- 1Clinical Medicine, China-Japan Union Hospital of Jilin University, Changchun, China
- 2Department of Cell Biology and Medical Genetics, College of Basic Medical Sciences, Jilin University, Changchun, China
- 3Department of Pharmacology, College of Basic Medical Sciences, Jilin University, Changchun, China
Surgery, chemotherapy, and endocrine therapy have improved the overall survival and postoperative recurrence rates of Luminal A, Luminal B, and HER2-positive breast cancers but treatment modalities for triple-negative breast cancer (TNBC) with poor prognosis remain limited. The effective application of the rapidly developing chimeric antigen receptor (CAR)-T cell therapy in hematological tumors provides new ideas for the treatment of breast cancer. Choosing suitable and specific targets is crucial for applying CAR-T therapy for breast cancer treatment. In this paper, we summarize CAR-T therapy’s effective targets and potential targets in different subtypes based on the existing research progress, especially for TNBC. CAR-based immunotherapy has resulted in advancements in the treatment of breast cancer. CAR-macrophages, CAR-NK cells, and CAR-mesenchymal stem cells (MSCs) may be more effective and safer for treating solid tumors, such as breast cancer. However, the tumor microenvironment (TME) of breast tumors and the side effects of CAR-T therapy pose challenges to CAR-based immunotherapy. CAR-T cells and CAR-NK cells-derived exosomes are advantageous in tumor therapy. Exosomes carrying CAR for breast cancer immunotherapy are of immense research value and may provide a treatment modality with good treatment effects. In this review, we provide an overview of the development and challenges of CAR-based immunotherapy in treating different subtypes of breast cancer and discuss the progress of CAR-expressing exosomes for breast cancer treatment. We elaborate on the development of CAR-T cells in TNBC therapy and the prospects of using CAR-macrophages, CAR-NK cells, and CAR-MSCs for treating breast cancer.
1 Introduction
Breast cancer, the most common malignancy in women, is a developmental dysplasia of malignant cells of the ductal or lobular compartment of the breast (1). According to global cancer statistics for 2020, breast cancer accounts for an estimated 11.7% of 19.3 million new cancer cases and 6.9% of 10 million cancer deaths (2). Based on the expression of estrogen receptor (ER), progesterone receptor (PR), human epidermal growth factor receptor 2 (HER-2) and Ki-67, breast cancers have been classified into Luminal A (HER2-/ER +/PR +, low proliferation), Luminal B (HER2-/ER +/PR +, high proliferation), HER2-amplified (HER2 +/ER + or PR + or ER +/PR +; HER2 +/ER-/PR-) and triple-negative breast cancer (TNBC) (HER2-/ER-/PR-) types (3). Classification according to the molecular phenotype of breast cancer guides the clinical treatment of breast cancer. Luminal A and Luminal B types are sensitive to chemotherapy and endocrine therapy (tamoxifen, fulvestrant, aromatase inhibitor) (4–6). Chemotherapy, anti-HER-2 therapy (transtuzumab), tyrosine kinase receptor inhibitors (TKIs) (lapatinib), and endocrine therapy have achieved treatment effects in HER2-positive breast cancer (7–9). However, given the lack of specific targets, endocrine therapy or HER-2 targeted therapy has a limited response in TNBC. Although chemotherapy (anthracycline plus taxane) is the main treatment modality for TNBC, problems of treatment resistance and long-term recurrence remain to be addressed (10). Improving the treatment effects and reducing the risk of TNBC recurrence are existing challenges meriting solutions.
In recent years, chimeric antigen receptor immunotherapy has emerged as a new research focus for tumor treatment (11). The first CAR-T therapy has been approved by the Food and Drug Administration (FDA) for the treatment of relapsed or refractory acute lymphoblastic leukemia (ALL), chronic lymphocytic leukemia (CLL), mantle cell lymphoma (MCL), refractory diffuse large B-cell lymphoma (DLBCL), and multiple myeloma (MM) (12). The successful application of CAR-T cell therapy to hematological tumors implies its use as a potential treatment strategy against solid tumors. Indeed, CAR-T therapy has been widely used to treat solid tumors of the respiratory, nervous system, digestive tract, urinary, breast, and female reproductive organs (13–25). The CAR-based immunotherapy has also been used in macrophages. For example, remarkable results have been achieved in the HER2-positive ovarian cancer cell line, SKOV3 (26). Interestingly, exosomes, extracellular vesicles composed of lipid bilayers released from source cells, can deliver biological molecules to target cells and exert relevant biological effects. Recent research suggests that CAR-containing exosomes show high expression of cytotoxic molecules and inhibit tumor growth, suggesting a relatively safe option compared with CAR-T therapy without acute toxicities, such as lung cancer (27, 28). Drawing on the effectiveness of CAR-therapy in tumor treatment, this review focuses on the application of CAR-based immunotherapy in breast cancer. The aim is to provide new insights and research directions for breast cancer treatment.
2 Application of CAR-T therapy in breast cancer
Although effective therapies are available for Luminal A, Luminal B, and HER-2 positive breast cancer, CAR-therapy has been investigated in therapeutic studies. Recent studies have reported good therapeutic results for CAR-T therapy in Luminal A type. CAR-T therapy has achieved remarkable success in treating HER-2-positive breast cancer (29). However, evidence for CAR-T therapy for Luminal B type is limited. CAR-T therapy for TNBC treatment has achieved a breakthrough (30). The following sections summarize the application of CAR-T therapy in different subtypes of breast cancer and focus on the relevant targets of TNBC.
2.1 Luminal A and Luminal B
Luminal A (HER2-/ER +/PR +, low proliferation) and Luminal B (HER2-/ER +/PR +, high proliferation) are breast cancer subtypes that have a better prognosis compared to other types of breast cancer (31, 32). Although endocrine therapy and chemotherapy show good therapeutic effects on Luminal A, CAR-T therapy is still under investigation to treat this subtype. For example, diganglioside GD2, a tumor-associated antigen, is expressed in a high proportion of patients with the luminal A subtype (33). Seitz et al. found that the GD2-targeted CAR-T cells exhibited good cytolytic activity against the GD2-positive Luminal A cell line MCF 7; however, no antitumor activity against the Luminal A cell line, T-47D, was demonstrated (34). Similarly, Zhang et al. found that CAR-T cells targeting MSLN could specifically kill MSLN-positive MCF 7 breast cancer cells and release secreted cytokines (35). Bajor et al. found that PD-L1 low MCF-7 cells were eliminated by PD-L1-CAR-T cells in long-term kill trials while PD-L1-CAR-T cells combined with HER-2-CAR-T cells stimulated the expression of PD-L1 on MCF-7 cells, thereby accelerating killing (36). CAR-T cells target AXL, B7-H4, EGFR, FcγRI (CD64), and HER2 and exert antitumor effects on MCF 7 and SK-BR-3 cell lines (27, 37–39). However, CAR-T treatment for Luminal B tumors is less explored. Thus, CAR-T cells for treating Luminal A and Luminal B subtypes merit investigation.
2.2 HER2-positive breast cancer
With overexpression of HER2 receptors driving proliferation, survival, and invasion of breast tumors, HER2-positive breast cancers (HER2 +/ER + or PR + or PR + or ER +/PR +; HER2 +/ER-/PR-) account for 15–20% of all cases (40, 41). Breast cancer with high HER2 expression has a high recurrence rate and a poor prognosis (42). Anti-HER2 monoclonal antibodies (trastuzumab and pertuzumab) exert antitumor effects in HER2-positive breast cancer by blocking HER2 signaling and activating antibody-dependent cytotoxicity (ADCC) (43, 44). However, a retrospective study of the combination of trastuzumab and pertuzumab in HER2-positive metastatic breast cancer (MBC) reported the most common adverse effects, including neutropenia (40.0%), leukopenia (34.5%), thrombocytopenia (32.7%), and diarrhea (29.1%) (45). Although TKIs show significant utility in the treatment of HER2-positive breast cancer, they can cause complications in multiple organs, especially the heart (46). Therefore, developing a treatment modality with fewer side effects and better efficacy is the research direction for HER-2-positive breast cancer.
2.2.1 HER2 serves as a target site
Significant breakthroughs have been made in achieving a killing effect on HER2-positive breast tumors using CAR-T cells targeting HER2. HER2-targeted CAR-T cells specifically recognize HER2-positive tumor cells, inhibiting tumor growth in vitro and in vivo (47). Similarly, CAR-T cells transduced with trastuzumab scFv efficiently lyse HER2-positive cancer cells, such as MCF-7 HER2 cells (genetically engineered to express HER2) and SK-BR-3 cells (27). HER2-targeted CAR-T cells and anti-PDL1 treatment together show a significant anti-tumor effect on HER2-positive breast cancer (48). However, HER2 targeting of CAR-T cells mediates off-target recognition in normal lung cells expressing HER2, in turn leading to multi-organ failure (41). Off-target toxicity of HER2-targeted CAR-T cells is challenging for achieving clinical utility of HER2-targeted CAR-T cells in breast cancer. Therefore, improving the therapeutic efficacy of HER2-targeted CAR-T cells in HER2-positive breast cancer is the research direction for treating HER2-positive breast cancer.
2.2.2 Progress in CAR-T therapy for treating HER2-positive breast cancer
As CAR-T cells targeting HER2 are immature for treating HER2-positive breast cancer, several new therapeutic ideas and approaches have been proposed to improve the therapeutic effects of HER-2-targeted CAR-T cells. For example, bispecific CAR-T cells have employed to treat HER2-positive breast cancer. More importantly, the emergence of Tandem CAR-T cells (TanCAR-T) may provide new ideas and approaches for treating HER2-positive breast cancer. These studies provide new research directions for CAR-T cell treatment of HER2-positive breast cancer and improve clinical outcomes.
2.2.2.1 Bispecific CAR-T cells
Bispecific CAR-T cells have shown tremendous progress in treating HER2-positive breast cancer. For instance, bispecific CAR-T cells targeting HER2 and the melanocytic protein (gp100) can eradicate various large solid tumors, including orthotopic mammary tumors expressing HER2 in the breast and brain of immunocompetent mice (49). Bispecific CAR-T cells targeting HER2 and MUC 1 showed cytotoxic activity in breast cancer (50). Thus, advancements in bispecific CAR-T cells are important for improving the therapeutic effect of HER2-targeted CAR-T cells in breast cancer.
2.2.2.2 TanCAR-T cells
A cutting-edge therapeutic approach is CAR-T cells with multi-targeting capabilities, carrying more than one specific CAR, to improve the ability to target tumor cells (51). TanCAR-T cells are a novel bispecific CAR containing two scFV domain junctions, allowing a single CAR-T cell to recognize multiple tumor antigens. The two specific CARs of TanCAR-T cells can synergistically enhance the activation of T cells when recognized simultaneously. For example, tandem CAR-T targeting HER2 and IL13Rα2 enhance the antitumor effect on glioblastoma (52). TanCAR cells targeting CD19 and HER2 lyse either HER2-positive or CD19-positive target cells, accompanied by the simultaneous secretion of IFN-γ and IL-2. The two specific CARs of tandem CAR-T cells enable near-free movement of TanCAR subunits and enhanced tandem recognition (53). Thus, TanCAR cells have shown great promise for their application in treating HER2-positive breast cancer. Construction of TanCAR-T cells targeting HER2 and tumor associated antigens (TAAs), expressed highly in breast cancer may provide novel approaches to improving HER2-positive breast cancer.
2.3 TNBC
TNBC accounts for approximately 30% of all breast cancer deaths (54). TNBC is highly invasive and distant metastatic, with a high histological grade compared to other subtypes of breast cancer (55). The main treatment for TNBC is postoperative adjuvant chemotherapy after curative surgical resection (56). However, the high recurrence rate and poor prognosis of TNBC remain challenging. Due to the lack of clear targets, treatment of TNBC is insensitive to targeted therapy. Anthracyclines and paclitaxel are chemotherapy regimens employed for TNBC. However, extreme toxicity and side effects do not improve patient survival (57). The FDA has recently approved PARP inhibitors, PD-1 inhibitors, and an anti-trop2 antibody-drug conjugate (sacituzumab govitecan) for the combination treatment of TNBC, benefiting more patients with TNBC (58–60). However, the adverse effects of PARP inhibitors in TNBC include gastrointestinal reactions, myelosuppression, and liver function impairment (61). Only a small proportion of patients benefit from PD-L1 inhibitor monotherapy (62). The anti-trop-2 antibody-drug conjugate shows adverse effects of neutropenia (39%), leukopenia (16%), anemia (14%), and diarrhea (13%) (63). Therefore, breakthrough evidence for CAR-T in solid tumors provides new opportunities for TNBC therapy.
We summarize the effective and potential targets of CAR-T cell therapy in TNBC in Table 1. Among them, the effective targets refer to TAAs in TNBC, which can recognize and activate CAR-T cells expressing a single specific CAR-To produce antitumor effects. These TAAs have been verified in clinical experiments or preclinical experiments. Similarly, potential targets refer to TAAs in TNBC, which can activate CAR-NK cells expressing a single specific CAR, or TanCAR-T cells expressing multiple specific CAR. Potential targets refer to TAAs in other solid tumors that can activate CAR-T cells expressing a single specific CAR, and these TAAs are highly expressed in TNBC. Although some studies suggest the potential targets of CAR for TNBC treatment, further investigations are needed to confirm their feasibility. This review attempts to facilitate the development of new ideas for subsequent research and existing clinical applications.
3 Effect of TME on CAR-T therapy for breast cancer
The tumor microenvironment (TME) is a dynamic cellular environment comprising innate and adaptive immune cells, stromal cells, vasculature, and acellular components, such as soluble factors, signaling molecules, and extracellular matrix (ECM) (128). TME is crucial in tumor angiogenesis, proliferation, metastasis, invasion, immune evasion, and therapeutic resistance (129). Hypoxia is a characteristic of TME. The hypoxic area is rich in MDSCs, TAMs, and Treg cells, which inhibit the activation, proliferation, and cytotoxicity of T cells, reducing the killing effect of immune response on tumor cells (130). Along with the selection of appropriate targets, the TME poses a challenge for the effective treatment of breast cancer. Targeting one or more components of the TME has research value for the treatment of malignancies. In the following sections, we present the effects of CAF, MDSCs, TAM, and Treg cells on CAR-T therapy in breast cancer treatment.
3.1 CAFs
CAFs are stromal cells in the breast TME, which are important for cancer progression and tumor drug resistance (131). CAFs are crucial in the TME as they participate in tumor proliferation, invasion, neoangiogenesis, inflammation, immune suppression, and the remodeling of the ECM (132). Wen et al. demonstrated that CAFs can promote tumor invasion in integrin β3-positive breast cancer cells. FAP is a therapeutic target for CAFs in the TME of HER2-positive breast cancer (133). Several studies have targeted the CAFs in the TME to improve breast cancer treatment. Rivas et al. found that targeted immunotherapy against CAFs overcame trastuzumab resistance in refractory HER2-positive breast tumors (134). In addition to treating HER2-positive breast tumors, FAP-targeted CAR-T cells can eliminate CAFs and enhance the antitumor effect in TNBC. Das et al. designed FAP-targeted CAR-T cells to ablate CAF, inhibit the recruitment of MDSC, and promote T cell infiltration, which enhanced the antitumor activity against TNBC (92). Therefore, regulating CAFs in the TME can improve the scope of CAR-T cells in breast cancer.
3.2 MDSCs
MDSCs, an important component of the TME, show increased levels in patients with breast cancer (135). In a xenograft mouse model constructed with TNBC cell lines, MDSCs promoted tumor progression, angiogenesis, tumor invasion, and increased metastasis (136). TR 2 expressed on MDSCs in the TME can bind TR2.41BB receptors leading to MDSC apoptosis. Therefore, treating breast cancer by targeting TR 2 has immense research potential (137). Nalawade et al. found that HER2-targeted CAR-T cells expressing the TR2.41BB costimulatory receptor exerted superior antitumor effects on HER2-positive breast cancer (138). Breast tumors recruit MDSCs to inhibit the activation and infiltration of T cells, which in turn affect the therapeutic efficacy of CAR-T cells. CAR-T cells targeting EGFR combined with olaparib or Poly I: C inhibit MDSC recruitment and enhance the efficacy of TNBC, wherein olaparib inhibit MDSCs through the SDF 1 a/CXCR 4 axis and increase the antitumor activity of CAR-T cell therapy (105, 106). Therefore, MDSCs have great research value in the application of CAR-T cells to treat breast cancer.
3.3 Tumor-associated macrophages
TAMs, a tumor-promoting cell type in the TME of breast cancer, include antitumor M1-like (M1-TAM) or pro-tumor M2-like (M2-TAM) TAM (139). Among them, M2-TAMs are the major components of the breast tumor stroma. TAMs are potential therapeutic targets in breast cancer. TAMs are involved in the growth, invasion, survival, angiogenesis, and metastasis of breast cancer cells (140, 141). For example, Liu et al. showed that the natural compound, emodin, blocked the epithelial mesenchymal transition (EMT) and cancer stem cell (CSC) formation in TAM-induced breast cancer cells (142). Meng et al. elucidated the molecular role of α PD-L1 in reversing TAM polarization toward the M2 phenotype in the TME of TNBC, providing novel therapeutic strategies for refractory TNBC (143). Combined treatment of FA-TLR 7-1A that transforms M2-like immune-suppressive myeloid cells into M1-like inflammatory myeloid cells with CD19-targeted CAR-T cells enhances the antitumor effects of 4T1-mCD19 breast cancer cells implanted in BALB/C mice. Therefore, CAR-T therapy has great potential for treating breast cancer by modulating TAMs.
3.4 Treg
Tregs are inhibitory CD4+ T cells regulated mainly by FoxP3 expression through the inhibition of T cell proliferation and secretion of inhibitory cytokines (144). Tregs are closely associated with the progression, metastasis, and local invasion of breast cancer. Núñez et al. found that Treg accumulation in patients with breast cancer was associated with breast cancer cell invasion and metastatic motility into draining lymph nodes (145). Qiu et al. demonstrated a positive correlation between CCL 5 expression levels and the degree of axillary lymph node metastasis in patients with breast cancer (146). Tregs are critical for establishing and maintaining the immunosuppressed TME to suppress antitumor immunity. Bai et al. showed that ANXA 1 enhanced the function of Treg cells and promoted the growth of breast cancer (147). Therefore, blocking ANXA 1 could reduce Treg cell function and suppress breast tumors. Small-molecule inhibitors of PDGFR β enhanced the antiproliferative activity of the anti-PD-L1 mAb against human and murine TNBC cells by reducing FOXP3 + Treg cells. Therefore, regulating the levels of Treg in the TME may be important for improving the effect of CAR-T cells in breast cancer treatment.
4 Toxic effects and limitations of CAR-T therapy in breast cancer
Despite the great success in tumor treatment, CAR-T therapy faces the challenges such as cytokine release syndrome (CRS), off-target effect, CAR-T cell exhaustion, and immunosuppression. Statistically, almost two-thirds of patients treated with CAR-T develop CRS, resulting in a cytokine storm, organ failure, and even death (148). When CAR-T cells were cocultured with 4T1-EGFR cells in vitro, they produce IL-2, IFN- γ, TNF- α, and granzyme B, which is a sign of CRS (107). CAR-T cells generated from Th/Tc17 cells combined with STING agonists or cGAMP greatly enhanced the antitumor effects on breast cancer in vivo, however, treatment efficacy correlated with the development of CRS (149). Although CAR-T treatment specifically kills tumor cells expressing target antigen, unexpectedly, CAR-T cells injected into the body kill normal cells expressing the target antigen, causing serious side effects (150). The first clinical trial using HER2-targeted CAR-T cells for the treatment of metastatic HER2-positive breast cancer (NCT00924287) was terminated due to organ failure of normal HER2-expressing lung tissue by off-target recognition (151). In addition, maintaining the CAR persistence force of T cells in vivo remains challenging. Due to continuous antigen stimulation, the expression of inhibitory receptors of the CAR construct was increased, which cause CAR-T cell exhaustion resulting in a dysfunctional state in the absence of antigen-specific T cells (152). Excitingly, the latest research developed an endogenous signaling molecule activating (ESMA) CARs which triggers robust cytotoxic activity and proliferation of the T cells when directed against the TNBC cell line MDA-MB-231 while exhibiting reduced cytokine secretion and exhaustion marker expression (153). This novel CAR design may provide a new approach to overcome T cell exhaustion. Thus, there are still several challenges to CAR-T therapy in treating breast cancer. To improve the role of CAR-T therapy in breast cancer treatment and reduce the toxic side effects of CAR-T therapy, identifying specific targets and developing persistent CAR-T cells are necessary. Accordingly, some studies have broken the innovative idea based on the existing CAR-T cell therapy, choosing to express CAR-through gene editing in other cells, such as NK cells, macrophages, and MSC surface. This breakthrough has yielded great results.
5 Modern advancements in CAR-based immunotherapy in breast cancer
In addition to CAR-T therapy, NK cells, macrophages, and mesenchymal stem cells (MSCs) can be modified using CAR-To serve as therapeutic tools for tumors (Figure 1). In the treatment of hematological tumors, specifically targeted CAR macrophages (CAR-Ms) can produce superior antitumor effects against CD19-positive ALL cancer cells and HER2-positive human chronic myeloid leukemia passage cells (154). Specific CAR-macrophages (CAR-Ms) can exert antitumor effects in solid tumors, such as in the HER2-positive ovarian cancer cell line, SKOV3, GD2-expressing neuroblastoma, and GD2-expressing melanomas (155–157).Thus, CAR-NK cells, CAR-M, and CAR-MSCs are beneficial for breast cancer treatment. We list effective targets in CAR-based immunotherapy for breast cancer in Table 2 and summarize the progress.
5.1 CAR-Ms
Based on the specificity of CAR, CAR-M results in antigen-specific phagocytosis and tumor clearance (26, 165). CAR-Ms express pro-inflammatory cytokines and chemokines, resulting in the transition of M2 macrophages into a pro-inflammatory (M1) phenotype, induction of a pro-inflammatory TME, and resistance to the effects of immunosuppressive cytokines (155). CAR-Ms have shown advancements in the treatment of breast cancer. Duan et al. found that VEGFR-targeted CAR macrophages activated by TLR 4 or IFN- γ receptors exerted antitumor effects in 4T1 breast cancer-bearing mice (164). Clinical experiments on CAR-M targeting MSLN for breast cancer are underway and may provide new methods for the treatment of breast cancer using CAR-M (163).
5.2 CAR-NK cells
Genetically engineered NK cells can express CAR. These CAR-NK cells can specifically recognize and kill tumor cells (166). Similar to CAR-T cells, the CAR structure of CAR-NK cells comprises an extracellular antigen-binding region, transmembrane domain, and intracellular activation domain (167). Due to the difference in the extracellular domain, CAR-NK cells can identify different targets to treat breast cancer. CAR-NK cells targeting CD44v6, HER2, TF, B7-H6, EGFR, and PD-L1 have been successfully used for treating different types of breast cancer (66, 127, 158, 161, 162). Raftery et al. demonstrated the significant anti-tumor effect of CD44v6-targeted CAR-NK cells in TNBC (66). Hu confirmed the ability of TF as a novel target for CAR-NK cell immunotherapy in TNBC. TF-targeted antibody-like immunoconjugate (L-ICON) enhanced its efficacy in vitro (127). Lin et al. found that targeting B7-H6 CAR-NK cells induced the death of fulvestrant-resistant breast cancer cells (158). CAR-NK cells can be used to treat HER2-positive breast cancer. CAR-NK cells targeting HER2 produced stronger antitumor effects on the HER2-expressing breast cancer cell lines, BT-474, SKBR 3, and MDA-MB453 (161). Compared with the HER2-targeted CAR-NK cells, Xia et al. found that HER2-targeted CAR-NK cells coexpressing sPD-1 exerted greater cytotoxicity against HER2-positive breast cancer cells with high HER2 and PD-L1 expression (162).
HER2-targeted CAR-NK cells showed stronger anti-tumor effects in HER2-positive breast cancer compared with HER2-targeted CAR-T cells. HER2-targeted CAR-NK cells maintained high cytotoxic functions in the presence of immunosuppressive factors enriched in the solid tumor TME. HER2-targeted CAR-NK cells did not cause off-target toxicity to human lung epithelial cells physically expressing HER2, which may predict the potential superiority of HER2-targeted CAR-NK cells in treating breast cancer (161).
The TME can affect the antitumor function of CAR-NK cells (168). CAR-NK cells target the matrix components to remodel the TME. Fabian et al. demonstrated that NK cells targeting PD-L1 cured TNBC by killing MDSCs (159). CAR-NK cells show good curative effect in treating distant metastatic lesions of breast cancer. Chen et al. demonstrated that CAR-NK cells targeting EGFR treated brain metastasis in breast cancer (160). Thus, the progress of CAR-NK cells in breast cancer treatment provides new ideas and methods for its clinical treatment.
5.3 CAR-MSCs
MSCs are involved in immune response, secrete cytokines, and enhance healing (169). MSCs target different antigens by genetically engineering expression-specific CAR, providing new ideas and approaches for cancer treatment. Golinelli et al. found that GD2-targeted CAR-MSC expressing TRAIL exerted antitumor effects on GD2-positive Ewing sarcoma (ES) and glioblastoma (GBM) (170, 171). Although studies on CAR-MSC therapy are scarce, its great potential in the treatment of breast cancer has been suggested.
6 Clinical studies on CAR-based immunotherapy for breast cancer
Ongoing clinical studies on CAR-based immunotherapy in breast cancer are presented in the table below. These include only one CAR-NK cell and one CAR-M cell therapy for breast cancer, and most of them are CAR-T cell therapies in the clinical studies of breast cancer treatment. With the development of clinical research, we believe that CAR-based immunotherapy can provide a reference for subsequent experimental research and contribute to the development of clinical treatment. Most clinical experiments are studies on the treatment of TNBC and HER2-positive breast cancer. Among them, only one is a study on CAR-M, and one is on CAR-NK cell therapy for breast cancer. Clinical experiments with CAR-TILs are underway, which may provide new perspectives for the treatment of breast cancer. With the development of clinical experiments, the application of CAR-based immunotherapy in breast cancer treatment can provide us with new experimental evidence and reference. We used (https://clinicaltrials.gov/search? Cond = Breast% 20 Cancer & amp; intr = CAR-T) for exploring clinical trials for breast cancer, and Table 3 provides a reference.
7 Therapeutic applications of CAR exosomes in breast cancer
Exosomes, comprising lipid bilayers, can transfer miRNA, mRNA, and proteins from the original cells to the corresponding target cells (172). Exosomes secreted by cells can be transmitted to target cells through the humor, causing changes in the physiological functions of target cells (173). Similarly, exosomes are crucial in tumor cell invasion and metastasis (174). Based on the disease-treating properties of exosomes, CAR-based cell-derived exosomes may have great potential for breast cancer (175). Interestingly, exosomal expression shows the same level of CAR as the protocells (27). Based on this feature, CAR-T cell-derived exosomes and CAR-NK cell-derived exosomes exerted antitumor effects in breast cancer cell lines (Table 4). Therefore, exosomes carrying CAR may provide new therapeutic means for breast cancer treatment. Exosomes carrying CAR offer hope for patients with breast cancer and may provide solutions to overcome the challenge of expense of CAR-T therapy and its wide accessibility (Figure 1).
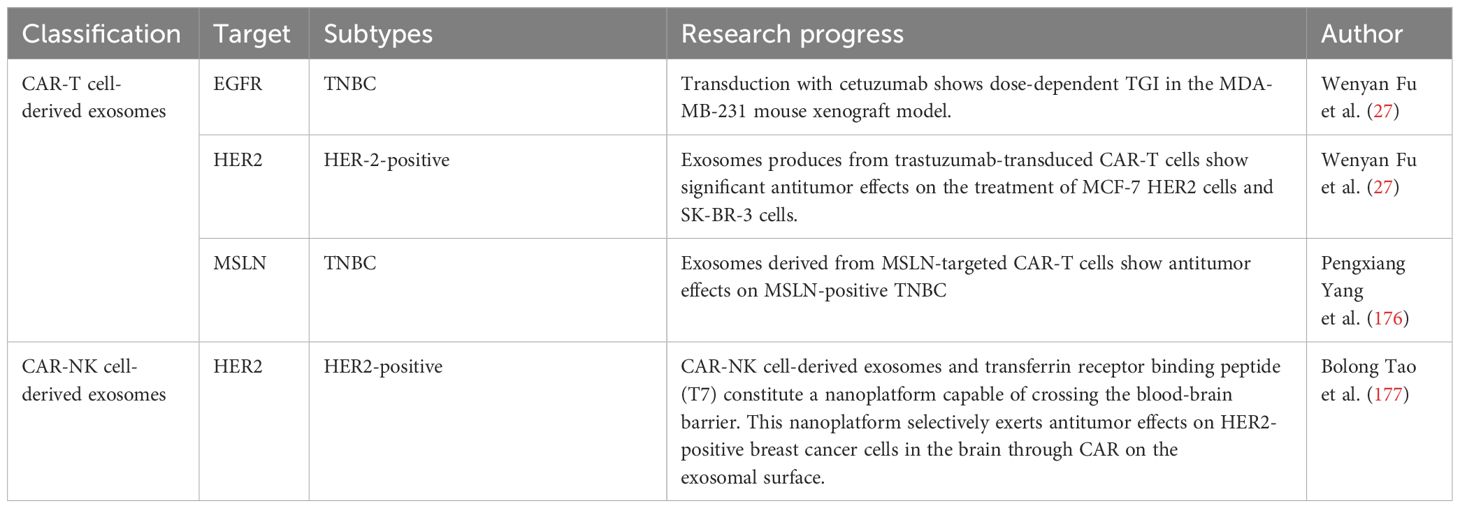
Table 4 Summary of advancements in research on CAR-T cell-derived exosomes and CAR-NK cell-derived exosomes for breast cancer.
Production and killing processes of CAR-M, CAR-NK, CAR-MSC, CAR-T cell-derived exosomes, and CAR-NK cell-derived exosomes. Macrophages are genetically engineered to express specific CARs. After CAR-M recognizes and phagocytoses tumor cells expressing a specific antigen, CAR-M cells present and activate T cells. Activated T cells exert anti-tumor effects. NK cells, T cells, and MSCs have been genetically engineered to express CAR. CAR-NK cells and CAR-MSC recognize and kill tumor cells. CAR-NK cells and CAR-T cell-derived exosomes can exert killing effects on tumor cells and are not inhibited by PDL 1.
7.1 CAR-T cell-derived exosomes in breast cancer
CAR expression has been investigated in CAR-T cell-derived exosomes. CAR-T cell-derived exosomes carry the same CAR as CAR-T cells, at comparable levels (27). Antigen-stimulated CAR-T cells show higher CAR content than exosomes stimulated with CD3/CD28 beads (27).With the deepening of research, the application scheme of CAR-T cell exosomes is gradually maturing. Peripheral blood samples from patients with cancer have been separated by density and co-cultured with CD3 and CD28 antibodies. Subsequently, CAR-T cells have been prepared using viral or non-viral transfection techniques and expanded in vitro in the presence of IL-2. CAR-T cell-derived exosomes have been isolated from the culture medium (178). CAR-T cell-derived exosomes have strong and specific cytotoxic activity against cancer cells. FasL, Apo 2 L, perforin, granzyme A and B play important roles (179).With the CAR and cytotoxic activities of exosomes, CAR-T cell-derived exosomes can be used to treat tumors. In the treatment of hematological tumors, targeting CD19 CAR-T cell-derived exosomes induce mRNA expression of the pro-apoptotic genes, BAD, BAX, and caspase-3 in leukemic B cells without the accompanying signs of cytotoxicity (180). CAR-T cell-derived exosomes can deliver biologically small molecules to the target cells, leading to changes in their physiological functions. For example, RN7SL1 is an RNA that can activate the RIG-I/MDA 5 signaling pathway to promote CAR-T cell expansion and effector memory differentiation (181). RN7SL1 improves the immunostimulatory properties of myeloid and DC subsets through the transfer of CAR-T cell-derived exosomes to MDSC (182). CAR-T cell-derived exosomes are less studied in drug transport. Therefore, the use of CAR-T-derived exosomes for transport drugs has great potential in the field of tumor therapy.
CAR exosomes have made tremendous progress in breast cancer treatment. With its specific CAR, CAR-T cell-derived exosomes transduced with cetuximab or trastuzumab demonstrated significant antitumor activity. Among them, exosomes expressing cetuximab scfv exerted dose-dependent tumor growth inhibition (TGI) against an EGFR-positive MDA-MB-231 mouse xenograft model. In contrast, those expressing trastuzumab scfv showed significant antitumor effects on HER-2-positive MCF-7 cells and SK-BR-3 cells in breast cancer tumor growth inhibition (TGI) (183). Yang et al. found that exosomes derived from MSLN-targeted CAR-T cells retained CAR-targeting MSLN. Exosomes carrying this CAR exerted antitumor effects on MSLN-positive TNBC without significant side effects. Retaining MSLN-targeted CAR, exosomes derived from MSLN-targeted CAR-T cells exerted significant antitumor effects on MSLN-positive TNBC without any significant side effects (176).
CAR-T cell-derived exosomes show several significant advantages. First, PD-L1 inhibited the antitumor immunity of CAR-T cells but did not affect exosomes. No CRS and off-tumor responses were observed after CAR exosomal immunotherapy. Exosomes can cross the blood-brain barrier, which may provide new therapeutic approaches for the treatment of intracranial metastases (27). CAR-T cell-derived exosomes are superior for solid tumors compared with CAR-T cells (184). In conclusion, CAR-T cell-derived exosomes possess specific cytotoxic activity against breast cancer cells and have great research potential in the development of novel breast cancer therapies.
7.2 CAR-NK cell-derived exosomes for the treatment of breast cancer
In addition to CAR-T cell-derived exosomes, CAR-NK cell-derived exosomes, an important component of immune cell function, carry several perforins and granzymes for the treatment of malignant tumors (185). CAR-NK are off-the-shelf cell therapies for solid tumors. For example, brain metastasis of HER2-positive breast cancer (HER2-positive BCBM) is a refractory malignancy with a high recurrence rate and poor prognosis. Interestingly, the CAR-NK cell-derived exosomes and transferrin receptor binding peptide (T7) can constitute a nanoplatform capable of crossing the blood-brain barrier. This nanoplatform selectively exerts antitumor effects on HER2-positive breast cancer cells in the brain through CAR on the surface of the exosomes (177). Studies on CAR-NK cell-derived exosomes are scarce, and there is great potential to investigate the therapeutic application of CAR-NK cell-derived exosomes in breast cancer.
8 Discussion
As the most common malignant tumor in women, the high incidence and mortality rate of breast cancer remains a pressing issue. With the increase in the number of clinical studies, although CAR-T therapy has achieved good therapeutic results in lumen type A, its therapeutic application in lumen B has not been reported. No CAR-T therapy for Luminal A and Luminal B has reached the clinical study stage, and huge gaps need to be supplemented. As for the therapeutic application of CAR-T cell therapy in HER2-positive breast cancer, although HER2-targeted CAR can effectively produce an antitumor effect on HER-2-positive breast cancer, the off-target effects in clinical research remains a difficult problem. Despite studies on immunomodulators, BsAbs, TanCAR, bi-specific CAR-T cells, and TME to improve the therapeutic status of HER2-targeted CAR-T cells, a recognized effective approach to improve HER2-targeted CAR-T cells for HER-2-positive breast cancer is lacking. For TNBC treatment, new effective targets of CAR-T cells are constantly being investigated. New potential targets await experimental confirmation. Clinical experiments of CAR-T cells for breast cancer are few and there is massive scope for development. We believe that with the increase of animal experiments and clinical experiments, CAR-T cell therapy with higher efficacy and fewer side effects will be applied to breast cancer treatment.
In addition to the booming development of CAR-T cell therapy in cancer treatment, research on CAR-NK cells, CAR-M, and CAR-MSC has made great progress in recent years. Although CAR-NK cells targeting CD44v6, HER2, TF, B7-H6, EGFR, and PD-L1 have made great progress in breast cancer, developing targets for CAR-NK cells in breast cancer has great research potential. CAR-Ms targeting VEGFR and MSLN have been investigated for breast cancer treatment, and suitable targets await further investigation. CAR-MSCs can be used to treat solid tumor therapy, which may provide new avenues for breast cancer treatment and merit further investigation. Although there are a few clinical studies on CAR-NK cells and CAR-Ms, their clinical effects await validation. Moreover, there are no studies of CAR-MSC for breast cancer and there is immense potential.
CAR-based immunotherapy faces great challenges in breast cancer treatment. On the one hand, the common toxic effects and limitations of CAR-T therapy hinder its application, and the development of more specific CAR-T cells remains a challenge. On the other hand, although CAR-NK and CAR-Ms have achieved more significant therapeutic effects in breast cancer treatment compared to CAR-T cells, clinical studies on their side effects are scarce. Therefore, the side effects of CAR-based immunotherapy merit further investigations to ensure that this treatment is effective and safe before entering the clinical stage.
In addition to studying specific targets to kill breast cancer cells, the TME of breast cancer is equally important in CAR-based immunotherapy and can serve as an adjuvant. Although CAFs, MDSCs, TAMs and Tregs in the TME exert a therapeutic effect, evidence remains scarce, especially for Tregs and TAMs. TME regulation can produce a synergistic effect on CAR-based immunotherapy and enhance the anti-tumor effect.
Interestingly, CAR-T cell-derived exosomes and CAR-NK cell-derived exosomes have been successfully used for breast cancer treatment, and show many advantages besides good anti-tumor effects. However, there are few studies on CAR-T cell-derived exosomes and CAR-NK cell-derived exosomes for breast cancer and no clinical studies. Exosomes expressing CAR can be used as an effective and extensive tool for breast cancer treatment.
In conclusion, we firmly believe that the present use of the target development and clinical research of CAR-based immunotherapy in breast cancer therapy could benefit a larger number of patients. The emergence of CAR exosomes may provide a more efficient, widely applicable, and low-cost tool for breast cancer treatment. In the future, CAR-based immunotherapy for breast cancer treatment is expected to benefit more patients.
Author contributions
ZN: Writing – original draft, Conceptualization. JW: Writing – original draft. QZ: Writing – original draft. PZ: Writing – original draft. JZ: Writing – review and editing, Data curation. YY: Writing – review & editing, Conceptualization, Funding acquisition, Supervision.
Funding
The author(s) declare financial support was received for the research, authorship, and/or publication of this article. This work received financial support from the National Natural Science Foundation of China (Grant No. 82203696), the Education Department of Jilin Province (Grant No. JJKH20220973KJ), the Health Science and Technology Capacity Improvement Project of Jilin Province (Grant No. 2022JC079).
Conflict of interest
The authors declare that the research was conducted in the absence of any commercial or financial relationships that could be construed as a potential conflict of interest.
Publisher’s note
All claims expressed in this article are solely those of the authors and do not necessarily represent those of their affiliated organizations, or those of the publisher, the editors and the reviewers. Any product that may be evaluated in this article, or claim that may be made by its manufacturer, is not guaranteed or endorsed by the publisher.
References
1. Andrianto A, Sudiana IK, Suprabawati DGA, Notobroto HB. Immune system and tumor microenvironment in early-stage breast cancer: different mechanisms for early recurrence after mastectomy and chemotherapy on ductal and lobular types. F1000Res. (2023) 12:841. doi: 10.12688/f1000research.134302.2
2. Sung H, Ferlay J, Siegel RL, Laversanne M, Soerjomataram I, Jemal A, et al. Global cancer statistics 2020: GLOBOCAN estimates of incidence and mortality worldwide for 36 cancers in 185 countries. CA Cancer J Clin. (2021) 71:209–49. doi: 10.3322/caac.21660
3. Yang YH, Liu JW, Lu C, Wei JF. CAR-T cell therapy for breast cancer: from basic research to clinical application. Int J Biol Sci. (2022) 18:2609–26. doi: 10.7150/ijbs.70120
4. Matsunuma R, Watanabe T, Hozumi Y, Koizumi K, Ito Y, Maruyama S, et al. Preoperative concurrent endocrine therapy with chemotherapy in luminal B-like breast cancer. Breast Cancer. (2020) 27:819–27. doi: 10.1007/s12282-020-01077-0
5. Lange CA, Yee D. Killing the second messenger: targeting loss of cell cycle control in endocrine-resistant breast cancer. Endocr Relat Cancer. (2011) 18:C19–24. doi: 10.1530/ERC-11-0112
6. Herr D, Wischnewsky M, Joukhadar R, Chow O, Janni W, Leinert E, et al. Does chemotherapy improve survival in patients with nodal positive luminal A breast cancer? A retrospective Multicenter Study. PloS One. (2019) 14:e0218434. doi: 10.1371/journal.pone.0218434
7. Gajria D, Chandarlapaty S. HER2-amplified breast cancer: mechanisms of trastuzumab resistance and novel targeted therapies. Expert Rev Anticancer Ther. (2011) 11:263–75. doi: 10.1586/era.10.226
8. Hodeib M, Serna-Gallegos T, Tewari KS. A review of HER2-targeted therapy in breast and ovarian cancer: lessons from antiquity - CLEOPATRA and PENELOPE. Future Oncol. (2015) 11:3113–31. doi: 10.2217/fon.15.266
9. Kreutzfeldt J, Rozeboom B, Dey N, De P. The trastuzumab era: current and upcoming targeted HER2+ breast cancer therapies. Am J Cancer Res. (2020) 10:1045–67.
10. Ferrari P, Scatena C, Ghilli M, Bargagna I, Lorenzini G, Nicolini A. Molecular mechanisms, biomarkers and emerging therapies for chemotherapy resistant TNBC. Int J Mol Sci. (2022) 23. doi: 10.3390/ijms23031665
11. Liu Q, Li J, Zheng H, Yang S, Hua Y, Huang N, et al. Adoptive cellular immunotherapy for solid neoplasms beyond CAR-T. Mol Cancer. (2023) 22:28. doi: 10.1186/s12943-023-01735-9
12. Bonini C, Chapuis AG, Hudecek M, Guedan S, Magnani C, Qasim W. Genome editing in engineered T cells for cancer immunotherapy. Hum Gene Ther. (2023) 34:853–69. doi: 10.1089/hum.2023.128
13. Benmebarek MR, Karches CH, Cadilha BL, Lesch S, Endres S, Kobold S. Killing mechanisms of chimeric antigen receptor (CAR) T cells. Int J Mol Sci. (2019) 20(6). doi: 10.3390/ijms20061283
14. Luksik AS, Yazigi E, Shah P, Jackson CM. CAR T cell therapy in glioblastoma: overcoming challenges related to antigen expression. Cancers. (2023) 15(5). doi: 10.3390/cancers15051414
15. Heitzeneder S, Bosse KR, Zhu Z, Zhelev D, Majzner RG, Radosevich MT, et al. GPC2-CAR T cells tuned for low antigen density mediate potent activity against neuroblastoma without toxicity. Cancer Cell. (2022) 40(1):53–69.e9. doi: 10.1016/j.ccell.2021.12.005
16. Schoutrop E, Poiret T, El-Serafi I, Zhao Y, He R, Moter A, et al. Tuned activation of MSLN-CAR T cells induces superior antitumor responses in ovarian cancer models. J Immunother Cancer. (2023) 11(2). doi: 10.1136/jitc-2022-005691
17. Zhang Q, Zhang Z, Liu G, Li D, Gu Z, Zhang L, et al. B7-H3 targeted CAR-T cells show highly efficient anti-tumor function against osteosarcoma both in vitro and in vivo. BMC Cancer. (2022) 22(1):1124. doi: 10.1186/s12885-022-10229-8
18. Krishnamurthy J, Rabinovich BA, Mi T, Switzer KC, Olivares S, Maiti SN, et al. Genetic engineering of T cells to target HERV-K, an ancient retrovirus on melanoma. Clin Cancer Res. (2015) 21(14):3241–51. doi: 10.1158/1078-0432.CCR-14-3197
19. Li X, Berahovich R, Zhou H, Liu X, Li F, Xu S, et al. PLAP -CAR T cells mediate high specific cytotoxicity against colon cancer cells. Front Biosci (Landmark Ed). (2020) 25(9):1765–86. doi: 10.2741/4877
20. Fan J, Yu Y, Yan L, Yuan Y, Sun B, Yang D, et al. GAS6-based CAR-T cells exhibit potent antitumor activity against pancreatic cancer. J Hematol Oncol. (2023) 16(1):77. doi: 10.1186/s13045-023-01467-9
21. Fang L, Tian W, Zhang C, Wang X, Li W, Zhang Q, et al. Oncolytic adenovirus-mediated expression of CCL5 and IL12 facilitates CA9-targeting CAR-T therapy against renal cell carcinoma. Pharmacol Res. (2023) 189:106701. doi: 10.1016/j.phrs.2023.106701
22. Makkouk A, Yang XC, Barca T, Lucas A, Turkoz M, Wong JTS, et al. Off-the-shelf Vδ1 gamma delta T cells engineered with glypican-3 (GPC-3)-specific chimeric antigen receptor (CAR) and soluble IL-15 display robust antitumor efficacy against hepatocellular carcinoma. J Immunother Cancer. (2021) 9(12). doi: 10.1136/jitc-2021-003441
23. Qi C, Gong J, Li J, Liu D, Qin Y, Ge S, et al. Claudin18.2-specific CAR T cells in gastrointestinal cancers: phase 1 trial interim results. Nat Med. (2022) 28(6):1189–98. doi: 10.1038/s41591-022-01800-8
24. Cao B, Liu M, Wang L, Zhu K, Cai M, Chen X, et al. Remodelling of tumour microenvironment by microwave ablation potentiates immunotherapy of AXL-specific CAR T cells against non-small cell lung cancer. Nat Commun. (2022) 13(1):6203. doi: 10.1038/s41467-022-33968-5
25. Yin L, Chen GL, Xiang Z, Liu YL, Li XY, Bi JW, et al. Current progress in chimeric antigen receptor-modified T cells for the treatment of metastatic breast cancer. BioMed Pharmacother. (2023) 162:114648. doi: 10.1016/j.biopha.2023.114648
26. Huo Y, Zhang H, Sa L, Zheng W, He Y, Lyu H, et al. M1 polarization enhances the antitumor activity of chimeric antigen receptor macrophages in solid tumors. J Transl Med. (2023) 21(1):225. doi: 10.1186/s12967-023-04061-2
27. Fu W, Lei C, Liu S, Cui Y, Wang C, Qian K, et al. CAR exosomes derived from effector CAR-T cells have potent antitumour effects and low toxicity. Nat Commun. (2019) 10(1):4355. doi: 10.1038/s41467-019-12321-3
28. Zheng W, Zhu T, Tang L, Li Z, Jiang G, Huang X. Inhalable CAR-T cell-derived exosomes as paclitaxel carriers for treating lung cancer. J Transl Med. (2023) 21(1):383. doi: 10.1186/s12967-023-04206-3
29. Xie X, Li X, Liu G, Zhao H, Zhou Z, Xiong S. T cells expressing a HER2-specific chimeric antigen receptor as treatment for breast cancer. J Cancer Res Clin Oncol. (2023) 149(13):11561–70. doi: 10.1007/s00432-023-04996-5
30. Corti C, Venetis K, Sajjadi E, Zattoni L, Curigliano G, Fusco N. CAR-T cell therapy for triple-negative breast cancer and other solid tumors: preclinical and clinical progress. Expert Opin Investig Drugs. (2022) 31(6):593–605. doi: 10.1080/13543784.2022.2054326
31. Li ZH, Hu PH, Tu JH, Yu NS. Luminal B breast cancer: patterns of recurrence and clinical outcome. Oncotarget. (2016) 7(40):65024–33. doi: 10.18632/oncotarget.11344
32. Gao JJ, Swain SM. Luminal A breast cancer and molecular assays: A review. Oncologist. (2018) 23(5):556–65. doi: 10.1634/theoncologist.2017-0535
33. Erber R, Kailayangiri S, Huebner H, Ruebner M, Hartmann A, Häberle L, et al. Variable expression of the disialoganglioside GD2 in breast cancer molecular subtypes. Cancers (Basel). (2021) 13(21). doi: 10.3390/cancers13215577
34. Seitz CM, Schroeder S, Knopf P, Krahl AC, Hau J, Schleicher S, et al. GD2-targeted chimeric antigen receptor T cells prevent metastasis formation by elimination of breast cancer stem-like cells. Oncoimmunology. (2020) 9(1):1683345. doi: 10.1080/2162402X.2019.1683345
35. Zhang Q, Liu G, Liu J, Yang M, Fu J, Liu G, et al. The antitumor capacity of mesothelin-CAR-T cells in targeting solid tumors in mice. Mol Ther Oncolytics. (2021) 20:556–68. doi: 10.1016/j.omto.2021.02.013
36. Bajor M, Graczyk-Jarzynka A, Marhelava K, Burdzinska A, Muchowicz A, Goral A, et al. PD-L1 CAR effector cells induce self-amplifying cytotoxic effects against target cells. J Immunother Cancer. (2022) 10(1). doi: 10.1136/jitc-2021-002500
37. Zhao Z, Li Y, Liu W, Li X. Engineered IL-7 receptor enhances the therapeutic effect of AXL-CAR-T cells on triple-negative breast cancer. BioMed Res Int. (2020) 2020:4795171. doi: 10.1155/2020/4795171
38. Zhou L, Wu J, Ruan M, Xiao Y, Lan H, Wu Q. The loss of B7-H4 expression in breast cancer cells escaping from T cell cytotoxicity contributes to epithelial-to-mesenchymal transition. Breast Cancer Res. (2023) 25(1):115. doi: 10.1186/s13058-023-01721-5
39. Cui Y, Yuan T, Wang Y, Zheng D, Qin L, Li S, et al. T lymphocytes expressing the switchable chimeric Fc receptor CD64 exhibit augmented persistence and antitumor activity. Cell Rep. (2023) 42(7):112797. doi: 10.1016/j.celrep.2023.112797
40. de Melo Gagliato D, Jardim DL, Marchesi MS, Hortobagyi GN. Mechanisms of resistance and sensitivity to anti-HER2 therapies in HER2+ breast cancer. Oncotarget. (2016) 7(39):64431–46. doi: 10.18632/oncotarget.7043
41. Duro-Sánchez S, Alonso MR, Arribas J. Immunotherapies against HER2-positive breast cancer. Cancers (Basel). (2023) 15(4). doi: 10.3390/cancers15041069
42. Najjar MK, Manore SG, Regua AT, Lo HW. Antibody-drug conjugates for the treatment of HER2-positive breast cancer. Genes (Basel). (2022) 13(11). doi: 10.3390/genes13112065
43. von Minckwitz G, Procter M, de Azambuja E, Zardavas D, Benyunes M, Viale G, et al. Adjuvant pertuzumab and trastuzumab in early HER2-positive breast cancer. N Engl J Med. (2017) 377(2):122–31. doi: 10.1056/NEJMoa1703643
44. Mandó P, Rivero SG, Rizzo MM, Pinkasz M, Levy EM. Targeting ADCC: A different approach to HER2 breast cancer in the immunotherapy era. Breast. (2021) 60:15–25. doi: 10.1016/j.breast.2021.08.007
45. Qian Y, Peng Y, Zhou H, Zhang L, Yuan Y. Trastuzumab plus pertuzumab in combination with chemotherapy in metastatic HER2-positive breast cancer: a retrospective single-armed cohort study in China. Ann Trans Med. (2022) 10(16):877. doi: 10.21037/atm-22-3592
46. Shyam Sunder S, Sharma UC, Pokharel S. Adverse effects of tyrosine kinase inhibitors in cancer therapy: pathophysiology, mechanisms and clinical management. Signal Transduction Targeted Ther. (2023) 8(1):262. doi: 10.1038/s41392-023-01469-6
47. Yang Y, Sun Q, Deng Z, Shi W, Cheng H. Cbl induced ubiquitination of HER2 mediate immune escape from HER2-targeted CAR-T. J Biochem Mol Toxicol. (2023) 37(10):e23446. doi: 10.1002/jbt.23446
48. Li P, Yang L, Li T, Bin S, Sun B, Huang Y, et al. The third generation anti-HER2 chimeric antigen receptor mouse T cells alone or together with anti-PD1 antibody inhibits the growth of mouse breast tumor cells expressing HER2 in vitro and in immune competent mice. Front Oncol. (2020) 10:1143. doi: 10.3389/fonc.2020.01143
49. Slaney CY, von Scheidt B, Davenport AJ, Beavis PA, Westwood JA, Mardiana S, et al. Dual-specific chimeric antigen receptor T cells and an indirect vaccine eradicate a variety of large solid tumors in an immunocompetent, self-antigen setting. Clin Cancer Res. (2017) 23(10):2478–90. doi: 10.1158/1078-0432.CCR-16-1860
50. Wilkie S, van Schalkwyk MC, Hobbs S, Davies DM, van der Stegen SJ, Pereira AC, et al. Dual targeting of ErbB2 and MUC1 in breast cancer using chimeric antigen receptors engineered to provide complementary signaling. J Clin Immunol. (2012) 32(5):1059–70. doi: 10.1007/s10875-012-9689-9
51. Tahmasebi S, Elahi R, Khosh E, Esmaeilzadeh A. Programmable and multi-targeted CARs: a new breakthrough in cancer CAR-T cell therapy. Clin Transl Oncol. (2021) 23(6):1003–19. doi: 10.1007/s12094-020-02490-9
52. Hegde M, Mukherjee M, Grada Z, Pignata A, Landi D, Navai SA, et al. Tandem CAR T cells targeting HER2 and IL13Rα2 mitigate tumor antigen escape. J Clin Invest. (2016) 126(8):3036–52. doi: 10.1172/JCI83416
53. Grada Z, Hegde M, Byrd T, Shaffer DR, Ghazi A, Brawley VS, et al. TanCAR: A novel bispecific chimeric antigen receptor for cancer immunotherapy. Mol Ther Nucleic Acids. (2013) 2(7):e105. doi: 10.1038/mtna.2013.32
54. Abuhadra N, Stecklein S, Sharma P, Moulder S. Early-stage triple-negative breast cancer: time to optimize personalized strategies. Oncologist. (2022) 27(1):30–9. doi: 10.1093/oncolo/oyab003
55. Bayraktar S, Glück S. Molecularly targeted therapies for metastatic triple-negative breast cancer. Breast Cancer Res Treat. (2013) 138(1):21–35. doi: 10.1007/s10549-013-2421-5
56. Li Y, Zhang H, Merkher Y, Chen L, Liu N, Leonov S, et al. Recent advances in therapeutic strategies for triple-negative breast cancer. J Hematol Oncol. (2022) 15(1):121. doi: 10.1186/s13045-022-01341-0
57. Furlanetto J, Loibl S. Optimal systemic treatment for early triple-negative breast cancer. Breast Care (Basel). (2020) 15(3):217–26. doi: 10.1159/000508759
58. Luo L, Keyomarsi K. PARP inhibitors as single agents and in combination therapy: the most promising treatment strategies in clinical trials for BRCA-mutant ovarian and triple-negative breast cancers. Expert Opin Investig Drugs. (2022) 31(6):607–31. doi: 10.1080/13543784.2022.2067527
59. Chen X, Feng L, Huang Y, Wu Y, Xie N. Mechanisms and strategies to overcome PD-1/PD-L1 blockade resistance in triple-negative breast cancer. Cancers (Basel). (2022) 15(1). doi: 10.3390/cancers15010104
60. Furlanetto J, Marmé F, Loibl S. Sacituzumab govitecan: past, present and future of a new antibody-drug conjugate and future horizon. Future Oncol. (2022) 18(28):3199–215. doi: 10.2217/fon-2022-0407
61. Yang R, Shi Y-Y, Han X-H, Liu S. The impact of platinum-containing chemotherapies in advanced triple-negative breast cancer: meta-analytical approach to evaluating its efficacy and safety. Oncol Res Treat. (2021) 44(6):333–43. doi: 10.1159/000515353
62. Li L, Zhang F, Liu Z, Fan Z. Immunotherapy for triple-negative breast cancer: combination strategies to improve outcome. Cancers. (2023) 15(1). doi: 10.3390/cancers15010321
63. Bardia A, Mayer IA, Diamond JR, Moroose RL, Isakoff SJ, Starodub AN, et al. Efficacy and safety of anti-trop-2 antibody drug conjugate sacituzumab govitecan (IMMU-132) in heavily pretreated patients with metastatic triple-negative breast cancer. J Clin Oncol Off J Am Soc Clin Oncol. (2017) 35(19):2141–8. doi: 10.1200/JCO.2016.70.8297
64. Zaib T, Cheng K, Liu T, Mei R, Liu Q, Zhou X, et al. Expression of CD22 in triple-negative breast cancer: A novel prognostic biomarker and potential target for CAR therapy. Int J Mol Sci. (2023) 24(3). doi: 10.3390/ijms24032152
65. Liu S, Zhang X, Dai H, Cui W, Yin J, Li Z, et al. Which one is better for refractory/relapsed acute B-cell lymphoblastic leukemia: Single-target (CD19) or dual-target (tandem or sequential CD19/CD22) CAR T-cell therapy? Blood Cancer J. (2023) 13(1):60. doi: 10.1038/s41408-023-00819-5
66. Raftery MJ, Franzén AS, Radecke C, Boulifa A, Schönrich G, Stintzing S, et al. Next generation CD44v6-specific CAR-NK cells effective against triple negative breast cancer. Int J Mol Sci. (2023) 24(10). doi: 10.3390/ijms24109038
67. Yang M, Tang X, Zhang Z, Gu L, Wei H, Zhao S, et al. Tandem CAR-T cells targeting CD70 and B7-H3 exhibit potent preclinical activity against multiple solid tumors. Theranostics. (2020) 10(17):7622–34. doi: 10.7150/thno.43991
68. Sauer T, Parikh K, Sharma S, Omer B, Sedloev D, Chen Q, et al. CD70-specific CAR T cells have potent activity against acute myeloid leukemia without HSC toxicity. Blood. (2021) 138(4):318–30. doi: 10.1182/blood.2020008221
69. Wu G, Guo S, Luo Q, Wang X, Deng W, Ouyang G, et al. Preclinical evaluation of CD70-specific CAR T cells targeting acute myeloid leukemia. Front Immunol. (2023) 14:1093750. doi: 10.3389/fimmu.2023.1093750
70. Park YP, Chen P, Lei W, Xu Y, Xu N, Pu JJ, et al. CD70 as a target for chimeric antigen receptor T cells in head and neck squamous cell carcinoma. Oral Oncol. (2018) 78:145–50. doi: 10.1016/j.oraloncology.2018.01.024
71. Deng W, Ge H, Long Y, Yang C, Chang YE, Mu L, et al. CD70-targeting CAR-T cells have potential activity against CD19-negative B-cell Lymphoma. Cancer Commun (Lond). (2021) 41(9):925–9. doi: 10.1002/cac2.12201
72. Jin L, Ge H, Long Y, Yang C, Chang YE, Mu L, et al. CD70, a novel target of CAR T-cell therapy for gliomas. Neuro Oncol. (2018) 20(1):55–65. doi: 10.1093/neuonc/nox116
73. Panowski SH, Srinivasan S, Tan N, Tacheva-Grigorova SK, Smith B, Mak YSL, et al. Preclinical development and evaluation of allogeneic CAR T cells targeting CD70 for the treatment of renal cell carcinoma. Cancer Res. (2022) 82(14):2610–24. doi: 10.1158/0008-5472.CAN-21-2931
74. Cimino A, Halushka M, Illei P, Wu X, Sukumar S, Argani P. Epithelial cell adhesion molecule (EpCAM) is overexpressed in breast cancer metastases. Breast Cancer Res Treat. (2010) 123(3):701–8. doi: 10.1007/s10549-009-0671-z
75. Fu J, Shang Y, Qian Z, Hou J, Yan F, Liu G, et al. Chimeric Antigen receptor-T (CAR-T) cells targeting Epithelial cell adhesion molecule (EpCAM) can inhibit tumor growth in ovarian cancer mouse model. J Vet Med Sci. (2021) 83(2):241–7. doi: 10.1292/jvms.20-0455
76. Zhang Q, Zhang H, Ding J, Liu H, Li H, Li H, et al. Combination therapy with epCAM-CAR-NK-92 cells and regorafenib against human colorectal cancer models. J Immunol Res. (2018) 2018:4263520. doi: 10.1155/2018/4263520
77. Gao G, Liao W, Shu P, Ma Q, He X, Zhang B, et al. Targeting sphingosine 1-phosphate receptor 3 inhibits T-cell exhaustion and regulates recruitment of proinflammatory macrophages to improve antitumor efficacy of CAR-T cells against solid tumor. J Immunother Cancer. (2023) 11(8). doi: 10.1136/jitc-2022-006343
78. Mei Z, Zhang K, Lam AK, Huang J, Qiu F, Qiao B, et al. MUC1 as a target for CAR-T therapy in head and neck squamous cell carinoma. Cancer Med. (2020) 9(2):640–52. doi: 10.1002/cam4.2733
79. Chen Y, E CY, Gong ZW, Liu S, Wang ZX, Yang YS, et al. Chimeric antigen receptor-engineered T-cell therapy for liver cancer. Hepatobiliary Pancreat Dis Int. (2018) 17(4):301–9. doi: 10.1016/j.hbpd.2018.05.005
80. Mao L, Su S, Li J, Yu S, Gong Y, Chen C, et al. Development of engineered CAR T cells targeting tumor-associated glycoforms of MUC1 for the treatment of intrahepatic cholangiocarcinoma. J Immunother. (2023) 46(3):89–95. doi: 10.1097/CJI.0000000000000460
81. Wang A, Lv T, Song Y. Tandem CAR-T cells targeting MUC1 and PSCA combined with anti-PD-1 antibody exhibit potent preclinical activity against non-small cell lung cancer. Cell Immunol. (2023) 391-392:104760. doi: 10.1016/j.cellimm.2023.104760
82. Maeda T, Hiraki M, Jin C, Rajabi H, Tagde A, Alam M, et al. MUC1-C induces PD-L1 and immune evasion in triple-negative breast cancer. Cancer Res. (2018) 78(1):205–15. doi: 10.1158/0008-5472.CAN-17-1636
83. Oshima T, Sato S, Kato J, Ito Y, Watanabe T, Tsuji I, et al. Nectin-2 is a potential target for antibody therapy of breast and ovarian cancers. Mol Cancer. (2013) 12:60. doi: 10.1186/1476-4598-12-60
84. Cifaldi L, Melaiu O, Giovannoni R, Benvenuto M, Focaccetti C, Nardozi D, et al. DNAM-1 chimeric receptor-engineered NK cells: a new frontier for CAR-NK cell-based immunotherapy. Front Immunol. (2023) 14:1197053. doi: 10.3389/fimmu.2023.1197053
85. Focaccetti C, Benvenuto M, Pighi C, Vitelli A, Napolitano F, Cotugno N, et al. DNAM-1-chimeric receptor-engineered NK cells, combined with Nutlin-3a, more effectively fight neuroblastoma cells in vitro: a proof-of-concept study. Front Immunol. (2022) 13:886319. doi: 10.3389/fimmu.2022.886319
86. Wallstabe L, Mades A, Frenz S, Einsele H, Rader C, Hudecek M. CAR T cells targeting α(v)β(3) integrin are effective against advanced cancer in preclinical models. Adv Cell Gene Ther. (2018) 1(2). doi: 10.1002/acg2.11
87. Whilding LM, Parente-Pereira AC, Zabinski T, Davies DM, Petrovic RMG, Kao YV, et al. Targeting of aberrant av 6 integrin expression in solid tumors using chimeric antigen receptor-engineered T cells. Mol Ther: J Am Soc Gene Ther. (2017) 25(1):259–73.
88. Ventin M, Cattaneo G, Maggs L, Jia J, Arya S, Ferrone S, et al. B7-H3-targeted CAR T cell activity is enhanced by radiotherapy in solid cancers. Front Oncol. (2023) 13:1193963. doi: 10.3389/fonc.2023.1193963
89. Harrer DC, Dörrie J, Schaft N. CSPG4 as target for CAR-T-cell therapy of various tumor entities-merits and challenges. Int J Mol Sci. (2019) 20(23). doi: 10.3390/ijms20235942
90. Song DG, Ye Q, Poussin M, Chacon JA, Figini M, Powell DJ Jr. Effective adoptive immunotherapy of triple-negative breast cancer by folate receptor-alpha redirected CAR T cells is influenced by surface antigen expression level. J Hematol Oncol. (2016) 9(1):56. doi: 10.1186/s13045-016-0285-y
91. Ye X, Deng X, Wen J, Li Y, Zhang M, Cai Z, et al. Folate receptor-alpha targeted 7x19 CAR-γδT suppressed triple-negative breast cancer xenograft model in mice. J Oncol. (2022) 2022:2112898. doi: 10.1155/2022/2112898
92. Das S, Valton J, Duchateau P, Poirot L. Stromal depletion by TALEN-edited universal hypoimmunogenic FAP-CAR T cells enables infiltration and anti-tumor cytotoxicity of tumor antigen-targeted CAR-T immunotherapy. Front Immunol. (2023) 14:1172681. doi: 10.3389/fimmu.2023.1172681
93. Li Y, Gao Q, Liu H, Lin S, Chen H, Ding R, et al. The targeting effect of cetuximab combined with PD-L1 blockade against EGFR-expressing tumors in a tailored CD16-CAR T-cell reporter system. Cancer Invest. (2021) 39(4):285–96. doi: 10.1080/07357907.2021.1894570
94. Caratelli S, Arriga R, Sconocchia T, Ottaviani A, Lanzilli G, Pastore D, et al. In vitro elimination of epidermal growth factor receptor-overexpressing cancer cells by CD32A-chimeric receptor T cells in combination with cetuximab or panitumumab. Int J Cancer. (2020) 146(1):236–47. doi: 10.1002/ijc.32663
95. Wei H, Wang Z, Kuang Y, Wu Z, Zhao S, Zhang Zv, et al. Intercellular adhesion molecule-1 as target for CAR-T-cell therapy of triple-negative breast cancer. Front Immunol. (2020) 11:573823. doi: 10.3389/fimmu.2020.573823
96. Li Y, Xiao F, Zhang A, Zhang D, Nie W, Xu T, et al. Oncolytic adenovirus targeting TGF-β enhances anti-tumor responses of mesothelin-targeted chimeric antigen receptor T cell therapy against breast cancer. Cell Immunol. (2020) 348:104041. doi: 10.1016/j.cellimm.2020.104041
97. Li F, Zhao S, Wei C, Hu Y, Xu T, Xin X, et al. Development of Nectin4/FAP-targeted CAR-T cells secreting IL-7, CCL19, and IL-12 for Malignant solid tumors. Front Immunol. (2022) 13:958082. doi: 10.3389/fimmu.2022.958082
98. Han Y, Xie W, Song DG, Powell DJ. Control of triple-negative breast cancer using ex vivo self-enriched, costimulated NKG2D CAR T cells. J Hematol Oncol. (2018) 11(1):92. doi: 10.1186/s13045-018-0635-z
99. Monzo HJ, Kalander K, Hyytiäinen MM, Elbasani E, Wall J, Moyano-Galceran L, et al. Efficacy and safety of glycosphingolipid SSEA-4 targeting CAR-T cells in an ovarian carcinoma model. Mol Cancer Ther. (2023) 22(11):1319–31. doi: 10.1158/1535-7163.MCT-23-0008
100. Pfeifer R, Al Rawashdeh W, Brauner J, Martinez-Osuna M, Lock D, Herbel C, et al. Targeting stage-specific embryonic antigen 4 (SSEA-4) in triple negative breast cancer by CAR T cells results in unexpected on target/off tumor toxicities in mice. Int J Mol Sci. (2023) 24(11). doi: 10.3390/ijms24119184
101. Pellizzari G, Martinez O, Crescioli S, Page R, Di Meo A, Mele S, et al. Immunotherapy using IgE or CAR T cells for cancers expressing the tumor antigen SLC3A2. J Immunother Cancer. (2021) 9(6). doi: 10.1136/jitc-2020-002140
102. Byrd TT, Fousek K, Pignata A, Szot C, Samaha H, Seaman S, et al. TEM8/ANTXR1-specific CAR T cells as a targeted therapy for triple-negative breast cancer. Cancer Res. (2018) 78(2):489–500. doi: 10.1158/0008-5472.CAN-16-1911
103. Liu H, Bai L, Huang L, Ning N, Li L, Li Y, et al. Bispecific antibody targeting TROP2xCD3 suppresses tumor growth of triple negative breast cancer. J Immunother Cancer. (2021) 9(10). doi: 10.1136/jitc-2021-003468
104. Tchou J, Zhao Y, Levine BL, Zhang PJ, Davis MM, Melenhorst JJ, et al. Safety and efficacy of intratumoral injections of chimeric antigen receptor (CAR) T cells in metastatic breast cancer. Cancer Immunol Res. (2017) 5(12):1152–61. doi: 10.1158/2326-6066.CIR-17-0189
105. Sun R, Luo H, Su J, Di S, Zhou M, Shi B, et al. Olaparib suppresses MDSC recruitment via SDF1α/CXCR4 axis to improve the anti-tumor efficacy of CAR-T cells on breast cancer in mice. Mol Ther. (2021) 29(1):60–74. doi: 10.1016/j.ymthe.2020.09.034
106. Di S, Zhou M, Pan Z, Sun R, Chen M, Jiang H, et al. Combined adjuvant of poly I:C improves antitumor effects of CAR-T cells. Front Oncol. (2019) 9:241. doi: 10.3389/fonc.2019.00241
107. Zhou M, Chen M, Shi B, Di S, Sun R, Jiang H, et al. Radiation enhances the efficacy of EGFR-targeted CAR-T cells against triple-negative breast cancer by activating NF-κB/Icam1 signaling. Mol Ther. (2022) 30(11):3379–93. doi: 10.1016/j.ymthe.2022.07.021
108. Jie Y, Liu G, Feng L, Li Y, E M, Wu L, et al. PTK7-targeting CAR T-cells for the treatment of lung cancer and other Malignancies. Front Immunol. (2021) 12:665970. doi: 10.3389/fimmu.2021.665970
109. Xing H, Yang X, Xu Y, Tang K, Tian Z, Chen Z, et al. Anti-tumor effects of vascular endothelial growth factor/vascular endothelial growth factor receptor binding domain-modified chimeric antigen receptor T cells. Cytotherapy. (2021) 23(9):810–9. doi: 10.1016/j.jcyt.2021.05.008
110. Stüber T, Monjezi R, Wallstabe L, Kühnemundt J, Nietzer SL, Dandekar G, et al. Inhibition of TGF-β-receptor signaling augments the antitumor function of ROR1-specific CAR T-cells against triple-negative breast cancer. J Immunother Cancer. (2020) 8(1). doi: 10.1136/jitc-2020-000676
111. Harrasser M, Gohil SH, Lau H, Della Peruta M, Muczynski V, Patel D, et al. Inducible localized delivery of an anti-PD-1 scFv enhances anti-tumor activity of ROR1 CAR-T cells in TNBC. Breast Cancer Res. (2022) 24(1):39. doi: 10.1186/s13058-022-01531-1
112. An W, Kang JS, Oh S, Tu A. MST1R as a potential new target antigen of chimeric antigen receptor T cells to treat solid tumors. Korean J Physiol Pharmacol. (2023) 27(3):241–56. doi: 10.4196/kjpp.2023.27.3.241
113. Hunt BG, Jones A, Lester C, Davis JC, Benight NM, Waltz SE. RON (MST1R) and HGFL (MST1) co-overexpression supports breast tumorigenesis through autocrine and paracrine cellular crosstalk. Cancers (Basel). (2022) 14(10). doi: 10.3390/cancers14102493
114. Ba H, Dai Z, Zhang Z, Zhang P, Yin B, Wang J, et al. Antitumor effect of CAR-T cells targeting transmembrane tumor necrosis factor alpha combined with PD-1 mAb on breast cancers. J Immunother Cancer. (2023) 11(1). doi: 10.1136/jitc-2021-003837
115. Bandara V, Foeng J, Gundsambuu B, Norton TS, Napoli S, McPeake DJ, et al. Pre-clinical validation of a pan-cancer CAR-T cell immunotherapy targeting nfP2X7. Nat Commun. (2023) 14(1):5546. doi: 10.1038/s41467-023-41338-y
116. Yuan Y, Fan J, Liang D, Wang S, Luo X, Zhu Y, et al. Cell surface GRP78-directed CAR-T cells are effective at treating human pancreatic cancer in preclinical models. Transl Oncol. (2024) 39:101803. doi: 10.1016/j.tranon.2023.101803
117. Tseng CC, Zhang P, Lee AS. The COOH-terminal proline-rich region of GRP78 is a key regulator of its cell surface expression and viability of tamoxifen-resistant breast cancer cells. Neoplasia. (2019) 21(8):837–48. doi: 10.1016/j.neo.2019.05.008
118. Zhang C, Wang L, Zhang Q, Shen J, Huang X, Wang M, et al. Screening and characterization of the scFv for chimeric antigen receptor T cells targeting CEA-positive carcinoma. Front Immunol. (2023) 14(12):1182409. doi: 10.3389/fimmu.2023.1182409
119. Zhang X, Sun S, Miao Y, Yuan Y, Zhao W, Li H, et al. Docetaxel enhances the therapeutic efficacy of PSMA-specific CAR-T cells against prostate cancer models by suppressing MDSCs. J Cancer Res Clin Oncol. (2022) 148:3511–20. doi: 10.1007/s00432-022-04248-y
120. Kloss CC, Lee J, Zhang A, Chen F, Melenhorst JJ, Lacey SF, et al. Dominant-negative TGF-β Receptor enhances PSMA-targeted human CAR T cell proliferation and augments prostate cancer eradication. Mol Ther. (2018) 26(7):1855–66. doi: 10.1016/j.ymthe.2018.05.003
121. Heesch A, Ortmanns L, Maurer J, Stickeler E, Sahnoun SEM, Mottaghy FM, et al. The potential of PSMA as a vascular target in TNBC. Cells. (2023) 12(4). doi: 10.3390/cells12040551
122. Reinhard K, Rengstl B, Oehm P, Michel K, Billmeier A, Hayduk N, et al. An RNA vaccine drives expansion and efficacy of claudin-CAR-T cells against solid tumors. Science. (2020) 367(6476):446–53. doi: 10.1126/science.aay5967
123. Mackensen A, Haanen J, Koenecke C, Alsdorf W, Wagner-Drouet E, Borchmann P, et al. CLDN6-specific CAR-T cells plus amplifying RNA vaccine in relapsed or refractory solid tumors: the phase 1 BNT211-01 trial. Nat Med. (2023) 29(11):2844–53. doi: 10.1038/s41591-023-02612-0
124. Shi H, Li A, Dai Z, Xue J, Zhao Q, Tian J, et al. IL-15 armoring enhances the antitumor efficacy of claudin 18.2-targeting CAR-T cells in syngeneic mouse tumor models. Front Immunol. (2023) 14:1165404. doi: 10.3389/fimmu.2023.1165404
125. Luo H, Su J, Sun R, Sun Y, Wang Y, Dong Y, et al. Coexpression of IL7 and CCL21 increases efficacy of CAR-T cells in solid tumors without requiring preconditioned lymphodepletion. Clin Cancer Res. (2020) 26(20):5494–505. doi: 10.1158/1078-0432.CCR-20-0777
126. Liu Y, Sun Y, Wang P, Li S, Dong Y, Zhou M, et al. FAP-targeted CAR-T suppresses MDSCs recruitment to improve the antitumor efficacy of claudin18.2-targeted CAR-T against pancreatic cancer. J Transl Med. (2023) 21(1):255. doi: 10.1186/s12967-023-04080-z
127. Hu Z. Tissue factor as a new target for CAR-NK cell immunotherapy of triple-negative breast cancer. Sci Rep. (2020) 10(1):2815. doi: 10.1038/s41598-020-59736-3
128. Liu L, Qu Y, Cheng L, Yoon CW, He P, Monther A, et al. Engineering chimeric antigen receptor T cells for solid tumour therapy. Clin Transl Med. (2022) 12(12):e1141. doi: 10.1002/ctm2.1141
129. Deepak KGK, Vempati R, Nagaraju GP, Dasari VR, S N, Rao DN, et al. Tumor microenvironment: Challenges and opportunities in targeting metastasis of triple negative breast cancer. Pharmacol Res. (2020) 153:104683. doi: 10.1016/j.phrs.2020.104683
130. Jarosz-Biej M, Smolarczyk R, Cichoń T, Kułach N. Tumor microenvironment as A "Game changer" in cancer radiotherapy. Int J Mol Sci. (2019) 20(13). doi: 10.3390/ijms20133212
131. Hu D, Li Z, Zheng B, Lin X, Pan Y, Gong P, et al. Cancer-associated fibroblasts in breast cancer: Challenges and opportunities. Cancer Commun (Lond). (2022) 42(5):401–34. doi: 10.1002/cac2.12291
132. Costa A, Kieffer Y, Scholer-Dahirel A, Pelon F, Bourachot B, Cardon M, et al. Fibroblast heterogeneity and immunosuppressive environment in human breast cancer. Cancer Cell. (2018) 33(3):463–479.e10. doi: 10.1016/j.ccell.2018.01.011
133. Wen S, Hou Y, Fu L, Xi L, Yang D, Zhao M, et al. Cancer-associated fibroblast (CAF)-derived IL32 promotes breast cancer cell invasion and metastasis via integrin β3-p38 MAPK signalling. Cancer Lett. (2019) 442:320–32. doi: 10.1016/j.canlet.2018.10.015
134. Rivas EI, Linares J, Zwick M, Gómez-Llonin A, Guiu M, Labernadie A, et al. Targeted immunotherapy against distinct cancer-associated fibroblasts overcomes treatment resistance in refractory HER2+ breast tumors. Nat Commun. (2022) 13(1):5310. doi: 10.1038/s41467-022-32782-3
135. Wu Y, Yi M, Niu M, Mei Q, Wu K. Myeloid-derived suppressor cells: an emerging target for anticancer immunotherapy. Mol Cancer. (2022) 21(1):184. doi: 10.1186/s12943-022-01657-y
136. Li W, Tanikawa T, Kryczek I, Xia H, Li G, Wu K, et al. Aerobic glycolysis controls myeloid-derived suppressor cells and tumor immunity via a specific CEBPB isoform in triple-negative breast cancer. Cell Metab. (2018) 28(1):87–103.e6. doi: 10.1016/j.cmet.2018.04.022
137. Condamine T, Kumar V, Ramachandran IR, Youn JI, Celis E, Finnberg N, et al. ER stress regulates myeloid-derived suppressor cell fate through TRAIL-R-mediated apoptosis. J Clin Invest. (2014) 124(6):2626–39. doi: 10.1172/JCI74056
138. Nalawade SA, Shafer P, Bajgain P, McKenna MK, Ali A, Kelly L, et al. Selectively targeting myeloid-derived suppressor cells through TRAIL receptor 2 to enhance the efficacy of CAR T cell therapy for treatment of breast cancer. J Immunother Cancer. (2021) 9(11). doi: 10.1136/jitc-2021-003237
139. Fernando V, Zheng X, Sharma V, Furuta S. Reprogramming of breast tumor-associated macrophages with modulation of arginine metabolism. bioRxiv. (2023). doi: 10.1101/2023.08.22.554238
140. Munir MT, Kay MK, Kang MH, Rahman MM, Al-Harrasi A, Choudhury M, et al. Tumor-associated macrophages as multifaceted regulators of breast tumor growth. Int J Mol Sci. (2021) 22(12). doi: 10.3390/ijms22126526
141. Mehta AK, Kadel S, Townsend MG, Oliwa M, Guerriero JL. Macrophage biology and mechanisms of immune suppression in breast cancer. Front Immunol. (2021) 12:643771. doi: 10.3389/fimmu.2021.643771
142. Liu Q, Hodge J, Wang J, Wang Y, Wang L, Singh U, et al. Emodin reduces Breast Cancer Lung Metastasis by suppressing Macrophage-induced Breast Cancer Cell Epithelial-mesenchymal transition and Cancer Stem Cell formation. Theranostics. (2020) 10(18):8365–81. doi: 10.7150/thno.45395
143. Meng Z, Zhang R, Wu X, Zhang M, Jin T. PD−L1 mediates triple−negative breast cancer evolution via the regulation of TAM/M2 polarization. Int J Oncol. (2022) 61(6). doi: 10.3892/ijo.2022.5440
144. Mukherjee S, Chakraborty S, Basak U, Pati S, Dutta A, Dutta S. Breast cancer stem cells generate immune-suppressive T regulatory cells by secreting TGFβ to evade immune-elimination. Discovery Oncol. (2023) 14(1):220. doi: 10.1007/s12672-023-00787-z
145. Núñez NG, Tosello Boari J, Ramos RN, Richer W, Cagnard N, Anderfuhren CD, et al. Tumor invasion in draining lymph nodes is associated with Treg accumulation in breast cancer patients. Nat Commun. (2020) 11(1):3272. doi: 10.1038/s41467-020-17046-2
146. Qiu J, Xu L, Zeng X, Wu H, Liang F, Lv Qv, et al. CCL5 mediates breast cancer metastasis and prognosis through CCR5/Treg cells. Front Oncol. (2022) 12:972383. doi: 10.3389/fonc.2022.972383
147. Bai F, Zhang P, Fu Y, Chen H, Zhang M, Huang Q, et al. Targeting ANXA1 abrogates Treg-mediated immune suppression in triple-negative breast cancer. J Immunother Cancer. (2020) 8(1). doi: 10.1136/jitc-2019-000169
148. Frey N, Porter D. Cytokine release syndrome with chimeric antigen receptor T cell therapy. Biol Blood Marrow Transplant. (2019) 25(4):e123–7. doi: 10.1016/j.bbmt.2018.12.756
149. Xu N, Palmer DC, Robeson AC, Shou P, Bommiasamy H, Laurie SJ, et al. STING agonist promotes CAR T cell trafficking and persistence in breast cancer. J Exp Med. (2021) 218(2). doi: 10.1084/jem.20200844
150. Miao L, Zhang Z, Ren Z, Li Y. Reactions related to CAR-T cell therapy. Front Immunol. (2021) 12:663201. doi: 10.3389/fimmu.2021.663201
151. Morgan RA, Yang JC, Kitano M, Dudley ME, Laurencot CM, Rosenberg SA. Case report of a serious adverse event following the administration of T cells transduced with a chimeric antigen receptor recognizing ERBB2. Mol Ther. (2010) 18(4):843–51. doi: 10.1038/mt.2010.24
152. Gumber D, Wang LD. Improving CAR-T immunotherapy: Overcoming the challenges of T cell exhaustion. EBioMedicine. (2022) 77:103941. doi: 10.1016/j.ebiom.2022.103941
153. Ebbinghaus M, Wittich K, Bancher B, Lebedeva V, Appelshoffer A, Femel J, et al. Endogenous signaling molecule activating (ESMA) CARs: A novel CAR design showing a favorable risk to potency ratio for the treatment of triple negative breast cancer. Int J Mol Sci. (2024) 25(1). doi: 10.3390/ijms25010615
154. Abdin SM, Paasch D, Kloos A, Oliveira MC, Jang MS, Ackermann M, et al. Scalable generation of functional human iPSC-derived CAR-macrophages that efficiently eradicate CD19-positive leukemia. J Immunother Cancer. (2023) 11(12). doi: 10.1136/jitc-2023-007705
155. Klichinsky M, Ruella M, Shestova O, Lu XM, Best A, Zeeman M, et al. Human chimeric antigen receptor macrophages for cancer immunotherapy. Nat Biotechnol. (2020) 38(8):947–53. doi: 10.1038/s41587-020-0462-y
156. Zhang J, Webster S, Duffin B, Bernstein MN, Steill J, Swanson S, et al. Generation of anti-GD2 CAR macrophages from human pluripotent stem cells for cancer immunotherapies. Stem Cell Rep. (2023) 18(2):585–96. doi: 10.1016/j.stemcr.2022.12.012
157. Chen Y, Sun C, Landoni E, Metelitsa L, Dotti G, Savoldo B. Eradication of neuroblastoma by T cells redirected with an optimized GD2-specific chimeric antigen receptor and interleukin-15. Clin Cancer Res. (2019) 25(9):2915–24. doi: 10.1158/1078-0432.CCR-18-1811
158. Lin YZ, Lee CC, Cho DY, Wang YL, Chen CY, Weng CY, et al. Suppression of breast cancer cells resistant to a pure anti-estrogen with CAR-transduced natural killer cells. Am J Cancer Res. (2021) 11(9):4455–69.
159. Fabian KP, Padget MR, Donahue RN, Solocinski K, Robbins Y, Allen CT, et al. PD-L1 targeting high-affinity NK (t-haNK) cells induce direct antitumor effects and target suppressive MDSC populations. J Immunother Cancer. (2020) 8(1). doi: 10.1136/jitc-2019-000450
160. Chen X, Han J, Chu J, Zhang L, Zhang J, Chen C, et al. A combinational therapy of EGFR-CAR NK cells and oncolytic herpes simplex virus 1 for breast cancer brain metastases. Oncotarget. (2016) 7(19):27764–77. doi: 10.18632/oncotarget.8526
161. Portillo AL, Hogg R, Poznanski SM, Rojas EA, Cashell NJ, Hammill JA, et al. Expanded human NK cells armed with CAR uncouple potent anti-tumor activity from off-tumor toxicity against solid tumors. iScience. (2021) 24(6):102619. doi: 10.1016/j.isci.2021.102619
162. Xia W, Chen J, Hou W, Chen J, Xiong Y, Li H, et al. Engineering a HER2-CAR-NK cell secreting soluble programmed cell death protein with superior antitumor efficacy. Int J Mol Sci. (2023) 24(7). doi: 10.3390/ijms24076843
163. Schepisi G, Gianni C, Palleschi M, Bleve S, Casadei C, Lolli C, et al. The new frontier of immunotherapy: chimeric antigen receptor T (CAR-T) cell and macrophage (CAR-M) therapy against breast cancer. Cancers (Basel). (2023) 15(5). doi: 10.3390/cancers15051597
164. Duan Z, Li Z, Wang Z, Chen C, Luo Y. Chimeric antigen receptor macrophages activated through TLR4 or IFN-γ receptors suppress breast cancer growth by targeting VEGFR2. Cancer Immunol Immunother. (2023) 72(10):3243–57. doi: 10.1007/s00262-023-03490-8
165. Liang Y, Xu Q, Gao Q. Advancing CAR-based immunotherapies in solid tumors: CAR- macrophages and neutrophils. Front Immunol. (2023) 14:1291619. doi: 10.3389/fimmu.2023.1291619
166. Zhang P, Zhang G, Wan X. Challenges and new technologies in adoptive cell therapy. J Hematol Oncol. (2023) 16(1):97. doi: 10.1186/s13045-023-01492-8
167. Biederstädt A, Rezvani K. Engineering the next generation of CAR-NK immunotherapies. Int J Hematol. (2021) 114(5):554–71. doi: 10.1007/s12185-021-03209-4
168. Valeri A, García-Ortiz A, Castellano E, Córdoba L, Maroto-Martín E, Encinas J, et al. Overcoming tumor resistance mechanisms in CAR-NK cell therapy. Front Immunol. (2022) 13:953849. doi: 10.3389/fimmu.2022.953849
169. Margiana R, Grisendi G, Prapa M, Bestagno M, Spano C, Rossignoli F, et al. Clinical application of mesenchymal stem cell in regenerative medicine: a narrative review. Stem Cell Res Ther. (2022) 13(1):366. doi: 10.1186/s13287-022-03054-0
170. Golinelli G, Grisendi G, Prapa M, Bestagno M, Spano C, Rossignoli F, et al. Targeting GD2-positive glioblastoma by chimeric antigen receptor empowered mesenchymal progenitors. Cancer Gene Ther. (2020) 27(7-8):558–70. doi: 10.1038/s41417-018-0062-x
171. Golinelli G, Grisendi G, Dall'Ora M, Casari G, Spano C, Talami R, et al. Anti-GD2 CAR MSCs against metastatic Ewing's sarcoma. Transl Oncol. (2022) 15(1):101240. doi: 10.1016/j.tranon.2021.101240
172. Chen W-H, Huang Q-Y, Wang Z-Y, Zhuang X-X, Lin S, Shi Q-Y. Therapeutic potential of exosomes/miRNAs in polycystic ovary syndrome induced by the alteration of circadian rhythms. Front In Endocrinol. (2022) 13:918805. doi: 10.3389/fendo.2022.918805
173. Li J, Zhang Y, Dong P-Y, Yang G-M, Gurunathan S. A comprehensive review on the composition, biogenesis, purification, and multifunctional role of exosome as delivery vehicles for cancer therapy. Biomedicine Pharmacotherapy = Biomedecine Pharmacotherapie. (2023) 165:115087. doi: 10.1016/j.biopha.2023.115087
174. Liu K, Gao X, Kang B, Liu Y, Wang D, Wang Y. The role of tumor stem cell exosomes in cancer invasion and metastasis. Front In Oncol. (2022) 12:836548. doi: 10.3389/fonc.2022.836548
175. Zhang M, Hu S, Liu L, Dang P, Liu Y, Sun Z, et al. Engineered exosomes from different sources for cancer-targeted therapy. Signal Transduction Targeted Ther. (2023) 8(1):124. doi: 10.1038/s41392-023-01382-y
176. Yang P, Cao X, Cai H, Feng P, Chen X, Zhu Y, et al. The exosomes derived from CAR-T cell efficiently target mesothelin and reduce triple-negative breast cancer growth. Cell Immunol. (2021) 360:104262. doi: 10.1016/j.cellimm.2020.104262
177. Tao B, Du R, Zhang X, Jia B, Gao Y, Zhao Y, et al. Engineering CAR-NK cell derived exosome disguised nano-bombs for enhanced HER2 positive breast cancer brain metastasis therapy. J Control Release. (2023) 363:692–706. doi: 10.1016/j.jconrel.2023.10.007
178. Tang XJ, Sun XY, Huang KM, Zhang L, Yang ZS, Zou DD, et al. Therapeutic potential of CAR-T cell-derived exosomes: a cell-free modality for targeted cancer therapy. Oncotarget. (2015) 6(42):44179–90. doi: 10.18632/oncotarget.6175
179. Calvo V, Izquierdo M. T lymphocyte and CAR-T cell-derived extracellular vesicles and their applications in cancer therapy. Cells. (2022) 11(5). doi: 10.3390/cells11050790
180. Haque S, Vaiselbuh SR. CD19 chimeric antigen receptor-exosome targets CD19 positive B-lineage acute lymphocytic leukemia and induces cytotoxicity. Cancers (Basel). (2021) 13(6). doi: 10.3390/cancers13061401
181. Nutt WS, Srivastava S. Special delivery! CAR-T cells transport RN7SL1 to the tumor microenvironment. Trends Mol Med. (2021) 27(11):1019–21. doi: 10.1016/j.molmed.2021.09.002
182. Johnson LR, Lee DY, Eacret JS, Ye D, June CH, Minn AJ. The immunostimulatory RNA RN7SL1 enables CAR-T cells to enhance autonomous and endogenous immune function. Cell. (2021) 184(19):4981–4995.e14. doi: 10.1016/j.cell.2021.08.004
183. Wang Z, Zhou G, Risu N, Fu J, Zou Y, Tang J, et al. Lenalidomide enhances CAR-T cell activity against solid tumor cells. Cell Transplant. (2020) 29:963689720920825. doi: 10.1177/0963689720920825
184. Yan L, Li J, Zhang C. The role of MSCs and CAR-MSCs in cellular immunotherapy. Cell Commun Signal. (2023) 21(1):187. doi: 10.1186/s12964-023-01191-4
Keywords: breast cancer, CAR-T, CAR-NK, CAR-M, exosome, TME, TNBC
Citation: Niu Z, Wu J, Zhao Q, Zhang J, Zhang P and Yang Y (2024) CAR-based immunotherapy for breast cancer: peculiarities, ongoing investigations, and future strategies. Front. Immunol. 15:1385571. doi: 10.3389/fimmu.2024.1385571
Received: 13 February 2024; Accepted: 27 March 2024;
Published: 12 April 2024.
Edited by:
Hongru Zhang, Nankai University, ChinaReviewed by:
Guoqiang Liu, University of Notre Dame, United StatesFanying Tang, Novartis, United States
Guiling Wang, China Medical University, China
Quanli Yang, Jinan University, China
Copyright © 2024 Niu, Wu, Zhao, Zhang, Zhang and Yang. This is an open-access article distributed under the terms of the Creative Commons Attribution License (CC BY). The use, distribution or reproduction in other forums is permitted, provided the original author(s) and the copyright owner(s) are credited and that the original publication in this journal is cited, in accordance with accepted academic practice. No use, distribution or reproduction is permitted which does not comply with these terms.
*Correspondence: Yiming Yang, eW1feWFuZ0BqbHUuZWR1LmNu