- 1Department of Medicine, Roswell Park Comprehensive Cancer Center, Buffalo, NY, United States
- 2Department of Internal Medicine, King Abdulaziz University Hospital, Jeddah, Saudi Arabia
- 3Department of Immunology, Roswell Park Comprehensive Cancer Center, Buffalo, NY, United States
- 4Department of Cancer Prevention and Control, Roswell Park Comprehensive Cancer Center, Buffalo, NY, United States
Triple negative breast cancer (TNBC) is a heterogenous disease that disproportionately affects Black women. TNBC outcomes among Black women are dismal secondary to multiple factors, such as poor healthcare accessibility resulting in delays in diagnosis, and aggressive disease biology in addition to a pro-tumor immune microenvironment (TME). Black women with breast cancer exhibit elevated levels of serum pro-inflammatory cytokines, and a pro-tumorigenic TME with higher immunosuppressive regulatory T cells (Tregs), M2 macrophages and exhausted CD8+ T cells. We have shown that the combined use of toll-like receptor 3 (TLR3) ligands with interferon-α (chemokine modulation: CKM) is able to enrich the tumor with CD8+ T cells, while not increasing immunosuppressive cells. Recent clinical trials have revealed the efficacy of immune checkpoint inhibitors (ICI) in rejuvenizing exhausted CD8+ T cells. We hypothesize that strategies to modulate the TME by enriching chemokines that attract CD8+T cells followed by reversal of CD8+ T cell exhaustion (ICI), when added to standard treatment, could potentially improve clinical outcomes, and mitigate the racial disparities in TNBC outcomes between Black and White Women.
Introduction
Triple negative breast cancer (TNBC) accounts for 15- 20% of all breast cancers (1), higher among Black population, with an age-adjusted incidence of 33.8 cases per 100,000, compared to 17.5 per 100,00 cases in White women (2). Additionally, TNBC in Black individuals carries a higher mortality rate of 29.2 out of 100,000 compared to 20.6 out of 100,000 in White women (3). Besides other factors associated with poor access to care, and delayed diagnosis; biological factors such as differences in tumor biology (4), tumor microenvironment (TME) and host immunity (5), contribute to racial disparities in clinical outcomes of TNBC; thus, there has been a recent interest in exploring the role of TME in breast cancer racial disparities. However, the under-representation of Black patients in clinical trials, including those with immunotherapy, has impeded the understanding of TNBC disparities, as evidenced by the lack of race data and race-specific clinical outcomes reported in KEYNOTE-522 (6) and GeparNuevo (7) and by the inclusion of only a few Black women (9%) in the pembrolizumab arm of I-SPY2 trial (8).
Understanding differences in tumor microenvironment across breast cancer from different racial groups
Tumor infiltrating lymphocytes and exhausted CD8+ T cells
TIL infiltration has been shown to be an independent prognostic marker in TNBC. Prior TNBC Phase III trials have shown that high stromal and intratumoral TIL numbers are associated with improved disease-free survival (DFS), distant recurrence-free survival (DRFS), and overall survival (OS) (9). A previous study from our group based on a cohort of 1315 patients (920 Black patients and 395 White patients) showed that tumors from Black patients expressed significantly higher pathological TIL score compared to White patients (P<0.001) (10). Although there is no racial difference in the fraction of CD8+ T cells, tumors from Black patients have higher expression of CD8+ T cell exhaustion markers (PD-1, LAG3) and eomesodermin (EOMES), a pivotal transcription factor upregulated in exhausted CD8+ T cells (11). An exhausted CD8+ T cell signature, defined as the ratio of aggregated expressions of PD-1, LAG-3, and EOMES to the absolute CD8+ T cell fraction, was also elevated in tumors from Black compared to White patients (P<0.001), and associated with worse survival (10). Thus, while Black patients have higher TILs in the TME, such TILs are more likely to be in an exhausted state, which may explain patients’ poorer survival (10). There are also more tumor-associated macrophages (TAM), specifically M2 macrophages in tumors in Black patients (12) which may contribute to CD8+ T cell exhaustion (13, 14).CD8+ T cell exhaustion is a dynamic process where effector CD8+ T cells progressively lose their cytotoxic function and ability to produce effector cytokines. This process happens due to overstimulation, chronic tumor antigenic exposure, hypoxia, presence of regulatory T cells (Tregs) or myeloid-derived suppressor cells (MDSCs), and immunosuppressive cytokines [interleukin-10 (IL-10) and transforming growth factor-β (TGF-β)] (15, 16). Exhausted CD8+ T cells may express inhibitory receptors, such as, cytotoxic T lymphocyte antigen-4 (CTLA-4), programmed cell death protein 1 (PD-1), lymphocyte activation gene 3 protein (LAG-3), B and T-lymphocyte attenuator (BTLA), and T cell immunoglobulin domain and mucin domain protein 3 (TIM-3) on their surface. Exhausted cells have limited anti-tumor potential. To counteract this exhausted state, strategies using single or combined checkpoint blockade such as anti-CTLA-4, anti-PD-1/PDL-1, or LAG-3 blockade combined with anti-PD-1, or combination of anti-TIM3 with anti-PD-1 have proven effective in reversing CD8+ T cell exhaustion and restoring its cytotoxic function through epigenetic reprogramming (17) (Figure 1).
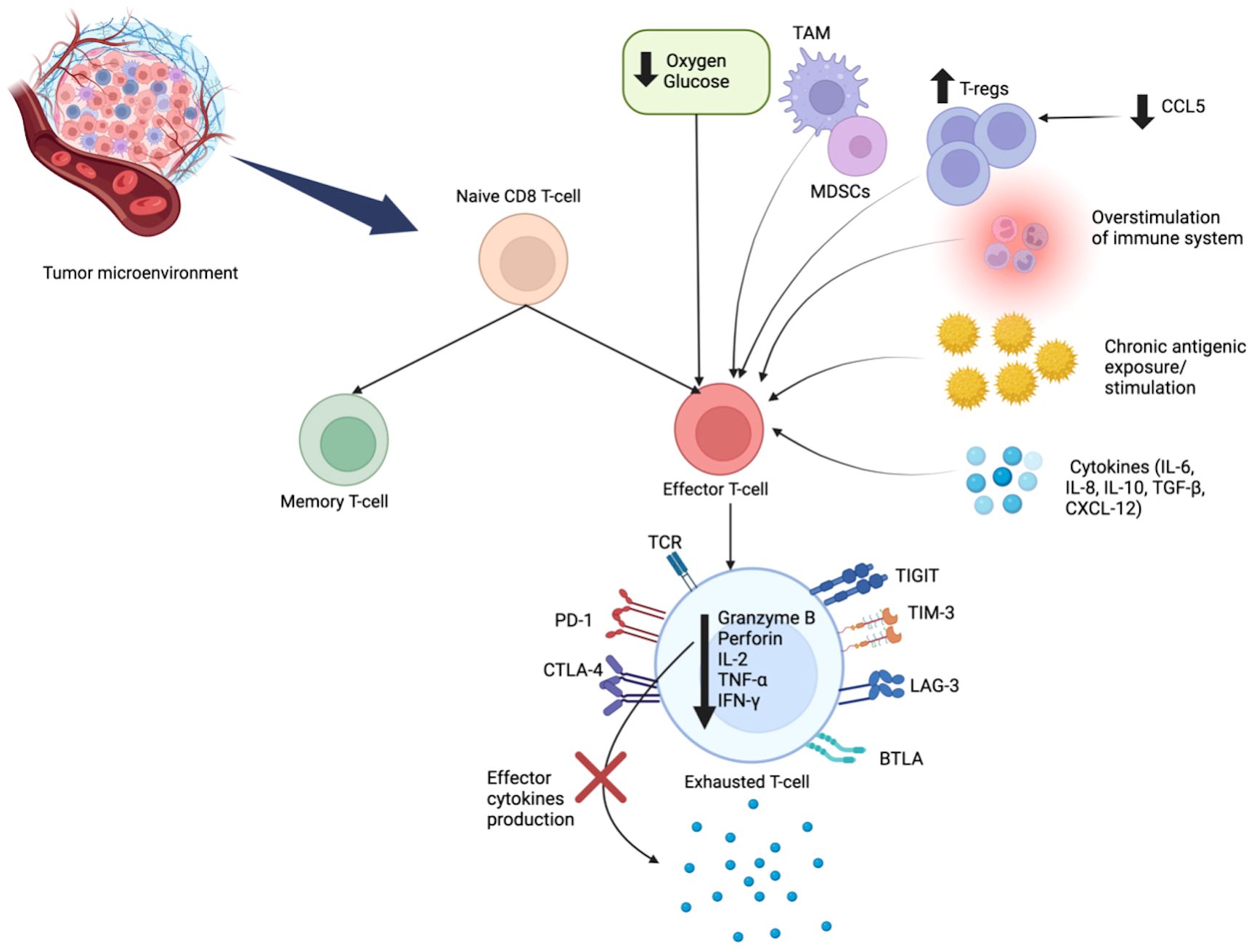
Figure 1 Mechanisms of CD8+ T Cell Exhaustion. CD8+ T cell exhaustion is a dynamic process, resulting from chronic antigenic overstimulation, hypoxia, and the immunosuppressive cells (TAM, MDSCs, Tregs) and cytokines (IL-10, TGF-β). In this state, effector cells progressively lose their cytotoxic ability. Exhausted CD8+ T cells have a unique metabolic pattern and epigenetic features, with sustained expression of inhibitory receptors: CTLA-4, PD-1, BTLA, LAG-3, TIM-3 and TIGIT. Exhausted T cells have decreased production of granzyme B, perforin, and effector cytokines (IL-2, TNF-α, IFN-γ). IL, interleukin; TNF-α, tumor necrosis factor alpha; IFN-γ, interferon gamma; Tregs, regulatory T cells; MDSCs, myeloid derived suppressor cells; TAM, tumor-associated macrophages; TGF-β, Transforming growth factor beta; CTLA-4, T lymphocyte antigen-4; PD-1, programmed cell death protein-1; LAG-3, lymphocyte activation gene 3 protein; BTLA, B and T-lymphocyte attenuator; TIM-3, T cell immunoglobulin domain and mucin domain protein 3; TIGIT, T cell immunoreceptor with Ig and ITIM domains.
Differences in cytokine and chemokine milieu across racial groups
Black patients with TNBC have higher plasma levels of IL-6, IL-8, and granulocyte colony-stimulating factor (12). Additionally, Black patients with breast cancer have an overall immunosuppressive TME due to the predominance of cytokines such as: IL-10 and TGF- β (18), and chemokines, such as, CCL7, CXCL8, CCL8, CCL17, CCL29, CCL25 (19) which may exert pro-tumor effects. Furthermore, tumors from Black women exhibit higher expression of Tregs (5) due to elevated level of CXCL12, which attracts pro-tumorigenic Tregs (18).
Differences in genetic expressions across racial groups
Studies have shown that the mutation rates and intra-tumoral heterogeneity are higher in Black patients with TNBC compared to whites (20, 21). In a study by Kennan et al, Black patients were noted to have higher incidence of TP53 mutations compared to whites (20). Chang CS et al. reported that Black and white patients with TNBC had similar frequency of mutations in the commonly mutated genes such as TP53, MUC4, and MUC6 (21); however, Black patients are less likely to harbor PIK3CA and BRCA alterations compared to white patients, making agents targeting these mutations to be less beneficial in these patients (22). Black patients also have a higher number of mutations in JAK/STAT5, HER2, and ERBB2/ ERBB3 signaling pathways compared to whites, conferring poor prognosis (21). Moreover, they have increased expressions of vascular endothelial growth factor activator genes, leading to high tumor vascularization, which promotes tumor growth and metastasis (23). There is emerging evidence that Black patients with TNBC have high Enhancer of Zeste homologue 2 (EZH2) overexpression, which has been shown to induce AKT-dependent genomic instability in the TME and block BRCA1 function (24). In addition, significant enrichment of dysregulated genes associated with the WNT-ß catenin pathway was observed in Black patients, suggesting that activation of this pathway could contribute to the aggressive nature of disease seen in Black women (24). Interestingly, younger Black patients (<50 years) with TNBC were found to have a distinct DNA methylation landscape with significant differences in the expression of hormone pathway mediators such as ESR1, AR, and ESRRA, compared to white patients and older Black patients, suggesting a hormone unresponsive phenotype that may be associated with aggressive disease in this patient group (25). The key differences in tumor intrinsic and TME parameters between Black and White patients are summarized in Table 1.
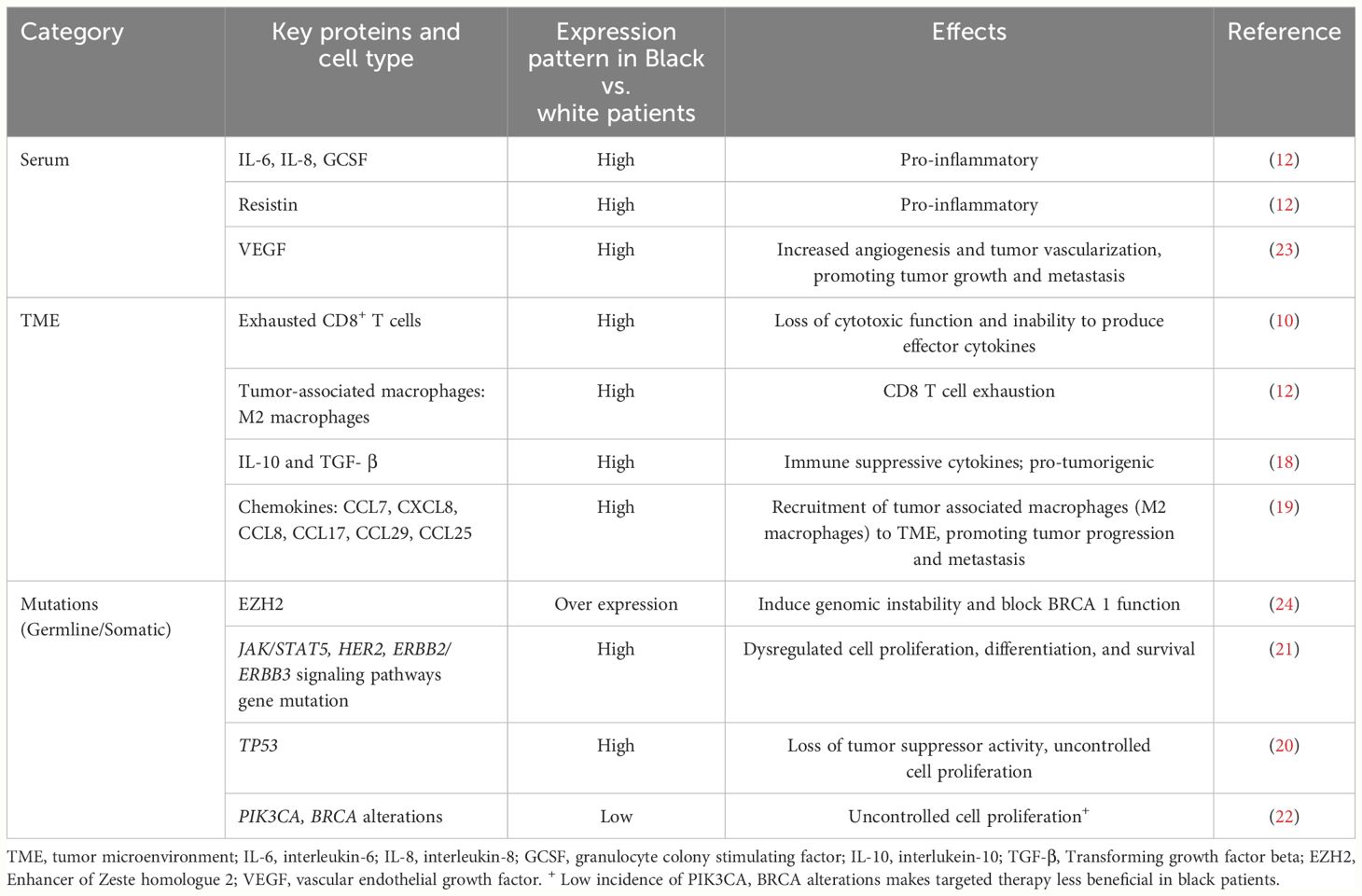
Table 1 Characteristics of blood, tumor microenvironment and mutations in breast cancer among Black women.
Potential strategies to counteract CD8+ T cell exhaustion in TNBC among black patients
Checkpoint inhibitors
Clinical trials that examined the impact of checkpoint inhibitors in TNBC in the neoadjuvant setting and assessed outcomes by race, are outlined in Table 2. In the NeoPACT (26) trial, which tested a combination of carboplatin, docetaxel and pembrolizumab, Black patients were observed to have higher stromal TILs than white patients. Black patients also achieved higher pathological complete response (pCR) rates compared to White patients (OR 3.27, 95% CI 1.01-10.64, P= 0.049) and longer 3-year event-free survival (EFS), supporting the notion of the potential benefit of immune checkpoint blockade in reversing CD8+ T cell exhaustion in Black patients. In contrast, findings from another trial by Foldi et al. (27) that included an extended cohort of Black patients in early-stage TNBC receiving neoadjuvant chemotherapy (doxorubicin-cyclophosphamide and paclitaxel) with durvalumab, showed no significant differences in pCR rate, EFS or overall survival by race. Additionally, there were no significant differences in stromal TILs or PDL-1 status by race. These varying outcomes by race in the two trials could be attributed to the use of different chemotherapeutic regimens and ICIs.
Chemokine modulation
Toll-like receptor 3 (TLR3) is an innate immune receptor expressed in immune cells and cancer cells that senses exogenous and endogenous endosomal double stranded RNA and is crucial in generating anti-microbial and tumor specific immune responses (28). Upon activation, it triggers a signal transduction pathway that leads to the production of pro-inflammatory cytokines, including type I interferon and chemokines, which recruits and activates immune cells (28). In addition to acting as an immunomodulator of TME, TLR3 can directly induce apoptosis of cancer cells (29). Studies have shown that interferon-α increases the surface expression of tumor-associated antigens in breast cancer cells and promote recognition of these antigens by T cells (30). Moreover, interferon-α promotes expression of molecules such as granzyme B, and perforin in effector T cells, which increases the cytotoxic effect of T cells (30). Our preclinical work demonstrated that combining toll-like receptor 3 (TLR3) ligands with interferon-α synergistically enhances the desirable CTL attractants and reduces the Treg and MDSC attractants in the TME (31, 32). In our recent clinical trial (NCT03599453) (33), the chemokine modulatory (CKM) regimen composed of a TLR3 agonist, interferon-α and celecoxib was administered to patients with metastatic TNBC, followed sequentially by pembrolizumab. CKM selectively increased intratumoral CTL attractants such as CCL5 or CXCL10, but not the Treg attractants CCL22 or CXCL12. Our trial further indicates an increased infiltration of CTLs into the tumor, and intratumoral granzyme B signature was also elevated. These observations point to the potential of CKM as a complementary strategy to immune checkpoint inhibitors, as CKM results in infiltration of CD8+T cells into the tumor and immune checkpoint inhibitors could reverse the exhaustion signature on these CD8+T cells. Both strategies would work synergistically to rejuvenate CD8+ T cell function (Figure 2).
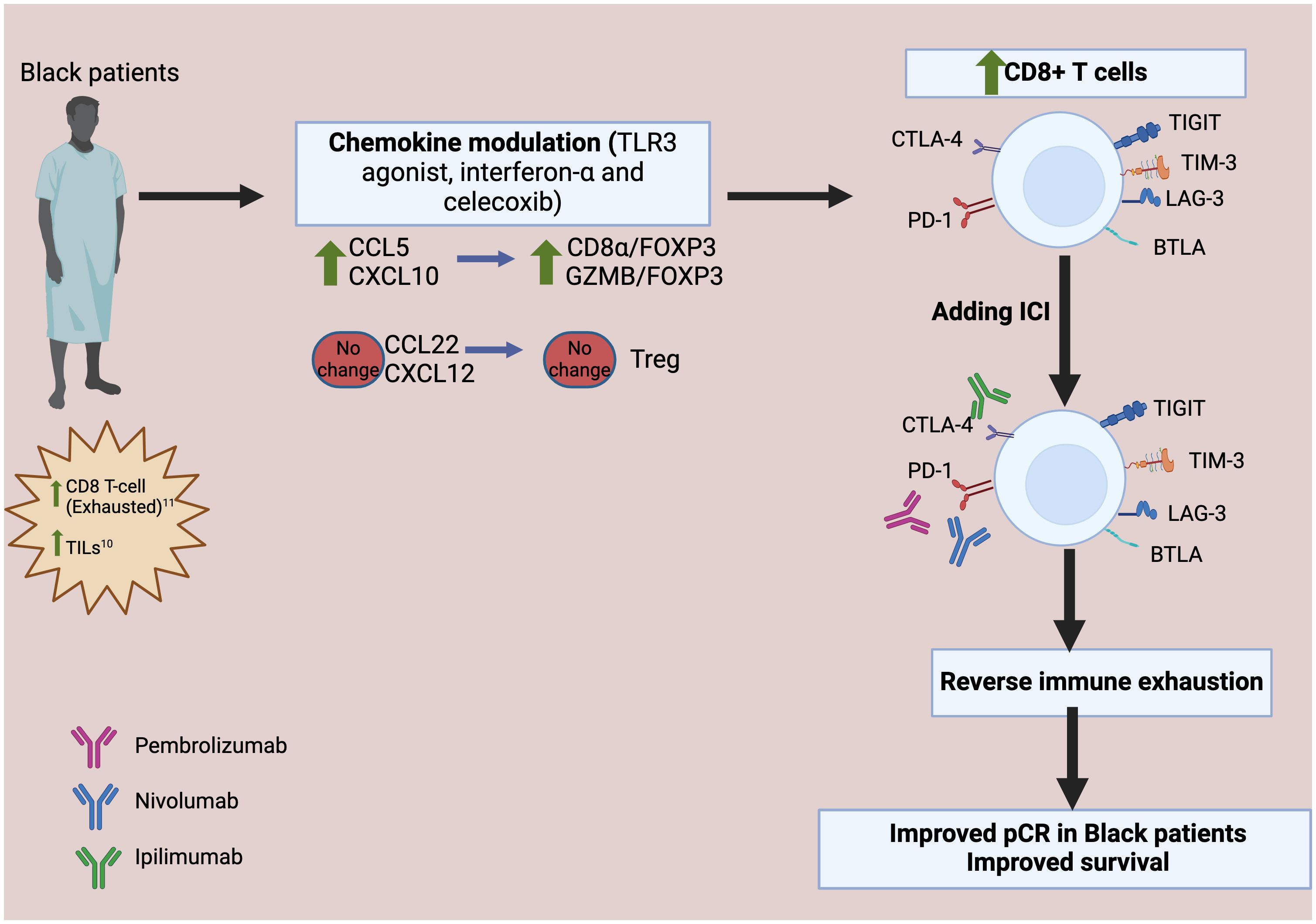
Figure 2 Proposed therapeutic interventions utilizing chemokine modulation and checkpoint blockade to reverse CD8+ T Cells exhaustion in Black Patients. The chemokine modulatory regimen selectively increases CCL5 and CXCL10, which attract cytotoxic T lymphocytes (CTL), while not increasing CCL22 or CXCL12. This leads to increased infiltration of the effector-type CTL, reflected by higher level of granzyme B FOXP3 is a marker of Treg; CD8α is a marker of CD8+ cytotoxic T cells. Upon the addition of immune checkpoint inhibitors (ICI), the exhaustion markers expressed in the exhausted CD8+T cells are blocked, thereby reversing immune exhaustion. TILs, tumor-infiltrating lymphocytes; GZMB, Granzyme B; CTL, cytotoxic T lymphocytes; Treg, regulatory T cells; pCR, pathologic complete response; CTLA-4, T lymphocyte antigen-4; PD-1, programmed cell death protein-1; LAG-3, lymphocyte activation gene 3 protein; BTLA, B and T-lymphocyte attenuator; TIM-3, T cell immunoglobulin domain and mucin domain protein 3; TIGIT, T cell immunoreceptor with Ig and ITIM domains.
Future directions
Given the link between biased chemokine patterns in Black TNBC patients, compared to Caucasian patients observed in our recent studies and the ability of CKM to correct such bias observed in our recent Phase I study, we propose that a dual strategy combining chemokine modulation with immune checkpoint inhibitors may have the potential to emerge as a novel approach to address racial disparity within the TME of TNBC, especially among Black patients.
Data availability statement
The original contributions presented in the study are included in the article/supplementary material. Further inquiries can be directed to the corresponding author.
Author contributions
MA: Writing – original draft, Writing – review & editing. AR: Writing – review & editing, Conceptualization. JK: Writing – review & editing. PK: Writing – review & editing. SY: Writing – review & editing. SG: Conceptualization, Supervision, Writing – original draft, Writing – review & editing.
Funding
The author(s) declare financial support was received for the research, authorship, and/or publication of this article. Research reported in this publication was supported by the National Center for Advancing Translational Sciences of the National Institutes of Health under award number KL2TR001413, UL1TR00412, and R03TR004607-01, and National Cancer Institute of the National Institutes of Health under Award Number K08CA279766.
Conflict of interest
The authors declare that the research was conducted in the absence of any commercial or financial relationships that could be construed as a potential conflict of interest.
Publisher’s note
All claims expressed in this article are solely those of the authors and do not necessarily represent those of their affiliated organizations, or those of the publisher, the editors and the reviewers. Any product that may be evaluated in this article, or claim that may be made by its manufacturer, is not guaranteed or endorsed by the publisher.
Author disclaimer
The content is solely the responsibility of the authors and does not necessarily represent the official views of the National Institutes of Health.
References
1. Yin L, Duan JJ, Bian XW, Yu SC. Triple-negative breast cancer molecular subtyping and treatment progress. Breast Cancer Res. (2020) 22:61. doi: 10.1186/s13058-020-01296-5
2. Du XL, Li Z. Incidence trends in triple-negative breast cancer among women in the United States from 2010 to 2019 by race/ethnicity, age and tumor stage. Am J Cancer Res. (2023) 13(2):678–91. doi: 10.3389/fonc.2023.1292577
3. Yedjou CG, Sims JN, Miele L, Noubissi F, Lowe L, Fonseca DD, et al. Health and racial disparity in breast cancer. Adv Exp Med Biol. (2019) 1152:31–49. doi: 10.1007/978-3-030-20301-6_3
4. Siddharth S, Sharma D. Racial disparity and triple-negative breast cancer in African-American women: A multifaceted affair between obesity, biology, and socioeconomic determinants. Cancers (Basel). (2018) 10(12):514. doi: 10.3390/cancers10120514
5. Kim G, Pastoriza JM, Condeelis JS, Sparano JA, Filippou PS, Karagiannis GS, et al. The contribution of race to breast tumor microenvironment composition and disease progression. Front Oncol. (2020) 10:1022. doi: 10.3389/fonc.2020.01022
6. Schmid P, Cortes J, Pusztai L, McArthur H, Kümmel S, Bergh J, et al. Pembrolizumab for early triple-negative breast cancer. N Engl J Med. (2020) 382:810–21. doi: 10.1056/NEJMoa1910549
7. Loibl S, Untch M, Burchardi N, Huober J, Sinn BV, Blohmer JU, et al. A randomised phase II study investigating durvalumab in addition to an anthracycline taxane-based neoadjuvant therapy in early triple-negative breast cancer: clinical results and biomarker analysis of GeparNuevo study. Ann Oncol. (2019) 30:1279–88. doi: 10.1093/annonc/mdz158
8. Nanda R, Liu MC, Yau C, Shatsky R, Pusztai L, Wallace A, et al. Effect of pembrolizumab plus neoadjuvant chemotherapy on pathologic complete response in women with early-stage breast cancer: an analysis of the ongoing phase 2 adaptively randomized I-SPY2 trial. JAMA Oncol. (2020) 6:676–84. doi: 10.1001/jamaoncol.2019.6650
9. Dieci MV, Mathieu MC, Guarneri V, Conte P, Delaloge S, Andre F, et al. Prognostic and predictive value of tumor-infiltrating lymphocytes in two phase III randomized adjuvant breast cancer trials. Ann Oncol. (2015) 26:1698–704. doi: 10.1093/annonc/mdv239
10. Yao S, Cheng TD, Elkhanany A, Yan L, Omilian A, Abrams SI, et al. Breast tumor microenvironment in black women: A distinct signature of CD8+ T-cell exhaustion. J Natl Cancer Inst. (2021) 113:1036–43. doi: 10.1093/jnci/djaa215
11. Li J, He Y, Hao J, Ni L, Dong C. High levels of eomes promote exhaustion of anti-tumor CD8(+) T cells. Front Immunol. (2018) 9:2981. doi: 10.3389/fimmu.2018.02981
12. Zajac KK, Malla S, Babu RJ, Raman D, Tiwari AK. Ethnic disparities in the immune microenvironment of triple negative breast cancer and its role in therapeutic outcomes. Cancer Rep (Hoboken). (2023) 6 Suppl 1:e1779. doi: 10.1002/cnr2.1779
13. Intlekofer AM, Takemoto N, Wherry EJ, Longworth SA, Northrup JT, Palanivel VR, et al. Effector and memory CD8+ T cell fate coupled by T-bet and eomesodermin. Nat Immunol. (2005) 6:1236–44. doi: 10.1038/ni1268
14. Wherry EJ, Kurachi M. Molecular and cellular insights into T cell exhaustion. Nat Rev Immunol. (2015) 15:486–99. doi: 10.1038/nri3862
15. Zhu W, Li Y, Han M, Jiang J. Regulatory mechanisms and reversal of CD8(+)T cell exhaustion: A literature review. Biol (Basel). (2023) 12(4):541. doi: 10.3390/biology12040541
16. Jin HT, Jeong YH, Park HJ, Ha SJ. Mechanism of T cell exhaustion in a chronic environment. BMB Rep. (2011) 44:217–31. doi: 10.5483/BMBRep.2011.44.4.217
17. Granier C, De Guillebon E, Blanc C, Roussel H, Badoual C, Colin E, et al. Mechanisms of action and rationale for the use of checkpoint inhibitors in cancer. ESMO Open. (2017) 2:e000213. doi: 10.1136/esmoopen-2017-000213
18. Habanjar O, Bingula R, Decombat C, Diab-Assaf M, Caldefie-Chezet F, Delort L. Crosstalk of inflammatory cytokines within the breast tumor microenvironment. Int J Mol Sci. (2023) 24(4):4002. doi: 10.3390/ijms24044002
19. Thomas JK, Mir H, Kapur N, Bae S, Singh S. CC chemokines are differentially expressed in Breast Cancer and are associated with disparity in overall survival. Sci Rep. (2019) 9:4014. doi: 10.1038/s41598-019-40514-9
20. Keenan T, Moy B, Mroz EA, Ross K, Niemierko A, Rocco JW, et al. Comparison of the genomic landscape between primary breast cancer in African American versus white women and the association of racial differences with tumor recurrence. J Clin Oncol. (2015) 33:3621–7. doi: 10.1200/JCO.2015.62.2126
21. Chang CS, Kitamura E, Johnson J, Bollag R, Hawthorn L. Genomic analysis of racial differences in triple negative breast cancer. Genomics. (2019) 111:1529–42. doi: 10.1016/j.ygeno.2018.10.010
22. Park SH, Khalife R, White E, Magliocco A. (2023). Distinct molecular differences between African American/Black and White women with Triple Negative Breast Cancer, in: Proceedings of the 2022 San Antonio Breast Cancer Symposium, San Antonio, TX. Philadelphia (PA) (Philadelphia, PA: American Association for Cancer Research), 2022 Dec 6-10, Vol. 83.
23. Lindner R, Sullivan C, Offor O, Lezon-Geyda K, Halligan K, Fischbach N, et al. Molecular phenotypes in triple negative breast cancer from African American patients suggest targets for therapy. PloS One. (2013) 8:e71915. doi: 10.1371/journal.pone.0071915
24. Dietze EC, Sistrunk C, Miranda-Carboni G, O'Regan R, Seewaldt VL. Triple-negative breast cancer in African-American women: disparities versus biology. Nat Rev Cancer. (2015) 15:248–54. doi: 10.1038/nrc3896
25. Ensenyat-Mendez M, Solivellas-Pieras M, Llinàs-Arias P, Íñiguez-Muñoz S, Baker JL, Marzese DM, et al. Epigenetic profiles of triple-negative breast cancers of African American and white females. JAMA Netw Open. (2023) 6:e2335821. doi: 10.1001/jamanetworkopen.2023.35821
26. Sharma P, Stecklein SR, Yoder R, Staley JM, Schwensen K, O'Dea A, et al. Clinical and biomarker results of neoadjuvant phase II study of pembrolizumab and carboplatin plus docetaxel in triple-negative breast cancer (TNBC) (NeoPACT). J Clin Oncol. (2022) 40:513. doi: 10.1200/JCO.2022.40.16_suppl.513
27. Foldi J, Kahn A, Silber A, Qing T, Reisenbichler E, Fischbach N, et al. Clinical outcomes and immune markers by race in a phase I/II clinical trial of durvalumab concomitant with neoadjuvant chemotherapy in early-stage TNBC. Clin Cancer Res. (2022) 28:3720–8. doi: 10.1158/1078-0432.CCR-22-0862
28. Thierry S, Maadadi S, Berton A, Dimier L, Perret C, Vey N, et al. TL-532, a novel specific Toll-like receptor 3 agonist rationally designed for targeting cancers: discovery process and biological characterization. Microb Cell. (2023) 10:117–32. doi: 10.15698/mic2023.06.797
29. Salaun B, Coste I, Rissoan MC, Lebecque SJ, Renno T. TLR3 can directly trigger apoptosis in human cancer cells. J Immunol. (2006) 176:4894–901. doi: 10.4049/jimmunol.176.8.4894
30. Zhang X, Wang S, Zhu Y, Zhang M, Zhao Y, Yan Z, et al. Double-edged effects of interferons on the regulation of cancer-immunity cycle. Oncoimmunology. (2021) 10:1929005. doi: 10.1080/2162402X.2021.1929005
31. Muthuswamy R, Berk E, Junecko BF, Zeh HJ, Zureikat AH, Normolle D, et al. NF-κB hyperactivation in tumor tissues allows tumor-selective reprogramming of the chemokine microenvironment to enhance the recruitment of cytolytic T effector cells. Cancer Res. (2012) 72:3735–43. doi: 10.1158/0008-5472.CAN-11-4136
32. Muthuswamy R, Corman JM, Dahl K, Chatta GS, Kalinski P. Functional reprogramming of human prostate cancer to promote local attraction of effector CD8(+) T cells. Prostate. (2016) 76:1095–105. doi: 10.1002/pros.v76.12
Keywords: triple negative breast cancer, racial disparities, tumor microenvironment, exhausted CD8 + T cells, TLR3 agonist
Citation: Alharbi M, Roy AM, Krishnan J, Kalinski P, Yao S and Gandhi S (2024) Targeting the tumor microenvironment to improve clinical outcomes in triple negative breast cancer patients and bridge the current disparity gap. Front. Immunol. 15:1428118. doi: 10.3389/fimmu.2024.1428118
Received: 05 May 2024; Accepted: 01 July 2024;
Published: 12 July 2024.
Edited by:
Chun Wai Mai, UCSI University, MalaysiaReviewed by:
Yaying Sun, Fudan University, ChinaSayali Onkar, Icahn School of Medicine at Mount Sinai, United States
Copyright © 2024 Alharbi, Roy, Krishnan, Kalinski, Yao and Gandhi. This is an open-access article distributed under the terms of the Creative Commons Attribution License (CC BY). The use, distribution or reproduction in other forums is permitted, provided the original author(s) and the copyright owner(s) are credited and that the original publication in this journal is cited, in accordance with accepted academic practice. No use, distribution or reproduction is permitted which does not comply with these terms.
*Correspondence: Shipra Gandhi, Shipra.gandhi@roswellpark.org