- 1Department of Dermatology, National Center for Tumor Diseases Heidelberg (NCT), Heidelberg, Germany
- 2Institute for Tumor Biology, University Medical Center Hamburg-Eppendorf, Hamburg, Germany
Tissue-resident memory T cells (TRM cells) have become an interesting subject of study for antitumor immunity in melanoma and other solid tumors. In the initial phases of antitumor immunity, they maintain an immune equilibrium and protect against challenges with tumor cells and the formation of primary melanomas. In metastatic settings, they are a prime target cell population for immune checkpoint inhibition (ICI) because they highly express inhibitory checkpoint molecules such as PD-1, CTLA-4, or LAG-3. Once melanoma patients are treated with ICI, TRM cells residing in the tumor are reactivated and expand. Tumor killing is achieved by secreting effector molecules such as IFN-γ. However, off-target effects are also observed. Immune-related adverse events, such as those affecting barrier organs like the skin, can be mediated by ICI-induced TRM cells. Therefore, a detailed understanding of this memory T-cell type is obligatory to better guide and improve immunotherapy regimens.
Introduction
Immunotherapy-based therapies have ushered in an era of unprecedented improvement in the prognosis of malignancies in metastatic states of the disease (1). Although immune checkpoint blockade (ICI) provides a long-lasting response in approximately one-third of melanoma patients, a high number of non-responders and immune-related adverse events (irAEs) need to be considered as well (1). Therefore, a high medical need exists to identify treatment responders and patients with a predisposition for irAEs early on. Recent studies have identified tissue-resident memory T cells (TRM cells), correlating with clinical endpoints such as prolonged survival in different ICI-treated cancer entities (2–6). In particular, CD103+CD39+ TRM cells were associated with better outcomes across different tumor entities (5). Within this review, we will highlight the functional aspects of TRM cells that underpin their prognostic role in melanoma antitumor immunity and ICI efficacy. However, TRM cells were also shown to be involved in irAEs of barrier organs such as the skin or the gut (7–10). We will elaborate on these off-target effects also.
Function and genesis of tissue-resident T cells in the skin
Tissue residency and memory functions enable TRM cells to rapidly defend against encountering viral infections and other pathogens these cells are previously primed against (11). In the course of a lifetime, inflammation or exposition to viral infections and microbiota contributes to the genesis of TRM cells and shapes an individual and diverse immunological landscape. Interestingly, TRM cells differ in their mode of action from primary T-cell responses. In their inactivated cell state, TRM cells were reported to pass throughout the tissue and express dendritic-cell-like pseudopods to recognize antigens (11). After pathogen sensing, TRM cells rapidly expand and proliferate, secreting IFN-γ, which evokes local inflammation and CXCL9 and CXCL10 secretion (12). CXCR3 ligands are known to attract more lymphocytes to the tissue which then can also correlate with antitumor immunity and ICI efficacy (13, 14). In mice lacking circulating T cells, TRM cells eradicate pathogens by upregulation of cytotoxic cellular function in an IFN-γ–dependent fashion (15). The functional features of TRM cells are predetermined by the expression profile of the cell: acquiring increased expression of CD69, Hobit, Blimp1, or Runx3 and downregulating CD62L, S1PR1, Tcf1, T-bet, or Eomes (16–19). The cytokine IL-15 induces CD69 expression (8, 20). In the skin, TRM genesis is triggered by inflammation and antigen presentation. In line with these findings, human keratinocytes cocultured with T cells induce CD103 expression by TGF-β, enabling TRM cells to consolidate (21). TRM cells showed more potent effector functions than recirculating T cells (21). Interestingly, TRM and TCM were shown to share the common naive T-cell progenitor/precursor (22). Progenitors of memory cells from the bloodstream extravasate and express CD103, induced by TGF-β (21). Entry into the epidermis is considered an essential step in the maturation of TRM cells identified by upregulated CD69 and the prosurvival marker Bcl-2 (23). In contrast, a comparable set of cells in the dermis was found to be CD103−, emphasizing the influence of environmental factors in the epidermis (23).
Induced by viral infection of the skin (modulated by HSV infection of the skin in mouse models), keratinocytes produce CXCL9 and CXCL10, attracting KLGR+ TRM progenitors more effectively due to their higher expression of CXCR3 than KLGR− effector cells (23). These cells, after entrance into the skin, are exposed to IL-15 and TGF-β, both known to influence memory T cells toward tissue residency. Interestingly, the longevity of memory T cells seems to be maintained by the KLGR transcription factor, regulating these effects in TCM cells as well as in TRM cells. The plasticity of TRM cells allows them to re-access circulation and differentiate into TCM which patrol lymph nodes (24). This interconversion of memory T-cell phenotype from resident to circulating can be referred to as “outside-in immunity” (24).
Single-cell RNA sequencing and knockout experiments revealed the essential role of transcriptional “master” regulators Runx3, Hobit, and Blimp1 (23). These regulators mediate particular TRM features like TGF-β sensitivity mediated by Smad3, which is positively regulated by Fosl2 or Blimp1 and interacts with Hobit to suppress lymph egress by CCR7 and S1P signaling suppression (23). Circulating memory T cells and tissue-adherent memory T cells differ in their expression profile of these transcription factors. Hobit, for example, was identified in precursors for resident T cells but not in the circulating fraction of T-cell precursors (25). For TRM survival, however, transcription factors like STAT5 and signaling of phosphatidylinositol 3-kinase (PI3K)/Akt and Wnt are mandatory (26). Interestingly, maintenance of TRM cells is achieved by the regulation of metabolic features like fatty acid uptake and beta-oxidation (27). Furthermore, hypoxia was shown to promote and maintain TRM fate (28).
Immune checkpoint inhibition and tissue-resident memory T cells
Mechanistically, ICI targets a subclass of lymphocytes that express regulatory molecules, called checkpoints. Due to (neo-)antigen overstimulation in the tumor microenvironment (TME), tumor-infiltrating lymphocytes (TILs) acquire a “self-protection” state, referred to as T-cell exhaustion (29). Due to their upregulated checkpoint proteins and their priming against tumor neoantigens, the subpopulation of exhausted T cells (TEX) is the target of ICI therapy and increases over the course of therapy, measured by the increase of proliferative markers Ki-67 and PD-1 expressing T cells from 50% prior to therapy up to 75% in the patients’ blood (29).
Elaboration of blood and tissue composition of responders and non-responders could reveal that the antitumor effects of ICI are facilitated by the accumulation of diverse clusters of lymphocytic cells representing adaptive immunity, widely captured as TILs. Distinct blood and tissue characterization further unraveled the subclusters of lymphocytes mediating the response to ICI, identifying memory-like T cells as the most abundant lymphocyte cluster (30). In high-risk patients with melanoma brain metastases, a sequencing scheme of radiotherapy first followed by immunotherapy led to higher frequencies of memory T cells (TMEM cells) in the blood and improved response (31). TMEM cells possess the ability of longevity after (neo-)antigen contact and form thereby a persisting pool of effector T cells facilitating an early adaptive response to previously encountered pathogens. A subset of TMEM cells is tumor-specific and resides within tumor-draining lymph nodes (32). TMEM cells maintain plasticity and can shift from being circulatory TMEM cells to become a TRM-like phenotype in melanoma tumors and persist after tumor eradication (33). Batf3-dependent DCs are essential for both memory cell compartments. They are required for the generation of skin TRM cells and they can reactivate circulating CD8+ TMEM cells, inducing antitumor immunity (33, 34). Intratumoral TRM cells were expressing PD-1 and expanded after anti-PD-1 therapy, arguing for a key role in ICI efficacy (33). TRM cells are expressing integrins (CD49a and CD103) and losing proteins required for lymph egress (S1PR1 suppressed by CD69). TRM cells represent a subset of TILs with the highest expression of various checkpoint molecules, making them the ideal targets for ICI (35). These cells are under extensive research, considered as secondary acquired defense against pathogens in barrier tissue. Due to their abundance and heterogeneity, memory T cells are thought to substantially contribute to antitumor immunity. Thus, TRM cells are eligible to counteract tumorigenesis and intervene in the different milestones in the metastatic cascade. A plethora of work showed the outgrowth of this fraction of TRM-like TEX cells correlating with clinical endpoints like prolonged survival in different ICI-treated cancer entities (2–6). Hence, these cells might hold the potential to eradicate cancerous cells across tumor entities. In particular, skin cancers such as melanoma seem predestined for the already present TRM cells patrolling the tissue and contributing to tumor immunity.
Tissue-resident memory T cells in melanoma
TRM cells were found to be most abundant in non-lymphatic tissue, rendering them as secondary effectors for bacterial or viral infection (12). For antitumor defense, their characteristics as the predominant T-cell population and their heterogeneity in the T-cell receptor (TCR) repertoire potentially enable effective antitumor responses (35). Residency, mediated by their constitutive expression profile of CD69 and CD103, allows early and immediate recognition and effector function in peripheral tissue, making them presumably important regulators of tumorigenesis. In mice, TRM cells mediated protection against melanoma development (36). CD8+ epidermal CD69+CD103+ TRM cells correlated with spontaneous disease control after inoculation with melanoma cells (36). Mice without TRM cell formation were more susceptible to tumor development (36). In a mouse model analysis, melanoma-specific TRM cells could also be identified in skin-draining lymph nodes (37). These TRM cells protected against melanoma tumor seeding in lymph nodes. TRM cell signatures were also found in sentinel lymph node metastases from patients and correlated positively with survival (37). In a small cohort comparison of four non-responders to four responders in human melanoma, the loss of CD63 and E-cadherin, the target of CD103, was associated with non-response in melanoma (38). E-cadherin resulted in a more mesenchymal phenotype of tumors which were non-responding tumors. In a B16F10 melanoma mouse model, deeper investigations unraveled the association of the loss of E-cadherin and reduced TRM activation in melanoma (39). TRM cells are capable of adhering to epithelial cells and melanoma cells by their CD103 (alphaE) expression (40). CD103 binds E-cadherin by forming a heterodimer with integrin beta7. This homing of T cells provokes a selective pressure for tumor cells to lose E-cadherin to resist reinforced TRM cell immunity (39). Shields et al. showed by implanting E-cadherin overexpressing B16F10 melanoma cells in wild-type, RAG−/−, and CD103−/− mice that E-cadherin expression, which initially led to reduced outgrowth in wild-type mice compared with CDH1 knockout B16F10s, requires functional CD103+ TRM cells for successful tumor eradication (39). In a mouse model of adoptive T-cell therapy for melanoma, knockout variants of Runx3 (identified as one of the main regulators of TRM fate) showed less T-cell invasion and accumulation in contrast to wild-type mice, resulting in worse outcomes in the knockouts (41). TRM cells can activate cross-presenting dermal DCs, resulting in priming of additional cytotoxic T cells against tumor-derived neo- and self-antigens (42). This TRM cell-induced antigen spreading suppresses local and disseminated melanoma in mice (42). Taken together, this is strong experimental evidence that TRM cells are essential for antitumor immunity in melanoma. CD103+CD69+ TRM cells showed a high expression profile of immune checkpoint proteins and were located within the tumor tissue of melanoma patients, suggesting them to be an ideal subpopulation of T cells to be reinvigorated by ICI (35). In fact, TRM cells were more efficient predictive biomarkers for the response to ICI than general CD8+ TILs in melanoma (43). In addition, within tumors, CD45RO+CD69+CCR7− TRM phenotypes were observed in 60% of CD8+ T cells and 50% of CD4+ T cells (35). Responders to ICI present an abundance of TRM cells in pretreatment melanoma tissue (44). In particular, CD8+/CD4+EOMES+CD69+CD45RO+ subpopulations were expanded in responder patients. Also, a gene expression signature obtained from tumor biopsies containing the gene (ITGAE) encoding for CD103 among other TIL-related genes (CD8A, CD8B, ITGAE [CD103], PDCD1 [PD-1], CCL5, CXCL13, and IL2) was associated with better outcomes to anti-PD1 therapy (44). Interestingly, CD45RO+ and EOMES+ memory T cells measured in baseline melanoma tissue also highly expressed CD69 and CD103. They were more abundantly found in responder patients, further emphasizing the predominant role of TRM cells as determinants of response and non-response (44). Another study in human melanoma samples found that 30% of the CD8+ T cells are TRM cells positive for CD103 and CD69 (43). These TRM cells showed moderate expression of granzyme B, CD137, and HLA-DR. CD103+CD69+ TRM cells expressed checkpoint molecules and thus expanded under therapy (43). Local IL-15 highly correlated with TRM cell numbers. IL-15 blocks tissue-egress signals on human T cells and induces the expression of CD69 (43). TRM cells can also co-express CD49. CD49+ TRM cells exhibited superior effector function and correlated with survival in a melanoma mouse model (45) (Table 1).
Immune-related adverse events and tissue-resident memory T cells
Although ICI can provoke a durable response for patients with advanced cancer, irAEs are challenging and limit the beneficial effects of treatment (49). They can be clinically categorized by the severity of the inflammatory side effects of ICI. IrAEs range from common and less severe cutaneous events like morbilliform or lichenoid rashes and pruritus to more severe toxicities such as dermatomyositis, Steven-Johnson syndrome, endocrinological dysfunction like diabetes, hepatitis, or myocarditis (50, 51). By clinical observation, the organ specificity of irAEs differs between CTLA-4 inhibitors and PD-1/PD-L1 inhibitors. For instance, patients treated with CTLA-4 inhibitors are more often affected by irAEs of higher severity like colitis and hypophysitis, whereas patients treated with PD-1/PD-L1 axis inhibitors more often develop pneumonitis and thyroiditis (52). Vitiligo is a side effect of ICI treatment specific to melanoma. Its association with a favorable outcome reveals the cross-reactivity of reinvigorated T cells (53, 54). In autoimmune vitiligo, CD8+ TRM cells are recruited via CXCL9 and CXCL10 and result in melanocyte damage by secreting granzyme B, perforin, and IFN-γ (55). In mice, TRM cells within vitiligo-affected tissue are also specific for melanoma antigens (46). These TRM cells were not only critical for vitiligo lesions but also for maintaining antitumor immunity (46). In melanoma patients with response to immunotherapy and vitiligo, long-term antitumor immunity even up to 9 years after ICI was mediated via TRM cells with high expression of IFN-γ and TNF-α (47). Interestingly, also other cutaneous irAEs and irAEs in general correlated with response to immunotherapy across cancers (56, 57). Recent studies of the cellular fraction in irAE-affected tissue revealed the abundance of TRM cells in irAE colitis and irAE dermatitis (8–10). In various cutaneous irAEs, CD4+ and CD8+ TRM cells were expanded and produced IFN-γ and TNF-α, arguing for Th1/Tc1 polarization (8). Downstream of IFN-γ, the CXCR3 ligands CXCL9–11 were upregulated, potentially recruiting more circulating T cells to the tissue. Furthermore, TRM cells highly expressed inhibitory checkpoint molecules such as PD-1, CTLA-4, LAG-3, TIM-3, or TIGIT in irAE dermatitis. A similar expression pattern was seen in CD4+ and CD8+ TRM cells of irAE colitis (8, 10). Upregulated checkpoints argue for potential reactivation and expansion of TRM cells in off-target tissue during ICI (Figure 1). In addition, local IL-15 expression was also upregulated, corresponding with the high TRM cell levels (8).
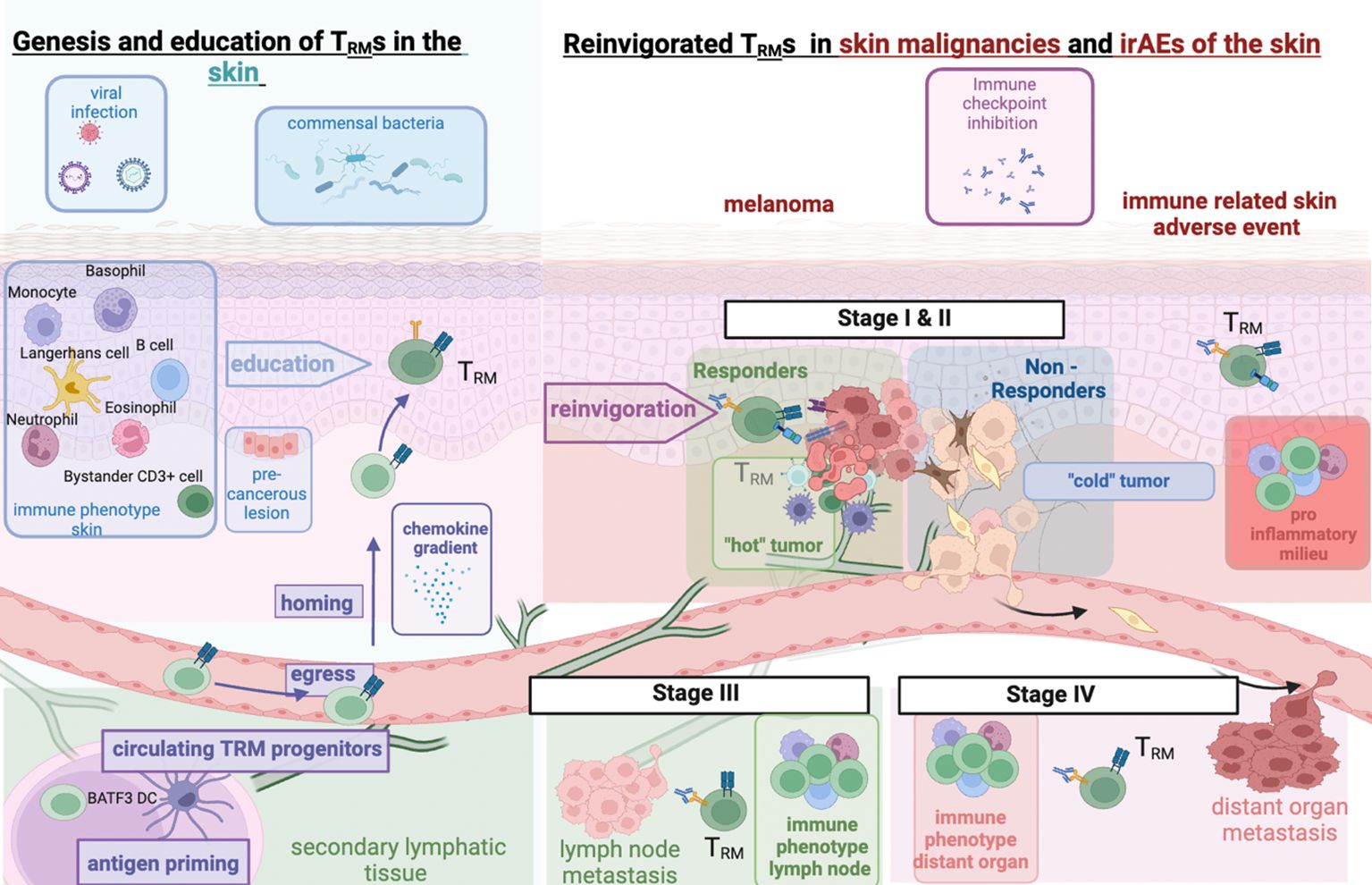
Figure 1 TRM interaction in healthy skin and in ICI-treated melanoma and cutaneous irAEs. Left: Genesis of TRM in healthy skin and impact of prior inflammation through commensal skin microbiota or prior immune responses to viral infections; right: immune checkpoint inhibition reinvigorates preformed TRM cells. Malignancies undergo metastatic invasion with interaction with differentially shaped immune micromilieus in lymph nodes or distant organs. Expansion of activated TRM cells to different cancer sites or off-target tissue sites (irAEs) upon ICI. Figure created with BioRender.
Discussion
We are still missing reliable and mechanistically motivated tissue-based biomarkers that guide ICI at different stages of melanoma therapy. The tumor-intrinsic risk of recurrence in stage I–III melanomas can be classified with an immunohistochemical signature consisting of Bax, Bcl-X, PTEN, COX-2, β-catenin, MTAP, and CD20 (58–60). This signature, however, does not contain markers relevant to T cells which are the main target of ICI. CXCR3 ligands such as CXCL9 and CXCL10 as indirect markers of T-cell infiltration have been identified in the past as indicating a response to ICI in stage IV melanoma (61, 62). Lately, TRM cells have become a subject of special interest and intensive research due to their unique combination of cytotoxic potential, combined with checkpoint protein expression, and their localization and high prevalence in tumor tissue (35). Microenvironmental factors, viruses, or microbiota can shape the appearance of TRM cell pools (12). They have been found to patrol various organs such as the brain, intestine, skin, or even the heart (11, 63). TRM cells are capable of plasticity and gene signature switches in agile and cytotoxic phenotypes (with cytolytic molecules) or endurance and senescence-like cell states in the absence of sustained antigen presentation (11). TRM cells have proven to be strong predictors of survival across tumor entities (2–6). Specifically, in cancers treated with immunotherapy such as melanoma, they are a highly relevant target cell population because they express inhibitory checkpoint molecules (35). Melanoma often appears as a high metastatic entity and tends toward invasiveness and distant organ metastasis. The first results in mice have shown that TRM cells can also colonize tumor-draining lymph nodes of melanoma patients and prevent metastatic spread from there (37). Investigation of the role of TRM cells in tumor surveillance in disseminated tumor stages under immunotherapy is an urgent clinical question. Recent development and expansion of approval of pembrolizumab in the stage II setting of melanoma raises the question for assessing the TRM profile and functional capacities in micrometastasis and MRD. Metastatic tumors face an altered immunologic niche. Adaptive immunity is confronted with transformed tumor cells undergoing epithelial–mesenchymal transition (EMT) (64). TRM cells are thought to diminish proliferating epithelial-like tumor cells with high turnover and expression of epithelial markers (40). The prognostic role of TRM cells within primary melanoma tissue and tumor-draining lymph nodes could become extremely interesting for the neoadjuvant ICI regimens and potentially guide neoadjuvant versus surgical approaches. In oral cancer patients, CD8+ TILs that clonally expanded during neoadjuvant ICI showed a cytotoxic TRM cell phenotype, underlining the capacity for a rapid response of pre-existing T-cell clones (3). Those treatment-expanded T-cell clones in responding patients also recognized self-antigens such as the cancer-specific antigen MAGEA1 (3). Melanoma patients with vitiligo or other (cutaneous) irAEs tend to have a better outcome than others (56, 57). We hypothesize that Th1/Tc1-polarized TRM cells that trigger cutaneous irAEs such as vitiligo can also be responsible for tumor cell killing through the production of effector molecules across melanoma stages I–IV. It is conceivable, downstream from IFN-γ-producing TRM cells, that other circulating T cells are recruited to the tissue via CXCL9 and CXCL10 gradients (62). Exhausted T cells within melanoma tumors also produce CCL4 and CXCL13, which in turn recruit other relevant immune cells from the circulation such as dendritic or B cells, potentially resulting in tertiary lymphoid structures (74). Treating toxicity, systemic inhibition of Tc1/Th1 TRM cells or corresponding cytokines such as IFN-γ might abrogate immunotherapy efficacy and should only be explored for severe irAEs. However, local and more targeted control of cutaneous irAEs might be achieved by topical treatment with JAK inhibitors instead of glucocorticoids without negatively affecting systemic antitumor immunity. In summary, TRM cells can function as biomarkers for antitumor immunity and ICI toxicity and have to be targeted with caution. TRM cells should be exploited as indicators for promising neoadjuvant ICI in melanoma and tested in larger trials.
Author contributions
RR: Conceptualization, Visualization, Writing – original draft, Writing – review & editing, Methodology, Project administration. BD: Visualization, Writing – original draft. AE: Writing – review & editing. JH: Writing – review & editing.
Funding
The author(s) declare financial support was received for the research, authorship, and/or publication of this article. For the publication fee, the authors acknowledge financial support by Heidelberg University.
Conflict of interest
The authors declare that the research was conducted in the absence of any commercial or financial relationships that could be construed as a potential conflict of interest.
Publisher’s note
All claims expressed in this article are solely those of the authors and do not necessarily represent those of their affiliated organizations, or those of the publisher, the editors and the reviewers. Any product that may be evaluated in this article, or claim that may be made by its manufacturer, is not guaranteed or endorsed by the publisher.
References
1. Larkin J, Chiarion-Sileni V, Gonzalez R, Grob J-J, Rutkowski P, Lao CD, et al. Five-year survival with combined nivolumab and ipilimumab in advanced melanoma. N Engl J Med. (2019) 381:1535–46. doi: 10.1056/NEJMoa1910836
2. Lee YJ, Kim JY, Jeon SH, Nam H, Jung JH, Jeon M, et al. CD39+ tissue-resident memory CD8+ T cells with a clonal overlap across compartments mediate antitumor immunity in breast cancer. Sci Immunol. (2022) 7:eabn8390. doi: 10.1126/sciimmunol.abn8390
3. Luoma AM, Suo S, Wang Y, Gunasti L, Porter CBM, Nabilsi N, et al. Tissue-resident memory and circulating T cells are early responders to pre-surgical cancer immunotherapy. Cell. (2022) 185:2918–2935.e29. doi: 10.1016/j.cell.2022.06.018
4. Banchereau R, Chitre AS, Scherl A, Wu TD, Patil NS, de Almeida P, et al. Intratumoral CD103+ CD8+ T cells predict response to PD-L1 blockade. J Immunother Cancer. (2021) 9:e002231. doi: 10.1136/jitc-2020-002231
5. Duhen T, Duhen R, Montler R, Moses J, Moudgil T, de Miranda NF, et al. Co-expression of CD39 and CD103 identifies tumor-reactive CD8 T cells in human solid tumors. Nat Commun. (2018) 9:2724. doi: 10.1038/s41467-018-05072-0
6. Savas P, Virassamy B, Ye C, Salim A, Mintoff CP, Caramia F, et al. Single-cell profiling of breast cancer T cells reveals a tissue-resident memory subset associated with improved prognosis. Nat Med. (2018) 24:986–93. doi: 10.1038/s41591-018-0078-7
7. Reschke R, Gajewski TF. Tissue-resident memory T cells in immune-related adverse events: friend or foe? Oncoimmunology. (2023) 12:2197358. doi: 10.1080/2162402X.2023.2197358
8. Reschke R, Shapiro JW, Yu J, Rouhani SJ, Olson DJ, Zha Y, et al. Checkpoint blockade–induced dermatitis and colitis are dominated by tissue resident memory T cells and Th1/Tc1 cytokines. Cancer Immunol Res. (2022), 10:1167–74. doi: 10.1158/2326-6066.CIR-22-0362
9. Luoma AM, Suo S, Williams HL, Sharova T, Sullivan K, Manos M, et al. Molecular pathways of colon inflammation induced by cancer immunotherapy. Cell. (2020) 182:655–671.e22. doi: 10.1016/j.cell.2020.06.001
10. Sasson SC, Slevin SM, Cheung VTF, Nassiri I, Olsson-Brown A, Fryer E, et al. Interferon-gamma–producing CD8+ Tissue resident memory T cells are a targetable hallmark of immune checkpoint inhibitor–colitis. Gastroenterology. (2021) 161:1229–1244.e9. doi: 10.1053/j.gastro.2021.06.025
11. Ariotti S, Beltman JB, Chodaczek G, Hoekstra ME, van Beek AE, Gomez-Eerland R, et al. Tissue-resident memory CD8+ T cells continuously patrol skin epithelia to quickly recognize local antigen. Proc Natl Acad Sci U.S.A. (2012) 109:19739–44. doi: 10.1073/pnas.1208927109
12. Schenkel JM, Fraser KA, Vezys V, Masopust D. Sensing and alarm function of resident memory CD8+ T cells. Nat Immunol. (2013) 14:509–13. doi: 10.1038/ni.2568
13. Reschke R, Gajewski TF. CXCL9 and CXCL10 bring the heat to tumors. Sci Immunol. (2022) 7:eabq6509. doi: 10.1126/sciimmunol.abq6509
14. Reschke R, Olson DJ. Leveraging STING, batf3 dendritic cells, CXCR3 ligands, and other components related to innate immunity to induce A “Hot” Tumor microenvironment that is responsive to immunotherapy. Cancers. (2022) 14:2458. doi: 10.3390/cancers14102458
15. Steinbach K, Vincenti I, Kreutzfeldt M, Page N, Muschaweckh A, Wagner I, et al. Brain-resident memory T cells represent an autonomous cytotoxic barrier to viral infection. J Exp Med. (2016) 213:1571–87. doi: 10.1084/jem.20151916
16. Mackay LK, Minnich M, Kragten NAM, Liao Y, Nota B, Seillet C, et al. Hobit and Blimp1 instruct a universal transcriptional program of tissue residency in lymphocytes. Science. (2016) 352:459–63. doi: 10.1126/science.aad2035
17. Rao RR, Li Q, Odunsi K, Shrikant PA. The mTOR kinase determines effector versus memory cell fate of CD8+ T cells by regulating T-bet and Eomesodermin expression. Immunity. (2010) 32:67–78. doi: 10.1016/j.immuni.2009.10.010
18. Skon CN, Lee J-Y, Anderson KG, Masopust D, Hogquist KA, Jameson SC. Transcriptional downregulation of S1pr1 is required for establishment of resident memory CD8+ T cells. Nat Immunol. (2013) 14:1285–93. doi: 10.1038/ni.2745
19. Behr FM, Kragten NAM, Wesselink TH, Nota B, van Lier RAW, Amsen D, et al. Blimp-1 rather than hobit drives the formation of tissue-resident memory CD8+ T cells in the lungs. Front Immunol. (2019) 10:400. doi: 10.3389/fimmu.2019.00400
20. Woon HG, Braun A, Li J, Smith C, Edwards J, Sierro F, et al. Compartmentalization of total and virus-specific tissue-resident memory CD8+ T cells in human lymphoid organs. PloS Pathog. (2016) 12:e1005799. doi: 10.1371/journal.ppat.1005799
21. Watanabe R, Gehad A, Yang C, Campbell L, Teague JE, Schlapbach C, et al. Human skin is protected by four functionally and phenotypically discrete populations of resident and recirculating memory T cells. Sci Transl Med. (2015) 7:279ra39. doi: 10.1126/scitranslmed.3010302
22. Gaide O, Emerson RO, Jiang X, Gulati N, Nizza S, Desmarais C, et al. Common clonal origin of central and resident memory T cells following skin immunization. Nat Med. (2015) 21:647–53. doi: 10.1038/nm.3860
23. Mackay LK, Rahimpour A, Ma JZ, Collins N, Stock AT, Hafon M-L, et al. The developmental pathway for CD103+CD8+ tissue-resident memory T cells of skin. Nat Immunol. (2013) 14:1294–301. doi: 10.1038/ni.2744
24. Fonseca R, Beura LK, Quarnstrom CF, Ghoneim HE, Fan Y, Zebley CC, et al. Developmental plasticity allows outside-in immune responses by resident memory T cells. Nat Immunol. (2020) 21:412–21. doi: 10.1038/s41590-020-0607-7
25. Parga-Vidal L, Behr FM, Kragten NAM, Nota B, Wesselink TH, Kavazović I, et al. Hobit identifies tissue-resident memory T cell precursors that are regulated by Eomes. Sci Immunol. (2021) 6:eabg3533. doi: 10.1126/sciimmunol.abg3533
26. Chen L, Shen Z. Tissue-resident memory T cells and their biological characteristics in the recurrence of inflammatory skin disorders. Cell Mol Immunol. (2020) 17:64–75. doi: 10.1038/s41423-019-0291-4
27. Pan Y, Tian T, Park CO, Lofftus SY, Mei S, Liu X, et al. Survival of tissue-resident memory T cells requires exogenous lipid uptake and metabolism. Nature. (2017) 543:252–6. doi: 10.1038/nature21379
28. Hasan F, Chiu Y, Shaw RM, Wang J, Yee C. Hypoxia acts as an environmental cue for the human tissue-resident memory T cell differentiation program. JCI Insight. (2021) 6:e138970. doi: 10.1172/jci.insight.138970
29. Huang AC, Postow MA, Orlowski RJ, Mick R, Bengsch B, Manne S, et al. T-cell invigoration to tumour burden ratio associated with anti-PD-1 response. Nature. (2017) 545:60–5. doi: 10.1038/nature22079
30. Ribas A, Shin DS, Zaretsky J, Frederiksen J, Cornish A, Avramis E, et al. PD-1 blockade expands intratumoral memory T cells. Cancer Immunol Res. (2016) 4:194–203. doi: 10.1158/2326-6066.CIR-15-0210
31. Hassel JC, Schank TE, Smetak H, Mühlbauer J, Salzmann M, Machiraju D, et al. Evaluation of radio-immunotherapy sequence on immunological responses and clinical outcomes in patients with melanoma brain metastases (ELEKTRA). Oncoimmunology. (2022) 11:2066609. doi: 10.1080/2162402X.2022.2066609
32. Huang Q, Wu X, Wang Z, Chen X, Wang L, Lu Y, et al. The primordial differentiation of tumor-specific memory CD8+ T cells as bona fide responders to PD-1/PD-L1 blockade in draining lymph nodes. Cell. (2022) 185:4049–4066.e25. doi: 10.1016/j.cell.2022.09.020
33. Enamorado M, Iborra S, Priego E, Cueto FJ, Quintana JA, Martínez-Cano S, et al. Enhanced anti-tumour immunity requires the interplay between resident and circulating memory CD8+ T cells. Nat Commun. (2017) 8:16073. doi: 10.1038/ncomms16073
34. Iborra S, Martínez-López M, Khouili SC, Enamorado M, Cueto FJ, Conde-Garrosa R, et al. Optimal Generation of Tissue-Resident but Not Circulating Memory T Cells during Viral Infection Requires Crosspriming by DNGR-1+ Dendritic Cells. Immunity. (2016) 45:847–60. doi: 10.1016/j.immuni.2016.08.019
35. Boddupalli CS, Bar N, Kadaveru K, Krauthammer M, Pornputtapong N, Mai Z, et al. Interlesional diversity of T cell receptors in melanoma with immune checkpoints enriched in tissue-resident memory T cells. JCI Insight. (2016) 1:e88955. doi: 10.1172/jci.insight.88955
36. Park SL, Buzzai A, Rautela J, Hor JL, Hochheiser K, Effern M, et al. Tissue-resident memory CD8+ T cells promote melanoma–immune equilibrium in skin. Nature. (2019) 565:366–71. doi: 10.1038/s41586-018-0812-9
37. Molodtsov AK, Khatwani N, Vella JL, Lewis KA, Zhao Y, Han J, et al. Resident memory CD8+ T cells in regional lymph nodes mediate immunity to metastatic melanoma. Immunity. (2021) 54:2117–2132.e7. doi: 10.1016/j.immuni.2021.08.019
38. Shields BD, Mahmoud F, Taylor EM, Byrum SD, Sengupta D, Koss B, et al. Indicators of responsiveness to immune checkpoint inhibitors. Sci Rep. (2017) 7:807. doi: 10.1038/s41598-017-01000-2
39. Shields BD, Koss B, Taylor EM, Storey AJ, West KL, Byrum SD, et al. Loss of E-cadherin inhibits CD103 anti-tumor activity and reduces checkpoint blockade responsiveness in melanoma. Cancer Res. (2019) 79:1113–23. doi: 10.1158/0008-5472.CAN-18-1722
40. Cepek KL, Shaw SK, Parker CM, Russell GJ, Morrow JS, Rimm DL, et al. Adhesion between epithelial cells and T lymphocytes mediated by E-cadherin and the αEβ7 integrin. Nature. (1994) 372:190–3. doi: 10.1038/372190a0
41. Milner JJ, Toma C, Yu B, Zhang K, Omilusik K, Phan AT, et al. Runx3 programs CD8+ T cell residency in non-lymphoid tissues and tumours. Nature. (2017) 552:253–7. doi: 10.1038/nature24993
42. Menares E, Gálvez-Cancino F, Cáceres-Morgado P, Ghorani E, López E, Díaz X, et al. Tissue-resident memory CD8+ T cells amplify anti-tumor immunity by triggering antigen spreading through dendritic cells. Nat Commun. (2019) 10:4401. doi: 10.1038/s41467-019-12319-x
43. Edwards J, Wilmott JS, Madore J, Gide TN, Quek C, Tasker A, et al. CD103+ Tumor-resident CD8+ T cells are associated with improved survival in immunotherapy-naïve melanoma patients and expand significantly during anti–PD-1 treatment. Clin Cancer Res. (2018) 24:3036–45. doi: 10.1158/1078-0432.CCR-17-2257
44. Gide TN, Quek C, Menzies AM, Tasker AT, Shang P, Holst J, et al. Distinct immune cell populations define response to anti-PD-1 monotherapy and anti-PD-1/anti-CTLA-4 combined therapy. Cancer Cell. (2019) 35:238–255.e6. doi: 10.1016/j.ccell.2019.01.003
45. Murray T, Fuertes Marraco SA, Baumgaertner P, Bordry N, Cagnon L, Donda A, et al. Very late antigen-1 marks functional tumor-resident CD8 T cells and correlates with survival of melanoma patients. Front Immunol. (2016) 7:573. doi: 10.3389/fimmu.2016.00573
46. Malik BT, Byrne KT, Vella JL, Zhang P, Shabaneh TB, Steinberg SM, et al. Resident memory T cells in the skin mediate durable immunity to melanoma. Sci Immunol. (2017) 2:eaam6346. doi: 10.1126/sciimmunol.aam6346
47. Han J, Zhao Y, Shirai K, Molodtsov A, Kolling FW, Fisher JL, et al. Resident and circulating memory T cells persist for years in melanoma patients with durable responses to immunotherapy. Nat Cancer. (2021) 2:300–11. doi: 10.1038/s43018-021-00180-1
48. Willemsen M, Tio D, Krebbers G, Kasiem FR, Jaspars EH, Matos TR, et al. Presence of skin tissue-resident memory T cells in human nonmalignant and premalignant melanocytic skin lesions and in melanoma. Am J Dermatopathology. (2022) 44:416. doi: 10.1097/DAD.0000000000002184
49. Topalian SL, Hodi FS, Brahmer JR, Gettinger SN, Smith DC, McDermott DF, et al. Safety, activity, and immune correlates of anti-PD-1 antibody in cancer. N Engl J Med. (2012) 366:2443–54. doi: 10.1056/NEJMoa1200690
50. Darnell EP, Mooradian MJ, Baruch EN, Yilmaz M, Reynolds KL. Immune-related adverse events (irAEs): diagnosis, management, and clinical pearls. Curr Oncol Rep. (2020) 22:39. doi: 10.1007/s11912-020-0897-9
51. Reschke R, Mockenhaupt M, Simon J-C, Ziemer M. Severe bullous skin eruptions on checkpoint inhibitor therapy – in most cases severe bullous lichenoid drug eruptions. JDDG: J Der Deutschen Dermatologischen Gesellschaft. (2019) 17:942–8. doi: 10.1111/ddg.13876
52. Callahan MK, Wolchok JD. At the Bedside: CTLA-4- and PD-1-blocking antibodies in cancer immunotherapy. J Leukoc Biol. (2013) 94:41–53. doi: 10.1189/jlb.1212631
53. Freeman-Keller M, Kim Y, Cronin H, Richards A, Gibney G, Weber JS. Nivolumab in resected and unresectable metastatic melanoma: characteristics of immune-related adverse events and association with outcomes. Clin Cancer Res. (2016) 22:886–94. doi: 10.1158/1078-0432.CCR-15-1136
54. Byrne EH, Fisher DE. Correlates of metastatic melanoma response to checkpoint inhibition. Cancer. (2017) 123:2143–53. doi: 10.1002/cncr.30444
55. Shah F, Patel S, Begum R, Dwivedi M. Emerging role of Tissue Resident Memory T cells in vitiligo: From pathogenesis to therapeutics. Autoimmun Rev. (2021) 20:102868. doi: 10.1016/j.autrev.2021.102868
56. Zhang S, Tang K, Wan G, Nguyen N, Lu C, Ugwu-Dike P, et al. Cutaneous immune-related adverse events are associated with longer overall survival in advanced cancer patients on immune checkpoint inhibitors: A multi-institutional cohort study. J Am Acad Dermatol. (2023), 88(5):1024–32. doi: 10.1016/j.jaad.2022.12.048
57. Rogado J, Sánchez-Torres JM, Romero-Laorden N, Ballesteros AI, Pacheco-Barcia V, Ramos-Leví A, et al. Immune-related adverse events predict the therapeutic efficacy of anti–PD-1 antibodies in cancer patients. Eur J Cancer. (2019) 109:21–7. doi: 10.1016/j.ejca.2018.10.014
58. Reschke R, Gussek P, Ziemer M. Identifying high-risk tumors within AJCC stage IB–III melanomas using a seven-marker immunohistochemical signature. Cancers (Basel). (2021) 13:2902. doi: 10.3390/cancers13122902
59. Meyer S, Fuchs TJ, Bosserhoff AK, Hofstädter F, Pauer A, Roth V, et al. A seven-marker signature and clinical outcome in Malignant melanoma: A large-scale tissue-microarray study with two independent patient cohorts. PloS One. (2012) 7:e38222. doi: 10.1371/journal.pone.0038222
60. Meyer S, Buser L, Haferkamp S, Berneburg M, Maisch T, Klinkhammer-Schalke M, et al. Identification of high-risk patients with a seven-biomarker prognostic signature for adjuvant treatment trial recruitment in American Joint Committee on Cancer v8 stage I–IIA cutaneous melanoma. Eur J Cancer. (2023) 182:77–86. doi: 10.1016/j.ejca.2023.01.002
61. Reschke R, Yu J, Flood BA, Higgs EF, Hatogai K, Gajewski TF. Immune cell and tumor cell-derived CXCL10 is indicative of immunotherapy response in metastatic melanoma. J Immunother Cancer. (2021) 9:e003521. doi: 10.1136/jitc-2021-003521
62. House IG, Savas P, Lai J, Chen AXY, Oliver AJ, Teo ZL, et al. Macrophage-derived CXCL9 and CXCL10 are required for antitumor immune responses following immune checkpoint blockade. Clin Cancer Res. (2020) 26:487–504. doi: 10.1158/1078-0432.CCR-19-1868
63. Kalinoski HM, Won T, Rusinkevich V, Talor MV, Hughes DM, Wood MK, et al. Myosin specific TRM cells mediate increased severity of immune checkpoint inhibitor myocarditis. J Immunol. (2021) 206:98.52–2. doi: 10.4049/jimmunol.206.Supp.98.52
Keywords: TRM cells, tissue resident memory T cells (TRM), irAE, immune related adverse effects (irAEs), immunotherapy, biomarker, immune checkpoint inhibitor (ICI), melanoma
Citation: Reschke R, Deitert B, Enk AH and Hassel JC (2024) The role of tissue-resident memory T cells as mediators for response and toxicity in immunotherapy-treated melanoma—two sides of the same coin? Front. Immunol. 15:1385781. doi: 10.3389/fimmu.2024.1385781
Received: 13 February 2024; Accepted: 05 March 2024;
Published: 18 March 2024.
Edited by:
Paul Nathan, Mount Vernon Cancer Centre, United KingdomReviewed by:
Dmitry Aleksandrovich Zinovkin, Gomel State Medical University, BelarusCopyright © 2024 Reschke, Deitert, Enk and Hassel. This is an open-access article distributed under the terms of the Creative Commons Attribution License (CC BY). The use, distribution or reproduction in other forums is permitted, provided the original author(s) and the copyright owner(s) are credited and that the original publication in this journal is cited, in accordance with accepted academic practice. No use, distribution or reproduction is permitted which does not comply with these terms.
*Correspondence: Robin Reschke, robinniklas.reschke@med.uni-heidelberg.de