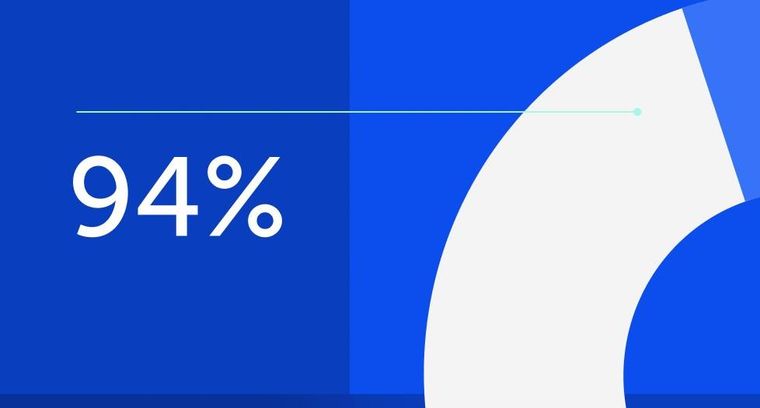
94% of researchers rate our articles as excellent or good
Learn more about the work of our research integrity team to safeguard the quality of each article we publish.
Find out more
BRIEF RESEARCH REPORT article
Front. Immunol., 03 May 2024
Sec. Multiple Sclerosis and Neuroimmunology
Volume 15 - 2024 | https://doi.org/10.3389/fimmu.2024.1391949
Dimethyl fumarate (DMF, Tecfidera) is an oral drug utilized to treat relapsing-remitting multiple sclerosis (MS). DMF treatment reduces disease activity in MS. Gastrointestinal discomfort is a common adverse effect of the treatment with DMF. This study aimed to investigate the effect of DMF administration in the gut draining lymph nodes cells of C57BL6/J female mice with experimental autoimmune encephalomyelitis (EAE), an animal model of MS. We have demonstrated that the treatment with DMF (7.5 mg/kg) significantly reduces the severity of EAE. This reduction of the severity is accompanied by the increase of both proinflammatory and anti-inflammatory mechanisms at the beginning of the treatment. As the treatment progressed, we observed an increasing number of regulatory Foxp3 negative CD4 T cells (Tr1), and anti-inflammatory cytokines such as IL-27, as well as the reduction of PGE2 level in the mesenteric lymph nodes of mice with EAE. We provide evidence that DMF induces a gradual anti-inflammatory response in the gut draining lymph nodes, which might contribute to the reduction of both intestinal discomfort and the inflammatory response of EAE. These findings indicate that the gut is the first microenvironment of action of DMF, which may contribute to its effects of reducing disease severity in MS patients.
Dimethyl fumarate (DMF, TECFIDERA, Biogen - USA) was approved as a first-line oral therapy for relapsing-remitting multiple sclerosis (MS). Its efficacy arises from neuroprotective and immunomodulatory effects, alongside a favorable benefit-risk profile. DMF’s neuroprotective benefits are primarily attributed to the activation of the Nrf2 antioxidant pathway in central nervous system (CNS) cells (1) and glutathione recycling (2). Additionally, its immunomodulatory properties are associated with the activation of the HCAR2/GPR109 pathway in microglia (3, 4) and the inhibition of the pro-inflammatory transcription factor NF-kB (5, 6).
Lymphopenia, flushing, and gastrointestinal events are the most common adverse effects of the treatment with DMF. DMF is converted into its main metabolite, monomethyl fumarate (MMF), by esterases from intestinal epithelial cells (7). The intestinal discomfort observed by oral administration of DMF seems to be the result of an inflammatory response induced by the treatment. These symptoms appear at the beginning and are reduced during treatment (8, 9), suggesting that an anti-inflammatory response is also activated by the DMF.
An important property of the cells of the intestinal mucosa is to prevent an exaggerated immune response, such as allergies or inflammation, to orally acquired antigens. The mesenteric lymph nodes are constantly draining immune cells from the gastrointestinal tract, either in a steady state or under inflammatory conditions, resulting in the induction of tolerance or immune cell activation. In the experimental autoimmune encephalomyelitis (EAE), the animal model for MS, the induction of regulatory T cells was demonstrated in classical experiments of adoptive cells transfer. The transfer of mesenteric lymph node cells and Peyer’s patch cells from rodents tolerized to myelin basic protein (MBP) protected against actively induced EAE (10–12). Here, we provide evidence that treatment with DMF gradually activated the expression of both regulatory T cells and IL-27 production in the mesenteric lymph nodes of mice with EAE.
Wild-type C57BL/6J female mice, 8 weeks old, were used in the experiments. They were obtained at the Multidisciplinary Center for Biological Research in the Area of Science in Laboratory Animals (CEMIB – UNICAMP), and they were housed in a specific pathogen-free facility of the Institute of Biology (UNICAMP). The temperature was kept between 21-23°C, and a standard light-dark cycle of 12 h was applied. The animals received sterile drinking water and food (Nuvilab - Quimtia) ad libitum. No animal was excluded during experiments. Mice were littermates and were randomly allocated into cohorts. Mice health was monitored daily. The study followed the updated recommendations of the National Council for the Control of Animal Experimentation (CONCEA), and it was approved by the UNICAMP Animal Ethics Committee (CEUA/UNICAMP), with registration number 4884-1/2018.
We used the neuroantigen myelin oligodendrocyte glycoprotein 35-55 peptide (MOG35-55) (N-MEVGWYRSPFSRVVHLYRNGK-C) (Genemed Synthesis, Tx, USA) for immunization. The mice were immunized blindly with 100 µg MOG35-55 diluted in 50 µL of phosphate-buffered saline (PBS) emulsified in equal volume with Complete Freund’s Adjuvant (CFA) (Sigma-Aldrich, F5881, MO, USA), which was supplemented with 4 mg/mL of inactivated Mycobacterium tuberculosis (MT, H37RA) (BD Difco, DF3114-33-8, USA). The emulsion was administered to the axillary region of each mouse subcutaneously. Immediately after immunization, and two days later, 200 ng of Pertussis toxin (Sigma-Aldrich, P7208, MO, USA) were injected intraperitoneally per animal. We monitored the clinical signs of EAE daily after the seventh day of immunization. The evolution of the disease was graded blindly as follows: score 0 = not sick; score 1 = loss of tail tone; score 2 = loss of movement of both hind legs, paraplegia; score 3 = paraplegia, loss of abdominal muscles, abdominal weight loss; score 4 = quadriplegia; score 5 = death. Mice at score 4 were closely monitored and a humane endpoint was applied before score 5 was reached. EAE onset was the first day a mouse showed clinical symptoms. Cumulative score corresponded to the sum of scores starting from onset until the last day of observation divided by the number of mice in the group.
We grounded DMF crystals (Sigma-Aldrich, 242926, MO, USA) and mixed them in PBS prepared with sterile tap water to obtain a dose of 7.5 mg/kg. We considered the standard mass of the mice as 20 g. We prepared fresh doses every day and mixed them vigorously before each administration. Mice with EAE were divided into groups receiving 100 µL of the vehicle (PBS) or DMF every 12 h, by gavage, starting from the third day post immunization (d.p.i.). For EAE monitoring, mice were treated daily until the 20th d.p.i. In other experiments, mice were treated daily for either 5, 10, or 15 days, corresponding to the days 7, 12, and 17 d.p.i., respectively. Tissues were collected in the morning after the final gavage for each time point.
For spinal cord histology on the 21st d.p.i., animals were anesthetized and euthanized, and the spinal cord was removed and immersed in 4% PFA for 48h. The tissues were transferred to a solution of 70% ethanol, and then they were sent to paraffin embedding, and hematoxylin and eosin (HE) staining at Histocell, SP, BR. Additionally, the longitudinal sections of the spinal cord (4 µm thick) were stained with Luxol fast blue-Cresyl violet to visualize myelin. Cellular infiltrates in the HE-stained samples were quantified using ImageJ 2.14.0. The white matter area was delineated, and infiltrates within were identified. Five sections were analyzed for each mouse.
We removed aseptically all mesenteric lymph nodes (mLN), small intestine and colonic mLN, and grounded them against a 70-µm cell strainer to obtain a cell suspension rich in leukocytes.
To obtain the total RNA from mLN cells, we used the Trizol (Invitrogen, 15596018, USA) extraction method, according to the manufacturer’s instructions. The purity and concentration of the nucleic acids were analyzed using nanodrop equipment (Thermo Scientific 2000). We converted the extracted mRNA to cDNA using the High-Capacity cDNA conversion kit (Applied Biosystems, 4368814, USA), also according to the manufacturer’s instructions. For each reaction, an amount of 1 µg of total RNA was added. The cDNA conversion was performed in a thermocycler (Eppendorf AG 6321 BK016651) with the following incubation steps: 25°C for 10 min, 37°C for 2 h, 85°C for 5 min, ending at 4°C.
For analyzing most cytokines and transcription factor expression, we applied the Taqman technique with the LuminoCt® qPCR ReadyMix™ (Sigma-Aldrich, L6669, USA). Briefly, we mixed the diluted (1:20) cDNA with the manufacturer’s master mix in duplicate. We added the following primers for the transcript’s amplification (all from Applied Biosystems, USA): Foxp3 Mm00475162_m1, Tgfb1 Mm01178820_m1, Tnf Mm00443258_m1, Il10 Mm01288386_m1, Ifng Mm01168134_m1, Il-12a Mm01208555_m1, Il17a Mm00439618_m1, and Il27 Mm00461164_m1. As a reference, we used Beta-2-Microglobulin (B2m) Mm00437762_m1. For the expression of IL-6, we used the SyBR Green chemistry with the LuminoCt® SYBR® Green qPCR ReadyMix™ (Sigma-Aldrich, L6544, USA). Primer sequences were Il6 (Forward CACGGCCTTCCCTACTTCAC; Reverse TGCAAGTGCATCATCGTTGT) and B2m (Forward ACAGTTCCACCCGCCTCACATT; Reverse TAGAAAGACCAGTCCTTG CTGAAG), (Exxtend, BR). The expression level was calculated as 2-ΔCt and the data were normalized by the mean expression of the control group.
To analyze cytokine production, the mLN cells were incubated with 25 ng/mL Phorbol 12-myristate 13-acetate (PMA), 1 µg/mL Ionomycin, and 5 µg/mL Brefeldin A in RPMI 1640 medium supplemented with 5% of Fetal Bovine Serum (FBS) during 4 h, at 37°C. Then, the cells were stained with extracellular markers, for 30 min, in PBS. For intracellular staining, a permeabilization and fixation buffer (Invitrogen, 00-5523-00, USA) was used. We incubated the cells with the intracellular markers in the permeabilization buffer overnight. The samples were washed, and the data were acquired and analyzed in a BD FACSymphony A5 flow cytometer (BD Bioscience, USA). We analyzed the cytometry data in the FlowJo software. Extracellular antibodies: CD3 PE (555275, BD), CD4 PerCP Cy 5.5 (116012, Biolegend), CD8a PE Cy 7 (100722, Biolegend), CD11c APC Cy 7 (561241, BD), CD11b BV421 (560625, BD), XCR1 APC (148206, Biolegend), CD103 PerCP Cy 5.5 (563637BD). Intracellular antibodies: Foxp3 APC (17577382, eBioscience), IL-10 BV650 (564083, BD), TGFbeta 1,2,3 Alexa 700 (IC1835N, R&D Systems).
The mLN cells (1 x 106 cells/mL) were cultured in RPMI 1640 medium supplemented with 10% FBS and stimulated with lipopolysaccharide (LPS), 0.1 µg/mL, for 24h. The levels of PGE2 in the cell culture supernatant were quantified by ELISA assay (R&D Systems, SKGE004B, USA) according to manufacturer instructions. We quantified the T cells’ cytokines of the undiluted cell culture supernatants using the BD™ CBA Mouse Th1/Th2/Th17 Cytokine Kit (BD, 560485, USA). The acquisition was performed in a BD FACSCalibur flow cytometer (BD Bioscience, USA).
Statistical analyses were performed using non-parametric tests in the GraphPad Prism 9 software. Outliers detected by the Grubbs method (alfa = 0.05) were excluded. The Kruskal-Wallis test was applied to compare three or more groups, and the Mann-Whitney test was used to compare pairs. Differences were considered significant with a P-value < 0.05. The n was determined according to the formula n= 1 + [2C*(s/d)2], for which we considered P < 0.05 (90% power and significance level 0.05 – C value is 10.51). Considering the maximum deviation of 20% and the difference between groups of 50%.
Dimethyl fumarate was administrated orally every 12h at 7.5 mg/kg, and PBS was used as a vehicle, following the literature (1, 13–15). Figure 1A shows the evolution of clinical signs of EAE for 20 days. The mean onsets of EAE were similar in both the Control (11 ± 1.5) and DMF (11 ± 1.7) groups. The control group had 100% incidence of symptoms, with a maximum score of 3.3 ± 1.2 and a cumulative score of 20.1 per mouse, whereas the DMF group showed a 62.5% of EAE incidence, with a maximum score of 1.1 ± 1.2 and a cumulative score of 8.4 per mouse. These results demonstrate a significant reduction in the severity of EAE in mice receiving 7.5 mg/kg DMF. Additionally, we compared cellular infiltration in the spinal cords of DMF-treated mice with EAE symptoms to those in the EAE control group, observing a trend towards decreased inflammatory infiltration in DMF-treated mice (Figure 1B). It should be noted, however, that only the most severely affected mice were selected for HE staining, which could introduce bias to our results by potentially masking a significant reduction in CNS inflammation. A previous study has reported decreased inflammatory infiltration and reduced demyelination with DMF treatment (14). After confirming the protective effects in EAE, we subsequently treated EAE mice with 7.5 mg/kg DMF or the vehicle for 5, 10, or 15 days and analyzed the immune response in the mesenteric lymph nodes (mLN) (Figure 1C).
Figure 1 Clinical evolution of EAE in C57BL/6J mice treated with dimethyl fumarate. (A) Evolution of EAE after DMF treatment. Representative results out of three experiments are shown. EAE control: without treatment (n = 3); DMF: dimethyl fumarate at a dose of 7.5 mg/kg (n = 8). (B) Longitudinal sections of the spinal cord of treated (n=3) and untreated EAE mice (n=3) were stained with HE and the number of inflammatory foci/mm2 of white matter in the tissue was counted. Samples were collected 21 days after the immunization. (A, B) Data represented as mean and SEM of each group. The Mann-Whitney test was applied for comparison between the EAE control group and DMF treated group. P < 0.05 was considered significant. ** P < 0.01. (C) Experimental design for the analysis of mesenteric lymph nodes cells (mLN). Group 5d: mice treated daily for 5 days; Group 10d: mice treated daily for 10 days; Group 15d: mice treated daily for 15 days. All mLN were collected the day after the treatment concluded. D.p.i: days post-immunization, ns: nonsignificant.
Flushing and intestinal discomfort are important side effects of DMF treatment. Previous studies demonstrated that the binding of MMF to HCAR2/GPR109A on Langerhans cells promotes the production of Prostaglandin E2 (PGE2), which may explain, at least in part, the flushing effects in the skin (16). The production of PGE2 by macrophages/dendritic cells plays an important role in the pathogenesis of EAE, mainly in the regulation of CD4 Th17 cells (17, 18).
Here, we show an increase in the CD11c+ cells from the mLN (Figure 2A) and in the release of PGE2 by mLN cells on the 10th day of treatment with DMF (Figure 2B). As the treatment and the disease progress (15th), the production of PGE2 in treated mice keeps steady, while there is a significant increase in PGE2 production by mLN cells of EAE mice without treatment. It should be noted that the 17th day of post-immunization (here the 15th day of treatment) usually corresponds to the peak of EAE, when autoreactive T lymphocytes are circulating throughout the body of mice, leading to systemic inflammation (19).
Figure 2 Pro-inflammatory mechanisms are activated in the mesenteric lymph nodes of C57BL/6J mice with EAE at the beginning of treatment with dimethyl fumarate. (A) The mesenteric lymph node (mLN) dendritic cells (CD11c+) were stained for flow cytometry. Conventional dendritic cells (cDCs) CD11c+ XCR1- were analyzed for the expression of CD103 and CD11b. (B, H) The mLN cells from EAE mice treated or not with 7.5 mg/kg DMF were cultured in RPMI 1640 medium supplemented with 10% FBS and stimulated with lipopolysaccharide (LPS), 0.1 µg/mL, for 24h. (B) PGE2 was measured in cell culture supernatant by an ELISA assay. (C) CD103-CD11b+ cDCs. (D–G) The expression of pro-inflammatory cytokines was measured in the freshly isolated mLN cells by qPCR, having B2m as the housekeeping gene. (D) Tnf mRNA. (E) Il12a mRNA. (F) Ifng mRNA. (G) Il17a mRNA. (H) The pro-inflammatory cytokines IL-6 and TNF were measured in the cell culture supernatants by flow cytometry using a cytometric bead array kit. n=5-10 for both groups, cohorts are littermates. *P < 0.05, **P < 0.01, ***P < 0.001.
EAE had long been considered the prototypic of both Th1 and Th17-mediated autoimmune disease (20). Here, we demonstrate that the initial treatment with DMF stimulates a pro-inflammatory environment in the gut. We analyzed the conventional DCs type 2 from the mLN, which are CD11c+ XCR1- (21), by flow cytometry. We observed an increase in the CD103- CD11b+ DC population (Figure 2C) after 5 days of treatment. Intestinal CD103- DCs might migrate to mLN and promote the production of pro-inflammatory cytokines by effector cells (22).
The treatment with DMF initially favored the expression of innate immune response pro-inflammatory cytokines in the mLN, such as TNF (Figure 2D) and IL-12a (Figure 2E) mRNA. In addition, we saw an expressive increase in the production of IFN-γ (Figure 2F) and IL-17A (Figure 2G) mRNA within 5 days of treatment. The expression of IL-6 mRNA was similar to the control group during the analysis period (Supplementary Figure 4A).
The mLN cells were also stimulated ex-vivo with LPS for 24h to evaluate cytokine production. The levels of TNF and IL-6 (Figure 2H) were higher in cells from DMF-treated animals after 5 days of treatment. There were no changes in other Th1, Th2, and Th17 cytokines levels after the LPS stimulus (Supplementary Figure 4B–F).
Interestingly, this initial pro-inflammatory profile is accompanied by regulation in DMF-treated mice. The oral administration of DMF increases the Foxp3 expression on CD4 T lymphocytes in mLN by the 5th day (Figure 3A). In addition, higher transcription of Foxp3 (Figure 3B), IL-10 (Figure 3C), and TGF-β (Figure 3D) by mLN cells are observed. A small population of Foxp3 CD4 T cells producing TGF-β increased during 5 days of DMF treatment (data not shown). We attribute this result to the characteristics of DMF. Within the intestine, DMF is transformed into its active metabolite MMF, which binds to the HCAR2 receptor on dendritic cells. It is well described in the literature that small-chain fatty acids, such as butyrate, utilize this same HCAR2 receptor to reduce the inflammatory response in the intestine and promote the activation of the CD4+Foxp3+ Treg population (23, 24). Moreover, Round and Mazmanian have demonstrated that oral administration of a fraction of Bacteroides fragilis leads to an increase in CD4+Foxp3+ T reg in mesenteric lymph nodes in mice, and either CD4+Foxp3+ or CD25+Foxp3+ had a regulatory function (25).
Figure 3 An anti-inflammatory response in the mesenteric lymph nodes of C57BL/6J mice with EAE accompanies the pro-inflammatory stimuli in the initial phase of dimethyl fumarate treatment. CD4 T lymphocytes from freshly isolated mesenteric lymph nodes (mLN) were analyzed by flow cytometry. (A) Median fluorescence intensity of Foxp3 in CD4 T cells. The expression of T reg-associated molecules was measured in the directly processed mLN cells by qPCR, having B2m as the housekeeping gene. (B) Foxp3 mRNA; (C) Il10 mRNA; (D) Tgfb1 mRNA; *P < 0.05; n=5-10 for both groups, cohorts are littermates. **P < 0.01.
After a few days, the expression of pro- and anti-inflammatory molecules was similar in DMF and control groups. In fact, by the 10th day, TNF transcription even decreased in DMF-treated mice (Figure 2D), and IFN-γ expression was also reduced (Figure 2F).
Further during treatment, the DMF stimulated an anti-inflammatory environment in the mLN of C57BL/6J mice. We observed an increase in the expression of IL-27 (after 15 days). IL-27 is a pleiotropic cytokine released by antigen-presenting cells (APC), which modulates several immune cells, including myeloid cells and T cells. A significantly higher IL-27 expression was observed in the group that received DMF for 15 days (Figure 4A).
Figure 4 An anti-inflammatory response predominates in the mesenteric lymph nodes of C57BL/6J mice with EAE as the treatment with dimethyl fumarate progresses. Mesenteric lymph nodes (mLN) cells from immunized mice were directly prepared for total RNA extraction and the qPCR protocol. (A) Il27 mRNA expression in the mesenteric lymph nodes (mLN) by qPCR, having B2m as the housekeeping gene. For analyzing the expression of IL-10 in T cells, a fraction of mLN cells were stimulated ex-vivo with PMA, Ionomycin, and Brefeldin A for 4h. T lymphocytes from the mLN were analyzed by flow cytometry. (B) Regulatory T cells in CD4+ Foxp3- population expressing IL-10 (Tr1). (C) Regulatory T cells CD8+ Foxp3- population expressing IL-10. n=5-10 for both groups, cohorts are littermates. *P < 0.05, **P < 0.01.
Our findings demonstrate that oral administration of DMF induces an increase of regulatory T cells of gut-draining lymph nodes of C57BL/6J mice with EAE. A significant increase in IL-10-producing CD4 T lymphocytes was observed after 15 days of treatment (Figure 4B). There is no expression of Foxp3 in this cell population, suggesting that it belongs to the Tr1 subset of regulatory T cells (26). We also showed a significant increase in IL-10 production by Foxp3- CD8 T lymphocytes after 15 days of treatment (Figure 4C). The proportion of Foxp3 T reg cells was the same in treated and control mice during the observed period (data not shown).
Here we demonstrate that DMF reduces the severity of EAE and gradually induces regulatory T cells in the mesenteric lymph nodes. Our data agree with previous observations that the treatment with DMF significantly reduces the severity of EAE (13, 14). The reduction of the severity of EAE may be explained, at least in part, by the ability of monomethyl fumarate (MMF), the bioactive metabolite of DMF, to cross the blood-brain barrier and downregulate the microglia activation (3).
The MMF is a potent agonist for hydroxycarboxylic acid receptor 2 (HCAR2) (4), which may either reduce the inflammatory response (3) or increase the inflammation in both the skin and gastrointestinal tract (8). The pro-inflammatory effect of MMF can be related to the activation of the COX-2 pathway in skin flushing under DMF administration (16, 27). Prostaglandins (PGs) are important in maintaining gastrointestinal homeostasis by contributing to the maintenance of mucosal integrity and limiting the inflammatory response (28). However, increased PG production occurs within the gastrointestinal mucosa of patients with inflammatory bowel disease (IBD) and in experimental models of IBD (29, 30). Treatment with DMF in a mouse model of colitis showed reduced COX-2 levels in the colon of treated mice, which were associated with the alleviation of colitis (31). Moreover, previous studies have demonstrated profound alterations in PGE2 levels in CNS-derived samples from animals with EAE (17, 18) indicating that PGE2 plays a role in the pathogenesis of the disease. In agreement with these observations, we demonstrated a significant increase of PGE2 by DC cells from mesenteric lymph nodes of mice at the peak of EAE when the inflammatory response is systemic, while the PGE2 levels of DMF-treated mice increase at the beginning of the treatment and later remain significantly reduced compared to the control animals, suggesting both pro-inflammatory and anti-inflammatory effects of DMF in the gut draining lymph nodes.
Besides the modulation of PGE2 in the mesenteric lymph nodes, we observed the increase of innate immune response cytokines, such as TNF, IL-12, and IL-6, and pro-inflammatory cytokines from lymphoid cells, such as IFN-g and IL-17A, in mice treated for five days with DMF. It has been shown that gut CD103- CD11b+ DCs can activate T cells and induce the expression of Th1 and Th17-related cytokines (22). We observe an increase in the population of those DCs after five days of treatment, which might induce the higher expression of IFN-γ and IL-17A mRNA. The expression of TGF-β and IL-10 was also higher in mice treated for five days with DMF, reinforcing that DMF treatment induces either pro-inflammatory cytokines or anti-inflammatory response in the initial phase of treatment.
In acute colitis models, DMF appears to promote a reduction in inflammatory activity within just 7 to 10 days of treatment. Previous studies have showed reductions in NF-κB, IL-6, IL-17a, and TNF levels (32, 33), as well as decreased oxidant activity (34) in the colonic lamina propria of DMF treated mice. It is noteworthy that these models induce severe damage to the epithelial barrier, leading to increased interaction between microbiota and lamina propria immune cells, thereby triggering an inflammatory response. In EAE, barrier dysfunction has also been reported, particularly in the early stages of the disease, although it is not as severe as in colitis models (35, 36). The intensity of stimuli in colitis models could contribute to favoring the activation of antioxidant pathways of DMF, as there is evidence of a dependency on Nrf2 for the alleviation of colitis (31, 34). However, studies with Nrf2 knockout mice in EAE have shown that DMF treatment can decrease disease severity even in the absence of the Nrf2 molecule (37), indicating this may not be a primary mechanism of action for DMF in the EAE model. Thus, the balance towards pro-inflammatory factors that we observed during the early stages of EAE in the gut draining lymph nodes may suggest that DMF could exert different effects in the gut depending on local environmental cues. Future studies with lamina propria immune cells could help to elucidate the specific pathways activated by DMF in the gut during EAE. As the treatment progressed, a significant increase of IL-10- producing type 1 regulatory T cells (Tr1 cells) was observed, as well as IL-27, while pro-inflammatory cytokines maintained the same levels as the control. The initial activation of Foxp3+ CD4 T cells may modulate the posterior generation of Tr1 cells since a previous study demonstrated that Foxp3+ T regs condition DCs to induce IL-10-secreting Tr1 cells by a mechanism dependent on IL-27 (38). IL-27 suppresses Th1 and Th17 responses and limits CNS inflammation in several experimental models (39). Consequently, the in vivo administration of IL-27 inhibits EAE development. In addition, the inhibition of IL-27 signaling results in exaggerated Th17 responses and the worsening of EAE (39, 40). IL-27 has been shown to inhibit the development of Th17 cells and to promote the differentiation of Tr1 cells (39, 41). Our data show that the increased production of IL-27 by mesenteric lymph node cells on the fifteenth day of treatment with DMF reduced both IL-17 levels, that were practically normal on the tenth day of treatment, and PGE2.
The IL-27 signaling may also induce the expression of IL-10 on CD8 T lymphocytes (42). Here, we see an increase in IL-10+ CD8 T cells in the gut lymph nodes with the continuation of DMF treatment. The production of IL-10 by CD8 T cells is enhanced in the CNS during the chronic phase of EAE (19), which might contribute to the control of the inflammatory immune response associated with the disease. In addition, a subset of CD122 CD8 regulatory T cells can express high amounts of IL-10 when stimulated by activated T cells and appear to regulate effector lymphocytes in the lymphoid organs (43, 44). These CD122 CD8 T regs reduce the severity of EAE after adoptive transference (45). Therefore, the cells we observed probably contribute to the anti-inflammatory microenvironment in the gut and may participate in the protection of EAE.
In the past decades, studies demonstrated that T cells in patients with autoimmune diseases such as MS might become refractory to T reg suppression (46). Many resistance-inducing mechanisms of effector T cells to suppress T regs have been associated with increased proinflammatory cytokines such as IL-6 and TNF (47, 48). However, another study reveals that DMF therapy enhances the responsiveness of the T effector to T reg in MS patients (49). In line with these observations, our data demonstrate that treatment of mice with EAE with DMF significantly reduces the expression of TNF and does not modify IL-6 levels in the gut draining lymph nodes in the 15th day of treatment, which may contribute to the responsiveness of the T effector to T regs generated in the intestinal mucosa.
Like all studies with disease models, we must acknowledge the primary limitations of our work. Although it appears that DMF treatment operates through various mechanisms over time in MS patients, directly correlating the duration of DMF treatment in humans with the treatment period in EAE is not feasible. One reason for this is the markedly different disease courses. Here, we consider the initial days of DMF treatment primarily as a five-day continuous treatment, aligning with the early stages of EAE (up to the 7th d.p.i), before disease onset. In MS patients the disease progresses over years, so the initial period could be considered in terms of months. Another challenge we encountered was determining a DMF dose that could be comparable to the dosage used by MS patients. Typically, an adult takes 240 mg of DMF every 12h. Initially, we tried different concentrations of DMF, ranging from 7.5 to 150 mg/kg. However, at the higher concentrations, we observed intestinal discomfort (diarrhea) in the animals. Nonetheless, these doses were considerably higher compared to those administered to MS patients. We therefore reduced the DMF dose as much as possible (7.5 mg/kg), which is still high. At this dosage, we observed a decrease in disease severity without the discomfort associated with higher doses. Thus, while we observed early pro-inflammatory activity induced by DMF, we were unable to directly correlate it with gastrointestinal symptoms in the mice.
Taken together, this study demonstrated that the administration of DMF to animals with EAE increases the inflammatory response with an increase in the population of CD103- dendritic cells and the production of pro-inflammatory molecules. After five days of treatment, there is also an increase in CD4+Foxp3+ lymphocytes and anti-inflammatory cytokines, which is followed by a consistent increase in IL-27 and Tr1 regulatory cells. These mechanisms may explain the adverse effect of DMF treatment and its resolution. Our data suggest that the intestinal mucosa may be the first microenvironment of generation of regulatory T cells in DMF-treated MS patients, which may contribute to reducing the inflammatory response.
The raw data supporting the conclusions of this article will be made available by the authors, without undue reservation.
The animal study was approved by UNICAMP Animal Ethics Committee (CEUA/UNICAMP), 4884-1/2018, Universidade Estadual de Campinas, Campinas, Brazil. The study was conducted in accordance with the local legislation and institutional requirements.
AL: Conceptualization, Data curation, Formal Analysis, Investigation, Methodology, Project administration, Validation, Visualization, Writing – original draft, Writing – review & editing. BF: Data curation, Formal Analysis, Investigation, Methodology, Project administration, Writing – review & editing. FP: Data curation, Formal Analysis, Investigation, Methodology, Validation, Writing – original draft, Writing – review & editing. RC: Methodology, Validation, Writing – review & editing. SR: Methodology, Validation, Writing – review & editing. RQ: Methodology, Writing – review & editing. MS: Methodology, Writing – review & editing. NB: Methodology, Writing – review & editing. AM: Methodology, Writing – review & editing. IS: Methodology, Writing – review & editing. AF: Formal Analysis, Supervision, Writing – review & editing. EO: Formal Analysis, Investigation, Methodology, Supervision, Validation, Writing – review & editing. LS: Formal Analysis, Funding acquisition, Investigation, Project administration, Resources, Supervision, Visualization, Conceptualization, Writing – original draft, Writing – review & editing.
The author(s) declare financial support was received for the research, authorship, and/or publication of this article. The following institutions provided funds for laboratory supplies, equipment and scholarship: São Paulo Research Foundation (FAPESP; #2014/26431-0; #2018/03432-2), Coordenação de Aperfeiçoamento de Pessoal de Nível Superior - Brasil (CAPES) - Finance Code 001, and the National Institute of Science and Technology in Neuroimmunomodulation - INCT-NIM (CNPq; #465489/2014-1). FAPESP also provided funds for research publication fees. The study also had the support of the Biogen-Idec research grant.
The authors would like to thank FAPESP, CAPES, and the INCT-NIM-CNPq. We also want to thank Dr. Valquíria A. Matheus (UNICAMP) for her assistance with the histological staining of samples.
LS received a Biogen-Idec research grant.
The remaining authors declare that the research was conducted in the absence of any commercial or financial relationships that could be construed as a potential conflict of interest.
All claims expressed in this article are solely those of the authors and do not necessarily represent those of their affiliated organizations, or those of the publisher, the editors and the reviewers. Any product that may be evaluated in this article, or claim that may be made by its manufacturer, is not guaranteed or endorsed by the publisher.
The Supplementary Material for this article can be found online at: https://www.frontiersin.org/articles/10.3389/fimmu.2024.1391949/full#supplementary-material
1. Linker RA, Lee D, Ryan S, van Dam AM, Conrad R, Bista P, et al. Fumaric acid esters exert neuroprotective effects in neuroinflammation via activation of the Nrf2 antioxidant pathway. Brain. (2011) 134:678–92. doi: 10.1093/brain/awq386
2. Albrecht P, Bouchachia I, Goebels N, Henke N, Hofstetter HH, Issberner A, et al. Effects of dimethyl fumarate on neuroprotection and immunomodulation. J Neuroinflamm. (2012) 9:638. doi: 10.1186/1742-2094-9-163
3. Parodi B, Rossi S, Morando S, Cordano C, Bragoni A, Motta C, et al. Fumarates modulate microglia activation through a novel HCAR2 signaling pathway and rescue synaptic dysregulation in inflamed CNS. Acta Neuropathologica. (2015) 130:279–95. doi: 10.1007/s00401-015-1422-3
4. von Glehn F, Dias-Carneiro RPC, Moraes AS, Farias AS, Silva VAPG, Oliveira FTM, et al. Dimethyl fumarate downregulates the immune response through the HCA2/GPR109A pathway: Implications for the treatment of multiple sclerosis. Multiple Sclerosis Related Disord. (2018) 23:46–50. doi: 10.1016/j.msard.2018.04.016
5. Gerdes S, Shakery K, Mrowietz U. Dimethylfumarate inhibits nuclear binding of nuclear factor kB but not of nuclear factor of activated T cells and CCAAT/enhancer binding protein b in activated human T cells. Br J Dermatol. (2007) 156:838–42. doi: 10.1111/j.1365-2133.2007.07779.x
6. Gillard GO, Collette B, Anderson J, Chao J, Scannevin RH, Huss DJ, et al. DMF, but not other fumarates, inhibits NF-κB activity in vitro in an Nrf2-independent manner. J Neuroimmunology. (2015) 283:74–85. doi: 10.1016/j.jneuroim.2015.04.006
7. Werdenberg D, Joshi R, Wolffram S, Merkle HP, Langguth P. Presystemic metabolism and intestinal absorption of antipsoriatic fumaric acid esters. Biopharmaceutics Drug Disposition. (2003) 24:259–73. doi: 10.1002/bdd.364
8. Phillips JT, Selmaj K, Gold R, Fox RJ, Havrdova E, Giovannoni G, et al. Clinical significance of gastrointestinal and flushing events in patients with multiple sclerosis treated with delayed-release dimethyl fumarate. Int J MS Care. (2015) 17:236–43. doi: 10.7224/1537-2073.2014-069
9. Gold R, Arnold DL, Bar-Or A, Fox RJ, Kappos L, Mokliatchouk O, et al. Long-term safety and efficacy of dimethyl fumarate for up to 13 years in patients with relapsing-remitting multiple sclerosis: Final ENDORSE study results. Multiple Sclerosis J. (2022) 28(5):801–16. doi: 10.1177/13524585211037909
10. Chen Y, Kuchroo VK, Inobe J, Hafler DA, Weiner HL. Regulatory T cell clones induced by oral tolerance: suppression of autoimmune encephalomyelitis. Science. (1994) 265:1237–40. doi: 10.1126/science.7520605
11. Santos LMB, Al-Sabbagh A, Londono A, Weiner HL. Oral tolerance to myelin basic protein induces regulatory TGF-β-secreting T cells in peyer’s patches of SJL mice. Cell Immunol. (1994) 157:439–47. doi: 10.1006/cimm.1994.1240
12. Weiner HL, Friedman A, Miller A, Khoury SJ, Al-Sabbagh A, Santos L, et al. Oral tolerance: immunologic mechanisms and treatment of animal and human organ-specific autoimmune diseases by oral administration of autoantigens. Annu Rev Immunol. (1994) 12:809–37. doi: 10.1146/annurev.iy.12.040194.004113
13. Schilling S, Goelz S, Linker R, Luehder F, Gold R. Fumaric acid esters are effective in chronic experimental autoimmune encephalomyelitis and suppress macrophage infiltration. Clin Exp Immunol. (2006) 145:101–7. doi: 10.1111/j.1365-2249.2006.03094.x
14. Chen H, Assmann JC, Krenz A, Rahman M, Grimm M, Karsten CM, et al. Hydroxycarboxylic acid receptor 2 mediates dimethyl fumarate’s protective effect in EAE. J Clin Invest. (2014) 124:2188–92. doi: 10.1172/JCI72151
15. Traub J, Traffehn S, Ochs J, Häusser‐Kinzel S, Stephan S, Scannevin R, et al. Dimethyl fumarate impairs differentiated B cells and fosters central nervous system integrity in treatment of multiple sclerosis. Brain Pathol. (2019) 29:640–57. doi: 10.1111/bpa.12711
16. Hanson J, Gille A, Zwykiel S, Lukasova M, Clausen BE, Ahmed K, et al. Nicotinic acid– and monomethyl fumarate–induced flushing involves GPR109A expressed by keratinocytes and COX-2–dependent prostanoid formation in mice. J Clin Invest. (2010) 120:2910–9. doi: 10.1172/JCI42273
17. Schiffmann S, Weigert A, Männich J, Eberle M, Birod K, Häussler A, et al. PGE2/EP4 signaling in peripheral immune cells promotes development of experimental autoimmune encephalomyelitis. Biochem Pharmacol. (2014) 87:625–35. doi: 10.1016/j.bcp.2013.12.006
18. Hernandez JB, Chang C, LeBlanc M, Grimm D, Le Lay J, Kaestner KH, et al. The CREB/CRTC2 pathway modulates autoimmune disease by promoting Th17 differentiation. Nat Commun. (2015) 6:7216. doi: 10.1038/ncomms8216
19. Sonobe Y, Jin S, Wang J, Kawanokuchi J, Takeuchi H, Mizuno T, et al. Chronological changes of CD4+ and CD8+ T cell subsets in the experimental autoimmune encephalomyelitis, a mouse model of multiple sclerosis. Tohoku J Exp Med. (2007) 213:329–39. doi: 10.1620/tjem.213.329
20. Korn T, Bettelli E, Oukka M, Kuchroo VK. IL-17 and th17 cells. Annu Rev Immunol. (2009) 27:485–517. doi: 10.1146/annurev.immunol.021908.132710
21. Stagg AJ. Intestinal dendritic cells in health and gut inflammation. Front Immunol. (2018) 9:2883. doi: 10.3389/fimmu.2018.02883
22. Cerovic V, Houston SA, Scott CL, Aumeunier A, Yrlid U, Mowat AM, et al. Intestinal CD103- dendritic cells migrate in lymph and prime effector T cells. Mucosal Immunol. (2013) 6:104–13. doi: 10.1038/mi.2012.53
23. Arpaia N, Campbell C, Fan X, Dikiy S, van der Veeken J, DeRoos P, et al. Metabolites produced by commensal bacteria promote peripheral regulatory T-cell generation. Nature. (2013) 504:451–5. doi: 10.1038/nature12726
24. Singh N, Gurav A, Sivaprakasam S, Brady E, Padia R, Shi H, et al. Activation of gpr109a, receptor for niacin and the commensal metabolite butyrate, suppresses colonic inflammation and carcinogenesis. Immunity. (2014) 40:128–39. doi: 10.1016/j.immuni.2013.12.007
25. Round JL, Mazmanian SK. Inducible Foxp3 + regulatory T-cell development by a commensal bacterium of the intestinal microbiota. Proc Natl Acad Sci. (2010) 107:12204–9. doi: 10.1073/pnas.0909122107
26. Groux H, O’Garra A, Bigler M, Rouleau M, Antonenko S, de Vries JE, et al. A CD4+T-cell subset inhibits antigen-specific T-cell responses and prevents colitis. Nature. (1997) 389:737–42. doi: 10.1038/39614
27. Sheikh SI, Nestorov I, Russell H, O’Gorman J, Huang R, Milne GL, et al. Tolerability and pharmacokinetics of delayed-release dimethyl fumarate administered with and without aspirin in healthy volunteers. Clin Ther. (2013) 35:1582–94. doi: 10.1016/j.clinthera.2013.08.009
28. Cheng H, Huang H, Guo Z, Chang Y, Li Z. Role of prostaglandin E2 in tissue repair and regeneration. Theranostics. (2021) 11:8836–54. doi: 10.7150/thno.63396
29. Raab Y, Sundberg C, Hällgren R, Knutson L, Gerdin B. Mucosal synthesis and release of prostaglandin E2 from activated eosinophils and macrophages in ulcerative colitis. Am J Gastroenterol. (1995) 90(4):614–20. Available at: http://www.ncbi.nlm.nih.gov/pubmed/7717321.
30. Melgar S, Drmotova M, Rehnström E, Jansson L, Michaëlsson E. Local production of chemokines and prostaglandin E2 in the acute, chronic and recovery phase of murine experimental colitis. Cytokine. (2006) 35:275–83. doi: 10.1016/j.cyto.2006.09.007
31. Li S, Takasu C, Lau H, Robles L, Vo K, Farzaneh T, et al. Dimethyl fumarate alleviates dextran sulfate sodium-induced colitis, through the activation of nrf2-mediated antioxidant and anti-inflammatory pathways. Antioxidants. (2020) 9(4):354. doi: 10.3390/antiox9040354
32. Casili G, Cordaro M, Impellizzeri D, Bruschetta G, Paterniti I, Cuzzocrea S, et al. Dimethyl fumarate reduces inflammatory responses in experimental colitis. J Crohn’s Colitis. (2016) 10:472–83. doi: 10.1093/ecco-jcc/jjv231
33. Chen H, Wu X, Sun R, Lu H, Lin R, Gao X, et al. Dysregulation of CD177 + neutrophils on intraepithelial lymphocytes exacerbates gut inflammation via decreasing microbiota-derived DMF. Gut Microbes. (2023) 15(1). doi: 10.1080/19490976.2023.2172668
34. Liu X, Zhou W, Zhang X, Lu P, Du Q, Tao L, et al. Dimethyl fumarate ameliorates dextran sulfate sodium-induced murine experimental colitis by activating Nrf2 and suppressing NLRP3 inflammasome activation. Biochem Pharmacol. (2016) 112:37–49. doi: 10.1016/j.bcp.2016.05.002
35. Nouri M, Bredberg A, Weström B, Lavasani S, Lees JR. editor. Intestinal barrier dysfunction develops at the onset of experimental autoimmune encephalomyelitis, and can be induced by adoptive transfer of auto-reactive T cells. PloS One. (2014) 9(9):e106335. doi: 10.1371/journal.pone.0106335
36. Secher T, Kassem S, Benamar M, Bernard I, Boury M, Barreau F, et al. Oral administration of the probiotic strain Escherichia coli Nissle 1917 reduces susceptibility to neuroinflammation and repairs experimental autoimmune encephalomyelitis-induced intestinal barrier dysfunction. Front Immunol. (2017) 8:1096. doi: 10.3389/fimmu.2017.01096
37. Schulze-Topphoff U, Varrin-Doyer M, Pekarek K, Spencer CM, Shetty A, Sagan SA, et al. Dimethyl fumarate treatment induces adaptive and innate immune modulation independent of Nrf2. Proc Natl Acad Sci. (2016) 113:4777–82. doi: 10.1073/pnas.1603907113
38. Awasthi A, Carrier Y, Peron JPS, Bettelli E, Kamanaka M, Flavell RA, et al. A dominant function for interleukin 27 in generating interleukin 10–producing anti-inflammatory T cells. Nat Immunol. (2007) 8:1380–9. doi: 10.1038/ni1541
39. Batten M, Li J, Yi S, Kljavin NM, Danilenko DM, Lucas S, et al. Interleukin 27 limits autoimmune encephalomyelitis by suppressing the development of interleukin 17–producing T cells. Nat Immunol. (2006) 7:929–36. doi: 10.1038/ni1375
40. Fitzgerald DC, Ciric B, Touil T, Harle H, Grammatikopolou J, Sarma Das J, et al. Suppressive effect of IL-27 on encephalitogenic th17 cells and the effector phase of experimental autoimmune encephalomyelitis. J Immunol. (2007) 179:3268–75. doi: 10.4049/jimmunol.179.5.3268
41. Casella G, Finardi A, Descamps H, Colombo F, Maiorino C, Ruffini F, et al. IL-27, but not IL-35, inhibits neuroinflammation through modulating GM-CSF expression. Sci Rep. (2017) 7:16547. doi: 10.1038/s41598-017-16702-w
42. Batten M, Kljavin NM, Li J, Walter MJ, de Sauvage FJ, Ghilardi N. Cutting edge: IL-27 is a potent inducer of IL-10 but not foxP3 in murine T cells. J Immunol. (2008) 180:2752–6. doi: 10.4049/jimmunol.180.5.2752
43. Endharti AT, Rifa’ M, Shi Z, Fukuoka Y, Nakahara Y, Kawamoto Y, et al. Cutting edge: CD8 + CD122 + Regulatory T cells produce IL-10 to suppress IFN-γ Production and proliferation of CD8 + T cells. J Immunol. (2005) 175(11):7093–7. doi: 10.4049/jimmunol.175.11.7093
44. Rifa’i M, Shi Z, Zhang S-Y, Lee YH, Shiku H, Isobe K, et al. CD8+CD122+ regulatory T cells recognize activated T cells via conventional MHC class I–αβTCR interaction and become IL-10-producing active regulatory cells. Int Immunol. (2008) 20:937–47. doi: 10.1093/intimm/dxn052
45. Lee Y-H, Ishida Y, Rifa’i M, Shi Z, Isobe K, Suzuki H. Essential role of CD8 + CD122 + Regulatory T cells in the recovery from experimental autoimmune encephalomyelitis. J Immunol. (2008) 180:825–32. doi: 10.4049/jimmunol.180.2.825
46. Viglietta V, Baecher-Allan C, Weiner HL, Hafler DA. Loss of functional suppression by CD4+CD25+ regulatory T cells in patients with multiple sclerosis. J Exp Med. (2004) 199:971–9. doi: 10.1084/jem.20031579
47. Valencia X, Stephens G, Goldbach-Mansky R, Wilson M, Shevach EM, Lipsky PE. TNF downmodulates the function of human CD4+CD25hi T-regulatory cells. Blood. (2006) 108:253–61. doi: 10.1182/blood-2005-11-4567
48. Schneider A, Long SA, Cerosaletti K, Ni CT, Samuels P, Kita M, et al. In active relapsing-remitting multiple sclerosis, effector T cell resistance to adaptive Tregs involves IL-6-mediated signaling. Sci Trans Med. (2013) 5(170). doi: 10.1126/scitranslmed.3004970
Keywords: dimethyl fumarate, experimental autoimmune encephalomyelitis, type 1 regulatory T cells, anti-inflammatory cytokines, gut draining lymph nodes
Citation: Lima ADR, Ferrari BB, Pradella F, Carvalho RM, Rivero SLS, Quintiliano RPS, Souza MA, Brunetti ŃS, Marques AM, Santos IP, Farias AS, Oliveira EC and Santos LMB (2024) Dimethyl fumarate modulates the regulatory T cell response in the mesenteric lymph nodes of mice with experimental autoimmune encephalomyelitis. Front. Immunol. 15:1391949. doi: 10.3389/fimmu.2024.1391949
Received: 26 February 2024; Accepted: 22 April 2024;
Published: 03 May 2024.
Edited by:
Marija Mostarica-Stojkovic, University of Belgrade, SerbiaReviewed by:
Djordje Miljkovic, University of Belgrade, SerbiaCopyright © 2024 Lima, Ferrari, Pradella, Carvalho, Rivero, Quintiliano, Souza, Brunetti, Marques, Santos, Farias, Oliveira and Santos. This is an open-access article distributed under the terms of the Creative Commons Attribution License (CC BY). The use, distribution or reproduction in other forums is permitted, provided the original author(s) and the copyright owner(s) are credited and that the original publication in this journal is cited, in accordance with accepted academic practice. No use, distribution or reproduction is permitted which does not comply with these terms.
*Correspondence: Amanda D. R. Lima, YW1hbmRhZGlhc3JsQGdtYWlsLmNvbQ==; Leonilda M. B. Santos, bGVvbmlsZGFAdW5pY2FtcC5icg==
Disclaimer: All claims expressed in this article are solely those of the authors and do not necessarily represent those of their affiliated organizations, or those of the publisher, the editors and the reviewers. Any product that may be evaluated in this article or claim that may be made by its manufacturer is not guaranteed or endorsed by the publisher.
Research integrity at Frontiers
Learn more about the work of our research integrity team to safeguard the quality of each article we publish.