- 1Guangdong Cardiovascular Institute, Guangdong Provincial People’s Hospital, Guangdong Academy of Medical Sciences, Guangzhou, China
- 2Department of Gastrointestinal Surgery, Department of General Surgery, Guangdong Provincial People’s Hospital (Guangdong Academy of Medical Sciences), Southern Medical University, Guangzhou, China
- 3Department of Gastrointestinal Surgery, First Affiliated Hospital of Jinan University, Guangzhou, Guangdong, China
- 4Digestive Diseases Center, The Seventh Affiliated Hospital of Sun Yat-sen University, Shenzhen, Guangdong, China
Chondroitin sulfate synthase 3 (CHSY3) is an important enzyme that regulates glycosylation, but its role in tumors has not been determined. Here, we showed that high CHSY3 expression promotes proliferation in gastric cancer (GC) cells and is associated with poor prognosis in GC patients. We analyzed the immunohistochemistry data of 150 gastric cancer patients to determine the clinicopathological and survival significance of CHSY3. Immunofluorescence was used to detect the colocalization of CHSY3 with infiltrating immune cells. Additionally, CHSY3 was predominantly found in tumor tissues and showed higher abundance compared to matched adjacent tissues. High CHSY3 expression was associated with more advanced tumor stage, higher recurrence risk and worse survival. Immunohistochemistry and bioinformatic analysis revealed that CHSY3 expression was significantly positively correlated with tumor-associated macrophage (TAM) infiltration. Moreover, after knocking down CHSY3, the proliferation of cells was decreased, and the migration ability was reduced, as shown by scratch, monoclonal and transwell assays. In conclusion, this study revealed that CHSY3 has a tumor-promoting effect on GC, suggesting a novel therapeutic strategy against this disease.
1 Introduction
Gastric cancer ranks as the fifth most prevalent cancer and the third leading cause of cancer-related mortality worldwide (1). Surgical resection with adjuvant chemotherapy is the main treatment for gastric cancer, but the prognosis remains unfavorable due to chemotherapy insensitivity and the emergence of chemoresistance, frequently resulting in postoperative recurrence (2). Notably, modifications in the tumor immune microenvironment hold predictive value for patient prognosis (3, 4). Hence, accurately forecasting patient responses to therapy emerges as a crucial challenge.
One of the common posttranslational modifications of proteins is glycosylation (5, 6), a process involving the transfer of sugar chains to form glycosidic bonds between proteins and specific amino acid residues, facilitated by glycosyltransferases. These modifications are closely linked to the development of malignant tumors and the prognosis of cancer patients, achieved through the alteration of sugar chains (7). In addition, aberrant alterations in glycosylation on the surface of tumor cells lead to tumor immune evasion, thereby providing new immune checkpoints (ICs) for immunotherapy (8, 9).
In this study, we revealed the function and expression of CHSY3 in gastric cancer. High CHSY3 expression was associated with poor patient prognosis, and the experiments showed that CHSY3 expression regulated the proliferation and migration of gastric cancer cells, and increased the infiltration of tumor-associated macrophages. In conclusion, these data suggest that CHSY3 can promote gastric cancer development and underscore its potential relevance as a prognostic biomarker for gastric cancer treatment.
2 Methods
2.1 LinkedOmics Database analysis
The LinkedOmics Database is a public portal that includes multiomics data from all 32 TCGA cancer types and 10 Clinical Proteomics Tumor Analysis Consortium (CPTAC) cancer cohorts, this portal provides biologists and clinicians with a unique platform for accessing, analyzing, and comparing multiomics data within and across tumor types. The genes related to CHSY3 were screened from the TCGA stomach adenocarcinoma (STAD) cohort by the LinkFinder module in the database, and the Pearson correlation coefficient was used to test the results; the results are shown as volcano plots and heatmaps. Functional module analysis of Gene Ontology biological process (GO_BP) and Kyoto Encyclopedia of Genes and Genomes (KEGG) pathway enrichment analysis (GSEA) of the LinkInterpreter module.
2.2 K−M plotter (gastric cancer)
KM plotter (http://kmplot.com/analysis/) was used to evaluate the survival prognosis associated with related genes by mapping the survival curve using 1,065 GC samples with an average follow-up of 33 months. The prognostic significance of CHSY3 in GC, as indicated by overall survival (OS), first progression (FP), and postprogression survival (PPS), was investigated using this database. The hazard ratio (HR) with 95% confidence intervals (CIs) was also estimated, as was the log-rank p value. p < 0.05 indicated statistical significance.
2.3 TIMER database analysis
TIMER (https://cistrome.shinyapps.io/timer/) and TIMER2.0 (https://timer.cistrome.org/) is a web-based interactive platform for the systematic analysis of immune infiltration in various malignancies. We investigated the expression of CHSY3 in various malignancies and the relationship between CHSY3 and TIL expression through gene modules. Furthermore, the link between CHSY3 expression and gene signatures of TILs, including CD8+/CD4+ T cells, tumor-associated macrophages (TAMs), M1 macrophages, M2 macrophages, T cells, and related subtypes has been analyzed. An expression scatter plot between Spearman’s correlation and estimated statistical significance for a pair of genes for GC was constructed using the correlation module. The levels of gene expression are represented as log2 RSEM.
2.4 Immunohistochemistry
This study involved the analysis of 150 paraffin-embedded gastric cancer (GC) specimens obtained from the Shanghai Outdo Biotech Company. The formalin-fixed and paraffin-embedded sections were deparaffinized with xylene and then rehydrated. Antigen retrieval was performed with Tris/EDTA buffer (pH 9.0) for 20 min at 95°C in paraffin-embedded tissue sections. The slides were incubated with antibodies against CD68 (1:200; Cell Signaling Technology, #97778) and CHSY3 (1:100; Novus, NBP1-85626) overnight at 4°C. The following steps were performed in accordance with the protocols provided by the manufacturer of the DAB Kit (DAB-0031, Maxim, China). Multiplex immunofluorescence was performed following the instructions of the PANOVUE kit (10234100050). Images of the tissues were observed and captured using a KFBIO Digital Slide Scanner.
2.5 Analysis of the DNA methylation status of the CpG islands of the CHSY3 gene
The DNA methylation status at the CpG sites of the CHSY3 gene was analyzed in the STAD-TCGA datasets using the MethSurv database (https://biit.cs.ut.ee/methsurv/). Furthermore, the prognostic value of the CpG methylation status of CHSY3 was evaluated in GC samples. Moreover, the association between the CpG methylation status of CHSY3 and overall survival (OS) in patients with GC was also evaluated. Moreover, the genetic alterations of CHSY3 from TCGA cancers were explored via the cBioPortal (https://www.cbioportal.org/) and are displayed as alteration frequencies.
2.6 Western blot assay
Cells and tissue samples were collected for protein extraction. A BCA protein assay kit (Thermo Scientific™, #23225, USA) was used to evaluate the protein concentration. The proteins were separated via sodium dodecyl sulfate–polyacrylamide gel electrophoresis. The polyvinylidene fluoride membrane containing proteins was blocked with 5% milk. Then, specific primary antibodies (CHSY3, Affinity, 1:1000; GAPDH, Proteintech, 1:10000) were applied to the membrane at 4°C overnight. After the membranes were incubated with secondary antibodies, an enhanced chemiluminescence (ECL) Western blotting Substrate (180-5001, Tanon, China) was used to detect the proteins.
2.7 Wound-healing and colony formation assays
AGS cells were cultured in plates for 24 hours. A pipette tip was used to draw the surface of the cell layer. A microscope was used to capture images at 0 h, 12 h and 24 h after injury. The distance to the injury area at 24 h was measured. To perform the colony formation assay, 1000–1500 cells were seeded into six-well plates and cultured for approximately 14 days. Cell colonies were fixed with 4% formaldehyde and stained with 0.1% crystal violet for 10 min.
2.8 Transwell assay
The cells were mixed with serum-free media and injected into the upper layer. The outside of the transwell chamber was filled with complete medium. After the cells were cultured under suitable conditions for 48 h, the Transwell chambers were removed for fixation and staining with 4% paraformaldehyde and 0.1% crystal violet (Solarbio, China). The cells on the bottom of the chambers were counted.
2.9 Immunofluorescence analyses
According to standard protocols, cells were fixed and incubated with primary antibodies (at a 1:100 dilution), fluorescent dye-conjugated secondary antibodies, and DAPI.
2.10 Statistical analysis
SPSS 22.0 (IBM, USA) was used for statistical analyses. The data were analyzed with Student’s t test or one-way analysis. All the results are displayed as the mean ± SD. P <0.05 was regarded as statistically significant.
3 Results
3.1 The expression of CHSY3 across cancers
To ascertain differences in CHSY3 expression between tumor and normal tissues, data from the TCGA database were used. The results showed that CHSY3 was differentially expressed in most tumors, some with high expression and some with low expression (Figure 1A). Additionally, the expression of CHSY3 in 23 kinds of tumors with paired samples in the TCGA cohort was also analyzed (Figure 1B). In summary, CHSY3 is highly expressed in most cancers.
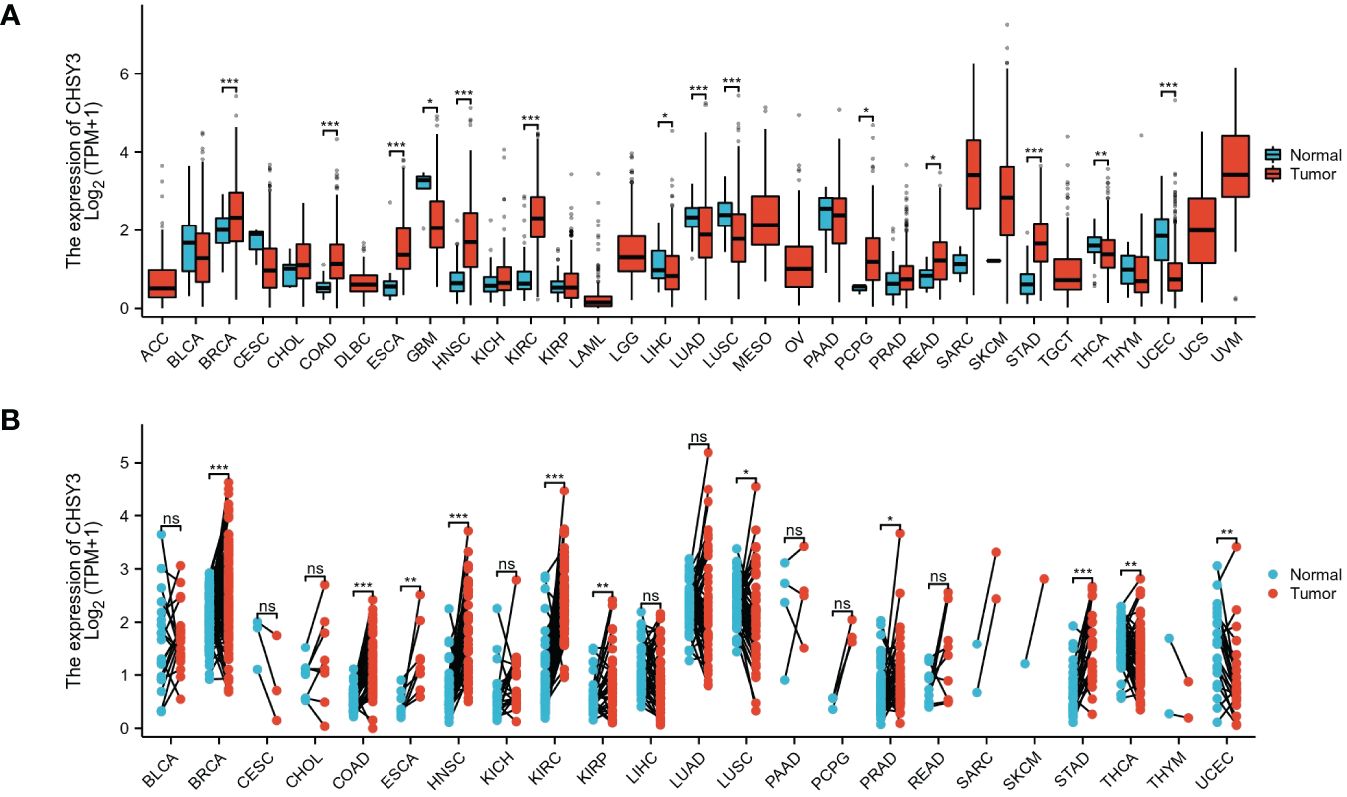
Figure 1 The pancancer mRNA expression of CHSY3. (A) The mRNA expression of CHSY3 in 33 tumors in the TCGA database. (B) Expression of CHSY3 in paired samples of 23 tumors in the TCGA database. ACC, adrenocortical carcinoma; BLCA, bladder urothelial carcinoma; BRCA, breast invasive carcinoma; CESC, cervical and endocervical cancers; CHOL, cholangiocarcinoma; COAD, colon adenocarcinoma; DLBC, lymphoid neoplasm diffuse large B-cell lymphoma; ESCA, esophageal carcinoma; GBM, glioblastoma multiforme; HNSC, head and neck squamous cell carcinoma; KICH, kidney chromophobe; KIRC, kidney renal clear cell carcinoma; KIRP, kidney renal papillary cell carcinoma; LAML, acute myeloid leukemia; LGG, brain lower grade glioma; LIHC, liver hepatocellular carcinoma; LUAD, lung adenocarcinoma; LUSC, lung squamous cell carcinoma; MESO, mesothelioma; OV, ovarian serous cystadenocarcinoma; PAAD, pancreatic adenocarcinoma; PCPG, pheochromocytoma and paraganglioma; PRAD, prostate adenocarcinoma; READ, rectum adenocarcinoma; SARC, sarcoma; SKCM, skin cutaneous melanoma; STAD, stomach adenocarcinoma; STES, stomach and esophageal carcinoma; TGCT, testicular germ cell tumor; THCA, thyroid carcinoma; THYM, thymoma; UCEC, uterine corpus endometrial carcinoma; UCS, uterine carcinosarcoma; UVM, uveal melanoma. (ns, p > 0.05; *p < 0.05; **p < 0.01; ***p < 0.001).
3.2 The expression of CHSY3 in gastric cancer
As shown in Figure 2A, the CHSY3 mRNA level was substantially greater in the GC samples (375 patients) than in the healthy samples (32 patients) (p < 0.05) from the TCGA. As shown in Figure 2B, the TCGA database showed that CHSY3 expression was greater in patients than in matched normal tissues (n = 27). Moreover, the area under the curve (AUC) was 0.890 (95% CI=0.835–0.945) for CHSY3 in GC (Figure 2C). The above data indicated that CHSY3 expression was strongly increased in GC tissues and may be a potential diagnostic biomarker for GC. Therefore, additional investigations are needed to determine whether CHSY3 expression is associated with tumor outcome. Notably, CHSY3 expression was associated with a favorable outcome in GC patients, and this study showed that increased CHSY3 expression was linked to a worse prognosis in the TCGA GC cohort (OS: HR = 1.58, p = 0.007; DSS: HR=1.54, p=0.046) (Figures 2D, E). Moreover, the same results were observed in the 242100-at cohort. High CHSY3 expression correlated with poorer prognosis in GC patients (hazard ratio [HR]=1.66, 95% confidence interval [CI]=1.33 to 2.06, p=0.0000049) (Figure 2F). Furthermore, western blotting and qRT-PCR were performed to assess the CHSY3 expression between 7 pairs of GC cases and their corresponding adjacent normal tissues. The results unveiled a notably heightened expression of both CHSY3 mRNA transcripts and proteins in the GC tissues relative to their adjacent healthy tissues (Figures 2G, H).
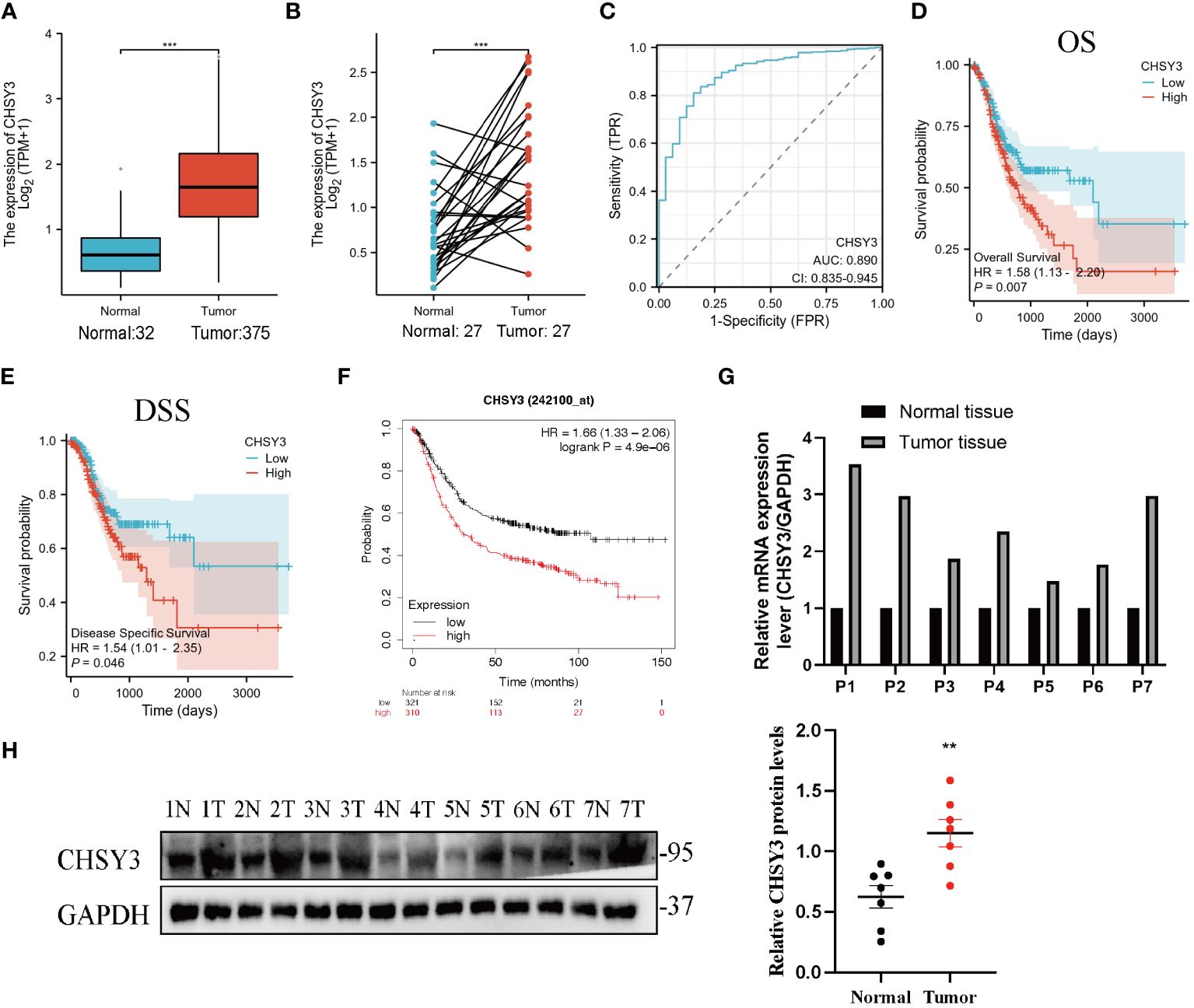
Figure 2 The expression profile of CHSY3 in gastric cancer. (A) Increased CHSY3 in gastric cancer tissues compared with normal tissues in the TCGA database. (B) Increased CHSY3 expression in gastric cancer tissue compared with matched normal tissue from the TCGA database (n = 27). (C) The ROC curve analysis of CHSY3 in GC patients. (D, E) High CHSY3 expression was correlated with poor OS and DSS in GC patients according to data from the TCGA database. (F) High CHSY3 expression was correlated with poor OS in GC patients according to the Kaplan–Meier plotter database. * P < 0.05, **P < 0.01, ***P < 0.001. (G) The mRNA level of CHSY3 in 7 pairs of GC cases and their corresponding adjacent normal tissues. (H) Western blotting was performed to detect the protein level of CHSY3 in 7 pairs of GC cases and their corresponding adjacent normal tissues.
3.3 CHSY3 co-expressed genes in gastric cancer
The above results revealed that CHSY3 expression was significantly associated with the prognosis in gastric cancer patients. Next, we explored CHSY3 co-expression networks using the LinkedOmics database to verify the potential function of CHSY3 in tumor tissue (Figure 3A). A total of 10689 co-expressed genes were significantly correlated with CHSY3 in GC (FDR < 0.05, P < 0.05, and |cor.| ≥ 0.3). Among the 10689 genes, 6290 were positively correlated with CHSY3 expression, whereas 4399 were negatively correlated with CHSY3 expression. Figures 3B, C shows heatmaps of the top 50 genes positively and negatively associated with CHSY3.
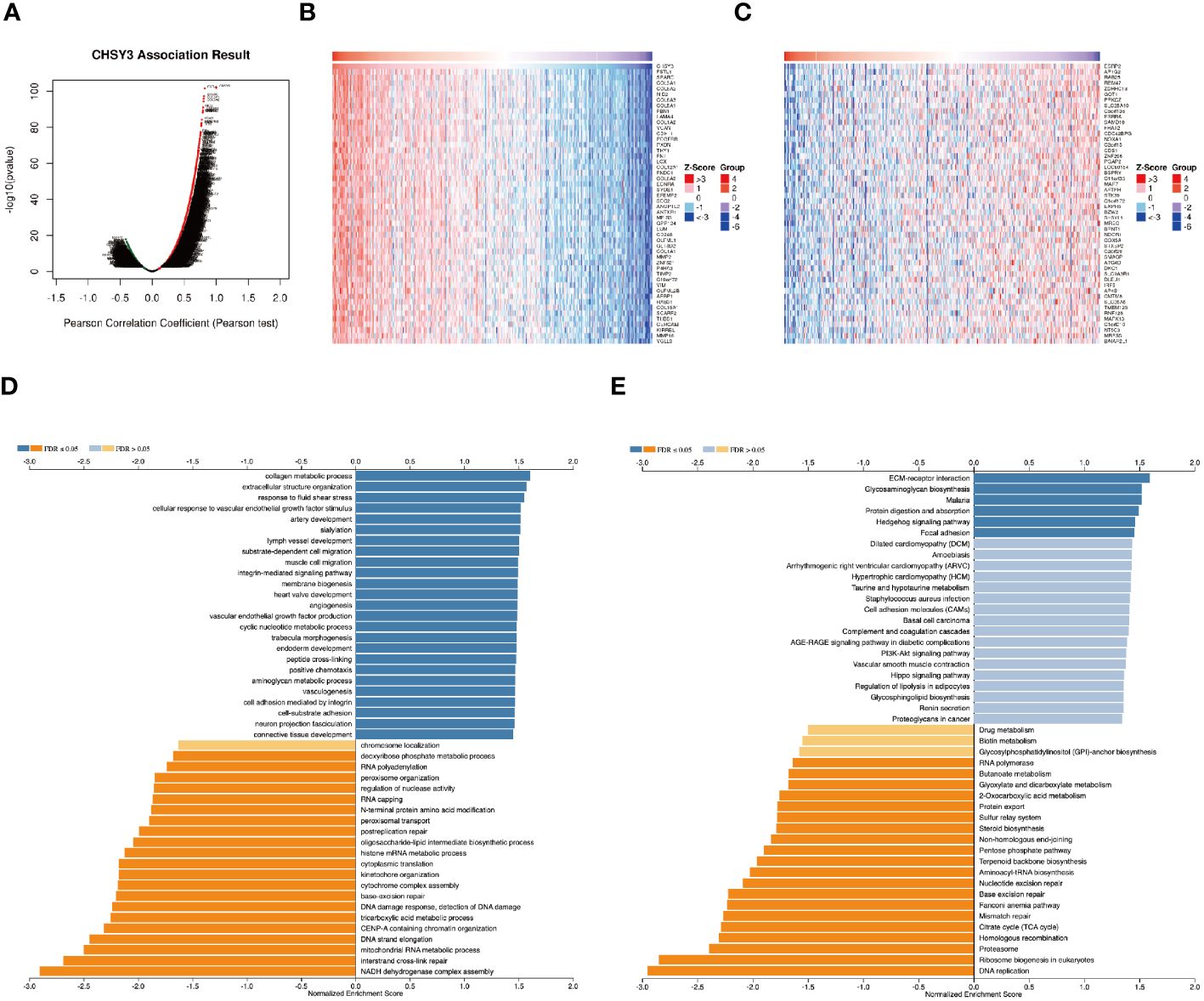
Figure 3 Genes co-expressed with CHSY3 in STAD were analyzed via the LinkedOmics database. (A) All genes significantly associated with CHSY3 were identified by Pearson correlation in the STAD cohort. (B, C) The top 50 genes positively and negatively related to CHSY3 in STAD are shown by heatmaps. Red represents positively linked genes, and blue represents negatively linked genes. (D, E) GO annotations and KEGG pathways associated with CHSY3 in the STAD cohort.
GO term annotation revealed that the genes co-expressed with CHSY3 were involved mainly in collagen metabolic processes, extracellular structure organization, response to fluid shear stress, cellular response to vascular endothelial growth factor stimulus, etc. (Figure 3D). KEGG pathway analysis revealed enrichment of ECM-rector interaction, glycosaminoglycan biosynthesis, malaria, protein digestion and absorption, and the Hedgehog signaling pathway (Figure 3E). These results revealed the wide influence of the CHSY3 expression network on the prognosis of patients with STAD.
3.4 Immune infiltration analysis
The immune microenvironment plays a crucial role in the occurrence and development of tumors. The relationship between CHSY3 and the immune microenvironment in GC was studied via the TCGA database, and the results of the R package “GSVA” demonstrated that CHSY3 was positively correlated with different immune cells in these cancers (Figure 4A). Furthermore, the relationship between the high/low expression of CHSY3 and immune cell infiltration was analyzed, and the results showed that the up-regulation of CHSY3 expression increased the infiltration level of various immune cells, especially tumor-associated macrophages (Figure 4B). By immunofluorescence, we found that CHSY3 (red) and CD68 (a marker of macrophages; green) were correlated, which was consistent with the findings of previous studies (Figure 4C). Heatmaps showing the correlations between CHSY3 expression and NK cells (Figure 4D) and macrophages (Figure 4E) are shown.
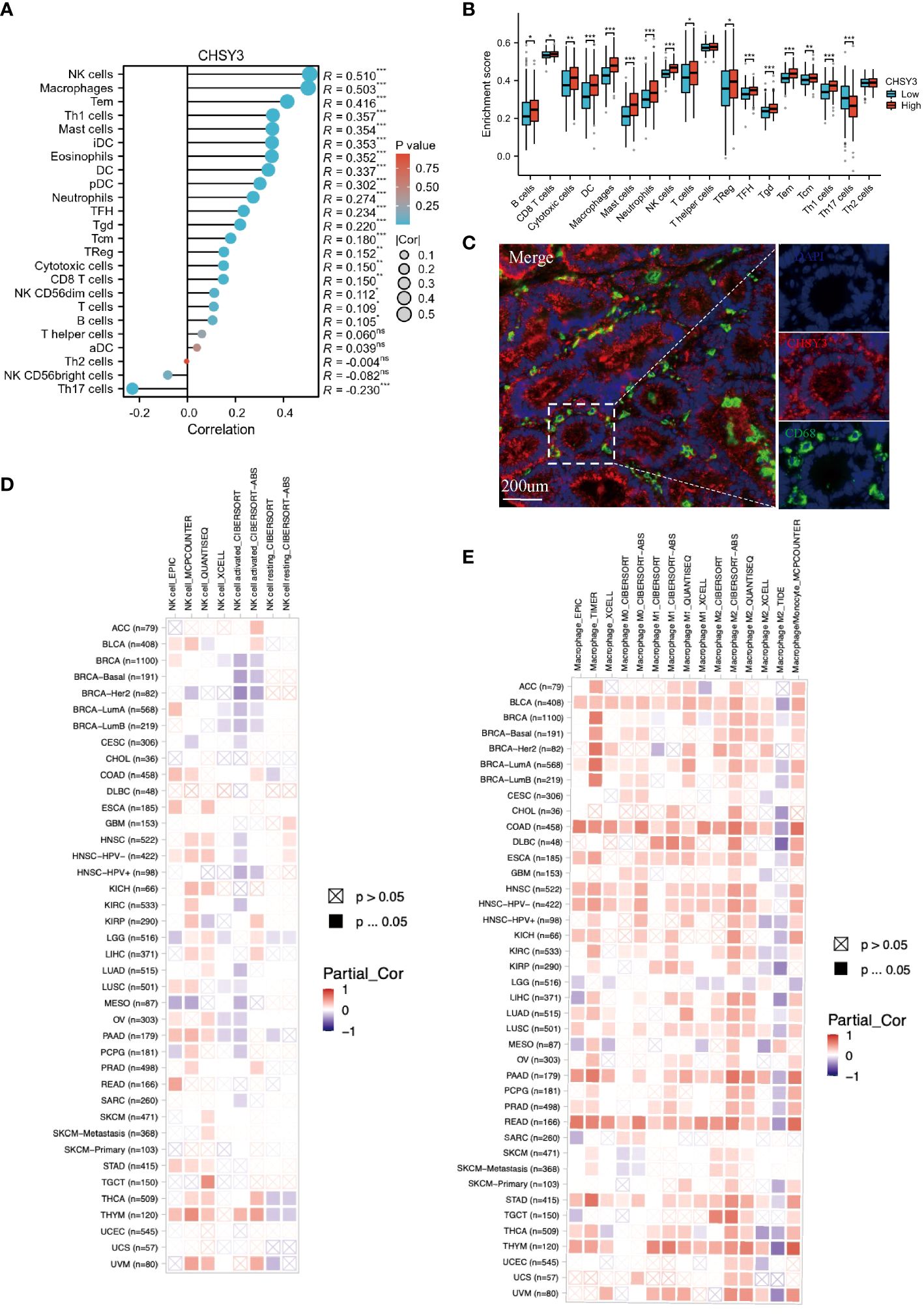
Figure 4 Correlations between CHSY3 expression and immune cell infiltration. (A) Relationships between CHSY3 expression and the infiltration levels of NK cells, macrophages, B cells, CD4+ T cells, CB8+ T cells, neutrophils, and dendritic cells in human cancers. (B) Relationship between the high/low expression of CHSY3 and immune cells infiltration. (C) mIHC staining demonstrating the colocalization of CHSY3 (red) with macrophages (green). (D, E) Heatmaps of the correlations between CHSY3 expression and NK cells and macrophages in the TIMER2 database. *p < 0.05, **p < 0.01, ***p < 0.001.
3.5 Correlation of CHSY3 expression with immune infiltration level and cumulative survival in patients with GC
As mentioned above, several tumor-infiltrating lymphocytes are independent predictors of cancer survival; thus, we investigated the association between CHSY3 expression and immune infiltration levels in GC patients. We selected CHSY3 expression levels that were positively correlated with tumor purity. The results showed that the level of CHSY3 expression was negatively correlated with the infiltration level of B cells (r = −0.071, p = 0.172) and positively correlated with the infiltration level of CD8+ T cells (r = 0.097, p = 0.062), CD4+ T cells (r = 0.302, p = 0.000), macrophages (r = 0.533, p = 0.000), neutrophils (r = 0.224, p = 0.000) and DCs (r = 0.347, p = 0.000) in GC (Figure 5A). Moreover, our findings revealed that B cells (p = 0.786), CD8+ T cells (p = 0.554), CD4+ T cells (p = 0.23), macrophages (p = 0.004), neutrophils (p = 0.436) and DCs (p = 0.12), but only macrophages, were related to the cumulative survival rate of patients with GC over time (Figure 5B). These data strongly indicate that CHSY3 is associated with macrophages infiltration in GC. CHSY3 was significantly associated with the majority of the macrophage marker sets in STAD. Specifically, this study revealed that the TAM markers chemokine ligand (CCL)-2, CD68 and interleukin 10 (IL10) are strongly correlated with CHSY3 in STAD, as are interferon regulatory factor 5 (IRF5) and prostaglandin-endoperoxide synthase 2 (PTGS2) in the M1 phenotype and with CD163, V-Set and immunoglobulin domain containing 4 (VSIG4), and the Membrane Spanning 4-Domains A4A (MS4A4A) in the M2 phenotype (p < 0.001; Figure 5C; Supplementary Table 1).
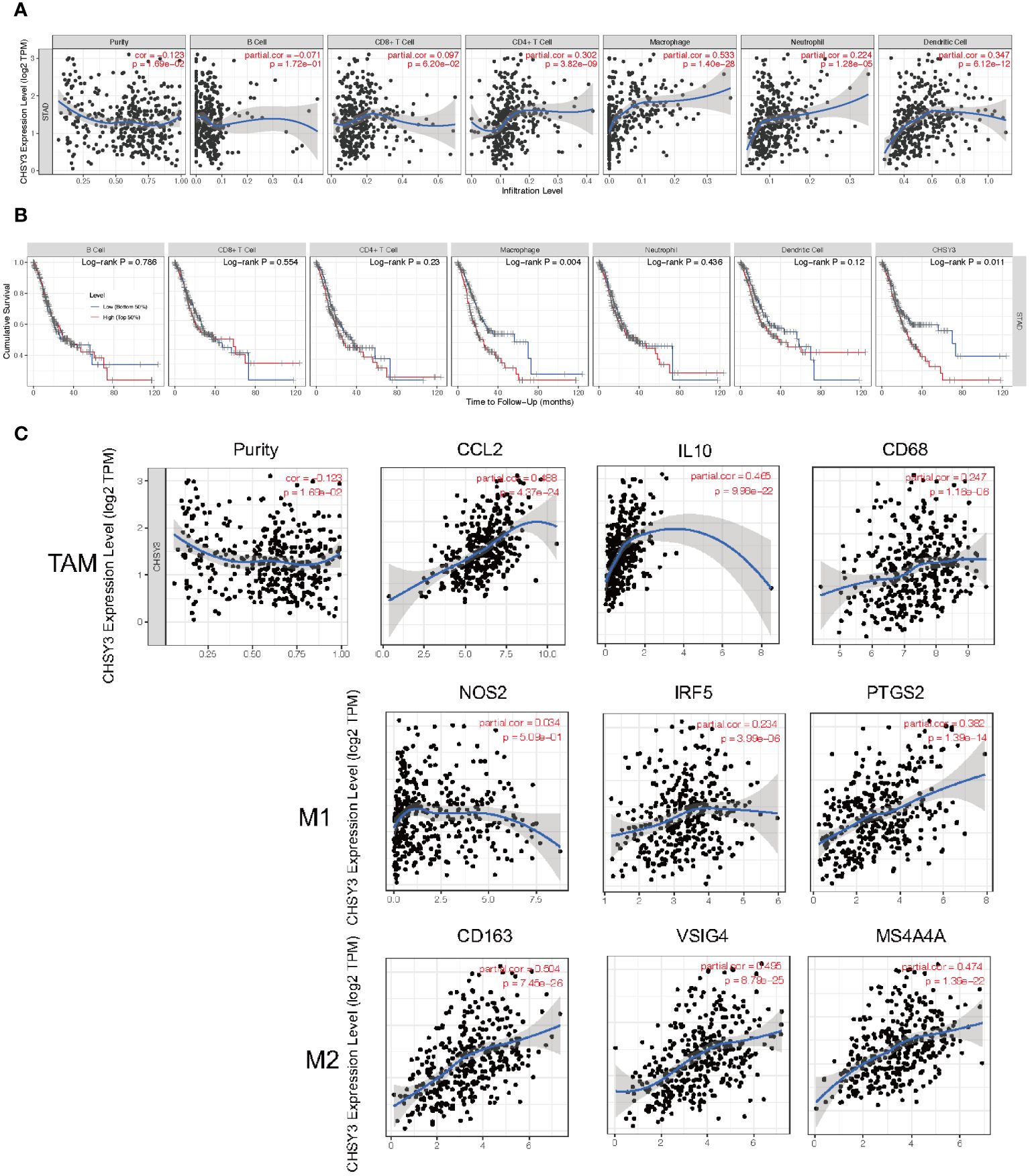
Figure 5 Correlation of CHSY3 expression with immune infiltration level in STAD. (A) The CHSY3 expression level was significantly correlated with the infiltration of B cells (r = −0.071, p = 0.172), CD8+ T cells (r = 0.097, p = 0.062), CD4+ T cells (r =0.302, p = 0.000), macrophages (r = 0.533, p = 0.000), neutrophils (r = 0.224, p = 0.000) and DCs (r = 0.347, p = 0.000) in patients with STAD. (B) Cumulative survival was related to B cells (p = 0.786), CD8+ T cells (p = 0.554), CD4+ T cells (p = 0.23), macrophages (p = 0.004), neutrophils (p = 0.436) and DCs (p = 0.011) in patients with STAD. (C) Scatterplots of the correlations between CHSY3 expression and the gene markers of TAMs and M1 and M2 macrophages in STAD.
3.6 CHSY3 is highly expressed in and influences the proliferation and migration of GC cells
To confirm the function of CHSY3, we designed three shRNAs that targeted different sites of CHSY3. Western blot analysis indicated that the three shRNAs had excellent efficacy in knocking down CHSY3 expression (Figure 6A). Moreover, wound healing and Transwell assays revealed that CHSY3 knockdown inhibited the migration of GC cells (Figures 6B, C). Colony formation and Ki67 staining assays demonstrated that knockdown of CHSY3 significantly decreased the proliferation of GC cells (Figures 6D, E).
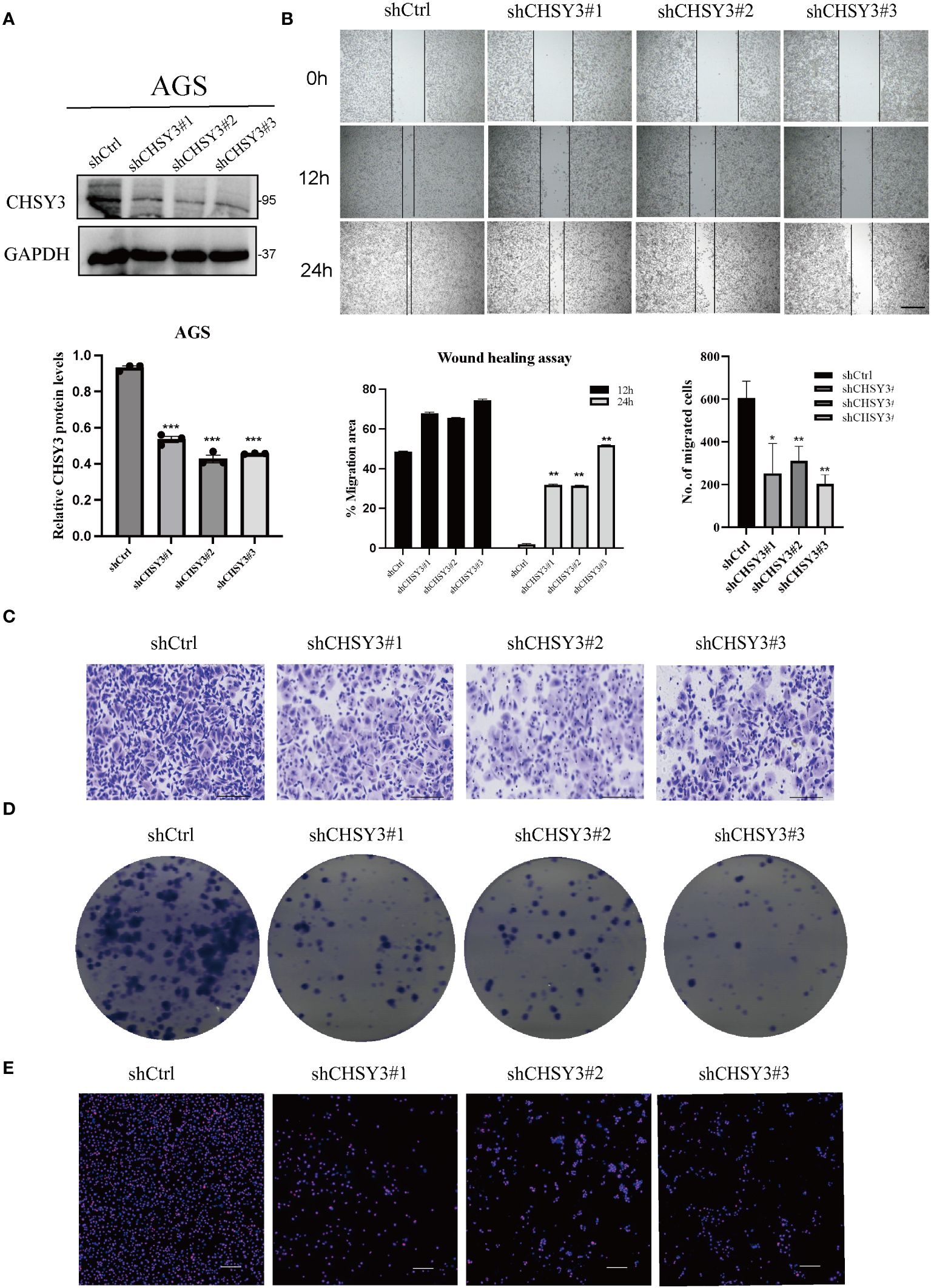
Figure 6 CHSY3 promotes the malignant properties of GC progression (A) WB was used to verify the efficiency of the shRNAs. (B) Wound healing assays indicated that CHSY3 knockdown could restrain the migration of GC cells. (C) Transwell assays showed that CHSY3 knockdown inhibited the migration of GC cells. (D) Colony formation assays were used to evaluate the effect of CHSY3 knockdown on the growth of AGS cells. (E) IF analysis of the relative expression of Ki-67 in CHSY3-knockdown cells. *p < 0.05, **p < 0.01, ***p < 0.001.
To assess the clinical significance of CHSY3 expression, we examined the protein expression of CHSY3 in GC using immunohistochemistry (IHC) and investigated the relationship between CHSY3 expression and clinicopathological characteristics in GC patients. We found that the expression of CHSY3 was associated with pathological TNM stage, lymph node metastasis, and LVI in gastric cancer patients (Figure 7A; Table 1). Moreover, we performed survival analysis and showed that increased CHSY3 expression was linked to worse OS and DFS in GC patients (Figures 7B, C; Tables 2, 3), which revealed that higher CHSY3 expression was significantly associated with worse prognosis in individuals diagnosed with GC.
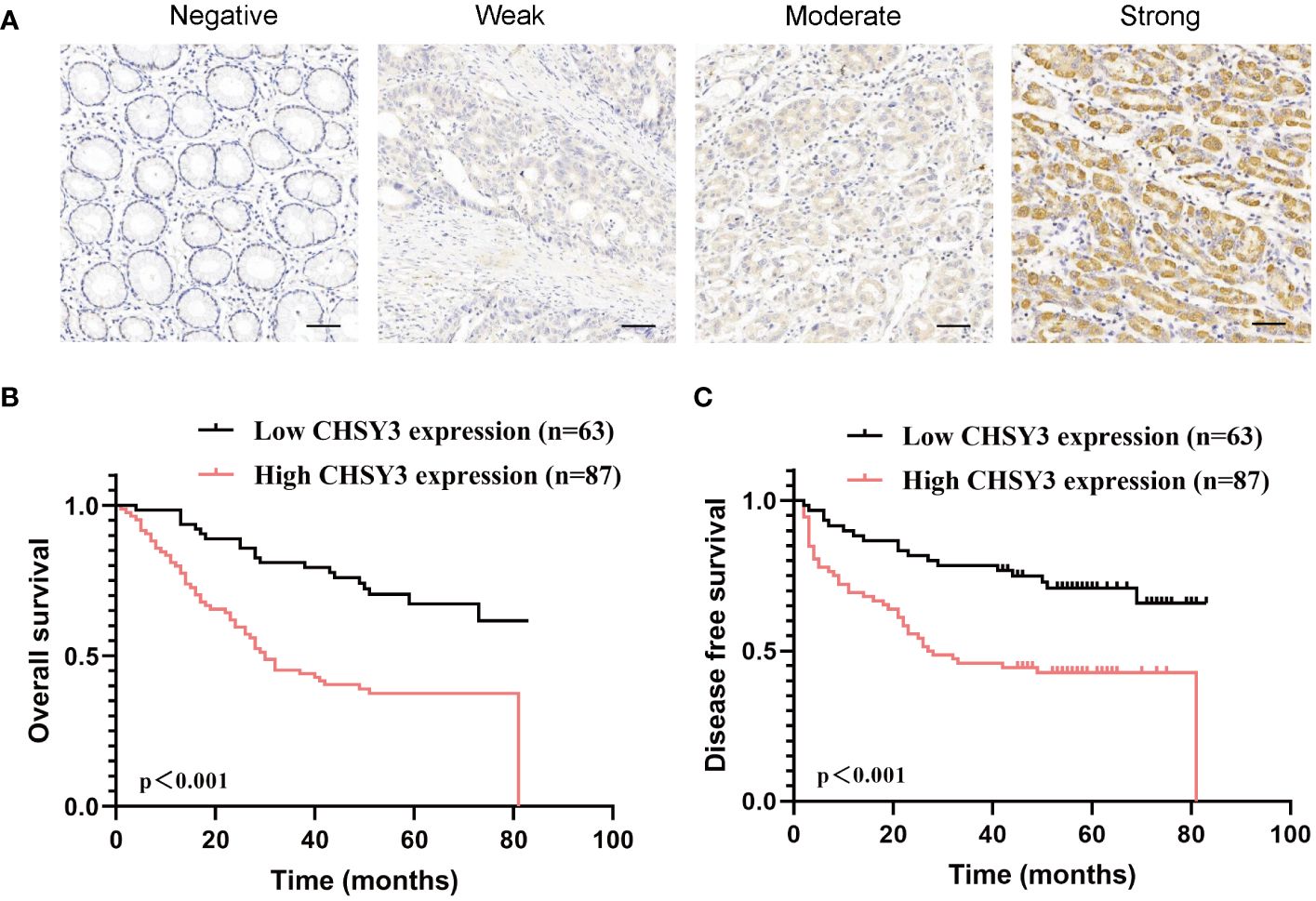
Figure 7 The expression and prognostic value of CHSY3 in gastric cancer (A) Gastric cancer tissue was quantified by scoring the staining intensity, which included negative (–) and weak (+) staining and moderate (++) and strong (+ + +) staining. Scale bar = 100 mm. (B-C) High CHSY3 expression was correlated with poor OS and DFS in GC patients.
3.7 DNA methylation analysis of the CHSY3 gene in GC
DNA methylation is an epigenetic alteration that is related to tumorigenesis and progression (10, 11). DNA methyltransferases that affect CpG island methylation are transcription factors that can suppress or promote cell growth, and the process is reversible (12, 13). A heatmap of DNA methylation clustering of the expression levels of the CHSY3 gene in GC was constructed (Figure 8A). Furthermore, the DNA methylation pattern of CHSY3, which has significant prognostic value, was also confirmed, as was that of cg06610705 (Figure 8B). Furthermore, the highest alteration frequency of CHSY3 (6%) was observed in uterine corpus endometrial carcinoma patients with “mutation”. In gastric cancer, and most patients had “mutation” or “deep deletion” as the primary alterations (Figure 8C).
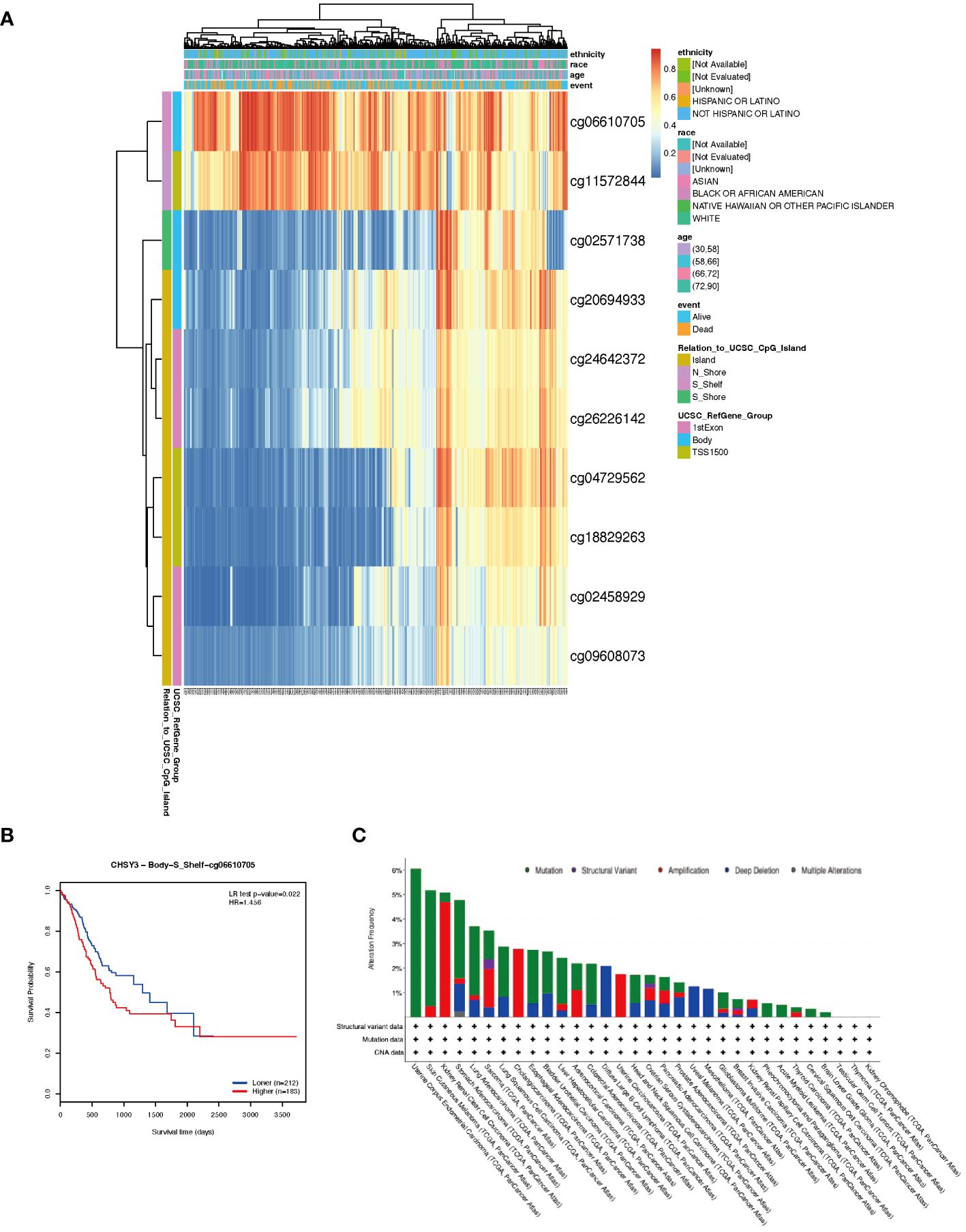
Figure 8 DNA methylation analysis and mutation features of CHSY3 in cancers. (A) DNA methylation of CHSY3 in STAD samples from the TCGA. (B) Prognostic value of a single CpG in the CHSY3 gene in STAD. The threshold of significance was an LR test p value <0.05. cg06610705 of CHSY3 indicates a significant level of DNA methylation in STAD. (C) The alteration frequency and mutation type of CHSY3 (https://www.cbioportal.org/).
4 Discussion
Immunotherapy stands at the forefront of cancer treatment, demonstrating remarkable success across various cancer types (14). Nonetheless, its efficacy varies among patients (15), potentially due to the intricate immunological microenvironment within tumors (16). Gastric cancer, characterized by a high global mortality rate, typically relies on surgical resection as the primary treatment modality. However, the outcomes of postoperative adjuvant chemotherapy and immunotherapy for advanced gastric cancer remain unsatisfactory. Notably, aberrant glycosylation plays a pivotal role in tumorigenesis and disease progression (1). The CHSY family of glycosyltransferases emerges as a crucial regulator of glycosylation. Specifically, upregulation of CHSY1 has been implicated in promoting the proliferation and metastasis of various cancers including gastric cancer (17), hepatocellular carcinoma (18), glioblastoma (19) and other tumors (20, 21). Recent findings underscore the role of CHSY1 in depleting CD8+ T cells through succinate metabolism activation and the PI3K/AKT/HIF1A pathway, facilitating liver metastasis in intestinal cancer (22). The CHSY2 and CHSY3 genes are identical. High CHSY2 expression has been associated with the occurrence of choriocarcinoma and its metastasis (23). However, only recent reports have shown that CHSY3 expression is associated with the development and metastasis of gastric cancer (24, 25). Like in their study, in which we evaluated the expression of CHSY3 via TIMER, TCGA and other databases, we found that CHSY3 was abnormally highly expressed in a variety of cancers, and compared with paraneoplastic tissues, CHSY3 was more highly expressed in tumors. We found that CHSY3 could promote the progression and migration of gastric cancer cells through cellular experiments, but we found that the expression of CHSY3 was associated with the infiltration of tumor-associated macrophages. We analyzed and verified gastric cancer tissue samples and found that high CHSY3 expression was associated with poorer prognosis and could be an independent risk factor for gastric cancer development.
Chondroitin sulfate, as a core component of the glycosaminoglycan (GAGs) family, plays a crucial role in the occurrence and development of tumors (26). Glycosaminoglycans exert key regulatory functions in the malignant transformation and metastasis of tumors, among which the biosynthesis of chondroitin sulfate is an indispensable part of this process. Specifically, the chondroitin sulfate synthase is essential for the generation of chondroitin sulfate molecules, with chondroitin sulfate synthase-2 being a necessary enzyme to ensure the effective extension of the chondroitin sulfate chain (27, 28). It is noteworthy that chondroitin sulfate has been confirmed as a ligand for the receptor for advanced glycation end products (RAGE) (29), and the role of RAGE in tumor biology is increasingly prominent, particularly in the field of gastric cancer research. The activation status of RAGE is closely associated with its mediation of tumor cell proliferation, enhanced invasive capacity, increased metastatic potential, and poor prognosis in gastric cancer patients (30). These studies further confirm that CHSY3 plays an important role in the development of malignant phenotypes in gastric cancer. Therefore, a thorough exploration of the complex interaction mechanisms between glycosaminoglycans, especially chondroitin sulfate synthase, and the RAGE signaling pathway holds the promise of revealing new anticancer therapeutic targets and strategies, thereby effectively inhibiting the progression of various tumors, including gastric cancer. This not only enriches our understanding of the regulatory mechanisms of the tumor microenvironment but also provides a theoretical basis for the development of targeted anticancer therapies.
In this paper, the role of CHSY3 in gastric cancer was shown to be related to the malignant phenotype of gastric cancer through pathological results and cellular experiments, and we also found that the expression of CHSY3 in gastric cancer was related mainly to the infiltration of macrophages. Tumor-associated macrophages (TAMs) are also special kinds of immune cells (31) that can be divided into M1 and M2 types and play important roles in tumor proliferation, migration, invasion, and tumor immune escape (32–34). M1 macrophages have antitumor functions, including direct cytotoxicity (35) and antibody-dependent cell-mediated cytotoxicity (36) (ADCC), to kill tumor cells. M2 macrophages can promote the occurrence and metastasis of tumor cells (37), inhibit the antitumor immune response mediated by T cells, promote tumor angiogenesis, and lead to tumor progression (38, 39). We found that CHSY3 was closely correlated with M2-type macrophage markers, including CD163, VSIG4, and MS4A4A, but the correlation between CHSY3 and M1-type macrophage markers, such as NOS2 and IRF5, was not strong. This finding suggested that CHSY3 is associated with the polarity of tumor-associated macrophages. Overall, we demonstrated that CHSY3 expression in gastric cancer is associated with immune infiltration. It is very important for tumor patients to choose the appropriate treatment by evaluating their prognosis. In recent years, the infiltration of immune cells in the tumor immune microenvironment has attracted increased attention, and we found that patients with high CHSY3 expression had worse OS.
This study has several limitations. First, we did not study the specific mechanism of the effect of CHSY3 on tumor proliferation and migration, which requires further research. Second, it is not sufficient to use only CD68 as a marker for macrophages. Again, we did not perform in vivo experiments to verify the function of CHSY3, and additional in-depth studies are needed.
5 Conclusions
Our study revealed a correlation between CHSY3 expression and clinical prognosis, immune infiltrates and DNA methylation. Furthermore, we confirmed that CHSY3 was highly expressed in GC cells and contributed to proliferation and migration. These results could lead to the use of a predictive biomarker and an inclusive understanding of CHSY3 expression in multiple tumor types, especially in GC.
Data availability statement
The original contributions presented in the study are included in the article/Supplementary Material. Further inquiries can be directed to the corresponding authors.
Ethics statement
The studies involving humans were approved by Shanghai Outdo Biotech Company and Guangdong Provincial People’s Hospital. The studies were conducted in accordance with the local legislation and institutional requirements. The participants provided their written informed consent to participate in this study.
Author contributions
HW: Conceptualization, Data curation, Formal analysis, Investigation, Methodology, Resources, Software, Supervision, Validation, Visualization, Writing – original draft. JuZ: Conceptualization, Data curation, Formal analysis, Funding acquisition, Investigation, Methodology, Resources, Software, Supervision, Validation, Writing – original draft. ZW: Data curation, Investigation, Methodology, Software, Writing – original draft. SC: Conceptualization, Data curation, Methodology, Software, Writing – original draft. JiZ: Funding acquisition, Project administration, Resources, Supervision, Validation, Visualization, Writing – review & editing. YL: Conceptualization, Funding acquisition, Project administration, Resources, Supervision, Validation, Visualization, Writing – review & editing.
Funding
The author(s) declare financial support was received for the research, authorship, and/or publication of this article. The study was supported by the National Natural Science Foundation of China (32370836), the National Key Clinical Specialty Construction Project (2021-2024, No. 2022YW030009), the Fundamental Research Funds for the Central Universities (21623303), the Guangdong Basic and Applied Basic Research Foundation (2023A1515110954), and the Funding by Science and Technology Projects in Guangzhou (2024A04J4101). All these study sponsors had no role in the study design or in the collection, analysis or interpretation of the data.
Conflict of interest
The authors declare that the research was conducted in the absence of any commercial or financial relationships that could be construed as a potential conflict of interest.
Publisher’s note
All claims expressed in this article are solely those of the authors and do not necessarily represent those of their affiliated organizations, or those of the publisher, the editors and the reviewers. Any product that may be evaluated in this article, or claim that may be made by its manufacturer, is not guaranteed or endorsed by the publisher.
Supplementary material
The Supplementary Material for this article can be found online at: https://www.frontiersin.org/articles/10.3389/fimmu.2024.1364979/full#supplementary-material
References
1. Smyth EC, Nilsson M, Grabsch HI, van Grieken NC, Lordick F. Gastric cancer. Lancet (London England). (2020) 396:635–48. doi: 10.1016/S0140-6736(20)31288-5
2. Yuan Z, Cui H, Xu Q, Gao J, Liang W, Cao B, et al. Total versus proximal gastrectomy for proximal gastric cancer after neoadjuvant chemotherapy: a multicenter retrospective propensity score-matched cohort study. Int J Surg. (2023) 110(2):1000–7. doi: 10.1097/JS9.0000000000000927
3. Park J, Hsueh P-C, Li Z, Ho P-C. Microenvironment-driven metabolic adaptations guiding CD8(+) T cell anti-tumor immunity. Immunity. (2023) 56:32–42. doi: 10.1016/j.immuni.2022.12.008
4. Rui R, Zhou L, He S. Cancer immunotherapies: advances and bottlenecks. Front Immunol. (2023) 14:1212476. doi: 10.3389/fimmu.2023.1212476
5. Vu LD, Gevaert K, De Smet I. Protein language: post-translational modifications talking to each other. Trends Plant Sci. (2018) 23:1068–80. doi: 10.1016/j.tplants.2018.09.004
6. Lee JM, Hammarén HM, Savitski MM, Baek SH. Control of protein stability by post-translational modifications. Nat Commun. (2023) 14:201. doi: 10.1038/s41467-023-35795-8
7. Pinho SS, Reis CA. Glycosylation in cancer: mechanisms and clinical implications. Nat Rev Cancer. (2015) 15:540–55. doi: 10.1038/nrc3982
8. Krug J, Rodrian G, Petter K, Yang H, Khoziainova S, Guo W, et al. N-glycosylation regulates intrinsic IFN-γ Resistance in colorectal cancer: implications for immunotherapy. Gastroenterology. (2023) 164(3):392–406.e5. doi: 10.1053/j.gastro.2022.11.018
9. Mereiter S, Balmaña M, Campos D, Gomes J, Reis CA. Glycosylation in the era of cancer-targeted therapy: where are we heading? Cancer Cell. (2019) 36:6–16. doi: 10.1016/j.ccell.2019.06.006
10. Koch A, Joosten SC, Feng Z, de Ruijter TC, Draht MX, Melotte V, et al. Analysis of DNA methylation in cancer: location revisited. Nat Rev Clin Oncol. (2018) 15(7):459–66. doi: 10.1038/s41571-018-0004-4
11. Papanicolau-Sengos A, Aldape K. DNA methylation profiling: an emerging paradigm for cancer diagnosis. Annu Rev Pathol. (2022) 17:295–321. doi: 10.1146/annurev-pathol-042220-022304
12. Jones PA, Ohtani H, Chakravarthy A, De Carvalho DD. Epigenetic therapy in immune-oncology. Nat Rev Cancer. (2019) 19:151–61. doi: 10.1038/s41568-019-0109-9
13. Esteller M. Relevance of DNA methylation in the management of cancer. Lancet Oncol. (2003) 4:351–8. doi: 10.1016/S1470-2045(03)01115-X
14. Kennedy LB, Salama AKS. A review of cancer immunotherapy toxicity. CA. Cancer J Clin. (2020) 70(2):86–104. doi: 10.3322/caac.21596
15. Riley RS, June CH, Langer R, Mitchell MJ. Delivery technologies for cancer immunotherapy. Nat Rev Drug Discovery. (2019) 18:175–96. doi: 10.1038/s41573-018-0006-z
16. Zhao Y, Shao Q, Peng G. Exhaustion and senescence: two crucial dysfunctional states of T cells in the tumor microenvironment. Cell Mol Immunol. (2020) 17:27–35. doi: 10.1038/s41423-019-0344-8
17. Liu J, Tian Z, Liu T, Wen D, Ma Z, Liu Y, et al. CHSY1 is upregulated and acts as tumor promotor in gastric cancer through regulating cell proliferation, apoptosis, and migration. Cell Cycle. (2021) 20(18):1861–74. doi: 10.1080/15384101.2021.1963553
18. Liu C-H, Lan C-T, Chou J-F, Tseng T-J, Liao W-C. CHSY1 promotes aggressive phenotypes of hepatocellular carcinoma cells via activation of the hedgehog signaling pathway. Cancer Lett. (2017) 403:280–8. doi: 10.1016/j.canlet.2017.06.023
19. Liao W-C, Liao CK, Tseng TJ, Ho YJ, Chen YR, Lin KH, et al. Chondroitin sulfate synthase 1 enhances proliferation of glioblastoma by modulating PDGFRA stability. Oncogenesis. (2020) 9(2):9. doi: 10.1038/s41389-020-0197-0
20. Zeng L, Qian J, Luo X, Zhou A, Zhang Z, Fang Q. CHSY1 promoted proliferation and suppressed apoptosis in colorectal cancer through regulation of the NFκB and/or caspase-3/7 signaling pathway. Oncol Lett. (2018) 16(5):6140–6. doi: 10.3892/ol
21. Jiang Y, Zhang H, Li W, Yan Y, Yao X, Gu W. FOXM1-activated LINC01094 promotes clear cell renal cell carcinoma development via microRNA 224-5p/CHSY1. Mol Cell Biol. (2020) 40(3):e00357-19. doi: 10.1128/MCB.00357-19
22. Sun G, Zhao S, Fan Z, Wang Y, Liu H, Cao H, et al. CHSY1 promotes CD8(+) T cell exhaustion through activation of succinate metabolism pathway leading to colorectal cancer liver metastasis based on CRISPR/Cas9 screening. J Exp Clin Cancer Res. (2023) 42(1):248. doi: 10.1186/s13046-023-02803-0
23. Zhang J, Chen Z, Wang B, Chen J, Xiao T, Zhang JV, et al. Reduction of pl-CSA through ChSy-2 knockout inhibits tumorigenesis and metastasis of choriocarcinoma in JEG3 cells. Int J Med Sci. (2021) 18(1):207–15. doi: 10.7150/ijms.51900
24. Huang X, Liu Y, Qian C, Shen Q, Wu M, Zhu B, et al. CHSY3 promotes proliferation and migration in gastric cancer and is associated with immune infiltration. J Transl Med. (2023) 21(1):474. doi: 10.1186/s12967-023-04333-x
25. Li X, Fan Y, Zhang Y, Wang Y, Zhao M, Tang M, et al. CHSY3 can be a Poor Prognostic Biomarker and Mediates Immune Evasion in Stomach Adenocarcinoma. Front Genet. (2022) 13:876588. doi: 10.3389/fgene.2022.876588
26. Zhang W, Xu R, Chen J, Xiong H, Wang Y, Pang B, et al. Advances and challenges in biotechnological production of chondroitin sulfate and its oligosaccharides. Int J Biol Macromol. (2023) 253:126551. doi: 10.1016/j.ijbiomac.2023.126551
27. Ogawa H, Hatano S, Sugiura N, Nagai N, Sato T, Shimizu K, et al. Chondroitin sulfate synthase-2 is necessary for chain extension of chondroitin sulfate but not critical for skeletal development. PloS One. (2012) 7(8):e43806. doi: 10.1371/journal.pone.0043806
28. Yada T, Gotoh M, Sato T, Shionyu M, Go M, Kaseyama H, et al. Chondroitin sulfate synthase-2. Molecular cloning and characterization of a novel human glycosyltransferase homologous to chondroitin sulfate glucuronyltransferase, which has dual enzymatic activities. J Biol Chem. (2003) 278(32):30235–47. doi: 10.1074/jbc.M303657200
29. Rojas A, Schneider I, Lindner C, Gonzalez I, Morales MA. The RAGE/multiligand axis: a new actor in tumor biology. Biosci Rep. (2022) 42(7):BSR20220395. doi: 10.1042/BSR20220395
30. Rojas A, Lindner C, Schneider I, González I, Morales MA. Contributions of the receptor for advanced glycation end products axis activation in gastric cancer. World J Gastroenterol. (2023) 29:997–1010. doi: 10.3748/wjg.v29.i6.997
31. Anderson NR, Minutolo NG, Gill S, Klichinsky M. Macrophage-based approaches for cancer immunotherapy. Cancer Res. (2021) 81:1201–8. doi: 10.1158/0008-5472.CAN-20-2990
32. Xiang X, Wang J, Lu D, Xu X. Targeting tumor-associated macrophages to synergize tumor immunotherapy. Signal Transduction Targeting Ther. (2021) 6:75. doi: 10.1038/s41392-021-00484-9
33. Pan Y, Yu Y, Wang X, Zhang T. Tumor-associated macrophages in tumor immunity. Front Immunol. (2020) 11:583084. doi: 10.3389/fimmu.2020.583084
34. Zhang H, Liu L, Liu J, Dang P, Hu S, Yuan W, et al. Roles of tumor-associated macrophages in anti-PD-1/PD-L1 immunotherapy for solid cancers. Mol Cancer. (2023) 22(1):58. doi: 10.1186/s12943-023-01725-x
35. Bernsmeier C, van der Merwe S, Périanin A. Innate immune cells in cirrhosis. J Hepatol. (2020) 73:186–201. doi: 10.1016/j.jhep.2020.03.027
36. Bruns H, Büttner M, Fabri M, Mougiakakos D, Bittenbring JT, Hoffmann MH, et al. Vitamin D-dependent induction of cathelicidin in human macrophages results in cytotoxicity against high-grade B cell lymphoma. Sci Transl Med. (2015) 7(282):282ra47. doi: 10.1126/scitranslmed.aaa3230
37. Yin M, Li X, Tan S, Zhou HJ, Ji W, Bellone S, et al. Tumor-associated macrophages drive spheroid formation during early transcoelomic metastasis of ovarian cancer. J Clin Invest. (2016) 126(11):4157–73. doi: 10.1172/JCI87252
38. Nie Y, Huang H, Guo M, Chen J, Wu W, Li W, et al. Breast phyllodes tumors recruit and repolarize tumor-associated macrophages via secreting CCL5 to promote Malignant progression, which can be inhibited by CCR5 inhibition therapy. Clin Cancer Res an Off J Am Assoc Cancer Res. (2019) 25(13):3873–86. doi: 10.1158/1078-0432.CCR-18-3421
Keywords: gastric cancer, CHSY3, tumor-associated macrophages, prognosis, tumor immune microenvironment
Citation: Wang H, Zhang J, Wei Z, Chen S, Zheng J and Li Y (2024) The prognostic implications and tumor-promoting functions of CHSY3 in gastric cancer. Front. Immunol. 15:1364979. doi: 10.3389/fimmu.2024.1364979
Received: 03 January 2024; Accepted: 22 April 2024;
Published: 15 May 2024.
Edited by:
Ping Wang, Michigan State University, United StatesReviewed by:
Yingpu Li, Harbin Medical University Cancer Hospital, ChinaArmando Rojas, Catholic University of the Maule, Chile
Copyright © 2024 Wang, Zhang, Wei, Chen, Zheng and Li. This is an open-access article distributed under the terms of the Creative Commons Attribution License (CC BY). The use, distribution or reproduction in other forums is permitted, provided the original author(s) and the copyright owner(s) are credited and that the original publication in this journal is cited, in accordance with accepted academic practice. No use, distribution or reproduction is permitted which does not comply with these terms.
*Correspondence: Jiabin Zheng, emhlbmdqaWFiaW5AZ2RwaC5vcmcuY24=; Yong Li, bGl5b25nQGdkcGgub3JnLmNu
†These authors have contributed equally to this work