- Department of Surgery, Universitätsklinikum Erlangen, Friedrich-Alexander Universität Erlangen-Nürnberg, Erlangen, Germany
Interleukin (IL)-3 has long been known for its hematopoietic properties. However, recent evidence has expanded our understanding of IL-3 function by identifying IL-3 as a critical orchestrator of inflammation in a wide array of diseases. Depending on the type of disease, the course of inflammation, the cell or the tissue involved, IL-3 promotes either pathologic inflammation or its resolution. Here, we describe the cell-specific functions of IL-3 and summarize its role in diseases. We discuss the current treatments targeting IL-3 or its receptor, and highlight the potential and the limitations of targeting IL-3 in clinics.
Introduction
Interleukin (IL)-3, a cytokine belonging to the β common chain family of cytokines with IL-5 and granulocyte-macrophage colony-stimulating factor (GM-CSF), is mainly produced by immune cells, but also by some non-immune cells, such as astrocytes or cells harboring a mesenchymal stem cell (MSC) phenotype (1, 2). IL-3 exerts its function through a heterodimeric receptor composed of the IL-3 receptor α-chain (CD123) and the common receptor β-chain (CD131), CD123 providing the specificity for IL-3, while CD131 is essential for signaling and assembly (3). Given the low affinity of IL-3 for CD123, heterodimerization with CD131 is crucial as it creates a high-affinity receptor complex (3). In addition to CD131, mice express another IL-3-specific β chain, this chain differing from CD131 in its ability to bind murine IL-3 directly (4), although the presence of CD123 is required for signaling (5) (Figure 1). Thus, the expression pattern of CD123 defines the target cell profiles and function of IL-3. CD123 is mainly expressed on hematopoietic cells, including hematopoietic stem and progenitor cells (HSPCs), basophils, eosinophils, mast cells, non-classical monocytes, macrophages, human plasmacytoid dendritic cells (pDCs) and activated T and B cells. CD123 expression is not limited to the hematopoietic compartment, but it extends to non-hematopoietic cells, such as endothelial and epithelial cells or osteoblasts and osteoclasts (Figure 2).
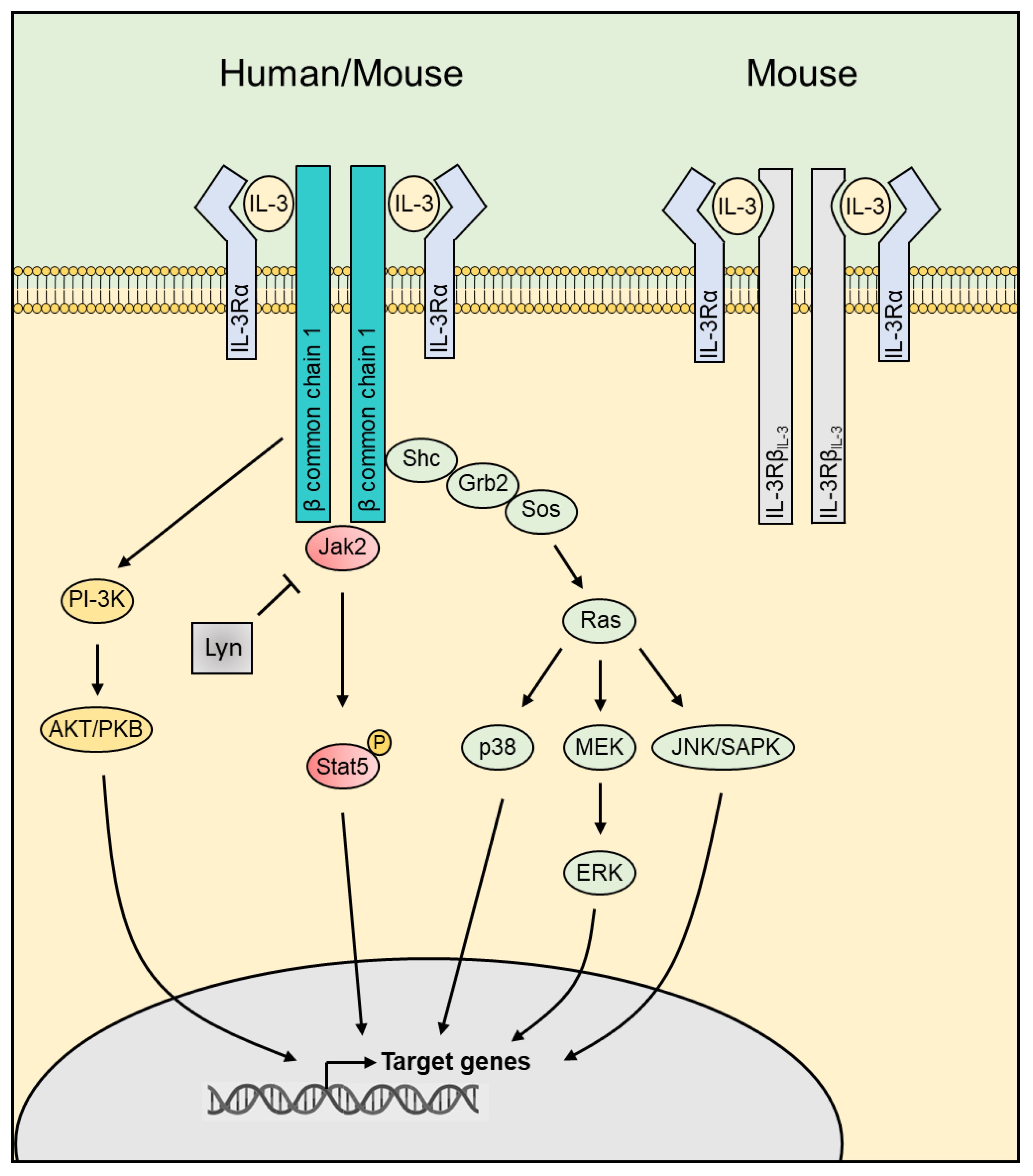
Figure 1 The IL-3 receptors and the downstream signaling. IL-3 exerts its function in humans through a heterodimeric receptor composed of the IL-3 receptor α-chain (CD123) and the common receptor β-chain (CD131). In addition to CD131, mice express another IL-3-specific β chain showing a strong homology at the amino acid level with CD131 but differing by its ability to bind IL-3. IL-3 binding leads to activation of downstream pathways including JAK2/STAT5, PI-3K/AKT and MAPK. JAK, Janus Kinase; STAT, signal transducer and activator of transcription; PI-3K, phosphoinositide 3-kinase; AKT, Protein kinase B; MAPK, Mitogen-Activated Protein Kinase.
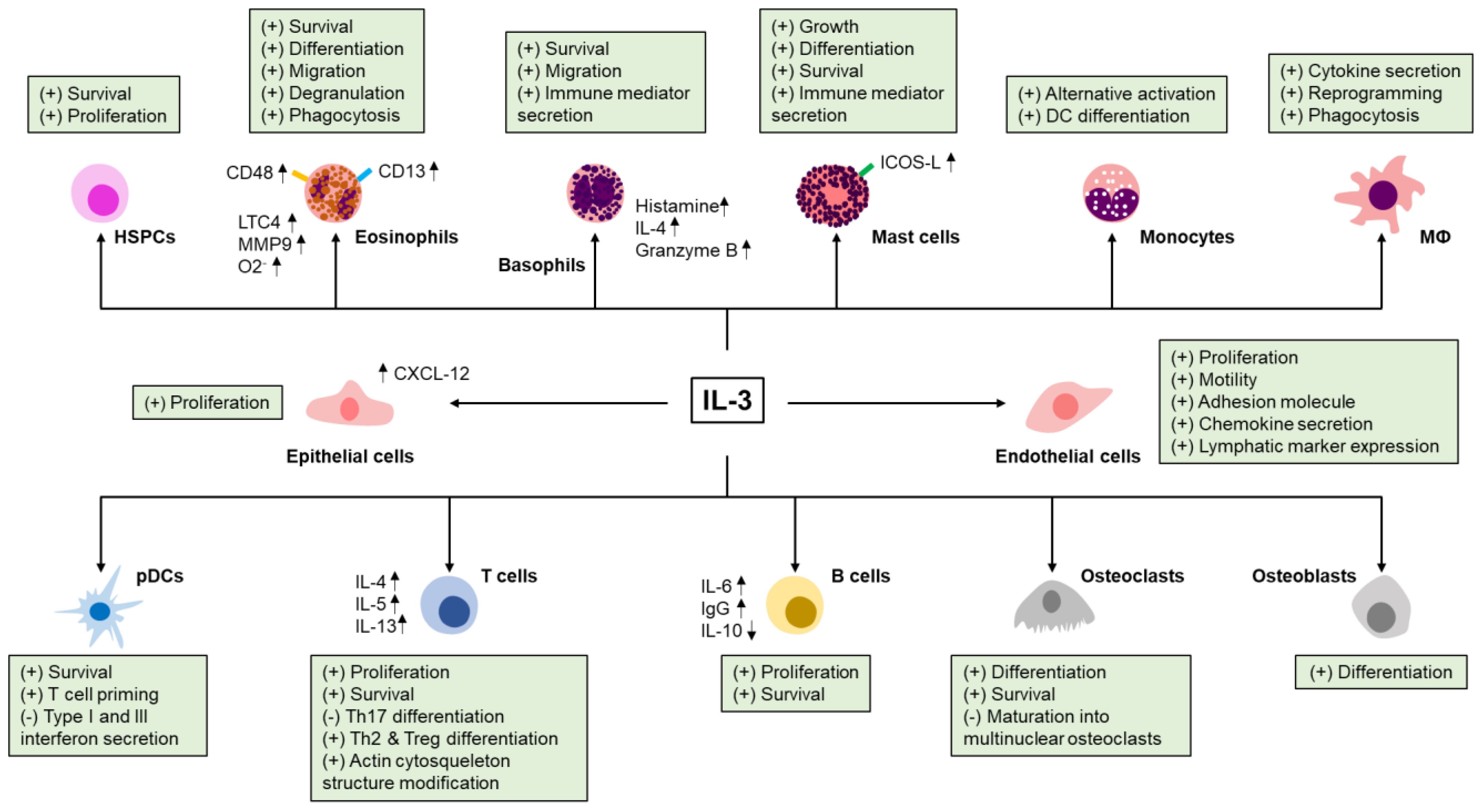
Figure 2 Effect of IL-3 on immune and non-immune cells. IL-3 modulates the survival, the growth, the differentiation, the migration, the phagocytosis and the secretion of immune mediators of many hematopoietic (hematopoietic stem and progenitor cells (HSPCs), eosinophils, basophils, mast cells, monocytes, macrophages, plasmacytoid dendritic cells (pDCs), T and B cells) and non-hematopoietic cells (endothelial cells, epithelial cells, osteoblasts and osteoclasts). CD, cluster of differentiation; LTC4, leukotriene C4; MMP9, matrix metallopeptidase 9; O2-, superoxide anion; IL-4, interleukin-4; ICOS-L, inducible costimulator-ligand; M⦶, macrophages; CXCL-12, C-X-C motif chemokine 12; Th, T helper; Treg, regulatory T cells; IgG, immunoglobulin G.
Blood levels of IL-3 are usually low to undetectable in healthy donors, although some have high IL-3 levels (6, 7). Likewise, IL-3 is hardly detected in the healthy or non-inflammatory conditions of many tissues but is produced at the site of inflammation, revealing IL-3 as an important regulator of the immune response. Indeed, IL-3 supports the survival, the proliferation, the differentiation, the polarization or the recruitment of immune and non-immune cells in infection, inflammatory diseases and cancer (Table 1).
Here, we review the function of IL-3 in health and disease. We describe the cell-specific effects of IL-3 and summarize its role in host defense, autoimmunity, and cancer. Likewise, we discuss the current treatments targeting IL-3 or its receptor and highlight the potential and the limitations of targeting IL-3 in clinics.
Cellular targets of IL-3
HSPCs
HSPCs give rise to all types of blood cells and play a pivotal role in building the immune system. As suggested by its former name (multi-colony stimulating factor), IL-3 stimulates the growth and differentiation of HSPCs from bone marrow cultures and in naive or sublethally irradiated mice into a large range of cell lineages, including basophils, neutrophils, eosinophils, macrophages, erythroid cells, megakaryocytes, and dendritic cells (DCs) (48, 49). Despite the abovementioned ability of IL-3 to stimulate hematopoiesis, mice deficient for Il-3 exhibit normal hematopoiesis under physiological conditions (33) indicating that IL-3 is not essential for the development of all blood cell lineages at steady state. However, IL-3 was described to play a key role in splenic extramedullary hematopoiesis during inflammatory diseases (2, 10, 40), which is consistent with the ability of recombinant IL-3 to increase hematopoiesis in the spleen and the liver of naive mice but not in the bone marrow (48, 49). Likewise, IL-3 increases the number of tissue mast cells and enhances basophil production during parasite infection, while it is dispensable for their generation under physiological conditions (33), thus making IL-3 an orchestrator of emergency hematopoiesis.
Eosinophils
Eosinophils are polymorphonuclear cells involved in the protection against multicellular parasites through the release of a variety of granular mediators and the production of toxic reactive oxygen species (50). IL-3 induces eosinophil survival (51) and is associated with early and end stages of eosinophilic differentiation and maturation (52). In addition, IL-3 increases human eosinophil adhesion, chemotaxis and migration (53) and stimulates cytotoxicity against antibody-sensitized helminths, superoxide anion production and phagocytosis of opsonized yeast particles (54).
Basophils
Basophils, one of the main effector cells during allergic diseases, are described to express both IL-3 (55) and its receptor (56). As for eosinophils, IL-3 plays a key role in basophil survival, migration and activation. Indeed, IL-3 protects basophil from apoptosis in vitro in a Pim1-dependent manner (57), increases basophil number in the blood during parasite infection (34), and enables basophil extravasation during allergic contact dermatitis (58). Moreover, IL-3, alone or in a combination with other molecules, such as anti-FcϵRI mAb, complement component 5a, N-Formylmethionine-leucyl-phenylalanine (fMLP), CCL2 or eotaxin, enhances the expression of various immune mediators important for the function of basophils, such as histamine (59) or granzyme B (60).
Mast cells
In mice, IL-3 promotes mast cell growth and differentiation (61) and enhances mediator release (62). However, CD123 was not detected on human mast cells purified from tonsils, lungs, uterus and skin (63) and no IL-3-binding sites were detected on enriched human lung mast cells (64) suggesting a limited impact of IL-3 on human mature mast cells in vivo.
Myeloid cells and pDCs
In steady-state, murine monocytes and human classical monocytes do not express the receptor for IL-3. Only human intermediate and non-classical monocytes express it (65). Although IL-3 alone does not seem to modulate the function of CD14+ monocytes, in vitro experiments show that IL-3 synergizes with IL-4 to enhance CCL17 expression in CD14+ monocytes, a marker of alternative activation, IL-4 increasing the responsiveness to IL-3 by inducing CD123 expression (66). Likewise, IL-3 enhances the production of TNFα by CD14+ monocytes upon lipopolysaccharide (LPS) stimulation by modulating TNFα posttranscriptional levels (67). In combination with IFNβ or IL-4, IL-3 drives the differentiation of CD14+ monocytes into DCs exhibiting pro- or anti-inflammatory properties, respectively (68, 69), and IL-3 induces the expression of the lectins mannose receptor, Dectin-1, and DC-SIGN during monocyte-derived macrophage differentiation allowing increased phagocytosis (70). Besides this, IL-3 modulates the expression of IL-1 and the chemokines CCL2, CCL3, CCL7, CCL12 in macrophages (8, 71, 72), increases peritoneal macrophage phagocytic activity (48), and elicits transcriptional, morphological, and functional programming of microglia (1, 14).
In addition to the monocyte-macrophage compartment, it was described that human pDCs express high levels of CD123, whereas murine pDCs do not express it (73). In humans, IL-3 promotes the survival of pDCs (74) as well as their differentiation in a sub-population of DCs characterized by a low ability to produce type I and III interferons but with a high capacity to prime T cells (27, 28). Interestingly, recent studies have revealed that CD123 is not only expressed on human pDCs but also on AXL+ DCs, dendritic cell precursors that look similar to conventional DC2s in terms of basic function and morphology (75).
T and B cells
T cells are a major source of IL-3 during inflammation (76) and IL-3-producing T cells are involved in many inflammatory and infectious diseases. In humans, CD123 is expressed on activated CD4+ and CD8+ T cells (77). Exposure to IL-3 enhances T cell proliferation and survival (77), inhibits Th17 differentiation (19) and enhances Th2 differentiation as well as IL-4, IL-5, and IL-13 expressions (78). Moreover, IL-3 promotes the differentiation of naive CD4+ T cells into regulatory T cells, and modulates regulatory T cell migration by modifying their actin cytoskeleton structure (43). Like T cells, activated human B cells express CD123 and IL-3 supports their proliferation and survival (77). In addition, IL-3 enhances IgG and IL-6 secretions but reduces IL-10 expression (77, 79). Recently, innate response activator (IRA) B cells, a subset of B-1a B cells residing in serosal sites, have been described as a source of IL-3 in infectious and inflammatory diseases (2, 40). After activation, IL-3-producing IRA B cells accumulate in the spleen, where they fuel the immune response by promoting extramedullary hematopoiesis (2, 40).
Endothelial and epithelial cells
In addition to hematopoietic cells, CD123 is expressed by endothelial and epithelial cells (2, 29). IL-3 induces intestinal epithelial cell proliferation (80) and stimulates CXCL12 expression in lung epithelial cells (29), while it promotes endothelial cell proliferation and motility in vitro (81, 82) as well as new vessel formation and tumor angiogenesis in vivo (82). Moreover, IL-3 induces the expression of adhesion molecules and chemokines in endothelial cells, thereby promoting immune cell rolling, adhesion, and transmigration (81).
Osteoblasts and osteoclasts
IL-3 plays an important role in bones by directly targeting both osteoblasts and osteoclasts. IL-3 promotes the differentiation of human mesenchymal stem cells into osteoblasts (83) and stimulates the formation and the survival of mononuclear osteoclasts (84). However, IL-3 inhibits the formation of mature multinucleated osteoclasts by diverting osteoclast precursors into macrophage or dendritic cell lineages, inhibiting NF-kB nuclear translocation induced by RANKL, and downregulating the expression of c-Fms (20, 21).
IL-3 in diseases
Cardiovascular diseases
Cardiovascular diseases are a group of disorders of the heart and blood vessels that are a leading cause of mortality worldwide (85). Although IL-3 is hardly detected in the heart in steady-state, its expression increased significantly during autoimmune myocarditis (8) and allograft heart transplantation (9). IL-3 is also expressed in early and advanced atherosclerotic plaques in humans (11) and plasma IL-3 levels predict for symptomatic restenosis (86). In experimental autoimmune myocarditis, IL-3 amplifies cardiac inflammation by promoting CCR2+ Ly-6Chigh monocyte accumulation in the heart via the stimulation of tissue macrophages. Recruited monocytes give rise to APCs that enhance local T cell proliferation and T cell-derived cytokine production, including IL-3, thus amplifying local inflammation (8). In addition, deletion or inhibition of IL-3 reduced the development of myocardial fibrosis and protects from chronic rejection of heart transplants by reducing the ability of infiltrating basophil to secrete IL-4 and IL-6 (9). In atherosclerosis, IL-3 sustains both the proliferation and the migration of vascular smooth muscle cells (11), a major source of plaque cells and extracellular matrix at all stages of atherosclerosis (87). Interestingly, IL-3 secreted in the spleen of Apoe-/- mice promotes HSPC expansion and differentiation into Ly6Chigh monocytes. Monocytes born in such extramedullary niches intravasate, circulate, and accumulate abundantly in atherosclerotic lesions where they secrete inflammatory cytokines, reactive oxygen species and proteases, and eventually become foam cells (10), thus promoting atheroma macrophage burden (12). Therefore, IL-3 seems to modulate inflammation in cardiac and vascular tissue by exerting its activity directly at the inflammatory site and indirectly in periphery.
Autoimmune diseases
Arthritis
In a model of inflammatory arthritis, IL-3 inhibits TNFα-induced bone resorption and prevents cartilage and bone loss in the joints (22). In osteoarthritis, IL-3 protects from cartilage degeneration and bone damage by reducing the expression of matrix metalloproteinases (MMPs) (23). IL-3 also reduces the severity of collagen-induced arthritis by modulating the development of Foxp3 regulatory T-cells (24) and by preventing the development of Th17 cells (19). Thus, it seems that IL-3 protects from joint inflammation by modulating the immune response in arthritis in addition to its role on osteoblast and osteoclast differentiation.
Diabetes
Diabetes is a chronic metabolic disease characterized by high levels of glucose in the blood. There are two main types of diabetes: type 1 diabetes (T1D) and type 2 diabetes (T2D). T1D develops when the immune system attacks and destroys pancreatic beta cells resulting in an inability of patients to produce insulin (88). T2D is characterized by a reduced production of insulin or a resistance to it, and is associated with obesity or high body fat percentage in the abdominal region (89). While its role during T2D is still unknown, IL-3 is thought to be protective during T1D. Indeed, IL-3 stimulates the development of immature T cells with strong immunoregulatory function in the bone marrow of non-obese diabetic (NOD) mice, which significantly delays the apparition of the first symptoms and reduces the overall incidence of the disease (25). In addition, deficiency in Il-3 and Csf2 results in insulitis, insulin-producing β cell destruction, and abnormal glucose tolerance (26).
Central nervous system inflammation
In the central nervous system (CNS), IL-3 is mainly expressed by neurons and a subset of astrocytes (1, 90). In patients with Alzheimer disease (AD), low plasma IL-3 levels are associated with AD risk (91), whereas IL-3 levels in frontal cortex tissue are unaltered by AD pathology (1). Only the expression of CD123 is increased in frontal cortex tissue and correlates with disease duration and β-amyloid (Aβ) levels (1). This increase of CD123 is specific to microglia, macrophages of the CNS, and is age-dependent (1). The stimulation of CD123+ microglia by IL-3 induces their reprogramming into an acute immune response program that improves their ability to clear Aβ and tau aggregates, thus limiting AD (1). By contrast, IL-3 seems to be detrimental in multiple sclerosis (MS). For instance, IL-3 administration to mice with experimental autoimmune encephalitis (EAE) worsens the disease (15), whereas mice deficient for Il-3 are protected from developing EAE (14). In MS, IL-3 is produced by astrocytes and infiltrating CD44hiCD4+ T cells, while CD123 is mainly expressed by microglia and recruited myeloid cells in the spinal cord (14). As observed in AD, the local production of IL-3 reprograms IL-3Rα+ myeloid cells of the CNS. However, this reprogramming amplifies CNS immune cell infiltration increasing MS severity. Thus, this discrepancy between AD and MS reveals a dual role of IL-3 during CNS inflammation, the same mechanism (reprogramming of IL-3R+ myeloid cells) leading to two different outcomes (beneficial in AD and detrimental in MS).
Systemic lupus erythematosus
In MLR/lpr mice, a mouse model of systemic lupus erythematosus, plasma IL-3 levels increase concomitantly with disease progression. Administration of IL-3 aggravates lupus nephritis in MLR/lpr mice, whereas injection of antibodies against IL-3 reduces the severity of the disease (92). In patients with SLE, pDCs are continuously activated by circulating immune complexes resulting in an aberrant production of type I IFNs, which contributes to autoreactive T cells stimulation and autoantibody-secreting plasma cell generation, thus highlighting IFN and pDCs as critical contributors to the disease. Interestingly, serum IL-3 levels correlate with serum IFNα and IFNλ levels in patients with SLE (7) and IL-3 potentiates the secretion of IFNα by pDCs upon immune complexes stimulation (13). Targeting CD123+ cells or IL-3 signaling could therefore constitute a future therapeutic target in SLE.
Asthma
Studies investigating the contribution of IL-3 in asthma pathogenesis, a chronic inflammatory disorder associated with airway hyper-responsiveness, show inconsistent results, both on IL-3 expression levels and function. Indeed, IL-3 levels were found either increased in sputum (93) and BALF (94) of asthmatic patients, reduced in nasopharyngeal fluid of asthmatic children (30), or similar in bronchial biopsies between asthmatic and non-asthmatic patients (95). Likewise, a report showed that mice deficient for Il-3 exhibit increased pulmonary inflammation during asthma, characterized by local eosinophil infiltration and increased secretion of IgE, IL-5, and IL-13 (31). On the contrary, another study reported that Il-3-/- mice show similar number of basophils and eosinophils in BALF as well as similar serum IgE levels when compared to control mice (32). In human, it was shown that (i) sputum IL-3 secretion correlates with levels of eosinophil granule proteins and decreased lung function (93); (ii) IL-3+ BALF cells are associated with asthma severity (96) and; (iii) serum IL-3 levels are higher in patients with uncontrolled chronic asthma (97). By contrast, other studies revealed that IL-3 produced by PBMCs is associated with amelioration of asthma in pre-school children (31) and that IL-3 expression in lung tissue is not correlated with eosinophil and metachromatic cell number, airway responsiveness, or the severity of the late asthmatic response (95). Mechanistically, IL-3 was described to protect against asthma by reducing the number of innate lymphoid cell type 2 in lungs and by increasing regulatory T cell number in the airways (30, 31). On the other hand, IL-3 was reported to be detrimental in asthma by enhancing the secretion of histamine and Th2 cytokines by basophils (32). This contradictory effect in mice might result from the different times of injection, application routes, and doses of IL-3, while the differences observed in humans might be explained by the age of the patients (children vs adults) or the stage of the disease.
Infectious diseases
Parasitic infections
Protozoa and helminths are the two main parasites infecting humans. Interestingly, polymorphism in the human IL-3 gene was described to be associated with the pathophysiology of malaria (98) and mice lacking IL-3 are more resistant to Plasmodium berghei NK65-induced cerebral malaria (35). Moreover, mice susceptible to Leishmania major infections exhibit higher IL-3-secreting draining lymph node cell number than resistant mice and reduced disease score after anti-IL-3 antibody treatment (36). Thus, these results suggest that IL-3 impairs protective immunity during protozoan infections. The mechanisms associated with IL-3-dependent susceptibility to protozoan infections seem to involve macrophages as shown by (i) the ability of mast cell-produced IL-3 to alter the antimicrobial activity of macrophages during Leishmania donovani infections (37) and; (ii) the aggravation of Leishmania major infections after adoptive transfer of macrophages treated with IL-3 (36). Unlike protozoan infections, Il-3-deficient mice exhibited defective immunity against Strongyloides venezuelensis (33) and treatment with recombinant IL-3 protects C57BL/6 mice against Strongyloides ratti but not against Nippostrongylus brasiliensis (38), indicating that IL-3 protects against some helminth infections. The anti-helminthic effect of IL-3 is exerted by its ability to increase the number of circulating basophils and tissue mast cells, to promote the recruitment of basophils into the lymph node, and to stimulate the secretion of IL-4 by basophils (33, 34, 39).
Viral infections
IL-3 was described as a predictive marker for clinical outcome and disease severity during SARS-CoV-2+ infections (29), IL-3 being mainly produced by CD4+ T cells (29) and induced by the viral ORF7a protein (99). In a mouse model of pulmonary HSV-1 infection, IL-3 protects against viral pneumonia by promoting the recruitment of pDC into the lung parenchyma in a CXCL12-dependent manner (29). In humans, IL-3 enhances T cell priming by pDCs but have no effect on type I or type III IFN production by pDCs upon viral activation (27, 28). Moreover, plasma IL-3 and IFNλ levels correlate in patients with SARS-CoV-2+ infections and in septic patients with pulmonary viral infections suggesting that IL-3 might also induce IFNλ expression during viral airway infections in a pDC-independent manner (28, 29). Thus, IL-3 seems to play a critical role in safeguarding environments frequently exposed to viruses.
Bacterial infections
The role of IL-3 during bacterial infections remains poorly described. Studies using LPS, a membrane component of Gram-negative bacteria, show that IL-3 enhances pro-inflammatory cellular response to LPS in vitro (41). In vivo, IL-3 augments LPS-induced murine lung injury by enhancing the recruitment of neutrophils and lymphocytes as well as the secretion of pro-inflammatory cytokines in BALF (42). Also, IL-3 potentiates inflammation during cecal ligature and puncture-induced sepsis by promoting the myelopoiesis of monocytes and neutrophils, which fuels the cytokine storm and leads to increased mortality (40) but protects against S. Typhimurium infections (2).
Inflammatory bowel diseases
Inflammatory bowel diseases (IBDs) are inflammatory disorders of the intestinal tract characterized by in an aberrant mucosal immune response driven by microbial factors of the commensal gut microbiota (100). Whereas the role of GM-CSF during colitis is clearly established, the role of IL-3 was until recently unknown. IL-3 and CD123 expressions are upregulated in inflamed tissue of patients with IBDs and correlate with the levels of inflammation (2, 43). In the colon, IL-3 is produced by T cells and cells harboring a mesenchymal stem cell phenotype, whereas CD123 is expressed by epithelial and infiltrating immune cells (2, 43). The use of different models of colitis has revealed that IL-3 has a dual role during acute colitis, which seems to depend on the intensity of the inflammation. On the one hand, IL-3 protects by (i) promoting the early recruitment of splenic neutrophils with high microbicidal capacity in the colon, which requires CCL5+ PD-1hi LAG-3hi T cells, STAT5 signaling, and CCL20 (2); (ii) preventing the egress of regulatory T cells from the colon through the modification of their actin cytoskeleton structure (43) and; (iii) stimulating the proliferation of basophils, which protects from acute inflammation by reducing the expression of IFNγ, IL-2, and TNFα in T cells (44). On the other hand, it was reported that IL-3 increases colitis severity during the acute phase of colitis by amplifying colonic inflammation (2). This phenomenon might result from the ability of IL-3 to promote extramedullary hematopoiesis in the spleen during colitis (2), which is important at the onset of the disease to sustain splenic neutrophil emigration into the colon, but might become detrimental during acute colitis by fueling the inflammatory immune response.
Cancers
Hematologic cancers
Hematologic cancers are characterized by an accumulation of abnormal hematopoietic cells in the bone marrow, blood or lymph nodes (101). As observed during normal hematopoiesis, IL-3 acts as a growth factor in many hematologic neoplasms. While expressed at low levels or absent on normal hematopoietic stem cells, CD123 is overexpressed in a variety of hematologic cancers, such as acute myeloid leukemia (AML), blastic plasmacytoid dendritic cell neoplasm (BPDCN), B-cell acute lymphoblastic leukemia, or Hodgkin lymphoma (3). In AML, high expression of CD123 on blasts or leukemic stem cells is associated with increased blast proliferation, resistance to apoptosis and worst prognosis (16). In addition, it enhances the response to low concentration of IL-3 and alters CXCR4/CXCL12 interaction in the BM (17). Moreover, IL-3 is significantly increased in serum of children with AML as well as in the bone marrow plasma of patients with multiple myeloma (MM), while no difference was observed in children with acute lymphoblastic leukemia (ALL) (18).
Solid cancers
Unlike hematologic cancers, the role of IL-3 in solid tumors is little described. In patients with pancreatic ductal adenocarcinomas (PDAC), serum IL-3 levels are significantly lower than in healthy donors and do not correlate with the most common symptoms, the tumor location and the cancer stage (102). In PDAC-draining lymph nodes, IL-3, produced by CD4+ T cells, stimulates basophils to secrete IL-4, which is necessary for the stabilization of the Th2 phenotype (45), a signature associated with reduced survival. In triple negative breast cancer, CD123 expression correlates with nodal metastasis and reduced survival (46), and IL-3 promotes epithelial-to-endothelial and epithelial-to-mesenchymal transition, lung metastasis, and increased programmed-cell death ligand-1 (PD-L1) expression on tumor and mononuclear immune cells (46). Finally, it was reported that serum IL-3 levels may act as a tumor marker for colorectal cancer (103) and that overexpression of IL-3 in a mouse lung carcinoma cell line inhibits tumor growth in vivo by enhancing the generation of tumor-specific cytotoxic CD8+ T cells (47).
IL-3 and CD123 in clinics
Given the ability of IL-3 to stimulate hematopoiesis, multiple clinical trials have evaluated IL-3 as a treatment for patients with cytopenia (Figure 3). Administration of IL-3 in healthy subjects and patients with bone marrow failure or myelodysplastic syndrome results in increased platelet, reticulocyte and leukocyte numbers with only mild side effects (104). Likewise, IL-3 alone or followed by G-CSF reduces the incidence and the severity of neutropenia and/or thrombocytopenia associated with chemotherapy in patients with solid tumors (105). Thus, IL-3 alone effectively abrogates cytopenia, but does not appear to have a real benefit after chemotherapy in comparison with GM-CSF or G-CSF. As well, IL-3 has limited effect in Human Immunodeficiency Virus (HIV)-infected patients with cytopenia (106). A fusion protein combining the active domain of IL-3 and GM-CSF was also assessed in clinical trials. This protein was described to be more efficient than GM-CSF in preventing cumulative thrombocytopenia associated with chemotherapy in patients with sarcoma but not in patients with advanced breast cancer (107, 108).
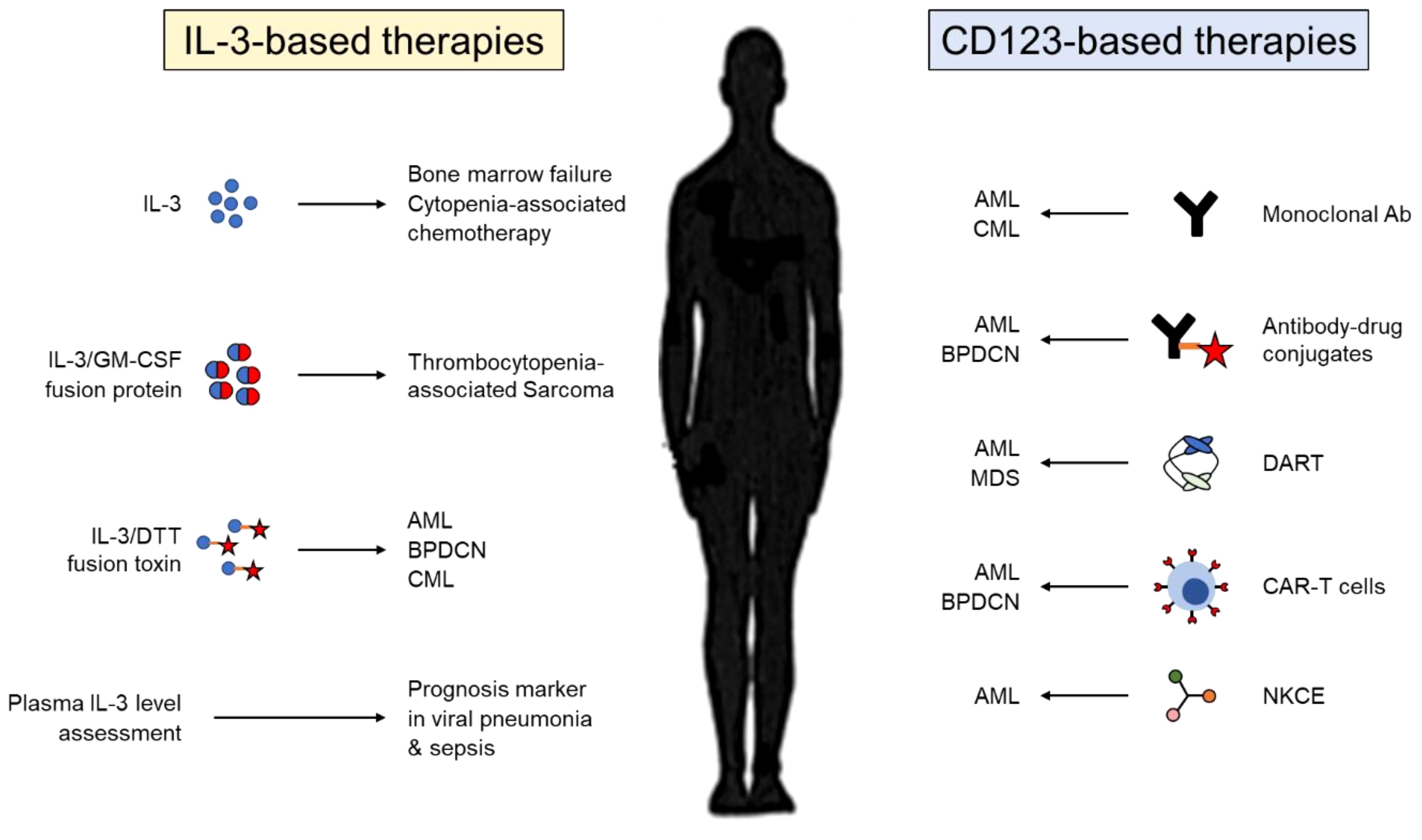
Figure 3 Therapeutic targeting of IL-3 and its receptor CD123. Therapies used in pre-clinical or clinical studies targeting either IL-3 (left) or CD123 (right). IL-3, interleukin-3; GM-CSF; granulocyte-macrophage colony-stimulating factor; DTT, diphtheria toxin; AML, acute myeloid leukemia; BPDCN, blastic plasmacytoid dendritic cell neoplasm; CML, chronic myelogenous leukemia; Ab, antibody; MDS, myelodysplastic syndrome; DART, dual affinity retargeting molecule; CAR-T cells, chimeric antigen receptor T cells; NKCE, natural killer cell engager.
As previously described, myeloid and lymphoid leukemic stem cells express high levels of CD123 in comparison with normal hematopoietic stem cells pointing out CD123 as a potential target for therapy against leukemia (Figure 3). Early treatments have used either monoclonal antibodies against CD123 or antibody-drug conjugates against CD123. While preclinical studies showed promising results (109, 110), many clinical trials revealed either insufficient antileukemic activity or high toxicity (111). By contrast, therapies using a recombinant toxin combining the catalytic and translocation domains of diphtheria toxin and human IL-3 show promising efficacity for the treatment of leukemias (112). Preclinical studies have also demonstrated that redirecting specifically polyclonal T cells or natural killer (NK) cells against CD123+ tumor cells, using either chimeric antigen receptor (CAR) T cells, bispecific antibodies or NK cell engager molecules, might be promising therapeutic option in the future (113–115). For that, it will be important to maximize affinity to CD123 in order to have an acceptable therapeutic effect between tumor eradication and toxicity.
Concluding remarks
Although the discovery of IL-3 dates back several decades, our knowledge on its function in immunity was quite limited, since most of the early studies focused on the hematopoietic properties of IL-3. However, recent studies have expanded our understanding of IL-3 function by identifying IL-3 as a critical orchestrator of inflammation in autoimmune diseases, cancer, and infection. Depending on the type of disease, the cell or the tissue involved, IL-3 can be either detrimental or beneficial. In addition to its role as a growth factor in cancers, IL-3 exerts its pathologic effect through the recruitment of monocytes into the inflammatory site (MS, experimental autoimmune myocarditis), the induction of inflammatory cytokines (asthma, SLE), or the stimulation of extramedullary hematopoiesis (sepsis, acute colitis). By contrast, IL-3 protects through the modulation of regulatory T cell development and migration (colitis, arthritis), the functional reprogramming of immune cells (AD, viral infection) and the early recruitment of splenic neutrophils or pDCs (early colitis, viral pneumonia). Moreover, through its ability to induce a Th2 response, IL-3 protects during helminth infection but is detrimental during asthma, protozoan infections and pancreatic cancers. Assessment of plasma IL-3 levels may therefore serve as a prognostic marker to identify patients at risk of developing severe disease. Since there are new technical possibilities to determine the plasma IL-3 value in a fast, affordable and at point-of-care manner (6), clinicians could adapt the therapeutic strategy for patients with the worst outcome. This is all the more important that genetic polymorphism of IL-3 or its receptor, which modulates IL-3 expression and signaling, has been identified as a potential factor in the pathophysiology of many diseases, such as Graves’ disease (116), schizophrenia (117, 118), atopy and asthma (119), acute kidney rejection (120) and rhinoconjunctivitis (121).
Given the impact of IL-3 in many inflammatory and infectious diseases, it would be interesting to assess if the therapies used in clinic for treating bone marrow failure or hematologic disorders may have a significant effect on the outcome of these diseases. It would be also of a potential interest to generate therapeutics with novel properties, such as dominant negative cytokines or drugs preventing either the heterodimerization of the IL-3 receptor or IL-3 expression itself. However, targeting IL-3 as a novel therapeutic approach may have ambivalent effects in certain diseases. Indeed, recent studies have highlighted that IL-3 may have a dual role depending on the course of the disease. In sepsis, IL-3 is detrimental during the acute phase by fueling the innate immune response but protects against pulmonary viral reactivation by improving antiviral defense mechanism. In colitis, IL-3 has a beneficial role at the onset of the disease by promoting the recruitment of splenic neutrophils with high microbicidal capability into the colon, whereas it has a detrimental effect during severe colitis by amplifying intestinal inflammation. Thus, it will be essential to identify in depth the cellular and molecular mechanisms associated with these dual effects for developing appropriated therapies.
For many years, IL-3 has been in the shadow of GM-CSF, but only recently has it been shown to be as important as GM-CSF in the regulation of inflammation. Nonetheless, the role of IL-3 is still elusive in many diseases, especially in solid cancers, so further research is still needed.
Author contributions
MP: Writing – original draft, Writing – review & editing. RG: Writing – review & editing. CP: Writing – review & editing. AB: Writing – original draft, Writing – review & editing.
Funding
The author(s) declare financial support was received for the research, authorship, and/or publication of this article. This work was supported by the grants of the German Research Foundation BE6981/1–1 and BE6981/4–1 (AB).
Conflict of interest
The authors declare that the research was conducted in the absence of any commercial or financial relationships that could be construed as a potential conflict of interest.
Publisher’s note
All claims expressed in this article are solely those of the authors and do not necessarily represent those of their affiliated organizations, or those of the publisher, the editors and the reviewers. Any product that may be evaluated in this article, or claim that may be made by its manufacturer, is not guaranteed or endorsed by the publisher.
References
1. McAlpine CS, Park J, Griciuc A, Kim E, Choi SH, Iwamoto Y, et al. Astrocytic interleukin-3 programs microglia and limits Alzheimer's disease. Nature. (2021) 595:701–6. doi: 10.1038/s41586-021-03734-6
2. Benard A, Mittelstadt A, Klosch B, Glanz K, Muller J, Schoen J, et al. IL-3 orchestrates ulcerative colitis pathogenesis by controlling the development and the recruitment of splenic reservoir neutrophils. Cell Rep. (2023) 42:112637. doi: 10.1016/j.celrep.2023.112637
3. Dougan M, Dranoff G, Dougan SK. GM-CSF, IL-3, and IL-5 family of cytokines: regulators of inflammation. Immunity. (2019) 50:796–811. doi: 10.1016/j.immuni.2019.03.022
4. Itoh N, Yonehara S, Schreurs J, Gorman DM, Maruyama K, Ishii A, et al. Cloning of an interleukin-3 receptor gene: a member of a distinct receptor gene family. Science. (1990) 247:324–7. doi: 10.1126/science.2404337
5. Hara T, Miyajima A. Two distinct functional high affinity receptors for mouse interleukin-3 (IL-3). EMBO J. (1992) 11:1875–84. doi: 10.1002/embj.1992.11.issue-5
6. Cho YK, Kim H, Benard A, Woo HK, Czubayko F, David P, et al. Electrochemiluminescence in paired signal electrode (ECLipse) enables modular and scalable biosensing. Sci Adv. (2022) 8:eabq4022. doi: 10.1126/sciadv.abq4022
7. Oon S, Monaghan K, Ng M, Hoi A, Morand E, Vairo G, et al. A potential association between IL-3 and type I and III interferons in systemic lupus erythematosus. Clin Transl Immunol. (2019) 8:e01097. doi: 10.1002/cti2.1097
8. Anzai A, Mindur JE, Halle L, Sano S, Choi JL, He S, et al. Self-reactive CD4(+) IL-3(+) T cells amplify autoimmune inflammation in myocarditis by inciting monocyte chemotaxis. J Exp Med. (2019) 216:369–83. doi: 10.1084/jem.20180722
9. Balam S, Schiechl-Brachner G, Buchtler S, Halbritter D, Schmidbauer K, Talke Y, et al. IL-3 triggers chronic rejection of cardiac allografts by activation of infiltrating basophils. J Immunol. (2019) 202:3514–23. doi: 10.4049/jimmunol.1801269
10. Robbins CS, Chudnovskiy A, Rauch PJ, Figueiredo JL, Iwamoto Y, Gorbatov R, et al. Extramedullary hematopoiesis generates Ly-6C(high) monocytes that infiltrate atherosclerotic lesions. Circulation. (2012) 125:364–74. doi: 10.1161/CIRCULATIONAHA.111.061986
11. Brizzi MF, Formato L, Dentelli P, Rosso A, Pavan M, Garbarino G, et al. Interleukin-3 stimulates migration and proliferation of vascular smooth muscle cells: a potential role in atherogenesis. Circulation. (2001) 103:549–54. doi: 10.1161/01.CIR.103.4.549
12. Wang M, Subramanian M, Abramowicz S, Murphy AJ, Gonen A, Witztum J, et al. Interleukin-3/granulocyte macrophage colony-stimulating factor receptor promotes stem cell expansion, monocytosis, and atheroma macrophage burden in mice with hematopoietic ApoE deficiency. Arterioscler Thromb Vasc Biol. (2014) 34:976–84. doi: 10.1161/ATVBAHA.113.303097
13. Leonard D, Eloranta ML, Hagberg N, Berggren O, Tandre K, Alm G, et al. Activated T cells enhance interferon-alpha production by plasmacytoid dendritic cells stimulated with RNA-containing immune complexes. Ann Rheum Dis. (2016) 75:1728–34. doi: 10.1136/annrheumdis-2015-208055
14. Kiss MG, Mindur JE, Yates AG, Lee D, Fullard JF, Anzai A, et al. Interleukin-3 coordinates glial-peripheral immune crosstalk to incite multiple sclerosis. Immunity. (2023) 56:1502–1514 e1508. doi: 10.1016/j.immuni.2023.04.013
15. Renner K, Hellerbrand S, Hermann F, Riedhammer C, Talke Y, Schiechl G, et al. IL-3 promotes the development of experimental autoimmune encephalitis. JCI Insight. (2016) 1:e87157. doi: 10.1172/jci.insight.87157
16. Testa U, Riccioni R, Militi S, Coccia E, Stellacci E, Samoggia P, et al. Elevated expression of IL-3Ralpha in acute myelogenous leukemia is associated with enhanced blast proliferation, increased cellularity, and poor prognosis. Blood. (2002) 100:2980–8. doi: 10.1182/blood-2002-03-0852
17. Wittwer NL, Brumatti G, Marchant C, Sandow JJ, Pudney MK, Dottore M, et al. High CD123 levels enhance proliferation in response to IL-3, but reduce chemotaxis by downregulating CXCR4 expression. Blood Adv. (2017) 1:1067–79. doi: 10.1182/bloodadvances.2016002931
18. Ehrlich LA, Chung HY, Ghobrial I, Choi SJ, Morandi F, Colla S, et al. IL-3 is a potential inhibitor of osteoblast differentiation in multiple myeloma. Blood. (2005) 106:1407–14. doi: 10.1182/blood-2005-03-1080
19. Rani L, Kumar A, Karhade J, Pandey G, Guha A, Mishra GC, et al. IL-3 regulates the differentiation of pathogenic Th17 cells. Eur J Immunol. (2022) 52:1842–58. doi: 10.1002/eji.202149674
20. Khapli SM, Mangashetti LS, Yogesha SD, Wani MR. IL-3 acts directly on osteoclast precursors and irreversibly inhibits receptor activator of NF-kappa B ligand-induced osteoclast differentiation by diverting the cells to macrophage lineage. J Immunol. (2003) 171:142–51. doi: 10.4049/jimmunol.171.1.142
21. Gupta N, Barhanpurkar AP, Tomar GB, Srivastava RK, Kour S, Pote ST, et al. IL-3 inhibits human osteoclastogenesis and bone resorption through downregulation of c-Fms and diverts the cells to dendritic cell lineage. J Immunol. (2010) 185:2261–72. doi: 10.4049/jimmunol.1000015
22. Yogesha SD, Khapli SM, Srivastava RK, Mangashetti LS, Pote ST, Mishra GC, et al. IL-3 inhibits TNF-alpha-induced bone resorption and prevents inflammatory arthritis. J Immunol. (2009) 182:361–70. doi: 10.4049/jimmunol.182.1.361
23. Kour S, Garimella MG, Shiroor DA, Mhaske ST, Joshi SR, Singh K, et al. IL-3 decreases cartilage degeneration by downregulating matrix metalloproteinases and reduces joint destruction in osteoarthritic mice. J Immunol. (2016) 196:5024–35. doi: 10.4049/jimmunol.1500907
24. Srivastava RK, Tomar GB, Barhanpurkar AP, Gupta N, Pote ST, Mishra GC, et al. IL-3 attenuates collagen-induced arthritis by modulating the development of Foxp3+ regulatory T cells. J Immunol. (2011) 186:2262–72. doi: 10.4049/jimmunol.1002691
25. Ito A, Aoyanagi N, Maki T. Regulation of autoimmune diabetes by interleukin 3-dependent bone marrow-derived cells in NOD mice. J Autoimmun. (1997) 10:331–8. doi: 10.1006/jaut.1997.0142
26. Enzler T, Gillessen S, Dougan M, Allison JP, Neuberg D, Oble DA, et al. Functional deficiencies of granulocyte-macrophage colony stimulating factor and interleukin-3 contribute to insulitis and destruction of beta cells. Blood. (2007) 110:954–61. doi: 10.1182/blood-2006-08-043786
27. Alculumbre SG, Saint-Andre V, Di Domizio J, Vargas P, Sirven P, Bost P, et al. Diversification of human plasmacytoid predendritic cells in response to a single stimulus. Nat Immunol. (2018) 19:63–75. doi: 10.1038/s41590-017-0012-z
28. Benard A, Hansen FJ, Uhle F, Klosch B, Czubayko F, Mittelstadt A, et al. Interleukin-3 protects against viral pneumonia in sepsis by enhancing plasmacytoid dendritic cell recruitment into the lungs and T cell priming. Front Immunol. (2023) 14:1140630. doi: 10.3389/fimmu.2023.1140630
29. Benard A, Jacobsen A, Brunner M, Krautz C, Klosch B, Swierzy I, et al. Interleukin-3 is a predictive marker for severity and outcome during SARS-CoV-2 infections. Nat Commun. (2021) 12:1112. doi: 10.1038/s41467-021-21310-4
30. Krammer S, Yang Z, Zimmermann T, Xepapadaki P, Geppert CI, Papadopoulos NG, et al. An immunoregulatory role of interleukin-3 in allergic asthma. Front Immunol. (2022) 13:821658. doi: 10.3389/fimmu.2022.821658
31. Kolle J, Zimmermann T, Kiefer A, Rieker RJ, Xepapadaki P, Zundler S, et al. Targeted deletion of Interleukin-3 results in asthma exacerbations. iScience. (2022) 25:104440. doi: 10.1016/j.isci.2022.104440
32. Rignault-Bricard R, Machavoine F, Mecheri S, Hermine O, Schneider E, Dy M, et al. IL-3-producing basophils are required to exacerbate airway hyperresponsiveness in a murine inflammatory model. Allergy. (2018) 73:2342–51. doi: 10.1111/all.13480
33. Lantz CS, Boesiger J, Song CH, Mach N, Kobayashi T, Mulligan RC, et al. Role for interleukin-3 in mast-cell and basophil development and in immunity to parasites. Nature. (1998) 392:90–3. doi: 10.1038/32190
34. Lantz CS, Min B, Tsai M, Chatterjea D, Dranoff G, Galli SJ. IL-3 is required for increases in blood basophils in nematode infection in mice and can enhance IgE-dependent IL-4 production by basophils. vitro Lab Invest. (2008) 88:1134–42. doi: 10.1038/labinvest.2008.88
35. Auclair SR, Roth KE, Saunders BL, Ogborn KM, Sheikh AA, Naples J, et al. Interleukin-3-deficient mice have increased resistance to blood-stage malaria. Infect Immun. (2014) 82:1308–14. doi: 10.1128/IAI.01140-13
36. Saha B, Saini A, Germond R, Perrin PJ, Harlan DM, Davis TA. Susceptibility or resistance to Leishmania infection is dictated by the macrophages evolved under the influence of IL-3 or GM-CSF. Eur J Immunol. (1999) 29:2319–29. doi: 10.1002/(ISSN)1521-4141
37. Saha B, Tonkal AM, Croft S, Roy S. Mast cells at the host-pathogen interface: host-protection versus immune evasion in leishmaniasis. Clin Exp Immunol. (2004) 137:19–23. doi: 10.1111/j.1365-2249.2004.02505.x
38. Abe T, Sugaya H, Yoshimura K. Different susceptibility to the IL-3 induced-protective effects between Strongyloides ratti and Nippostrongylus brasiliensis in C57BL/6 mice. Parasite Immunol. (1993) 15:643–5. doi: 10.1111/j.1365-3024.1993.tb00578.x
39. Kim S, Prout M, Ramshaw H, Lopez AF, LeGros G, Min B. Cutting edge: basophils are transiently recruited into the draining lymph nodes during helminth infection via IL-3, but infection-induced Th2 immunity can develop without basophil lymph node recruitment or IL-3. J Immunol. (2010) 184:1143–7. doi: 10.4049/jimmunol.0902447
40. Weber GF, Chousterman BG, He S, Fenn AM, Nairz M, Anzai A, et al. Interleukin-3 amplifies acute inflammation and is a potential therapeutic target in sepsis. Science. (2015) 347:1260–5. doi: 10.1126/science.aaa4268
41. Cohen L, David B, Cavaillon JM. Interleukin-3 enhances cytokine production by LPS-stimulated macrophages. Immunol Lett. (1991) 28:121–6. doi: 10.1016/0165-2478(91)90109-N
42. Tong Y, Lear TB, Evankovich J, Chen Y, Londino JD, Myerburg MM, et al. The RNFT2/IL-3Ralpha axis regulates IL-3 signaling and innate immunity. JCI Insight. (2020) 5(3):5. doi: 10.1172/jci.insight.133652
43. Ullrich KA, Derdau J, Baltes C, Battistella A, Rosso G, Uderhardt S, et al. IL-3 receptor signalling suppresses chronic intestinal inflammation by controlling mechanobiology and tissue egress of regulatory T cells. Gut. (2023) 72:2081–94. doi: 10.1136/gutjnl-2023-329818
44. Gomez MR, Talke Y, Hofmann C, Ketelsen I, Hermann F, Reich B, et al. Basophils control T-cell responses and limit disease activity in experimental murine colitis. Mucosal Immunol. (2014) 7:188–99. doi: 10.1038/mi.2013.38
45. De Monte L, Wormann S, Brunetto E, Heltai S, Magliacane G, Reni M, et al. Basophil recruitment into tumor-draining lymph nodes correlates with th2 inflammation and reduced survival in pancreatic cancer patients. Cancer Res. (2016) 76:1792–803. doi: 10.1158/0008-5472.CAN-15-1801-T
46. Koni M, Castellano I, Venturelli E, Sarcinella A, Lopatina T, Grange C, et al. Interleukin-3-receptor-alpha in triple-negative breast cancer (TNBC): an additional novel biomarker of TNBC aggressiveness and a therapeutic target. Cancers (Basel). (2022) 14(16):14. doi: 10.3390/cancers14163918
47. Pulaski BA, Yeh KY, Shastri N, Maltby KM, Penney DP, Lord EM, et al. Interleukin 3 enhances cytotoxic T lymphocyte development and class I major histocompatibility complex "re-presentation" of exogenous antigen by tumor-infiltrating antigen-presenting cells. Proc Natl Acad Sci U.S.A. (1996) 93:3669–74. doi: 10.1073/pnas.93.8.3669
48. Metcalf D, Begley CG, Johnson GR, Nicola NA, Lopez AF, Williamson DJ. Effects of purified bacterially synthesized murine multi-CSF (IL-3) on hematopoiesis in normal adult mice. Blood. (1986) 68:46–57. doi: 10.1182/blood.V68.1.46.bloodjournal68146
49. Kindler V, Thorens B, de Kossodo S, Allet B, Eliason JF, Thatcher D, et al. Stimulation of hematopoiesis in vivo by recombinant bacterial murine interleukin 3. Proc Natl Acad Sci U.S.A. (1986) 83:1001–5. doi: 10.1073/pnas.83.4.1001
50. Weller PF, Spencer LA. Functions of tissue-resident eosinophils. Nat Rev Immunol. (2017) 17:746–60. doi: 10.1038/nri.2017.95
51. Kita H, Ohnishi T, Okubo Y, Weiler D, Abrams JS, Gleich GJ. Granulocyte/macrophage colony-stimulating factor and interleukin 3 release from human peripheral blood eosinophils and neutrophils. J Exp Med. (1991) 174:745–8. doi: 10.1084/jem.174.3.745
52. Robinson DS, Damia R, Zeibecoglou K, Molet S, North J, Yamada T, et al. CD34(+)/interleukin-5Ralpha messenger RNA+ cells in the bronchial mucosa in asthma: potential airway eosinophil progenitors. Am J Respir Cell Mol Biol. (1999) 20:9–13. doi: 10.1165/ajrcmb.20.1.3449
53. Ebisawa M, Liu MC, Yamada T, Kato M, Lichtenstein LM, Bochner BS, et al. Eosinophil transendothelial migration induced by cytokines. II. Potentiation of eosinophil transendothelial migration by eosinophil-active cytokines. J Immunol. (1994) 152:4590–6. doi: 10.4049/jimmunol.152.9.4590
54. Rothenberg ME, Owen WF Jr., Silberstein DS, Woods J, Soberman RJ, Austen KF, et al. Human eosinophils have prolonged survival, enhanced functional properties, and become hypodense when exposed to human interleukin 3. J Clin Invest. (1988) 81:1986–92. doi: 10.1172/JCI113547
55. Schroeder JT, Chichester KL, Bieneman AP. Human basophils secrete IL-3: evidence of autocrine priming for phenotypic and functional responses in allergic disease. J Immunol. (2009) 182:2432–8. doi: 10.4049/jimmunol.0801782
56. MacDonald SM, Schleimer RP, Kagey-Sobotka A, Gillis S, Lichtenstein LM. Recombinant IL-3 induces histamine release from human basophils. J Immunol. (1989) 142:3527–32. doi: 10.4049/jimmunol.142.10.3527
57. Didichenko SA, Spiegl N, Brunner T, Dahinden CA. IL-3 induces a Pim1-dependent antiapoptotic pathway in primary human basophils. Blood. (2008) 112:3949–58. doi: 10.1182/blood-2008-04-149419
58. Hachem CE, Marschall P, Hener P, Karnam A, Bonam SR, Meyer P, et al. IL-3 produced by T cells is crucial for basophil extravasation in hapten-induced allergic contact dermatitis. Front Immunol. (2023) 14:1151468. doi: 10.3389/fimmu.2023.1151468
59. Tung R, Lichtenstein LM. In vitro histamine release from basophils of asthmatic and atopic individuals in D2O. J Immunol. (1982) 128:2067–72. doi: 10.4049/jimmunol.128.5.2067
60. Tschopp CM, Spiegl N, Didichenko S, Lutmann W, Julius P, Virchow JC, et al. Granzyme B, a novel mediator of allergic inflammation: its induction and release in blood basophils and human asthma. Blood. (2006) 108:2290–9. doi: 10.1182/blood-2006-03-010348
61. Valent P, Akin C, Hartmann K, Nilsson G, Reiter A, Hermine O, et al. Mast cells as a unique hematopoietic lineage and cell system: From Paul Ehrlich's visions to precision medicine concepts. Theranostics. (2020) 10:10743–68. doi: 10.7150/thno.46719
62. Sabbaghi F, Ullner L, Bohn T, Hahlbrock J, Bopp T, Schmitt E, et al. In activated murine mast cells, NFATc2 is critical for the production of autocrine IL-3, thereby promoting the expression of IL-9. J Immunol. (2021) 206:67–76. doi: 10.4049/jimmunol.1900310
63. Fureder W, Bankl HC, Toth J, Walchshofer S, Sperr W, Agis H, et al. Immunophenotypic and functional characterization of human tonsillar mast cells. J Leukoc Biol. (1997) 61:592–9. doi: 10.1002/jlb.61.5.592
64. Valent P, Besemer J, Sillaber C, Butterfield JH, Eher R, Majdic O, et al. Failure to detect IL-3-binding sites on human mast cells. J Immunol. (1990) 145:3432–7. doi: 10.4049/jimmunol.145.10.3432
65. Sampath P, Moideen K, Ranganathan UD, Bethunaickan R. Monocyte subsets: phenotypes and function in tuberculosis infection. Front Immunol. (2018) 9:1726. doi: 10.3389/fimmu.2018.01726
66. Borriello F, Longo M, Spinelli R, Pecoraro A, Granata F, Staiano RI, et al. IL-3 synergises with basophil-derived IL-4 and IL-13 to promote the alternative activation of human monocytes. Eur J Immunol. (2015) 45:2042–51. doi: 10.1002/eji.201445303
67. Borriello F, Iannone R, Di Somma S, Loffredo S, Scamardella E, Galdiero MR, et al. GM-CSF and IL-3 modulate human monocyte TNF-alpha production and renewal in in vitro models of trained immunity. Front Immunol. (2016) 7:680. doi: 10.4049/jimmunol.1900310
68. Buelens C, Bartholome EJ, Amraoui Z, Boutriaux M, Salmon I, Thielemans K, et al. Interleukin-3 and interferon beta cooperate to induce differentiation of monocytes into dendritic cells with potent helper T-cell stimulatory properties. Blood. (2002) 99:993–8. doi: 10.1182/blood.V99.3.993
69. Ebner S, Hofer S, Nguyen VA, Furhapter C, Herold M, Fritsch P, et al. A novel role for IL-3: human monocytes cultured in the presence of IL-3 and IL-4 differentiate into dendritic cells that produce less IL-12 and shift Th cell responses toward a Th2 cytokine pattern. J Immunol. (2002) 168:6199–207. doi: 10.4049/jimmunol.168.12.6199
70. Cardone M, Ikeda KN, Varano B, Belardelli F, Millefiorini E, Gessani S, et al. Opposite regulatory effects of IFN-beta and IL-3 on C-type lectin receptors, antigen uptake, and phagocytosis in human macrophages. J Leukoc Biol. (2014) 95:161–8. doi: 10.1189/jlb.0313168
71. Frendl G, Fenton MJ, Beller DI. Regulation of macrophage activation by IL-3. II. IL-3 and lipopolysaccharide act synergistically in the regulation of IL-1 expression. J Immunol. (1990) 144:3400–10. doi: 10.4049/jimmunol.144.9.3400
72. Jarmin DI, Nibbs RJ, Jamieson T, de Bono JS, Graham GJ. Granulocyte macrophage colony-stimulating factor and interleukin-3 regulate chemokine and chemokine receptor expression in bone marrow macrophages. Exp Hematol. (1999) 27:1735–45. doi: 10.1016/S0301-472X(99)00115-0
73. Swiecki M, Colonna M. The multifaceted biology of plasmacytoid dendritic cells. Nat Rev Immunol. (2015) 15:471–85. doi: 10.1038/nri3865
74. Grouard G, Rissoan MC, Filgueira L, Durand I, Banchereau J, Liu YJ. The enigmatic plasmacytoid T cells develop into dendritic cells with interleukin (IL)-3 and CD40-ligand. J Exp Med. (1997) 185:1101–11. doi: 10.1084/jem.185.6.1101
75. Villani AC, Satija R, Reynolds G, Sarkizova S, Shekhar K, Fletcher J, et al. Single-cell RNA-seq reveals new types of human blood dendritic cells, monocytes, and progenitors. Science. (2017) 356(6335):356. doi: 10.1126/science.aah4573
76. Niemeyer CM, Sieff CA, Mathey-Prevot B, Wimperis JZ, Bierer BE, Clark SC, et al. Expression of human interleukin-3 (multi-CSF) is restricted to human lymphocytes and T-cell tumor lines. Blood. (1989) 73:945–51. doi: 10.1182/blood.V73.4.945.bloodjournal734945
77. Renner K, Metz S, Metzger AM, Neumayer S, Schmidbauer K, Talke Y, et al. Expression of IL-3 receptors and impact of IL-3 on human T and B cells. Cell Immunol. (2018) 334:49–60. doi: 10.1016/j.cellimm.2018.09.005
78. Kumar A, Rani L, Mhaske ST, Pote ST, Behera S, Mishra GC, et al. IL-3 receptor expression on activated human th cells is regulated by IL-4, and IL-3 synergizes with IL-4 to enhance th2 cell differentiation. J Immunol. (2020) 204:819–31. doi: 10.4049/jimmunol.1801629
79. Tadmori W, Feingersh D, Clark SC, Choi YS. Human recombinant IL-3 stimulates B cell differentiation. J Immunol. (1989) 142:1950–5. doi: 10.4049/jimmunol.142.6.1950
80. Saxena SK, Crouse DA, Sharp JG. Effect of systemic interleukin-3 administration on epithelial cell proliferation in mouse intestine. Life Sci. (1993) 53:473–7. doi: 10.1016/0024-3205(93)90698-3
81. Brizzi MF, Garbarino G, Rossi PR, Pagliardi GL, Arduino C, Avanzi GC, et al. Interleukin 3 stimulates proliferation and triggers endothelial-leukocyte adhesion molecule 1 gene activation of human endothelial cells. J Clin Invest. (1993) 91:2887–92. doi: 10.1172/JCI116534
82. Dentelli P, Del Sorbo L, Rosso A, Molinar A, Garbarino G, Camussi G, et al. Human IL-3 stimulates endothelial cell motility and promotes in vivo new vessel formation. J Immunol. (1999) 163:2151–9. doi: 10.4049/jimmunol.163.4.2151
83. Barhanpurkar AP, Gupta N, Srivastava RK, Tomar GB, Naik SP, Joshi SR, et al. IL-3 promotes osteoblast differentiation and bone formation in human mesenchymal stem cells. Biochem Biophys Res Commun. (2012) 418:669–75. doi: 10.1016/j.bbrc.2012.01.074
84. Lorenzo JA, Sousa SL, Fonseca JM, Hock JM, Medlock ES. Colony-stimulating factors regulate the development of multinucleated osteoclasts from recently replicated cells. vitro J Clin Invest. (1987) 80:160–4. doi: 10.1172/JCI113042
85. Benjamin EJ, Virani SS, Callaway CW, Chamberlain AM, Chang AR, Cheng S, et al. Heart disease and stroke statistics-2018 update: a report from the american heart association. Circulation. (2018) 137:e67–e492. doi: 10.1161/CIR.0000000000000558
86. Stauss RD, Grosse GM, Neubert L, Falk CS, Jonigk D, Kuhnel MP, et al. Distinct systemic cytokine networks in symptomatic and asymptomatic carotid stenosis. Sci Rep. (2020) 10:21963. doi: 10.1038/s41598-020-78941-8
87. Basatemur GL, Jorgensen HF, Clarke MCH, Bennett MR, Mallat Z. Vascular smooth muscle cells in atherosclerosis. Nat Rev Cardiol. (2019) 16:727–44. doi: 10.1038/s41569-019-0227-9
88. Herold KC, Delong T, Perdigoto AL, Biru N, Brusko TM, Walker LSK. The immunology of type 1 diabetes. Nat Rev Immunol. (2024) 24(6):435–51. doi: 10.1038/s41577-023-00985-4
89. DeFronzo RA, Ferrannini E, Groop L, Henry RR, Herman WH, Holst JJ, et al. Type 2 diabetes mellitus. Nat Rev Dis Primers. (2015) 1:15019. doi: 10.1038/nrdp.2015.19
90. Konishi Y, Kamegai M, Takahashi K, Kunishita T, Tabira T. Production of interleukin-3 by murine central nervous system neurons. Neurosci Lett. (1994) 182:271–4. doi: 10.1016/0304-3940(94)90814-1
91. Ray S, Britschgi M, Herbert C, Takeda-Uchimura Y, Boxer A, Blennow K, et al. Classification and prediction of clinical Alzheimer's diagnosis based on plasma signaling proteins. Nat Med. (2007) 13:1359–62. doi: 10.1038/nm1653
92. Renner K, Hermann FJ, Schmidbauer K, Talke Y, Rodriguez Gomez M, Schiechl G, et al. IL-3 contributes to development of lupus nephritis in MRL/lpr mice. Kidney Int. (2015) 88:1088–98. doi: 10.1038/ki.2015.196
93. Koller DY, Wojnarowski C, Herkner KR, Weinlander G, Raderer M, Eichler I, et al. High levels of eosinophil cationic protein in wheezing infants predict the development of asthma. J Allergy Clin Immunol. (1997) 99:752–6. doi: 10.1016/S0091-6749(97)80007-3
94. Johansson MW, Kelly EA, Busse WW, Jarjour NN, Mosher DF. Up-regulation and activation of eosinophil integrins in blood and airway after segmental lung antigen challenge. J Immunol. (2008) 180:7622–35. doi: 10.4049/jimmunol.180.11.7622
95. Woolley KL, Adelroth E, Woolley MJ, Ramis I, Abrams JS, Jordana M, et al. Interleukin-3 in bronchial biopsies from nonasthmatics and patients with mild and allergen-induced asthma. Am J Respir Crit Care Med. (1996) 153:350–5. doi: 10.1164/ajrccm.153.1.8542142
96. Robinson DS, Ying S, Bentley AM, Meng Q, North J, Durham SR, et al. Relationships among numbers of bronchoalveolar lavage cells expressing messenger ribonucleic acid for cytokines, asthma symptoms, and airway methacholine responsiveness in atopic asthma. J Allergy Clin Immunol. (1993) 92:397–403. doi: 10.1016/0091-6749(93)90118-Y
97. Patil SP, Wisnivesky JP, Busse PJ, Halm EA, Li XM. Detection of immunological biomarkers correlated with asthma control and quality of life measurements in sera from chronic asthmatic patients. Ann Allergy Asthma Immunol. (2011) 106:205–13. doi: 10.1016/j.anai.2010.11.019
98. Meyer CG, Calixto Fernandes MH, Intemann CD, Kreuels B, Kobbe R, Kreuzberg C, et al. IL3 variant on chromosomal region 5q31–33 and protection from recurrent malaria attacks. Hum Mol Genet. (2011) 20:1173–81. doi: 10.1093/hmg/ddq562
99. Su CM, Wang L, Yoo D. Activation of NF-kappaB and induction of proinflammatory cytokine expressions mediated by ORF7a protein of SARS-CoV-2. Sci Rep. (2021) 11:13464. doi: 10.1038/s41598-021-92941-2
100. Abraham C, Cho JH. Inflammatory bowel disease. N Engl J Med. (2009) 361:2066–78. doi: 10.1056/NEJMra0804647
101. Redondo-Munoz J, Garcia-Pardo A, Teixido J. Molecular players in hematologic tumor cell trafficking. Front Immunol. (2019) 10:156. doi: 10.3389/fimmu.2019.00156
102. Vasiliades G, Kopanakis N, Vasiloglou M, Zografos G, Margaris H, Masselou K, et al. Role of the hematopoietic cytokines SCF, IL-3, GM-CSF and M-CSF in the diagnosis of pancreatic and ampullary cancer. Int J Biol Markers. (2012) 27:e186–194. doi: 10.5301/JBM.2012.9348
103. Mroczko B, Szmitkowski M, Wereszczynska-Siemiatkowska U, Okulczyk B. Stem cell factor (SCF) and interleukin 3 (IL-3) in the sera of patients with colorectal cancer. Dig Dis Sci. (2005) 50:1019–24. doi: 10.1007/s10620-005-2697-3
104. Ganser A, Lindemann A, Seipelt G, Ottmann OG, Herrmann F, Eder M, et al. Effects of recombinant human interleukin-3 in patients with normal hematopoiesis and in patients with bone marrow failure. Blood. (1990) 76:666–76. doi: 10.1182/blood.V76.4.666.666
105. Palmeri S, Leonardi V, Danova M, Porta C, Ferrari S, Fincato G, et al. Prospective, randomized trial of sequential interleukin-3 and granulocyte- or granulocyte-macrophage colony-stimulating factor after standard-dose chemotherapy in cancer patients. Haematologica. (1999) 84:1016–23.
106. Scadden DT, Levine JD, Bresnahan J, Gere J, McGrath J, Wang Z, et al. In vivo effects of interleukin 3 in HIV type 1-infected patients with cytopenia. AIDS Res Hum Retroviruses. (1995) 11:731–40. doi: 10.1089/aid.1995.11.731
107. Vadhan-Raj S, Papadopoulos NE, Burgess MA, Linke KA, Patel SR, Hays C, et al. Effects of PIXY321, a granulocyte-macrophage colony-stimulating factor/interleukin-3 fusion protein, on chemotherapy-induced multilineage myelosuppression in patients with sarcoma. J Clin Oncol. (1994) 12:715–24. doi: 10.1200/JCO.1994.12.4.715
108. O'Shaughnessy JA, Tolcher A, Riseberg D, Venzon D, Zujewski J, Noone M, et al. Prospective, randomized trial of 5-fluorouracil, leucovorin, doxorubicin, and cyclophosphamide chemotherapy in combination with the interleukin-3/granulocyte-macrophage colony-stimulating factor (GM-CSF) fusion protein (PIXY321) versus GM-CSF in patients with advanced breast cancer. Blood. (1996) 87:2205–11. doi: 10.1182/blood.V87.6.2205.bloodjournal8762205
109. Lee EM, Yee D, Busfield SJ, McManus JF, Cummings N, Vairo G, et al. Efficacy of an Fc-modified anti-CD123 antibody (CSL362) combined with chemotherapy in xenograft models of acute myelogenous leukemia in immunodeficient mice. Haematologica. (2015) 100:914–26. doi: 10.3324/haematol.2014.113092
110. Busfield SJ, Biondo M, Wong M, Ramshaw HS, Lee EM, Ghosh S, et al. Targeting of acute myeloid leukemia in vitro and in vivo with an anti-CD123 mAb engineered for optimal ADCC. Leukemia. (2014) 28:2213–21. doi: 10.1038/leu.2014.128
111. Aldoss I, Clark M, Song JY, Pullarkat V. Targeting the alpha subunit of IL-3 receptor (CD123) in patients with acute leukemia. Hum Vaccin Immunother. (2020) 16:2341–8. doi: 10.1080/21645515.2020.1788299
112. Syed YY. Tagraxofusp: first global approval. Drugs. (2019) 79:579–83. doi: 10.1007/s40265-019-01087-z
113. Al-Hussaini M, Rettig MP, Ritchey JK, Karpova D, Uy GL, Eissenberg LG, et al. Targeting CD123 in acute myeloid leukemia using a T-cell-directed dual-affinity retargeting platform. Blood. (2016) 127:122–31. doi: 10.1182/blood-2014-05-575704
114. Gauthier L, Virone-Oddos A, Beninga J, Rossi B, Nicolazzi C, Amara C, et al. Control of acute myeloid leukemia by a trifunctional NKp46-CD16a-NK cell engager targeting CD123. Nat Biotechnol. (2023) 41:1296–306. doi: 10.1038/s41587-022-01626-2
115. Cai T, Gouble A, Black KL, Skwarska A, Naqvi AS, Taylor D, et al. Targeting CD123 in blastic plasmacytoid dendritic cell neoplasm using allogeneic anti-CD123 CAR T cells. Nat Commun. (2022) 13:2228. doi: 10.1038/s41467-022-29669-8
116. Tu Y, Fan G, Zeng T, Cai X, Kong W. Association between polymorphism within interleukin related genes and Graves' disease: a meta-analysis of 22 case-control studies. Oncotarget. (2017) 8:98993–9002. doi: 10.18632/oncotarget.v8i58
117. Chen X, Wang X, Hossain S, O'Neill FA, Walsh D, van den Oord E, et al. Interleukin 3 and schizophrenia: the impact of sex and family history. Mol Psychiatry. (2007) 12:273–82. doi: 10.1038/sj.mp.4001932
118. Sun S, Wang F, Wei J, Cao LY, Wu GY, Lu L, et al. Association between interleukin-3 receptor alpha polymorphism and schizophrenia in the Chinese population. Neurosci Lett. (2008) 440:35–7. doi: 10.1016/j.neulet.2008.05.029
119. Park BL, Kim LH, Choi YH, Lee JH, Rhim T, Lee YM, et al. Interleukin 3 (IL3) polymorphisms associated with decreased risk of asthma and atopy. J Hum Genet. (2004) 49:517–27. doi: 10.1007/s10038-004-0184-x
120. Lee DY, Song SB, Moon JY, Jeong KH, Park SJ, Kim HJ, et al. Association between interleukin-3 gene polymorphism and acute rejection after kidney transplantation. Transplant Proc. (2010) 42:4501–4. doi: 10.1016/j.transproceed.2010.09.153
Keywords: interleukin-3, CD123, inflammation, infection, cancer
Citation: Podolska MJ, Grützmann R, Pilarsky C and Bénard A (2024) IL-3: key orchestrator of inflammation. Front. Immunol. 15:1411047. doi: 10.3389/fimmu.2024.1411047
Received: 02 April 2024; Accepted: 03 June 2024;
Published: 13 June 2024.
Edited by:
Athanasia Mouzaki, University of Patras, GreeceReviewed by:
Julio César Flores González, National Institute of Respiratory Diseases-Mexico (INER), MexicoJavier Mora, University of Costa Rica, Costa Rica
Copyright © 2024 Podolska, Grützmann, Pilarsky and Bénard. This is an open-access article distributed under the terms of the Creative Commons Attribution License (CC BY). The use, distribution or reproduction in other forums is permitted, provided the original author(s) and the copyright owner(s) are credited and that the original publication in this journal is cited, in accordance with accepted academic practice. No use, distribution or reproduction is permitted which does not comply with these terms.
*Correspondence: Alan Bénard, YWxhbi5iZW5hcmRAdWstZXJsYW5nZW4uZGU=