- 1Institute of Immunology, Center for Biotechnology and Biomedicine, Faculty of Veterinary Medicine, Leipzig University, Leipzig, Germany
- 2Department of Pathology, Microbiology and Immunology, School of Veterinary Medicine, University of California, Davis, Davis, CA, United States
The dog is an important companion animal and also serves as model species for human diseases. Given the central role of T cells in immune responses, a basic understanding of canine conventional T cell receptor (TCR)αβ+ T cells, comprising CD4+ single-positive (sp) T helper (Th) and CD8α+ sp cytotoxic T cell subsets, is available. However, characterization of canine non-conventional TCRαβ+ CD4+CD8α+ double-positive (dp) and TCRαβ+ CD4−CD8α− double-negative (dn) T cells is limited. In this study, we performed a comprehensive analysis of canine dp and dn T cells in comparison with their conventional counterparts. TCRαβ+ T cells from peripheral blood of healthy dogs were sorted according to their CD4/CD8α phenotype into four populations (i.e. CD4+ sp, CD8α+ sp, dp, and dn) and selected surface markers, transcription factors and effector molecules were analyzed ex vivo and after in vitro stimulation by RT-qPCR. Novel characteristics of canine dp T cells were identified, expanding the previously characterized Th1-like phenotype to Th17-like and Th2-like properties. Overall, mRNA expression of various Th cell-associated cytokines (i.e. IFNG, IL17A, IL4, IL13) in dp T cells upon stimulation highlights their versatile immunological potential. Furthermore, we demonstrated that the CD4-CD8α- dn phenotype is stable during in vitro stimulation. Strikingly, dn T cells were found to express highest mRNA levels of type 2 effector cytokines (IL4, IL5, and IL13) upon stimulation. Their strong ability to produce IL-4 was confirmed at the protein level. Upon stimulation, the percentage of IL-4-producing cells was even higher in the non-conventional dn than in the conventional CD4+ sp population. Constitutive transcription of IL1RL1 (encoding IL-33Rα) further supports Th2-like properties within the dn T cell population. These data point to a role of dn T cells in type 2 immunity. In addition, the high potential of dn T cells to transcribe the gene encoding the co-inhibitory receptor CTLA-4 and to produce the inhibitory cytokine IL-10 indicates putative immunosuppressive capacity of this population. In summary, this study reveals important novel aspects of canine non-conventional T cells providing the basis for further studies on their effector and/or regulatory functions to elucidate their role in health and disease.
1 Introduction
Dogs develop a number of spontaneous immune-mediated disorders, such as autoimmune diseases (1) and allergies (2), as well as cancers (3). Similarities to these complex diseases in humans make the dog particularly interesting as an animal model. Given the importance of T cells in health and disease, comprehensive knowledge of canine T cells is essential to elucidate pathogenesis mechanisms, to develop new treatment strategies, and to appropriately use dogs as a model for these strategies.
Besides the conventional TCRαβ+ T cells expressing either CD4 or CD8α as co-receptor (i.e. CD4+ single-positive (sp) and CD8α+ sp T cells), dogs harbor non-conventional TCRαβ+ CD4+CD8α+ double-positive (dp) (4) and TCRαβ+ CD4-CD8α- double-negative (dn) T cells (5). Whereas conventional cytotoxic CD8 T cells mainly express the CD8αβ heterodimer, canine non-conventional dp T cells predominantly express the CD8αα homodimer (6, 7). Due to their special co-receptor equipment, dp T cells possess unique immunological potential. In humans, dp T cells have been shown to be functionally relevant in cancer, infections and autoimmune diseases (8, 9), while in mice, they have been found to accumulate at the site of injection following subcutaneous vaccination (10). In dogs, increased frequencies of CD4+CD8α+ dp T cells were reported after infection with Ehrlichia chaffeensis (11) and in the context of canine leishmaniasis (12). As shown by our group, mature extrathymic TCRαβ+ dp T cells comprise about 2% of circulating T cells in healthy dogs and are characterized by an activated phenotype indicated by expression of CD25 and MHC-II (6, 13). Furthermore, consistent with their effector memory phenotype, these cells have a strong IFN-γ production capability (6, 7).
Whereas dn T cells account for only 1–3% of all T cells in the peripheral blood of mice and humans (14, 15), in dogs, approximately 15% of all circulating TCRαβ+ T cells are double-negative (5). This substantial population of non-conventional T cells is characterized by a remarkably high expression of CD25. CD25 is either co-expressed with FoxP3 (reminiscent of a regulatory phenotype), or without FoxP3 (reminiscent of an effector phenotype). In addition, subsets expressing IFN-γ, GATA-3, or IL-17A suggest properties of conventional type 1 T helper (Th1), Th2, and Th17 cells, respectively (5).
The recently reported increase of dn T cells after allergen desensitization, as well as the greatly increased frequency of dp T cells in dogs with adverse food reactions (16) indicates that canine non-conventional T cells can be involved in immune responses in vivo. However, knowledge about these cells is still limited.
To gain deeper insight into their phenotype(s) and functional potential, we performed a comprehensive ex vivo and in vitro analysis of canine peripheral blood dp and dn T cells in comparison with their conventional CD4+ sp and CD8α+ sp counterparts.
While dp T cells have versatile immunological potential, our results point to a stable dn phenotype and highlight a remarkable Th2-like potential of dn T cells. Furthermore, their high ability to express inhibitory molecules indicates putative immunosuppressive capacity of canine dn T cells.
2 Materials and methods
2.1 Dogs, blood samples
Peripheral blood was taken by venipuncture of the vena cephalica antebrachii into heparinized collection tubes (BD Vacutainer®, 10 ml, Li-Heparin 17 IU/ml Becton Dickinson, Heidelberg, Germany) from healthy experimental Beagle dogs (7 female, 4 male, age: 4-10 years) of the Faculty of Veterinary Medicine (Leipzig University, Leipzig, Germany). The number of dogs used for individual analyses is indicated in the figure legends. All dogs received routine vaccinations against canine distemper, rabies, canine infectious hepatitis, parvovirus infection, parainfluenza, and leptospirosis. The study was authorized by the Saxony State Office (Landesdirektion Sachsen) in Leipzig, Germany (approval numbers: DD24.1-5131/444/30; DD24.1-5131/468/16).
2.2 Isolation of canine peripheral blood mononuclear cells
PBMC were isolated by density gradient centrifugation. Briefly, blood was diluted with phosphate buffered saline (PBS) at a ratio of 1:1, layered above Biocoll Separating Solution (density 1077 g/l, Biochrom AG, Berlin, Germafny) and centrifuged at 500g for 30 min at room temperature (minimal acceleration and braking). PBMC at the interphase were harvested into PBS and centrifuged at 500g for 10 min at RT. After another washing step with PBS, remaining erythrocytes were lysed by incubation in 150 mM NH4Cl, 8 mM KHCO3, 2 mM EDTA (pH 7.0) for 5 min at RT. The reaction was stopped by addition of PBS containing 3% fetal bovine serum (FBS, Thermo Fisher Scientific, Carlsbad, USA; and PAN-Biotech, Aidenbach, Germany). After washing with PBS, PBMC were counted in Trypan blue (Sigma-Aldrich, Taufkirchen, Germany) using a hemocytometer (Laboroptik, Lancing, UK).
2.3 Fluorescence-activated cell sorting of conventional and non-conventional TCRαβ+ T cell subpopulations
All incubation steps performed to stain cells for fluorescence-activated cell sorting were performed for 15-20 min on ice in the dark. First, PBMC were incubated with the fixable viability dye eFluor 780 (Thermo Fisher Scientific, Carlsbad, USA) according to the manufacturer’s protocol to discriminate viable from dead cells. The subsequent staining steps were preceded by washing with PBS, 3% FCS. To block binding of Fc receptors, cells were preincubated for 5 min with a mixture of heat-inactivated normal serum derived from dog and rat (each 15% in PBS) before addition of the anti-canine TCRαβ (clone CA15.8G7) hybridoma supernatant (Table 1) and further incubation. In the next incubation step, a PerCP/Cy5.5-conjugated goat-anti-mouse IgG secondary antibody (Biolegend, San Diego, USA) was used for detection of TCRαβ. Finally, PBMC were incubated with FITC-conjugated anti-canine CD4 (clone YKIX302.9) and APC-conjugated CD8α (clone YCATE55.9) antibodies (Table 1). Stained cells were applied over a 30 µm filter (Sysmex, Norderstedt, Germany) and immediately sorted using a BD FACSAria™ III Cell Sorter (Becton Dickinson, Heidelberg, Germany). The sorting strategy is shown in Figure 1A. After exclusion of dead cells and doublets, TCRαβ+ T cells from the lymphocyte gate were selected and CD4+ single-positive (sp), CD8α+ sp, CD4brightCD8αbright double-positive (dp), and CD4-CD8α- double-negative (dn) T cell subpopulations were sorted with a purity >99% (Re-analysis using the FlowJo™10 software (Treestar Inc., Ashland, OR, USA).
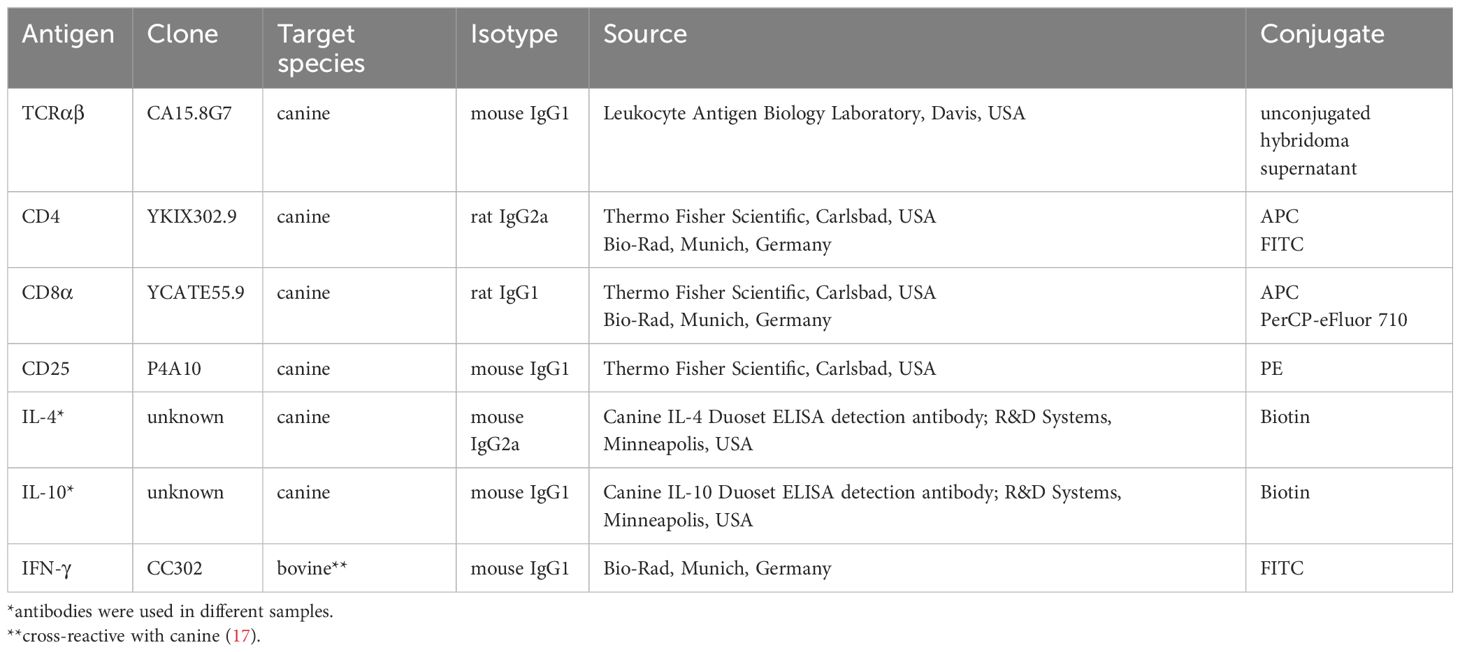
Table 1 Primary antibodies used for fluorescence-activated cell sorting and flow cytometry analysis.
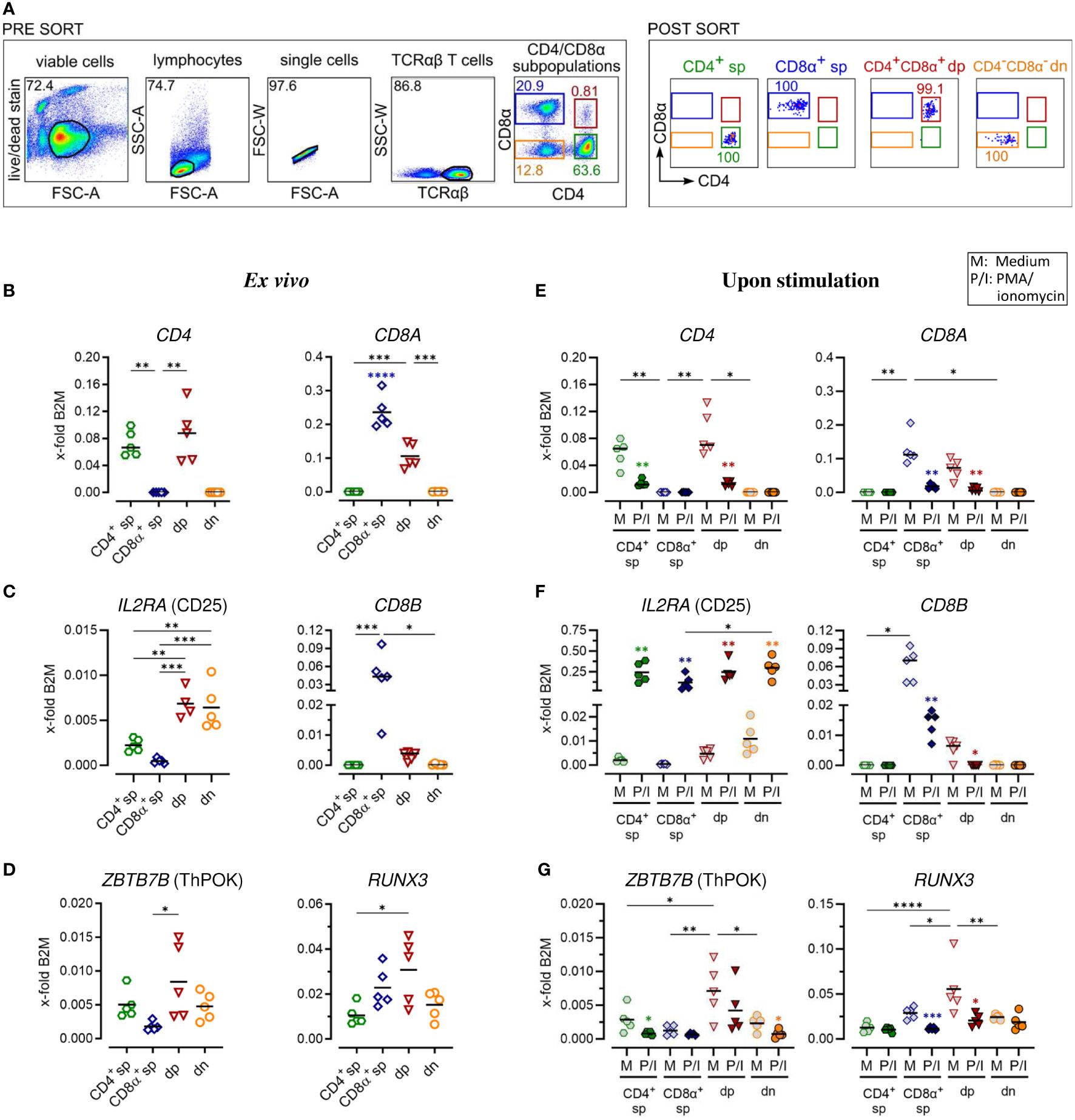
Figure 1 Gene expression analysis of purified canine peripheral conventional and non-conventional TCRαβ+ T cell subpopulations reveals stability of the CD4-CD8α- double-negative phenotype after PMA/Ionomycin stimulation. (A) Strategy for isolation of indicated CD4/CD8α TCRαβ+ subpopulations (sp: single-positive, dp: double-positive, dn: double-negative) by fluorescence-activated cell sorting and the purity (percentages) of the sorted populations are shown (representative data of one dog). (B–G) These populations were analyzed by RT-qPCR. Expression of indicated genes ex vivo (B–D) and after 5 h of medium (M) incubation or stimulation with PMA/ionomycin (P/I) (E–G) defines the sp/dp/dn phenotype of sorted T cell subpopulations. Note that the expression of ThPOK (which is known to be associated with the gene program of CD4 T helper cells) and Runx3 (which establishes the gene program characteristic of cytotoxic CD8 T cells) is significantly higher in CD4+CD8α+ dp T cells compared to CD8α+ sp and CD4+ T cells, respectively. (D, G). Each dot represents one individual dog analyzed separately in independent experiments (n=4 or 5). Depending on normal distribution, data sets are presented with the mean or median (horizontal bars). Statistical differences between subpopulations are marked with lines. Additionally, in (E–G), statistical significance of stimulation-induced effects was calculated for each subpopulation by direct comparison of M versus the P/I equivalent (colored asterisks without lines). (* p < 0.05; ** p < 0.01; *** p < 0.001; **** p < 0.0001). Blue asterisks in (B) indicate significantly increased expression of CD8A in the purified CD8A sp population compared to all other populations. B2M, Beta-2-Microglobulin (reference gene for normalization).
2.4 Stimulation of sorted TCRαβ+ T cell subpopulations and whole PBMC
Isolated TCRαβ+ T cell subpopulations or whole PBMC were seeded at a density of 5x105 per well in IMDM medium with L-Glutamine and 25 mM HEPES (PAA Laboratories, Cölbe, Germany) supplemented with 10% FBS (Thermo Fisher Scientific, Carlsbad, USA), 100 U/ml penicillin, and 100 μg/ml streptomycin (both purchased from PAA Laboratories) into 96-well round bottom cell culture plates (Techno Plastic Products AG, Trasadingen, Switzerland) and rested over night at 37°C and 5% CO2. Then, cells were polyclonally stimulated for 5 hours at 37°C with phorbol myristate acetate and Ionomycin (P/I) (both: 0.22 µg/ml; Sigma-Aldrich, Taufkirchen, Germany). Medium incubation (M) served as negative control. For subsequent intracellular cytokine staining, 5 µg/ml Brefeldin A (BrefA) (Enzo Life Sciences, New York, USA) was added to the culture.
2.5 RNA isolation, reverse transcription and real-time PCR analysis
Total RNA from sorted TCRαβ+ T cell subpopulations was isolated using 1 ml RNA-Solv® Reagent (Omega Bio-tek, Norcross, USA) following manufacturer instructions. To increase the RNA yield, 2 µl glycogen (Thermo Fisher Scientific, Vilnius, Lithuania) were added per sample. The concentration of the RNA was determined spectrophotometrically (NanoDrop, PEQLAB, Erlangen). DNA was digested with one unit DNase (Thermo Fisher Scientific)/µg RNA for 30 min at 37°C. For isolation of poly(A)+ RNA, an additional ethanol precipitation was conducted with 1/8 volume RNase-free 3 M NaOAc, pH 5.7. Reverse transcription was performed using the ‘High Capacity cDNA Reverse Transcription Kit’ (Applied Biosystems, Foster City, USA), containing RiboLock RNase inhibitor (Thermo Fisher Scientific), and a mixture of oligo (dT)18 (Thermo Fisher Scientific) and random hexamer primers in a PTC-200 (MJ Research, Watertown, USA). Specific primers were designed using the primer-BLAST Software (National Center for Biotechnology Information, Bethesda, USA) and synthesized by Metabion (Planegg, Germany) (Table 2). The primers for IL2RA (CD25), IL4, and IL5 were commercially available (Bio-Rad, Munich, Germany). Real-time PCR with SYBR® Green detection was performed using the iTaq Universal SYBR® Green Supermix (Bio-Rad, Munich, Germany) and a CFX Connect real time PCR detection system (Bio-Rad). Specificity was tested by melting curve analysis and an agarose gel electrophoresis (LE agarose, Biozym, Hess. Oldendorf; Germany). Only primers resulting in a single peak in melting curve analysis and a single band with the expected fragment size in gel electrophoresis were used. RT-qPCR efficiencies were calculated with the CFX Maestro Software version 2.2 (Bio-Rad) from standard curves generated by serial dilutions of appropriate cDNA samples (2-fold dilutions, 8 point-curve, conducted in duplicates). The efficiency of all reactions ranged between 90 and 110%. Beta-2-microglobulin (B2M) and succinate dehydrogenase subunit A (SDHA), were used as reference genes of different functional classes with stability verified using the CFX Maestro software based on the GeNorm algorithm (18) (Supplementary Table 1). The same amount of cDNA was used for analysis of expression of the respective reference gene and target genes from the same sample. Relative quantification of the transcripts was done by the 2(-ΔCt) method (19, 20). Results obtained with B2M as reference gene are shown in Figures 1–4, those normalized to SDHA in Supplementary Figures 1–4.
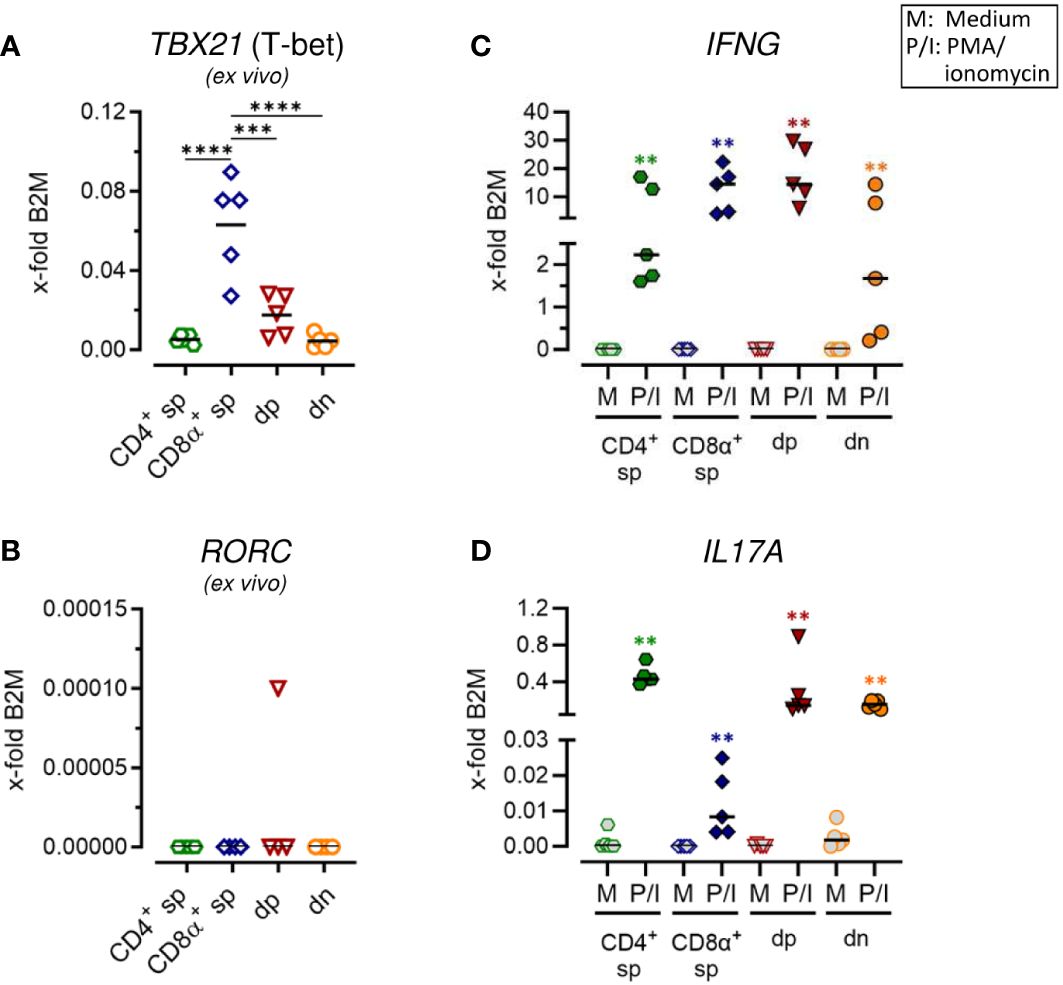
Figure 2 Canine CD4+CD8α+ double-positive T cells comprise T helper (Th) 1 and Th17 expression properties. (A–D) Sorted TCRαβ+ T cell subpopulations, as shown in Figure 1A, were analyzed for expression of indicated genes ex vivo (A, B) or after 5 h of medium (M) incubation or stimulation with PMA/ionomycin (P/I) (C, D) (sp, single-positive; dp, double-positive; dn, double-negative). Each dot represents one individual dog analyzed separately in independent experiments (n=5). Depending on normal distribution, data sets are presented with the mean or median. Statistical differences between subpopulations are marked with lines. Additionally, in (C, D), statistical significance of stimulation-induced effects was calculated for each subpopulation by direct comparison of M versus the P/I equivalent (colored asterisks without lines). (** p < 0.01; *** p < 0.001; **** p < 0.0001).
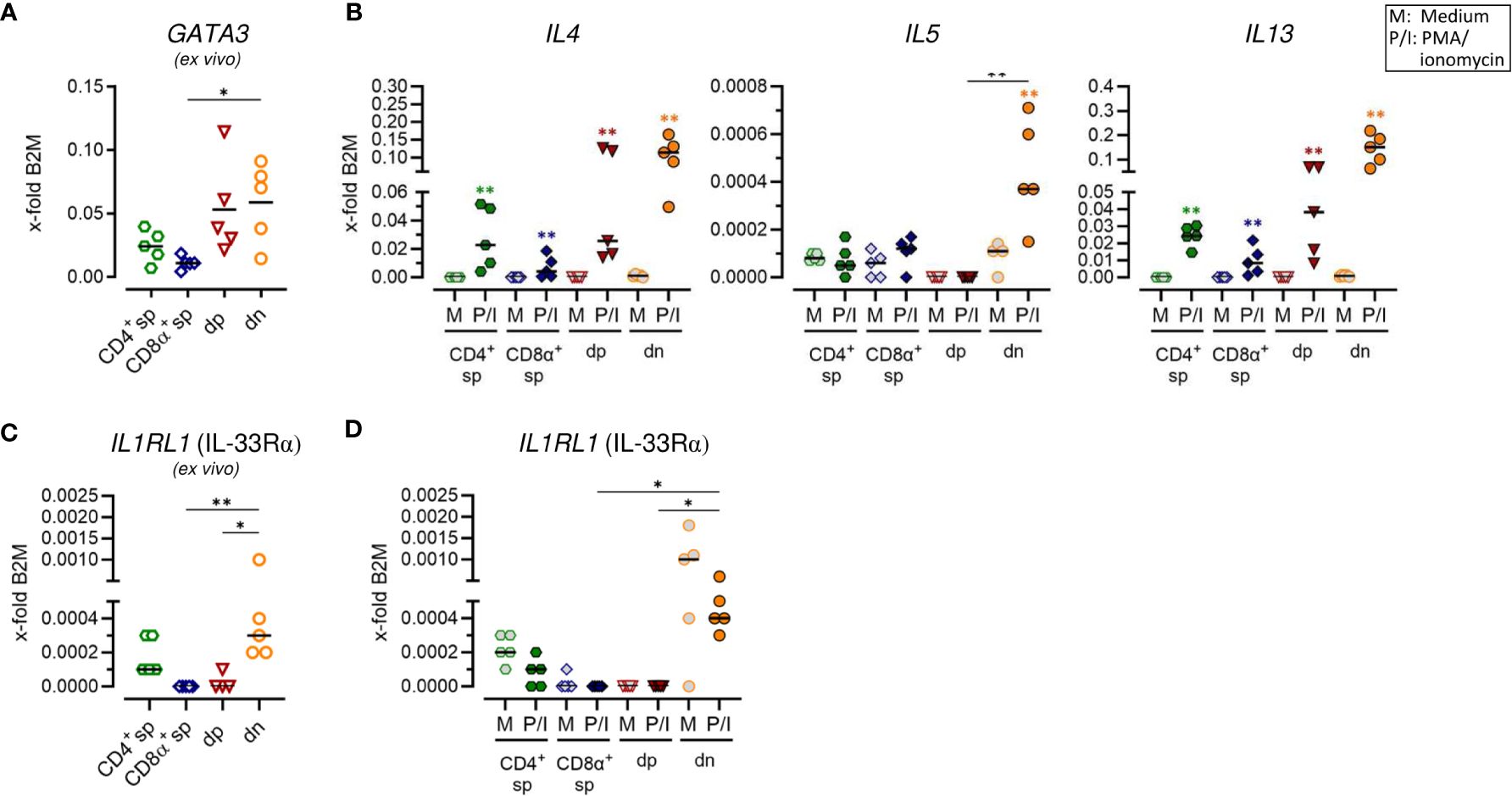
Figure 3 Canine CD4+CD8α+ double-positive and particularly CD4-CD8α- double-negative T cells have a remarkable type 2 potential. (A–D) TCRαβ+ T cell subpopulations sorted as shown in Figure 1A were analyzed for expression of indicated type 2 immune response-related genes ex vivo (A, C) and/or after 5 h of medium (M) incubation or stimulation with PMA/ionomycin (P/I) (B, D) (sp: single-positive, dp: double-positive, dn: double-negative). Each dot represents one individual dog analyzed separately in independent experiments (n=5). Depending on normal distribution, data sets are presented with the mean or median. Statistical differences between subpopulations are marked with lines. Additionally, in (B, D), statistical significance of stimulation-induced effects was calculated for each subpopulation by direct comparison of M versus the P/I equivalent using the Mann-Whitney test (colored asterisks without lines). (* p < 0.05; ** p < 0.01).
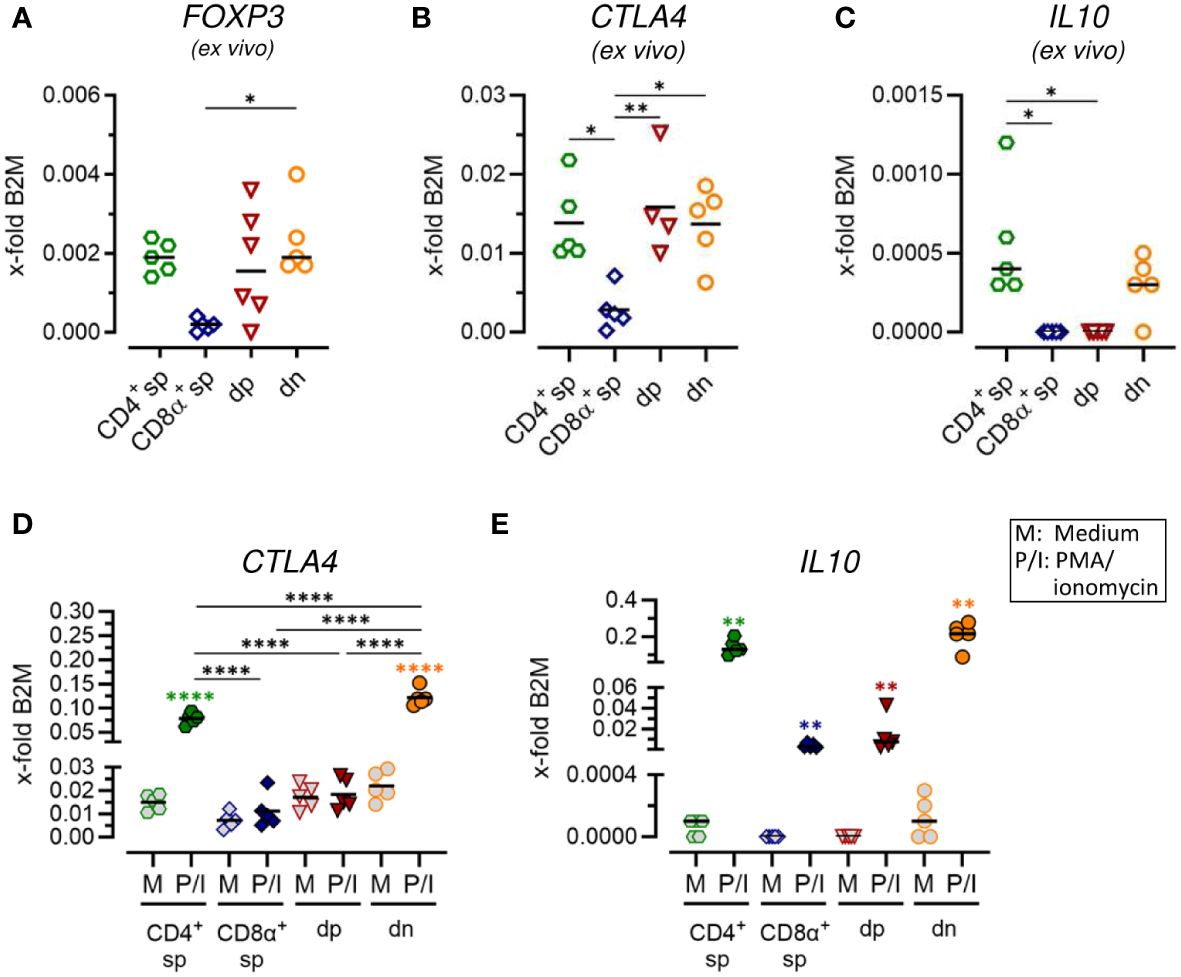
Figure 4 Constitutive expression of key regulatory markers and stimulation-induced upregulation of inhibitory effector molecules indicate immunosuppressive potential of CD4-CD8α- double-negative T cells. (A–E) TCRαβ+ T cell subpopulations sorted as shown in Figure 1A were analyzed for expression of indicated Treg-related genes ex vivo (A–C) or after 5 h of medium (M) incubation or stimulation with PMA/ionomycin (P/I) (D, E) (sp, single-positive; dp, double-positive; dn, double-negative). Each dot represents one individual dog analyzed separately in independent experiments (n=5). Depending on normal distribution, data sets are presented with the mean or median. Statistical differences between subpopulations are marked with lines. Additionally, in (D, E), statistical significance of stimulation-induced effects was calculated for each subpopulation by direct comparison of M versus the P/I equivalent (colored asterisks without lines). (* p < 0.05; ** p < 0.01; **** p < 0.0001).
2.6 Analysis of canine TCRαβ+ T cell subpopulations by flow cytometry
Viability-dye and surface staining of stimulated canine PBMC were essentially performed as described in paragraph 2.3, except for using flow cytometry buffer (3% FBS, 0.1% sodium azide in PBS) instead of PBS/3% FBS. Primary antibodies used are listed in Table 1. For intracellular staining of IL-4, the FoxP3 staining kit (Thermo Fisher Scientific) was used according to the manufacturer’s protocol. For intracellular detection of IL-10, alternatively, cells were fixed with 2% paraformaldehyde (Serva, Heidelberg, Germany) for 15 minutes at 4°C and subsequently permeabilized using 0.5% saponin (w/v, Serva) in flow cytometry buffer. After permeabilization, blocking of Fc receptors was performed by incubation with a mixture of rat, mouse and dog serum (each 15% in PBS) for 10 minutes at room temperature. Cells were incubated for 30 minutes at room temperature with the monoclonal antibodies specific for the selected cytokines (Table 1). Finally, cells were incubated for 20 minutes with PE-Cy7-conjugated streptavidin to detect IL-4 and IL-10, respectively. Data were acquired with a BD LSR Fortessa (Becton Dickinson, Heidelberg, Germany). FlowJo 10.7.1 software (FlowJo, LLC, Ashland, OR, USA) was used for analysis. Gates were set using appropriate controls: unstimulated controls, and fluorescence minus one (FMO) controls, which include all specific antibodies of the staining panel, except the one of interest, which is replaced by its isotype control.
2.7 Statistical analysis
Statistical analysis was performed using Graph Pad Prism 10.2.0 software (San Diego, CA, USA). Depending on normal distribution determined using the Shapiro-Wilk test, data sets are presented with the mean or median. Statistical analysis was performed using One-way ANOVA with Tukey’s post-test or Kruskal-Wallis test with Dunn’s post-test to compare for differences between T cell subpopulations. Additionally, significance of stimulation-induced effects was calculated for each subpopulation by direct comparison of medium (M) versus the PMA/ionomycin-stimulated (P/I) equivalent using the unpaired t test or Mann-Whitney test (two-tailed). The level of confidence for significance is shown in figure legends.
3 Results
3.1 RT-qPCR analysis of sorted canine conventional and non-conventional TCRαβ+ T cell subpopulations demonstrates stability of the CD4-CD8α- double-negative phenotype upon activation
For a detailed investigation, non-conventional TCRαβ+ CD4+CD8α+ double-positive (dp) and CD4-CD8α- double-negative (dn) T cells were compared to conventional CD4+ and CD8α+ single-positive (sp) T cells. All four subpopulations were isolated with high purity (>99%) from peripheral blood of healthy Beagle dogs by fluorescence-activated cell sorting (Figure 1A) and gene expression was analyzed by reverse transcription-quantitative PCR (RT-qPCR). Noteworthy, a very high transcriptional activity of the dp T cell subset was observed. Despite a >20x lower cell yield of the dp T cell subset compared to conventional T cell subpopulations, the resulting amount of RNA was similar in all groups (Table 3), which allowed comparison of gene expression between the populations.
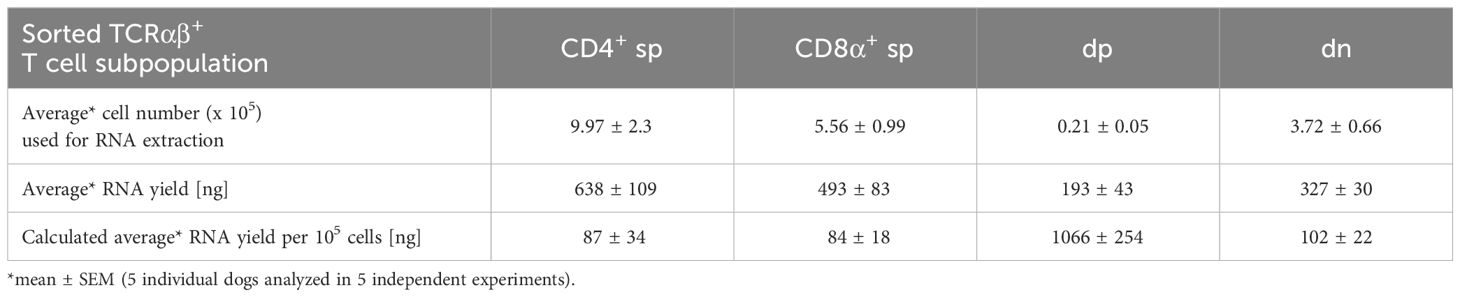
Table 3 RNA yield from sorted T cell populations: The low cell number of dp T cells is compensated by their high transcriptional activity.
The transcription of selected key markers was analyzed ex vivo (Figures 1B–D; Supplementary Figures 1B–D) and after stimulation with PMA/Ionomycin (P/I) (Figures 1E–G; Supplementary Figures 1E–G). In general, gene expression was normalized to the expression levels of two stable reference genes (Supplementary Table 1) of different functional classes, B2M (Figures 1–4) and SDHA (Supplementary Figures 1–4), yielding comparable results. The expression of CD4 and CD8A mRNA ex vivo confirmed the sp/dp/dn phenotype of the sorted T-cell fractions (Figure 1B; Supplementary Figure 1B). Increased IL2RA (CD25) transcription levels ex vivo support the high constitutive activation and/or the IL-2 dependence of both non-conventional T cell subpopulations (Figure 1C; Supplementary Figure 1C) (5, 13). Upregulation of IL2RA (CD25) mRNA expression following P/I incubation confirmed efficient stimulation of each T cell subpopulation (Figure 1F; Supplementary Figure 1F).
In accordance with previous flow cytometric results (13), dp T cells differ from their CD8α+ sp counterparts by only weak constitutive transcription of CD8B (Figure 1C; Supplementary Figure 1C), indicating preferential expression of the CD8αα homodimer. After P/I stimulation, CD8B was almost not detectable in dp T cells, whereas in CD8α+ sp T cells a downregulation was observed (Figure 1F; Supplementary Figure 1F).
Consistent with their CD4+CD8A+ phenotype (Figure 1B; Supplementary Figure 1B), canine peripheral blood dp T cells were characterized by constitutive mRNA expression of genes encoding the transcription factors ThPOK [known to be associated with the CD4 T helper cell gene program (21)] and Runx3 [establishing the gene program characteristic of CD8 cytotoxic T cells (22)] (Figure 1D). Albeit showing broader variation among the five dogs studied, ThPOK and Runx3 expression was significantly elevated in dp in comparison to the CD8α+ sp and CD4+ sp T cell subpopulation, respectively. Dn T cells showed a comparable transcription level of ZBTB7b (encoding ThPOK) and RUNX3 like their CD4+ sp counterparts (Figure 1D; Supplementary Figure 1D). The expected downregulation of CD4 or CD8A mRNA expression in sp and dp populations upon P/I stimulation (5) (Figure 1E; Supplementary Figure 1E) was accompanied by a downregulation of ZBTB7b/RUNX3 (Figure 1G; Supplementary Figure 1G). Notably, the CD4-CD8α- phenotype of the double-negative T cell population was unaltered upon stimulation (Figure 1E; Supplementary Figure 1E).
Overall, RT-qPCR analysis of sort-purified canine TCRαβ+ T cell subpopulations ex vivo and after stimulation confirmed key features of non-conventional dp and dn T cells identified by flow cytometry in previous studies and revealed stability of the dn phenotype upon stimulation in vitro.
3.2 Canine CD4+CD8α+ double-positive T cells show a comparable ability to transcribe IL17A as CD4-CD8α- double-negative T cells and the expected high potential for IFNG expression
Expression of characteristic markers of T helper (Th)1 and Th17 cells was analyzed by RT-qPCR in sorted non-conventional and conventional TCRαβ+ T cell subpopulations. Consistent with previous flow cytometric results showing expression of the transcription factor T-bet only in a small proportion of dp T cells with a CD4bright/CD8αbright phenotype (7), mRNA levels of the corresponding gene TBX21 was significantly lower in this population as compared to CD8α+ sp T cells (Figure 2A; Supplementary Figure 2A). Upon stimulation, high transcription of IFNG (encoding the effector cytokine IFN-γ) was confirmed in both populations (Figure 2C; Supplementary Figure 2C) (5, 13). Albeit to a lesser extent than in CD8α+ sp and dp T cells, a stimulation-dependent increase in transcription of IFNG was also observed in CD4+ sp and dn T cells (Figure 2C; Supplementary Figure 2C), consistent with previous flow cytometric results (5). Expression of RORC, which encodes the lineage-defining transcription factor of Th17 cells RORγt (23, 24), was hardly detectable in any of the sorted populations ex vivo (Figure 2B; Supplementary Figure 2B) and upon stimulation (data not shown). Nevertheless, transcription of the gene encoding the Th17 effector cytokine IL-17A was highly upregulated in CD4+ sp T cells and in dn T cells upon PMA/Iono stimulation (Figure 2D; Supplementary Figure 2D). This is in line with previous flow cytometric findings (5). Interestingly, high stimulation-induced upregulation of IL17A mRNA expression was also found in dp T cells (Figure 2D; Supplementary Figure 2D) suggesting additional Th17 properties in this population.
3.3 Canine CD4+CD8α+ double-positive and particularly CD4-CD8α- double-negative T cells have remarkable T helper 2-like features
To test for a potential role of non-conventional dn and dp T cells in type 2 immune reactions, characteristic features of conventional Th2 cells, including mRNA expression of genes encoding the master transcription factor GATA-3, the key surface marker IL-33Rα as well as the effector cytokines IL-4, IL-5 and IL-13, were analyzed. In line with previous flow cytometric results (5), CD4+ sp T cells constitutively expressed GATA3 and minimal expression was also detected in CD8α sp T cells (Figure 3A; Supplementary Figure 3A). Furthermore, both, dp and dn T cells constitutively transcribed GATA3 (Figure 3A; Supplementary Figure 3A). For dn T cells, this is consistent with previously published flow cytometric results showing an even higher percentage of GATA-3+ cells in the dn than in the CD4+ sp subpopulation (5). For dp T cells, this is the first indication of a role in type 2 immunity. Indeed, dp T cells showed a high capacity for transcription of genes encoding the Th2-associated cytokines IL-4 and IL-13 comparable to their CD4+ sp counterparts (Figure 3B; Supplementary Figure 3B). However, in dp T cells and their CD4+ sp counterparts, stimulation-induced transcription levels of the gene encoding IL-5 were not increased similarly as in CD8α+ sp T cells (Figure 3B; Supplementary Figure 3B). Strikingly, highest mRNA expression levels of all three type 2 effector cytokines IL-4, IL-5, and IL-13 were detected in dn T cells upon PMA/ionomycin stimulation (Figure 3B; Supplementary Figure 3B). Constitutive transcription of IL1RL1 (encoding IL33Rα) in this population further supports the Th2-like phenotype (Figures 3C, D; Supplementary Figures 3C, D).
Taken together, canine non-conventional dp and especially dn T cells show characteristics of conventional Th2 cells and their high ability to transcribe key effector cytokines points to an important involvement in type 2 immune responses.
3.4 Canine CD4-CD8α- double-negative T cells constitutively express key regulatory markers and show stimulation-induced up-regulation of inhibitory molecules
In a previous study, we identified expression of the master transcription factor of conventional CD4+ regulatory T (Treg) cells FoxP3 in a subset of dn T cells (5). Here, we further assessed the potential immunoregulatory phenotype of canine non-conventional dn and dp T cells. Comparable mRNA expression levels of FOXP3 and the inhibitory receptor CTLA4 were observed ex vivo in sorted dn, dp, and conventional CD4+ sp T cells (Figures 4A, B; Supplementary Figures 4A, B). Interestingly, the gene encoding the immunosuppressive cytokine IL-10 was constitutively transcribed by dn and CD4+ sp but not dp T cells (Figure 4C; Supplementary Figure 4C). High up-regulation of IL10 and CTLA4 mRNA expression upon stimulation was detected in CD4+ sp but not CD8α+ sp and dp T cells (Figures 4D, E; Supplementary Figures 4D, E). Intriguingly, dn T cells showed highest stimulation-induced expression levels of both inhibitory molecules, which even exceeded those of conventional CD4+ T cells (Figures 4D, E; Supplementary Figures 4D, E). Taken together, these data point to a putative regulatory role of canine non-conventional dn T cells.
3.5 Canine CD4-CD8α- double-negative T cells are potent IL-4 and IL-10 producers
The capacity of dp and dn T cells to produce IL-4 and IL-10 was analyzed at the protein level by flow cytometry. Analysis of IFN-γ by intracellular cytokine staining (ICS) served as a positive control. As expected (7), IFN-γ was detected in all TCRαβ+ T cell populations analyzed after 5 h of PMA/Iono stimulation, with highest frequencies in dp and CD8α+ T cells, followed by CD4+ sp and dn T cells (Figure 5A).
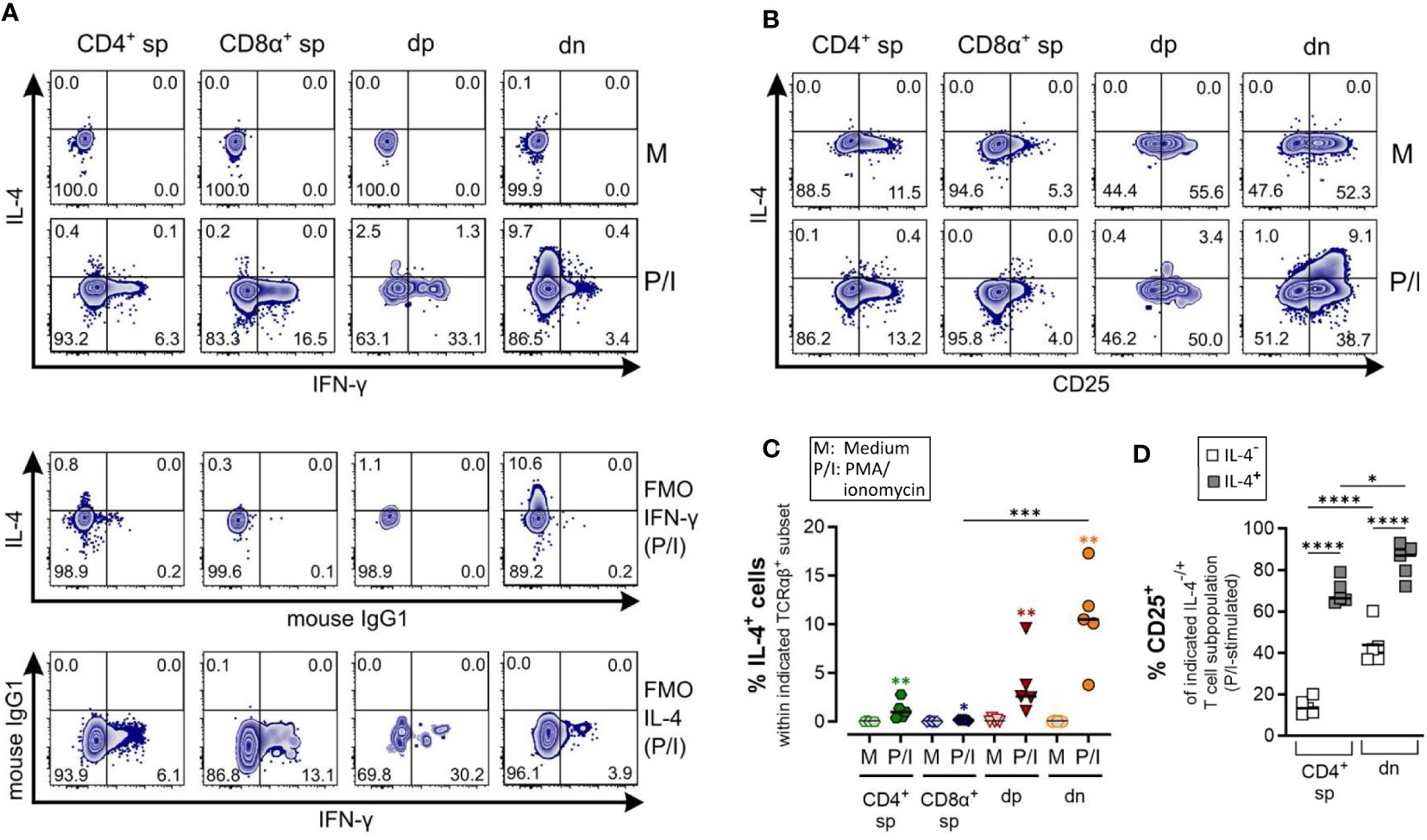
Figure 5 Canine CD4-CD8α- double-negative T cells are potent IL-4 producers upon PMA/Ionomycin stimulation. (A–D) Whole PBMC of five dogs analyzed in three independent experiments were incubated for 5 h in medium (M) or stimulated with PMA/ionomycin (P/I) in the presence of Brefeldin A and intracellular cytokine staining was performed. (A) Representative zebra plots illustrating IL-4 and IFN-γ expression by canine CD4+ single-positive (sp), CD8α+ sp, CD4+CD8α+ double-positive (dp) and CD4-CD8α- double-negative (dn) T cell subpopulations gated as in Figure 1A. Appropriate cytokine gates were set using unstimulated control (medium), and fluorescence minus one (FMO) controls. The dn population shows the highest frequency of IL-4-producing cells upon stimulation. (B) Representative zebra diagrams show that upon stimulation, IL-4 is primarily produced by dn T cells with an activated CD25+ phenotype. (C) Quantification of IL-4+ cells within the indicated TCRαβ+ subpopulations after 5 h of medium (M) incubation or stimulation with PMA/ionomycin (P/I). Horizontal bars indicate median values. Statistical differences between subpopulations are marked with lines. Additionally, statistical significance of stimulation-induced effects was calculated for each subpopulation by direct comparison of M versus the P/I equivalent (colored asterisks without lines) (* p < 0.05; ** p < 0.01; *** p < 0.001). (D) The frequency of CD25+ cells within the IL-4- and IL-4+ fraction of P/I-stimulated CD4+ sp and dn populations was quantified. The horizontal bars indicate mean values. (* p < 0.05; **** p < 0.0001). (CD8α+ sp and dp populations were excluded from this analysis due to limiting absolute numbers of IL-4+ cells).
Specific detection of IL-4 and IL-10 by ICS upon PMA/Iono stimulation was confirmed using several controls, including unstimulated and fluorescence minus one (FMO) controls. CD8α+ sp T cells showed almost no IL-4/IL-10 expression after stimulation (Figures 5, 6).
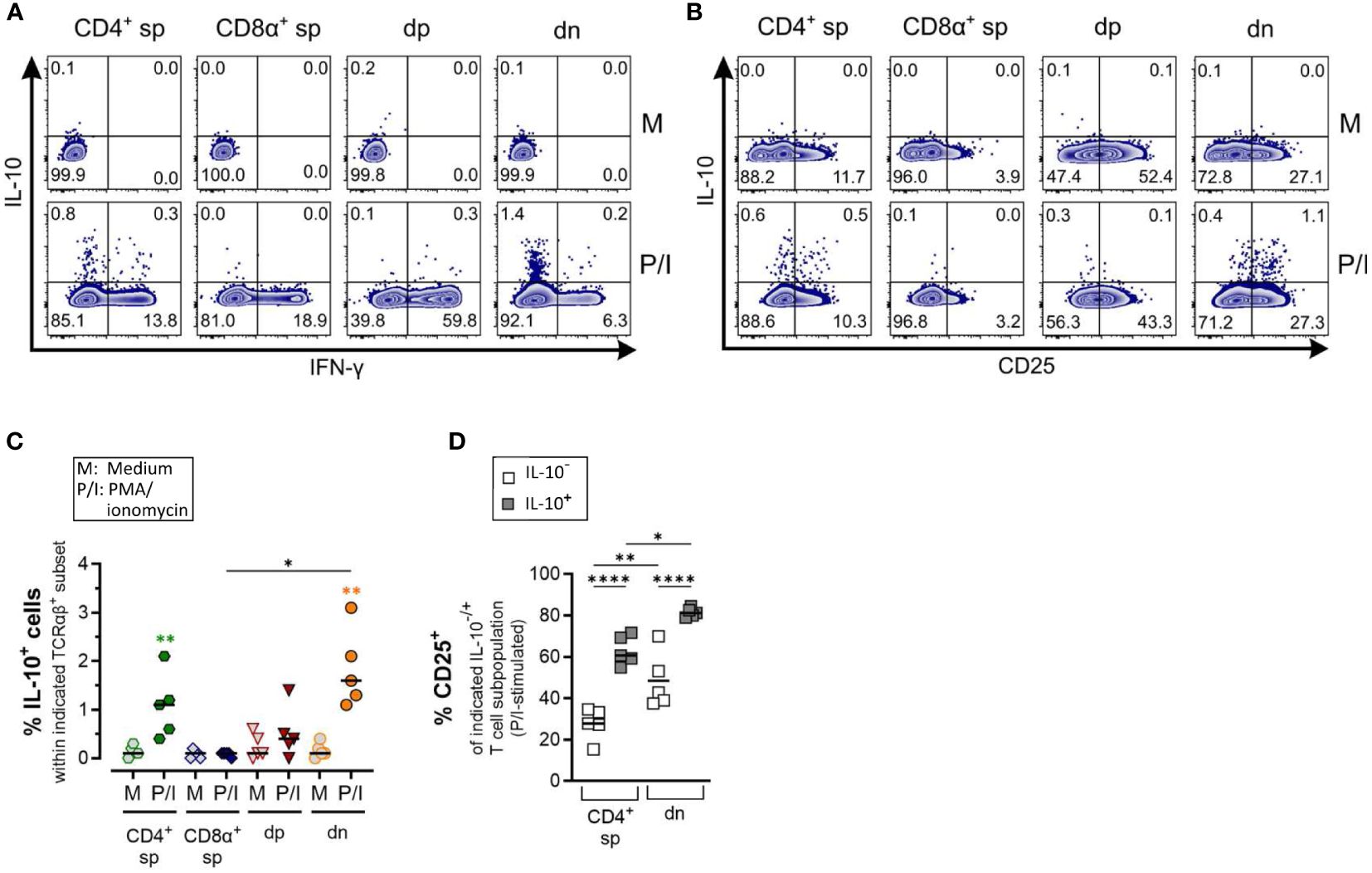
Figure 6 Canine CD4-CD8α- double-negative T cells produce IL-10 upon stimulation (A) Whole PBMC were incubated for 5 h in medium (M) or stimulated with PMA/ionomycin (P/I) in the presence of Brefeldin A and intracellular cytokine staining was performed. (A) Representative zebra plots illustrating IL-10 and IFN-γ expression by canine CD4+ single-positive (sp), CD8α+ sp, CD4+CD8α+ double-positive (dp) and CD4+CD8α+ double-negative (dn) T cell subpopulations gated as in Figure 1A. Appropriate cytokine gates were set using the unstimulated control (medium). Dn T cells show the highest frequency of IL-10-producing cells. (B) IL-10 producers within dn T cells are mainly CD25+. (C) Quantification of IL-10+ cells within the indicated TCRαβ+ subpopulations after 5 h of medium (M) incubation or stimulation with PMA/ionomycin (P/I) (n=5). Horizontal bars indicate median values. Statistical differences between subpopulations are marked with lines. Additionally, statistical significance of stimulation-induced effects was calculated for each subpopulation by direct comparison of M versus the P/I equivalent (colored asterisks without lines) (* p < 0.05; ** p < 0.01). (D) The frequency of CD25+ cells was quantified within the IL-10- and IL-10+ fraction of P/I-stimulated CD4+ sp and dn populations with significant induction of IL-10. Horizontal bars indicate mean values. (* p < 0.05; ** p < 0.01, **** p < 0.0001).
As expected, the Th1 and Th2 effector cytokines, IFN-γ and IL-4, are barely co-produced (Figure 5A). Polyclonal activation-induced IL-4 expression was detected in CD4+ sp T cells, and especially in dn T cells. Of note, the frequency of IL-4+ cells was higher in the dn than in the CD4+ sp population (Figures 5A, C). Furthermore, IL-4+ dn and CD4+ sp T cells exhibit an activated phenotype, indicated by CD25 expression (Figures 5B, D).
Production of the immunosuppressive cytokine IL-10 was significantly induced in dn T cells and CD4+ sp (but not in CD8α+ sp and dp T cells) in response to PMA/Ionomycin stimulation (Figures 6A–C). As expected, the majority of dn T cells producing IL-10 are CD25+ reminiscent of a T regulatory phenotype (Figure 6D).
Taken together, ICS analysis confirmed the high ability of dn T cells to produce IL-4 and IL-10, further supporting a potential role of these non-conventional T cells in type 2 and regulatory immune responses.
4 Discussion
Despite indications of their in vivo relevance, e.g. in the context of desensitization of dogs with food hypersensitivity (16), canine non-conventional dp and dn T cells have been poorly characterized. To gain deeper insight into their phenotype(s) and potential function(s), in this study, peripheral blood non-conventional dp and dn T cells were comprehensively analyzed ex vivo and in vitro upon stimulation in comparison to their conventional CD4 and CD8α+ sp counterparts. Besides identification of novel characteristics of canine dp T cells (e.g. Th17 potential), three remarkable novel features of dn T cells were revealed, i.e. (1) the CD4-CD8α- dn phenotype appears to be stable during in vitro stimulation, (2) dn T cells have a high ability to express Th2-associated factors, and in addition to a putative role in type 2 immunity, (3) canine dn T cells seem to have immunosuppressive capacity indicated by strong expression of regulatory molecules.
A role of canine dn T cells in type 2 immunity was hypothesized based on the previous finding that a significant proportion of these cells express GATA-3 (5), the master transcription factor of conventional Th2 cells (25, 26). To better assess a potential type 2 phenotype of canine dn T cells here, characteristic features of Th2 cells including the ability to transcribe the genes encoding IL-4, IL-5, and IL-13 were investigated. These effector cytokines are associated with distinct functions in vivo: IL-4 is critical for induction of IgE production, IL-5 mediates recruitment of eosinophils, and IL-13 induces goblet cell hyperplasia, mucus production, and smooth muscle contraction (27). In line with GATA3 expression, dn T cells showed highest stimulation-induced mRNA expression of IL4, IL5, and IL13. Their strong ability to produce IL-4 was confirmed at the protein level. Noteworthy, upon stimulation, the percentage of IL-4-producing cells was even higher in the non-conventional dn compared to the conventional CD4+ sp population. This supports an important role of canine GATA-3+ dn T cells in type 2 immunity, like anti-parasite responses and/or allergy. A potential involvement of murine TCRαβ+ dn T cells in type 2 immune responses has been suggested in a single study, showing that murine splenic TCRαβ+ dn T cells are able to produce high amounts of IL-4 upon activation (28). Interestingly, TCRαβ+ dn T cells of healthy humans hardly secrete IL-4 and only some IL-5 upon stimulation (14). The distinct type 2 phenotype of canine non-conventional dn T cells identified in this study was further supported by their constitutive transcription of the gene encoding the IL-33Rα. IL-33 is a well-known key mediator to promote Th2-associated immunity exerting its biological effects via the IL-33R (29). Thus, the impact of IL-33 on the effector functions of canine dn T cells will be an interesting aspect to further investigate in future experiments. Noteworthy, some features of Th2 cells, i.e. transcription of GATA3 and stimulation-dependent up-regulation of IL-4 and IL13 expression were also identified in non-conventional dp T cells in the present study. Similar to human allergic disease, canine allergy is characterized by increased expression of the type 2 cytokines IL-4, IL-5 and IL-13, e.g. in dogs with atopic dermatitis (30–32). In light of our present in vitro data it will be interesting to evaluate whether canine dn T cells are a major cellular source of type 2 cytokines also in vivo and contribute to the pathogenesis of allergic disease.
Besides a potential role in type 2 immunity, canine non-conventional dn T cells are likely involved in immune regulation: In accordance with expression of FoxP3 already shown previously by us (5), we demonstrated a high capacity of dn T cells to transcribe the co-inhibitory receptor CTLA-4 and to produce the immunosuppressive cytokine IL-10 upon stimulation here. Interestingly, the increase of peripheral blood dn T cells in dogs with adverse food reaction after specific immunotherapy could be based on an immunoregulatory role of dn T cells in vivo (16). However, FoxP3 expression as well as immunosuppressive effector molecules were not analyzed in this study. Pinheiro et al., already suspected but did not analyze a possible regulatory function of canine FoxP3+ dn T cells (33). Noteworthy, albeit murine and human dn T cells lack FoxP3 expression (14, 34–36), they were shown to control immune responses both in vitro and in vivo and thus have been termed dn regulatory T cells (14, 37–40). Therefore, it can be speculated that in dogs both, FoxP3+ and FoxP3- regulatory T cells exist. Their actual suppressive capacity needs to be assessed in vitro and in vivo in future experiments.
Noteworthy, in canine dp T cells, in contrast to their conventional CD4+ sp and dn counterparts, FOXP3 mRNA expression is not associated with constitutive transcription of IL10. Additionally, only weak induction of IL10 transcription comparably low as in the CD8α+ sp population and no upregulation of CTLA4 mRNA expression was observed in dp T cells upon stimulation. Whereas FoxP3 is a distinct marker of murine regulatory CD4+ T cells, in humans, activated non-regulatory CD4+ T cells were shown to also express FoxP3 albeit transiently, and at lower levels than human regulatory CD4+ T cells (41, 42). In future experiments, the investigation of further regulatory effector molecules and mechanisms will shed light onto the actual regulatory capacity of dp T cells.
The diverse immunological potential of dp T cells was further highlighted in this study showing induction of mRNA expression of various T helper cell-associated cytokines (i.e., IFNG, IL17A, IL4, IL13) upon stimulation. The increased transcriptional activity of canine dp T cells shown here represents an important prerequisite for future single-cell RNA sequencing analyses, that could shed light on their potential polyfunctionality, i.e. their ability to produce combinations of cytokines at the single-cell level. Furthermore, scRNA-seq including TCR repertoire analysis, recently established for total canine TCRαβ+ T cells by our group (43), should be applied to both, purified dp and dn T cells in future experiments as this could provide initial insights into a potential biased recognition of antigens.
Taken together, the data presented here reveal new insights into phenotypic features and potential functions of canine dp and dn T cells and provide the basis for future in vitro and in vivo studies to elucidate their role in host defense and immunopathological diseases of dogs.
Data availability statement
The original contributions presented in the study are included in the article/Supplementary Material. Further inquiries can be directed to the corresponding author.
Ethics statement
The animal study was approved by Saxony State Office (Landesdirektion Sachsen), Leipzig, Germany (approval numbers: DD24.1-5131/444/30; DD24.1-5131/468/16). The study was conducted in accordance with the local legislation and institutional requirements.
Author contributions
MP: Data curation, Formal analysis, Investigation, Methodology, Visualization, Writing – original draft, Writing – review & editing. DD: Data curation, Formal analysis, Investigation, Methodology, Writing – review & editing. PM: Resources, Writing – review & editing. MB: Conceptualization, Writing – review & editing. GA: Conceptualization, Funding acquisition, Resources, Supervision, Writing – review & editing. ME: Conceptualization, Data curation, Formal analysis, Funding acquisition, Investigation, Methodology, Project administration, Supervision, Visualization, Writing – original draft, Writing – review & editing.
Funding
The author(s) declare financial support was received for the research, authorship, and/or publication of this article. This study was funded by grants AL 371/8-3 (to GA) and ES 645/1-1 (to ME) from the German Research Foundation (Deutsche Forschungsgemeinschaft, DFG). The authors further acknowledge support from the German Research Foundation (DFG) and Universität Leipzig within the program of OpenAccess Publishing.
Acknowledgments
The authors thank Ina Hochheim and Caroline Schöller from the Institute of Pharmacology, Pharmacy and Toxicology, College of Veterinary Medicine, University of Leipzig for extensive care of the dogs and providing blood samples. Aileen Wingenfeld and Anett Grohs are thanked for their excellent technical assistance. Dr. Christiane Schnabel and Dr. Uwe Müller are thanked for critical reading of the manuscript. Flow cytometry was performed at the Core Unit Flow Cytometry (CUDZ) of the Faculty of Veterinary Medicine, Leipzig University.
Conflict of interest
The authors declare that the research was conducted in the absence of any commercial or financial relationships that could be construed as a potential conflict of interest.
Publisher’s note
All claims expressed in this article are solely those of the authors and do not necessarily represent those of their affiliated organizations, or those of the publisher, the editors and the reviewers. Any product that may be evaluated in this article, or claim that may be made by its manufacturer, is not guaranteed or endorsed by the publisher.
Supplementary material
The Supplementary Material for this article can be found online at: https://www.frontiersin.org/articles/10.3389/fimmu.2024.1400550/full#supplementary-material
References
1. Gershwin LJ. Veterinary autoimmunity: autoimmune diseases in domestic animals. Ann N Y Acad Sci. (2007) 1109:109–16. doi: 10.1196/annals.1398.013
2. Mueller RS, Jensen-Jarolim E, Roth-Walter F, Marti E, Janda J, Seida AA, et al. Allergen immunotherapy in people, dogs, cats and horses - differences, similarities and research needs. Allergy. (2018) 73:1989–99. doi: 10.1111/all.13464
3. Overgaard NH, Fan TM, Schachtschneider KM, Principe DR, Schook LB, Jungersen G. Of mice, dogs, pigs, and men: choosing the appropriate model for immuno-oncology research. ILAR J. (2018) 59:247–62. doi: 10.1093/ilar/ily014
4. Buttlar Hv, Bismarck D, Alber G. Peripheral canine CD4(+)CD8(+) double-positive T cells - unique amongst others. Vet Immunol Immunopathol. (2015) 168:169–75. doi: 10.1016/j.vetimm.2015.09.005
5. Rabiger FV, Rothe K, Buttlar Hv, Bismarck D, Büttner M, Moore PF, et al. Distinct features of canine non-conventional CD4-CD8α- double-negative TCRαβ+ vs. TCRγδ+ T cells. Front Immunol. (2019) 10:2748. doi: 10.3389/fimmu.2019.02748
6. Bismarck D, Schütze N, Moore P, Büttner M, Alber G, Buttlar Hv. Canine CD4+CD8+ double positive T cells in peripheral blood have features of activated T cells. Vet Immunol Immunopathol. (2012) 149:157–66. doi: 10.1016/j.vetimm.2012.06.014
7. Rothe K, Bismarck D, Büttner M, Alber G, Buttlar Hv. Canine peripheral blood CD4+CD8+ double-positive Tcell subpopulations exhibit distinct Tcell phenotypes and effector functions. Vet Immunol Immunopathol. (2017) 185:48–56. doi: 10.1016/j.vetimm.2017.01.005
8. Nishida K, Kawashima A, Kanazawa T, Kidani Y, Yoshida T, Hirata M, et al. Clinical importance of the expression of CD4+CD8+ T cells in renal cell carcinoma. Int Immunol. (2020) 32:347–57. doi: 10.1093/intimm/dxaa004
9. Overgaard NH, Jung J-W, Steptoe RJ, Wells JW. CD4+/CD8+ double-positive T cells: more than just a developmental stage? J Leukoc Biol. (2015) 97:31–8. doi: 10.1189/jlb.1RU0814-382
10. Overgaard NH, Cruz JL, Bridge JA, Nel HJ, Frazer IH, La Gruta NL, et al. CD4+CD8β+ double-positive T cells in skin-draining lymph nodes respond to inflammatory signals from the skin. J Leukoc Biol. (2017) 102:837–44. doi: 10.1189/jlb.1AB0217-065R
11. McGill JL, Wang Y, Ganta CK, Boorgula GD, Ganta RR. Antigen-specific CD4+CD8+ Double-positive T cells are increased in the blood and spleen during ehrlichia chaffeensis infection in the canine host. Front Immunol. (2018) 9:1585. doi: 10.3389/fimmu.2018.01585
12. Alexandre-Pires G, Brito MTde, Algueró C, Martins C, Rodrigues OR, Da Fonseca IP, et al. Canine leishmaniasis. Immunophenotypic profile of leukocytes in different compartments of symptomatic, asymptomatic and treated dogs. Vet Immunol Immunopathol. (2010) 137:275–83. doi: 10.1016/j.vetimm.2010.06.007
13. Rabiger FV, Bismarck D, Protschka M, Köhler G, Moore PF, Büttner M, et al. Canine tissue-associated CD4+CD8α+ double-positive T cells are an activated T cell subpopulation with heterogeneous functional potential. PloS One. (2019) 14:e0213597. doi: 10.1371/journal.pone.0213597
14. Fischer K, Voelkl S, Heymann J, Przybylski GK, Mondal K, Laumer M, et al. Isolation and characterization of human antigen-specific TCR alpha beta+ CD4(-)CD8- double-negative regulatory T cells. Blood. (2005) 105:2828–35. doi: 10.1182/blood-2004-07-2583
15. Zhang ZX, Yang L, Young KJ, DuTemple B, Zhang L. Identification of a previously unknown antigen-specific regulatory T cell and its mechanism of suppression. Nat Med. (2000) 6:782–9. doi: 10.1038/77513
16. Maina E, Devriendt B, Cox E. Food allergen-specific sublingual immunotherapy modulates peripheral T cell responses of dogs with adverse food reactions. Vet Immunol Immunopathol. (2019) 212:38–42. doi: 10.1016/j.vetimm.2019.05.003
17. Pedersen LG, Castelruiz Y, Jacobsen S, Aasted B. Identification of monoclonal antibodies that cross-react with cytokines from different animal species. Vet Immunol Immunopathol. (2002) 88:111–22. doi: 10.1016/S0165-2427(02)00139-3
18. Vandesompele J, Preter K, Pattyn F, Poppe B, van Roy N, Paepe A, et al. Accurate normalization of real-time quantitative RT-PCR data by geometric averaging of multiple internal control genes. Genome Biol. (2002) 3:RESEARCH0034. doi: 10.1186/gb-2002-3-7-research0034
19. Pfaffl MW. A new mathematical model for relative quantification in real-time RT-PCR. Nucleic Acids Res. (2001) 29:e45. doi: 10.1093/nar/29.9.e45
20. Livak KJ, Schmittgen TD. Analysis of relative gene expression data using real-time quantitative PCR and the 2(-Delta Delta C(T)) Method. Methods. (2001) 25:402–8. doi: 10.1006/meth.2001.1262
21. He X, Park K, Kappes DJ. The role of ThPOK in control of CD4/CD8 lineage commitment. Annu Rev Immunol. (2010) 28:295–320. doi: 10.1146/annurev.immunol.25.022106.141715
22. Park J-H, Adoro S, Guinter T, Erman B, Alag AS, Catalfamo M, et al. Signaling by intrathymic cytokines, not T cell antigen receptors, specifies CD8 lineage choice and promotes the differentiation of cytotoxic-lineage T cells. Nat Immunol. (2010) 11:257–64. doi: 10.1038/ni.1840
23. Ivanov II, McKenzie BS, Zhou L, Tadokoro CE, Lepelley A, Lafaille JJ, et al. The orphan nuclear receptor RORgammat directs the differentiation program of proinflammatory IL-17+ T helper cells. Cell. (2006) 126:1121–33. doi: 10.1016/j.cell.2006.07.035
24. Manel N, Unutmaz D, Littman DR. The differentiation of human T(H)-17 cells requires transforming growth factor-beta and induction of the nuclear receptor RORgammat. Nat Immunol. (2008) 9:641–9. doi: 10.1038/ni.1610
25. Zhang DH, Cohn L, Ray P, Bottomly K, Ray A. Transcription factor GATA-3 is differentially expressed in murine Th1 and Th2 cells and controls Th2-specific expression of the interleukin-5 gene. J Biol Chem. (1997) 272:21597–603. doi: 10.1074/jbc.272.34.21597
26. Zheng W, Flavell RA. The transcription factor GATA-3 is necessary and sufficient for Th2 cytokine gene expression in CD4 T cells. Cell. (1997) 89:587–96. doi: 10.1016/S0092-8674(00)80240-8
27. Finkelman FD, Hogan SP, Hershey GK, Rothenberg ME, Wills-Karp M. Importance of cytokines in murine allergic airway disease and human asthma. J Immunol. (2010) 184:1663–74. doi: 10.4049/jimmunol.0902185
28. Zlotnik A, Godfrey DI, Fischer M, Suda T. Cytokine production by mature and immature CD4-CD8- T cells. Alpha beta-T cell receptor+ CD4-CD8- T cells produce IL-4. J Immunol. (1992) 149:1211–5. doi: 10.4049/jimmunol.149.4.1211
29. Peine M, Marek RM, Löhning M. IL-33 in T cell differentiation, function, and immune homeostasis. Trends Immunol. (2016) 37:321–33. doi: 10.1016/j.it.2016.03.007
30. Majewska A, Gajewska M, Dembele K, Maciejewski H, Prostek A, Jank M. Lymphocytic, cytokine and transcriptomic profiles in peripheral blood of dogs with atopic dermatitis. BMC Vet Res. (2016) 12:174. doi: 10.1186/s12917-016-0805-6
31. Nuttall TJ, Knight PA, McAleese SM, Lamb JR, Hill PB. Expression of Th1, Th2 and immunosuppressive cytokine gene transcripts in canine atopic dermatitis. Clin Exp Allergy. (2002) 32:789–95. doi: 10.1046/j.1365-2222.2002.01356.x
32. Olivry T, Mayhew D, Paps JS, Linder KE, Peredo C, Rajpal D, et al. Early activation of th2/th22 inflammatory and pruritogenic pathways in acute canine atopic dermatitis skin lesions. J Invest Dermatol. (2016) 136:1961–9. doi: 10.1016/j.jid.2016.05.117
33. Pinheiro D, Singh Y, Grant CR, Appleton RC, Sacchini F, Walker KR, et al. Phenotypic and functional characterization of a CD4(+) CD25(high) FOXP3(high) regulatory T-cell population in the dog. Immunology. (2011) 132:111–22. doi: 10.1111/j.1365-2567.2010.03346.x
34. Voelkl S, Gary R, Mackensen A. Characterization of the immunoregulatory function of human TCR-αβ+ CD4- CD8- double-negative T cells. Eur J Immunol. (2011) 41:739–48. doi: 10.1002/eji.201040982
35. Zhang D, Yang W, Degauque N, Tian Y, Mikita A, Zheng XX. New differentiation pathway for double-negative regulatory T cells that regulates the magnitude of immune responses. Blood. (2007) 109:4071–9. doi: 10.1182/blood-2006-10-050625
36. Hillhouse EE, Lesage S. A comprehensive review of the phenotype and function of antigen-specific immunoregulatory double negative T cells. J Autoimmun. (2013) 40:58–65. doi: 10.1016/j.jaut.2012.07.010
37. Tian D, Yang L, Wang S, Zhu Y, Shi W, Zhang C, et al. Double negative T cells mediate Lag3-dependent antigen-specific protection in allergic asthma. Nat Commun. (2019) 10:4246. doi: 10.1038/s41467-019-12243-0
38. Sun G, Zhao X, Li M, Zhang C, Jin H, Li C, et al. CD4 derived double negative T cells prevent the development and progression of nonalcoholic steatohepatitis. Nat Commun. (2021) 12:650. doi: 10.1038/s41467-021-20941-x
39. Haug T, Aigner M, Peuser MM, Strobl CD, Hildner K, Mougiakakos D, et al. Human double-negative regulatory T-cells induce a metabolic and functional switch in effector T-cells by suppressing mTOR activity. Front Immunol. (2019) 10:883. doi: 10.3389/fimmu.2019.00883
40. Zhang ZX, Young K, Zhang L. CD3+CD4-CD8- alphabeta-TCR+ T cell as immune regulatory cell. J Mol Med (Berl). (2001) 79:419–27. doi: 10.1007/s001090100238
41. Allan SE, Crome SQ, Crellin NK, Passerini L, Steiner TS, Bacchetta R, et al. Activation-induced FOXP3 in human T effector cells does not suppress proliferation or cytokine production. Int Immunol. (2007) 19:345–54. doi: 10.1093/intimm/dxm014
42. Wang J, Ioan-Facsinay A, van der Voort EI, Huizinga TW, Toes RE. Transient expression of FOXP3 in human activated nonregulatory CD4+ T cells. Eur J Immunol. (2007) 37:129–38. doi: 10.1002/eji.200636435
Keywords: dog, canine TCRαβ+ T cells, CD4-CD8α- double-negative, CD4+CD8α+ double-positive, non-conventional T cells, Th2 Cells, Treg cells, peripheral blood
Citation: Protschka M, Di Placido D, Moore PF, Büttner M, Alber G and Eschke M (2024) Canine peripheral non-conventional TCRαβ+ CD4-CD8α- double-negative T cells show T helper 2-like and regulatory properties. Front. Immunol. 15:1400550. doi: 10.3389/fimmu.2024.1400550
Received: 13 March 2024; Accepted: 29 April 2024;
Published: 21 May 2024.
Edited by:
Enric M. Mateu, Autonomous University of Barcelona, SpainReviewed by:
Tobias Kaeser, University of Veterinary Medicine Vienna, AustriaFumihiko Katakura, Nihon University, Japan
Copyright © 2024 Protschka, Di Placido, Moore, Büttner, Alber and Eschke. This is an open-access article distributed under the terms of the Creative Commons Attribution License (CC BY). The use, distribution or reproduction in other forums is permitted, provided the original author(s) and the copyright owner(s) are credited and that the original publication in this journal is cited, in accordance with accepted academic practice. No use, distribution or reproduction is permitted which does not comply with these terms.
*Correspondence: Maria Eschke, maria.eschke@bbz.uni-leipzig.de
†Present address: Daniela Di Placido, Department of Infectious Diseases, Istituto Superiore di Sanità, Rome, Italy