- 1Stanford Center for Sleep Science and Medicine, Stanford University, Palo Alto, CA, United States
- 2French Reference Center on Paraneoplastic Neurological Syndrome and Autoimmune Encephalitis, Hospices Civils de Lyon, Lyon, France
- 3Institut MeLiS INSERM U1314/CNRS UMR 5284, Université Claude Bernard Lyon 1, Lyon, France
- 4Department of Neurology, Erasmus Medical Center, Rotterdam, Netherlands
- 5Department of Neurology and Experimental Neurology, Charité-Universitätsmedizin Berlin, Berlin, Germany
- 6Berlin School of Mind and Brain, Humboldt-Universität zu Berlin, Berlin, Germany
- 7Department of Neurology, Christian-Albrechts-University/University Hospital Schleswig-Holstein, Kiel, Germany
- 8Neuroimmunology, Institute of Clinical Chemistry, University Hospital Schleswig-Holstein Kiel/Lübeck, Kiel, Germany
- 9German Network for Research on Autoimmune Encephalitis, Germany
- 10Starship Hospital, Centre for Brain Research, Faculty of Medical and Health Sciences, University of Auckland, Auckland, New Zealand
- 11Kids Neuroscience Centre, Children’s Hospital at Westmead clinical school, University of Sydney, Sydney, NSW, Australia
- 12Oxford Autoimmune Neurology Group, Nuffield Department of Clinical Neurosciences, University of Oxford, Oxford, United Kingdom
- 13Department of Neurology, John Radcliffe Hospital, Oxford, United Kingdom
- 14Departments of Neurology and Neurosciences, Mayo Clinic, Jacksonville, FL, United States
- 15Department of Immunogenetics and Transplantation Immunology, Leiden University Medical Center, Leiden, Netherlands
- 16Department of Neurology, Ajou University School of Medicine, Suwon, Republic of Korea
- 17Department of Neurology, Seoul National University Hospital, Seoul National University College of Medicine, Seoul, Republic of Korea
- 18Department of Neurology, Teikyo University School of Medicine, Tokyo, Japan
- 19Department of Biomedical Informatics, University of Colorado School of Medicine, Aurora, CO, United States
- 20Department of Immunology and Microbiology, University of Colorado School of Medicine, Aurora, CO, United States
Introduction: Genetic predisposition to autoimmune encephalitis with antibodies against N-methyl-D-aspartate receptor (NMDAR) is poorly understood. Given the diversity of associated environmental factors (tumors, infections), we hypothesized that human leukocyte antigen (HLA) and killer-cell immunoglobulin-like receptors (KIR), two extremely polymorphic gene complexes key to the immune system, might be relevant for the genetic predisposition to anti-NMDAR encephalitis. Notably, KIR are chiefly expressed by Natural Killer (NK) cells, recognize distinct HLA class I allotypes and play a major role in anti-tumor and anti-infection responses.
Methods: We conducted a Genome Wide Association Study (GWAS) with subsequent control-matching using Principal Component Analysis (PCA) and HLA imputation, in a multi-ethnic cohort of anti-NMDAR encephalitis (n=479); KIR and HLA were further sequenced in a large subsample (n=323). PCA-controlled logistic regression was then conducted for carrier frequencies (HLA and KIR) and copy number variation (KIR). HLA-KIR interaction associations were also modeled. Additionally, single cell sequencing was conducted in peripheral blood mononuclear cells from 16 cases and 16 controls, NK cells were sorted and phenotyped.
Results: Anti-NMDAR encephalitis showed a weak HLA association with DRB1*01:01~DQA1*01:01~DQB1*05:01 (OR=1.57, 1.51, 1.45; respectively), and DRB1*11:01 (OR=1.60); these effects were stronger in European descendants and in patients without an underlying ovarian teratoma. More interestingly, we found increased copy number variation of KIR2DL5B (OR=1.72), principally due to an overrepresentation of KIR2DL5B*00201. Further, we identified two allele associations in framework genes, KIR2DL4*00103 (25.4% vs. 12.5% in controls, OR=1.98) and KIR3DL3*00302 (5.3% vs. 1.3%, OR=4.44). Notably, the ligands of these KIR2DL4 and KIR3DL3, respectively, HLA-G and HHLA2, are known to act as immune checkpoint with immunosuppressive functions. However, we did not find differences in specific KIR-HLA ligand interactions or HLA-G polymorphisms between cases and controls. Similarly, gene expression of CD56dim or CD56bright NK cells did not differ between cases and controls.
Discussion: Our observations for the first time suggest that the HLA-KIR axis might be involved in anti-NMDAR encephalitis. While the genetic risk conferred by the identified polymorphisms appears small, a role of this axis in the pathophysiology of this disease appears highly plausible and should be analyzed in future studies.
Introduction
Encephalitis with antibodies against N-methyl-D-aspartate receptor (NMDAR) is one of the most common autoimmune encephalitides and has recently been considered of intermediate risk for a paraneoplastic origin based on a tumor association rate of approximately 40% (1, 2). However, tumors are extremely rare in children and young men, ovarian teratomas are present in about 50% of women during their fertile age and heterogeneous carcinomas identified in 30% of elderly patients (3–6). Strikingly, it has been proven that ovarian teratomas associated with anti-NMDAR encephalitis harbor particular immunopathological characteristics, including more frequent glial GluN1 expression, and harbor B cells whose B cell receptors directly bind the NMDAR (7–9). Similarly, other malignant tumors may also express this NMDAR subunit (4). Additionally, there are rare cases of autoimmune encephalitis with NMDAR antibodies following herpetic encephalitis (10). However, despite 70% of patients presenting with prodromal, mild viral-like symptoms, the pathogenesis of the remaining cases of anti-NMDAR encephalitis remains obscure (11). Thus, although the aforementioned tumors and perhaps herpetic encephalitis seem to be able to trigger an autoimmune reaction in a subset of subjects, the mechanisms underlying the initiation of the autoimmune response are still unknown in most cases. A genetic predisposition conferring some risk has been postulated but results remain heavily debated (12–16).
The human leukocyte antigen (HLA) region is one of the most gene dense, complex, and polymorphic regions of the human genome. It harbors polymorphic genes involved in antigen presentation, where the HLA subtypes modulate immune responses to specific peptides and antigens. As a result, genetic associations with HLA are commonly found in infectious diseases, although these are typically strongest with autoimmune disorders, including specific subtypes of autoimmune encephalitis (12, 17–22). To date, weak HLA association findings have been reported in small samples of patients with anti-NMDAR encephalitis (13, 14), although these were not replicated in a large, recent cohort (16). Even more recently, however, a genome-wide association study (GWAS) performed in a large Chinese cohort described strong associations with DQB1*05:02, A*11:01 and A*02:07 (23).
Interestingly, some HLA class I molecules are also ligands for killer-cell immunoglobulin-like receptors (KIRs), a less studied group of polymorphic immune regulators. KIRs are immune surface receptors encoded by up to 13 polymorphic genes in each individual (24, 25). KIRs are principally expressed by natural killer (NK) cells, which play roles in immune responses against both cancer and infections. Recent data also suggest specific KIRs also regulate tolerance of CD8+ T cells (26). KIRs are key to anti-tumor and anti-viral immune responses (27, 28), and deficiencies in NK cell numbers are associated with increased susceptibility to infection by Herpesviridae, such as human cytomegalovirus (CMV), Epstein-Barr virus (EBV), herpes simplex virus (HSV) and varicella-zoster virus, as well as by human papillomavirus and other viruses (29). Because of the above, NK cells and associated receptors are plausible candidates genetically predisposing to anti-NMDAR encephalitis. Herein, we aimed to investigate potential HLA and KIR associations in a large, multiethnic cohort of anti-NMDAR encephalitis.
The analysis of KIR associations is complex, as specific receptors may become functional only in the presence of their cognate HLA ligand. The ligands include subsets of HLA-A, B and C alleles that carry A3/11, Bw4, C1 or C2 motifs (24, 25, 27, 30, 31). Thus, a given individual may not carry all the KIR or their HLA class I ligands. To explore a possible HLA-KIR association, we first assume that the KIR allele association could be strong enough to manifest dominantly independent of the presence of its ligand in the same individual. This was followed by an analysis of the number of existing paired interactions in each patient versus controls, as previously done in other studies (32–34). Doing so, we also explored both presence and number of KIR genes that are copy number variations in cases versus controls, allelic differences within these genes when present in cases versus controls, and presence or absence of KIR-HLA ligand pairs in cases versus controls. Finally, as these receptors can be either inhibitory or activating, we approximated and compared the amount of inhibitory and activating inputs NK cells were likely to receive in cases versus controls.
Materials and methods
Patients and controls
A multi-ethnic cohort of patients fulfilling diagnostic criteria for anti-NMDAR encephalitis (35) and available DNA were retrospectively recruited (Supplementary Table 1). This sample contains GWAS typed samples of 479 cases and 2,806 PCA (principal component analysis) and genotyping platform-matched controls (Supplementary Table 1). A total of 15 patients with post-herpetic autoimmune encephalitis and presence of NMDAR antibodies were excluded from this study, given their known clinical, immunological and genetic particularities (10, 36). All samples were de-identified. This cohort was primarily used for HLA imputation and allele comparison across the entire cohort.
A subsample of 323 cases and 1,519 controls, the main object of this study, was drawn from this larger sample of anti-NMDAR encephalitis cases and was analyzed using KIR and HLA sequencing. Ethnicity distribution for the sequenced sample is reported in Supplementary Table 2. In this sample, 71 (21.9%) cases had teratomas, 12 (3.7%) had other tumors and 238 (73.7%) were non-paraneoplastic; information regarding a possible underlying tumor was unavailable for 2 (0.6%) patients.
Genome wide association
Patients and controls were genotyped using Affymetrix or Illumina chips (see Supplementary Table 1). Only high-imputation calls (R2>0.9) were used to conduct genotype data calculations. All genotype data operations were performed using PLINK 1.9 (www.cog-genomics.org/plink/1.9/) (37). Patients were genome-wide imputed to the 1000 Genome Phase III dataset (38) after haplotype phasing and merged using QCTOOL and were subsequently PCA-matched by ethnicity to their closest 10 controls based on Euclidean distance using PLINK. Ethnicity was manually defined from PCAs. Genotypes were used, together with geographic origin, to identify PCA matched controls for KIR/HLA sequencing, and to conduct HLA imputation.
KIR and HLA sequencing
The subset of 323 cases and 1,519 PCA-matched control samples were sequenced for all KIR and HLA genes, as previously described (39–41). After sequencing, raw FASTQ files were analyzed using our custom bioinformatics pipeline PING (Pushing Immunogenetics into the Next Generation) to obtain KIR gene content and KIR allelic genotypes from next-generation sequencing (NGS) data (39). We applied an updated version of the pipeline that precisely determines the copy number of each locus through multiple alignment and filtration steps, also accurately identifying KIR genotypes. The updated pipeline increased the accuracy of KIR genotype determination and is publicly available (40, 42). In cases where KIR sequencing was ambiguous due to multiple allele combinations, the most frequent set of alleles was selected based on previously reported KIR frequencies (41). In parallel with KIR, HLA class I and class II alleles were determined from the sequence data using the consensus calls obtained using three algorithms: NGSengine® 2.10.0 (GenDX, Utrecht, the Netherlands), HLA Explore™ (Omixon Biocomputing Ltd. Budapest, Hungary) and HLA*LA (43), as previously described (44). Included were genotyping for alleles of HLA-G, and HLA-G 14-bp insertion/deletion genotyping, a 3’UTR variant suggested to modulate expression (45).
HLA imputation
HLA imputation was also conducted in a larger cohort of 479 cases and 2,806 PCA-matched controls using HLA Genotype Imputation with Attribute Bagging (HIBAG), performed post-genome wide-imputation and using ethnic and platform-specific models (Supplementary Table 1) (21, 46). HLA imputation was validated using the sequenced cohort with an overall allele accuracy of >99.9%.
10x sequencing of peripheral blood mononuclear cells
PBMCs were obtained from 16 French patients with anti-NMDAR encephalitis and 16 French matched controls using Ficoll isolation and stored in liquid nitrogen until use. Median age at disease onset for cases was 24 years (range 3–48) and 12 (75%) were female. Four (25%) had ovarian teratomas and the remaining ones were non-paraneoplastic. Median delay between disease onset and PBMC collection was 44 days (range 8–1220); 4 patients were untreated at sample collection, 6 received immunotherapy close to the blood drawn (< 3 weeks, “short-term treatment”), and 6 were treated more than 3 weeks before sample collection (“long-term treatment”; see Supplementary Table 3).
Single cell libraries were prepared using 10x as instructed by the manufacturer. Briefly, individual PMBCs were thawed and washed with complete RPMI medium (RPMI (Cat# 61870–036, Gibco) supplemented with 10% fetal bovine serum (FBS) and 1% penicillin/streptomycin) and then counted. An equal number of live PBMCs from each sample was pooled and loaded into a 10x chip. Single cell 3’ and 5’ libraries were prepared by Chromium Single Cell 3’ Reagent Kits (v3.1 Chemistry) and Chromium Next GEM Single Cell V(D)J Reagent Kits v1.1 with Feature Barcode technology for Cell Surface Protein, respectively. These libraries were next sequenced on a HiSeq4000 platform at Stanford Genomics for a paired end 2x150 run with a depth of >20,000 read pairs per cell. Sequencing data were processed using the 10x Genomix Cell Ranger v6.0. Single cell was identified using demuxlet (47).
Phenotype clustering
10x sequencing results of single cell 3’ and 5’ libraries were imported into Python, concatenated into a single dataset, and analyzed using Scanpy standard workflow (48). Briefly, cells with less than 200 genes were removed from the dataset. Genes that were found in less than 4 cells or encoding hemoglobin/immunoglobulin sequences were removed. Total counts per cell were normalized. Each cell was assigned to a respective stage of cell cycle using a publicly available list of cell cycle genes (49). Next, total counts, cell cycle and mitochondrial content were regressed out. Highly variable genes were identified and used to perform PCA. The first 20 PC were used as an input for Shared Nearest Neighbor clustering and for embedding using the Uniform Manifold Approximation and Projection (UMAP) (Figure 1). Marker genes for each cluster were identified computationally using default Scanpy settings. The clusters were manually annotated according to their gene expression pattern. To further identify and cluster NK cells, single cells of PBMCs expressing high level of NKG7 and GNLY were extracted and re-clustered as above, from which NK cells that were NKG7/GNLY/CD7 positive and CD3/CD14/CD19 negative were extracted and re-clustered (50–53).
FACS sorting
NK cells were sorted as previously described (54). Briefly, PBMCs were recovered in complete RPMI medium overnight at 37°C, 5% CO2. Cells were stained with the following combination of antibodies: Brilliant Violet 421® (BV421) (Pacific Blue)-CD7 (clone, CD7–6B7, Cat# 343132), Alexa Fluor® 488 (AF488)-CD14 (clone, HCD14, Cat# 325610), AF700-CD16 (clone, B73.1, Cat# 360718), BV605-CD57 (clone, HNK-1, Cat# 393304), APC-CD3 (clone, UCHT1, Cat# 300412), BV650-CD19 (clone, HIB19, Cat# 302238), APC/Cy7-CD20 (clone, 2H7, Cat# 302314), and PE-CD56 (clone, QA17A16, Cat# 985902). Cells of CD7+CD3-CD14-CD19- were sorted in bulk with BD ARIA II at Stanford shared FACS facility (SSFF). Propidium iodide (PI) was added to separate live cells. Data were analyzed using FlowJo (v10.0.8r1). Sorted cells were immediately loaded into a 10x chip and phenotype analysis were conducted as above.
Standard protocol approvals, registrations, and patient consents
This study was approved by local ethics committees (IGNITE, #65073), and written informed consent was obtained from all the patients for the storage and use of biological samples and clinical information for research purposes.
Statistical analysis
KIR and HLA allele association
To avoid stratification issues due to different case-control matching ratios in each ethnic group, a logistic regression controlling for the three first PC (ethnicity) was conducted for each ethnic group and a meta-regression on each allele’s effects was performed using METAL (55). For clarity, we report HLA and KIR associations with anti-NMDAR encephalitis in all cases and controls in one set of analyses, and in European descent (the largest group) only in another.
HLA allele analysis was explored as previously conducted (21); multiple testing was controlled by comparing alleles having a carrier frequency of at least 5% in any group (cases or control) and by using Bonferroni correction. For non-framework KIR genes, we first studied if cases versus matched controls differed in presence/absence of each KIR genes and in the mean number of each specific KIR genes. Following this, we studied allelic associations comparing % individuals with each allele in cases versus matched controls (carrier frequency differences). In cases where a positive association was found (specific KIR2DL4 and KIR3DL3 alleles, presence of KIR2DL5B), we next analyzed if associations were independent of each other (versus the result of linkage). To do so, we iteratively controlled for the most significant alleles/presence of genes until no significant association remained. Associations were also conducted in teratoma vs. non-teratoma cases, and in teratoma plus non-teratoma vs. controls.
Following these analyses, for non-framework genes, we next explored if cases versus matched-controls differed in effective pairs of KIR-HLA ligand. Finally, as these receptors can be either inhibitory or activating (24), we compared the amount of inhibitory and activating inputs NK cells were likely to receive in cases versus controls.
Combined HLA-KIR association studies
HLA-KIR interaction associations in anti-NMDAR patients vs. controls were also modeled (30, 32). To do so, we listed all possible KIR interactions with HLA (categorized as A3, A11, Bw4, C1, C2) and assigned them a negative or a positive value from 1–3 depending of each pair effect based on the existing literature for each HLA-KIR pair (24, 25, 27, 30, 31). We then computed and compared the resulting scores in cases and controls using generalized linear and ordinal regression after control of first three PC.
Single cell analysis
For single cell RNA-sequencing studies, a linear regression was fitted to study differences in frequency between CD56dim and CD56bright and in gene expression between cases and controls, and controls and each stage of treatment using an ordinal categorization (in order: control, long-term treatment, short-term treatment, no treatment). Similarly, cells with a T lymphocyte (CD3+) and NK cell (CD3-CD56+) were separated and % population carrying KIR genes of interest compared between controls and patients as described above. Differences in other gene expression between cells positive for these KIRs were also compared in disease vs control. Population age, sex, and PC were controlled for but removed from the model due a lack of significance.
Statistical significance
We report FDR (false discovery rate) corrected p-values in alleles with >5% in either case or control carrier frequency for all analyses. Uncorrected p-values are also reported in exploratory analyses and when sample sizes are small, as indicated in each table. Analyses were conducted in R 4.1.0 (R Core Team, 2021) (56), using a significance level of p values < 0.05.
Results
Weak HLA class II association with DRB1*01:01~DQA1*01:01~DQB1*05:01 in anti-NMDAR encephalitis
Using the largest sample possible (imputed HLA), controlling for ethnicity/PC (within each ethnic group) and conducting a meta-analysis, a complex HLA association pattern was revealed, with multiple weakly significant HLA class II allele associations. These included an increase in frequency of DRB1*01:01~DQA1*01:01~DQB1*05:01, and DRB1*11:01, and a protective effect of DRB3*03:01 (Table 1). The DRB1*01:01~DQA1*01:01~DQB1*05:01 increase was more significant in non-teratoma cases (Supplementary Table 4). This finding contrast with recent works have outlined that when autoimmune encephalitis is paraneoplastic, HLA associations are frequently weaker or non-existent (19, 57). Further, DRB1*01:01~DQA1*01:01~ DQB1*05:01 was also most significant in the sequenced European descent population, doubling the risk of anti-NMDAR encephalitis (Supplementary Tables 5, 6). Finally, we specifically analyzed the carrier frequencies of DQB1*05:02, A*11:01 and A*02:07, as these have recently been reported in a large Chinese cohort (23); we confirm the association with the former but not with the class I alleles (Supplementary Table 7).
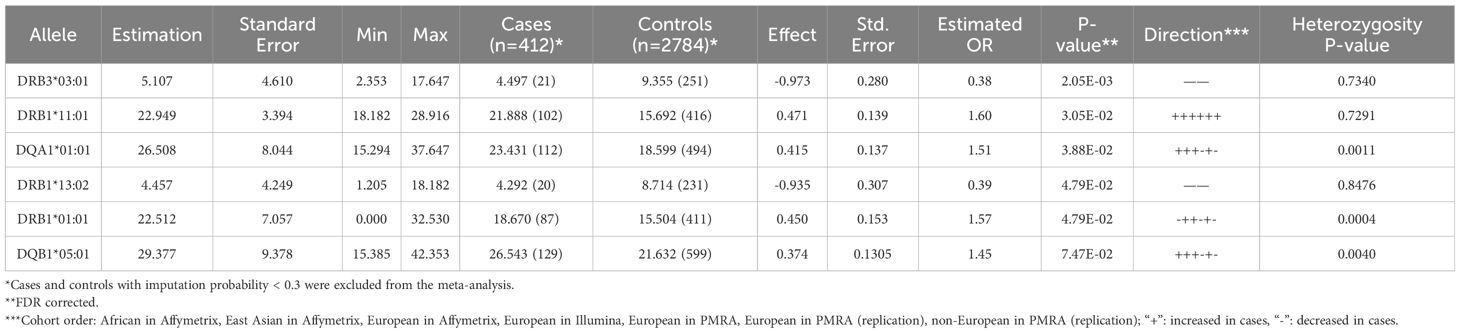
Table 1 Meta-regression of HLA association effects in imputed anti-NMDAR cases vs. controls analyzed using a Generalized Linear Equation modeling after control of first three intra-ethnic Principal Components.
Copy number variation analysis effects suggest increased presence of KIR2DL5B in anti-NMDAR encephalitis
Most KIR genes display copy number variations (CNVs). Using a meta-analysis of all cohorts we found that the number of KIR2DL5B copies was higher in cases versus controls overall (Table 2; Supplementary Table 8). This was significant exclusively in European descent cases (Supplementary Tables 9, 10); only in the very small cohort of Asian American was the trend direction reversed (Table 2; Supplementary Table 8). Of note, as KIR2DL5 was largely represented by KIR2DL5B*00201, only presence of KIR2DL5B was significantly increased (Supplementary Table 11).
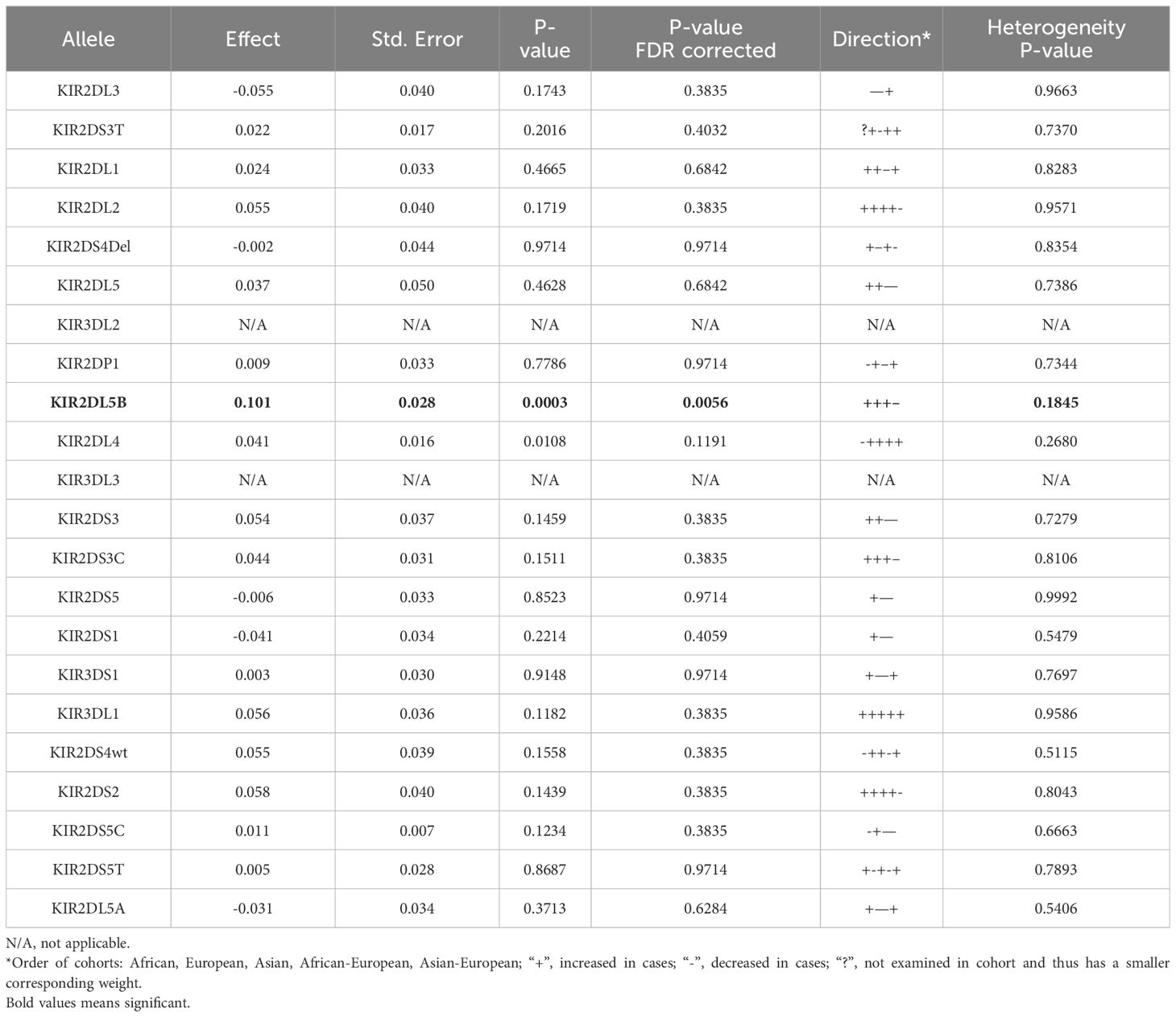
Table 2 Meta-regression of Additive Count Number Variation effects in all cases and controls, analyzed using General Linear Regression modelling after control of first three intra-ethnic Principal Components.
KIR2DL4*00103 and KIR3DL3*00302 associations in anti-NMDAR encephalitis
Comparison of alleles across other loci was first conducted only considering alleles that have amino acid changes, with FDR correction for each locus. As this revealed no significant differences, we explored all known alleles and this revealed three other independent FDR-corrected significant findings (Table 3), two of which were present in the European descent only cohort (Supplementary Table 12). The same findings were found using a meta-analysis (Supplementary Table 13), and the finding did not differ in patients with teratoma versus non-teratoma cases (Supplementary Table 14).
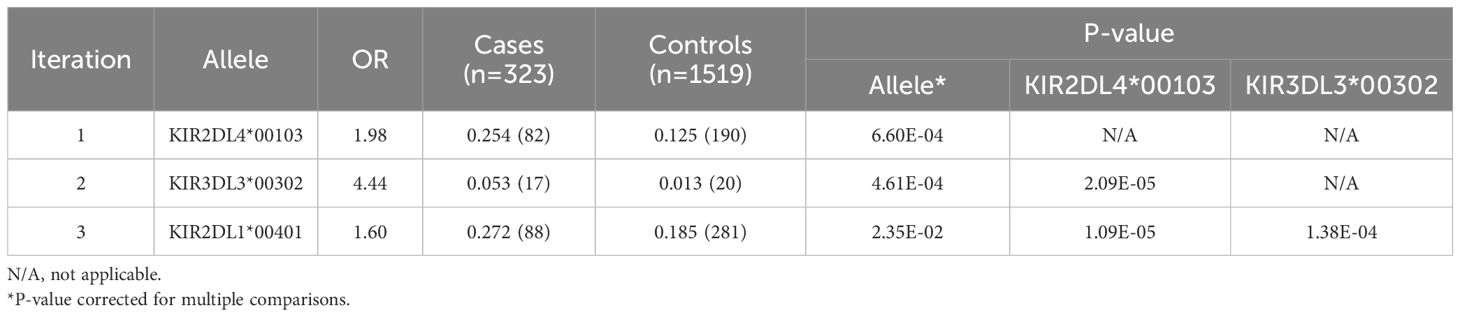
Table 3 KIR association effects in all anti-NMDAR cases versus controls, analyzed using a Generalized Linear Equation modeling after control of three Principal Components and iteratively controlling for significant alleles.
One association signal was due to increased telomeric framework gene allele KIR2DL4*00103 in patients with anti-NMDAR encephalitis overall (25.4% vs. 12.5%, OR=1.98, p=6.60×10-4, Table 3). In addition, presence of centromeric framework gene KIR3DL3*00302, a rare allele only carried by 1.3% of European descent controls was also increased to 5.3% (OR=4.44, p=4.61×10-4, Table 3). As mentioned, the two effects were independent of each other and carrying KIR2DL4*00103 was independently associated, not surprisingly since they are located on two unlinked segments. Finally, a weak effect of KIR2DL1*00401 remained (OR=1.6, p=2.35×10-2), which as mentioned above was not significant in European descent alone and is thus not discussed further (Table 3).
As KIR3DL3 and KIR2DL5B are both centromeric, and KIR3DL3*00302 is rare, we conditioned the KIR3DL3*00302 association by presence of KIR2DL5B and found these effects to be largely independent (Supplementary Table 15). The KIR2DL4*00103, KIR2DL5B, KIR3DL3*00302 effects were significantly increased in patients with teratoma and non-teratoma cases with a larger effect in the former group (Supplementary Table 14). Similar results were obtained using a meta-analysis per ethnic groups (Supplementary Table 13). Meta-regression analysis also identified another rare allele, KIR3DL2*10701, associated with the disease (OR=5.47, p=0.007) and which was removed from the Caucasian analysis due to low carrier frequency. KIR2DL1*00401 was associated with a higher risk of developing the disease in the whole cohort but was not confirmed in the meta-regression.
Balance of KIR activation/inhibition and HLA ligand pairs
We next explored if specific KIR-HLA ligand interactions (presence of ligand and receptor in the same individual) differed between European descent cases and controls using generalized linear regression after control of first three PC (Supplementary Tables 16, 17). This was also done by meta-regression across all ethnic groups (Supplementary Tables 18, 19) using approximate scores reflecting strength of signaling as reported in Supplementary data. As can be seen, KIR2DL1_C2 and KIR2DL2_C1_C2 inhibitory scores were significantly higher in cases versus controls. As a final analysis, Supplementary Table 20, a total inhibitory score, total excitatory score, and a sum of both (excitatory minus inhibitory) was compared using a formulation described in Supplementary data, revealing no significant difference. It is noteworthy that none of the KIR we identified in the association analysis bind to classical HLA class I ligands.
HLA-G genotyping
Since HLA-G is the ligand of KIR2DL4 (58), we also analyzed differences in HLA-G genotypes between cases and controls. However, we found no differences in either HLA-G 14-bp Insertion/Deletion 3’UTR promotor polymorphism (Supplementary Table 21) or in the frequency of HLA-G*01:05, the rare null allele (Supplementary Table 22).
Differences in NK cell frequencies and gene expression
To assess the immune cell type most related to KIRs, CD56dim or CD56bright cells were next sorted and examined for gene expression (Table 4). This was then used to cluster NK cells into CD56dim or CD56bright subtypes across cases by phase of therapy and controls (Table 4; Supplementary Table 21). Using this method, percentage of CD56dim or CD56bright NK cells did not differ between cases and controls nor across stages of therapies (coded as controls, long-term treatment, short-term treatment, no treatment), although a non-significant decrease in NK CD56bright cells was found in untreated cases vs. controls.
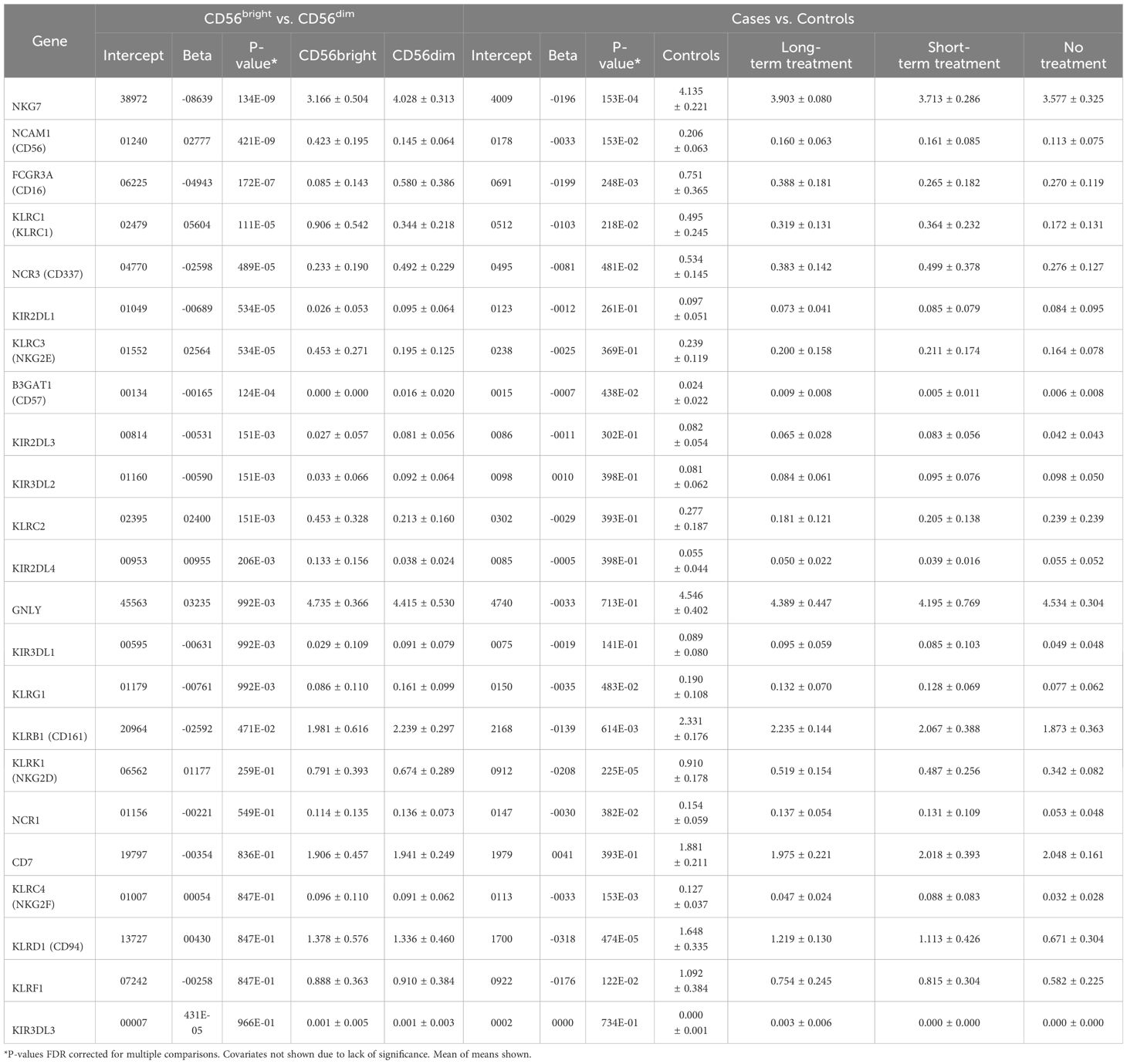
Table 4 Gene expression analysis between CD56bright and CD56dim NK cells using Generalized Linear Models and controlling for Age and Sex.
In term of gene expression profile, as expected, CD56bright mostly differed from CD56dim as follows: increased CD56/NCAM1 (neural cell adhesion molecule 1), KLRC3/NKG2E (killer cell lectin like receptor C3) and KLRC1/NKG2A plus decreased NKG7 (natural killer cell granule protein 7), CD16/FCGR3A (Fc gamma receptor IIIa), KIR2DL1 and CD57/B3GAT1 (beta-1,3-Glucuronyltransferase 1). KIR2DL4 was also significantly increased in CD56bright as expected.
We next compared gene expression within NK cells in cases versus controls. Because only four untreated cases were available (in almost all cases, anti-NMDAR encephalitis cases receive immunosuppression early in the disease course), to test a difference by disease status, we expected a gene to be most different in these four cases and to recover closer to control values as treatment progressed and the case resolved. For this reason, an ordinal model (controls, long-term treatment, short-term treatment, no treatment) was set up looking at expression, also controlling for age and sex; however, the results did not change (data not shown).
In general, we found that expression of many genes was decreased in anti-NMDAR encephalitis vs. controls and by phase of therapy, most notably KLRD1/CD94, KLRK1/NKG2D, NKG7, NCAM1/CD56, KLRB1/CD161 and KLRC4/NKG2F. Importantly, KIR2DL4 did not differ. KIR3DL3 expression was also examined but it was low (data not shown) and based on its recently reported cellular distribution in γδ and CD8+ T cells (59) that was not clearly observed in our dataset (data not shown), data for this gene was not reported. KIR2DL5B expression was also not observed, in line with reports that it is generally silenced (60–62).
Discussion
In this work, we conducted HLA imputation/sequencing and KIR sequencing in the largest reported genetic analysis of anti-NMDAR encephalitis cases, using a recently described pipeline. Anti-NMDAR encephalitis was a particularly interesting candidate disease in which to explore effects of KIR and HLA, given it is autoimmune and associated with both viral infection (post-HSV encephalitis) and tumors.
We found a weak HLA association with DRB1*01:01~DQA1*01:01~DQB1*05:01, a frequent haplotype, that will need to be replicated. Previously, weak associations were reported with B*07:02 in White adult patients (13), DRB1*16:02 in Chinese populations (14), and DRB1*15:02 in a pediatric Thai cohort (63). More recently, a large Chinese study revealed rather strong associations with DQB1*05:02, A*11:01 and A*02:07 (23). Altogether, these results suggest that, in contrast to other autoimmune encephalitides with strong and homogeneous HLA associations across different ethnicities, such as those related to antibodies against leucine-rich glioma-inactivated 1 (LGI1) or IgLON5 (21, 64), the HLA associations in anti-NMDAR encephalitis are more diverse and likely reflect a less significant role in the pathogenesis of the disease.
More interestingly, we found an association with increased number of copies of KIR2DL5B and two allele associations in framework genes, KIR2DL4*00103 and KIR3DL3*00302. KIR2DL4 is a framework gene. KIR2DL4 is likely an activating receptor that recognizes HLA-G as its ligand (58). HLA-G is highly expressed in trophoblast, although expression is also present in thymus, cornea, and pancreas islet (65, 66). HLA-G is also present as a secreted protein. The KIR2DL4-HLA-G interaction may be particularly important in the context of the feto-maternal interface. Indeed, KIR2DL4 is primarily expressed in CD56bright NK cells (as confirmed in this study), cells that also include decidual NK cells. Interestingly, KIR2DL4 is mostly intracellular and only a small portion is expressed at the surface of NK cells (58). In this context, CD56bright NK cells, a subtype of NK cells considered less differentiated and more cytokine-producing than cytotoxic, is likely useful to induce tolerance to trophoblastic invasion though KIR2DL4-HLA-G interactions. A similar role may be played in the context of virally infected or cancerous cells. Ectopic expression of HLA-G in tumor and in virally infected cells has also been reported, where it binds leukocyte immunoglobulin-like receptors (LILR) subfamily B and likely operates as a checkpoint inhibitor, dampening NK cell activity (66). KIR2DL4*00103 amino-acid sequence does not differ from the most common KIR2DL4*00102, an allele that was not associated with anti-NMDAR encephalitis in our dataset (31.2% vs. 33.6% in controls, ns, data not shown). Thus, the difference observed here with KIR2DL4*00103, if replicated, is more likely to result from differences in expression.
To complement this finding, we also explored whether HLA-G genotypes differ across cases and controls, finding no differences in either HLA-G promotor polymorphisms or in the frequency of the rare HLA-G null allele across cases and controls (Supplementary Tables 19, 20). This, together with the fact KIR2DL4*00103 does not differ in sequence with KIR2DL4*00102 makes this pathway unlikely to be strongly involved in anti-NMDAR encephalitis. Sequencing of additional samples will be needed to confirm the association with non-coding allele KIR2DL4*001.
Another finding was an association with the rare KIR3DL3*00302 allele. Similar to the above, KIR3DL3*00302 does not differ in amino acid sequence from the more common KIR3DL3*00301, which did not differ in our data set (22.9 vs. 25.0, ns, data not shown). KIR3DL3 ligand is HHLA2 (Human endogenous retrovirus-H long terminal repeat-associating 2), a variant of the B7 family that is mostly expressed in monocytes, but is also present in trophoblastic cells. Like HLA-G, it may function as a checkpoint inhibitor. Interestingly, KIR3DL3 was recently shown to be mostly expressed in CD8+ T cells and γδ T cells (59), a finding we could not confirm in our single cell sequencing due to low expression of this receptor in peripheral blood. As for the KIR2DL4 association, the fact the allele does not differ from a more common variant that is not associated is not strongly supportive of involvement, although the fact both KIR2DL4 and KIR3DL3 have related function is intriguing.
A more solid finding pertained to increased copy number variation of KIR2DL5B. KIR2DL5 is present as two extremely similar genes, KIR2DL5A (telomeric) and KIR2DL5B (centromeric). KIR2DL5 was considered an orphan molecule until recent studies identified it as a new binding partner of poliovirus receptor (PVR, also known as CD155) using high-throughput screening of receptor-ligand interactions (67–69). KIR2DL5A frequency, a receptor expressed in both innate (NK and γδ T cells) and adaptive (CD8+ T cells) immune cells from human peripheral blood (70), did not differ globally in our study. While most KIR5DL5B alleles are considered transcriptionally silent because of an impaired RUNX binding site conserved in the promoter region of most KIRs (-97A), most known KIR2DL5A alleles and a few KIR2DL5B alleles have intact RUNX binding sites and are expressed (70).
Expression of PVR is low or absent in most healthy tissues; however, it is overexpressed on numerous types of tumors, including colorectal cancer, glioma, myeloid leukemia, ovarian cancer, lung cancer, pancreatic cancer, melanoma, and other tumors (71). Accumulating evidence suggests that PVR overexpression induces the immune escape of tumor cells and is associated with a poor prognosis and enhanced tumor progression (70). Besides its tumor-intrinsic roles, PVR participates in multiple immunoregulatory events through finely tuned interaction with the stimulatory receptor DNAX accessory molecule 1 (DNAM-1, also known as CD226) and the inhibitory receptors T cell immunoreceptor with Ig and ITIM domains (TIGIT) and CD96 (72). Further, the KIR2DL5-PVR pathway has been shown to be important in modulating responses of HIV infected cells by NK cells (73). Finally, KIR2DL5 polymorphisms have been associated with multiple sclerosis, a disease associated with EBV infection (74).
In our study, increased KIR2DL5B copy number was found in anti-NMDAR encephalitis (Table 1). As expected, the increase was mostly due to KIR2DL5B*00201 (27.5 vs. 19.5%, p=0.03), the most frequent allele, although other alleles, which are rare, were also increased. Interestingly, KIR2DL5B*00201 and most other alleles are characterized by the presence of 162Asp and 174Gly in the D2 domain (exon-5) of the KIR molecule, an area known to be essential to PVR binding (70). As such, most KIR2DL5B alleles are not only known to be expressed under normal conditions but also unlikely to bind PVR as their ligand. These changes are characteristic of most KIR2DL5B alleles and also shared by KIR2DL5A*00501, the only KIR2DL5A allele slightly increased in anti-NMDAR encephalitis (10.6% vs. 8.7%, ns). Interestingly, KIR2DL5A*00501 is also transcriptionally silent (60, 70). Although most KIR2DL5B alleles including KIR2DL5B*00201 (and KIR2DL5A*00501) are transcriptionally silent in normal conditions in blood (other tissues have not been tested), demethylation of the promotor could restore expression, thus whether increased KIR2DL5B and allele differences in KIR2DL5A are relevant to anti-NMDAR encephalitis is still possible. Of note, however, all alleles associated with anti-NMDAR encephalitis are not those binding PVR, thus interaction with another yet unknown ligand would have to be involved.
We next explored gene expression differences in NK cells of patients versus controls across therapy. Interestingly, decreased KLRD1/CD94, KLRK1/NKG2D, NKG7, NCAM1/CD56, KLRB1/CD161 and KLRC4/NKG2D were found (Table 4). Most of the changes were due to changes observed within the NK CD56dim population, were present in untreated patients and improved partially with therapy (data not shown), as predicted from the analysis we designed. CD94, which is decreased, heterodimerizes with NKG2A and C to interact with HLA-E (27), and is either inhibitory or activating. KLRK1/NKG2D is activating and recognizes MIC and RAET1/ULBP families which appear on the surface of stressed, malignant transformed, and infected cells. It is thought to be important in viral and cancer control and viruses/cancer have adapted mechanisms by which to evade NKG2D responses such as with CMV (75). As for intracellular NKG2F, NKG2D does not pair with CD94; its function is largely unknown. Overall, the profile of these cells is consistent with a population of NK cells that may be more difficult to activate, although it is important to realize circulating NK cells are not representative of tissue homing NK cells, which are mostly CD56bright and cytokine producing. Additional studies will be needed to confirm and expend on these findings.
The main limitations of the current study are the relatively low number of non-European and teratoma-related patients included, which hindered the identification of specific HLA and KIR associations in these subsets of patients, as well as the small number of untreated patients from whom PBMCs were used to investigate NK cell frequencies and gene expression.
In conclusion, our study of anti-NMDAR encephalitis revealed minor changes in KIR polymorphism distribution, although increased KIR2DL5B copy number was found. No significant differences in cell numbers for all major cell categories were found, although a trend in decreased CD56bright was observed. Many of these changes could reflect past or current viral infection, or be the result of the autoimmune process. Gene expression in NK cells revealed changes that are predicted to result in less easily activatable cells.
Data availability statement
Publicly available datasets were analyzed in this study. This data can be found here: https://doi.org/10.7910/DVN/W7QAIR and https://doi.org/10.7910/DVN/AOIFIO.
Ethics statement
The studies involving humans were approved by IGNITE (#65073) Stanford University. The studies were conducted in accordance with the local legislation and institutional requirements. Written informed consent for participation in this study was provided by the participants’ legal guardians/next of kin.
Author contributions
VS: Conceptualization, Data curation, Formal analysis, Investigation, Methodology, Software, Validation, Writing – original draft, Writing – review & editing. GL: Conceptualization, Data curation, Formal analysis, Investigation, Methodology, Software, Writing – original draft, Writing – review & editing. SM-C: Data curation, Formal analysis, Investigation, Methodology, Writing – original draft, Writing – review & editing. A-LP: Data curation, Writing – review & editing. GP: Data curation, Writing – review & editing. VR: Data curation, Writing – review & editing. MT: Data curation, Investigation, Writing – original draft, Writing – review & editing. CF: Data curation, Investigation, Writing – original draft, Writing – review & editing. FL: Data curation, Investigation, Methodology, Writing – original draft, Writing – review & editing. GK: Data curation, Investigation, Methodology, Writing – original draft, Writing – review & editing. HJ: Data curation, Writing – review & editing. RD: Data curation, Writing – review & editing. SB: Data curation, Investigation, Methodology, Writing – review & editing. SI: Data curation, Investigation, Methodology, Writing – original draft, Writing – review & editing. AB: Data curation, Writing – review & editing. JV: Data curation, Writing – review & editing. MB: Data curation, Writing – review & editing. DR: Data curation, Investigation, Writing – review & editing. T-JK: Data curation, Writing – review & editing. KC: Data curation, Writing – review & editing. S-TL: Data curation, Writing – review & editing. TK: Data curation, Writing – review & editing. NP: Data curation, Formal analysis, Investigation, Methodology, Writing – review & editing. KK: Data curation, Formal analysis, Investigation, Methodology, Writing – review & editing. AM-M: Data curation, Formal analysis, Investigation, Methodology, Writing – review & editing. JH: Conceptualization, Data curation, Formal analysis, Funding acquisition, Investigation, Methodology, Project administration, Resources, Software, Supervision, Validation, Visualization, Writing – original draft, Writing – review & editing. PN: Conceptualization, Data curation, Formal analysis, Funding acquisition, Investigation, Methodology, Project administration, Resources, Software, Supervision, Validation, Visualization, Writing – original draft, Writing – review & editing. EM: Conceptualization, Data curation, Formal analysis, Funding acquisition, Investigation, Methodology, Project administration, Resources, Software, Supervision, Validation, Visualization, Writing – original draft, Writing – review & editing.
Funding
The author(s) declare financial support was received for the research, authorship, and/or publication of this article. This study was supported by research grant NIH-1U01NS120885 (EM). PN is supported by research grant NIH U01AI090905. JH, A-LP, GP, and VR are supported by a public grant overseen by the Agence Nationale de la Recherche (ANR) as part of the “Investissements d’Avenir” program (ANR-18-RHUS-0012), and performed within the framework of the LABEX CORTEX of the Université Claude Bernard Lyon 1, and the program “ Investissements d’Avenir “ (ANR-11-LABX-0042) operated by the ANR. SI was supported by the Wellcome Trust (104079/Z/14/Z), Medical Research Council (MR/V007173/1), BMA Research Grants—Vera Down grant (2013), Margaret Temple (2017), Epilepsy Research UK (P1201), the Fulbright UK-US commission (MS Society research award), the NIHR Oxford Biomedical Research Centre, and by the Wellcome Trust (Grant number 104079/Z/14/Z). SB has received support from the NIHR and Wellcome Trust. MT is supported by the Dutch Epilepsy Foundation (NEF 14-19 & 19-08), ZonMW (Memorabel initiative), Dioraphte (2001 0403), E-RARE JTC 2018 (UltraAIE, 90030376505), and an Interlaken Leadership Award. FL and GK are supported by German Ministry of Education and Research (01GM1908A und 01GM2208, CONNECT-GENERATE), FL also by E-Rare Joint Transnational research support (ERA-Net, LE3064/2-1), Stiftung Pathobiochemie of the German Society for Laboratory Medicine and HORIZON MSCA 2022 Doctoral Network 101119457 — IgG4-TREAT.
Acknowledgments
The authors thank all the patients for their invaluable participation in this study. JH thanks NeuroBioTec Hospices Civils de Lyon BRC (France, AC-2013–1867, NFS96–900) for banking samples. FL and GK thank all GENERATE contributors for their help in recruiting patients.
Conflict of interest
MT has filed a patent, on behalf of the Erasmus MC, for methods for typing neurologic disorders and cancer, and devices for use therein; has received research funds for serving on a scientific advisory board of AmGen, for consultation at Guidepoint Global LLC, for consultation at UCB; and has received an unrestricted research grant from Euroimmun AG and from CSL Behring. FL discloses speaker honoraria from Grifols, Teva, Biogen, Bayer, Roche, Novartis, Fresenius, travel funding from Merck, Grifols and Bayer and serving on advisory boards for Roche, Biogen and Alexion. SI is a co-applicant and receives royalties on patent application WO/210/046716 U.K. patent no., PCT/GB2009/051441 entitled “Neurological Autoimmune Disorders”; the patent has been licensed for the development of assays for LGI1 and other VGKC-complex antibodies; has filed a patent Diagnostic Strategy to improve specificity of CASPR2 antibody detection; Ref. JA94536P.GBA; has received research support from and/or consultancy with UCB, RocheADC therapeutics, CSL Behring and ONO Pharmaceuticals.
The remaining authors declare that the research was conducted in the absence of any commercial or financial relationships that could be construed as a potential conflict of interest.
Publisher’s note
All claims expressed in this article are solely those of the authors and do not necessarily represent those of their affiliated organizations, or those of the publisher, the editors and the reviewers. Any product that may be evaluated in this article, or claim that may be made by its manufacturer, is not guaranteed or endorsed by the publisher.
Supplementary material
The Supplementary Material for this article can be found online at: https://www.frontiersin.org/articles/10.3389/fimmu.2024.1423149/full#supplementary-material
References
1. Graus F, Vogrig A, Muñiz-Castrillo S, Antoine J-CG, Desestret V, Dubey D, et al. Updated diagnostic criteria for paraneoplastic neurologic syndromes. Neurol - Neuroimmunol Neuroinflamm. (2021) 8:e1014. doi: 10.1212/NXI.0000000000001014
2. Hébert J, Riche B, Vogrig A, Muñiz-Castrillo S, Joubert B, Picard G, et al. Epidemiology of paraneoplastic neurologic syndromes and autoimmune encephalitides in France. Neurol - Neuroimmunol Neuroinflamm. (2020) 7:e883. doi: 10.1212/NXI.0000000000000883
3. Titulaer MJ, McCracken L, Gabilondo I, Armangué T, Glaser C, Iizuka T, et al. Treatment and prognostic factors for long-term outcome in patients with anti-NMDA receptor encephalitis: an observational cohort study. Lancet Neurol. (2013) 12:157–65. doi: 10.1016/S1474–4422(12)70310–1
4. Bost C, Chanson E, Picard G, Meyronet D, Mayeur M-E, Ducray F, et al. Malignant tumors in autoimmune encephalitis with anti-NMDA receptor antibodies. J Neurol. (2018) 265:2190–200. doi: 10.1007/s00415–018-8970–0
5. Irani SR, Bera K, Waters P, Zuliani L, Maxwell S, Zandi MS, et al. N-methyl-d-aspartate antibody encephalitis: temporal progression of clinical and paraclinical observations in a predominantly non-paraneoplastic disorder of both sexes. Brain. (2010) 133:1655–67. doi: 10.1093/brain/awq113
6. Bastiaansen AEM, de Bruijn MAAM, Schuller SL, Martinez-Hernandez E, Brenner J, Paunovic M, et al. Anti-NMDAR encephalitis in the Netherlands, focusing on late-onset patients and antibody test accuracy. Neurol Neuroimmunol Neuroinflamm. (2022) 9:e1127. doi: 10.1212/NXI.0000000000001127
7. Chefdeville A, Treilleux I, Mayeur M-E, Couillault C, Picard G, Bost C, et al. Immunopathological characterization of ovarian teratomas associated with anti-N-methyl-D-aspartate receptor encephalitis. Acta Neuropathol Commun. (2019) 7(1):38. doi: 10.1186/s40478–019-0693–7
8. Makuch M, Wilson R, Al-Diwani A, Varley J, Kienzler A-K, Taylor J, et al. N-methyl-D-aspartate receptor antibody production from germinal center reactions: Therapeutic implications. Ann Neurol. (2018) 83:553–61. doi: 10.1002/ana.25173
9. Al-Diwani A, Theorell J, Damato V, Bull J, McGlashan N, Green E, et al. Cervical lymph nodes and ovarian teratomas as germinal centres in NMDA receptor-antibody encephalitis. Brain J Neurol. (2022) 145:2742–54. doi: 10.1093/brain/awac088
10. Armangue T, Spatola M, Vlagea A, Mattozzi S, Cárceles-Cordon M, Martinez-Heras E, et al. Frequency, symptoms, risk factors, and outcomes of autoimmune encephalitis after herpes simplex encephalitis: a prospective observational study and retrospective analysis. Lancet Neurol. (2018) 17:760–72. doi: 10.1016/S1474–4422(18)30244–8
11. Dalmau J, Lancaster E, Martinez-Hernandez E, Rosenfeld MR, Balice-Gordon R. Clinical experience and laboratory investigations in patients with anti-NMDAR encephalitis. Lancet Neurol. (2011) 10:63–74. doi: 10.1016/S1474–4422(10)70253–2
12. Kim TJ, Lee ST, Moon J, Sunwoo JS, Byun JI, Lim JA, et al. Anti-LGI1 encephalitis is associated with unique HLA subtypes. Ann Neurol. (2017) 81:183–92. doi: 10.1002/ana.24860
13. Mueller SH, Färber A, Prüss H, Melzer N, Golombeck KS, Kümpfel T, et al. Genetic predisposition in anti-LGI1 and anti-NMDA receptor encephalitis. Ann Neurol. (2018) 83:863–9. doi: 10.1002/ana.25216
14. Shu Y, Qiu W, Zheng J, Sun X, Yin J, Yang X, et al. HLA class II allele DRB1*16:02 is associated with anti-NMDAR encephalitis. J Neurol Neurosurg Psychiatry. (2019) 90:652–8. doi: 10.1136/jnnp-2018–319714
15. Shu Y, Guo J, Ma X, Yan Y, Wang Y, Chen C, et al. Anti-N-methyl-D-aspartate receptor (NMDAR) encephalitis is associated with IRF7, BANK1 and TBX21 polymorphisms in two populations. Eur J Neurol. (2021) 28:595–601. doi: 10.1111/ene.14596
16. Tietz AK, Angstwurm K, Baumgartner T, Doppler K, Eisenhut K, Elisak M, et al. Genome-wide association study identifies 2 new loci associated with anti-NMDAR encephalitis. Neurol - Neuroimmunol Neuroinflamm. (2021) 8:e1085. doi: 10.1212/NXI.0000000000001085
17. Van Sonderen A, Roelen DL, Stoop JA, Verdijk RM, Haasnoot GW, Thijs RD, et al. Anti-LGI1 encephalitis is strongly associated with HLA-DR7 and HLA-DRB4. Ann Neurol. (2017) 81:193–8. doi: 10.1002/ana.24858
18. Binks S, Varley J, Lee W, Makuch M, Elliott K, Gelfand JM, et al. Distinct HLA associations of LGI1 and CASPR2-antibody diseases. Brain. (2018) 141:2263–71. doi: 10.1093/brain/awy109
19. Muñiz-Castrillo S, Joubert B, Elsensohn M-H, Pinto A-L, Saint-Martin M, Vogrig A, et al. Anti-CASPR2 clinical phenotypes correlate with HLA and immunological features. J Neurol Neurosurg Psychiatry. (2020) 91:1076–84. doi: 10.1136/JNNP-2020–323226
20. Muñiz-Castrillo S, Haesebaert J, Thomas L, Vogrig A, Pinto AL, Picard G, et al. Clinical and prognostic value of immunogenetic characteristics in anti-LGI1 encephalitis. Neurol Neuroimmunol Neuroinflamm. (2021) 8(3):e974. doi: 10.1212/NXI.0000000000000974
21. Sempere VP, Muñiz-Castrillo S, Ambati A, Binks S, Pinto A-L, Rogemond V, et al. Human leukocyte antigen association study reveals DRB1*04:02 effects additional to DRB1*07:01 in anti-LGI1 encephalitis. Neurol - Neuroimmunol Neuroinflamm. (2022) 9:e1140. doi: 10.1212/NXI.0000000000001140
22. Gaig C, Ercilla G, Daura X, Ezquerra M, Fernández-Santiago R, Palou E, et al. HLA and microtubule-associated protein tau H1 haplotype associations in anti-IgLON5 disease. Neurol - Neuroimmunol Neuroinflamm. (2019) 6:e605. doi: 10.1212/NXI.0000000000000605
23. Liu X, Zheng X, Shu Y, Qu X, Wang Q, Liu X, et al. Genome-wide association study identifies IFIH1 and HLA-DQB1*05:02 loci associated with anti-NMDAR encephalitis. Neurol Neuroimmunol Neuroinflamm. (2024) 11:e200221. doi: 10.1212/NXI.0000000000200221
24. Saunders PM, Vivian JP, O’Connor GM, Sullivan LC, Pymm P, Rossjohn J, et al. A bird’s eye view of NK cell receptor interactions with their MHC class I ligands. Immunol Rev. (2015) 267:148–66. doi: 10.1111/imr.12319
25. Pollock NR, Harrison GF, Norman PJ. Immunogenomics of killer cell immunoglobulin-like receptor (KIR) and HLA class I: coevolution and consequences for human health. J Allergy Clin Immunol Pract. (2022) 10:1763–75. doi: 10.1016/j.jaip.2022.04.036
26. Li J, Zaslavsky M, Su Y, Guo J, Sikora MJ, van Unen V, et al. KIR+CD8+ T cells suppress pathogenic T cells and are active in autoimmune diseases and COVID-19. Science. (2022) 376:eabi9591. doi: 10.1126/science.abi9591
27. Pende D, Falco M, Vitale M, Cantoni C, Vitale C, Munari E, et al. Killer ig-like receptors (KIRs): their role in NK cell modulation and developments leading to their clinical exploitation. Front Immunol. (2019) 10:1179. doi: 10.3389/fimmu.2019.01179
28. Mora-Bitria L, Asquith B. Innate receptors modulating adaptive T cell responses: KIR-HLA interactions and T cell-mediated control of chronic viral infections. Immunogenetics. (2023) 75:269–82. doi: 10.1007/s00251-023-01293-w
29. Björkström NK, Strunz B, Ljunggren H-G. Natural killer cells in antiviral immunity. Nat Rev Immunol. (2022) 22:112–23. doi: 10.1038/s41577–021-00558–3
30. Blunt MD, Khakoo SI. Activating killer cell immunoglobulin-like receptors: Detection, function and therapeutic use. Int J Immunogenet. (2020) 47:1–12. doi: 10.1111/iji.12461
31. Parham P, Norman PJ, Abi-Rached L, Guethlein LA. Human-specific evolution of killer cell immunoglobulin-like receptor recognition of major histocompatibility complex class I molecules. Philos Trans R Soc Lond B Biol Sci. (2012) 367:800–11. doi: 10.1098/rstb.2011.0266
32. Agrawal S, Prakash S. Significance of KIR like natural killer cell receptors in autoimmune disorders. Clin Immunol Orlando Fla. (2020) 216:108449. doi: 10.1016/j.clim.2020.108449
33. Ahn R, Vukcevic D, Motyer A, Nititham J, Squire DM, Hollenbach JA, et al. Large-scale imputation of KIR copy number and HLA alleles in north American and European psoriasis case-control cohorts reveals association of inhibitory KIR2DL2 with psoriasis. Front Immunol. (2021) 12:684326. doi: 10.3389/fimmu.2021.684326
34. Anderson KM, Augusto DG, Dandekar R, Shams H, Zhao C, Yusufali T, et al. Killer cell immunoglobulin-like receptor variants are associated with protection from symptoms associated with more severe course in Parkinson disease. J Immunol Baltim Md 1950. (2020) 205:1323–30. doi: 10.4049/jimmunol.2000144
35. Graus F, Titulaer MJ, Balu R, Benseler S, Bien CG, Cellucci T, et al. A clinical approach to diagnosis of autoimmune encephalitis. Lancet Neurol. (2016) 15:391–404. doi: 10.1016/S1474–4422(15)00401–9
36. Armangué T, Olivé-Cirera G, Martínez-Hernandez E, Rodes M, Peris-Sempere V, Guasp M, et al. Neurologic complications in herpes simplex encephalitis: clinical, immunological and genetic studies. Brain J Neurol. (2023) 146:4306–19. doi: 10.1093/brain/awad238
37. Chang CC, Chow CC, Tellier LC, Vattikuti S, Purcell SM, Lee JJ. Second-generation PLINK: rising to the challenge of larger and richer datasets. GigaScience. (2015) 4:7. doi: 10.1186/s13742–015-0047–8
38. Auton A, Abecasis GR, Altshuler DM, Durbin RM, Bentley DR, Chakravarti A, et al. A global reference for human genetic variation. Nat. (2015) 526:68–74. doi: 10.1038/nature15393
39. Norman PJ, Hollenbach JA, Nemat-Gorgani N, Marin WM, Norberg SJ, Ashouri E, et al. Defining KIR and HLA Class I Genotypes at Highest Resolution via High-Throughput Sequencing. Am J Hum Genet. (2016) 99:375–91. doi: 10.1016/j.ajhg.2016.06.023
40. Marin WM, Dandekar R, Augusto DG, Yusufali T, Heyn B, Hofmann J, et al. High-throughput interpretation of killer-cell immunoglobulin-like receptor short-read sequencing data with PING. PloS Comput Biol. (2021) 17:e1008904. doi: 10.1371/journal.pcbi.1008904
41. Amorim LM, Augusto DG, Nemat-Gorgani N, Montero-Martin G, Marin WM, Shams H, et al. High-resolution characterization of KIR genes in a large north american cohort reveals novel details of structural and sequence diversity. Front Immunol. (2021) 12:674778. doi: 10.3389/fimmu.2021.674778
42. Wagner I, Schefzyk D, Pruschke J, Schöfl G, Schöne B, Gruber N, et al. Allele-level KIR genotyping of more than a million samples: workflow, algorithm, and observations. Front Immunol. (2018) 9:2843. doi: 10.3389/fimmu.2018.02843
43. Dilthey AT, Mentzer AJ, Carapito R, Cutland C, Cereb N, Madhi SA, et al. HLA*LA-HLA typing from linearly projected graph alignments. Bioinforma Oxf Engl. (2019) 35:4394–6. doi: 10.1093/bioinformatics/btz235
44. Farias TDJ, Brugiapaglia S, Croci S, Magistroni P, Curcio C, Zguro K, et al. HLA-DPB1*13:01 associates with enhanced, and KIR2DS4*001 with diminished protection from developing severe COVID-19. HLA. (2024) 103:e15251. doi: 10.1111/tan.15251
45. de Almeida BS, Muniz YCN, Prompt AH, Castelli EC, Mendes-Junior CT, Donadi EA. Genetic association between HLA-G 14-bp polymorphism and diseases: A systematic review and meta-analysis. Hum Immunol. (2018) 79:724–35. doi: 10.1016/j.humimm.2018.08.003
46. Zheng X, Shen J, Cox C, Wakefield JC, Ehm MG, Nelson MR, et al. HIBAG—HLA genotype imputation with attribute bagging. Pharmacogenomics J. (2014) 14:192–200. doi: 10.1038/tpj.2013.18
47. Kang HM, Subramaniam M, Targ S, Nguyen M, Maliskova L, McCarthy E, et al. Multiplexed droplet single-cell RNA-sequencing using natural genetic variation. Nat Biotechnol. (2018) 36:89–94. doi: 10.1038/nbt.4042
48. Wolf FA, Angerer P, Theis FJ. SCANPY: large-scale single-cell gene expression data analysis. Genome Biol. (2018) 19:15. doi: 10.1186/s13059–017-1382–0
49. Tirosh I, Izar B, Prakadan SM, Wadsworth MH, Treacy D, Trombetta JJ, et al. Dissecting the multicellular ecosystem of metastatic melanoma by single-cell RNA-seq. Science. (2016) 352:189–96. doi: 10.1126/science.aad0501
50. Turner WH, Collin J. Seamstress’s finger: a cause of Heberden’s nodes. BMJ. (1988) 297:1636. doi: 10.1136/bmj.297.6664.1636-b
51. Smith SL, Kennedy PR, Stacey KB, Worboys JD, Yarwood A, Seo S, et al. Diversity of peripheral blood human NK cells identified by single-cell RNA sequencing. Blood Adv. (2020) 4:1388–406. doi: 10.1182/bloodadvances.2019000699
52. Crinier A, Milpied P, Escalière B, Piperoglou C, Galluso J, Balsamo A, et al. High-dimensional single-cell analysis identifies organ-specific signatures and conserved NK cell subsets in humans and mice. Immunity. (2018) 49:971–986.e5. doi: 10.1016/j.immuni.2018.09.009
53. Ranganath T, Simpson LJ, Ferreira A-M, Seiler C, Vendrame E, Zhao N, et al. Characterization of the impact of daclizumab beta on circulating natural killer cells by mass cytometry. Front Immunol. (2020) 11:714. doi: 10.3389/fimmu.2020.00714
54. Yang C, Siebert JR, Burns R, Gerbec ZJ, Bonacci B, Rymaszewski A, et al. Heterogeneity of human bone marrow and blood natural killer cells defined by single-cell transcriptome. Nat Commun. (2019) 10:3931. doi: 10.1038/s41467–019-11947–7
55. Willer CJ, Li Y, Abecasis GR. METAL: fast and efficient meta-analysis of genomewide association scans. Bioinforma Oxf Engl. (2010) 26:2190–1. doi: 10.1093/bioinformatics/btq340
57. Muñiz-Castrillo S, Vogrig A, Honnorat J. Associations between HLA and autoimmune neurological diseases with autoantibodies. Auto- Immun Highlights. (2020) 11:2. doi: 10.1186/s13317–019-0124–6
58. Rajagopalan S, Long EO. KIR2DL4 (CD158d): An activation receptor for HLA-G. Front Immunol. (2012) 3:258. doi: 10.3389/fimmu.2012.00258
59. Palmer WH, Leaton LA, Campos Codo A, Crute B, Roest J, Zhu S, et al. Polymorphic KIR3DL3 expression modulates tissue-resident and innate-like T cells. Sci Immunol. (2023) 8:eade5343. doi: 10.1126/sciimmunol.ade5343
60. Cisneros E, Moraru M, Gómez-Lozano N, López-Botet M, Vilches C. KIR2DL5: an orphan inhibitory receptor displaying complex patterns of polymorphism and expression. Front Immunol. (2012) 3:289. doi: 10.3389/fimmu.2012.00289
61. Cisneros E, Estefanía E, Vilches C. Allelic polymorphism determines surface expression or intracellular retention of the human NK cell receptor KIR2DL5A (CD158f). Front Immunol. (2016) 7:698. doi: 10.3389/fimmu.2016.00698
62. Cisneros E, Moraru M, Gómez-Lozano N, Muntasell A, López-Botet M, Vilches C. Haplotype-based analysis of KIR-gene profiles in a South European population-distribution of standard and variant haplotypes, and identification of novel recombinant structures. Front Immunol. (2020) 11:440. doi: 10.3389/fimmu.2020.00440
63. Anurat K, Watcharakuldilok P, Sakpichaisakul K, Khongkhatithum C, Mahasirimongkol S, Kunhapan P, et al. HLA-DRB1∗1502 is associated with anti-N-methyl-D-aspartate receptor encephalitis in thai children. Pediatr Neurol. (2022) 134:93–9. doi: 10.1016/j.pediatrneurol.2022.06.014
64. Yogeshwar SM, Muñiz-Castrillo S, Sabater L, Peris-Sempere V, Mallajosyula V, Luo G, et al. HLA-DQB1*05 subtypes and not DRB1*10:01 mediates risk in anti-IgLON5 disease. Brain J Neurol. (2024), awae048. doi: 10.1093/brain/awae048
65. Weiss E, Andrade HS, Lara JR, Souza AS, Paz MA, Lima THA, et al. KIR2DL4 genetic diversity in a Brazilian population sample: implications for transcription regulation and protein diversity in samples with different ancestry backgrounds. Immunogenetics. (2021) 73:227–41. doi: 10.1007/s00251–021-01206–9
66. Jasinski-Bergner S, Schmiedel D, Mandelboim O, Seliger B. Role of HLA-G in viral infections. Front Immunol. (2022) 13:826074. doi: 10.3389/fimmu.2022.826074
67. Verschueren E, Husain B, Yuen K, Sun Y, Paduchuri S, Senbabaoglu Y, et al. The immunoglobulin superfamily receptome defines cancer-relevant networks associated with clinical outcome. Cell. (2020) 182:329–344.e19. doi: 10.1016/j.cell.2020.06.007
68. Husain B, Ramani SR, Chiang E, Lehoux I, Paduchuri S, Arena TA, et al. A platform for extracellular interactome discovery identifies novel functional binding partners for the immune receptors B7-H3/CD276 and PVR/CD155. Mol Cell Proteomics MCP. (2019) 18:2310–23. doi: 10.1074/mcp.TIR119.001433
69. Shilts J, Severin Y, Galaway F, Müller-Sienerth N, Chong Z-S, Pritchard S, et al. A physical wiring diagram for the human immune system. Nature. (2022) 608:397–404. doi: 10.1038/s41586-022-05028-x
70. Ren X, Peng M, Xing P, Wei Y, Galbo PM, Corrigan D, et al. Blockade of the immunosuppressive KIR2DL5/PVR pathway elicits potent human NK cell-mediated antitumor immunity. J Clin Invest. (2022) 132:e163620. doi: 10.1172/JCI163620
71. Kučan Brlić P, Lenac Roviš T, Cinamon G, Tsukerman P, Mandelboim O, Jonjić S. Targeting PVR (CD155) and its receptors in anti-tumor therapy. Cell Mol Immunol. (2019) 16:40–52. doi: 10.1038/s41423-018-0168-y
72. Bottino C, Castriconi R, Pende D, Rivera P, Nanni M, Carnemolla B, et al. Identification of PVR (CD155) and Nectin-2 (CD112) as cell surface ligands for the human DNAM-1 (CD226) activating molecule. J Exp Med. (2003) 198:557–67. doi: 10.1084/jem.20030788
73. Fittje P, Hœlzemer A, Garcia-Beltran WF, Vollmers S, Niehrs A, Hagemann K, et al. HIV-1 Nef-mediated downregulation of CD155 results in viral restriction by KIR2DL5+ NK cells. PloS Pathog. (2022) 18:e1010572. doi: 10.1371/journal.ppat.1010572
74. García-León JA, Pinto-Medel MJ, García-Trujillo L, López-Gómez C, Oliver-Martos B, Prat-Arrojo I, et al. Killer cell immunoglobulin-like receptor genes in Spanish multiple sclerosis patients. Mol Immunol. (2011) 48:1896–902. doi: 10.1016/j.molimm.2011.05.018
Keywords: anti-NMDAR encephalitis, KIR, KIR3DL3, KIR2DL4, HLA, DQA1, DQB1, DRB1
Citation: Peris Sempere V, Luo G, Muñiz-Castrillo S, Pinto A-L, Picard G, Rogemond V, Titulaer MJ, Finke C, Leypoldt F, Kuhlenbäumer G, GENERATE study group, Jones HF, Dale RC, Binks S, Irani SR, Bastiaansen AE, de Vries JM, de Bruijn MAAM, Roelen DL, Kim T-J, Chu K, Lee S-T, Kanbayashi T, Pollock NR, Kichula KM, Mumme-Monheit A, Honnorat J, Norman PJ and Mignot E (2024) HLA and KIR genetic association and NK cells in anti-NMDAR encephalitis. Front. Immunol. 15:1423149. doi: 10.3389/fimmu.2024.1423149
Received: 25 April 2024; Accepted: 06 June 2024;
Published: 10 July 2024.
Edited by:
Markus Reindl, Clinical Department of Neurology, AustriaCopyright © 2024 Peris Sempere, Luo, Muñiz-Castrillo, Pinto, Picard, Rogemond, Titulaer, Finke, Leypoldt, Kuhlenbäumer, GENERATE study group, Jones, Dale, Binks, Irani, Bastiaansen, de Vries, de Bruijn, Roelen, Kim, Chu, Lee, Kanbayashi, Pollock, Kichula, Mumme-Monheit, Honnorat, Norman and Mignot. This is an open-access article distributed under the terms of the Creative Commons Attribution License (CC BY). The use, distribution or reproduction in other forums is permitted, provided the original author(s) and the copyright owner(s) are credited and that the original publication in this journal is cited, in accordance with accepted academic practice. No use, distribution or reproduction is permitted which does not comply with these terms.
*Correspondence: Emmanuel Mignot, bWlnbm90QHN0YW5mb3JkLmVkdQ==
†These authors have contributed equally to this work and share first authorship
‡These authors have contributed equally to this work and share last authorship