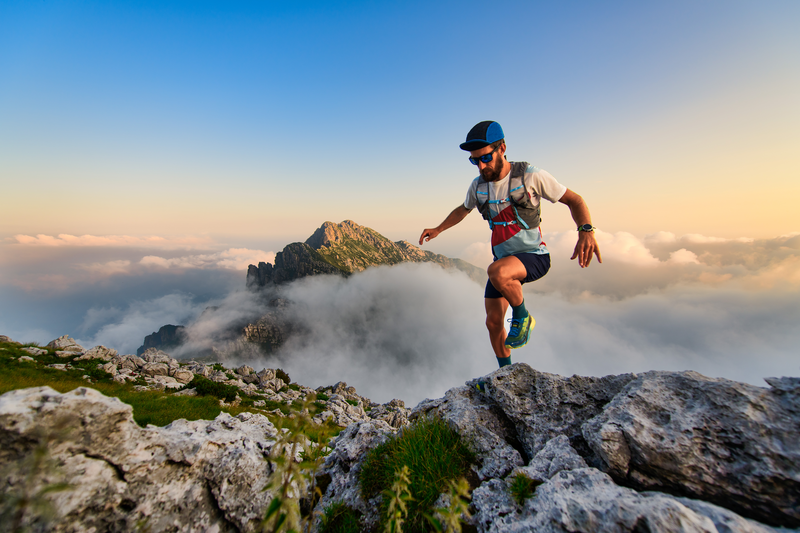
94% of researchers rate our articles as excellent or good
Learn more about the work of our research integrity team to safeguard the quality of each article we publish.
Find out more
OPINION article
Front. Immunol. , 18 June 2024
Sec. Cancer Immunity and Immunotherapy
Volume 15 - 2024 | https://doi.org/10.3389/fimmu.2024.1403124
This article is part of the Research Topic The Interplay between Immuno-Inflammatory Response and Oxidative Stress in Cancer Pathogenesis View all articles
Inflammation and oxidative stress contribute in cancer development, severity and aggression (1–3). Many malignancies arise from tissues affected by chronic inflammation. Tumor microenvironment consists of both cancer and immune cells which secrete growth factors, cytokines and chemokines, leading to cancer spread (1). Anti-inflammatory and immunomodulatory therapeutic approaches are commonly used in oncology (3).
Reactive oxygen species (ROS) are highly active molecules, arising from physiological and pathological processes, and our body balances their excess utilizing anti-oxidative defense mechanisms. Cancers suppress antioxidative mechanisms via enzyme modulation/mutation. Under physiological conditions ROS act as signaling molecules in cell growth, migration and differentiation. Chronic inflammation may lead to the excessive generation of ROS and reactive nitrogen species (RNS), altering immune responses, which in turn lead to oncogenic transformations (4).
The innate immune system depends on ROS, since macrophages and natural killer (NK) cells utilize those highly active molecules to maintain human tissue integrity and combat pathogens. ROS generation by mitochondria is due to activation of several proinflammatory pathways (e.g. MAPK, AMPK, PI3K/ACT) in coordination with NF-κB and HIF1α (2).
Growth hormone - releasing hormone (GHRH) is secreted by the hypothalamus and binds to the GHRH receptor (GHRH-R) of the pituitary cells to trigger the release of GH from the somatotrophs. GHRH is a 44-amino acid peptide, however its full intrinsic biological activity is retained by the NH2-terminal 29-amino acid sequence. The pituitary type GHRH receptor (pGHRH-R) is a class II G-protein-coupled receptor with seven transmembrane domains, homologous to the receptors for VIP, PACAP and calcitonin. Activation of pGHRH-R results in increased cAMP production, which acts as the second messenger in the GHRH related signal transduction. Splice variants (SVs) of the GHRH-R have been identified in various cancers. SV1 receptor possesses ligand independent activities (5, 6) and activates the mitogen-activated protein kinase (MAPK) pathway (5, 7).
The expression of GHRH has been demonstrated in prostatic, endometrial, ovarian, breast, gastroenteropatic, and lung carcinomas, glioblastomas, malignant bone tumors, human adrenal carcinomas and colorectal cancers (8). GHRH may act as an autocrine and/or paracrine growth factor in cancers (8). Knocking down of GHRH gene expression suppressed the proliferation of T47D, MDA-MB-435S, MDA-MB-468 breast cancers, LNCaP prostate cancer and NCI H838 non-SCLC (6). Moreover, GHRH can increase IL-17 secretion (9), a cytokine involved in the pathogenesis of non-alcoholic and alcoholic steatohepatitis (10). It has been reported that both conditions are associated with increased risk of hepatocellular carcinoma (HCC) development. In mice, targeting IL-17 suppressed the development of NASH-associated HCC (10). In another study, IL-17 was able to blunt the anticancer efficacy of chemotherapeutic agents in vivo (11). GHRH was able to promote TH17 cell differentiation and autoimmune inflammation (12), and MIA-690 – a GHRH antagonist - inhibited LPS-induced inflammatory and pro-oxidative markers (13).
Several splice variants of the GHRH receptor (SVs) were identified and sequenced, including SV1 (14). The major part of its cDNA sequence is identical to the corresponding sequence of pGHRH-R, with the exception of the first 334 SV1 nucleotides. The protein sequence of this transduced receptor differs from the full length receptor in the amino-terminal extracellular domain, in which a 25 amino-acid sequence replaces the first 89 amino acids of pGHRH-R (14). SV1 has been associated with strong ligand independent activities (15). Moreover, it is expressed in many cancers, including prostatic, breast, colorectal, gastric, melanomas, bone sarcomas, glioblastomas and SW13 human adrenal carcinoma cells (16). The pGHRH-R is present in human cancer tissues isolated from breast, ovarian, lung cancers, glioblastomas and lymphoma cells (16).
Antagonists of growth hormone-releasing hormone inhibit the growth of various experimental cancers including prostate, breast, ovarian, colorectal, lung, renal, endometrial cancers; glioblastomas and lymphomas (16, 17). The inhibitory effect of GHRH antagonists is partially dependent on the suppression of GH secretion from the pituitary, which results in decreased IGF-I production. GHRH antagonists can also suppress tumor growth in a direct manner through blockade of autocrine GHRH action (8, 16). HeLa cells, which do not express GHRH receptors, responded to GHRH and GHRH antagonists after being transfected with the pGHRH-R or SV1 receptor (18).
Reactive oxygen species (ROS) and reactive nitrogen species (RNS) act as signaling molecules. They promote human tumors by contributing to oxidative stress, a common condition in cancer (2). In LNCaP prostate cancer cells GHRH antagonists exerted antioxidative properties, and in A549 lung cancer cells JV-1–36 suppressed hydrogen-peroxide induced ROS (19, 20). In bovine pulmonary artery endothelial cells, human cerebral microvascular endothelial cells, and human lung microvascular endothelial cells those peptides reduced ROS generation. 3T3 cells which do not express GHRH receptors were not affected by GHRH analog treatment (21).
It was also recently revealed that GHRH antagonists suppress IFN-γ (22), hydrogen peroxide (23, 24) and hydrochloric acid (25) - induced inflammation. P53 is a tumor suppressor exerting anti-oxidative activities, which is induced by GHRH antagonists (17, 26–29). Those data further our knowledge on the mechanisms mediating the protective effects of those peptides against human disease (30). P53 and unfolded protein response are interrelated in the intracellular niche, since UPR activation induces P53 (31). It appears that UPR – which exerts anti-inflammatory and anti-oxidative activities (32–37) - is involved in the effects of GHRH antagonists in endothelial cells (32). These peptides were able to induce the three UPR sensors and its downstream target, namely BiP, in normal lung cells. There is very limited information on these effects of GHRH-related analogs in cancer cells (38).
It has been demonstrated that the SV1 receptor and pGHRH-R activate mitogen activated protein kinases ERK1/2 (7), which are strongly related to the generation and metabolism of ROS. GHRH can also activate: i) JAK2/STAT3, which contributes to oxidative phenomena (39), and ii) inducible nitric oxide synthase (iNOS) in A549 lung cancer cells. GHRH antagonist treatment counteracts those events (40). This is important because iNOS is one of the three NOS isoforms. It catalyzes the oxidative deamination of L-arginine to produce cytruline and nitric oxide (NO) and it is essential for immunity and vascular function. Moreover, it has been involved in the pathogenesis of various diseases through ROS/RNS induction. Indeed, ERK1/2 activation leads to increased iNOS and NO production (41, 42).
The aforementioned studies report that GHRH induces ROS/RNS generation. GHRH antagonists can counteract those effects eliciting anti-inflammatory responses, which contribute to their anti-cancer activities. The exact mechanisms involved in those events are not completely understood, and are currently under investigation.
AS: Conceptualization, Writing – original draft, Writing – review & editing. NB: Writing – review & editing.
The author(s) declare financial support was received for the research, authorship, and/or publication of this article. The study was supported by the grant of the Medical University of Lodz No 503/1-153-06/503-11-001-19-00 (to AS). NB is supported by an IDeA award from NIGMS/NIH under grant number P20 GM103424-21.
The authors declare that the research was conducted in the absence of any commercial or financial relationships that could be construed as a potential conflict of interest.
The author(s) declared that they were an editorial board member of Frontiers, at the time of submission. This had no impact on the peer review process and the final decision.
All claims expressed in this article are solely those of the authors and do not necessarily represent those of their affiliated organizations, or those of the publisher, the editors and the reviewers. Any product that may be evaluated in this article, or claim that may be made by its manufacturer, is not guaranteed or endorsed by the publisher.
2. Morris G, Gevezova M, Sarafian V, Maes M. Redox regulation of the immune response. Cell Mol Immunol. (2022) 19:1079–101. doi: 10.1038/s41423-022-00902-0
3. Hou J, Karin M, Sun B. Targeting cancer-promoting inflammation - have anti-inflammatory therapies come of age? Nat Rev Clin Oncol. (2021) 18:261–79. doi: 10.1038/s41571-020-00459-9
4. Yu W, Tu Y, Long Z, Liu J, Kong D, Peng J, et al. Reactive oxygen species bridge the gap between chronic inflammation and tumor development. Oxid Med Cell Longev. (2022) 2022:2606928. doi: 10.1155/2022/2606928
5. Barabutis N, Tsellou E, Schally AV, Kouloheri S, Kalofoutis A, Kiaris H. Stimulation of proliferation of MCF-7 breast cancer cells by a transfected splice variant of growth hormone-releasing hormone receptor. Proc Natl Acad Sci U.S.A. (2007) 104:5575–9. doi: 10.1073/pnas.0700407104
6. Barabutis N, Schally AV. Knocking down gene expression for growth hormone-releasing hormone inhibits proliferation of human cancer cell lines. Br J Cancer. (2008) 98:1790–6. doi: 10.1038/sj.bjc.6604386
7. Barabutis N, Siejka A, Schally AV, Block NL, Cai R, Varga JL. Activation of mitogen-activated protein kinases by a splice variant of GHRH receptor. J Mol Endocrinol. (2010) 44:127–34. doi: 10.1677/JME-09-0121
8. Barabutis N, Schally AV. Growth hormone-releasing hormone: extrapituitary effects in physiology and pathology. Cell Cycle. (2010) 9:4110–6. doi: 10.4161/cc.9.20.13787
9. Stepien T, Lawnicka H, Komorowski J, Stepien H, Siejka A. Growth hormone-releasing hormone stimulates the secretion of interleukin 17 from human peripheral blood mononuclear cells in vitro. Neuro Endocrinol Lett. (2010) 31(6):852–856.
10. Ma HY, Yamamoto G, Xu J, Liu X, Karin D, Kim JY, et al. IL-17 signaling in steatotic hepatocytes and macrophages promotes hepatocellular carcinoma in alcohol-related liver disease. J Hepatol. (2020) 72:946–59. doi: 10.1016/j.jhep.2019.12.016
11. Bruchard M, Mignot G, Derangere V, Chalmin F, Chevriaux A, Vegran F, et al. Chemotherapy-triggered cathepsin B release in myeloid-derived suppressor cells activates the Nlrp3 inflammasome and promotes tumor growth. Nat Med. (2013) 19:57–64. doi: 10.1038/nm.2999
12. Du L, Ho BM, Zhou L, Yip YWY, He JN, Wei Y, et al. Growth hormone releasing hormone signaling promotes Th17 cell differentiation and autoimmune inflammation. Nat Commun. (2023) 14:3298. doi: 10.1038/s41467-023-39023-1
13. Recinella L, Chiavaroli A, Orlando G, Ferrante C, Marconi GD, Gesmundo I, et al. Antinflammatory, antioxidant, and behavioral effects induced by administration of growth hormone-releasing hormone analogs in mice. Sci Rep. (2020) 10:732. doi: 10.1038/s41598-019-57292-z
14. Rekasi Z, Czompoly T, Schally AV, Halmos G. Isolation and sequencing of cDNAs for splice variants of growth hormone-releasing hormone receptors from human cancers. Proc Natl Acad Sci U.S.A. (2000) 97:10561–6. doi: 10.1073/pnas.180313297
15. Kiaris H, Chatzistamou I, Schally AV, Halmos G, Varga JL, Koutselini H, et al. Ligand-dependent and -independent effects of splice variant 1 of growth hormone-releasing hormone receptor. Proc Natl Acad Sci U.S.A. (2003) 100:9512–7. doi: 10.1073/pnas.1533185100
16. Schally AV, Varga JL, Engel JB. Antagonists of growth-hormone-releasing hormone: an emerging new therapy for cancer. Nat Clin Pract Endocrinol Metab. (2008) 4:33–43. doi: 10.1038/ncpendmet0677
17. Barabutis N, Schally AV, Siejka A. P53. GHRH Inflammation cancer EBioMedicine. (2018) 37:557–62. doi: 10.1016/j.ebiom.2018.10.034
18. Barabutis N, Schally AV. Antioxidant activity of growth hormone-releasing hormone antagonists in LNCaP human prostate cancer line. Proc Natl Acad Sci U.S.A. (2008) 105:20470–5. doi: 10.1073/pnas.0811209106
19. Kubra KT, Akhter MS, Apperley K, Barabutis N. Growth hormone-releasing hormone antagonist JV-1–36 suppresses reactive oxygen species generation in A549 lung cancer cells. Endocrines. (2022) 3:813–20. doi: 10.3390/endocrines3040067
20. Barabutis N, Akhter MS, Kubra KT, Jackson K. Growth hormone-releasing hormone in endothelial inflammation. Endocrinology (2022) 164(2):1–11. doi: 10.1210/endocr/bqae064
21. Akhter MS, Barabutis N. Suppression of reactive oxygen species in endothelial cells by an antagonist of growth hormone-releasing hormone. J Biochem Mol Toxicol. (2021) 35:e22879. doi: 10.1002/jbt.22879
22. Fakir S, Barabutis N. Growth hormone-releasing hormone antagonists counteract interferon-gamma - induced barrier dysfunction in bovine and human endothelial cells. Cytokine. (2024) 173:156416. doi: 10.1016/j.cyto.2023.156416
23. Barabutis N, Siejka A, Akhter MS. Growth hormone-releasing hormone antagonists counteract hydrogen peroxide - induced paracellular hyperpermeability in endothelial cells. Growth Horm IGF Res. (2023) 69–70:101534. doi: 10.1016/j.ghir.2023.101534
24. Akhter MS, Kubra KT, Barabutis N. Protective effects of GHRH antagonists against hydrogen peroxide-induced lung endothelial barrier disruption. Endocrine. (2023) 79:587–92. doi: 10.1007/s12020-022-03226-1
25. Barabutis N, Kubra KT, Akhter MS. Growth hormone-releasing hormone antagonists protect against hydrochloric acid-induced endothelial injury in vitro. Environ Toxicol Pharmacol. (2023) 99:104113. doi: 10.1016/j.etap.2023.104113
26. Akhter MS, Uddin MA, Barabutis N. P53 regulates the redox status of lung endothelial cells. Inflammation. (2020) 43:686–91. doi: 10.1007/s10753-019-01150-7
27. Akhter MS, Uddin MA, Kubra KT, Barabutis N. P53-induced reduction of lipid peroxidation supports brain microvascular endothelium integrity. J Pharmacol Sci. (2019) 141:83–5. doi: 10.1016/j.jphs.2019.09.008
28. Kubra KT, Uddin MA, Akhter MS, Barabutis N. P53 is subjected to lipoteichoic acid-induced phosphorylation in the lungs. TH Open. (2020) 4:e173–4. doi: 10.1055/s-0040-1714695
29. Uddin MA, Akhter MS, Siejka A, Catravas JD, Barabutis N. P53 supports endothelial barrier function via APE1/Ref1 suppression. Immunobiology. (2019) 224:532–8. doi: 10.1016/j.imbio.2019.04.008
30. Barabutis N, Fakir S. Growth hormone-releasing hormone beyond cancer. Clin Exp Pharmacol Physiol. (2024) 51:40–1. doi: 10.1111/1440-1681.13829
31. Akhter MS, Uddin MA, Barabutis N. Unfolded protein response regulates P53 expression in the pulmonary endothelium. J Biochem Mol Toxicol. (2019) 33:e22380. doi: 10.1002/jbt.22380
32. Akhter MS, Uddin MA, Schally AV, Kubra KT, Barabutis N. Involvement of the unfolded protein response in the protective effects of growth hormone releasing hormone antagonists in the lungs. J Cell Commun Signal. (2021) 15:125–9. doi: 10.1007/s12079-020-00593-0
33. Barabutis N. Activating transcription factor 6 in the endothelial context. Pulm Pharmacol Ther. (2023) 80:102216. doi: 10.1016/j.pupt.2023.102216
34. Kubra KT, Akhter MS, Saini Y, Kousoulas KG, Barabutis N. Activating transcription factor 6 protects against endothelial barrier dysfunction. Cell Signal. (2022) 99:110432. doi: 10.1016/j.cellsig.2022.110432
35. Kubra KT, Barabutis N, Brefeldin A. kifunensine modulate LPS-induced lung endothelial hyperpermeability in human and bovine cells. Am J Physiol Cell Physiol. (2021) 321:C214–20. doi: 10.1152/ajpcell.00142.2021
36. Kubra KT, Barabutis N. Ceapin-A7 potentiates lipopolysaccharide-induced endothelial injury. J Biochem Mol Toxicol. (2023) 37:e23460. doi: 10.1002/jbt.23460
37. Kubra KT, Uddin MA, Akhter MS, Barabutis N. Luminespib counteracts the Kifunensine-induced lung endothelial barrier dysfunction. Curr Res Toxicol. (2020) 1:111–5. doi: 10.1016/j.crtox.2020.09.003
38. Barabutis N. Growth hormone releasing hormone in the unfolded protein response context. Endocrine. (2020) 67:291–3. doi: 10.1007/s12020-020-02205-8
39. Siejka A, Schally AV, Barabutis N. Activation of Janus kinase/signal transducer and activator of transcription 3 pathway by growth hormone-releasing hormone. Cell Mol Life Sci. (2010) 67:959–64. doi: 10.1007/s00018-009-0224-y
40. Barabutis N, Siejka A, Schally AV. Growth hormone releasing hormone induces the expression of nitric oxide synthase. J Cell Mol Med. (2011) 15:1148–55. doi: 10.1111/jcmm.2011.15.issue-5
41. Ratajczak-Wrona W, Jablonska E, Garley M, Jablonski J, Radziwon P, Iwaniuk A. The role of MAP kinases in the induction of iNOS expression in neutrophils exposed to NDMA: the involvement transcription factors. Adv Med Sci. (2013) 58:265–73. doi: 10.2478/v10039-012-0074-y
Keywords: tumors, redox biology, endothelium, lungs, hypothalamus, pituitary
Citation: Siejka A and Barabutis N (2024) Growth hormone – releasing hormone in the context of inflammation and redox biology. Front. Immunol. 15:1403124. doi: 10.3389/fimmu.2024.1403124
Received: 18 March 2024; Accepted: 07 June 2024;
Published: 18 June 2024.
Edited by:
Yunxiang Zhou, Zhejiang University, ChinaReviewed by:
Ioannis Eleftherianos, George Washington University, United StatesCopyright © 2024 Siejka and Barabutis. This is an open-access article distributed under the terms of the Creative Commons Attribution License (CC BY). The use, distribution or reproduction in other forums is permitted, provided the original author(s) and the copyright owner(s) are credited and that the original publication in this journal is cited, in accordance with accepted academic practice. No use, distribution or reproduction is permitted which does not comply with these terms.
*Correspondence: Agnieszka Siejka, YWduaWVzemthLnNpZWprYUB1bWVkLmxvZHoucGw=
Disclaimer: All claims expressed in this article are solely those of the authors and do not necessarily represent those of their affiliated organizations, or those of the publisher, the editors and the reviewers. Any product that may be evaluated in this article or claim that may be made by its manufacturer is not guaranteed or endorsed by the publisher.
Research integrity at Frontiers
Learn more about the work of our research integrity team to safeguard the quality of each article we publish.