- 1Postgraduate Program in Parasitic Biology in the Amazon (PPGBPA), University of Pará State (UEPA), Belém, Brazil
- 2Department of Biomedicine, Federal University of Ceará (UFC), Fortaleza, Brazil
- 3Bacteriology and Mycology Section (SABMI), Evandro Chagas Institute (IEC), Ananindeua, Brazil
- 4Department of Pathology and Legal Medicine, Faculty of Medicine, Federal University of Ceará (UFC), Fortaleza, Brazil
Introduction: Leprosy is a chronic infectious condition and the main cause of neuropathy that occurs brought on by M. leprae. It is known that the biological characteristics of the human host, such as the immunological ones, have a higher influence on the pathology of this disease than the intrinsic mechanisms of the bacterium. The objective of this work was to review the scientific knowledge about the relationship between immunopathology and the severity of leprosy.
Methods: A systematic review following the PRISMA 2020 recommendations was conducted in the PUBMED, LILACS, SciELO and Science Direct databases using articles in English, Portuguese or Spanish between January 2011 and May 2022 with the descriptors “Leprosy/Immunology”, “Cytokines” and “Mycobacterium leprae”. A methodological quality assessment was carried out using the JBI checklists.
Results: A total of 49 articles were included. There is a relationship of greater severity of infection associated with lower release of MHC molecules in response to PGL-1 that inhibit the promotion of resolving T lymphocytes arising from dendritic cells (DCs) stimulation. In addition, the differentiation of macrophage phenotypes dependent on the activation of PRRs can define activation and the distinct type of T helper (Th) cells involved according to severity. Activated CD8+ T cells also have distinct types at the appropriate poles of the disease, and B cells show at the most severe pole of the LL, specific induction of IgA and more Treg-type CD8+ T cells that further contribute to T cell anergy.
Conclusion: Therefore, the adaptive immune system aggravates nerve damage and defines the type of leprosy, while the innate immune system is considerably more significant in the onset of nerve damage, symptomatic of the initial presentation of illness and in several critical immune responses, including inflammation and elimination of dead M. leprae.
Introduction
Leprosy or Hansen’s Disease (HD) is a chronic infectious condition and the main cause of infectious neuropathy caused by the obligate intracellular organisms Mycobacterium leprae (M. leprae) and M. lepromatosis. This mycobacteriosis is characterized by high infectivity and minimal pathogenicity (1). The epidermis and peripheral nerves are the primary targets of this micobacteria. Damage to the peripheral nervous system can result in sensory and motor loss, as well as hand and foot abnormalities (2).
The most common cause of leprosy in humans is Mycobacterium leprae. It affects people all over the world and, in 2019, over 200,000 new instances from more than 100 countries were reported, although around the globe, Brazil, India, and Indonesia account for roughly 80% of all newly reported cases (3). High endemicity regions can be observed inside nations with lower levels of development (4).
Physical impairment can be developed before leprosy diagnosis, throughout therapy, and after discharge from treatment (5). Leprosy-related disabilities affect about 15% of people worldwide (6). Disability and nerve thickening was already linked in leprosy (7). The peripheral nerves’ Schwann cells, which are crucial for transmitting nerve impulses, and dermal histiocytes (tissue macrophages) are the two main cell types that this bacterium primarily invades. In the form of erythematous infiltration, skin lesions manifest as whitish papules or rashes (8).
A wide range of clinical symptoms are brought on by delayed diagnosis and inadequate leprosy treatments. Due to its morbidity and socioeconomic effect, which are both results of sequelae (such as physical impairment and deformities) that arise during the clinical course of the illness, leprosy is regarded as a significant public health issue (9). Regarding the clinical, bacilloscopic, histological and immunological presentation, the Ridley-Jopling classification of leprosy has been divided into five types related to the order of increasing severity: tuberculoid (TT), borderline tuberculoid (BT), mid-borderline (BB), borderline lepromatous (BL), and lepromatous (LL) (10). In this sense, the clinical spectrum of the disease can be summarized in a variation of severity between the Tuberculoid and Lepromatous poles, being separated by Borderline forms in the middle (11).
People who have weak cell immune responses against the bacillus also have strong humoral immune reactions and large levels of serum antibodies, which makes it difficult for them to control the spread of M. leprae. While LL is characterized by robust humoral immunity, TT is linked with strong cellular immunity, poor humoral immunity, and granulomatous local skin lesions (12).
Individual differences in the clinical manifestation of the illness are mostly caused by unique host characteristics rather than genetic variants of the bacterium (13). So, this study sought to review the correlation between the immunological characteristics of the human host and the distinct clinical presentations of leprosy based on its severity poles (with no focus on leprosy reactions, such as reverse reaction - RR and type 2 leprosy reaction, also known as erythema nodosum leprosum – ENL forms, and the Lucio’s phenomenon – LP), caused by M. leprae. The aim of this article was to write an updated review on the immunopathogenesis of leprosy.
Material and methods
Study design
This work is a systematic review conducted in accordance with the Preferred Reporting Items for Systematic Reviews and Meta-Analyses (PRISMA) 2020 statement (14). To create the guiding question, the PICO strategy was used with the following anagrams: population, intervention, comparison, and outcome (15). In this context, it was developed from Population: patients with leprosy; Intervention: to assess immunopathogenic features in Mycobacterium leprae-infected patients; Comparison: mycobacteria’s immunopathogenic properties to human immunity; Outcome: the disease’s progression. So, the following question was formed: “Which immunopathological aspects of the host are associated with the types of clinical forms of leprosy?”.
Search strategy
The descriptors ((“Leprosy/immunology”[Mesh]) AND “Cytokines”[Mesh]) AND “ Mycobacterium leprae “[Mesh] were combined to identify and select relevant articles from the databases Science Direct, the National Library of Medicine National Institutes of Health of the USA (PUBMED), Latin American and Caribbean Literature in Health Sciences (LILACS) and Scientific Electronic Library Online (SciELO). The period included the period from January 2011 to May 2022. The information was gathered on July 20, 2022. The study only considered English, Portuguese, and Spanish as languages. Study titles and abstracts were reviewed, and those that addressed this topic were given greater consideration for analysis. All of those published before 2011 were excluded, as well as those that were duplicated, only available as an abstract, letters to the editor, and papers with inaccessible key material.
Extraction of data and quality methodological assessment
All relevant data, including any discrepancies and ambiguities discovered in the articles, were separately gathered, extracted, and reviewed for quality by two authors (MJAS and CSS), with the assistance of a third author (TPB) in situations where the selection of the data was discordant. The data extracted from the articles were: author and year of publication; data base; kind of study; goals; results. The data found were organized in Microsoft Office Excel 365 and arranged in tabular form.
The quality assessment was done by completing the Joanna Briggs Institute (JBI) Appraisal Checklists for the kind of studies included, such as: Checklist for Cohort studies (ranging from 0 to 11); Checklist for Systematic reviews and research syntheses (ranging from 0 to 11); Checklist for Quasi-experimental studies (ranging from 0 to 9) (16). The scores for answering the checklist questions were considered only when the conditioned answer was “Yes” (17).
Results
Literature search
A total of 130 studies were found in an initial search in the analyzed databases, being collected for the selection phase. During the selection, 5 incomplete or duplicated studies between the databases and 40 researches not relevant to the theme considering the reading of the abstract and title were excluded. So, 85 studies selected for reading in full were verified if they concerned the subject and then, 36 of them were excluded from the analysis due to eligibility. In the end, 49 studies were included for qualitative synthesis (Figure 1).
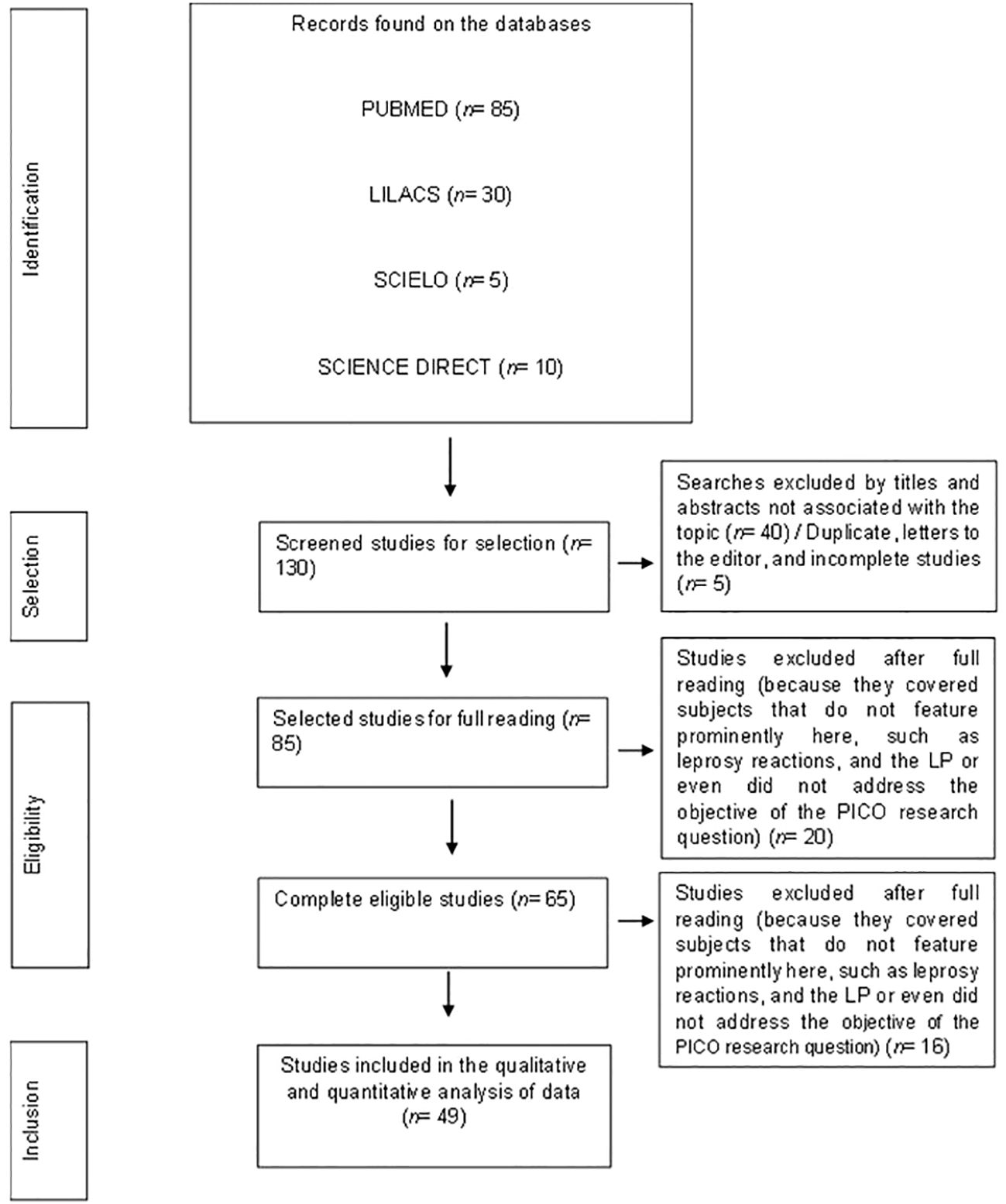
Figure 1. PRISMA flowchart of the steps related to the inclusion of articles in this systematic review.
Presentation of the data included in this review
The final framework consists of 49 papers. The articles included in this review were mostly international (n= 32; 65%), in the English language in its entirety (n= 49; 100%), coming from PUBMED (n= 49; 100%) and referring to the type of cohort study (n= 25; 51%), experimental (n= 16; 33%) and review (n= 8; 16%). The methodological quality assessment score demonstrated low bias in these data. These extracted data were arranged in tabular form below (Table 1).
Didactic illustrative schemes of the influence of the immune response against infection by Mycobacterium leprae with characterization based on the poles of severity of leprosy
Figure 2 demonstrates the immune responses induced by the infection of this pathogen. The chronological order of the steps is described by letters from A-B in parentheses.
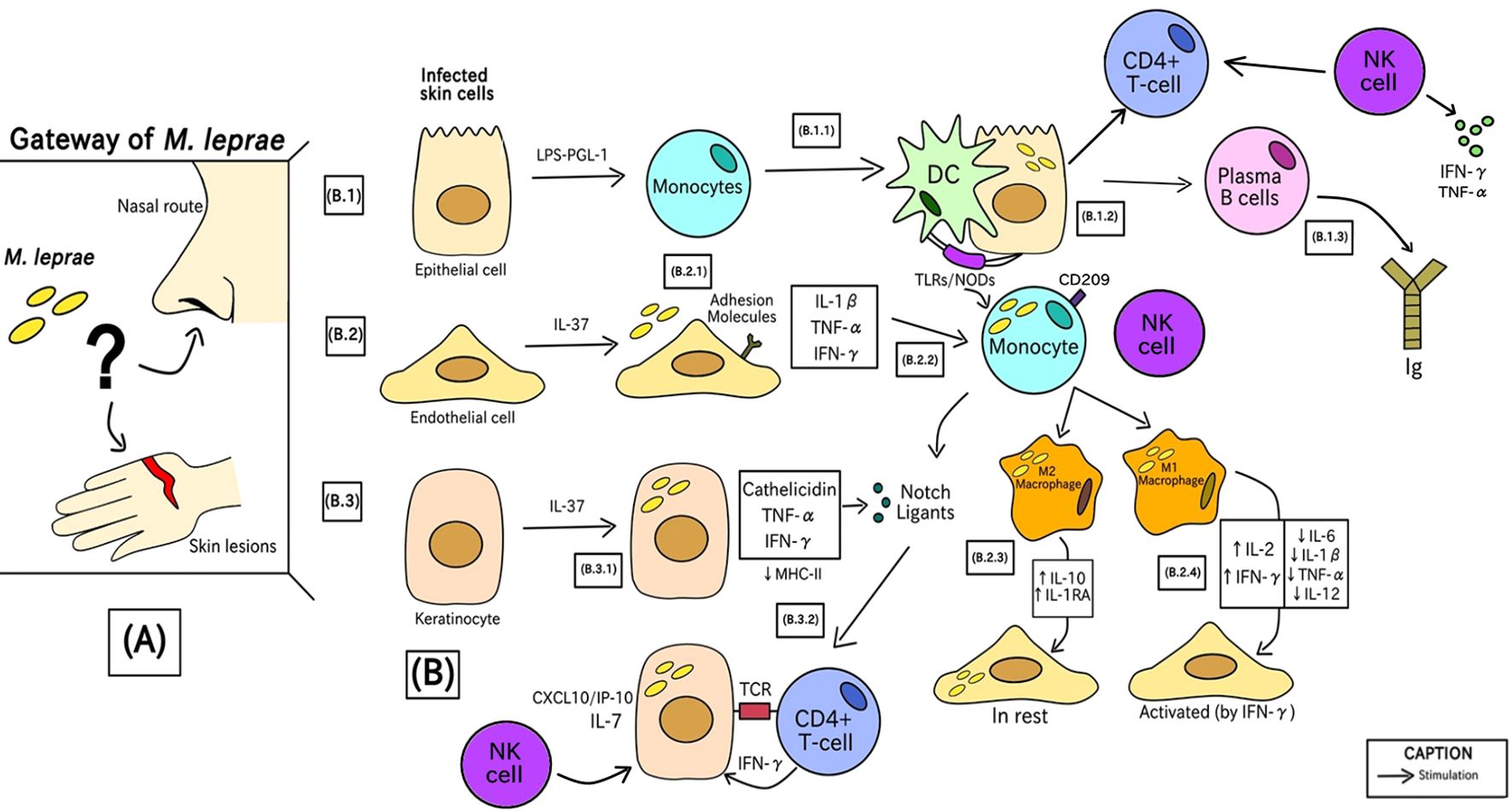
Figure 2. Phases of the generation of the human immune response against M. leprae since bacterial entry into the initial site of infection.
In the first stage (A), bacterial entry into humans occurs through a still uncertain transmission route. The hypotheses behind this process involve the route of nasal epithelial cells or through keratinocytes and poorly differentiated endothelial cells due to minor injuries. Recently, the nasal route is the most accepted form of entry by the scientific community (1, 68, 69).
In (B) there is the characterization of cells infected in the first instance by Mycobacterium leprae. These cells are epithelial (B.1), endothelial (B.2), and keratinocytes (B.3) cells. These three cell types are the initial line of defense against M. leprae, and mammalian cell entry protein 1A (Mce1A) play a role in the cells’ ability to attract other cells (70–72).
(B.1.1) Epithelial cells, in contact with bacterial liposaccharide (LPS) and PGL-1 antigen, induce inflammatory mediators that can instruct monocytes to differentiate into mucosal dendritic cells (DCs) (73). (B.1.2) Through Pattern Recognition Receptors (PRRs), such as TLRs and NODs, DCs attach to the infected cell, process PAMPs from the bacteria and call for more monocytes (which can differentiate into other immune cells, such as macrophages, for example) (74, 75). (B.1.3) Next, there is the recruitment of B lymphocytes, responsible for the production and class switching of Immunoglobulins (Ig), acting as humoral immunity against infection, in addition to there being a Th1 response with cell-mediated immunity (CMI). Besides that, NK cells can be activated by cytokines produced during the initial immune response, such as IFN-γ, which is crucial in the defense against M. leprae, increasing antigen presentation of DC cells and T lymphocyte activation, strengthening the response against infection (57, 64).
(B.2.1) Endothelial cells stimulated by IL-37 expression through the secretion of inflammatory mediators, including IL-1β, TNF-α, and IFN-γ, which positively induce adhesion molecules like E-selectin, ICAM-1, VCAM- 1, and VLA-4 that allow immune cells to go to the infection site. This overexpression of molecules was not present in LL patients, suggesting that M. leprae influences this upregulation (55, 65). (B.2.2) Monocytes with high expression of CD209 are recruited and this cell signaling process also stimulates the induction of molecules called Notch Ligands (57). (B.2.3) Resting endothelial cells urge monocytes to differentiate into anti-inflammatory M2 macrophages, with elevated levels of IL-10 and IL-1RA, which do not generate an antimicrobial response (76). (B.2.4) IFN-γ–activated endothelium cells direct monocytes to develop into pro-inflammatory M1 macrophages, showing high levels of IL-2 and IFN-γ and lower concentrations of IL-6, IL-1β, IL-12 and TNF-α, which can limit bacterial growth. Patients with tuberculoid leprosy have active endothelial cells at the site of infection, which prompt M1 macrophages to provide an efficient antimicrobial response (47). After activation, NK cells release cytokines, such as TNF-α and IFN-γ, which not only promote the death of infected cells, but also stimulate other cells of the immune system, such as macrophages (77).
(B.3.1) Keratinocytes through the antimicrobial activity of IL-37 release MHC class II molecules to present M. leprae antigens by the release of cathelicidin, TNF-α and IFN-γ which stimulates the cellular immune signaling of Notch ligands (62). (B.3.2) Only lesions of patients with tuberculoid leprosy show these IFN-γ–induced keratinocytes (54). The interaction of the NK cell with the infected cell can also further increase the defense response with the activation of CD4+ T lymphocytes (78).
Figure 3 is the visual identification of the summarized findings of this systematic review in relation to the association between observed immunopathological and the clinical forms of leprosy represented from left going to right like characteristics (according to the clinical spectrum of leprosy described by Ridley and Jopling classification). The chronological order of the steps is described by letters from A-E in parentheses.
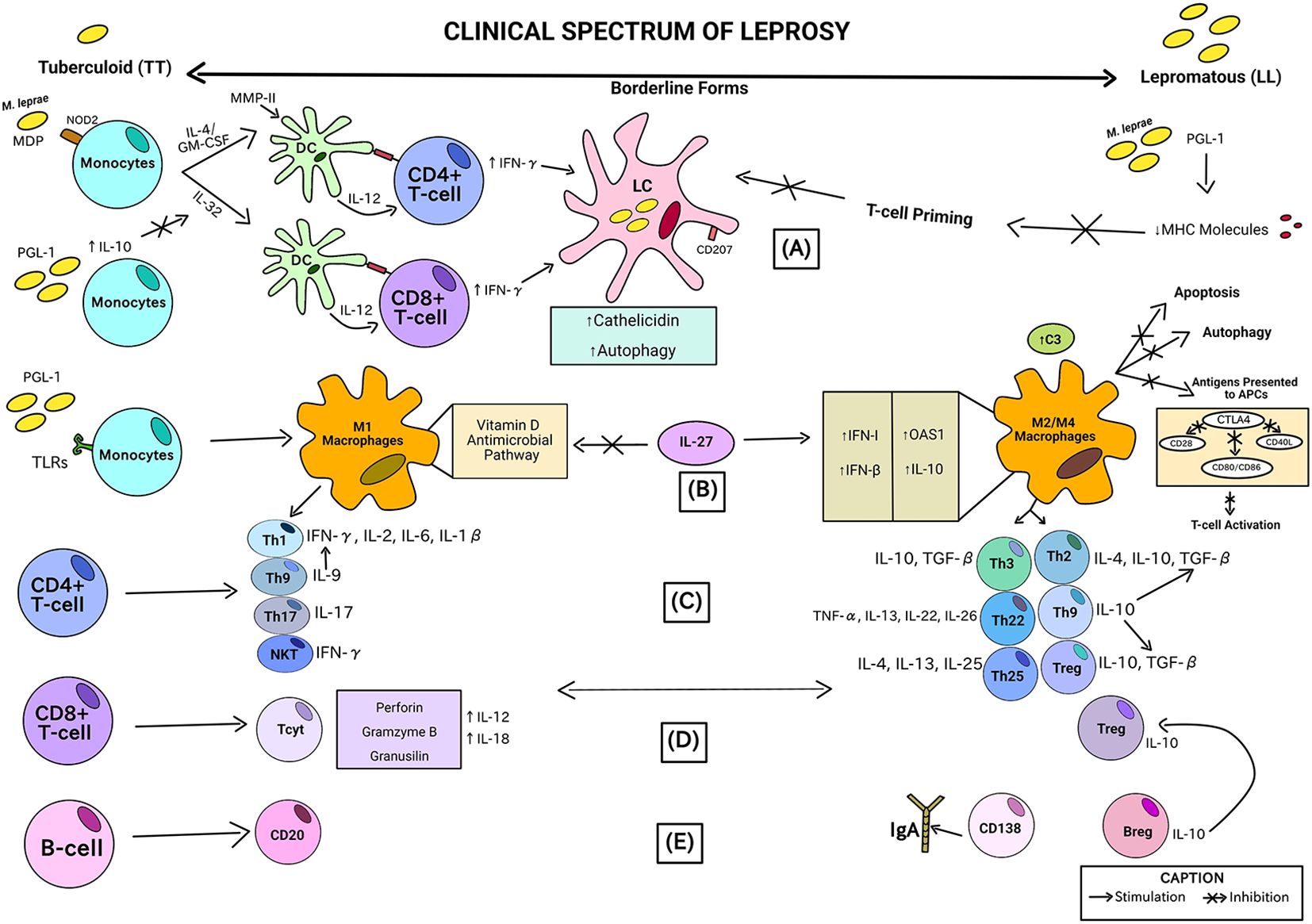
Figure 3. Association between immunopathological characteristics observed in the analyzes of the studies included in this review and the clinical forms of leprosy severity. This picture consists of a theoretical proposal for leprosy, based on individual data extracted from the studies included in this review, but which have not yet been proven in a joint and methodologically organized manner. No original study has been performed in clinically classified leprosy cases to prove such a clear differentiation using immunological parameters.
In the initial stage (A), the innate immune response through monocytes can develop into dendritic cells (DCs) that act like APCs by a mechanism independent of IL-4/GM-CSF and IL-32. Muramyl dipeptides (MDP) from M. leprae are recognized by NOD2 and cause an increase in these pathways in monocytes. In the presence of IL-10 (induced by M. leprae PGL-1), the IL-32 pathway can be inhibited. Another M. leprae cell-wall antigen, MMP-II, activates CD4+ and CD8+ T lymphocytes via DC cells, stimulating the recruitment of adaptive immune cells through IL-12 induction, and producing IFN-γ. In lepromatous patients, the IL-12 production in response to M. leprae is poor. CD4+ T-cells and CD8+ T-cells receive antigens from DCs via MHC class II and class I, respectively. Less T-cell priming results from M. leprae/PGL-1 downregulating the production of MHC molecules. The skin or mucosa’s Langerhans cells (LCs) are the only cells that express langerin (CD207). When Langerhans cells infected with M. leprae are treated with IFN-γ, autophagy is induced and cathelicidin synthesis is increased. Cathelicidin is needed to activate the antimicrobial activity in these cells. M. leprae can be broken down and released, releasing antigens that can be presented to local T lymphocytes thanks to the enhanced autophagy in LCs (23, 31, 43).
In (B), monocytes exposed to M. leprae/PGL-1 in interaction with TLRs results in an increase in CXCL10/IP-10 and CCL2/MCP-1 production in M1 macrophages and a decrease in IL-10 and IL-1RA (M2/M4 macrophages) in Tuberculoid patients. Type I interferon genes, including IFN-β and OAS1, and IL-10, which are increased by IL-27, disrupt the vitamin D-dependent pathway, which is necessary for bacterial death in M1 macrophages. In lepromatous leprosy lesions, all these genes exhibit high levels of expression, which is consistent with the M2 macrophage phenotype, characterized by the high production of C3 (an element of the complement system). Antigens are not as readily available for T-cell antigen presentation when IL-10 levels are high because these processes are suppressed, along with autophagy and apoptosis. This lower availability of antigens to APCs is caused by the high release of the CTLA4 molecule, capable of inhibiting T cell receptors, such as CD28, CD40 ligand (CD40L) and CD86/CD80, generating less activation of T lymphocytes (52, 61).
In (C), activated CD4+ T cells can further differentiate by means of the type of phenotype of the recruited macrophage (M1 or M2/M4) into distinct T helper subpopulations according to the severity of the leprosy clinical form. In tuberculoid patients (TT), there is induction by M1 macrophages of Natural Killer T cell subpopulations - NKT (with high production of IFN-γ), Th17 (with high levels of IL-17), Th9 (with high production of IL-9) related to the release of IFN-γ by Th1 cells (subpopulation that produces IFN-γ, IL-2, IL-6, and IL-1β). On the other hand, in lepromatous patients (LL), there is secretion of subpopulations of T lymphocytes arising from the M2/M4 phenotype of macrophages referring to Th3 (with production of IL-10 and TGF-β), Th22 (elevated levels of TNF-α, IL-13, IL-22 and IL-26), Th25 (releasing IL-4, IL-13 and IL-25), Th2 (secreting IL-4, IL-10 and TGF-β), Treg (secreting IL-10 and TGF-β) and also Th9, but here it is related to the release of IL-10, a cytokine that is capable of inducing more TGF-β from both Th2 and Treg responses (18, 29, 49, 79, 80).
In (D), activated CD8+ T cells can differentiate into distinct subpopulations according to the severity of the leprosy clinical form. CD8+ cytotoxic T cells (Tcyt) are considered effectors and typical of patients with the TT form, as they produce potentially defensive proteins, such as perforins, gramzymes B and granusilins through IL-12 and IL-18 pathways. As regards the LL pole, there is an abundance of Treg-type CD8+ T cells, contributing to the T-cell anergy (81).
In the stage (E), in terms of B-cell differentiation, lepromatous individuals had more CD138+ cells, which is responsible for secreting high levels of IgA, although at the other end of the range, CD20+, a B-cell marker typically lacking on the terminally differentiated plasma cells, was more commonly seen. Patients with LL leprosy and, to a lesser extent, those with tuberculoid infection have been found to contain regulatory B-cells (Bregs) that produce IL-10. Bregs promote T effector cells to change into regulatory T cells concurrently as these cells expressed more FoxP3 and Programmed Cell Death Protein 1 (PD-1) (64, 82).
Didactic illustrative scheme of the immunopathogenesis of leprosy
Leprosy is characterized by significant nerve involvement, with perineural inflammation being a hallmark of the disease. This inflammation may facilitate the entry of M. leprae into peripheral nerves via a vascular route. Once inside, the bacteria are ingested by Schwann cells, leading to various detrimental effects, including early axonal atrophy and eventual segmental demyelination of the affected nerves (83).
In Figure 4, there is the characterization of the histological damage in human nerves caused by the negative immune response in patients with leprosy, particularly related to the most severe clinical conditions of leprosy. The chronological order of the steps is described by letters from A-C in parentheses.
In the initial phase (A), PGL-1 specific to M. leprae binds to laminin-2 on Schwann cells and is necessary for its internalization in Schwann cells where it causes the upregulation of mannose receptors (MRs), such as CD206, via peroxisome proliferator-activated receptor gamma (PPAR-γ). Simultaneously, the interaction between the glycolipid lipoarabinomannan (LAM), one of the components of the most external part of the mycobacteria, which acts like a kind of PAMP and activates the Schwann cell by the formation of opsonin C3 and Membrane Attack Complex – MAC, and the cellular receptor Dectin-2, responsible for β-glucan recognition implicated in the phagocytic process. To survive in Schwann cells, M. leprae induces the production of IFN-I-related genes, lipid synthesis, and regulates glucose absorption, whereas it downregulates oxidative stress apoptosis, autophagy, and the expression of antimicrobial peptides (AMPs). Through shrinking the expression of genes necessary for myelination, such as Krox-20, myelin basic protein (MBP), and myelin protein zero (MPZ), it results in dedifferentiated Schwann cells (20).
In (B), Dedifferentiated Schwann cells generate matrix metalloproteinases (MMPs), CXCL10/IP-10, and CCL2/MCP-1 (which are chemoattractants of macrophages and CD4+ T cells) and are very prone to invasion. Nitric oxide (NO) is produced by macrophages in response to M. leprae PGL-1 via CXCL10/IP-10 and CCL2/MCP-1 (76).
The final stage (C) is characterized by the subsequent mitochondrial damage in axons that initiates nerve demyelination and can cause cell death. Along with the activities of macrophages and NO, antigen presentation via CD4+ T-cells results in the death of infected Schwann cells. These factors contribute to the nerve damage and subsequent pathological clinical conditions in leprosy (84).
Innate immunity in leprosy
It is known that the M. leprae bacillus resides in macrophages, dendritic cells and Schwann cells and penetrates the cells through the action of a component of the bacterial cell wall, called glycolipid-phenolic-1 (PGL-1), which binds to that of complement C3 through complement receptors CR1, CR3 and CR4 (13, 18). Doz-Deblauwe et al. (2019) identified in mice infected with M. leprae that the binding of PGL-1 to CR3 increased bacterial phagocytosis by polymorphonuclear neutrophils (PMNs) and in lung macrophages, in addition to increasing bacterial invasion in dendritic cells (DCs), increasing the selective production of IL-2 by these cells and IL-10 by macrophages. Study data indicate that by targeting CR3, PGL-1 triggers the Syk/calcineurin/NFATc pathway that rewires the innate immune response in three key innate cells. rBCG: PGL-1 allows effective uptake by PMNs and lung macrophages if CR3 is present and Syk is active. Lung cells infected by rBCG: PGL-1 produce high levels of IL-10 when CR3 was present (20).
The presence of NK cells is indicative of a robust immune response, which may help limit the spread of the bacillus. In cases of lepromatous leprosy, where there is a depression of the cellular immune response, NK cell activity may be insufficient to control the infection, resulting in more severe manifestations of the disease (85).
Furthermore, studies identified that in the MB form of leprosy, high levels of antibodies and IFN-y directed by PGL-1 were present, showing the efficiency of the immune system in identifying this antigen (51, 62). The results showed that IFN-γ induces the autophagy-dependent antimicrobial activity of Langerhans Cells - LC against cutaneous pathogens, such as S. aureus, S. pyogenes and C. albicans. Autophagy in leprosy contributes to an antimicrobial response in CL, which allows DC to process pathogen-derived antigens to T cells and direct the adaptive response of T cells (23).
Monocytes that differentiate into DC in the presence of PGL-1 and bacterial LPS demonstrate immunosuppression with respect to the production of inflammatory cytokines and immune regulatory molecules. Considering that DCs can produce the PGL-1 antigen, masking it enhanced the DC-mediated T cell response, demonstrating that PGL-1 presentation dampens the T cell response. Activation of DCs can be detected by increased expression of MHC class I and II molecules, CD86 and CD83 when DCs are stimulated by pure M. leprae matrix metalloproteinase (MMP)-II (through TLR signaling). These MMP-II pulsed DCs trigger T cell IL-12 and IFN-γ production, resulting in an efficient Th1 response (73). High amounts of IL-10 prevent DC development via NOD2/IL-32 in lepromatous patients’ monocytes, but recombinant IL-32 added in vitro restored DC differentiation in these individuals.
IL-32-induced DCs are crucial to the host’s defense against M. leprae (74).The activity of macrophages involves the production of oxygen species and reactive nitrogen, through the complex NADPH-oxidase and nitric oxide (NO) (75). Oldenburg et al. (2018) demonstrated a limited ability of macrophages to induce nitric oxide synthase and generate NO to fight bacterial infection (58).
Toll-like receptors (TLRs) are present on macrophages, DCs and Langerhans cells, and mainly TLR1/2, TLR2, TLR4 and TLR6 are involved in the recognition of M. leprae PAMPs (57). The TLR1/TLR2 heterodimers are known to be responsible for recognizing mycobacterial lipoproteins and activating the pro-inflammatory response. Krutzik et al. (2003) identified that TLR1 and TLR2 are expressed in greater amounts in individuals with tuberculoid leprosy than in patients with lepromatous leprosy (86).
Polycarpou et al. (2016) found that this bacillus has a ligand that activates TLR4 leading to signal transduction and is present in human macrophages, the preferred environment for M. leprae. Furthermore, it was identified that the use of a neutralizing monoclonal antibody against TLR4 influenced the decrease in the production of pro-inflammatory cytokines, such as TNF-α, CXCL10/IP-10 and IL-6. The CD16, a signaling marker for immune cell recruitment, acts by mediating the TLR4 response. Furthermore, it has been shown that PGL affects the TLR4 signaling pathway, increasing the exposure of macrophages to PGL and leading to a reduction in the levels of the interferon-β inducing protein (TRIF), containing the TIR domain, resulting in a low production of INF-β and CXCL10/IP-10 (47).
Monocytes in the presence of galectin-3 have a reduced antigen presentation, indicating an influence of the lectin through glucose uptake on the low immune response in leprosy patients (33). GM-CSF-derived APCs stimulate CD1B-restricted T cells to proliferate in response to antigen. Macrophages are generally classified into M1 and M2, according to the type of Th response (Th1 or Th2), and M2 macrophages are directly associated with the Th2 response present in LL leprosy (52). M2 macrophages from patients who have the disease in a disseminated form present co-stimulation of the expression of molecules such as: CD68 and CD163, in addition to the anti-inflammatory cytokines IL-10 and TGF-β (31). Other types of macrophages were also disregarded, such as the M4 phenotype, which is more highly expressed in lepromatous leprosy patients and may indicate that this subpopulation is less successful in eradicating the bacillus, favoring disseminated presentations (87).
The bacterial death in M1 macrophages depends on the vitamin D-dependent pathway. In macrophages infected with M. leprae, this response is innately active, but it is inhibited by M. leprae ‘s production of type I IFN. CYP27B1, which transforms the inactive prohormone substrate 25-hydroxyvitamin D (25D) into the active vitamin D hormone 1,25-dihydroxyvitamin D, is necessary for the antimicrobial response, was blocked by the generation of IFN-β and OAS1 in infected monocytes/macrophages in vitro (67, 76, 88).
The M2 phenotype is advantageous for M. leprae to survive in macrophages. This is consistent with the relationship between the in vitro distorting of macrophages infected with M. leprae toward the M2 phenotype and the suppression of autophagy and apoptosis. This inhibition of macrophages can stop T cells from receiving an effective antigen presentation. Uninfected macrophages were able to deliver antigen by consuming apoptotic cells, while infected macrophages were not effectively engulfed (89). The negative feedback loop that caused the suppression of autophagy is as follows: Initially, live M. leprae increased macrophage autophagy, which resulted in decreased pro-inflammatory cytokine expression. These macrophages suppressed further activation of autophagy by selectively priming anti-inflammatory T cells that produced high amounts of IL-10 (62, 64).
Leprous neuropathy was shown to have downregulated expression of multiple mitochondrial genes when compared to non-leprous neuropathy in nerve lesions from individuals with peripheral neuropathy (65). Then, MB and PB patients can be distinguished using indicators of mitochondrial dysfunction, which have also been shown in other neurodegenerative illnesses (90).
M. leprae controls the autophagy mechanism of human monocytes to create a safe space inside cells for bacterial replication. The survival of M. leprae is not influenced by the lack of L-tryptophan, and this is due to the activation of the IDO-1 signaling pathway dependent on IL-10 and iron (Fe). M. leprae uses glucose transporter 1 to enhance glucose uptake in infected cells. Live bacilli, during M. leprae infection, prevent the formation of free radicals by activating carbons in the electron transport chain for the formation of lipids (60).
In this sense, in leprosy patients, lipid metabolism is also impacted. High-density lipoprotein (HDL) becomes dysfunctional because of the oxidation of ApoA1, which is a major HDL protein and impairs macrophages’ ability to remove cholesterol (65). The presence of foamy macrophages, which are typical of lepromatous leprosy, actively contributes to M. leprae ‘s improved survival in the host; hence, it is not unexpected that these patients’ lesions tend to have upregulated lipid metabolism-related genes (90). While HDL from healthy people retained DC function, defective HDL in these patients also led to a reduction in the clearance of oxidized lipids, which in turn inhibited the DCs’ ability to deliver antigen to T-cells. Therefore, increased ApoA1 production in response to delayed neuronal damage (91).
Adaptive immunity in leprosy
The recognized antigens can recruit T lymphocytes through CD4+ T Cell Receptors (TCR). In turn, keratinocytes secrete CXCL10/IP-10 and IL-7 because of CD4+ Th-cells producing IFN-γ. There are several categories for which naive T cells can differentiate, such as Th1, Th2, Th3, Th9, Th17, Th22 and Th25, and for this differentiation to occur there is the involvement of Notch. Therefore, it is possible to point out which individuals are vulnerable to producing anergic forms of leprosy due to the Notch ligands demonstrated in APC cells of M. leprae carriers (57). Cytotoxic CD8 T-cells mediated by NK cells activity can directly kill infected cells, among others via the secretion of IFN-γ and TNF-α or the release of cytotoxic granules and their frequency is higher in tuberculoid patients than the lepromatous form. The first illness for which CD8 suppressor T cells cloned from a patient with borderline lepromatous disease were discovered was leprosy. Depending on the function of the CD8 T‐cells they are either associated with PB leprosy (cytotoxic - Tcyt) or MB leprosy (Tregs) (81).
It is known that Th1 and Th2 play an important role in the immune response against mycobacteria. IL-4 levels, produced by Th2, were significantly higher in LL patients than even in healthy subjects and in tuberculoid patients (64). IL-10 is an anti-inflammatory and immunosuppressive cytokine produced by T cells and macrophages, whose overactivation decreases lymphocyte-induced immunity and is present in lesions of MB patients (54).
However, contrary to most of the literature, the work carried out by Kumar et al. (2011), one of the studies included in this review sought to analyze this immunological relationship between Th1 and Th2 and demonstrated that the values of IL-4 and IL-13, cytokines associated with Th2, had their levels unchanged in BL/LL, with no correlation with the bacteriological index. Th3 immune responses (in the absence of Th1 and Th2) allow M. leprae to progress. It deduces that through raising the expression of Cbl-b, a member of mammalian Cbl family proteins (ubiquitin ligases thought to negatively regulate TCR signaling), the overexpression of TGF-β and CTLA4 causes T cell hyporesponsiveness, a significant leprosy characteristic (29).
The Th17 response, responsible for producing the cytokine IL-17 and which has a RORC and STAT3 transcription factor. This type of response plays a protective role in individuals who lack the ability to present Th1-type immune responses (37). Increased production of IL-17 in tuberculoid leprosy was related to greater recruitment of inflammatory cells, increased activation of endothelial cells and help in maintaining the chronic inflammatory process (18).
Other types of T cells have essential roles in the formation of host immunity, such as natural killer T cells (NKT) and regulatory T cells (Tregs). These cells recognize mycobacterial antigens that are presented by CD1, which may reduce IL-4 production and increase IFN-γ production, increasing the potential for bacilli elimination (59). In cases where NKT cells may present a late development, the cells produce a low level of IFN-γ, causing non-differentiation of CD4+ T cells in the Th1 phenotype, helping in the development of the LL form of the disease (57).
The development of the Treg and Th17 lineages is connected by the fact that TGF-β also induces RORγt, a key transcription factor in Th17 cell differentiation. FOXP3 can decrease RORγt activity and promote Treg development in the absence of a second signal from a proinflammatory cytokine. However, FOXP3 activity is suppressed and the Th17 differentiation pathway is triggered when the cell also gets a signal from a pro-inflammation cytokine (for example, IL-6) (45).
Tregs in leprosy are in greater quantity in individuals with the lepromatous type. It is considered that the circulation of Treg cells to the sites of lepromatous lesions is related to the presence of tissue chemokines produced at the site of the lesion. Tregs use PD-1 ligands to block B cell activation directly, which prevents the generation of antibodies, hampers B cell growth, and triggers B cell death. In the same way, the regulation of key genes linked to the phenotype of Treg cells is controlled by a vast protein complex that FOXP3 is one of its components (37).
FoxP3-positive Treg cells act by reducing the activity of effector T cells, such as Th17 cells, and thereby negatively controlling the immune response within the host during intracellular infections. Th17 cells are helper T cells that act by increasing the number of Th1 effector cells, in the recruitment of neutrophils and in the activation of macrophages. Secretion of IL-10 by Treg cells in individuals with the lepromatous form is considerably associated with polarized immunity distinct to small IL-17 by CD4+ T cells of the cluster itself (59).
To survive, Hansen’s bacillus can induce TCRγδ + FoxP3 + immunosuppressive cells, through the control of phosphoantigens. A small proportion of T cells possess the TCRγ/δ complex, whereas most T cells possess the αβ complex. γ/δ TCRs identify phosphoantigens present in mycobacteria without depending on the major histocompatibility complex - MHC. A high amount of γ/δ T cells in the skin and blood has been associated with granulomatous reactions in leprosy people of the LL (54).
NKT cells, which correspond to innate lymphocytes and share surface characteristics with NK cells, are thought to be diminished in leprosy patients. Once triggered by antigen recognition, NKT cells can release a variety of pro-inflammatory mediators quickly. However, NKT cell numbers grew following stimulation with PGL-1 or mannose-capped LAM, particularly in tuberculoid individuals (with more IFN-γ) (79).
Th9 lymphocytes are associated with the pole tuberculoid and arise from Th0 cells, which in the presence of IL-4 and TGF-β differentiate to Th9, producing cytokines such as IL-9, IL-10, and IL-21. The IL-9 cytokine is directly involved with the regulatory activities of cells involved in innate and adaptive immunity. SOUSA et al. (2016) observed the predominance of IL-9 in TT individuals, mainly in lymphocytic granulomas and granulomas composed of macrophages. It has been shown that an increase in IL-10 caused by Th9 activation in tissue lesions of LL patients inhibits macrophage activation and adversely controls the production of IL-12, IFN-γ, and TNF-α. Furthermore, it was verified that IL-9 had the blocking effect of IL-4 and IL-10, being able to positively regulate the expression of Th9 lymphocytes (48).
Th22 cells are recognized for being part of a group of CD4+ T cells that are responsible for the production of isoforms belonging to the Fibroblast Growth Factor (FGF) family and cytokines such as TNF-α, IL-13, IL-22, and IL-26. These cytokines by Th22 subpopulation, such as Th2 cells, are linked to humoral responses and the activation of tissue regeneration processes, leading to a clinically less potent immune response to intracellular infections (80).
Once regards to the Th25 response in leprosy, it appears to be closely linked to the anti-inflammatory pattern in the LL disease mode, i.e., promoting tissue repair or immunosuppression. It is well established that the cytokines IL-4 and IL-13 have a direct role in the Th2 lymphocyte response and M2 macrophage development. Furthermore, IL-4 and IL-13 control the humoral immune response and B cell activation. IL-25/IL-17E is a member of the IL-17 cytokine family and, unlike IL-17A and IL-17F, inhibits the development of both Th1 and Th17 cytokines (92).
Moreover, B-cells is involved in disease etiology in lepromatous patients and related to the inverted gradient between CD138- and CD20-positive phenotype by B cells from the tuberculoid to the lepromatous pole. Patients with lepromatous disease and, to a lesser extent, those with tuberculoid disease have regulatory B-cells (Bregs) in the blood that produce IL-10 (22). Bregs promote T effector cells to change into regulatory T cells while these cells expressed more FoxP3 and PD-1. This B-cell fraction led to the creation of the immunosuppressive IL-10 and may play a significant role in M. leprae maintenance (82).
In addition to chemokines, epithelial cells actively control the local immune response. The discovery of IgA antibodies against entire M. leprae in saliva suggested that mucosal immunity may play a protective role in responses to M. leprae infection. Contacts of untreated leprosy patients, who are often exposed to M. leprae, had greater salivary IgA levels than endemic controls, indicating an active mucosal immune response. Additionally, high salivary IgA levels were seen in household contacts, particularly in those who often interacted with MB patients, in response to LAM or PGL-1 compared to controls. Therefore, it appears that the IgA levels in saliva correspond with the degree of exposure to M. leprae (93).
Discussion
This article presents a comprehensive overview of the immunopathological aspects of leprosy, focusing on the relationship between the immune response and the severity of the disease caused by Mycobacterium leprae. The study employs a systematic review methodology to synthesize findings from various studies published between 2011 and 2022. This review highlights a significant correlation between the severity of leprosy and the immune response.
In order to survive while immunologically hidden within host cells, the bacteria may need to evade immune surveillance systems, allowing long-term parasitization (94). By boosting inducible nitric oxide synthase (iNOS) in the host macrophages, M. leprae ‘s adherence and invasion of Schwann cells encourage brain injury. When the bacterial PGN is recognized, the nucleotide-binding oligomerization domain (NOD)-like receptors (NLRs) start an immunological response (74).
Defense against the M. leprae bacillus is initiated by the host’s innate immune response, followed by the adaptive immune response (41). Innate immune cells act such as APCs, like macrophages and dendritic cells (DCs) can be activated by the functional expression of pattern recognition receptors by epithelial cells, including Toll-like Receptors (TLRs). According to the traditional Th response (Th1/Th2), macrophages may be divided into two categories, M1 and M2. However, the traditional Th1/Th2 antagonistic response pattern associated with the prognosis of Hansen’s disease has recently come under scrutiny due to the wide range of immune response data reported by new studies linked to other T helper subpopulations that differ in the disease’s classic symptomatology (18).
It was reported here that lower release of MHC molecules in response to phenolic glycolipid-1 (PGL-1) is associated with more severe forms of the disease (20, 95). This suggests that a robust immune response, characterized by effective antigen presentation, is crucial for controlling the infection. The differentiation of macrophages into distinct phenotypes plays a critical role in the immune response to leprosy (88, 89). M1 macrophages, associated with the Th1 response, are linked to the tuberculoid form of leprosy, while M2 macrophages, associated with the Th2 response, are more prevalent in lepromatous leprosy (47). This polarization affects the overall immune landscape and the body’s ability to combat the infection.
Activated CD8+ T cells exhibit different profiles depending on the clinical form of leprosy. The review notes that in severe cases, there is a specific induction of regulatory T cells (Tregs), which contribute to T cell anergy, further complicating the immune response (49, 82). The findings emphasize the importance of the innate immune system in the initial presentation of leprosy, while the adaptive immune system is more influential in the progression of nerve damage and the clinical manifestation of the disease (21, 81).
Numerous reviews have already been conducted on the topic of immunology in leprosy (13, 41, 52, 57, 59, 60, 65). This is due to several factors, such as being an ancient disease and with recorded data present in the literature for a long time, the need to associate different complex data and because it is a public health problem. However, it is necessary and important that there are updated reviews on the subject since many new immunological discoveries in leprosy have been made in the scientific community in recent years and are dispersed in the literary environment.
When compared to other literature on the immunological aspects of leprosy, such as the studies by Froes et al. (2022) and Karamova et al. (2020), the findings of this present review align with the understanding that distinct immune responses are critical in determining the clinical outcomes of leprosy (18, 19). For instance, Froes et al. (2022) discuss the roles of various T cell subsets and cytokines in different clinical presentations, reinforcing the notion that the balance between Th1 and Th2 responses is pivotal (18). Additionally, this review’s emphasis on macrophage polarization and its impact on disease severity resonates with findings from other studies, such as those by Dang et al. (2019), which highlights the role of Langerhans cells in antimicrobial responses and antigen presentation (23).
Molecular epidemiology studies with M. leprae in humans and primates are important for identifying genetic profiles and, through this, understanding the origin, transmission pattern and concentration of bacterial strains related to leprosy events. In particular, the geographical restriction of M. leprae strains refers to the specific geographical distribution of the different strains of Mycobacterium leprae around the globe (96, 97).
This geographical restriction consists of a theory under investigation that has not yet been proven, but is under investigation. It is supposed that it can be observed in different regions of the world, influencing the incidence and prevalence of the disease. Multibacillary leprosy (LL form) is more common in areas like Saudi Arabia, while paucibacillary disease (TT form) is more frequent in localities such as Brazil (98–100).
Moreover, evidence suggests that differences in the genome of the M. leprae strain may be able to distinguish between distinct clinical manifestations of leprosy, in addition to the host response, and to cause differential gene expression in human macrophages. According to analysis of the samples in India, TTC repeats are prevalent in Indian LL skin samples, which set them apart from other M. leprae strains found in the country (101). The genotypic difference is related to the spread and transmission of leprosy and related to disease types (102).
Specific genotypes of M. leprae were also linked to a particular clinical pole. Genotype 1 of M. leprae, linked to European geographic origins, has been associated with the tuberculoid pole (TT form), while genotype 2, linked to Brazilian regions such as Pernambuco and Ceará, has been related to the lepromatous pole (LL) of leprosy (103–106). Furthermore, there is evidence of the contribution of the specific characteristics of the strain linked to the leprosy event in the regulation/modulation of clinical states. An example of this is the evidence that leprosy cases caused by Mycobacterium lepromatosis were associated with diffuse lepromatous leprosy (DLL), although it should be mentioned that the population size screened in this kind of papers for the prevalence are too small to conclude (107, 108).
Regarding the future perspectives, the condensation of these information of this present article can help scientists and health professionals to see answers to immunopathogenic gaps, generate new hypotheses for the progression of leprosy, new ways of targeting to fight the disease, as well as new questions for both Mycobacterium leprae as well as other infections. In addition, clarification of the pathogenic interface of interaction between bacterial agent and host can help in the search for diagnostic and prognostic biomarkers for leprosy. In this sense, it is necessary to carry out more studies on the complete proteome and transcriptome profile of leprosy patients and household contacts with the disease.
The limitations of this study come up against the following conditions: a) the case definition of a leprosy patient according to the different classifications of the disease in each study; b) different forms of diagnosis; c) different strains of M. leprae; d) different genotypes of M. leprae. In order to find novel solutions to issues about the immunological aspects of leprosy, further study must be done along methodological lines. This includes investigating which speculations of biological elements, in particular, raise the likelihood that an individual would become lepromatous and erode their defenses: (1) examination of experimental research on the immunoregulation of the cell-mediated immune response in relation to nonreplicating antigens; (2) investigation of immune responses and their regulation in leprosy in mice; (3) evaluation of the outcomes of human immunotherapy trials; and (4) meticulous scrutiny of immune responses in other persistent infections in humans.
Conclusions
The progression factors of leprosy are linked to several interconnected complex molecular of host-parasite interaction. The early development of the host infection depends on these cells’ capacity to deliver antigens and control the polarization of macrophages. Apoptosis and autophagy may be possible with differing phenotypes of the macrophages that have been attracted to the infection site, which may result in a biphasic response, i.e., allowing replication and further dissemination or its elimination.
Innate immune cells try to reduce the bacterial burden by activating the adaptive immune system. The capacity of antigen presentation is actively downregulated by M. leprae as the bacterial load rises, which lowers the activation of T cell-mediated immunity (TT or LL form). As a result, the innate immune system plays a far larger role in the initiation of nerve damage, which is a symptom of the illness’s first presentation, and in many key immunological reactions, such as inflammation and the removal of dead M. leprae, even while the adaptive immune system intensifies nerve damage and determines the kind of leprosy.
Data availability statement
The original contributions presented in the study are included in the article/Supplementary Material. Further inquiries can be directed to the corresponding author.
Author contributions
MS: Conceptualization, Data curation, Formal analysis, Investigation, Methodology, Software, Writing – original draft. CS: Conceptualization, Data curation, Formal analysis, Investigation, Methodology, Resources, Writing – original draft. TB: Data curation, Formal analysis, Investigation, Resources, Validation, Visualization, Writing – original draft. AA: Data curation, Formal analysis, Investigation, Methodology, Software, Writing – original draft. Ed: Formal analysis, Investigation, Methodology, Resources, Validation, Visualization, Writing – original draft, Writing – review & editing. CF: Data curation, Formal analysis, Investigation, Methodology, Resources, Software, Supervision, Validation, Visualization, Writing – review & editing. KL: Formal analysis, Funding acquisition, Investigation, Methodology, Resources, Software, Supervision, Validation, Visualization, Writing – review & editing. LL: Conceptualization, Data curation, Funding acquisition, Methodology, Project administration, Resources, Software, Supervision, Validation, Visualization, Writing – review & editing.
Funding
The author(s) declare that no financial support was received for the research, authorship, and/or publication of this article.
Conflict of interest
The authors declare that the research was conducted in the absence of any commercial or financial relationships that could be construed as a potential conflict of interest.
Publisher’s note
All claims expressed in this article are solely those of the authors and do not necessarily represent those of their affiliated organizations, or those of the publisher, the editors and the reviewers. Any product that may be evaluated in this article, or claim that may be made by its manufacturer, is not guaranteed or endorsed by the publisher.
References
1. Bratschi MW, Steinmann P, Wickenden A, Gillis TP. Current knowledge on Mycobacterium leprae transmission: a systematic literature review. Leprosy Rev. (2015) 86:142–55. doi: 10.47276/lr.86.2.142
2. Bhat RM, Prakash C. Leprosy: an overview of pathophysiology. Interdiscip Perspect Infect Dis. (2012) 2012:181089. doi: 10.1155/2012/181089
3. mondiale de la Santé O, Organization WH. Global leprosy (Hansen disease) update, 2019: time to step-up prevention initiatives–Situation de la lèpre (maladie de Hansen) dans le monde, 2019: le moment est venu d’intensifier les initiatives de prévention. Weekly Epidemiological Record= Relevé épidémiologique hebdomadaire. (2020) 95:417–38.
4. Carlock S, Fulton EH, Rosenbaum E, Mukasa L, Gardner JM, Shalin SC, et al. Hansen disease (leprosy) and Armadillo exposure in Arkansas: a case series. Am J Dermatopathology. (2020) 42:769–73. doi: 10.1097/DAD.0000000000001660
5. Withington SG, Joha S, Baird D, Brink M, Brink J. Assessing socio-economic factors in relation to stigmatization, impairment status, and selection for socio-economic rehabilitation: a 1-year cohort of new leprosy cases in north Bangladesh. Leprosy Rev. (2003) 74:120–32. doi: 10.47276/lr.74.2.120
6. World Health Organization. World report on disability 2011. São Paulo, Brazil: World Health Organization (2011).
7. Masresha BM, Yesuf KM, Moyehodie YA, Biresaw HB, Mulugeta SS, Addisia GD. Determinant factors of leprosy-related disability; comparison of acceleration failure time and parametric shared frailty models. PloS One. (2023) 18:e0271883. doi: 10.1371/journal.pone.0271883
8. Young RA, Mehra V, Sweetser D, Buchanan T, Clark-Curtiss J, Davis RW, et al. Genes for the major protein antigens of the leprosy parasite Mycobacterium leprae. Nature. (1985) 316:450–2. doi: 10.1038/316450a0
9. Moschioni C, Antunes CM de F, Grossi MAF, Lambertucci JR. Risk factors for physical disability at diagnosis of 19,283 new cases of leprosy. Rev Soc Bras Med Trop. (2010) 43:19–22. doi: 10.1590/s0037-86822010000100005
10. Ridley DS. Classification of leprosy. In: Window in leprosy. Wardha: Gandhi Memorial Leproy foundation. (1978) 1978.
11. Jacobson RR, Krahenbuhl JL. Leprosy. Lancet. (1999) 353:655–60. doi: 10.1016/S0140-6736(98)06322-3
12. Walker SL, Lockwood DNJ. The clinical and immunological features of leprosy. Br Med Bull. (2006) 77:103–21. doi: 10.1093/bmb/ldl010
13. Froes LAR, Sotto MN, Trindade MAB. Leprosy: clinical and immunopathological characteristics. Anais Brasileiros Dermatologia. (2022) 97:338–47. doi: 10.1016/j.abd.2021.08.006
14. Page MJ, McKenzie JE, Bossuyt PM, Boutron I, Hoffmann TC, Mulrow CD, et al. PRISMA 2020 statement: an updated guideline for reporting systematic reviews. BMJ. (2021) 372:n71. doi: 10.1136/bmj.n71
15. Santos CM da C, Pimenta CA de M, Nobre MRC. The PICO strategy for the research question construction and evidence search. Rev Latino-Am Enfermagem. (2007) 15:508–11. doi: 10.1590/S0104-11692007000300023
16. Aromataris E, Munn Z. JBI manual for evidence synthesis. Adelaide, Australia: Joanna Briggs Institute (2020).
17. Munn Z, Aromataris E, Tufanaru C, Stern C, Porritt K, Farrow J, et al. The development of software to support multiple systematic review types: the Joanna Briggs Institute System for the Unified Management, Assessment and Review of Information (JBI SUMARI). Int J Evidence-Based Healthcare. (2019) 17:36–43. doi: 10.1097/XEB.0000000000000152
18. Froes LAR, Trindade MAB, Sotto MN. Immunology of leprosy. Int Rev Immunol. (2022) 41:72–83. doi: 10.1080/08830185.2020.1851370
19. Karamova AE, Semenova VG, Verbenko DA, Obraztsova OA, Vanchugova KHM, Nikonorov AA, et al. Experimental modeling of leprosy in BALB/c, BALB/c nude, CBA, and C57BL/6ТNF—/— Mice. Bull Exp Biol Med. (2020) 169:836–9. doi: 10.1007/s10517-020-04991-7
20. Doz-Deblauwe É, Carreras F, Arbues A, Remot A, Epardaud M, Malaga W, et al. CR3 engaged by PGL-I triggers syk-calcineurin-NFATc to rewire the innate immune response in leprosy. Front Immunol. (2019) 10:2913. doi: 10.3389/fimmu.2019.02913
21. Silva PHL, Santos LN, Mendes MA, Nery JAC, Sarno EN, Esquenazi D. Involvement of TNF-producing CD8+ Effector memory T cells with immunopathogenesis of erythema nodosum leprosum in leprosy patients. Am J Trop Med Hyg. (2019) 100:377–85. doi: 10.4269/ajtmh.18-0517
22. Montoya DJ, Andrade P, Silva BJA, Teles RMB, Ma F, Bryson B, et al. Dual RNA-seq of human leprosy lesions identifies bacterial determinants linked to host immune response. Cell Rep. (2019) 26:3574–3585.e3. doi: 10.1016/j.celrep.2019.02.109
23. Dang AT, Teles RMB, Liu PT, Choi A, Legaspi A, Sarno EN, et al. Autophagy links antimicrobial activity with antigen presentation in Langerhans cells. JCI Insight. (2019) 4:e126955. doi: 10.1172/jci.insight.126955
24. Vilani-Moreno FR, Barbosa ASAA, Sartori BGC, Diório SM, Silva SMUR, Rosa PS, et al. Murine experimental leprosy: Evaluation of immune response by analysis of peritoneal lavage cells and footpad histopathology. Int J Exp Pathol. (2019) 100:161. doi: 10.1111/iep.12319
25. Queiroz EA, Medeiros NI, Mattos RT, Carvalho APM, Rodrigues-Alves ML, Dutra WO, et al. Immunological biomarkers of subclinical infection in household contacts of leprosy patients. Immunobiology. (2019) 224:518–25. doi: 10.1016/j.imbio.2019.05.002
26. Andrade P R, Mehta M, Lu J, Teles R MB, Montoya D, Scumpia P O, et al. The cell fate regulator NUPR1 is induced by Mycobacterium leprae via type I interferon in human leprosy. PloS Negl Trop Dis. (2019) 13:e0007589. doi: 10.1371/journal.pntd.0007589
27. Rastogi R, Kaur G, Maan P, Bhatnagar A, Narang T, Dogra S, et al. Molecular characterization and immunogenic function of ML1899 (LipG) of Mycobacterium leprae. J Med Microbiol. (2019) 68:1629–40. doi: 10.1099/jmm.0.001080
28. de Souza Sales J, Lara FA, Amadeu TP, de Oliveira Fulco T, da Costa Nery JA, Sampaio EP, et al. The role of indoleamine 2, 3-dioxygenase in lepromatous leprosy immunosuppression. Clin Exp Immunol. (2011) 165:251–63. doi: 10.1111/j.1365-2249.2011.04412.x
29. Kumar S, Naqvi RA, Khanna N, Pathak P, Rao DN. Th3 Immune responses in the progression of leprosy via molecular cross-talks of TGF-β, CTLA-4 and Cbl-b. Clin Immunol. (2011) 141:133–42. doi: 10.1016/j.clim.2011.06.007
30. Sampaio LH, Sousa ALM, Barcelos MC, Reed SG, Stefani MMA, Duthie MS. Evaluation of various cytokines elicited during antigen-specific recall as potential risk indicators for the differential development of leprosy. Eur J Clin Microbiol Infect Dis. (2012) 31:1443–51. doi: 10.1007/s10096-011-1462-0
31. Moura DF, De Mattos KA, Amadeu TP, Andrade PR, Sales JS, Schmitz V, et al. CD163 favors Mycobacterium leprae survival and persistence by promoting anti-inflammatory pathways in lepromatous macrophages: Immunity to infection. Eur J Immunol. (2012) 42:2925–36. doi: 10.1002/eji.201142198
32. Dagur PK, Sharma B, Upadhyay R, Dua B, Rizvi A, Khan NA, et al. Phenolic-glycolipid-1 and lipoarabinomannan preferentially modulate TCR- and CD28-triggered proximal biochemical events, leading to T-cell unresponsiveness in mycobacterial diseases. Lipids Health Dis. (2012) 11:119. doi: 10.1186/1476-511X-11-119
33. Chung AW, Sieling PA, Schenk M, Teles RM, Krutzik SR, Hsu DK, et al. Galectin-3 regulates the innate immune response of human monocytes. J Infect Dis. (2013) 207:947–56. doi: 10.1093/infdis/jis920
34. Teles RM, Graeber TG, Krutzik SR, Montoya D, Schenk M, Lee DJ, et al. Type I interferon suppresses type II interferon–triggered human anti-mycobacterial responses. Science. (2013) 339:1448–53. doi: 10.1126/science.1233665
35. Kumar S, Naqvi RA, Bhat AA, Rani R, Ali R, Agnihotri A, et al. IL-10 production from dendritic cells is associated with DC SIGN in human leprosy. Immunobiology. (2013) 218:1488–96. doi: 10.1016/j.imbio.2013.05.004
36. Kumar S, Naqvi RA, Ali R, Rani R, Khanna N, Rao DN. Fox P 3 provides competitive fitness to CD 4+ CD 25+ T cells in leprosy patients via transcriptional regulation. Eur J Immunol. (2014) 44:431–9. doi: 10.1002/eji.201343649
37. Saini C, Ramesh V, Nath I. Increase in TGF-β secreting CD4+CD25+ FOXP3+ T regulatory cells in anergic lepromatous leprosy patients. PloS Negl Trop Dis. (2014) 8:e2639. doi: 10.1371/journal.pntd.0002639
38. Oliveira RM, Hungria EM, de Araújo Freitas A, De Sousa A, Costa MB, Reed SG, et al. Synergistic antigen combinations for the development of interferon gamma release assays for paucibacillary leprosy. Eur J Clin Microbiol Infect Dis. (2014) 33:1415–24. doi: 10.1007/s10096-014-2077-z
39. Bobosha K, Wilson L, van Meijgaarden KE, Bekele Y, Zewdie M, van der Ploeg-van Schip JJ, et al. T-cell regulation in lepromatous leprosy. PloS Negl Trop Dis. (2014) 8:e2773. doi: 10.1371/journal.pntd.0002773
40. Hagge DA, Scollard DM, Ray NA, Marks VT, Deming AT, Spencer JS, et al. IL-10 and NOS2 modulate antigen-specific reactivity and nerve infiltration by T cells in experimental leprosy. PloS Negl Trop Dis. (2014) 8::e3149. doi: 10.1371/journal.pntd.0003149
41. Nath I, Saini C, Valluri VL. Immunology of leprosy and diagnostic challenges. Clinics Dermatol. (2015) 33:90–8. doi: 10.1016/j.clindermatol.2014.07.005
42. Lyrio ECD, Campos-Souza IC, Corrêa LCD, Lechuga GC, Verícimo M, Castro HC, et al. Interaction of Mycobacterium leprae with the HaCaT human keratinocyte cell line: new frontiers in the cellular immunology of leprosy. Exp Dermatol. (2015) 24:536–42. doi: 10.1111/exd.12714
43. Braga AF, Moretto DF, Gigliotti P, Peruchi M, Vilani-Moreno FR, Campanelli AP, et al. Activation and cytokine profile of monocyte derived dendritic cells in leprosy: in vitro stimulation by sonicated mycobacterium leprae induces decreased level of IL-12p70 in lepromatous leprosy. Memórias do Instituto Oswaldo Cruz. (2015) 110:655–61. doi: 10.1590/0074-02760140230
44. de Sousa Aarão TL, de Sousa JR, Botelho BS, Fuzii HT, Quaresma JAS. Correlation between nerve growth factor and tissue expression of IL-17 in leprosy. Microbial pathogenesis. (2016) 90:64–8. doi: 10.1016/j.micpath.2015.11.019
45. Sadhu S, Khaitan BK, Joshi B, Sengupta U, Nautiyal AK, Mitra DK. Reciprocity between regulatory T cells and th17 cells: relevance to polarized immunity in leprosy. PloS Negl Trop Dis. (2016) 10:e0004338. doi: 10.1371/journal.pntd.0004338
46. Schenk M, Mahapatra S, Le P, Kim HJ, Choi AW, Brennan PJ, et al. Human NOD2 recognizes structurally unique muramyl dipeptides from Mycobacterium leprae. Infection Immun. (2016) 84:2429–38. doi: 10.1128/IAI.00334-16
47. Polycarpou A, Holland MJ, Karageorgiou I, Eddaoudi A, Walker SL, Willcocks S, et al. Mycobacterium leprae activates toll-like receptor-4 signaling and expression on macrophages depending on previous bacillus Calmette-Guerin vaccination. Front Cell infection Microbiol. (2016) 6:72. doi: 10.3389/fcimb.2016.00072
48. De Sousa JR, Pagliari C, De Almeida DSM, Barros LFL, Carneiro FRO, Dias LB, et al. Th9 cytokines response and its possible implications in the immunopathogenesis of leprosy. J Clin Pathol. (2017) 70:521–7. doi: 10.1136/jclinpath-2016-204110
49. Tarique M, Saini C, Naqvi RA, Khanna N, Rao DN. Increased IL-35 producing Tregs and CD19+IL-35+ cells are associated with disease progression in leprosy patients. Cytokine. (2017) 91:82–8. doi: 10.1016/j.cyto.2016.12.011
50. Tarique M, Naqvi RA, Ali R, Khanna N, Rao DN. CD 4+ TCR γδ+ FoxP3+ cells: An unidentified population of immunosuppressive cells towards disease progression leprosy patients. Exp Dermatol. (2017) 26:946–8. doi: 10.1111/exd.13302
51. Hungria EM, Freitas AA, Pontes MAA, Gonçalves HS, Sousa ALOM, Costa MB, et al. Antigen-specific secretion of IFNγ and CXCL10 in whole blood assay detects Mycobacterium leprae infection but does not discriminate asymptomatic infection from symptomatic leprosy. Diagn Microbiol Infect Dis. (2017) 87:328–34. doi: 10.1016/j.diagmicrobio.2017.01.002
52. Saini C, Tarique M, Rai R, Siddiqui A, Khanna N, Sharma A. T helper cells in leprosy: an update. Immunol Lett. (2017) 184:61–6. doi: 10.1016/j.imlet.2017.02.013
53. Santos MB, De Oliveira DT, Cazzaniga RA, Varjão CS, Dos Santos PL, Santos MLB, et al. Distinct roles of Th17 and Th1 cells in inflammatory responses associated with the presentation of paucibacillary leprosy and leprosy reactions. Scandinavian J Immunol. (2017) 86:40–9. doi: 10.1111/sji.12558
54. Tarique M, Saini C, Naz H, Naqvi RA, Khan FI, Sharma A. Fate of T cells and their secretory proteins during the progression of leprosy. Curr Protein Pept Sci. (2018) 19:889–99. doi: 10.2174/1389203718666170829120729
55. de Sousa JR, Prudente RL, Junior LBD, Carneiro FRO, Sotto MN, Quaresma JAS. IL-37 and leprosy: A novel cytokine involved in the host response to Mycobacterium leprae infection. Cytokine. (2018) 106:89–94. doi: 10.1016/j.cyto.2017.10.016
56. Chaves AT, Ribeiro-Junior AF, Lyon S, Medeiros NI, Cassirer-Costa F, Paula KS, et al. Regulatory T cells: Friends or foe in human Mycobacterium leprae infection? Immunobiology. (2018) 223:397–404. doi: 10.1016/j.imbio.2017.11.002
57. Serrano-Coll H, Acevedo-Saenz L, Cardona-Castro N. A hypothetical role for Notch signaling pathway in immunopathogenesis of leprosy. Med Hypotheses. (2017) 109:162–9. doi: 10.1016/j.mehy.2017.10.009
58. Oldenburg R, Mayau V, Prandi J, Arbues A, Astarie-Dequeker C, Guilhot C, et al. Mycobacterial phenolic glycolipids selectively disable TRIF-dependent TLR4 signaling in macrophages. Front Immunol. (2018) 9:2. doi: 10.3389/fimmu.2018.00002
59. Sadhu S, Mitra DK. Emerging concepts of adaptive immunity in leprosy. Front Immunol. (2018) 9:604. doi: 10.3389/fimmu.2018.00604
60. Toledo Pinto TG, Batista-Silva LR, Medeiros RCA, Lara FA, Moraes MO. Type I interferons, autophagy and host metabolism in leprosy. Front Immunol. (2018) 9:806. doi: 10.3389/fimmu.2018.00806
61. Kim EW, Teles RM, Haile S, Liu PT, Modlin RL. Vitamin D status contributes to the antimicrobial activity of macrophages against Mycobacterium leprae. PloS Negl Trop Dis. (2018) 12:e0006608. doi: 10.1371/journal.pntd.0006608
62. Dua B, Upadhyay R, Natrajan M, Arora M, Kithiganahalli Narayanaswamy B, Joshi B. Notch signaling induces lymphoproliferation, T helper cell activation and Th1/Th2 differentiation in leprosy. Immunol Lett. (2019) 207:6–16. doi: 10.1016/j.imlet.2019.01.003
63. Bezerra-Santos M, do Vale-Simon M, Barreto AS, Cazzaniga RA, De Oliveira DT, Barrios MR, et al. Mycobacterium leprae recombinant antigen induces high expression of multifunction T lymphocytes and is promising as a specific vaccine for leprosy. Front Immunol. (2018) 9:2920. doi: 10.3389/fimmu.2018.02920
64. Upadhyay R, Dua B, Sharma B, Natrajan M, Jain AK, Kithiganahalli Narayanaswamy B, et al. Transcription factors STAT-4, STAT-6 and CREB regulate Th1/Th2 response in leprosy patients: effect of M. leprae antigens. BMC Infect Dis. (2019) 19:1–11. doi: 10.1186/s12879-018-3601-z
65. Hooij A, Geluk A. In search of biomarkers for leprosy by unraveling the host immune response to Mycobacterium leprae. Immunol Rev. (2021) 301:175–92. doi: 10.1111/imr.12966
66. Idrissi NBE, Iyer AM, Ramaglia V, Rosa PS, Soares CT, Baas F, et al. In Situ complement activation and T-cell immunity in leprosy spectrum: An immunohistological study on leprosy lesional skin. PloS One. (2017) 12:e0177815. doi: 10.1371/journal.pone.0177815
67. Teles RMB, Kelly-Scumpia KM, Sarno EN, Rea TH, Ochoa MT, Cheng G, et al. IL-27 suppresses antimicrobial activity in human leprosy. J Invest Dermatol. (2015) 135:2410–7. doi: 10.1038/jid.2015.195
68. Huang CL. The transmission of leprosy in man. Int J Leprosy Other Mycobacterial Diseases: Off Organ Int Leprosy Assoc. (1980) 48:309–18.
69. Ramaprasad P, Fernando A, Madhale S, Rao JR, Edward VK, Samson PD, et al. Transmission and protection in leprosy: indications of the role of mucosal immunity. Leprosy Rev. (1997) 68:301–15. doi: 10.5935/0305-7518.19970038
70. Idris I, Abdurrahman AH, Fatulrachman, Aftitah VB, Fadlitha VB, Sato N, et al. Invasion of human microvascular endothelial cells by Mycobacterium leprae through Mce1A protein. J Dermatol. (2019) 46:853–8. doi: 10.1111/1346-8138.15047
71. Fadlitha VB, Yamamoto F, Idris I, Dahlan H, Sato N, Aftitah VB, et al. The unique tropism of Mycobacterium leprae to the nasal epithelial cells can be explained by the mammalian cell entry protein 1A. PloS Negl Trop Dis. (2019) 13:e0006704. doi: 10.1371/journal.pntd.0006704
72. Sato N, Fujimura T, Masuzawa M, Yogi Y, Matsuoka M, Kanoh M, et al. Recombinant Mycobacterium leprae protein associated with entry into mammalian cells of respiratory and skin components. J Dermatol Sci. (2007) 46:101–10. doi: 10.1016/j.jdermsci.2007.01.006
73. Pinheiro RO, Schmitz V, Silva BJ de A, Dias AA, de Souza BJ, de Mattos Barbosa MG, et al. Innate immune responses in leprosy. Front Immunol. (2018) 9:518. doi: 10.3389/fimmu.2018.00518
74. Girardin SE, Travassos LH, Hervé M, Blanot D, Boneca IG, Philpott DJ, et al. Peptidoglycan molecular requirements allowing detection by Nod1 and Nod2. J Biol Chem. (2003) 278:41702–8. doi: 10.1074/jbc.M307198200
75. Wynn TA, Chawla A, Pollard JW. Macrophage biology in development, homeostasis and disease. Nature. (2013) 496:445–55. doi: 10.1038/nature12034
76. Fulco T de O, Andrade PR, Barbosa MG de M, Pinto TGT, Ferreira PF, Ferreira H, et al. Effect of apoptotic cell recognition on macrophage polarization and mycobacterial persistence. Infection Immun. (2014) 82:3968. doi: 10.1128/IAI.02194-14
77. Del M, Sasiain C, de la Barrera S, Fink S, Finiasz M, Alemán M, et al. Interferon-gamma (IFN-γ) and tumour necrosis factor-alpha (TNF-α) are necessary in the early stages of induction of CD4 and CD8 cytotoxic T cells by Mycobacterium leprae heat shock protein (hsp) 65 kD. Clin Exp Immunol. (1998) 114:196–203. doi: 10.1046/j.1365-2249.1998.00702.x
78. Chiplunkar SV, Deshmukh MV, Samson PD, Butlin R, Bhatki WS, Chulawalla RG, et al. Natural killer-cell-mediated and antibody-dependent cellular cytotoxicity in leprosy. Int J Lepr Other Mycobact Dis. (1990) 58:334–41.
79. Im JS, Kang T-J, Lee S-B, Kim C-H, Lee S-H, Venkataswamy MM, et al. Alteration of the relative levels of iNKT cell subsets is associated with chronic mycobacterial infections. Clin Immunol. (2008) 127:214–24. doi: 10.1016/j.clim.2007.12.005
80. De Lima Silveira E, De Sousa JR, De Sousa Aarão TL, Fuzii HT, Dias LB, Carneiro FRO, et al. New immunologic pathways in the pathogenesis of leprosy: Role for Th22 cytokines in the polar forms of the disease. J Am Acad Dermatol. (2015) 72:729–30. doi: 10.1016/j.jaad.2014.11.023
81. Fernandes C, Gonçalves HS, Cabral PB, Pinto HC, Pinto MIM, Câmara LMC. Increased frequency of CD4 and CD8 regulatory T cells in individuals under 15 years with multibacillary leprosy. PloS One. (2013) 8:e79072. doi: 10.1371/journal.pone.0079072
82. Tarique M, Naz H, Kurra SV, Saini C, Naqvi RA, Rai R, et al. Interleukin-10 producing regulatory B cells transformed CD4+CD25– into tregs and enhanced regulatory T cells function in human leprosy. Front Immunol. (2018) 9:1636. doi: 10.3389/fimmu.2018.01636
83. Scollard DM, Truman RW, Ebenezer GJ. Mechanisms of nerve injury in leprosy. Clinics Dermatol. (2015) 33:46–54. doi: 10.1016/j.clindermatol.2014.07.008
84. Madigan CA, Cambier CJ, Kelly-Scumpia KM, Scumpia PO, Cheng T-Y, Zailaa J, et al. A macrophage response to mycobacterium leprae phenolic glycolipid initiates nerve damage in leprosy. Cell. (2017) 170:973–985.e10. doi: 10.1016/j.cell.2017.07.030
85. Barrera SDL, Finiasz M, Fink S, Ilarregui J, Alemán M, Olivares L, et al. NK cells modulate the cytotoxic activity generated by Mycobacterium leprae-hsp65 in leprosy patients: role of IL-18 and IL-13. Clin Exp Immunol. (2004) 135:105. doi: 10.1111/j.1365-2249.2004.02334.x
86. Krutzik SR, Ochoa MT, Sieling PA, Uematsu S, Ng YW, Legaspi A, et al. Activation and regulation of Toll-like receptors 2 and 1 in human leprosy. Nat Med. (2003) 9:525–32. doi: 10.1038/nm864
87. De Sousa JR, Lucena Neto FD, Sotto MN, Quaresma JAS. Immunohistochemical characterization of the M4 macrophage population in leprosy skin lesions. BMC Infect Dis. (2018) 18:576. doi: 10.1186/s12879-018-3478-x
88. Marin A, Bateman E, Barragan J, Vance J, Van Huss K, Cervantes J. Macrophage polarization leads to differential inflammatory responses to live and dead. Mycobacterium leprae. Clin Infect Immun. (2023) 8:31–6. doi: 10.14740/cii163
89. Ma Y, Pei Q, Zhang L, Lu J, Shui T, Chen J, et al. Live Mycobacterium leprae inhibits autophagy and apoptosis of infected macrophages and prevents engulfment of host cell by phagocytes. Am J Transl Res. (2018) 10:2929–39.
90. Guerreiro LTA, Robottom-Ferreira AB, Ribeiro-Alves M, Toledo-Pinto TG, Rosa Brito T, Rosa PS, et al. Gene expression profiling specifies chemokine, mitochondrial and lipid metabolism signatures in leprosy. PloS One. (2013) 8:e64748. doi: 10.1371/journal.pone.0064748
91. Lemes RMR, Silva CAdeMe, Marques MÂdeM, Atella GC, Nery JAdaC, Nogueira MRS, et al. Altered composition and functional profile of high-density lipoprotein in leprosy patients. PloS Negl Trop Dis. (2020) 14:e0008138. doi: 10.1371/journal.pntd.0008138
92. De Sousa JR, Quaresma JAS. The role of T helper 25 cells in the immune response to Mycobacterium leprae. J Am Acad Dermatol. (2018) 78:1009–11. doi: 10.1016/j.jaad.2017.11.025
93. Nahas AA, Lima MI de S, Goulart IMB, Goulart LR. Anti-lipoarabinomannan-specific salivary IgA as prognostic marker for leprosy reactions in patients and cellular immunity in contacts. Front Immunol. (2018) 9:1205. doi: 10.3389/fimmu.2018.01205
94. Sugawara-Mikami M, Tanigawa K, Kawashima A, Kiriya M, Nakamura Y, Fujiwara Y, et al. Pathogenicity and virulence of Mycobacterium leprae. Virulence. (2022) 13:1985–2011. doi: 10.1080/21505594.2022.2141987
95. Spencer JS, Brennan PJ. The role of Mycobacterium leprae phenolic glycolipid I (PGL-I) in serodiagnosis and in the pathogenesis of leprosy. Leprosy Rev. (2011) 82:344–57. doi: 10.47276/lr.82.4.344
96. Matsuoka M, Roa RIL, Budiawan T, Kyaw K, Chae G-T. Genotypic analysis of Mycobacterium leprae isolates from Japan and other Asian countries reveals a global transmission pattern of leprosy. FEMS Microbiol Lett. (2006) 261:150–4. doi: 10.1111/j.1574-6968.2006.00346.x
97. Honap TP, Pfister L-A, Housman G, Mills S, Tarara RP, Suzuki K, et al. Mycobacterium leprae genomes from naturally infected nonhuman primates. PloS Negl Trop Dis. (2018) 12:e0006190. doi: 10.1371/journal.pntd.0006190
98. Alotaibi MH, Bahammam SA, Ur Rahman S, Bahnassy AA, Hassan IS, Alothman AF, et al. The demographic and clinical characteristics of leprosy in Saudi Arabia. J Infection Public Health. (2016) 9:611–7. doi: 10.1016/j.jiph.2015.12.015
99. Woodall P, Scollard D, Rajan L. Hansen disease among Micronesian and marshallese persons living in the United States. Emerg Infect Dis. (2011) 17:1202–8. doi: 10.3201/eid1707.102036
100. Marciano LHSC, Belone A de FF, Rosa PS, Coelho NMB, Ghidella CC, Nardi SMT, et al. Epidemiological and geographical characterization of leprosy in a Brazilian hyperendemic municipality. Cad Saúde Pública. (2018) 34:e00197216. doi: 10.1590/0102-311X00197216
101. Chokkakula S, Dasari K, Attitalla IH, Aparna S, Ponnada P, Male MM. Strain typing and strain differentiation of Mycobacterium leprae by TTC repeats. International J Pharmacol. (2014) 10: 168–74. doi: 10.4172/2161-0703
102. Marin A, Van Huss K, Corbett J, Kim S, Mohl J, Hong B, et al. Human macrophage polarization in the response to Mycobacterium leprae genomic DNA. Curr Res Microbial Sci. (2021) 2:100015. doi: 10.1016/j.crmicr.2020.100015
103. Matsuoka M, Zhang L, Morris MF, Legua P, Wiens C. Polymorphism in the rpoT gene in Mycobacterium leprae isolates obtained from Latin American countries and its possible correlation with the spread of leprosy. FEMS Microbiol Lett. (2005) 243:311–5. doi: 10.1016/j.femsle.2004.12.031
104. Matsuoka M, Maeda S, Kai M, Nakata N. Mycobacterium leprae typing by genomic diversity and global distribution of genotypes. Int J leprosy other mycobacterial Dis. (2000) 68:121.
105. de Holanda MV, Marques LEC, de Macedo MLB, Pontes MA de A, Sabadia JAB, Kerr LRFS, et al. Presence of Mycobacterium leprae genotype 4 in environmental waters in Northeast Brazil. Rev Soc Bras Med Trop. (2017) 50:216–22. doi: 10.1590/0037-8682-0424-2016
106. Pfrengle S, Neukamm J, Guellil M, Keller M, Molak M, Avanzi C, et al. Mycobacterium leprae diversity and population dynamics in medieval Europe from novel ancient genomes. BMC Biol. (2021) 19:220. doi: 10.1186/s12915-021-01120-2
107. Singh P, Benjak A, Schuenemann VJ, Herbig A, Avanzi C, Busso P, et al. Insight into the evolution and origin of leprosy bacilli from the genome sequence of Mycobacterium lepromatosis. Proc Natl Acad Sci U.S.A. (2015) 112:4459–64. doi: 10.1073/pnas.1421504112
Keywords: leprosy, immunity, pathology, molecular, mycobacterium leprae, nerve damage
Citation: Silva MJA, Silva CS, Brasil TP, Alves AK, dos Santos EC, Frota CC, Lima KVB and Lima LNGC (2024) An update on leprosy immunopathogenesis: systematic review. Front. Immunol. 15:1416177. doi: 10.3389/fimmu.2024.1416177
Received: 11 April 2024; Accepted: 22 August 2024;
Published: 06 September 2024.
Edited by:
Jean-louis Mege, Aix-Marseille Université, FranceReviewed by:
Christian Albert Devaux, Centre National de la Recherche Scientifique (CNRS), FranceUtpal Sengupta, The Leprosy Mission Trust India, India
Hongsheng Wang, Chinese Academy of Medical Sciences and Peking Union Medical College, China
Rosane Mb Teles, University of California, Los Angeles, United States
Copyright © 2024 Silva, Silva, Brasil, Alves, dos Santos, Frota, Lima and Lima. This is an open-access article distributed under the terms of the Creative Commons Attribution License (CC BY). The use, distribution or reproduction in other forums is permitted, provided the original author(s) and the copyright owner(s) are credited and that the original publication in this journal is cited, in accordance with accepted academic practice. No use, distribution or reproduction is permitted which does not comply with these terms.
*Correspondence: Marcos Jessé Abrahão Silva, jesseabrahao10@gmail.com
†These authors have contributed equally to this work