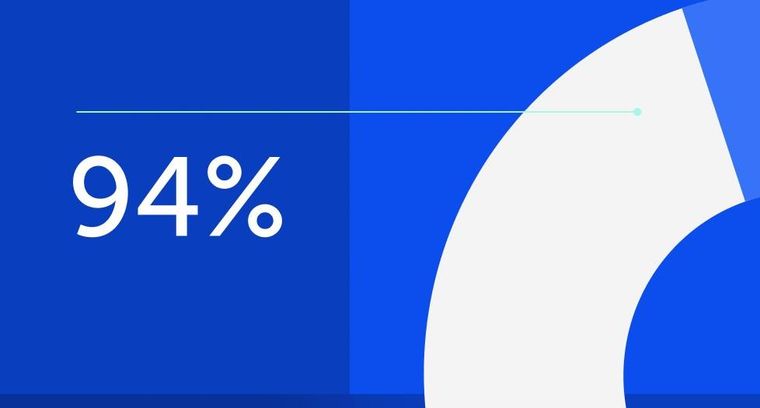
94% of researchers rate our articles as excellent or good
Learn more about the work of our research integrity team to safeguard the quality of each article we publish.
Find out more
MINI REVIEW article
Front. Immunol., 28 May 2024
Sec. T Cell Biology
Volume 15 - 2024 | https://doi.org/10.3389/fimmu.2024.1402412
This article is part of the Research TopicCommunity Series in the Role of CD1- and MR1-restricted T cells in Immunity and Disease, volume IIView all 18 articles
Due to the COVID-19 pandemic, the importance of developing effective vaccines has received more attention than ever before. To maximize the effects of vaccines, it is important to select adjuvants that induce strong and rapid innate and acquired immune responses. Invariant natural killer T (iNKT) cells, which constitute a small population among lymphocytes, bypass the innate and acquired immune systems through the rapid production of cytokines after glycolipid recognition; hence, their activation could be used as a vaccine strategy against emerging infectious diseases. Additionally, the diverse functions of iNKT cells, including enhancing antibody production, are becoming more understood in recent years. In this review, we briefly describe the functional subset of iNKT cells and introduce the glycolipid antigens recognized by them. Furthermore, we also introduce novel vaccine development taking advantages of iNKT cell activation against infectious diseases.
Invariant natural killer T (iNKT) cells, which express an invariant T cell receptor (TCR) α chain, are a subpopulation of T cells that possess both T cell and NK cell phenotypes (1–3). Conventional T cells recognize peptides presented on MHC class I or II molecules, whereas iNKT cells recognize endogenous or exogenous glycolipids presented by CD1d (4–6). Additionally, iNKT cells have already acquired effector functions during thymic development, and similar to memory T cells, they rapidly produce large amounts of cytokines, such as IFNγ and IL-4, after activation. Thus, iNKT cells play a role in bridging innate and acquired immune responses. Since the discovery of iNKT cells, specific ligands recognized by iNKT cells have been explored and discovered (7, 8). iNKT cells recognize several glycolipid antigens with similar structures through an invariant TCR, a unique feature of these cells. In this article, we review the features of iNKT cells especially in response to microbes.
The invariant TCR of iNKT cells consists of Vα14-Jα18 chains and a restricted repertoire of Vβ chains (Vβ8, 7, 2) in mice, and Vα24-Jα18 chains and Vβ11 in humans (1–3). Upon TCR stimulation, iNKT cells rapidly produce various cytokines, including IFNγ, IL-2, IL-4, IL-13, and IL-17A, and stimulate other immune cells. iNKT cells are classified into several effector subsets similar to conventional T cells based on cytokine production and regulatory transcription factors (9–11). NKT1 cells predominantly produce IFNγ as T helper (Th) 1 cells, NKT2 cells produce IL-4 and IL-13 as Th2 cells, and NKT17 cells have functions similar to those of Th17 cells. These effector subsets are already mature in the thymus and are distributed to the tissues via their specific chemokine receptors and adhesion molecules. However, depending on the intensity of TCR stimulation and the environment, iNKT cells may produce cytokines such as IL-4 from NKT1 cells and IFNγ from NKT2 cells. This suggests that iNKT cells function as “tuning players” in immune responses. iNKT subsets localize differently among tissues (12). NKT1 cells are mostly found in the liver, while NKT2 cells are found in lung and mesenteric lymph nodes. In contrast, NKT17 cells are found more abundantly in lymph nodes throughout the body. This distribution bias may be caused by differences in the cytokines required for homeostasis in each organ (13). Other functional subsets of iNKT cells include NKT10 cells (14), which is an immunosuppressive NKT subset that produces IL-10, and follicular helper NKT (NKTFH) cells (15, 16), which are phenotypically similar to follicular helper T (TFH) cells and stimulate B cells with IL-21 and costimulatory molecules. NKT10 and NKTFH cells localized in peripheral tissues, such as adipose and lymphoid tissue respectively. Although the detailed mechanisms of differentiation into NKT10 cells remain unknown, the quality and intensity of TCR stimulation may likely be involved (17, 18).
In contrast to the above effector subset classification, functional NKT cells may be classified based on the differential surface expression of CD244 and CXCR6 (19). CD244+ CXCR6+ iNKT cells (C2 NKT cells) are systemically circulating cells and produce more IFNγ and granzymes than CD244− CXCR6+ tissue-resident iNKT cells (C1 NKT cells). Hence, C2 NKT cells participate in antitumor and antimicrobial responses. As iNKT cells in human blood can be identified by similar surface molecules, this classification would be useful for the analysis of iNKT cells in humans.
The prototype antigen for iNKT cells is α-galactosylceramide (αGalCer) that is synthesized based on Agelasphin, which was isolated from a marine sponge and has antitumor activity (5, 20). Even a minimal amount of αGalCer induces IFNγ and IL-4 production by iNKT cells. αGalCer has also been used to study iNKT cell function and vaccines based on their activation, because this glycolipid induces more robust TCR stimulation in iNKT cells than known endogenous and pathogen-derived glycolipid antigens (21). αGalCer-activated iNKT cells reportedly become anergic after transient activation and proliferation (22). Additionally, when activated with αGalCer, NKT1 cells produce large amounts of IFNγ and highly express IL-4, which is produced mainly by NKT2 cells in steady state and is considered a characteristic of NKT2 cells (23). Therefore, while understanding the function of iNKT cells under physiological conditions, αGalCer results should be carefully interpreted. However, αGalCer has become a promising ligand in vaccine studies utilizing the effects of iNKT cell activation (24, 25). Moreover, iNKT cells have the potential for replacing conventional adjuvants. Vaccine studies using αGalCer will be discussed later in this article.
The physiological glycolipid antigen that most iNKT cells recognize was identified with the intestinal symbiont Sphingomonas sp. The α-linked glycosphingolipids (GSLs; containing a galacturonic acid or glucuronic acid moiety) of Sphingomonas sp. induce iNKT cells to produce IFNγ and IL-4 in a CD1d-dependent manner (26, 27). Bacteroides, a gram-negative bacterium that comprises 50% of human intestinal bacteria, produces sphingolipids similar to αGalCer (28–30). Bacteroides fragilis produces sphingolipids to regulate both activation and inhibition of iNKT cells. Among these sphingolipids, GSL-Bf717 inhibits proliferation and IFNγ and IL-4 production in iNKT cells; this effect is important to regulate the number and function of iNKT cells in intestinal tissues (29). In that study, it was shown that the regulation of iNKT cells by GSL-Bf717 in the neonatal stages influences the sensitivity of iNKT cell-mediated colitis in adult mice. Another study showed that B. fragilis regulates intestinal iNKT cell function via sphingolipid synthesis and is dependent on branched amino acids ingested by the host (31). Antibiotic-associated dysbiosis reportedly affects the number and function of iNKT cells, resulting in pathological conditions (32), although gut microbiota composition was not changed in iNKT cell-deficient or transgenic mice (33). This suggests that indigenous bacteria contribute to intestinal homeostasis by regulating iNKT cells.
From the perspective of host protection, exogenous glycolipid antigens recognized by iNKT cells were identified in several pathogens. Borrelia burgdorferi—the pathogenic bacterium that causes Lyme disease—produces a diacylglycerol (DAG)-based glycolipid containing α-linked galactose (αGal-DAG). iNKT cells recognize B. burgdorferi αGal-DAG via TCR and produce IFNγ and IL-4 (34–36). B. burgdorferi infections in iNKT cell-deficient mice results in more severe arthritis and carditis compared to non-iNKT cell-deficient control mice. Additionally, numerous spirochetes accumulate in the lesions, suggesting that antigen recognition by iNKT cells is important for antibacterial immunity. Streptococcus pneumoniae causes pneumonia, which can result in bacteremia and meningitis, especially in children and the elderly, and produces an α-linked glucose (Glc) containing DAG (αGlc-DAG). S. pneumoniae αGlc-DAG was recognized by TCR on iNKT cells (37). Neutrophil accumulation in the lungs via IFNγ and cytokines and chemokines, including IL-17 and GM-CSF produced by iNKT cells, is reportedly important during pneumococcal infections (38–40). Dendritic cells (DCs) play an important role in the stimulation of iNKT cells. Maturation of DCs through Toll like receptors upregulates CD1d and introduces TCR stimulation into surrounding iNKT cells together with IL-12. Activated iNKT cells then produce IFNγ and express CD40L, enhancing IL-12 production from DCs that further augments the immune response. More importantly, these glycolipids can activate not only mouse but also human iNKT cells.
Not all pathogens have glycolipid antigens that are recognized by iNKT cells. The filamentous fungus Aspergillus fumigatus and the gram-negative bacterium Salmonella typhimurium reportedly activate iNKT cells despite not having microbial glycolipid antigens (41, 42), and that this effect is CD1d dependent. This suggests that iNKT cells are activated by endogenous antigen/CD1d-mediated TCR stimulation as well as indirect stimulation by cytokines from antigen-presenting cells that were activated via pattern recognition receptors.
How are iNKT cells able to recognize a wide variety of glycolipids? Previous structural analysis of the iNKT cell TCR-glycolipid-CD1d complex has demonstrated that the TCR of iNKT cells changes the conformation of the CD1d-glycolipid antigen complex (43, 44), which may allow iNKT cells to recognize different but structurally similar glycolipid antigens. Contextually, iNKT cells reportedly produce IFNγ when exposed to IL-12 from antigen-presenting cells stimulated by LPS (21, 45). However, during TCR-independent activation, iNKT cells produced IFNγ but not IL-4 (21). Thus, although iNKT cells can be stimulated in the absence of TCR stimulation similarly as NK cells, CD1d-dependent TCR stimulation is important for IL-4 production.
There have been attempts to employ glycolipids as therapeutics for various infectious diseases as well as in vaccines. iNKT cell-mediated vaccines augment cellular and humoral immunity. For cellular immunity, mice immunized with malarial antigen plus αGalCer were more protected against malaria than those immunized with the antigen alone (46). Additionally, mice immunized with the Mycobacterium tuberculosis antigen plus αGalCer were also protected from bacterial infection (47). Following administration, αGalCer stimulates iNKT cells, thereby increasing the number of antigen-specific CD8 T cells in an IFNγ-dependent manner. Other synthetic glycolipids were used in several studies. Although αGalCer induces IFNγ and IL-4 production in iNKT cells, α-C-GalCer (48) and OCH (49) induce relatively biased cytokine production toward IFNγ and IL-4, respectively, in these cells.
iNKT cells reportedly enhance B cell responses during influenza infections under physiological conditions (50). iNKT cells play an essential role in the initial formation of germinal centers (GC), which is microstructure that regulates selection and proliferation of antigen-specific B cells and is essential for antibody production. During infection with the influenza virus, iNKT cells become a source of IL-4 that is important not only for the induction of GC but also for class switching and production of IgG1. Also, vaccines containing a glycolipid as an adjuvant efficiently induce antibody-producing responses mediated by B cells through the activation of iNKT cells. Intranasal influenza hemagglutinin (HA) and αGalCer vaccines more strongly induce HA-specific IgG in serum and HA-specific IgA in mucosa than HA vaccine alone as well as exert a potent protective effect even against lethal doses of influenza virus infection (51–53). Although follicular helper T (TFH) cells play an important role in the antibody-producing response in vivo (54), follicular helper NKT (NKTFH) cells play a more central role in the antibody-producing response induced by αGalCer-contained vaccines (15, 16).
A vaccine containing liposome-encapsulated PBS57, an αGalCer analog and pneumococcal capsular polysaccharide (CPS) induces NKTFH cells (55). CPS-specific IgG1 induction by this vaccine is dependent on CD1d expression on B cells and DCs, indicate that the interaction of CPS-specific B cells and NKTFH cells is important for specific antibody production. NKTFH cells contribute to antigen-specific B cell responses via stimulatory molecules, including as IL-21 and ICOS, and their follicular differentiation is controlled by transcription factor Bcl6 (15). However, the detailed differentiation mechanisms have not been elucidated. Normally, NKTFH cells are not found in the thymus and appear in the spleen and lymph nodes only upon the activation of iNKT cells with αGalCer. This indicates that potent TCR stimulation by αGalCer is important for NKTFH cell differentiation. However, αGalCer stimulation alone does not induce NKTFH cell differentiation in vitro, suggesting that environmental factors are essential for acquiring follicular phenotypes. Moreover, our recent study clarified that Gr-1+ cells promote NKTFH cell differentiation by producing interleukin-27 (IL-27) post-αGalCer administration (56). IL-27 modulates mitochondrial metabolism in activated iNKT cells and optimizes the energy demand required for NKTFH cell differentiation. Gr-1+ cell-derived IL-27 is induced by iNKT cells via IFNγ production.
Recently, it was shown that administration of nanoparticles embedded with αGalCer activates iNKT cells in vivo more efficiently than soluble αGalCer. Additionally, delivery by nanoparticles enables activation of iNKT cells at doses 1,000× lower than those used for in vivo studies and does not induce iNKT cell anergy. Although nanoparticle vaccines inhibit T-independent reactions by tolerizing or eliminating polysaccharide-specific B cells, T-dependent reactions following vaccination efficiently induces antigen-specific antibodies and protects mice from lethal S. pneumoniae infection (57).
The administration of NKT-specific glycolipid alone induces protection against microbial infections. 7DW8-5, the primary compound of αGalCer, activates human and mouse iNKT cells more potently than αGalCer (58). Intranasal administration of 7DW8-5 provided protection against respiratory pathogens, including SARS-COV2, RSV, and influenza viruses. However, as administrating 7DW8-5 to mice postinfection was ineffective, glycolipids may be more suitable for vaccine-like therapy. Herein, the authors underscore that αGalCer and 7DW8-5 may potentially be used in clinical applications as long as their safety is considered. More importantly, their simplicity and affordability will assist in the development of next-generation vaccines. αGalCer has already been used to treat patients with tumors, and that no significant toxicity was reportedly observed (59). Additionally, toxicity was not observed in monkeys treated with excessive amounts of 7DW8-5 as a vaccine adjuvant (60). Although additional safety studies are warranted, αGalCer and 7DW8-5 are expected to be used in clinical studies.
iNKT cells play an important role in microbial infections. Their importance is evidenced by the fact that in some infections, pathogens adopt strategies to reduce CD1d expression (61, 62). Although iNKT cells constitute a small population of T cells with a poor diversity of TCR, they enhance as well as regulate immune responses through the recognition of various glycolipid ligands (Table 1). iNKT cells are more prominent in subtle responses to environment as compared with conventional T cells, i.e., as a “tuning player” in immune reactions, which may be important for induction of proper immune reactions. These functions would be why iNKT cells have persisted despite evolution. Studies have also shown the functional significance of iNKT cells not only for protection against pathogens but also in the establishment and maintenance of tissue homeostasis (Figure 1). Tissue accumulation of iNKT cells during fetal life is necessary to maintain intestinal tract and skin homeostasis (63, 64), and iNKT cells are also involved in regulating peripheral serotonin release. These show that iNKT cells contribute not only to the immune system but also to systemic homeostasis (65).
Figure 1 Glycolipid-dependent or -independent reaction of iNKT cells direct multiple outcomes. 1) Several microbial lipid antigens, which are derived Streptococcus pneumoniae and Borrelia burgdorferi, activate iNKT cells through CD1d presentation on antigen-presenting cells (APCs). Activated iNKT cells rapidly produce IFNγ and augment innate and acquired immune responses, which are essential for protection against acute bacterial infections. Not only IFNγ but IL-4, IL-17 and GM-CSF are also involved in iNKT cell-mediated protective responses. 2) Infection with Salmonella typhimurium stimulates APCs by LPS and induces IL-12 production (probably also IL-18 production). iNKT cell activation and IFNγ production are dependent not only on IL-12 but also partially on TCR stimulation by endogenous ligands presented by CD1d. 3) During viral infections involving murine cytomegalovirus (MCMV), iNKT cells produce IFNγ even in the absence of glycolipid antigens and is dependent on IL-12 and Toll like receptor signaling. 4) In intestinal tissues, among symbionts, Bacteroides fragilis affect the number of iNKT cells in the young and regulate homeostasis throughout life via multiple α-galactosylceramide (BfαGCs), which has immunomodulatory signaling and actions. In the skin, iNKT cells also support tissue homeostasis by regulating local iron metabolism with transferrin production, which depends on CD1d.
Although iNKT cells rapidly respond during microbial infections, their functions are diverse, and their importance in various tissues need to be elucidated. iNKT cell-mediated vaccines are potent and are expected to be components of next-generation vaccines. However, due to their diverse functions, the route of administration, timing, and duration warrants further investigation.
KH: Writing – original draft, Writing – review & editing. YaK: Writing – original draft. YuK: Writing – original draft, Writing – review & editing.
The author(s) declare financial support was received for the research, authorship, and/or publication of this article. This work was supported by the Ministry of Education, Culture, Sports, Science and Technology, Japan [Grants-in Aid for Scientific Research (B) #19H03705, #22H03123, #23K24382 (C) #21K07085, Grants-in Aid for Early-Career Scientists #19K16704], The Uehara Memorial Foundation, Yakult Bio-Science Foundation, Takeda Science Foundation, Senshin Medical Research Foundation, Daiichi Sankyo Foundation of Life Science, Astellas Foundation for Research on Metabolic Disorders, and The Jikei University Graduate Research Fund.
We are grateful to the NIH Tetramer Core facility for kindly providing PBS57 loaded CD1d tetramers.
The authors declare that the research was conducted in the absence of any commercial or financial relationships that could be construed as a potential conflict of interest.
All claims expressed in this article are solely those of the authors and do not necessarily represent those of their affiliated organizations, or those of the publisher, the editors and the reviewers. Any product that may be evaluated in this article, or claim that may be made by its manufacturer, is not guaranteed or endorsed by the publisher.
1. Kronenberg M. Toward an understanding of NKT cell biology: progress and paradoxes. Annu Rev Immunol. (2005) 23:877–900. doi: 10.1146/annurev.immunol.23.021704.115742
2. Godfrey DI, Pellicci DG, Patel O, Kjer-Nielsen L, McCluskey J, Rossjohn J. Antigen recognition by CD1d-restricted NKT T cell receptors. Semin Immunol. (2010) 22:61–7. doi: 10.1016/j.smim.2009.10.004
3. Gapin L. Development of invariant natural killer T cells. Curr Opin Immunol. (2016) 39:68–74. doi: 10.1016/j.coi.2016.01.001
4. Bendelac A, Lantz O, Quimby ME, Yewdell JW, Bennink JR, Brutkiewicz RR. CD1 recognition by mouse NK1+ T lymphocytes. Science. (1995) 268:863–5. doi: 10.1126/science.7538697
5. Kawano T, Cui J, Koezuka Y, Toura I, Kaneko Y, Motoki K, et al. CD1d-restricted and TCR-mediated activation of valpha14 NKT cells by glycosylceramides. Science. (1997) 278:1626–9. doi: 10.1126/science.278.5343.1626
6. McEwen-Smith RM, Salio M, Cerundolo V. CD1d-dependent endogenous and exogenous lipid antigen presentation. Curr Opin Immunol. (2015) 34:116–25. doi: 10.1016/j.coi.2015.03.004
7. Tupin E, Kinjo Y, Kronenberg M. The unique role of natural killer T cells in the response to microorganisms. Nat Rev Microbiol. (2007) 5:405–17. doi: 10.1038/nrmicro1657
8. Kinjo Y, Ueno K. iNKT cells in microbial immunity: recognition of microbial glycolipids. Microbiol Immunol. (2011) 55:472–82. doi: 10.1111/mim.2011.55.issue-7
9. Georgiev H, Ravens I, Benarafa C, Forster R, Bernhardt G. Distinct gene expression patterns correlate with developmental and functional traits of iNKT subsets. Nat Commun. (2016) 7:13116. doi: 10.1038/ncomms13116
10. Lee YJ, Starrett GJ, Lee ST, Yang R, Henzler CM, Jameson SC, et al. Lineage-Specific Effector Signatures of Invariant NKT Cells Are Shared amongst gammadelta T, Innate Lymphoid, and Th Cells. J Immunol. (2016) 197:1460–70. doi: 10.4049/jimmunol.1600643
11. Engel I, Seumois G, Chavez L, Samaniego-Castruita D, White B, Chawla A, et al. Innate-like functions of natural killer T cell subsets result from highly divergent gene programs. Nat Immunol. (2016) 17:728–39. doi: 10.1038/ni.3437
12. Lee YJ, Wang H, Starrett GJ, Phuong V, Jameson SC, Hogquist KA. Tissue-specific distribution of iNKT cells impacts their cytokine response. Immunity. (2015) 43:566–78. doi: 10.1016/j.immuni.2015.06.025
13. Crosby CM, Kronenberg M. Tissue-specific functions of invariant natural killer T cells. Nat Rev Immunol. (2018) 18:559–74. doi: 10.1038/s41577-018-0034-2
14. Lynch L, Michelet X, Zhang S, Brennan PJ, Moseman A, Lester C, et al. Regulatory iNKT cells lack expression of the transcription factor PLZF and control the homeostasis of T(reg) cells and macrophages in adipose tissue. Nat Immunol. (2015) 16:85–95. doi: 10.1038/ni.3047
15. Chang PP, Barral P, Fitch J, Pratama A, Ma CS, Kallies A, et al. Identification of Bcl-6-dependent follicular helper NKT cells that provide cognate help for B cell responses. Nat Immunol. (2011) 13:35–43. doi: 10.1038/ni.2166
16. King IL, Fortier A, Tighe M, Dibble J, Watts GF, Veerapen N, et al. Invariant natural killer T cells direct B cell responses to cognate lipid antigen in an IL-21-dependent manner. Nat Immunol. (2011) 13:44–50. doi: 10.1038/ni.2172
17. Lang ML. The influence of invariant natural killer T cells on humoral immunity to T-dependent and -independent antigens. Front Immunol. (2018) 9:305. doi: 10.3389/fimmu.2018.00305
18. Vieth JA, Das J, Ranaivoson FM, Comoletti D, Denzin LK, Sant’Angelo DB. TCRalpha-TCRbeta pairing controls recognition of CD1d and directs the development of adipose NKT cells. Nat Immunol. (2017) 18:36–44. doi: 10.1038/ni.3622
19. Cui G, Shimba A, Jin J, Ogawa T, Muramoto Y, Miyachi H, et al. A circulating subset of iNKT cells mediates antitumor and antiviral immunity. Sci Immunol. (2022) 7:eabj8760. doi: 10.1126/sciimmunol.abj8760
20. Yamaguchi Y, Motoki K, Ueno H, Maeda K, Kobayashi E, Inoue H, et al. Enhancing effects of (2S,3S,4R)-1-O-(alpha-D-galactopyranosyl)-2-(N-hexacosanoylamino) -1,3,4-octadecanetriol (KRN7000) on antigen-presenting function of antigen-presenting cells and antimetastatic activity of KRN7000-pretreated antigen-presenting cells. Oncol Res. (1996) 8:399–407.
21. Holzapfel KL, Tyznik AJ, Kronenberg M, Hogquist KA. Antigen-dependent versus -independent activation of invariant NKT cells during infection. J Immunol. (2014) 192:5490–8. doi: 10.4049/jimmunol.1400722
22. Parekh VV, Wilson MT, Olivares-Villagomez D, Singh AK, Wu L, Wang CR, et al. Glycolipid antigen induces long-term natural killer T cell anergy in mice. J Clin Invest. (2005) 115:2572–83. doi: 10.1172/JCI24762
23. Lee YJ, Holzapfel KL, Zhu J, Jameson SC, Hogquist KA. Steady-state production of IL-4 modulates immunity in mouse strains and is determined by lineage diversity of iNKT cells. Nat Immunol. (2013) 14:1146–54. doi: 10.1038/ni.2731
24. Fujii SI, Yamasaki S, Sato Y, Shimizu K. Vaccine designs utilizing invariant NKT-licensed antigen-presenting cells provide NKT or T cell help for B cell responses. Front Immunol. (2018) 9:1267. doi: 10.3389/fimmu.2018.01267
25. Kinjo Y, Takatsuka S, Kitano N, Kawakubo S, Abe M, Ueno K, et al. Functions of CD1d-restricted invariant natural killer T cells in antimicrobial immunity and potential applications for infection control. Front Immunol. (2018) 9:1266. doi: 10.3389/fimmu.2018.01266
26. Kinjo Y, Wu D, Kim G, Xing GW, Poles MA, Ho DD, et al. Recognition of bacterial glycosphingolipids by natural killer T cells. Nature. (2005) 434:520–5. doi: 10.1038/nature03407
27. Mattner J, Debord KL, Ismail N, Goff RD, Cantu C 3rd, Zhou D, et al. Exogenous and endogenous glycolipid antigens activate NKT cells during microbial infections. Nature. (2005) 434:525–9. doi: 10.1038/nature03408
28. Wieland Brown LC, Penaranda C, Kashyap PC, Williams BB, Clardy J, Kronenberg M, et al. Production of alpha-galactosylceramide by a prominent member of the human gut microbiota. PloS Biol. (2013) 11:e1001610. doi: 10.1371/journal.pbio.1001610
29. An D, Oh SF, Olszak T, Neves JF, Avci FY, Erturk-Hasdemir D, et al. Sphingolipids from a symbiotic microbe regulate homeostasis of host intestinal natural killer T cells. Cell. (2014) 156:123–33. doi: 10.1016/j.cell.2013.11.042
30. Cameron G, Nguyen T, Ciula M, Williams SJ, Godfrey DI. Glycolipids from the gut symbiont Bacteroides fragilis are agonists for natural killer T cells and induce their regulatory differentiation. Chem Sci. (2023) 14:7887–96. doi: 10.1039/D3SC02124F
31. Oh SF, Praveena T, Song H, Yoo JS, Jung DJ, Erturk-Hasdemir D, et al. Host immunomodulatory lipids created by symbionts from dietary amino acids. Nature. (2021) 600:302–7. doi: 10.1038/s41586-021-04083-0
32. Strati F, Pujolassos M, Burrello C, Giuffre MR, Lattanzi G, Caprioli F, et al. Antibiotic-associated dysbiosis affects the ability of the gut microbiota to control intestinal inflammation upon fecal microbiota transplantation in experimental colitis models. Microbiome. (2021) 9:39. doi: 10.1186/s40168-020-00991-x
33. Lin Q, Kuypers M, Liu Z, Copeland JK, Chan D, Robertson SJ, et al. Invariant natural killer T cells minimally influence gut microbiota composition in mice. Gut Microbes. (2022) 14:2104087. doi: 10.1080/19490976.2022.2104087
34. Kinjo Y, Tupin E, Wu D, Fujio M, Garcia-Navarro R, Benhnia MR, et al. Natural killer T cells recognize diacylglycerol antigens from pathogenic bacteria. Nat Immunol. (2006) 7:978–86. doi: 10.1038/ni1380
35. Wang J, Li Y, Kinjo Y, Mac TT, Gibson D, Painter GF, et al. Lipid binding orientation within CD1d affects recognition of Borrelia burgdorferi antigens by NKT cells. Proc Natl Acad Sci U S A. (2010) 107:1535–40. doi: 10.1073/pnas.0909479107
36. Lee WY, Moriarty TJ, Wong CH, Zhou H, Strieter RM, van Rooijen N, et al. An intravascular immune response to Borrelia burgdorferi involves Kupffer cells and iNKT cells. Nat Immunol. (2010) 11:295–302. doi: 10.1038/ni.1855
37. Kinjo Y, Illarionov P, Vela JL, Pei B, Girardi E, Li X, et al. Invariant natural killer T cells recognize glycolipids from pathogenic Gram-positive bacteria. Nat Immunol. (2011) 12:966–74. doi: 10.1038/ni.2096
38. Kawakami K, Yamamoto N, Kinjo Y, Miyagi K, Nakasone C, Uezu K, et al. Critical role of Valpha14+ natural killer T cells in the innate phase of host protection against Streptococcus pneumoniae infection. Eur J Immunol. (2003) 33:3322–30. doi: 10.1002/eji.200324254
39. Nakamatsu M, Yamamoto N, Hatta M, Nakasone C, Kinjo T, Miyagi K, et al. Role of interferon-gamma in Valpha14+ natural killer T cell-mediated host defense against Streptococcus pneumoniae infection in murine lungs. Microbes Infect. (2007) 9:364–74. doi: 10.1016/j.micinf.2006.12.003
40. Murray MP, Crosby CM, Marcovecchio P, Hartmann N, Chandra S, Zhao M, et al. Stimulation of a subset of natural killer T cells by CD103(+) DC is required for GM-CSF and protection from pneumococcal infection. Cell Rep. (2022) 38:110209. doi: 10.1016/j.celrep.2021.110209
41. Cohen NR, Tatituri RV, Rivera A, Watts GF, Kim EY, Chiba A, et al. Innate recognition of cell wall beta-glucans drives invariant natural killer T cell responses against fungi. Cell Host Microbe. (2011) 10:437–50. doi: 10.1016/j.chom.2011.09.011
42. Brigl M, Bry L, Kent SC, Gumperz JE, Brenner MB. Mechanism of CD1d-restricted natural killer T cell activation during microbial infection. Nat Immunol. (2003) 4:1230–7. doi: 10.1038/ni1002
43. Girardi E, Yu ED, Li Y, Tarumoto N, Pei B, Wang J, et al. Unique interplay between sugar and lipid in determining the antigenic potency of bacterial antigens for NKT cells. PloS Biol. (2011) 9:e1001189. doi: 10.1371/journal.pbio.1001189
44. Zajonc DM, Girardi E. Recognition of microbial glycolipids by natural killer T cells. Front Immunol. (2015) 6:400. doi: 10.3389/fimmu.2015.00400
45. Velazquez P, Cameron TO, Kinjo Y, Nagarajan N, Kronenberg M, Dustin ML. Cutting edge: activation by innate cytokines or microbial antigens can cause arrest of natural killer T cell patrolling of liver sinusoids. J Immunol. (2008) 180:2024–8. doi: 10.4049/jimmunol.180.4.2024
46. Gonzalez-Aseguinolaza G, Van Kaer L, Bergmann CC, Wilson JM, Schmieg J, Kronenberg M, et al. Natural killer T cell ligand alpha-galactosylceramide enhances protective immunity induced by malaria vaccines. J Exp Med. (2002) 195:617–24. doi: 10.1084/jem.20011889
47. Khan A, Singh S, Galvan G, Jagannath C, Sastry KJ. Prophylactic sublingual immunization with mycobacterium tuberculosis subunit vaccine incorporating the natural killer T cell agonist alpha-galactosylceramide enhances protective immunity to limit pulmonary and extra-pulmonary bacterial burden in mice. Vaccines (Basel). (2017) 5(4):47. doi: 10.3390/vaccines5040047
48. Schmieg J, Yang G, Franck RW, Tsuji M. Superior protection against malaria and melanoma metastases by a C-glycoside analogue of the natural killer T cell ligand alpha-Galactosylceramide. J Exp Med. (2003) 198:1631–41. doi: 10.1084/jem.20031192
49. Miyamoto K, Miyake S, Yamamura T. A synthetic glycolipid prevents autoimmune encephalomyelitis by inducing TH2 bias of natural killer T cells. Nature. (2001) 413:531–4. doi: 10.1038/35097097
50. Gaya M, Barral P, Burbage M, Aggarwal S, Montaner B, Warren Navia A, et al. Initiation of antiviral B cell immunity relies on innate signals from spatially positioned NKT cells. Cell. (2018) 172:517–33 e20. doi: 10.1016/j.cell.2017.11.036
51. Ko SY, Ko HJ, Chang WS, Park SH, Kweon MN, Kang CY. alpha-Galactosylceramide can act as a nasal vaccine adjuvant inducing protective immune responses against viral infection and tumor. J Immunol. (2005) 175:3309–17. doi: 10.4049/jimmunol.175.5.3309
52. Youn HJ, Ko SY, Lee KA, Ko HJ, Lee YS, Fujihashi K, et al. A single intranasal immunization with inactivated influenza virus and alpha-galactosylceramide induces long-term protective immunity without redirecting antigen to the central nervous system. Vaccine. (2007) 25:5189–98. doi: 10.1016/j.vaccine.2007.04.081
53. Kamijuku H, Nagata Y, Jiang X, Ichinohe T, Tashiro T, Mori K, et al. Mechanism of NKT cell activation by intranasal coadministration of alpha-galactosylceramide, which can induce cross-protection against influenza viruses. Mucosal Immunol. (2008) 1:208–18. doi: 10.1038/mi.2008.2
54. Crotty S. T follicular helper cell biology: A decade of discovery and diseases. Immunity. (2019) 50:1132–48. doi: 10.1016/j.immuni.2019.04.011
55. Bai L, Deng S, Reboulet R, Mathew R, Teyton L, Savage PB, et al. (NKT)-B-cell interactions promote prolonged antibody responses and long-term memory to pneumococcal capsular polysaccharides. Proc Natl Acad Sci U S A. (2013) 110:16097–102. doi: 10.1073/pnas.1303218110
56. Kamii Y, Hayashizaki K, Kanno T, Chiba A, Ikegami T, Saito M, et al. IL-27 regulates the differentiation of follicular helper NKT cells via metabolic adaptation of mitochondria. Proc Natl Acad Sci U S A. (2024) 121:e2313964121. doi: 10.1073/pnas.2313964121
57. Shute T, Amiel E, Alam N, Yates JL, Mohrs K, Dudley E, et al. Glycolipid-Containing Nanoparticle Vaccine Engages Invariant NKT Cells to Enhance Humoral Protection against Systemic Bacterial Infection but Abrogates T-Independent Vaccine Responses. J Immunol. (2021) 206:1806–16. doi: 10.4049/jimmunol.2001283
58. Tsuji M, Nair MS, Masuda K, Castagna C, Chong Z, Darling TL, et al. An immunostimulatory glycolipid that blocks SARS-CoV-2, RSV, and influenza infections in vivo. Nat Commun. (2023) 14:3959. doi: 10.1038/s41467-023-39738-1
59. Giaccone G, Punt CJ, Ando Y, Ruijter R, Nishi N, Peters M, et al. A phase I study of the natural killer T-cell ligand alpha-galactosylceramide (KRN7000) in patients with solid tumors. Clin Cancer Res. (2002) 8:3702–9.
60. Padte NN, Boente-Carrera M, Andrews CD, McManus J, Grasperge BF, Gettie A, et al. A glycolipid adjuvant, 7DW8-5, enhances CD8+ T cell responses induced by an adenovirus-vectored malaria vaccine in non-human primates. PloS One. (2013) 8:e78407. doi: 10.1371/journal.pone.0078407
61. Rey-Jurado E, Bohmwald K, Galvez NMS, Becerra D, Porcelli SA, Carreno LJ, et al. Contribution of NKT cells to the immune response and pathogenesis triggered by respiratory viruses. Virulence. (2020) 11:580–93. doi: 10.1080/21505594.2020.1770492
62. Traves R, Opadchy T, Slobedman B, Abendroth A. Varicella zoster virus downregulates expression of the non-classical antigen presentation molecule CD1d. J Infect Dis. (2023). doi: 10.1093/infdis/jiad512
63. Gensollen T, Lin X, Zhang T, Pyzik M, See P, Glickman JN, et al. Embryonic macrophages function during early life to determine invariant natural killer T cell levels at barrier surfaces. Nat Immunol. (2021) 22:699–710. doi: 10.1038/s41590-021-00934-0
64. Wang WB, Lin YD, Zhao L, Liao C, Zhang Y, Davila M, et al. Developmentally programmed early-age skin localization of iNKT cells supports local tissue development and homeostasis. Nat Immunol. (2023) 24:225–38. doi: 10.1038/s41590-022-01399-5
Keywords: iNKT, glycolipid, infection, follicular helper, vaccine
Citation: Hayashizaki K, Kamii Y and Kinjo Y (2024) Glycolipid antigen recognition by invariant natural killer T cells and its role in homeostasis and antimicrobial responses. Front. Immunol. 15:1402412. doi: 10.3389/fimmu.2024.1402412
Received: 17 March 2024; Accepted: 14 May 2024;
Published: 28 May 2024.
Edited by:
Kazuya Iwabuchi, Kitasato University School of Medicine, JapanReviewed by:
Seokmann Hong, Sejong University, Republic of KoreaCopyright © 2024 Hayashizaki, Kamii and Kinjo. This is an open-access article distributed under the terms of the Creative Commons Attribution License (CC BY). The use, distribution or reproduction in other forums is permitted, provided the original author(s) and the copyright owner(s) are credited and that the original publication in this journal is cited, in accordance with accepted academic practice. No use, distribution or reproduction is permitted which does not comply with these terms.
*Correspondence: Yuki Kinjo, eWtpbmpvQGppa2VpLmFjLmpw
Disclaimer: All claims expressed in this article are solely those of the authors and do not necessarily represent those of their affiliated organizations, or those of the publisher, the editors and the reviewers. Any product that may be evaluated in this article or claim that may be made by its manufacturer is not guaranteed or endorsed by the publisher.
Research integrity at Frontiers
Learn more about the work of our research integrity team to safeguard the quality of each article we publish.