- School of Molecular Biosciences, Center for Reproductive Biology, Washington State University, Pullman, WA, United States
Endometriosis is a chronic inflammatory disease that causes debilitating pelvic pain in women. Macrophages are considered to be key players in promoting disease progression, as abundant macrophages are present in ectopic lesions and elevated in the peritoneum. In the present study, we examined the role of GATA6+ peritoneal macrophages on endometriosis-associated hyperalgesia using mice with a specific myeloid deficiency of GATA6. Lesion induction induced the disappearance of TIM4hi MHCIIlo residential macrophages and the influx of increased Ly6C+ monocytes and TIM4lo MHCIIhi macrophages. The recruitment of MHCIIhi inflammatory macrophages was extensive in MacGata6 KO mice due to the severe disappearance of TIM4hi MHCIIlo residential macrophages. Ki67 expression confirmed GATA6-dependent proliferative ability, showing different proliferative phenotypes of TIM4+ residential macrophages in Gata6f/f and MacGata6 KO mice. Peritoneal proinflammatory cytokines were elevated after lesion induction. When cytokine levels were compared between Gata6f/f and MacGata6 KO mice, TNFα at day 21 in Gata6f/f mice was higher than in MacGata6 KO mice. Lesion induction increased both abdominal and hind paw sensitivities. Gata6f/f mice tended to show higher sensitivity in the abdomen after day 21. Elevated expression of TRPV1 and CGRP was observed in the dorsal root ganglia after ELL induction in Gata6f/f mice until days 21 and 42, respectively. These results support that peritoneal GATA6+ macrophages are involved in the recruitment and reprogramming of monocyte-derived macrophages. The extensive recruitment of monocyte-derived macrophages in MacGata6 KO mice might protect against inflammatory stimuli during the resolution phase, whereas GATA6 deficiency did not affect lesion initiation and establishment at the acute phase of inflammation. GATA6+ residential macrophages act to sustain local inflammation in the peritoneum and sensitivities in the neurons, reflecting endometriosis-associated hyperalgesia.
1 Introduction
Endometriosis is a chronic inflammatory disease and affects approximately 10% of reproductive-aged women, representing ~190 million women worldwide (1, 2). It is associated with debilitating chronic pelvic pain, dysmenorrhea, and dyspareunia that dramatically reduces the quality of life of women (3–6). Endometriosis is commonly classified into four stages according to the revised criteria from the American Society of Reproductive Medicine (rASRM) or American Fertility Society (AFS) based on lesion size, location, and the extent of adhesions (6, 7). However, endometriosis-associated pain does not correlate with the staging system (6, 8, 9). Patients with stage I disease can have severe pain, while stage IV patients can be asymptomatic (2, 10), indicating that several other factors contribute to disease symptoms.
Rigorous prior research suggests that aberrant inflammation contributes to the onset and progression of endometriosis (11–21). Macrophages are considered to be key players in promoting disease progression (14, 20, 21), as abundant macrophages are present in ectopic lesions (22) and elevated in the peritoneal cavity (20, 23, 24). Transcriptionally and functionally dysregulated macrophages can establish an inflammatory environment by secreting cytokines and chemokines, which encourage lesion growth and progression (13, 17, 20, 21, 24, 25) and contribute to endometriosis-associated pain (24, 26, 27). Peritoneal macrophages also contribute to the inflammatory condition by releasing cytokines and growth factors that stimulate lesion infiltration, growth, and vascularization (20, 24, 28, 29). Indeed, depletion of peritoneal macrophages by clodronate liposomes reduced lesion progression (24).
Macrophages are highly heterogeneous immune cells that play various roles in homeostasis, innate immunity, and tissue development and repair depending on the signals from their local environment (30). In the peritoneum, two major subsets of macrophages have been distinguished based on their ontogeny and differential expression of F4/80 and MHCII (31). Large peritoneal macrophages (LPM) expressing high F4/80 and low MHCII, F4/80hi MHCIIlo, are derived from embryonic progenitors in the yolk sac and are maintained in the peritoneum by self-renewal (32), while some replenishment from bone marrow (BM)-derived monocytes continuously occurs with aging (33, 34). Small peritoneal macrophages stemmed from hematopoiesis in the BM, expressing low F4/80 and high MHCII, F4/80lo MHCIIhi (31). When inflammatory stimuli are induced in the peritoneum, large numbers of BM-derived monocytes are recruited and differentiate into monocyte-derived macrophages that secrete proinflammatory cytokines as an immune response (35). Monocyte-derived macrophages are transcriptionally and functionally distinct from tissue-resident macrophages even though they serve to replenish resident macrophages (36, 37). Replenishment or reprogramming of monocyte-derived inflammatory macrophages to resident macrophages depends on the degree of inflammatory stimuli (37). Recent evidence (15) indicates that monocyte-derived macrophages may protect the peritoneum against lesion establishment, whereas endometrial macrophages are pro-endometriosis, suggesting further diverse functions of macrophage contribution in endometriosis pathophysiology.
LPM represent most of the macrophage population in the peritoneum, and GATA6 is selectively expressed in LPM (38, 39). See (21) https://kanakohayashilab.org/hayashi/en/mouse/peritoneal.immune.cells/ A zinc finger transcription factor, GATA6, is known to transcriptionally regulate residential LPM proliferation, differentiation, metabolism, and survival (38–40). LPM are essential as immunosurveillance patrollers in maintaining peritoneal homeostasis, as summarized in (41, 42). In response to inflammatory stimuli such as infection or sterile inflammation, LPM undergo the macrophage disappearance reaction (MDR) at the early phase of the inflammatory reaction, in which LPM disappear from the peritoneum due to LPM death and/or adherence to the mesothelium (41, 42). Fate-mapping studies exhibit that MDR, due to the death of large numbers of GATA6+ LPM, induces significant replenishment with BM-derived monocytes (33, 34). GATA6+ LPM also adhere to the mesothelium to repair sterile injury on the surface of visceral organs, such as the liver or intestine (43, 44). They generally cannot interact with mesothelium under normal homeostasis as they are free-floating cells (45). In contrast, multiple studies have demonstrated that peritoneal macrophages contribute to disease advancement, such as ovarian cancer progression and metastasis (46–49), as deleting peritoneal macrophages reduces tumor progression and peritoneal metastasis (46, 47). Gastric cancer patients bearing more peritoneal macrophages show poor prognosis (50). GATA6+ LPM invade liver metastases directly from the peritoneum and contribute to metastatic tumor growth and recurrence (51). Thus, GATA6+ LPM can also become adapted and act as tumor-associated macrophages (41, 42).
In endometriosis, CD14hi peritoneal macrophages, which are the predominant resident population in the peritoneum in humans (52), are increased in women with endometriosis (9). However, CD14hi peritoneal macrophages negatively correlate with the severity of pelvic pain, which is independent of disease outcome (9). We have previously reported that GATA6+ LPM were involved in the establishment of the aberrant inflammatory environment in the peritoneum, which was associated with increased levels of cytokines and chemokines in the peritoneum and lesion vascularization and innervation (20), indicating dysregulation of GATA6+ LPM are involved in disease pathophysiology. In the present study, we thus examined the role of peritoneal GATA6+ LPM following endometriotic lesion induction on the recruitment and differentiation of peritoneal macrophages and how they affect disease progression, inflammation, and endometriosis-associated hyperalgesia using Lyz2cre Gata6f/f mice, selectively deficient for Gata6 only in the macrophage lineage to reduce GATA6+ LPM (38–40).
2 Materials and methods
2.1 Animals
Gata6-floxed mice (Gata6f/f, Gata6tm2.1Sad/, JAX #008196) and Lysozyme M (Lyz2) Cre mice (Lyz2Cre, Lyz2tm1(cre)Ifo/J, JAX #004781) were purchased from the Jackson Laboratory and Lyz2Cre/+Gata6f/f (denoted as MacGata6 KO) and Lyz2+/+Gata6f/f (denoted as Gata6f/f) mice were generated. All animal experiments were performed at Washington State University according to the NIH guidelines for the care and use of laboratory animals (protocol #6751). Characterizations of peritoneal macrophages in Gata6f/f and MacGata6 KO mice are shown in Figure 1.
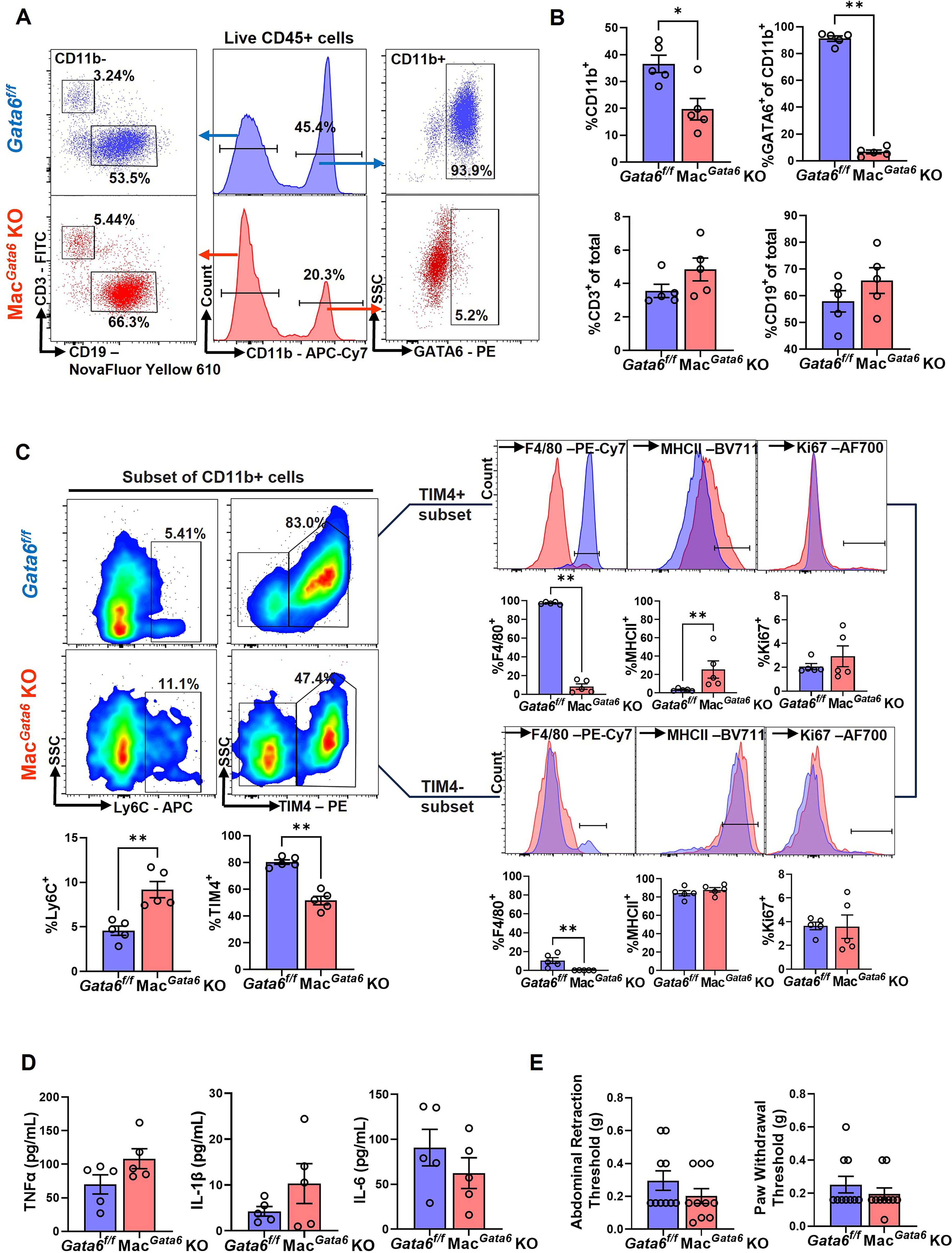
Figure 1. Comparison of peritoneal immune cell profiles between Gata6f/f and MacGata6 KO mice. (A) Representative flow plots illustrating the composition of CD11b+, GATA6+, CD3+, and CD19+ cells in Gata6f/f and MacGata6 KO mice. (B) Proportions of CD11b+, GATA6+, CD3+, and CD19+ in Gata6f/f and MacGata6 KO mice. (C) Flow cytometric analysis of CD11b+ subsets. Bottom left: Proportions of Ly6C+ and TIM4+ cells from CD11b+ subsets. Right panel: TIM4+ (top) and TIM4- (bottom) cells were further gated with F4/80, MHCII, and Ki67. Results were shown as representative overlaid histogram plots (Gata6f/f in blue and MacGata6 KO in red) with summarized bar plots below. (D) Proinflammatory cytokine levels (TNFα, IL-1β, and IL-6) in the peritoneal fluid were analyzed by IQELISA. (E) Abdominal and hind paw hyperalgesia were analyzed using the von Frey test. Data were analyzed by the Mann-Whitney test and shown mean ± SEM (n=5). *P < 0.05, **P < 0.01.
2.2 Mouse model of endometriosis
An experimental mouse model of endometriosis was employed by adopting procedures we have used and described previously with minor modifications (20, 21, 53). Briefly, a ‘menses-like’ event was induced in ovariectomized estradiol-17β- and progesterone-primed donor mice following an established protocol (54). Then, mouse menses-like endometrium scraped from myometrium and cut into fragments (1-2 mm per side) were introduced as the source of syngeneic mouse endometrium (donor: Gata6f/f mice) via injection (in 0.2 mL PBS) into the peritoneal cavity in untreated naïve mice (recipient: Gata6f/f or MacGata6 KO mice) under anesthesia via inhaled isoflurane. MacGata6 KO mice served only as recipient mice, as we have already confirmed that peritoneal macrophages do not arise from donor tissue in this model (20).
2.3 Study design
Endometriosis-like lesions (ELL) were induced in the recipient, Gata6f/f or MacGata6 KO mice, as described above (Figure 2A). On Day -1, 3, 7, 21, and 42 (Day 0 = ELL induction), a behavioral test was performed, and mice were then euthanized for sample collections: peritoneal fluid (PF) was recovered by lavage (4 mL x 2 of ice-cold PBS with 3% FBS), ELL, and bilateral lumbar (L4-6) dorsal root ganglia (DRG) were collected for further analysis.
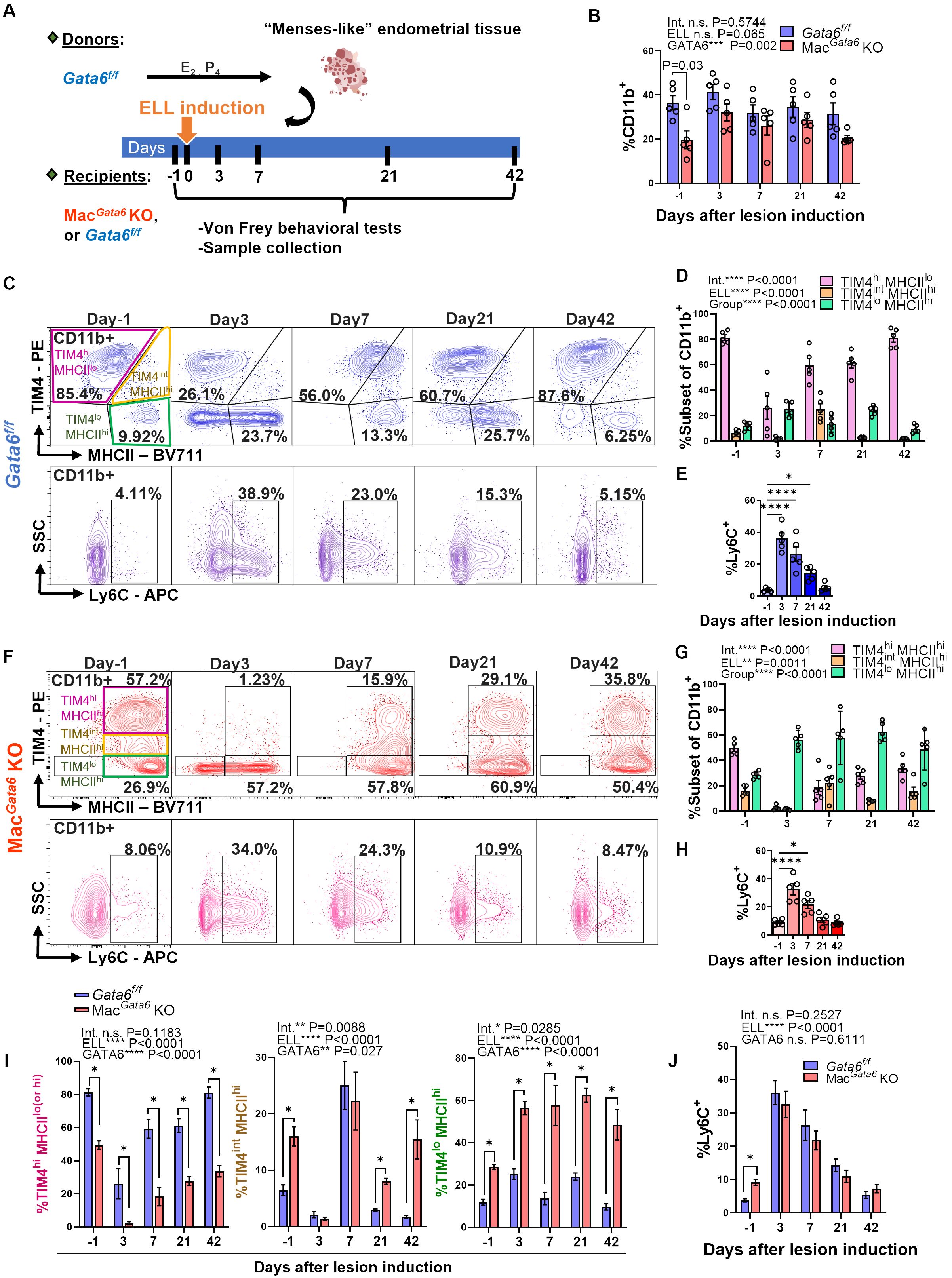
Figure 2. Characterization of peritoneal macrophage profiling following lesion induction in Gata6f/f and MacGata6 KO mice. (A) Experimental study design as described in Materials and Methods. (B) Graph summarizing flow cytometry data for the proportion of CD11b+ cells on Days 3, 7, 21, and 42 after endometriosis-like lesions (ELL) induction (n=5). Two-way ANOVA was performed to determine the main effects of ELL induction (depicted as ELL), GATA6 status (depicted as GATA6), and the interaction effect between the two factors (depicted as Int.). The differences between Gata6f/f and MacGata6 KO mice at each time point were compared by the Multiple Mann-Whitney test. (C, F) Representative flow cytometric plots showing the dynamics of macrophages following ELL induction in Gata6f/f (C) and MacGata6 KO mice (F). CD11b+ cells were further gated by TIM4 and MHCII (top) or Ly6C (bottom) (n=5). Statistical analysis of TIM4high(hi) MHCIIlow(lo) (pink), TIM4inter(int) MHCIIhi (yellow), and TIM4lo MHCIIhi (green) cells in Gata6f/f mice (D), or TIM4hi MHCIIhi (pink), TIM4int MHCIIhi (yellow), and TIM4lo MHCIIhi (green) cells in MacGata6 KO mice (G) was performed with two-way ANOVA to determine the time-dependent difference after ELL induction (depicted as ELL), the mean difference among cell populations (depicted as Group), and the interaction effect between the two factors (depicted as Int.). Time course analysis for Ly6C+ cells in (E, H) was conducted by one-way ANOVA with Dunnett’s multiple comparisons test. *P < 0.05, and ****P < 0.0001. (I) Time-dependent proportions of TIM4hi (pink), TIM4int (yellow), TIM4lo (green) cells, and (J) Ly6C+ cells in Gata6f/f and MacGata6 KO mice (n=5). Data were analyzed by two-way ANOVA and Multiple Mann-Whitney tests as described in (B). *P < 0.05. Data are presented as the mean ± SEM.
2.4 Flow cytometry
Single-cell suspensions of peritoneal exudate cells were used for analyzing immune cell profiles by flow cytometry as described previously (20, 21). Briefly, peritoneal exudate cells were lysed using Red Blood Cell Lysis Buffer (BioLegend) and incubated at room temperature for 20 min with Zombie Aqua™ Fixable Viability dye (Bio-Legend). The cells were blocked on ice for 20 min with Fc Block anti-CD16/CD32 (ThermoFisher) and stained with fluorochrome-conjugated monoclonal antibodies for 1 hour (Supplementary Table S1). For GATA6 and Ki67 staining, the cells were fixed and permeabilized with Foxp3/Transcription Factor Staining Buffer Set and Permeabilization Buffer (ThermoFisher). Samples (n=5/group) were acquired with the Attune NxT Acoustic Focusing Cytometer using Attune NxT software (ThermoFisher), and data were analyzed with FlowJo v10.4 software (FLOWJO).
2.5 Von Frey test
A standard behavioral (mechanical sensitivity) test was performed before sample collection, as we have performed and others described previously (26, 55, 56). Mice were allowed to acclimate in the testing room for 30 min, and then the von Frey test was performed using von Frey filaments (BIO-VF-M, Bioseb). Filaments were applied 10 times to the skin perpendicular to the lower abdomen and bilateral hind paws. The force in grams (g) of the filament evoking a withdrawal response (50% response count as sensitive) was recorded. Three behaviors were considered positive responses to filament stimulation: 1) sharp retraction of the abdomen, 2) immediate licking and/or scratching of the area of filament stimulation, or 3) jumping. All behavioral tests were performed blindly without describing the identity and details of treatment groups to investigators assessing pain. These data were then analyzed by another independent investigator.
2.6 IQELISA
Protein yield from peritoneal fluid was quantitated by BCA assay (Pierce), and TNFα (IQM-TNFA-1), IL-1β (IQM-IL1b-1), and IL-6 (IQM-IL6-1) were further quantified by IQELISA kits (Ray Biotech) according to the manufacturer’s instructions (n=5/group).
2.7 Immunohistochemistry
Immunostaining of TRPV1, SP, CGRP, PGP 9.5, and CD68 was performed with cross-sections (5 µm) of paraffin-embedded tissues using specific primary antibodies (Supplementary Table S1) and AlexaFluor 488 or 568-conjugated F(ab’) secondary antibody (Molecular Probe) or VECTASTAIN ABC kit (Vector lab). Immunostaining images were acquired by Leica DM4 B microscopy. Cell-specific CD68 positive and total cell numbers were counted by Image J in the area of 0.07244 mm2, and the percentage of CD68+ cells was shown. PGP9.5 was used as a pan-neuronal marker and was co-stained with TRPV1, SP, or CGRP. TRPV1, SP, or CGRP positive DRG neurons in the section were counted by Image J in the area of 0.07244 mm2, and the percentages of TRPV1, SP, or CGRP positive cells per PGP9.5-positive DRG were shown (n=5/group).
2.8 Quantitative real-time RT-PCR
Total RNA was isolated from peritoneal exudate cells collected from Gata6f/f and MacGata6 KO mice using TRIzol reagent (Sigma). cDNA templates were synthesized from 1 μg of purified RNA using the High-Capacity cDNA Reverse Transcription Kit (Thermo Fisher). Relative gene expression was examined by SYBR Green Master Mix (Thermo Fisher) using a CFX Opus 96 Real-time PCR system (BioRad) as described previously (20, 21). Rpl19 was used as the reference gene to normalize mRNA expression levels. Data were analyzed using the 2-ΔΔCt method, and shown as the relative mRNA expression (n=5/group). Specific primer sets were designed using NCBI’s design suite and are shown in Supplementary Table S2.
2.9 Statistical analysis
Statistical analyses were performed using GraphPad Prism (version 9.5). Mann-Whitney test was used to compare the differences between Gata6f/f and MacGata6 KO mice. One-way ANOVA followed by Dunnett’s multiple comparison tests was used to analyze time-dependent differences within a single group. For grouped data, two-way ANOVA was used to analyze the main effects of ELL induction (depicted as ELL on graphs) and group factor (i.e., GATA6 status, depicted as GATA6; or cell populations, depicted as Group). The interaction effect between the two factors was then calculated (depicted as Int. on graphs). Following two-way ANOVA, to determine the group differences at each time point, multiple Mann-Whitney test with Holm-Šídák correction was used. A P value less than 0.05 was considered to be statistically significant.
3 Results
3.1 Loss of GATA6 in the peritoneal macrophages
Before studying the impact of ELL induction on macrophage populations, we sought to establish the baseline parameters for immune cell distribution, cytokine levels, and pain sensitivity in mice lacking GATA6+ macrophages. In agreement with reported values in three significant papers in 2014 characterizing GATA6+ LPM (38–40), we observed an approximately 50% reduction of CD11b+ cell populations (macrophages) in the peritoneum in MacGata6 KO mice (Figures 1A, B). The percentages of CD11b+ cell populations in Gata6f/f mice (36.5 ± 3.2%) and MacGata6 KO mice (19.7 ± 4.0 %) were shown in Figure 1B (P < 0.05). GATA6+ CD11b+ cells (Figures 1A, B; Gata6f/f, 91.2 ± 2.0 % vs MacGata6 KO, 6.7 ± 1.5 %, P < 0.01 in Figures 1B), or a selective population of F4/80hi MHCIIlo LPM (Supplementary Figure S1A; Gata6f/f, 87.9 ± 0.8 % vs MacGata6 KO, 3.8 ± 1.7 %, P < 0.05) were greatly reduced in the peritoneum, confirming that the Lyz2Cre successfully deleted Gata6, leading to the loss of GATA6+ LPM expressing high F4/80 in MacGata6 KO mice (38, 40). In addition, GATA6-regulated genes were also down-regulated in the peritoneal exudate cells of mice lacking GATA6, confirming the loss of GATA6-driven transcriptional factor action (Supplementary Figure S1B). However, GATA6 deficiency did not affect CD3+ and CD19+ cells in the peritoneum (Figures 1A, B). When MDR occurs by sterile stimuli, inflammation leads to the recruitment of BM-derived monocytes to replace partially long-lived LPM (32, 34, 57, 58), although remaining LPM proliferate substantially (59). In the persistent loss of GATA6+ F4/80hi LPM, an increase in F4/80lo MHCIIhi macrophages has been reported (39). We have also observed an increased F4/80lo MHCIIhi (Supplementary Figure S1A; Gata6f/f, 7.8 ± 1.0 % vs MacGata6 KO, 38.8 ± 2.0 %, P < 0.05) and Ly6C+ cells in MacGata6 KO mice (Figure 1C; Gata6f/f, 4.6 ± 0.5 % vs MacGata6 KO, 9.2 ± 0.9 %, P < 0.01). Furthermore, F4/80int cells had high levels of MHCII in MacGata6 KO mice (F4/80int MHCIIint, 54.0 ± 3.4 %), which was lowly expressed in GATA6f/f mice (3.7 ± 0.8 %) (Supplementary Figure S1A; P < 0.05), indicating that GATA6 deficiency induces persistent replenishment from BM in the peritoneum. TIM4+ residential macrophages were reduced due to the loss of GATA6+ LPM (Figure 1C; Gata6f/f, 80.3 ± 1.7 % vs MacGata6 KO, 51.5 ± 3.1 %, P < 0.01). As expected, F4/80+ cells in MacGata6 KO mice were extremely low in either TIM4+ macrophage populations (Figure 1C; Gata6f/f, 97.2 ± 0.6 % vs MacGata6 KO, 8.2 ± 2.9 %, P < 0.01), or TIM4- macrophage populations (Figure 1C; Gata6f/f, 10.6 ± 3.0 % vs MacGata6 KO, 0.2 ± 0.1 %, P < 0.01). In support of persistent replacement from BM-derived recruited inflammatory macrophages, a high level of MHCII in TIM4+ macrophages was observed in MacGata6 KO mice (Figure 1C; Gata6f/f, 3.3 ± 0.6 % vs MacGata6 KO, 25.5 ± 9.4 %, P < 0.01). While a higher basal proliferation rate (SG2M phases) of peritoneal macrophages was reported in MacGata6 KO mice (39), Ki67+ cells in either TIM4+ or – macrophages were not altered by loss of GATA6+ LPM in the steady state (Figure 1C). Peritoneal cytokine levels, such as TNFα, IL-1β, and IL-6 (Figure 1D), as well as abdominal and paw hypersensitivity, were not significantly different between Gata6f/f and MacGata6 KO mice (Figure 1E).
3.2 Dysregulated peritoneal macrophage resolutions in the absence of GATA6 during endometriosis lesion development
We next induced ELL in Gata6f/f and MacGata6 KO mice to examine how the loss of GATA6+ LPM affects BM-derived and peritoneal residential macrophages and their resolutions from the stimuli of lesion induction (Figures 2, 3). While the CD11b+ population was reduced in MacGata6 KO mice prior to ELL induction, within 42 days after ELL induction, there were no significant differences in the CD11b+ population between Gata6f/f and MacGata6 KO mice after ELL induction (Figure 2B). As shown in Figures 2C, D, Supplementary Figure S2A, ELL induction in GATA6f/f mice induced the disappearance of TIM4hi MHCIIlo residential macrophages 3 days after ELL induction (day -1, 81.3 ± 2.2 % vs. day 3, 26.2 ± 9.1 %, P < 0.0001 in Supplementary Figure S2A). Concurrently, the influx of increased Ly6C+ monocytes was observed 3 days after ELL induction (Figure 2E; day -1, 3.8 ± 0.5 % vs. day 3, 36.1 ± 3.6 %, P < 0.0001). At the same time, TIM4lo MHCIIhi macrophages, as shown in Figures 2C, D and Supplementary Figure S2A, were significantly elevated (day -1, 11.8 ± 1.4 % vs. day 3, 25.2 ± 2.5 %,P < 0.001 in Supplementary Figure S2A) following an increase of Ly6C+ and TIM4lo MHCIIlo cells as the recruitment of inflammatory macrophages (Figures 2C–E, Supplementary Figure S2A) (15, 21, 60). By day 7, MHCIIhi inflammatory macrophages exhibited TIM4int MHCIIhi phenotypes consistent with inflammatory macrophages. From days 21-42, TIM4hi MHCIIlo residential macrophages recovered and repopulated to the baseline distribution (Figures 2C, D, Supplementary Figure S2A). In contrast, a more significant disappearance of TIM4hi MHCIIhi residential macrophages occurred in MacGata6 KO mice within 3 days (Figures 2F, G, I, Supplementary Figure S2B). The percentages of TIM4hi MHCIIhi macrophages were 49.5 ± 2.5 % at day -1 and 2.3 ± 0.9 % at day 3 as shown in Supplementary Figure S2B (P < 0.0001). Enhanced recruitment of Ly6C+ monocytes (Figures 2H; day -1, 9.2 ± 0.9 % vs. day 3, 32.6 ± 8.8 %, P < 0.0001), or TIM4lo MHCIIhi inflammatory macrophages (Figures 2F, G; day -1, 28.5 ± 1.2 % vs. day 3, 56.5 ± 3.2 %, P < 0.01 shown in Supplementary Figure S2B) was also observed by day 3. Interestingly, the recruitment of MHCIIhi inflammatory macrophages was extensive in MacGata6 KO mice (Figures 2F, G, I), probably due to the severe disappearance of TIM4hi MHCIIhi residential macrophages. While repopulation of TIM4hi MHCIIhi macrophages continuously occurred in MacGata6 KO, the replenishment of TIM4hi MHCIIhi residential macrophages was slower than in Gata6f/f mice (Figures 2F, G, I, Supplementary Figure S2B). As shown in Supplementary Figure S2AB, TIM4hi matured residential macrophages recovered to the baseline by day 21 in Gata6f/f mice (Supplementary Figure S2A; day -1, 81.3 ± 2.2 % vs. day 21, 61.3 ± 4.0 %, N.S.), while replenishment of TIM4hi macrophages was still ongoing at day 42 in MacGata6 KO mice (Supplementary Figure S2B; day -1, 49.5 ± 2.5 % vs. day 21, 27.8 ± 2.6 %, P < 0.001). Indeed, TIM4int MHCIIhi macrophages (unmatured) were elevated at only day 7 in Gata6f/f mice (Figures 2C, D, Supplementary Figure S2A), whereas TIM4int MHCIIhi macrophages in MacGata6 KO mice disappeared within 3 days and then repopulated but remained persistent at day 42 during the resolution phase of resident macrophages (Figures 2F, G, I, Supplementary Figure S2B). Thus, GATA6 deficiency in LPM limits the ability of TIM4hi residential macrophage repopulation following MDR within 6 weeks due to probably loss of proliferative ability with transcriptional signatures (38, 39) and persistently recruits MHCIIhi inflammatory macrophages but fails to acquire a mature resident phenotype. Subsequently, Ki67 expression confirmed GATA6-dependent proliferative ability, showing different proliferative phenotypes of TIM4+ residential macrophages (Figure 3). After the initial disappearance of TIM4+ residential macrophages by ELL induction in Gata6f/f mice, the remaining TIM4+ residential macrophages actively proliferated by self-renewal within day 3 (Figures 3A, B). As shown in Figure 3B, the percentages of TIM4+ Ki67+ macrophages were significantly increased on day 3 (10.15 ± 4.0 %) compared to day -1 (2.4 ± 0.6 %). This active proliferation probably contributed to the recovery of the TIM4+ residential macrophage population by day 21 (Supplementary Figure S2A). In MacGata6 KO mice, TIM4+ residential macrophages almost entirely disappeared and lost their proliferative ability, although they gradually gained proliferative activity to the baseline (Figures 3A, B). In contrast, TIM4- macrophages possess a higher proliferative state in MacGata6 KO mice, possibly due to the continuous recruitment of inflammatory macrophages.
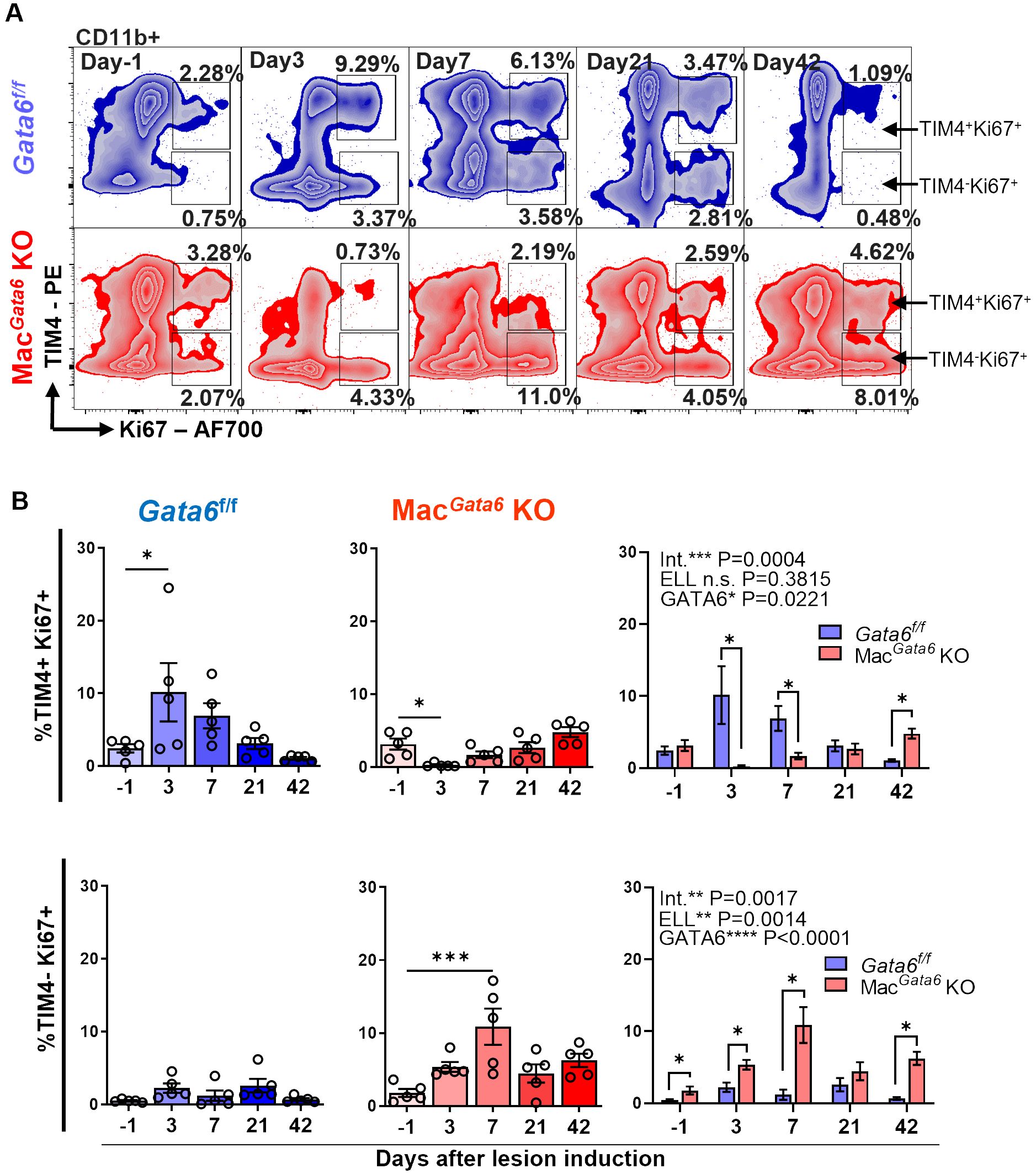
Figure 3. Analysis of TIM4+ Ki67+ and TIM4- Ki67+ cells in CD11b+ subsets after ELL induction in Gata6f/f and MacGata6 KO mice. (A) Representative flow cytometric plots of CD11b+ cells gated with TIM4 and Ki67. (B) Bar graphs showing the TIM4+ Ki67+ (top panel) and TIM4- Ki67+ (bottom panel) cell percentages in Gata6 f/f in blue, or MacGata6 KO in red, or together. Data are presented as the mean ± SEM (n=5). One-way ANOVA, followed by Dunnett’s multiple comparison test, was used to analyze the time-dependent differences in each group. Two-way ANOVA was performed to determine the main effects of ELL induction (depicted as ELL), GATA6 status (depicted as GATA6), and the interaction effect between the two factors (depicted as Int.). The differences between Gata6f/f and MacGata6 KO mice at each time point were compared by the Multiple Mann-Whitney test. *P < 0.05, ***P < 0.001.
3.3 Peritoneal inflammation in the absence of GATA6 during endometriosis lesion development
While abundant cytokines and chemokines have been observed in the pelvic cavity of endometriosis patients (13, 16), TNFα, IL-1β, and IL-6 are considered the key factors involved in maintaining the aberrant peritoneal inflammatory environment, promoting lesion growth, and mediating peripheral sensitization (61–63). Elevated TNFα, IL-1β, and IL-6 levels have been reported in the blood, peritoneal fluids, and/or eutopic and ectopic endometrial tissues of women with endometriosis (13, 64–66). Specifically, the levels of TNFα, IL-1β, and IL-6 are increased in peritoneal macrophages isolated from endometriosis patients (67). Thus, we next analyzed the secretion of proinflammatory cytokines, TNFα, IL-1β, and IL-6, in the peritoneal fluids to assess how dysregulated peritoneal macrophage resolutions in the absence of GATA6 affect cytokine secretions (Figure 4). ELL induction time-dependently affected cytokine secretions (Figure 4A). Peritoneal TNFα, IL-1β, and IL-6 were elevated immediately after ELL induction on day 3 and remained high on day 7 but returned to the baseline by day 21 in both Gata6f/f and MacGata6 KO mice (Figure 4A). When cytokine levels were compared between Gata6f/f and MacGata6 KO mice at each time point, TNFα at day 21 in Gata6f/f mice was higher than in MacGata6 KO mice (Figure 4B; Gata6f/f, 412.1 ± 60.7 pg/ml vs. MacGata6 KO, 186.1 ± 8.9 pg/ml, P < 0.05). Although MHCIIhi macrophages were continuously recruited 3-6 weeks after ELL induction in MacGata6 KO mice (Figures 2F, G, I), the persistent recruitment of inflammatory macrophages did not accelerate the cytokine levels in the peritoneum.
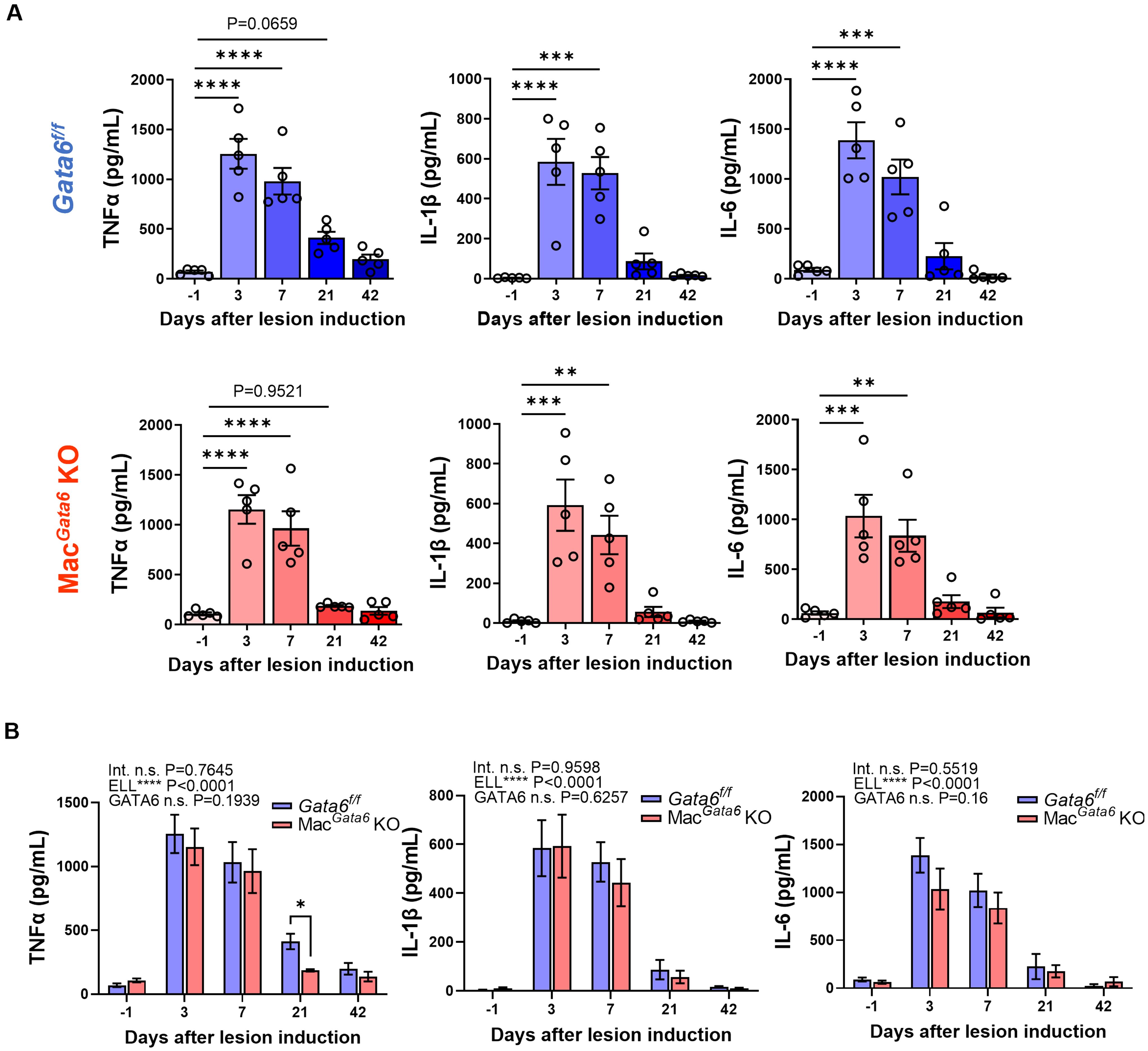
Figure 4. Proinflammatory cytokine levels (TNFα, IL-1β, and IL-6) after ELL induction in Gata6f/f and MacGata6 KO mice. Cytokine levels were shown in a single group (A) or grouped plots (B). Data are presented as the mean ± SEM (n=5). (A) Data were analyzed with one-way ANOVA followed by Dunnett’s multiple comparisons test. **P < 0.01, ***P < 0.001, and ****P < 0.0001. (B) Two-way ANOVA was performed to analyze the main effects of ELL induction (depicted as ELL), GATA6 status (depicted as GATA6), and the interaction effect between the two factors (depicted as Int.). The difference between Gata6f/f and MacGata6 KO mice at each time point was determined by the Multiple Mann-Whitney test. *P < 0.05.
3.4 Endometriosis-associated hyperalgesia and pain-related mediators in the absence of GATA6 during endometriosis lesion development
Inflammatory factors in the peritoneum from endometriosis patients can be inflammatory mediators that stimulate neurite outgrowth and endometriosis-associated hyperalgesia (20, 26, 68). We next performed the von Frey test to examine the abdominal and hind paw retraction threshold to determine whether loss of GATA6+ LPM affects endometriosis-associated hyperalgesia (Figure 5). ELL induction affected time-dependently both abdominal and hind paw sensitivities (Figure 5A). Gata6f/f and MacGata6 KO mice withdrew abdominal retraction thresholds with significantly lighter stimuli during the entire study period (Figure 5A), even though cytokines levels were only elevated by day 7 (Figure 4). Gata6f/f mice tended to show higher sensitivity after day 21 when the abdominal retraction was compared between Gata6f/f and MacGata6 KO mice at each time point (Figure 5B; Gata6f/f, 0.04 ± 0.01 g vs. MacGata6 KO, 0.09 ± 0.02 g at day 21, P = 0.18; Gata6f/f, 0.04 ± 0.01 g vs. MacGata6 KO, 0.08 ± 0.01 g at day 42, P = 0.08). The hind paw retraction thresholds were sensitive in Gata6f/f mice until day 21, but after day 7 in MacGata6 KO mice, sensitivity returned to the preinduction threshold (Figure 5A), whereas no different sensitivity was observed between the groups (Figure 5B). The results suggested that Gata6f/f mice tended to sustain higher endometriosis-associated hyperalgesia for extended periods.
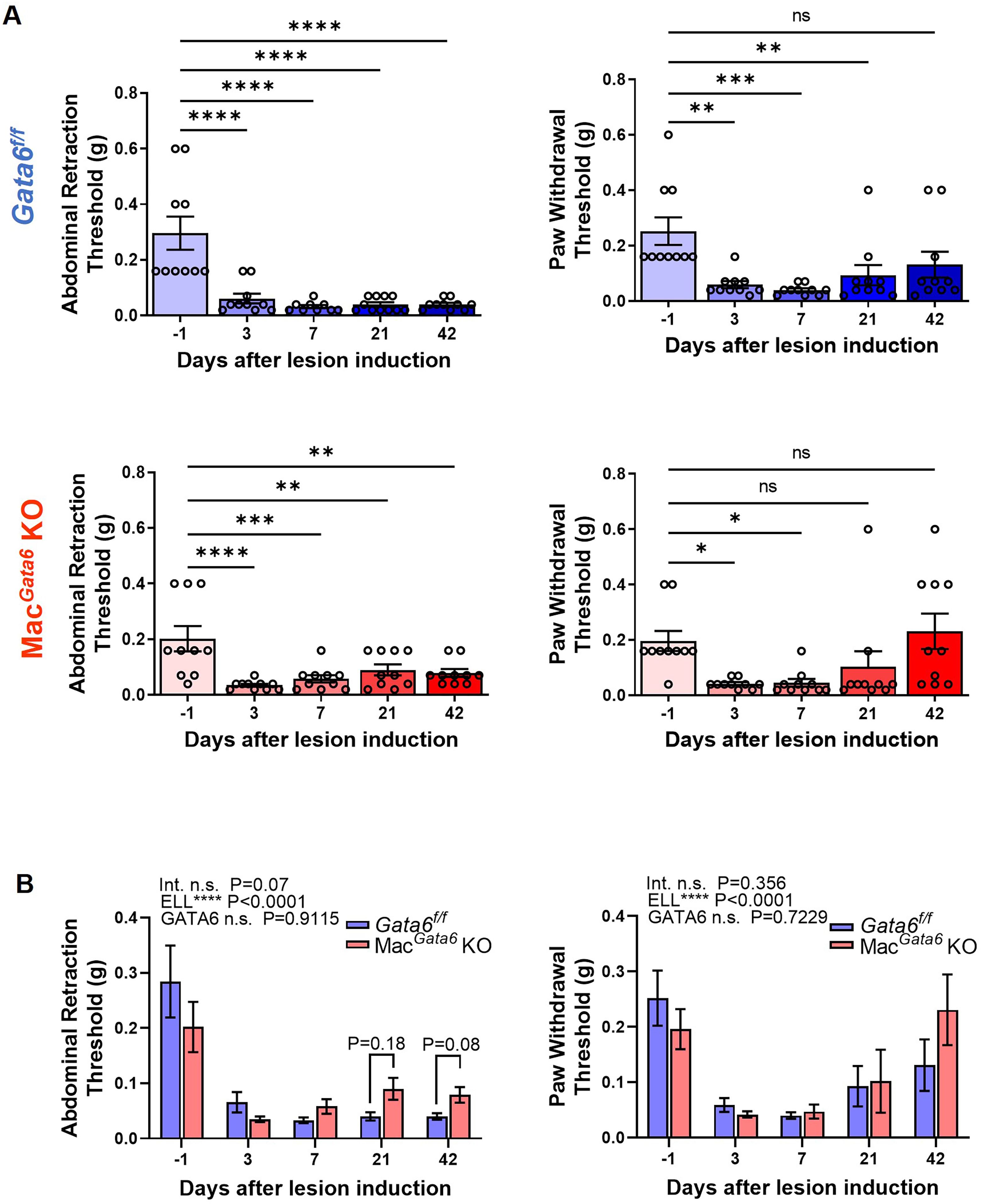
Figure 5. Evaluation of endometriosis-associated hyperalgesia followed by ELL induction in Gata6f/f and MacGata6 KO mice. Abdominal and hind paw withdrawal thresholds were assessed by the von Frey test in Gata6f/f and MacGata6 KO mice. Data are shown by individual group (A) and grouped graphs (B) as mean ± SEM (n = 10). (A) Date were analyzed with one-way ANOVA followed by Dunnett’s multiple comparison test. *P < 0.05, **P < 0.01, ***P < 0.001, ****P < 0.0001. (B) Two-way ANOVA was performed to analyze the main effects of ELL induction (depicted as ELL), GATA6 status (depicted as GATA6), and the interaction effect between the two factors (depicted as Int.). The difference between Gata6f/f and MacGata6 KO mice at each time point was determined by the Multiple Mann-Whitney test.
Endometriosis-associated hyperalgesia, especially local abdominal sensitivity, should be correlated with the stimuli in the peripheral nerve terminals of nociceptor neurons (69), such as an increase in the expression of transient receptor potential channels, TRPV1. Peripheral nerves can be activated with the increased release of neurotransmitters, such as SP and CGRP. We thus examined TRPV1, SP, and CGRP in the L4-6 DRG, the primary spinal ganglia receiving sensory input from pelvic organs (Figure 6). ELL induction increased TRPV1, SP, and CGRP expression time-dependently (Figures 6A, B). More TRPV1+ DRG were observed until day 21 in Gata6f/f mice and until day 7 in MacGata6 KO mice (Figures 6A, B). Higher TRPV1+ neurons were detected in Gata6f/f mice at day 21 compared with those in MacGata6 KO mice (Figure 6B; Gata6f/f, 14.1 ± 1.2 % vs. MacGata6 KO, 7.1 ± 1.3 % at day 21, P = 0.039). SP+ DRG were significantly high until day 21 compared with that in the preinduction level in both mouse groups (Figures 6A, B). CGRP+ DRG were elevated until day 42 in Gata6f/f mice and until day 21 in MacGata6 KO mice (Figures 6A, B). Thus, the results support behavior analysis, in which Gata6f/f mice potentially sustained higher endometriosis-associated hyperalgesia than MacGata6 KO mice at later periods.
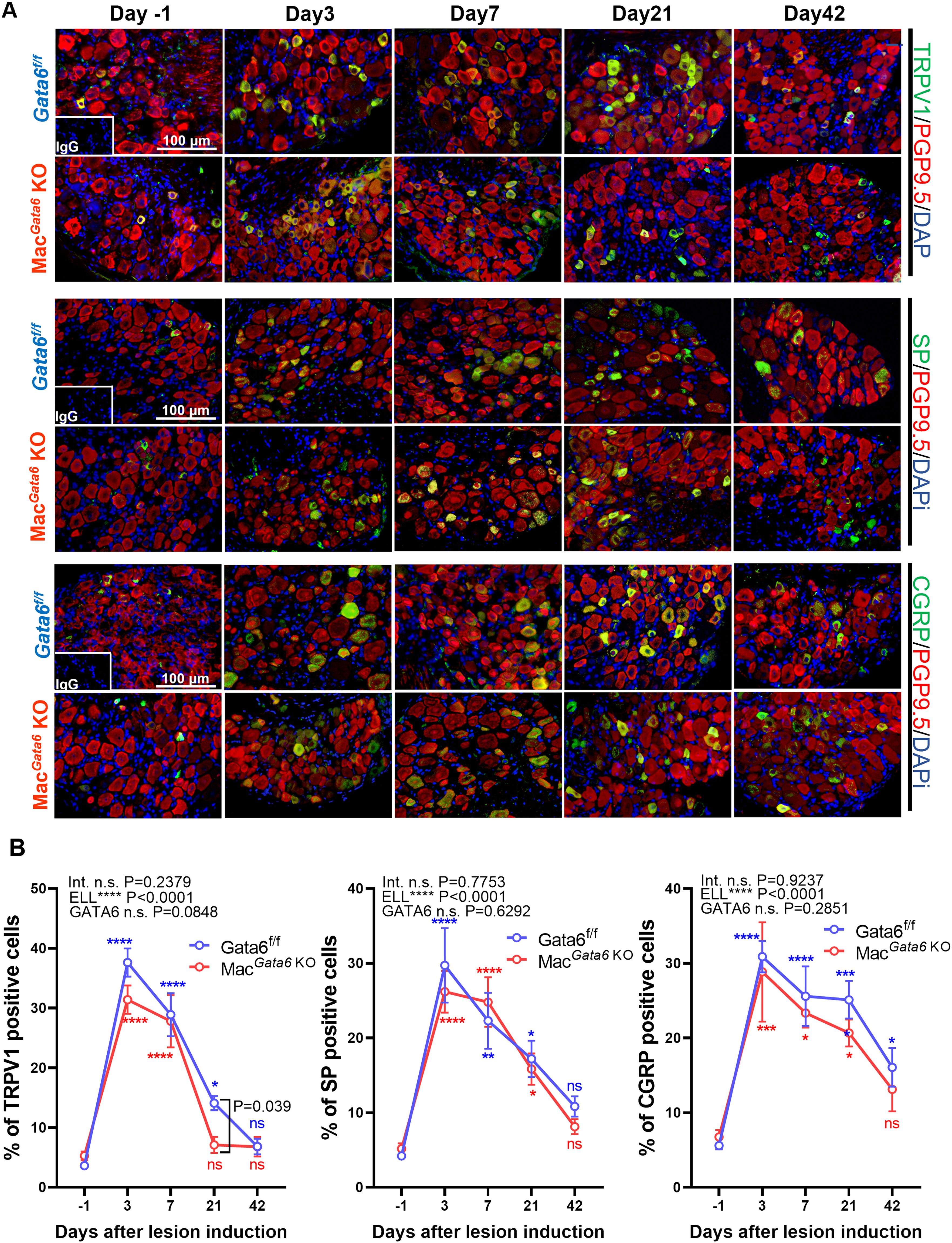
Figure 6. Expression of TRPV1, SP, and CGRP in DRG from Gata6f/f and MacGata6 KO mice after ELL induction. (A) Representative images showing DRG sections double stained with TRPV1, SP, or CGRP (green), and PGP9.5 (red), as a marker of DRG cell body. (B) Quantification of TRPV1+, SP+, or CGRP+ cells in PGP9.5+ cells. Data are shown as the mean ± SEM (n=5). Two-way ANOVA was performed to analyze the main effects of ELL induction (depicted as ELL), GATA6 status (depicted as GATA6), and the interaction effect between the two factors (depicted as Int.). Following two-way ANOVA, Dunnett’s multiple comparison test was used to analyze time-dependent differences compared with day -1 within a group labeled blue (Gata6f/f) or red (MacGata6 KO). The difference between Gata6f/f and MacGata6 KO mice at each time point was determined by the Multiple Mann-Whitney test. *P < 0.05, **P < 0.01, ***P < 0.001, ****P < 0.0001.
3.5 Endometriosis lesion progression and macrophage presence in the absence of GATA6
Lastly, we assessed how GATA6 deficiency affects the progression and growth of endometriosis lesions. Loss of GATA6+ LPM did not alter lesion number during the study period (Figure 7A). Because we previously reported that peritoneal macrophages can infiltrate ELL (20), we addressed macrophage infiltration in the lesion staining with CD68, a macrophage marker. We only examined macrophage infiltration in the lesion on days 21 and 42, as lesion number, endometriosis-associate hyperalgesia, cytokine levels in the peritoneal cavity, and TRPV1, SP, and CGRP expression in the DRG were not altered by GATA6 deficiency until day 7 in Gata6f/f and MacGata6 KO mice. CD68+ macrophages were comparable in both mouse groups at days 21 and 42 (Figure 7B). Thus, the absence of GATA6 in the LPM did not affect lesion progression and macrophage infiltration.
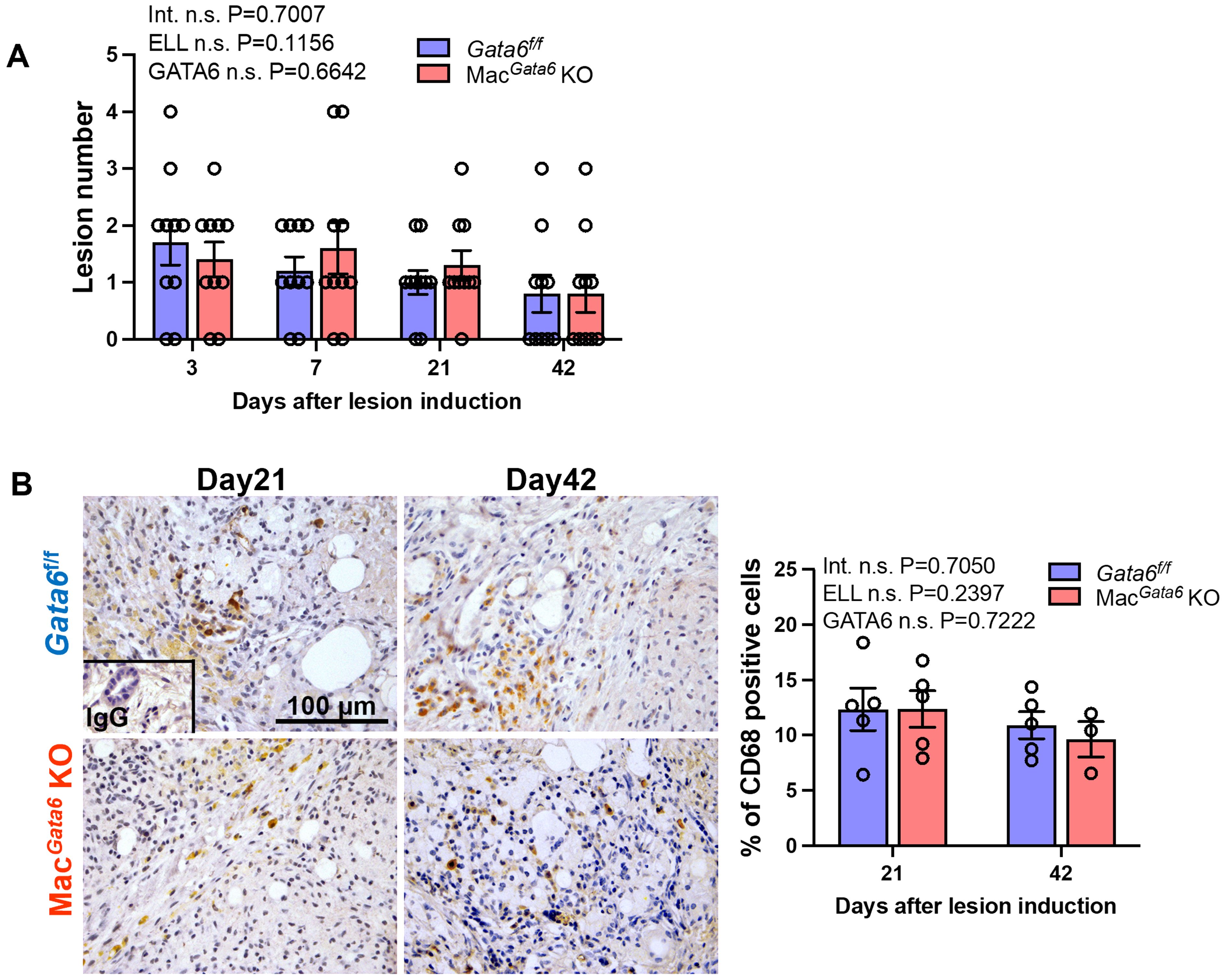
Figure 7. Analysis of ELL in Gata6f/f and MacGata6 KO mice. (A) Quantification of lesion numbers in Gata6f/f and MacGata6 KO mice after ELL induction (n=10). (B) Representative immunohistochemical images (left) and quantification (right) of CD68+ macrophages in the lesions of Gata6f/f and MacGata6 KO mice on days 21 and 42 (n=5). Data are shown as the mean ± SEM. Two-way ANOVA was performed to analyze the main effects of ELL induction (depicted as ELL), GATA6 status (depicted as GATA6), and the interaction effect between the two factors (depicted as Int.). The difference between Gata6f/f and MacGata6 KO mice at each time point was determined by the Multiple Mann-Whitney test. No significant difference was detected.
4 Discussion
Peritoneal macrophages are known to contribute to endometriosis pathophysiology, as dysregulated macrophages increase the secretion of proinflammatory cytokines and reduce phagocytic functions in women with endometriosis (23, 25, 70, 71). Bacci et al. (24) demonstrate that peritoneal macrophages are required for lesion establishment, growth, and vascularization in a mouse model of endometriosis. However, peritoneal macrophages are highly diverse (21, 72), differ in their ontogeny (31), and have transcriptionally and functionally divergent features depending on the signals of the local environment (30). Hogg et al. (15) have reported different characteristics of macrophages depending on their ontogeny in the pathogenesis of endometriosis. They found that monocytopenic mice, which limit monocyte recruitment and subsequently reduce LPM and SPM replenishment, develop increased lesions (15). Furthermore, fewer lesions are developed in mice when embryo-derived LPM are replaced by monocyte-derived LPM, indicating that monocyte-derived LPM protect against endometriosis development (15). In the present study, we focused on the roles of embryo-derived GATA6+ LPM, which represent the majority of peritoneal residential macrophages, during ELL establishment and progression. When menses-like tissues are introduced in the peritoneum, it causes acute inflammatory responses. GATA6+ LPM are important for the initial uptake where they adhere to the mesothelium to cover organs (41, 42) or die via pyroptosis to release proinflammatory cytokines, such as IL-1β (73), called MDR. If LPM die/disappear, they appear to be replaced by BM-derived macrophages (34). However, when LPM adhere to the walls or mesothelium, they eventually detach and return to the residential LPM in the peritoneum (34). In the present study of Gata6f/f mice, peritoneal residential LPM (TIM4+) disappeared after ELL induction, BM-derived monocytes (Ly6C+) were recruited/increased, and MHCIIhi macrophages were elevated in the peritoneum. This likely happens immediately after ELL induction, as sterile inflammation induced by intraperitoneal injection with a low dose of zymosan (10 µg) causes MDR and recruited monocytes and inflammatory macrophages in the peritoneum within 4 hours (37). MDR from ELL induction was still observed on day 3 in our study. The significant recruitment of Ly6C+ monocytes and MHCIIhi macrophages was present until day 21, indicating that LPM pyroptosis with replenishment from BM-derived macrophages also occurs. Supporting the MDR via pyroptosis (34), proinflammatory cytokines, such as TNFα, IL-1β, and IL-6, were significantly elevated in the first week after ELL induction. In contrast, because resident macrophages did not wholly disappear, the remaining macrophages rebounded in number by day 21. In support of this, proliferation in residential LPM was active in the first 3 days and tended to be high even on day 7. Based on the results of increased Ly6C+ cells, cytokine levels, and proliferation, “acute inflammatory reactions” continue until 7 days after ELL induction, which is longer than that is induced by a low dose of zymosan (37). During days 21-42, repopulated TIM4hi residential macrophages and BM-derived TIM4lo MHCIIhi and TIM4int MHCIIhi macrophages returned to the baseline level. LPM in the peritoneum appear to be reestablished by replenishment from BM-derived macrophages and proliferation of remaining residential LPM. Thus, Days 21-42 are likely to be a resolution phase from the inflammatory stimuli (ELL induction). However, we are uncertain whether LPM are fully functional, as monocyte-derived LPM are functionally distinct from embryo-derived residential LPM (37).
Deficiency of GATA6 in the peritoneum induces loss of F4/80hi MHCIIlo macrophages (LPM), as previously reported (38, 40). We also observed a significantly reduced macrophage population of highly expressed F4/80. Loss of GATA6 reduced TIM4+ resident macrophages as well. Due to the persistent reduction of LPM or resident macrophages, consistent recruitment from BM-derived monocytes and macrophages was seen in our study, as Ly6C+ monocytes and MHCIIhi macrophages were elevated. In contrast, proinflammatory cytokines were not elevated in the peritoneum of MacGata6 KO mice, suggesting that genetically engineered deletion of GATA6 is different from MDR via pyroptosis to release proinflammatory cytokines. When ELL was induced in MacGata6 KO mice, severe MDR occurred, as TIM4+ resident macrophages almost completely disappeared in addition to the loss of F4/80hi macrophages on day 3. At the same time, MHCIIhi macrophages were continuously recruited and maintained at high levels, even on day 42. GATA6 deficiency disrupts peritoneal macrophage proliferation, as GATA6 controls peritoneal macrophage proliferation via its target genes (38, 39). After ELL induction, the remaining residential macrophages are likely less proliferative due to the severe disappearance of TIM4+ residential macrophages in MacGata6 KO mice on day 3. Instead, recruited TIM4lo MHCIIhi macrophages presented higher proliferative activity. However, TIM4+ resident macrophages did not fully recover by day 42, indicating that BM-derived TIM4+ macrophages probably have a less proliferative ability due to GATA6 deficiency; they are probably distinct from embryo-derived LPM.
Severe MDR also elevated proinflammatory cytokine levels in the peritoneum of MacGata6 KO mice, although we only evaluated TNFα, IL-1β, and IL-6, probably via pyroptosis, similar to those in Gata6f/f mice. Interestingly, TNFα was higher on day 21 in Gata6f/f mice than in MacGata6 KO mice. GATA6+ macrophages use an inhibitory pathway to suppress IL-1β processing, a potent activator of the inflammatory cascade in response to inflammatory stimuli, to solve early stages of inflammation (74). It is unclear whether and how GATA6+ macrophages are still involved in cytokine secretion after the acute phase of inflammation, and it remains to be examined. However, newly recruited monocyte-derived LPM can protect against lesion development (15). Since GATA6 deficiency enhanced the recruitment of BM-derived macrophages to replenish resident macrophages, they might be involved in the reduction of cytokine levels in the peritoneal cavity. Alternatively, the persistent recruitment of BM-derived macrophages is not directly correlated with cytokine secretion in the peritoneal cavity. On the other hand, GATA6+ macrophages can become tumor-associated macrophages to contribute to tumor progression (41, 42), as deletion of peritoneal macrophages reduces tumor progression and peritoneal metastasis in ovarian cancer (46, 47). Peritoneal macrophages expressing higher amounts of a proangiogenic factor, VEGF, are associated with peritoneal metastasis in gastric cancer (50, 75). GATA6+ LPM can invade liver malignant tumors and contribute to metastatic tumor growth and recurrence (51). In the mouse model of endometriosis, >25% of macrophages in the lesions are LPM in the peritoneum, indicating that significant trafficking of LPM into the endometriotic lesion might be favorable to maintaining chronic inflammation and disease progression (15).
We showed that the GATA6-deficient peritoneal macrophages did not affect abdominal and paw hyperalgesia in a steady state. When ELL was induced, both Gata6f/f and MacGata6 KO mice immediately exhibited increased endometriosis-associated hypersensitivity in the abdomen on day 3 and sustained until day 42. Increased expression of pain-related mediators supports the result of endometriosis-associated hyperalgesia that was sustained for nearly 6 weeks. However, the sensitivity in the abdomen tended to be severe in Gata6f/f mice on days 21-42, when most peritoneal macrophage replenishment was resolved. In support of local abdominal sensitivity in Gata6f/f mice, TRPV1 expression was higher on day 21. Systemic paw sensitivity was significantly high in Gata6f/f mice until day 21, whereas it was no longer significant on day 21 in MacGata6 KO mice. The results suggest that ELL induce acute inflammation in the peritoneum, immediately sensitizing the pain-related neurons. While the resolution of the immune cell population from the stimuli of ELL induction is relatively fast, endometriosis-associated hyperalgesia is sustained longer because the stimuli likely further sensitize pain-related neurons.
MacGata6 KO mice showed slightly less hyperalgesia, which can be supported by TNFα level in the peritoneum and TRPV1 expression in the DRG. Hogg et al. (15) demonstrate that monocyte-derived peritoneal macrophages are anti-endometriosis to protect the cavity from lesion development. MacGata6 KO mice constantly recruited SPM (monocyte-derived macrophages) due to the deficiency of GATA6 and further enhanced the replenishment of residential macrophages after ELL induction. Thus, large numbers of BM-origin monocyte-derived macrophages exist in the peritoneum. Indeed, TIM4int macrophages differentiated from monocytes are significantly higher in MacGata6 KO mice on days 21-42. Therefore, although its mechanism is unclear, monocyte-derived macrophages might also protect against inflammatory stimuli during the resolution phase of inflammation. However, increased TIM4int macrophages were observed in both Gata6f/f and MacGata6 KO mice, suggesting that GATA6+ macrophages do not affect earlier lesion development, including establishment. As a supporting result, the lesion numbers were not altered at any stage.
Gibson et al. (9) have reported a human study about endometriosis and peritoneal macrophages. The group shows that CD14hi peritoneal macrophages considered resident peritoneal macrophages in humans (52) are significantly higher than CD14lo peritoneal macrophages in women with endometriosis (9). CD14hi peritoneal macrophages negatively correlate with pain score, whereas CD14lo peritoneal macrophages are positively correlated, independent of endometriosis diagnostic outcome at laparoscopy (9). They assume CD14hi and CD14lo in humans to be equivalent to LPM and SPM in mice (76). However, the authors have noted that CD14hi macrophages likely account for BM-derived macrophages, as monocyte-derived LPM (CD14hi macrophages) are not distinguished. The group concludes that pain symptoms do not correlate with disease extent, as pain scores do not differ among patients at the rASRM stages, indicating inflammatory or immune cell profiles might be a better predictor of endometriosis pain than lesion extent identified during laparoscopy (9).
Evolutionarily, retained body cavities protect internal organs from the external toxic environment, and cavity macrophages contribute to various immune responses (42). LPM maintain peritoneal cavity homeostasis, providing the first line of defense mechanisms (41, 42). Rigorous studies present the vital roles of LPM in repairing injuries and controlling infections by GATA6-driven transcriptional program (41, 42). In the present study, we used a mouse model of endometriosis to study the role of GATA6 resident macrophages following the resolution of inflammatory stimuli (lesion induction). The results suggest the involvement of peritoneal GATA6+ macrophages in the peritoneal inflammatory environment, including the reprogramming of monocyte-derived macrophages. Because GATA6 deficiency induced the extensive recruitment of monocyte-derived macrophages and enhanced the replenishment of residential macrophages after ELL induction, they might protect against inflammatory stimuli, especially during the resolution phase. Therefore, GATA6+ LPM might be favorable toward sustaining local inflammation in the peritoneum and sensitivities in the neurons, reflecting potentially endometriosis-associated pain during the process of resolution of inflammatory stimuli. Retrograde menstruation causes massive inflammatory responses in the peritoneum. However, menstrual cycles repeatedly occur in women; each retrograde menstruation induces composite inflammation in the pelvic cavity, and unsolved inflammation is expected to worsen to develop chronic conditions further. It might be necessary to induce multiple inflammatory stimuli (multiple ELL induction) to understand the role of residential macrophages and their resolution process in the peritoneum associated with endometriosis-associated hyperalgesia.
Data availability statement
The original contributions presented in the study are included in the article/Supplementary Material. Further inquiries can be directed to the corresponding author.
Ethics statement
The animal study was approved by Washington State University IACUC. The study was conducted in accordance with the local legislation and institutional requirements.
Author contributions
MS: Conceptualization, Data curation, Writing – review & editing, Formal analysis, Investigation, Methodology, Validation, Visualization. JM: Writing – review & editing, Supervision. KH: Supervision, Writing – review & editing, Conceptualization, Data curation, Funding acquisition, Project administration, Resources, Writing – original draft.
Funding
The author(s) declare financial support was received for the research, authorship, and/or publication of this article. This work was supported by NIH/NICHD, R01HD104619 (to KH).
Conflict of interest
The authors declare that the research was conducted in the absence of any commercial or financial relationships that could be construed as a potential conflict of interest.
Publisher’s note
All claims expressed in this article are solely those of the authors and do not necessarily represent those of their affiliated organizations, or those of the publisher, the editors and the reviewers. Any product that may be evaluated in this article, or claim that may be made by its manufacturer, is not guaranteed or endorsed by the publisher.
Supplementary material
The Supplementary Material for this article can be found online at: https://www.frontiersin.org/articles/10.3389/fimmu.2024.1396000/full#supplementary-material
Supplementary Figure 1 | Verification of Gata6 depletion in peritoneal macrophages. (A) CD11b+ cells were gated with F4/80 and MHCII to identify LPM, IM, and SPM. SPM, small peritoneal macrophage; IM, intermediate macrophage; LPM, large peritoneal macrophage. Two-way ANOVA was performed to analyze the mean difference of cell populations (depicted as Population), GATA6 status (depicted as GATA6), and the interaction effect between the two factors (depicted as Int.). The difference between Gata6f/f and MacGata6 KO mice was determined by the Multiple Mann-Whitney test. *P < 0.05. (B) The expression of Gata6-regulated genes was examined by Real-time RT-PCR in peritoneal cells (n=5). The Mann-Whitney test was performed to compare the differences between Gata6f/f and MacGata6 KO mice. Data are shown as the mean ± SEM. *P < 0.05, **P < 0.01.
Supplementary Figure 2 | Time course analysis of TIM4 and MHCII gated four cell proportions of CD11b+ subsets in Gata6f/f and MacGata6 KO mice after ELL induction. Percentages of TIM4hi MHCIIlo(hi), TIM4int MHCIIhi, TIM4lo MHCIIhi, and TIM4lo MHCIIlo cells in Gata6f/f mice (A) and MacGata6 KO mice (B) following ELL induction. Data were analyzed by one-way ANOVA followed by Dunnett’s multiple comparison test and presented as mean ± SEM (n=5). *P < 0.05, **P < 0.01, ***P < 0.001, ****P < 0.0001.
References
1. Saunders PTK, Horne AW. Endometriosis: etiology, pathobiology, and therapeutic prospects. Cell. (2021) 184:2807–24. doi: 10.1016/j.cell.2021.04.041
2. Zondervan KT, Becker CM, Missmer SA. Endometriosis. New Engl J Med. (2020) 382:1244–56. doi: 10.1056/NEJMra1810764
3. Nnoaham KE, Hummelshoj L, Webster P, d'Hooghe T, de Cicco Nardone F, de Cicco Nardone C, et al. Impact of endometriosis on quality of life and work productivity: A multicenter study across ten countries. Fertility Sterility. (2011) 96:366–73 e8. doi: 10.1016/j.fertnstert.2011.05.090
4. Sepulcri Rde P, do Amaral VF. Depressive symptoms, anxiety, and quality of life in women with pelvic endometriosis. Eur J Obstet Gynecol Reprod Biol. (2009) 142:53–6. doi: 10.1016/j.ejogrb.2008.09.003
5. Simoens S, Dunselman G, Dirksen C, Hummelshoj L, Bokor A, Brandes I, et al. The burden of endometriosis: costs and quality of life of women with endometriosis and treated in referral centres. Hum Reprod. (2012) 27:1292–9. doi: 10.1093/humrep/des073
6. Zondervan KT, Becker CM, Koga K, Missmer SA, Taylor RN, Vigano P. Endometriosis. Nat Rev Dis Primers. (2018) 4:9. doi: 10.1038/s41572-018-0008-5
7. Revised american society for reproductive medicine classification of endometriosis: 1996. Fertility Sterility. (1997) 67:817–21. doi: 10.1016/S0015-0282(97)81391-X
8. Vercellini P, Fedele L, Aimi G, Pietropaolo G, Consonni D, Crosignani PG. Association between endometriosis stage, lesion type, patient characteristics and severity of pelvic pain symptoms: A multivariate analysis of over 1000 patients. Hum Reprod. (2007) 22:266–71. doi: 10.1093/humrep/del339
9. Gibson DA, Collins F, De Leo B, Horne AW, Saunders PTK. Pelvic pain correlates with peritoneal macrophage abundance not endometriosis. Reprod Fertil. (2021) 2:47–57. doi: 10.1530/RAF-20-0072
10. Johnson NP, Hummelshoj L, Adamson GD, Keckstein J, Taylor HS, Abrao MS, et al. World endometriosis society consensus on the classification of endometriosis. Hum Reprod. (2017) 32:315–24. doi: 10.1093/humrep/dew293
11. Berkkanoglu M, Arici A. Immunology and endometriosis. Am J Reprod Immunol. (2003) 50:48–59. doi: 10.1034/j.1600-0897.2003.00042.x
12. Capobianco A, Monno A, Cottone L, Venneri MA, Biziato D, Di Puppo F, et al. Proangiogenic tie2(+) macrophages infiltrate human and murine endometriotic lesions and dictate their growth in a mouse model of the disease. Am J Pathol. (2011) 179:2651–9. doi: 10.1016/j.ajpath.2011.07.029
13. Rana N, Braun DP, House R, Gebel H, Rotman C, Dmowski WP. Basal and stimulated secretion of cytokines by peritoneal macrophages in women with endometriosis. Fertility Sterility. (1996) 65:925–30. doi: 10.1016/S0015-0282(16)58262-4
14. Hogg C, Horne AW, Greaves E. Endometriosis-associated macrophages: origin, phenotype, and function. Front Endocrinol (Lausanne). (2020) 11:7. doi: 10.3389/fendo.2020.00007
15. Hogg C, Panir K, Dhami P, Rosser M, Mack M, Soong D, et al. Macrophages inhibit and enhance endometriosis depending on their origin. Proc Natl Acad Sci U.S.A. (2021) 118:e2013776118. doi: 10.1073/pnas.2013776118
16. Symons LK, Miller JE, Kay VR, Marks RM, Liblik K, Koti M, et al. The immunopathophysiology of endometriosis. Trends Mol Med. (2018) 24:748–62. doi: 10.1016/j.molmed.2018.07.004
17. Prather GR, MacLean JA 2nd, Shi M, Boadu DK, Paquet M, Hayashi K. Niclosamide as a potential nonsteroidal therapy for endometriosis that preserves reproductive function in an experimental mouse model. Biol Reprod. (2016) 95:76. doi: 10.1095/biolreprod.116.140236
18. Sekulovski N, Whorton AE, Shi M, MacLean JA II, Hayashi K. Endometriotic inflammatory microenvironment induced by macrophages can be targeted by niclosamidedagger. Biol Reprod. (2019) 100:398–408. doi: 10.1093/biolre/ioy222
19. Sekulovski N, Whorton AE, Tanaka T, Hirota Y, Shi M, MacLean JA, et al. Niclosamide suppresses macrophage induced inflammation in endometriosis. Biol Reprod. (2020) 102:1011–19. doi: 10.1093/biolre/ioaa010
20. Shi M, Sekulovski N, Whorton AE, MacLean JA 2nd, Greaves E, Hayashi K. Efficacy of niclosamide on the intra-abdominal inflammatory environment in endometriosis. FASEB J. (2021) 35:e21584. doi: 10.1096/fj.202002541RRR
21. Zhao L, Shi M, Winuthayanon S, MacLean JA 2nd, Hayashi K. Niclosamide targets the dynamic progression of macrophages for the resolution of endometriosis in a mouse model. Commun Biol. (2022) 5:1225. doi: 10.1038/s42003-022-04211-0
22. Capobianco A, Rovere-Querini P. Endometriosis, a disease of the macrophage. Front Immunol. (2013) 4:9. doi: 10.3389/fimmu.2013.00009
23. Hill JA, Faris HM, Schiff I, Anderson DJ. Characterization of leukocyte subpopulations in the peritoneal fluid of women with endometriosis. Fertility Sterility. (1988) 50:216–22. doi: 10.1016/S0015-0282(16)60062-6
24. Bacci M, Capobianco A, Monno A, Cottone L, Di Puppo F, Camisa B, et al. Macrophages are alternatively activated in patients with endometriosis and required for growth and vascularization of lesions in a mouse model of disease. Am J Pathol. (2009) 175:547–56. doi: 10.2353/ajpath.2009.081011
25. Beste MT, Pfaffle-Doyle N, Prentice EA, Morris SN, Lauffenburger DA, Isaacson KB, et al. Molecular network analysis of endometriosis reveals a role for C-jun-regulated macrophage activation. Sci Trans Med. (2014) 6:222ra16. doi: 10.1126/scitranslmed.3007988
26. Forster R, Sarginson A, Velichkova A, Hogg C, Dorning A, Horne AW, et al. Macrophage-derived insulin-like growth factor-1 is a key neurotrophic and nerve-sensitizing factor in pain associated with endometriosis. FASEB J. (2019) 33:fj201900797R. doi: 10.1096/fj.201900797R
27. Wu J, Xie H, Yao S, Liang Y. Macrophage and nerve interaction in endometriosis. J Neuroinflamm. (2017) 14:53. doi: 10.1186/s12974-017-0828-3
28. Cheong YC, Shelton JB, Laird SM, Richmond M, Kudesia G, Li TC, et al. Il-1, il-6 and tnf-alpha concentrations in the peritoneal fluid of women with pelvic adhesions. Hum Reprod. (2002) 17:69–75. doi: 10.1093/humrep/17.1.69
29. Sekiguchi K, Ito Y, Hattori K, Inoue T, Hosono K, Honda M, et al. Vegf receptor 1-expressing macrophages recruited from bone marrow enhances angiogenesis in endometrial tissues. Sci Rep. (2019) 9:7037. doi: 10.1038/s41598-019-43185-8
30. Wynn TA, Chawla A, Pollard JW. Macrophage biology in development, homeostasis and disease. Nature. (2013) 496:445–55. doi: 10.1038/nature12034
31. Ghosn EE, Cassado AA, Govoni GR, Fukuhara T, Yang Y, Monack DM, et al. Two physically, functionally, and developmentally distinct peritoneal macrophage subsets. Proc Natl Acad Sci U.S.A. (2010) 107:2568–73. doi: 10.1073/pnas.0915000107
32. Yona S, Kim KW, Wolf Y, Mildner A, Varol D, Breker M, et al. Fate mapping reveals origins and dynamics of monocytes and tissue macrophages under homeostasis. Immunity. (2013) 38:79–91. doi: 10.1016/j.immuni.2012.12.001
33. Bain CC, Hawley CA, Garner H, Scott CL, Schridde A, Steers NJ, et al. Long-lived self-renewing bone marrow-derived macrophages displace embryo-derived cells to inhabit adult serous cavities. Nat Commun. (2016) 7:ncomms11852. doi: 10.1038/ncomms11852
34. Liu Z, Gu Y, Chakarov S, Bleriot C, Kwok I, Chen X, et al. Fate mapping via ms4a3-expression history traces monocyte-derived cells. Cell. (2019) 178:1509–25 e19. doi: 10.1016/j.cell.2019.08.009
35. Jenkins SJ, Allen JE. The expanding world of tissue-resident macrophages. Eur J Immunol. (2021) 51:1882–96. doi: 10.1002/eji.202048881
36. Zigmond E, Varol C, Farache J, Elmaliah E, Satpathy AT, Friedlander G, et al. Ly6c hi monocytes in the inflamed colon give rise to proinflammatory effector cells and migratory antigen-presenting cells. Immunity. (2012) 37:1076–90. doi: 10.1016/j.immuni.2012.08.026
37. Louwe PA, Badiola Gomez L, Webster H, Perona-Wright G, Bain CC, Forbes SJ, et al. Recruited macrophages that colonize the post-inflammatory peritoneal niche convert into functionally divergent resident cells. Nat Commun. (2021) 12:1770. doi: 10.1038/s41467-021-21778-0
38. Okabe Y, Medzhitov R. Tissue-specific signals control reversible program of localization and functional polarization of macrophages. Cell. (2014) 157:832–44. doi: 10.1016/j.cell.2014.04.016
39. Rosas M, Davies LC, Giles PJ, Liao CT, Kharfan B, Stone TC, et al. The transcription factor gata6 links tissue macrophage phenotype and proliferative renewal. Science. (2014) 344:645–8. doi: 10.1126/science.1251414
40. Gautier EL, Ivanov S, Williams JW, Huang SC, Marcelin G, Fairfax K, et al. Gata6 regulates aspartoacylase expression in resident peritoneal macrophages and controls their survival. J Exp Med. (2014) 211:1525–31. doi: 10.1084/jem.20140570
41. Ardavin C, Alvarez-Ladron N, Ferriz M, Gutierrez-Gonzalez A, Vega-Perez A. Mouse tissue-resident peritoneal macrophages in homeostasis, repair, infection, and tumor metastasis. Adv Sci (Weinh). (2023) 10:e2206617. doi: 10.1002/advs.202206617
42. Salm L, Shim R, Noskovicova N, Kubes P. Gata6(+) large peritoneal macrophages: an evolutionarily conserved sentinel and effector system for infection and injury. Trends Immunol. (2023) 44:129–45. doi: 10.1016/j.it.2022.12.002
43. Wang J, Kubes P. A reservoir of mature cavity macrophages that can rapidly invade visceral organs to affect tissue repair. Cell. (2016) 165:668–78. doi: 10.1016/j.cell.2016.03.009
44. Honda M, Kadohisa M, Yoshii D, Komohara Y, Hibi T. Directly recruited gata6 + Peritoneal cavity macrophages contribute to the repair of intestinal serosal injury. Nat Commun. (2021) 12:7294. doi: 10.1038/s41467-021-27614-9
45. Zindel J, Peiseler M, Hossain M, Deppermann C, Lee WY, Haenni B, et al. Primordial gata6 macrophages function as extravascular platelets in sterile injury. Science. (2021) 371:eabe0595. doi: 10.1126/science.abe0595
46. Casanova-Acebes M, Menendez-Gutierrez MP, Porcuna J, Alvarez-Errico D, Lavin Y, Garcia A, et al. Rxrs control serous macrophage neonatal expansion and identity and contribute to ovarian cancer progression. Nat Commun. (2020) 11:1655. doi: 10.1038/s41467-020-15371-0
47. Robinson-Smith TM, Isaacsohn I, Mercer CA, Zhou M, Van Rooijen N, Husseinzadeh N, et al. Macrophages mediate inflammation-enhanced metastasis of ovarian tumors in mice. Cancer Res. (2007) 67:5708–16. doi: 10.1158/0008-5472.CAN-06-4375
48. Moughon DL, He H, Schokrpur S, Jiang ZK, Yaqoob M, David J, et al. Macrophage blockade using csf1r inhibitors reverses the vascular leakage underlying Malignant ascites in late-stage epithelial ovarian cancer. Cancer Res. (2015) 75:4742–52. doi: 10.1158/0008-5472.CAN-14-3373
49. Xia H, Li S, Li X, Wang W, Bian Y, Wei S, et al. Autophagic adaptation to oxidative stress alters peritoneal residential macrophage survival and ovarian cancer metastasis. JCI Insight. (2020) 5:e141115. doi: 10.1172/jci.insight.141115
50. Song H, Wang T, Tian L, Bai S, Chen L, Zuo Y, et al. Macrophages on the peritoneum are involved in gastric cancer peritoneal metastasis. J Cancer. (2019) 10:5377–87. doi: 10.7150/jca.31787
51. Hossain M, Shim R, Lee WY, Sharpe AH, Kubes P. Gata6(+) resident peritoneal macrophages promote the growth of liver metastasis. Nat Commun. (2022) 13:4406. doi: 10.1038/s41467-022-32080-y
52. Ruiz-Alcaraz AJ, Carmona-Martinez V, Tristan-Manzano M, MaChado-Linde F, Sanchez-Ferrer ML, Garcia-Penarrubia P, et al. Characterization of human peritoneal monocyte/macrophage subsets in homeostasis: phenotype, gata6, phagocytic/oxidative activities and cytokines expression. Sci Rep. (2018) 8:12794. doi: 10.1038/s41598-018-30787-x
53. Greaves E, Cousins FL, Murray A, Esnal-Zufiaurre A, Fassbender A, Horne AW, et al. A novel mouse model of endometriosis mimics human phenotype and reveals insights into the inflammatory contribution of shed endometrium. Am J Pathol. (2014) 184:1930–9. doi: 10.1016/j.ajpath.2014.03.011
54. Cousins FL, Murray A, Esnal A, Gibson DA, Critchley HO, Saunders PT. Evidence from a mouse model that epithelial cell migration and mesenchymal-epithelial transition contribute to rapid restoration of uterine tissue integrity during menstruation. PloS One. (2014) 9:e86378. doi: 10.1371/journal.pone.0086378
55. Greaves E, Horne AW, Jerina H, Mikolajczak M, Hilferty L, Mitchell R, et al. Ep2 receptor antagonism reduces peripheral and central hyperalgesia in a preclinical mouse model of endometriosis. Sci Rep. (2017) 7:44169. doi: 10.1038/srep44169
56. Herup-Wheeler T, Shi M, Harvey ME, Talwar C, Kommagani R, MacLean JA II, et al. High-fat diets promote peritoneal inflammation and augment endometriosis-associated abdominal hyperalgesia. Front Endocrinol (Lausanne). (2024) 15. doi: 10.1101/2023.11.09.566474
57. Newson J, Stables M, Karra E, Arce-Vargas F, Quezada S, Motwani M, et al. Resolution of acute inflammation bridges the gap between innate and adaptive immunity. Blood. (2014) 124:1748–64. doi: 10.1182/blood-2014-03-562710
58. Davies LC, Rosas M, Jenkins SJ, Liao CT, Scurr MJ, Brombacher F, et al. Distinct bone marrow-derived and tissue-resident macrophage lineages proliferate at key stages during inflammation. Nat Commun. (2013) 4:1886. doi: 10.1038/ncomms2877
59. Davies LC, Rosas M, Smith PJ, Fraser DJ, Jones SA, Taylor PR. A quantifiable proliferative burst of tissue macrophages restores homeostatic macrophage populations after acute inflammation. Eur J Immunol. (2011) 41:2155–64. doi: 10.1002/eji.201141817
60. Gautier EL, Ivanov S, Lesnik P, Randolph GJ. Local apoptosis mediates clearance of macrophages from resolving inflammation in mice. Blood. (2013) 122:2714–22. doi: 10.1182/blood-2013-01-478206
61. Garcia-Gomez E, Vazquez-Martinez ER, Reyes-Mayoral C, Cruz-Orozco OP, Camacho-Arroyo I, Cerbon M. Regulation of inflammation pathways and inflammasome by sex steroid hormones in endometriosis. Front Endocrinol (Lausanne). (2019) 10:935. doi: 10.3389/fendo.2019.00935
62. Harada T, Iwabe T, Terakawa N. Role of cytokines in endometriosis. Fertility Sterility. (2001) 76:1–10. doi: 10.1016/S0015-0282(01)01816-7
63. Machairiotis N, Vasilakaki S, Thomakos N. Inflammatory mediators and pain in endometriosis: A systematic review. Biomedicines. (2021) 9:54. doi: 10.3390/biomedicines9010054
64. Bergqvist A, Bruse C, Carlberg M, Carlstrom K. Interleukin 1beta, interleukin-6, and tumor necrosis factor-alpha in endometriotic tissue and in endometrium. Fertility Sterility. (2001) 75:489–95. doi: 10.1016/S0015-0282(00)01752-0
65. Keenan JA, Chen TT, Chadwell NL, Torry DS, Caudle MR. Il-1 beta, tnf-alpha, and il-2 in peritoneal fluid and macrophage-conditioned media of women with endometriosis. Am J Reprod Immunol. (1995) 34:381–5. doi: 10.1111/j.1600-0897.1995.tb00968.x
66. Malutan AM, Drugan T, Costin N, Ciortea R, Bucuri C, Rada MP, et al. Pro-inflammatory cytokines for evaluation of inflammatory status in endometriosis. Cent Eur J Immunol. (2015) 40:96–102. doi: 10.5114/ceji.2015.50840
67. Montagna P, Capellino S, Villaggio B, Remorgida V, Ragni N, Cutolo M, et al. Peritoneal fluid macrophages in endometriosis: correlation between the expression of estrogen receptors and inflammation. Fertility Sterility. (2008) 90:156–64. doi: 10.1016/j.fertnstert.2006.11.200
68. Arnold J, Barcena de Arellano ML, Ruster C, Vercellino GF, Chiantera V, Schneider A, et al. Imbalance between sympathetic and sensory innervation in peritoneal endometriosis. Brain Behav Immun. (2012) 26:132–41. doi: 10.1016/j.bbi.2011.08.004
69. Ji RR, Xu ZZ, Gao YJ. Emerging targets in neuroinflammation-driven chronic pain. Nat Rev Drug Discovery. (2014) 13:533–48. doi: 10.1038/nrd4334
70. Chuang PC, Wu MH, Shoji Y, Tsai SJ. Downregulation of cd36 results in reduced phagocytic ability of peritoneal macrophages of women with endometriosis. J Pathol. (2009) 219:232–41. doi: 10.1002/path.2588
71. Richter ON, Dorn C, Rosing B, Flaskamp C, Ulrich U. Tumor necrosis factor alpha secretion by peritoneal macrophages in patients with endometriosis. Arch Gynecol Obstet. (2005) 271:143–7. doi: 10.1007/s00404-003-0591-9
72. Bain CC, Gibson DA, Steers NJ, Boufea K, Louwe PA, Doherty C, et al. Rate of replenishment and microenvironment contribute to the sexually dimorphic phenotype and function of peritoneal macrophages. Sci Immunol. (2020) 5:eabc4466. doi: 10.1126/sciimmunol.abc4466
73. Vega-Perez A, Villarrubia LH, Godio C, Gutierrez-Gonzalez A, Feo-Lucas L, Ferriz M, et al. Resident macrophage-dependent immune cell scaffolds drive anti-bacterial defense in the peritoneal cavity. Immunity. (2021) 54:2578–94 e5. doi: 10.1016/j.immuni.2021.10.007
74. Ipseiz N, Pickering RJ, Rosas M, Tyrrell VJ, Davies LC, Orr SJ, et al. Tissue-resident macrophages actively suppress il-1beta release via a reactive prostanoid/il-10 pathway. EMBO J. (2020) 39:e103454. doi: 10.15252/embj.2019103454
75. Yamaguchi T, Fushida S, Yamamoto Y, Tsukada T, Kinoshita J, Oyama K, et al. Tumor-associated macrophages of the M2 phenotype contribute to progression in gastric cancer with peritoneal dissemination. Gastric Cancer. (2016) 19:1052–65. doi: 10.1007/s10120-015-0579-8
Keywords: endometriosis, peritoneal macrophages, GATA6, inflammation, pain
Citation: Shi M, MacLean JA II and Hayashi K (2024) The involvement of peritoneal GATA6+ macrophages in the pathogenesis of endometriosis. Front. Immunol. 15:1396000. doi: 10.3389/fimmu.2024.1396000
Received: 05 March 2024; Accepted: 26 July 2024;
Published: 12 August 2024.
Edited by:
Philippa T. Saunders, University of Edinburgh, United KingdomReviewed by:
Fiona L. Cousins, Hudson Institute of Medical Research, AustraliaJocelyn M. Wessels, McMaster University, Canada
Copyright © 2024 Shi, MacLean and Hayashi. This is an open-access article distributed under the terms of the Creative Commons Attribution License (CC BY). The use, distribution or reproduction in other forums is permitted, provided the original author(s) and the copyright owner(s) are credited and that the original publication in this journal is cited, in accordance with accepted academic practice. No use, distribution or reproduction is permitted which does not comply with these terms.
*Correspondence: Kanako Hayashi, ay5oYXlhc2hpQHdzdS5lZHU=