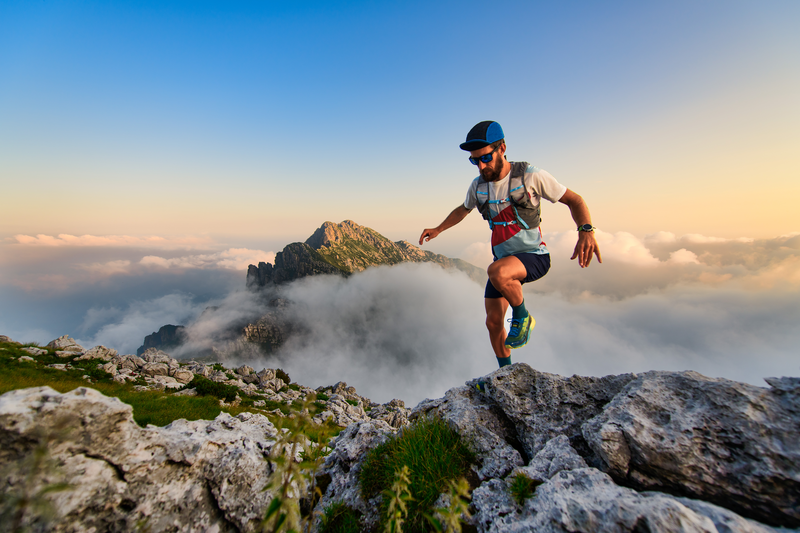
94% of researchers rate our articles as excellent or good
Learn more about the work of our research integrity team to safeguard the quality of each article we publish.
Find out more
ORIGINAL RESEARCH article
Front. Immunol. , 13 August 2024
Sec. Parasite Immunology
Volume 15 - 2024 | https://doi.org/10.3389/fimmu.2024.1373498
This article is part of the Research Topic Leishmaniasis and Immunity: Challenges, Advances and Future Perspective View all 8 articles
Introduction: Visceral leishmaniasis (VL) is an important tropical and neglected disease and represents a serious global health problem. The initial interaction between the phagocytes and the parasite is crucial to determine the pathogen’s capacity to initiate infection and it shapes the subsequent immune response that will develop. While type-1 T-cells induce IL-6, IL-1β, TNF-α, and IL-12 production by monocytes/macrophages to fight the infection, type-2 T-cells are associated with a regulatory phenotype (IL-10 and TGF-β) and successful infection establishment. Recently, our group demonstrated the role of an important Th1/Th17 T-cell population, the mucosal-associated invariant T (MAIT) cells, in VL. MAIT cells can respond to L. infantum by producing TNF-α and IFN-γ upon MR1-dependent activation.
Objective and methods: Here, we describe the impact of the MR1-blockage on L. infantum internalization on the functional profile of circulating neutrophils and monocytes as well as the impact of the MR1-blockage on the soluble mediator signatures of in vitro whole blood cultures.
Results: Overall, our data showed that VL patients presents higher percentage of activated neutrophils than asymptomatic and non-infected controls. In addition, MR1 blockade led to lower TNF-α and TGF-β production by non-activated neutrophils from asymptomatic individuals. Moreover, TNF-α and IL-10 production by monocytes was higher in VL patients. In the analysis of soluble mediators produced in vitro, MR1-blockade induced a decrease of IFN-γ and an increase of IL-10, IL-27 and IL-33 in the cell cultures of AS group, a cytokine pattern associated with type 2 deleterious response.
Discussion and conclusion: These data corroborate the hypothesis that MR1-restricted responses are associated to a protective role during Leishmania infection.
Visceral leishmaniasis (VL) is one of the seven most important tropical and neglected diseases that presents a wide spectrum of clinical manifestations and can be fatal in more than 95% of cases if not treated (1). Patients with classical VL may present anemia, leukopenia, hepatosplenomegaly, and intermittent fever, while severe VL is associated with bleeding, splenomegaly, edema, weakness, jaundice, bacterial pneumonia, and sepsis (2, 3). Indeed, severe VL is related to exacerbation of the systemic inflammatory cytokine response, especially IL-6, IL-1β, TNF-α and IFN-γ. This enhanced cytokine response is associated with macrophage activation syndrome (MAS) along with increased production of localized regulatory cytokines, notably IL-10, IL-27 and TGF-β (4–7).
Neutrophils take a crucial role as the initial cellular responders to the infections caused by Leishmania donovani (8) and Leishmania infantum (9), shaping the course of the infection by regulating parasite control. These cells play diverse functions in this regard, including phagocytosis, secretion of enzymes and antimicrobial proteins, oxidative bursts, and the formation of Neutrophil Extracellular Trap (NETs) (10, 11). Furthermore, neutrophils contribute actively to the modulation of adaptive immune responses by inducing the secretion of several cytokines such as TNF-α, IL-12, and IFN-γ (12–15). Leishmania primarily targets monocytes/macrophages as its host cells, and the initial interaction between the parasite and the target cell is crucial to determine the pathogen’s capacity to initiate the infection and shapes the subsequent immune response (16). In response to cytokines produced by Type 1 T-cells (Th1), monocytes/macrophages exhibit a pro-inflammatory phenotype, which is characterized by increased production of IL-6, IL-1β, TNF-α, and IL-12, as well as by important antimicrobial agents such as NO (Nitric Oxide) and ROS (Reactive Oxygen Species). Conversely, when stimulated by Type 2 T-cells (Th2) cytokines, monocytes/macrophages undertake a regulatory phenotype marked by elevated levels of IL-10 and TGF-β, which are deleterious to the host and are associated with successful establishment of the parasites in the host (17–21).
In addition to Th1 and Th2 immune responses, the importance of cytokines produced by Th17/22 axis in controlling L. infantum and L. donovani infections has been demonstrated (22). For instance, Th17 and γδ T-cells were found to be important sources of IL-17A in the liver and spleen of mice infected with L. donovani (23). An important subset of unconventional innate-like T-cells are the mucosal-associated invariant T (MAIT) cells. These cells have unique circulation patterns and a significant impact in the host’s immune response against the infection, characterized as cytotoxic (24–26) and effector memory (27) profile. MAIT cells possess an invariant TCR α-chain consisting of the semi-variable region (TRAV) 1-2 and joining region (TRAJ) 33 that recognize antigens limited to the non-classical antigen-presenting molecule, the MHC class I-related protein 1 (MR1), which is expressed by diverse cell types as antigen-presenting cells (APCs) (28, 29). Although, MAIT cells can be found in various tissues, they are found at high frequencies in organs that play crucial role in the pathology of VL, such as liver, intestine, lymphoid organs, and spleen (30–33). Regarding this context, recently our group have demonstrated that, upon MR1-dependent activation, MAIT cells can respond to L. infantum by producing TNF-α and IFN-γ (34). However, it is not known how the blockade of these MR1-interactive pathways may impact the response of phagocytes during VL.
Here, we describe the impact of the MR1 blockade on L. infantum internalization and in the functional profile of circulating neutrophils and monocytes as well as the impact of the MR1 blockade in the soluble mediator signatures of in vitro whole blood cultures.
Clinical samples from children (age 2 – 9) with classical visceral leishmaniasis (LV) were obtained before (LV-BT) and after (LV-AT) treatment at the João Paulo II Children’s Hospital, Belo Horizonte, Minas Gerais, Brazil. All participants had diagnosis confirmed by positive serology using the IT Leish® assay (Bio-Rad Laboratories, Hercules, CA, USA). Whole blood samples were collected before (VL-BT group) and after (VL-AT group) 20-40 days of treatment with meglumine antimoniate (used in 64% of the cases) or liposomal amphotericin B (used in 29% of the cases). Asymptomatic patients presenting positive tests for ELISA (rk39) or whole blood real-time PCR were also included in the study. In addition, endemic groups, recruited from metropolitan region of Belo Horizonte, were included, comprising the asymptomatic (AS group, n=18) and non-infected healthy children (NI group, n=15) groups. Written informed consent was obtained from parents and/or legal guardians of the participants before inclusion in the study. The research adhered to the guidelines outlined in the Brazilian resolution 466/2012 established by the National Commission on Research Ethics (CONEP). This study protocol was submitted and approved by the Ethics Committee of the René Rachou Institute, IRR/FIOCRUZ-MINAS (protocol #782.042) and the João Paulo II Children’s Hospital (protocol #860.893).
Promastigotes of L. infantum (MHOM/BR/1974/PP75) were obtained from in vitro axenic culture in biphasic blood-agar medium (Novy-McNeal-Nicolle plus Liver Infusion Triptose supplemented with 10% of fetal bovine serum (FBS) over rabbit blood agar). Cultures were maintained at 26°C in a Biochemical Oxygen Demand incubator. A metacyclic-enriched promastigote suspension was collected at the stationary growth phase (7-days of culture) and submitted to differential low-speed centrifugation at 100 x g for 10 minutes, at 18°C, to spin down parasites clumps and debris. After 20 minutes incubation at 26°C, single cell parasites suspension was recovered in the supernatant and transferred to a 50 mL polypropylene tube. The parasites were washed twice with phosphate-buffered saline (PBS) supplemented with 10% FBS by high-speed centrifugation at 900 x g for 10 minutes at 18°C. The pellet was resuspended in 1 mL of PBS with 10% FBS, the parasites counted in a Neubauer chamber on optical microscopy and adjusted to obtain a promastigote suspension at 1.0 x 108 parasites/mL. An aliquot of parasite suspension (2 mL) was incubated with 100 μL of Alexa Fluor 647 solution (Invitrogen, Thermo Fisher Scientific – MA, USA) at 3.2 g/mL final concentration, for 30 minutes at 37°C in the dark. Following incubation, fluorescent-labeled parasites were submitted to three washing steps with PBS supplemented with 10% FBS at 900 x g for 10 minutes at 18°C and the promastigote suspension adjusted to a final concentration of 1.0 x 108 fluorescent parasites/mL in a final volume of 1 mL. The labeling quality control of fluorescent-labeled parasites was carried out on a BD LRS Fortessa Flow Cytometer (BD Biosciences, San Jose, CA, USA), considering the mean fluorescence intensity (MFI) target between 103 and 104 monitored on single parameter histograms.
Heparinized whole blood samples were centrifuged at 660 x g for 10 minutes at 18°C for plasma removal. The cells were resuspended in RPMI 1640 medium (GIBCO – Grand Island, NY, USA), gently homogenized and centrifugated at 660 x g for 10 minutes at 18°C. After supernatant removal, the cellular concentration was adjusted to 1.0 x 107 leukocytes/mL. To assess the parasite internalization and intracytoplasmic cytokine profile, three different in vitro whole blood cultures were performed, referred as: Control Culture (CC), L. infantum (1:2 parasite:cells ratio) and L. infantum (1:2 parasite:cells ratio) + α-MR1 antibody (10μg/mL; clone 26.5). Briefly, after 1 hour of incubation, at 37°C in a 5% CO2 humidified atmosphere, 10μg/mL of Brefeldin A (BFA - Sigma, MO, USA) was added to each whole blood culture to evaluate the intracytoplasmic cytokine profile. After 4 hours of incubation, 20mM of EDTA (Sigma, MO, USA) was added in the cultures, following assessment of intracytoplasmic cytokine profile by flow cytometry. Cultures without BFA were performed for assessing immune soluble factors secreted in the supernatant.
For the monocyte and neutrophil phagocyte surface marker analysis, 200μL of the cell suspension of each culture condition, was incubated with anti-HLA-DR-FITC (clone: Tu39), anti-CD16-AF700 (clone: 3G8) and anti-CD14-V450 (clone: MφP9) monoclonal antibodies (BD Biosciences, San Jose, CA, USA). After 20 minutes incubation in the dark at room temperature, erythrocytes were lysed (FACS Lysing Solution – BD, San Jose, CA, EUA) and leucocytes were permeabilizated with 200μL of PBS-P (PBS solution with 0.5% bovine serum albumin, 0,5% saponin and 0.1% sodium azide). After permeabilization, 200μL of PBS-W (PBS solution with 0.5% bovine serum albumin and 0.1% sodium azide) was added to the cultures and 40μL of the cell suspensions were transferred to 96 wells U-bottom plates (Sarstedt, Inc – Newton) containing the phycoerythrin (PE)-labeled monoclonal antibodies: anti-IFN-γ (clone: 4S.B3), anti-TNF-α (clone: MAb11), anti-IL-10 (clone: JES3-9D7), anti-IL-12 (clone: C11.5) and anti-TGF-β (clone: TB21), all purchased from BD Biosciences (San Jose, CA, USA). The plates were incubated for 30 minutes in the dark, at room temperature. After incubation, the cells were washed with PBS-P followed by PBS-W. After centrifugation at 600 x g, for 7 minutes at 18°C, cells were resuspended with 200μL of PBS and the cell suspensions were maintained at 4°C for 15 minutes. A total of 3,000 events within the CD14+ monocyte gate was acquired using BD LRS Fortessa Flow Cytometer (BD Biosciences, San Jose, CA, USA). The phenotypic profile, internalization rate of L. infantum, and intracellular cytokine production by phagocytes were analyzed using FlowJo (version 10.1, Tree Star Inc. Ashland, OR, USA), at the Flow Cytometry Platform facility at FIOCRUZ-MINAS.
Aiming at assessing the intracellular nitric oxide (NO) production in peripheral blood phagocytes, a flow cytometric assay was performed according to Schachnik and colleagues (35). Briefly, the assay is based on 4,5-diaminofluorescein diacetate probe (DAF-2DA) that after being deacetylated by intracellular esterases to DAF-2, it reacts with NO to yield fluorescent DAF-2T (triazolofluorescein) compound, which shows a green fluorescence detected by the flow cytometer. Briefly, the cultures (control, L. infantum and L. infantum + α-MR1) were conducted using leukocytes aliquots (1.0 x 107 cells/mL), which were incubated for 1 hour with the DAF-2DA, at 37°C in a 5%CO2 humidified atmosphere. Lipopolysaccharide from Escherichia coli (LPS, 10mg/mL; Sigma, MO, USA) was employed as iNOS inducer (positive control culture). For specificity assays, Aminoguanidine (AG, 10 mM; Sigma, MO, USA), an iNOS inhibitor, was used. Following, the samples were incubated for 20 minutes in the dark at room temperature in the presence of anti-HLA-DR-PE (clone: G46-6), anti-CD16-AF700 (clone: 3G8) and anti-CD14-V450 (clone: MφP9) monoclonal antibodies (BD Biosciences, San Jose, CA, USA). Following incubation, the samples were washed with PBS at 600 x g, for 7 minutes at 18°C and a total of 3,000 events/CD14+ monocyte gate, were acquired using BD LRS Fortessa Flow Cytometer (BD Biosciences, San Jose, CA, USA). The data were analyzed by FlowJo (version 10.1, Tree Star Inc. Ashland, OR, USA), at the Flow Cytometry Platform facility at FIOCRUZ-MINAS.
The supernatants from Control (CC), L. infantum and L. infantum + α-MR1 cultures were collected, and the levels of soluble mediators were measured by Luminex immunoassay. The quantification of chemokines, pro-inflammatory cytokines, Th17/22 axis cytokines, regulatory cytokines, and growth factors was performed using the “Milliplex MAP Kit – Th17” system (Merck Millipore, Burlington, MA, EUA) and the “The Bio-Plex Pro Human Cytokine 27-Plex” system (BioRad Laboratories, Hercules, CA, USA), following the manufacturer’s instruction. The results were expressed in pg/mL according to standard curves inserted on each experimental batch. The data was analyzed by Bio-plex 200 System (BioRad Laboratories, CA, USA) at Flow Cytometry Platform facility at FIOCRUZ-MINAS.
Data analysis was performed using GraphPad Prism v.9.1.1 software (San Diego, CA, USA). Considering the non-parametric distribution of the data, comparative analyzes were performed using Mann-Whitney test or Kruskal-Walli’s test, followed by Dunn’s post-test. Significant differences at p<0.05 are represented by the letters “a” “b” “c” and “d” as compared to NI, AS, VL-BT and VL-AT groups, respectively. Heatmap constructs were assembled using Microsoft Excel (Albuquerque, NM, USA) to illustrate the functional profiles of neutrophils and monocytes in NI, AS, VL-BT and VL-AT groups. Lollipop charts show the relationship between a numeric and a categoric variable. Here, lollipop charts were plotted to represent the proportion (%) of samples with soluble mediator index (L.i/CC and L.i + α-MR1/CC) above the global median cut-off in NI, AS, VL-BT and VL-AT groups. Correlation analysis was employed to build integrative networks of soluble mediators. Pearson and Spearman correlation tests were used to obtain the significant “r” scores at p<0.05 and the Cytoscape software platform (https://cytoscape.org) was employed to construct the integrative networks. The integrative networks were organized using cluster layouts comprising five categories of parameters including: chemokines, pro-inflammatory, regulatory, and Th17/Th22 cytokines along with growth factors. The node sizes are proportional to the number of correlations between biomarkers. Descriptive analysis was represented by orbital charts summarizing the number of correlations for each study group. Changes in biomarker connectivity were estimated as L.i. + α-MR1/L.i. ratio to identify soluble mediators with increased (>2x) or decreased (<0.5x) connectivity.
To evaluate the impact of MR1 blockade on the phagocytic capacity and oxidative potential of circulating neutrophils and monocytes, whole blood samples from non-infected (NI) and asymptomatic (AS) individuals, as well as patients with visceral leishmaniasis before (LV-BT) and after (LV-AT) treatment were stimulated with live L. infantum promastigotes in the absence/presence of α-MR1 mAb. The rates of L. infantum internalization and nitric oxide production were assessed by flow cytometry and the results are presented in Figure 1. Data analysis demonstrated that regardless of the lower frequency of total neutrophils (CD16+SSChigh) in comparison to NI, VL-BT presented higher percentage of activated neutrophils (HLA-DR+) than NI and AS. Likewise, VL-AT also exhibited more activated neutrophils as compared to NI and AS (Figure 1A). No differences were observed for the frequency or activation of total monocytes (CD14+SSCint) amongst groups (Figure 1B). Additionally, the ability of neutrophils to internalize L. infantum in patients before and after treatment (VL-BT and VL-AT) was impaired as compared to NI individuals, regardless of MR1 blockade. Conversely, AS individuals exhibited higher Leishmania internalization by non-activated neutrophils in L. infantum culture in comparison to all other groups (Figure 1C – top panels). Neutrophils from VL-BT and VL-AT patients, and non-activated neutrophils from AS individuals produced higher levels of NO in L. infantum-stimulated culture as compared to NI donors. Interestingly, the MR1 blockade inhibited the NO production by neutrophils in those three groups, reaching similar levels as observed for NI, both in non-activated and activated cells (Figure 1C – bottom panels).
Figure 1 Impact of MR1 blockade on L. infantum internalization and nitric oxide production by circulating neutrophils and monocytes. (A) The frequencies (%) of circulating neutrophil and (B) total monocytes were quantified in whole blood samples from non-infected endemic controls (NI), asymptomatic individuals (AS) along with visceral leishmaniasis patients before (VL-BT) and after treatment (VL-AT) by flow cytometry, as described in Material and Methods. Data are shown as scattering distribution of individual values over bar charts representing the median values. Significant differences amongst groups were considered at p<0.05 and indicated by connecting lines. (C) The percentage of L. infantum internalization and NO production by activated and non-activated neutrophils and (D) total monocytes were determined upon in vitro whole blood cultures referred as: L. infantum and L. infantum + α-MR1, as described in Material and Methods. The results are shown in bar charts representing the median (IQR) values for the percentage of L. infantum internalization and the geometric mean of Mean Fluorescence Intensity (gMFI) for NO production. Significant differences amongst groups were indicated by connecting lines, and the asterisks represent the significancy levels: *p < 0.05, **p < 0.01, and ***p < 0.001. (E) Representative flow cytometric charts using pseudocolor density plots illustrate the strategies used to selected neutrophils subsets (Total – CD16+SSCHigh, non-activated - HLA-DR–CD16+ and activated - HLA-DR+CD16+) and total monocytes (CD14+SSCInt; CD14+DR+) and histograms to quantify the percentage of L. infantum internalization and NO production.
Lower rates of Leishmania internalization were observed in monocytes from VL-BT and VL-AT groups as compared to NI and AS, in both culture conditions (Figure 1D – top panels). Monocytes from VL-BT produced less NO as compared to NI and AS in the L. infantum stimulated culture, and the addition of MR1 blockade to the cultures lead to lower NO production by monocytes from AS compared to NI (Figure 1D – bottom panels).
Representative flow cytometric charts containing the gating strategies used to selected neutrophils and monocytes subsets as well as the approaches used to quantify the L. infantum internalization and NO production are illustrated on Figure 1E.
The functional profile of circulating neutrophils and monocytes was assessed in whole blood cultures upon stimuli with live L. infantum promastigotes in the absence/presence of α-MR1 blocking mAb. The results are expressed as ratio of L. infantum + α-MR1/L. infantum cultures and are presented in Figure 2. Data analysis demonstrated that the MR1 blockade led to lower expression of TNF-α by non-activated neutrophils from AS group in comparison to all other groups (Figure 2A). Moreover, MR1-blockage reduced the TGF-β production by activated neutrophils from AS when compared to all other groups (Figure 2B). Additionally, TNF-α and IL-10 production by monocytes was higher in VL-BT and VL-AT as compared to NI and even higher in AS in comparison to NI and VL-BT (Figure 2C). Heatmap constructs further corroborate these findings illustrating that, overall, the presence of MR1 blockade impaired the functional response of neutrophils in NI, VL-BT and VL-AT, while it improved the response of monocytes, particularly in AS individuals (Figure 2D).
Figure 2 Impact of MR1 blockade on the functional profile of circulating neutrophils and monocytes. (A) The frequencies (%) of non-activated neutrophils and (B) activated neutrophils expressing (TNF+, IL-12+, IFN-γ+, IL-10+ and TGF-β+) and (C) total monocytes expressing (TNF+, IL-12+, IL-10+ and TGF-β+) were quantified in whole blood samples from non-infected endemic controls (NI), asymptomatic individuals (AS) along with visceral leishmaniasis patients before (VL-BT) and after treatment (VL-AT) by intracytoplasmic flow cytometry, as described in Material and Methods. Data are shown in bar charts representing the median (IQR) values of L. infantum + α-MR1/L. infantum ratio. Significant differences amongst groups were indicated by connecting lines, and the asterisks represent the significancy levels: *p < 0.05, and **p < 0.01. (D) Heatmap constructs illustrate the functional profiles neutrophils and monocytes in NI, AS, VL-BT and VL-AT groups.
To investigate the impact of the MR1 blockade on the expression of soluble mediators regulating anti-Leishmania immunity, we established signatures of chemokines, cytokines, and growth factors present in the supernatant of our in vitro cultures stimulated with live L. infantum promastigotes in the absence/presence of α-MR1 mAb. The results expressed as the proportion (%) of samples with soluble mediator index (L. infantum/CC and L. infantum + α-MR1/CC) above the global median cut-off and are presented in Figure 3. Data demonstrated that MR1 blockade induced distinct signatures in each clinical group. From the quantitative point of view, MR1 blockade reduced the set of soluble mediators with an increased index in NI and VL-AT. Conversely, the MR1 blockade increased the number of soluble mediators with increased index in AS and VL-BT (Figure 3A). Qualitative analysis indicated that MR1 blockade was associated with a decrease of IFN-γ and IL-17A in NI, while in AS, it was associated with a decrease of IFN-γ and an increase of IL-10, IL-27 and IL-33. Increase of IL-27 and IL-33 upon MR1 blockade were reported for VL-BT, whereas reduction of IL-17 and IL-23 with upregulation of IL-10 was identified in VL-AT (Figure 3A, dashed color rectangles). Ascendant soluble mediator signatures further pointed out the selected soluble mediators increased in the absence or presence of MR1 blockade (Figure 3B). The analysis of common and selective soluble mediators revealed distinct sets of attributes associated with each clinical group, however, increased CXCL10 secretion was universally attributed to MR1 blockade (Figure 3B).
Figure 3 Impact of MR1 blockade on soluble mediator signatures of in vitro whole blood cultures. (A) The soluble mediators chemokine (CXCL8, CCL11, CCL3, CCL4, CCL2, CCL20, CXCL10), pro-inflammatory cytokines (IL-1β, IL-6, TNF, IL-12, IL-15, IFN-γ), regulatory cytokines (IL-1Ra, IL-4, IL-5, IL-9, IL-10, IL-27, IL-33), Th17/Th22 axis cytokines (IL-17, IL-21, IL-22, IL-23) and growth factors (FGF, PDGF, VEGF, G-CSF, GM-CSF, IL-2, IL-7) were measured in the supernatant of in vitro whole blood cultures from non-infected endemic controls (NI), asymptomatic individuals (AS) along with visceral leishmaniasis patients before (VL-BT) and after treatment (VL-AT) by Luminex Bio-plex platform, as described in Material and Methods. (A) The data are shown in Lollipop charts representing the proportion (%) of samples with soluble mediator index (L.i/CC and L.i + α-MR1/CC) above the global median cut-off (grey zone). Qualitative features associated with MR1 blockade on each group was underscored by color dashed rectangles. (B) Heatmap constructs illustrate the ascendant soluble mediator signatures. The biomarkers with proportion above the 50th percentile were highlighted by dashed rectangles and the soluble mediators increased in L.i/CC and L.i + α-MR1/CC culture condition on each group underscored by bold underline format.
Integrative networks of soluble mediators were built to further characterize the impact of the MR1 blockade in the in vitro whole blood culture. The results are presented in Figure 4. Data analysis demonstrated that while NI (n=546) and AS (n=632) groups exhibited a higher number of correlations upon L. infantum stimuli, lower connectivity between soluble mediators was observed for VL-BT (n=260) and VL-AT (n=138). Conversely, the connections between soluble mediators waned upon MR1 blockade in NI (n=74) and AS (n=128), whereas a slight increase in connectivity was identified for VL-BT (n=334) and VL-AT (n=274) (Figure 4).
Figure 4 Impact of MR1 blockade on soluble mediator integrative networks of in vitro whole blood cultures. Comprehensive correlation matrices and networks of chemokines (CXCL8, CCL11, CCL3, CCL4, CCL2, CCL20, CXCL10), pro-inflammatory cytokines (IL-1β, IL-6, TNF, IL-12, IL-15, IFN-γ), regulatory cytokines (IL-1Ra, IL-4, IL-5, IL-9, IL-10, IL-27, IL-33), Th17/Th22 axis cytokines (IL-17, IL-21, IL-22, IL-23) and growth factors (FGF, PDGF, VEGF, G-CSF, GM-CSF, IL-2, IL-7) were assembled based on the significant (p<0.05) “r” correlation scores obtained for in vitro whole blood cultures (L. infantum and L. infantum + α-MR1) from non-infected endemic controls (NI) and asymptomatic individuals (AS) along with visceral leishmaniasis patients before (VL-BT) and after treatment (VL-AT), as described in Material and Methods. Triangle matrices were assembled to compile the “r” correlation scores for each group. The integrative networks were constructed using cluster layouts comprising five categories of parameters including: chemokines (1-7), pro-inflammatory (8-13), regulatory (14-20) and Th17/Th22 cytokines (21-24) along with growth factors (25-31). The node sizes are proportional to the number of correlations between biomarkers. The number of correlations involving each category as well as the total correlation number are provided in the figure. Circular backgrounds underscore the proportional contribution of each category of parameters to the overall connectivity.
To further characterize the distinct involvement of soluble mediators in the overall connectivity profile, we quantified the connections in each soluble mediator cluster C (chemokine), Pro (pro-inflammatory), Reg (regulatory), Th17/22 and GF (grow factor), for each clinical group (Figure 5). Data demonstrated that the depletion of connections observed in NI and AS upon MR1 blockade impacted all soluble mediator clusters, while in VL-BT and VL-AT, the increase in connectivity was more prominent in the Reg and GF clusters (Figure 5).
Figure 5 Involvement of soluble mediator categories in integrative networks of in vitro whole blood cultures. The number of correlations involving categories of soluble mediators, including: chemokines (C), pro-inflammatory (Pro), regulatory (Reg), Th17/Th22 axis (Th17/22) cytokines and growth factors (GF) was calculated from integrative networks of in vitro whole blood cultures (L. infantum and L. infantum + α-MR1) from non-infected endemic controls (NI) and asymptomatic individuals (AS) along with visceral leishmaniasis patients before (VL-BT) and after treatment (VL-AT). Data are shown as line charts with symbol sizes proportional to the number of correlations involving each category of soluble mediator.
Aiming at further understanding the connectivity profile between soluble mediators, descriptive analysis of the integrative networks was performed to identify sets of soluble mediators with increased (>2x) or decreased (<0.5x) connectivity upon MR1 blockade. These results are presented in Figure 6. The results further evidenced the differential impact of MR1 blockade on the integrative networks across the distinct clinical groups. While MR1 blockade substantially reduced the connectivity throughout all sets of soluble mediators in NI and AS, minor increases were observed in VL-BT and VL-AT (Figure 6A). In NI and AS, the loss of connectivity in L. infantum + α-MR1/L. infantum ratio involved all sets of soluble mediators (Figure 6B). Conversely, a restricted set of soluble mediators (CCL3, IFN-γ, IL-27, IL-22 and PDGF) drove the increased connectivity observed in VL-BT. Of note, the impact of MR1 blockade in VL-AT was more robust and affected a larger number of soluble mediators (CXCL8, CCL11, CCL4, IL-6, IL-12, IFN-γ, IL-1Ra, IL-4, IL-5, IL-9, IL-27, IL-23, PFGF, VEGF, G-CSF and GM-CSF) (Figure 6B).
Figure 6 Descriptive analysis of soluble mediator integrative networks of in vitro whole blood cultures. (A) The number of correlations involving chemokines (CXCL8, CCL11, CCL3, CCL4, CCL2, CCL20, CXCL10), pro-inflammatory cytokines (IL-1β, IL-6, TNF, IL-12, IL-15, IFN-γ), regulatory cytokines (IL-1Ra, IL-4, IL-5, IL-9, IL-10, IL-27, IL-33), Th17/Th22 axis cytokines (IL-17, IL-21, IL-22, IL-23) and growth factors (FGF, PDGF, VEGF, G-CSF, GM-CSF, IL-2, IL-7) was assembled from integrative networks of in vitro whole blood cultures (L. infantum and L. infantum + α-MR1) from non-infected endemic controls (NI) and asymptomatic individuals (AS) along with visceral leishmaniasis patients before (VL-BT) and after treatment (VL-AT). Data are shown as orbital charts summarizing the number of correlations for each group. (B) Changes in biomarker connectivity upon MR1 blockade were estimated as L. infantum + α-MR1/L. infantum ratio to identify soluble mediators with increased (>2x) or decreased (<0.5x) connectivity. Color frames and backgrounds highlight the sets of soluble mediators with changes in connectivity. * Underscores the biomarkers with increased connectivity upon MR1 blockade. Squares represents the biomarkers that did not presented changes (0.5>x>2x) in connectivity and triangles represents the biomarkers that did present changes.
Additionally, analysis was carried out to further identify the impact of MR1 blockade on selective circuits within each clinical group (Supplementary Figure 1). Data demonstrated that changes in the pattern of intra-circuit connectivity upon MR1 blockade are specific for each clinical group (Supplementary Figure 1A). The NI group was characterized by loss of connectivity in chemokines and by the increase in Th17/22 and growth factors clusters. In AS, increase in connectivity was exclusively observed in the pro-inflammatory cytokine cluster. Both VL-BT and VL-AT presented a decrease in connectivity involving Th17/22 and an increase in connectivity of clusters containing growth factors. Particularly in VL-BT, the MR1 blockade led to a decrease of connectivity within the pro-inflammatory cluster (Supplementary Figure 1B). All in all, these results suggest important impact of MR1 blockade in cluster connectivity of soluble mediators of VL before and after treatment as well as, AS and endemic controls.
MR1 is a non-classical antigen-presenting molecule MHC class I-related. Unlike other antigen-presenting molecules (MHC-I and MHC-II), in the absence of infection, MR1 is hardly detectable on the surface of human cells. The MR1 molecule is retained in the endoplasmic reticulum, and it is readily upregulated in ligands presence (28, 29).
The importance of MR1 molecule and MR1-restricted cells has been described in a wide spectrum of infectious diseases caused by fungi, bacteria, and viruses (36–40). Notably, our research group previously revealed the involvement of MR1-restricted cells in a protozoan infection caused by Leishmania (34).
In this study, we describe the impact of the MR1 blockade on L. infantum internalization and on the functional profile of circulating neutrophils and monocytes as well as the impact of the MR1 blockade in the soluble mediator signatures in vitro. These analyses were carried out upon in vitro whole blood cultures referred as: L. infantum and L. infantum + α-MR1. Due to ethical restriction for blood samples collect from children, we were unable to conduct additional cultures, including: Cells + α-MR1, Cells + α-IL-12/Il-18, and L. infantum + α- IL-12/IL-18.
Numerous studies have characterized the phenotypic and functional changes in phagocytes, including both polymorphonuclear and mononuclear cells, in the context of Leishmania spp. and various other pathogens. These investigations have contributed significantly to our understanding about MHC-I and MHC-II antigen presentation (41). However, there is a notable lack of literature aiming at elucidating specific alterations occurring in phagocytes concerning antigen presentation via MR1 and the consequences of blocking this molecule within these cells. To our knowledge, the current study stands out as the pioneering effort to shed light on the impact of MR1 blockade on phagocytes upon Leishmania spp. infection.
Considering the Leishmania infection, neutrophils are the initial innate immune cells to be recruited to the infection site (8, 9, 42). Neutrophils exhibit various anti-parasitic mechanisms, such as the phagocytosis of parasites (10, 11). Previous studies have demonstrated that neutrophils from patients with VL can phagocyte a comparable number of L. donovani as neutrophils from healthy controls (43, 44). However, our analysis revealed a different outcome: both activated and non-activated neutrophils from VL patients exhibit reduced Leishmania internalization as compared to non-infected (NI) individuals, which could be related to differences in our in vitro method. The impaired rates of Leishmania internalization profiles are found even after treatment. In line with these observations, a secondary role of neutrophils has been suggested regarding the ability of those cells in facilitating Leishmania infection of monocytes when they undergo apoptosis (45, 46). Monocytes play a pivotal role in Leishmania infection as the primary host cells. The primary route of infection involves direct phagocytosis of the remaining parasites by these monocytes (47). In a study performed by Singh and colleagues (48), monocytes from patients with VL exhibited a reduced phagocytic ability as compared to those from endemic controls, corroborating with our findings. Monocytes from VL patients presented reduced phagocytic capacity when compared to monocytes from NI and AS individuals, before and after treatment. Furthermore, our investigation revealed that the MR1 blockade did not alter this phagocytic profile, highlighting the persistence of this impairment in VL patients. Previous findings have established a connection between Toll-Like Receptors (TLRs) and the internalization of Leishmania by neutrophils and monocytes (49, 50). In our study, we investigated whether the blockade of the MR1 molecule could modify the internalization pattern. However, our analysis demonstrated that the internalization pattern remained unaltered, indicating that MR1 does not exert a significant influence on this process.
Both neutrophils and monocytes produce NO as an anti-Leishmania mechanism. Our data showed increase in the NO production by neutrophils in VL patients as compared to NI group. Neutrophils have been shown to play a protective role during visceral leishmaniasis (8, 9, 51). In experimental models it has been showed that neutrophils are activated and produce TNF-α and NO in a TLR2-dependent manner, after L. infantum stimulation (51). In fact, neutrophils have an independent role in orchestrating the early cytokine/chemokine responses, and actively contribute to molding adaptive immune responses against the parasite (8, 16). Our data corroborate with these studies showing elevated frequency of neutrophils IL-12+, IFN-γ+, TNF-α+, IL-10+, and TGF-β+, especially in VL groups (Figure 2D).
Despite the relevance of elucidating the role of monocytes subsets (CD14++CD16- and CD14dimCD16+) in Leishmania infection (52) in the present study, the functional profile of monocytes was evaluated using total CD14+, since it was not possible to accurately access the functional profile of monocytes subsets, mainly due to the low monocytes counts in peripheral blood from VL patients and the ethical restriction to collect large amount of samples from children. Several reports indicate that patients with VL exhibit lower levels of NO production by total monocytes in comparison to healthy controls (51, 52). Considering the relevance of characterizing the functional aspects of classical (CD14++CD16-) and non-classical (CD14dimCD16++) monocytes upon MR1-blockade, additional studies would contribute to bring about insightful and interesting findings to elucidate the role of monocytes subsets in Leishmania infection. Our results are in accordance with these observations.
Here, we showed a high NO production by total monocytes in NI and AS individuals as compared to VL patients. It has been described that monocyte exhibited distinct behaviors depending on the type of activation. Several studies have established that the development of Th1 responses, characterized by the production of cytokines such as TNF-α and IL-12, leads to the clearance of parasites and resistance to leishmaniasis. In contrast, a Th2 type response, marked by elevated levels of IL-10 and TGF-β, is associated with susceptibility to infection, disease progression, parasite replication and persistence (17, 19, 20, 53). Our findings indicate that the presence of the MR1 blockade leads to an increase in IL-10 levels in AS individuals. This result aligns with the observation that MR1 blockade contributes to reducing the IFN-γ levels and increasing the IL-10, IL-27 and IL-33 levels in the supernatant of in vitro cultures from the AS group. This scenario raises the possibility that the MR1 molecule may have a protective role in the context of VL, since high levels of IL-10 is a well-established feature of patients with classical VL (54). Furthermore, IL-27 has been recognized as a factor that promotes disease, by facilitating the differentiation and expansion of T cells that produce IL-10 in vitro (55, 56). The induction of IL-10 can occur through various pathways involving IL-27 (57). Recently, elevated IL-27 levels were associated to dysfunctional immune response to infectious diseases, potentially leading to significant tissue damage (58).
Further analysis of the supernatant compartment revealed that blocking MR1 increased the secretion of CXCL10, which was found as a common feature present across all the clinical groups. Previous studies have illustrated that parasite-infected Kupffer cells swiftly release CXCL10 to attract inflammatory monocytes and T-cells, thereby forming a granuloma that initiates anti-parasitic immune responses (59, 60). Moreover, CXCL10 has the potential to enhance protective immune responses during VL, by upregulating pro-inflammatory mediators, Th1-cell cytokines, and NO production in vivo (61). In a recent study, it was noted that CXCL10 could suppress IL-10+ Treg-cells within the spleen of mice infected with L. infantum, resulting in a reduction of the parasite load in the spleen of these mice (62). Conversely, excessive production of CXCL10 and CXCL9 has been associated with disease severity in VL, potentially leading to tissue damage due to uncontrolled inflammation (63, 64).
In addition to the well-recognized Th1 and Th2 immune responses, Th17 cells appear as a significant protective subset that contributes to controlling VL infection (65, 66). It has been demonstrated that IL-17A collaborates with IFN-γ to enhance NO production in infected macrophages (67). Besides, the cytokines associated with the Th17 axis strongly correlates with the asymptomatic VL. This protective effect is related to an increase in CXCL chemokines, which serve as potent chemoattractants for both neutrophils and Th1-cells, ultimately contributing to parasite clearance (66, 68). Terrazas and colleagues reported that Tγδ cells are a significant source of IL-17A in the spleen of VL patients (23). Tγδ cells are known to produce IL-17A in response to stimuli like dendritic cell-derived cytokines, such as IL-1β or IL-23, or direct interaction with pathogens (69). In addition, another significant “innate-like” T cell subset comprise MAIT cells, which is recognized for expressing high levels of IL-17A in a variety of infections and non-infectious diseases (70–72).
Despite our ability to extract a substantial amount of data from the provided samples, it is crucial to recognize the encountered limitations. The “VL-AT” group, evaluated within the initial 20-40 days after treatment, might not comprehensively depict the long-term dynamics of cytokine kinetics. Future studies are yet required in order to evaluate the immunological profile of patients after 90 and 180 days of treatment to expand our understanding on the impact of treatment on cytokine production.
In summary, our findings provide evidence of the impact of the MR1 blockade in the functional profile of phagocytes and the overall production of soluble mediators in the context of VL. Our results also support the hypothesis about the protective role of MR1 and MR1-restricted cells in asymptomatic VL individuals. Nevertheless, further investigations are warranted to address extensively and precisely the roles played by MR1 and MR1-restricted cells in protozoan infections, particularly in the Visceral Leishmaniasis.
The original contributions presented in the study are included in the article/Supplementary Material. Further inquiries can be directed to the corresponding author/s.
The studies involving humans were approved byComitê de Ética – Instituto René Rachou - Fiocruz/Minas Av. Augusto de Lima, 1715 – Barro Preto - Belo Horizonte (Cep: 30190-002) Telefone (31): 3349 7825 e-mail: Y2VwY29vcmQubWluYXNAZmlvY3J1ei5icg==. The studies were conducted in accordance with the local legislation and institutional requirements. Written informed consent for participation in this study was provided by the participants’ legal guardians/next of kin.
LB-F: Conceptualization, Data curation, Formal analysis, Investigation, Methodology, Writing – original draft, Writing – review & editing. MD: Conceptualization, Data curation, Investigation, Methodology, Validation, Writing – original draft. VP: Formal analysis, Investigation, Methodology, Writing – original draft, Writing – review & editing. MP-X: Conceptualization, Formal analysis, Writing – review & editing. ÁL: Formal analysis, Writing – review & editing. IC-R: Formal analysis, Methodology, Writing – original draft, Writing – review & editing. LL: Formal analysis, Investigation, Methodology, Writing – review & editing. GM: Formal analysis, Investigation, Methodology, Writing – review & editing. MA: Formal analysis, Investigation, Methodology, Writing – review & editing. AT-C: Conceptualization, Formal analysis, Investigation, Methodology, Writing – review & editing. JPB: Formal analysis, Visualization, Writing – original draft, Writing – review & editing. AC: Methodology, Writing – review & editing. MM: Methodology, Writing – review & editing. FC: Methodology, Writing – review & editing. MB: Methodology, Writing – review & editing. MC: Methodology, Writing – review & editing. MT: Conceptualization, Formal analysis, Writing – review & editing. OM-F: Conceptualization, Formal analysis, Writing – original draft, Writing – review & editing. JC-R: Conceptualization, Data curation, Formal analysis, Funding acquisition, Investigation, Methodology, Project administration, Resources, Software, Supervision, Validation, Visualization, Writing – original draft, Writing – review & editing. VP-M: Conceptualization, Data curation, Formal analysis, Funding acquisition, Investigation, Methodology, Project administration, Resources, Software, Supervision, Validation, Visualization, Writing – original draft, Writing – review & editing.
The author(s) declare financial support was received for the research, authorship, and/or publication of this article. This study was supported by Conselho Nacional de Desenvolvimento Científico e Tecnológico – CNPq [Grant# 458134/2014-7], Fundação de Amparo à Pesquisa do Estado de Minas Gerais – FAPEMIG [Grant# APQ-01754-2014; PPM-00497-2016; APQ-02182-2018] and Fundação Oswaldo Cruz (FIOCRUZ). The study was carried out by students enrolled at Programa de Pós-graduação em Ciências da Saúde (FIOCRUZ-Minas), supported by the Coordenação de Aperfeiçoamento de Pessoal de Nível Superior (CAPES). JC-R received a fellowship from Brazilian National Postdoctoral Fellowship Program (PNPD/CAPES). MA, MC, AT-C, OM-F, and JC-R thank the CNPq for the Senior Research Productivity Fellowships (PQ). OM-F participated in the fellow program supported by the Universidade do Estado do Amazonas (PROVISIT N° 005/2023-PROPESP/UEA). MT received financial support from the National Institutes of Health [R01-AI070258]. The authors thank the Program for Technological Development in Tools for Health-RPT-FIOCRUZ for using the flow cytometry facilities. We thank Fernanda Vianni and Daniela Caldas Teixeira for medical assistance.
The authors declare that the research was conducted in the absence of any commercial or financial relationships that could be construed as a potential conflict of interest.
The author(s) declared that they were an editorial board member of Frontiers, at the time of submission. This had no impact on the peer review process and the final decision
All claims expressed in this article are solely those of the authors and do not necessarily represent those of their affiliated organizations, or those of the publisher, the editors and the reviewers. Any product that may be evaluated in this article, or claim that may be made by its manufacturer, is not guaranteed or endorsed by the publisher.
The Supplementary Material for this article can be found online at: https://www.frontiersin.org/articles/10.3389/fimmu.2024.1373498/full#supplementary-material
1. Arenas R, Torres-Guerrero E, Quintanilla-Cedillo MR, Ruiz-Esmenjaud J. Leishmaniasis: A review. F1000Res. (2017) 6:1–15. doi: 10.12688/f1000research.11120.1
2. Costa CH, Werneck GL, Costa DL, Holanda TA, Aguiar GB, Carvalho AS, et al. Is severe visceral leishmaniasis a systemic inflammatory response syndrome? A case control study. Rev Soc Bras Med Trop. (2010) 43(4):386–92. doi: 10.1590/s0037-86822010000400010
3. Rodrigues V, Cordeiro-Da-Silva A, Laforge M, Silvestre R, Estaquier J. Regulation of immunity during visceral Leishmania infection. Parasit Vectors. (2016) 9:1–13. doi: 10.1186/s13071-016-1412-x
4. Sacks D, Lal S, Shrivastava S, Blackwell S, Neva F. An analysis of T cell responsiveness in Indian kala-azar. J Immunol. (1987) 138:908–913. doi: 10.4049/jimmunol.138.3.908
5. Pérez-Cabezas B, Cecílio P, Robalo AL, Silvestre R, Carrillo E, Moreno J, et al. Interleukin-27 early impacts Leishmania infantum infection in mice and correlates with active visceral disease in humans. Front Immunol. (2016) 7:478. doi: 10.3389/fimmu.2016.00478
6. dos Santos PL, de Oliveira FA, Santos MLB, Cunha LCS, Lino MTB, de Oliveira MFS, et al. The Severity of Visceral Leishmaniasis Correlates with Elevated Levels of Serum IL-6, IL-27 and sCD14. PLoS Negl Trop Dis. (2016) 10:1–16. doi: 10.1371/journal.pntd.0004375
7. Coura-Vital W, de Araújo VEM, Reis IA, Amancio FF, Reis AB, Carneiro M. Prognostic Factors and Scoring System for Death from Visceral Leishmaniasis: An Historical Cohort Study in Brazil. PLoS Negl Trop Dis. (2014) 8:1–12. doi: 10.1371/journal.pntd.0003374
8. McFarlane E, Perez C, Charmoy M, Allenbach C, Carter KC, Alexander J, et al. Neutrophils contribute to development of a protective immune response during onset of infection with Leishmania donovani. Infect Immun. (2008) 76:532–41. doi: 10.1128/IAI.01388-07
9. Thalhofer CJ, Chen Y, Sudan B, Love-Homan L, Wilson ME. Leukocytes infiltrate the skin and draining lymph nodes in response to the protozoan leishmania infantum chagasi. Infect Immun. (2011) 79:108–17. doi: 10.1128/IAI.00338-10
10. Hurrell BP, Regli IB, Tacchini-Cottier F. Different Leishmania Species Drive Distinct Neutrophil Functions. Trends Parasitol. (2016) 32:392–401. doi: 10.1016/j.pt.2016.02.003
11. Kolaczkowska E, Kubes P. Neutrophil recruitment and function in health and inflammation. Nat Rev Immunol. (2013) 13:159–75. doi: 10.1038/nri3399
12. Romani L, Mencacci A, Cenci E, Del Sero G, Bistoni F, Puccetti P. An immunoregulatory role for neutrophils in CD4+ T helper subset selection in mice with candidiasis. J Immunol. (1997) 158:2356–62. doi: 10.4049/jimmunol.158.5.2356
13. Ribeiro-Gomes FL, Otero AC, Gomes NA, Carolina M, Moniz-De-Souza A, Cysne-Finkelstein L, et al. Macrophage Interactions with Neutrophils Regulate Leishmania major Infection. J Immunol (2004) 172:4454–62. doi: 10.4049/jimmunol.172.7.4454
14. Tsuda Y, Takahashi H, Kobayashi M, Hanafusa T, Herndon DN, Suzuki F. Three Different Neutrophil Subsets Exhibited in Mice with Different Susceptibilities to Infection by Methicillin-Resistant Staphylococcus aureus immunities (Verhoef, 1997). Polymorphonuclear neutro-phils (PMNs) have been described as critical effector cells in the host’s immune response against microbial invasion (Verhoef, 1997). PMNs adhere to endothelium at the sites of infection in response to signals generated. Immunity (2004) 21:215–26. doi: 10.1016/j.immuni.2004.07.006
15. Charmoy M, Megnekou R, Allenbach C, Zweifel C, Perez C, Monnat K, et al. Leishmania major induces distinct neutrophil phenotypes in mice that are resistant or susceptible to infection. J Leukoc Biol. (2007) 82:288–99. doi: 10.1189/jlb.0706440
16. Mantovani A, Cassatella MA, Costantini C, Jaillon S. Neutrophils in the activation and regulation of innate and adaptive immunity. Nat Rev Immunol. (2011) 11:519–31. doi: 10.1038/nri3024
17. Gordon S. Alternative activation of macrophages. Nat Rev Immunol. (2003) 3:23–35. doi: 10.1038/nri978
18. Mantovani A, Sica A, Sozzani S, Allavena P, Vecchi A, Locati M. The chemokine system in diverse forms of macrophage activation and polarization. Trends Immunol. (2004) 25:677–86. doi: 10.1016/j.it.2004.09.015
19. Peruhype-Magalhães V, Martins-Filho OA, Prata A, Silva LDA, Rabello A, Teixeira-Carvalho A, et al. Mixed inflammatory/regulatory cytokine profile marked by simultaneous raise of interferon-γ and interleukin-10 and low frequency of tumour necrosis factor-α+ monocytes are hallmarks of active human visceral Leishmaniasis due to Leishmania chagasi infection. Clin Exp Immunol. (2006) 146:124–32. doi: 10.1111/j.1365-2249.2006.03171.x
20. Martinez FO, Helming L, Gordon S. Alternative activation of macrophages: An immunologic functional perspective. Annu Rev Immunol. (2009) 27:451–83. doi: 10.1146/annurev.immunol.021908.132532
21. Shapouri-Moghaddam A, Mohammadian S, Vazini H, Taghadosi M, Esmaeili SA, Mardani F, et al. Macrophage plasticity, polarization, and function in health and disease. J Cell Physiol. (2018) 233(9):6425–6440. doi: 10.1002/jcp.26429
22. Khatonier R, Ahmed G, Sarmah P, Narain K, Khan AM. Immunomodulatory role of Th17 pathway in experimental visceral leishmaniasis. Immunobiology. (2021) 226:152148. doi: 10.1016/j.imbio.2021.152148
23. Terrazas C, Varikuti S, Kimble J, Moretti E, Boyaka PN, Satoskar AR. IL-17A promotes susceptibility during experimental visceral leishmaniasis caused by Leishmania donovani. FASEB J. (2016) 30:1135–43. doi: 10.1096/fj.15-277202
24. Le Bourhis L, Dusseaux M, Bohineust A, Bessoles S, Martin E, Premel V, et al. MAIT Cells Detect and Efficiently Lyse Bacterially-Infected Epithelial Cells. PLoS Pathog. (2013) 9:1–12. doi: 10.1371/journal.ppat.1003681
25. Kurioka A, Ussher JE, Cosgrove C, Clough C, Fergusson JR, Smith K, et al. MAIT cells are licensed through granzyme exchange to kill bacterially sensitized targets. Mucosal Immunol. (2015) 8:429–40. doi: 10.1038/mi.2014.81
26. Leeansyah E, Svärd J, Dias J, Buggert M, Nyström J, Quigley MF, et al. Arming of MAIT Cell Cytolytic Antimicrobial Activity Is Induced by IL-7 and Defective in HIV-1 Infection. PLoS Pathog. (2015) 11:1–23. doi: 10.1371/journal.ppat.1005072
27. Salou M, Legoux F, Gilet J, Darbois A, Du Halgouet A, Alonso R, et al. A common transcriptomic program acquired in the thymus defines tissue residency of MAIT and NKT subsets. J Exp Med. (2019) 216:133–51. doi: 10.1084/jem.20181483
28. Tilloy F, Treiner E, Park S-H, Garcia C, Lemonnier F, de la Salle H, et al. An Invariant T Cell Receptor Chain Defines a Novel TAP-independent Major Histocompatibility Complex Class Ib-restricted / T Cell Subpopulation in Mammals. J Exp Med (1999) 189(12):1907–21. doi: 10.1084/jem.189.12.1907
29. Treiner E, Duban L, Bahram S, Radosavljevic M, Wanner V, Tilloy F, et al. Selection of evolutionarily conserved mucosal-associated invariant T cells by MR1. Nature (2003) 422:164–169. doi: 10.1038/nature01433
30. Martin E, Treiner E, Duban L, Guerri L, Laude H, Toly C, et al. Stepwise Development of MAIT Cells in Mouse and Human. PLoS Biol. (2009) 7:e1000054. doi: 10.1371/journal.pbio.1000054
31. Gapin L. Where do mait cells fit in the family of unconventional t cells? PLoS Biol. (2009) 7:0435–8. doi: 10.1371/journal.pbio.1000070
32. Godfrey DI, Rossjohn J, McCluskey J. Fighting infection with your MAITs. Nat Immunol. (2010) 11:693–5. doi: 10.1038/ni0810-693
33. Dusseaux M, Martin E, Serriari N, Péguillet I, Premel V, Louis D, et al. Human MAIT cells are xenobiotic-resistant, tissue-targeted, CD161 hi IL-17-secreting T cells. Blood. (2011) 117:1250–9. doi: 10.1182/blood-2010-08-303339
34. Moreira M de L, Borges-Fernandes LO, Pascoal-Xavier MA, Ribeiro ÁL, Pereira VHS, Pediongco T, et al. The role of mucosal-associated invariant T cells in visceral leishmaniasis. Front Immunol. (2022) 13:926446. doi: 10.3389/fimmu.2022.926446
35. Schachnik NCC, Peruhype-Magalhães V, Paula GMM, Lucas F, Freitas VM, Martins-Filho OA, et al. Intracellular nitric oxide assessment in whole blood leukocytes by flow cytometry: Optimization and applicability to monitor patients with chronic graft nephropathy. J Immunol Methods. (2009) 343:103–11. doi: 10.1016/j.jim.2009.01.010
36. Le Bourhis L, Martin E, Péguillet I, Guihot A, Froux N, Coré M, et al. Antimicrobial activity of mucosal-associated invariant T cells. Nat Immunol. (2010) 11:701–8. doi: 10.1038/ni.1890
37. Georgel P, Radosavljevic M, Macquin C, Bahram S. The non-conventional MHC class I MR1 molecule controls infection by Klebsiella pneumoniae in mice. Mol Immunol. (2011) 48:769–75. doi: 10.1016/j.molimm.2010.12.002
38. Leeansyah E, Ganesh A, Quigley MF, Sönnerborg A, Andersson J, Hunt PW, et al. Activation, exhaustion, and persistent decline of the antimicrobial MR1-restricted MAIT-cell population in chronic HIV-1 infection. Blood. (2013) 121:1124–35. doi: 10.1182/blood-2012-07-445429
39. Gold MC, Napier RJ, Lewinsohn DM. MR1-restricted mucosal associated invariant T (MAIT) cells in the immune response to Mycobacterium tuberculosis. Immunol Rev (2015) 264(1):154–66. doi: 10.1111/imr.12271
40. Wang H, D’Souza C, Lim XY, Kostenko L, Pediongco TJ, Eckle SBG, et al. MAIT cells protect against pulmonary Legionella longbeachae infection. Nat Commun. (2018) 9:1–15. doi: 10.1038/s41467-018-05202-8
41. Serbina NV, Jia T, Hohl TM, Pamer EG. Monocyte-mediated defense against microbial pathogens. Annu Rev Immunol. (2008) 26:421–52. doi: 10.1146/annurev.immunol.26.021607.090326
42. Ribeiro-Gomes FL, Sacks D. The influence of early neutrophil-Leishmania interactions on the host immune response to infection. Front Cell Infect Microbiol. (2012) 2:59. doi: 10.3389/fcimb.2012.00059
43. Yizengaw E, Getahun M, Tajebe F, Cervera EC, Adem E, Mesfin G, et al. Visceral leishmaniasis patients display altered composition and maturity of neutrophils as well as impaired neutrophil effector functions. Front Immunol. (2016) 7:517. doi: 10.3389/fimmu.2016.00517
44. Sharma S, Srivastva S, Davis RE, Singh SS, Kumar R, Nylén S, et al. The phenotype of circulating neutrophils during visceral leishmaniasis. Am J Trop Med Hygiene. (2017) 97:767–70. doi: 10.4269/ajtmh.16-0722
45. Peters NC, Egen JG, Secundino N, Debrabant A, Kimblin N, Kamhawi S, et al. In Vivo Imaging Reveals an Essential Role for Neutrophils in Leishmaniasis Transmitted by Sand Flies. Sci (1979). (2008) 321:970–4. doi: 10.1126/science.1159194
46. Ritter U, Frischknecht F, van Zandbergen G. Are neutrophils important host cells for Leishmania parasites? Trends Parasitol. (2009) 25:505–10. doi: 10.1016/j.pt.2009.08.003
47. Liu D, Uzonna JE. The early interaction of Leishmania with macrophages and dendritic cells and its influence on the host immune response. Front Cell Infect Microbiol. (2012) 2:83. doi: 10.3389/fcimb.2012.00083
48. Singh N, Kumar R, Chauhan SB, Engwerda C, Sundar S. Peripheral blood monocytes with an antiinflammatory phenotype display limited phagocytosis and oxidative burst in patients with visceral leishmaniasis. J Infect Dis. (2018) 218:1130–41. doi: 10.1093/infdis/jiy228
49. Tuon FF, Amato VS, Bacha HA, AlMusawi T, Duarte MI, Neto VA. Toll-like receptors and leishmaniasis. Infect Immun. (2008) 76:866–72. doi: 10.1128/IAI.01090-07
50. Gurung P, Kanneganti TD. Innate immunity against Leishmania infections. Cell Microbiol. (2015) 17:1286–94. doi: 10.1111/cmi.12484
51. Sacramento LA, da Costa JL, de Lima MHF, Sampaio PA, Almeida RP, Cunha FQ, et al. Toll-Like Receptor 2 Is Required for Inflammatory Process Development during Leishmania infantum Infection. Front Microbiol. (2017) 8:262. doi: 10.3389/fmicb.2017.00262
52. Ribeiro CV, Rocha BFB, Oliveira E, Teixeira-Carvalho A, Martins-Filho OA, Murta SMF, et al. Leishmania infantum induces high phagocytic capacity and intracellular nitric oxide production by human proinflammatory monocyte. Mem Inst Oswaldo Cruz. (2020) 115:1–10. doi: 10.1590/0074-02760190408
53. Peruhype-Magalhães V, Martins-Filho OA, Prata A, Silva LDA, Rabello A, Teixeira-Carvalho A, et al. Immune response in human visceral leishmaniasis: Analysis of the correlation between innate immunity cytokine profile and disease outcome. Scand J Immunol. (2005) 62:487–95. doi: 10.1111/j.1365-3083.2005.01686.x
54. Roy S, Mukhopadhyay D, Mukherjee S, Moulik S, Chatterji S, Brahme N, et al. An IL-10 dominant polarization of monocytes is a feature of Indian Visceral Leishmaniasis. Parasite Immunol. (2018) 40:1–9. doi: 10.1111/pim.12535
55. Ansari NA, Kumar R, Gautam S, Nylén S, Singh OP, Sundar S, et al. IL-27 and IL-21 Are Associated with T Cell IL-10 Responses in Human Visceral Leishmaniasis. J Immunol. (2011) 186:3977–85. doi: 10.4049/jimmunol.1003588
56. Wynick C, Petes C, Gee K. Interleukin-27 mediates inflammation during chronic disease. J Interferon Cytokine Res. (2014) 34:741–9. doi: 10.1089/jir.2013.0154
57. Hunter CA, Kastelein R. Interleukin-27: Balancing Protective and Pathological Immunity. Immunity. (2012) 37:960–9. doi: 10.1016/j.immuni.2012.11.003
58. Montes De Ocaid M, De F, Rivera L, Winterford C, Frameid TCM, Ngid SS, et al. IL-27 signalling regulates glycolysis in Th1 cells to limit immunopathology during infection. PLoS Pathog. (2020) 16(10):e1008994. doi: 10.1371/journal.ppat.1008994
59. Cotterell SEJ, Engwerda CR, Kaye PM. Leishmania donovani infection initiates T cell-independent chemokine responses, which are subsequently amplified in a T cell-dependent manner. Eur J Immunol. (1999) 29(1):203–14. doi: 10.1002/(SICI)1521-4141(199901)29:01<203::AID-IMMU203>3.0.CO;2-B
60. Stanley AC, Engwerda CR. Balancing immunity and pathology in visceral leishmaniasis. Immunol Cell Biol. (2007) 85:138–47. doi: 10.1038/sj.icb.7100011
61. Gupta G, Bhattacharjee S, Bhattacharyya S, Bhattacharya P, Adhikari A, Mukherjee A, et al. CXC chemokine-mediated protection against visceral leishmaniasis: involvement of the proinflammatory response. J Infect Dis. (2009) 200:1300–10. doi: 10.1086/605895
62. Eufrásio de Figueiredo WM, Heredia FF, Santos AS, da Rocha Braga R, Marciano Fonseca FR, Lúcia de Castro Rodrigues N, et al. CXCL10 treatment promotes reduction of IL-10+ regulatory T (Foxp3+ and Tr1) cells in the spleen of BALB/c mice infected by Leishmania infantum. Exp Parasitol. (2019) 207:1–7. doi: 10.1016/j.exppara.2019.107789
63. Amato VS, Franco De Andrade H, Irma M, Duarte S. Mucosal leishmaniasis: in situ characterization of the host inflammatory response, before and after treatment. Acta Trop. (2003) 85(1):39–49. doi: 10.1016/s0001-706x(02)00260-7
64. Ritter U, Körner H. Divergent expression of inflammatory dermal chemokines in cutaneous leishmaniasis. Parasite Immunol (2002) 24(6):295–301. doi: 10.1046/j.1365-3024.2002.00467.x
65. Nascimento MSL, Carregaro V, Lima-Júnior DS, Costa DL, Ryffel B, Duthie MS, et al. Interleukin 17A Acts Synergistically With Interferon γ to Promote Protection Against Leishmania infantum Infection. J Infect Dis. (2015) 211:1015–1026. doi: 10.1093/infdis/jiu531
66. Pitta MGR, Romano A, Cabantous S, Henri S, Hammad A, Kouriba B, et al. IL-17 and IL-22 are associated with protection against human kala azar caused by Leishmania donovani. J Clin Invest. (2009) 119:2379–87. doi: 10.1172/JCI38813
67. Nascimento MS, Carregaro V, Lima-Júnior DS, Costa DL, Ryffel B, Duthie MS, et al. Interleukin 17A acts synergistically with interferon γ to promote protection against Leishmania infantum infection. J Infect Dis. (2015) 211(6):1015–26. doi: 10.1093/infdis/jiu531
68. Ghosh K, Sharma G, Saha A, Kar S, Das PK, Ukil A. Successful therapy of visceral leishmaniasis with curdlan involves T-helper 17 cytokines. J Infect Dis. (2013) 207:1016–25. doi: 10.1093/infdis/jis771
69. Sutton CE, Lalor SJ, Sweeney CM, Brereton CF, Lavelle EC, Mills KHG. Interleukin-1 and IL-23 Induce Innate IL-17 Production from γδ T Cells, Amplifying Th17 Responses and Autoimmunity. Immunity. (2009) 31:331–41. doi: 10.1016/j.immuni.2009.08.001
70. Guggino G, Di Liberto D, Lo PM, Saieva L, Alessandro R, Dieli F, et al. IL-17 polarization of MAIT cells is derived from the activation of two different pathways. Eur J Immunol. (2017) 47:2002–3. doi: 10.1002/eji.201747140
71. Willing A, Jäger J, Reinhardt S, Kursawe N, Friese MA. Production of IL-17 by MAIT Cells Is Increased in Multiple Sclerosis and Is Associated with IL-7 Receptor Expression. J Immunol. (2018) 200:974–82. doi: 10.4049/jimmunol.1701213
Keywords: MR1 blockade, L. infantum, phagocytes, soluble mediators, integrative circuits, cytokines, nitric oxide, internalization
Citation: Borges-Fernandes LO, de Lima Moreira M, Pereira VHS, Pascoal-Xavier MA, Lopes Ribeiro Á, Costa-Rocha IAd, Lopes LR, Moreira GTC, Araújo MSdS, Teixeira-Carvalho A, Brito-de-Sousa JP, de Carvalho AL, Mourão MVA, Campos FA, Borges M, Carneiro M, Tsuji M, Martins-Filho OA, Coelho-dos-Reis JGA and Peruhype-Magalhães V (2024) MR1 blockade drives differential impact on integrative signatures based on circuits of circulating immune cells and soluble mediators in visceral leishmaniasis. Front. Immunol. 15:1373498. doi: 10.3389/fimmu.2024.1373498
Received: 19 January 2024; Accepted: 15 July 2024;
Published: 13 August 2024.
Edited by:
Neetu Singh, University of Texas MD Anderson Cancer Center, United StatesReviewed by:
Camila Farias Amorim, University of Pennsylvania, United StatesCopyright © 2024 Borges-Fernandes, de Lima Moreira, Pereira, Pascoal-Xavier, Lopes Ribeiro, Costa-Rocha, Lopes, Moreira, Araújo, Teixeira-Carvalho, Brito-de-Sousa, de Carvalho, Mourão, Campos, Borges, Carneiro, Tsuji, Martins-Filho, Coelho-dos-Reis and Peruhype-Magalhães. This is an open-access article distributed under the terms of the Creative Commons Attribution License (CC BY). The use, distribution or reproduction in other forums is permitted, provided the original author(s) and the copyright owner(s) are credited and that the original publication in this journal is cited, in accordance with accepted academic practice. No use, distribution or reproduction is permitted which does not comply with these terms.
*Correspondence: Vanessa Peruhype-Magalhães, dmFuZXNzYS5wYXNjb2FsQGZpb2NydXouYnI=
†These authors have contributed equally to this work
‡These authors share senior authorship
Disclaimer: All claims expressed in this article are solely those of the authors and do not necessarily represent those of their affiliated organizations, or those of the publisher, the editors and the reviewers. Any product that may be evaluated in this article or claim that may be made by its manufacturer is not guaranteed or endorsed by the publisher.
Research integrity at Frontiers
Learn more about the work of our research integrity team to safeguard the quality of each article we publish.