- 1Department of Urology, The Second Hospital & Clinical Medical School, Lanzhou University, Lanzhou, Gansu, China
- 2Key Laboratory of Space Radiobiology of Gansu Province & Key Laboratory of Heavy Ion Radiation Biology and Medicine of Chinese Academy of Sciences, Institute of Modern Physics, Chinese Academy of Sciences, Lanzhou, China
- 3College of Life Science, University of Chinese Academy of Sciences, Beijing, China
Renal cell carcinoma (RCC) is considered radio- and chemo-resistant. Immune checkpoint inhibitors (ICIs) have demonstrated significant clinical efficacy in advanced RCC. However, the overall response rate of RCC to monotherapy remains limited. Given its immunomodulatory effects, a combination of radiotherapy (RT) with immunotherapy is increasingly used for cancer treatment. Heavy ion radiotherapy, specifically the carbon ion radiotherapy (CIRT), represents an innovative approach to cancer treatment, offering superior physical and biological effectiveness compared to conventional photon radiotherapy and exhibiting obvious advantages in cancer treatment. The combination of CIRT and immunotherapy showed robust effectiveness in preclinical studies of various tumors, thus holds promise for overcoming radiation resistance of RCC and enhancing therapeutic outcomes. Here, we provide a comprehensive review on the biophysical effects of CIRT, the efficacy of combination treatment and the underlying mechanisms involved in, as well as its therapeutic potential specifically within RCC.
1 Introduction
Globally, renal tumors are the 14th most prevalent malignant tumors, registering 430,000 new cases in 2020. With a steadily rising incidence rate, the burden of RCC continues to grow, resulting in numerous patients dying annually due to inadequate treatment (1, 2). 90% of renal tumors are renal cell carcinoma (RCC). At the time of diagnosis, 30% of RCC patients already exhibit metastasis, and a prognostic analysis indicates that nearly 30% of postoperative patient possess recurrence or metastasis. Since RCC responds poorly to both radiation and chemotherapy, a more effective treatment is critical for current RCC therapy (3–5).
Immune checkpoint inhibitors (ICIs), such as anti-programmed death receptor 1 (anti-PD-1), anti-programmed death-ligand 1 (anti-PD-L1), and anti-cytotoxic T-lymphocyte-associated protein-4 (anti-CTLA-4), constitute the primary approach to immunotherapy, achieving a considerable effect in patient with non-small cell lung cancer (NSCLC) or malignant melanoma among other cancer patient (6, 7). For RCC, ICIs treatment notably enhances the objective response and overall survival rates in patients with advanced disease, serving as the first- and second-line therapies for metastatic RCC. However, the total clinical efficacy rate stands at roughly 20%-40%, with only a subset of patients truly benefiting from immunotherapy (8–10). Hence, augmenting the effects of immunotherapy especially a combining treatment of immunotherapy with different therapeutic strategies is the major concern of RCC therapy currently. Studies indicate that RT can modulate immune responses and induce immunogenic cell death, thereby enhancing the systemic anti-tumor effects of immunotherapy. Extensive research focusing on the combination of RT with ICIs is underway (11, 12). Since RCC exhibits limited sensitivity to X-ray and γ-ray radiation, few studies reported the application of combination treatment in RCC.
Charged particles, especially hydrogen ions (protons) and carbon ions, are being increasingly used for cancer therapy. Notably, carbon ion radiotherapy (CIRT), the most prevalent used heavy ion radiation, has superior physical and biological characteristics to conventional photon radiation such as X-rays or γ-rays (13, 14). In addition, the combination treatment of CIRT and immunotherapy of malignant melanoma and breast cancer showed a better tumor suppression effect than the combination treatment of proton radiation thus present an encouraging prospect of CIRT combination treatment (13, 15). The combination treatment of CIRT and ICIs might offer a solution to RCC therapy.
In this article, we reviewed the biophysical effects of CIRT, the efficacy of combination treatment and the underlying mechanisms involved in, as well as its therapeutic potential specifically within RCC.
2 Radiation therapy and radiation therapy of RCC
2.1 Radiation therapy and CIRT
Approximately half of global tumor patients are treated with ionizing radiation (IR) therapy nowadays, either alone or as a complement to other treatments (16). Photon radiation such as X-rays and γ-rays are the most common cancer treatments and recently RT has emerged as a primary approach for cancer treatments (17). Though clinical photon radiotherapy enables the effective dosage distribution of tumor area, the concurrently irradiation of surrounding healthy tissues is inevitable and nonnegligible. Clinically, the late effects of radiotherapy gravely impact patients’ quality of life. Consequently, an important aspect of current radiation oncology research is still focusing on strategies to mitigate the influence of conventional radiation therapy on healthy tissues by other treatments such as chemotherapy, surgery, immunotherapy, or comprehensive treatments.
Preclinical studies and clinical usage of particle radiation, such as protons, heavy ions beam, neutrons, and boron neutrons therapy, indicate its distinct physical and biological attributes. Presently, carbon ion beams represent the most prevalent types of medical heavy ions beam radiation and its elevated linear energy transfer (LET) endows it with distinctive radiobiological traits. When carbon ions enter tissue, they will release the vast majority of their energy at the end of trajectory, form an inverted energy deposition curve which called Bragg peak (18). Modulating the energy of ions allows for adjusting the depth of the Bragg peak in tissue, leading to the focused release of energy within tumor sites. This characteristic of energetic charge particle radiation greatly reduces energy deposition in healthy tissues, providing precise targeting of tumor area and minimizing side effects. Yet, the Bragg peak’s duration is sharp, with its half-maximal width spanning just a few millimeters. In clinical settings, a spread-out Bragg peak (SOBP) is frequently utilized to encompass tumor areas. The high linear energy transfer (LET) characteristic ensures that the SOBP has elevated radiation dose deposition, facilitated accurate and thorough lesion irradiation while also significantly reduced harm to healthy tissue (19). Additionally, CIRT typically causes clustered DNA damage, making it difficult for cells to repair, thus facilitating the elimination of tumor cells (20). Furthermore, CIRT is less sensitive to the alterations in tumor cell cycle, radiation susceptibility, and oxygen concentration, allowing them to substantially counteract tumor radio-resistance.
Currently, the US, Japan, Germany, and China are leading in the field of heavy ion cancer therapy, achieving significant results in breast cancers, skin cancer, cranial cancers, prostate cancer, and more. In China, several hospitals now offer heavy ion cancer treatment services. For instance, the The Wuwei Tumor Hospital in northwest China’s Gansu Province boasts China’s first self-developed carbon ion therapy facility which was co-developed by the Institute of Modern Physics, Chinese Academy of Sciences (CAS) and a subsidiary company. Since 2020, the facility has completed over 1,500 cases of tumor patient, achieving considerable success. According to the data from the Particle Therapy Co-Operative Group (PTCOG), by the end of 2020, 40,000 patients globally had undergone heavy ion radiotherapy (primarily CIRT).
Currently, many clinical studies on heavy ion radiotherapy have been completed (Table 1) or are in progress (Table 2). Among 216 NSCLC patients, single-fraction CIRT showed comparable efficacy to the previous fractionated regimens and only one case of serious late toxicity was observed (21). 21 patients with melanoma treated with heavy ion beam irradiation showed that the 3-year local control rate was 92.3% (22). 75 patients with locally recurrent nasopharyngeal carcinoma were treated with CIRT, the 1-year overall survival was 98.1% and no severe acute or late toxicities were found (23). Among 2,157 prostate cancer patients receiving CIRT, the 5-year biochemical relapse-free survival rates for low, intermediate, and high-risk patients were 100%and no serious toxicities were identified (24). In addition, in cervical cancer, uterine adenocarcinoma, rectal cancer, hepatocellular carcinoma, chondrosarcoma, pancreatic cancer, and chordoma, heavy ion radiotherapy has also shown better clinical results and lower radiation toxicities (25–29). As compared with XRT, the 5-year overall survival rate for malignant melanoma post-CIRT (44%) was significantly higher than conventional radiation therapy (CRT) (25%) (30); In stage I inoperable NSCLC patients, the 5-year overall survival rate for CIRT (42%) was significantly higher than CRT (20%) (31). All these studies demonstrated the safety and efficacy of heavy ion radiotherapy especially the CIRT.
2.2 Radiation therapy of RCC
In vivo and in vitro studies have confirmed that RCC is a radio-resistant tumor, and photon radiation is primarily used for symptom relief in patients with advanced renal cell carcinoma (32–34).
Recently, Stereotactic Body Radiation Therapy (SBRT) has shown intriguing therapeutic effects in the treatment of RCC (35). SBRT is a radiotherapy technique that utilizes image-guided technology and highly conformal doses, achieving significant local control rates for primary and metastatic tumors. It can be applied to patients who are not surgical candidates as well as those with extensive or oligometastatic disease. Several studies have already confirmed the excellent efficacy and safety of SBRT in RCC (36, 37), considering the side effect of photon radiation, more attempts based on different radiation strategies would still be worthful.
There are few reports of RCC heavy ion radiotherapy, but the two papers showed that CIRT has notable therapeutic effects and lower toxicity. One study exhibited intriguing outcomes. Long-term follow-up revealed that the 5-year local control rate, disease-free survival rate, tumor-specific survival rate, and overall survival rate were 94.1%, 68.9%, 100% and 89.2%, respectively (38). In another study, 10 RCC patients treated with CIRT achieved 100% local control and 74% overall survival at 5 years, with only one patient experiencing grade 4 skin toxicity. These studies indicated the safety and favorable therapeutic results of CIRT in RCC (39).
Overall, though research on CIRT for RCC is quite limited, given the biological advantages of CIRT over CRT, it holds application benefits and research value in the treatment of RCC.
3 Immunotherapy and ICIs in RCC
3.1 immunotherapy
Immunotherapy aims to enhance a patient’s own immune system’s ability to recognize and attack cancer cells, thus achieving a better tumor control effect. This approach has significantly improved the current status of cancer treatment (40). Current immunotherapeutic strategies mainly include ICIs, adoptive T cell therapy, and cancer vaccines (41). Immune checkpoints refer to a set of negative regulatory molecules owned by immune cells. Among them, the immunosuppressive transmembrane protein PD-1 is expressed on T cells, B cells and NK cells, with PD-L1 and PD-L2 being its ligands (42). Within the Tumor Microenvironment (TME), tumor cells can upregulate the expression of PD-L1 or PD-L2, by which binding to PD-1 on the surface of immune cells, thereby inducing apoptosis of immune cells and keeping surviving of themselves (43, 44). Another immune checkpoint, CTLA-4 (CD152), is a transmembrane protein expressed on CD4+ T cells, CD8+ T cells and Treg cells, with its ligands primarily being CD80 and CD86 expressed on Antigen-Presenting Cells (APCs). When CTLA-4 binds to its ligands, it promotes Treg cells to secrete immunosuppressive factors, thereby inhibiting T cell responses (45).
ICIs aim to block the inhibition of immune cells to increase the number of anti-tumor cells in the TME (mainly T cells) to achieve tumor suppression (Figure 1). In recent years, many immunotherapeutic drugs have received FDA approval, such as anti-PD-1, anti-PD-L1, and anti-CTLA-4 monoclonal antibodies (e.g., Pembrolizumab, Atezolizumab, Nivolumab, and Ipilimumab). Clinically, ICIs have been widely used and have achieved excellent results in cancer treatment. The approval of these drugs indicates the efficacy and prospects of immunotherapy. Although immunotherapy has achieved these successes, the efficacy of monotherapy is limited, and its mechanism is not yet fully understood (46). How to make it effective for more types of tumors and benefit more patients remains an unresolved issue.
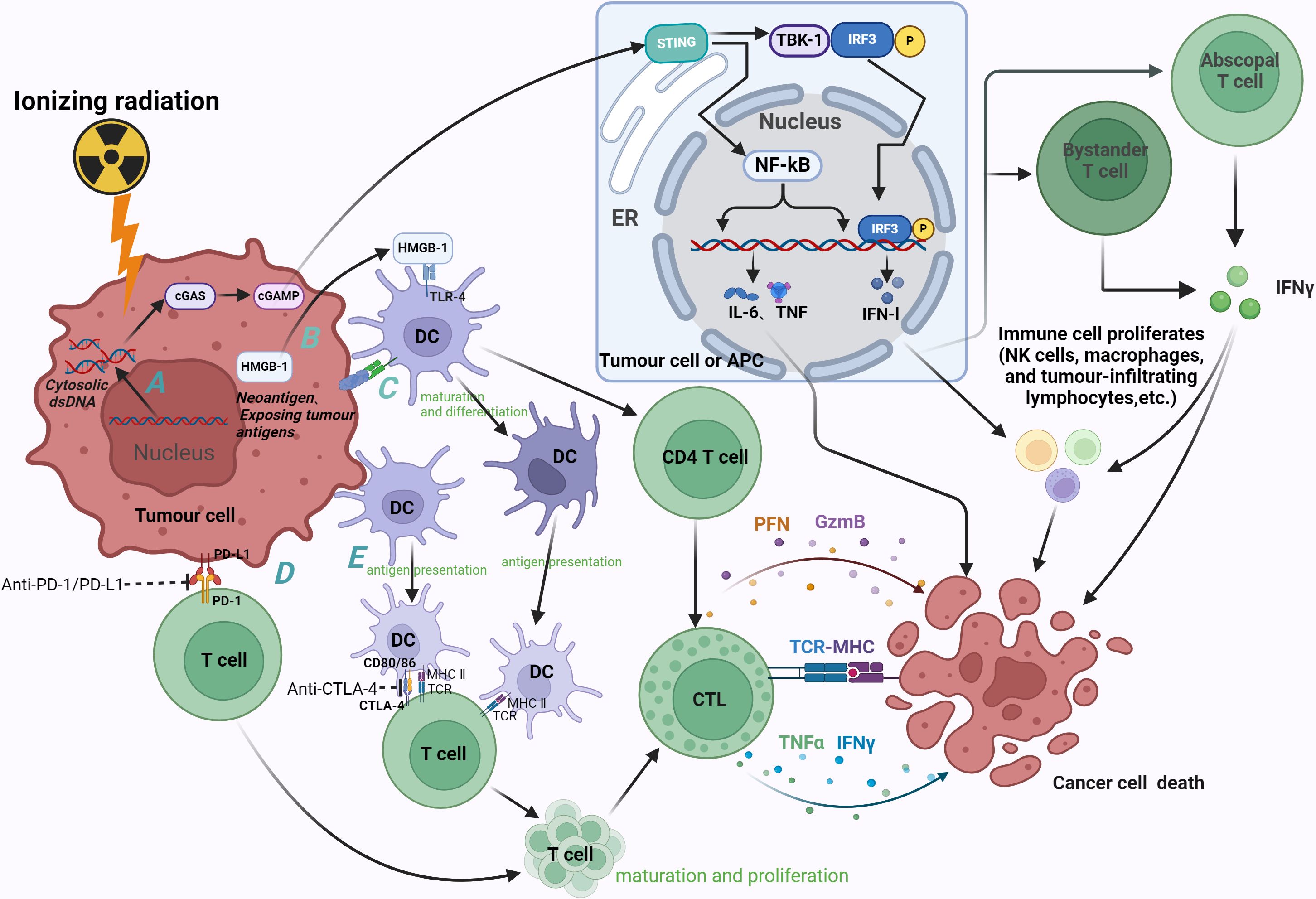
Figure 1 Radiation-induced immune effects and therapeutic mechanism of ICIs (anti-PD-1, anti-PD-L1 and anti-CTLA-4). (A) Radiation causes accumulation of cytoplasmic dsDNA in tumor cells, which can induce activation of the cGAS-STING pathway in tumor cells and APC. The enrichment of cGAS promotes the synthesis of second messenger cGAMP, which in turn binds to STING, recruits and phosphorylates downstream TBK-1 and IRF-3. Nuclear translocation of IRF-3 induces the transcription of IFN-1, and IFN-β stimulates the release of IFN-γ by bystander T cells and distant T cells. IFN can inhibit tumor cell division while inducing apoptosis, activate effector cells and increase the activity of NK cells, macrophages and tumor infiltrating lymphocytes, thereby enhancing the anti-tumor immune effect. STING induces tumor cell death by promoting the secretion of pro-inflammatory cytokines such as IL-6 and TNF through NF-kB. (B) Irradiation promotes HMGB-1 efflux, and HMGB-1 binds to TLR-4 to promote the differentiation and maturation of DC and antigen cross-presentation, thereby activating CD4 T cells and CTL. CTL induces apoptosis of tumor cells through secretion of PFN, GzmB, etc., or recognition of tumor cell MHC by TCR. (C) Radiation induces the exposure of tumor antigens and the generation of neoantigens (as indicated by calreticulin exposure to tumor cells), thereby exerting anti-tumor effects through DCs. (D) Irradiation can up-regulate the expression of PD-1 on immune cells and PD-L1 on tumor cells, and the combination of the two can promote the apoptosis of immune cells, resulting in immunosuppression. Anti-pd-1 and anti-PD-L1 can promote the proliferation and differentiation of immune cells (mainly T cells) by antagonizing this process, and finally play an anti-tumor role. (E) The immune checkpoint CTLA-4 expressed on T cells exerts immunosuppressive effects by binding to its ligands CD80 and CD86, which are mainly expressed on DC. Blockade of this process by anti-CTLA-4 can promote the proliferation and maturation of T cells. cGAS, cGMP-AMP synthase; STING, interferon gene stimulator; TBK-1, TANK-binding kinase 1; IRF-3, interferon regulatory factor 3; IFN, interferon; IL, interleukin; TNF, tumor necrosis factor; HMGB1, human high mobility group protein; TLR-4, Toll-like receptor 4; CTL, cytotoxic T cells; PFN, perforin; GzmB, Granzyme B; TCR, T cell receptor; MHC, major histocompatibility complex; ER, endoplasmic reticulum. Image created with BioRender.com, with permission.
3.2 ICIs in RCC
Higher expression levels of PD-L1 in tumor often categorized it as an immune “hot” tumor which is inclined sensitive to ICIs (47). A majority of RCC subtypes, including clear cell RCC (ccRCC) which constitutes approximately 75% of RCC, papillary RCC, translocation RCC, and collecting duct carcinoma, exhibit a PD-L1 expression ratio exceeding 10% (48, 49). Concurrently, the preclinical use of ICIs (anti-CTLA-4 and anti-PD-1) alone or in combination in treating ccRCC has shown notable results (50–53), as illustrated in Table 3. Unfortunately, in clinical, RCC often develops resistance to first-line ICIs treatments, and the majority of RCC patients do not derive long-term benefits from them. Therefore, developing new treatment modalities to overcome these resistance mechanisms is crucial for the treatment of RCC.
Considering the limitations of monotherapy with immunotherapy, researchers have become increasingly interested in combination strategies of ICIs with other treatment modalities. Among them, RT has garnered significant attention due to its immune-stimulating effects, and numerous related studies have been conducted. However, there is limited research on the combination treatment of ICIs and RT in RCC. The radio-resistance of RCC might be one of the factors limiting work in this area. CIRT demonstrates superior efficacy in RCC compared to CRT. The future utilization of CIRT is anticipated to be extensive in the management of RCC. Considering the commendable efficacy demonstrated by ICIs in RCC treatment, the amalgamation of CIRT and ICIs has the potential to further augment the anti-tumor effect exerted by ICIs. This combination warrants further investigation in subsequent studies. CIRT has gained attention for its immune-stimulating effects in RCC. The synergy between CIRT and ICIs holds promise for enhancing anti-tumor effects, emphasizing the need for further investigation in future studies.
4 The combination of RT and immunotherapy
Radiation causes direct damage to tumor cells while also inducing systemic biological effects that generate anti-tumor responses (Figure 1). Through these mechanisms, RT can transform tumors with low immune cell infiltration (referred to as “cold” tumors) into tumors with high immune cell infiltration (referred to as “hot” tumors), thereby enhancing the effect of tumor immunotherapy (54). This role of RT has been widely recognized, forming the fundamental principle behind the combination of radiation and immunotherapy.
4.1 The combination of CRT and immunotherapy
Current RT combined with immunotherapy includes combinations with DC vaccine, ICIs, and CAR-T cell therapy. Nesslinger et al. assessed the serum samples of prostate cancer patients who received cancer vaccine combined with RT or RT alone. The results indicated that the combination therapy enhanced the spread of antigens and the immune response to other tumor antigens (55). In a Phase II clinical trial, most prostate cancer patients who received cancer vaccine combined with RT showed a significant increase in PAS-specific T cells, indicating a PAS-specific cellular immune response, which was not observed in the solo radiotherapy group (56). A meta-analysis revealed that the combination of PD-1/PD-L1 inhibitors with RT extended the overall survival of NSCLC patients, simultaneously improving the objective response rate and disease control rate, without increasing the incidence of adverse events above grade 3. It was also found that SBRT or SBRT combined with surgery and PD-1/PD-L1 inhibitors is more effective than CRT combined with PD-1/PD-L1 inhibitors (57). Formenti et al. confirmed that in chemo-resistant metastatic NSCLC, RT combined with CTLA-4 inhibitor induced systemic anti-tumor T cells. Treatment with anti-CTLA-4 antibodies alone or in combination with chemotherapy was less effective (58). DeSelm et al. found that low-dose radiation sensitized tumor cells to the immune rejection response of locally activated CAR-T cells, reducing the recurrence of antigen-negative tumors (59). Current research in the CAR-T cell and radiation fields is limited, but it’s clear that RT can play an essential role in the combination treatment of patients undergoing CAR-T therapy in various clinical settings (60). However, RT also has a negative immune regulatory effect, promoting immune suppression to some extent. When radiation kills tumor cells, it can also cause a certain degree of lethal radiation. The local hypoxic environment caused by RT induces the aggregation of immune suppressive cells and M2-type macrophages in the TME and the elevation of immune suppressive factors.
In summary, RT, as a local treatment modality, can trigger a systemic immune response, suppressing the growth of distant non-irradiated tumors, also known as the abscopal effect. This became the fundamental principle of combining RT and immunotherapy. However, it can also lead to immune suppression. Therefore, when RT triggers a systemic anti-tumor immune response, administering immunotherapy as an adjunct to counteract the negative immune regulatory effects of RT and amplify its immune-stimulating effects holds promise as a curative treatment for tumors and achieving control over systemic metastatic tumors.
4.2 The combination of CIRT and immunotherapy
Carbon ion has unique biological and physical efficacy compared to CRT (61). Compared to X-rays, carbon ions have a stronger cytotoxic effect on tumor cells, and the enhancement of radiotherapy-induced immune cell infiltration and remodeling of the TME is more pronounced. For deep tumors like RCC that are resistant to CRT, carbon ions achieve a cytotoxic effect that CRT cannot achieve and can effectively promote immune responses. Thus, the application of “CIRT combining immunotherapy” shows a very promising prospect. Currently, the combination of CIRT and immunotherapy has made some progress in NSCLC (62), and this combined approach is increasingly being seen as an effective cancer treatment modality.
Carbon ions induce immune stimulation. Research has found that carbon ion beams can regulate anti-tumor immunity in various ways and have unique advantages in remodeling the TME. A large amount of literature has reported the advantages of carbon ion beams in immune regulation. Spina et al. compared the immune regulatory effects of CIRT and biologically equivalent doses of X-ray radiotherapy (XRT) in an in situ 4T1 breast cancer mouse model. The results found that low-dose CIRT can usually retain lymphocytes that are crucial for the anti-tumor immune response, and higher dose CIRT more effectively induced the secretion of pro-inflammatory cytokines, while even at low doses, XRT had lymphotoxicity (15). Guo et al.’s study showed that the activation of the cGAS-STING pathway caused by CIRT is greater than that of XRT, and it also promoted the infiltration of NK cells, CD4+ and CD8+ T cells (63). In Luo et al.’s research, CIRT was able to reduce the phosphorylation of STAT3 mediated by the JAK3/STAT2 pathway, thereby inhibiting the formation of regulatory T cells (Treg) (64), and in TME, Tregs can suppress the anti-tumor activity of NK cells (65). In a study of 32 localized prostate patients treated with CIRT, it was found that the carbon ion could effectively retain lymphocyte numbers, promote their proliferation, enhance T-cell function, and also weaken the induction of immunosuppressive cells and the expression of immunosuppressive cell factors, thus effectively inducing immune activation (66). These results indicate that compared to XRT, CIRT can significantly promote the proliferation of immune cells and the secretion of pro-inflammatory cytokines in both animals and humans, exerting a more effective anti-tumor effect.
Carbon ion beams have a superior ability to induce immunogenic cell death compared to CRT. While irradiating and killing tumor cells, they also induce immunogenic changes in these cells, which is one of the key mechanisms by which radiotherapy modulates anti-tumor immunity. Ran et al. discovered that after XRT and CIRT treatments, A549, H520, and Lewis Lung Carcinoma (LLC) cells both showed time-dependent increases in the levels of HMGB1, IL-10, and TGF-β. However, only XRT exhibited a dose-dependent increase in HMGB1 levels. Simultaneously, carbon ions induced a higher level of HMGB1, while relatively reducing the levels of immunosuppressive factors IL-10 and TGF-β (67). Onishi M and colleagues irradiated HeLa, SiHa and KYSE-70 cells with carbon ions of different LET, finding that HMGB1 release levels significantly increased in all these cancer cell lines and intensified with increasing LET (68). HMGB1, upon binding with TLR-4, promotes cytokine secretion, antigen cross-presentation, and the differentiation and maturation of DC, further activating helper and effector T cells (69); TGF-β and IL-10 are significant immunosuppressive factors in the TME, working by reducing DC function and T-cell activation, promoting Treg cell transformation, and facilitating MDSC maturation (70–73). Huang and colleagues irradiated four human cancer cell lines with photons, protons, and carbon ions. The results showed that 48 hours after irradiation, carbon ions at 2 Gy and 4 Gy significantly promoted the cell surface translocation of calreticulin, which was much more pronounced than with protons and photons (74). In normal cells, calreticulin is primarily found in the endoplasmic reticulum. When exposed on the cell surface, it can be recognized, taken up and processed by DCs, thus upregulating the immunogenic death of the tumor. This makes the irradiated tumor serve as an in-situ vaccine (75). Thus, carbon ion beams induce tumor cell death in various ways and release pro-inflammatory cytokines, chemokines, and express tumor antigens, referred to as Damage-Associated Molecular Patterns (DAMP). DAMPs can mediate the infiltration of immune cells in the tumor region, thereby activating the immune system (76).
Seventy years ago, the term “abscopal effect” was established by Mole and began to gain attention. This refers to the phenomenon where irradiating a tumor can lead to the regression of distant non-irradiated metastatic tumors. The biological mechanism behind this is not yet fully understood, but it is related to the immune system (77, 78). RT can trigger the release of new antigens (Tumor-Associated Antigens, TAA) from irradiated dying tumor cells, acting as in-situ vaccines. Once TAAs are phagocytosed by APCs, they are presented to CD8+ T cells. Activated CD8+ T cells can recognize and attack the primary tumor and distant metastatic tumors. Irradiated tumor cells can also release the aforementioned DAMPs and cytokines, further enhancing the activation of immune cells (79). However, the abscopal effect induced by RT is not common (80). Notably, some studies have confirmed that immunotherapy can enhance the radiotherapy-induced abscopal effect. In Grimaldi’s research, 21 patients with advanced melanoma underwent RT after ICIs (Ipilimumab) treatment. The results showed that 11 patients (52%) observed remission of distant tumors. The median OS for all 21 patients was 13 months, with the median OS being 22.4 months for those with the abscopal effect and only 8.3 months for those without (81). Komatsu reported a primary lung cancer patient treated with ICIs. After three weeks of treatment with Nivolumab, the tumor increased. Immediate RT was administered, showing a significant reduction in the irradiated tumor and also a decrease in the non-irradiated lung metastasis (82). The enhancement of the radiotherapy-induced abscopal effect by immunotherapy indicates the potential of this combined treatment approach. Further exploration is needed to understand the specific mechanisms of the radiotherapy-immunotherapy strategy, as well as determining the best dosages, intervention times, etc., to achieve optimal tumor therapeutic outcomes and effective treatment of cancer metastasis.
Carbon ions have been shown to be more effective than CRT in inducing the abscopal effect. In one case, a patient with recurrent thymic carcinoma showed a significant reduction in both the irradiated tumor and the non-irradiated distant tumor on a CT scan on day 1 after only receiving CIRT (60 Gy/12 fractions). Acute adverse reactions were limited to grade 1 radiodermatitis and mild erythema, with no adverse reactions greater than grade 2 observed, and no late adverse reactions found within 10 months (83). In another study, 3 out of 5 patients with assessable distant tumors observed the abscopal effect. Among them, two had multiple metastatic lesions, and all metastatic lesions achieved a tumor volume reduction, with an average volume reduction of 42% (84). Additionally, animal experiments have shown that CIRT is more effective than CRT in inhibiting the number of metastatic tumors in radiation-induced abscopal effect (85). In 4T1 tumor-bearing mice, both the irradiated and non-irradiated tumors’ growth was significantly delayed compared to the control group after receiving CIRT, indicating the direct and abscopal anti-tumor effects of carbon ions (86). However, the abscopal effect induced by either CRT or CIRT is not common (87). But when combined with immunotherapy like ICIs, significant control of both primary and distant tumors was observed, indicating the therapeutic potential of combined treatments in advanced tumors (85, 88).
In recent years, with research into the immunomodulatory effects of RT, a substantial body of evidence has demonstrated the effectiveness of combined RT and immunotherapy in cancer treatment. CIRT, based on its unique physical and biological advantages, has shown significant promise in combination therapy and holds potential for the treatment of certain radiation-resistant tumors and immunologically “cold” tumors.
In animal experiments involving the combination of carbon ion beams with ICIs, Zhou et al. irradiated subcutaneous melanomas in C57BL/6 mice with 5 Gy of X-rays and carbon ion beams. They administered anti-PD-1 treatment on days 1, 2 and 4 after irradiation. The results showed that in the combined treatment, CIRT promoted more exposure of calreticulin and the release of HMGB1 compared to XRT. It also induced a stronger IFN-1 response, demonstrating that CIRT effectively triggers immunogenic cell death. In the CIRT combined with anti-PD-1 group, there was an increase in the infiltration of CD4+ and CD8+ T cells into tumor tissues, leading to significant tumor control and an extended survival period for tumor-bearing mice (89). Guo et al. reported similar outcomes, showing that compared to X-ray, the combination of CIRT with anti-PD-L1 significantly increased the infiltration of CD4+, CD8+ T cells and NK cells into tumors, while delaying melanoma growth. Additionally, CIRT up-regulated the expression of PD-1 on the surface of immune cells, whereas XRT did not. Furthermore, CIRT induced the exposure of PD-L1 on tumor cells. High PD-1 expression in tumor-infiltrating immune cells is considered a characteristic of immunologically “hot” tumors (63, 90). Thus, these results demonstrate the synergistic effects of carbon ion beams combined with anti-PD-L1 (or anti-PD-1) in cancer therapy.
In a mouse osteosarcoma model, both CIRT and XRT, in combination with two ICIs (anti-PD-1 and anti-CTLA-4), inhibited the growth of distant tumors and lung metastasis. The combination of CIRT and ICIs showed the most significant effect, while monotherapy with radiation or ICIs did not achieve control of distant tumors (85).In another mouse breast cancer model, the combination of CIRT with ICIs achieved even more remarkable therapeutic effects. Analysis of tumors irradiated with carbon ion beams and treated with anti-CTLA-4 showed activation of NK cells, up-regulation of tumor-associated macrophages, TNF and IL-1 response genes, along with improved activity of naive T cells in distant non-irradiated tumors. Furthermore, cured mice exhibited long-lasting anti-tumor immunity (14). These results indicate that combination therapy effectively optimizes the TME, which may be one of the reasons for distant effects and specific tumor immune memory. In RT combined with DC-based therapy, after CIRT and XRT combined with intratumoral or intravenous DC administration in mice, the former significantly inhibited lung metastasis of mouse tumors when combined with DC, while the latter required higher doses to achieve lung metastasis suppression. Compared to XRT, CIRT significantly up-regulated the exposure level of extracellular calreticulin in tumor cells (91). Therefore, CIRT may promote the maturation of DC by increasing the immunogenicity of tumors, further stimulating anti-tumor immunity and reducing lung metastasis.
In terms of clinical trials, there are currently two ongoing clinical trials regarding carbon ion radiation combined with immunotherapy. One is a Phase II clinical trial combining CIRT and pamrelizumab for nasopharyngeal carcinoma patients after chemotherapy, with a plan to recruit 146 nasopharyngeal carcinoma patients (NCT04143984). The other is a multi-center Phase II clinical trial planned to include 27 patients (NCT05229614), using large fraction CIRT and pembrolizumab to treat non-small cell lung cancer, head and neck squamous cell carcinoma, melanoma, and urothelial carcinoma; results from both clinical trials have not yet been published.
5 Conclusions and perspectives
As research into radiotherapy and immunotherapy progresses, the synergistic effects of radiotherapy and immunotherapy become more apparent, especially for tumors that are not sensitive to conventional photon radiotherapy. Carbon ions can effectively kill tumors resistant to XRT, such as RCC, and exert an immune-activating effect. The synergistic effects in anti-tumor of this combined method offer the hope of eliciting a durable and potent immune response against tumors and having a new method of cancer treatment. Although preclinical and clinical research have shown the advantages and efficacy of CIRT. However, research combining carbon ions with immunotherapy are still rare and have not been reported in renal cell carcinoma. This may be due to the fact that heavy ion accelerators are not yet widely available globally and the high cost of treatment limits related research.
There are possible risks and problems associated with the combination therapy. Adverse reactions such as radiation pneumonitis and dermatitis also accompany carbon ion irradiation but are generally comparable or milder than CRT. Over-activation of the immune system may cause systemic toxicity and damage the body. With the optimization of accelerators, the cost of CIRT will be lower in the future, and more cancer patients can receive this advanced treatment. And new RT technologies, such as SBRT and Flash RT, are constantly emerging, which can control the radiation dose distribution in the tumor target more accurately, further improve the efficacy of carbon ion therapy and reduce adverse reactions. The adverse reactions of tumor immunotherapy are milder and usually reversible than those of conventional chemotherapy, and most patients can benefit from it. The potent immune-activating effect of CIRT is expected to reduce the dose of immunotherapeutic agents, thereby further minimizing the adverse reactions. In the future, CIRT will benefit more cancer patients, and its combination with other treatment methods, such as immunotherapy, is expected to achieve the ultimate goal of cancer treatment from “remission” to “cure”.
Author contributions
ZZ: Conceptualization, Investigation, Visualization, Writing – original draft, Writing – review & editing. TY: Conceptualization, Writing – original draft. YL: Conceptualization, Funding acquisition, Writing – original draft. PQ: Writing – review & editing. ZS: Writing – review & editing. YW: Writing – review & editing. WC: Writing – review & editing. SU: Writing – review & editing. WW: Supervision, Writing – review & editing, Conceptualization, Funding acquisition, Project administration. ND: Funding acquisition, Project administration, Supervision, Writing – review & editing. JW: Supervision, Writing – review & editing.
Funding
The author(s) declare financial support was received for the research, authorship, and/or publication of this article. This work was supported by the Gansu Provincial Science Fund for Distinguished Young Scholars (22JR5RA942), CuiYing Science and Technology Innovation Program of the Second Hospital of Lanzhou University (CY2023–MS–A03), the Natural Science Foundation of China (12175289 and 12275329), the West Light Foundation of The Chinese Academy of Sciences (xbzglzb2022003), the Science and Technology Research Project of Gansu Province (21JR7RA107 and 22JR5RA1009), Lanzhou Science and Technology Plan Project (2023–2–43), the scientific research project of health industry of Gansu province (GSWSKY2020-10), Innovation and Entrepreneurship Incubation Project of Lanzhou University Medical Center (lzuyxcx–2022–96), Lanzhou university education teaching reform research key project (202328), the National Innovation and Entrepreneurship Training plan for college students in 2024 (202410730002).
Conflict of interest
The authors declare that the research was conducted in the absence of any commercial or financial relationships that could be construed as a potential conflict of interest.
Publisher’s note
All claims expressed in this article are solely those of the authors and do not necessarily represent those of their affiliated organizations, or those of the publisher, the editors and the reviewers. Any product that may be evaluated in this article, or claim that may be made by its manufacturer, is not guaranteed or endorsed by the publisher.
References
1. Rini BI, Campbell SC, Escudier B. Renal cell carcinoma. Lancet. (2009) 373:1119–32. doi: 10.1016/S0140-6736(09)60229-4
2. Sung H, Ferlay J, Siegel RL, Laversanne M, Soerjomataram I, Jemal A, et al. Global cancer statistics 2020: GLOBOCAN estimates of incidence and mortality worldwide for 36 cancers in 185 countries. CA Cancer J Clin. (2021) 71:209–49. doi: 10.3322/caac.21660
3. Hepgur M, Sadeghi S, Dorff TB, Quinn DI. Tivozanib in the treatment of renal cell carcinoma. Biologics. (2013) 7:139–48. doi: 10.2147/BTT.S32958
4. Yamamoto T, Kadoya N, Takeda K, Matsushita H, Umezawa R, Sato K, et al. Renal atrophy after stereotactic body radiotherapy for renal cell carcinoma. Radiat Oncol. (2016) 11:72. doi: 10.1186/s13014-016-0651-5
5. Hsieh JJ, Purdue MP, Signoretti S, Swanton C, Albiges L, Schmidinger M, et al. Renal cell carcinoma. Nat Rev Dis Primers. (2017) 3:17009. doi: 10.1038/nrdp.2017.9
6. Kalaora S, Nagler A, Wargo JA, Samuels Y. Mechanisms of immune activation and regulation: lessons from melanoma. Nat Rev Cancer. (2022) 22:195–207. doi: 10.1038/s41568-022-00442-9
7. Reck M, Remon J, Hellmann MD. First-line immunotherapy for non-small-cell lung cancer. J Clin Oncol. (2022) 40:586–97. doi: 10.1200/JCO.21.01497
8. Horn L, Spigel DR, Vokes EE, Holgado E, Ready N, Steins M, et al. Nivolumab versus docetaxel in previously treated patients with advanced non-small-cell lung cancer: two-year outcomes from two randomized, open-label, phase III trials (CheckMate 017 and CheckMate 057). J Clin Oncol. (2017) 35:3924–33. doi: 10.1200/JCO.2017.74.3062
9. Brahmer J, Reckamp KL, Baas P, Crinò L, Eberhardt WE, Poddubskaya E, et al. Nivolumab versus docetaxel in advanced squamous-cell non-small-cell lung cancer. N Engl J Med. (2015) 373:123–35. doi: 10.1056/NEJMoa1504627
10. Deleuze A, Saout J, Dugay F, Peyronnet B, Mathieu R, Verhoest G, et al. Immunotherapy in renal cell carcinoma: the future is now. Int J Mol Sci. (2020) 21:2532. doi: 10.3390/ijms21072532
11. Galluzzi L, Aryankalayil MJ, Coleman CN, Formenti SC. Emerging evidence for adapting radiotherapy to immunotherapy. Nat Rev Clin Oncol. (2023) 20:543–57. doi: 10.1038/s41571-023-00782-x
12. Herrera FG, Irving M, Kandalaft LE, Coukos G. Rational combinations of immunotherapy with radiotherapy in ovarian cancer. Lancet Oncol. (2019) 20:e417–e33. doi: 10.1016/S1470-2045(19)30401-2
13. Zhou H, Tu C, Yang P, Li J, Kepp O, Li H, et al. Carbon ion radiotherapy triggers immunogenic cell death and sensitizes melanoma to anti-PD-1 therapy in mice. Oncoimmunology. (2022) 11:2057892. doi: 10.1080/2162402X.2022.2057892
14. Hartmann L, Osen W, Eichmüller OL, Kordaß T, Furkel J, Dickes E, et al. Carbon ion irradiation plus CTLA4 blockade elicits therapeutic immune responses in a murine tumor model. Cancer Lett. (2022) 550:215928. doi: 10.1016/j.canlet.2022.215928
15. Spina CS, Tsuruoka C, Mao W, Sunaoshi MM, Chaimowitz M, Shang Y, et al. Differential immune modulation with carbon-ion versus photon therapy. Int J Radiat Oncol Biol Phys. (2021) 109:813–8. doi: 10.1016/j.ijrobp.2020.09.053
16. Qu P, Shao ZA, Wang B, He JP, Zhang YN, Wei WJ, et al. MiR-663a inhibits radiation-induced epithelium-to-mesenchymal transition by targeting TGF-β1. BioMed Environ Sci. (2022) 35:437–47. doi: 10.3967/bes2022.059
17. Schaue D, McBride WH. Opportunities and challenges of radiotherapy for treating cancer. Nat Rev Clin Oncol. (2015) 12:527–40. doi: 10.1038/nrclinonc.2015.120
18. Grau C, Durante M, Georg D, Langendijk JA, Weber DC. Particle therapy in europe. Mol Oncol. (2020) 14:1492–9. doi: 10.1002/1878-0261.12677
19. Kanai T, Furusawa Y, Fukutsu K, Itsukaichi H, Eguchi-Kasai K, Ohara H. Irradiation of mixed beam and design of spread-out Bragg peak for heavy-ion radiotherapy. Radiat Res. (1997) 147:78–85. doi: 10.2307/3579446
20. Durante M, Loeffler JS. Charged particles in radiation oncology. Nat Rev Clin Oncol. (2010) 7:37–43. doi: 10.1038/nrclinonc.2009.183
21. Yamamoto N, Miyamoto T, Nakajima M, Karube M, Hayashi K, Tsuji H, et al. A dose escalation clinical trial of single-fraction carbon ion radiotherapy for peripheral stage I non-small cell lung cancer. J Thorac Oncol. (2017) 12:673–80. doi: 10.1016/j.jtho.2016.12.012
22. Takayasu Y, Kubo N, Shino M, Nikkuni O, Ida S, Musha A, et al. Carbon-ion radiotherapy combined with chemotherapy for head and neck mucosal melanoma: Prospective observational study. Cancer Med. (2019) 8:7227–35. doi: 10.1002/cam4.2614
23. Hu J, Bao C, Gao J, Guan X, Hu W, Yang J, et al. Salvage treatment using carbon ion radiation in patients with locoregionally recurrent nasopharyngeal carcinoma: Initial results. Cancer. (2018) 124:2427–37. doi: 10.1002/cncr.31318
24. Nomiya T, Tsuji H, Kawamura H, Ohno T, Toyama S, Shioyama Y, et al. A multi-institutional analysis of prospective studies of carbon ion radiotherapy for prostate cancer: A report from the Japan Carbon ion Radiation Oncology Study Group (J-CROS). Radiother Oncol. (2016) 121:288–93. doi: 10.1016/j.radonc.2016.10.009
25. Wakatsuki M, Kato S, Ohno T, Karasawa K, Kiyohara H, Tamaki T, et al. Clinical outcomes of carbon ion radiotherapy for locally advanced adenocarcinoma of the uterine cervix in phase 1/2 clinical trial (protocol 9704). Cancer. (2014) 120:1663–9. doi: 10.1002/cncr.28621
26. Yamada S, Kamada T, Ebner DK, Shinoto M, Terashima K, Isozaki Y, et al. Carbon-ion radiation therapy for pelvic recurrence of rectal cancer. Int J Radiat Oncol Biol Phys. (2016) 96:93–101. doi: 10.1016/j.ijrobp.2016.04.022
27. Okonogi N, Wakatsuki M, Kato S, Karasawa K, Miyasaka Y, Murata H, et al. A phase 1/2 study of carbon ion radiation therapy with concurrent chemotherapy for locally advanced uterine cervical squamous cell carcinoma (Protocol 1302). Int J Radiat Oncol Biol Phys. (2019) 104:631–9. doi: 10.1016/j.ijrobp.2019.02.042
28. Hong Z, Zhang W, Cai X, Yu Z, Sun J, Wang W, et al. Carbon ion radiotherapy with pencil beam scanning for hepatocellular carcinoma: Long-term outcomes from a phase I trial. Cancer Sci. (2023) 114:976–83. doi: 10.1111/cas.15633
29. Schulz-Ertner D, Nikoghosyan A, Thilmann C, Haberer T, Jäkel O, Karger C, et al. Carbon ion radiotherapy for chordomas and low-grade chondrosarcomas of the skull base. Results in 67 patients. Strahlenther Onkol. (2003) 179:598–605. doi: 10.1007/s00066-003-1120-2
30. Ramaekers BL, Pijls-Johannesma M, Joore MA, van den Ende P, Langendijk JA, Lambin P, et al. Systematic review and meta-analysis of radiotherapy in various head and neck cancers: comparing photons, carbon-ions and protons. Cancer Treat Rev. (2011) 37:185–201. doi: 10.1016/j.ctrv.2010.08.004
31. Grutters JP, Kessels AG, Pijls-Johannesma M, De Ruysscher D, Joore MA, Lambin P. Comparison of the effectiveness of radiotherapy with photons, protons and carbon-ions for non-small cell lung cancer: a meta-analysis. Radiother Oncol. (2010) 95:32–40. doi: 10.1016/j.radonc.2009.08.003
32. Ning S, Trisler K, Wessels BW, Knox SJ. Radiobiologic studies of radioimmunotherapy and external beam radiotherapy in vitro and in vivo in human renal cell carcinoma xenografts. Cancer. (1997) 80:2519–28. doi: 10.1002/(ISSN)1097-0142
33. Deschavanne PJ, Fertil B. A review of human cell radiosensitivity in vitro. Int J Radiat Oncol Biol Phys. (1996) 34:251–66. doi: 10.1016/0360-3016(95)02029-2
34. Garcia-Barros M, Paris F, Cordon-Cardo C, Lyden D, Rafii S, Haimovitz-Friedman A, et al. Tumor response to radiotherapy regulated by endothelial cell apoptosis. Science. (2003) 300:1155–9. doi: 10.1126/science.1082504
35. Siva S, Ali M, Correa RJM, Muacevic A, Ponsky L, Ellis RJ, et al. 5-year outcomes after stereotactic ablative body radiotherapy for primary renal cell carcinoma: an individual patient data meta-analysis from IROCK (the International Radiosurgery Consortium of the Kidney). Lancet Oncol. (2022) 23:1508–16. doi: 10.1016/S1470-2045(22)00656-8
36. Hannan R, McLaughlin MF, Pop LM, Pedrosa I, Kapur P, Garant A, et al. Phase 2 trial of stereotactic ablative radiotherapy for patients with primary renal cancer. Eur Urol. (2023) 84:275–86. doi: 10.1016/j.eururo.2023.02.016
37. Correa RJM, Louie AV, Zaorsky NG, Lehrer EJ, Ellis R, Ponsky L, et al. The emerging role of stereotactic ablative radiotherapy for primary renal cell carcinoma: A systematic review and meta-analysis. Eur Urol Focus. (2019) 5:958–69. doi: 10.1016/j.euf.2019.06.002
38. Kasuya G, Tsuji H, Nomiya T, Makishima H, Haruyama Y, Kobashi G, et al. Updated long-term outcomes after carbon-ion radiotherapy for primary renal cell carcinoma. Cancer Sci. (2018) 109:2873–80. doi: 10.1111/cas.13727
39. Nomiya T, Tsuji H, Hirasawa N, Kato H, Kamada T, Mizoe J, et al. Carbon ion radiation therapy for primary renal cell carcinoma: initial clinical experience. Int J Radiat Oncol Biol Phys. (2008) 72:828–33. doi: 10.1016/j.ijrobp.2008.01.043
40. Szeto GL, Finley SD. Integrative approaches to cancer immunotherapy. Trends Cancer. (2019) 5:400–10. doi: 10.1016/j.trecan.2019.05.010
41. Huang R, Zhao B, Hu S, Zhang Q, Su X, Zhang W. Adoptive neoantigen-reactive T cell therapy: improvement strategies and current clinical researches. biomark Res. (2023) 11:41. doi: 10.1186/s40364-023-00478-5
42. Jiang X, Wang J, Deng X, Xiong F, Ge J, Xiang B, et al. Role of the tumor microenvironment in PD-L1/PD-1-mediated tumor immune escape. Mol Cancer. (2019) 18:10. doi: 10.1186/s12943-018-0928-4
43. Zhang X, Schwartz JC, Guo X, Bhatia S, Cao E, Lorenz M, et al. Structural and functional analysis of the costimulatory receptor programmed death-1. Immunity. (2004) 20:337–47. doi: 10.1016/S1074-7613(04)00051-2
44. Baumeister SH, Freeman GJ, Dranoff G, Sharpe AH. Coinhibitory pathways in immunotherapy for cancer. Annu Rev Immunol. (2016) 34:539–73. doi: 10.1146/annurev-immunol-032414-112049
45. Rowshanravan B, Halliday N, Sansom DM. CTLA-4: a moving target in immunotherapy. Blood. (2018) 131:58–67. doi: 10.1182/blood-2017-06-741033
46. Nagasaki J, Ishino T, Togashi Y. Mechanisms of resistance to immune checkpoint inhibitors. Cancer Sci. (2022) 113:3303–12. doi: 10.1111/cas.15497
47. Thompson RH, Dong H, Lohse CM, Leibovich BC, Blute ML, Cheville JC, et al. PD-1 is expressed by tumor-infiltrating immune cells and is associated with poor outcome for patients with renal cell carcinoma. Clin Cancer Res. (2007) 13:1757–61. doi: 10.1158/1078-0432.CCR-06-2599
48. Choueiri TK, Fay AP, Gray KP, Callea M, Ho TH, Albiges L, et al. PD-L1 expression in nonclear-cell renal cell carcinoma. Ann Oncol. (2014) 25:2178–84. doi: 10.1093/annonc/mdu445
49. Thompson RH, Dong H, Kwon ED. Implications of B7-H1 expression in clear cell carcinoma of the kidney for prognostication and therapy. Clin Cancer Res. (2007) 13:709s–15s. doi: 10.1158/1078-0432.CCR-06-1868
50. Rini BI, Powles T, Atkins MB, Escudier B, McDermott DF, Suarez C, et al. Atezolizumab plus bevacizumab versus sunitinib in patients with previously untreated metastatic renal cell carcinoma (IMmotion151): a multicentre, open-label, phase 3, randomised controlled trial. Lancet. (2019) 393:2404–15. doi: 10.1016/S0140-6736(19)30723-8
51. Motzer RJ, Powles T, Atkins MB, Escudier B, McDermott DF, Alekseev BY, et al. Final overall survival and molecular analysis in IMmotion151, a phase 3 trial comparing atezolizumab plus bevacizumab vs sunitinib in patients with previously untreated metastatic renal cell carcinoma. JAMA Oncol. (2022) 8:275–80. doi: 10.1001/jamaoncol.2021.5981
52. Motzer RJ, Powles T, Burotto M, Escudier B, Bourlon MT, Shah AY, et al. Nivolumab plus cabozantinib versus sunitinib in first-line treatment for advanced renal cell carcinoma (CheckMate 9ER): long-term follow-up results from an open-label, randomised, phase 3 trial. Lancet Oncol. (2022) 23:888–98. doi: 10.1016/S1470-2045(22)00290-X
53. Rini BI, Plimack ER, Stus V, Gafanov R, Hawkins R, Nosov D, et al. Pembrolizumab plus Axitinib versus Sunitinib for Advanced Renal-Cell Carcinoma. N Engl J Med. (2019) 380:1116–27. doi: 10.1056/NEJMoa1816714
54. Dong M, Liu R, Zhang Q, Luo H, Wang D, Wang Y, et al. Efficacy and safety of carbon ion radiotherapy for bone sarcomas: a systematic review and meta-analysis. Radiat Oncol. (2022) 17:172. doi: 10.1186/s13014-022-02089-0
55. Nesslinger NJ, Ng A, Tsang KY, Ferrara T, Schlom J, Gulley JL, et al. A viral vaccine encoding prostate-specific antigen induces antigen spreading to a common set of self-proteins in prostate cancer patients. Clin Cancer Res. (2010) 16:4046–56. doi: 10.1158/1078-0432.CCR-10-0948
56. Gulley JL, Arlen PM, Bastian A, Morin S, Marte J, Beetham P, et al. Combining a recombinant cancer vaccine with standard definitive radiotherapy in patients with localized prostate cancer. Clin Cancer Res. (2005) 11:3353–62. doi: 10.1158/1078-0432.CCR-04-2062
57. Geng Y, Zhang Q, Feng S, Li C, Wang L, Zhao X, et al. Safety and Efficacy of PD-1/PD-L1 inhibitors combined with radiotherapy in patients with non-small-cell lung cancer: a systematic review and meta-analysis. Cancer Med. (2021) 10:1222–39. doi: 10.1002/cam4.3718
58. Formenti SC, Rudqvist NP, Golden E, Cooper B, Wennerberg E, Lhuillier C, et al. Radiotherapy induces responses of lung cancer to CTLA-4 blockade. Nat Med. (2018) 24:1845–51. doi: 10.1038/s41591-018-0232-2
59. DeSelm C, Palomba ML, Yahalom J, Hamieh M, Eyquem J, Rajasekhar VK, et al. Low-dose radiation conditioning enables CAR T cells to mitigate antigen escape. Mol Ther. (2018) 26:2542–52. doi: 10.1016/j.ymthe.2018.09.008
60. Fang PQ, Gunther JR, Wu SY, Dabaja BS, Nastoupil LJ, Ahmed S, et al. Radiation and CAR T-cell therapy in lymphoma: future frontiers and potential opportunities for synergy. Front Oncol. (2021) 11:648655. doi: 10.3389/fonc.2021.648655
61. Kanai T, Endo M, Minohara S, Miyahara N, Koyama-ito H, Tomura H, et al. Biophysical characteristics of HIMAC clinical irradiation system for heavy-ion radiation therapy. Int J Radiat Oncol Biol Phys. (1999) 44:201–10. doi: 10.1016/S0360-3016(98)00544-6
62. Liang S, Zhou G, Hu W. Research progress of heavy ion radiotherapy for non-small-cell lung cancer. Int J Mol Sci. (2022) 23:2316. doi: 10.3390/ijms23042316
63. Guo Y, Shen R, Wang F, Wang Y, Xia P, Wu R, et al. Carbon ion irradiation induces DNA damage in melanoma and optimizes the tumor microenvironment based on the cGAS-STING pathway. J Cancer Res Clin Oncol. (2023) 149:6315–28. doi: 10.1007/s00432-023-04577-6
64. Luo H, Yang Z, Zhang Q, Shao L, Wei S, Liu R, et al. Carbon ion therapy inhibits esophageal squamous cell carcinoma metastasis by upregulating STAT3 through the JAK2/STAT3 signaling pathway. Front Public Health. (2020) 8:579705. doi: 10.3389/fpubh.2020.579705
65. Ghiringhelli F, Ménard C, Martin F, Zitvogel L. The role of regulatory T cells in the control of natural killer cells: relevance during tumor progression. Immunol Rev. (2006) 214:229–38. doi: 10.1111/j.1600-065X.2006.00445.x
66. Hu W, Pei Y, Ning R, Li P, Zhang Z, Hong Z, et al. Immunomodulatory effects of carbon ion radiotherapy in patients with localized prostate cancer. J Cancer Res Clin Oncol. (2022) 149:4533–45. doi: 10.1007/s00432-022-04194-9
67. Ran J, Wang J, Dai Z, Miao Y, Gan J, Zhao C, et al. Irradiation-induced changes in the immunogenicity of lung cancer cell lines: based on comparison of X-rays and carbon ions. Front Public Health. (2021) 9:666282. doi: 10.3389/fpubh.2021.666282
68. Onishi M, Okonogi N, Oike T, Yoshimoto Y, Sato H, Suzuki Y, et al. High linear energy transfer carbon-ion irradiation increases the release of the immune mediator high mobility group box 1 from human cancer cells. J Radiat Res. (2018) 59:541–6. doi: 10.1093/jrr/rry049
69. Green DR, Ferguson T, Zitvogel L, Kroemer G. Immunogenic and tolerogenic cell death. Nat Rev Immunol. (2009) 9:353–63. doi: 10.1038/nri2545
70. De Martino M, Daviaud C, Vanpouille-Box C. Activin A backs-up TGF-ß to promote regulatory T cells. Oncoimmunology. (2021) 10:1883288. doi: 10.1080/2162402X.2021.1883288
71. De Martino M, Daviaud C, Diamond JM, Kraynak J, Alard A, Formenti SC, et al. Activin A promotes regulatory T-cell-mediated immunosuppression in irradiated breast cancer. Cancer Immunol Res. (2021) 9:89–102. doi: 10.1158/2326-6066.CIR-19-0305
72. Neumann C, Scheffold A, Rutz S. Functions and regulation of T cell-derived interleukin-10. Semin Immunol. (2019) 44:101344. doi: 10.1016/j.smim.2019.101344
73. Bah I, Kumbhare A, Nguyen L, McCall CE, El Gazzar M. IL-10 induces an immune repressor pathway in sepsis by promoting S100A9 nuclear localization and MDSC development. Cell Immunol. (2018) 332:32–8. doi: 10.1016/j.cellimm.2018.07.003
74. Huang Y, Dong Y, Zhao J, Zhang L, Kong L, Lu JJ. Comparison of the effects of photon, proton and carbon-ion radiation on the ecto-calreticulin exposure in various tumor cell lines. Ann Transl Med. (2019) 7:542. doi: 10.21037/atm
75. Obeid M, Tesniere A, Ghiringhelli F, Fimia GM, Apetoh L, Perfettini JL, et al. Calreticulin exposure dictates the immunogenicity of cancer cell death. Nat Med. (2007) 13:54–61. doi: 10.1038/nm1523
76. Carvalho HA, Villar RC. Radiotherapy and immune response: the systemic effects of a local treatment. Clinics (Sao Paulo). (2018) 73:e557s. doi: 10.6061/clinics/2018/e557s
77. Mole RH. Whole body irradiation; radiobiology or medicine? Br J Radiol. (1953) 26:234–41. doi: 10.1259/0007-1285-26-305-234
78. Formenti SC, Demaria S. Systemic effects of local radiotherapy. Lancet Oncol. (2009) 10:718–26. doi: 10.1016/S1470-2045(09)70082-8
79. Grass GD, Krishna N, Kim S. The immune mechanisms of abscopal effect in radiation therapy. Curr Probl Cancer. (2016) 40:10–24. doi: 10.1016/j.currproblcancer.2015.10.003
80. Rodríguez-Ruiz ME, Vanpouille-Box C, Melero I, Formenti SC, Demaria S. Immunological mechanisms responsible for radiation-induced abscopal effect. Trends Immunol. (2018) 39:644–55. doi: 10.1016/j.it.2018.06.001
81. Grimaldi AM, Simeone E, Giannarelli D, Muto P, Falivene S, Borzillo V, et al. Abscopal effects of radiotherapy on advanced melanoma patients who progressed after ipilimumab immunotherapy. Oncoimmunology. (2014) 3:e28780. doi: 10.4161/onci.28780
82. Komatsu T, Nakamura K, Kawase A. Abscopal effect of nivolumab in a patient with primary lung cancer. J Thorac Oncol. (2017) 12:e143–e4. doi: 10.1016/j.jtho.2017.05.004
83. Zhang YS, Zhang YH, Li XJ, Hu TC, Chen WZ, Pan X, et al. Bystander effect and abscopal effect in recurrent thymic carcinoma treated with carbon-ion radiation therapy: A case report. World J Clin cases. (2021) 9:6538–43. doi: 10.12998/wjcc.v9.i22.6538
84. Tubin S, Fossati P, Carlino A, Martino G, Gora J, Stock M, et al. Novel carbon ion and proton partial irradiation of recurrent unresectable bulky tumors (Particle-PATHY): early indication of effectiveness and safety. Cancers (Basel). (2022) 14:2232. doi: 10.3390/cancers14092232
85. Helm A, Tinganelli W, Simoniello P, Kurosawa F, Fournier C, Shimokawa T, et al. Reduction of lung metastases in a mouse osteosarcoma model treated with carbon ions and immune checkpoint inhibitors. Int J Radiat Oncol Biol Phys. (2021) 109:594–602. doi: 10.1016/j.ijrobp.2020.09.041
86. Huang Q, Sun Y, Wang W, Lin LC, Huang Y, Yang J, et al. Biological guided carbon-ion microporous radiation to tumor hypoxia area triggers robust abscopal effects as open field radiation. Front Oncol. (2020) 10:597702. doi: 10.3389/fonc.2020.597702
87. Janopaul-Naylor JR, Shen Y, Qian DC, Buchwald ZS. The abscopal effect: A review of pre-clinical and clinical advances. Int J Mol Sci. (2021) 22:11061. doi: 10.3390/ijms222011061
88. Daguenet E, Louati S, Wozny AS, Vial N, Gras M, Guy JB, et al. Radiation-induced bystander and abscopal effects: important lessons from preclinical models. Br J Cancer. (2020) 123:339–48. doi: 10.1038/s41416-020-0942-3
89. Marcus D, Lieverse RIY, Klein C, Abdollahi A, Lambin P, Dubois LJ, et al. Charged particle and conventional radiotherapy: current implications as partner for immunotherapy. Cancers (Basel). (2021) 13:1468. doi: 10.3390/cancers13061468
90. Binnewies M, Roberts EW, Kersten K, Chan V, Fearon DF, Merad M, et al. Understanding the tumor immune microenvironment (TIME) for effective therapy. Nat Med. (2018) 24:541–50. doi: 10.1038/s41591-018-0014-x
Keywords: renal cell carcinoma (RCC), radiotherapy, immunotherapy, carbon ion radiotherapy, combination therapy
Citation: Zheng Z, Yang T, Li Y, Qu P, Shao Z, Wang Y, Chang W, Umar SM, Wang J, Ding N and Wang W (2024) A future directions of renal cell carcinoma treatment: combination of immune checkpoint inhibition and carbon ion radiotherapy. Front. Immunol. 15:1428584. doi: 10.3389/fimmu.2024.1428584
Received: 06 May 2024; Accepted: 05 July 2024;
Published: 18 July 2024.
Edited by:
Haoran Feng, Ruijin Hospital, ChinaReviewed by:
Hailong Pei, Soochow University, ChinaDafei Xie, Academy of Military Medical Sciences (AMMS), China
Copyright © 2024 Zheng, Yang, Li, Qu, Shao, Wang, Chang, Umar, Wang, Ding and Wang. This is an open-access article distributed under the terms of the Creative Commons Attribution License (CC BY). The use, distribution or reproduction in other forums is permitted, provided the original author(s) and the copyright owner(s) are credited and that the original publication in this journal is cited, in accordance with accepted academic practice. No use, distribution or reproduction is permitted which does not comply with these terms.
*Correspondence: Jufang Wang, anVmYW5nd2FuZ0BpbXBjYXMuYWMuY24=; Nan Ding, ZG5AaW1wY2FzLmFjLmNu; Wei Wang, d2FuZ3dlaTIwMTFAbHp1LmVkdS5jbg==