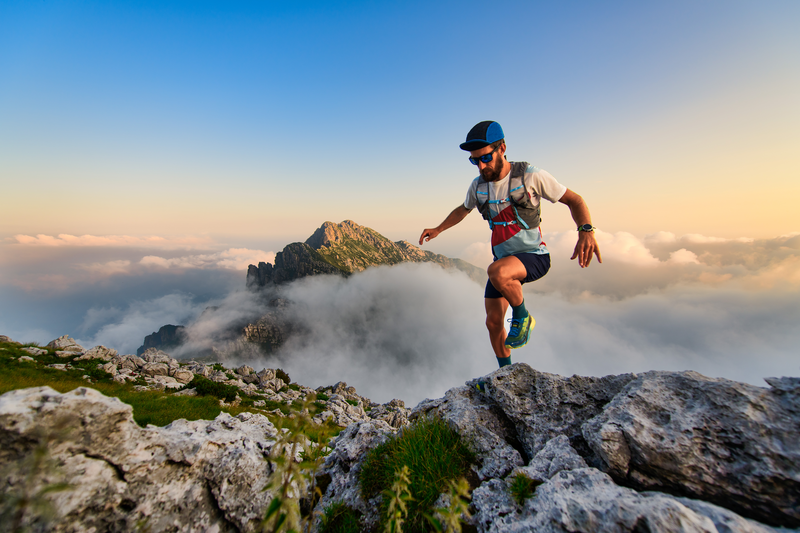
94% of researchers rate our articles as excellent or good
Learn more about the work of our research integrity team to safeguard the quality of each article we publish.
Find out more
ORIGINAL RESEARCH article
Front. Immunol. , 07 June 2024
Sec. Viral Immunology
Volume 15 - 2024 | https://doi.org/10.3389/fimmu.2024.1397052
Background: Immunocompromised patients are at particular risk of Severe Acute Respiratory Syndrome Corona Virus 2 (SARS-CoV-2) infection and previous findings suggest that the infection or vaccination induced immune response decreases over time. Our main goal was to investigate the SARS-CoV-2-specific immune response in rheumatoid arthritis patients and healthy controls over prolonged time.
Methods: The SARS-CoV-2-specific humoral immune response was measured by Elecsys Anti-SARS-CoV-2 Spike (S) immunoassay, and antibodies against SARS-CoV-2 nucleocapsid protein (NCP) were also evaluated by Euroimmun enzyme-linked immunosorbent assay (ELISA) test. The SARS-CoV-2-specific T-cell response was detected by an IFN- γ release assay.
Results: We prospectively enrolled 84 patients diagnosed with rheumatoid arthritis (RA) and 43 healthy controls in our longitudinal study. Our findings demonstrate that RA patients had significantly lower anti-S antibody response and reduced SARS-CoV-2-specific T-cell response compared to healthy controls (p<0.01 for healthy controls, p<0.001 for RA patients). Furthermore, our results present evidence of a notable increase in the SARS-CoV-2-specific humoral immune response during the follow-up period in both study groups (p<0.05 for healthy volunteers, p<0.0001 for RA patients, rank-sum test). Participants who were vaccinated against Coronavirus disease-19 (COVID-19) during the interim period had 2.72 (CI 95%: 1.25–5.95, p<0.05) times higher anti-S levels compared to those who were not vaccinated during this period. Additionally, individuals with a confirmed SARS-CoV-2 infection exhibited 2.1 times higher (CI 95%: 1.31–3.37, p<0.01) anti-S levels compared to those who were not infected during the interim period. It is worth noting that patients treated with targeted therapy had 52% (CI 95%: 0.25–0.94, p<0.05) lower anti-S levels compared to matched patients who did not receive targeted therapy. Concerning the SARS-CoV-2-specific T-cell response, our findings revealed that its level had not changed substantially in the study groups.
Conclusion: Our present data revealed that the level of SARS-CoV-2-specific humoral immune response is actually higher, and the SARS-CoV-2-specific T-cell response remained at the same level over time in both study groups. This heightened humoral response, the nearly permanent SARS-CoV-2-specific T-cell response and the coexistence of different SARS-CoV-2 variants within the population, might be contributing to the decline in severe COVID-19 cases.
The emergence of a new kind of coronavirus in 2019, the SARS-CoV-2, caused severe worldwide consequences and led to millions of deaths all over the world (1, 2).
The SARS-CoV-2 specific adaptive immune responses induced either by natural infection or vaccination play a crucial role in the control of the infection (3–7). In most acute viral infections, neutralizing antibodies increase rapidly after infection and persist for many years due to long-lasting plasma cells and memory B cells, playing vital roles in viral clearance and protection against viral diseases (8). However, it was shown that the antibody response triggered by coronaviruses in humans tends to wane over time (9). Currently, only few studies have investigated the dynamic change and the longevity of SARS-CoV-2 specific immune responses (10–13), although it is important to understand how natural reinfections, booster vaccination doses, different immunosuppressive drugs could influence the long-term immune response.
Patients with autoimmune rheumatic diseases have demonstrated heightened susceptibility to developing SARS-CoV-2 infection and hospitalization (14, 15), and as a consequence, they have been deemed a priority target group for the distribution of basic and booster vaccinations, too (16–18). Previously, we investigated the factors influencing the SARS-CoV-2 infection and vaccination-induced immune response in RA (19). In accordance with earlier investigations (20–22), we showed that following vaccination against COVID-19 (one or two vaccination doses) or SARS-CoV-2 infection, the induced anti-S antibodies decreased rapidly: approximately by 25% in each month, and we predicted that many RA patients would have sub-therapeutic antibody levels after 12 months. Additionally, based on our results, RA patients treated with tumor necrosis factor (TNF)-α-inhibitors, interleukin (IL)-6-inhibitor had reduced SARS-CoV-2-specific antibody response and anti-CD20 therapy inhibited both SARS-CoV-2-induced humoral and cellular immune responses (19).
Here, we studied the SARS-CoV-2-specific immunity longitudinally in rheumatoid arthritis patients and healthy controls.
All 84 patients with RA included in this study were recruited from the rheumatology outpatient department of Semmelweis University (Buda Hospital of the Hospitaller Order of Saint John of God) in Budapest, Hungary. The study was conducted with the approval number IV/2021–1/2021/EKU from 19.10.2022 until 01.03.2023. Patients for the study were 18 years old or above who received COVID-19 vaccination and/or had previous confirmed SARS-CoV-2 infection and were diagnosed with RA according to the 2010 American College of Rheumatology/European League Against Rheumatism classification criteria (23). A control group consisting of 43 healthy individuals was also included in the study. These individuals were vaccinated against COVID-19 and/or had previous confirmed SARS-CoV-2 infection. Peripheral blood samples were collected to measure the SARS-CoV-2 induced humoral and cellular immune responses in all participants. Written, informed consent was obtained from all participants.
The analysis of SARS-CoV-2-specific antibodies was performed using the Elecsys Anti-SARS-CoV-2 S immunoassay, developed by Roche. This assay was conducted on the Cobas e6000 instrument. The test is designed to detect antibodies specifically targeting the receptor binding domain (RBD) of the S protein of SARS-CoV-2 in human serum and plasma samples. The immunoassay uses a recombinant protein that represents the RBD of the S antigen in a double-antigen sandwich assay format. This format is particularly effective in detecting high-affinity antibodies (IgG, IgA, IgM) against SARS-CoV-2 in a quantitative manner. The method uses electrochemiluminescence for the quantitative determination of these antibodies. The limit of quantification for this assay is 0.4 U/ml, which means that results below this threshold are considered negative. Results equal to or above 0.8 U/ml are considered positive, as described in the test documentation (24).
The level of specific antibodies (IgG) against NCP from the SARS-CoV-2 virus in a blood sample was measured by Euroimmun ELISA test. It provides a semi-quantitative assessment, meaning it gives an approximate measurement rather than an exact value. The results are presented as a ratio, comparing the absorbance of the sample to a standard calibrator. If the ratio is below 0.8, it is considered negative for the presence of antibodies. Ratios equal to or above 1.1 are considered positive, indicating the presence of antibodies according to the test’s guidelines (25).
A small amount (1 ml) of heparinized whole blood was stimulated with specific antigens (S1, S2, RBD subdomains) that induce immune response in certain types of T-cells (CD4+ and CD4+CD8+ T-cells). In one test tube, additional specific peptides were added to cover various parts of the SARS-CoV-2 virus (spike, nucleocapsid, and M protein domains) to provoke a more comprehensive immune response from T-cells (Ag3). After incubation at 37°C for 16–24 hours, the levels of IFN-γ were measured using the ELISA QuantiFERON test. The measurement followed the instructions provided by the manufacturer. The manufacturer’s data sheet suggested that an IFN-γ level between 0.15 IU/ml and 0.2 IU/ml should be considered positive (26). However, in our study, we defined the positive cut-off as 0.15 IU/ml.
The time interval in days following the last event (vaccination against COVID-19 or SARS-CoV-2 infection) until the first sampling is the “Sampling 1 interval”. “Sampling 2 interval” is the time in days between Sampling 1 and Sampling 2. “Covid interval” is the time in days between the confirmed case of SARS-CoV-2 infection by a positive NCP IgG test result and the Sampling 2. “Vaccine interval” is the time in days between additional vaccination against COVID-19 during the interim period and the Sampling 2. “Event interval” is defined as a time interval between vaccination against COVID-19 or SARS-CoV-2 infection during the interim period and sampling, whichever is closer to Sampling 2. If following Sampling 1, none of these events happened, then we set the “Event interval” to equal to the “Sampling 2 interval”. Figure 1 illustrates these interval definitions and the median (IQR) elapsed time in days in both groups.
Figure 1 Definitions of the interval variables in the control group (A) and in the RA group (B). The data shown in the figure represent median values. The time interval following the last event (SARS-CoV-2 infection or vaccination against COVID-19) until the first sampling is the “Sampling 1 interval” (control: 83 (37, 190) days; RA: 130 (81, 169) days). “Sampling 2 interval” is the time in days between Sampling 1 and Sampling 2 (control: 458 (410, 547) days; RA: 386 (311, 448) days). “Covid interval” is the time between confirmed case of SARS-CoV-2 infection by a positive NCP IgG test result and the Sampling 2 (control: 403 (267, 543) days; RA: 357 (245, 448) days). “Vaccine interval” is the time between additional vaccination against COVID-19 during the interim period and the Sampling 2 (control: 370 (330, 405) days; RA: 394 (342, 453) days). “Event interval” is defined as a time interval between vaccination against COVID-19 or SARS-CoV-2 infection during the interim period and sampling whichever is closer to the Sampling 2 (control: 194 (67, 327) days; RA: 321 (201, 378) days). If following the Sampling 1 none of these events happened then we set “Event interval” to equal to the “Sampling 2 interval”. This figure was created with BioRender.com.
Concentrations in the “Sampling 1” subgroup were measured in the first period. Subgroup “Sampling 2” represents cases with no confirmed SARS-CoV-2 infection or additional vaccination against COVID-19 between the first and second measurements. Subgroup “Sampling 2+Vaccination” and “Sampling 2+Infection” consist of subjects who were either vaccinated against COVID-19 prior to the second measurement or had confirmed SARS-CoV-2 infection before the second measurement. It is important to note that the term “prior to measurement” indicates that latest vaccination against COVID-19 or latest SARS-CoV-2 infection was the most recent event that triggered the SARS-CoV-2-specific immune response. For instance, if a subject received a COVID-19 vaccine first and later had a SARS-CoV-2 infection, their sample would still be classified as “Sampling 2+Infection” (Figure 2).
Figure 2 Boxplot of anti-S concentration by Group (healthy control or RA) and by subgroups. To ease the statistical and graphical analysis we classified observations into subgroups. Concentrations in subgroup “Sampling 1” were measured in the first period. Concentrations in subgroup “Sampling 2” were measured in the second period and neither vaccination against COVID-19 nor SARS-CoV-2 infection had been recorded during the interim period. Concentrations in subgroup “Sampling 2+Vaccination” were measured in the second period and during the interim period the participant received another vaccination dose against COVID-19. Concentrations in subgroup “Sampling 2+Infection” were measured in the second period and during the interim period there was a clinically documented SARS-CoV-2 infection. In a case of multiple events (vaccination against COVID-19 and SARS-CoV-2 infection) the classification is based on the type of the last event.
It should be noted that data from the first period could not be split this way. Before taking the first sample, every participant experienced at least one event of SARS-CoV-2 infection or vaccination against COVID-19. Furthermore, in the first period, vaccines with varying efficacies against COVID-19 were administered (BNT162b2, mRNA-1273, ChAdOx1s, Gam-COVID, BBIBP-CorV) whereas, in the interim period, only RNA-based vaccines such as BNT162b2 vaccine and mRNA-1273 vaccine were used.
The data shown in Figure 2 underwent analysis using stepwise linear regression. The stepwise algorithm identified and eliminated variables such as sex, group (healthy control or RA), MTX use, and low dose of corticosteroid therapy as not statistically significant.
Continuous variables are presented as median (IQR) and compared using the Wilcoxon rank-sum test or unpaired t-test. Categorical variables are presented as n (percent) and compared using the chi-square test. The reported p-values are the nominal p-values without correction for multiple hypothesis testing.
Linear regression was used to model anti-S dependence on external factors. Based on our previous results, the initial linear regression model contained the following variables: Sex, Age, Group (healthy or RA), “Event interval”, the nature of the last event (vaccination against COVID-19 or SARS-CoV-2 infection), and Period. Period is a categorical variable that can take two values, demonstrating if a measured value comes from the first (Sampling 1) or follow-up period (Sampling 2).
Anti-S concentrations were highly right-skewed. Therefore, concentrations were logarithmically transformed before entering into regressions models. Anti-S half-life was estimated assuming using the log (0.5)/” Event interval” formula where “Event interval” is the estimated regression coefficient of the “Event interval” variable.
Additionally, only RA patients received targeted therapy. Therefore, from a statistical perspective, variables such as group (healthy control or RA), age, and targeted therapy are interdependent. In such cases, it is recommended to look beyond individual p-values and consider the overall fit of the model. Accordingly, we fit the two regression models. Model 1 included SARS-CoV-2 infection, vaccination against COVID-19, “Event interval” and “Sampling time point” variables, while Model 2 contained the variables mentioned before plus the group, age, and targeted therapy variables. The two models were compared with the F test. The corresponding F is highly significant (F= 7.34, df= 208, 211, p= 0.0001059), showing that the patient group differs considerably from the healthy volunteer group.
The correlation between anti-S level and CW4–8 T-cell response was evaluated by non-parametric Spearman’s rank test. A Spearman’s rho >0.7 indicated a high correlation, 0.7 > rho > 0.5 indicated a moderate one, and rho <0.5 indicated a low correlation. Two-tailed p values were considered significant if <0.05.
Data were analyzed using R (27), and tables and figures were prepared with the gtsummary (28) and ggplot2 (29).
84 RA patients and 43 healthy controls were included in our longitudinal study (Table 1). The activity of RA was assessed by examining the patient clinically using a scoring system called the Disease Activity Score 28-CRP (DAS28-CRP). The median DAS28-CRP score was 2.6. In this scoring system, a DAS28-CRP value above 5.1 indicates high disease activity, values between 3.2 and 5.1 indicate moderate activity, values between 2.6 and 3.2 indicate low activity and values between 0 and less than 2.6 indicate remission of the disease (30). In the RA group, 63 (75%) patients were treated with methotrexate (MTX) as monotherapy or in combination, and 20 patients (24%) received a low dose of corticosteroid therapy (all of them below 7.5 mg/day prednisone or equivalent). The majority of RA patients (N=75; 89%) were treated with targeted therapy (TNF-α inhibitors, IL-6-inhibitor, rituximab, JAK inhibitors). In the baseline study (19), RA patients received COVID-19 vaccination 3.5 ± 0.87 months (SD/SEM, n=4) after rituximab treatment. Of the 84 RA patients in the follow-up study, only one was treated with rituximab and received the COVID-19 vaccine four months after the rituximab dose. In the first phase, SARS-CoV-2 infection was confirmed with a positive PCR test. In the second phase, if a SARS-CoV-2 infection occurred, the role of SARS-CoV-2 was confirmed by detecting IgG antibodies against NCP as a response marker. During the interim period, only RNA-based vaccines, such as the BNT162b2 vaccine and the mRNA-1273 vaccine, were administered. Figure 1 reveals that during the follow-up phase, the duration between the events that triggered the SARS-CoV-2-specific immune response and the time of sampling was considerably extended compared to the first period.
Regardless of the period, patients with RA had lower anti-S levels compared to healthy controls (p<0.01 for healthy controls, p<0.001 for RA patients, Supplementary Table 1). The median (IQR) concentration of the anti-S antibodies was 4548 U/ml (1567, 13949) in the RA group and 12813 U/ml (5632, 25000) in the healthy group based on the follow-up results (Supplementary Table 1). Additionally, there was a massive increase in the SARS-CoV-2-specific humoral immune response in each study population during the follow-up period (p<0.05 for healthy volunteers, p<0.0001 for RA patients, rank-sum test) (Figure 3A). 63% (53/84) of RA patients and 82% (14/17) of healthy individuals had increased SARS-CoV-2-specific humoral immune response compared to the baseline results (Figure 4). The median values of the anti-S levels increased sevenfold in the RA group and fivefold in the control group compared to the baseline results.
Figure 3 Comparative analysis of the SARS-CoV-2-specific immune responses. (A) shows the median of anti-S concentrations in both groups. Regardless of the Sampling time point (Sampling 1 or Sampling 2), patients with RA showed statistically significantly lower levels of anti-S antibodies when compared to the healthy individuals (Sampling 1: 636 U/ml (RA) vs 2516 U/ml (control) p=0.008; Sampling 2: 4548 U/ml (RA) vs 12813 U/ml (control) p<0.001). Furthermore, compared to the first measurements in the second period, there was a large increase in the SARS-CoV-2-specific humoral immune response. These differences were significant for both groups (p<0.05 for healthy volunteers, p<0.0001 for RA patients, rank-sum test). (B) shows the median of whole COVID virus-stimulated COVID-specific CD4+ and CD8+ T-cell response (CW4–8) in healthy controls and patients diagnosed with RA. After considering measurements from both periods (Sampling 1 and Sampling 2), it was observed that RA patients had a diminished CW4–8 T-cell response in comparison to healthy controls.
Figure 4 The change of the anti-S concentration and the whole COVID virus-stimulated COVID-specific CD4+ and CD8+ T-cell response (CW 4–8) in both study groups. We compared the results of Sampling 1 and Sampling 2 and we defined a change of at least 10% decrease or increase, “unchanged” means the rate of the change is less than 10%.
Table 2 shows that the “Sampling time point” effect, with an estimate of 9.34 (CI95%: 4.58–19.00, p<0.001), indicates that the anti-S concentrations in the second sampling period were several times higher than those in the first period after adjusting for covariates. Furthermore, the “Sampling time point” effect is multiplied by a factor of 2.72 (CI 95%: 1.25–5.95, p < 0.05) if a study subject received a vaccination against COVID-19 during the interim period (Figure 2). Additionally, individuals who had a confirmed SARS-CoV-2 infection during the interim period had anti-S levels that were 2.1 times higher (CI 95%: 1.31–3.37) compared to those who did not have SARS-CoV-2 infection (p<0.01) (Figure 2). However, the effects of these two events gradually diminish over time, with an estimated half-life of 176.8 days (CI 95%: 51.9–301.8 days, p<0.01). But at the same time, the multiplying factor targeted therapy is 0.48 (CI 95% 0.25–0.94, p<0.05), meaning that compared to matched patients, the anti-S levels are 52% lower. Moreover, anti-S concentrations decrease with age, with each 20-year difference corresponding to a decrease of approximately 45.6% (calculated as 100(1–0.9720)).
Based on the COVID-19 vaccination (three doses) history of the participants, 77% (44/57) of RA patients and 76% (16/21) of healthy controls received homologous COVID-19 vaccinations. In contrast, 23% (13/57) of RA patients and 24% (5/21) of healthy controls received heterologous vaccine doses. For those who received homologous vaccinations, the median (IQR) concentration of anti-S antibodies was 6362 U/ml (1989, 15696) in the RA group and 14163 U/ml (5632, 24842) in the healthy group. For those who received heterologous vaccinations, the median (IQR) concentration of anti-S antibodies was 4625 U/ml (1664, 13532) in the RA group and 21986 U/ml (19524, 25000) in the healthy group. Based on our results there was no significant difference between homologous (9382 U/ml (2582, 21347)) and heterologous (9588 (2858, 21604)) vaccination groups, if we calculate the median (IQR) concentration for the whole study population.
Figure 5A shows the number of weekly deaths caused by SARS-CoV-2 infection in Hungary between 29.11.2021 and 28.11.2022 versus the median of anti-S levels over time in each study population. Based on these data, the number of weekly deaths caused by SARS-CoV-2 infection in Hungary and the median of anti-S levels changed in opposite ways over time. The heightened level of anti-S antibodies might explain the decline in the number of weekly deaths caused by SARS-CoV-2 infection.
Figure 5 (A) The number of weekly deaths caused by SARS-CoV-2 infection in the Hungarian population versus the median of anti-S levels over time in each study population (https://covid19.who.int/region/euro/country/hu). The median values of the results of Sampling 1 and Sampling 2 were connected with a line in the Figure. (B) The number of weekly deaths caused by SARS-CoV-2 infection in the Hungarian population versus the median of whole COVID virus-stimulated COVID-specific CD4+ and CD8+ T-cell response (CW 4–8) over time in each study population (https://covid19.who.int/region/euro/country/hu). The median values of the results of Sampling 1 and Sampling 2 were connected with a line in the Figure.
By investigating the SARS-CoV-2-specific T-cell response, we were able to study how it changes when stimulated by three specific antigens (I: spike antigen stimulated COVID-specific CD4+ (CD4); II: spike antigen stimulated COVID-specific CD4+ and CD8+ (CD4–8) and III: whole COVID virus-stimulated COVID-specific CD4+ and CD8+ (CW4–8) T-cell responses). We further analyzed the CW4–8 T-cell response because it seemed to be the most informative marker.
Based on both measurements (Sampling 1, Sampling 2), RA patients had reduced CW4–8 T-cell response compared to healthy controls (p<0.01 for healthy controls, p<0.001 for RA patients, Figure 3B). We identified a median IFN-γ level (reflecting CW4–8 T-cell response) of 0.72 IU/ml (0.52, 1.25) in healthy controls; however, the magnitude of the RA patients’ response was 0.25 IU/ml (0.05, 1.28) (Supplementary Table 1). 43% (33/76) of RA patients and 75% (9/12) of healthy individuals had increased CW4–8 T-cell response compared to the baseline results (Figure 4). A significant, low correlation was observed between the anti-S antibody level and the CW4–8 T-cell response in each study population (r=0.3891, p<0.0001).
Supplementary Figure 1 shows markers of SARS-CoV-2-specific T-cell response. No apparent trend or pattern is observable. Indeed, a similar linear regression modeling strategy applied for anti-S did not yield any significant effect, except CW4–8, where SARS-CoV-2 infection (but not vaccination against COVID-19) increased the CW4–8 response to 2.09-fold (95% CI: 1.30 - 3.36, p<0.01) in RA patients.
Figure 5B shows the number of weekly deaths caused by SARS-CoV-2 infection in Hungary between 29.11.2021 and 28.11.2022 versus the median of CW4–8 T-cell response over time in each study population. Based on these data, the number of weekly deaths caused by SARS-CoV-2 infection in Hungary decreased over time, however, the level of CW4–8 T-cell response had not changed substantially in the study groups.
In this longitudinal study, we followed a cohort of rheumatoid arthritis patients and healthy individuals to assess their SARS-CoV-2-specific humoral and cellular immune response over one year.
Neutralizing antibodies are specific to viral epitopes that are predominantly in the spike protein and they play an important protective role by blocking the virus from entering the host cells, which limits infection (31). However, studies revealed that the level of neutralizing antibodies decreases over time following either SARS-CoV-2 infection or vaccination against COVID-19, thereby increasing the chance of potential reinfections (32–34). The SARS-CoV-2 virus has been notable for its extensive array of genomic variations observed since the beginning of the pandemic (35). These variations have led to significant changes across all structural proteins, driven by natural selection, resulting in the emergence of variants with heightened abilities in transmission and replication (36). From November 2021, the majority of infections were caused by the omicron (B.1.1.529) coronavirus variant, which caused the most infections (37) and since then, it has undergone numerous mutations (38). One reason for the rapid spread of mutants is the infection spread by mild and asymptomatic virus carriers (39). It has remained unclear, how asymptomatic SARS-CoV-2 infections could affect the dynamic and the longevity of the SARS-CoV-2-specific immunity, especially in patients receiving immunosuppressive therapy.
Previous studies (15, 40–42) announced that immunocompromised patients, including RA patients, have a higher prevalence of SARS-CoV-2 infection and have a higher mortality risk of COVID-19 compared to the general population. We confirmed the results of our first report (6) and earlier findings (18–23), patients diagnosed with RA had substantially lower anti-S levels even after more than a yearlong interim period compared to the healthy control group (p<0.01 for healthy controls, p<0.001 for RA patients). We also evaluated the CW4–8 T-cell response during the follow-up period, and similarly to other research groups (43–46), we found that patients diagnosed with RA showed a diminished CW4–8 T-cell response in comparison to the healthy control group (p<0.01 for healthy controls, p<0.001 for RA patients). It was found that patients with immune-mediated inflammatory diseases have a lower and less long-lasting SARS-CoV-2-specific immune response than healthy individuals, so earlier booster doses or more frequent re-doses, or both, are recommended in order to achieve an adequate immune response in this population (47). Furthermore, it was shown that booster vaccination doses against COVID-19 enhances the SARS-CoV-2-specific immune response of immunocompromised patients (48) and increases the vaccine effectiveness against COVID-19-related death in both immunocompromised patients and healthy individuals (42). Although glucocorticoid therapy may affect the vaccination-induced immune response, in line with the appropriate ACR guidance (43) glucocorticoid therapy at the lowest effective dose was continued, because (not delaying the COVID-19 vaccination) uncontrolled disease activity poses a greater risk than the potential modification of vaccine response due to glucocorticoid use.
In contrast with other studies (32–34), throughout the follow-up period, both RA patients and healthy volunteers showed several times higher anti-S concentrations, regardless of whether they were vaccinated against COVID-19 or had previous SARS-CoV-2 infection during the time between the first and second samplings (as shown in Figures 2, 3 and Supplementary Table 1). The same laboratory methods were used in order to measure the level of SARS-CoV-2-specific humoral and cellular immune responses at the two Sampling time points. These findings were somewhat unexpected, as based on our previous analysis (19), we expected lower rather than substantially higher anti-S levels under these conditions. Additionally, according to Figures 5A, B as the number of weekly deaths caused by SARS-CoV-2 infection in the Hungarian population decreased, the median anti-S concentrations increased and CW4–8 T-cell response remained relatively stable in both study populations. Our results confirmed a previously described fact that anti-S antibody concentrations have been shown to correlate with protection against COVID-19 (46, 49, 50). Moreover, earlier studies marked that retained CD4+ and CD8+ T-cell responses were associated with reduced COVID-19 severity (44–46) and T-cell immunity to the SARS-CoV-2 may help to compensate the absence of neutralizing antibodies in preventing or limiting severe COVID-19 (33, 49). In addition, there are many protective and risk factors of COVID-19 severity (50) as shown in Figure 6. The importance of the CW4–8 T-cell response was confirmed by our previous work (51), where we assessed the potential predictive value of CW4–8 T-cell response and SARS-CoV-2-specific humoral immune response for survival in critically ill COVID-19 patients requiring venovenous extracorporeal membrane oxygenation (VV-ECMO). We found that CW4–8 T-cell response was significantly higher among survivors compared to the deceased patients.
Figure 6 Protective and risk factors of COVID-19 severity. BMI: Body mass index. CCS: corticosteroids. csDMARDs: conventional synthetic disease-modifying antirheumatic drugs. tsDMARDs: targeted synthetic disease-modifying antirheumatic drugs. This figure was created with BioRender.com.
This is an observational study with its inherent limitations. However, because of these limitations, we cannot say for sure whether the reduced humoral response in the RA group was due to the disease itself, the targeted therapy, or simply the fact that the patients were older than the healthy volunteers. Statistical analysis suggests that the last two (treatment with targeted therapy and age, Table 2) better explain the difference between the healthy volunteer and patient group than the disease itself, which aligns with our previous results (19). There is a significant difference between the healthy and the RA groups in terms of age and gender. However, the gender effect on the anti-S response is negligible, and the significant age effect was adjusted for all other variables. The reported p-values have been “cleaned” of the age effect.
In summary, the increased SARS-CoV-2-specific humoral immune response, the nearly permanent CW4–8 T-cell response, and possibly the simultaneous presence of various SARS-CoV-2 variants in the population potentially resulting in mild or symptom-free cases of COVID-19, may contribute to the low number of severe COVID-19 cases in the whole population.
The original contributions presented in the study are included in the article. Further inquiries can be directed to the corresponding author.
The studies involving humans were approved by National Public Health Center, Hungary. IV/2021-1/2021/EKU. The studies were conducted in accordance with the local legislation and institutional requirements. The participants provided their written informed consent to participate in this study.
DN: Writing – original draft, Writing – review & editing. HV: Writing – review & editing. LT: Writing – review & editing. ZU: Writing – review & editing. DB: Writing – review & editing. ZS: Writing – review & editing. BR: Writing – review & editing. LG: Writing – review & editing. BM: Writing – original draft, Writing – review & editing. GN: Writing – original draft, Writing – review & editing.
The author(s) declare financial support was received for the research, authorship, and/or publication of this article. This project was financed by the National Research, Development and Innovation Office Fund in Hungary (2020–2.1.1-ED-2022–00198), the Thematic Excellence Programme (Tématerületi Kiválósági Program, TKP2021-EGA-29) of the Ministry for Innovation and Technology in Hungary, OTKA Grant (K 131479). This study was supported by the National Research, Development and Innovation Office of Hungary under the Investment in the Future funding scheme (2020–1.1.6-JÖVŐ-2021–00013) and by the grant of European Union RRF-2.3.1–21-2022–00003. TKP2021-EGA-23 has been implemented with the support provided by the Ministry of Innovation and Technology of Hungary from the National Research, Development and Innovation Fund, financed under the TKP2021-EGA funding scheme.
We thank Ilona Dargai for her contribution to our work. We acknowledge the laboratory support of Zsolt Pósa.
The authors declare that the research was conducted in the absence of any commercial or financial relationships that could be construed as a potential conflict of interest.
All claims expressed in this article are solely those of the authors and do not necessarily represent those of their affiliated organizations, or those of the publisher, the editors and the reviewers. Any product that may be evaluated in this article, or claim that may be made by its manufacturer, is not guaranteed or endorsed by the publisher.
The Supplementary Material for this article can be found online at: https://www.frontiersin.org/articles/10.3389/fimmu.2024.1397052/full#supplementary-material
1. Berlin DA, Gulick RM, Martinez FJ. Severe covid-19. N Engl J Med. (2020) 383:2451–60. doi: 10.1056/NEJMcp2009575
2. Gandhi RT, Lynch JB, Del Rio C. Mild or moderate covid-19. N Engl J Med. (2020) 383:1757–66. doi: 10.1056/NEJMcp2009249
3. Braun J, Loyal L, Frentsch M, Wendisch D, Georg P, Kurth F, et al. Sars-cov-2-reactive T cells in healthy donors and patients with covid-19. Nature. (2020) 587:270–4. doi: 10.1038/s41586–020-2598–9
4. Long QX, Liu BZ, Deng HJ, Wu GC, Deng K, Chen YK, et al. Antibody responses to sars-cov-2 in patients with covid-19. Nat Med. (2020) 26:845–8. doi: 10.1038/s41591–020-0897–1
5. Robbiani DF, Gaebler C, Muecksch F, Lorenzi JCC, Wang Z, Cho A, et al. Convergent antibody responses to sars-cov-2 in convalescent individuals. Nature. (2020) 584:437–42. doi: 10.1038/s41586–020-2456–9
6. Schulien I, Kemming J, Oberhardt V, Wild K, Seidel LM, Killmer S, et al. Characterization of pre-existing and induced sars-cov-2-specific cd8(+) T cells. Nat Med. (2021) 27:78–85. doi: 10.1038/s41591–020-01143–2
7. Sekine T, Perez-Potti A, Rivera-Ballesteros O, Stralin K, Gorin JB, Olsson A, et al. Robust T cell immunity in convalescent individuals with asymptomatic or mild covid-19. Cell. (2020) 183:158–68 e14. doi: 10.1016/j.cell.2020.08.017
8. Amanna IJ, Carlson NE, Slifka MK. Duration of humoral immunity to common viral and vaccine antigens. New Engl J Med. (2007) 357:1903–15. doi: 10.1056/nejmoa066092
9. Sariol A, Perlman S. Lessons for covid-19 immunity from other coronavirus infections. Immunity. (2020) 53:248–63. doi: 10.1016/j.immuni.2020.07.005
10. Hou H, Zhang Y, Tang G, Luo Y, Liu W, Cheng C, et al. Immunologic memory to sars-cov-2 in convalescent covid-19 patients at 1 year postinfection. J Allergy Clin Immunol. (2021) 148:1481–92 e2. doi: 10.1016/j.jaci.2021.09.008
11. Lau EHY, Tsang OTY, Hui DSC, Kwan MYW, Chan WH, Chiu SS, et al. Neutralizing antibody titres in sars-cov-2 infections. Nat Commun. (2021) 12:63. doi: 10.1038/s41467–020-20247–4
12. Seow J, Graham C, Merrick B, Acors S, Pickering S, Steel KJA, et al. Longitudinal observation and decline of neutralizing antibody responses in the three months following sars-cov-2 infection in humans. Nat Microbiol. (2020) 5:1598–607. doi: 10.1038/s41564–020-00813–8
13. Shi D, Weng T, Wu J, Dai C, Luo R, Chen K, et al. Dynamic characteristic analysis of antibodies in patients with covid-19: A 13-month study. Front Immunol. (2021) 12:708184. doi: 10.3389/fimmu.2021.708184
14. GianFrancesco M, Hyrich KL, Al-Adely S, Carmona L, Danila MI, Gossec L, et al. Characteristics associated with hospitalisation for covid-19 in people with rheumatic disease: data from the covid-19 global rheumatology alliance physician-reported registry. Ann Rheum Dis. (2020) 79:859–66. doi: 10.1136/annrheumdis-2020–217871
15. Akiyama S, Hamdeh S, Micic D, Sakuraba A. Prevalence and clinical outcomes of covid-19 in patients with autoimmune diseases: A systematic review and meta-analysis. Ann Rheum Dis. (2021) 80:384–91. doi: 10.1136/annrheumdis-2020–218946
16. Belleudi V, Rosa AC, Poggi FR, Armuzzi A, Nicastri E, Goletti D, et al. Direct and indirect impact of covid-19 for patients with immune-mediated inflammatory diseases: A retrospective cohort study. J Clin Med. (2021) 10:2388. doi: 10.3390/jcm10112388
17. Landewe RB, MaChado PM, Kroon F, Bijlsma HW, Burmester GR, Carmona L, et al. Eular provisional recommendations for the management of rheumatic and musculoskeletal diseases in the context of sars-cov-2. Ann Rheum Dis. (2020) 79:851–8. doi: 10.1136/annrheumdis-2020–217877
18. Assawasaksakul T, Sathitratanacheewin S, Vichaiwattana P, Wanlapakorn N, Poovorawan Y, Avihingsanon Y, et al. Immunogenicity of the third and fourth bnt162b2 mrna covid-19 boosters and factors associated with immune response in patients with sle and rheumatoid arthritis. Lupus Sci Med. (2022) 9:e000726. doi: 10.1136/lupus-2022–000726
19. Nemeth D, Vago H, Tothfalusi L, Ulakcsai Z, Becker D, Szabo Z, et al. Factors influencing the sars-cov-2 infection and vaccination induced immune response in rheumatoid arthritis. Front Immunol. (2022) 13:960001. doi: 10.3389/fimmu.2022.960001
20. Levin EG, Lustig Y, Cohen C, Fluss R, Indenbaum V, Amit S, et al. Waning immune humoral response to bnt162b2 covid-19 vaccine over 6 months. N Engl J Med. (2021) 385:e84. doi: 10.1056/NEJMoa2114583
21. Shrotri M, Navaratnam AMD, Nguyen V, Byrne T, Geismar C, Fragaszy E, et al. Spike-antibody waning after second dose of bnt162b2 or Chadox1. Lancet. (2021) 398:385–7. doi: 10.1016/S0140–6736(21)01642–1
22. Dan JM, Mateus J, Kato Y, Hastie KM, Yu ED, Faliti CE, et al. Immunological Memory to Sars-Cov-2 Assessed for up to 8 Months after Infection. Science. (2021) 371:eabf4063. doi: 10.1126/science.abf4063
23. Aletaha D, Neogi T, Silman AJ, Funovits J, Felson DT, Bingham CO 3rd, et al. Rheumatoid arthritis classification criteria: an american college of rheumatology/european league against rheumatism collaborative initiative. Arthritis Rheum. (2010) 62:2569–81. doi: 10.1002/art.27584
24. Riester E, Findeisen P, Hegel JK, Kabesch M, Ambrosch A, Rank CM, et al. Performance evaluation of the roche elecsys anti-sars-cov-2 S immunoassay. J Virol Methods. (2021) 297:114271. doi: 10.1016/j.jviromet.2021.114271
25. Weidner L, Gansdorfer S, Unterweger S, Weseslindtner L, Drexler C, Farcet M, et al. Quantification of sars-cov-2 antibodies with eight commercially available immunoassays. J Clin Virol. (2020) 129:104540. doi: 10.1016/j.jcv.2020.104540
26. Kruttgen A, Klingel H, Haase G, Haefner H, Imohl M, Kleines M. Evaluation of the quantiferon sars-cov-2 interferon- release assay in mrna-1273 vaccinated health care workers. J Virol Methods. (2021) 298:114295. doi: 10.1016/j.jviromet.2021.114295
27. Team RC. R: A Language and Environment for Statistical Computing. Vienna, Austria. (2021). Available at: https://www.R-project.org/.
28. Sjoberg DD, Whiting K, Curry M, Lavery JA, Larmarange J. Reproducible summary tables with the gtsummary package. R J. (2021) 13:570–80. doi: 10.32614/RJ-2021–053
29. Wickham H. Ggplot2: Elegant Graphics for Data Analysis. Verlag New York: Springer (2016). doi: 10.1007/978-3-319-24277-4
30. Pincus T, Furer V, Keystone E, Yazici Y, Bergman MJ, Luijtens K. Rapid3 (Routine assessment of patient index data 3) severity categories and response criteria: similar results to das28 (Disease activity score) and cdai (Clinical disease activity index) in the rapid 1 (Rheumatoid arthritis prevention of structural damage) clinical trial of certolizumab pegol. Arthritis Care Res (Hoboken). (2011) 63:1142–9. doi: 10.1002/acr.20481
31. Brouwer PJM, Caniels TG, van der Straten K, Snitselaar JL, Aldon Y, Bangaru S, et al. Potent neutralizing antibodies from covid-19 patients define multiple targets of vulnerability. Science. (2020) 369:643–50. doi: 10.1126/science.abc5902
32. Bilich T, Nelde A, Heitmann JS, Maringer Y, Roerden M, Bauer J, et al. Cell and antibody kinetics delineate sars-cov-2 peptides mediating long-term immune responses in covid-19 convalescent individuals. Sci Trans Med. (2021) 13:eabf7517. doi: 10.1126/scitranslmed.abf7517
33. Geurtsvankessel CH, Geers D, Schmitz KS, Mykytyn AZ, Lamers MM, Bogers S, et al. Divergent sars-cov-2 omicron–reactive T and B cell responses in covid-19 vaccine recipients. Sci Immunol. (2022) 7:eabo2202. doi: 10.1126/sciimmunol.abo2202
34. Koerber N, Priller A, Yazici S, Bauer T, Cheng CC, Mijocevic H, et al. Dynamics of spike-and nucleocapsid specific immunity during long-term follow-up and vaccination of sars-cov-2 convalescents. Nat Commun. (2022) 13:153. doi: 10.1038/s41467-021-27649-y
35. Lauring AS, Hodcroft EB. Genetic variants of sars-cov-2—What do they mean? JAMA. (2021) 325:529. doi: 10.1001/jama.2020.27124
36. Dimonte S, Babakir-Mina M, Hama-Soor T, Ali S. Genetic variation and evolution of the 2019 novel coronavirus. Public Health Genomics. (2021) 24:54–66. doi: 10.1159/000513530
37. Araf Y, Akter F, Tang YD, Fatemi R, Parvez MSA, Zheng C, et al. Omicron variant of sars-cov-2: genomics, transmissibility, and responses to current covid-19 vaccines. J Med Virol. (2022) 94:1825–32. doi: 10.1002/jmv.27588
38. Saxena SK, Kumar S, Ansari S, Paweska JT, Maurya VK, Tripathi AK, et al. Characterization of the novel sars-cov-2 omicron (B.1.1.529) variant of concern and its global perspective. J Med Virol. (2022) 94:1738–44. doi: 10.1002/jmv.27524
39. Yang X, Dai T, Zhou X, Qian H, Guo R, Lei L, et al. Naturally activated adaptive immunity in covid-19 patients. J Cell Mol Med. (2020) 24:12457–63. doi: 10.1111/jcmm.15771
40. Bournia VK, Fragoulis GE, Mitrou P, Mathioudakis K, Konstantonis G, Tektonidou MG, et al. Outcomes of covid-19 omicron variant in patients with rheumatoid arthritis: A nationwide greek cohort study. Rheumatol (Oxford). (2023) 63:1130–8. doi: 10.1093/rheumatology/kead354
41. Conway R, Grimshaw AA, Konig MF, Putman M, Duarte-Garcia A, Tseng LY, et al. Sars-cov-2 infection and covid-19 outcomes in rheumatic diseases: A systematic literature review and meta-analysis. Arthritis Rheumatol. (2022) 74:766–75. doi: 10.1002/art.42030
42. Szekanecz Z, Voko Z, Surjan O, Rakoczi E, Szamosi S, Szucs G, et al. Effectiveness and waning of protection with the bnt162b2 vaccine against the sars-cov-2 delta variant in immunocompromised individuals. Front Immunol. (2023) 14:1247129. doi: 10.3389/fimmu.2023.1247129
43. Curtis JR, Johnson SR, Anthony DD, Arasaratnam RJ, Baden LR, Bass AR, et al. American college of rheumatology guidance for COVID-19 vaccination in patients with rheumatic and musculoskeletal diseases: version 5. Arthritis Rheumatol. (2023) 75:E1–E16. doi: 10.1002/art.42372
44. Liao M, Liu Y, Yuan J, Wen Y, Xu G, Zhao J, et al. Single-cell landscape of bronchoalveolar immune cells in patients with covid-19. Nat Med. (2020) 26:842–4. doi: 10.1038/s41591–020-0901–9
45. Rydyznski Moderbacher C, Ramirez SI, Dan JM, Grifoni A, Hastie KM, Weiskopf D, et al. Antigen-specific adaptive immunity to sars-cov-2 in acute covid-19 and associations with age and disease severity. Cell. (2020) 183:996–1012 e19. doi: 10.1016/j.cell.2020.09.038
46. Zhou R, To KK, Wong YC, Liu L, Zhou B, Li X, et al. Acute sars-cov-2 infection impairs dendritic cell and T cell responses. Immunity. (2020) 53:864–77 e5. doi: 10.1016/j.immuni.2020.07.026
47. Simon D, Tascilar K, Fagni F, Kleyer A, Kronke G, Meder C, et al. Intensity and longevity of sars-cov-2 vaccination response in patients with immune-mediated inflammatory disease: A prospective cohort study. Lancet Rheumatol. (2022) 4:e614–e25. doi: 10.1016/S2665–9913(22)00191–6
48. Farroni C, Aiello A, Picchianti-Diamanti A, Lagana B, Petruccioli E, Agrati C, et al. Booster dose of sars-cov-2 messenger rna vaccines strengthens the specific immune response of patients with rheumatoid arthritis: A prospective multicenter longitudinal study. Int J Infect Dis. (2022) 125:195–208. doi: 10.1016/j.ijid.2022.10.035
49. Guo L, Wang G, Wang Y, Zhang Q, Ren L, Gu X, et al. Sars-cov-2-specific antibody and T-cell responses 1 year after infection in people recovered from covid-19: A longitudinal cohort study. Lancet Microbe. (2022) 3:e348–e56. doi: 10.1016/S2666–5247(22)00036–2
50. Zhang JJ, Dong X, Liu GH, Gao YD. Risk and protective factors for covid-19 morbidity, severity, and mortality. Clin Rev Allergy Immunol. (2023) 64:90–107. doi: 10.1007/s12016–022-08921–5
Keywords: SARS-CoV-2, humoral immune response, cellular immune response, longitudinal study, immunosuppression
Citation: Nemeth D, Vago H, Tothfalusi L, Ulakcsai Z, Becker D, Szabo Z, Rojkovich B, Gunkl-Toth L, Merkely B and Nagy G (2024) Escalating SARS-CoV-2 specific humoral immune response in rheumatoid arthritis patients and healthy controls. Front. Immunol. 15:1397052. doi: 10.3389/fimmu.2024.1397052
Received: 07 March 2024; Accepted: 28 May 2024;
Published: 07 June 2024.
Edited by:
Zoltan Szekanecz, University of Debrecen, HungaryCopyright © 2024 Nemeth, Vago, Tothfalusi, Ulakcsai, Becker, Szabo, Rojkovich, Gunkl-Toth, Merkely and Nagy. This is an open-access article distributed under the terms of the Creative Commons Attribution License (CC BY). The use, distribution or reproduction in other forums is permitted, provided the original author(s) and the copyright owner(s) are credited and that the original publication in this journal is cited, in accordance with accepted academic practice. No use, distribution or reproduction is permitted which does not comply with these terms.
*Correspondence: Dora Nemeth, bmVtZXRoLmRvcmFAc3R1ZC5zZW1tZWx3ZWlzLmh1
†These authors have contributed equally to this work and share senior authorship
Disclaimer: All claims expressed in this article are solely those of the authors and do not necessarily represent those of their affiliated organizations, or those of the publisher, the editors and the reviewers. Any product that may be evaluated in this article or claim that may be made by its manufacturer is not guaranteed or endorsed by the publisher.
Research integrity at Frontiers
Learn more about the work of our research integrity team to safeguard the quality of each article we publish.