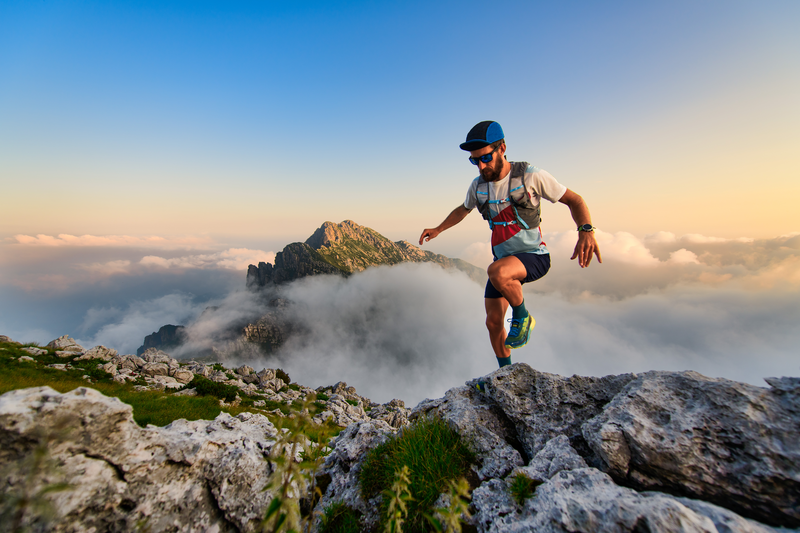
95% of researchers rate our articles as excellent or good
Learn more about the work of our research integrity team to safeguard the quality of each article we publish.
Find out more
REVIEW article
Front. Immunol. , 20 September 2024
Sec. Cytokines and Soluble Mediators in Immunity
Volume 15 - 2024 | https://doi.org/10.3389/fimmu.2024.1410273
Immunity is a critical self-defense mechanism of the human body, wherein immune cells and immune molecules play a crucial role. Extracellular vesicles (EVs), derived from immune cells or other cells, play a significant role in tumors, autoimmune diseases and other immune-related disorders by serving as carriers and facilitating intercellular communication through the transfer of cargoes. Numerous studies have revealed that EVs can exacerbate disease development by modulating immune responses. Therefore, this paper focuses on the effects of EVs on the number, activity and function of different types of immune cells and the release of immune molecules (such as cytokines, antigens, antibodies, etc) in various diseases, as well as the roles of EVs associated with different types of immune cells in various diseases. We aim to provide a comprehensive review of the negative effects that EVs play in the immune system to provide more ideas and strategies for the management of clinical immune diseases.
Extracellular vesicles (EVs) represent a diverse class of vesicles that are present in various body fluids, including plasma, urine, saliva and milk. They can be produced by almost all eukaryotic cells. EVs encompass various subtypes of cell-released membrane structures such as exosomes, microvesicles, microparticles, ectosomes, oncosomes, apoptotic bodies and many other names (1). EVs protect their contents from degradation during recycling and are capable of transporting a variety of cargoes, including proteins, lipids, sugars and nucleic acids (2). EVs can directly bind to cellular receptors or transfer their contents to target cells, thereby triggering signaling and downstream transport, and regulating a range of pathophysiological functions in target cells, such as dynamic homeostasis, induction/inhibition of inflammation and promotion of repair (3).
The human body’s health is intimately linked with its immune system. The immune system is a complex network of immune organs, immune cells and immune molecules that work together to identify and remove foreign invading antigens and mutated or senescent cells in the body, thus maintaining a stable internal environment. Any abnormalities in the composition or function of the immune system can lead to immune diseases. In recent years, increasing evidence have highlighted the significant role of EVs in the pathogenesis of immune diseases (4).
EVs play a critical role in mediating communication between immune cells and other cells, as well as maintaining homeostasis in the body through multiple actions. Firstly, EVs can maintain homeostasis by regulating T cell (Treg) function. For instance, intrapericardial-injected exosomes were absorbed by major histocompatibility complex (MHC)-II+ antigen-presenting cells, and induced Foxo3 activation via modulating PP2A/p-Akt/Foxo3 pathway. Foxo3 promoted expression and secretion of Interleukin (IL)-10, IL-33, IL-34 to establish a Treg-inducing niche in the mediastinal lymph node. Following myocardial deployment, Treg orchestrate inflammation resolution and cardiac reparation (5). Additionally, small EVs containing surface-bound IL-2 have been shown to promote the recruitment of T cells, dendritic cells and macrophages in the tumor tissue, thereby enhanced the anticancer activity of T cells (6). Secondly, EVs can exert anti-inflammatory, anti-tumor and other effects by affecting macrophage polarization. For example, mesenchymal stem cells (MSCs)-derived EVs repolarized M1 pro-inflammatory macrophages to M2 anti-inflammatory macrophages by transmitting DUSP2 and DUSP3, effectively attenuated the inflammatory response in tendinopathy (7). Additionally, MSCs-EVs delivered miR-744-5p to block glioma progression by inhibiting macrophage TGFB1 expression and M2 polarization via miR-744-5p/TGFB1/MAPK axis (8). Thirdly, interactions between EVs and neutrophils have also been shown to maintain homeostasis. In asthmatic mouse model, micrococcus luteus-derived EVs significantly attenuated neutrophilic airway inflammation by decreasing IL-1β and IL-17 production in bronchoalveolar lavage fluid and the number of group 3 innate lymphoid cells in lung tissue (9). Additionally, after partial hepatectomy, liver-specific apoptotic EVs were released into the circulation and internalized by neutrophils to promote the secretion of hepatocyte growth factor, inducing a non-inflammatory, pro-regenerative neutrophil phenotype, and promoting liver regeneration (10). Circulating neutrophils released EVs containing mitochondria that carried superoxide dismutase 2, which reduced intravascular reactive oxygen species and maintained endothelial homeostasis, and acted as an anticoagulant in sepsis (11).
Recent studies have also shown that EVs can be used for vaccine development and drug delivery. Outer membrane vesicles (OMVs), which are EVs shed from bacterial outer membrane, have demonstrated a superior safety profile and the capacity to induce a full immune response, making them an attractive candidate for the development of mucosal vaccines (12). Furthermore, OMVs have also been considered as a promising drug delivery tool due to their inherent immune response properties, their accumulation in tumors and flexibility in engineering design (13).
Although EVs exhibit a non-negligible role in maintaining homeostasis and promoting repair in the organism, a large number of studies have indicated that EVs can also contribute to the development of diseases through their modulation of immunity. Therefore, this paper aims to provide a systematic review of the effects of EVs on various aspects of immune cells, including their number, activity, function and immune molecule release, as well as the role of immune cell-associated EVs in diseases to provide a more comprehensive review of the negative effects of EVs as in the immune system to provide more ideas and strategies for the management of clinical immune diseases.
T cell is one of the major cell types in the human immune system and primarily mediates cellular immunity. Additionally, EVs play an important role as immunomodulators in the communication between T cells and other cells.
On the one hand, EVs in the context of disease exacerbate disease pathology by attenuating T-cell responses. Firstly, tumor-derived EVs have been shown to evade immune surveillance by reducing the function or number of T cells (Table 1). For example, in gastric cancer cells, lysine-specific demethylase 1 inhibited T-cell activation and proliferation via exosomal programmed cell death 1 ligand 1, promoting the development of gastric cancer (14). Invasive breast cancer cell-derived EVs transferred transforming growth factor-β type II receptor to CD8+ T cells (15, 16), while chronic myeloid leukemia cell-derived EVs to T cells (17), promoting T cell depletion, resulting in the failure of immunotherapy. Additionally, myeloma cell line MOPC315.BM-derived EVs promoted myeloma cell escape and progression by modulating the lymphoid-like cell phenotype, in which CD4+ T cells exhibited pro-tumorigenic features, increasing the expression of immune checkpoint PD-1 and CTLA-4 while decreasing the expression of CD27, and facilitating the generation of an immunosuppressive environment (18). Interestingly, CD19+ leukemia cells NALM6-derived EVs carrying CD19 antigen could activate CD19 chimeric antigen receptor-T (CAR-T) cells, and transient activation dramatically promoted the release of a variety of inflammatory cytokines, including IL-2, IL-4, interferon (IFN)-γ, IL-10, IL-6, tumor necrosis factor (TNF)-α, IL2RA, IL-13, LTA and TNFSF18. However, sustained activation caused CD19 CAR-T cells to enter a depleted state, with increased inhibitory receptors PD1 and CTLA4, and significantly increased in the markers of exhaustion CD244 and CD160, and weakened their anti-tumor activity (19). Secondly, EVs are also believed to accelerate the progression of autoimmune diseases (Table 1). (1) In terms of the number of T cells, one research has demonstrated that lncRNA HOXA transcript at the distal tip was upregulated in rheumatoid arthritis (RA) synovial fibroblast cell-derived exosomes and promoted the expression of STAT3 by inhibiting the expression of miR-1908-5p, which increased the proportion of T-helper17(Th17) cells and decreased the proportion of Treg cells in the CD4+ T cell population, leading to increased synovial inflammation and cartilage erosion (20). Similarly, Schneider et al. found that in patients with juvenile idiopathic arthritis, the release of CD73-containing EVs from activated CD8+ T cells promoted adenosine production and inhibited T cell proliferation and function, which synergized with the high ATPase activity of the Treg cells and played a key role in terminating the immune response (21). (2) In terms of the function of T cells, Sun et al. reported that exosomes harvested from the sera of mice with experimental autoimmune myocarditis induced immunometabolic dysfunction of CD4+ T cells through cargo miR-142, thus promoted disease progression (22). These findings underscore the critical role of EVs in modulating T cell responses.
On the other hand, T cells are not only the target cells of EVs, but also can secrete EVs into the target cells to alter or impair biological functions of target cells, leading to disease development. First of all, T cell-derived EVs can induce disease onset and progression by impairing the function of epithelial or endothelial cells. The Ca2+ and cAMP pathways are the major signaling systems of the secretory epithelium and control almost all secretory gland functions, and Ca2+ signaling is essential for controlling fluid and enzyme secretion from exocrine glands. It has been indicated that miR-142-3p was highly expressed in salivary glands of Sjögren’s syndrome patients, including inflammation-infiltrated T cells, which secreted exosomes containing miR-142-3p that significantly down-regulated key components of Ca2+ signaling and cAMP production in salivary gland epithelial cells—SERCA2B, RyR2 and AC9—limited cAMP production, altered calcium signaling, reduced protein production in salivary gland cells, ultimately directly impaired epithelial cell function (23). Furthermore, CD4+ T lymphocytes-derived exosomes transported MEK1/2 and ERK1/2, and activated NADPH oxidase in cardiac microvascular endothelial cells, which played a key role in the pathogenesis of angiosclerosis and coronary microcirculatory dysfunction (24). Secondly, T cell-derived EVs could also cause the disorder of the endocrine system. For instance, exosomes released from T cells contained specific microRNAs that triggered chemokine expression and apoptosis in recipient pancreatic β cells, increasing the incidence of diabetes in non-obese diabetic mice (25).
In conclusion, these studies suggested that T cells can play an immunomodulatory role through EVs regardless of whether they are the source or target cells of EVs; however, T cell subtypes are varied. The specific regulatory mechanisms between EVs and T cell subtypes in different disease settings still need more scientific exploration.
B cells stimulated by antigen can differentiate into effector B cells, which subsequently produce antibodies to mediate humoral immunity. In addition, B cells participate in immune regulation via presenting antigens and secreting cytokines.
It has been observed that when the body is in a pathological condition, EVs can release their contents into B cells, which affects the function of B cells. For example, RA is a chronic autoimmune inflammatory disease. B cells are the central component of the immune pathology of RA. Rincón-Arévalo et al. investigated the effect of medium/large EVs (m/lEVs) and m/lEVs-forming immune complexes from RA patients on B cell activation. They found that these EVs reduced the expression of activation markers, diminished calcium mobilization and decreased tyrosine phosphorylation in activated B cells in vitro (Table 1) (26). These findings suggested that EVs may contribute to the dysregulation of B cell function in RA.
In addition, mixed cryoglobulinemia is a systemic vasculitis mediated mainly by the immune complex and is the most common extrahepatic manifestation associated with hepatitis C virus (HCV), characterized by B cell proliferation and autoantibody production, in which HCV-associated exosomes have been shown to induce B cell activating factor upregulation in macrophages through activation of Toll-like receptor 7 and Nuclear factor-κB (NF-κB), accompanied by phosphorylation of the p65 subunit, stimulating B cell activation to produce excessive anti-HCV antibodies and then leading to the development of mixed cryoglobulinemia symptoms (27).
The above studies suggested that there may be a dual role for EVs in B cell activation, which meant that the effect of EVs on B cell immunoregulatory function in specific diseases remains largely unknown.
Neutrophils are the most abundant granulocyte type, and they are the main effect cells of the body against infection by extracellular pathogens and can be recruited from the blood to act at the site of inflammation of infection. In addition, interactions between EVs and neutrophils have been shown to influence immune regulation.
Lung EVs have been shown to contain protein and dsDNA components, both of which played a critical role in the recruitment of neutrophils during the early stages of bacterial infection (28). In another study, pyroptotic bodies (micron-sized EVs) were characterized in the alveolar lavage fluid during the early phases of acute lung injury. This study confirmed that the release of caspase-1-mediated pyroptosis-dependent vesicles from alveolar macrophages in the early stages of lipopolysaccharide-induced acute lung injury in mice. These EVs encapsulated several injury-associated molecular patterns—including TNF-α, IL-6, IL-1β, fibronectin, HMGB1, heat shock proteins, and s100s—promoting the activation of lung epithelial cells through the p38 MAPK signaling pathway, which led to interstitial vascular edema, recruited neutrophils and participated in uncontrolled inflammatory responses (29).
Neutrophil-derived EVs can cause tissue cell dysfunction and inflammatory chemotaxis, thereby contributing to the development of autoimmune diseases. Firstly, in terms of endothelial cell injury, activated neutrophil-derived EVs containing miR-142-3p and miR-451 have been found to target endothelial cells to trigger an inflammatory cascade reactions and induce direct vascular injury in anti-neutrophil cytoplasmic antibody-associated small vessel vasculitis (30). Moreover, the exosomes in microscopic polyangiitis have also been shown to induce endothelial damage (31). Secondly, in terms of inflammatory chemotaxis, a study by Shao et al. revealed that neutrophils from patients with generalized pustular psoriasis secreted more exosomes than those from healthy individuals, which were then rapidly internalized by keratinocytes. This resulted in the increased expression of inflammatory molecules through the activation of NF-κB and MAPK signaling pathways, exacerbating neutrophil migration and the formation of an autoinflammatory cycle in generalized pustular psoriasis (32).
Generally speaking, EVs can indeed promote the recruitment and migration of neutrophils, but to some extent, it can lead to self inflammatory cycle and cause inflammatory cascade reaction. Further research is needed to clarify the exact mechanism of neutrophil immunoregulation of EVs in diseases.
Monocytes originate from the bone marrow, circulate to the whole body through the blood, and differentiate into macrophages, which play an essential role in immune surveillance and response (33). In addition to direct cell-cell communication, monocytes and macrophages also communicate with other cells through EVs for intercellular communication. The communication mediated by these EVs can also impair immune function and contribute to the pathogenesis of various diseases (33, 34).
On the one hand, EVs have been found to modulate the phenotype and function of monocytes or macrophages, which has a significant impact on the immune function of the body (shown in Figure 1). First of all, EVs can induce the immune state of the body toward a pro-inflammatory direction, as demonstrated in Table 1. (1) In the respiratory system, It has been shown that exosomes presented in alveolar lavage fluid from patients with Lumpy disease induced elevation of IL-1β, IL-6, TNF, CCL2 and reactive oxygen species in monocytes, demonstrating the effect of exosomes on nodular inflammation (35). In addition, SARS-CoV-2 Spike transfected cells could activate microglia through exosomal miR-148a to target the Ubiquitin Specific Peptidase 33/Interferon regulatory factor 9 axis, leading to severe neuroinflammation in the central nervous system (36). (2) In the nervous system, in patients with multiple sclerosis, neurons secreted EVs containing interferon stimulated gene 15 protein. Interferon stimulated gene 15 protein directly activated microglia in a CD11b-dependent manner and promoted the production of chemokines, inflammatory factors and iNOS in microglia (37). (3) In the circulatory system, EVs secreted by trophoblast cells in the placenta of patients with preeclampsia can act as key inflammatory signals driving macrophage M1 polarization to a pro-inflammatory phenotype. It has been shown that trophoblast-derived EVs in preeclampsia women significantly up-regulated macrophage M1 gene markers, such as IL-1β, IL-6 and TNF-α, and significantly down-regulated macrophage CD163 expression compared to trophoblast-derived EVs in normal pregnant women. These results suggested that trophoblast-derived EVs in preeclampsia may be a key inflammatory signal driving macrophage polarization toward pro-inflammatory phenotypes (38). (4) In the digestive system, Crohn’s disease-related EVs were transported from intestinal epithelial cells to macrophages, which not only triggered the inflammatory response (39), but also expanded the replication of secondary bacterial infections in host cells (40). (5) In the urinary and endocrine systems, Leucine-rich α-2-glycoprotein 1-rich lipotoxic renal tubular epithelium-derived EVs induced inflammation and injury in diabetic nephropathy by activating macrophages through a TGFβR1-dependent process (41). Additionally, adipocytes delivered miR-34a to neighboring macrophages via exosomes, resulting in a shift to a pro-inflammatory M1 phenotype that exacerbated obesity-associated systemic inflammation and metabolic dysregulation (42).
Secondly, EVs have been shown to play a role in promoting tumor progression through various oncogenic mechanisms (Table 1). (1) Immunosuppression. For instance, the nasopharyngeal carcinoma cell-derived exosomes RNF126 (43), glioma cell-derived EVs miR-3184-3p (44) and miR-10b-5p (45) altered the tumor immune microenvironment and aggravated tumor progression by up-regulating the expression of M2 polarization-associated factors IL-10, TGF-β, CD163, and Arg-1, as well as down-regulated the expression of M1 polarization-associated factor TNF-α. (2) Proliferation. For example, after radiotherapy, glioblastoma cell exosomal circ_0012381 could induce microglia M2 polarization, reduce their phagocytosis and then promote tumor proliferative capacity (46). Pancreatic cancer cell exosomal FGD5-AS1 have been shown to induce M2 polarization of tumor-associated macrophages, resulting in increased proliferation, migration and invasion (47). (3) Angiogenesis. As shown by gastric cancer cell exosomal miR-519a-3p, which mediated angiogenesis by inducing M2 polarization in intrahepatic macrophages, thus promoted liver metastasis (48). (4) Anti-apoptosis. For example, melanoma cell EVs recombinant heat shock protein 90α could enhance the resistance of monocytes to apoptosis (49).
On the other hand, EVs released by monocytes or macrophages can also aggravate the severity of diseases through different mechanisms. Firstly, they can induce tissue cells damage. For example, in the exosomes secreted by macrophages treated with HIV virus protein, miR-23a and miR-27a impaired the tight junction barrier and mitochondrial function of lung epithelial cells respectively, increasing the susceptibility to alveolar infection and injury (50). Macrophage-derived EVs containing high levels of leucine-rich α-2-glycoprotein 1 (51) or tumor necrosis factor-related apoptosis-inducing ligand (41) have been shown to induce renal tubular epithelial cell injury and apoptosis via corresponding transforming growth factor β receptor 1 (51) or death receptor 5 (41) dependent processes. Secondly, EVs can enhance tumor cell resistance. For instance, the tumor-associated macrophage-derived exosomal lncMMPA promoted tumor proliferation by activating the glycolytic pathway in hepatocellular carcinoma cells (52). MiR-222-3p, an M2 macrophage-derived EVs cargo, mediated gemcitabine resistance in pancreatic cancer (53). Thirdly, EVs can also modulate other immune cells. In the animal model of Guillain-Barré syndrome, M1 macrophage-derived exosomes exacerbated the disease by directly regulating the differentiation (increased proportion) and function (increased IFN-γ intensity) of Th1 effector cells (54). Finally, EVs can inhibit precursor cell differentiation. For example, miR-98, an M1 macrophage-derived exosomal cargo, was transferred to MC3T3-E1 cells and exacerbated bone loss in osteoporosis by inhibiting osteogenic differentiation through downregulation of DUSP1 and activation of the JNK signaling pathway (55).
Although a large number of studies have shown that EVs promoted diseases progression by inducing M2 polarization of macrophages, M2 macrophages could be subdivided into four subtypes, so more future researches are needed to clarify the more accurate influence of EVs on M2 macrophage subtypes.
Immune molecules, such as cytokines, antibodies, antigens, complements, MHC and other substances, are synthesized and secreted by immune cells to regulate the immune response. Many evidences have suggested that EVs can play an immunomodulatory role by promoting the secretion or delivery of immune molecules.
Specific proteins in plasma (56) and tear-derived (57) exosomes from patients with autoimmune thyroid disease promoted the secretion of TNF-α (56) and inflammatory cytokines (IL-6, IL-8 and MCP-1) (57) respectively, activating inflammatory responses, orbital tissue remodeling and fibrosis. Plasma-derived EVs from patients with dermatomyositis triggered the release of STING phosphorylated pro-inflammatory cytokines, including IFN-β, TNF-α and IL-6, and the severity of the disease correlated with the levels of these cytokines (58). In addition, after immunization of mice with exosomes from the cerebrospinal fluid of patients with antibody-positive autoimmune encephalitis, Gu et al. showed an increase in the frequency of neuronal antigen-specific inflammatory cytokines IFN-γ and IL-17 production (59). Similarly, it has been shown that patients with minimal hepatic encephalopathy (60) and preeclampsia (61) have a pro-inflammatory state, and their circulating EVs altered the body toward a pro-inflammatory state by up-regulating the expression of pro-inflammatory cytokines IL-17, IL-21 and others.
In a study of antibody-mediated allograft rejection, it was found that plasma-derived EVs led to the upregulation of C3 gene expression and downregulation of CFH expression in renal tubular epithelial cells, and deposition of C4d in endothelial cells, these had pro-inflammatory, pro-aging, and pro-fibrotic effects (62). Another study in vivo showed that mouse skin and heart allografts as well as human skin grafts transferred cell-free donor MHC antigens to secondary lymphoid tissues via EVs, triggering upregulation of calcium fluxes and CD86 in alloreactive B cells and eliciting B-cell alloimmunity (63). Similarly, using a mouse heart transplantation model, Liu et al. found that donor dendritic cells transferred EVs with functional MHC antigens to recipient lymphoid tissues, and recipient dendritic cells were activated by acquisition of EVs and directly presented to allogeneic reactive T cells (64).These suggested that EVs may play a role in mediating allograft rejection, serve as noninvasive diagnostic biomarkers of allograft rejection, and promise potential therapeutic targets for the prevention of allograft rejection. Heart transplant patients were divided into two groups according to whether they developed rejection within 1 year: the group that developed rejection and the group that did not develop rejection. The surface protein profiles of plasma-derived EVs of the two groups were studied, which revealed that the acute cellular rejection response differentiation factors CD3, CD2, ROR1, SSEA-4, HLA-I and CD41b; and the antibody-mediated rejection differentiation factors HLA-II, CD326, CD19, CD25, CD20 (65).In renal transplantation, detection of T-cell-derived EVs in urine samples from renal transplant patients by the iKEA method demonstrated the highest differential expression of CD3 in transplant-rejected patients compared to patients with no signs of rejection on renal biopsy (66). Regarding antibody-mediated rejection, the gene combination scores of the four genes gp130, SH2D1B, TNF-α, and CCL4 were significantly higher in plasma exosomes of HLA-sensitized renal transplant recipients than in cellular rejection responders and non-rejection responders. Thus, mRNA transcriptional profiles based on the four genes mentioned above in plasma exosomes can be used to predict ongoing and/or impending antibody-mediated rejection reactions (67).
In addition, it has been found that the cerebrospinal fluid EVs of autoimmune encephalitis and herpes simplex encephalitis had different properties. Herpes simplex virus-induced exosomes played a key role in the development of herpes simplex encephalitis by delivering surface/cellular neuronal autoantigens to trigger brain autoimmunity (68).
The immune system is an interactive network of immune organs, immune cells, and immune molecules (69). The immune system disorders can occur if there is a defective or overactive immune function in the body. As important mediators of intercellular communication, the role of EVs in immune regulation has attracted much attention in recent years. Although EVs play a variety of positive effects in immune responses, the negative effects cannot be ignored as well. As mentioned earlier, several studies have demonstrated the involvement of EVs in the development of immune diseases, including tumors (14–19), autoimmune diseases (20–23), allograft rejection (62–67), and so on. Not only can non-immune cell derived EVs aggravate diseases by affecting the number and function of immune cells and the release of immune molecules, but immune cell derived EVs can also participate in the occurrence and development of diseases by suppressing immunity, promoting tissue and cell damage, and triggering excessive immune response.
Although some studies have revealed the negative role of EVs in the immune system, there is still some controversy in this area. The immunomodulatory role of EVs is very complex and has not been fully elucidated. Different sources and compositions of EVs may have different effects in the same immune environment. The function of EVs can be influenced by a variety of factors, such as source cell type, composition, the microenvironment in which they are found, time factor, the method of isolation and purification, etc. Traditional batch analysis methods often fail to dissect the inherent heterogeneity in EVs population, so various methods for single extracellular vesicle analysis have been introduced in recent years, such as nanoflow cytometry, the ExoView platform, super-resolution fluorescence imaging, single-particle dark-field imaging, single particle automated Raman trapping analysis, and so on (70). The complexity of EVs makes the reproducibility and consistency of research results a great challenge. In addition, most of the current researches on the negative effects of EVs were based on in vitro experiments or animal models. There is a lack of direct evidence for clinical applications, so how to translate laboratory research into clinical application still needs to be further explored.
Therefore, the future direction can be developed in several directions. Firstly, the development of more advanced isolation and analytical techniques to improve the purity and identification accuracy of EVs and ensure the reliability and repeatability of research results will also be an important direction for future research. Secondly, the specific action mechanism of EVs in immune regulation should be further explored, including their interactions with immune cell subtypes, signaling pathways, and functional changes at different stages of different disease states. In addition, large scale clinical studies should be conducted to verify the effectiveness and safety of EVs as biomarkers and therapeutic targets.
In conclusion, despite numerous studies exploring the mechanisms of EVs in immune diseases, their scope remains limited, and there are several disease areas and developmental mechanisms that require further in-depth and detailed investigation.
YW: Writing – original draft. CL: Writing – review & editing. FW: Writing – review & editing. JM: Writing – review & editing. JZ: Writing – review & editing. HX: Writing – review & editing. XZ: Writing – review & editing. CW: Conceptualization, Supervision, Writing – review & editing. JT: Conceptualization, Supervision, Writing – review & editing.
The author(s) declare financial support was received for the research, authorship, and/or publication of this article. This work was supported by grants from the National Natural Science Foundation of China (No. 82070758), Hunan Provincial Key R&D Program Project (No. 2020SK2084).
The authors declare that the research was conducted in the absence of any commercial or financial relationships that could be construed as a potential conflict of interest.
All claims expressed in this article are solely those of the authors and do not necessarily represent those of their affiliated organizations, or those of the publisher, the editors and the reviewers. Any product that may be evaluated in this article, or claim that may be made by its manufacturer, is not guaranteed or endorsed by the publisher.
CAR-T, Chimeric antigen receptor-T; EVs, Extracellular vesicles IFN, Interferon; IL, Interleukin; MSCs, Mesenchymal stem cells; NF-κB, Nuclear factor-κB; RA, Rheumatoid arthritis; Th, T-helper; TNF, Tumor necrosis factor; Treg, Regulatory T.
1. Théry C, Witwer KW, Aikawa E, Alcaraz MJ, Anderson JD, Andriantsitohaina R, et al. Minimal information for studies of extracellular vesicles 2018 (MISEV2018): a position statement of the international society for extracellular vesicles and update of the MISEV2014 guidelines. J Extracell Vesicles. (2018) 7:1535750. doi: 10.1080/20013078.2018.1535750
2. Gill S, Catchpole R, Forterre P. Extracellular membrane vesicles in the three domains of life and beyond. FEMS Microbiol Rev. (2019) 43:273–303. doi: 10.1093/femsre/fuy042
3. Bazzan E, Tinè M, Casara A, Biondini D, Semenzato U, Cocconcelli E, et al. Critical review of the evolution of extracellular vesicles’ knowledge: from 1946 to today. Int J Mol Sci. (2021) 22:6417. doi: 10.3390/ijms22126417
4. Zeng F, Morelli AE. Extracellular vesicle-mediated MHC cross-dressing in immune homeostasis, transplantation, infectious diseases, and cancer. Semin Immunopathol. (2018) 40:477–90. doi: 10.1007/s00281-018-0679-8
5. Zhu D, Liu S, Huang K, Wang Z, Hu S, Li J, et al. Intrapericardial exosome therapy dampens cardiac injury via activating Foxo3. Circ Res. (2022) 131:e135–50. doi: 10.1161/CIRCRESAHA.122.321384
6. Jung D, Shin S, Kang SM, Jung I, Ryu S, Noh S, et al. Reprogramming of T cell-derived small extracellular vesicles using IL2 surface engineering induces potent anti-cancer effects through miRNA delivery. J Extracell Vesicles. (2022) 11:e12287. doi: 10.1002/jev2.12287
7. Ye T, Chen Z, Zhang J, Luo L, Gao R, Gong L, et al. Large extracellular vesicles secreted by human iPSC-derived MSCs ameliorate tendinopathy via regulating macrophage heterogeneity. Bioact Mater. (2023) 21:194–208. doi: 10.1016/j.bioactmat.2022.08.007
8. Liu L, Cheng M, Zhang T, Chen Y, Wu Y, Wang Q. Mesenchymal stem cell-derived extracellular vesicles prevent glioma by blocking M2 polarization of macrophages through a miR-744-5p/TGFB1-dependent mechanism. Cell Biol Toxicol. (2022) 38:649–65. doi: 10.1007/s10565-021-09652-7
9. Sim S, Lee DH, Kim KS, Park HJ, Kim YK, Choi Y, et al. Micrococcus luteus-derived extracellular vesicles attenuate neutrophilic asthma by regulating miRNAs in airway epithelial cells. Exp Mol Med. (2023) 55:196–204. doi: 10.1038/s12276-022-00910-0
10. Brandel V, Schimek V, Göber S, Hammond T, Brunnthaler L, Schrottmaier WC, et al. Hepatectomy-induced apoptotic extracellular vesicles stimulate neutrophils to secrete regenerative growth factors. J Hepatol. (2022) 77:1619–30. doi: 10.1016/j.jhep.2022.07.027
11. Bao W, Xing H, Cao S, Long X, Liu H, Ma J, et al. Neutrophils restrain sepsis associated coagulopathy via extracellular vesicles carrying superoxide dismutase 2 in a murine model of lipopolysaccharide induced sepsis. Nat Commun. (2022) 13:4583. doi: 10.1038/s41467-022-32325-w
12. Tan K, Li R, Huang X, Liu Q. Outer membrane vesicles: current status and future direction of these novel vaccine adjuvants. Front Microbiol. (2018) 9:783. doi: 10.3389/fmicb.2018.00783
13. Suri K, D’Souza A, Huang D, Bhavsar A, Amiji M. Bacterial extracellular vesicle applications in cancer immunotherapy. Bioact Mater. (2023) 22:551–66. doi: 10.1016/j.bioactmat.2022.10.024
14. Shen DD, Pang JR, Bi YP, Zhao LF, Li YR, Zhao LJ, et al. LSD1 deletion decreases exosomal PD-L1 and restores T-cell response in gastric cancer. Mol Cancer. (2022) 21:75. doi: 10.1186/s12943-022-01557-1
15. Xie F, Zhou X, Su P, Li H, Tu Y, Du J, et al. Breast cancer cell-derived extracellular vesicles promote CD8+ T cell exhaustion via TGF-β type II receptor signaling. Nat Commun. (2022) 13:4461. doi: 10.1038/s41467-022-31250-2
16. Xie F, Zhou X, Li H, Su P, Liu S, Li R, et al. USP8 promotes cancer progression and extracellular vesicle-mediated CD8+ T cell exhaustion by deubiquitinating the TGF-β receptor TβRII. EMBO J. (2022) 41:e108791. doi: 10.15252/embj.2021108791
17. Böttcher M, Böttcher-Loschinski R, Kahlfuss S, Aigner M, Gießl A, Mackensen A, et al. CLL-derived extracellular vesicles impair T-Cell activation and foster T-Cell exhaustion via multiple immunological checkpoints. Cells. (2022) 11:2176. doi: 10.3390/cells11142176
18. Lopes R, Caetano J, Barahona F, Pestana C, Ferreira BV, Lourenço D, et al. Multiple Multiple myeloma-derived extracellular vesicles modulate the bone marrow immune microenvironment. Front Immunol. (2022) 13:909880. doi: 10.3389/fimmu.2022.909880
19. Zhu X, Hu H, Xiao Y, Li Q, Zhong Z, Yang J, et al. Tumor-derived extracellular vesicles induce invalid cytokine release and exhaustion of CD19 CAR-T Cells. Cancer Lett. (2022) 536:215668. doi: 10.1016/j.canlet.2022.215668
20. Yao X, Wang Q, Zeng P, Hou L, Yang Y, Lu D, et al. LncRNA HOTTIP from synovial fibroblast-derived exosomes: A novel molecular target for rheumatoid arthritis through the miR-1908-5p/STAT3 axis. Exp Cell Res. (2021) 409:112943. doi: 10.1016/j.yexcr.2021.112943
21. Schneider E, Winzer R, Rissiek A, Ricklefs I, Meyer-Schwesinger C, Ricklefs FL, et al. CD73-mediated adenosine production by CD8 T cell-derived extracellular vesicles constitutes an intrinsic mechanism of immune suppression. Nat Commun. (2021) 12:5911. doi: 10.1038/s41467-021-26134-w
22. Sun P, Wang N, Zhao P, Wang C, Li H, Chen Q, et al. Circulating exosomes control CD4+ T cell immunometabolic functions via the transfer of miR-142 as a novel mediator in myocarditis. Mol Ther. (2020) 28:2605–20. doi: 10.1016/j.ymthe.2020.08.015
23. Cortes-Troncoso J, Jang SI, Perez P, Hidalgo J, Ikeuchi T, Greenwell-Wild T, et al. T cell exosome-derived miR-142-3p impairs glandular cell function in Sjögren’s syndrome. JCI Insight. (2020) 5:e133497. doi: 10.1172/jci.insight.133497
24. Rolski F, Czepiel M, Tkacz K, Fryt K, Siedlar M, Kania G, et al. T lymphocyte-derived exosomes transport MEK1/2 and ERK1/2 and induce NOX4-dependent oxidative stress in cardiac microvascular endothelial cells. Oxid Med Cell Longev. (2022) 2022:2457687. doi: 10.1155/2022/2457687
25. Guay C, Kruit JK, Rome S, Menoud V, Mulder NL, Jurdzinski A, et al. Lymphocyte-derived exosomal microRNAs promote pancreatic β Cell death and may contribute to type 1 diabetes development. Cell Metab. (2019) 29:348–361.e6. doi: 10.1016/j.cmet.2018.09.011
26. Rincón-Arévalo H, Burbano C, Atehortúa L, Rojas M, Vanegas-García A, Vásquez G, et al. Modulation of B cell activation by extracellular vesicles and potential alteration of this pathway in patients with rheumatoid arthritis. Arthritis Res Ther. (2022) 24:169. doi: 10.1186/s13075−022−02837−3
27. Liao TL, Chen YM, Hsieh SL, Tang KT, Chen DY, Yang YY, et al. Hepatitis C virus-induced exosomal microRNAs and Toll-like receptor 7 polymorphism regulate B-cell activating factor. mBio. (2021) 12:e0276421. doi: 10.1128/mBio.02764-21
28. Liu B, Jin Y, Yang J, Han Y, Shan H, Qiu M, et al. Extracellular vesicles from lung tissue drive bone marrow neutrophil recruitment in inflammation. J Extracell Vesicles. (2022) 11:e12223. doi: 10.1002/jev2.12223
29. Qin X, Zhou Y, Jia C, Chao Z, Qin H, Liang J, et al. Caspase-1-mediated extracellular vesicles derived from pyroptotic alveolar macrophages promote inflammation in acute lung injury. Int J Biol Sci. (2022) 18:1521–38. doi: 10.7150/ijbs.66477
30. Glémain A, Néel M, Néel A, André-Grégoire G, Gavard J, Martinet B, et al. Neutrophil-derived extracellular vesicles induce endothelial inflammation and damage through the transfer of miRNAs. J Autoimmun. (2022) 129:102826. doi: 10.1016/j.jaut.2022.102826
31. Wang Y, Bai Y, Liu Y, Wilfried Noel S, Yan Q, Pham Thi H, et al. Plasma exosomal miRNAs involved in endothelial injury in microscopic polyangiitis patients. FASEB J. (2020) 34:6215–28. doi: 10.1096/fj.201902964R
32. Shao S, Fang H, Zhang J, Jiang M, Xue K, Ma J, et al. Neutrophil exosomes enhance the skin autoinflammation in generalized pustular psoriasis via activating keratinocytes. FASEB J. (2019) 33:6813–28. doi: 10.1096/fj.201802090RR
33. Shapouri-Moghaddam A, Mohammadian S, Vazini H, Taghadosi M, Esmaeili SA, Mardani F, et al. Macrophage plasticity, polarization, and function in health and disease. J Cell Physiol. (2018) 233:6425–40. doi: 10.1002/jcp.26429
34. Wang Y, Zhao M, Liu S, Guo J, Lu Y, Cheng J, et al. Macrophage-derived extracellular vesicles: diverse mediators of pathology and therapeutics in multiple diseases. Cell Death Dis. (2020) 11:924. doi: 10.1038/s41419-020-03127-z
35. Wahlund CJE, Gucluler Akpinar G, Steiner L, Ibrahim A, Bandeira E, Lepzien R, et al. Sarcoidosis exosomes stimulate monocytes to produce pro-inflammatory cytokines and CCL2. Sci Rep. (2020) 10:15328. doi: 10.1038/s41598-020-72067-7
36. Mishra R, Banerjea AC. SARS-CoV-2 spike targets USP33-IRF9 axis via exosomal miR-148a to activate human microglia. Front Immunol. (2021) 12:656700. doi: 10.3389/fimmu.2021.656700
37. Clarkson BDS, Grund E, David K, Johnson RK, Howe CL. ISGylation is induced in neurons by demyelination driving ISG15-dependent microglial activation. J Neuroinflammation. (2022) 19:258. doi: 10.1186/s12974-022-02618-4
38. Liu X, Fei H, Yang C, Wang J, Zhu X, Yang A, et al. Trophoblast-derived extracellular vesicles promote preeclampsia by regulating macrophage polarization. Hypertension. (2022) 79:2274–87. doi: 10.1161/HYPERTENSIONAHA.122.19244
39. Zhao F, Zheng T, Gong W, Wu J, Xie H, Li W, et al. Extracellular vesicles package dsDNA to aggravate Crohn’s disease by activating the STING pathway. Cell Death Dis. (2021) 12:815. doi: 10.1038/s41419-021-04101-z
40. Carrière J, Bretin A, Darfeuille-Michaud A, Barnich N, Nguyen HTT. Exosomes released from cells infected with Crohn’s disease-associated adherent-invasive escherichia coli activate host innate immune responses and enhance bacterial intracellular replication. Inflammation Bowel Dis. (2016) 22:516–28. doi: 10.1097/MIB.0000000000000635
41. Jiang WJ, Xu CT, Du CL, Dong JH, Xu SB, Hu BF, et al. Tubular epithelial cell-to-macrophage communication forms a negative feedback loop via extracellular vesicle transfer to promote renal inflammation and apoptosis in diabetic nephropathy. Theranostics. (2022) 12:324–39. doi: 10.7150/thno.63735
42. Pan Y, Hui X, Hoo RLC, Ye D, Chan CYC, Feng T, et al. Adipocyte-secreted exosomal microRNA-34a inhibits M2 macrophage polarization to promote obesity-induced adipose inflammation. J Clin Invest. (2019) 129:834–49. doi: 10.1172/JCI123069
43. Yu C, Xue B, Li J, Zhang Q. Tumor cell-derived exosome RNF126 affects the immune microenvironment and promotes nasopharyngeal carcinoma progression by regulating PTEN ubiquitination. Apoptosis. (2022) 27:590–605. doi: 10.1007/s10495-022-01738-9
44. Xu H, Li M, Pan Z, Zhang Z, Gao Z, Zhao R, et al. miR-3184-3p enriched in cerebrospinal fluid exosomes contributes to progression of glioma and promotes M2-like macrophage polarization. Cancer Sci. (2022) 113:2668–80. doi: 10.1111/cas.15372
45. Li B, Yang C, Zhu Z, Chen H, Qi B. Hypoxic glioma-derived extracellular vesicles harboring microRNA-10b-5p enhance M2 polarization of macrophages to promote the development of glioma. CNS Neurosci Ther. (2022) 28:1733–47. doi: 10.1111/CNS.13905
46. Zhang C, Zhou Y, Gao Y, Zhu Z, Zeng X, Liang W, et al. Radiated glioblastoma cell-derived exosomal circ_0012381 induce M2 polarization of microglia to promote the growth of glioblastoma by CCL2/CCR2 axis. J Transl Med. (2022) 20:388. doi: 10.1186/s12967-022-03607-0
47. He Z, Wang J, Zhu C, Xu J, Chen P, Jiang X, et al. Exosome-derived FGD5-AS1 promotes tumor-associated macrophage M2 polarization-mediated pancreatic cancer cell proliferation and metastasis. Cancer Lett. (2022) 548:215751. doi: 10.1016/j.canlet.2022.215751
48. Qiu S, Xie L, Lu C, Gu C, Xia Y, Lv J, et al. Gastric cancer-derived exosomal miR-519a-3p promotes liver metastasis by inducing intrahepatic M2-like macrophage-mediated angiogenesis. J Exp Clin Cancer Res CR. (2022) 41:296. doi: 10.1186/s13046-022-02499-8
49. Arkhypov I, Özbay Kurt FG, Bitsch R, Novak D, Petrova V, Lasser S, et al. HSP90α induces immunosuppressive myeloid cells in melanoma via TLR4 signaling. J Immunother Cancer. (2022) 10:e005551. doi: 10.1136/JITC-2022-005551
50. Yuan Z, Petree JR, Lee FEH, Fan X, Salaita K, Guidot DM, et al. Macrophages exposed to HIV viral protein disrupt lung epithelial cell integrity and mitochondrial bioenergetics via exosomal microRNA shuttling. Cell Death Dis. (2019) 10:580. doi: 10.1038/s41419-019-1803-y
51. Jiang W, Xu C, Xu S, Su W, Du C, Dong J, et al. Macrophage-derived, LRG1-enriched extracellular vesicles exacerbate aristolochic acid nephropathy in a TGFβR1-dependent manner. Cell Biol Toxicol. (2022) 38:629–48. doi: 10.1007/s10565-021-09666-1
52. Xu M, Zhou C, Weng J, Chen Z, Zhou Q, Gao J, et al. Tumor associated macrophages-derived exosomes facilitate hepatocellular carcinoma Malignance by transferring lncMMPA to tumor cells and activating glycolysis pathway. J Exp Clin Cancer Res. (2022) 41:253. doi: 10.1186/s13046-022-02458-3
53. Guo Y, Wu H, Xiong J, Gou S, Cui J, Peng T. miR-222-3p-containing macrophage-derived extracellular vesicles confer gemcitabine resistance via TSC1-mediated mTOR/AKT/PI3K pathway in pancreatic cancer. Cell Biol Toxicol. (2023) 39:1203–14. doi: 10.1007/s10565-022-09736-y
54. Du T, Yang CL, Ge MR, Liu Y, Zhang P, Li H, et al. M1 macrophage derived exosomes aggravate experimental autoimmune neuritis via modulating Th1 response. Front Immunol. (2020) 11:1603. doi: 10.3389/fimmu.2020.01603
55. Yu L, Hu M, Cui X, Bao D, Luo Z, Li D, et al. M1 macrophage-derived exosomes aggravate bone loss in postmenopausal osteoporosis via a microRNA-98/DUSP1/JNK axis. Cell Biol Int. (2021) 45:2452–63. doi: 10.1002/cbin.11690
56. Jia X, Zhai T, Zhang JA. Circulating exosome involves in the pathogenesis of autoimmune thyroid diseases through immunomodulatory proteins. Front Immunol. (2021) 12:730089. doi: 10.3389/fimmu.2021.730089
57. Han JS, Kim SE, Jin JQ, Park NR, Lee JY, Kim HL, et al. Tear-derived exosome proteins are increased in patients with thyroid eye disease. Int J Mol Sci. (2021) 22:1115. doi: 10.3390/ijms22031115
58. Li Y, Bax C, Patel J, Vazquez T, Ravishankar A, Bashir MM, et al. Plasma-derived DNA containing-extracellular vesicles induce STING-mediated proinflammatory responses in dermatomyositis. Theranostics. (2021) 11:7144–58. doi: 10.7150/thno.59152
59. Gu J, Jin T, Li Z, Chen H, Xia H, Xu X, et al. Exosomes expressing neuronal autoantigens induced immune response in antibody-positive autoimmune encephalitis. Mol Immunol. (2021) 131:164–70. doi: 10.1016/j.molimm.2020.12.034
60. Gallego JJ, Fiorillo A, Casanova-Ferrer F, Urios A, Ballester MP, Durbán L, et al. Plasma extracellular vesicles play a role in immune system modulation in minimal hepatic encephalopathy. Int J Mol Sci. (2022) 23:12335. doi: 10.3390/ijms232012335
61. Chen HY, Wang XY, Lu KM, Yu CH, Su MT, Kang L, et al. Maternal Th17/Treg cytokines and small extracellular vesicles in plasma as potential biomarkers for preeclampsia. Int J Med Sci. (2022) 19:1672–9. doi: 10.7150/ijms.71047
62. Franzin R, Stasi A, Sallustio F, Bruno S, Merlotti G, Quaglia M, et al. Extracellular vesicles derived from patients with antibody-mediated rejection induce tubular senescence and endothelial to mesenchymal transition in renal cells. Am J Transplant. (2022) 22:2139–57. doi: 10.1111/ajt.17097
63. Zeng F, Chen Z, Chen R, Shufesky WJ, Bandyopadhyay M, Camirand G, et al. Graft-derived extracellular vesicles transported across subcapsular sinus macrophages elicit B cell alloimmunity after transplantation. Sci Transl Med. (2021) 13:eabb0122. doi: 10.1126/scitranslmed.abb0122
64. Liu Q, Rojas-Canales DM, Divito SJ, Shufesky WJ, Stolz DB, Erdos G, et al. Donor dendritic cell-derived exosomes promote allograft-targeting immune response. J Clin Invest. (2016) 126:2805–20. doi: 10.1172/JCI84577
65. Castellani C, Burrello J, Fedrigo M, Burrello A, Bolis S, Di Silvestre D, et al. Circulating extracellular vesicles as non-invasive biomarker of rejection in heart transplant. J Heart Lung Transplant. (2020) 39:1136–48. doi: 10.1016/j.healun.2020.06.011
66. Park J, Lin HY, Assaker JP, Jeong S, Huang CH, Kurdi A, et al. Integrated kidney exosome analysis for the detection of kidney transplant rejection. ACS Nano. (2017) 11:11041–6. doi: 10.1021/acsnano.7b05083
67. Zhang H, Huang E, Kahwaji J, Nast CC, Li P, Mirocha J, et al. Plasma exosomes from HLA-sensitized kidney transplant recipients contain mRNA transcripts which predict development of antibody-mediated rejection. Transplantation. (2017) 101:2419–28. doi: 10.1097/TP.0000000000001834
68. Li Y, Gu J, Mao Y, Wang X, Li Z, Xu X, et al. Cerebrospinal fluid extracellular vesicles with distinct properties in autoimmune encephalitis and herpes simplex encephalitis. Mol Neurobiol. (2022) 59:2441–55. doi: 10.1007/s12035-021-02705-2
69. Parkin J, Cohen B. An overview of the immune system. Lancet. (2001) 357:1777–89. doi: 10.1016/S0140-6736(00)04904-7
Keywords: extracellular vesicles, T cell, B cell, neutrophil, monocyte, macrophage, immune
Citation: Wang Y, Li C, Wu F, Mao J, Zhu J, Xie H, Zhou X, Wen C and Tian J (2024) The negative effects of extracellular vesicles in the immune system. Front. Immunol. 15:1410273. doi: 10.3389/fimmu.2024.1410273
Received: 31 March 2024; Accepted: 03 September 2024;
Published: 20 September 2024.
Edited by:
Vu Ngo, City of Hope, United StatesReviewed by:
Jill Johnson, Aston University, United KingdomCopyright © 2024 Wang, Li, Wu, Mao, Zhu, Xie, Zhou, Wen and Tian. This is an open-access article distributed under the terms of the Creative Commons Attribution License (CC BY). The use, distribution or reproduction in other forums is permitted, provided the original author(s) and the copyright owner(s) are credited and that the original publication in this journal is cited, in accordance with accepted academic practice. No use, distribution or reproduction is permitted which does not comply with these terms.
*Correspondence: Chuan Wen, Y2h1YW53ZW5AY3N1LmVkdS5jbg==; Jidong Tian, dGlhbmppZG9uZ0Bjc3UuZWR1LmNu
Disclaimer: All claims expressed in this article are solely those of the authors and do not necessarily represent those of their affiliated organizations, or those of the publisher, the editors and the reviewers. Any product that may be evaluated in this article or claim that may be made by its manufacturer is not guaranteed or endorsed by the publisher.
Research integrity at Frontiers
Learn more about the work of our research integrity team to safeguard the quality of each article we publish.