- 1Medical Oncology Department of Thoracic Cancer 1, Cancer Hospital of China Medical University, Liaoning Cancer Hospital & Institute, Shenyang, Liaoning, China
- 2Department of Radiotherapy, Cancer Hospital of China Medical University, Liaoning Cancer Hospital & Institute, Shenyang, Liaoning, China
Brain metastatic cancer poses a significant clinical challenge, with limited treatment options and poor prognosis for patients. In recent years, immunotherapy has emerged as a promising strategy for addressing brain metastases, offering distinct advantages over conventional treatments. This review explores the evolving landscape of tumor immunotherapy in the context of brain metastatic cancer, focusing on the intricate interplay between the tumor microenvironment (TME) and immunotherapeutic approaches. By elucidating the complex interactions within the TME, including the role of immune cells, cytokines, and extracellular matrix components, this review highlights the potential of immunotherapy to reshape the treatment paradigm for brain metastases. Leveraging immune checkpoint inhibitors, cellular immunotherapies, and personalized treatment strategies, immunotherapy holds promise in overcoming the challenges posed by the blood-brain barrier and immunosuppressive microenvironment of brain metastases. Through a comprehensive analysis of current research findings and future directions, this review underscores the transformative impact of immunotherapy on the management of brain metastatic cancer, offering new insights and opportunities for personalized and precise therapeutic interventions.
1 Introduction
Recent advancements in diagnostic and therapeutic modalities have significantly improved survival rates in patients with malignant tumors. However, the development of symptomatic brain metastases (BM) presents a considerable challenge and remains a leading cause of mortality in these patients. Brain metastatic carcinoma, characterized by the metastasis of malignant tumors from other parts of the body to the skull, represents one of the most prevalent intracranial tumors encountered in clinical practice (1, 2). This condition infiltrates various intracranial tissues, including the brain parenchyma, spinal cord membranes, nerves, and capillaries, with brain parenchymal metastasis being the most frequent. Consequently, patients often experience epilepsy, cognitive dysfunction, sensory impairment, motor dysfunction, and cranial nerve damage, leading to a significant decline in their overall quality of life (3). The primary treatment options for metastatic brain tumors currently encompass surgical resection and radiation therapy. While conventional chemotherapeutic agents have shown limited efficacy in the central nervous system (CNS), the emergence of targeted small molecule tyrosine kinase inhibitors has revolutionized therapeutic approaches by effectively crossing the BBB and displaying activity within the CNS (4). Additionally, immunotherapy has emerged as a promising treatment modality for brain metastatic carcinoma, overcoming the traditional challenges associated with pharmacotherapy by demonstrating intracranial activity. Notably, two major factors impeding pharmacotherapy efficacy in BM include the unpredictable molecular profiles of BM relative to the primary tumor and their variable responsiveness to drugs, alongside the constraints posed by limited drug penetration through the human BBB and blood-tumor barrier (BTB) (5).
The tumor immune microenvironment (TME) plays a crucial role in tumor development, progression, and metastasis. It consists of tumor-associated immune cells and associated cytokines (6, 7). Tumor cells are a prime component of the immune microenvironment and can hinder immune responses by releasing various molecules and cytokines, allowing them to escape immune surveillance (8). Meanwhile, diverse immune cells play essential roles in mounting an effective immune response. Additionally, fibroblasts, vascular endothelial cells, and other cell types contribute to the construction of the TME and influence tumor cell growth and metastasis. Interleukins (ILs), tumor necrosis factors (TNFs), and transforming growth factors (TGFs) are pivotal in regulating immune responses and tumor growth (9). Immunotherapy, the manipulation of the body’s immune system to enhance its ability to target and eliminate tumor cells, intimately links with the tumor immune microenvironment (10). On one hand, the tumor immune microenvironment greatly influences the effectiveness and response rates of immunotherapy. For instance, certain tumor cells employ the upregulation of programmed death-ligand 1 (PD-L1) to evade attacks from immunotherapy by inhibiting T cell activation and cytotoxicity. On the other hand, immunotherapy actively influences tumor growth and metastasis by modulating the immune microenvironment. For instance, targeting the cytotoxic T-lymphocyte-associated protein 4 (CTLA-4) molecule promotes T cell activation and cytotoxicity, thereby inhibiting tumor growth and metastasis.
In recent years, rapid advancements in biomedical science have led to novel ideas for immunotherapy in the treatment of malignant brain metastases. This article provides a comprehensive summary of research pertaining to various immunotherapies for brain metastatic cancer. Additionally, it outlines recent advances in immunotherapy for brain metastatic cancer in conjunction with the specific tumor immune microenvironment found in brain metastatic foci.
2 Brain-specific structures and microenvironment
2.1 BBB
The BBB consists of a heterogeneous composition of cell types, including microvascular endothelial cells, the basement membrane, and adjacent astrocytes Among these cell types, microvascular endothelial cells serve as the primary constituents of the BBB, which are interconnected by tight junction proteins such as TJP-1 (ZO-1), claudin, and occludin, forming a cohesive barrier (Figure 1) (11). These tight junction proteins are crucial for maintaining cellular interconnectivity and preventing the entry of macromolecules into the neural tissue Moreover, the basement membrane plays a vital role in the establishment and maintenance of the BBB by establishing adhesive connections between microvascular endothelial cells and astrocytes via proteins, polyamines, and sugars (12, 13). The adjacent astrocytes, being a specialized subtype of glial cells, not only provide structural support but also influence neuronal activity (14). Functionally, the BBB selectively filters substances from the bloodstream, permitting the passage of only small, specific molecules. This “selective permeability” feature of the BBB is crucial for the stable functioning of neural tissue (15). However, after the onset of brain metastases, the integrity of the BBB is disrupted to various extents, leading to the formation of what is referred to as the BTB. Experimental models of brain metastases have revealed that the BTB tends to be more permeable to drugs and contrast media than the BBB.
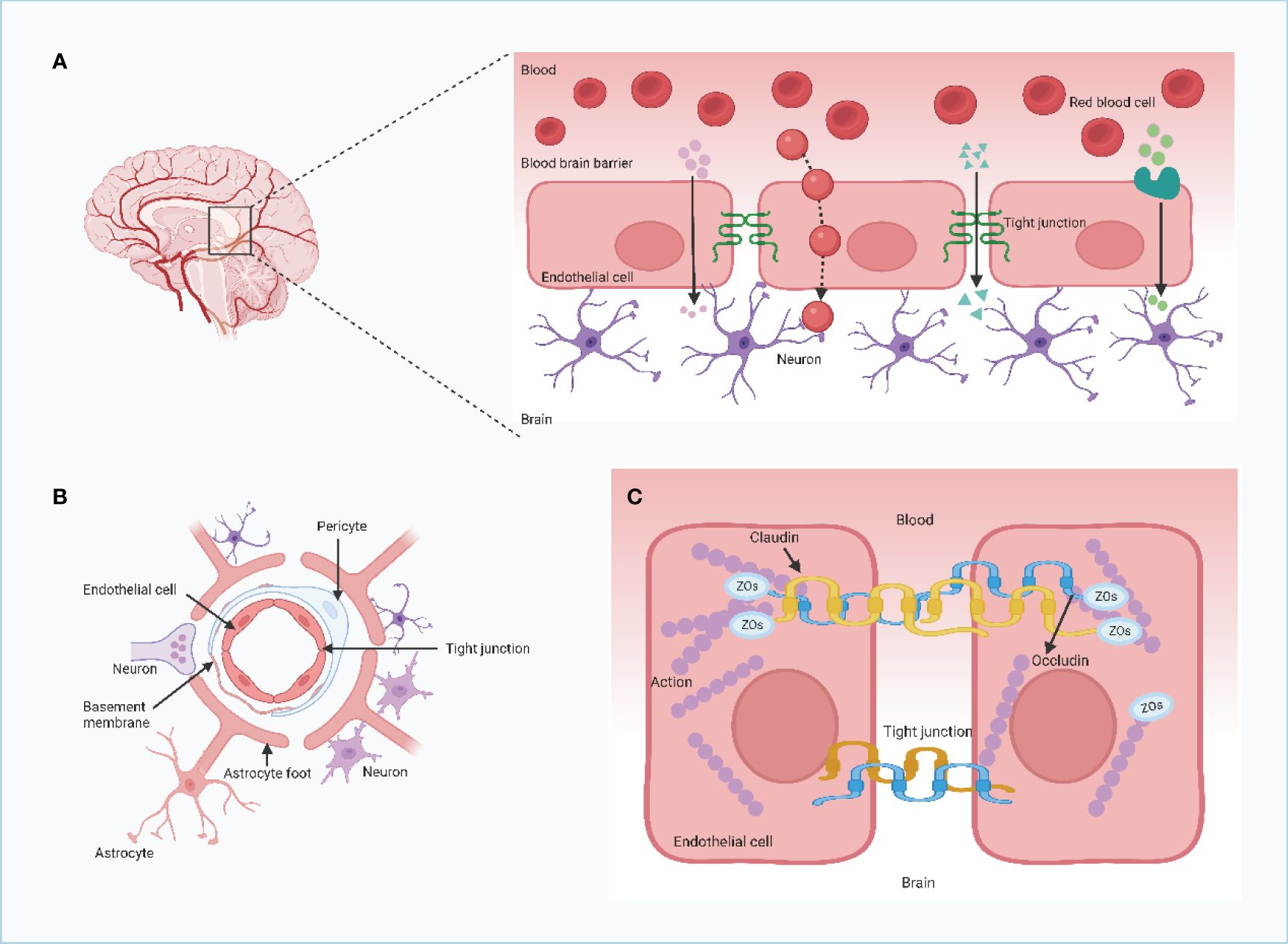
Figure 1 The structure and feature of the blood brain barrier (BBB). (A) Claudins and occludin compress two neighboring endothelial cells together. These proteins are connected to cytoskeletal proteins through auxiliary proteins such as ZO, which promote the formation of TJs. The blood-brain barrier is formed by endothelial cells connected by tight junction proteins (TJs) and separates the brain from components of the circulating blood. (B, C) The capillary lumen of the blood-brain barrier is surrounded by endothelial cells, and TJs are located between the endothelial cells of the brain thus preventing the flow of most substances from the blood into the brain. TJs allow essential nutrients to enter the brain parenchyma by simple diffusion, passive diffusion between cells, and transport proteins that transport essential macromolecules, but they limit the entry of potentially harmful molecules from the blood into the brain.Endothelial and pericytes are surrounded by a common basement membrane. The ends of astrocytes surround the endothelium and pericytes and provide the connection between neurons and the blood-brain barrier. (Created with BioRender.com).
The BBB, on the other hand, is the barrier between plasma and brain cells formed by the walls of brain capillaries and glial cells, and between plasma and cerebrospinal fluid formed by the choroid plexus (16, 17). During tumor treatment, circulating tumor cells may enter the brain and form brain metastases. However, because of the presence of the BBB, most circulating tumor cells cannot pass through, limiting the occurrence of BM to some extent (18). Recent studies have shown that circulating tumor cells disrupt the integrity of the BBB, thereby promoting the development of brain metastases (19). Circulating tumor cells may disrupt the BBB by interacting with endothelial and pericyte cells of the BBB and releasing substances such as VEGF that alter the permeability of the BBB (20). Circulating tumor cells can also directly invade the endothelial and pericyte cells of the BBB, thereby inducing BBB disruption (21). In addition, circulating tumor cells can further promote the development of BM by interacting with glial cells and inducing their activation, thereby releasing substances that alter the permeability of the BBB, such as interleukin-8 (IL-8) (22, 23). There is growing evidence that MMPs play a role in disrupting tight junctions and promoting tumor brain metastasis. During cerebral ischemia, MMP2 and MMP9 are activated and degrade tight junction proteins such as claudin-5, occludin, and ZO-1 in brain microvascular endothelial cells, increasing the permeability of the blood-cerebrospinal fluid barrier and causing brain edema and hemorrhage (24).
Treatment options for encephalopathy often involve the use of lipid-soluble drugs with low polarity. Studies have demonstrated that the rate at which different drugs enter the brain and cerebrospinal fluid from the blood can vary greatly. Drugs that have a high binding affinity to plasma encounter difficulties in traversing the BBB and reaching brain tissue. Under normal physiological conditions, drugs that exist in a non-dissociated form are more likely to pass through the BBB, while drugs with a high oil-water partition coefficient tend to have easier access to the CNS. In normal circumstances, the presence of the BBB provides a protective effect for the CNS, limiting the entry of certain substances from the bloodstream into the brain. The restrictive properties of the BBB are more pronounced in comparison to the capillaries of other organs. The BBB acts as a barrier, allowing essential substances for brain metabolism to pass through while preventing the entry of foreign matter, such as bacteria and viruses, thereby safeguarding the brain tissue from potential harm. The invasion of viruses and bacteria into the central nervous system typically occurs through dissemination via the bloodstream, necessitating passage through the BBB. Thus, the integrity of the BBB contributes to determining the occurrence and severity of CNS infections. Moreover, the existence of the BBB reinforces the stability of brain cells and enhances the resistance of brain tissue to environmental changes, thereby promoting the organism’s adaptive capacity. In pathological conditions, the functioning of the BBB becomes compromised. For instance, in cases of brain tumors, substances such as 32P or other fluorescent materials that normally have limited BBB permeability can penetrate the brain tissue. This principle is applied in radiological diagnoses of brain tumors. Notably, more than 89% of experimental brain metastatic lesions exhibit some disruption in BBB permeability, varied in terms of significance, with only 10% achieving therapeutic drug concentrations. Therefore, increasing BBB permeability to peripheral immune cells and antitumor drugs has become a crucial area of focus within therapeutic research for brain metastatic cancer.
2.2 Immune microenvironment of metastatic brain tumors
The TME is a complex and intricately coordinated system consisting of multiple components. These include tumor cells, immune cells, inflammatory cells, tumor-associated fibroblasts (TAMs), neighboring mesenchymal tissue, microvasculature, as well as various cytokines and chemokines (25). The TME can be further divided into two distinct compartments: the immune microenvironment, predominantly governed by immune cells, and the non-immune microenvironment, primarily controlled by fibroblasts. Within the TME, there exist intricate interactions and regulatory relationships among its diverse constituents, which exert considerable influence on numerous processes such as tumorigenesis, metastasis, and drug resistance. Furthermore, these complex components contribute to the metabolic heterogeneity of the TME and dictate the behavior of immune cells. Tumor metastasis represents a significant hurdle in tumor management and is a leading cause of mortality in many cancer patients. One key driver of tumor metastasis is the establishment of pre-metastatic niches (PMNs), which are specific locations where a microenvironment conducive to tumor metastasis forms. Remarkably, certain tumor cells exhibit organ-specific tropism, suggesting the selective nature of tumor metastasis. The formation of PMNs is mediated by a tripartite interplay involving tumor-derived secreted factors (TDSFs), extracellular vesicles (EVs), and bone marrow-derived cells (BMDCs). Specifically, TDSFs and EVs derived from primary tumors induce the recruitment of BMDCs to target organs, thereby facilitating the creation of an inflammatory microenvironment conducive to tumor metastasis. Consequently, this inflammatory milieu promotes tumor cell colonization, survival, and growth within PMNs.
Brain metastasis shares similarities with metastasis in other organs, as it follows a highly selective, nonrandom, multistep process. Tumor cells act as the “seeds” of metastasis, with the microenvironment of the metastatic site serving as the “soil” for their growth (26). The genetic and biological heterogeneity of tumor cells from different subclones within a primary tumor influences their metastatic potential, with only subclones possessing strong invasive properties capable of crossing the blood-cerebrospinal fluid (BSF) barrier to establish brain metastases (27, 28). The brain provides a unique microenvironment featuring astrocytes, stromal cells, cytokines, vascular networks, and metabolic components that either promote or inhibit tumor growth, influencing the development and progression of brain metastasis. Upon hematogenous dissemination, tumor cells interact with brain endothelial cells, secreting cytokines like vascular endothelial growth factor (VEGF) and matrix metalloproteinases (MMPs) that modify the brain microenvironment to support tumor growth (29, 30). Additionally, some researchers suggest that tumor cells may carry activated tumor-associated fibroblasts, acting as part of the microenvironment for brain metastasis initiation, survival, and proliferation. The dynamic interplay between tumor cells and the brain microenvironment ultimately leads to the rapid and irreversible growth of brain metastases. For a detailed illustration of the TME characteristics in brain metastasis, refer to Figure 2.
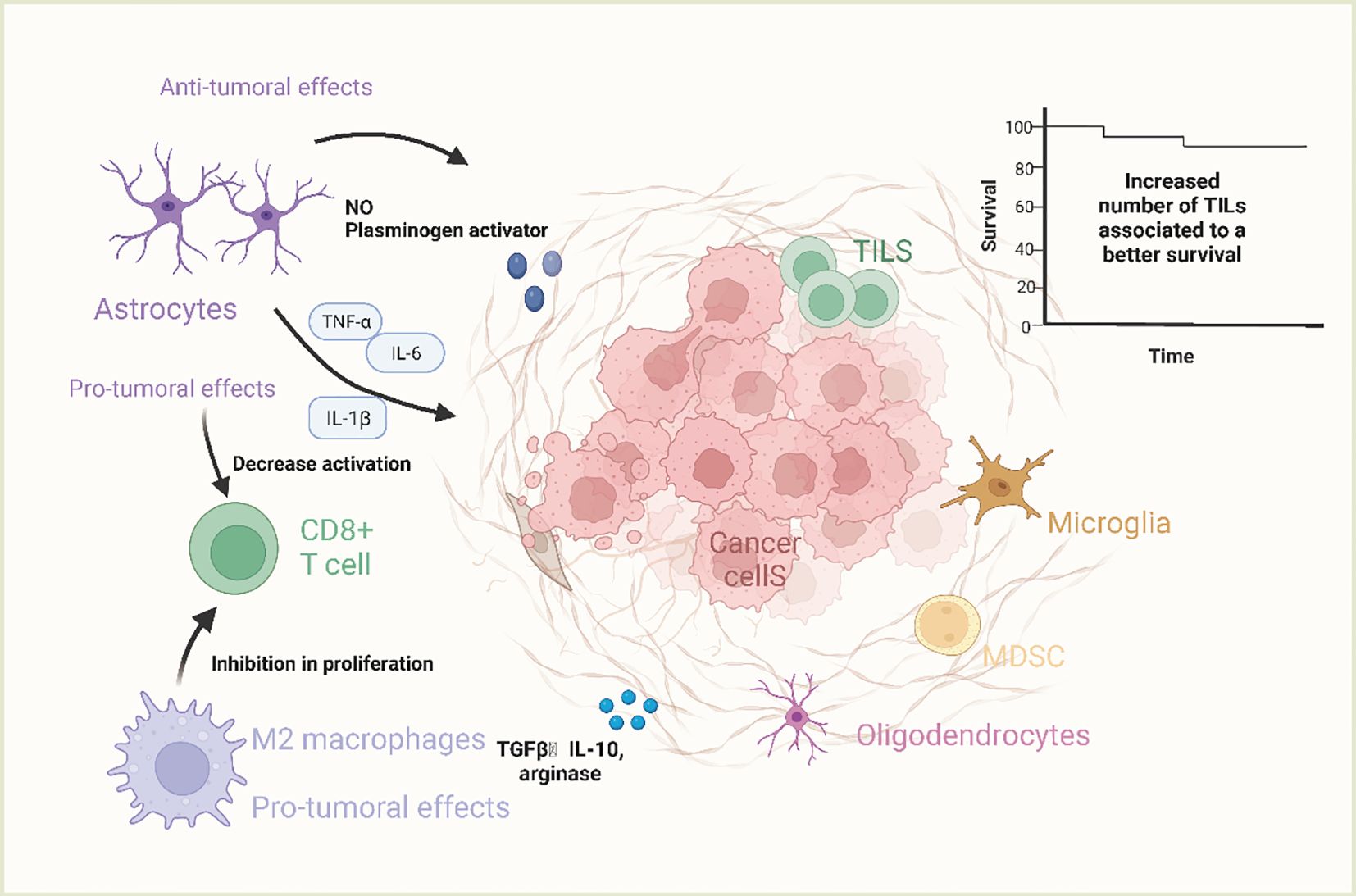
Figure 2 The characteristics of tumor microenvironment of brain metastases. Microglia are resident macrophages in the tumor microenvironment of brain metastases and are not of bone marrow origin. Only when the blood-brain barrier is disrupted can bone marrow-derived macrophages reach the CNS and act as a response to CNS disturbances. These cells are known as tumor-associated macrophages (TAMs), in which M1-like macrophages are usually pro-inflammatory and are stimulated by Toll-like receptor ligands as well as IFN-g and TNF-a. They exert tumor suppressor functions by producing factors such as IL-1, IL-12 and nitric oxide. M2-like macrophages are anti-inflammatory and can be activated by IL-4 and IL-13 to produce molecules such as =TGF-b, arginase, IL-10, and pro-fibrotic factors. M2-like macrophages can also be associated with tumor-promoter functions by inhibiting the proliferation of CD8+ T-cells.M2-like macrophages can also be associated with tumor-promoter functions by inhibiting CD8+ T-cell proliferation.M1-like macrophages are usually pro-inflammatory and stimulated by Toll-like receptor ligands as well as IFN-g and TNF-a. (Created with BioRender.com).
The immune microenvironment of brain metastatic lesions is notably distinct from that of metastatic or primary lesions in other anatomical sites. The brain harbors distinctive microenvironmental characteristics, such as the BBB, specialized environmental cells (including microglia, astrocytes, oligodendrocytes, and neurons), a lymphoid system draining to the neck, and an extracellular matrix. Furthermore, the brain exhibits a unique immunological profile (31, 32). Despite the limited presence of immune cells in the normal brain microenvironment, there is documentation of CNS immune surveillance through CD4+ T lymphocytes and CD8+ T lymphocytes in healthy individuals. Notably, astrocytes assume a crucial role in the microenvironment of brain metastatic lesions (33). In the initial phases of brain metastatic lesion development, astrocytes predominantly participate in inhibiting the survival of metastatic tumor cells. However, upon the establishment of metastatic tumor cells in the brain, they release a multitude of cytokines that facilitate the polarization of astrocytes from type M1 (tumor suppressor) to type M2 (tumor activator), thereby leading to a significant involvement of astrocytes in promoting tumor cell proliferation (34).
2.3 Drivers of brain metastasis development and treatment challenges
The development of brain metastases is influenced by various factors, including tumor cell invasive and metastatic potential, as well as oncogenes and genetic factors (35). The invasive and metastatic potential of tumor cells plays a crucial role in this process. Malignant tumor cells, with their high proliferative and invasive capabilities, are able to breach the cerebral vascular wall and cerebrospinal fluid barrier. Subsequently, they enter the cerebrospinal fluid circulation and establish metastatic foci within the brain. Moreover, tumor cells secrete specific proteins that facilitate their adhesion to brain tissue, enabling their passage through the cerebral vascular wall and successful infiltration into brain tissue via the bloodstream. In addition to tumor cell behavior, the impact of oncogenes and genetic factors on the development of brain metastasis should not be overlooked. Overexpression of cancer genes leads to the emergence of tumors. If primary tumors in other parts of the body are not effectively controlled, cancer genes can be disseminated to the brain via systemic circulation, resulting in intracranial metastases. Certain patients, such as those with neurofibromatosis, retinoblastoma, and angioretinoblastoma, possess a higher susceptibility to familial intracranial metastases. Treatment of brain metastases is complicated by several challenges, including the BBB, drug resistance of tumor cells, and the phenotypic heterogeneity of tumor cells. The BBB serves as a major impediment, preventing many drugs from entering the brain and hampering the efficacy of treatment. Furthermore, tumor cells can acquire resistance to drugs following repeated therapies, thus diminishing the therapeutic impact. The individualized treatment of brain metastases is necessitated by the distinct gene expression profiles and biological properties of various tumor cells. Consequently, an in-depth understanding of tumor cell invasion and metastasis mechanisms, along with a comprehensive comprehension of the physiological and pathological aspects of the BBB, is imperative for the development of more effective treatment strategies and therapeutic agents that can successfully address brain metastasis.
3 Immunotherapy for metastatic brain tumors
3.1 Immune checkpoint inhibitors
The immune system is known to fulfill a crucial role in safeguarding against cancer (36, 37). This function is commonly referred to as the immune surveillance of tumors, attributing to the immune system’s capacity to recognize and eliminate tumor cells through the recognition of tumor-specific antigens and molecular specificity induced by cell activation (38, 39). The concept of “cancer immune editing” elucidates the dual role of the immune system during tumor pathogenesis, encompassing both host-protective and tumor-sculpting functions (40, 41). The emergence of immune checkpoint inhibitors marks a significant advancement in the realm of tumor therapy, as these inhibitors obstruct autologous tumor antigens, bolster zvex-induced T cell responses, and enhance antitumor effects (42). ICIs are pharmaceutical agents designed to stimulate or augment the immune system’s assault on tumor cells by intervening with specific immune checkpoint molecules. The utilization of immune checkpoint inhibitors necessitates careful consideration of various aspects including the patient’s overall health and ability to manage potential adverse reactions associated with immunotherapy. It is vital to note that not all tumor types are amenable to treatment with immune checkpoint inhibitors, highlighting the importance of selecting the appropriate tumor type for this therapeutic approach. Additionally, understanding the patient’s genetic mutation status is essential, as certain genetic variations may impact the efficacy of immunotherapy. Combining ICIs with other treatment modalities like chemotherapy and radiation therapy can enhance therapeutic outcomes. It is imperative to remain vigilant for potential adverse reactions such as immune-related adverse events, skin issues, and gastrointestinal disturbances, necessitating close monitoring and prompt intervention. Clinical trials with ICIs are shown in Table 1.
3.1.1 Adrebrelimab
Adrebrelimab is a high-affinity humanized monoclonal antibody against PD-L1 that has demonstrated efficacy and safety in advanced esophageal squamous cell carcinoma (ESCC) (43), extensive SCLC (SCLC) (44), and resectable non-small cell lung cancer (NSCLC) (45). Lung cancer is currently one of the most common malignancies in the world, and its morbidity and mortality are increasing year by year. Local recurrence and metastasis are the main causes of poor prognosis for many lung cancer patients, among which the incidence of brain metastasis reaches 30%~50% (46, 47). NSCLC 20%~50% of patients develop brain metastasis during the course (48, 49) and survive more than 2 years. SCLC The incidence of brain metastasis in patients with SCLC is as high as 60% to 80% (50). The main treatment modalities for BM are whole brain radiotherapy (WBRT), stereotactic radiosurgery (SRS), surgery, and chemotherapy (51). The mechanism of brain metastasis of lung cancer is shown in Figure 3. Wang et al (44), evaluated the efficacy and safety of adrebrelimab (SHR-1316) versus standard chemotherapy in the primary treatment of extensive SCLC (ES-SCLC). The main inclusion criteria were age 18-75 years, ES-SCLC patients without a previously confirmed histologic or cytologic diagnosis, and Eastern Cooperative Oncology Group (ECOG) grade 0-1. Ultimately, 230 patients received adrebrelimab combination chemotherapy (adrebrelimab arm) and 232 patients received placebo combination chemotherapy (placebo arm). Patients received carboplatin and etoposide for 4-6 cycles, with concurrent adrebrelimab or corresponding placebo. Adrebrelimab or placebo was administered as maintenance therapy. Results of the research demonstrated a significant improvement in median overall survival in the adrebrelimab group in comparison with the placebo group and an acceptable safety profile, suggesting that this combination treatment may be a new first-line therapy for ES-SCLC.
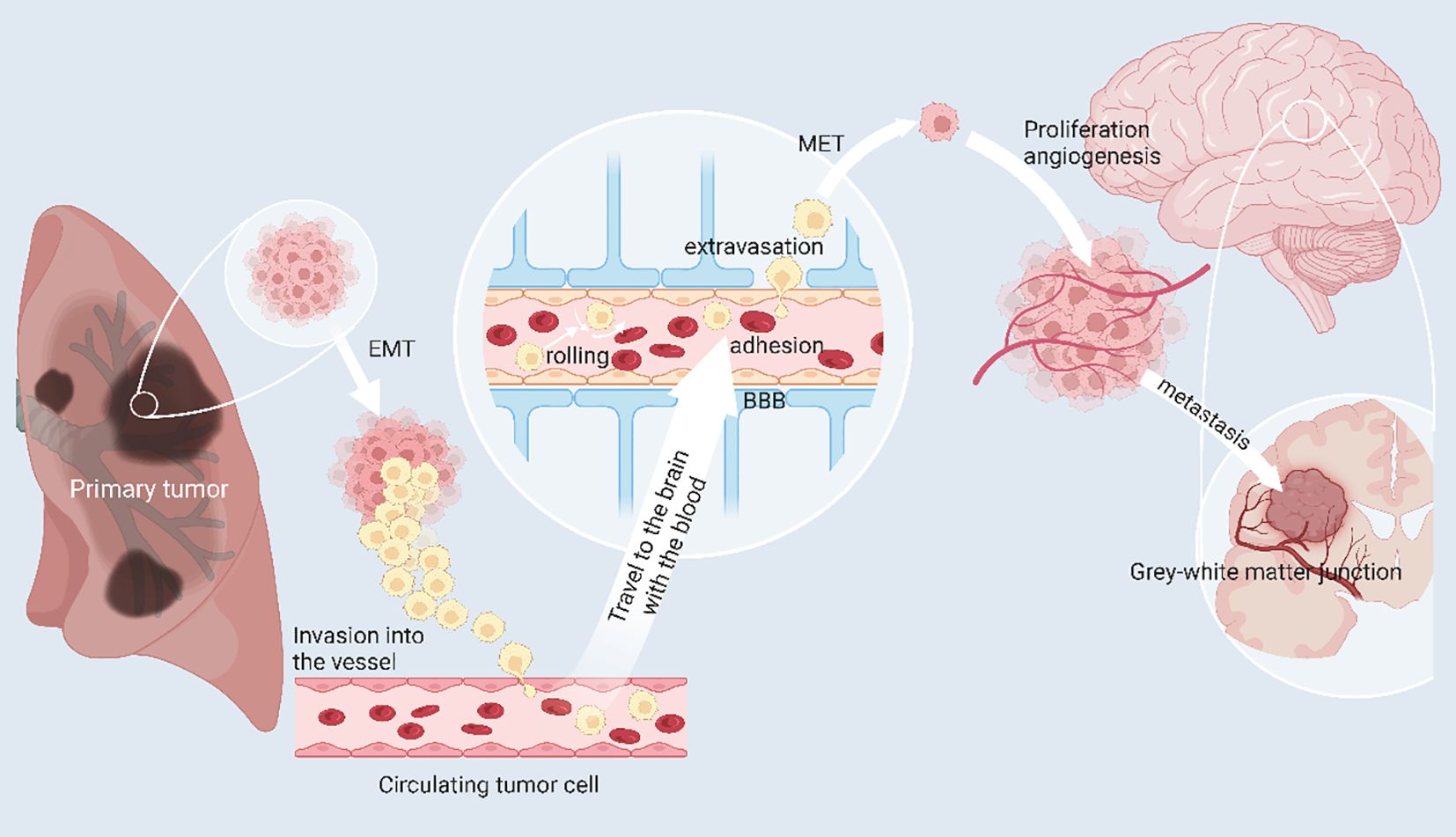
Figure 3 The mechanism of brain metastasis of lung cancer. Primary lung cancer cells can escape from the primary tumor site to invade and circulate in blood vessels, called circulating tumor cells (CTC). In response to chemokines, CTCs can reach the brain and cross the blood-brain barrier by rolling, adherence and extravasation in response to E-ligands and integrins, undergo mesenchymal epithelial transformation (MET) thereby restoring primary tumor properties and generating and adapting to the new tumor microenvironment. Angiogenesis is necessary for the growth of brain metastases. When pure oxygen diffusion is insufficient for the tumor, the tumor can gradually develop a hypoxic microenvironment and promote angiogenesis by overexpressing angiogenesis-stimulating factors. (Created with BioRender.com).
3.1.2 Atezolizumab
Atezolizumab is a humanized IgG1 anti-PD-L1 drug approved for the treatment of breast cancer (52), SCLC (53), and NSCLC (54). Lin et al (55), reported a case of poorly differentiated adenocarcinoma of the right lobe of the lung treated with atezolizumab monotherapy. The patient was diagnosed in April 2016 with stage IV poorly differentiated adenocarcinoma of the right lobe of the lung and had no driver gene mutations. The primary tumor remained enlarged after 6 cycles of nedaplatin and paclitaxel, and on October 14, 2016, the patient started atezolizumab monotherapy, which showed significant reduction in both primary tumor and mediastinal lymph nodes. Subsequently, the patient developed headaches, and on May 11, 2018, right parietal lobe metastasis of the tumor was confirmed. On May 23, 2018, the patient underwent brain X-knife stereotactic radiotherapy. One month later, the patient developed cough and shortness of breath, a new nodule in the right inferior lung basement area, a mild subpleural large lesion in the left inferior lobar area, a small right-sided A small pleural effusion was noted on the right side. Continued treatment with atezolizumab resulted in a decrease in the number of nodules in the basal right lower lobe, a decrease in the number of subpleural lesions in the basal left lower lobe, and a decrease in the number of mediastinal lymph nodes. This indicates significant efficacy of atezolizumab monotherapy in the treatment of lung adenocarcinoma. IMpower130 reported an evaluation of the efficacy and safety of atezolizumab plus chemotherapy versus chemotherapy alone as primary treatment for nonsquamous non-SCLC (56). Patient inclusion criteria were age 18 years or older, histologically or cytologically confirmed diagnosis of stage IV non-squamous non-SCLC, Eastern Cooperative Oncology Group performance status of 0 or 1, and no prior stage IV chemotherapy. Patients were randomized to receive either atezolizumab plus chemotherapy (carboplatin [area under the curve 6 mg/mL/min IV every 3 weeks] plus nab-paclitaxelor chemotherapy alone. Patients were randomized in a 2:1 ratio to receive either chemotherapy alone (four or six 21-day cycles, followed by maintenance therapy). The primary endpoints were progression-free survival and overall survival in the intention-to-treat wild-type population. The results of this study showed that atezolizumab plus chemotherapy significantly improved overall and progression-free survival compared with chemotherapy alone as a primary treatment option for stage IV non-squamous non-SCLC without ALK or epidermal growth factor receptor mutations.
3.1.3 Camrelizumab
Camrelizumab is a human IgG4-κ monoclonal antibody with high affinity for PD-1. Kamrelizumab binds to PD-1 with a binding affinity of up to 3 nM and has an inhibitory effect on PD-1/PD-L1 with an IC50 of 0.70 nM. Camrelizumab has antitumor activity and is well-tolerated in experimental cancers such as NSCLC (57), Hodgkin lymphoma (58) and HCC (59). The safety and efficacy of immune checkpoint inhibitors (including durvalumab, atezolizumab, nivolumab, tripalimumab, tisulizumab, cintilizumab, and camrelizumab) in patients with BMfrom SCLC were evaluated (60). The group retrospectively reviewed the medical records of patients with SCLC who received chemotherapy and radiation therapy for BMwith or without immune checkpoint inhibitors from January 2019 to January 2021 at our institution. Patients were divided into two groups: Group A received chemotherapy and radiation therapy for brain metastases; Group B received chemotherapy, radiation therapy for brain metastases, and immunotherapy for at least four cycles. Overall survival and intracranial progression-free survival were evaluated using Kaplan-Meier estimation and Cox regression modeling. The analysis showed that the intracranial objective response rate was higher in group B than in group A, but the intracranial disease control rate was similar in both groups, indicating that immunotherapy plus chemotherapy plus radiation therapy was favorably effective in patients with BM from SCLC.
3.1.4 Durvalumab
Durvalumab is a humanized antibody that affects the immune response by binding to PD-L1. Durvalumab fights tumors by inhibiting the binding of PD-L1 to PD-1 and enhances the killing of tumor cells by T cells. Currently, durvalumab is FDA-approved for the treatment of lung cancer (61), esophageal cancer (62), stomach cancer (63), and prostate cancer (64). Researcher describes the therapeutic efficacy of durvalumab in a patient with stage III SCLC (65). The patient developed lung and BM after concurrent chemoradiation therapy (cCRT) and achieved complete radiologic local regression after whole brain irradiation (WBI) using the simultaneous integrated boost (SIB) technique. Durvalumab was then used as maintenance therapy. After the second dose of durvalumab, the patient developed an asymptomatic multifocal brain tumor recurrence. In contrast, with the combination of durvalumab and amlotinib, the myeloma regressed almost completely without severe toxicity. This suggests that the combination of durvalumab and amlotinib may have a synergistic effect on myeloma in previously treated SCLC patients.
3.1.5 Ipilimumab
Ipilimumab is an IgG1 kappa immunoglobulin with a molecular weight of approximately 148 kDa. Ipilimumab binds to CTLA-4 and blocks the interaction of CTLA-4 with its ligand CD80/CD86. blockade of CTLA-4 increases T cell activity and proliferation, including tumor-infiltrating effector T cells, and increases growth. inhibition of CTLA-4 signaling similarly decreases regulatory T cell function and may contribute to a general increase in T cell responsiveness, including anti-tumor immune responses. Ipilimumab is used primarily for the treatment of unresectable or metastatic melanoma and for adjuvant therapy in patients with cutaneous melanoma who have undergone total lymphadenectomy, including total lymph node excision, and have localized lymph node lesions greater than 1 mm. Adjuvant therapy. Metastatic malignant melanoma has a poor prognosis and lacks effective treatment. Patients with this disease have a median survival of only 6-9 months for stage IV and a 5-year survival rate of only 10-20% (66, 67). Once the tumor has spread to the brain, only conservative treatment is available (68). Surgery and radiation therapy are effective but often cause other lesions (69). Radiation therapy, now commonly used to treat many types of brain metastases, can palliate but not eliminate lesions (4). Chemotherapy has also been applied to treat brain metastases, but with poor efficacy and short median survival (70). Chen et al (71), investigated the impact of concurrent SRS-SRT and immune checkpoint inhibitors on the prognosis and safety of patients with BM (metastatic non-SCLC, melanoma, and renal cell carcinoma). Patients receiving SRS-SRT treatment with anti-cytotoxic T lymphocyte-related protein 4 (ipilimumab) and anti-programmed cell death protein 1 receptor (nivolumab and pembrolizumab) were included. Patients using immune checkpoint inhibitors in ongoing or unreported clinical trials were excluded, and concomitant use of ICIs was defined as ICI use within 2 weeks of SRS-SRT treatment. Patients were treated with SRS-SRT, SRS-SRT without ICI, or SRS-SRT with ICI. The results of this study suggest that ICI concurrent with SRS-SRT may reduce the incidence of new BM without increasing the incidence of adverse events and may result in favorable survival outcomes. Long et al (72), evaluated the efficacy and safety of nitolizumab alone or in combination with ibritumomab in patients with active melanoma brain metastases. They randomized asymptomatic patients with BM who had never received local brain therapy to group A (nivolumab plus ibritumomab) or group B (nivolumab), while patients with BM who were refractory to local therapy and had neurological symptoms or meningeal lesions were enrolled in a non-randomized subgroup C (nivolumab). The treatment regimen consisted of nivolumab 1 mg/kg plus ibritumomab 3 mg/kg every 3 weeks for a total of 4 doses, followed by nivolumab 3 mg/kg intravenously every 2 weeks for patients in group A and nivolumab 3 mg/kg intravenously every 2 weeks for patients in group B or C. The primary endpoint was intracranial response from week 12. The primary and safety analyses were performed on an intention-to-treat basis for all patients who received at least one treatment. The results of this study showed that both the combination of nivolumab and ipilimumab and nivolumab monotherapy had a favorable effect on melanoma BM and that the combination of nivolumab and ipilimumab is applicable to asymptomatic untreated brain metastasis patients. Amaral et al (73), also reported that asymptomatic and symptomatic Amaral et al. also evaluated the efficacy of nituzumab plus ibritumomab alone or in combination with local therapy in patients with asymptomatic and symptomatic melanoma BM(MBM). Results showed no difference in OS between patients treated with BRAF and MEK inhibitors or nivolumab plus ibritumomab, and no difference in OS between initial and subsequent treatment with nituzumab plus ibritumomab in BRAF wild-type patients. In contrast, patients who received stereotactic radiosurgery or surgical local therapy had improved OS compared to patients who did not receive local therapy. Thus, the combination of nivolumab plus ibritumomab immunotherapy and stereotactic radiosurgery or surgery was found to improve OS in both asymptomatic and symptomatic MBM. Borzillo et al (74), used the CyberKnife system to compare SRT/SRS with ibritumomab (IPI) to evaluate the association and timing. They tested the correlation in 53 patients treated with RT+IPI and 10 patients treated with RT alone. Results showed that IPI combined with SRS/SRT improved LC in the treatment of MBM, but the impact and timing of both therapies on patient prognosis is unknown. Tawbi et al (75), tested the efficacy of nivolumab and ibritumomab combination therapy in patients with symptomatic MBM. The study protocol consisted of nivolumab 1 mg/kg and ipilimumab 3 mg/kg IV every 3 weeks for 4 doses, followed by nivolumab 3 mg/kg IV every 2 weeks for up to 2 years until disease progression or unacceptable toxicity. The primary endpoint was to assess the intracranial clinical response rate in all patients treated. Secondary endpoints were intracranial progression-free survival and overall survival. The results of this study showed that the combination of nituzumab and ibritumomab improved progression-free survival in patients with symptomatic MBM without causing serious side effects. A multivariate predictive model of response and survival to anti-programmed cell death protein-1 (anti-PD-1) monotherapy or in combination with anti-cytotoxic T-cell lymphocyte-4 (ipilimumab [IPI]; anti-PD-1 + IPI) was developed in metastatic melanoma (76). Study endpoints were objective response rate (ORR), progression-free survival (PFS), and overall survival (OS). And the area under the curve of the final model predicting ORR for immunotherapy-treated patients was 0.71, indicating that the model can predict response and survival outcomes for metastatic melanoma patients receiving immunotherapy.
3.1.6 Nivolumab
Nivolumab is an immunotherapeutic drug that targets PD-1 and inhibits the interaction between PD-1 and its ligand PD-L1, thereby restoring immune cell activity and strengthening the body’s defense against cancer. Nivolumab is widely used to treat a variety of cancers and has shown good efficac (77). Unresectable or metastatic melanoma, melanoma as adjuvant therapy, resectable or metastatic non-SCLC, SCLC, advanced renal cell carcinoma, classical Hodgkin lymphoma, head and neck squamous cell carcinoma, urothelial carcinoma, metastatic colorectal cancer with high microsatellite instability or defective mismatch repair, liver cell carcinoma, esophageal cancer, and others with high microsatellite instability or defective mismatch repair. Chen et al (71), investigated the impact of concurrent administration of SRS-SRT and immune checkpoint inhibitors on prognosis and safety in patients with BM (metastatic non-SCLC, melanoma, and renal cell carcinoma). Patients receiving SRS-SRT treatment with anti-cytotoxic T lymphocyte-related protein 4 (ipilimumab) and anti-programmed cell death protein 1 receptor (nivolumab and pembrolizumab) were included. Patients using immune checkpoint inhibitors in ongoing or unreported clinical trials were excluded, and concomitant use of ICIs was defined as ICI use within 2 weeks of SRS-SRT treatment. Patients were treated with SRS-SRT, SRS-SRT without ICI, or SRS-SRT with ICI. The results of this study showed that ICI concurrent with SRS-SRT may reduce the incidence of new BM without increasing the incidence of adverse events and may result in favorable survival outcomes. Crinò et al (78), reported the efficacy and safety of nivolumab in nonsquamous NSCLC. In their study, nivolumab was indicated for patients with stage IIIB/IV non-squamous NSCLC whose disease had progressed after at least one prior therapy. Patients with brain metastases, on the other hand, were included as long as they were asymptomatic, neurologically stable, had discontinued corticosteroids, or their prednisone dose was stable or reduced to less than 10 mg per day. The results of this study suggest that patients with BMfrom non-squamous NSCLC are asymptomatic or have controlled brain metastases. Renal cell carcinoma accounts for approximately 4% of all solid tumors, with an incidence of approximately 16.1 per 100,000 population; approximately 1/3 of RCC patients are in an advanced stage at diagnosis (79, 80). The first-line treatment for advanced renal cell carcinoma is targeted therapy combined with immunotherapy, and metastasectomy, radiofrequency ablation, and targeted therapy are the preferred treatment for patients with oligometastatic or low tumor burden trans metastatic renal cell carcinoma (mRCC) (81). Although these therapies help prolong OS in patients with mRCC, the side effects of drug therapy affect patients’ quality of life. An overview of the challenges of brain metastasis in renal cell carcinoma is shown in Figure 4. Flippot et al (82), evaluated the activity of nivolumab in patients with brain metastatic clear cell renal cell carcinoma (ccRCC) after failure of angiogenic therapy. The study population consisted of patients with previously treated or untreated brain metastases. The primary endpoint was intracranial response efficiency in patients. Study results showed limited activity of nivolumab in patients with untreated ccRCC brain metastases. Brain imaging and treatment of the lesion should be considered before using immune checkpoint inhibitors in patients with metastatic ccRCC. Reardon et al (83) examined the role and value of bevacizumab and nivolumab monotherapy in improving survival in patients with recurrent glioblastoma. The study recruited 439 patients (369 of whom were randomized) with first recurrence of glioblastoma after treatment with standard radiation therapy and temozolomide. Patients were randomized 1:1 to either nivolumab 3 mg/kg or bevacizumab 10 mg/kg every 2 weeks until disease progression, unacceptable toxicity, or death was confirmed. The primary endpoint was OS. The results of this study suggest that overall mOS values for nivolumab and bevacizumab in patients with recurrent glioblastoma are similar, and the safety profile of nivolumab in patients with glioblastoma is consistent with that of other tumor types. The CheckMate 920 trial investigated the safety and efficacy of nivolumab plus ipilimumab in patients with advanced renal cell carcinoma (aRCC) and brain metastases. The study revealed a 32% objective response rate (ORR) in response-evaluable patients, with a median duration of response (DOR) of 24.0 months and a median time to response (TTR) of 2.8 months. Some patients experienced intracranial progression, and the safety profile of the treatment regimen, including immune-mediated adverse events, was assessed (83).
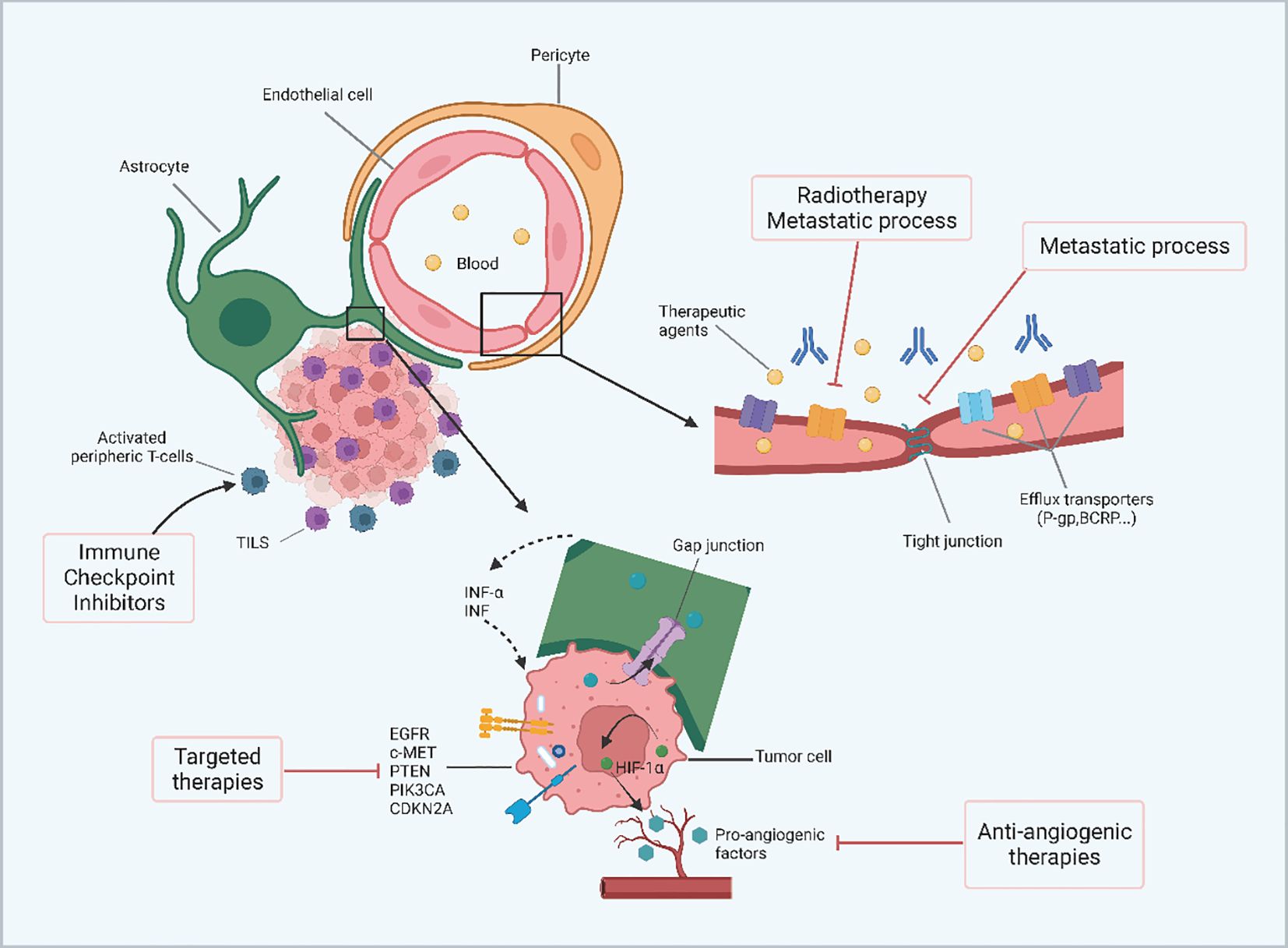
Figure 4 The overview of the challenges of brain metastasis from renal cell carcinoma. In the microenvironment of renal cancer brain metastases, the BBB can limit the permeability of therapeutic agents. The number of efflux transporters is significantly reduced during metastasis and radiotherapy thereby increasing the permeability of peripheral molecules. In addition, tight junctions are impaired by metastatic development leading to increased permeability of the blood-brain barrier. Gap junctions may allow metabolite transfer between renal cancer cells and astrocytes and induce secretion of INF-α and TNF by astrocytes, leading to chemoresistance. The molecular characteristics of renal cancer cells, including highly angiogenic features, molecular inconsistencies between primary and brain metastases, and inherent radio resistance also affect the outcome of renal cancer brain metastases. (Created with BioRender.com).
3.1.7 Pembrolizumab
Pembrolizumab exerts its antitumor effects by inhibiting the binding of PD-1 receptors on immune cells to PD-L1 on tumor cells, thereby restoring the ability of immune cells to attack tumors. pembrolizumab has been used in the treatment of many different Nasopharyngeal carcinoma (84) with broad indications for the treatment of many different types of malignancies. Studies have shown that pembrolizumab is an alternative to cytotoxic chemotherapy as first-line therapy in patients with PD-L1 tumor percentage scores of 50% or greater. The study reported the use of pembrolizumab in patients with giant cell lung cancer (85). A 69-year-old female patient with giant cell lung cancer, clinically classified as IVB (T2bN0M1c, BRA) and with a high percentage of tumors expressing PD-L1, received stereotactic radiotherapy targeting two cerebellar metastases, followed by immunotherapy with an anti-PD-1 antibody (pembrolizumab) for four treatment cycles. The tumor shrank significantly after 4 cycles of treatment. However, treatment was discontinued due to renal dysfunction. This suggests that pembrolizumab combined with radiotherapy also has a favorable therapeutic effect in giant cell lung cancer. reported the management of a patient with multiple metastases of NSCLC with exon 19 deletion and PD-L1 deletion. Pembrolizumab plus chemotherapy and SBRT were then initiated for the supraclavicular metastases and spinal cord lesions; examination after four cycles showed resolution of adenopathy, reduction in lung mass, liver and spinal cord lesions, and no lesions or new metastases were detected on brain MRI. The patient then continued treatment with pembrolizumab plus pemetrexed for almost a year and is now in good disease control. A study was conducting a phase II trial of pembrolizumab in untreated NSCLC or melanoma patients with BM to investigate the activity of PD-1 blocking agents in the central nervous system (86). Cohort 1 consisted of patients with PD-L1 ≥1% and cohort 2 consisted of patients with PD-L1 <1% or not evaluable. The primary endpoint was the proportion of patients who achieved a response in brain metastases. The results of this study showed that pembrolizumab is effective for NSCLC BM with PD-L1 expression of 1% or greater and is safe in some untreated patients with brain metastases. Breast cancer is the most common malignancy in women worldwide, and the incidence of BM from breast cancer is on the rise, ranging from 5% to 21% (87, 88), as new therapeutic agents and imaging techniques advance (Figure 5). The incidence of BM from breast cancer is highest in HER2-positive types, accounting for about 30% to 55% (89, 90). There are mainly parenchymal and meningeal metastases, with parenchymal metastases being more common (91, 92). The prognosis for BM from breast cancer is usually very poor because the BBB severely limits the entry of most chemotherapeutic agents into the nervous system (93, 94). Local radiation therapy and surgical treatment can slow the progression of the disease, but it is difficult to completely kill the cancer cells in the body (95, 96). Therefore, the development of systemic therapeutics and the selection of new therapies are crucial for the treatment of brain metastasis of breast cancer. Wu et al (97),. reported on the combination of anti-estrogenic drugs and immunotherapy in patients with HR-positive metastatic breast cancer. The first patient was a patient with recurrent breast cancer with ovarian and BM after endocrine therapy. After surgery for the ovarian lesions and three cycles of chemotherapy, a high degree of T-cell receptor (TCR) complexes were observed in the tumor. The patient then received a combination of trazodone and pembrolizumab. The patient achieved a partial response and had a PFS of more than 21 months; the second patient was a breast cancer patient with multiple bone metastases. The second patient had multiple bone metastases and was treated with a combination of tamoxifen and pembrolizumab because the combination of radiation and chemotherapy was ineffective. Another patient with BM from lung cancer underwent local resection of two BM by SRS and received systemic immunochemotherapy consisting of four cycles of cisplatin, pemetrexed, and pembrolizumab. The patient then underwent left posterolateral thoracotomy, left lower lobe expansion resection, segment 1 wedge resection, and systematic clearance of hilar, mediastinal, and interlobar lymph nodes. Maintenance therapy with pembrolizumab was resumed postoperatively and was uneventful for 2 years. Thirty-five months after the initial diagnosis, CT scan of the chest and abdomen and MRI of the cranium showed no signs of local recurrence or metastasis. The ORR (objective response rate) of brain metastasis when using pembrolizumab was found to be 28.6%. The PFS (progression-free survival) was reported to be 4.0 months. These outcomes suggest that pembrolizumab may have some efficacy in the treatment of brain metastasis. However, further research and clinical trials are needed to establish its effectiveness and safety in this specific patient population (98).
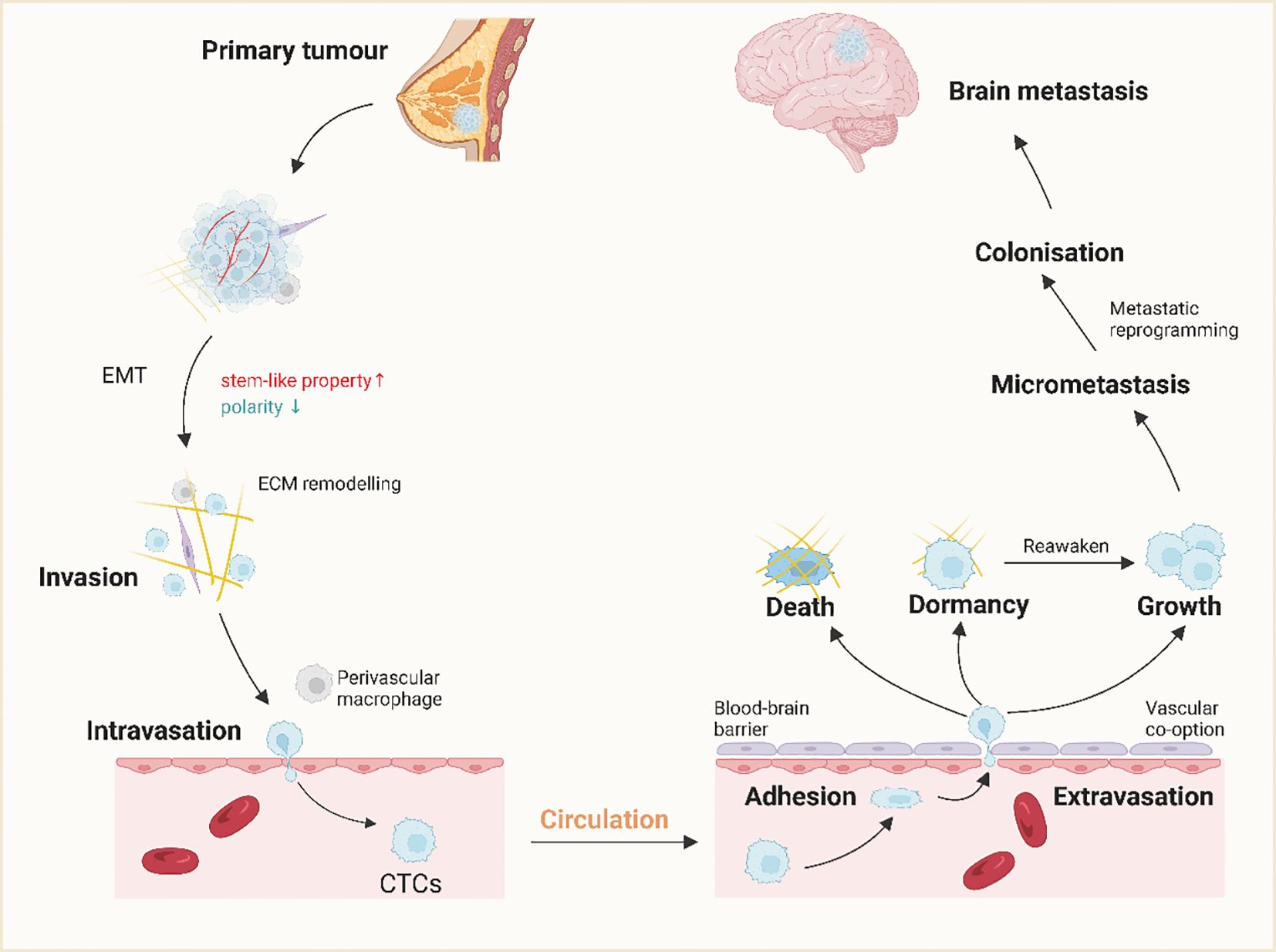
Figure 5 The mechanism of brain metastasis of breast cancer. A small population of breast cancer cells at the primary site acquires stem cell-like properties and, through epithelial-mesenchymal transition (EMT), invasive properties. Invasive breast cancer cells infiltrate the surrounding tissue through ECM remodeling and become circulating tumor cells (CTCs) with the help of perivascular macrophages and interactions with vascular endothelial cells (ECs).CTCs spread throughout the body through the bloodstream and cross the BBB through extravasation after adhering to endothelial cells in the brain. The majority of the cells die or become dormant, while a small number of cells proliferate in this new microenvironment. this new microenvironment proliferate. In addition, dormant cells are often reawakened under certain conditions and participate in colonization, leading to tumor recurrence. (Created with BioRender.com).
3.1.8 Sintilimab
Sintilimab is a novel immunotherapeutic agent that is a humanized monoclonal antibody. Sintilimab is widely used to treat a variety of malignancies, including but not limited to non-SCLC, melanoma, ESCC, renal cell carcinoma, and bladder cancer (99, 100). It is considered an innovative therapy that has the potential to change the landscape of conventional tumor treatment. Nong et al. Nong et al (101), reported the diagnosis and treatment with sintilimab in a patient with lung adenocarcinoma with brain metastases. The patient had an in-frame insertion of epidermal growth factor receptor exon 20 and was treated with pemetrexed and carboplatin plus the programmed cell death-1 inhibitor sintilimab After six cycles of treatment, the patient received sintilimab plus pemetrexed for patients received maintenance therapy every 3 weeks with sintilimab plus pemetrexed, which was effective without toxicity. This suggests an important role for sintilimab in patients with brain metastatic NSCLC who have an insertional mutation in exon 20 of the epidermal growth factor receptor.
3.1.9 Tisulizumab
Tislelizumab is a new generation targeted immunotherapy drug widely used to treat many malignancies. It is a humanized monoclonal antibody that strengthens a patient’s own immune system to fight tumor cells by targeting the immune checkpoint PD-1. It has shown excellent antitumor activity in a wide range of cancer types (102), including non-SCLC, melanoma, renal cell carcinoma, esophageal cancer, and nasopharyngeal cancer. The use of tislelizumab can further improve survival and quality of life for patients. Fu et al (103),. reported the progress of tislelizumab in a patient with invasive lung adenocarcinoma with brain metastases. The patient underwent right upper lung lobectomy and lymph node dissection. Postoperative pathology revealed invasive adenocarcinoma (alveolar, papillary, and enhancing) with pleural invasion, staged cT0N0M1c, stage IVC. POLE and TP53 mutations were found. The patient then received two cycles of combination therapy with pemetrexed + carboplatin + bevacizumab + tislelizumab. After two cycles of treatment, the patient’s intracranial metastases became smaller; after four cycles of combination therapy, the patient’s metastases completely resolved After four cycles of combination therapy, the patient’s metastases had completely disappeared. The patient then received two cycles of consolidation therapy with tislelizumab, pemetrexed, and bevacizumab. 6 cycles of treatment later, the patient felt fatigue and anorexia. Treatment was then switched to tislelizumab and bevacizumab for six cycles to date. The patient responded well and had no treatment-related adverse events 11 months after starting the combination.
3.1.10 Toripalimab
Toripalimab is a PD-1 antibody that inhibits the immune escape mechanism of melanoma cells by targeting the immune checkpoint PD-1, a membrane surface receptor that regulates immune response homeostasis and prevents over-activated immune cells from attacking normal tissue. In melanoma, tumor cells normally overexpress PD-L1, which binds to PD-L1 to inhibit immune cell function and evade immune attack. Reported on the diagnosis and treatment with toripalimab in a patient with SCLC with brain metastases (104). The patient was diagnosed with localized stage small cell carcinoma of the left lower lung and received 6 cycles of initial chemotherapy with etoposide and nedaplatin followed by adaptive intensity modulated radiation therapy (IMRT) to the thorax and prophylactic brain radiation therapy to achieve CR. Approximately 3 months after completion of radiotherapy, chest CT and brain-enhanced MRI confirmed that CR was sustained. Approximately 6 months after completion of radiotherapy, a cranial-weighted MRI showed metastasis in the left cerebellar hemisphere. The patient was then treated with IMRT and anlotinib; at the end of IMRT, irinotecan and lopressor were added to anlotinib. Due to grade 3 adverse events, patients received 3 cycles of maintenance therapy with sindilizumab plus anlotinib (104). However, 2.5 months after achieving CR, the BM recurred. Because the recurrent lesion was small and asymptomatic, treatment with sindilizumab in combination with erlotinib was continued for 3 more cycles. A skull-weighted MRI showed no change in the target lesion. The physician then switched from sindilizumab to toripalimab. After two cycles of treatment with toripalimab in combination with anlotinib, the recurrent BM reached CR and were maintained for 6 months. In conclusion, the safety profile of toripalimab in combination with erlotinib was favorable and no serious adverse events were observed during treatment.
3.2 Immune cell therapy
Lymphocytes that possess cytotoxic abilities in vivo encompass natural killer cells and cytotoxic T cells, both of which can effectively counteract tumor cell proliferation. Empirical evidence suggests that several hundred lymphocytes are required to combat a single tumor cell. Hence, a larger population of lymphocytes confers a greater capacity for tumor cell elimination and inhibition of tumor cell production. This fundamental principle forms the basis of cellular immunotherapy. Presently, cellular biopharmacotherapy, exemplified by cellular immunotherapy, represents a significant advancement in the field of tumor biotherapy. Adoptive cellular immunotherapy (ACI) is the accepted nomenclature for this modality and entails the infusion of immune cells with antitumor properties (both specific and nonspecific) into tumor patients, either for direct tumor eradication or to induce the patient’s immune response to target tumor cells (105). Clinically, ACI entails the administration of autologous or allogeneic immune effector cells that have been activated in vitro, thereby inducing tumor cell death within the patient’s body (106, 107). In recent years, cellular immunotherapy has emerged as a vibrant domain within tumor biotherapy, specifically suited for patients with compromised cellular immunity, particularly those afflicted with hematologic and immune system malignancies, such as those arising after intense chemotherapy, radiotherapy, bone marrow transplantation, or viral infections leading to depletion and dysfunction of immune cells (108, 109). Cellular immunotherapy possesses the ability to selectively suppress and eliminate tumor cells, independent of the patient’s inherent immune function, and can be effectively combined with radiation therapy and chemotherapy. The efficacy, specificity, overall therapeutic effectiveness, and side effect profile of this approach have shown progressive enhancements during the evolutionary stages of LAK, TIL, CD3AK, CIK, DC-CIK, and EAAL (110, 111).
3.3 Tumor vaccine
Tumor vaccines have gained significant attention in recent years as a focal point of research in the medical field. The fundamental concept behind tumor vaccines involves the administration of tumor antigens into the patient’s system by various means, such as tumor cells, tumor-related proteins and peptides, and genetic material encoding tumor antigens. This approach is aimed at counteracting the immune-suppressive environment induced by the tumor, enhancing immunogenicity, and stimulating the patient’s endogenous immune response. Activation and stimulation of both cellular and humoral immune responses in the body are crucial components in achieving the ultimate goal of controlling or eradicating tumors (112, 113). There are three primary classifications of tumor vaccines: prophylactic tumor vaccines, therapeutic tumor vaccines, and immuno-cellular therapy vaccines (114). Prophylactic tumor vaccines are primarily utilized to prevent the development of specific malignancies, such as cervical and liver cancer vaccines. In contrast, therapeutic tumor vaccines are designed for the treatment of patients with existing tumors. Examples of therapeutic tumor vaccines encompass tumor cell vaccines and tumor-associated antigen vaccines. Finally, immuno-cellular therapeutic vaccines involve the manipulation of the patient’s immune cells to combat tumors, including tumor-infiltrating lymphocyte vaccines and dendritic cell vaccines.
As scientific knowledge and technological capabilities continue to progress, the categorization of tumor vaccines is evolving, presenting new possibilities and optimism for tumor prevention and treatment. Current research predominantly concentrates on the advancement and assessment of tumor vaccines as a potential therapeutic modality for a range of malignancies. In recent times, there have been significant advancements in comprehending the mechanisms through which tumors evade the immune system and devising strategies to overcome these challenges. Various types of tumor vaccines, such as peptide-based, dendritic cell-based, and whole tumor cell-based vaccines, are undergoing scrutiny in preclinical and clinical trials. These vaccines are designed to provoke a targeted immune response against tumor antigens, with the objective of facilitating the specific destruction of tumor cells, averting tumor recurrence, and enhancing patient outcomes.
While early-phase trials have shown promise, further exploration and refinement of vaccine design, delivery modalities, and patient selection are imperative to amplify their effectiveness and delineate their role in cancer management. Consequently, ongoing research in this domain holds substantial promise for introducing novel therapeutic avenues to combat malignancies and elevate the quality of patient care.
In summary, immune checkpoint inhibitors, such as CTLA-4 inhibitors (e.g., Ipilimumab), PD-1 inhibitors (e.g., Pembrolizumab, Nivolumab), PD-L1 inhibitors (e.g., Atezolizumab, Durvalumab), LAG-3 inhibitors (e.g., Relatlimab), and TIM-3 inhibitors (e.g., Sabatolimab), have distinct characteristics and mechanisms of action. These inhibitors target specific receptors on T cells or ligands on cancer cells, aiming to enhance the immune response against cancer by restoring T cell activity, promoting anti-tumor immune responses, and potentially reversing T cell exhaustion. Immune checkpoint inhibitors play a vital role in cancer immunotherapy, offering potential in improving the body’s ability to combat cancer (Table 2). Immune checkpoint inhibitors are pivotal in modulating the immune response directed towards cancer cells through the targeting of specific regulatory checkpoints governing T cell functionality. Integral to cancer immunotherapy, these agents exhibit significant potential in bolstering the host’s anti-cancer defenses.
4 Discussion
The tumor microenvironment (TME) is a complex and dynamic system that plays a crucial role in tumor development and growth. It consists of four major components: non-tumor cells, extracellular matrix, vasculature, and soluble products (115, 116). Non-tumor cells in the TME include immune cells, fibroblasts, endothelial cells, and neurons. The extracellular matrix provides structural support for cells and regulates their functions through a network of proteins. The vasculature supplies oxygen and nutrients to tumor cells, often forming a dense network around tumors. Soluble products, such as chemokines, within the TME significantly influence cellular activities (117). The interactions among these components have profound effects on tumorigenesis, metastasis, and drug resistance, ultimately impacting the metabolic patterns and immune responses within the TME. Brain metastases (BM) are a common and challenging complication in cancer patients, affecting over 10% of patients at diagnosis and escalating to 30-40% during disease progression (118, 119). Patients with BM have a grim prognosis, marked by high mortality rates, poor quality of life, and a median overall survival of merely 4-6 months. The blood-brain barrier (BBB) poses challenges for the treatment of BM by limiting the efficacy of systemic chemotherapy. Current treatment options for BM include surgical resection, stereotactic radiosurgery, and whole-brain radiation therapy. However, their effectiveness varies depending on the number and size of metastatic brain lesions, with whole-brain radiation therapy typically yielding response rates between 50% and 75%, and survival rates ranging from 4 to 9 months Recent advances in tumor immunology research have led to the development of targeted therapies, such as CTLA-4 and PD-1/PD-L1 inhibitors, which have shown promising efficacy rates compared to traditional chemotherapeutic agents (120, 121). However, the effectiveness of immunotherapy alone may be limited due to immunosuppressive factors in tumor patients. Therefore, combination therapies involving tumor immunotherapy and other treatment modalities have become a future direction in cancer treatment (122, 123). Although immunotherapy has demonstrated significant benefits in treating advanced tumors, its efficacy is limited to certain tumor types, and individual differences may result in poor response rates. Immune-related complications are also common. Therefore, efforts to improve the efficiency and reduce the risk of tumor recurrence of immunotherapy, particularly PD-1/PD-L1 inhibitors, are needed (124, 125). Predictive markers and an understanding of drug resistance mechanisms are crucial for treatment selection and prognostic assessment.
The presence of the BBB poses a challenge for conventional chemotherapeutic agents to effectively reach brain metastases. The successful application of immune checkpoint inhibitors (ICIs) in patients with brain metastatic cancer has raised expectations for the potential of immunotherapy in the treatment of primary and metastatic brain cancer. However, the clinical implementation of immunotherapy in patients with brain metastases faces substantial obstacles due to the lack of robust predictors and appropriate animal models for evaluating efficacy. A comprehensive understanding of the biological underpinnings and specific mechanisms of immunosuppression in brain metastatic cancer is imperative for the development of novel immunological interventions. Clinical trials are needed to establish the effectiveness of immunotherapy in treating brain metastatic cancer and to identify precise biomarkers for patient selection. Furthermore, the heterogeneity of brain metastases and the limited infiltration of immune cells present challenges for effective immunotherapy. Strategies to enhance the penetration of immunotherapeutic agents through the BBB and to increase immune cell recruitment and infiltration into brain metastases are being explored. Resistance mechanisms, such as immune checkpoint upregulation and alterations in antigen presentation, can also develop in brain metastases. Combination therapies that target multiple resistance mechanisms and immunotherapies are necessary to overcome resistance and improve treatment outcomes. Managing immune-related adverse events and developing more targeted and selective immunotherapies are essential for the safe and effective use of immunotherapy in brain metastases. Combination therapies, targeted delivery systems, and personalized immunotherapies are being investigated to improve the efficacy and safety of immunotherapy for brain metastases.
In conclusion, immunotherapy holds promise as a treatment approach for brain metastases. However, challenges related to the BBB, tumor heterogeneity, limited immune cell infiltration, resistance mechanisms, and immune-related adverse events need to be addressed to optimize the efficacy and safety of immunotherapy in patients with brain metastases. Various strategies, such as enhanced BBB penetration, combination therapies, and personalized immunotherapies, are being explored to overcome these challenges and improve treatment outcomes.
Author contributions
TL: Writing – original draft. SS: Conceptualization, Investigation, Writing – original draft. YL: Writing – original draft. YZ: Writing – review & editing. LW: Writing – original draft, Writing – review & editing.
Funding
The author(s) declare that no financial support was received for the research, authorship, and/or publication of this article.
Acknowledgments
This work was supported by the China Medical University (Shenyang).
Conflict of interest
The authors declare that the research was conducted in the absence of any commercial or financial relationships that could be construed as a potential conflict of interest.
Publisher’s note
All claims expressed in this article are solely those of the authors and do not necessarily represent those of their affiliated organizations, or those of the publisher, the editors and the reviewers. Any product that may be evaluated in this article, or claim that may be made by its manufacturer, is not guaranteed or endorsed by the publisher.
Abbreviation
NK cells, Natural killer cells; ILs, interleukins; TNF, tumor necrosis factor; TGF, transforming growth factor; BBB, blood-brain barrier; TJP1, tight junction protein 1; TME, tumor microenvironment; PMN, pre-metastatic niche; TDSFs, tumor-derived secreted factors; EVs, extracellular vesicles; BMDCs, bone marrow-derived cells; VEGF, vascular endothelial growth factor; MMP, matrix metalloproteinase; CTCs, circulating tumor cells; ICI, immune checkpoint inhibitor; NSCLC, non- small cell lung cancer; SCLC, small cell lung cancer; WBRT, whole brain radiotherapy; SRS, stereotactic radiosurgery; ORR, objective response rate; PFS, progression-free survival; OS, overall survival; mRCC, metastatic renal cell carcinoma; ccRCC, clear cell renal cell carcinoma; EGFR, epidermal growth factor receptor; TCR, T cell receptor; ACI, adoptive cellular immunotherapy; ECM, extracellular matrix; CTLA – 4, cytotoxic T-lymphocyte - associated antigen 4; PD-1, programmed cell death protein 1; PD - L1, programmed cell death 1 ligand 1.
References
1. Tabor JK, Onoichenco A, Narayan V, Wernicke AG, D'Amico RS, Vojnic M. Brain metastasis screening in the molecular age. Neuro-oncology advances. (2023) 5:vdad080. doi: 10.1093/noajnl/vdad080
2. Luo T, Kang Y, Liu Y, Li J, Li J. Small extracellular vesicles in breast cancer brain metastasis and the prospect of clinical application. Front Bioeng Biotechnol. (2023) 11:1162089. doi: 10.3389/fbioe.2023.1162089
3. Brozos-Vázquez EM, Rodríguez-López C, Cortegoso-Mosquera A, Lopez-Landrove S, Muinelo-Romay L, García-González J, et al. Immunotherapy in patients with brain metastasis: advances and challenges for the treatment and the application of circulating biomarkers. Front Immunol. (2023) 14:1221113. doi: 10.3389/fimmu.2023.1221113
4. Alsabbagh R, Ahmed M, Alqudah MAY, Hamoudi R, Harati R. Insights into the molecular mechanisms mediating extravasation in brain metastasis of breast cancer, melanoma, and lung cancer. Cancers (Basel). (2023) 15(8). doi: 10.3390/cancers15082258
5. Nakhjavani M, Shigdar S. Natural blockers of PD-1/PD-L1 interaction for the immunotherapy of triple-negative breast cancer-brain metastasis. Cancers (Basel). (2022) 14(24). doi: 10.3390/cancers14246258
6. Aili Y, Maimaitiming N, Qin H, Ji W, Fan G, Wang Z, et al. Tumor microenvironment and exosomes in brain metastasis: Molecular mechanisms and clinical application. Front Oncol. (2022) 12:983878. doi: 10.3389/fonc.2022.983878
7. Yue M, Hu S, Sun H, Tuo B, Jia B, Chen C, et al. Extracellular vesicles remodel tumor environment for cancer immunotherapy. Mol Cancer. (2023) 22:203. doi: 10.1186/s12943-023-01898-5
8. Cui Z. Transitioning cancer cells and the surrounding tumor environment in pancreatic cancer. Nat Genet. (2022) 54:1269–70. doi: 10.1038/s41588-022-01162-4
9. Huang X, Li L, Ou C, Meiling S, Xinchao L, Miaomiao Z, et al. Tumor environment regression therapy implemented by switchable prune-to-essence nanoplatform unleashed systemic immune responses. Adv Sci (Weinh). Dec. (2023) 10:e2303715. doi: 10.1002/advs.202303715
10. Gao Y, Chen S, Wang H, Chenghao W, Rui A, Guoli L, et al. Liver metastases across cancer types sharing tumor environment immunotolerance can impede immune response therapy and immune monitoring. J Adv Res. (2023) 61(0):151–64. doi: 10.1016/j.jare.2023.08.011
11. Gong X, Ma Y, Deng X, Aiqing L, Xingjie L, Xueying K, et al. Intestinal dysbiosis exacerbates susceptibility to the anti-NMDA receptor encephalitis-like phenotype by changing blood brain barrier permeability and immune homeostasis. Brain behavior immunity. (2023) 116(0):34–51. doi: 10.1016/j.bbi.2023.11.030
12. Le Guennec L, Balcerac A, Weiss N. A letter concerning a role for blood-brain barrier dysfunction in delirium following noncardiac surgery in older adults. Ann neurology. (2024) 95(2):410–1. doi: 10.1002/ana.26837
13. Tang J, Kang Y, Zhou Y, Nianying S, Xinnan L, Hongyue W, et al. TIMP2 ameliorates blood-brain barrier disruption in traumatic brain injury by inhibiting Src-dependent VE-Cadherin internalization. J Clin Invest. (2023) 115:705–17. doi: 10.1172/JCI164199
14. Weng Y, Chen N, Zhang R, Jian H, Xukai D, Guo C, et al. An integral blood-brain barrier in adulthood relies on microglia-derived PDGFB. Brain behavior immunity. (2024) 115:705–17. doi: 10.1016/j.bbi.2023.11.023
15. Chagnot A, Montagne A. Connexins and blood-brain barrier: Beyond the gap. Neuron. Nov 15. (2023) 111:3499–501. doi: 10.1016/j.neuron.2023.10.032
16. Sun ZW, Wang X, Zhao Y, Sun ZX, Wu YH, Hu H, et al. Blood-brain barrier dysfunction mediated by the EZH2-Claudin-5 axis drives stress-induced TNF-alpha infiltration and depression-like behaviors. Brain behavior immunity. Jan. (2024) 115:143–56. doi: 10.1016/j.bbi.2023.10.010
17. Wang K, Zhao B, Ao Y, Zhao J, Zhao C, Wang W, et al. Super-small zwitterionic micelles enable the improvement of blood-brain barrier crossing for efficient orthotopic glioblastoma combinational therapy. J Control Release. Dec. (2023) 364:261–71. doi: 10.1016/j.jconrel.2023.10.019
18. Manuel TJ, Sigona MK, Phipps MA, Huiwen J, Kusunose L, Pai Feng Y, et al. Small volume blood-brain barrier opening in macaques with a 1 MHz ultrasound phased array. J Control Release. (2023) 363:707–20. doi: 10.1016/j.jconrel.2023.10.015
19. Grasso G, Torregrossa F, Noto M, Eleonora B, Paola F, Felice B, et al. MR-guided focused ultrasound-induced blood-brain barrier opening for brain metastasis: a review. Neurosurgical focus. (2023) 55:E11. doi: 10.3171/2023.5.FOCUS23227
20. Chhichholiya Y, Ruthuparna M, Velagaleti H, Munshi A. Brain metastasis in breast cancer: focus on genes and signaling pathways involved, blood-brain barrier and treatment strategies. Clin Transl Oncol May. (2023) 25:1218–41. doi: 10.1007/s12094-022-03050-z
21. Rehman AU, Khan P, Maurya SK, Siddiqui JA, Santamaria-Barria JA, Batra SK, et al. Liquid biopsies to occult brain metastasis. Mol Cancer. (2022) 21:113. doi: 10.1186/s12943-022-01577-x
22. Burn L, Gutowski N, Whatmore J, Giamas G, Pranjol MZI. The role of astrocytes in brain metastasis at the interface of circulating tumour cells and the blood brain barrier. Front Biosci (Landmark Ed). (2021) 26:590–601. doi: 10.52586/4969
23. Doron H, Pukrop T, Erez N. A blazing landscape: neuroinflammation shapes brain metastasis. Cancer Res. (2019) 79:423–36. doi: 10.1158/0008-5472.CAN-18-1805
24. Masmudi-Martín M, Zhu L, Sanchez-Navarro M, Priego N, Casanova AM, Ruiz RV, et al. Brain metastasis models: What should we aim to achieve better treatments? Adv Drug Delivery Rev Feb. (2021) 169:79–99. doi: 10.1016/j.addr.2020.12.002
25. Miller CR, Hjelmeland AB. Breaking the feed forward inflammatory cytokine loop in the tumor microenvironment of PDGFB-driven glioblastomas. J Clin Invest. (2023) 133(22). doi: 10.1172/JCI175127
26. Maas RR, Soukup K, Fournier N, Massara M, Galland S, Kornete M, et al. The local microenvironment drives activation of neutrophils in human brain tumors. Cell. (2023) 186:4546–4566.e27. doi: 10.1016/j.cell.2023.08.043
27. Chen Q, Zhou S, Ding Y, Chen D, Dahiru NS, Tang H, et al. A bio-responsive, cargo-catchable gel for postsurgical tumor treatment via ICD-based immunotherapy. J Control Release. (2022) 346:212–25. doi: 10.1016/j.jconrel.2022.04.015
28. Shi Y, Jiang J, Cui Y, Chen Y, Dong T, An H, et al. MSH6 aggravates the hypoxic microenvironment via regulating HIF1A to promote the metastasis of glioblastoma multiforme. DNA Cell Biol. (2021) 40:93–100. doi: 10.1089/dna.2020.5442
29. Zhang Q, Wang Y, Liu F. Cancer-associated fibroblasts: Versatile mediators in remodeling the tumor microenvironment. Cell Signal. (2023) 103:110567. doi: 10.1016/j.cellsig.2022.110567
30. Ben-Shlomo A. Exploring the role of the tumor microenvironment in refractory pituitary tumor pathogenesis. Pituitary. (2023) 26:263–5. doi: 10.1007/s11102-023-01301-y
31. Messiaen J, Jacobs SA, De Smet F. The tumor micro-environment in pediatric glioma: friend or foe? Front Immunol. (2023) 14:1227126. doi: 10.3389/fimmu.2023.1227126
32. Li Y, Wang J, Song SR, Lv SQ, Qin JH, Yu SC. Models for evaluating glioblastoma invasion along white matter tracts. Trends Biotechnol. (2023) 42(3):293–309. doi: 10.1016/j.tibtech.2023.09.005
33. Nguyen DT, Liu R, Ogando-Rivas E, Pepe A, Pedro D, Qdaisat S, et al. Bioconjugated liquid-like solid enhances characterization of solid tumor - chimeric antigen receptor T cell interactions. Acta Biomater. (2023) 172:466–79. doi: 10.1016/j.actbio.2023.09.042
34. Wang Y, Liu W, Geng P, Du W, Guo C, Wang Q, et al. Role of crosstalk between glial cells and immune cells in blood-brain barrier damage and protection after acute ischemic stroke. Aging Dis. (2023). doi: 10.14336/AD.2023.1010
35. Deshpande K, Buchanan I, Martirosian V, Neman J. Clinical perspectives in brain metastasis. Cold Spring Harbor Perspect Med. (2020) 10(6). doi: 10.1101/cshperspect.a037051
36. Yong T, Li X, Wei Z, Gan L, Yang X. Extracellular vesicles-based drug delivery systems for cancer immunotherapy. J Control Release. (2020) 328:562–74. doi: 10.1016/j.jconrel.2020.09.028
37. Allais BS, Fay CJ, Kim DY, Semenov YR, LeBoeuf NR. Cutaneous immune-related adverse events from immune checkpoint inhibitor therapy: Moving beyond "maculopapular rash". Immunol Rev. (2023) 318:22–36. doi: 10.1111/imr.13257
38. Berner F, Flatz L. Autoimmunity in immune checkpoint inhibitor-induced immune-related adverse events: A focus on autoimmune skin toxicity and pneumonitis. Immunol Rev. (2023) 318:37–50. doi: 10.1111/imr.13258
39. Goodman RS, Jung S, Balko JM, Johnson DB. Biomarkers of immune checkpoint inhibitor response and toxicity: Challenges and opportunities. Immunol Rev. (2023) 318:157–66. doi: 10.1111/imr.13249
40. Song R, Liu F, Ping Y, Zhang Y, Wang L. Potential non-invasive biomarkers in tumor immune checkpoint inhibitor therapy: response and prognosis prediction. biomark Res. (2023) 11:57. doi: 10.1186/s40364-023-00498-1
41. Zhang C, Zhang C, Wang H. Immune-checkpoint inhibitor resistance in cancer treatment: Current progress and future directions. Cancer Lett. (2023) 562:216182. doi: 10.1016/j.canlet.2023.216182
42. Tan S, Day D, Nicholls SJ, Segelov E. Immune checkpoint inhibitor therapy in oncology: current uses and future directions: JACC: cardioOncology state-of-the-art review. JACC CardioOncology. Dec. (2022) 4:579–97. doi: 10.1016/j.jaccao.2022.09.004
43. Mu L, Song Y, Zhao K, Liu Y, Fan Q, Wang X, et al. SHR-1316, an anti-PD-L1 antibody, plus chemotherapy as the first-line treatment for advanced esophageal squamous cell carcinoma: A multicentre, phase 2 study. Thorac cancer. (2021) 12:1373–81. doi: 10.1111/1759-7714.13913
44. Wang J, Zhou C, Yao W, Han S, Li D. Adebrelimab or placebo plus carboplatin and etoposide as first-line treatment for extensive-stage small-cell lung cancer (CAPSTONE-1): a multicentre, randomised, double-blind, placebo-controlled, phase 3 trial. Lancet Oncol. (2022) 23:739–47. doi: 10.1016/S1470-2045(22)00224-8
45. Yan W, Zhong WZ, Liu YH, Chen Q, Xing W, Zhang Q, et al. Adebrelimab (SHR-1316) in combination with chemotherapy as perioperative treatment in patients with resectable stage II to III NSCLCs: an open-label, multicenter, phase 1b trial. J Thorac Oncol. (2023) 18:194–203. doi: 10.1016/j.jtho.2022.09.222
46. Pan K, Concannon K, Li J, Zhang J, Heymach JV, Le X. Emerging therapeutics and evolving assessment criteria for intracranial metastases in patients with oncogene-driven non-small-cell lung cancer. Nat Rev Clin Oncol. (2023) 20:716–32. doi: 10.1038/s41571-023-00808-4
47. Gillespie CS, Mustafa MA, Richardson GE, Alam AM, Lee KS, Hughes DM, et al. Genomic alterations and the incidence of brain metastases in advanced and metastatic NSCLC: A systematic review and meta-analysis. J Thorac Oncol. (2023) 18:1703–13. doi: 10.1016/j.jtho.2023.06.017
48. Passaro A, Mok TSK, Attili I, Wu YL, Tsuboi M, de Marinis F, et al. Adjuvant treatments for surgically resected non-small cell lung cancer harboring EGFR mutations: A review. JAMA Oncol. (2023) 9:1124–31. doi: 10.1001/jamaoncol.2023.0459
49. O'Dowd EL, Lee RW, Akram AR, Bartlett EC, Bradley SH, Brain K, et al. Defining the road map to a UK national lung cancer screening programme. Lancet Oncol. (2023) 24:e207–18. doi: 10.1016/S1470-2045(23)00104-3
50. Nardone V, Romeo C, D'Ippolito E, Pastina P, D'Apolito M, Pirtoli L, et al. The role of brain radiotherapy for EGFR- and ALK-positive non-small-cell lung cancer with brain metastases: a review. La Radiologia medica. (2023) 128:316–29. doi: 10.1007/s11547-023-01602-z
51. Ozcan G, Singh M, Vredenburgh JJ. Leptomeningeal metastasis from non-small cell lung cancer and current landscape of treatments. Clin Cancer Res. (2023) 29:11–29. doi: 10.1158/1078-0432.CCR-22-1585
52. Liu HC, Capuani S, Badachhape AA, Di TN, Davila GD, Vander PR, et al. Intratumoral nanofluidic system enhanced tumor biodistribution of PD-L1 antibody in triple-negative breast cancer. Bioeng Transl Med. (2023) 8:e10594. doi: 10.1002/btm2.10594
53. Rudin CM, Liu SV, Soo RA, Lu S, Hong MH, Lee JS, et al. SKYSCRAPER-02: tiragolumab in combination with atezolizumab plus chemotherapy in untreated extensive-stage small-cell lung cancer. J Clin Oncol. (2024) 42(3):324–35. doi: 10.1200/JCO.23.01363
54. Wang Y, Zhu J, Zhou N, Wang Y, Zhang X. Changes in T lymphocyte subsets predict the efficacy of atezolizumab in advanced non-small cell lung cancer: a retrospective study. J Thorac Dis. (2023) 15:5669–79. doi: 10.21037/jtd-23-1169
55. Lin X, Lu T, Xie Z, Qin Y, Liu M, Xie X, et al. Extracranial abscopal effect induced by combining immunotherapy with brain radiotherapy in a patient with lung adenocarcinoma: A case report and literature review. Thorac cancer. (2019) 10:1272–5. doi: 10.1111/1759-7714.13048
56. West H, McCleod M, Hussein M, Morabito A, Rittmeyer A, Conter HJ, et al. Atezolizumab in combination with carboplatin plus nab-paclitaxel chemotherapy compared with chemotherapy alone as first-line treatment for metastatic non-squamous non-small-cell lung cancer (IMpower130): a multicentre, randomised, open-label, phase 3 trial. Lancet Oncol. (2019) 20:924–37. doi: 10.1016/S1470-2045(19)30167-6
57. Jin Y, Xu J, Zhuang D, Dong L, Sun Y, Zhao L, et al. Hepatic cavernous hemangioma developed in non-small cell lung cancer patients after receiving Camrelizumab treatment: two case reports. Front Oncol. (2023) 13:1221309. doi: 10.3389/fonc.2023.1221309
58. Wu J, Song Y, Chen X, Tang Y, Zheng W, Liu W, et al. Camrelizumab for relapsed or refractory classical Hodgkin lymphoma: Extended follow-up of the multicenter, single-arm, Phase 2 study. Int J Cancer. (2022) 150:984–92. doi: 10.1002/ijc.33852
59. Xiao Y, Zhu G, Xie J, Luo L, Deng W, Lin L, et al. Pretreatment neutrophil-to-lymphocyte ratio as prognostic biomarkers in patients with unresectable hepatocellular carcinoma treated with hepatic arterial infusion chemotherapy combined with lenvatinib and camrelizumab. J hepatocellular carcinoma. (2023) 10:2049–58. doi: 10.2147/JHC.S432134
60. Chang J, Jing X, Hua Y, Geng K, Li R, Lu S, et al. Programmed cell death 1 pathway inhibitors improve the overall survival of small cell lung cancer patients with brain metastases. J Cancer Res Clin Oncol. (2023) 149:1825–33. doi: 10.1007/s00432-022-04121-y
61. Terashima Y, Hakozaki T, Uehara Y, Miyanaga A, Kasahara K, Seike M, et al. Prognostic significance of initial tumor shrinkage in patients with stage III non-small cell lung cancer treated with durvalumab following chemoradiotherapy. Int J Clin Oncol. (2023). doi: 10.1007/s10147-023-02436-5
62. Park S, Oh D, Choi YL, Chi SA, Kim K, Ahn MJ, et al. Durvalumab and tremelimumab with definitive chemoradiotherapy for locally advanced esophageal squamous cell carcinoma. Cancer. Jun 1. (2022) 128:2148–58. doi: 10.1002/cncr.34176
63. Cathomas R, Rothschild SI, Hayoz S, Bubendorf L, Özdemir BC, Kiss B, et al. Perioperative chemoimmunotherapy with durvalumab for muscle-invasive urothelial carcinoma: primary analysis of the single-arm phase II trial SAKK 06/17. J Clin Oncol. (2023) 41:5131–9. doi: 10.1200/JCO.23.00363
64. Gao P, Li T, Zhang K, Luo G. Recent advances in the molecular targeted drugs for prostate cancer. Int Urol nephrology. (2023) 55(4):777–89. doi: 10.1007/s11255-023-03487-3
65. Wu Y, Zhang T, Liu Y, Wang J, Bi N. Anlotinib combined with durvalumab in a patient with recurrent multifocal brain metastases of small cell lung cancer after definitive concurrent chemoradiotherapy and palliative radiotherapy of the lung and brain: a case report. Ann palliative Med. (2021) 10:2379–86. doi: 10.21037/apm-20-2390
66. Wohlfeil SA, Géraud C. Endothelial and tumor-intrinsic mechanisms of hepatic melanoma metastasis. J der Deutschen Dermatologischen Gesellschaft = J German Soc Dermatol JDDG. (2024) 22(1):18–21. doi: 10.1111/ddg.15233
67. Nowacka A, Fajkiel-Madajczyk A, Ohla J, Kamila W, browska D, Sara L, et al. Current treatment of melanoma brain metastases. Cancers (Basel). (2023) 15(16). doi: 10.3390/cancers15164088
68. Steininger J, Gellrich FF, Engellandt K, Matthias M, Dana W, Stefan B, et al. Leptomeningeal metastases in melanoma patients: an update on and future perspectives for diagnosis and treatment. Int J Mol Sci. (2023) 24(14). doi: 10.3390/ijms241411443
69. Boutros C, Belkadi-Sadou D, Marchand A, Roy S, Routier E, Robert C. Cured or not? Long-term outcomes of immunotherapy responders. Focus Melanoma. Curr Oncol Rep. (2023) 25:989–96. doi: 10.1007/s11912-023-01429-x
70. Tracz JA, Donnelly BM, Ngu S, Vojnic M, Wernicke AG, D'Amico RS. The abscopal effect: inducing immunogenicity in the treatment of brain metastases secondary to lung cancer and melanoma. J Neurooncol. (2023) 163:1–14. doi: 10.1007/s11060-023-04312-8
71. Chen L, Douglass J, Kleinberg L, Kenneth B, Douglas K, Ajay N, et al. Concurrent immune checkpoint inhibitors and stereotactic radiosurgery for brain metastases in non-small cell lung cancer, melanoma, and renal cell carcinoma. Int J Radiat Oncol Biol Phys. (2018) 100:916–25. doi: 10.1016/j.ijrobp.2017.11.041
72. Long GV, Atkinson V, Lo S, Shahneen S, Alexander DG, Michael PB, et al. Combination nivolumab and ipilimumab or nivolumab alone in melanoma brain metastases: a multicentre randomised phase 2 study. Lancet Oncol. (2018) 19:672–81. doi: 10.1016/S1470-2045(18)30139-6
73. Amaral T, Kiecker F, Schaefer S, Henner S, Katharina K, Patrick T, et al. Combined immunotherapy with nivolumab and ipilimumab with and without local therapy in patients with melanoma brain metastasis: a DeCOG* study in 380 patients. J Immunother Cancer. (2020) 8(1). doi: 10.1136/jitc-2019-000333
74. Borzillo V, Di Franco R, Giannarelli D, Cammarota F, Scipilliti E, D'Ippolito E, et al. Ipilimumab and stereotactic radiosurgery with cyberKnife((R)) system in melanoma brain metastases: A retrospective monoinstitutional experience. Cancers (Basel). (2021) 13(8). doi: 10.3390/cancers13081857
75. Tawbi HA, Forsyth PA, Hodi FS, Alain PA, Omid H, Christopher D, et al. Long-term outcomes of patients with active melanoma brain metastases treated with combination nivolumab plus ipilimumab (CheckMate 204): final results of an open-label, multicentre, phase 2 study. Lancet Oncol. (2021) 22:1692–704. doi: 10.1016/S1470-2045(21)00545-3
76. Pires da Silva I, Ahmed T, McQuade JL, Caroline AN, John JP, Judith MV, et al. Clinical models to define response and survival with anti-PD-1 antibodies alone or combined with ipilimumab in metastatic melanoma. J Clin Oncol. (2022) 40:1068–80. doi: 10.1200/JCO.21.01701
77. Mullick N, Nambudiri VE. Relatlimab-nivolumab: A practical overview for dermatologists. J Am Acad Dermatol. (2023) 89:1031–7. doi: 10.1016/j.jaad.2023.06.024
78. Crino L, Bronte G, Bidoli P, Paola C, Elisa M, Enrico C, et al. Nivolumab and brain metastases in patients with advanced non-squamous non-small cell lung cancer. Lung Cancer. (2019) 129:35–40. doi: 10.1016/j.lungcan.2018.12.025
79. Guo T, Zhang X, Chen S, Wang X, Wang X. Targeting lipid biosynthesis on the basis of conventional treatments for clear cell renal cell carcinoma: A promising therapeutic approach. Life Sci. (2023) 336:122329. doi: 10.1016/j.lfs.2023.122329
80. Sofia D, Zhou Q, Shahriyari L. Mathematical and machine learning models of renal cell carcinoma: A review. Bioengineering (Basel Switzerland). (2023) 10(11). doi: 10.3390/bioengineering10111320
81. Huang C, Esfani Sarafraz P, Enayati P, Mortazavi Mamaghani E, Babakhanzadeh E, Nazari M. Circular RNAs in renal cell carcinoma: from mechanistic to clinical perspective. Cancer Cell Int. (2023) 23:288. doi: 10.1186/s12935-023-03128-w
82. Flippot R, Dalban C, Laguerre B, Delphine B, Gwénaelle G, Sylvie N, et al. Safety and efficacy of nivolumab in brain metastases from renal cell carcinoma: results of the GETUG-AFU 26 NIVOREN multicenter phase II study. J Clin Oncol. (2019) 37:2008–16. doi: 10.1200/JCO.18.02218
83. Emamekhoo H, Olsen MR, Carthon BC, Alexandra D, Ivor JP, Ana MM, et al. Safety and efficacy of nivolumab plus ipilimumab in patients with advanced renal cell carcinoma with brain metastases: CheckMate 920. Cancer. (2022) 128:966–74. doi: 10.1002/cncr.34016
84. Stark MC, Joubert AM, Visagie MH. Molecular farming of pembrolizumab and nivolumab. Int J Mol Sci. (2023) 24(12). doi: 10.3390/ijms241210045
85. Kakimoto T, Sasaki M, Yamamoto T, Arifumi I, Kentaro O, Ko L, et al. A histologically complete response to immunotherapy using pembrolizumab in a patient with giant cell carcinoma of the lung: an additional report and literature review. Case Rep Oncol Med. (2019) 2019:1763625. doi: 10.1155/2019/1763625
86. Goldberg SB, Schalper KA, Gettinger SN, Amit M, Roy SH, Anne CC, et al. Pembrolizumab for management of patients with NSCLC and brain metastases: long-term results and biomarker analysis from a non-randomised, open-label, phase 2 trial. Lancet Oncol May. (2020) 21:655–63. doi: 10.1016/S1470-2045(20)30111-X
87. Kong X, Song J, Gao P, Ran G, Lin Z, Yi F, et al. Revolutionizing the battle against locally advanced breast cancer: A comprehensive insight into neoadjuvant radiotherapy. Med Res Rev. (2020) 21(5):655–63. doi: 10.1002/med.21998
88. Thakur C, Qiu Y, Pawar A, Chen F. Epigenetic regulation of breast cancer metastasis. Cancer Metastasis Rev. (2024) 44(2):606–31. doi: 10.1007/s10555-023-10146-7
89. Adam-Artigues A, Arenas EJ, Arribas J, Prat A, Cejalvo JM. AXL - a new player in resistance to HER2 blockade. Cancer Treat Rev. (2023) 121:102639. doi: 10.1016/j.ctrv.2023.102639
90. Zhang J, Wu J, Zhou XS, Shi F, Shen D. Recent advancements in artificial intelligence for breast cancer: Image augmentation, segmentation, diagnosis, and prognosis approaches. Semin Cancer Biol. (2023) 96:11–25. doi: 10.1016/j.semcancer.2023.09.001
91. Criscitiello C, Corti C, De Laurentiis M, Giampaolo B, Barbara P, Saverio C, et al. Tucatinib's journey from clinical development to clinical practice: New horizons for HER2-positive metastatic disease and promising prospects for brain metastatic spread. Cancer Treat Rev. (2023) 120:102618. doi: 10.1016/j.ctrv.2023.102618
92. Gil-Henn H, Girault JA, Lev S. PYK2, a hub of signaling networks in breast cancer progression. Trends Cell Biol. (2023) 120:102618. doi: 10.1016/j.tcb.2023.07.006
93. Pasquier D, Bidaut L, Oprea-Lager DE, Nandita M, deSouza Krug D, Collette L, et al. Designing clinical trials based on modern imaging and metastasis-directed treatments in patients with oligometastatic breast cancer: a consensus recommendation from the EORTC Imaging and Breast Cancer Groups. Lancet Oncol. (2023) 24:e331–43. doi: 10.1016/S1470-2045(23)00286-3
94. Medford AJ, Moy B, Spring LM, Hurvitz SA, Turner NC, Bardia A. Molecular residual disease in breast cancer: detection and therapeutic interception. Clin Cancer Res. (2023) 29:4540–8. doi: 10.1158/1078-0432.CCR-23-0757
95. Epaillard N, Bassil J, Pistilli B. Current indications and future perspectives for antibody-drug conjugates in brain metastases of breast cancer. Cancer Treat Rev. (2023) 119:102597. doi: 10.1016/j.ctrv.2023.102597
96. Yoshimura T, Li C, Wang Y, Matsukawa A. The chemokine monocyte chemoattractant protein-1/CCL2 is a promoter of breast cancer metastasis. Cell Mol Immunol. (2023) 20:714–38. doi: 10.1038/s41423-023-01013-0
97. Wu D, Tang S, Ye R, Li DM, Gu DJ, Chen RR, et al. Case report: long-term response to pembrolizumab combined with endocrine therapy in metastatic breast cancer patients with hormone receptor expression. Front Immunol. (2021) 12:610149. doi: 10.3389/fimmu.2021.610149
98. Lemery S, Keegan P, Pazdur R. First FDA approval agnostic of cancer site - when a biomarker defines the indication. N Engl J Med. (2017) 377:1409–12. doi: 10.1056/NEJMp1709968
99. Zhang L, Lin W, Tan F, Li N, Xue Q, Gao S, et al. Sintilimab for the treatment of non-small cell lung cancer. biomark Res. (2022) 10:23. doi: 10.1186/s40364-022-00363-7
100. Zhang L, Mai W, Jiang W, Geng Q. Sintilimab: A promising anti-tumor PD-1 antibody. Front Oncol. (2020) 10:594558. doi: 10.3389/fonc.2020.594558
101. Nong J, Gu Y, Yao S, Zhang Y. Durable response to immunotherapy plus chemotherapy in a patient with untreated, brain-metastatic, EGFR exon 20 insertion mutation lung adenocarcinoma: A case report. Med (Baltimore). (2021) 100:e26650. doi: 10.1097/MD.0000000000026650
102. Lee A, Keam SJ. Tislelizumab: first approval. Drugs. (2020) 80:617–24. doi: 10.1007/s40265-020-01286-z
103. Fu Y, Zheng Y, Wang PP, Chen YY, Ding ZY. Immunotherapy for a POLE mutation advanced non-small-cell lung cancer patient. Front Pharmacol. (2022) 13:817265. doi: 10.3389/fphar.2022.817265
104. Chen Q, Zheng K, Xu M, Yan N, Hai G, Yu X. Anlotinib combined with radiotherapy and chemotherapy for recurrent pelvic osteosarcoma treatment: a case report and literature review. Front Oncol. (2023) 13:1283932. doi: 10.3389/fonc.2023.1283932
105. Zhao D, Zhu D, Cai F, Jiang M, Liu X, Li T, et al. Current situation and prospect of adoptive cellular immunotherapy for Malignancies. Technol Cancer Res Treat. (2023) 22:15330338231204198. doi: 10.1177/15330338231204198
106. Liu Q, Li J, Zheng H, Yang S, Hua Y, Huang N, et al. Adoptive cellular immunotherapy for solid neoplasms beyond CAR-T. Mol Cancer. (2023) 22:28. doi: 10.1186/s12943-023-01735-9
107. Biederstädt A, Rezvani K. How I treat high-risk acute myeloid leukemia using preemptive adoptive cellular immunotherapy. Blood. (2023) 141:22–38. doi: 10.1182/blood.2021012411
108. Zhu Y, Qian Y, Li Z, Li Y, Li B. Neoantigen-reactive T cell: An emerging role in adoptive cellular immunotherapy. MedComm. (2021) 2:207–20. doi: 10.1002/mco2.41
109. Etxebeste-Mitxeltorena M, Del Rincón-Loza I, Martín-Antonio B. Tumor secretome to adoptive cellular immunotherapy: reduce me before I make you my partner. Front Immunol. (2021) 12:717850. doi: 10.3389/fimmu.2021.717850
110. Taborska P, Stakheev D, Bartunkova J, Smrz D. Thapsigargin-stimulated LAD2 human mast cell line is a potent cellular adjuvant for the maturation of monocyte-derived dendritic cells for adoptive cellular immunotherapy. Int J Mol Sci. (2021) 22(8). doi: 10.3390/ijms22083978
111. Chen H, Fan Y, Hao X, Yang C, Peng , Guo R, et al. Adoptive cellular immunotherapy of tumors via effective CpG delivery to dendritic cells using dendrimer-entrapped gold nanoparticles as a gene vector. J Mater Chem B. (2020) 8:5052–63. doi: 10.1039/D0TB00678E
112. Guo L, Ding J, Zhou W. Converting bacteria into autologous tumor vaccine via surface biomineralization of calcium carbonate for enhanced immunotherapy. Acta Pharm Sin B Dec. (2023) 13:5074–90. doi: 10.1016/j.apsb.2023.08.028
113. Ma G, Li F, Wang X, Li Q, Hong Y, Wei Q, et al. A bionic yeast tumor vaccine using the co-loading strategy to prevent post-operative tumor recurrence. ACS Nano. (2023) 17:21394–410. doi: 10.1021/acsnano.3c06115
114. Wang X, Zhang Y, Chung Y, Tu C, Zhang W, Mu X, et al. Tumor vaccine based on extracellular vesicles derived from γδ-T cells exerts dual antitumor activities. J Extracell Vesicles. (2023) 12:e12360. doi: 10.1002/jev2.12360
115. Zeng F, Fan Z, Li S, Li L, Sun T, Qiu Y, et al. Tumor microenvironment activated photoacoustic-fluorescence bimodal nanoprobe for precise chemo-immunotherapy and immune response tracing of glioblastoma. ACS Nano. (2023) 17:19753–66. doi: 10.1021/acsnano.3c03378
116. Tobin JWD, Bednarska K, Campbell A, Keane C. PD-1 and LAG-3 checkpoint blockade: potential avenues for therapy in B-cell lymphoma. Cells. (2021) 10(5). doi: 10.3390/cells10051152
117. Chen M, Bie L, Ying J. Cancer cell-intrinsic PD-1: Its role in Malignant progression and immunotherapy. BioMed Pharmacother. (2023) 167:115514. doi: 10.1016/j.biopha.2023.115514
118. Filippone A, Lanza M, Mannino D, Raciti G, Colarossi C, Sciacca D, et al. PD1/PD-L1 immune checkpoint as a potential target for preventing brain tumor progression. Cancer Immunol Immunother. (2022) 71:2067–75. doi: 10.1007/s00262-021-03130-z
119. Zhao J, Roberts A, Wang Z, Savage J, Ji RR. Emerging role of PD-1 in the central nervous system and brain diseases. Neurosci bulletin. (2021) 37:1188–202. doi: 10.1007/s12264-021-00683-y
120. Kreidieh FY, Tawbi HA. The introduction of LAG-3 checkpoint blockade in melanoma: immunotherapy landscape beyond PD-1 and CTLA-4 inhibition. Ther Adv Med Oncol. (2023) 15:17588359231186027. doi: 10.1177/17588359231186027
121. Khasraw M, Reardon DA, Weller M, Sampson JH. PD-1 Inhibitors: Do they have a Future in the Treatment of Glioblastoma? Clin Cancer Res. (2020) 26:5287–96. doi: 10.1158/1078-0432.CCR-20-1135
122. Villacampa G, Cresta Morgado P, Navarro V, Viaplana C, Dienstmann R. Comprehensive evaluation of surrogate endpoints to predict overall survival in trials with PD1/PD-L1 immune checkpoint inhibitors plus chemotherapy. Cancer Treat Rev. (2023) 116:102542. doi: 10.1016/j.ctrv.2023.102542
123. Hao Y, Cook MC. Inborn errors of immunity and their phenocopies: CTLA4 and PD-1. Front Immunol. (2021) 12:806043. doi: 10.3389/fimmu.2021.806043
124. Chen RY, Zhu Y, Shen YY, Xu QY, Tang HY, Cui NX, et al. The role of PD-1 signaling in health and immune-related diseases. Front Immunol. (2023) 14:1163633. doi: 10.3389/fimmu.2023.1163633
Keywords: metastatic brain tumors, immunotherapy, immune checkpoint inhibitors, BBB, cancer
Citation: Li T, Sun S, Li Y, Zhang Y and Wei L (2024) Immunotherapy revolutionizing brain metastatic cancer treatment: personalized strategies for transformative outcomes. Front. Immunol. 15:1418580. doi: 10.3389/fimmu.2024.1418580
Received: 16 April 2024; Accepted: 10 July 2024;
Published: 29 July 2024.
Edited by:
Elena Voronov, Ben-Gurion University of the Negev, IsraelReviewed by:
Zhijin Fan, Sun Yat-sen University, ChinaJiaji Yue, Shenzhen Second People’s Hospital, China
Copyright © 2024 Li, Sun, Li, Zhang and Wei. This is an open-access article distributed under the terms of the Creative Commons Attribution License (CC BY). The use, distribution or reproduction in other forums is permitted, provided the original author(s) and the copyright owner(s) are credited and that the original publication in this journal is cited, in accordance with accepted academic practice. No use, distribution or reproduction is permitted which does not comply with these terms.
*Correspondence: Linlin Wei, d2VpbGlubGluX2xuc3psQDEyNi5jb20=