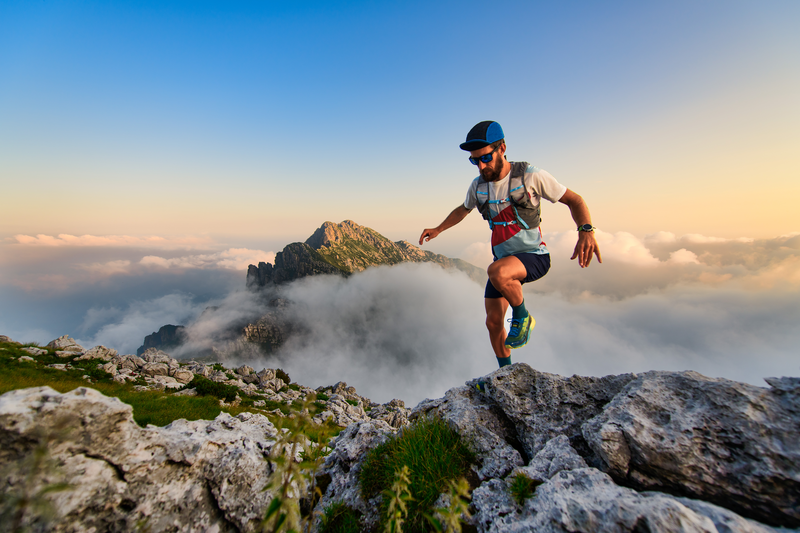
94% of researchers rate our articles as excellent or good
Learn more about the work of our research integrity team to safeguard the quality of each article we publish.
Find out more
REVIEW article
Front. Immunol. , 08 May 2024
Sec. Inflammation
Volume 15 - 2024 | https://doi.org/10.3389/fimmu.2024.1387292
Peritoneal dialysis is a widely used method for treating kidney failure. However, over time, the peritoneal structure and function can deteriorate, leading to the failure of this therapy. This deterioration is primarily caused by infectious and sterile inflammation. Sterile inflammation, which is inflammation without infection, is particularly concerning as it can be subtle and often goes unnoticed. The onset of sterile inflammation involves various pathological processes. Peritoneal cells detect signals that promote inflammation and release substances that attract immune cells from the bloodstream. These immune cells contribute to the initiation and escalation of the inflammatory response. The existing literature extensively covers the involvement of different cell types in the sterile inflammation, including mesothelial cells, fibroblasts, endothelial cells, and adipocytes, as well as immune cells such as macrophages, lymphocytes, and mast cells. These cells work together to promote the occurrence and progression of sterile inflammation, although the exact mechanisms are not fully understood. This review aims to provide a comprehensive overview of the signals from both stromal cells and components of immune system, as well as the reciprocal interactions between cellular components, during the initiation of sterile inflammation. By understanding the cellular and molecular mechanisms underlying sterile inflammation, we may potentially develop therapeutic interventions to counteract peritoneal membrane damage and restore normal function.
Peritoneal dialysis (PD) is a widely used method for renal replacement therapy, similar to hemodialysis and renal transplantation. It involves using the peritoneal membrane as a dialysis membrane to treat end-stage renal disease (ERSD) (1–3). In this procedure, the peritoneal membrane cavity serves as a medium for the transfer of waste products and solutes between the body and the peritoneal dialysis fluids (PDFs). A permanent catheter is inserted to introduce PDFs into the peritoneal cavity. These PDFs contain glucose as an osmotic agent, which facilitates the movement of fluid from the circulation to the peritoneal membrane cavity, eliminating the metabolic waste products and excess water (4). Globally, PD is utilized by over 272,000 patients, accounting for approximately 11% of dialysis patients (5). PD therapy offers a higher quality of life and lower cost for individuals with ESRD compared to hemodialysis, owing to its simplicity of operation, low risk of cross-infectious, and preservation of residual renal function (6–8). Traditionally, the most commonly used PDFs have an acidic pH and rely on high osmotic glucose solutions to facilitate water and solute exchange. However, in recent years, various types of biocompatible PDFs, such as bicarbonate-based, icodextrin-based, amino acid-based solutions, have also become available in the market (9).
Although PD therapy greatly improves the quality of life for individuals with ESRD, it is not without its drawbacks. One notable concern is the occurrence of PD-related peritonitis, which can lead to chronic inflammation and damage to peritoneal cells (10–13). The development of peritonitis in PD can be attributed to both infectious and non-infectious factors (14). In particular, infection-related peritonitis remains the most common cause of technique failure and subsequent transition to hemodialysis in academic settings. The peritoneal membrane often undergoes significant changes in both structure and function during prolonged dialysis, leading to chronic inflammation. These alterations include mesothelial-to-mesenchymal transition, the growth of new blood vessels (neoangiogenesis), the development of fibrosis beneath the mesothelium (sub‐mesothelial fibrosis), and the occurrence of hyalinizing vasculopathy. These factors can cause irreversible damage to the peritoneal tissue, resulting in the failure of ultrafiltration and a decline in the effectiveness of dialysis (15–17). Unfortunately, the chronic sterile inflammation caused by these various factors frequently goes unnoticed or receives limited attention.
The induction of sterile inflammation is a complex pathological process in which resident cells sense pro-inflammatory signals and release extracellular mediators, leading to the recruitment of circulating immune cells that contribute to the initiation and escalation of the inflammatory response. Consequently, the development of sterile inflammation is the result of intricate signaling interactions between stromal resident cells and circulating immune cells, understanding of which has the potential to improve the medical management of this harmful condition. In recent years, there have been some successful attempts to address this issue. This paper focuses on the interactions between immune cells and peritoneal stroma cells in sterile inflammation. Furthermore, potential interventions for sterile inflammation caused by PD are discussed, which could be significantly important in preventing aseptic changes in PD.
The peritoneum is structured into three distinct layers: the mesothelium, the basal lamina, and the submesothelial stroma. The submesothelial stroma provides support to the mesothelial cells and the basal lamina, and is mainly composed of collagen fibers, (myo)fibroblasts, adipocytes, as well as lymphatic and blood vessels (18). In the context of PD, the prolonged exposure of the mesothelium to bioincompatible PDFs and their breakdown products triggers an inflammatory response in mesothelial cell. Consequently, this response stimulates the production of cytokines, chemokines, and extracellular matrix (ECM) proteins (19–23). These factors have the potential to initiate damage to the peritoneum (24). The occurrence of cellular stress and tissue damage triggers the production of ECM degradation products and the release of endogenous cellular constituents, which are referred to as damage-associated molecular patterns (DAMPs) (25). These DAMPs activate pro-inflammatory and pro-fibrotic signaling pathways. Toll-like receptors (TLRs), specifically TLR2 and TLR4, as well as the receptor of advanced glycation end products (RAGEs), plays a crucial role in recognizing and responding to DAMPs (25–28). They recognize a wide range of DAMPs that are released during tissue injury, including hyaluronan and fibronectin resulting from matrix degradation, as well as heat shock proteins and high mobility group box-1 (HMGB1) released due to cellular stress (25, 29–33). Activation of TLRs triggers the production of inflammatory and fibrotic cytokines, such as tumor necrosis factor-α (TNF-α), interleukin 6 (IL-6), IL-8 and transforming growth factor β1 (TGF-β1) (34, 35). However, the use of soluble TLR2 (a TLR inhibitor) has been found to reduce the development of sterile peritoneal inflammation and fibrosis (28, 36). Additionally, when mesothelial cells are exposed to PDFs, they release HMGB1 from the nucleus. This HMGB1 acts on the mesothelial cells in an autocrine manner, stimulating the expression of IL-8 and monocyte chemoattractant protein-1 (MCP-1) through the mitogen-activated protein kinase (MAPK) signaling pathways (36–38). Importantly, elevated levels of HMGB1 in the serum have been associated with microinflammatory conditions in continuous ambulatory peritoneal dialysis (CAPD) patients. Inhibition of HMGB1 has shown a protective effect on peritoneal function in peritonitis models (39, 40).
Mitochondrial dysfunction plays a role in the inflammatory response of human peritoneal mesothelial cells (HPMCs) derived from PDFs (41). A key function of mitochondria is the production of reactive oxygen species (ROS), which are involved in oxidative stress (42). Recent research has shown that mitochondria also release DAMPs, which play a significant role in inflammatory and immune responses (43). In addition, impaired mitochondria release DAMPs, such as mitochondrial ROS and mitochondrial DNA, which are recognized by the immune system and trigger an immune response (44). This response can further damage mitochondrial, leading to a cycle of mitochondrial dysfunction and activation of inflammation. Specifically, mitochondrial DNA has been shown to activate of TLRs, leading to an inflammatory response (45). Additionally, mitochondrial DNA can activate the NACHT, LRR and PYD domains-containing protein 3 (NLRP3) inflammasome, resulting in the secretion of pro-inflammatory cytokines IL-1β and IL-18 (46–49). High glucose levels in PDFs also contribute to mitochondrial damage and apoptosis in HPMCs (50, 51). Moreover, mitochondrial dysfunction in HPMCs is implicated in T cells differentiation, further exacerbating the inflammatory response (52). The inhibition of ROS could have a significant impact on the activation of NLRP3, production of ROS, and expression of IL-1β (53). Furthermore, the application of resveratrol has been shown to induce mitophagy/autophagy through adenosine monophosphate-activated protein kinase, resulting in a decrease in the inflammatory response by suppressing the NLRP3 inflammasome (49). Additionally, recent research has shown that paricalcitol can alleviate the epithelial-to-mesenchymal transition (EMT) of HPMCs triggered by TGF-β1 through the inhibition of the NLRP3 inflammasome and oxidative stress (54).
Numerous studies have provided evidence that nuclear factor-κB (NF-κB) pathway plays a crucial role in the PD-related sterile inflammation. In patients undergoing CAPD, the presence of various compounds and glycated proteins strongly activates the NF-κB pathway in HPMCs, leading to the release of inflammatory mediators such as IL-1β, IL-6 and TNF-α, as well as the activation of cyclooxygenase-2 (55). In rat models, NF-κB signaling pathways are also activated by high glucose and hypertonic PDFs through a protein kinase C (PKC)-dependent mechanism. This activation results in an increased synthesis of MCP-1 (22, 56). Additionally, the EMT process observed in PD patients, which is a common disorder is also influenced by the activation of NF-κB (20, 23). A study has shown that p38 plays a role in maintaining the expression of E-cadherin by suppressing TGF-β-activated kinase 1 (TAK1) NF-κB pathway, thereby inhibiting EMT in primary HPMCs (57). The activation of p38 may also lead to an increase in IL-8 transcription through NF-κB and post-transcriptional molecular mechanisms (58–60). Another study has indicated that the process of EMT is regulated by the ERK/NF-κB/Snail1 pathway in primary mesothelial cells from PD patients (20). However, the administration of parthenolide, a NF-κB inhibitor, has been shown to reduce inflammation and peritoneal fibrosis (PF) through the NF-κB/TGF-β/Smad pathway, thereby reducing the level of IL-6, TNF-α, and MCP-1 (61).
Recent researches have demonstrated that peroxisome proliferator-activated receptor-gamma (PPAR-γ), a nuclear hormone receptor that regulates glucose and lipid homeostasis, possesses anti-inflammatory properties (62, 63). Exposure of the peritoneum to PDFs leads to EMT of HPMCs, fibrosis, angiogenesis, and an inflammatory response. However, administration of the PPAR-γ agonist rosiglitazone in mice has been found to alleviate these changes (64). The PPARβ/δ agonist GW501516 has also been found to have a mitigating effect on peritonitis in rat models of PF through the inhibition of the TAK1–NF-κB pathway (65). Furthermore, microsomal prostaglandin E synthase-1 and its derived prostaglandin E2 also play a crucial role in PF (66). Recent researches have shown that the treatment of ONO-AE3–208, a prostaglandin E2 receptor 4, can suppress both the activation of the NLRP3 inflammasome and the expression of inflammatory cytokines induced by high glucose in rat peritoneal mesothelial cells. This is achieved by regulating NF-κB signaling (36, 67, 68). Additionally, the janus kinase/signal transducer and activator of transcription (JAK/STAT) signaling pathway has been found to contribute to the inflammatory response in the peritoneum of patients undergoing PD (69). Furthermore, both the p38 MAPK pathway and the PKC signaling have been shown to be activated in high glucose-induced inflammation in HPMCs (70, 71). It has also been observed that high glucose treatment stimulates IL-6 synthesis in Met-5A cell, and IL-6 subsequently stimulates vascular endothelial growth factor (VEGF) synthesis, partially dependent on the JAK/STAT3 signaling pathway (Figure 1) (72).
Figure 1 The role of peritoneal mesothelial cells in sterile inflammation. DAMPs accumulate during chronic exposure to bioincompatible PDFs. These DAMPs subsequently activate pro-inflammatory and profibrotic responses by activating TLRs and RAGE. In the condition of PDFs, the mesothelial cells secrets HMGB1, a type of DAMPs, which in turn enhances the inflammatory response. Furthermore, mitochondrial dysfunction induced by PDFs leads to the activation of NLRP3, triggering the secretion of the pro-inflammatory cytokines.
Mesothelial cells possess the remarkable ability to synthesize and release a variety of complement factors, specifically C4, C3, and C5-C9 (73, 74). These complement proteins play a crucial role in the immune response. Additionally, other tissue-resident cells, including immune cells, may also contribute to the production of complement proteins within the peritoneal cavity. Studies have confirmed the presence of complement system molecules, such as C3-C9 and factor D, in the fluid that is drained during PD (75, 76). In fact, proteomic analysis has identified up to 18 different complement proteins, including C3, C4, C9, factors D, B, H, and I, in the PDFs (77–81). It is noteworthy that the complement system can be activated within the peritoneal cavity due to the production and expression of various effectors (such as C3, C4, C5, and C6–C9) and their regulators (such as membrane cofactor protein, CD55, and CD59) by mesothelial cells derived from healthy individuals and patients with kidney disease who are undergoing PD (73, 74, 82, 83). Activation of the complement system in PD patients is believed to occur due to exposure to PDFs, particularly those containing high levels of glucose and glucose degradation products. This exposure to high glucose PDFs can trigger an excessive activation of the complement system, leading to local inflammation, cellular damage, and ultimately causing structural alterations in the peritoneal membrane. These alterations may include vascular proliferation, vasculopathy and PF (84, 85).
Glucose and its degradation products found in PDFs have been identified as triggers of EMT in mesothelial cells. This transition causes the cells to transform into fibroblast-like cells with increased migratory, invasive, and fibrogenic properties (37, 86). As a result, mesothelial cells undergoing EMT secrete large amounts of TGF-β and VEGF. The local production of VEGF by transitioning mesothelial cells appears to play a pivotal role in the mechanisms involved in peritoneal angiogenesis and vascular permeability. This process may contribute to the inflammation within the peritoneum (87, 88).
The EMT of mesothelial cells is a critical source of peritoneal fibroblasts, which have been implicated in the aseptic inflammation induced by PD. Similar to mesothelium cells, peritoneal fibroblasts, have the ability to synthesize various chemokines. One example is the release of chemokines MCP-1/CCL2 and IL-8/CXCL8, which are chemokines involved in recruiting immune cells, by peritoneal fibroblasts. This process is mediated by the NF-κB family, a group of transcription factors that play a role in inflammation (88). Additionally, peritoneal fibroblasts can produce cytokines of CXCL1 and CXCL8, which target neutrophils. The production of these cytokines is primarily induced by IL-1β (89). Furthermore, peritoneal fibroblasts have the capability to produce CCL5, a potent chemoattractant for mononuclear leukocytes. The production of CCL5 is intricately regulated by IFN-γ, a cytokine with immunomodulatory functions. Additionally, the treatment of peritoneal fibroblasts with IFN-γ can induce the production of CCL5 in response to CD40 ligand, a protein involved in immune cell activation (90).
Furthermore, peritoneal fibroblasts have been found to produce chemokines in response to exposure to high levels of glucose. In vitro studies have demonstrated that stimulation of peritoneal fibroblasts with high glucose PDFs leads to increased expression of chemokine (C-C motif) ligand mRNA and nuclear factor of activated T cells 5 (NFAT5) (91). Previous research has indicated that NFAT5 modulates the activity of NF-κB in response to osmotic stress (92). The increased expression of NFAT5 in human peritoneal fibroblasts is associated with NF-κB activation in patients undergoing PD, potentially leading to the recruitment of macrophages.
The presence of PD appears to have a notable proinflammatory effect on the endothelium (93). In healthy individuals, the expression of IL-17, a pro-inflammatory cytokine, is minimal in the peritoneum, but it is highly expressed in peritoneal biopsies of long-term PD patients (94). This increase in IL-17 contributes to angiogenic stimulation and direct damage to the peritoneum in PD patients. IL-17 up-regulates the expression of VEGF, leading to enhanced angiogenesis. It also promotes the production of CXC chemokines such as CXCL1 and CXCL8, which further contribute to inflammation.
Additionally, IL-17 stimulates the release of IL-6, another pro-inflammatory cytokine, from various sources including HPMCs, endothelial cells, macrophages, and monocytes (95, 96). IL-6 upon, specific binding to its receptor, further enhances the production of angiogenic molecules like VEGF and adhesion molecules such as intercellular adhesion molecule 1 (97–99). With the stimulation of PDFs, the above inflammation factors were significantly higher in the endothelial cells via activating both p38 MAPK and NF-κB pathway. This observation was consistent with the findings in PD patients, where increased levels of these inflammatory factors were observed (93). The stimulation of endothelial cells by these inflammatory factors, such as VEGF, resulted in microvascular alterations characterized by increased vascular permeability, microcirculation density, and transendothelial migration of infiltrating cells. These alterations can contribute to endothelial cells proliferation, inflammation, and injury, ultimately leading to peritoneal inflammation, fibrosis, and reduced efficacy of PD (Figure 2) (100–102).
Figure 2 Endothelial cells alterations in PD. Both inflammatory cytokines IL-6 and IL-17 secreted from HPMCs and immune cells contribute to increase vascular permeability, microcirculation density, and transendothelial migration of infiltrating cells via up-regulating the expression of VEGF.
Previous studies have shown that cultured HPMCs produce VEGF-A and VEGF-C in response to glucose degradation products or cytokines (103, 104). However, it has been observed that targeting of VEGF-A by miR-15a-5p can suppress inflammation and fibrosis in HPMCs induced by PD (104). In addition, the use of biocompatible PDFs has been found to result in fewer adverse reactions in endothelial dysfunction compared to conventional PDFs in PD patients (105).
In recent years, the potential impact of adipocytes in PD has been overlooked. There is a noticeable increase in body mass among PD patients, with visceral fat being the main contributor (106, 107). Long-term PD patients also display elevated levels of adipokines in their plasma (108). Ultrastructural investigations have shown that dialysate can penetrate adipose tissue in the presence of an injured mesothelial monolayer (109). Adipocytes have various functions, including autocrine, paracrine, and endocrine activities. They release a range of adipokines and cytokines, such as leptin, adiponectin, resistin, visfatin, IL-6, TNF-a, TGF-β, VEGF, and others (110). On one hand, adipokines such as leptin and resistin have pro-inflammatory and pro-angiogenic effects. Leptin, a hormone secreted in large amounts by adipocytes, can phosphorylate VEGFR2 and activate the p38 MAPK/Akt/COX-2 signaling pathway, thereby promoting angiogenesis (111). In addition, hyperleptinemia induced by PD also stimulates macrophages and monocytes to secret IL-6 and TNF-α (112). Conversely, certain adipokines, such as adiponectin and omentin, have anti-inflammatory and anti-angiogenic properties. However, their expression is suppressed in the peritoneum (113). Adiponectin plays a crucial role in inhibiting the production of adhesion molecules by endothelial cells, thereby preventing the attachment of monocytes (114). Moreover, it reduces the activation of NF-κB, which is induced by TNF-α. However, there is a negative feedback loop between TNF-α and adiponectin, as TNF-α downregulates the production of adiponectin, creating a vicious cycle that leads to lower adiponectin release (115). Furthermore, adipose-derived cytokine, promotes the propagation of inflammation by stimulation macrophage infiltration into the interstitial spaces within adipose tissue (116). Prolonged dialysis has been found to result in an accumulation of adipocytes and infiltrated macrophages within the body, which in turn secrete various adipokines and cytokines that contribute to inflammation and tissue damage (117–119). Interestingly, the omentum, a fatty tissue layer within the abdomen, contains a significant number of progenitor cells (120). The stromal vascular fraction derived from adipose tissues also contains pluripotent mesenchymal stem cells that have the ability to regenerate damaged tissue (121). This discovery offers a promising approach for preserving the functionality of the peritoneal membrane (Figure 3).
Figure 3 Adipocytes in inflammation and angiogenesis during PD. Adipocytes would be stimulated in the condition of injury mesothelial. The activated adipocytes subsequently release pro-inflammatory and pro-angiogenic cytokines (e.g., leptin and resistin) and suppress anti-inflammatory cytokines (e.g., adiponectin and omentin). Additionally, MCP-1 derived from adipose promotes macrophages infiltration to fat tissue.
The activation of macrophages plays a crucial role in the advancement of kidney diseases and the ineffectiveness of treatment for PD (Figure 4). Monocytes and macrophages constitute a significant proportion (50%-90%) of the infiltrated leukocytes in peritoneum (122, 123). Generally, macrophages can be divided into two categories: tissue resident macrophages and monocyte-derived macrophages (124). While monocyte-derived macrophages have been shown to contribute to the progression of inflammation and fibrosis, tissue resident macrophages have a protective role. Surprisingly, researchers have discovered that peritoneal resident macrophages are gradually losing their homeostatic properties and anti-inflammatory properties, instead exhibiting a heightened inflammatory response (125). Furthermore, it has been observed that M2 macrophages are elevated in both the effluents and peritoneal membrane biopsies of PD patients (126, 127). In mice induced with chlorhexidine gluconate and PDFs, there is also an increase in the infiltration of T cells and macrophages (128, 129). Additionally, the presence of high glucose PDFs leads to a significant increase in inflammation and almost complete depletion of tissue resident cells (125). The inflammatory macrophages that infiltrate the affected area produce inflammatory cytokines and collaborate with adipocytes, resulting in a substantial escalation of the inflammatory cascade (116).
Figure 4 The effect of macrophages in peritoneal damage by PDFs. Prolonged dialysis further leads to an increased capacity of recruited macrophages and a depletion of tissue resident cells. Prolonged exposure to high glucose PDFs and the cytokines leads to the differentiation and activation of macrophages, which onsets inflammatory response.
Among these receptors, TLR4 has been extensively studied in macrophage polarization and peritonitis (59, 130). The injury and necrosis of mesothelial cells induced by high glucose exacerbate the accumulation of extracellular TLR4 and HMGB1, resulting in the recruitment of a significant number of macrophages to the abdominal cavity (131, 132). Additionally, HMGB1 has the ability to activate TLRs, initiating innate immunity and subsequently increasing the expression of MCP-1 and the activation of macrophages (133). Advanced glycation end products (AGEs) and glucose degradation products can stimulate peritoneal macrophages, leading to the secretion of cytokines such as IL-1β, IL-6, and IL-8, in HPMCs (134). The NLRP3 inflammasome and its downstream pathway enhances the activation of caspase-1 and the maturation of IL-1β specifically in macrophages, contributing to the progression of inflammation (135, 136). Prolonged exposure to high glucose PDFs and the cytokines can induce macrophage polarizations, which contributes to an inflammatory response and PF (137–139). M1 macrophages release inflammatory cytokines such as IL-1β, IL-6, and TNF-α, while M2 macrophages secrete anti-inflammatory substances like IL-10 and arginase-1 (140, 141). Previous research has shown that high glucose induces peritoneal injury via the PKC-β pathway and promotes M1 macrophage polarization in mouse models (142). It has been observed that PD induces a shift in adipose tissue macrophages from M2 to M1, promoting a pro-inflammatory state (116). Co-culturing HMrSV5 with M1 macrophages resulted in a loss of the typical epithelial cell morphology, indicating that HMrSV5 undergoes EMT through TLR4 receptors (143). Additionally, previous studies have proposed a role of M2 macrophages in peritoneal inflammation and PF (126, 144). It is worth mentioning that exposure to PDFs has been shown to induce polarization of M2 macrophages and the subsequent inflammatory response, which may be associated with the transmission of exosomes (145).
In previous research, it has been demonstrated that the administration of the probiotic Lactobacillus casei Zhang effectively corrects gut dysbiosis, leading to an improvement in PF. This improvement is achieved through the inhibition of macrophage-related inflammation via the PPAR-γ/NF-κB pathway (122). Moreover, the use of biocompatible PDFs has been found to increase the recruitment of M1 macrophages in uremic mice (146). In the context of dialysis-induced PF, mesenchymal stem cells have been shown to induce the polarization of macrophages into the M2 phenotype through the release of IL-6 (147). Astragalus membranaceus, a traditional Chinese medicine with anti-inflammatory properties, has been found to inhibit the recruitment and activation of monocytes/macrophages via the MCP-1 pathway in rats undergoing PD (148). Moreover, treatment with hepatocyte growth factor has been shown to reduce the infiltration of macrophages in mouse models of PF, while also mitigating the upregulation of proinflammatory and profibrotic genes associated with PF (149).
Lymphocytes can be roughly divided into T lymphocytes and B lymphocytes. B lymphocyte cells have been identified as important contributors to innate immunity and autoimmunity. However, there is little evidence suggesting their involvement in PD-related sterile inflammation (150). On the other hand, an increased number of T lymphocytes has been observed in the effluent of patients undergoing PD (151). Thus, targeting the differentiation of T cells may offer potential therapeutic strategies for treating peritoneal damage by regulating immune and inflammatory responses. Notably, there are notable differences in the levels of CD4+ and CD8+ T cells in patients undergoing PD compared to healthy individuals (152, 153). Regarding CD4+ cells subsets, Th1 cells are characterized by increased production of IFN-γ, while Th2 cells primarily secrete IL-4 (154). Interestingly, patients undergoing CAPD have shown a significant increase in the proportion of Th2 cells (155). This dysregulation of Th1/Th2 balance in PD patients leads to changes in proinflammatory and anti-inflammatory cytokines in their serum (156–158). Surprisingly, preliminary studies have indicated that PF mice induced by PDFs exhibit an enhanced immune response, characterized by the presence of Th17 and T cells in the peritoneum, rather than Th1 or Th2 cells (159).
Regulatory T cells (Tregs) play a crucial role in limiting inflammation, while Th17 cells secrete various proinflammatory cytokines. Tregs are responsible for regulating the expansion of T cells, including Th17 cells, which have been implicated in peritoneal damage and the development of fibrosis (151, 160). A primary study has demonstrated that exposure to PDFs leads to an imbalance of Th17/Treg cells, with an increase in Th17 cells and a decrease in Treg cells, ultimately resulting in peritoneal damage in mouse models (151). Additionally, CD69, a leukocyte membrane glycoprotein, contributes to activation of Th17 cells and the expression of IL-17 in PD mouse models through the JAK3/STAT5 signaling pathway (160, 161). Therapeutic inhibition of TLR2 activity in the peritoneum has been shown to protect against inflammation and PF induced by PDFs, resulting in an increase in the ratio of Tregs to Th17 cells (162). Another study suggests that T lymphocytes stimulates IL-17 expression by releasing calpains to regulate TLR2 (163).
Recent evidence also suggests that the IL-17A-induced inflammatory response may contribute to peritoneal injury in both experimental models induced by PDFs and in patients undergoing PD (94, 146, 160, 164). Studies in mice and human peritoneal biopsies have also demonstrated an overexpression of IL-17 (151). It is possible that endogenous factors in the peritoneum, such as AGEs, might regulate IL-17 levels by promoting pro-inflammatory cytokines IL-6 and TGF-β (165, 166). Binding IL-17A to its receptor in mesothelial cells has the ability to trigger proinflammatory responses. Studies using cultured HPMCs have shown that IL-17A can activate the NF-κB pathway and the release of downstream cytokines, such as CXCL1 (167, 168). Additionally, IL-17A has been found to stimulate peritoneal cells, resulting in the upregulation of proinflammatory cytokines like IL-1β, IL-6 and MCP-1, which contribute to the persistence of inflammation (48, 169). Resident γδ T cells in peritoneum produce IL-17, which in turn contributes to fibrosis and ultrafiltration failure (94). Conversely, studies have shown that intraperitoneal injections of a neutralizing IL-17A antibody can prevent peritoneal changes and reduced PF in mice exposed to PDFs (94).
Various therapeutic approaches aimed at preventing peritoneal damage by targeting the Th17/Treg axis have been investigated (Figure 5). In PD patients, treatment with rosiglitazone has been shown to lower levels of IL-17 and IL-23 and increase levels of FoxP3+Treg activity (64). Paricalcitol, the vitamin D activator, also inhibits IL-17 production and slows the progression of PF (170). The dipeptide alanyl-glutamine has also been found to ameliorate PF and attenuate IL-17-dependent pathways during PD (171). Additionally, it has been discovered that the use of conventional PDFs triggers the activation of the Th17 immune response in the peritoneum, while the use of biocompatible PDFs does not (146). Moreover, potential therapeutic options include statins, mammalian target of rapamycin inhibitors, cyclooxygenase-2 inhibitors, and angiotensin converting enzyme inhibitors, as they have shown the ability to modulate the Th17/IL-17A response in the injured peritoneum (172).
Figure 5 Th17/Treg axis contributes to peritoneal damage induced by PDFs. Prolong exposure to dialysis fluids results in an imbalance of Th17/Treg cells, with an up-regulation of Th17 cells and down-regulation of Treg cells, ultimately leading to peritoneal damage. IL-17A released by activated Th17 cells stimulate peritoneal cells, leading to the upregulation of proinflammatory cytokines. Nevertheless, therapeutic approaches targeting the T17/Treg-axis may be beneficial to reduce peritoneal injury.
Besides, the presence of AGEs has been hypothesized to be responsible for the proliferation of CD8+ lymphocytes (153, 173). It is believed that repeated exposure to the dialysate can lead to the recruitment and transformation of CD8+ naïve T cells into CD8+ effector memory cells (174).
The role and specific mechanism of mast cells in PD remain a topic of controversy. Several studies have reported an elevated presence of mast ocytes in samples obtained from PD patients (175). Additionally, both chronic renal failure rat models and PD rat models have shown a significant increase in mast cell numbers in the peritoneum (4, 176, 177). However, a preliminary investigation observed a decrease in mast cell quantification in peritoneal biopsies of patients (178). The influence of mast cells on inflammatory and fibrotic processes is depends on the timing, intensity, or nature of the damaging stimulus (179, 180). Mast cells that reside in the peritoneum have the potential to modulate the functioning of mesothelial cells through the release of their mediators, primarily histamine. This modulation occurs partly through calcium-dependent pathways and can affect the functionality of the peritoneum in the context of PD (181). In addition, mast cells play a role in the remodeling of omental tissue caused by PDFs, leading to the migration of peritoneal cells and the formation of adhesion (182).
Both hemodialysis and PD patients experience a significant decrease in the population of natural killer cells, which are essential components of the innate immune system (183). This highlights a strong link between ESRD and immune activation, as well as immune deficiency. Notably, another study indicates that the count of natural killer cells does not show any correlation with the duration of CAPD (184).
As previously discussed, TLRs, specifically TLR2 and TLR4, play a crucial role in the development of sterile peritoneal inflammation due to prolonged exposure to PDFs. However, inhibiting TLR2 has been shown to reduce the number of leukocytes in the peritoneum, particularly the infiltrated neutrophils (185). Furthermore, research suggests that the migration of neutrophil during inflammation is influenced by the compatibility of PDFs (101). Despite the documented biocompatibility of currently used catheters, there is extensive evidence of increased eosinophil counts in both peripheral blood and peritoneal fluid following catheter replacement. This response can range from a mild increase in asymptomatic individuals to a severe elevation that can ultimately lead to eosinophilic peritonitis (186–191). Eosinophilic peritonitis is characterized by aseptic inflammation, manifested as cloudy peritoneal dialysis effluent, mild clinical symptoms, negative dialysate culture, and lack of response to antibiotic treatment (191). Tissue invasion, resulting from infection and/or nonspecific stimulation of the PDFs, triggers the release of danger signals that activate eosinophils. This activation initiates the pathogenesis of the innate immune system, aimed at protecting the body but ultimately inducing tissue fibrosis (192).
The cellular and molecular mechanisms mentioned above emphasize the complex nature of the pathophysiological response observed in the peritoneum. The interaction between stromal resident cells and immune cells is mutually influential. Resident cells play an active role in influencing the recruitment, survival, and differentiation of immune cells, On the other hand, immune cells regulate the expression of proinflammatory factors and cytokines, which can cause damage to peritoneal resident cells.
Despite notable progress in PD in recent years, the issue of sterile inflammation remains a significant complication that can result in technique failure and unfavorable clinical outcomes. The repeated exposure to bioincompatible PDFs continues to be the primary cause of sterile inflammation. To overcome this challenge, future research efforts should focus on enhancing the biocompatibility of PDFs to improve peritoneal viability and extend the duration of PD therapy. Currently, no PDFs meet all the ideal solution requirements, which include efficient ultrafiltration, long-term preservation of the peritoneal membrane, and correction of nutritional and metabolic abnormalities.
However, the use of novel PDFs in conjunction has the potential to achieve these objectives. Initial findings from clinical investigations suggest that these biocompatible PDFs can provide comparable effectiveness to conventional regimens while also offering superior preservation of mesothelial cell mass (193). Furthermore, there are evidences to suggest that biocompatible PDFs can induce peritoneal inflammation and angiogenesis in children undergoing PD treatment (194, 195). The activation of peritoneal cells, mediators, and pathways can result in long-lasting functional and structural changes in the peritoneal membrane during long-term PD therapy. However, certain modifications may be reversible through the implementation of peritoneal rest (4, 196). The possibility of remesothelialization or healing depends on allowing the peritoneum to rest. During this resting period, pluripotent cells migrate to the surface and differentiate into fully developed mesothelial cells (197–199). Normal stem cells also play a crucial in tissue regeneration after injury, either by being mobilized from the bone marrow or already present in damaged tissues (147, 200). Furthermore, gene therapy can be used to modify the peritoneal membrane by targeting intervention to control inflammation, fibrosis and angiogenesis. These novel approaches show promising potential in preserving the integrity of the peritoneal membrane (201, 202). However, their clinical efficacy is still to be determined. Additionally, there is ongoing investigation into the potential clinical significance of using catheters infused with antimicrobial agents to prevent infections associated with PD (203). Consequently, we anticipate the potential use of catheters impregnated with anti-inflammatory medications as a therapeutic approach to reduce catheter-induced alterations and offer benefits to patients undergoing PD. However, it is important to conduct additional testing to ensure the safety and effectiveness of these PD tubes.
These events can potentially trigger both acute or chronic inflammation, resulting in damage to the peritoneal membrane and a gradual deterioration of its functioning. The role of mesothelial cells and other recruited cells is essential in initiating sterile inflammation and the subsequent decline of the peritoneal membrane. The inflammatory response is intricately intertwined with a complex network of extracellular signals produced by various types of cells residing or circulating within the peritoneal membrane. Here, we offer a thorough depiction of the key extracellular factors and cellular components that contribute to the communication between the immune system and peritoneal stromal cells. The exploration of the intricate cellular and molecular mechanisms involved in the sterile inflammation of the peritoneal membrane carries substantial significant in both fundamental research and clinical practices. This comprehension contributes to the development of therapeutic approaches focused on alleviating deterioration and reinstating the homeostasis of the peritoneal membrane.
HS: Writing – original draft. RZ: Writing – original draft. JS: Writing – review & editing. XC: Writing – review & editing. HY: Writing – review & editing. NA: Writing – review & editing. CY: Writing – review & editing. JT: Writing – review & editing. HL: Writing – review & editing. CY: Writing – review & editing.
The author(s) declare financial support was received for the research, authorship, and/or publication of this article. This work was supported by the Funds for the National Natural Science Foundation of China (grant numbers: 81700627, 81670654, and 81974095), National Clinical Key Specialty Construction Project (Institute of Nephrology, Affiliated Hospital of Guangdong Medical University), Science and Technology Innovation Strategy of Guangdong Province (grant numbers: 2023A1515030024 and 2021A1515011581), the Guangdong Medical Science and Technology Research Fund Project (grant numbers: A2023437), and the Science and Technology Planning Project of Zhanjiang City (grant number:2021A05067 and 2021A05083).
We thank Figdraw (www.figdraw.com) for figure drawing.
The authors declare that the research was conducted in the absence of any commercial or financial relationships that could be construed as a potential conflict of interest.
All claims expressed in this article are solely those of the authors and do not necessarily represent those of their affiliated organizations, or those of the publisher, the editors and the reviewers. Any product that may be evaluated in this article, or claim that may be made by its manufacturer, is not guaranteed or endorsed by the publisher.
1. Li PK, Chow KM, Van de Luijtgaarden MW, Johnson DW, Jager KJ, Mehrotra R, et al. Changes in the worldwide epidemiology of peritoneal dialysis. Nat Rev Nephrol. (2017) 13:90–103. doi: 10.1038/nrneph.2016.181
2. Velloso MS, Otoni A, de Paula Sabino A, de Castro WV, Pinto SW, Marinho MA, et al. Peritoneal dialysis and inflammation. Clinica chimica acta; Int J Clin Chem. (2014) 430:109–14. doi: 10.1016/j.cca.2013.12.003
3. Mehrotra R, Devuyst O, Davies SJ, Johnson DW. The current state of peritoneal dialysis. J Am Soc Nephrology: JASN. (2016) 27:3238–52. doi: 10.1681/asn.2016010112
4. Stavenuiter AW, Farhat K, Schilte MN, Ter Wee PM, Beelen RH. Bioincompatible impact of different peritoneal dialysis fluid components and therapeutic interventions as tested in a rat peritoneal dialysis model. Int J Nephrol. (2011) 2011:742196. doi: 10.4061/2011/742196
5. Balzer MS. Molecular pathways in peritoneal fibrosis. Cell signalling. (2020) 75:109778. doi: 10.1016/j.cellsig.2020.109778
6. Yeates K, Zhu N, Vonesh E, Trpeski L, Blake P, Fenton S. Hemodialysis and peritoneal dialysis are associated with similar outcomes for end-stage renal disease treatment in Canada. Nephrology dialysis transplantation: Off Publ Eur Dialysis Transplant Assoc - Eur Renal Assoc. (2012) 27:3568–75. doi: 10.1093/ndt/gfr674
7. Mehrotra R, Chiu YW, Kalantar-Zadeh K, Bargman J, Vonesh E. Similar outcomes with hemodialysis and peritoneal dialysis in patients with end-stage renal disease. Arch Internal Med. (2011) 171:110–8. doi: 10.1001/archinternmed.2010.352
8. Wong B, Ravani P, Oliver MJ, Holroyd-Leduc J, Venturato L, Garg AX, et al. Comparison of patient survival between hemodialysis and peritoneal dialysis among patients eligible for both modalities. Am J Kidney diseases: Off J Natl Kidney Foundation. (2018) 71:344–51. doi: 10.1053/j.ajkd.2017.08.028
9. Htay H, Johnson DW, Wiggins KJ, Badve SV, Craig JC, Strippoli GF, et al. Biocompatible dialysis fluids for peritoneal dialysis. Cochrane Database systematic Rev. (2018) 10(10):Cd007554. doi: 10.1002/14651858.CD007554.pub3
10. Morgan LW, Wieslander A, Davies M, Horiuchi T, Ohta Y, Beavis MJ, et al. Glucose degradation products (GDP) retard remesothelialization independently of D-glucose concentration. Kidney Int. (2003) 64:1854–66. doi: 10.1046/j.1523–1755.2003.00265.x
11. Topley N. Membrane longevity in peritoneal dialysis: impact of infection and bio-incompatible solutions. Adv Renal replacement Ther. (1998) 5:179–84. doi: 10.1016/s1073–4449(98)70030–5
12. Williams JD, Craig KJ, Topley N, Von Ruhland C, Fallon M, Newman GR, et al. Morphologic changes in the peritoneal membrane of patients with renal disease. J Am Soc Nephrology: JASN. (2002) 13:470–9. doi: 10.1681/asn.V132470
13. Witowski J, Wisniewska J, Korybalska K, Bender TO, Breborowicz A, Gahl GM, et al. Prolonged exposure to glucose degradation products impairs viability and function of human peritoneal mesothelial cells. J Am Soc Nephrology: JASN. (2001) 12:2434–41. doi: 10.1681/asn.V12112434
14. Su HY, Yang JJ, Zou R, An N, Chen XC, Yang C, et al. Autophagy in peritoneal fibrosis. Front Physiol. (2023) 14:1187207. doi: 10.3389/fphys.2023.1187207
15. Baroni G, Schuinski A, de Moraes TP, Meyer F, Pecoits-Filho R. Inflammation and the peritoneal membrane: causes and impact on structure and function during peritoneal dialysis. Mediators Inflammation. (2012) 2012:912595. doi: 10.1155/2012/912595
16. Lai KN, Leung JC. Inflammation in peritoneal dialysis. Nephron Clin Pract. (2010) 116:c11–8. doi: 10.1159/000314544
17. Terri M, Trionfetti F, Montaldo C, Cordani M, Tripodi M, Lopez-Cabrera M, et al. Mechanisms of peritoneal fibrosis: focus on immune cells-peritoneal stroma interactions. Front Immunol. (2021) 12:607204. doi: 10.3389/fimmu.2021.607204
18. van Baal JO, Van de Vijver KK, Nieuwland R, van Noorden CJ, van Driel WJ, Sturk A, et al. The histophysiology and pathophysiology of the peritoneum. Tissue Cell. (2017) 49:95–105. doi: 10.1016/j.tice.2016.11.004
19. Visser CE, Tekstra J, Brouwer-Steenbergen JJ, Tuk CW, Boorsma DM, Sampat-Sardjoepersad SC, et al. Chemokines produced by mesothelial cells: huGRO-alpha, IP-10, MCP-1 and RANTES. Clin Exp Immunol. (1998) 112:270–5. doi: 10.1046/j.1365–2249.1998.00592.x
20. Strippoli R, Benedicto I, Pérez Lozano ML, Cerezo A, López-Cabrera M, del Pozo MA. Epithelial-to-mesenchymal transition of peritoneal mesothelial cells is regulated by an ERK/NF-kappaB/Snail1 pathway. Dis Models Mech. (2008) 1:264–74. doi: 10.1242/dmm.001321
21. Lanfrancone L, Boraschi D, Ghiara P, Falini B, Grignani F, Peri G, et al. Human peritoneal mesothelial cells produce many cytokines (granulocyte colony-stimulating factor [CSF], granulocyte-monocyte-CSF, macrophage-CSF, interleukin-1 [IL-1], and IL-6) and are activated and stimulated to grow by IL-1. Blood. (1992) 80:2835–42.
22. Sosińska P, Baum E, Maćkowiak B, Staniszewski R, Jasinski T, Umezawa K, et al. Inhibition of NF-kappaB with Dehydroxymethylepoxyquinomicin modifies the function of human peritoneal mesothelial cells. Am J Trans Res. (2016) 8:5756–65.
23. Zhou G, Su X, Ma J, Wang L, Li D. Pioglitazone inhibits high glucose-induced synthesis of extracellular matrix by NF-κB and AP-1 pathways in rat peritoneal mesothelial cells. Mol Med Rep. (2013) 7:1336–42. doi: 10.3892/mmr.2013.1309
24. Santamaría B, Ucero AC, Benito-Martin A, Vicent MJ, Orzáez M, Celdrán A, et al. Biocompatibility reduces inflammation-induced apoptosis in mesothelial cells exposed to peritoneal dialysis fluid. Blood purification. (2015) 39:200–9. doi: 10.1159/000374103
25. Anders HJ, Schaefer L. Beyond tissue injury-damage-associated molecular patterns, toll-like receptors, and inflammasomes also drive regeneration and fibrosis. J Am Soc Nephrology: JASN. (2014) 25:1387–400. doi: 10.1681/asn.2014010117
26. Tang D, Kang R, Livesey KM, Cheh CW, Farkas A, Loughran P, et al. Endogenous HMGB1 regulates autophagy. J Cell Biol. (2010) 190:881–92. doi: 10.1083/jcb.200911078
27. Chen YC, Statt S, Wu R, Chang HT, Liao JW, Wang CN, et al. High mobility group box 1-induced epithelial mesenchymal transition in human airway epithelial cells. Sci Rep. (2016) 6:18815. doi: 10.1038/srep18815
28. Raby AC, González-Mateo GT, Williams A, Topley N, Fraser D, López-Cabrera M, et al. Targeting Toll-like receptors with soluble Toll-like receptor 2 prevents peritoneal dialysis solution-induced fibrosis. Kidney Int. (2018) 94:346–62. doi: 10.1016/j.kint.2018.03.014
29. Lechner M, Kratochwill K, Lichtenauer A, Rehulka P, Mayer B, Aufricht C, et al. A proteomic view on the role of glucose in peritoneal dialysis. J Proteome Res. (2010) 9:2472–9. doi: 10.1021/pr9011574
30. Kratochwill K, Lechner M, Lichtenauer AM, Herzog R, Lederhuber HC, Siehs C, et al. Interleukin-1 receptor-mediated inflammation impairs the heat shock response of human mesothelial cells. Am J Pathol. (2011) 178:1544–55. doi: 10.1016/j.ajpath.2010.12.034
31. Yung S, Chan TM. Pathophysiology of the peritoneal membrane during peritoneal dialysis: the role of hyaluronan. J biomedicine Biotechnol. (2011) 2011:180594. doi: 10.1155/2011/180594
32. Bender TO, Böhm M, Kratochwill K, Vargha R, Riesenhuber A, Witowski J, et al. Peritoneal dialysis fluids can alter HSP expression in human peritoneal mesothelial cells. Nephrology dialysis transplantation: Off Publ Eur Dialysis Transplant Assoc - Eur Renal Assoc. (2011) 26:1046–52. doi: 10.1093/ndt/gfq484
33. Chen GY, Nuñez G. Sterile inflammation: sensing and reacting to damage. Nat Rev Immunol. (2010) 10:826–37. doi: 10.1038/nri2873
34. Fielding CA, Jones GW, McLoughlin RM, McLeod L, Hammond VJ, Uceda J, et al. Interleukin-6 signaling drives fibrosis in unresolved inflammation. Immunity. (2014) 40:40–50. doi: 10.1016/j.immuni.2013.10.022
35. Kawasaki T, Kawai T. Toll-like receptor signaling pathways. Front Immunol. (2014) 5:461. doi: 10.3389/fimmu.2014.00461
36. Chu Y, Wang Y, Zheng Z, Lin Y, He R, Liu J, et al. Proinflammatory effect of high glucose concentrations on HMrSV5 cells via the autocrine effect of HMGB1. Front Physiol. (2017) 8:762. doi: 10.3389/fphys.2017.00762
37. Strippoli R, Moreno-Vicente R, Battistelli C, Cicchini C, Noce V, Amicone L, et al. Molecular mechanisms underlying peritoneal EMT and fibrosis. Stem Cells Int. (2016) 2016:3543678. doi: 10.1155/2016/3543678
38. Malarkey CS, Churchill ME. The high mobility group box: the ultimate utility player of a cell. Trends Biochem Sci. (2012) 37:553–62. doi: 10.1016/j.tibs.2012.09.003
39. Zhu N, Yuan W, Zhou Y, Liu J, Bao J, Hao J, et al. High mobility group box protein-1 correlates with microinflammatory state and nutritional status in continuous ambulatory peritoneal dialysis patients. J Artif organs: Off J Japanese Soc Artif Organs. (2011) 14:125–32. doi: 10.1007/s10047–011-0561–0
40. Cao S, Li S, Li H, Xiong L, Zhou Y, Fan J, et al. The potential role of HMGB1 release in peritoneal dialysis-related peritonitis. PloS One. (2013) 8:e54647. doi: 10.1371/journal.pone.0054647
41. Ramil-Gómez O, López-Pardo M, Fernández-Rodríguez JA, Rodríguez-Carmona A, Pérez-López T, Vaamonde-García C, et al. Involvement of mitochondrial dysfunction in the inflammatory response in human mesothelial cells from peritoneal dialysis effluent. Antioxidants. (2022) 11. doi: 10.3390/antiox11112184
42. López-Armada MJ, Riveiro-Naveira RR, Vaamonde-García C, Valcárcel-Ares MN. Mitochondrial dysfunction and the inflammatory response. Mitochondrion. (2013) 13:106–18. doi: 10.1016/j.mito.2013.01.003
43. Riley JS, Quarato G, Cloix C, Lopez J, O'Prey J, Pearson M, et al. Mitochondrial inner membrane permeabilisation enables mtDNA release during apoptosis. EMBO J. (2018) 37. doi: 10.15252/embj.201899238
44. Mills EL, Kelly B, O'Neill LAJ. Mitochondria are the powerhouses of immunity. Nat Immunol. (2017) 18:488–98. doi: 10.1038/ni.3704
45. Carvalho JTG, Schneider M, Cuppari L, Grabulosa CC, TA D, QR BM, et al. Cholecalciferol decreases inflammation and improves vitamin D regulatory enzymes in lymphocytes in the uremic environment: A randomized controlled pilot trial. PloS One. (2017) 12:e0179540. doi: 10.1371/journal.pone.0179540
46. Lorenz G, Darisipudi MN, Anders HJ. Canonical and non-canonical effects of the NLRP3 inflammasome in kidney inflammation and fibrosis. Nephrology dialysis transplantation: Off Publ Eur Dialysis Transplant Assoc - Eur Renal Assoc. (2014) 29:41–8. doi: 10.1093/ndt/gft332
47. Jo EK, Kim JK, Shin DM, Sasakawa C. Molecular mechanisms regulating NLRP3 inflammasome activation. Cell Mol Immunol. (2016) 13:148–59. doi: 10.1038/cmi.2015.95
48. Hautem N, Morelle J, Sow A, Corbet C, Feron O, Goffin E, et al. The NLRP3 inflammasome has a critical role in peritoneal dialysis-related peritonitis. J Am Soc Nephrology: JASN. (2017) 28:2038–52. doi: 10.1681/asn.2016070729
49. Wu J, Li X, Zhu G, Zhang Y, He M, Zhang J. The role of Resveratrol-induced mitophagy/autophagy in peritoneal mesothelial cells inflammatory injury via NLRP3 inflammasome activation triggered by mitochondrial ROS. Exp Cell Res. (2016) 341:42–53. doi: 10.1016/j.yexcr.2016.01.014
50. Ishibashi Y, Sugimoto T, Ichikawa Y, Akatsuka A, Miyata T, Nangaku M, et al. Glucose dialysate induces mitochondrial DNA damage in peritoneal mesothelial cells. Peritoneal Dialysis international: J Int Soc Peritoneal Dialysis. (2002) 22:11–21.
51. Hung KY, Liu SY, Yang TC, Liao TL, Kao SH. High-dialysate-glucose-induced oxidative stress and mitochondrial-mediated apoptosis in human peritoneal mesothelial cells. Oxid Med Cell Longevity. (2014) 2014:642793. doi: 10.1155/2014/642793
52. Helmke A, Hüsing AM, Gaedcke S, Brauns N, Balzer MS, Reinhardt M, et al. Peritoneal dialysate-range hypertonic glucose promotes T-cell IL-17 production that induces mesothelial inflammation. Eur J Immunol. (2021) 51:354–67. doi: 10.1002/eji.202048733
53. Wu J, Zhang YF, Li JS, Zhu GL, Bi ZM, Li XY. The effect of high glucose-based peritoneal dialysis fluids on thioredoxin-interacting protein expression in human peritoneal mesothelial cells. Int Immunopharmacol. (2019) 66:198–204. doi: 10.1016/j.intimp.2018.11.027
54. Ko J, Kang HJ, Kim DA, Ryu ES, Yu M, Lee H, et al. Paricalcitol attenuates TGF-β1-induced phenotype transition of human peritoneal mesothelial cells (HPMCs) via modulation of oxidative stress and NLRP3 inflammasome. FASEB journal: Off Publ Fed Am Societies Exp Biol. (2019) 33:3035–50. doi: 10.1096/fj.201800292RR
55. Nevado J, Peiró C, Vallejo S, El-Assar M, Lafuente N, Matesanz N, et al. Amadori adducts activate nuclear factor-kappaB-related proinflammatory genes in cultured human peritoneal mesothelial cells. Br J Pharmacol. (2005) 146:268–79. doi: 10.1038/sj.bjp.0706309
56. Matsuo H, Tamura M, Kabashima N, Serino R, Tokunaga M, Shibata T, et al. Prednisolone inhibits hyperosmolarity-induced expression of MCP-1 via NF-kappaB in peritoneal mesothelial cells. Kidney Int. (2006) 69:736–46. doi: 10.1038/sj.ki.5000131
57. Strippoli R, Benedicto I, Foronda M, Perez-Lozano ML, Sánchez-Perales S, López-Cabrera M, et al. p38 maintains E-cadherin expression by modulating TAK1-NF-kappa B during epithelial-to-mesenchymal transition. J Cell Sci. (2010) 123:4321–31. doi: 10.1242/jcs.071647
58. Zarubin T, Han J. Activation and signaling of the p38 MAP kinase pathway. Cell Res. (2005) 15:11–8. doi: 10.1038/sj.cr.7290257
59. Kato S, Yuzawa Y, Tsuboi N, Maruyama S, Morita Y, Matsuguchi T, et al. Endotoxin-induced chemokine expression in murine peritoneal mesothelial cells: the role of toll-like receptor 4. J Am Soc Nephrology: JASN. (2004) 15:1289–99.
60. Riesenhuber A, Kratochwill K, Bender TO, Vargha R, Kasper DC, Herzog R, et al. Peritoneal dialysis fluid induces p38-dependent inflammation in human mesothelial cells. Peritoneal Dialysis international: J Int Soc Peritoneal Dialysis. (2011) 31:332–9. doi: 10.3747/pdi.2009.00206
61. Zhang Y, Feng W, Peng X, Zhu L, Wang Z, Shen H, et al. Parthenolide alleviates peritoneal fibrosis by inhibiting inflammation via the NF-κB/ TGF-β/Smad signaling axis. Lab investigation; J Tech Methods Pathol. (2022) 102:1346–54. doi: 10.1038/s41374–022-00834–3
62. Wohlfert EA, Nichols FC, Nevius E, Clark RB. Peroxisome proliferator-activated receptor gamma (PPARgamma) and immunoregulation: enhancement of regulatory T cells through PPARgamma-dependent and -independent mechanisms. J Immunol. (2007) 178:4129–35. doi: 10.4049/jimmunol.178.7.4129
63. Hontecillas R, Bassaganya-Riera J. Peroxisome proliferator-activated receptor gamma is required for regulatory CD4+ T cell-mediated protection against colitis. J Immunol (Baltimore Md.: 1950). (2007) 178:2940–9. doi: 10.4049/jimmunol.178.5.2940
64. Sandoval P, Loureiro J, González-Mateo G, Pérez-Lozano ML, Maldonado-Rodríguez A, Sánchez-Tomero JA, et al. PPAR-γ agonist rosiglitazone protects peritoneal membrane from dialysis fluid-induced damage. Lab investigation; J Tech Methods Pathol. (2010) 90:1517–32. doi: 10.1038/labinvest.2010.111
65. Su X, Zhou G, Wang Y, Yang X, Li L, Yu R, et al. The PPARβ/δ agonist GW501516 attenuates peritonitis in peritoneal fibrosis via inhibition of TAK1-NFκB pathway in rats. Inflammation. (2014) 37:729–37. doi: 10.1007/s10753–013-9791-z
66. Luo Q, Hu Q, Zheng Q, Gong L, Su L, Ren B, et al. Enhanced mPGES-1 Contributes to PD-Related Peritoneal Fibrosis via Activation of the NLRP3 Inflammasome. Front Med. (2021) 8:675363. doi: 10.3389/fmed.2021.675363
67. Luo Q, Liu M, Tan Y, Chen J, Zhang W, Zhong S, et al. Blockade of prostaglandin E2 receptor 4 ameliorates peritoneal dialysis-associated peritoneal fibrosis. Front Pharmacol. (2022) 13:1004619. doi: 10.3389/fphar.2022.1004619
68. Liu Y, Dong Z, Liu H, Zhu J, Liu F, Chen G. Transition of mesothelial cell to fibroblast in peritoneal dialysis: EMT, stem cell or bystander? Peritoneal Dialysis international: J Int Soc Peritoneal Dialysis. (2015) 35:14–25. doi: 10.3747/pdi.2014.00188
69. Dai T, Wang Y, Nayak A, Nast CC, Quang L, LaPage J, et al. Janus kinase signaling activation mediates peritoneal inflammation and injury in vitro and in vivo in response to dialysate. Kidney Int. (2014) 86:1187–96. doi: 10.1038/ki.2014.209
70. Xu ZG, Kim KS, Park HC, Choi KH, Lee HY, Han DS, et al. High glucose activates the p38 MAPK pathway in cultured human peritoneal mesothelial cells. Kidney Int. (2003) 63:958–68. doi: 10.1046/j.1523–1755.2003.00836.x
71. Lee HB, Yu MR, Song JS, Ha H. Reactive oxygen species amplify protein kinase C signaling in high glucose-induced fibronectin expression by human peritoneal mesothelial cells. Kidney Int. (2004) 65:1170–9. doi: 10.1111/j.1523–1755.2004.00491.x
72. Yang X, Lin A, Jiang N, Yan H, Ni Z, Qian J, et al. Interleukin-6 trans-signalling induces vascular endothelial growth factor synthesis partly via Janus kinases-STAT3 pathway in human mesothelial cells. Nephrology. (2017) 22:150–8. doi: 10.1111/nep.12746
73. Barbano G, Cappa F, Prigione I, Tedesco F, Pausa M, Gugliemino R, et al. (1999). Peritoneal mesothelial cells produce complement factors and express CD59 that inhibits C5b-9-mediated cell lysis, in: Advances in peritoneal dialysis. Conference on Peritoneal Dialysis, , Vol. 15. pp. 253–7.
74. Tang S, Leung JC, Chan LY, Tsang AW, Chen CX, Zhou W, et al. Regulation of complement C3 and C4 synthesis in human peritoneal mesothelial cells by peritoneal dialysis fluid. Clin Exp Immunol. (2004) 136:85–94. doi: 10.1111/j.1365–2249.2004.02407.x
75. Young GA, Kendall S, Brownjohn AM. Complement activation during CAPD. Nephrology dialysis transplantation: Off Publ Eur Dialysis Transplant Assoc - Eur Renal Assoc. (1993) 8:1372–5.
76. Reddingius RE, Schröder CH, Daha MR, Willems HL, Koster AM, Monnens LA. Complement in serum and dialysate in children on continuous ambulatory peritoneal dialysis. Peritoneal Dialysis international: J Int Soc Peritoneal Dialysis. (1995) 15:49–53.
77. Zavvos V, Buxton AT, Evans C, Lambie M, Davies SJ, Topley N, et al. A prospective, proteomics study identified potential biomarkers of encapsulating peritoneal sclerosis in peritoneal effluent. Kidney Int. (2017) 92:988–1002. doi: 10.1016/j.kint.2017.03.030
78. Wang HY, Tian YF, Chien CC, Kan WC, Liao PC, Wu HY, et al. Differential proteomic characterization between normal peritoneal fluid and diabetic peritoneal dialysate. Nephrology dialysis transplantation: Off Publ Eur Dialysis Transplant Assoc - Eur Renal Assoc. (2010) 25:1955–63. doi: 10.1093/ndt/gfp696
79. Wen Q, Zhang L, Mao HP, Tang XQ, Rong R, Fan JJ, et al. Proteomic analysis in peritoneal dialysis patients with different peritoneal transport characteristics. Biochem Biophys Res Commun. (2013) 438:473–8. doi: 10.1016/j.bbrc.2013.07.116
80. Raaijmakers R, Pluk W, Schröder CH, Gloerich J, Cornelissen EA, Wessels HJ, et al. Proteomic profiling and identification in peritoneal fluid of children treated by peritoneal dialysis. Nephrology dialysis transplantation: Off Publ Eur Dialysis Transplant Assoc - Eur Renal Assoc. (2008) 23:2402–5. doi: 10.1093/ndt/gfn212
81. Oliveira E, Araújo JE, Gómez-Meire S, Lodeiro C, Perez-Melon C, Iglesias-Lamas E, et al. Proteomics analysis of the peritoneal dialysate effluent reveals the presence of calcium-regulation proteins and acute inflammatory response. Clin Proteomics. (2014) 11:17. doi: 10.1186/1559–0275-11–17
82. Kitterer D, Biegger D, Segerer S, Braun N, Alscher MD, Latus J. Alteration of membrane complement regulators is associated with transporter status in patients on peritoneal dialysis. PloS One. (2017) 12:e0177487. doi: 10.1371/journal.pone.0177487
83. Sei Y, Mizuno M, Suzuki Y, Imai M, Higashide K, Harris CL, et al. Expression of membrane complement regulators, CD46, CD55 and CD59, in mesothelial cells of patients on peritoneal dialysis therapy. Mol Immunol. (2015) 65:302–9. doi: 10.1016/j.molimm.2015.02.005
84. Borceux P, Morelle J, Goffin E. Complement system activation and peritoneal membrane alterations: Culprit or innocent bystander? Peritoneal Dialysis international: J Int Soc Peritoneal Dialysis. (2020) 40:115–23. doi: 10.1177/0896860819896242
85. Poppelaars F, Faria B, Gaya da Costa M, Franssen CFM, van Son WJ, Berger SP, et al. The complement system in dialysis: A forgotten story? Front Immunol. (2018) 9:71. doi: 10.3389/fimmu.2018.00071
86. Selgas R, Bajo A, Jiménez-Heffernan JA, Sánchez-Tomero JA, Del Peso G, Aguilera A, et al. Epithelial-to-mesenchymal transition of the mesothelial cell–its role in the response of the peritoneum to dialysis. Nephrology dialysis transplantation: Off Publ Eur Dialysis Transplant Assoc - Eur Renal Assoc. (2006) 21 Suppl 2:ii2–7. doi: 10.1093/ndt/gfl183
87. Margetts PJ, Bonniaud P. Basic mechanisms and clinical implications of peritoneal fibrosis. Peritoneal Dialysis international: J Int Soc Peritoneal Dialysis. (2003) 23:530–41.
88. Mandl-Weber S, Cohen CD, Haslinger B, Kretzler M, Sitter T. Vascular endothelial growth factor production and regulation in human peritoneal mesothelial cells. Kidney Int. (2002) 61:570–8. doi: 10.1046/j.1523–1755.2002.00143.x
89. Witowski J, Tayama H, Ksiazek K, Wanic-Kossowska M, Bender TO, Jörres A. Human peritoneal fibroblasts are a potent source of neutrophil-targeting cytokines: a key role of IL-1beta stimulation. Lab investigation; J Tech Methods Pathol. (2009) 89:414–24. doi: 10.1038/labinvest.2009.1
90. Kawka E, Witowski J, Fouqet N, Tayama H, Bender TO, Catar R, et al. Regulation of chemokine CCL5 synthesis in human peritoneal fibroblasts: a key role of IFN-γ. Mediators Inflammation. (2014) 2014:590654. doi: 10.1155/2014/590654
91. Kitterer D, Latus J, Ulmer C, Fritz P, Biegger D, Ott G, et al. Activation of nuclear factor of activated T cells 5 in the peritoneal membrane of uremic patients. Am J Physiol Renal Physiol. (2015) 308:F1247–58. doi: 10.1152/ajprenal.00617.2014
92. Roth I, Leroy V, Kwon HM, Martin PY, Féraille E, Hasler U. Osmoprotective transcription factor NFAT5/TonEBP modulates nuclear factor-kappaB activity. Mol Biol Cell. (2010) 21:3459–74. doi: 10.1091/mbc.E10–02-0133
93. Caballo C, Palomo M, Cases A, Galán AM, Molina P, Vera M, et al. NFκB in the development of endothelial activation and damage in uremia: an in vitro approach. PloS One. (2012) 7:e43374. doi: 10.1371/journal.pone.0043374
94. Rodrigues-Díez R, Aroeira LS, Orejudo M, Bajo MA, Heffernan JJ, Rodrigues-Díez RR, et al. IL-17A is a novel player in dialysis-induced peritoneal damage. Kidney Int. (2014) 86:303–15. doi: 10.1038/ki.2014.33
95. Witowski J, Kamhieh-Milz J, Kawka E, Catar R, Jörres A. IL-17 in peritoneal dialysis-associated inflammation and angiogenesis: conclusions and perspectives. Front Physiol. (2018) 9:1694. doi: 10.3389/fphys.2018.01694
96. Shi Y, Hu Y, Cui B, Zhuang S, Liu N. Vascular endothelial growth factor-mediated peritoneal neoangiogenesis in peritoneal dialysis. Peritoneal Dialysis international: J Int Soc Peritoneal Dialysis. (2022) 42:25–38. doi: 10.1177/08968608211004683
97. Salgado R, Benoy I, Weytjens R, Van Bockstaele D, Van Marck E, Huget P, et al. Arterio-venous gradients of IL-6, plasma and serum VEGF and D-dimers in human cancer. Br J Cancer. (2002) 87:1437–44. doi: 10.1038/sj.bjc.6600655
98. Feurino LW, Zhang Y, Bharadwaj U, Zhang R, Li F, Fisher WE, et al. IL-6 stimulates Th2 type cytokine secretion and upregulates VEGF and NRP-1 expression in pancreatic cancer cells. Cancer Biol Ther. (2007) 6:1096–100. doi: 10.4161/cbt.6.7.4328
99. de Lima SM, Otoni A, Sabino Ade P, Dusse LM, Gomes KB, Pinto SW, et al. Inflammation, neoangiogenesis and fibrosis in peritoneal dialysis. Clinica chimica acta; Int J Clin Chem. (2013) 421:46–50. doi: 10.1016/j.cca.2013.02.027
100. Bartosova M, Herzog R, Ridinger D, Levai E, Jenei H, Zhang C, et al. Alanyl-glutamine restores tight junction organization after disruption by a conventional peritoneal dialysis fluid. Biomolecules. (2020) 10. doi: 10.3390/biom10081178
101. Schilte MN, Fabbrini P, Wee PM, Keuning ED, Zareie M, Tangelder GJ, et al. Peritoneal dialysis fluid bioincompatibility and new vessel formation promote leukocyte-endothelium interactions in a chronic rat model for peritoneal dialysis. Microcirculation. (2010) 17:271–80. doi: 10.1111/j.1549–8719.2010.00024.x
102. Sacnun JM, Hoogenboom R, Eibensteiner F, Sobieszek IJ, Unterwurzacher M, Wagner A, et al. Proteome-wide differential effects of peritoneal dialysis fluid properties in an in vitro human endothelial cell model. Int J Mol Sci. (2022) 23. doi: 10.3390/ijms23148010
103. Yang WS, Tsai TJ, Shih CL, Huang JW, Chuang HF, Chen MH, et al. Intraperitoneal vascular endothelial growth factor C level is related to peritoneal dialysis ultrafiltration. Blood purification. (2009) 28:69–74. doi: 10.1159/000218008
104. Shang J, He Q, Chen Y, Yu D, Sun L, Cheng G, et al. miR-15a-5p suppresses inflammation and fibrosis of peritoneal mesothelial cells induced by peritoneal dialysis via targeting VEGFA. J Cell Physiol. (2019) 234:9746–55. doi: 10.1002/jcp.27660
105. Park SH, Do JY, Kim YH, Lee HY, Kim BS, Shin SK, et al. Effects of neutral pH and low-glucose degradation product-containing peritoneal dialysis fluid on systemic markers of inflammation and endothelial dysfunction: a randomized controlled 1-year follow-up study. Nephrology dialysis transplantation: Off Publ Eur Dialysis Transplant Assoc - Eur Renal Assoc. (2012) 27:1191–9. doi: 10.1093/ndt/gfr451
106. Bal Z, Uyar ME, Tutal E, Guliyev O, Sezer S, Haberal M. Body composition analysis of patients on waiting list for cadaveric renal transplantation: a comparison of hemodialysis and peritoneal dialysis patients. Transplant Proc. (2013) 45:3489–93. doi: 10.1016/j.transproceed.2013.08.097
107. Choi SJ, Kim NR, Hong SA, Lee WB, Park MY, Kim JK, et al. Changes in body fat mass in patients after starting peritoneal dialysis. Peritoneal Dialysis international: J Int Soc Peritoneal Dialysis. (2011) 31:67–73. doi: 10.3747/pdi.2009.00131
108. Rodríguez-Carmona A, Pérez-Fontán M, Guitián A, Peteiro J, García-Falcón T, López-Muñiz A, et al. Effect of low-GDP bicarbonate-lactate-buffered peritoneal dialysis solutions on plasma levels of adipokines and gut appetite-regulatory peptides. A randomized crossover study. Nephrology dialysis transplantation: Off Publ Eur Dialysis Transplant Assoc - Eur Renal Assoc. (2012) 27:369–74. doi: 10.1093/ndt/gfr244
109. Aoki S, Udo K, Morimoto H, Ikeda S, Takezawa T, Uchihashi K, et al. Adipose tissue behavior is distinctly regulated by neighboring cells and fluid flow stress: a possible role of adipose tissue in peritoneal fibrosis. J Artif organs: Off J Japanese Soc Artif Organs. (2013) 16:322–31. doi: 10.1007/s10047–013-0702–8
110. Achike FI, To NH, Wang H, Kwan CY. Obesity, metabolic syndrome, adipocytes and vascular function: A holistic viewpoint. Clin Exp Pharmacol Physiol. (2011) 38:1–10. doi: 10.1111/j.1440–1681.2010.05460.x
111. Garonna E, Botham KM, Birdsey GM, Randi AM, Gonzalez-Perez RR, Wheeler-Jones CP. Vascular endothelial growth factor receptor-2 couples cyclo-oxygenase-2 with pro-angiogenic actions of leptin on human endothelial cells. PloS One. (2011) 6:e18823. doi: 10.1371/journal.pone.0018823
112. Leung JC, Chan LY, Lam MF, Tang SC, Chow CW, Lim AI, et al. The role of leptin and its short-form receptor in inflammation in db/db mice infused with peritoneal dialysis fluid. Nephrology dialysis transplantation: Off Publ Eur Dialysis Transplant Assoc - Eur Renal Assoc. (2012) 27:3119–29. doi: 10.1093/ndt/gfr774
113. Costacou T, Orchard TJ. Adiponectin: good, bad, or just plain ugly? Kidney Int. (2008) 74:549–51. doi: 10.1038/ki.2008.262
114. Teta D, Maillard M, Halabi G, Burnier M. The leptin/adiponectin ratio: potential implications for peritoneal dialysis. Kidney Int Supplement. (2008) 108):S112–8. doi: 10.1038/sj.ki.5002611
115. He Y, Lu L, Wei X, Jin D, Qian T, Yu A, et al. The multimerization and secretion of adiponectin are regulated by TNF-alpha. Endocrine. (2016) 51:456–68. doi: 10.1007/s12020–015-0741–4
116. Shi J, Yu M, Sheng M. Angiogenesis and inflammation in peritoneal dialysis: the role of adipocytes. Kidney Blood Pressure Res. (2017) 42:209–19. doi: 10.1159/000476017
117. Weisberg SP, McCann D, Desai M, Rosenbaum M, Leibel RL, Ferrante AW Jr. Obesity is associated with macrophage accumulation in adipose tissue. J Clin Invest. (2003) 112:1796–808. doi: 10.1172/jci19246
118. Lee CT, Ng HY, Hsu CY, Tsai YC, Yang YK, Chen TC, et al. Proinflammatory cytokines, hepatocyte growth factor and adipokines in peritoneal dialysis patients. Artif organs. (2010) 34:E222–9. doi: 10.1111/j.1525–1594.2010.01000.x
119. Taskapan MC, Taskapan H, Sahin I, Keskin L, Atmaca H, Ozyalin F. Serum leptin, resistin, and lipid levels in patients with end stage renal failure with regard to dialysis modality. Renal failure. (2007) 29:147–54. doi: 10.1080/08860220601095827
120. Litbarg NO, Gudehithlu KP, Sethupathi P, Arruda JA, Dunea G, Singh AK. Activated omentum becomes rich in factors that promote healing and tissue regeneration. Cell Tissue Res. (2007) 328:487–97. doi: 10.1007/s00441–006-0356–4
121. Gimble JM, Katz AJ, Bunnell BA. Adipose-derived stem cells for regenerative medicine. Circ Res. (2007) 100:1249–60. doi: 10.1161/01.Res.0000265074.83288.09
122. Wu Z, Zuo X, Wang X, Shi M, Zhu H, Cao C, et al. The probiotic Lactobacillus casei Zhang-mediated correction of gut dysbiosis ameliorates peritoneal fibrosis by suppressing macrophage-related inflammation via the butyrate/PPAR-γ/NF-κB pathway. Food Funct. (2023) 14:6840–52. doi: 10.1039/d3fo01518a
123. Capobianco A, Cottone L, Monno A, Manfredi AA, Rovere-Querini P. The peritoneum: healing, immunity, and diseases. J Pathol. (2017) 243:137–47. doi: 10.1002/path.4942
124. Zhao Y, Zou W, Du J, Zhao Y. The origins and homeostasis of monocytes and tissue-resident macrophages in physiological situation. J Cell Physiol. (2018) 233:6425–39. doi: 10.1002/jcp.26461
125. Sutherland TE, Shaw TN, Lennon R, Herrick SE, Rückerl D. Ongoing exposure to peritoneal dialysis fluid alters resident peritoneal macrophage phenotype and activation propensity. Front Immunol. (2021) 12:715209. doi: 10.3389/fimmu.2021.715209
126. Habib SM, Abrahams AC, Korte MR, Zietse R, de Vogel LL, Boer WH, et al. CD4-positive T cells and M2 macrophages dominate the peritoneal infiltrate of patients with encapsulating peritoneal sclerosis. PloS One. (2015) 10:e0120174. doi: 10.1371/journal.pone.0120174
127. Liao CT, Andrews R, Wallace LE, Khan MW, Kift-Morgan A, Topley N, et al. Peritoneal macrophage heterogeneity is associated with different peritoneal dialysis outcomes. Kidney Int. (2017) 91:1088–103. doi: 10.1016/j.kint.2016.10.030
128. Yokoi H, Kasahara M, Mori K, Ogawa Y, Kuwabara T, Imamaki H, et al. Pleiotrophin triggers inflammation and increased peritoneal permeability leading to peritoneal fibrosis. Kidney Int. (2012) 81:160–9. doi: 10.1038/ki.2011.305
129. Wang L, Balzer MS, Rong S, Menne J, von Vietinghoff S, Dong L, et al. Protein kinase C α inhibition prevents peritoneal damage in a mouse model of chronic peritoneal exposure to high-glucose dialysate. Kidney Int. (2016) 89:1253–67. doi: 10.1016/j.kint.2016.01.025
130. Zhou Y, Zhang T, Wang X, Wei X, Chen Y, Guo L, et al. Curcumin modulates macrophage polarization through the inhibition of the toll-like receptor 4 expression and its signaling pathways. Cell Physiol biochemistry: Int J Exp Cell physiology biochemistry Pharmacol. (2015) 36:631–41. doi: 10.1159/000430126
131. Choi SY, Ryu HM, Choi JY, Cho JH, Kim CD, Kim YL, et al. The role of Toll-like receptor 4 in high-glucose-induced inflammatory and fibrosis markers in human peritoneal mesothelial cells. Int Urol Nephrol. (2017) 49:171–81. doi: 10.1007/s11255–016-1430–9
132. Wang W, Ai J, Liao B, Xiao K, Lin L, Chen H, et al. The roles of MCP-1/CCR2 mediated macrophage recruitment and polarization in bladder outlet obstruction (BOO) induced bladder remodeling. Int Immunopharmacol. (2021) 99:107947. doi: 10.1016/j.intimp.2021.107947
133. Chen G, Hou Y, Li X, Pan R, Zhao D. Sepsis-induced acute lung injury in young rats is relieved by calycosin through inactivating the HMGB1/MyD88/NF-κB pathway and NLRP3 inflammasome. Int Immunopharmacol. (2021) 96:107623. doi: 10.1016/j.intimp.2021.107623
134. Ranzinger J, Rustom A, Schwenger V. Membrane nanotubes between peritoneal mesothelial cells: functional connectivity and crucial participation during inflammatory reactions. Front Physiol. (2014) 5:412. doi: 10.3389/fphys.2014.00412
135. Kadoya H, Hirano A, Umeno R, Kajimoto E, Iwakura T, Kondo M, et al. Activation of the inflammasome drives peritoneal deterioration in a mouse model of peritoneal fibrosis. FASEB journal: Off Publ Fed Am Societies Exp Biol. (2023) 37:e23129. doi: 10.1096/fj.202201777RRR
136. Kadoya H, Satoh M, Sasaki T, Taniguchi S, Takahashi M, Kashihara N. Excess aldosterone is a critical danger signal for inflammasome activation in the development of renal fibrosis in mice. FASEB journal: Off Publ Fed Am Societies Exp Biol. (2015) 29:3899–910. doi: 10.1096/fj.15–271734
137. Duffield JS. Macrophages and immunologic inflammation of the kidney. Semin Nephrol. (2010) 30:234–54. doi: 10.1016/j.semnephrol.2010.03.003
138. Wynn TA, Barron L. Macrophages: master regulators of inflammation and fibrosis. Semin liver Dis. (2010) 30:245–57. doi: 10.1055/s-0030–1255354
139. Amorim A, De Feo D, Friebel E, Ingelfinger F, Anderfuhren CD, Krishnarajah S, et al. IFNγ and GM-CSF control complementary differentiation programs in the monocyte-to-phagocyte transition during neuroinflammation. Nat Immunol. (2022) 23:217–28. doi: 10.1038/s41590–021-01117–7
140. Cao Q, Wang Y, Harris DC. Macrophage heterogeneity, phenotypes, and roles in renal fibrosis. Kidney Int Suppl. (2014) 4:16–9. doi: 10.1038/kisup.2014.4
141. Cao Q, Harris DC, Wang Y. Macrophages in kidney injury, inflammation, and fibrosis. Physiol (Bethesda Md.). (2015) 30:183–94. doi: 10.1152/physiol.00046.2014
142. Balzer MS, Helmke A, Ackermann M, Casper J, Dong L, Hiss M, et al. Protein kinase C beta deficiency increases glucose-mediated peritoneal damage via M1 macrophage polarization and up-regulation of mesothelial protein kinase C alpha. Nephrology dialysis transplantation: Off Publ Eur Dialysis Transplant Assoc - Eur Renal Assoc. (2019) 34:947–60. doi: 10.1093/ndt/gfy282
143. Shi J, Li Q, Sheng M, Zheng M, Yu M, Zhang L. The role of TLR4 in M1 macrophage-induced epithelial-mesenchymal transition of peritoneal mesothelial cells. Cell Physiol biochemistry: Int J Exp Cell physiology biochemistry Pharmacol. (2016) 40:1538–48. doi: 10.1159/000453204
144. Hu W, Jiang Z, Zhang Y, Liu Q, Fan J, Luo N, et al. Characterization of infiltrating macrophages in high glucose-induced peritoneal fibrosis in rats. Mol Med Rep. (2012) 6:93–9. doi: 10.3892/mmr.2012.890
145. Shao Q, Jiang C, Zhang Q, Liu J, Jin B, Zhao M, et al. Knockdown of AK142426 suppresses M2 macrophage polarization and inflammation in peritoneal fibrosis via binding to c-Jun. J Gene Med. (2023) 25:e3524. doi: 10.1002/jgm.3524
146. Vila Cuenca M, Keuning ED, Talhout W, Paauw NJ, van Ittersum FJ, Ter Wee PM, et al. Differences in peritoneal response after exposure to low-GDP bicarbonate/lactate-buffered dialysis solution compared to conventional dialysis solution in a uremic mouse model. Int Urol Nephrol. (2018) 50:1151–61. doi: 10.1007/s11255–018-1872–3
147. Yang CY, Chang PY, Chen JY, Wu BS, Yang AH, Lee OK. Adipose-derived mesenchymal stem cells attenuate dialysis-induced peritoneal fibrosis by modulating macrophage polarization via interleukin-6. Stem Cell Res Ther. (2021) 12:193. doi: 10.1186/s13287–021-02270–4
148. Li Z, Zhang L, He W, Zhu C, Yang J, Sheng M. Astragalus membranaceus inhibits peritoneal fibrosis via monocyte chemoattractant protein (MCP)-1 and the transforming growth factor-β1 (TGF-β1) pathway in rats submitted to peritoneal dialysis. Int J Mol Sci. (2014) 15:12959–71. doi: 10.3390/ijms150712959
149. Yoshimine H, Tanoue S, Ibi Y, Minami M, Nakahara M, Tokunaga K, et al. Hepatocyte growth factor ameliorates methylglyoxal-induced peritoneal inflammation and fibrosis in mouse model. Clin Exp Nephrol. (2021) 25:935–43. doi: 10.1007/s10157–021-02067-y
150. Viau M, Zouali M. B-lymphocytes, innate immunity, and autoimmunity. Clin Immunol. (2005) 114:17–26. doi: 10.1016/j.clim.2004.08.019
151. Liappas G, Gónzalez-Mateo GT, Majano P, Sánchez-Tomero JA, Ruiz-Ortega M, Rodrigues Díez R, et al. T helper 17/regulatory T cell balance and experimental models of peritoneal dialysis-induced damage. BioMed Res Int. (2015) 2015:416480. doi: 10.1155/2015/416480
152. Griveas I, Fleva A, Karanikas E, Gogos K, Sakellariou G. CD4/CD8 T-cell ratio in peritoneal dialysis effluents predicts the outcome of peritonitis in patients undergoing continuous ambulatory peritoneal dialysis. Artif organs. (2009) 33:1091–5. doi: 10.1111/j.1525–1594.2009.00802.x
153. Griveas I, Visvardis G, Fleva A, Papadopoulou D, Mitsopoulos E, Nikopoulos K, et al. Lymphocytes subsets in the course of continuous ambulatory peritoneal dialysis (CAPD). Renal failure. (2004) 26:641–6. doi: 10.1081/jdi-200037156
154. Mosmann TR, Coffman RL. TH1 and TH2 cells: different patterns of lymphokine secretion lead to different functional properties. Annu Rev Immunol. (1989) 7:145–73. doi: 10.1146/annurev.iy.07.040189.001045
155. Yokoyama T, Nitta K, Futatsuyama K, Hayashi T, Honda K, Uchida K, et al. Identification of T helper cell subsets in continuous ambulatory peritoneal dialysis patients. Nephron. (2001) 89:215–8. doi: 10.1159/000046070
156. Stenvinkel P, Ketteler M, Johnson RJ, Lindholm B, Pecoits-Filho R, Riella M, et al. IL-10, IL-6, and TNF-alpha: central factors in the altered cytokine network of uremia–the good, the bad, and the ugly. Kidney Int. (2005) 67:1216–33. doi: 10.1111/j.1523–1755.2005.00200.x
157. Libetta C, Rampino T, Dal Canton A. Polarization of T-helper lymphocytes toward the Th2 phenotype in uremic patients. Am J Kidney diseases: Off J Natl Kidney Foundation. (2001) 38:286–95. doi: 10.1053/ajkd.2001.26092
158. Zamauskaite A, Yaqoob MM, Madrigal JA, Cohen SB. The frequency of Th2 type cells increases with time on peritoneal dialysis in patients with diabetic nephropathy. Eur Cytokine network. (1999) 10:219–26.
159. González-Mateo GT, Loureiro J, Jiménez-Hefferman JA, Bajo MA, Selgas R, López-Cabrera M, et al. Chronic exposure of mouse peritoneum to peritoneal dialysis fluid: structural and functional alterations of the peritoneal membrane. Peritoneal Dialysis international: J Int Soc Peritoneal Dialysis. (2009) 29:227–30.
160. Liappas G, González-Mateo GT, Sánchez-Díaz R, Lazcano JJ, Lasarte S, Matesanz-Marín A, et al. Immune-regulatory molecule CD69 controls peritoneal fibrosis. J Am Soc Nephrology: JASN. (2016) 27:3561–76. doi: 10.1681/asn.2015080909
161. Martín P, Gómez M, Lamana A, Cruz-Adalia A, Ramírez-Huesca M, Ursa MA, et al. CD69 association with Jak3/Stat5 proteins regulates Th17 cell differentiation. Mol Cell Biol. (2010) 30:4877–89. doi: 10.1128/mcb.00456–10
162. Raby AC, Labéta MO. Preventing peritoneal dialysis-associated fibrosis by therapeutic blunting of peritoneal toll-like receptor activity. Front Physiol. (2018) 9:1692. doi: 10.3389/fphys.2018.01692
163. Perez J, Dansou B, Hervé R, Levi C, Tamouza H, Vandermeersch S, et al. Calpains released by T lymphocytes cleave TLR2 to control IL-17 expression. J Immunol. (2016) 196:168–81. doi: 10.4049/jimmunol.1500749
164. Kumar P, Natarajan K, Shanmugam N. High glucose driven expression of pro-inflammatory cytokine and chemokine genes in lymphocytes: molecular mechanisms of IL-17 family gene expression. Cell signalling. (2014) 26:528–39. doi: 10.1016/j.cellsig.2013.11.031
165. Maksic D, Vasilijic S, Colic M, Stankovic-Popovic V, Bokonjic D. (2009). Systemic and intraperitoneal proinflammatory cytokine profiles in patients on continuous ambulatory peritoneal dialysis, in: Advances in peritoneal dialysis. Conference on Peritoneal Dialysis, , Vol. 25. pp. 50–5.
166. Maksić D, Colić M, Stanković-Popović V, Radojević M, Bokonjić D. Systemic and intraperitoneal proinflammatory cytokines profiles in patients on chronic peritoneal dialysis. Medicinski pregled. (2007) 60 Suppl 2:53–7.
167. Witowski J, Pawlaczyk K, Breborowicz A, Scheuren A, Kuzlan-Pawlaczyk M, Wisniewska J, et al. IL-17 stimulates intraperitoneal neutrophil infiltration through the release of GRO alpha chemokine from mesothelial cells. J Immunol. (2000) 165:5814–21. doi: 10.4049/jimmunol.165.10.5814
168. Witowski J, Ksiazek K, Warnecke C, Kuźlan M, Korybalska K, Tayama H, et al. Role of mesothelial cell-derived granulocyte colony-stimulating factor in interleukin-17-induced neutrophil accumulation in the peritoneum. Kidney Int. (2007) 71:514–25. doi: 10.1038/sj.ki.5002082
169. Catar R, Witowski J, Zhu N, Lücht C, Derrac Soria A, Uceda Fernandez J, et al. IL-6 trans-signaling links inflammation with angiogenesis in the peritoneal membrane. J Am Soc Nephrology: JASN. (2017) 28:1188–99. doi: 10.1681/asn.2015101169
170. González-Mateo GT, Fernández-Míllara V, Bellón T, Liappas G, Ruiz-Ortega M, López-Cabrera M, et al. Paricalcitol reduces peritoneal fibrosis in mice through the activation of regulatory T cells and reduction in IL-17 production. PloS One. (2014) 9:e108477. doi: 10.1371/journal.pone.0108477
171. Ferrantelli E, Liappas G, Vila Cuenca M, Keuning ED, Foster TL, Vervloet MG, et al. The dipeptide alanyl-glutamine ameliorates peritoneal fibrosis and attenuates IL-17 dependent pathways during peritoneal dialysis. Kidney Int. (2016) 89:625–35. doi: 10.1016/j.kint.2015.12.005
172. Marchant V, Tejera-Muñoz A, Marquez-Expósito L, Rayego-Mateos S, Rodrigues-Diez RR, Tejedor L, et al. IL-17A as a potential therapeutic target for patients on peritoneal dialysis. Biomolecules. (2020) 10. doi: 10.3390/biom10101361
173. Glik A, Douvdevani A. T lymphocytes: the "cellular" arm of acquired immunity in the peritoneum. Peritoneal Dialysis international: J Int Soc Peritoneal Dialysis. (2006) 26:438–48.
174. Ohashi A, Yamanishi A, Kondo M, Ihara F, Tanaka T, Maeda Y. Transition of lymphocyte subsets in peritoneal dialysis effluent and its relationship to peritoneal damage. J Rural medicine: JRM. (2021) 16:200–5. doi: 10.2185/jrm.2021–009
175. Alscher DM, Braun N, Biegger D, Fritz P. Peritoneal mast cells in peritoneal dialysis patients, particularly in encapsulating peritoneal sclerosis patients. Am J Kidney diseases: Off J Natl Kidney Foundation. (2007) 49:452–61. doi: 10.1053/j.ajkd.2006.11.040
176. Zareie M, Hekking LH, Driesprong BA, ter Wee PM, Beelen RH, van den Born J. Accumulation of omental mast cells during peritoneal dialysis. Peritoneal Dialysis international: J Int Soc Peritoneal Dialysis. (2001) 21 Suppl 3:S373–6. doi: 10.1177/089686080102103S71
177. Kazama I, Baba A, Endo Y, Toyama H, Ejima Y, Matsubara M, et al. Mast cell involvement in the progression of peritoneal fibrosis in rats with chronic renal failure. Nephrology. (2015) 20:609–16. doi: 10.1111/nep.12489
178. Jiménez-Heffernan JA, Bajo MA, Perna C, del Peso G, Larrubia JR, Gamallo C, et al. Mast cell quantification in normal peritoneum and during peritoneal dialysis treatment. Arch Pathol Lab Med. (2006) 130:1188–92. doi: 10.5858/2006–130-1188-mcqinp
179. Krystel-Whittemore M, Dileepan KN, Wood JG. Mast cell: A multi-functional master cell. Front Immunol. (2015) 6:620. doi: 10.3389/fimmu.2015.00620
180. Bradding P, Pejler G. The controversial role of mast cells in fibrosis. Immunol Rev. (2018) 282:198–231. doi: 10.1111/imr.12626
181. Bird SD, Walker RJ. Mast cell histamine-induced calcium transients in cultured human peritoneal mesothelial cells. Peritoneal Dialysis international: J Int Soc Peritoneal Dialysis. (1998) 18:626–36.
182. Zareie M, Fabbrini P, Hekking LH, Keuning ED, Ter Wee PM, Beelen RH, et al. Novel role for mast cells in omental tissue remodeling and cell recruitment in experimental peritoneal dialysis. J Am Soc Nephrology: JASN. (2006) 17:3447–57. doi: 10.1681/asn.2005111173
183. Peraldi MN, Berrou J, Métivier F, Toubert A. Natural killer cell dysfunction in uremia: the role of oxidative stress and the effects of dialysis. Blood purification. (2013) 35 Suppl 2:14–9. doi: 10.1159/000350839
184. Leander M, Grzegorzewska AE. Natural killer cells in continuous ambulatory peritoneal dialysis patients. Nephrology dialysis transplantation: Off Publ Eur Dialysis Transplant Assoc - Eur Renal Assoc. (2009) 24:1696–7. doi: 10.1093/ndt/gfp097
185. Raby AC, Colmont CS, Kift-Morgan A, Köhl J, Eberl M, Fraser D, et al. Toll-like receptors 2 and 4 are potential therapeutic targets in peritoneal dialysis-associated fibrosis. J Am Soc Nephrology: JASN. (2017) 28:461–78. doi: 10.1681/asn.2015080923
186. Wang Z, Li Z, Luo S, Yang Z, Xing Y, Pu C, et al. Cefoperazone and sulbactam-related eosinophilic peritonitis: a case report and literature review. J Int Med Res. (2021) 49:3000605211025367. doi: 10.1177/03000605211025367
187. Kenan BU, Büyükkaragöz B, Leventoğlu E, Bakkaloğlu SA. Eosinophilic peritonitis in children undergoing maintenance peritoneal dialysis: A case report and literature review. Semin Dialysis. (2022) 35:548–55. doi: 10.1111/sdi.13113
188. Uchiyama K, Washida N, Kusahana E, Nakayama T, Morimoto K, Itoh H. Eosinophilic reaction at the time of catheter insertion predicts survival in patients initiating peritoneal dialysis. Blood purification. (2022) 51:355–64. doi: 10.1159/000517349
189. Cetin N, Sav NM, Ciftci E, Yildiz B. Foreign body reaction to dialysis chatheter and peritoneal fluid eosinophilia in a child on continuous ambulatory peritoneal dialysis. Iranian J Kidney Dis. (2017) 11:319–21.
190. Shigemoto E, Mizuno M, Suzuki Y, Kobayashi K, Sakata F, Kariya T, et al. Increase of eosinophil in dialysate during induction of peritoneal dialysis. Peritoneal Dialysis international: J Int Soc Peritoneal Dialysis. (2019) 39:90–2. doi: 10.3747/pdi.2017.00205
191. Qingyan Z, Yangyang X, Miao Z, Chunming J. Peritoneal dialysis related eosinophilic peritonitis: a case report and review of the literature. BMC Nephrol. (2023) 24:10. doi: 10.1186/s12882–022-03027–8
192. Kobayashi T, Noguchi T, Saito K, Shirasaki F, Kita H, Nagata M, et al. Eosinophilic inflammation in peritoneal fibrosis patients undergoing peritoneal dialysis. Contributions to Nephrol. (2018) 196:1–4. doi: 10.1159/000485689
193. Saxena R. Pathogenesis and treatment of peritoneal membrane failure. Pediatr Nephrol. (2008) 23:695–703. doi: 10.1007/s00467–007-0580–5
194. Bartosova M, Zhang C, Schaefer B, Herzog R, Ridinger D, Damgov I, et al. Glucose derivative induced vasculopathy in children on chronic peritoneal dialysis. Circ Res. (2021) 129:e102–18. doi: 10.1161/circresaha.121.319310
195. Schaefer B, Bartosova M, Macher-Goeppinger S, Sallay P, Vörös P, Ranchin B, et al. Neutral pH and low-glucose degradation product dialysis fluids induce major early alterations of the peritoneal membrane in children on peritoneal dialysis. Kidney Int. (2018) 94:419–29. doi: 10.1016/j.kint.2018.02.022
196. Zareie M, Keuning ED, ter Wee PM, Beelen RH, van den Born J. Peritoneal dialysis fluid-induced changes of the peritoneal membrane are reversible after peritoneal rest in rats. Nephrology dialysis transplantation: Off Publ Eur Dialysis Transplant Assoc - Eur Renal Assoc. (2005) 20:189–93. doi: 10.1093/ndt/gfh559
197. Herrick SE, Mutsaers SE. Mesothelial progenitor cells and their potential in tissue engineering. Int J Biochem Cell Biol. (2004) 36:621–42. doi: 10.1016/j.biocel.2003.11.002
198. Lai KN, Leung JC. Peritoneal adipocytes and their role in inflammation during peritoneal dialysis. Mediators Inflammation. (2010) 2010:495416. doi: 10.1155/2010/495416
199. Mutsaers SE, Prêle CM, Lansley SM, Herrick SE. The origin of regenerating mesothelium: a historical perspective. Int J Artif organs. (2007) 30:484–94. doi: 10.1177/039139880703000606
200. Yu F, Yang J, Chen J, Wang X, Cai Q, He Y, et al. Bone marrow mesenchymal stem cell-derived exosomes alleviate peritoneal dialysis-associated peritoneal injury. Stem Cells Dev. (2023) 32:197–211. doi: 10.1089/scd.2022.0244
201. Li XJ, Sun L, Xiao L, Liu FY. Gene delivery in peritoneal dialysis related peritoneal fibrosis research. Chin Med J. (2012) 125:2219–24.
202. Igarashi Y, Hoshino T, Ookawara S, Ishibashi K, Morishita Y. Nano-sized carriers in gene therapy for peritoneal fibrosis in vivo. Nano Rev experiments. (2017) 8:1331100. doi: 10.1080/20022727.2017.1331100
Keywords: peritoneal dialysis fluids, non-bioincompatible, sterile inflammation, immune cells, mesothelial cells
Citation: Su H-Y, Zou R, Su J-Q, Chen X-C, Yang H-J, An N, Yang C, Tang J-X, Liu H-F and Yao C-W (2024) Sterile inflammation of peritoneal membrane caused by peritoneal dialysis: focus on the communication between immune cells and peritoneal stroma. Front. Immunol. 15:1387292. doi: 10.3389/fimmu.2024.1387292
Received: 17 February 2024; Accepted: 16 April 2024;
Published: 08 May 2024.
Edited by:
Raffaele Strippoli, Sapienza University of Rome, ItalyReviewed by:
Maria Bartosova, Heidelberg University Hospital, GermanyCopyright © 2024 Su, Zou, Su, Chen, Yang, An, Yang, Tang, Liu and Yao. This is an open-access article distributed under the terms of the Creative Commons Attribution License (CC BY). The use, distribution or reproduction in other forums is permitted, provided the original author(s) and the copyright owner(s) are credited and that the original publication in this journal is cited, in accordance with accepted academic practice. No use, distribution or reproduction is permitted which does not comply with these terms.
*Correspondence: Huafeng Liu, bGl1aGZAZ2RtdS5lZHUuY24=; Cuiwei Yao, eWFvY3Vpd2VpQGdkbXUuZWR1LmNu
†These authors have contributed equally to this work
Disclaimer: All claims expressed in this article are solely those of the authors and do not necessarily represent those of their affiliated organizations, or those of the publisher, the editors and the reviewers. Any product that may be evaluated in this article or claim that may be made by its manufacturer is not guaranteed or endorsed by the publisher.
Research integrity at Frontiers
Learn more about the work of our research integrity team to safeguard the quality of each article we publish.