- 1Division of Immunology and Allergy, Department of Medicine Solna, and Center for Molecular Medicine, Karolinska Institutet, and Karolinska University Hospital, Stockholm, Sweden
- 2Unit of Integrative Metabolomics, Institute of Environmental Medicine, Karolinska Institutet, Stockholm, Sweden
- 3Department of Respiratory Medicine and Allergy, Karolinska University Hospital, Stockholm, Sweden
- 4Department of Molecular Medicine and Surgery, Karolinska Institutet, Stockholm, Sweden
- 5Department of Cardiothoracic Surgery, Karolinska University Hospital, Stockholm, Sweden
- 6Department of Medical Sciences, Uppsala University, Uppsala, Sweden
Background: Mast cells are critically involved in IgE-mediated diseases, e.g., allergies and asthma. Human mast cells are heterogeneous, and mast cells from different anatomical sites have been shown to respond differently to certain stimuli and drugs. The origin of the mast cells is therefore of importance when setting up a model system, and human lung mast cells are highly relevant cells to study in the context of asthma. We therefore set out to optimize a protocol of IgE-mediated activation of human lung mast cells.
Methods: Human lung mast cells were extracted from lung tissue obtained from patients undergoing pulmonary resection by enzyme digestion and mechanical disruption followed by CD117 magnetic-activated cell sorting (MACS) enrichment. Different culturing media and conditions for the IgE-mediated degranulation were tested to obtain an optimized method.
Results: IgE crosslinking of human lung mast cells cultured in serum-free media gave a stronger response compared to cells cultured with 10% serum. The addition of stem cell factor (SCF) did not enhance the degranulation. However, when the cells were put in fresh serum-free media 30 minutes prior to the addition of anti-IgE antibodies, the cells responded more vigorously. Maximum degranulation was reached 10 minutes after the addition of anti-IgE. Both CD63 and CD164 were identified as stable markers for the detection of degranulated mast cells over time, while the staining with anti-CD107a and avidin started to decline 10 minutes after activation. The levels of CD203c and CD13 did not change in activated cells and therefore cannot be used as degranulation markers of human lung mast cells.
Conclusions: For an optimal degranulation response, human lung mast cells should be cultured and activated in serum-free media. With this method, a very strong and consistent degranulation response with a low donor-to-donor variation is obtained. Therefore, this model is useful for further investigations of IgE-mediated mast cell activation and exploring drugs that target human lung mast cells, for instance, in the context of asthma.
Introduction
Mast cells are granulated cells that play a major role in allergic diseases and asthma. The allergic activation of mast cells occurs by crosslinking of allergen-specific IgE antibodies that are bound to the high-affinity IgE receptor, FcεRI. The mast cells respond to this activation by releasing their pre-formed granule content, including histamine, mast cell proteases (tryptase and chymase carboxypeptidase A3), and heparin, and by de novo synthesizing lipid mediators (prostaglandin D2, cysteine leukotrienes, etc.) and cytokines. These mediators will in turn cause the allergic symptoms, such as smooth muscle cell constriction, mucus production, edema, and itch (1).
Mast cells have been shown to be heterogeneous, and human mast cells are divided into the MCT and MCTC subtypes, depending on their expression of mast cell proteases. MCT expresses tryptase and MCTC, in addition to tryptase, also express chymase, carboxypeptidase A3, and cathepsin G (2–4). The prevalence of these subtypes varies in various tissues, where, for example, mast cells in the skin are predominantly of the MCTC type, and in the lung, they are predominantly of the MCT type (2). Mast cells from the skin have also been shown to express MRGPRX2, a receptor that binds numerous basic compounds, such as compound 48/80, substance P, LL-37, and various drugs, causing degranulation and pseudo-allergic reactions (5–7). Lung mast cells of the MCT type, in contrast, lack the expression of this receptor and therefore do not respond to these stimuli (8, 9). In addition, it is known that mast cells from different tissues have different levels of sensitivity to drugs. For example, lung but not skin mast cells are inhibited by sodium cromoglycate (10). Therefore, the origin of the mast cells is important when setting up a model system and testing the effect of potential activators and drugs on human mast cells. Thus, using mast cells obtained from human lungs is a highly relevant model for studying IgE-mediated degranulation in the context of allergic asthma.
We previously developed a method to extract mast cells from human lung tissue with high yield (11). These human lung mast cells can be used to study degranulation in different contexts and the potential effect of various drugs on the reaction. However, we and others found very high donor-to-donor and experiment-to-experiment variations in the degranulation response, with some donors showing very limited activation while others show a very strong response, and a minor change of the conditions will make a significant difference in terms of activation (12–15). We therefore set out to optimize the conditions in the experiments to obtain a high and stable degranulation response of human lung mast cells.
Materials and methods
Ethical approval
The study was approved by the local ethics committee (Regionala Etikprövningsnämnden Stockholm, 2018/1819–31/1), and all patients gave their informed consent for the collection of lung tissue during pulmonary resection procedures due to cancer. Lung tissue distal to the tumor was utilized.
Cell preparation
Human lung mast cells were obtained from lung tissues as essentially described in a previous study (11). In brief, human lung tissue was cut into small pieces and enzymatically digested for 30 minutes with 0.2 mg/mL DNase I (Sigma-Aldrich, St. Louis, MO, USA) and 0.1 mg/mL Liberase TL (Sigma-Aldrich). Thereafter, the tissue was vigorously cut by scissors and mechanically disrupted by plunging through a syringe. The cells were purified by 30% Percoll centrifugation, and red blood cells were lysed using ACK lysis buffer (Thermo Fisher, Waltham, MA, USA). The cells were then cultured in StemPro™-34 SFM media (Gibco, Grand Island, NY, USA) [StemPro™-34 SFM media include the supplied nutrient supplement and 2 mM l-glutamine (Sigma-Aldrich) unless otherwise stated] overnight, dead cells were thereafter removed by Dead Cell Removal Kit (Miltenyi, Bergisch Gladbach, Germany), and mast cells were enriched by magnetic-activated cell sorting (MACS) enrichment using CD117 MicroBead Kit (Miltenyi). Enriched cells were cultured in StemPro™-34 SFM media with 2 mM l-glutamine or in RPMI 1640 media (HyClone-Cytiva) with 10% fetal bovine serum (FBS; Sigma-Aldrich). Both media were supplemented with 1% penicillin/streptomycin (Sigma) and 100 ng/mL stem cell factor (SCF) (Sobi, Stockholm, Sweden). The cells were cultured for at least 4 days before IgE-crosslinking experiments were performed.
IgE crosslinking
One day prior to crosslinking, 1 μg/mL of human IgE (Myeloma, Calbiochem, San Diego, CA, USA) was added to the media. After washing away unbound IgE, the cells were crosslinked with various concentrations of anti-IgE (clone GE-1, Sigma-Aldrich) for different time periods at 37°C in StemPro™-34 SFM media or PIPES-BSA buffer [0.2% bovine serum albumin (BSA) (Sigma-Aldrich), 9.3 mM PIPES sodium salt (Sigma-Aldrich), 140.0 mM sodium acetate trihydrate, 5.0 mM potassium acetate, 0.6 mM calcium chloride dihydrate, and 0.11 mM magnesium chloride hexahydrate, pH adjusted to 7.4 with 1 M Tris]. After activation, cells were washed with phosphate-buffered saline (PBS) + 2% FBS and thereafter stained with different antibodies and analyzed by flow cytometry.
MRGPRX2-mediated activation
The cells were plated and allowed to pre-incubate in fresh StemPro™-34 SFM media for 30 minutes prior to the addition of 10 µg/mL compound 48/80 (Sigma-Aldrich). After 10 minutes, the cells were washed with PBS + 2% FBS and thereafter stained with antibodies and analyzed by flow cytometry.
Flow cytometry
The following antibodies/stains were used: anti-CD117-APC (clone 104D2, BD Biosciences, San Jose, CA, USA), anti-CD107a-APC-H7 (clone H4A3, BD Biosciences), anti-CD164-PE (clone 67D2, BioLegend, San Diego, CA, USA), anti-CD63-FITC (clone H5C6, BD Biosciences), anti-CD63-PE-Cy7 (clone H5C6, BD Biosciences), anti-CD13-BV421 (clone WM15, BioLegend), anti-CD203c-FITC (clone NP4D6, BioLegend), and Avidin-Alexa Fluor 488 (Thermo Fisher). Staining was performed by incubation for at least 8 minutes at 4°C with the antibodies 1:100 diluted in PBS + 2% FBS, followed by washing with PBS + 2% FBS. The cells were resuspended in PBS + 2% FBS, and DAPI (BD Biosciences) was added to a final dilution of 1:10,000 prior to running the flow cytometry on a BD FACSCanto (BD Biosciences). Live Human lung mast cells (HLMCs) were gated as DAPI negative and CD117 high. Gates for positive degranulation were set according to the anti-CD63 (or other markers when they were used) stained non-activated control. Flow cytometry data analysis was conducted using FlowJo software version 10.8.1 (FlowJo LLC, Ashland, OR, USA).
Statistical analysis
Student’s two-tailed t-test was used to compare differences between the two groups; the Mann–Whitney test was performed when the normality test (α = 0.05) was not passed. One-way ANOVA or two-way ANOVA tests with Bonferroni correction for multiple comparisons were performed when comparing more than two groups. Statistical analyses were performed using GraphPad Prism software version 9.4.1. * p < 0.05; ** p < 0.01; *** p < 0.001; **** p < 0.0001.
Stepwise protocol for optimized human lung mast cell degranulation
Materials
StemPro™-34 SFM media (Gibco) [with the supplied nutrient supplement and 2 mM l-glutamine (Sigma-Aldrich)] or RPMI 1640 media (HyClone-Cytiva)
Cell culture flask (T-25, surface: suspension, filter cap, SARSTEDT, Nümbrecht Germany)
96-well cell culture plate (surface: suspension, bottom shape: conical, SARSTEDT)
SCF (Sobi)
Human IgE, Myeloma (Calbiochem)
Anti-IgE, clone GE-1 (Sigma-Aldrich)
Fluorescence-activated cell sorting (FACS) buffer (PBS + 2% FBS)
Anti-CD63-PE-Cy7 (clone H5C6, BD Biosciences)
Anti-CD117-APC (clone 104D2, BD Biosciences)
DAPI (BD Biosciences)
BD FACSCanto (BD Biosciences or similar flow cytometer)
Stepwise protocol
1. Culture MACS-enriched human lung mast cells with StemPro™-34 SFM media with 100 ng/mL SCF at a concentration of 0.5 × 106 cells/mL in cell culture flasks for at least 4 days.
2. Add 1 μg/mL of human IgE to the media 1 day prior to crosslinking and incubate in the cell incubator for one night.
3. Before IgE crosslinking, pre-heat StemPro™-34 SFM media (or serum-free RPMI) with and without 4 μg/mL anti-IgE antibodies to 37°C.
4. Collect cells from the flask and count the cell number.
5. Spin down the cells at 400 ×g for 5 minutes at room temperature and discard the supernatant.
6. Resuspend the cells to the concentration of 104 cells/mL using pre-heated StemPro™-34 SFM media (or serum-free RPMI media).
7. Add 50 μL of cells per well (5,000 cells per well) into a conical bottom 96-well cell culture plate.
8. Pre-incubate at 37°C for 30 minutes.
9. Add 50 μL pre-heated 4 μg/mL anti-IgE to wells to make the final concentration 2 μg/mL or 50 μL pre-heated media to the control sample.
10. Incubate at 37°C for 10 minutes (optimal degranulation is reached at 10 minutes, but this time can be increased to investigate other effects).
11. Spin down the cells at 400 ×g for 5 minutes at 4°C. Optional: If the supernatant is collected, transfer the supernatant and store it at −20°C for later analysis of released mediators; otherwise, discard the supernatant.
12. Add 200 μL FACS buffer per well, spin down the cells at 400 ×g for 5 minutes at 4°C (maximum break), and discard the supernatant.
13. Resuspend with 50 μL per well 1:100 diluted anti-CD63 and anti-CD117 antibodies in FACS buffer and stain for 8 minutes at 4°C (maximum staining is reached at 8 minutes, but this time can also be increased).
14. Spin down the cells at 400 ×g for 5 minutes at 4°C (maximum break) and resuspend the cell pellet with 100 μL FACS buffer per well.
15. Prior to running the samples on the flow cytometer, add 100 μL per well of DAPI 1:5,000 diluted in FACS buffer.
16. Gate mast cells as live (DAPI low) and CD117 high and set a cutoff for degranulated cells according to the anti-CD63 stained non-stimulated control.
Results
HLMC degranulation is increased when cultured in serum-free StemPro™-34 SFM media
Mast cells were extracted from human lung tissue using enzymatic and mechanical disruption of the tissue followed by CD117 MACS enrichment. The cells failed to degranulate in response to IgE-crosslinking directly after the preparation, they needed to rest for a couple of days to be able to respond (data not shown), they needed to rest for a couple of days to be able to respond. To investigate the effect of different cell culture media on HLMC degranulation, the cells were cultured for 4 days with serum-free StemPro™-34 SFM media or RPMI 1640 media with different FBS batches. Although we optimized the extraction of human lung mast cells from the tissue (11), the obtained number of these primary cells is limited. We therefore used flow cytometry with surface CD63 staining as a surrogate marker for degranulation to be able to assess degranulation at the single cell level and determine the degranulation percentage with relatively few cells utilized. The gating strategies and representative CD63 histograms are shown in Figure 1A. The degranulation peaked at 2 μg/mL anti-IgE antibodies, and cells cultured in StemPro™-34 SFM media had significantly higher degranulation percentages than cells cultured in RPMI 1640 with either of the two FBS batches (Figure 1B). Despite having different degranulation percentages, the high-affinity IgE receptor (FcϵRI) expression was similar in the different culturing conditions (Figure 1C). Because of this, we chose to culture the cells in StemPro™-34 SFM media and use 2 μg/mL anti-IgE antibodies for the rest of the experiments.
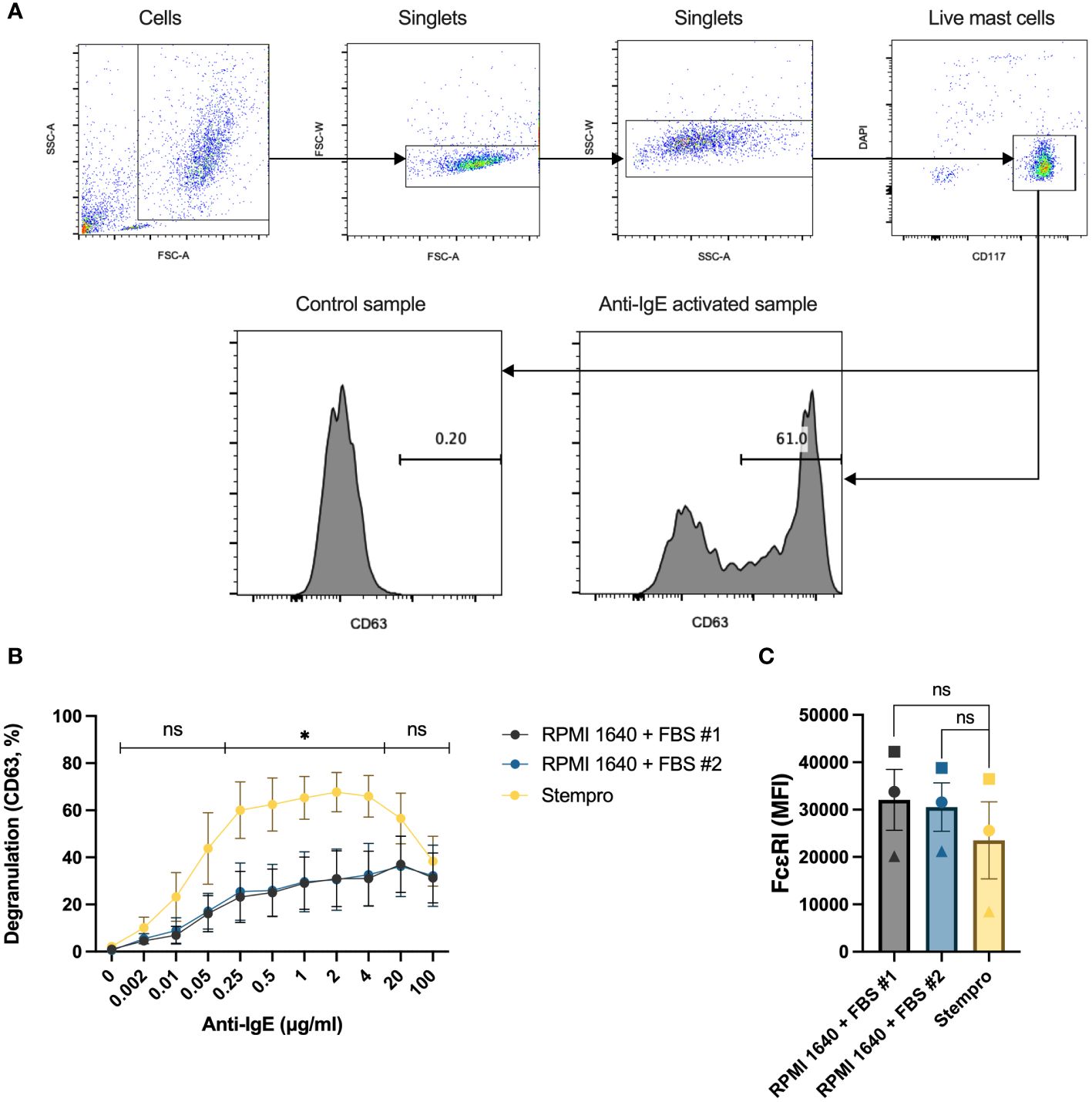
Figure 1 The effect of different culture media on HLMC degranulation. (A) Gating strategies for the degranulation experiments and representative CD63 histograms for control and anti-IgE activated samples. (B) HLMCs were cultured with serum-free StemPro™-34 SFM media and RPMI 1640 media supplemented with two different batches of FBS for 4 days before they were crosslinked with anti-IgE in PIPES-BSA media. Degranulation was evaluated by flow cytometric detection of anti-CD63 staining. n = 5; means with SEMs. (C) The FcϵRI expression [median fluorescence intensity (MFI)] in the different treatment groups was detected by flow cytometry; each individual donor is represented by a unique symbol. n = 3; means with SEMs. FBS, fetal bovine serum. ns, non significant. *p < 0.05.
HLMC degranulation response is higher in StemPro™-34 SFM media than in PIPES-BSA buffer
The media that HLMCs are IgE-crosslinked in, i.e., the degranulation media, also affect their response. We traditionally degranulated mast cells in a PIPES buffer with 2% BSA; however, we have not investigated how this buffer affects mast cell degranulation in time-course experiments where the cells are kept in the degranulation buffer for a longer period. We therefore compared the degranulation percentage when the cells were activated in the PIPES-BSA buffer or in StemPro™-34 SFM media, the media in which the HLMCs were cultured. To make this time course experimentally feasible, the media were changed to the degranulation media, i.e., PIPES-BSA or StemPro™-34 SFM media, the cells were plated, and the anti-IgE antibodies were added at different timepoints so that the endpoint and staining of the cells with anti-CD63 antibodies were performed at the same time (Figure 2A, lower x-axis). This means that the cells had been sitting in the degranulation media for different periods of time prior to the addition of the anti-IgE antibodies (Figure 2A, upper x-axis). At the 120-minute timepoint, when the anti-IgE was added immediately after the media change, there was no difference in degranulation response between the media (Figure 2A). However, at the timepoint of 90 minutes down to 2 minutes of activation, cells crosslinked in StemPro™-34 SFM media had a substantially higher degranulation than those in the PIPES-BSA buffer (Figure 2A). PIPES media only contain basic salts, and since the degranulation response was the highest for this buffer when the anti-IgE was added directly after the media change (120 timepoint), we hypothesized that cells that have been incubated for some time in this media may show lower degranulation. To test this, we performed IgE crosslinking for 10 minutes on cells pre-incubated in PIPES-BSA for 30 minutes or immediately after the media change, and the pre-incubated cells did indeed show lower degranulation (Figure 2B). Thus, the late peak in degranulation in the PIPES buffer is not due to a slow degranulation but rather a lowering in the degranulation response by pre-incubation in this media prior to IgE crosslinking (Figure 2A). The degranulation response reached maximum in the StemPro™-34 SFM media at 10 minutes and was stable up until 90 minutes (Figure 2A). Therefore, henceforth, we used StemPro™-34 SFM media as degranulation media.
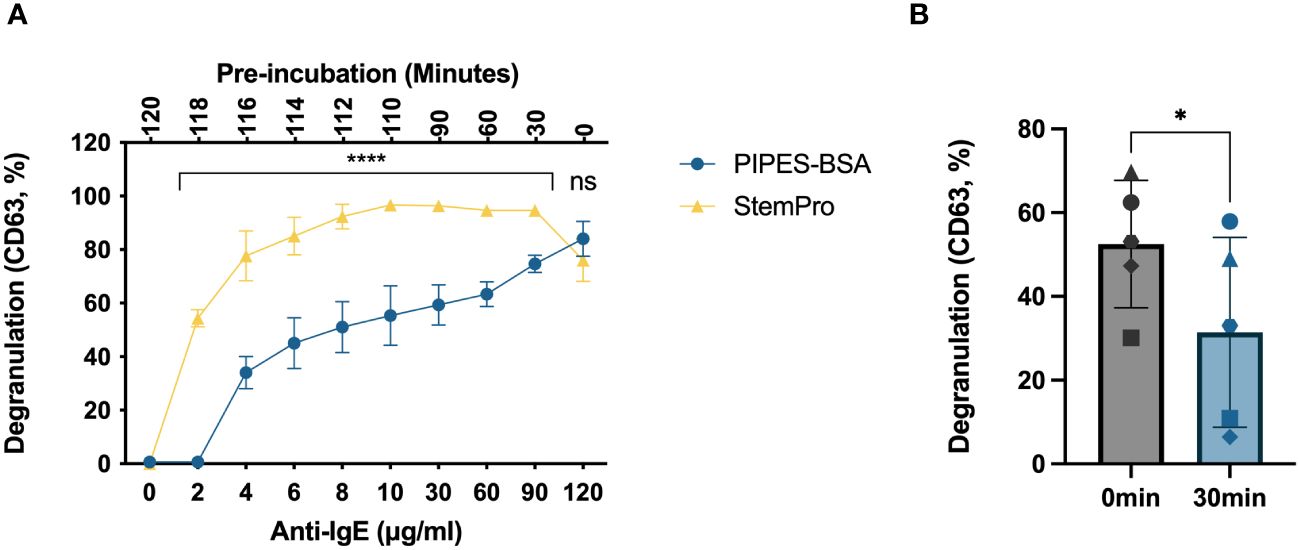
Figure 2 Degranulation time course of HLMCs crosslinked in different buffers. (A) The media were changed to either PIPES-BSA buffer or StemPro™-34 SFM media, the cells were plated on a 96-well plate, and 2 μg/mL anti-IgE was added immediately to the 120-minute timepoint; thereafter, anti-IgE was added at different timepoint to ensure that the cells had been incubated with anti-IgE for the indicated periods (lower x-axis) of time at the endpoint of the experiment. This also leads to the cells being pre-incubated in the media for various timepoints prior to the addition of the anti-IgE (upper x-axis). (B) Anti-IgE was added immediately after the media change or after 30-minute pre-incubation in PIPES-BSA media, and the reaction was stopped after 10 minutes; each individual donor is represented by a unique symbol. Degranulation was evaluated by flow cytometric detection of anti-CD63 staining. n = 3–5; means with SEMs. ns, non significant. *p < 0.05; ****p < 0.0001.
CD63 is a stable marker for HLMC degranulation
In addition to CD63 (16), CD107a, CD164, CD203c, CD13 (17), and fluorochrome-labeled avidin (18, 19) can also be used to evaluate degranulation by flow cytometry. To test the stability of these markers for degranulation over time, HLMCs were stained after being crosslinked with 2 μg/mL anti-IgE at 37°C for different time periods. The surface expression of CD203c and CD13 did not increase in degranulated HLMCs (data not shown). In contrast, CD63, CD107a, CD164, and avidin performed similarly and reached their maximum 10 minutes after the addition of anti-IgE (Figure 3A). These markers also correlated well with each other, staining the same degranulated mast cells at the 10 minutes timepoint (Figures 3B–D). After 10 minutes, avidin and CD107a started to decline, indicating that these markers were not stable over time. In contrast, CD63 and CD164 were stable up until 120 minutes (Figure 3A). We also investigated the optimal staining time for the detection of the different surface markers and found that staining the cells for 8 minutes was enough to reach maximum signal and clear distinction of degranulated and non-degranulated mast cells (Figures 3E–I).
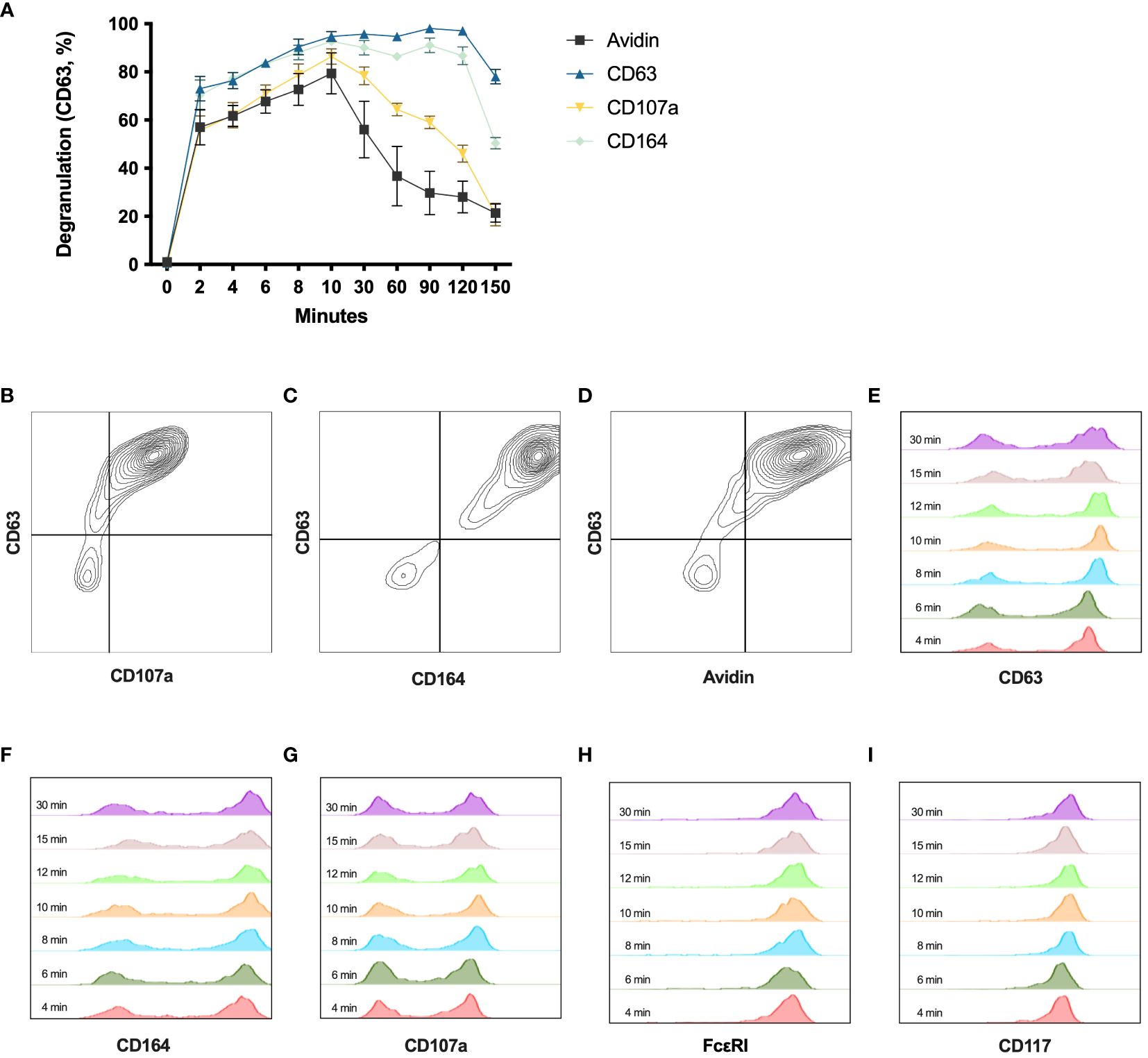
Figure 3 Degranulation time course with different degranulation markers. (A) HLMCs were incubated with 2 μg/mL anti-IgE from 0 to 150 minutes before being stained with avidin, anti-CD63, anti-CD107a, or anti-CD164 and analyzed by flow cytometry. n = 3; means with SEMs. (B–D) Co-staining of indicated markers at 10 minutes after IgE crosslinking is shown. (E–I) Anti-IgE crosslinked HLMCs (10 minutes) were stained with indicated markers for different timepoints (indicated in the figure) on ice before being washed and analyzed on the flow cytometer.
Change of media and addition of SCF have no significant effect on HLMC degranulation
SCF is a pivotal growth factor for human mast cells that they require for their survival. Additionally, it is reported that SCF also enhances mast cell degranulation (15). We therefore next investigated if the degranulation response would differ if it was performed at different times in relation to media change and the addition of SCF. We hence tested the degranulation response on different days after the media change. We replaced the media of the HLMCs every 7–8 days, and the degranulation response was stable throughout this period (Figure 4A); therefore, it does not matter when degranulation experiments are performed in relation to media change. We also cultured HLMCs in different SCF concentrations (10 ng/mL and 100 ng/mL) in StemPro™-34 SFM media for 1 day and found no significant difference between the two groups (Figure 4B).
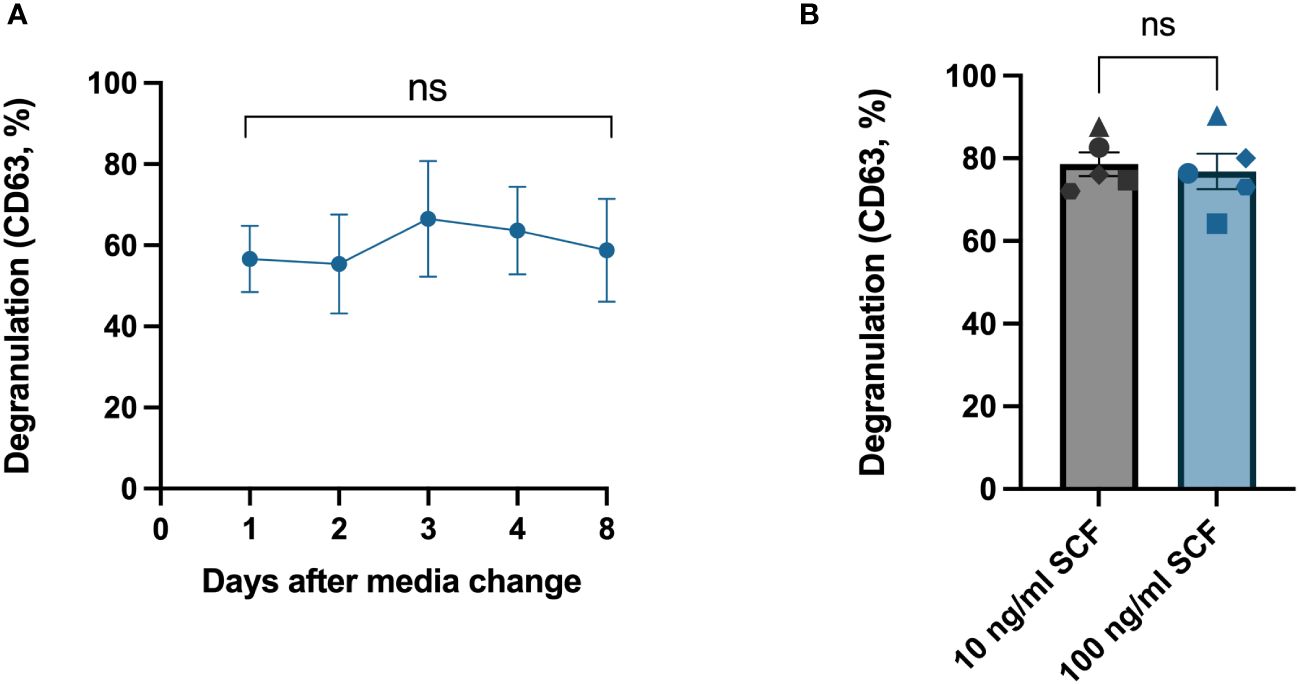
Figure 4 Change of media and addition of fresh SCF had no significant effect on HLMC degranulation. (A) IgE crosslinking was performed on different days after media change to fresh StemPro™-34 SFM media and 100 ng/mL SCF. n = 4; means with SEMs. (B) HLMCs were cultured in StemPro™-34 SFM media with different concentrations of SCF for 1 day before IgE crosslinking; each individual donor is represented by a unique symbol. n = 5; means with SEMs. Degranulation was evaluated by flow cytometric detection of anti-CD63 staining. SCF, stem cell factor. ns, non significant.
Pre-incubation in fresh StemPro™-34 SFM media before crosslinking significantly increases HLMC degranulation
We noticed in our time course experiments that the degranulation response dropped at the last timepoint (Figures 2A, 3A). Since in the latest timepoint the anti-IgE antibodies were added immediately after the media change while in the other timepoints the cells had been incubated in the media for various timepoints (Figure 2A, upper x-axis), we decided to test whether pre-incubating in fresh media could have an impact on the response. We found that the degranulation response increased significantly when the cells were allowed to be pre-incubated in fresh StemPro™-34 SFM media for 30 minutes before activation, compared to cells activated immediately after the media change (Figure 5A). In other words, giving the cells fresh StemPro™-34 SFM media primed HLMCs for degranulation. To exclude that the presence of SCF in the StemPro™-34 SFM media was the cause of the effect, we compared StemPro™-34 SFM media with or without SCF, and with or without the nutrient supplement that was supplied with StemPro™-34 SFM media (and normally always added to the StemPro™-34 SFM media), and the results were comparable (Figure 5A). We also tested to pre-incubate the cells in RPMI media (without serum added) for 30 minutes prior to degranulation, and also in this case, the response was increased to a similar extent as in StemPro™-34 SFM media. However, when cells were pre-incubated in RPMI media with 10% serum, the degranulation was significantly reduced (Figure 5B).
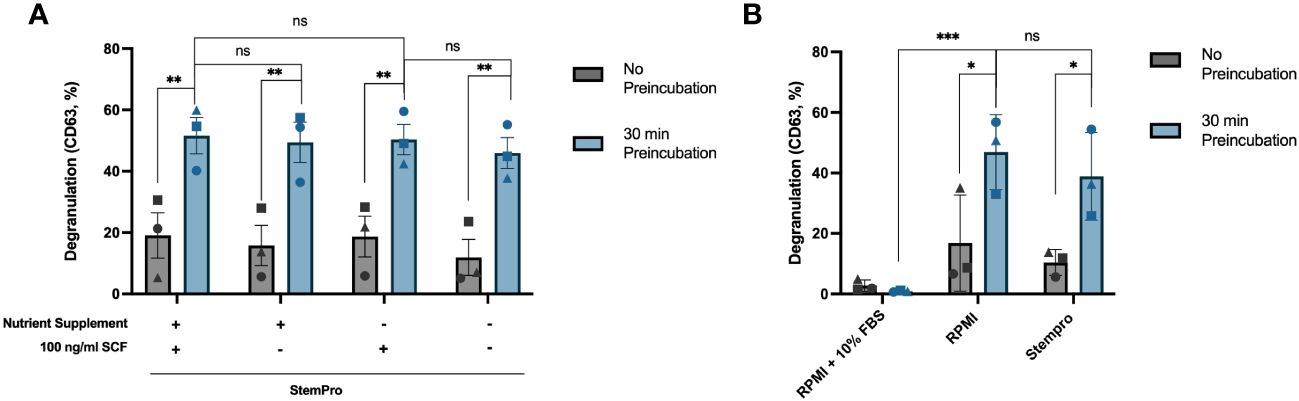
Figure 5 Pre-incubation in fresh StemPro™-34 SFM media increases HLMC degranulation. (A) HLMCs were pre-incubated in fresh StemPro™-34 SFM media with or without SCF/nutrient supplement for 30 minutes at 37°C before IgE crosslinking, or the degranulation was performed immediately after the media change (no pre-incubation). (B) HLMCs were pre-incubated in different media, RPMI 1640 media with 10% serum, RPMI alone, or StemPro™-34 SFM media (with nutrient supplement), for 30 minutes at 37°C before IgE crosslinking, or the degranulation was performed immediately after the media change (no pre-incubation). Degranulation was evaluated by flow cytometric detection of anti-CD63 staining. Each individual donor is represented by a unique symbol, n = 3; means with SEMs. SCF, stem cell factor; RPMI, RPMI-1640 media. ns, non significant. *p < 0.05; **p < 0.01; *** p < 0.001.
Human lung mast cells do not respond to the MRGPRX2 agonist compound 48/80
It has been published that HLMCs do not express the MRGPRX2 receptor and that they do not degranulate upon exposure to MRGPRX2 agonists (8, 9). We tested whether this is also true using our optimized culturing/degranulation method with MRGPRX2 agonist compound 48/80. In line with previously published results, we found that compound 48/80 does not induce significant degranulation in HLMCs, while the mast cell line LAD-2, in contrast, readily degranulates upon exposure to compound 48/80 (Figure 6).
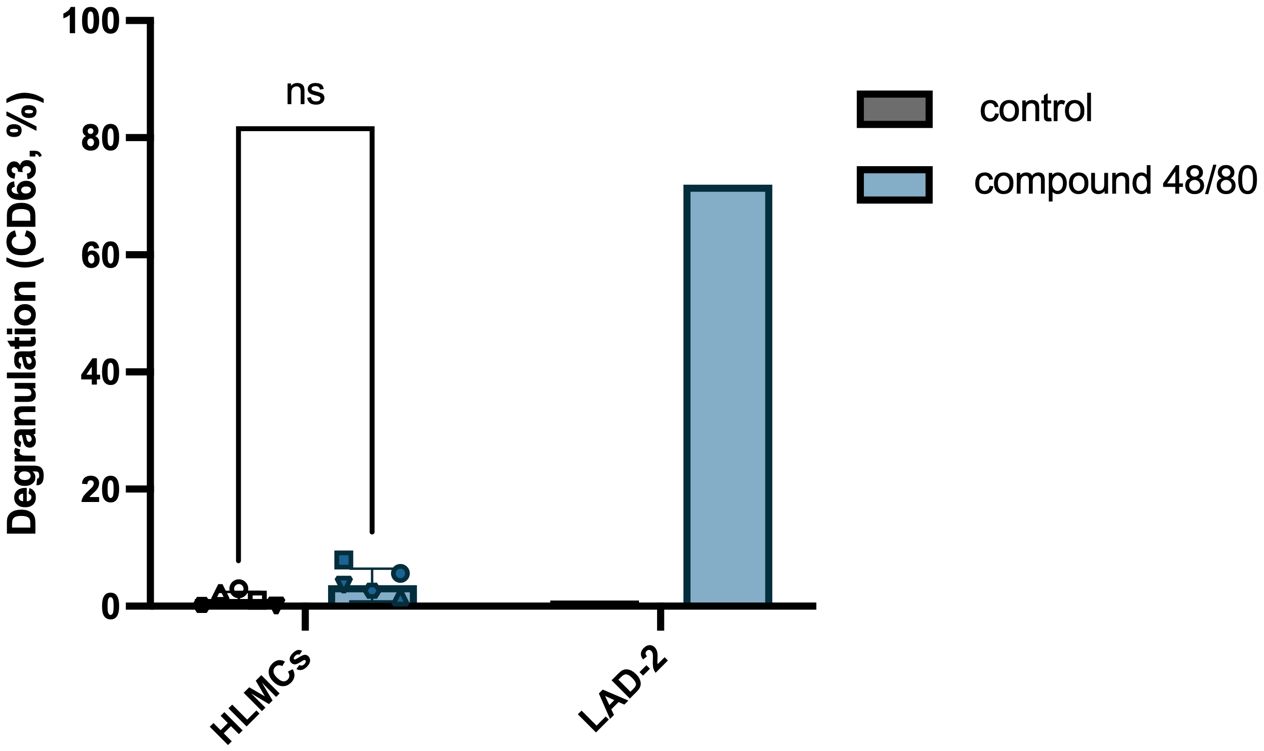
Figure 6 HLMCs do not degranulate in response to MRGPRX2 agonist compound 48/80. HLMCs were pre-incubated in fresh StemPro™-34 SFM media for 30 minutes prior to addition of 10 µg/mL compound 48/80. LAD-2 cells were used as positive control for MRGPRX2-induced degranulation. Degranulation was evaluated by flow cytometric detection of anti-CD63 staining. Each individual donor is represented by a unique symbol, n = 6; means with SEMs. ns, non significant.
Discussion
Because of a high variation in the IgE-mediated degranulation response that we have observed in HLMCs, we set out to optimize the conditions to obtain a higher, more stable, and reproducible response by the IgE crosslinking of the HLMCs.
HLMCs fail to degranulate when the IgE crosslinking is performed directly after extraction of the cells from the lung tissue (data not shown). The extraction and purification protocols are very harsh on the cells, and it is likely that the cells are activated in this process. This could be a reason why they cannot degranulate in response to the IgE crosslinking; however, it is not possible to control for this since we need to extract the cells to perform the experiment. Regardless of the reason, the cells need to rest for a few days before they can respond to IgE crosslinking by degranulation. We found that culturing the HLMCs in a serum-free media results in increased IgE-mediated degranulation compared to cells cultured in RPMI with 10% serum. There was no statistical difference in the surface expression of the FcεRI receptor; thus, the receptor expression is not likely to be the cause of the lower response.
SCF has been reported to prime mast cells and increase the degranulation of the cells upon IgE crosslinking (20), also specifically in HLMCs (15). In our experiments, SCF did not influence the degree of degranulation (Figures 4A, B, 5A). The rationale for this inconsistency remains speculative. One possibility is that the level of degranulation is already maximized under our optimized conditions, leaving no scope for any additional priming effect of SCF.
We found that merely changing the media of the cells, i.e., placing them in fresh StemPro™-34 SFM 30 minutes before activation, increased the rate of degranulation (Figure 5A), which suggests that there may be some ingredients in StemPro™-34 SFM media that prime mast cells for degranulation, or there is an inhibitory factor in the culture media that is removed. StemPro™-34 SFM media are a trademark serum-free media, consisting of a basal liquid medium and a frozen nutrient supplement. Initially, we hypothesized that the nutrient supplement contained ingredients that primed the mast cells; however, using only the basal liquid media without the supplement had the same priming effect (Figure 5A). In addition, we found that pre-incubating the cells in fresh RPMI media without serum added also had the same priming effect (Figure 5B). Pre-incubation in serum-containing RPMI 1640 media in contrast significantly reduced degranulation, suggesting inhibitory factor(s) present in the serum. It has previously been published that a factor in serum can inhibit mast cell degranulation (21); however, the identity of this factor is unknown.
Several different markers have been used to identify degranulated cells, including CD63, CD13, CD203c, CD107a, CD164, and avidin (which binds electrostatically to the exteriorized proteoglycans) (17–19). To measure the dynamics of these degranulation markers over time on the HLMCs, we set up a time course experiment, staining the mast cells at different timepoints after the IgE crosslinking. CD203c and CD13 staining did not increase in degranulated HLMCs (data not shown), while the staining for all the other markers increased and reached maximum 10 minutes after the activation (Figure 3A). Furthermore, the surface expression of the different markers also correlated strongly with each other (Figures 3B–D), showing that the markers identify the same degranulated mast cells. CD63 and CD164 were quite stable over time, while the CD107a and avidin staining started to drop earlier (Figure 3A). This is in contrast to a study on basophils where the CD203c, CD164, and CD13 reached maximum staining within 5–15 minutes, while CD63 and CD107a reached maximum after only 20–40 minutes (17), indicating that the degranulation process somewhat differs in basophils and mast cells. In summary, the CD63 staining was the marker that showed the highest degranulation percentage with a clear distinction between degranulated and non-degranulated cells, and it was stable over time; therefore, we believe that this is a useful marker for studying the degranulation of HLMCs.
In summary, our optimized method for degranulation of HLMCs consists of culturing the HLMCs in serum-free StemPro™-34 SFM media and pre-incubating the cells for 30 minutes in fresh StemPro™-34 SFM media (or serum-free RPMI) prior to activation by 2 μg/mL anti-IgE. This leads to peak degranulation at 10 minutes, which can be measured through flow cytometric analysis of CD63.
This optimized method consistently yields a high and stable activation percentage, proving valuable for the analysis of IgE-mediated activation in HLMCs and for assessing the impact of drugs targeting HLMCs in the context of lung diseases, such as asthma.
Data availability statement
The original contributions presented in the study are included in the article/supplementary material. Further inquiries can be directed to the corresponding author.
Ethics statement
The studies involving humans were approved by Regionala etikprövningsnämnden i Stockholm. The studies were conducted in accordance with the local legislation and institutional requirements. The participants provided their written informed consent to participate in this study.
Author contributions
YG: Conceptualization, Data curation, Formal analysis, Investigation, Methodology, Software, Validation, Visualization, Writing – original draft, Writing – review & editing. AJ: Investigation, Methodology, Resources, Writing – review & editing. JS: Funding acquisition, Project administration, Resources, Writing – review & editing. ES: Resources, Writing – review & editing. MA: Resources, Writing – review & editing. KV: Resources, Writing – review & editing. GN: Conceptualization, Funding acquisition, Project administration, Supervision, Writing – review & editing. ER: Conceptualization, Data curation, Formal analysis, Funding acquisition, Investigation, Methodology, Project administration, Resources, Software, Supervision, Validation, Visualization, Writing – original draft, Writing – review & editing.
Funding
The author(s) declare financial support was received for the research, authorship, and/or publication of this article. This study was supported by grants from the Swedish Research Council, the Heart-Lung Foundation, The Swedish Cancer Society, the Ellen, Walter and Lennart Hesselman Foundation, the Åke Wiberg Foundation, The Konsul ThC Berghs Foundation, The Tornspiran Foundation, The Magnus Bergvall Foundation, and the Karolinska Institutet. The funding sources were not involved in the study design, collection, and interpretation of data; writing the report; or the decision to submit the article for publication.
Conflict of interest
The authors declare that the research was conducted in the absence of any commercial or financial relationships that could be construed as a potential conflict of interest.
Publisher’s note
All claims expressed in this article are solely those of the authors and do not necessarily represent those of their affiliated organizations, or those of the publisher, the editors and the reviewers. Any product that may be evaluated in this article, or claim that may be made by its manufacturer, is not guaranteed or endorsed by the publisher.
References
1. Dahlin JS, Maurer M, Metcalfe DD, Pejler G, Sagi-Eisenberg R, Nilsson G. The ingenious mast cell: Contemporary insights into mast cell behavior and function. Allergy. (2022) 77:83–99. doi: 10.1111/all.14881
2. Irani AA, Schechter NM, Craig SS, DeBlois G, Schwartz LB. Two types of human mast cells that have distinct neutral protease compositions. Proc Natl Acad Sci U S A. (1986) 83:4464–8. doi: 10.1073/pnas.83.12.4464
3. Irani AM, Goldstein SM, Wintroub BU, Bradford T, Schwartz LB. Human mast cell carboxypeptidase. Selective localization to MCTC cells. J Immunol. (1991) 147:247–53. doi: 10.4049/jimmunol.147.1.247
4. Schechter NM, Irani AM, Sprows JL, Abernethy J, Wintroub B, Schwartz LB. Identification of a cathepsin G-like proteinase in the MCTC type of human mast cell. J Immunol. (1990) 145:2652–61. doi: 10.4049/jimmunol.145.8.2652
5. Tatemoto K, Nozaki Y, Tsuda R, Konno S, Tomura K, Furuno M, et al. Immunoglobulin E-independent activation of mast cell is mediated by Mrg receptors. Biochem Biophys Res Commun. (2006) 349:1322–8. doi: 10.1016/j.bbrc.2006.08.177
6. McNeil BD, Pundir P, Meeker S, Han L, Undem BJ, Kulka M, et al. Identification of a mast-cell-specific receptor crucial for pseudo-allergic drug reactions. Nature. (2015) 519:237–41. doi: 10.1038/nature14022
7. Subramanian H, Gupta K, Guo Q, Price R, Ali H. Mas-related gene X2 (MrgX2) is a novel G protein-coupled receptor for the antimicrobial peptide LL-37 in human mast cells: resistance to receptor phosphorylation, desensitization, and internalization. J Biol Chem. (2011) 286:44739–49. doi: 10.1074/jbc.M111.277152
8. Fujisawa D, Kashiwakura J, Kita H, Kikukawa Y, Fujitani Y, Sasaki-Sakamoto T, et al. Expression of Mas-related gene X2 on mast cells is upregulated in the skin of patients with severe chronic urticaria. J Allergy Clin Immunol. (2014) 134:622–33.e629. doi: 10.1016/j.jaci.2014.05.004
9. Kajiwara N, Sasaki T, Bradding P, Cruse G, Sagara H, Ohmori K, et al. Activation of human mast cells through the platelet-activating factor receptor. J Allergy Clin Immunol. (2010) 125:1137–45.e1136. doi: 10.1016/j.jaci.2010.01.056
10. Okayama Y, Benyon RC, Rees PH, Lowman MA, Hillier K, Church MK. Inhibition profiles of sodium cromoglycate and nedocromil sodium on mediator release from mast cells of human skin, lung, tonsil, adenoid and intestine. Clin Exp Allergy. (1992) 22:401–9. doi: 10.1111/j.1365-2222.1992.tb03102.x
11. Ravindran A, Ronnberg E, Dahlin JS, Mazzurana L, Safholm J, Orre AC, et al. An optimized protocol for the isolation and functional analysis of human lung mast cells. Front Immunol. (2018) 9:2193. doi: 10.3389/fimmu.2018.02193
12. Havard S, Scola AM, Kay LJ, Ishmael SS, MacGlashan DW Jr., Peachell PT. Characterization of syk expression in human lung mast cells: relationship with function. Clin Exp Allergy. (2011) 41:378–88. doi: 10.1111/cea.2011.41.issue-3
13. Duffy SM, Lawley WJ, Conley EC, Bradding P. Resting and activation-dependent ion channels in human mast cells. J Immunol. (2001) 167:4261–70. doi: 10.4049/jimmunol.167.8.4261
14. Lavens SE, Proud D, Warner JA. A sensitive colorimetric assay for the release of tryptase from human lung mast cells. vitro J Immunol Methods. (1993) 166:93–102. doi: 10.1016/0022-1759(93)90332-2
15. Bischoff SC, Dahinden CA. c-kit ligand: a unique potentiator of mediator release by human lung mast cells. J Exp Med. (1992) 175:237–44. doi: 10.1084/jem.175.1.237
16. Schafer T, Starkl P, Allard C, Wolf RM, Schweighoffer T. A granular variant of CD63 is a regulator of repeated human mast cell degranulation. Allergy. (2010) 65:1242–55. doi: 10.1111/j.1398-9995.2010.02350.x
17. Hennersdorf F, Florian S, Jakob A, Baumgartner K, Sonneck K, Nordheim A, et al. Identification of CD13, CD107a, and CD164 as novel basophil-activation markers and dissection of two response patterns in time kinetics of IgE-dependent upregulation. Cell Res. (2005) 15:325–35. doi: 10.1038/sj.cr.7290301
18. Bergstresser PR, Tigelaar RE, Tharp MD. Conjugated avidin identifies cutaneous rodent and human mast cells. J Invest Dermatol. (1984) 83:214–8. doi: 10.1111/1523-1747.ep12263584
19. Joulia R, L'Faqihi FE, Valitutti S, Espinosa E. IL-33 fine tunes mast cell degranulation and chemokine production at the single-cell level. J Allergy Clin Immunol. (2017) 140:497–509.e410. doi: 10.1016/j.jaci.2016.09.049
20. Halova I, et al. Changing the threshold-Signals and mechanisms of mast cell priming. Immunol Rev. (2018) 282:73–86. doi: 10.1111/imr.12625
Keywords: human lung mast cells, IgE-mediated degranulation, FcεRI, degranulation, MRGPRX2
Citation: Gong Y, Johnsson A-K, Säfholm J, Al-Ameri M, Sachs E, Vali K, Nilsson G and Rönnberg E (2024) An optimized method for IgE-mediated degranulation of human lung mast cells. Front. Immunol. 15:1393802. doi: 10.3389/fimmu.2024.1393802
Received: 29 February 2024; Accepted: 10 May 2024;
Published: 31 May 2024.
Edited by:
Glenn Cruse, North Carolina State University, United StatesReviewed by:
Graham Mackay, The University of Melbourne, AustraliaMargarita Martin, University of Barcelona, Spain
Copyright © 2024 Gong, Johnsson, Säfholm, Al-Ameri, Sachs, Vali, Nilsson and Rönnberg. This is an open-access article distributed under the terms of the Creative Commons Attribution License (CC BY). The use, distribution or reproduction in other forums is permitted, provided the original author(s) and the copyright owner(s) are credited and that the original publication in this journal is cited, in accordance with accepted academic practice. No use, distribution or reproduction is permitted which does not comply with these terms.
*Correspondence: Elin Rönnberg, ZWxpbi5yb25uYmVyZy5ob2NrZXJsaW5kQGtpLnNl