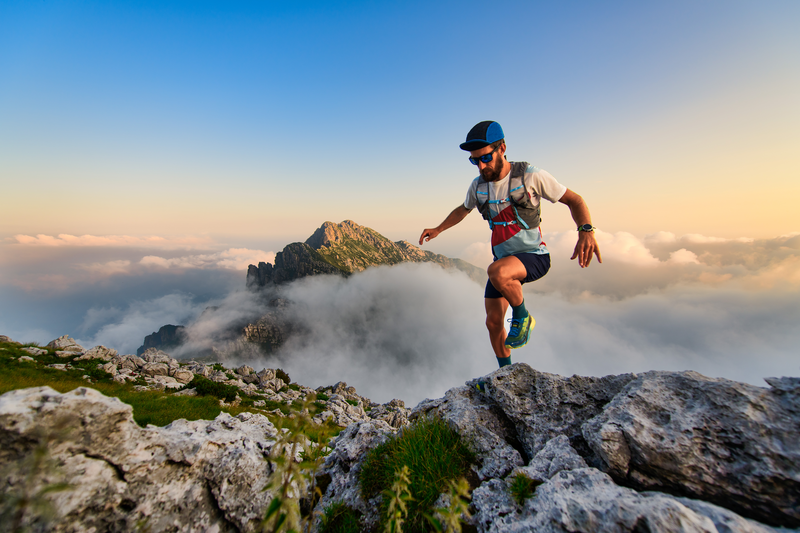
95% of researchers rate our articles as excellent or good
Learn more about the work of our research integrity team to safeguard the quality of each article we publish.
Find out more
REVIEW article
Front. Immunol. , 14 May 2024
Sec. Systems Immunology
Volume 15 - 2024 | https://doi.org/10.3389/fimmu.2024.1394161
Excessive fluoride intake from residential environments may affect multiple tissues and organs; however, the specific pathogenic mechanisms are unclear. Researchers have recently focused on the damaging effects of fluoride on the immune system. Damage to immune function seriously affects the quality of life of fluoride-exposed populations and increases the incidence of infections and malignant tumors. Probing the mechanism of damage to immune function caused by fluoride helps identify effective drugs and methods to prevent and treat fluorosis and improve people’s living standards in fluorosis-affected areas. Here, the recent literature on the effects of fluoride on the immune system is reviewed, and research on fluoride damage to the immune system is summarized in terms of three perspectives: immune organs, immune cells, and immune-active substances. We reviewed that excessive fluoride can damage immune organs, lead to immune cells dysfunction and interfere with the expression of immune-active substances. This review aimed to provide a potential direction for future fluorosis research from the perspective of fluoride-induced immune function impairment. In order to seek the key regulatory indicators of fluoride on immune homeostasis in the future.
Fluorine is a common non-metallic chemical element that in its elemental form is light yellow gas. Fluorine exists widely in nature primarily as fluorite (CaF2), cryolite (Na3AlF6), and fluorapatite (Ca5(PO4)3F). Fluoride is commonly ingested via drinking water, and excessive intake can affect the development and function of various tissues and organs (1, 2). Fluoridation of drinking water has become a prominent public health problem, and China also has unique health issues stemming from coal-burning fluorosis and tea-drinking fluorosis (3–5). Any form of fluorosis is a serious problem, however, as it can not only cause toxic effects, but also result in cell damage and affect the normal physiological activities of the body. A daily intake of more than 2.4 mg for children aged 8–16 years and 3.5 mg for people aged >16 years may lead to fluorosis and adverse health effects (6). In Ethiopia, India, Tanzania, Mexico, China and other countries, the average fluoride concentration in drinking water is approximately 2 mg/L (7–12). Therefore, the World Health Organization has set the maximum fluoride concentration in water to 1.5 mg/L (13). Long-term ingestion of fluoride causes fluorine to accumulate in the body, which can damage bone tissues and the cardiovascular, nervous, reproductive and digestive systems, as well as result in a range of adverse effects on immune system (14, 15). In recent years, more and more researchers have paid attention to the effect of fluoride on the immune system, founding that fluoride has immunotoxicity, although its pathogenesis is remains unclear. A better understanding of the damage to the immune system by fluorosis is essential.
The immune system plays important roles in immune responses and functions. It may recognize and exclude antigenic foreign bodies, coordinate with other body systems, and maintain the stability of the body’s internal environment and physiological balance (16, 17). The immune system is a special distributed system, that is closely connected with other biological systems, and it is the most effective weapon against pathogen invasion (18, 19). The immune system comprises a network of interactions between immune organs, cells, and active substances, including innate immunity, which provides direct host defense, and adaptive immunity, which has precise memory. Many parameters such as environmental factors and lifestyle can cause immune system abnormalities, leading to the occurrence and development of diseases (20, 21). As the damage caused by fluoride to the immune system may be permanent and systemic, an in-depth understanding of the mechanism of damage to the immune system is of immense importance for the prevention and treatment of endemic fluorosis. In recent years, more and more researchers have studied the immune system damage caused by fluoride and its pathogenesis. Here, we reviewed the effects of drinking water fluorosis on immune system and its potential pathogenesis.
We searched through multiple journal databases (PubMed@, CNKI, Google Scholar, etc.) for articles published between 2013 and 2023 in the field of epidemiological investigations and mechanism exploration of fluoride damage to the immune system, and we found that fluoride affects cellular and humoral immunity. Here, the effects of fluoride on immune system in recent years are reviewed in terms of three perspectives: immune organs, immune cells and immune-active substances. This review aimed to provide a potential direction for future fluorosis research from the perspective of fluoride-induced immune function impairment. In light of the non-negligible cumulative damage caused by fluoride to the human body, it is necessary to focus on the changes in tissues and cells at lower doses of fluoride in the future. The results of the authors’ original analysis were used, and no statistical re-analysis was performed.
Bone marrow is the hematopoietic tissue of the human body. Hematopoietic stem cells in the bone marrow may proliferate and differentiate into erythroid, granuloid, lymphoid, mononuclear, and other blood cells and macrophages (22). Platelets exert hemostatic effects, and white blood cells may kill and inhibit various pathogens, including bacteria and viruses. Some lymphocytes specifically recognize antigens and produce antibodies (23). Therefore, bone marrow is a blood-forming and important immune organ. Owing to its strong affinity for bone, most fluoride accumulates in the calcified tissues of adults, resulting in skeletal fluorosis (24). Therefore, fluoride toxicity in human bones has been widely studied (25). However, the immunotoxic mechanism of fluoride exposure in bone injury remains unclear.
Bone marrow mesenchymal stem cells (BMSCs) are widely used as model cells to evaluate bone toxicity after exposure to environmental toxins. They may self-renew, undergo pluripotent differentiation and maintain immune regulation. Multi-omics analysis combining transcriptomics and metabolomics has shown that the influence of excessive fluoride on BMSCs is primarily caused by the alteration of lysosomes, which are severely damaged by fluoride exposure (26). Osteocytes have a strong tolerance to high fluoride concentrations, while osteoclasts are more sensitive to changes of fluoride dose (25). Fluoride can cause chromosome aberration and micronuclei increase in bone marrow cells, resulting in inhibited proliferation of bone marrow cells (27). In addition, fluoride can not only induce osteoblast differentiation, initiate endoplasmic reticulum stress and unfolded protein response, but also induce genotoxicity, oxidative stress and bone mineralization, thereby affecting cell proliferation and producing inflammatory factors (25, 28–30). However, the limited evidence available suggests that low level of fluoride have no immunosuppressive effect on bone marrow and do not cause immune damage. And low levels of NaF can promote soft tissue healing and hard tissue regeneration (31). Analysis at the transcriptomic level showed that human osteosarcoma (HOS) cells stimulated with sublethal concentration of sodium fluoride (8mg/L) for 30 days significantly changed the expression of genes associated with oxidative stress, inflammation, osteoblast differentiation, and bone development pathways. This suggests that chronic oxidative and inflammatory stress may occur in osteoblasts at sublethal fluoride concentrations, affecting the immune function of bone marrow (27, 32–34). These findings provide new insights to study the mechanism of bone marrow immune damage induced by fluoride.
The thymus is an important immune and secretory organ that releases hormones. It is the third line of defense in the human body, providing surveillance and protection and specifically recognizing a variety of pathogens, tumors, antigens, and tissue damage agents. The thymus is the main lymphoid organ involved in T lymphocyte development and regulates T cell development and function. Specific differentiation and generation of T lymphocytes occur in the cortical and medullary regions and are controlled by age (35–37).
Excessive fluoride intake in water may induce immunosuppression, decreasing the number of immune cells and damaging the immune function of the thymus, which can lead to damage via various apoptotic mechanisms and signaling pathways (38). In mature rats, the thymus medulla accounts for 1/3 of the entire lobule, and the ratio of the thymic cortex to the medulla is approximately 2:1 under normal conditions. Fluoride treatment may lead to a decreased ratio of the cortex to the medulla of the thymus, uneven distribution of the cortical layer, irregular ultrastructure and morphology of thymus lymphocytes, and enlargement or cavitation of the mitochondrial crista and apoptotic bodies (39). In addition, fluoride causes enlargement of the thymic nuclear space, condensation, and marginalization of chromatin (40). Moreover, high fluoride levels may decrease the thymus immune index and reduce the number of thymus cells by inhibiting lymphocyte division and promoting cell apoptosis, thereby inhibiting immune function. According to the literature on high fluoride-induced apoptosis, mitochondria-mediated, endoplasmic reticulum (ER)-mediated, and death receptor-mediated pathways are involved (41). However, current studies on the fluoride-induced apoptotic pathways in thymus cells have primarily focused on oxidative stress mediated by the endoplasmic reticulum. Excessive intake of fluoride leads to endoplasmic reticulum stress, which in turn leads to excessive accumulation of the unfolded protein response, activation of RNA activated the protein kinase-like endoplasmic reticulum kinase (PERK), inosin-required protein-1 (IRE1), and activated transcription factor (ATF)-6 signaling pathways. It also triggers eukaryotic initiation factor-2α and tumor necrosis factor (TNF) receptor-associated factor 2 expression. Under continuous external stimulation, ATF4 induces the expression of GADD153, which leads to apoptosis. The immunosuppressant effect of fluoride in the thymus primarily restores cell division reduced by fluoride treatment by inhibiting the PERK and IRE1 signaling pathways, thus inhibiting fluoride-induced apoptosis (42–44).
Fluoride induces the apoptosis of thymus cells and leads to immunosuppression; additionally, high concentrations of fluoride damages thymic epithelial cells (TECs) by altering the expression of T cell function–related genes and the production of immunomodulatory cytokines (45). In TECs, the expression of the Foxn1, Cbx4, DLL4, and IL-7 genes related to T cell development and differentiation are reduced (46). Thus, a decrease in CD4+ and CD8+ thymus T cells was induced. The mRNA levels of the immunoregulatory cytokines interleukin-2 (IL-2) and interleukin-10 (IL-10), which are involved in T cell proliferation, were also decreased, and the expression of the T cell function–related genes CD2, PTPRC, CD69, and CD101 decreased with the action of fluoride. This leads to dysfunction of the thymus gland (47–50). The ingestion of high fluoride levels can hence lead to decreased T cell numbers and T cell dysfunction by reducing the expression of these genes, which may become a new entry point for the study of fluoride-induced immune damage.
The spleen is the largest immune organ in the body, accounting for 25% of the total lymphoid tissue. It contains many lymphocytes and macrophages and is the center of cellular and humoral immunity. The spleen’s main function is to filter blood, which also plays an antitumor role via various mechanisms. Removal of the spleen leads to cellular and humoral immunity disorders (51–53).
The immune damage caused by high fluoride levels primarily manifests as a decline in humoral immune function and immune cell apoptosis, thereby reducing the immune response (50). Through the establishment of Wistar rat model of water-induced fluorosis, fluoride was shown to inhibits splenic cell division in rats, decreases the splenic immune index, promotes apoptosis, and leads to the expansion and vesiculation of the endoplasmic reticulum of splenic lymphocytes, with the presence of a large number of vacuoles between cells. Under fluoride treatment, the nuclear space was evident, the chromatin of lymphocytes in the spleen tissue was marginalized, and the nuclear chromatin coagulated (39, 54). In addition, fluoride has been shown to induce endoplasmic reticulum-mediated oxidative stress, activation of PERK and IRE1 signaling, and associated apoptosis. After PERK and IRE1 knockout, cell division ability was restored and apoptosis was reduced in fluoride-treated lymphocytes. This result correlated well with the expression of PERK and IRE1 signaling–related proteins, confirming the key role of these pathways in excessive fluoride immunosuppression. Therefore, the mechanism of fluoride immune damage in the spleen is related to the activation of the PERK and IRE1 signaling pathways. Inhibition of PERK and IRE1 signaling pathways may reduce cell division and apoptotic damage, and high fluoride levels can affect the development and differentiation of immune cells and induce immune cell apoptosis by activating PERK and IRE1 signaling pathways (42, 55–57).
In addition, fluoride may inhibit the activation of the p38/mitogen-activated protein kinase (MAPK) pathway in mouse splenic lymphocytes. p38/MAPK mRNA is expressed in macrophages, T lymphocytes, and B lymphocytes in mice. Fluoride may reduce the transcription of MLK3, MKK6, and p38/MAPK genes and thus reduce the expression of MKK6 and p38/MAPK proteins, thereby affecting the immune function of lymphocytes. With an increase in fluoride concentration, the expression of MKK6, p38/MAPK, and their protein products decreased, which may have been caused by the disruption of homeostasis by chronic fluorosis. These genes are not phosphorylated properly and exhibit differences in gene expression that directly alter protein expression, thereby reducing pathway activity. The p38/MAPK pathway is activated, and the c-Jun N-terminal kinase (JNK) and extracellular regulated protein kinases (ERK) pathways may also be activated, and high fluoride concentrations have a substantial inhibitory effect on mouse B lymphocytes, reducing the proliferative activity of lymphocytes, indicating that sodium fluoride (NaF) may inhibit the proliferation and differentiation of lymphocytes via the p38/MAPK pathway (58–61).
The damage of fluoride to the spleen is not only manifested in morphology and apoptosis but also leads to an increase in the percentage of G0/G1 phase cells and a decrease in the number of S phase cells in the spleen (62). Cell cycle arrest is the molecular basis for NaF toxicity caused by NaF during spleen development. Moreover, the protein expression levels of IL-2, transforming growth factor-β (TGF-β), TNF-α, interferon-γ (IFN-γ), and cyclin E/D and CDK2/4 were significantly decreased. In contrast, the IL-10 protein expression level was significantly increased. The expression levels of cyclin E/CDK2 and cyclin D/CDK4 proteins were significantly reduced, indicating that NaF treatment slowed the G1 cell cycle progression, blocked the G1/S transition, inhibited splenic lymphocyte proliferation, and damaged DNA synthesis, thereby resulting in cytotoxicity in mice (49, 63–66).
The intestine is an important digestive organ in the human body by playing key roles in food digestion and absorption, water and electrolyte balance, and immune regulation. The intestines can absorb the necessary nutrients in food, regulate the osmotic pressure of plasma crystals, and monitor and eliminate pathogenic bacteria via specific and non-specific immunity to protect the body’s internal environment (67). People living in fluoride-endemic areas often report stomach abnormalities with symptoms including loss of appetite, nausea, anorexia, gas, constipation, and intermittent diarrhea (68). Fluoride is absorbed in the gastrointestinal tract, and numerous studies in laboratory animals and humans have shown that ingestion of NaF may cause gastrointestinal damage (69–71). In addition, long-term excessive intake of fluoride has a certain damaging effect on the intestine, inhibiting the proliferation of epithelial cells (72), leading to increased intestinal permeability, and thus destroying the mechanical and immune barrier function of the intestine (73).
Dietary high fluoride–induced oxidative damage decreased the percentage of T cell subgroups in the cecal tonsil and decreased Immunoglobulin A (IgA), Immunoglobulin G (IgG), and Immunoglobulin M (IgM) levels in the cecal tonsil, which ultimately affected the local mucosal immune function of broilers (71, 74). Morever, ingested fluoride is absorbed from the stomach and intestinal epithelium. Excess fluoride may inhibit intestinal epithelial cell and mast cell proliferation, promote the release of bioactive molecules, stimulate inflammatory cells, activate lymphocytes, reduce the concentrations of immunoglobulins, reduce the ability to resist pathogen infection, and affect the immune function of the intestinal mucosal (69, 75–78). The intestinal barrier is considered to be the most important defense against microbial pathogens that enter the host through the gut (79). Excessive fluoride can stimulate the expression of pro-inflammatory factors, reduce the expression level of tight-junction related genes and proteins, activate inflammatory response, promote cell pyrogen, inhibit intestinal development, and thus cause intestinal inflammation and diarrhea (80). In addition, using high-throughput 16S rRNA gene sequencing technology, it has been found that fungi may be one of the important factors affecting intestinal function, and excessive fluorine can affect the species richness of intestinal fungi in mice, suggesting that fluorine may cause adverse intestinal symptoms by causing an imbalance in the intestinal fungal population, thus affecting the normal intestinal function (81, 82). Moreover, fluoride exposure not only disturbed the compositional balance of the gut microbiome, but also triggered metabolic disorders (83). Alterations in intestinal microbiota and metabolome play a critical role in regulating disease susceptibility and multi-organ damage after excessive fluoride exposure (73). More and more studies have shown that there is a significant difference in the abundance of bacteria in the gut after excessive fluoride intake, and excessive fluoride changes the composition of intestinal microbes in animals (77, 84, 85).
The innate immune system is the body’s second line of defense. The cells involved in innate immune response are primarily phagocytes, including macrophages, neutrophils, and natural killer (NK) cells. The adaptive immune system is activated after the body comes in contact with foreign microorganisms after birth. Adaptive immunity includes cellular and humoral immunity, and the cells involved in the adaptive immune response are primarily lymphocytes, including T lymphocytes and B lymphocytes (86–89).
Macrophages are an important part of the innate immune system, and their activation is indispensable for several aspects of immune defense, inflammatory responses, tissue remodeling, and homeostasis. Macrophages are found in almost all body tissues and are highly heterogeneous. Macrophages function in different physiological states primarily via cell polarization (90).
High fluoride stimulation may affect macrophage polarization, resulting in decreased M2 differentiation and inhibition of osteogenic differentiation and formation (91). Moreover, macrophages exposed to fluoride concentrations ≥50 mg/L (2.6 mM NaF) produce active substances, significantly increasing lipid peroxidation. Even within a short period, it leads to macrophage redox imbalance, resulting in a decline in mitochondrial activity and phagocyte vitality (49). Additionally low fluoride levels can also damage the immune system. Even 1 and 3μM NaF can also affect the expression and activity of 15 lipoxygenases, the number and activity of cyclo-oxygenase, and the activation of phospholipase A2 in human peripheral blood mononuclear cell differentiation mononuclear cells, and promote the biosynthesis of inflammatory mediators, leading to inflammation (92, 93). Macrophages are the main source of reactive oxygen species (ROS) in the human body, participate in the body’s response to various pathogenic factors, and play a key role in inflammation. Under the effect of high fluoride concentrations, the formation of ROS in macrophages increases oxidative stress, thus affecting the expression of inflammatory factors (94).
Neutrophils are effective antibacterial cells, capable of attacking microorganisms within the limited intracellular compartment with powerful antibacterial properties (95). Inappropriate or dysregulated neutrophil activation may damage the host and lead to autoimmune and inflammatory diseases (96).
Due to the harmful effects of fluoride on the bone marrow and hematopoietic organs, fluoride may lower white blood cell, platelet, and neutrophil counts (97). Fluoride stimulation of neutrophils isolated from the tail venous blood of healthy carp showed that NaF significantly increased the release of neutrophil extracellular traps (NET) by regulating the Adenosine 5’-monophosphate (AMP)-activated protein kinase/p38 (AMPK/p38) signaling pathway, suggesting that the toxic effect of fluoride on the immune system of carp may be caused by the release of NET (98). In addition, NaF-induced NET formation in bovine neutrophils is accompanied by increased ROS production and decreased antioxidant enzyme activity, and oxidative stress and NET formation may cause immunotoxicity in neutrophils via the p38/MAPK and ERK pathways (99).
NK cells are lymphocytes of the innate immune system that play an important role in innate and adaptive immune responses to tumors and viral infections. They not only exert cell-mediated cytotoxicity toward tumor and infected cells but also play a regulatory role by secreting cytokines and chemokines to promote or inhibit the functions of other immune cells (100, 101).
NaF has immunosuppressive activity, and fluoride may enhance the in vitro reactivity of human lymphocytes to mitogen or specific antigens. Increased levels of IFN-γ and sIL-2R, which are products of activated T and NK cells, suggest that NaF may primarily act on T and/or NK cells (102). The levels of primary immune response cells, namely CD4 cells, IgG1 cells, and NK cells, may be reduced by different fluoride concentrations. Lipid peroxidation increases significantly, and the antioxidant defense system is damaged (103).
T lymphocytes are derived from pluripotent stem cells in the bone marrow. During the embryonic and neonatal stages, some pluripotent stem cells or pro-T cells in the bone marrow migrate to the thymus and mature into immunoactive T cells under the induction of thymus hormones (104–106). T lymphocytes are divided into two functionally distinct subsets: CD4+ T helper (Th) cells and CD8+ cytotoxic T lymphocytes (CTL). These cells are indirectly involved in clearing infections by regulating the activities of other immune cells. CTL cells induce apoptosis by secreting pro-inflammatory cytokines. Adequate CD4+ and CD8+ T cell activation, proliferation, clonal amplification, and effector functions are essential to clear the infection effectively (107, 108).
Exposure to excessive fluoride reduces the number of lymphocytes, resulting in a decrease in the percentage of CD4+ and CD8+ T cells in the thymus, leading to T cell dysfunction (109). Excessive fluoride intake can alter T-bet and GATA3 protein expression levels in the spleen. T-bet and GATA3 are transcription factors that effectively regulate the development, differentiation, and memory formation of Th1/Th2 cells, disrupting the balance of Th1/Th2 cells (110). Splenic injury is eventually induced by alterations in the expression of Th1/Th2 cell–related cytokines in the splenic microenvironment. In addition, excessive fluoride intake has been found to affect the number of Th17 cells and the expression of related cytokines, leading to changes in the immune system (111–113).
B lymphocytes are derived from the mammalian bone marrow or bird bursa lymphoid stem cells, also called bone marrow- or sac-dependent lymphocytes. They primarily settles in the superficial cortical area of the lymph nodes and lymph nodules of the white pulp of the spleen. In peripheral blood, B cells account for approximately 10%-15% of lymphocytes (113). B cells are the main cells involved in humoral immunity and produce immune effects primarily by secreting antibodies (114–117).
A study using flow cytometry to determine the percentages of CD3+, CD3+CD4+, CD3+CD8+ T, and CD19+ B lymphocytes in the spleens of mice (118). The results showed that the percentage of B lymphocytes was significantly reduced under high fluoride conditions. In addition, a high fluoride diet reduced the number of tonsil B lymphocytes in the cecum and B lymphocytes in the intestinal mucosal of broilers (71, 74, 119). The decrease in the number and activity of B lymphocytes may be caused by the apoptosis of B lymphocytes induced by NaF or the inhibition of lymphocyte proliferation. Some studies have shown that NaF may induce apoptosis and inhibit the proliferation of splenic B lymphocytes both in vivo and in vitro, thus impairing humoral immunity (120).
Immun-active substances are produced by immune cells and other cells involved in immune responses for resisting or preventing infection by microorganisms or parasites or invasion by other organisms. They primarily comprise immunoglobulins, interferons, interleukins, TNFs, and other cytokines.
Immunoglobulins are used to assess the immune system (121). The downregulation of IgA, IgG and IgM is mainly due to the decrease in the number of B lymphocytes and the inhibition of Th2 cytokine expression. Fluoride reduces the number of B lymphocytes, which in turn leads to decreased expression of IgA, IgG, and IgM in serum (122, 123). In conclusion, NaF can reduce lymphocyte proliferation and inhibit humoral immune function.
Cytokines are a class of small molecular proteins that are synthesized and secreted by immune cells and some non-immune cells in response to stimulation and have a wide range of biological activities, including the regulation of innate and adaptive immunity, hematogenesis, cell growth, and repair of damaged tissues. Cytokines include interleukins, interferons, the TNF superfamily, colony-stimulating factors, chemokines, and growth factors. Th1 cells produce IL-2, IFN-γ, and TNF, which are involved in cellular immunity. Th2 cells produce IL-4, IL-6, and IL-10 and participate in humoral immunity. Th17 cells produce pro-inflammatory cytokines such as IL-17, which are important for inducing inflammation (124). NaF may reduce serum Th1 cytokines (IL-2, IFN-γ and TNF) and Th2 cytokines (IL-4, IL-6 and IL-10) and lead to a decrease in the number of CD3+CD4+ T cells (125). It has also been found that Th17 cells primarily produce IL-17 (126). Th17 cells may eliminate pathogens inadequately eliminated by Th1 or Th2 cells. The results showed that IL-17A levels were significantly decreased in the cecum and increased in the rectum after exposure to NaF (127). At the same time, fluoride exposure significantly up-regulated the expression of intestinal IL-1β, IL-23 and IL-22, while IL-6 levels were down-regulated (72). Morever, high fluoride levels may reduce IL-2, IL-4, IL-6, TNF-α, and IFN-γ levels in broilers’ cecal tonsil and intestinal tract (128, 129). High fluoride levels may decrease the percentage of CD4+ cells and the mRNA expression of IL-2 and IL-10 in the thymus (130). In addition, NaF has been reduced the expression of IL-10 mRNA in mouse macrophages, and the decrease in cytokine levels indicates that excessive intake of NaF may inhibit cellular immunity and immune function in mice. In Pakistan, pro-inflammatory cytokines (TNF-α, IL-1β, and IL-6) were increased in people who drank high fluoride-contained water over a long period of time, and there was a significant correlation between fluoride exposure and cholinergase. This may be due to low-grade systemic inflammation through the cholinergic pathway (131). Besides, NaF significantly increases the mRNA and protein levels of MAPK signaling pathways and significantly inhibits the production of pro-inflammatory mediators such as IL-1β, IL-6, IL-8, monocyte chemotactic protein 1, and cyclooxygenase-2 and anti-inflammatory mediators, including IL-4 and TGF-β, thus resulting in the body’s immune dysfunction and inflammatory response (132–134).
An increasing number of researchers have focused on the damage to the immune system caused by endemic fluorosis, and substantial progress has been made in understanding the mechanism of immune system damage. Recent studies have shown that fluoride damages the immune system primarily via oxidative stress pathways, signaling pathways, cell apoptosis, and cell activity. For example, several studies have found that fluoride regulates cell differentiation and development primarily via the oxidative stress pathway when it damages immune organs such as the thymus and spleen, thus leading to cell apoptosis and inhibition of relevant signaling pathways. Therefore, immune system damage warrants further exploration. These findings provide a new direction for studying other fluoride-induced immune dysfunctions and lay the foundation for subsequent studies at the molecular level.
In this review, we found that fluoride affects both cellular and humoral immunity. Fluoride decreases the number and activity of immune cells, causing an imbalance in the Th1/Th2 cells. However, an imbalance in the Th1/Th2 cell ratio may lead to a response from the body’s immune system to its components, leading to autoimmune diseases. In contrast, T lymphocyte subsets in peripheral blood are changed, the number of auxiliary T lymphocytes is reduced and the number of inhibitory T lymphocytes is relatively high, leading to tumor immunosuppression, which affects the occurrence and development of tumors (Figure 1).
In addition to further exploring the molecular mechanisms underlying the relationship between fluoride and immune organs, immune cells, and immune-active substances, future studies on fluoride-induced immune damage should also explore the relevant immune homeostasis, stress response, immunotherapy, and signal transduction pathways regulated by fluoride. Second, multi-omics and epigenetic technologies, such as transcriptomics and proteomics, are used to find a breakthrough conducive to a deeper understanding of whether fluoride-induced body damage affects the occurrence and development of tumors in different genetic backgrounds. Can fluoride be used as an immune adjuvant in the development of autoimmune diseases and cancer? In addition, studies have found that more and more diseases can be treated with immune cells to suppress the immune response, thus reducing the occurrence of diseases. It is estimated that approximately 100 million people worldwide have fluoride levels in their drinking water above the maximum safe level of fluoride specified by the World Health Organization (WHO) regulations (135). Can immune cell therapy treat patients with fluorosis and improve their quality of life? Finally, several studies to date have focused on the irreversible damage to the immune system caused by high fluoride concentrations (Tables 1–3), mostly at concentrations unavailable to humans in nature. The most recent standard for drinking water in China has a limit of 1.2mg/L for fluoride, and different types of fluorosis persist in China (139). The United States requires that the final concentration of fluoride added to bottled water does not exceed 0.7mg/L (140), and Taiwan requires that the concentration of fluoride in drinking water does not exceed 0.8mg/L (141). Considering the non-negligible cumulative damage caused by fluoride to the human body, it is necessary to focus on the changes in tissues and cells at lower doses of fluoride in the future.
SZ: Conceptualization, Formal Analysis, Investigation, Methodology, Validation, Writing – original draft. WW: Funding acquisition, Project administration, Resources, Supervision, Writing – review & editing.
The author(s) declare financial support was received for the research, authorship, and/or publication of this article. The National Natural Science Foundation of China (Nos. 82373699 and 81972984) for supporting this work.
We would like to thank the reviewers for their constructive comments, which are very helpful in revising the review. All the authors have read and approved the final version of the manuscript. We would like to thank Editage (www.editage.cn) for English language editing.
The authors declare that the research was conducted in the absence of any commercial or financial relationships that could be construed as a potential conflict of interest.
All claims expressed in this article are solely those of the authors and do not necessarily represent those of their affiliated organizations, or those of the publisher, the editors and the reviewers. Any product that may be evaluated in this article, or claim that may be made by its manufacturer, is not guaranteed or endorsed by the publisher.
1. Wei W, Pang S, Sun D. The pathogenesis of endemic fluorosis: Research progress in the last 5 years. J Cell Mol Med. (2019) 23:2333–42. doi: 10.1111/jcmm.14185
2. Solanki YS, Agarwal M, Gupta AB, Gupta S, Shukla P. Fluoride occurrences, health problems, detection, and remediation methods for drinking water: A comprehensive review. Sci Total Environ. (2022) 807:150601. doi: 10.1016/j.scitotenv.2021.150601
3. Yang Y, Zhao Q, Liu Y, Liu X, Chu Y, Yan H, et al. FRZB1 rs2242070 polymorphisms is associated with brick tea type skeletal fluorosis in Kazakhs, but not in Tibetans, China. Arch Toxicol. (2018) 92:2217–25. doi: 10.1007/s00204-018-2217-9
4. Lou Q, Guo N, Huang W, Wu L, Su M, Liu Y, et al. Association between bone morphogenetic protein 2 gene polymorphisms and skeletal fluorosis of the brick-tea type fluorosis in Tibetans and Kazakhs, China. Int J Environ Health Res. (2022) 32:1489–99. doi: 10.1080/09603123.2021.1892037
5. Wang J, Yu C, Zhang J, Liu R, Xiao J. Aberrant gut microbiota and fecal metabolites in patients with coal-burning endemic fluorosis in Guizhou, China. Environ Sci pollut Res Int. (2023) 30(27):69913–26. doi: 10.1007/s11356-023-27051-9
6. Saeed M, Malik RN, Kamal A. Fluorosis and cognitive development among children (6-14 years of age) in the endemic areas of the world: a review and critical analysis. Environ Sci pollut Res Int. (2020) 27:2566–79. doi: 10.1007/s11356-019-06938-6
7. Suthar S, Garg VK, Jangir S, Kaur S, Goswami N, Singh S. Fluoride contamination in drinking water in rural habitations of northern Rajasthan, India. Environ Monit Assess. (2008) 145:1–6. doi: 10.1007/s10661-007-0011-x
8. Demelash H, Beyene A, Abebe Z, Melese A. Fluoride concentration in ground water and prevalence of dental fluorosis in Ethiopian Rift Valley: systematic review and meta-analysis. BMC Public Health. (2019) 19:1298. doi: 10.1186/s12889-019-7646-8
9. Alarcón-Herrera MT, Martin-Alarcon DA, Gutiérrez M, Reynoso-Cuevas L, Martín-Domínguez A, Olmos-Márquez MA, et al. Co-occurrence, possible origin, and health-risk assessment of arsenic and fluoride in drinking water sources in Mexico: Geographical data visualization. Sci Total Environ. (2020) 698:134168. doi: 10.1016/j.scitotenv.2019.134168
10. Zhang K, Li X, Zheng X, Qiang C, Zhang X. Human health risk assessment and spatial distribution of fluoride from shallow groundwater in a region of southwest China. J Water supply: Res Technol. (2020) 7/8):69. doi: 10.2166/aqua.2020.060
11. Hu Y, You M, Liu G, Dong Z. Spatial distribution and potential health risk of fluoride in drinking groundwater sources of Huaibei, Anhui Province. Sci Rep. (2021) 11:8371. doi: 10.1038/s41598-021-87699-6
12. Ijumulana J, Ligate F, Irunde R, Bhattacharya P, Ahmad A, Tomašek I, et al. Spatial variability of the sources and distribution of fluoride in groundwater of the Sanya alluvial plain aquifers in northern Tanzania. Sci Total Environ. (2022) 810:152153. doi: 10.1016/j.scitotenv.2021.152153
13. Ling Y, Podgorski J, Sadiq M, Rasheed H, Eqani S, Berg M. Monitoring and prediction of high fluoride concentrations in groundwater in Pakistan. Sci Total Environ. (2022) 839:156058. doi: 10.1016/j.scitotenv.2022.156058
14. Strunecka A, Blaylock RL, Patocka J, Strunecky O. Immunoexcitotoxicity as the central mechanism of etiopathology and treatment of autism spectrum disorders: A possible role of fluoride and aluminum. Surg Neurol Int. (2018) 9:74. doi: 10.4103/sni.sni_407_17
15. Zhou J, Sun D, Wei W. Necessity to pay attention to the effects of low fluoride on human health: an overview of skeletal and non-skeletal damages in epidemiologic investigations and laboratory studies. Biol Trace Elem Res. (2023) 201:1627–38. doi: 10.1007/s12011-022-03302-7
16. Parkin J, Cohen B. An overview of the immune system. Lancet. (2001) 357:1777–89. doi: 10.1016/S0140-6736(00)04904-7
17. Tomar N, De RK. A brief outline of the immune system. Methods Mol Biol. (2014) 1184:3–12. doi: 10.1007/978-1-4939-1115-8_1
18. McComb S, Thiriot A, Akache B, Krishnan L, Stark F. Introduction to the immune system. Methods Mol Biol. (2019) 2024:1–24. doi: 10.1007/978-1-4939-9597-4_1
19. Daëron M. The immune system as a system of relations. Front Immunol. (2022) 13:984678. doi: 10.3389/fimmu.2022.984678
21. Pieren DKJ, Boer MC, de Wit J. The adaptive immune system in early life: The shift makes it count. Front Immunol. (2022) 13:1031924. doi: 10.3389/fimmu.2022.1031924
22. Travlos GS. Normal structure, function, and histology of the bone marrow. Toxicol Pathol. (2006) 34:548–65. doi: 10.1080/01926230600939856
23. Lucas D. Structural organization of the bone marrow and its role in hematopoiesis. Curr Opin Hematol. (2021) 28:36–42. doi: 10.1097/MOH.0000000000000621
24. Srivastava S, Flora SJS. Fluoride in drinking water and skeletal fluorosis: a review of the global impact. Curr Environ Health Rep. (2020) 7:140–46. doi: 10.1007/s40572-020-00270-9
25. Jiang N, Guo F, Sun B, Zhang X, Xu H. Different effects of fluoride exposure on the three major bone cell types. Biol Trace Elem Res. (2020) 193:226–33. doi: 10.1007/s12011-019-01684-9
26. Faruk EM, Alasmari WA, Fouad H, Nafea OE, Hasan RAA. Extracellular vesicles derived from bone marrow mesenchymal stem cells repair functional and structural rat adrenal gland damage induced by fluoride. Life Sci. (2021) 270:119122. doi: 10.1016/j.lfs.2021.119122
27. Sharma A, John P, Bhatnagar P. Fluoride and endosulfan together potentiate cytogenetic effects in Swiss albino mice bone marrow cells. Toxicol Ind Health. (2021) 37:68–76. doi: 10.1177/0748233720979423
28. Huo L, Liu K, Pei J, Yang Y, Ye Y, Liu Y, et al. Fluoride promotes viability and differentiation of osteoblast-like Saos-2 cells via BMP/Smads signaling pathway. Biol Trace Elem Res. (2013) 155:142–9. doi: 10.1007/s12011-013-9770-0
29. Lee M, Arikawa K, Nagahama F. Micromolar levels of sodium fluoride promote osteoblast differentiation through Runx2 signaling. Biol Trace Elem Res. (2017) 178:283–91. doi: 10.1007/s12011-017-0930-5
30. Garcia ALH, Picinini J, Silveira MD, Camassola M, Visentim APV, Salvador M, et al. Fluorosilicic acid induces DNA damage and oxidative stress in bone marrow mesenchymal stem cells. Mutat Res Genet Toxicol Environ Mutagen. (2021) 861-862:503297. doi: 10.1016/j.mrgentox.2020.503297
31. Bhawal UK, Li X, Suzuki M, Taguchi C, Oka S, Arikawa K, et al. Treatment with low-level sodium fluoride on wound healing and the osteogenic differentiation of bone marrow mesenchymal stem cells. Dent Traumatol. (2020) 36:278–84. doi: 10.1111/edt.12532
32. Gandhi D, Naoghare PK, Bafana A, Kannan K, Sivanesan S. Fluoride-induced oxidative and inflammatory stress in osteosarcoma cells: does it affect bone development pathway? Biol Trace Elem Res. (2017) 175:103–11. doi: 10.1007/s12011-016-0756-6
33. Chen YX, Zhu DY, Gao J, Xu ZL, Tao SC, Yin WJ, et al. Diminished membrane recruitment of Akt is instrumental in alcohol-associated osteopenia via the PTEN/Akt/GSK-3β/β-catenin axis. FEBS J. (2019) 286:1101–19. doi: 10.1111/febs.14754
34. Ebrahim FM, Nguyen TN, Shyshkanov S, Gładysiak A, Favre P, Zacharia A, et al. Selective, fast-response, and regenerable metal-organic framework for sampling excess fluoride levels in drinking water. J Am Chem Soc. (2019) 141:3052–58. doi: 10.1021/jacs.8b11907
35. Palmer DB. The effect of age on thymic function. Front Immunol. (2013) 4:316. doi: 10.3389/fimmu.2013.00316
36. Takahama Y, Ohigashi I, Baik S, Anderson G. Generation of diversity in thymic epithelial cells. Nat Rev Immunol. (2017) 17:295–305. doi: 10.1038/nri.2017.12
37. Thapa P, Farber DL. The role of the thymus in the immune response. Thorac Surg Clin. (2019) 29:123–31. doi: 10.1016/j.thorsurg.2018.12.001
38. Wei W, Wang Q, Pang S, Tan S, Sun J, Li M, et al. Excessive fluoride exposure induces thymocyte apoptosis and impairs cell division: Roles of the PERK and IRE1 pathways. Toxicol Lett. (2020) 328:35–44. doi: 10.1016/j.toxlet.2020.04.014
39. Das SS, Maiti R, Ghosh D. Fluoride-induced immunotoxicity in adult male albino rat: a correlative approach to oxidative stress. J Immunotoxicol. (2006) 3:49–55. doi: 10.1080/15476910600631587
40. Wang H, Zhou B, Cao J, Gu X, Cao C, Wang J. Effects of dietary protein and calcium on thymus apoptosis induced by fluoride in female rats (Wistar rats). Environ Toxicol. (2009) 24:218–24. doi: 10.1002/tox.20421
41. Wei Q, Deng H, Cui H, Fang J, Zuo Z, Deng J, et al. A mini review of fluoride-induced apoptotic pathways. Environ Sci pollut Res Int. (2018) 25:33926–35. doi: 10.1007/s11356-018-3406-z
42. Deng H, Kuang P, Cui H, Chen L, Luo Q, Fang J, et al. Sodium fluoride (NaF) induces the splenic apoptosis via endoplasmic reticulum (ER) stress pathway in vivo and in vitro. Aging (Albany NY). (2016) 8:3552–67. doi: 10.18632/aging.101150
43. Ma Y, Zhang K, Ren F, Wang J. Developmental fluoride exposure influenced rat’s splenic development and cell cycle via disruption of the ERK signal pathway. Chemosphere. (2017) 187:173–80. doi: 10.1016/j.chemosphere.2017.08.067
44. Pabst R. The thymus is relevant in the migration of mature lymphocytes. Cell Tissue Res. (2019) 376:19–24. doi: 10.1007/s00441-019-02994-z
45. Yin S, Wu H, Song C, Chen X, Zhang Y. Modulation and the underlying mechanism of T cells in thymus of mice by oral administration of sodium fluoride. Biol Trace Elem Res. (2016) 170:194–200. doi: 10.1007/s12011-015-0458-5
46. Ribeiro AR, Rodrigues PM, Meireles C, Di Santo JP, Alves NL. Thymocyte selection regulates the homeostasis of IL-7-expressing thymic cortical epithelial cells in vivo. J Immunol. (2013) 191:1200–9. doi: 10.4049/jimmunol.1203042
47. Hayball JD, Robinson BW, Lake RA. CD4+ T cells cross-compete for MHC class II-restricted peptide antigen complexes on the surface of antigen presenting cells. Immunol Cell Biol. (2004) 82:103–11. doi: 10.1046/j.0818-9641.2004.01233.x
48. Barbier O, Arreola-Mendoza L, Del Razo LM. Molecular mechanisms of fluoride toxicity. Chem Biol Interact. (2010) 188:319–33. doi: 10.1016/j.cbi.2010.07.011
49. De la Fuente B, Vázquez M, Rocha RA, Devesa V, Vélez D. Effects of sodium fluoride on immune response in murine macrophages. Toxicol In Vitro. (2016) 34:81–7. doi: 10.1016/j.tiv.2016.03.001
50. Guo H, Kuang P, Luo Q, Cui H, Deng H, Liu H, et al. Effects of sodium fluoride on blood cellular and humoral immunity in mice. Oncotarget. (2017) 8:85504–15. doi: 10.18632/oncotarget.v8i49
51. Cesta MF. Normal structure, function, and histology of the spleen. Toxicol Pathol. (2006) 34:455–65. doi: 10.1080/01926230600867743
52. Lewis SM, Williams A, Eisenbarth SC. Structure and function of the immune system in the spleen. Sci Immunol. (2019) 4(33):eaau6085. doi: 10.1126/sciimmunol.aau6085
53. Crane GM, Liu YC, Chadburn A. Spleen: Development, anatomy and reactive lymphoid proliferations. Semin Diagn Pathol. (2021) 38:112–24. doi: 10.1053/j.semdp.2020.06.003
54. Peng X, Cui H, Deng J, Zuo Z, Lai W. Histological lesion of spleen and inhibition of splenocyte proliferation in broilers fed on diets excess in selenium. Biol Trace Elem Res. (2011) 140:66–72. doi: 10.1007/s12011-010-8679-0
55. Deng H, Kuang P, Cui H, Chen L, Fang J, Zuo Z, et al. Sodium fluoride induces apoptosis in cultured splenic lymphocytes from mice. Oncotarget. (2016) 7:67880–900. doi: 10.18632/oncotarget.12081
56. Wei W, Pang S, Fu X, Tan S, Wang Q, Wang S, et al. The role of PERK and IRE1 signaling pathways in excessive fluoride mediated impairment of lymphocytes in rats’ spleen in vivo and in vitro. Chemosphere. (2019) 223:1–11. doi: 10.1016/j.chemosphere.2019.02.031
57. Geng Y, Qiu Y, Liu X, Chen X, Ding Y, Liu S, et al. Sodium fluoride activates ERK and JNK via induction of oxidative stress to promote apoptosis and impairs ovarian function in rats. J Hazard Mater. (2014) 272:75–82. doi: 10.1016/j.jhazmat.2014.03.011
58. Sun Y, Liu WZ, Liu T, Feng X, Yang N, Zhou HF. Signaling pathway of MAPK/ERK in cell proliferation, differentiation, migration, senescence and apoptosis. J Recept Signal Transduct Res. (2015) 35:600–4. doi: 10.3109/10799893.2015.1030412
59. Shi Z, Zhan Y, Zhao J, Wang J, Ma H. Effects of fluoride on the expression of p38MAPK signaling pathway-related genes and proteins in spleen lymphocytes of mice. Biol Trace Elem Res. (2016) 173:333–8. doi: 10.1007/s12011-016-0656-9
60. Zhao L, Su J, Liu S, Li Y, Xi T, Ruan J, et al. MAP kinase phosphatase MKP-1 regulates p-ERK1/2 signaling pathway with fluoride treatment. Biochem Biophys Res Commun. (2021) 542:65–72. doi: 10.1016/j.bbrc.2020.12.100
61. Kuang P, Cui H, Yu L. Sodium fluoride suppresses spleen development through MAPK/ERK signaling pathway in mice. Ecotoxicol Environ Saf. (2022) 241:113764. doi: 10.1016/j.ecoenv.2022.113764
62. Liu J, Wang HW, Zhao WP, Li XT, Lin L, Zhou BH. Induction of pathological changes and impaired expression of cytokines in developing female rat spleen after chronic excess fluoride exposure. Toxicol Ind Health. (2019) 35:43–52. doi: 10.1177/0748233718809773
63. Lozupone CA, Stombaugh JI, Gordon JI, Jansson JK, Knight R. Diversity, stability and resilience of the human gut microbiota. Nature. (2012) 489:220–30. doi: 10.1038/nature11550
64. Liu L, Zhang Y, Gu H, Zhang K, Ma L. Fluorosis induces endoplasmic reticulum stress and apoptosis in osteoblasts in vivo. Biol Trace Elem Res. (2015) 164:64–71. doi: 10.1007/s12011-014-0192-4
65. Clénet ML, Gagnon F, Moratalla AC, Viel EC, Arbour N. Peripheral human CD4(+)CD8(+) T lymphocytes exhibit a memory phenotype and enhanced responses to IL-2, IL-7 and IL-15. Sci Rep. (2017) 7:11612. doi: 10.1038/s41598-017-11926-2
66. Wang H, Zhou B, Niu R, Wang J, Zhang J, Wang J. Analysis of the roles of dietary protein and calcium in fluoride-induced changes in T-lymphocyte subsets in rat. Environ Toxicol. (2017) 32:1587–95. doi: 10.1002/tox.22386
67. Camilleri M, Madsen K, Spiller R, Greenwood-Van Meerveld B, Verne GN. Intestinal barrier function in health and gastrointestinal disease. Neurogastroenterol Motil. (2012) 24:503–12. doi: 10.1111/j.1365-2982.2012.01921.x
68. Job JT, Rajagopal R, Alfarhan A, Ramesh V, Narayanankutty A. Toxic effects of fluoride in intestinal epithelial cells and the mitigating effect of methanol extract of coconut haustorium by enhancing de novo glutathione biosynthesis. Environ Res. (2021) 200:111717. doi: 10.1016/j.envres.2021.111717
69. Follin-Arbelet B, Moum B. Fluoride: a risk factor for inflammatory bowel disease? Scand J Gastroenterol. (2016) 51:1019–24. doi: 10.1080/00365521.2016.1177855
70. Li A, Wang Y, He Y, Liu B, Iqbal M, Mehmood K, et al. Environmental fluoride exposure disrupts the intestinal structure and gut microbial composition in ducks. Chemosphere. (2021) 277:130222. doi: 10.1016/j.chemosphere.2021.130222
71. Liu J, Cui H, Peng X, Fang J, Zuo Z, Deng J, et al. Decreased IgA+ B cells population and IgA, IgG, IgM contents of the cecal tonsil induced by dietary high fluorine in broilers. Int J Environ Res Public Health. (2013) 10:1775–85. doi: 10.3390/ijerph10051775
72. Gao XY, Jin Y, Zhao J, Zhang YL, Wang HW, Zhou BH. Th17-related cytokines involved in fluoride-induced cecal and rectal barrier damage of ovariectomized rats. Biol Trace Elem Res. (2023) 201:4497–507. doi: 10.1007/s12011-022-03519-6
73. Wang Y, Xu J, Chen H, Shu Y, Peng W, Lai C, et al. Effects of prolonged fluoride exposure on innate immunity, intestinal mechanical, and immune barriers in mice. Res Vet Sci. (2023) 164:105019. doi: 10.1016/j.rvsc.2023.105019
74. Miao L, Zhu M, Li H, Xu Q, Dong X, Zou X. Dietary high sodium fluoride impairs digestion and absorption ability, mucosal immunity, and alters cecum microbial community of laying hens. Anim (Basel). (2020) 10(2):179. doi: 10.3390/ani10020179
75. Liu J, Cui H, Peng X, Fang J, Zuo Z, Wang H, et al. Dietary high fluorine induces apoptosis and alters Bcl-2, Bax, and caspase-3 protein expression in the cecal tonsil lymphocytes of broilers. Biol Trace Elem Res. (2013) 152:25–30. doi: 10.1007/s12011-012-9595-2
76. Chauhan SS, Mahmood A, Ojha S. Ethanol and age enhances fluoride toxicity through oxidative stress and mitochondrial dysfunctions in rat intestine. Mol Cell Biochem. (2013) 384:251–62. doi: 10.1007/s11010-013-1804-6
77. Cao Q, Li R, Fu R, Zhang X, Yue B, Wang J, et al. Intestinal fungal dysbiosis in mice induced by fluoride. Chemosphere. (2020) 245:125617. doi: 10.1016/j.chemosphere.2019.125617
78. Hillion S, Arleevskaya MI, Blanco P, Bordron A, Brooks WH, Cesbron JY, et al. The innate part of the adaptive immune system. Clin Rev Allergy Immunol. (2020) 58:151–54. doi: 10.1007/s12016-019-08740-1
79. Garcia-Hernandez V, Quiros M, Nusrat A. Intestinal epithelial claudins: expression and regulation in homeostasis and inflammation. Ann N Y Acad Sci. (2017) 1397:66–79. doi: 10.1111/nyas.13360
80. Luo Q, Cui H, Peng X, Fang J, Zuo Z, Deng J, et al. Suppressive effects of dietary high fluorine on the intestinal development in broilers. Biol Trace Elem Res. (2013) 156:153–65. doi: 10.1007/s12011-013-9845-y
81. Wheeler ML, Limon JJ, Bar AS, Leal CA, Gargus M, Tang J, et al. Immunological consequences of intestinal fungal dysbiosis. Cell Host Microbe. (2016) 19:865–73. doi: 10.1016/j.chom.2016.05.003
82. Iliev ID, Leonardi I. Fungal dysbiosis: immunity and interactions at mucosal barriers. Nat Rev Immunol. (2017) 17:635–46. doi: 10.1038/nri.2017.55
83. Mo Z, Wang J, Meng X, Li A, Li Z, Que W, et al. The dose-response effect of fluoride exposure on the gut microbiome and its functional pathways in rats. Metabolites. (2023) 13:1159. doi: 10.3390/metabo13111159
84. Fu R, Niu R, Li R, Yue B, Zhang X, Cao Q, et al. Fluoride-induced alteration in the diversity and composition of bacterial microbiota in mice colon. Biol Trace Elem Res. (2020) 196:537–44. doi: 10.1007/s12011-019-01942-w
85. Wang HW, Miao CY, Liu J, Zhang Y, Zhu SQ, Zhou BH. Fluoride-induced rectal barrier damage and microflora disorder in mice. Environ Sci pollut Res Int. (2020) 27:7596–607. doi: 10.1007/s11356-019-07201-8
86. Sadighi Akha AA. Aging and the immune system: An overview. J Immunol Methods. (2018) 463:21–6. doi: 10.1016/j.jim.2018.08.005
87. Sun L, Wang X, Saredy J, Yuan Z, Yang X, Wang H. Innate-adaptive immunity interplay and redox regulation in immune response. Redox Biol. (2020) 37:101759. doi: 10.1016/j.redox.2020.101759
88. Kaur BP, Secord E. Innate immunity. Immunol Allergy Clin North Am. (2021) 41:535–41. doi: 10.1016/j.iac.2021.07.003
89. Wang C, Ma C, Gong L, Guo Y, Fu K, Zhang Y, et al. Macrophage polarization and its role in liver disease. Front Immunol. (2021) 12:803037. doi: 10.3389/fimmu.2021.803037
90. Du C, Xiao P, Gao S, Chen S, Chen B, Huang W, et al. High fluoride ingestion impairs bone fracture healing by attenuating M2 macrophage differentiation. Front Bioeng Biotechnol. (2022) 10:791433. doi: 10.3389/fbioe.2022.791433
91. Goschorska M, Gutowska I, Baranowska-Bosiacka I, Piotrowska K, Metryka E, Safranow K, et al. Influence of acetylcholinesterase inhibitors used in alzheimer’s disease treatment on the activity of antioxidant enzymes and the concentration of glutathione in THP-1 macrophages under fluoride-induced oxidative stress. Int J Environ Res Public Health. (2018) 16(1):10. doi: 10.3390/ijerph16010010
92. Gutowska I, Baranowska-Bosiacka I, Safranow K, Jakubowska K, Olszewska M, Telesiński A, et al. Fluoride in low concentration modifies expression and activity of 15 lipoxygenase in human PBMC differentiated monocyte/macrophage. Toxicology. (2012) 295:23–30. doi: 10.1016/j.tox.2012.02.014
93. Gutowska I, Baranowska-Bosiacka I, Siennicka A, Telesiński A, Stańczyk-Dunaj M, Wesołowska T, et al. Activation of phospholipase A(2) by low levels of fluoride in THP1 macrophages via altered Ca(2+) and cAMP concentration. Prostaglandins Leukot Essent Fatty Acids. (2012) 86:99–105. doi: 10.1016/j.plefa.2012.02.002
94. Liew PX, Kubes P. The neutrophil’s role during health and disease. Physiol Rev. (2019) 99(2):1223–48. doi: 10.1152/physrev.00012.2018
95. Burn GL, Foti A, Marsman G, Patel DF, Zychlinsky A. The neutrophil. Immunity. (2021) 54:1377–91. doi: 10.1016/j.immuni.2021.06.006
96. Atmaca N, Yıldırım E, Güner B, Kabakçı R, Bilmen FS. Effect of resveratrol on hematological and biochemical alterations in rats exposed to fluoride. BioMed Res Int. (2014) 2014:698628. doi: 10.1155/2014/698628
97. Song Y, Zhang Y, Zhang P, Yu P, Shang X, Lu Y, et al. Effects of fluorine on neutrophil extracellular trap formation through regulating AMPK/p38 signaling pathway. Oxid Med Cell Longev. (2021) 2021:6693921. doi: 10.1155/2021/6693921
98. Wang JJ, Wei ZK, Han Z, Liu ZY, Zhang Y, Zhu XY, et al. Sodium fluoride exposure triggered the formation of neutrophil extracellular traps. Environ pollut. (2020) 257:113583. doi: 10.1016/j.envpol.2019.113583
99. O’Brien KL, Finlay DK. Immunometabolism and natural killer cell responses. Nat Rev Immunol. (2019) 19:282–90. doi: 10.1038/s41577-019-0139-2
100. Loftenius A, Andersson B, Butler J, Ekstrand J. Fluoride augments the mitogenic and antigenic response of human blood lymphocytes in vitro. Caries Res. (1999) 33:148–55. doi: 10.1159/000016509
101. Zhang C, Tian Z. NK cell subsets in autoimmune diseases. J Autoimmun. (2017) 83:22–30. doi: 10.1016/j.jaut.2017.02.005
102. Reddy YP, Tiwari SK, Shaik AP, Alsaeed A, Sultana A, Reddy PK. Effect of sodium fluoride on neuroimmunological parameters, oxidative stress and antioxidative defenses. Toxicol Mech Methods. (2014) 24:31–6. doi: 10.3109/15376516.2013.843224
103. Braun MY. The natural history of T cell metabolism. Int J Mol Sci. (2021) 22(13):6779. doi: 10.3390/ijms22136779
104. Pearse G. Normal structure, function and histology of the thymus. Toxicol Pathol. (2006) 34:504–14. doi: 10.1080/01926230600865549
105. Rezzani R, Bonomini F, Rodella LF. Histochemical and molecular overview of the thymus as site for T-cells development. Prog Histochem Cytochem. (2008) 43:73–120. doi: 10.1016/j.proghi.2008.03.001
106. Sprent J, Cho JH, Boyman O, Surh CD. T cell homeostasis. Immunol Cell Biol. (2008) 86:312–9. doi: 10.1038/icb.2008.12
107. Mason DW. Subpopulations of T lymphocytes. Immunol Lett. (1987) 14:269–70. doi: 10.1016/0165-2478(87)90002-2
108. Wik JA, Skålhegg BS. T cell metabolism in infection. Front Immunol. (2022) 13. doi: 10.3389/fimmu.2022.840610
109. Li Y, Du X, Zhao Y, Wang J, Wang J. Fluoride can damage the spleen of mice by perturbing th1/th2 cell balance. Biol Trace Elem Res. (2021) 199:1493–500. doi: 10.1007/s12011-020-02264-y
110. Capone A, Volpe E. Transcriptional regulators of T helper 17 cell differentiation in health and autoimmune diseases. Front Immunol. (2020) 11:348. doi: 10.3389/fimmu.2020.00348
111. Johnson MO, Wolf MM, Madden MZ, Andrejeva G, Sugiura A, Contreras DC, et al. Distinct regulation of th17 and th1 cell differentiation by glutaminase-dependent metabolism. Cell. (2018) 175:1780–95.e19. doi: 10.1016/j.cell.2018.10.001
112. Zhao Y, Li Y, Wang J, Manthari RK, Wang J. Fluoride induces apoptosis and autophagy through the IL-17 signaling pathway in mice hepatocytes. Arch Toxicol. (2018) 92:3277–89. doi: 10.1007/s00204-018-2305-x
113. Siu JHY, Pitcher MJ, Tull TJ, Velounias RL, Guesdon W, Montorsi L, et al. Two subsets of human marginal zone B cells resolved by global analysis of lymphoid tissues and blood. Sci Immunol. (2022) 7:eabm9060. doi: 10.1126/sciimmunol.abm9060
114. Wang Y, Liu J, Burrows PD, Wang JY. B cell development and maturation. Adv Exp Med Biol. (2020) 1254:1–22. doi: 10.1007/978-981-15-3532-1
115. Banchereau J, Rousset F. Human B lymphocytes: phenotype, proliferation, and differentiation. Adv Immunol. (1992) 52:125–262. doi: 10.1016/S0065-2776(08)60876-7
116. MacConmara M, Lederer JA. B cells. Crit Care Med. (2005) 33:S514–6. doi: 10.1097/01.CCM.0000190616.15952.4B
117. Seifert M, Küppers R. Human memory B cells. Leukemia. (2016) 30:2283–92. doi: 10.1038/leu.2016.226
118. Ma H, Shi Z, Dong Y, Liang R, Chen J, Wang J, et al. Effect of fluoride on endocytosis and surface marker expression levels of mouse B cells in vitro. Cell Physiol Biochem. (2016) 39:596–603. doi: 10.1159/000445651
119. Deng Y, Cui H, Peng X, Fang J, Zuo Z, Deng J, et al. Effects of high dietary fluorine on erythrocytes and erythrocyte immune adherence function in broiler chickens. Biol Trace Elem Res. (2013) 155:247–52. doi: 10.1007/s12011-013-9793-6
120. Kuang P, Deng H, Cui H, Chen L, Guo H, Fang J, et al. Suppressive effects of sodium fluoride on cultured splenic lymphocyte proliferation in mice. Oncotarget. (2016) 7:61905–15. doi: 10.18632/oncotarget.v7i38
122. Kuang P, Deng H, Cui H, Chen L, Fang J, Zuo Z, et al. Sodium fluoride (NaF) causes toxic effects on splenic development in mice. Oncotarget. (2017) 8:4703–17. doi: 10.18632/oncotarget.v8i3
123. Mehany HM, El-Shafai NM, Attia AM, Ibrahim MM, El-Mehasseb IM. Potential of chitosan nanoparticle/fluoride nanocomposite for reducing the toxicity of fluoride an in-vivo study on the rat heart functions: Hematopoietic and immune systems. Int J Biol Macromol. (2022) 216:251–62. doi: 10.1016/j.ijbiomac.2022.06.171
124. Owen DL, Sjaastad LE, Farrar MA. Regulatory T cell development in the thymus. J Immunol. (2019) 203:2031–41. doi: 10.4049/jimmunol.1900662
125. Miller CN, Proekt I, von Moltke J, Wells KL, Rajpurkar AR, Wang H, et al. Thymic tuft cells promote an IL-4-enriched medulla and shape thymocyte development. Nature. (2018) 559:627–31. doi: 10.1038/s41586-018-0345-2
126. Tabarkiewicz J, Pogoda K, Karczmarczyk A, Pozarowski P, Giannopoulos K. The role of IL-17 and th17 lymphocytes in autoimmune diseases. Arch Immunol Ther Exp (Warsz). (2015) 63:435–49. doi: 10.1007/s00005-015-0344-z
127. Zhao Y, Wang J, Zhang J, Sun Z, Niu R, Manthari RK, et al. Fluoride exposure induces mitochondrial damage and mitophagy via activation of the IL-17A pathway in hepatocytes. Sci Total Environ. (2022) 804:150184. doi: 10.1016/j.scitotenv.2021.150184
128. Chorro L, Suzuki M, Chin SS, Williams TM, Snapp EL, Odagiu L, et al. Interleukin 2 modulates thymic-derived regulatory T cell epigenetic landscape. Nat Commun. (2018) 9:5368. doi: 10.1038/s41467-018-07806-6
129. Ross SH, Cantrell DA. Signaling and function of interleukin-2 in T lymphocytes. Annu Rev Immunol. (2018) 36:411–33. doi: 10.1146/annurev-immunol-042617-053352
130. Zhang B, Sun J, Yuan Y, Ji D, Sun Y, Liu Y, et al. Proximity-enabled covalent binding of IL-2 to IL-2Rα selectively activates regulatory T cells and suppresses autoimmunity. Signal Transduct Target Ther. (2023) 8:28. doi: 10.1038/s41392-022-01208-3
131. Bibi S, Habib R, Shafiq S, Abbas SS, Khan S, Eqani SAMAS, et al. Influence of the chronic groundwater fluoride consumption on cholinergic enzymes, ACHE and BCHE gene SNPs and pro-inflammatory cytokines: A study with Pakistani population groups. Sci Total Environ. (2023) 880:163359. doi: 10.1016/j.scitotenv.2023.163359
132. Karpuzoglu E, Gogal RM Jr., Ansar Ahmed S. Serine protease inhibitor, 4-(2-aminoethyl)-benzene sulfonyl fluoride, impairs IL-12-induced activation of pSTAT4β, NFκB, and select pro-inflammatory mediators from estrogen-treated mice. Immunobiology. (2011) 216:1264–73. doi: 10.1016/j.imbio.2011.07.003
133. Ma Y, Niu R, Sun Z, Wang J, Luo G, Zhang J, et al. Inflammatory responses induced by fluoride and arsenic at toxic concentration in rabbit aorta. Arch Toxicol. (2012) 86:849–56. doi: 10.1007/s00204-012-0803-9
134. Aslan A, Gok O, Beyaz S, Can MI, Parlak G, Gundogdu R, et al. Royal jelly regulates the caspase, Bax and COX-2, TNF-α protein pathways in the fluoride exposed lung damage in rats. Tissue Cell. (2022) 76:101754. doi: 10.1016/j.tice.2022.101754
135. Khatkar R, Nagpal S. Conventional and advanced detection approaches of fluoride in water: a review. Environ Monit Assess. (2023) 195:325. doi: 10.1007/s10661-022-10888-x
136. Manivannan J, Sinha S, Ghosh M, Mukherjee A. Evaluation of multi-endpoint assay to detect genotoxicity and oxidative stress in mice exposed to sodium fluoride. Mutat Res. (2013) 751:59–65. doi: 10.1016/j.mrgentox.2012.11.006
137. Wang Y, Zhao W, Shi J, Wang J, Hao J, Pang X, et al. Intestinal microbiota contributes to altered glucose metabolism in simulated microgravity mouse model. FASEB J. (2019) 33:10140–51. doi: 10.1096/fj.201900238RR
138. Yu Y, Niu R, Zhao F, Zhao Y, Wang J, Wang J, et al. Moderate exercise relieves fluoride-induced liver and kidney inflammatory responses through the IKKβ/NFκB pathway. Environ Sci Pollut Res Int. (2022) 29:78429–43. doi: 10.1007/s11356-022-21360-1
139. Yu X, Chen J, Li Y, Liu H, Hou C, Zeng Q, et al. Threshold effects of moderately excessive fluoride exposure on children's health: A potential association between dental fluorosis and loss of excellent intelligence. Environ Int. (2018) 118:116–24. doi: 10.1016/j.envint.2018.05.042
140. Lin M, Griffin SO, Park S, Li C, Robison V, Espinoza L. Associations between household water fluoridation status and plain tap or bottled water consumption. JDR Clin Trans Res. (2021) 6:440–7. doi: 10.1177/2380084420960419
Keywords: fluoride, inflammatory response, pathogenesis, immune balance, immune organs, immune cells, immun-active substances
Citation: Zhu S and Wei W (2024) Progress in research on the role of fluoride in immune damage. Front. Immunol. 15:1394161. doi: 10.3389/fimmu.2024.1394161
Received: 04 March 2024; Accepted: 29 April 2024;
Published: 14 May 2024.
Edited by:
Yanzhu Zhu, Jilin Agricultural Science and Technology College, ChinaReviewed by:
Bangyuan Wu, China West Normal University, ChinaCopyright © 2024 Zhu and Wei. This is an open-access article distributed under the terms of the Creative Commons Attribution License (CC BY). The use, distribution or reproduction in other forums is permitted, provided the original author(s) and the copyright owner(s) are credited and that the original publication in this journal is cited, in accordance with accepted academic practice. No use, distribution or reproduction is permitted which does not comply with these terms.
*Correspondence: Wei Wei, aHJibXV3d0AxNjMuY29t
Disclaimer: All claims expressed in this article are solely those of the authors and do not necessarily represent those of their affiliated organizations, or those of the publisher, the editors and the reviewers. Any product that may be evaluated in this article or claim that may be made by its manufacturer is not guaranteed or endorsed by the publisher.
Research integrity at Frontiers
Learn more about the work of our research integrity team to safeguard the quality of each article we publish.