- 1Department of Microbiology and Parasitology, Faculty of Pharmacy, University of Seville, Seville, Spain
- 2Health Center Amante Laffón, Seville, Spain
Background: Exposure to antigens is crucial for child immune system development, aiding disease prevention and promoting infant health. Some common food antigen proteins are found in human breast milk. However, it is unclear whether gluten antigens linked to celiac disease (CD) are transmitted through breast milk, potentially impacting the development of the infant’s immune system.
Objective: This study aimed to analyze the passage of gluten immunogenic peptides (GIP) into human breast milk. We evaluated the dynamics of GIP secretion after lactating mothers adopted a controlled gluten-rich diet.
Methods: We prospectively enrolled 96 non-CD and 23 CD lactating mothers, assessing total proteins and casein in breast milk, and GIP levels in breast milk and urine. Subsequently, a longitudinal study was conducted in a subgroup of 12 non-CD lactating mothers who adopted a controlled gluten-rich diet. GIP levels in breast milk and urine samples were assayed by multiple sample collections over 96 hours.
Results: Analysis of a single sample revealed that 24% of non-CD lactating mothers on a regular unrestricted diet tested positive for GIP in breast milk, and 90% tested positive in urine, with significantly lower concentrations in breast milk. Nevertheless, on a controlled gluten-rich diet and the collection of multiple samples, GIP were detected in 75% and 100% of non-CD participants in breast milk and urine, respectively. The transfer dynamics in breast milk samples were long-enduring and GIP secretion persisted from 0 to 72 h. In contrast, GIP secretion in urine samples was limited to the first 24 h, with inter-individual variations. In the cohort of CD mothers, 82.6% and 87% tested negative for GIP in breast milk and urine, respectively.
Conclusions: This study definitively established the presence of GIP in breast milk, with substantial inter-individual variations in secretion dynamics. Our findings provide insights into distinct GIP kinetics observed in sequentially collected breast milk and urine samples, suggesting differential gluten metabolism patterns depending on the organ or system involved. Future research is essential to understand whether GIP functions as sensitizing or tolerogenic agents in the immune system of breastfed infants.
1 Introduction
Celiac disease (CD) is a systemic disorders triggered by exposure to dietary gluten in genetically predisposed individuals (1, 2). It is a common disease that can affect individuals of all ages and is characterized by a wide spectrum of clinical manifestations. The symptoms include abdominal pain, bloating, nausea, vomiting, and/or diarrhea. Additionally, extra-intestinal manifestations can occur, such as blistering skin rashes, ataxia, bone disease, and issues in the reproductive and endocrine systems, among others (3).
The diagnostic rate for this pathology has increased over the last 10 years (4). Worldwide epidemiological data show that CD is ubiquitous, with a prevalence of 1.4% (5), and is higher in females than in males (4–8). The increase in this prevalence may, in part, be attributed to improved recognition and testing of the disease. However, there may also be a real increase in the incidence of this immune disorder, related to environmental factors that promote a loss of tolerance to dietary gluten (9). Breastfeeding and the timing of gluten introduction have been particularly considered influential factors (10). Nevertheless, the available evidence regarding the relationship between CD and breast milk remains inconclusive. Extended breastfeeding and, notably, breastfeeding at the time of gluten introduction seem to decrease the risk of developing CD or at least postpone its onset (11, 12). However, conflicting findings persist, as some studies have been unable to establish breastfeeding as protective against the development of CD (13–15).
Breastfeeding significantly influences the composition of the intestinal microbiota in the infant and offers substantial potential to prevent allergic diseases (16, 17). The study of breast milk composition is crucial not only for understanding the nutritional requirements of infants, but also for evaluating its impact on neurodevelopment, immune system maturation, and gut development, among others (18). Additionally, breastfeeding plays a dual role in promoting tolerance through the presence of immunomodulatory substances and facilitating antigen transfer. The presence of food antigens in breast milk is a significant source of early oral exposure that is critical for establishing and reinforcing oral tolerance (19).
Common food antigens found in human breast milk include proteins derived from egg (ovalbumin) (20), cow milk (casein and beta-lactoglobulin) (21), and peanuts (arah h1 and arah h2) (22, 23). The frequency and concentration of these dietary proteins in breast milk vary widely among women, with approximately 50% excreting them at concentrations ranging from 0.1 to over 1000 ng/ml (24–26). However, the presence of certain proteins/peptides including gluten remains unclear (27, 28). Gluten proteins have unique properties compared to other dietary proteins. In particular, they contain high proportions of glutamine and proline residues, making them resistant to degradation by gastrointestinal proteases. Consequently, relatively long gluten peptides with intact immunotoxic epitopes accumulate in the lumen of the small intestine and cross the epithelial barrier, ultimately entering the systemic circulation (29–31). This raises the possibility of their subsequent secretion through the mammary glands into breast milk. Therefore, the possible presence of gluten peptides in breast milk could promote oral tolerance or sensitivity to gluten in breastfed infants.
Our research group is a pioneer in detecting and analyzing gluten in human samples. In particular, monoclonal antibodies (moAbs) have been used to develop immunoassays for the sensitive and specific detection of the gluten immunogenic peptides (GIP). These assays have enabled to measure GIP concentrations in human samples, including feces and urine, confirming their absorption into the bloodstream (32–36). Given their resistance to gastrointestinal digestion, GIP play a key role in triggering immunogenic responses within T cells of individuals diagnosed with CD. However, to date, there have been no studies that confirm the existence of gluten peptides in breast milk and if they are immunogenic. On this basis, the aim of this study is to understand the transfer and secretion dynamics of GIP in breast milk. Initially, we carried out a cross-sectional study to analyze the GIP in breast milk samples within a cohort of mothers with and without CD and assessed total protein and caseins secretion. Secondly, we conducted an exploratory longitudinal clinical study to elucidate the differential kinetics of GIP secretion in human milk and urine by examining non-CD mothers after a controlled gluten-rich diet.
2 Materials and methods
2.1 Study design and participants
2.1.1 Cross-sectional study
This cross-sectional study was conducted between September 2020 and September 2023, and included lactating mothers with and without CD. Potential volunteers were invited to participate via the following official notification: Centro de Salud Amante Laffón (Sevilla, Spain), Federación de Asociaciones de Celíacos de España (FACE), Asociación Provincial de Celíacos de Sevilla (Asprocese) and social networks (https://celiacos.org/la-universidad-de-sevilla-estudia-la-presencia-de-peptidos-del-gluten-en-la-leche-materna/).
A questionnaire was administered to assess the gestational age of the children at delivery and during sample collection process. Furthermore, mothers with CD were asked about the date of CD diagnosis, results of the last duodenal biopsy study, and serum CD antibodies. The inclusion criteria for the cohort of non-CD mothers were as follows: (1) over 18 years of age; (2) regular gluten consumption; and (3) no prior diagnosis of CD, non-CD gluten sensitivity, food allergies, food intolerances, or other gastrointestinal diseases. The inclusion criteria for the cohort of mothers with CD were as follows: (1) over 18 years of age; (2) breastfeeding mothers previously diagnosed with CD, and (3) all volunteers had followed a gluten-free diet (GFD) for at least 2 years prior to enrolling in the study. The exclusion criteria were as follows: (1) participants with associated pathologies or severe psychiatric diseases; and (2) participants who did not collect the samples properly.
This study was conducted in accordance with the principles of the Declaration of Helsinki. The study protocol was approved by the regional research ethics committee (ID/Numbers: 0364-N-20, Comunidad Autónoma de Andalucía, Spain), and written informed consent was obtained from all participants.
Detailed instructions were provided to all participants at the beginning of the study. Breast milk and urine samples were consecutively collected from each participant. The parameters analyzed were total protein, casein and GIP level in breast milk, and GIP level in urine.
2.1.2 Longitudinal study
A longitudinal study was conducted in a selected subgroup of non-CD mothers who were instructed to complete a two-phase study: (1) an initial 3-day phase with a controlled gluten-rich diet and (2) a second 5-day phase with a strict GFD. During this study, participants were provided with a menu planning with foods rich in gluten (pizza, sandwich, wheat pancakes, pasta, croutons, lasagna, etc.) for the first period, and menus with gluten-free (unprocessed or minimally processed) foods for the second phase (Supplementary Figure S1). All volunteers collected consecutive urine and milk samples at 0 h during the controlled gluten-rich diet and at 3, 6, 24, 48, 72, and 96 h after the start of the GFD (Figure 1).
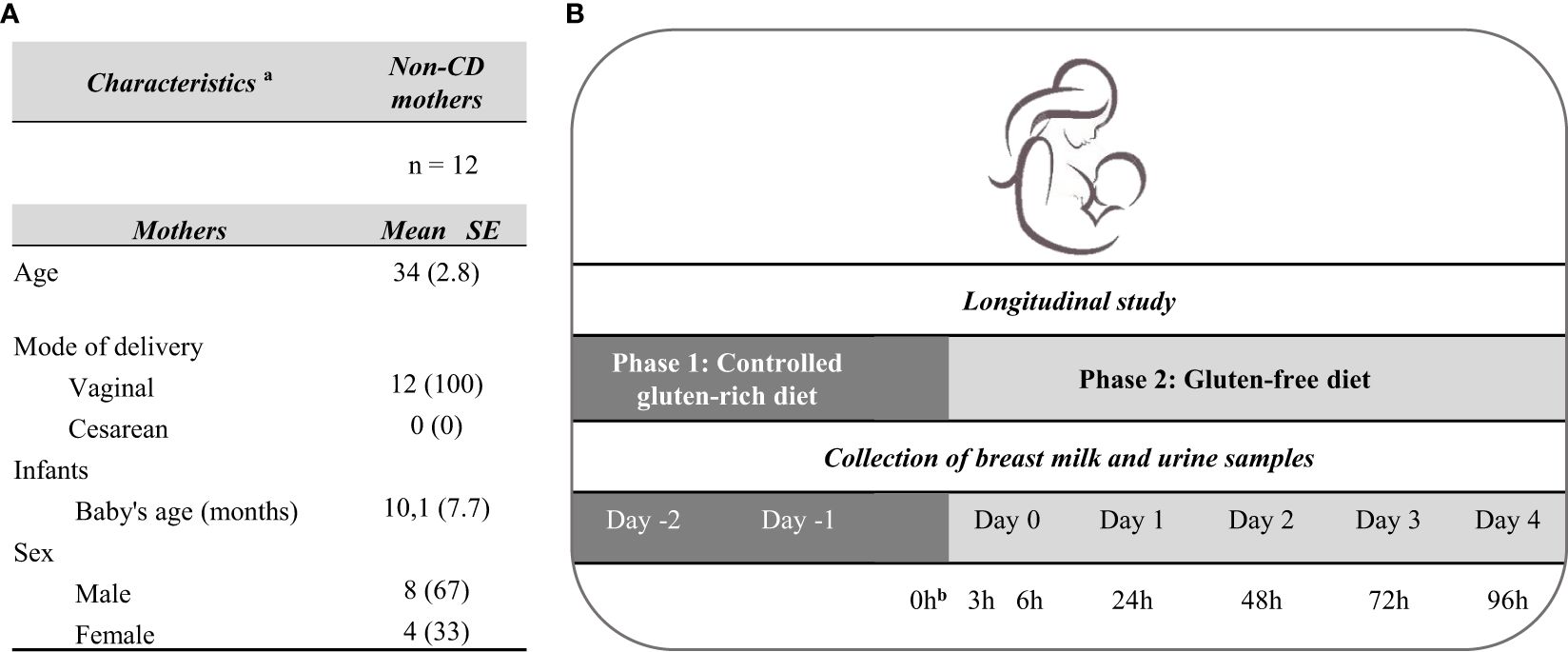
Figure 1 Characteristics of the participants and design of the longitudinal study. (A) Characteristics of the participants included (B) Study design for the determination of the kinetics of GIP secretion in breast milk and urine. aData of continuous variables are expressed as mean and standard error (SE) (in brackets), and data of categorical variables are expressed as absolute numbers and percentage (in brackets). bCollection of breast milk and urine samples after breakfast with gluten. CD, celiac disease; GFD, gluten-free diet.
2.2 Milk and urine collection
The participants were instructed to collect 10 mL of mature milk and 5 mL of urine in a sealed sterile container. Mothers expressed the milk samples manually or by pump and immediately froze the milk and urine sample at −20°C until collected by the researchers. Once in the laboratory, the samples were homogenized, and after aliquoting, they were stored at −20°C. To optimize the milk protocol, the following were used: 1) whole breast milk samples, and 2) whey samples obtained by centrifuging an aliquot of the milk sample for 5 min at 8000 x g (removal of fat content and cellular elements that could interfere with immunoassays). To ensure temporal coherence and minimize potential data variations, milk and urine samples were collected consecutively over time.
2.3 Total protein concentration
The protein concentration in the whole milk and whey was quantified using a Pierce BCA Protein Assay Kit (Thermo Fisher Scientific Inc. Waltham, Massachusetts, USA) adapted to microplates. Briefly, 25 μL of milk/whey or standard diluted was added to 96-well plates in duplicate. After covering the plates and incubating at 37°C for 30 minutes, optical densities were read at 562 nm in the Multiskan Sky Reader (Thermo Fisher Scientific Inc. Waltham, Massachusetts, USA). Each sample was participants to duplicate analyses on different dates.
2.4 Casein concentration
The casein concentration in breast milk samples was measured by sandwich-type enzyme-linked immunosorbent assay (ELISA) using the AlerTox ELISA Casein Kits (AlerTox ELISA, Hygiena Diagnostic España S.L., Seville, Spain), following the manufacturer’s guidelines with minor modifications. Briefly, breast milk samples were incubated for 15 min at 60°C with gentle agitation in extraction buffer and centrifuged. ELISA was performed using a microtiter plate and standards previously diluted 1:100. The samples were then incubated with a casein-conjugated antibody for 20 min. The TMB casein substrate solution was then added. Color development was stopped with a stop solution of casein, and absorbance at 450 nm was measured using a Multiskan Sky Reader microplate reader (Thermo Fisher Scientific Inc., Waltham, Massachusetts, USA). A minimum of two separate aliquots from each sample were examined on different days.
2.5 Determination of GIP in milk and urine samples
GIP in milk and urine were measured using a lateral flow immunoassay (LFIA) (iVYCHECK GIP Urine kit based on G12 and A1 moAbs, Biomedal S.L., Seville, Spain) following the manufacturer’s recommendations. Samples were homogenized and mixed with a conditioning solution. Thereafter, 100 µL of the mixture was added to the immunochromatographic cassette and visual interpretation of the results was carried out after 30 min. The limit of detection (LoD) was established through visual examination (2.25 ng GIP/mL), and the measuring range was 3.12–25 ng GIP/mL that was determined using the iVYCHECK Reader (Biomedal S.L.). Each sample was run in duplicate, and at least two different aliquots of each sample were tested on different days.
2.6 Statistical analysis
The results of the quantitative variables were expressed using the median and interquartile range (IQR), and those of the qualitative variables were expressed as percentages. The goodness-of-fit to normality was calculated using the Shapiro–Wilk test.
The Mann–Whitney U test was used to compare quantitative variables in independent groups, and the Wilcoxon signed-rank test was used to compare quantitative variables in dependent groups. The McNemar test was used to compare qualitative variables within dependent groups. For all the cases, P < 0.05 was considered statistically significant. The data analysis was performed using SPSS 26.0 software for Windows (SPSS Inc.).
3 Results
3.1 Participants
We recruited 96 non-CD lactating mothers taking a normal unrestricted diet with a median age of 35 (IQR: 32–37) and 23 CD lactating mothers under long-term GFD with a median age of 35 (IQR: 31–37). In non-CD mothers, the mode of delivery of the fetus was: 81% (78/96) vaginal and, 17% (16/96) cesarean. For CD mothers, the mode of delivery of the fetus was: 17% (4/23) vaginal and, 4% (1/23) cesarean. The gestational age, 40 (IQR: 39–40) weeks, was similar in both cohorts. However, the median of the baby´s age was 2 (IQR:1–5) months in non-CD mothers and 8 (IQR: 4–20) months in CD-mothers. The demographic and clinical characteristics are summarized in Table 1.
3.2 Cross-sectional study
As this was a non-interventional study, and neither the amount of fluid ingested, nor the feeding pattern of the lactating mothers was modified. Additionally, urine and breast milk samples were collected only once.
3.2.1 Determination of total protein and casein in breast milk
To evaluate whether differences in the diets of non-CD and CD mothers could affect the protein content of their breast milk, we analyzed protein concentrations before and after whey separation. The protein concentration was higher in whole milk than in whey in both cohorts (Wilcoxon test, P<0.001) (Figure 2A). In CD mothers, the median was 9.7 (IQR= 9.1–12.8) and 9 mg/mL (IQR =8–11) for whole breast milk and whey samples, respectively, while in non-CD mothers was 10.7 (IQR= 9.7–12) and 9.8 mg/mL (IQR=8.6–11.6). Protein levels values were similar between the study cohorts, with no significant differences (Mann–Whitney U test, P=0.273).
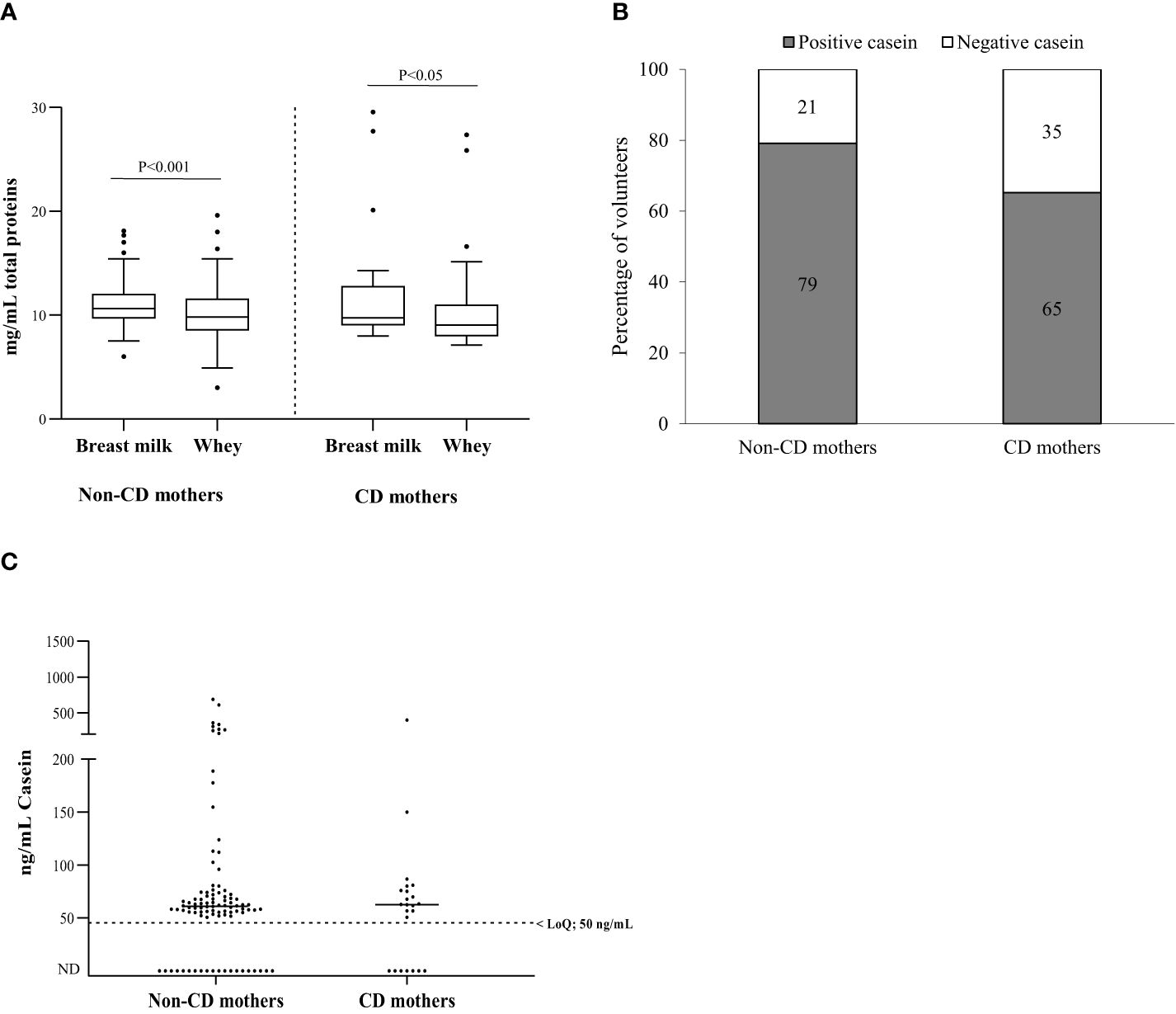
Figure 2 Total proteins and bovine caseins in breast milk. (A) Total protein content (mg/mL) in breast milk and whey (milk serum) of non-CD and CD mothers; (B) percentage of non-CD and CD mothers with casein in breast milk; (C) casein concentration in non-CD and CD mothers. Differences between groups were tested with a Wilcoxon signed-rank test in dependent groups and Mann–Whitney U test in independent groups. CD, celiac disease; LoQ, limit of quantification; ND, not detectable.
Bovine casein is one of the non-human proteins most frequently found in human breast milk (21, 37, 38). In this study, casein was detected in 78.15% of the samples (93/119) (Figure 2B), specifically 65% (16/23) in the cohort of CD mothers and 79% (76/96) in non-CD mothers (median 62.5 ng/mL; IQR 50–76 and median 60.7 ng/mL; IQR 52.2–73.4, respectively). As expected, the results showed no significant differences between the bovine casein values of the control and CD mothers (Mann–Whitney U test, P=0.797) (Figure 2C).
3.2.2 Determination of GIP in breast milk
We investigated the presence of gluten in breast milk by quantifying GIP using specific moAbs (G12/A1). In the cohort of non-CD mothers without gluten restriction in their diet, 24% (23/96) of breast milk samples contained detectable levels of GIP. There were no significant differences in the GIP levels of whole milk and whey for these mothers. Urine samples tested positive for GIP in 90% (86 out of 96) of volunteers within this cohort. The milk and urine samples were collected consecutively over time, and our findings revealed that the concentrations of GIP in the milk were significantly lower than those in the urine (average range according to the Mann–Whitney U test: 60.71 and 132.39, respectively) (P < 0.001) (Figure 3). However, 96% (22/23) of mothers who tested positive in breast milk also had a GIP positive result in urine.
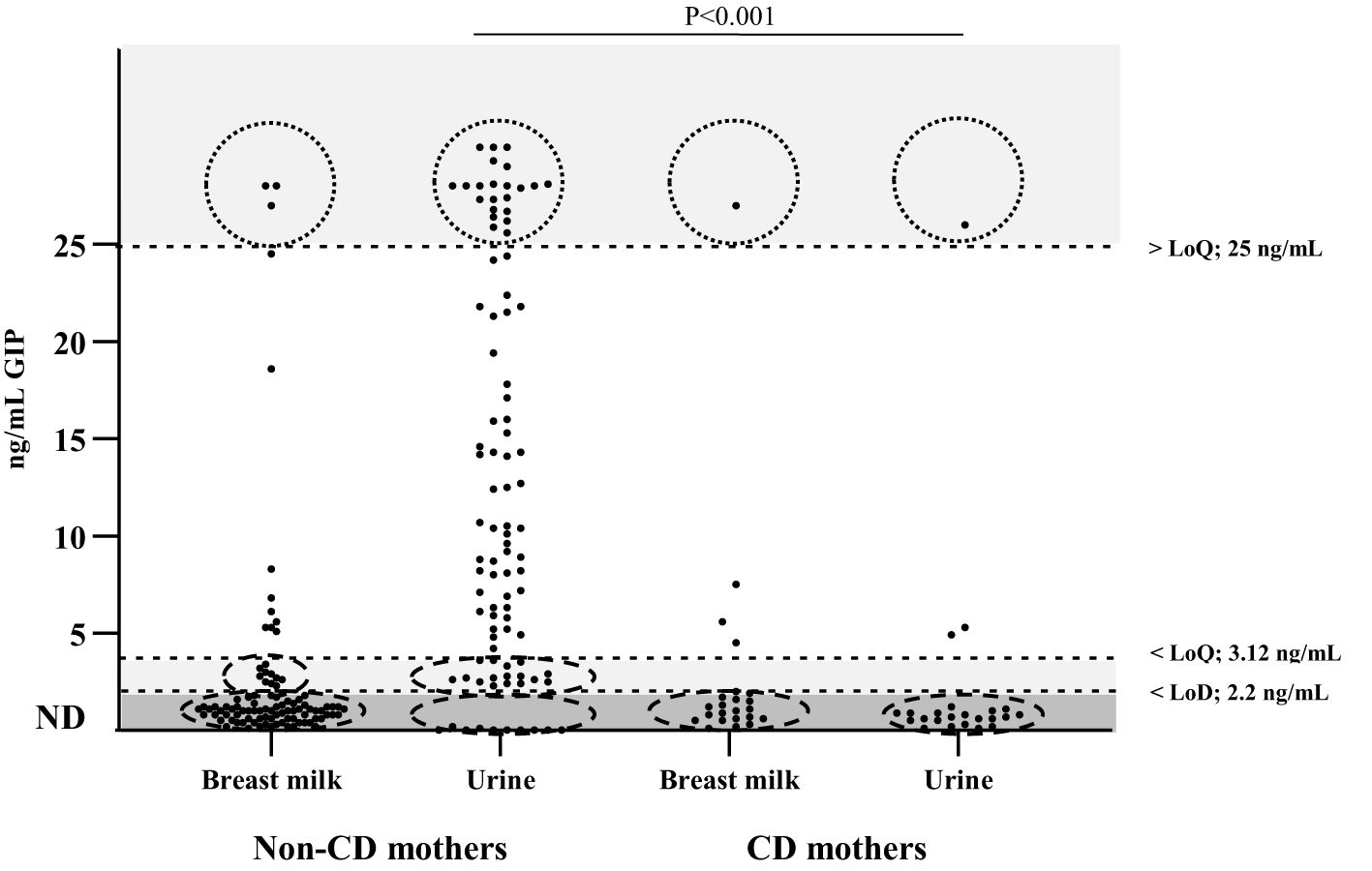
Figure 3 Detection and quantification of GIP in urine and breast milk of non-CD mothers and CD mothers. n = 96 (non-CD mothers), n = 23 (CD mothers). The data points include 2 values per volunteer. Differences in GIP levels in breast milk and urine between the 2 groups were tested with a Mann–Whitney U test. CD, celiac disease; GIP, gluten immunogenic peptides; LoD, limit of detection; LoQ, limit of quantification; ND, not detectable.
In the cohort of CD mothers, 82.6% (19/23) tested negative for GIP in breast milk. GIP were detected in only four breast milk samples and three urine samples from 23 participants, and only one of them showed significant GIP levels (> limit of quantification, LoQ) for both samples. No significant differences were found between the GIP values of the milk and urine samples (P > 0.05).
These results suggest the presence of GIP across both biological fluids, albeit at varying levels, underscoring the importance of comprehensive assessment methods in elucidating GIP dynamics in lactating mothers with CD.
3.3 Longitudinal study
3.3.1 Kinetics of GIP secretion in breast milk
A parallel longitudinal descriptive study was conducted using a subgroup of 12 volunteers from the cohort of non-CD mothers. This study aimed to elucidate GIP kinetics in breast milk after maternal ingestion of gluten. For this purpose, all volunteers were instructed to complete a two-phase study: phase 1 on a controlled gluten-rich diet, and phase 2 on a strict GFD. GIP levels were quantified in breast milk and urine samples taken at various time points, from 0 to 96 h after gluten ingestion and at the onset of the GFD. The study design and the characteristics of mothers are summarized in Figure 1.
The results were GIP positive in 75% (9/12) of the participants in breast milk samples and 100% (12/12) of the urine samples (Figure 4), considering at least one positive sample per participant. After adopting a gluten-rich diet (0 h), 54.5% (6/11) of the volunteers tested positive for GIP in their breast milk. At 3 and 6 h from the beginning of the GFD, GIP was detected in 33.3% (4/12) and 16.7% (2/12) of the volunteers, respectively. In addition, we found positive GIP in breast milk on the following hours: at 24, 48 and 72 h in 16.7% (2/12), 25% (3/12) and 8.3% (1/12) of the participants, respectively. The means GIP values detected in breast milk were similar between 0 and 48 h, however, significant differences were found after 72 h (P<0.05) (Figure 5A). No GIP was detected in breast milk after 72 h in the volunteers studied. However, the period of GIP excretion in urine after the start of GFD is limited to the first 24 h (100% of urine samples at 0h, 92% at 3 and 6 h, and 17% at 24 h) (Figure 5B).
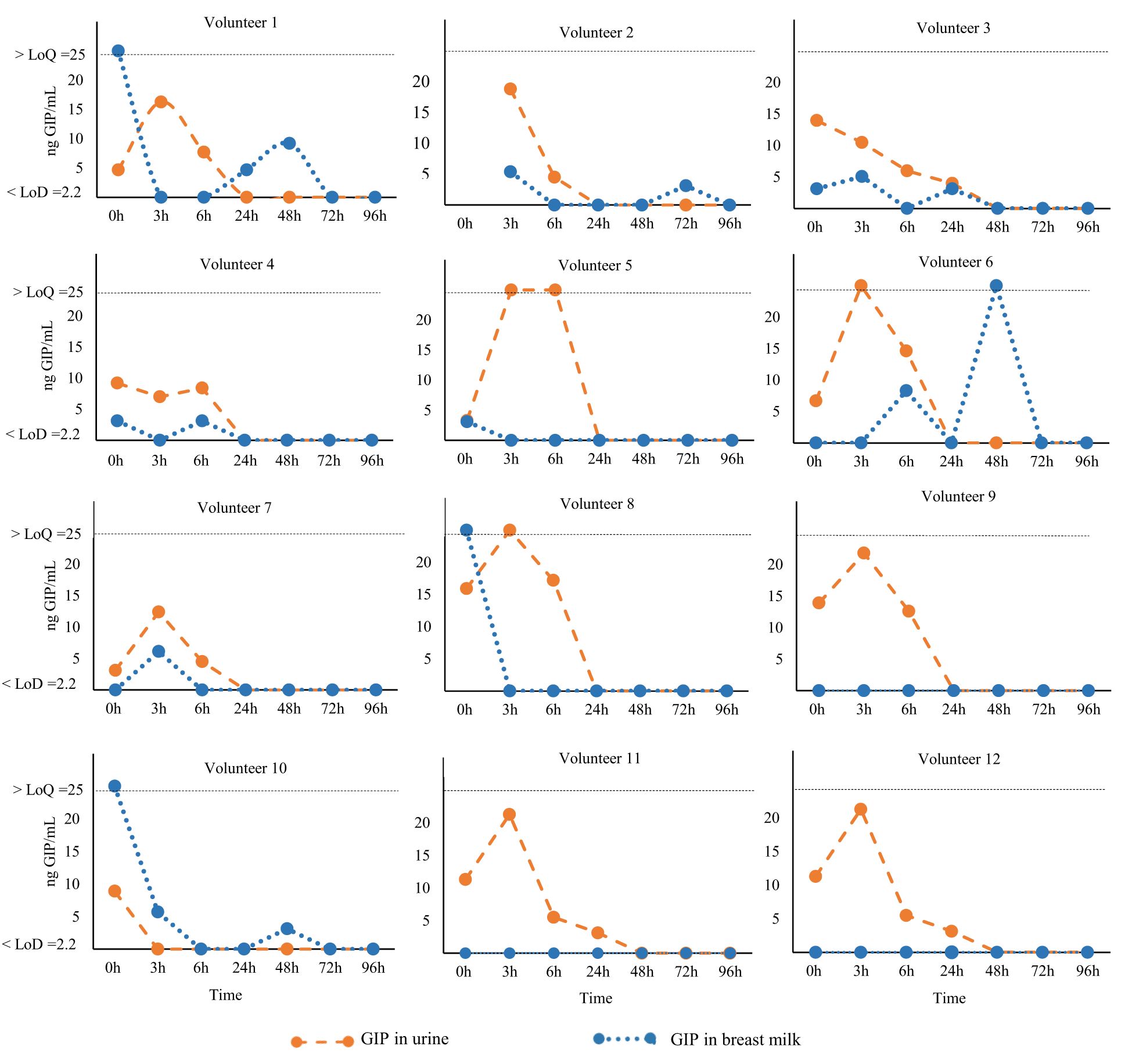
Figure 4 Kinetics of GIP secretion in breast milk and urine. Individual GIP excretion kinetics of 12 non-CD mothers on a GFD for over 96 h. The dashed orange lines correspond to breast milk samples, and blue lines represent urine samples. GFD, gluten-free diet; GIP, gluten immunogenic peptides; LoQ, limit of quantification; LoD, limit of detection.
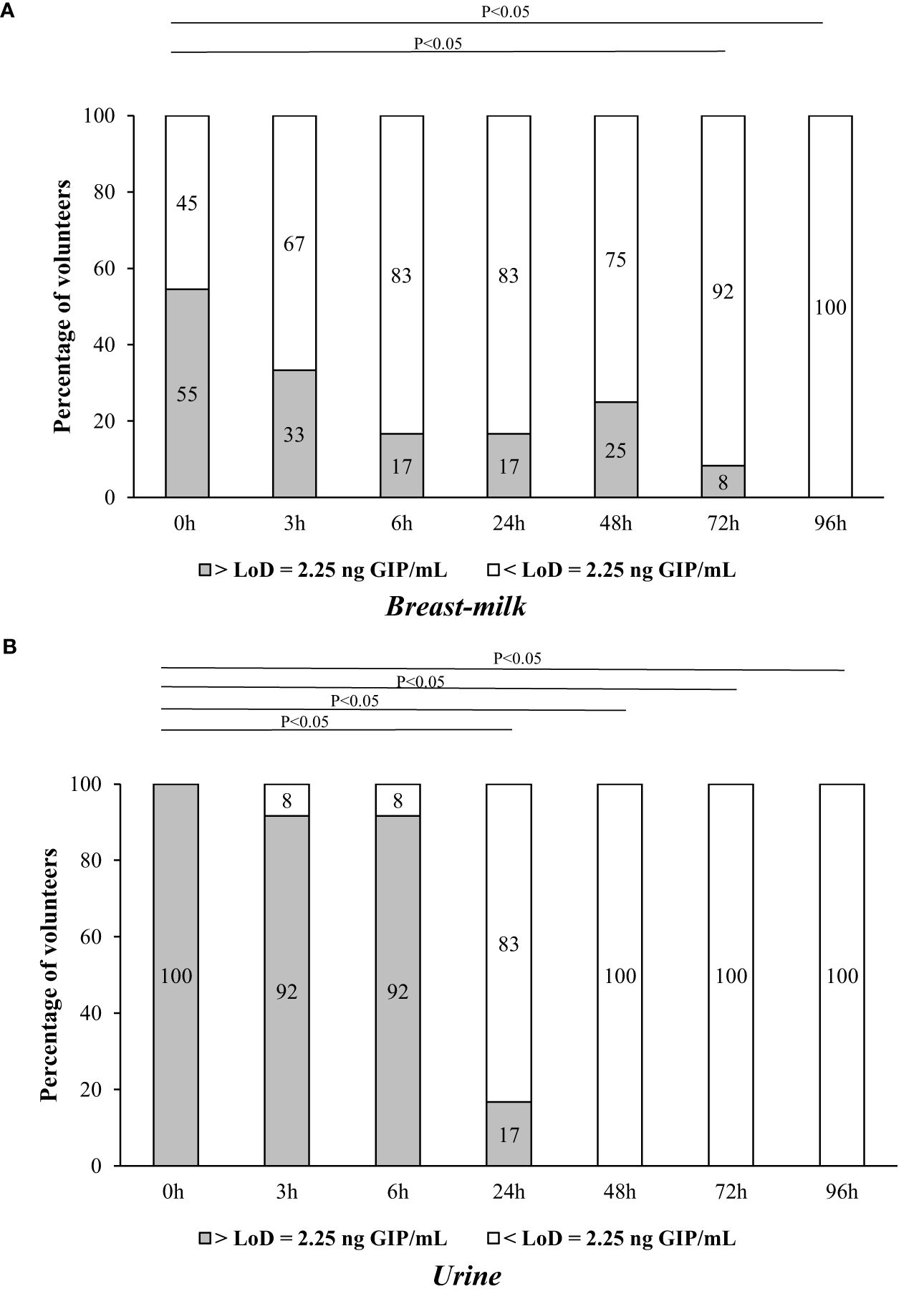
Figure 5 Comparison of GIP levels in breast milk and urine of 12 non-CD mothers on a GFD for over 96 h after gluten ingestion. (A) GIP secretion in breast milk; (B) GIP excretion in urine. Comparative analysis via the McNemar test of GIP levels before and after consumption of gluten. GIP, gluten immunogenic peptides; LoD, limit of detection.
4 Discussion
To our knowledge, this study represents the first instance of detecting immunogenic gluten peptides in breast milk samples. The presence of GIP in breast milk indicates that gluten is absorbed through the intestinal mucosa, enters the bloodstream, and is subsequently secreted by the mammary gland. The detection of measurable amounts of GIP in breast milk suggests that infants are exposed to gluten antigens before their introduction into the diet, which could potentially impact CD development and other gluten-related disorders.
Our findings reveal that the secretion of GIP in breast milk exhibits dynamic and fluctuating changes over time, likely influenced by various factors such as maternal diet, hormonal fluctuations, and the feeding patterns of the infant, among others. This dynamic nature underscores the inherent complexity involved in studying GIP in breast milk. Following a gluten-containing diet challenge and the collection of multiple breast milk samples (ensuring at least one positive sample from each participant), the percentage of GIP-positive participants was to 75%. The secretion kinetics extended from 0 to 72 hours after the gluten-containing diet challenge and the commencement of the GFD. It is important to note that a considerable degree of intra- and interindividual variation was observed in the secretion of GIP, as previously reported for other food antigens (39–42).
Chirdo et al. (28) detected undegraded gliadins in all milk samples analyzed from lactating mothers following a normal diet, using a polyclonal antibody. The concentrations of gliadins observed exceeded those reported in our study to immunogenic gluten peptides. These variations may be attributed to differences in the study populations, the identified epitopes, and the specificity of the antibodies. The polyclonal antibodies are produced from a mixture of different immune cells, and they may bind to unrelated proteins, causing cross-reactivity. This cross-reactivity can lead to false signals or interference in assays. In contrast, our study has the advantage of detecting GIP using G12 and A1 moAbs, known for their reactivity with tandem epitopes contained in the major immunogenic peptides associated with CD (36, 43).
As expected, in the cohort of mothers with CD on a GFD, the majority of the breast milk samples (82.6%, 19/23) tested negative for GIP. Only four breast milk samples tested positive for GIP. Although the mothers with CD in our study followed a GFD, the possibility of dietary lapses cannot rule out. A GFD is difficult to maintain, and adult patients with CD consume unsafe amounts of gluten on average (44–46). Therefore, these results may be attributed to possible transgressions in the GFD as no food intake control was implemented for mothers with CD.
Understanding how other proteins manifest in breast milk is fundamental it provides crucial insights into the composition of breast milk and its role in infant nutrition and development. In our study, no significant differences were detected in the total protein levels in breast milk between non-CD and CD mothers. Thus, our findings suggest that a GFD does not influence variations in the levels of this macronutrient in breast milk. These results agree with those of prior studies that have demonstrated a remarkable consistency in the macronutrient composition of breast milk across different populations despite potential variations in maternal nutritional status (47). With respect to food antigens, we also proved the transference of bovine casein into breast milk that represents one of the most commonly secreted non-human proteins (21). The levels of this protein in breast milk were in the ng/mL range, similar to those of GIP and other food antigens (48). No significant statistical differences in bovine casein levels were observed between the non-CD and CD cohorts. This result was expected, given that cow milk proteins are not only present in dairy products and their derivatives, but are also widely incorporated into a myriad of manufactured items such as bread, cold cuts, sausages, frozen fish, sweeteners, preserves, and medicines. Additionally, numerous additives used in the food industry are derived from milk (49).
The transfer of proteins from the maternal intestinal tract to the mammary gland is not yet well understood (24, 48, 50–52). Additionally, food proteins secreted into the breast milk appear at highly variable concentrations at different time points following exposure and in different forms, either as free proteins or as components of immune complexes (24). This makes it impossible to precisely predict the concentration at which food antigens/allergens will appear in breast milk and their impact on the immune response of the breast-fed children. For example, ovalbumin was detected in 68% of breast milk samples following egg ingestion, while beta-lactoglobulin was found in 62.5% of lactating women after consuming cow milk, exhibiting variable appearance and disappearance over a 15-hour sampling period. Another study observed the presence of beta-lactoglobulin in breast milk up to 7 days after oral intake of cow’s milk (37). Kinetic analysis of peanut protein transfer into breast milk revealed that 11 out of 23 mothers excreted peanut proteins, with 73% showing appearance within 1 hour of ingestion. In this work, in a cohort of non-CD mothers, taking a single sample of breast milk and urine consecutively over time, we have demonstrated that the percentage of GIP- positive milk samples was significantly lower as compared to urine samples. The detection of GIP in the urine of 90% of non-CD mothers implied that the vast majority of volunteers in this cohort consumed gluten; however, this gluten intake was not reflected in breast milk (with only 24% breast milk being GIP positive). To understand this differential excretion/secretion GIP pattern between urine and breast milk and the excretion kinetics of gluten peptides in breast milk we designed a two-phase longitudinal trial: phase 1 involved a gluten-rich diet by menu planning, and phase 2 enforced a strict GFD (Figure 1). We measured GIP levels in the breast milk at various time points, ranging from 0 to 96 h after gluten ingestion and at the commencement of a GFD. The period of GIP excretion in urine after initiating the GFD was confined to the initial 24 h, with 100% of the volunteers testing positive for GIP during this period. In contrast, the period of GIP secretion in breast milk was extended to 72 h, with 75% of volunteers showing positive GIP results. This result confirmed the differential kinetics of GIP in breast milk and urine samples collected sequentially.
Gluten proteins are initially digested by the enzymes in the mother’s intestinal tract. However, this digestion is often incomplete, leaving behind resistant peptides that can cross the intestinal barrier. It is probable that these peptides reach the mammary glands throughout the entire lactation process. We hypothesize that these peptides may undergo partial degradation before being secreted into the mammary glands, potentially extending the time of secretion. Consequently, the mammary gland could serve as a reservoir for certain proteins, including gluten. This speculation arises from observations that mothers who followed the same diet, with menu planning, exhibited fluctuating gluten secretion and interindividual differences.
In this longitudinal trial, the children were breastfed during the milk sample collection period, and this may limit the scope of our results. It should be noted that not all breast milk samples could be collected within the designated 0 to 96 h timeframe, and GIP could have been secreted in some uncollected milk samples. Pooled 24h milk samples are generally considered gold standards for collection; however, they are not possible if the mother wants to maintain normal breastfeeding behaviors (42). Furthermore, it is likely that the variations in the rate and concentration at which gluten is secreted into breast milk can be attributed to the complexity and content of meals consumed before or after gluten intake within our study group, and this could also contribute to the variations observed.
Our findings provide a conclusive demonstration of the presence of GIP in breast milk. Notable aspects of this study include the analysis of breast milk samples and the sequential analysis of urine samples using a specific assay designed for detecting GIP, coupled with the collection of multiple samples over several days. We now have the molecular tool to further investigate whether and how GIP are processed and secreted into breast milk. This investigation has raised important questions about the role that these proteins may play in the development of the immune system of infants, particularly considering that exposure to gluten in children occurs before the introduction of complementary feeding, as was previously understood. Therefore, future studies are necessary to know whether GIP act as sensitizing or tolerogenic agents in the immune system of breastfed babies.
Data availability statement
The original contributions presented in the study are included in the article/Supplementary Material. Further inquiries can be directed to the corresponding author.
Ethics statement
The studies involving humans were approved by Dictamen Único En La Comunidad Autónoma De Andalucía. The studies were conducted in accordance with the local legislation and institutional requirements. The participants provided their written informed consent to participate in this study. Written informed consent was obtained from the individual(s) for the publication of any potentially identifiable images or data included in this article.
Author contributions
ÁR-C: Conceptualization, Data curation, Formal analysis, Investigation, Methodology, Writing – original draft, Writing – review & editing, Resources. VS: Conceptualization, Data curation, Formal analysis, Investigation, Methodology, Writing – original draft, Writing – review & editing, Resources. MLM: Writing – review & editing, Funding acquisition, Project administration, Investigation. CC: Writing – review & editing, Investigation. CS: Writing – original draft, Writing – review & editing, Investigation. IC: Conceptualization, Funding acquisition, Project administration, Supervision, Writing – original draft, Writing – review & editing, Investigation, Methodology, Resources.
Funding
The author(s) declare financial support was received for the research, authorship, and/or publication of this article. This work was supported by grant from Consejería de Economía y Conocimiento, Junta de Andalucía (project P18-RT-3004) PAIDI 2018 Spain.
Acknowledgments
We would like to thank the Federation of Celiac Associations of Spain (FACE) and the Provincial Association of Celiacs of Seville (Asprocese) for their outreach to the population, as well as the volunteer mothers who enrolled in the study.
Conflict of interest
The authors declare that the research was conducted in the absence of any commercial or financial relationships that could be construed as a potential conflict of interest.
Publisher’s note
All claims expressed in this article are solely those of the authors and do not necessarily represent those of their affiliated organizations, or those of the publisher, the editors and the reviewers. Any product that may be evaluated in this article, or claim that may be made by its manufacturer, is not guaranteed or endorsed by the publisher.
Supplementary material
The Supplementary Material for this article can be found online at: https://www.frontiersin.org/articles/10.3389/fimmu.2024.1405344/full#supplementary-material
References
1. Al-Toma A, Volta U, Auricchio R, Castillejo G, Sanders DS, Cellier C, et al. European Society for the Study of Coeliac Disease (ESsCD) guideline for coeliac disease and other gluten-related disorders. United Eur Gastroenterol J. (2019) 7:583–613. doi: 10.1177/2050640619844125
2. Besser HA, Khosla C. Celiac disease: mechanisms and emerging therapeutics. Trends Pharmacol Sci. (2023) 44:949–62. doi: 10.1016/j.tips.2023.09.006
3. Caio G, Volta U, Sapone A, Leffler DA, De Giorgio R, Catassi C, et al. Celiac disease: a comprehensive current review. BMC Med. (2019) 17:142. doi: 10.1186/s12916-019-1380-z
4. King JA, Jeong J, Underwood FE, Quan J, Panaccione N, Windsor JW, et al. Incidence of celiac disease is increasing over time: a systematic review and meta-analysis. Am J Gastroenterol. (2020) 115:507–25. doi: 10.14309/ajg.0000000000000523
5. Singh P, Arora A, Strand TA, Leffler DA, Catassi C, Green PH, et al. Global prevalence of celiac disease: systematic review and meta-analysis. Clin Gastroenterol Hepatol. (2018) 16:823–36.e2. doi: 10.1016/j.cgh.2017.06.037
6. Mardini HE, Westgate P, Grigorian AY. Racial differences in the prevalence of celiac disease in the us population: national health and nutrition examination survey (NHANES) 2009-2012. Dig Dis Sci. (2015) 60:1738–42. doi: 10.1007/s10620-014-3514-7
7. Lebwohl B, Green PHR, Söderling J, Roelstraete B, Ludvigsson JF. Association between celiac disease and mortality risk in a swedish population. JAMA. (2020) 323:1277–85. doi: 10.1001/jama.2020.1943
8. Ludvigsson JF, Murray JA. Epidemiology of celiac disease. Gastroenterol Clin North Am. (2019) 48:1–18. doi: 10.1016/j.gtc.2018.09.004
9. Lebwohl B, Rubio-Tapia A. Epidemiology, presentation, and diagnosis of celiac disease. Gastroenterology. (2021) 160:63–75. doi: 10.1053/j.gastro.2020.06.098
10. Villamil E, Rodríguez-Camejo C, Puyol A, Fazio L, Colistro V, Hernández A. Immune profiling of breast milk from mothers with treated celiac disease. Pediatr Res. (2021) 89:488–95. doi: 10.1038/s41390-020-0901-y
11. Akobeng AK, Ramanan AV, Buchan I, Heller RF. Effect of breast feeding on risk of coeliac disease: a systematic review and meta-analysis of observational studies. Arch Dis Child. (2006) 91:39–43. doi: 10.1136/adc.2005.082016
12. Aronsson CA, Agardh D. Intervention strategies in early childhood to prevent celiac disease-a mini-review. Front Immunol. (2023) 14:1106564. doi: 10.3389/fimmu.2023.1106564
13. Lionetti E, Castellaneta S, Francavilla R, Pulvirenti A, Tonutti E, Amarri S, et al. Introduction of gluten, HLA status, and the risk of celiac disease in children. N Engl J Med. (2014) 371:1295–303. doi: 10.1056/NEJMoa1400697
14. Vriezinga SL, Auricchio R, Bravi E, Castillejo G, Chmielewska A, Crespo-Escobar P, et al. Randomized feeding intervention in infants at high risk for celiac disease. N Engl J Med. (2014) 371:1304–15. doi: 10.1056/NEJMoa1404172
15. Silano M, Agostoni C, Sanz Y, Guandalini S. Infant feeding and risk of developing celiac disease: a systematic review. BMJ Open. (2016) 6:e009163. doi: 10.1136/bmjopen-2015-009163
16. Borba V, Sharif K, Shoenfeld Y. Breastfeeding and autoimmunity: Programing health from the beginning. Am J Reprod Immunol. (2018) 79(1). doi: 10.1111/aji.12778
17. van den Elsen LWJ, Garssen J, Burcelin R, Verhasselt V. shaping the gut microbiota by breastfeeding: the gateway to allergy prevention? Front Pediatr. (2019) 7:47. doi: 10.3389/fped.2019.00047
18. Samuel TM, Zhou Q, Giuffrida F, Munblit D, Verhasselt V, Thakkar SK. Nutritional and non-nutritional composition of human milk is modulated by maternal, infant, and methodological factors. Front Nutr. (2020) 7:576133. doi: 10.3389/fnut.2020.576133
19. Nuzzi G, Di Cicco ME, Peroni DG. Breastfeeding and allergic diseases: What's new? Children (Basel). (2021) 8:330. doi: 10.3390/children8050330
20. Kosmeri C, Rallis D, Kostara M, Siomou E, Tsabouri S. Characteristics of exogenous allergen in breast milk and their impact on oral tolerance induction. Front Pediatr. (2022) 10:830718. doi: 10.3389/fped.2022.830718
21. Franco C, Fente C, Sánchez C, Lamas A, Cepeda A, Leis R, et al. Cow's milk antigens content in human milk: a scoping review. Foods. (2022) 11:1783. doi: 10.3390/foods11121783
22. Bernard H, Ah-Leung S, Drumare MF, Feraudet-Tarisse C, Verhasselt V, Wal JM, et al. Peanut allergens are rapidly transferred in human breast milk and can prevent sensitization in mice. Allergy. (2014) 69:888–97. doi: 10.1111/all.12411
23. Vadas P, Wai Y, Burks W, Perelman B. Detection of peanut allergens in breast milk of lactating women. JAMA. (2001) 285:1746–8. doi: 10.1001/jama.285.13.1746
24. Macchiaverni P, Tulic MK, Verhasselt V. Antigens in breast milk: possible impact on immune system education. In: Handbook of dietary and nutritional aspects of human breast milk, vol. 5. Wageningen Academic, Leiden, The Netherlands (2013). p. 447–60. doi: 10.3920/978-90-8686-764-6_25
25. Palmer DJ, Gold MS, Makrides M. Effect of cooked and raw egg consumption on ovalbumin content of human milk: a randomized, double-blind, cross-over trial. Clin Exp Allergy. (2005) 35:173–8. doi: 10.1111/j.1365-2222.2005.02170.x
26. Fukushima Y, Kawata Y, Onda T, Kitagawa M. Consumption of cow milk and egg by lactating women and the presence of beta-lactoglobulin and ovalbumin in breast milk. Am J Clin Nutr. (1997) 65:30–5. doi: 10.1093/ajcn/65.1.30
27. Troncone R, Scarcella A, Donatiello A, Cannataro P, Tarabuso A, Auricchio S. Passage of gliadin into human breast milk. Acta Paediatr Scand. (1987) 76:453–6. doi: 10.1111/j.1651-2227.1987.tb10498.x
28. Chirdo FG, Rumbo M, Añón MC, Fossati CA. Presence of high levels of non-degraded gliadin in breast milk from healthy mothers. Scand J Gastroenterol. (1998) 33:1186–92. doi: 10.1080/00365529850172557
29. Shan L, Molberg Ø, Parrot I, Hausch F, Filiz F, Gray GM, et al. Structural basis for gluten intolerance in celiac sprue. Science. (2002) 297:2275–9. doi: 10.1126/science.1074129
30. Heyman M, Abed J, Lebreton C, Cerf-Bensussan N. Intestinal permeability in coeliac disease: insight into mechanisms and relevance to pathogenesis. Gut. (2012) 61:1355–64. doi: 10.1136/gutjnl-2011-300327
31. Palanski BA, Weng N, Zhang L, Hilmer AJ, Fall LA, Swaminathan K, et al. An efficient urine peptidomics workflow identifies chemically defined dietary gluten peptides from patients with celiac disease. Nat Commun. (2022) 13:888. doi: 10.1038/s41467-022-28353-1
32. Comino I, Real A, Vivas S, Síglez MÁ, Caminero A, Nistal E, et al. Monitoring of gluten-free diet compliance in celiac patients by assessment of gliadin 33-mer equivalent epitopes in feces. Am J Clin Nutr. (2012) 95:670–7. doi: 10.3945/ajcn.111.026708
33. Comino I, Fernández-Bañares F, Esteve M, Ortigosa L, Castillejo G, Fombuena B, et al. Fecal gluten peptides reveal limitations of serological tests and food questionnaires for monitoring gluten-free diet in celiac disease patients. Am J Gastroenterol. (2016) 111:1456–65. doi: 10.1038/ajg.2017.110
34. Comino I, Segura V, Ortigosa L, Espín B, Castillejo G, Garrote JA, et al. Prospective longitudinal study: Use of faecal gluten immunogenic peptides to monitor children diagnosed with coeliac disease during transition to a gluten-free diet. Aliment Pharmacol Ther. (2019) 49:1484–92. doi: 10.1111/apt.15277
35. Moreno M, Cebolla Á, Muñoz-Suano A, Carrillo-Carrion C, Comino I, Pizarro Á, et al. Detection of gluten immunogenic peptides in the urine of patients with coeliac disease reveals transgressions in the gluten-free diet and incomplete mucosal healing. Gut. (2017) 66:250–7. doi: 10.1136/gutjnl-2015-310148
36. Ruiz-Carnicer Á, Garzón-Benavides M, Fombuena B, Segura V, García-Fernández F, Sobrino-Rodríguez S, et al. Negative predictive value of the repeated absence of gluten immunogenic peptides in the urine of treated celiac patients in predicting mucosal healing: new proposals for follow-up in celiac disease. Am J Clin Nutr. (2020) 112:1240–51. doi: 10.1093/ajcn/nqaa188
37. Picariello G, De Cicco M, Nocerino R, Paparo L, Mamone G, Addeo F, et al. Excretion of dietary cow's milk derived peptides into breast milk. Front Nutr. (2019) 6:25. doi: 10.3389/fnut.2019.00025
38. Dekker PM, Boeren S, Wijga AH, Koppelman GH, Vervoort JJM, Hettinga KA. Maternal allergy and the presence of nonhuman proteinaceous molecules in human milk. Nutrients. (2020) 12:1169. doi: 10.3390/nu12041169
39. Daly SE, Di Rosso A, Owens RA, Hartmann PE. Degree of breast emptying explains changes in the fat content, but not fatty acid composition, of human milk. Exp Physiol. (1993) 78:741–55. doi: 10.1113/expphysiol.1993.sp003722
40. Ruel MT, Dewey KG, Martínez C, Flores R, Brown KH. Validation of single daytime samples of human milk to estimate the 24-h concentration of lipids in urban Guatemalan mothers. Am J Clin Nutr. (1997) 65:439–44. doi: 10.1093/ajcn/65.2.439
41. Kent JC, Mitoulas LR, Cregan MD, Ramsay DT, Doherty DA, Hartmann PE. Volume and frequency of breastfeedings and fat content of breast milk throughout the day. Pediatrics. (2006) 117:e387–395. doi: 10.1542/peds.2005-1417
42. Keikha M, Bahreynian M, Saleki M, Kelishadi R. Macro- and micronutrients of human milk composition: are they related to maternal diet? A comprehensive systematic review. Breastfeed Med. (2017) 12:517–27. doi: 10.1089/bfm.2017.0048
43. Morón B, Bethune MT, Comino I, Manyani H, Ferragud M, López MC, et al. Toward the assessment of food toxicity for celiac patients: characterization of monoclonal antibodies to a main immunogenic gluten peptide. PloS One. (2008) 3:e2294. doi: 10.1371/journal.pone.0002294
44. Silvester JA, Comino I, Kelly CP, Sousa C, Duerksen DR, DOGGIE BAG Study Group. Most patients with celiac disease on gluten-free diets consume measurable amounts of gluten. Gastroenterology. (2020) 158:1497–99.e1. doi: 10.1053/j.gastro.2019.12.016
45. Silvester JA, Comino I, Rigaux LN, Segura V, Green KH, Cebolla A, et al. Exposure sources, amounts and time course of gluten ingestion and excretion in patients with coeliac disease on a gluten-free diet. Aliment Pharmacol Ther. (2020) 52:1469–79. doi: 10.1111/apt.16075
46. Wieser H, Ruiz-Carnicer Á, Segura V, Comino I, Sousa C. Challenges of Monitoring the gluten-free diet adherence in the management and follow-up of patients with celiac disease. Nutrients. (2021) 13:2274. doi: 10.3390/nu13072274
47. Fields DA, Demerath EW. Human milk composition: nutrients and bioactive factors. Pediatr Clin North Am. (2013) 60:49–74. doi: 10.1016/j.pcl.2012.10.002
48. Schocker F, Jappe U. Breastfeeding: maternally transferred allergens in breast milk: protective or sensitizing? Mol Nutr Food Res. (2022) 66:e2200066. doi: 10.1002/mnfr.202200066
49. Al-Beltagi M, Saeed NK, Bediwy AS, Elbeltagi R. Cow's milk-induced gastrointestinal disorders: From infancy to adulthood. World J Clin Pediatr. (2022) 11:437–54. doi: 10.5409/wjcp.v11.i6.437
50. Benn CS, Böttcher MF, Pedersen BV, Filteau SM, Duchén K. Permeabilidad paracelular epitelial mamaria en madres atópicas y no atópicas versus atopia infantil. Pediatra Inmunol alérgico. (2004) 15:123–6. doi: 10.1046/j.1399-3038.2003.00138.x
51. Monks J, Neville MC. Albumin transcytosis across the epithelium of the lactating mouse mammary gland. J Physiol. (2004) 560(Pt 1):267–80. doi: 10.1113/jphysiol
Keywords: antigens, breast milk, celiac disease, gluten exposure, gluten-free diet, gluten immunogenic peptides
Citation: Ruiz-Carnicer Á, Segura V, Moreno MdL, Coronel-Rodríguez C, Sousa C and Comino I (2024) Transfer of celiac disease-associated immunogenic gluten peptides in breast milk: variability in kinetics of secretion. Front. Immunol. 15:1405344. doi: 10.3389/fimmu.2024.1405344
Received: 22 March 2024; Accepted: 10 June 2024;
Published: 05 July 2024.
Edited by:
Ger Rijkers, University College Roosevelt, NetherlandsReviewed by:
Ana Maria Calderon De La Barca, National Council of Science and Technology (CONACYT), MexicoRichard E. Goodman, University of Nebraska-Lincoln, United States
Copyright © 2024 Ruiz-Carnicer, Segura, Moreno, Coronel-Rodríguez, Sousa and Comino. This is an open-access article distributed under the terms of the Creative Commons Attribution License (CC BY). The use, distribution or reproduction in other forums is permitted, provided the original author(s) and the copyright owner(s) are credited and that the original publication in this journal is cited, in accordance with accepted academic practice. No use, distribution or reproduction is permitted which does not comply with these terms.
*Correspondence: Isabel Comino, icomino@us.es
†These authors have contributed equally to this work and share first authorship