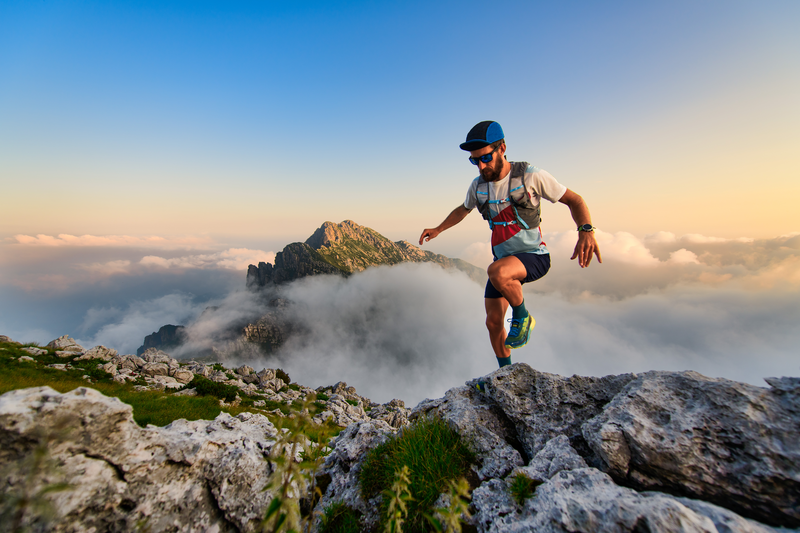
94% of researchers rate our articles as excellent or good
Learn more about the work of our research integrity team to safeguard the quality of each article we publish.
Find out more
OPINION article
Front. Immunol. , 17 May 2024
Sec. Comparative Immunology
Volume 15 - 2024 | https://doi.org/10.3389/fimmu.2024.1404360
The exceptional versatility and broad applicability of plastics in various industries and daily life is resulting in escalating release rates of plastics across environments. Under varying environmental conditions, plastics undergo fragmentation into so-called microplastic particles (<5 mm) and nanoplastic particles (≤100 nm) with increased risks for exposure and scarcely explored hazardous effects on humans and environmental species (1–3). Considering their small size, micro and nanoplastic particles (MNPs) are expected to interact with the epithelial barriers in, for example, gills, lungs, digestive tract, or exoskeleton of various species. Such interactions may result in receptor-dependent or -independent modulation of cellular signaling machinery maintaining the overall function and homeostasis of the cell. As the essential protective system defending the organism’s integrity and well-being, the innate immune system’s structures and mechanisms stand as the first pillar in the recognition and elimination of foreign materials and potential threats, including MNPs. Scientific efforts to explore MNPs-innate immune interactions are well undergoing, however, mainly by separate studies with the focus on individual environmental species, ranging from bivalves and earthworms to echinoderms and vertebrates. In order to grasp the full picture of the MNPs-innate immune interactions and understand their outcomes, leveraging the evolutionary immunology approach offers numerous advantages. Critically, a broad-taxa approach covering different phyla and hierarchical levels of organization is an important concept in (eco)toxicological studies and pollution monitoring (4) and provides significant potential in tackling MNP challenges.
The innate immune system holds several molecular and structural traits that are conserved throughout evolution, as summarized in Figure 1. Those include subsets of circulating or tissue-resident innate effector cells and an intricate network of receptors (e.g., pattern recognition receptors - PRRs) and signaling cascades (5). Cross-species effector functions of innate cells predominantly rely on phagocytosis combined with the generation of reactive oxygen or nitrogen species (ROS/NOS), production and release of lysosomal enzymes, antimicrobial peptides, cytokines, fibrinogen-related peptides, leucine-rich repeats (LRRs), and complement-related proteins. Additional innate effector functions described across phyla are agglutination, clotting, coagulation, and melanization (4). Among PRRs, Toll-like receptors (TLRs) are present in all multicellular organisms and are typically expressed on sentinel cells, such as phagocytes, and have been recognized as key regulators of the immune responses. The prototypical TLR gene emerged in the eumetazoan ancestor approximately 600 million years ago, subsequently undergoing selection throughout evolution, likely to accommodate coevolving microbial threat cues. Genes involved in TLR-signaling pathways are highly conserved across both protostomes and deuterostomes and include the presence of TIR domain-containing adaptors, signaling mediators, effector molecules, and transcription factors activated by pathogens (6, 7). This compelling evidence speaks in favor of using innate immune response as a focal point in understanding common and distinct mechanisms and pathways modulated by MNPs, and in identifying predictive cross-species biomarkers of MNP exposure. Nevertheless, PRRs, including TLRs, exhibit genetic diversity, and to find gene homology between different metazoan species is challenging (8–10). This divergence in homology may lead to distinct functions between species (8–10), a factor that needs consideration when employing a cross-species approach in assessing MNP-innate interactions.
Based on the existing knowledge about cross-species immunosafety assessment of engineered nanomaterials (ENMs) or air pollution particles, there are many lessons learned that could be applied in the immunosafety assessment of MNPs. It is assumed that MNPs follow similar mechanisms of action as ENMs. Consequently, they ought to have the capacity to trigger the activation of innate immune mechanisms ranging from recognition to internalization and inflammatory response (6). However, when evaluating such interactions, it is of crucial importance to consider several aspects including physicochemical properties of particles, for example, size, shape, intrinsic and surface chemistry, aggregation/agglomeration affinity, etc. (11). Importantly, ENMs readily interact with biomolecules present in the environment and/or biological systems and form biomolecular interface or (eco)corona that is largely shaping innate immune responses (12, 13). Similar concepts are also being applied in the case of MNPs (14). Notably, studies on potential recognition of MNPs by surface and intracellular PRRs are still in their early stages. Currently, these studies primarily provide evidence of increased protein levels or gene expression of PRRs or downstream signaling molecules following MNP exposure, rather than direct evidence of receptor binding (15–17). Moreover, when defining the immune responses to MNPs, and as learned for ENMs, it is of paramount importance to clearly distinguish between physiological and anomalous innate response. Inflammatory response per se is considered a physiological defensive response across species. However, failure of the inflammatory resolution or defective response may result in pathological consequences for the organism (12).
Based on the extensive body of evidence, studying the MNP effects on the innate immune system in the evolutionary immunology context would yield manifold benefits. Primarily, it would provide important insight into the common and distinct mechanisms that innate immunity, as a first and conserved line of defense, employs to cope with MNP particles, from basal eukaryotic organisms to humans. Moreover, different species occupy different trophic levels and ecosystems, and understanding MNPs-innate interactions may provide essential insights on how MNP exposure affect species lower down the food chain, or how MNPs move through the food chains and potentially impact human health. Species with high commercial value (e.g., mussels, crustaceans, sea urchins) as well as those with a crucial role in ecosystems (e.g., benthic organisms, fish, seabirds) are potentially exposed to MNPs. Hence, discerning the effects of MNPs on the innate immune system of these species could help in designing mitigation measures and regulatory actions in order to preserve their survival and biodiversity. Ecosystems are interconnected, and immunological and health changes in one species can have cascading effects on others. Studying the impacts of MNPs of innate reactivity across different species may further approach these complex interactions. The innate immune system of some species is more susceptible toward MNPs (18), and these species could serve as early-warning sentinels of MNPs as an urging pollution problem that is here to stay. On the contrary, some species have a more robust innate immune system [e.g., echinoderms have highly diversified panel of PRRs and effector molecules (19)] that could provide a base for understanding the mechanisms that help them cope with MNP pollution. In addition, cross-species hazard identification will enhance reliability when strategies for MNPs are set based on their physical and/or chemical properties. In addition, wide and multi-species understanding of MNPs-innate interactions could serve as an excellent platform to develop predictive bioassays, empowered with omics and high-throughput techniques, that will have the potential to replace, reduce, and refine (3R) animal use in research, mirroring the efforts with ENMs (4, 20).
Even though the cross-species approach in exploring MNP-innate immune system interactions holds promise, it faces several challenges. Firstly, it is highly important to establish standardized experimental models, both in vitro and in vivo, that simulate realistic exposure conditions across species, while also considering MNP physicochemical properties and environmental conditions affecting MNP behavior and interactions with the innate immune system. Further strategies should prioritize the development of in vitro models derived from key innate immune cell types, such as phagocytes, to investigate MNP-innate interactions from a mode of action perspective. Notably, mechanistic studies need to be designed feasibly, focusing on certain PRRs found in various species to allow for comparison. One example could be TLRs, the best-known PRRs present in all multicellular organisms, demonstrated to be promising in capturing cross-species effects of ENMs (5). Apart from focusing on individual molecules/pathways, additional endpoints such as phagocytic activity, cytokine/chemokine profiling, oxidative stress markers, and cell morphology should also be included. To evaluate the genetic determinants of MNP-innate interactions, genome-wide association studies and multi-omics analyses can identify genes, metabolites, and proteins involved in modulating innate immune responses to MNPs. Such an approach can identify gene candidates and target pathways, with further research scrutinizing their roles in MNP-innate interactions. Complementary broad-taxa in vivo models can validate in vitro findings and provide insights into systemic effects and organismal responses to MNP exposure. Longitudinal studies assessing chronic effects of MNP exposure on innate immune system functioning can provide important insights into the persistence of MNP-triggered effects on the innate immune system. Finally, integrating ecologically relevant endpoints, such as effects on species abundance and community structure, can serve as a connection between laboratory findings and real-life consequences of MNP pollution.
The innate immune system, from basal eukaryotes to humans, holds several functional and structural characteristics that are conserved throughout evolution. The proper functioning of the innate immune system plays a crucial role in the survival and overall fitness of organisms. Therefore, in this opinion article, we emphasize the importance of adopting an evolutionary immunology framework to unveil the interactions between MNPs and the innate immune system across diverse species. For instance, assessing how invertebrate innate immune system responds to MNPs may reveal novel endpoints worth exploring in vertebrates and vice versa. Additionally, a broad-taxa approach may identify common innate mechanisms that transcend species boundaries. Leveraging evolutionarily conserved pathways and mechanisms identified in other organisms may be useful in translating findings to human health. Importantly, this broad-taxa approach aligns with the One Health initiative, which underscores the interconnectedness of human, animal, and environmental health. Furthermore, the proposed approach seeks to consolidate fragmented efforts and knowledge in exploring MNP-innate immune interactions in the time of escalating plastic pollution. We believe that the proposed evolutionary immunology approach, rooted in interdisciplinary research, will pave the way to a more accurate and reliable understanding of MNP-associated environmental and human health risks and informed decision making.
AA: Conceptualization, Formal Analysis, Funding acquisition, Investigation, Project administration, Software, Writing – original draft, Writing – review & editing. ES: Funding acquisition, Project administration, Writing – original draft, Writing – review & editing.
The author(s) declare financial support was received for the research, authorship, and/or publication of this article. This work was supported by the Swedish Knowledge Foundation (Grants No. 20220122; 20230020). Scientific support from the Exploring Inflammation in Health and Disease (X-HiDE) Consortium, which is a strategic research profile at Örebro University funded by the Knowledge Foundation (Grant No. 20200017) is also acknowledged.
The authors declare that the research was conducted in the absence of any commercial or financial relationships that could be construed as a potential conflict of interest.
All claims expressed in this article are solely those of the authors and do not necessarily represent those of their affiliated organizations, or those of the publisher, the editors and the reviewers. Any product that may be evaluated in this article, or claim that may be made by its manufacturer, is not guaranteed or endorsed by the publisher.
1. Vethaak AD, Legler J. Microplastics and human health. Science. (2021) 371:672–4. doi: 10.1126/science.abe5041
2. Koelmans AA, Belay BMG, Mintenig SM, Nor NHM, Redondo-Hasselerharm PE, de Ruijter VN. Towards a rational and efficient risk assessment for microplastics. Nat Rev Mater. (2023) 7:138–52. doi: 10.1016/j.trac.2023.117142
3. Lai H, Liu X, Qu M. Nanoplastics and human health: hazard identification and biointerface. Nanomaterials. (2022) 12:p.1298. doi: 10.3390/nano12081298
4. Rosner A, Ballarin L, Barnay‐Verdier S, Borisenko I, Drago L, Drobne D, et al. A broad-taxa approach as an important concept in ecotoxicological studies and pollution monitoring. Biol Rev. (2024) 99:131–76. doi: 10.1111/brv.13015
5. Boraschi D, Canesi L, Drobne D, Kemmerling B, Pinsino A, Prochazkova P, et al. Interaction between nanomaterials and the innate immune system across evolution. Biol Rev. (2023) 98:747–74. doi: 10.1111/brv.12928
6. Tassia MG, Whelan NV, Halanych KM. Toll-like receptor pathway evolution in deuterostomes. Proc Natl Acad Sci USA. (2017) 114:7055–60. doi: 10.1073/pnas.1617722114
7. Gerdol M, Venier P, Edomi P, Pallavicini A. Diversity and evolution of TIR-domain-containing proteins in bivalves and Metazoa: new insights from comparative genomics. Dev Comp Immunol. (2017) 70:145–64. doi: 10.1016/j.dci.2017.01.014
8. Orús-Alcalde A, Lu TM, Børve A, Hejnol A. The evolution of the metazoan Toll receptor family and its expression during protostome development. BMC Ecol Evol. (2021) 21:1–21. doi: 10.1186/s12862-021-01927-1
9. Saco A, Novoa B, Greco S, Gerdol M, Figueras A. Bivalves present the largest and most diversified repertoire of Toll-like receptors in the animal kingdom, suggesting broad-spectrum pathogen recognition in marine waters. Mol Biol Evol. (2023) 40:msad133. doi: 10.1093/molbev/msad133
10. Liu G, Zhang H, Zhao C, Zhang H. Evolutionary history of the Toll-like receptor gene family across vertebrates. Genome Biol Evol. (2020) 12:3615–34. doi: 10.1093/gbe/evz266
11. Xiao B, Liu Y, Chandrasiri I, Overby C, Benoit DS. Impact of nanoparticle physicochemical properties on protein corona and macrophage polarization. ACS Appl Mater Interfaces. (2023) 15:13993–4004. doi: 10.1021/acsami.2c22471
12. Boraschi D, Alijagic A, Auguste M, Barbero F, Ferrari E, Hernadi S, et al. Addressing nanomaterial immunosafety by evaluating innate immunity across living species. Small. (2020) 16:2000598. doi: 10.1002/smll.202000598
13. Cai R, Ren J, Ji Y, Wang Y, Liu Y, Chen Z, et al. Corona of thorns: the surface chemistry-mediated protein corona perturbs the recognition and immune response of macrophages. ACS Appl Mater Interfaces. (2019) 12:1997–2008. doi: 10.1021/acsami.9b15910
14. Shi X, Chen Z, Wei W, Chen J, Ni BJ. Toxicity of micro/nanoplastics in the environment: Roles of plastisphere and eco-corona. Soil Environ Health. (2023) 1:100002. doi: 10.1016/j.seh.2023.100002
15. Antunes J, Sobral P, Martins M, Branco V. Nanoplastics activate a TLR4/p38-mediated pro-inflammatory response in human intestinal and mouse microglia cells. Environ Toxicol Pharmacol. (2023) 104:104298. doi: 10.1016/j.etap.2023.104298
16. Zhang Q, Wang F, Xu S, Cui J, Li K, Shiwen X, et al. Polystyrene microplastics induce myocardial inflammation and cell death via the TLR4/NF-κB pathway in carp. Fish Shellfish Immunol. (2023) 135:108690. doi: 10.1016/j.fsi.2023.108690
17. Alijagic A, Hedbrant A, Persson A, Larsson M, Engwall M, Särndahl E. NLRP3 inflammasome as a sensor of micro-and nanoplastics immunotoxicity. Front Immunol. (2023) 14:1178434. doi: 10.3389/fimmu.2023.1178434
18. Franzellitti S, Canesi L, Auguste M, Wathsala RH, Fabbri E. Microplastic exposure and effects in aquatic organisms: a physiological perspective. Environ Toxicol Pharmacol. (2019) 68:37–51. doi: 10.1016/j.etap.2019.03.009
19. Smith LC, Ghosh J, Buckley KM, Clow LA, Dheilly NM, Haug T, et al. Echinoderm immunity. Invertebrate Immun. (2010), 260–301. doi: 10.1007/978-1-4419-8059-5_14
Keywords: innate immunity, particles, environmental species, humans, pattern recognition receptors (PRRs)
Citation: Alijagic A and Särndahl E (2024) Can evolutionary immunology decode micro and nanoplastic challenges?. Front. Immunol. 15:1404360. doi: 10.3389/fimmu.2024.1404360
Received: 20 March 2024; Accepted: 06 May 2024;
Published: 17 May 2024.
Edited by:
Jiong Chen, Ningbo University, ChinaReviewed by:
Umberto Rosani, University of Padua, ItalyCopyright © 2024 Alijagic and Särndahl. This is an open-access article distributed under the terms of the Creative Commons Attribution License (CC BY). The use, distribution or reproduction in other forums is permitted, provided the original author(s) and the copyright owner(s) are credited and that the original publication in this journal is cited, in accordance with accepted academic practice. No use, distribution or reproduction is permitted which does not comply with these terms.
*Correspondence: Andi Alijagic, YW5kaS5hbGlqYWdpY0BvcnUuc2U=
Disclaimer: All claims expressed in this article are solely those of the authors and do not necessarily represent those of their affiliated organizations, or those of the publisher, the editors and the reviewers. Any product that may be evaluated in this article or claim that may be made by its manufacturer is not guaranteed or endorsed by the publisher.
Research integrity at Frontiers
Learn more about the work of our research integrity team to safeguard the quality of each article we publish.