- 1Laboratory of Immunology, Mossakowski Medical Research Institute Polish Academy of Sciences, Warsaw, Poland
- 2Department of Immunology, Medical University of Warsaw, Warsaw, Poland
CD20 located predominantly on the B cells plays a crucial role in their development, differentiation, and activation, and serves as a key therapeutic target for the treatment of B-cell malignancies. The breakthrough of monoclonal antibodies directed against CD20, notably exemplified by rituximab, revolutionized the prognosis of B-cell malignancies. Rituximab, approved across various hematological malignancies, marked a paradigm shift in cancer treatment. In the current landscape, immunotherapies targeting CD20 continue to evolve rapidly. Beyond traditional mAbs, advancements include antibody-drug conjugates (ADCs), bispecific antibodies (BsAbs), and chimeric antigen receptor-modified (CAR) T cells. ADCs combine the precision of antibodies with the cytotoxic potential of drugs, presenting a promising avenue for enhanced therapeutic efficacy. BsAbs, particularly CD20xCD3 constructs, redirect cytotoxic T cells to eliminate cancer cells, thereby enhancing both precision and potency in their therapeutic action. CAR-T cells stand as a promising strategy for combatting hematological malignancies, representing one of the truly personalized therapeutic interventions. Many new therapies are currently being evaluated in clinical trials. This review serves as a comprehensive summary of CD20-targeted therapies, highlighting the progress and challenges that persist. Despite significant advancements, adverse events associated with these therapies and the development of resistance remain critical issues. Understanding and mitigating these challenges is paramount for the continued success of CD20-targeted immunotherapies.
Introduction
CD20 is a surface protein that exhibits ubiquitous expression in B cells with minimal occurrence in other tissues, rendering it an ideal target for immunotherapy against B cell-derived malignancies. CD20 expression initiates during the pre-B cell stage and persists until B cells undergo terminal differentiation into plasma cells (Figure 1). Immunotherapy directed at CD20 is extensively employed for treating mature B cell-derived malignancies, such as chronic lymphocytic leukemia (CLL) and various B cell-derived non-Hodgkin lymphomas (B-NHL), including follicular lymphoma (FL), diffuse large B-cell lymphoma (DLBCL), and mantle cell lymphoma (MCL). CD20 is also present in multiple subtypes of B cell precursor acute lymphoblastic leukemia (B-ALL), albeit its expression at diagnosis is heterogeneous and frequently low (1–3). Notably, documented upregulation of CD20 after induction treatment suggests a potential expansion of CD20-directed immunotherapy applications for B-ALL (4, 5). CD20-specific therapies offer precise B cell targeting, minimizing impact on other cell types. These therapies efficiently deplete CD20-expressing B cells without hindering the replenishment of the B-cell compartment from early B cell precursors. Hence, upon cessation of anti-CD20 treatment, the B-cell population can recover (6). Notably, the absence of CD20 on fully mature plasma cells enables patients to maintain protective humoral immunity against previously encountered pathogens during treatment (6).
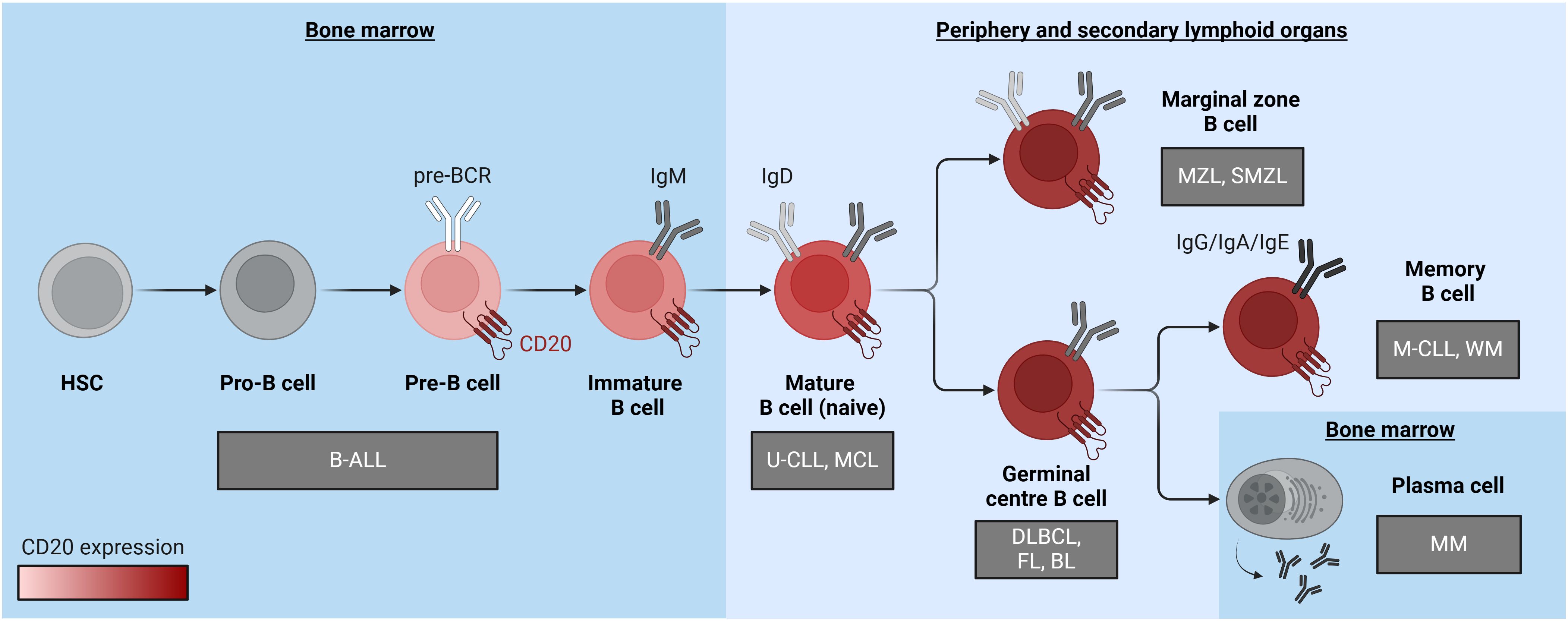
Figure 1 A diagram illustrating B cell differentiation and maturation, emphasizing the pronounced increase in CD20 expression levels depicted through a red color gradient. Associated malignancies are positioned near the cell of origin and represented within grey boxes. B-ALL, B cell acute lymphoblastic leukemia; BL, Burkitt lymphoma; DLBCL, diffuse large B cell lymphoma; FL, follicular lymphoma; HSC, hematopoietic stem cell; MCL, mantle cell lymphoma; M-CLL, mutated chronic lymphocytic leukemia; MM, multiple myeloma; MZL, marginal zone lymphoma; SMZL, splenic marginal zone lymphoma; U-CLL, unmutated chronic lymphocytic leukemia; WM, Waldenstrom macroglobulinaemia. The figure was created using BioRender.com.
CD20-targeted immunotherapy encompasses diverse modalities administered at various treatment stages. Rituximab, the pioneering anti-CD20 monoclonal antibody (mAb) introduced in 1997, stands out as a well-studied, low-toxicity immunotherapy with manageable side effects. It is a crucial component of the common therapy regimens, such as BR (bendamustine + rituximab) or FCR (fludarabine + cyclophosphamide + rituximab), which are often used as a first-line treatment in specific groups of CLL and B-NHL patients. In addition, following positive phase 3 trial results, rituximab has been recently integrated into chemotherapy for adult B-ALL patients with at least 20% CD20-positive leukemic cells (7). Beyond rituximab, the engineered anti-CD20 mAb obinutuzumab is registered and employed in combination with chemotherapy as first-line therapy for defined cases of CLL and FL. In addition to mAbs, new immunotherapies targeting CD20 have been developed and successfully introduced into the clinic for patients refractory to first-line therapy or with relapsed disease (r/r). These include bispecific antibodies (BsAbs) targeting the CD20 molecule and simultaneously recruiting cytotoxic T cells, as well as adoptive therapies using autologous T cells modified with chimeric antigen receptors (CAR-T). Three BsAbs targeting CD20 have received FDA approval, while CD20-specific CAR-T cells are presently undergoing clinical trials. Notably, CAR-T cells simultaneously targeting CD19 and CD20 aim to address CD19-negative clones, with ongoing clinical trials in advanced r/r B-cell malignancies (Table 1).
This comprehensive review explores various CD20-directed immunotherapies, including mAbs, radio-immunoconjugates, BsAbs, and CD20 CAR-T cells. The discussion encompasses both approved drugs and novel solutions undergoing investigation in preclinical and clinical trials (Figures 2, 3; Tables 1–3). Mechanisms of resistance to CD20-directed immunotherapies are presented (Figure 4), and the potential for various combinations with immunotherapies is discussed.
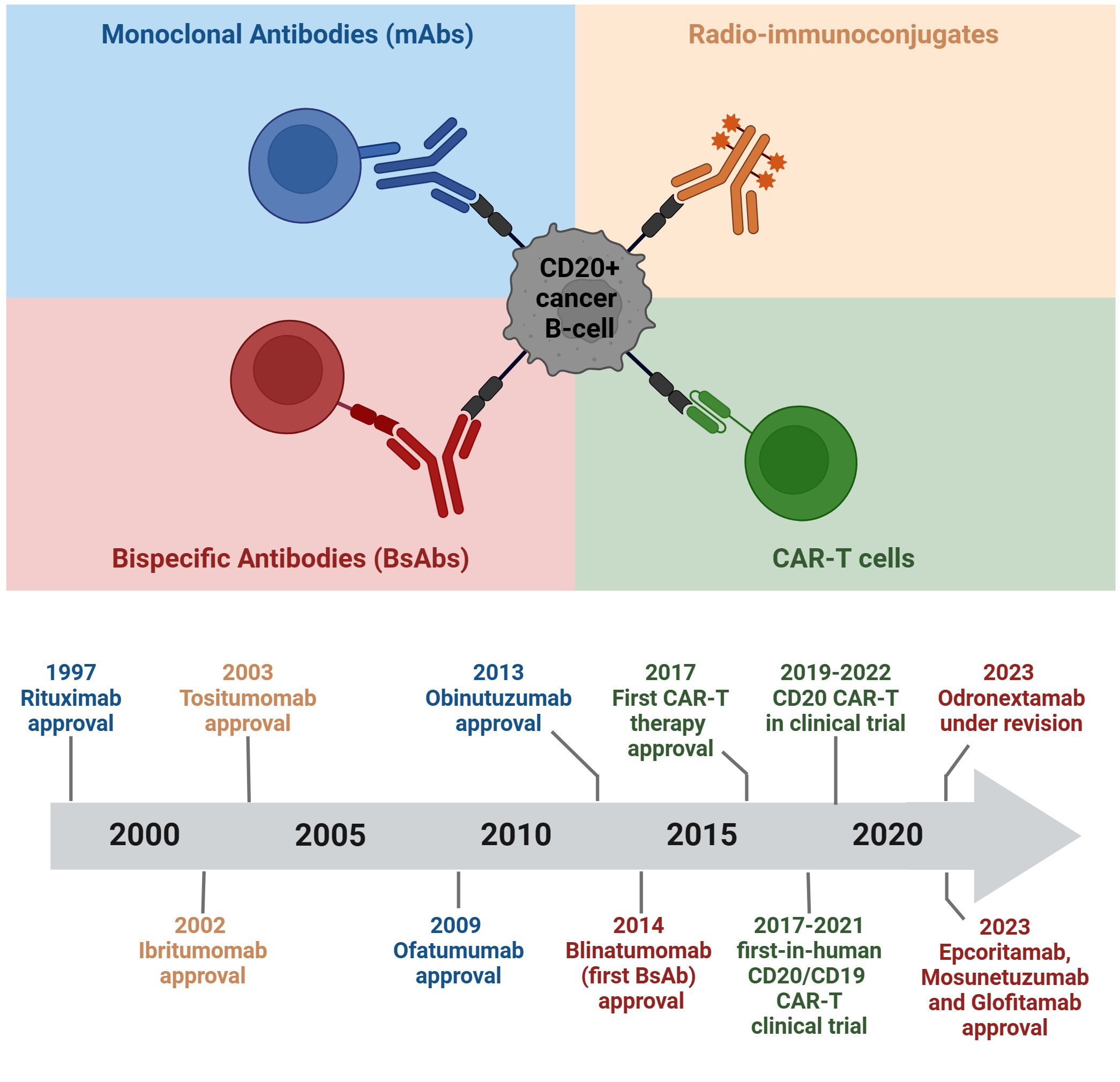
Figure 2 Chronology of clinical approvals and recent breakthroughs in CD20-targeted immunotherapies. The figure was created using BioRender.com.
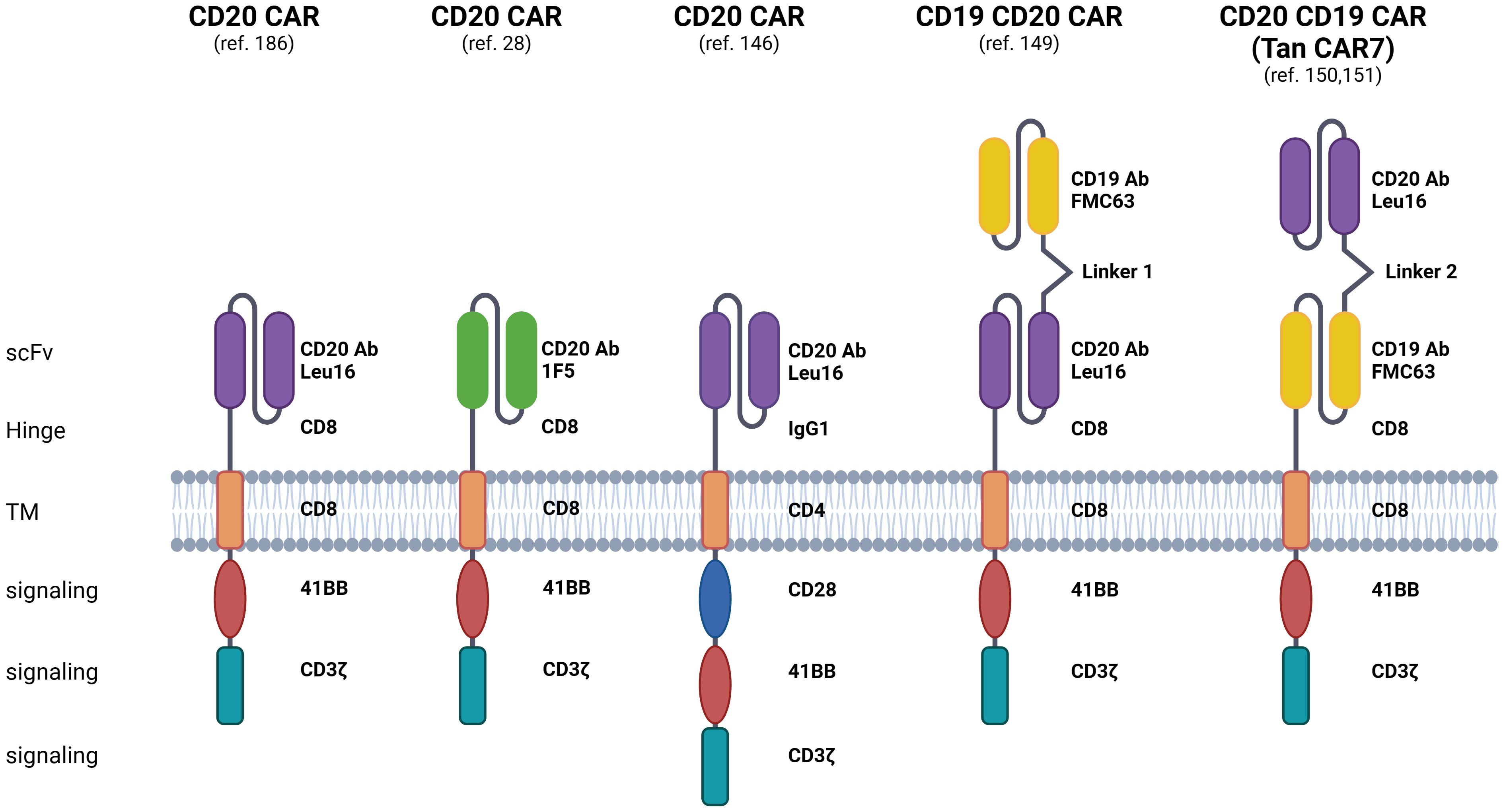
Figure 3 Displayed are CAR constructs, including second- and third-generation designs specifically recognizing the CD20 molecule, alongside dual-specificity CAR constructs recognizing both CD20 and CD19 molecules. Additionally, preclinical and clinical investigations explore the efficacy of bi- or tri-cistronic vectors for the expression of these CARs. Linker 1: (G4S)5, Linker 2: (EAAAK)3. The figure was created using BioRender.com.
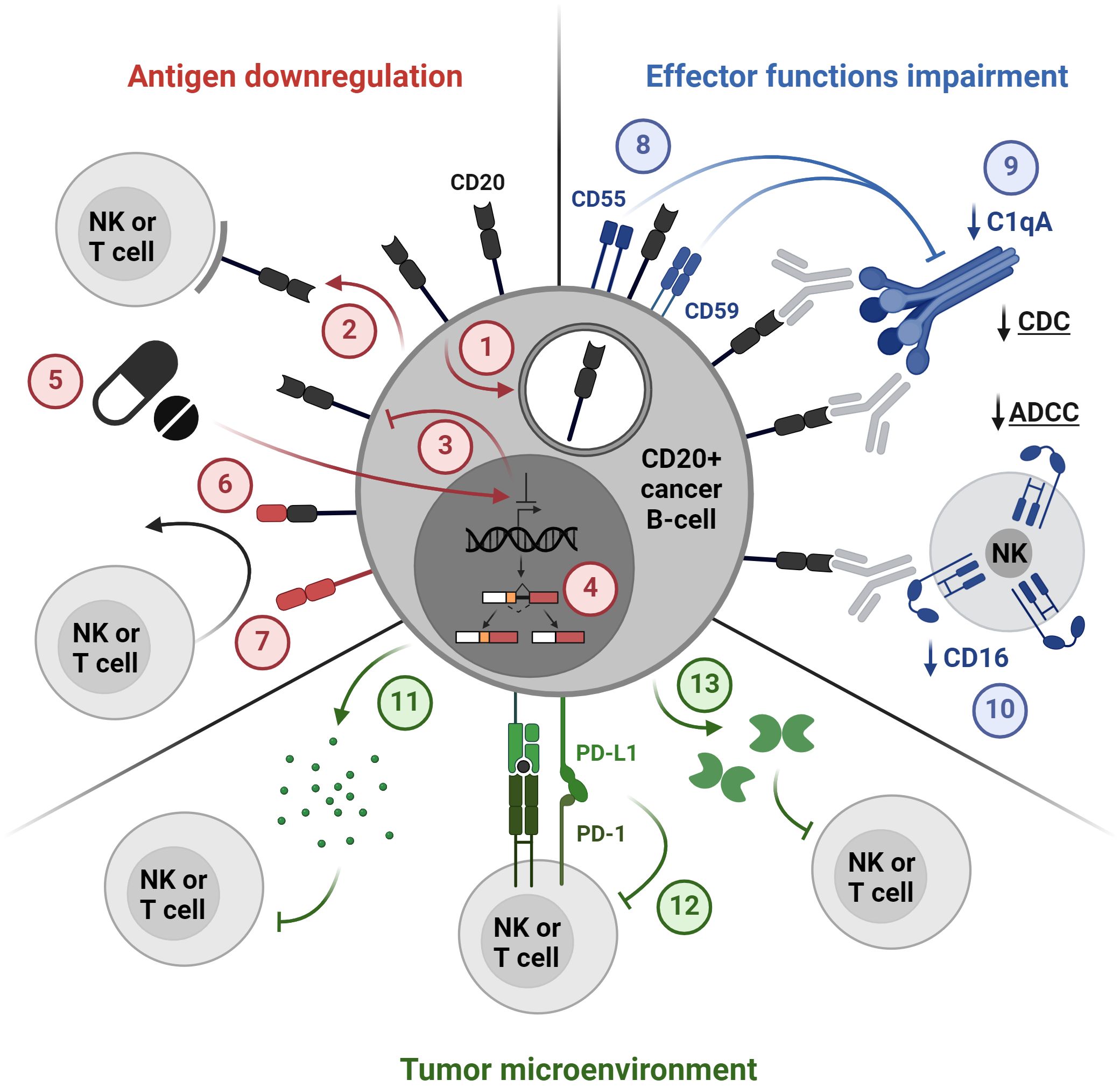
Figure 4 Mechanisms of resistance to CD20-directed immunotherapies. 1. Internalization, 2. Trogocytosis, 3. Loss of antigen expression, 4. Alternative splicing, 5. Drug-induced antigen downregulation, 6. Loss of an epitope, 7. Lineage switch, 8. Overexpression of complement regulatory proteins, 9. Downregulation of complement proteins, 10. CD16 downregulation, 11. Secretion of immunosuppressive cytokines, 12. Immune checkpoints, 13. Secretion of suppressive molecules, e.g. galectin 1. The figure was created using BioRender.com.
CD20 antigen: structure, function, and expression regulation
CD20 is a transmembrane protein whose significance as a target for immunotherapy is well recognized, although its biological role remains elusive. Encoded by the MS4A1 gene, CD20 is part of the MS4A family, which consists of 18 proteins with similar structures. The CD20 protein spans the cell membrane with four transmembrane helices and features two extracellular loops, which are the main epitopes recognized by anti-CD20 mAbs. Notably, both the N-terminal and C-terminal ends of CD20 are situated inside the cell. The detailed structure and dimeric assembly of CD20 on the cell membrane have recently been extensively characterized (9). This structural research sheds light on the molecular architecture of CD20 and anti-CD20 mAbs biding modes, contributing to our understanding of its potential as a target for immunotherapy (9).
While no identified physiological ligand binds to CD20, it is known to form nanoclusters on the B cell membrane with proteins such as IgD or IgM-class B cell receptors (BCR), CD19, CXCR4, and CD40 (10). A recent study utilizing CRISPR/Cas9-mediated CD20 elimination from mature B cells revealed CD20’s role as a gatekeeper in maintaining the resting state. The knockout of CD20 resulted in the translocation of the BCR toward the CD19 coreceptor, transient B cell activation, and internalization of various B cell-specific proteins (10). Additionally, initial research proposed that CD20 may function as a calcium channel (11), however, subsequent findings suggested that calcium flux induction is mediated by the CD20-BCR complex rather than CD20 alone (12). Therefore, the role of CD20 as a regulator of B cell activity seems to be inherently linked to interactions of CD20 with other surface proteins, primarily with the BCR complex.
The regulation of the MS4A1 gene was attributed to several transcription factors, summarized in (13). The described positive regulators include transcription factors essential for B cell development and maturation, such as PU.1, PiP (IRF4), NFκB (14–16), as well as other factors, such as USF, TFE3.1 (16), OCT1, OCT2 (17), ELK1, ETS1 (18), SP1, CHD4 and MBD2 (19). Recently, another member of interferon regulatory factors (IRF) engaged in B cell development, IRF8, was shown to promote CD20 expression in DLBCL as well as in healthy B cells (20). Negative regulators of CD20 include FOXO1 (21), CREM (19), SMAD2/3 (22), and MYC (23, 24).
Additional regulation of CD20 expression occurs on the epigenetic level. Histone deacetylase (HDAC) family members HDAC1/2, HDAC1/4, and HDAC6 as well as methyltransferase enzyme EZH2 can repress CD20 in healthy and malignant B cells (25–27). Recently, the occurrence of four 5’-UTR variants of MS4A1 mRNA with differential translation efficacy was described (28).
Anti-CD20 mAbs and immunoconjugates
The evolution of anti-CD20 mAbs marks a progression toward enhanced compatibility and reduced immunogenicity. The first therapeutic anti-CD20 mAb, rituximab, comprises a chimeric murine-human structure, contributing to the development of immune response and infusion-related reactions due to its limited resemblance to natural human antibodies (29, 30). Enhanced human content in subsequent mAbs correlates with decreased immunogenicity and improved binding affinity to human Fc receptors. The newer generations of anti-CD20 mAbs, exhibiting humanized (obinutuzumab) and fully human (ofatumumab) designs have reduced immunogenicity (31–33). Furthermore, heightened human sequence content enhances interactions with immune effector cells and FcRn receptors on hepatic and epithelial cells, thereby prolonging IgG antibodies’ half-life (34).
CD20-targeting mAbs elicit their cytotoxic function by at least four different mechanisms (35). Upon binding CD20 on target cells, they can activate complement-mediated cytotoxicity (CDC), engage immune effector cells to mediate antibody-dependent cytotoxicity (ADCC) and phagocytosis (ADCP), as well as directly induce cell death. The anti-CD20 mAbs currently employed in cancer treatment vary in their degree of activating specific mechanisms. These differences are the basis for a categorization of anti-CD20 mAbs into two types. The majority of mAbs are characterized as type I, which exhibit the ability to cluster CD20 into membrane lipid rafts, which is associated with potent induction of CDC. On the other hand, type I antibodies display a higher rate of internalization, which can limit their therapeutic efficacy (36). Type II mAbs do not stabilize CD20 in lipid rafts and are weak inducers of CDC, but they potently evoke direct cell death (37). Rituximab, ofatumumab, and ublituximab are classified as the type I anti-CD20 mAbs, whereas obinutuzumab represents a type II anti-CD20 mAb.
CD20-directed mAbs are also used in the form of immunoconjugates – Abs linked with drugs (ADCs), toxins (immunotoxins or engineered toxin bodies – ETBs) or radioactive isotopes (radio-immunoconjugates). Unlike conventional antibodies, which largely depend on the immune effector cells or the complement system for their cytotoxic effects, immunoconjugates can directly induce apoptosis of cancer cells. Due to the relatively poor internalization of CD20, few ADCs and immunotoxins were developed. A single-chain variable fragment-based targeting CD20 and conjugated with Shiga-like toxin A subunit, MT-3724, presented promising preclinical and early clinical results, but its development was ceased by the manufacturer (38). Another strategy that does not require CD20 internalization for direct and targeted cell killing is the use of radio-immunoconjugates. This approach has gained significant attention in targeting lymphoma cells, which are highly radiosensitive (39). Radio-immunoconjugates utilize ionizing radiation to induce cytotoxicity of the target cell. Concurrently, they can trigger classical effector mechanisms such as CDC, ADCC, and ADCP.
In this section, we describe anti-CD20 mAbs and conjugates that were approved for clinical use in lymphoid malignancies. Additionally, other anti-CD20 agents that displayed effectiveness in clinical trials of B-cell neoplasms are listed in Table 2.
Rituximab
Rituximab is the first mAb used for cancer therapy. It is a chimeric mouse/human IgG1 anti-CD20 mAb targeting the epitope on a large extracellular loop of CD20. As a type I mAb, rituximab elicits its function mostly by CDC, ADCC, and ADCP (35). Since gaining its first approval for low grade FL in 1997 (40), rituximab has consistently demonstrated its efficacy, both as part of combination drug regimens and as a standalone agent, across various clinical trials. Rituximab is currently employed in a broad spectrum of conditions including DLBCL, Burkitt lymphoma (BL), MCL, FL, marginal zone lymphoma (MZL), hairy cell leukemia (HCL), and CLL, as comprehensively reviewed in (41). A relatively recent hematologic application of rituximab involves its use in CD20+ adult B-ALL, serving as an adjunct to chemotherapy throughout all stages of treatment (7). The popularity and effectiveness of rituximab, as well as the expiration of its patent, has catalyzed an increase in the production of biosimilars. Following prior studies confirming its bioavailability, a new formulation of rituximab with hyaluronidase has been approved for subcutaneous use in FL, CLL, and DLBCL (42). Despite the success of rituximab, some patients experience relapses due to various resistance mechanisms, including trogocytosis, complement exhaustion, internalization of CD20 and others, described in the section Resistance to CD20-directed immunotherapies (43, 44). Attempts to increase the efficacy of rituximab prompted the trials combining rituximab with other drugs that could potentiate its cytotoxicity, ideally in chemotherapy-free schemes. A phase 3 study AUGUMENT confirmed the benefit of the addition of the immunomodulatory drug lenalidomide to the rituximab in r/r FL and MZL (45). Strategies involving the addition of mTOR inhibitors to rituximab combined with classic chemotherapeutics are also under investigation for the treatment of patients with r/r DLBCL, with promising results from phase 1 and 2 trials (46, 47).
Ofatumumab
Ofatumumab (2F2) is a fully human anti-CD20 IgG1κ mAb developed by Genmab and Glaxo PLC. It binds to an epitope distinct from that of rituximab, targeting both small and large extracellular loops of CD20 (48). Preclinical tests have shown that ofatumumab induces CDC more potently than rituximab, while the ADCC efficacy is comparable to that of rituximab (49, 50). The superior CDC efficacy of ofatumumab may be in part associated with the location of its target epitope more proximally to the cell membrane than the epitope recognized by rituximab (51). Recent structural studies also revealed that ofatumumab complexes show optimal geometry for complement recruitment (52). Additionally, ofatumumab demonstrates a slower off-rate than rituximab (49), allowing prolonged binding to the target cells. The first approval of ofatumumab was granted in 2009 for refractory CLL. Despite promising preclinical results, there is limited clinical evidence to confirm its superiority over other anti-CD20 agents (53). Clinical trials comparing ofatumumab to rituximab in FL (54) and DLBCL (55) relapsed after a rituximab-containing therapy showed no superiority of ofatumumab. On the other hand, the comparison of the treatment composed of hyper-fractionated cyclophosphamide, vincristine, doxorubicin, dexamethasone with ofatumumab (HCVAD-O) to the historical cohort of B-ALL CD20+ Ph- patients treated with HCVAD with rituximab (HCVAD-R) showed improvement in event-free survival (EFS) and overall survival (OS) (56). Currently, ofatumumab is rarely used in its initial indication, being replaced by newer agents such as obinutuzumab or ibrutinib (57–59).
Obinutuzumab
Obinutuzumab (GA101) is a humanized, glycoengineered IgG1 type II mAb that targets the epitope on the large extracellular loop of CD20, which partially overlaps with the rituximab epitope. The novelty of obinutuzumab design lies predominantly in the glycoengineering modifications, which were applied to improve affinity to the FcγR receptors on effector cells (60). Specifically, obinutuzumab exhibits reduced fucosylation of oligosaccharides attached to Asp297 in its Fc region, which results in improved binding of FcγRIII (61). In preclinical tests, obinutuzumab presented a slower internalization rate after binding to CD20 and superior efficacy in ADCC than rituximab and ofatumumab (62). The ADCP efficacy was comparable between the three antibodies (63). As a type II mAb, it exhibits reduced levels of CDC (60, 63), but was suggested to have the ability to induce direct cell death (DCD) via a non-apoptotic, lysosome-mediated mechanism, in some, but not all target cell types (64, 65). While obinutuzumab has consistently demonstrated greater effectiveness than equivalent doses of rituximab in the preclinical in vivo models (60, 66, 67), the exact reasons behind this advantage remain incompletely understood. The underlying mechanism appears to be multifaceted and potentially attributable to the combination of several factors including greater induction of ADCC and DCD, as well as being less prone to internalization (63). Importantly, obinutuzumab demonstrated superior efficacy as a part of the chemotherapy regimen in comparison with the same chemotherapy but with rituximab in first-line treatment of CLL patients, demonstrating improved progression-free survival (PFS) and OS in a phase 3 trial (68). This resulted in the approval of obinutuzumab in combination with chlorambucil for the treatment of patients with previously untreated CLL in 2013 (69). Recently published results from the phase 3 trial have also demonstrated the benefit of obinutuzumab over rituximab when used as a part of immunochemotherapy in the first-line treatment of FL (70). On the other hand, no advantage over rituximab was observed in advanced DLBCL (9, 71, 72). It is also important to note that the overall doses of obinutuzumab in the clinical trials were higher for most patients (68, 70, 73). An ongoing trial will assess the efficacy of obinutuzumab versus rituximab in B-ALL (NCT04920968). Additionally, promising results of the phase 1 trial of the combination of obinutuzumab with the novel oral cereblon-modulating agent avadomide suggest the potential for new chemotherapy-free regimens for NHL (74). Comprehensive information about obinutuzumab and its efficacy is reviewed in (69, 73, 75).
Radio-immunoconjugates: 90-Y-Ibritumomab tiuxetan and 131I-Tositumomab
Y-90-Ibritumomab tiuxetan is a murine anti-CD20 IgG1 mAb linked with Y-90 isotope of yttrium, which emits beta radiation and decays to non-radioactive Zirconium-90. A randomized controlled trial of 90-Y ibritumomab tiuxetan in r/r low-grade, follicular, or transformed NHL showed a significant improvement in overall response rate (ORR) and complete response (CR) rates (ORR 80% vs. 56%; CR 30% vs. 16%) in comparison to the rituximab treatment (76). 90-Y ibritumomab tiuxetan was approved in 2002 for r/r NHL patients, and in 2014 the approval was expanded for the first-line consolidation in NHL (77, 78).
131I-Tositumomab is a murine anti-CD20 monoclonal IgG2 antibody linked with Iodium-131, which emits beta and gamma radiation. It was approved for use in r/r NHL in 2003. Despite the documented efficacy in FL and r/r NHL (79, 80) 131I-Tositumomab was replaced by modern agents and its sale was discontinued in 2014.
While the use of radio-immunoconjugates is linked to an increased risk of secondary malignancies and myelotoxicity, their overall toxicity profile was considered acceptable and comparable to other therapies (39, 79, 81–84). Nonetheless, neither of the two radio-immunotherapeutic agents has been widely used in clinical practice, mainly due to economic and logistic problems, such as radiation safety concerns (85). Radio-immunotherapeutics targeting CD20 are extensively reviewed in (86).
Side effects of anti-CD20 mAbs and their management
The toxicity of anti-CD20 mAbs is relatively low, with hypersensitivity reactions, myelosuppression, and immunosuppression being the most common. Other common side effects include chest pain, arrythmia, paresthesia, nausea, diarrhea, abdominal pain, and muscle pain (87, 88). Rarely, more severe complications may occur, including tumor lysis syndrome or progressive multifocal leukoencephalopathy (PML). Common strategies for reducing hypersensitivity reaction incidence include premedication by steroids or antihistamine drugs and a slow rate of first infusion (89). For CLL patients with high lymphocyte counts (over 25 x 10^9/L), administration of i.v. prednisone or prednisolone is recommended before the infusion of rituximab to decrease the risk of acute infusion reactions and/or cytokine release syndrome (CRS) (88). Additionally, a recent study confirmed that obinutuzumab - as a humanized and potentially less immunogenic antibody - can be used as an alternative to rituximab after a hypersensitivity reaction (31). In the case of hypogammaglobulinemia, intravenous immunoglobulin (IVIG) replacement should be considered to reduce the risk of infections (90). Radiolabeled antibodies exhibit additional toxicities related to the emitted radiation, including the risk of myelodysplastic syndrome (MDS) and acute myeloid leukemia (AML) (86). Additionally, the use of 131I-Tositumomab can lead to hypothyroidism. This was addressed by oral administration of potassium iodide to inhibit the thyroid uptake of Iodium-131 (86).
CD20-directed BsAbs
BsAbs represent one of the most promising classes of off-the-shelf immunotherapies for the treatment of r/r B cell malignancies (91, 92). Notably, several BsAbs targeting the CD20 antigen received clinical approvals in 2023, with numerous others showing promising results in ongoing clinical trials (Figure 2, Table 3) (93). These engineered proteins, featuring dual binding sites, can simultaneously target two different antigens or two epitopes of the same antigen. This dual specificity allows BsAbs to bridge immune cells, such as T cells, with target tumor cells, promoting their interaction and subsequent cytotoxicity against the tumor cells.
Over the past few years, there has been a rapid development of this technology, resulting in various molecular BsAb formats, including IgG-like and non-IgG-like platforms (91, 92). IgG-like BsAbs mimic the structure of IgG, featuring an Fc region for effector functions, like ADCC and CDC, and provide a larger molecular weight, which increases solubility, stability, serum half-life. This allows for a wider spectrum of dosing frequency from daily to weekly or even less frequent and its administration both intravenously and subcutaneously. In contrast, non-IgG-like BsAbs lack the Fc region, typically have a smaller molecular weight, and primarily exert therapeutic effects through direct antigen binding (92). This applies also to blinatumomab, the first BsAb approved for medical use. Blinatumomab is a CD3xCD19 bispecific T-cell engager that consists only of two scFvs connected by a linker, which contributes to a relatively short half-life and the necessity for frequent dosing in prolonged infusions (94).
Among the various formats, the anti-CD20xCD3 BsAb engaging cytotoxic T cells is the most popular format. Here we focus on CD20-targeting IgG-like BsAbs as a new therapeutic option for patients with B-cell malignancies who have already undergone several lines of mAbs and CD19 CAR-T therapy.
Epcoritamab (DuoBody-CD3xCD20, GEN3013)
It is a full-length IgG1 BsAb generated by Fab-arm exchange of a humanized CD3 mAb and human CD20 mAb (95). In preclinical studies, epcoritamab has demonstrated its efficacy by eliciting robust T-cell activation and T-cell-mediated cytotoxicity against NHL cell lines in vitro (95). It also showed high effectiveness against primary cells derived from lymph node biopsies from newly diagnosed and r/r B-NHL patients (96). Moreover, epcoritamab-mediated cytotoxicity was observed against primary CLL cells in vitro and in vivo in patient-derived xenografts (PDX), where epcoritamab demonstrated a reduction in both blood and spleen disease burden. This effect was enhanced when used in combination with Bruton’s tyrosine kinase (BTK) and BCL2 inhibitors (97).
These promising results led to the testing of epcoritamab in clinical trials. In the first-in-human trial in patients with r/r B-cell lymphoma, including DLBCL, FL, MCL, high-grade B-cell lymphoma (HGBCL), primary mediastinal large B-cell lymphoma (PMBCL), small lymphocytic lymphoma (SLL) and MZL (EPCORE™ NHL-1, NCT03625037), epcoritamab administered as a single agent subcutaneously in 68 patients exhibited notable efficacy (88% ORR and 38% CR at 48 mg) (98). In an ongoing clinical trial evaluating the safety and efficacy of epcoritamab in patients with r/r CLL and Richter’s syndrome (EPCORE™ CLL-1, NCT04623541) so far epcoritamab was well tolerated (99). Several other clinical trials using this BsAb are currently underway, including testing a combination with rituximab for the first-line FL (NCT05783609). In 2023 the encouraging outcomes of clinical trials resulted in FDA and EMA approval of epcoritamab for r/r DLBCL after at least two lines of systemic therapy (100, 101).
Odronextamab (REGN1979)
This hinge-stabilized, fully human IgG4 BsAb targeting CD20 and CD3, has demonstrated both in vitro and in vivo efficacy (102, 103), leading to further evaluation of its effectiveness in clinical trials. In a phase 1, multicenter trial (ELM-1, NCT02290951) investigating the safety and tolerability of odronextamab in 145 patients with CD20+ B-NHL pretreated with CD19 CAR-T therapy or refractory to the last line of therapy, ORR among all patients reached 51% (72 of 142 patients), but among those with FL who received doses of 5 mg or higher 91% ORR (29 of 32 patients) and 72% CRR (23 of 32 patients). DLBCL patients who received doses of 80 mg or higher without previous CAR T-cell therapy reached 53% ORR and all responses were complete, and those pretreated with CAR T-cell 33% ORR and 27% CR (104). Additionally, the efficacy and safety of odronextamab were demonstrated in a case report of two patients with r/r B-NHL refractory to CAR-T therapy, who achieved complete responses that persisted for over 2 years of follow-up (105). In the light of these results, currently the phase 2 clinical trial is conducted. It assesses the anti-tumor activity and safety of odronextamab in pretreated patients with B-NHL (ELM-2, NCT03888105).
Odronextamab is not yet fully approved for marketing, but in September 2023 FDA accepted it for Priority Review for the treatment of adult patients with r/r FL and r/r DLBCL after at least two prior systemic therapies (106). Almost at the same time, EMA has accepted it for review in the same medical indications (107). Previously this drug was designated by EMA as an orphan drug for FL and DLBCL.
Mosunetuzumab (BTCT4465A)
It is a full-length, humanized IgG1 CD20xCD3 BsAb, generated using “knobs-into-holes” heterodimerization technology, which allows the combination of two heavy chains, one with the ‘knob’ mutation and the other with the ‘hole’ mutation, into one BsAb (108, 109). It was effective in vitro against tumor B cells obtained from PBMC of CLL patients, and in vivo in mice and cynomolgus monkeys, causing complete B cell depletion in peripheral blood and lymphoid tissues also in the presence of a competitive anti-CD20 mAb (108).
It has been tested in phase 1/2 clinical trial verifying it as a single agent and combined with atezolizumab (anti-PD-L1 mAb) in NHL and CLL (NCT02500407) and demonstrated notable efficacy and a manageable safety profile in patients with r/r FL (ORR 78%, CR 60%) (110). In patients with r/r DLBCL, including those previously treated with CAR-T cells, ORR was 42% and CR 23.9% (111). It is also being investigated in combination with polatuzumab vedotin (CD79b-directed ADC approved for patients with previously untreated DLBCL, NOS and HGBL with International Prognostic Index (IPI) score of at least 2) in B-NHL (NCT03671018) where it shows a favorable safety profile with highly durable responses (112). Currently, many other single-agent and combination studies of mosunetuzumab in r/r and previously untreated B-NHL are ongoing. In June 2022 mosunetuzumab obtained conditional approval from EMA (113) and in January 2023 FDA approved it for adult patients with r/r FL after two or more lines of systemic therapy (114).
Glofitamab (RO7082859)
It is CD20xCD3 heterodimeric human IgG1 BsAb with two anti-CD20 and one anti-CD3 Fabs (115). In preclinical studies, it showed higher potency than classical 1:1 IgG BsAbs, and its main treatment-related risk of CRS was mitigated by prior treatment with obinutuzumab (115). This combination of anti-CD20 therapies was evaluated in phase 1/2 clinical trial in patients with r/r B-NHL (NCT03075696), where it demonstrated durable responses, with most patients in CR (116, 117). These clinical findings were also confirmed in a group of 46 heavily pretreated patients with r/r DLBCL, who were given the drug under compassionate use and reached 7 months median OS (118). Glofitamab is also tested in combination with polatuzumab vedotin plus rituximab, cyclophosphamide, doxorubicin and prednisone (R-pola-CHP) and shows promising early results (ORR 100% and CR 76,5% among 17 patients) (119). Therefore in June 2023 FDA granted accelerated approval to glofitamab for r/r DLBCL, not otherwise specified (NOS) or large B-cell lymphoma (LBCL) arising from FL, after two or more lines of systemic therapy (120), and in July EMA approved it for conditional use for adults with r/r DLBCL after at least two previous treatments (121).
Imvotamab (IGM-2323)
This CD20xCD3 IgM BsAb is generated from 10 high-affinity CD20 binding domains and a single anti-CD3 scFv fused through the recombinant J-chain (122, 123). It exhibits a higher avidity for the CD20 binding and induces CDC against CD20-expressing cells with a greater potency than IgG BsAbs in vitro (122). Moreover, it exhibits vastly reduced cytokine release in vitro and in vivo (122) and seems to maintain higher effectiveness in the presence of rituximab than IgG BsAbs (124). A combination of imvotamab and loncastuximab tesirine (CD19-directed ADC approved in r/r LBCL after two or more lines of systemic therapy) demonstrated enhanced cytotoxic effect in preclinical studies (125) and is currently tested in first-in-human clinical trial in patients with r/r NHL (NCT04082936). So far imvotamab shows notable safety and tolerability profile due to repeatable IFNγ-dominant cytokine profile (123, 126).
Plamotamab (XmAb13676)
This humanized CD20xCD3 IgG1 BsAb is heterodimer with one IgG Fab arm exchanged for a scFv (127, 128). Preclinical in vivo data show its efficiency both in circulation and lymphoid organs (127). A phase 1 clinical trial (NCT02924402) evaluating its safety and tolerability in patients with CD20-expressing hematologic malignancies is ongoing and demonstrated so far evidence of clinical activity in heavily pretreated patients with DLBCL and FL, including earlier treatment with CAR-T therapy (129, 130).
Other BsAbs
There are several new directions in the further development of BsAbs, involving the use of antigens other than CD3. These include, among others, CD20xNKG2D antibodies, which engage the cytotoxic activity of NK cells against leukemic cells in vitro (131, 132). Another novel type of BsAb tested in preclinical studies is the CD95xCD20 antibody, which induces apoptosis in malignant B cells both in vitro and in vivo (133, 134). Finally, CD20xCD28 antibodies, which were first created over 20 years ago, however, due to the high production costs using conventional methods, were not developed for a long time (135, 136).
Side effects of CD20-directed BsAbs
Although significant therapeutic successes have been observed in clinical trials, CD20xCD3 BsAbs are associated with certain side effects. The most common is CRS, primarily associated with the initial doses and confined to the first cycle of treatment (110, 137). This is related to the simultaneous binding of BsAb to CD3 of effector cells and FcγR of other immune system cells or complement factor C1q, which results in premature activation and release of cytokines, hampering the effectiveness of therapy and increasing its toxicity. Therefore, currently used BsAbs have silencing mutations in the Fc regions that prevent binding to FcγR and C1q but retain binding to FcRn, which ensures extended plasma half-life (138). Another strategies to overcome CRS are step-up dosing of BsAbs (111, 139) and premedication with anti-CD20 mAb, which depletes B-cells in both peripheral blood and secondary lymphoid organs and decreases T cells activation (115). Other common adverse events include pyrexia, fatigue, injection-site reaction, nausea, diarrhea, hypophosphatemia, hematological toxicities: neutropenia, anemia, lymphopenia, thrombocytopenia, as well as neurological adverse events: headache, insomnia, dizziness (98, 99, 104, 110–112, 117–119, 126, 129, 130, 137, 139–143). Immune effector cell-associated neurotoxicity syndrome (ICANS) is a rare complication that occurs in less than 5% of patients treated with BsAb (119, 137, 139). The frequency and severity of these side effects vary. To mitigate risks, careful patient monitoring, premedication with anti-CD20 mAb, and dose adjustments are implemented to enhance the safety profile of CD20xCD3 BsAb therapies.
CD20-directed CAR-T cells
CD20 is also under exploration as a target for CAR-T cells in preclinical and clinical trials. CARs are synthetic constructs comprising extracellular antigen recognition domains, hinge and transmembrane regions, and intracellular signaling domains responsible for their activation and proliferation. Approved CAR T-cell therapy involves genetically engineered autologous products, utilizing the patient’s CAR T cells to target tumor cell antigens. Currently, four CD19-targeted CAR T-cell therapies are approved for treating r/r B-ALL and r/r B-NHL. Despite its efficacy, around 60% of patients experience disease relapse post-CD19 CAR-T treatment, often due to mechanisms like CD19 antigen loss. Also, in some patients, life-threatening toxicities occur, including severe CRS and ICANS (144, 145). Ongoing clinical trials suggest that CD20 CAR T-cell therapy could be a promising treatment for r/r NHL, even in cases of CD19-negative disease post-CD19 CAR-T cell relapse (Table 1). The structure of the clinically tested CD20 CAR T-cells is presented in Figure 3.
Phase 1/2 clinical trials utilizing second- and third-generation CAR constructs have confirmed the feasibility and efficacy of autologous anti-CD20 CAR-T cells in r/r CD20+ B-NHL (146). Particularly noteworthy is the efficacy of CD20 CAR T-cell therapy in treating r/r B-NHL patients who had previously failed chemotherapy, including R-CHOP. Studies indicate that CD20-targeted CAR T cells exhibit effectiveness even in cases of low antigen expression, proposing their potential utility for patients with CD20-downregulated B-NHL refractory to CD20 mAb therapy (28, 147). A comprehensive overview of ongoing and completed clinical trials for single CD20 CAR T-cell therapy in hematologic malignancies can be found in Table 2 of a recent review (148).
CD20 is also a pivotal target in CAR-T cell immunotherapies designed to mitigate antigen escape risks. Strategies targeting both CD19 and CD20 include bispecific/tandem CARs, co-administration of CD19 and CD20-directed CAR-T cells as well as sequential treatment with CD19 and CD20-directed CAR-T cells. Tan CAR7 T cells are bispecific CAR T cells composed of tandem extracellular domains targeting CD20 and CD19 tumor antigens linked in frame to the tisa-cel backbone, capable of activation via binding to either CD19 or CD20 tumor antigens, or both (149). Long-term remissions were observed following the use of Tan CAR7 T cells in r/r B-NHL with a safety profile that included CRS but few cases of high-grade CRS (150, 151). In a recent phase 1 dose-escalation trial, autologous CD19/CD20 bispecific CAR-T cells derived from naïve and memory T cells demonstrated safety and strong efficacy (90% ORR, 70% CR rate) in patients with r/r B-NHL (152). Beyond bispecific CD19/CD20 CAR T-cells, ongoing clinical trials explore sequential CD20 CAR-T after CD19 CAR-T infusion and combined infusion of CD19 and CD20-specific CAR-T cells for r/r B-ALL or DLBCL. However, a phase 2 trial combining anti-CD19 and anti-CD20 CAR-T cells in r/r DLBCL showed limited long-term responses (153). Recently, a combinatorial CAR-T cell approach targeting three antigens, CD19, CD20, and CD22, demonstrated efficacy in preclinical models, including leukemic cells that do not express CD19, thereby showcasing the promising potential for treating CD19-negative relapses (154). This approach is now undergoing testing in a clinical trial (NCT05418088).
Resistance to CD20-directed immunotherapies
Despite substantial progress in CD20-targeting immunotherapies, the issue of resistance and post-treatment relapse remains prominent. Resistance to CD20-targeted therapies encompasses a spectrum of mechanisms, ranging from alterations in CD20 antigen levels to compromised immune system effector functions, and extending to diverse mechanisms of immune evasion (Figure 4). One of the main causes of resistance is the loss of the CD20 antigen on the surface of the target cell, which can be caused by changes in the expression of the MS4A1 gene, including silenced expression and alternative splicing (28, 155–158). A recent study has shown that the gene encoding CD20 in both healthy and malignant B cells is alternatively spliced into four 5’-UTRs variants, of which especially variants V3 and V4 support robust translation. It has also been demonstrated that resistance to the BsAbs therapy targeting CD20 results from the V3-to-V1 shift (28). A potential strategy to combat this resistance through the use of phosphorodiamidate morpholino oligomers or antisense oligonucleotides was presented in preclinical studies (28). Other mechanisms of antigen loss which may also cause resistance include the internalization of the CD20-mAb complex by cancer cells through endocytosis as well as the transfer of membrane fragments containing CD20 from a cancer cell to an effector cell called trogocytosis (159–161).
Resistance to CD20-directed immunotherapies may also be caused by impaired effector functions of the immune system, such as CDC and ADCC. Therapies targeting CD20 may cause complement depletion and overexpression of its inhibitors CD55 and CD59 (44, 162). Additionally, downregulation of the complement component C1qA was associated with the resistance of DLBCL cells to rituximab in vitro (163). Potential strategies to overcome these mechanisms may include the use of inhibitors of complement regulatory proteins as well as the use of the new asymmetric CD55-binding bispecific antibodies (164). Moreover, rituximab-coated tumor cells were shown to significantly downregulate CD16 (FcγRIII), leading to impaired ADCC (165). Mutations that modify the binding of the Fc fragment of antibodies to FcγR can be used to increase the effector functions of antibodies (166, 167). However, as previously mentioned, this approach may not always be optimal when utilizing BsAbs, as it carries an increased risk of premature activation of T cells, cytokine release, and tissue damage.
Additionally, genetic alterations within signaling pathways can also contribute to resistance to CD20-directed therapies, especially in the context of T cell activation, which is crucial for the activity of BsAbs and CAR-T cells (168, 169). Moreover, the tumor microenvironment can play a role in resistance by creating an immunosuppressive milieu. Tumor cells and immunosuppressive cells in the tumor microenvironment, e.g. myeloid-derived suppressive cells (MDSCs), tumor-associated macrophages (TAMs), and regulatory T cells (Tregs), can secrete suppressive cytokines that inhibit the activity of effector cells (T cells, NK cells, phagocytes), thereby reducing the effectiveness of immunotherapy (170, 171). It has also been shown that some proteins secreted by the tumor may have a suppressive effect, including galectin-1, which inhibited CD20 mAb-induced phagocytosis in the lymphoma microenvironment (172). Moreover, overexpression of PD-L1 by tumor cells can contribute to resistance to CD20-targeting therapies by dampening the activity of effector T cells induced by these therapies. Tumor cells may increase PD-L1 expression in response to treatment, leading to T cell exhaustion and reduced efficacy of CD20-targeting therapies (173). Combining CD20-targeting therapies with immune checkpoint inhibitors is a potential strategy to overcome resistance and is currently tested in clinical trials, as discussed in more detail in section Combination therapies with CD20 immunotherapeutics.
Due to the multitude of resistance mechanisms, it is crucial to actively search for new methods that can increase the effectiveness of immunotherapy. Research efforts encompass the identification of novel therapeutic targets beyond CD20, the refinement of patient stratification, and the incorporation of combination therapeutic strategies. A recent review summarizes potential solutions to overcome resistance to CAR-T therapy (174).
Combination therapies with CD20 immunotherapeutics
To enhance their efficacy, anti-CD20 mAbs are commonly administered in combination with other drugs. One notable combination is the R-CHOP regimen, which integrates rituximab with cyclophosphamide, doxorubicin, vincristine, and prednisone, and has been extensively employed in treating patients with DLBCL and MCL. Similarly, R-pola-CHP (rituximab, polatuzumab, cyclophosphamide, doxorubicin) regimen is an approved treatment for advanced-stage DLBCL. Other regimens include a combination of rituximab, dexamethasone, high-dose cytarabine and a platinum-based agent (R-DHAP) used in the treatment of MCL, and the addition of lenalidomide to rituximab (R-lenalidomide) which has shown promising results, particularly in patients with r/r FL. Combinations such as bendamustine and rituximab (BR) and fludarabine, cyclophosphamide, and rituximab (FCR) have demonstrated efficacy in the treatment of CLL. Furthermore, novel combinations of rituximab with targeted agents have shown significant potential. Rituximab in combination with venetoclax, a BCL-2 inhibitor, as well as with idelalisib, a PI3K inhibitor, has been approved for the treatment of CLL. Furthermore, the R-GemOx regimen, which combines rituximab with gemcitabine and oxaliplatin, has exhibited notable efficacy in r/r B-NHL in phase 2 clinical trial (175), and is currently being compared to a similar regimen using glofitamab instead of rituximab (glofit-GemOx) in a phase 3 clinical trial (176). In the treatment of CD20+ B-ALL, rituximab is added to standard chemotherapy regimens in patients with a Philadelphia chromosome-negative (Ph-) B-ALL. In patients with Philadelphia chromosome-positive (Ph+) CD20+ B-ALL, rituximab is combined with chemotherapy and BCR-ABL1 tyrosine kinase inhibitors, such as imatinib and dasatinib.
Several clinical trials have investigated the efficacy of other combination therapies involving CD20-targeting in patients with B-NHLs. Among these trials, the combination of R-DHAP regimen with temsirolimus, an mTOR inhibitor, has shown encouraging results, demonstrating improved outcomes in patients with r/r DLBCL (47). Temsirolimus has also demonstrated effectiveness in combination with rituximab alone in patients with r/r MCL in phase 2 clinical trial (177). Moreover, checkpoint inhibitors are also being tested in phase 1 and 2 clinical trials in combination with anti-CD20 therapies. The addition of atezolizumab to an R-CHOP regimen in previously untreated DLBCL patients resulted in 77,5% CR (178). It is also tested in combination with BsAbs glofitamab and mosunetuzumab in phase 1/2 clinical trials in patients with NHL (NCT02500407, NCT03533283) (179). Additionally, pembrolizumab (anti-PD1 mAb) was evaluated in combination with rituximab in a single-arm phase 2 clinical trial and resulted in 67% ORR with 50% CR among patients with r/r FL (180).
Notably, drugs that are used in combinations with CD20-targeting immunotherapies may have a bidirectional impact on CD20 antigen expression and thus the effectiveness of these therapies. Prednisolone, a glucocorticosteroid present in many chemotherapeutic regimens, was shown to upregulate CD20 on some primary B-ALL samples in vitro (4). On the other hand, some drugs that are used together with anti-CD20 mAbs, such as BTK inhibitor ibrutinib, PI3Kδ inhibitor idelalisib, or SYK inhibitor dasatinib, were shown to downregulate CD20 and demonstrated inhibitory effects on cytotoxic effector cells (181–184). These drugs decreased the efficacy of anti-CD20 mAbs in vitro (183, 184). It may also be one of the reasons for the failure of an attempt to improve ibrutinib efficacy in CLL by the addition of rituximab, as demonstrated in a randomized clinical trial showing no improvement in PFS in the rituximab+ibrutinib group versus ibrutinib alone (185). This highlights the need for further research on the drug-induced changes in cellular signaling and related CD20 regulation. Understanding these relationships may be important for selecting the most effective therapies and improving therapeutic results. Interestingly, several classes of CD20-upregulating drugs were described, including aurora kinase inhibitors, FOXO1 inhibitors, and chromatin modulators, enabling the increase in anti-CD20-mAbs efficacy in preclinical settings (13). Combining these drugs with CD20-targeting therapies could be a potentially valuable strategy to overcome resistance, however, it requires further evaluation in a clinical setting.
Concluding remarks
A breakthrough in the treatment of B cell malignancies is evident with recent approvals of CD19 CAR-T cells and BsAbs, particularly those targeting CD20xCD3, offering effective treatment and potential cure for r/r patients. Over the past 25 years, CD20, an early target in immunotherapy, has demonstrated remarkable effectiveness. However, the widespread use of cytotoxic T cell-based therapies appeared with new challenges such as treatment-related complications and side effects. Effective management requires the accumulation of comprehensive knowledge and experience, including identifying risk factors for CRS, ICAN, and refining treatment guidelines. These improvements are crucial for the widespread use of these innovative drugs.
With diverse treatment modalities emerging, from naked mAbs to BsAbs and CAR-T cells, understanding determinants of activity and resistance mechanisms for the specific types of treatment are crucial for their optimal selection and clinical efficacy. Decent levels of CD20 are essential for the efficacy of all types of CD20-directed immunotherapies, however, recent preclinical reports emphasize that different types of anti-CD20 therapies require different amounts of CD20 protein on the cell surface to be effective. While a certain level of reduction in CD20 compromises the activity of anti-CD20 mAbs and BsAbs, it may still be adequate for the effectiveness of CD20 CAR-T cells (28). Although the CD20 CAR-T constructs currently being tested in the clinic show great efficacy, further refinements to the CD20 CAR constructs, including changes around the scFv sequence, have shown significant superiority in preclinical models and offer the prospect of even better outcomes for patients (186).
Key directions for CD20 immunotherapy improvement also include combination strategies with small molecule drugs and simultaneous targeting of multiple immunotherapy targets to enhance precision and minimize relapse risks. Simultaneous targeting of CD20 with other antigens like CD19 and CD22 demonstrates efficacy in preclinical models (154) and ongoing clinical trials. Noteworthy, a better understanding of the determinants of response and resistance will be critical for patient selection and future rational combinations.
Author contributions
AD: Conceptualization, Writing – original draft, Writing – review & editing, Visualization, Investigation. KD: Conceptualization, Writing – original draft, Writing – review & editing, Investigation. MF: Conceptualization, Investigation, Writing – original draft, Writing – review & editing, Funding acquisition, Supervision, Visualization.
Funding
The author(s) declare that financial support was received for the research, authorship, and/or publication of this article. This work was supported by the National Science Centre (Poland) grant: 2019/35/B/NZ5/01428 (MF).
Conflict of interest
The authors declare that the research was conducted in the absence of any commercial or financial relationships that could be construed as a potential conflict of interest.
Publisher’s note
All claims expressed in this article are solely those of the authors and do not necessarily represent those of their affiliated organizations, or those of the publisher, the editors and the reviewers. Any product that may be evaluated in this article, or claim that may be made by its manufacturer, is not guaranteed or endorsed by the publisher.
References
1. Sędek Ł, Bulsa J, Sonsala A, Twardoch M, Wieczorek M, Malinowska I, et al. The immunophenotypes of blast cells in B-cell precursor acute lymphoblastic leukemia: how different are they from their normal counterparts? Cytometry B Clin Cytom. (2014) 86:329–39. doi: 10.1002/cyto.b.v86.5
2. Thomas DA, O’Brien S, Jorgensen JL, Cortes J, Faderl S, Garcia-Manero G, et al. Prognostic significance of CD20 expression in adults with de novo precursor B-lineage acute lymphoblastic leukemia. Blood. (2009) 113:6330–7. doi: 10.1182/blood-2008-04-151860
3. Jeha S, Behm F, Pei D, Sandlund JT, Ribeiro RC, Razzouk BI, et al. Prognostic significance of CD20 expression in childhood B-cell precursor acute lymphoblastic leukemia. Blood. (2006) 108:3302–4. doi: 10.1182/blood-2006-04-016709
4. Dworzak MN, Schumich A, Printz D, Pötschger U, Husak Z, Attarbaschi A, et al. CD20 up-regulation in pediatric B-cell precursor acute lymphoblastic leukemia during induction treatment: setting the stage for anti-CD20 directed immunotherapy. Blood. (2008) 112:3982–8. doi: 10.1182/blood-2008-06-164129
5. Sędek Ł, Theunissen P, Sobral da Costa E, van der Sluijs-Gelling A, Mejstrikova E, Gaipa G, et al. Differential expression of CD73, CD86 and CD304 in normal vs. leukemic B-cell precursors and their utility as stable minimal residual disease markers in childhood B-cell precursor acute lymphoblastic leukemia. J Immunol Methods. (2019) 475:112429. doi: 10.1016/j.jim.2018.03.005
6. Worch J, Makarova O, Burkhardt B. Immunreconstitution and infectious complications after rituximab treatment in children and adolescents: what do we know and what can we learn from adults? Cancers. (2015) 7:305–28. doi: 10.3390/cancers7010305
7. Maury S, Chevret S, Thomas X, Heim D, Leguay T, Huguet F, et al. Rituximab in B-lineage adult acute lymphoblastic leukemia. New Engl J Med. (2016) 375:1044–53. doi: 10.1056/NEJMoa1605085
8. Klein C, Lammens A, Schäfer W, Georges G, Schwaiger M, Mössner E, et al. Epitope interactions of monoclonal antibodies targeting CD20 and their relationship to functional properties. MAbs. (2013) 5:22–33. doi: 10.4161/mabs.22771
9. Rougé L, Chiang N, Steffek M, Kugel C, Croll TI, Tam C, et al. Structure of CD20 in complex with the therapeutic monoclonal antibody rituximab. Science. (2020) 367:1224–30. doi: 10.1126/science.aaz9356
10. Kläsener K, Jellusova J, Andrieux G, Salzer U, Böhler C, Steiner SN, et al. CD20 as a gatekeeper of the resting state of human B cells. Proc Natl Acad Sci U S A. (2021) 118:e2021342118. doi: 10.1073/pnas.2021342118
11. Li H, Ayer LM, Lytton J, Deans JP. Store-operated cation entry mediated by CD20 in membrane rafts. J Biol Chem. (2003) 278:42427–34. doi: 10.1074/jbc.M308802200
12. Walshe CA, Beers SA, French RR, Chan CHT, Johnson PW, Packham GK, et al. Induction of cytosolic calcium flux by CD20 is dependent upon B Cell antigen receptor signaling. J Biol Chem. (2008) 283:16971–84. doi: 10.1074/jbc.M708459200
13. Pavlasova G, Mraz M. The regulation and function of CD20: an “enigma” of B-cell biology and targeted therapy. Haematologica. (2020) 105:1494–506. doi: 10.3324/haematol.2019.243543
14. Shimizu R, Kikuchi J, Wada T, Ozawa K, Kano Y, Furukawa Y. HDAC inhibitors augment cytotoxic activity of rituximab by upregulating CD20 expression on lymphoma cells. Leukemia. (2010) 24:1760–8. doi: 10.1038/leu.2010.157
15. Laidlaw BJ, Cyster JG. Transcriptional regulation of memory B cell differentiation. Nat Rev Immunol. (2021) 21:209–20. doi: 10.1038/s41577-020-00446-2
16. Himmelmann A, Riva A, Wilson GL, Lucas BP, Thevenin C, Kehrl JH. PU.1/Pip and basic helix loop helix zipper transcription factors interact with binding sites in the CD20 promoter to help confer lineage- and stage-specific expression of CD20 in B lymphocytes. Blood. (1997) 90:3984–95. doi: 10.1182/blood.V90.10.3984
17. Thévenin C, Lucas BP, Kozlow EJ, Kehrl JH. Cell type- and stage-specific expression of the CD20/B1 antigen correlates with the activity of a diverged octamer DNA motif present in its promoter. J Biol Chem. (1993) 268:5949–56. doi: 10.1016/S0021-9258(18)53411-6
18. Wojciechowski W, Li H, Marshall S, Dell’Agnola C, Espinoza-Delgado I. Enhanced expression of CD20 in human tumor B cells is controlled through ERK-dependent mechanisms. J Immunol. (2005) 174:7859–68. doi: 10.4049/jimmunol.174.12.7859
19. Słabicki M, Lee KS, Jethwa A, Sellner L, Sacco F, Walther T, et al. Dissection of CD20 regulation in lymphoma using RNAi. Leukemia. (2016) 30:2409–12. doi: 10.1038/leu.2016.230
20. Grzelak L, Roesch F, Vaysse A, Biton A, Legendre R, Porrot F, et al. IRF8 regulates efficacy of therapeutic anti-CD20 monoclonal antibodies. Eur J Immunol. (2022) 52:1648–61. doi: 10.1002/eji.202250037
21. Pyrzynska B, Dwojak M, Zerrouqi A, Morlino G, Zapala P, Miazek N, et al. FOXO1 promotes resistance of non-Hodgkin lymphomas to anti-CD20-based therapy. Oncoimmunology. (2018) 7:e1423183. doi: 10.1080/2162402X.2017.1423183
22. Kawabata KC, Ehata S, Komuro A, Takeuchi K, Miyazono K. TGF-β-induced apoptosis of B-cell lymphoma Ramos cells through reduction of MS4A1/CD20. Oncogene. (2013) 32:2096–106. doi: 10.1038/onc.2012.219
23. Seitz V, Butzhammer P, Hirsch B, Hecht J, Gütgemann I, Ehlers A, et al. Deep sequencing of MYC DNA-binding sites in Burkitt lymphoma. PLoS One. (2011) 6:e26837. doi: 10.1371/journal.pone.0026837
24. Yu D, Dews M, Park A, Tobias JW, Thomas-Tikhonenko A. Inactivation of Myc in murine two-hit B lymphomas causes dormancy with elevated levels of interleukin 10 receptor and CD20: implications for adjuvant therapies. Cancer Res. (2005) 65:5454–61. doi: 10.1158/0008-5472.CAN-04-4197
25. Tomita A, Hiraga J, Kiyoi H, Ninomiya M, Sugimoto T, Ito M, et al. Epigenetic regulation of CD20 protein expression in a novel B-cell lymphoma cell line, RRBL1, established from a patient treated repeatedly with rituximab-containing chemotherapy. Int J Hematol. (2007) 86:49–57. doi: 10.1532/IJH97.07028
26. Scialdone A, Hasni MS, Damm JK, Lennartsson A, Gullberg U, Drott K. The HDAC inhibitor valproate induces a bivalent status of the CD20 promoter in CLL patients suggesting distinct epigenetic regulation of CD20 expression in CLL in vivo. Oncotarget. (2017) 8:37409–22. doi: 10.18632/oncotarget.v8i23
27. Frys S, Simons Z, Hu Q, Barth MJ, Gu JJ, Mavis C, et al. Entinostat, a novel histone deacetylase inhibitor is active in B-cell lymphoma and enhances the anti-tumour activity of rituximab and chemotherapy agents. Br J Haematol. (2015) 169:506–19. doi: 10.1111/bjh.13318
28. Ang Z, Paruzzo L, Hayer KE, Schmidt C, Torres Diz M, Xu F, et al. Alternative splicing of its 5′-UTR limits CD20 mRNA translation and enables resistance to CD20-directed immunotherapies. Blood. (2023) 142:1724–39. doi: 10.1182/blood.2023020400
29. Almagro JC, Daniels-Wells TR, Perez-Tapia SM, Penichet ML. Progress and challenges in the design and clinical development of antibodies for cancer therapy. Front Immunol. (2017) 8:1751. doi: 10.3389/fimmu.2017.01751
30. Fouda GE, Bavbek S. Rituximab hypersensitivity: from clinical presentation to management. Front Pharmacol. (2020) 11:572863. doi: 10.3389/fphar.2020.572863
31. Schieber T, Li A, Lundberg J, Wiczer T, Voorhees T. Successful obinutuzumab administration after rituximab discontinuation due to intolerance in patients with hematologic disorders. Blood Adv. (2023) 7:3431–4. doi: 10.1182/bloodadvances.2022009548
32. Ali SB, Yuson C, Hissaria P. Rituximab hypersensitivity reactions and tolerance of ofatumumab therapy. Clin Exp Rheumatol. (2021) 39:648–50. doi: 10.55563/clinexprheumatol/z3mbin
33. Coiffier B, Lepretre S, Pedersen LM, Gadeberg O, Fredriksen H, van Oers MHJ, et al. Safety and efficacy of ofatumumab, a fully human monoclonal anti-CD20 antibody, in patients with relapsed or refractory B-cell chronic lymphocytic leukemia: a phase 1-2 study. Blood. (2008) 111:1094–100. doi: 10.1182/blood-2007-09-111781
34. Ko S, Jo M, Jung ST. Recent achievements and challenges in prolonging the serum half-lives of therapeutic IgG antibodies through Fc engineering. BioDrugs. (2021) 35:147–57. doi: 10.1007/s40259-021-00471-0
35. Weiner GJ. Rituximab: mechanism of action. Semin Hematol. (2010) 47:115–23. doi: 10.1053/j.seminhematol.2010.01.011
36. Lim SH, Vaughan AT, Ashton-Key M, Williams EL, Dixon SV, Chan HTC, et al. Fc gamma receptor IIb on target B cells promotes rituximab internalization and reduces clinical efficacy. Blood. (2011) 118:2530–40. doi: 10.1182/blood-2011-01-330357
37. Deans JP, Li H, Polyak MJ. CD20-mediated apoptosis: signalling through lipid rafts. Immunology. (2002) 107:176–82. doi: 10.1046/j.1365-2567.2002.01495.x
38. Hamlin PA, Musteata V, Park SI, Burnett C, Dabovic K, Strack T, et al. Safety and efficacy of engineered toxin body MT-3724 in relapsed or refractory B-cell non-Hodgkin’s lymphomas and diffuse large B-cell lymphoma. Cancer Res Commun. (2022) 2:307–15. doi: 10.1158/2767-9764.CRC-22-0056
39. Kimball AS, Webb TJ. The roles of radiotherapy and immunotherapy for the treatment of lymphoma. Mol Cell Pharmacol. (2013) 5:27–38.
40. Grillo-López AJ, White CA, Dallaire BK, Varns CL, Shen CD, Wei A, et al. Rituximab: the first monoclonal antibody approved for the treatment of lymphoma. Curr Pharm Biotechnol. (2000) 1:1–9. doi: 10.2174/1389201003379059
41. Pierpont TM, Limper CB, Richards KL. Past, present, and future of rituximab-the world’s first oncology monoclonal antibody therapy. Front Oncol. (2018) 8:163. doi: 10.3389/fonc.2018.00163
42. Yelvington BJ. Subcutaneous rituximab in follicular lymphoma, chronic lymphocytic leukemia, and diffuse large B-cell lymphoma. J Adv Pract Oncol. (2018) 9:530–4.
43. Pérez-Callejo D, González-Rincón J, Sánchez A, Provencio M, Sánchez-Beato M. Action and resistance of monoclonal CD20 antibodies therapy in B-cell Non-Hodgkin Lymphomas. Cancer Treat Rev. (2015) 41:680–9. doi: 10.1016/j.ctrv.2015.05.007
44. Kennedy AD, Beum PV, Solga MD, DiLillo DJ, Lindorfer MA, Hess CE, et al. Rituximab infusion promotes rapid complement depletion and acute CD20 loss in chronic lymphocytic leukemia1. J Immunol. (2004) 172:3280–8. doi: 10.4049/jimmunol.172.5.3280
45. Leonard JP, Trneny M, Izutsu K, Fowler NH, Hong X, Zhu J, et al. AUGMENT: A phase III study of lenalidomide plus rituximab versus placebo plus rituximab in relapsed or refractory indolent lymphoma. J Clin Oncol. (2019) 37:1188–99. doi: 10.1200/JCO.19.00010
46. Johnston PB, LaPlant B, McPhail E, Habermann TM, Inwards DJ, Micallef IN, et al. Everolimus combined with R-CHOP-21 for new, untreated, diffuse large B-cell lymphoma (NCCTG 1085 [Alliance]): safety and efficacy results of a phase 1 and feasibility trial. Lancet Haematol. (2016) 3:e309–316. doi: 10.1016/S2352-3026(16)30040-0
47. Witzens-Harig M, Viardot A, Keller U, Wosniok J, Deuster O, Klemmer J, et al. The mTOR inhibitor temsirolimus added to rituximab combined with dexamethasone, cytarabine, and cisplatinum (R-DHAP) for the treatment of patients with relapsed or refractory DLBCL - results from the phase-II STORM trial. Hemasphere. (2021) 5:e636. doi: 10.1097/HS9.0000000000000636
48. Lin TS. Ofatumumab: a novel monoclonal anti-CD20 antibody. Pharmgenomics Pers Med. (2010) 3:51–9. doi: 10.2147/PGPM
49. Teeling JL, French RR, Cragg MS, van den Brakel J, Pluyter M, Huang H, et al. Characterization of new human CD20 monoclonal antibodies with potent cytolytic activity against non-Hodgkin lymphomas. Blood. (2004) 104:1793–800. doi: 10.1182/blood-2004-01-0039
50. Barth MJ, Mavis C, Czuczman MS, Hernandez-Ilizaliturri FJ. Ofatumumab exhibits enhanced in vitro and in vivo activity compared to rituximab in preclinical models of mantle cell lymphoma. Clin Cancer Res. (2015) 21:4391–7. doi: 10.1158/1078-0432.CCR-15-0056
51. Cleary KLS, Chan HTC, James S, Glennie MJ, Cragg MS. Antibody distance from the cell membrane regulates antibody effector mechanisms. J Immunol. (2017) 198:3999–4011. doi: 10.4049/jimmunol.1601473
52. Kumar A, Planchais C, Fronzes R, Mouquet H, Reyes N. Binding mechanisms of therapeutic antibodies to human CD20. Science. (2020) 369:793–9. doi: 10.1126/science.abb8008
53. Desikan SP, Keating MJ, Ferrajoli A, Jain N, Ohanian M, Pemmaraju N, et al. Early treatment with ofatumumab in patients with high-risk CLL. Blood. (2022) 140:9876–8. doi: 10.1182/blood-2022-167284
54. van Imhoff GW, McMillan A, Matasar MJ, Radford J, Ardeshna KM, Kuliczkowski K, et al. Ofatumumab versus rituximab salvage chemoimmunotherapy in relapsed or refractory diffuse large B-cell lymphoma: the ORCHARRD study. J Clin Oncol. (2017) 35:544–51. doi: 10.1200/JCO.2016.69.0198
55. Maloney DG, Ogura M, Fukuhara N, Davis J, Lasher J, Izquierdo M, et al. A phase 3 randomized study (HOMER) of ofatumumab vs rituximab in iNHL relapsed after rituximab-containing therapy. Blood Advances. (2020) 4:3886–93. doi: 10.1182/bloodadvances.2020001942
56. Jabbour E, Richard-Carpentier G, Sasaki Y, Konopleva M, Patel K, Roberts K, et al. Hyper-CVAD regimen in combination with ofatumumab as frontline therapy for adults with Philadelphia chromosome-negative B-cell acute lymphoblastic leukaemia: a single-arm, phase 2 trial. Lancet Haematol. (2020) 7:e523–33. doi: 10.1016/S2352-3026(20)30144-7
57. Byrd JC, Brown JR, O’Brien S, Barrientos JC, Kay NE, Reddy NM, et al. Ibrutinib versus ofatumumab in previously treated chronic lymphoid leukemia. N Engl J Med. (2014) 371:213–23. doi: 10.1056/NEJMoa1400376
58. Sandhu S, Mulligan SP. Ofatumumab and its role as immunotherapy in chronic lymphocytic leukemia. Haematologica. (2015) 100:411–4. doi: 10.3324/haematol.2015.124107
59. Flinn IW, Hillmen P, Montillo M, Nagy Z, Illés Á, Etienne G, et al. The phase 3 DUO trial: duvelisib vs ofatumumab in relapsed and refractory CLL/SLL. Blood. (2018) 132:2446–55. doi: 10.1182/blood-2018-05-850461
60. Mössner E, Brünker P, Moser S, Püntener U, Schmidt C, Herter S, et al. Increasing the efficacy of CD20 antibody therapy through the engineering of a new type II anti-CD20 antibody with enhanced direct and immune effector cell-mediated B-cell cytotoxicity. Blood. (2010) 115:4393–402. doi: 10.1182/blood-2009-06-225979
61. Ferrara C, Stuart F, Sondermann P, Brünker P, Umaña P. The carbohydrate at FcgammaRIIIa Asn-162. An element required for high affinity binding to non-fucosylated IgG glycoforms. J Biol Chem. (2006) 281:5032–6. doi: 10.1074/jbc.M510171200
62. Patz M, Isaeva P, Forcob N, Müller B, Frenzel LP, Wendtner CM, et al. Comparison of the in vitro effects of the anti-CD20 antibodies rituximab and GA101 on chronic lymphocytic leukaemia cells. Br J Haematol. (2011) 152:295–306. doi: 10.1111/j.1365-2141.2010.08428.x
63. Herter S, Herting F, Mundigl O, Waldhauer I, Weinzierl T, Fauti T, et al. Preclinical activity of the type II CD20 antibody GA101 (obinutuzumab) compared with rituximab and ofatumumab in vitro and in xenograft models. Mol Cancer Ther. (2013) 12:2031–42. doi: 10.1158/1535-7163.MCT-12-1182
64. Alduaij W, Ivanov A, Honeychurch J, Cheadle EJ, Potluri S, Lim SH, et al. Novel type II anti-CD20 monoclonal antibody (GA101) evokes homotypic adhesion and actin-dependent, lysosome-mediated cell death in B-cell Malignancies. Blood. (2011) 117:4519–29. doi: 10.1182/blood-2010-07-296913
65. Bologna L, Gotti E, Manganini M, Rambaldi A, Intermesoli T, Introna M, et al. Mechanism of action of type II, glycoengineered, anti-CD20 monoclonal antibody GA101 in B-chronic lymphocytic leukemia whole blood assays in comparison with rituximab and alemtuzumab. J Immunol. (2011) 186:3762–9. doi: 10.4049/jimmunol.1000303
66. Illidge T, Klein C, Sehn LH, Davies A, Salles G, Cartron G. Obinutuzumab in hematologic Malignancies: lessons learned to date. Cancer Treat Rev. (2015) 41:784–92. doi: 10.1016/j.ctrv.2015.07.003
67. Chu Y, Awasthi A, Lee S, Edani D, Yin C, Hochberg J, et al. Obinutuzumab (GA101) vs. rituximab significantly enhances cell death, antibody-dependent cytotoxicity and improves overall survival against CD20+ primary mediastinal B-cell lymphoma (PMBL) in a xenograft NOD-scid IL2Rgnull (NSG) mouse model: a potential targeted agent in the treatment of PMBL. Oncotarget. (2020) 11:3035–47. doi: 10.18632/oncotarget.v11i32
68. Goede V, Fischer K, Engelke A, Schlag R, Lepretre S, Montero LFC, et al. Obinutuzumab as frontline treatment of chronic lymphocytic leukemia: updated results of the CLL11 study. Leukemia. (2015) 29:1602–4. doi: 10.1038/leu.2015.14
69. Tobinai K, Klein C, Oya N, Fingerle-Rowson G. A review of obinutuzumab (GA101), a novel type II anti-CD20 monoclonal antibody, for the treatment of patients with B-cell Malignancies. Adv Ther. (2017) 34:324–56. doi: 10.1007/s12325-016-0451-1
70. Townsend W, Hiddemann W, Buske C, Cartron G, Cunningham D, Dyer MJS, et al. Obinutuzumab versus rituximab immunochemotherapy in previously untreated iNHL: final results from the GALLIUM study. Hemasphere. (2023) 7:e919. doi: 10.1097/HS9.0000000000000919
71. Le Gouill S, Ghesquières H, Oberic L, Morschhauser F, Tilly H, Ribrag V, et al. Obinutuzumab vs rituximab for advanced DLBCL: a PET-guided and randomized phase 3 study by LYSA. Blood. (2021) 137:2307–20. doi: 10.1182/blood.2020008750
72. Sehn LH, Martelli M, Trněný M, Liu W, Bolen CR, Knapp A, et al. A randomized, open-label, Phase III study of obinutuzumab or rituximab plus CHOP in patients with previously untreated diffuse large B-Cell lymphoma: final analysis of GOYA. J Hematol Oncol. (2020) 13:71. doi: 10.1186/s13045-020-00900-7
73. Freeman CL, Sehn LH. A tale of two antibodies: obinutuzumab versus rituximab. Br J Haematol. (2018) 182:29–45. doi: 10.1111/bjh.15232
74. Michot JM, Bouabdallah R, Vitolo U, Doorduijn JK, Salles G, Chiappella A, et al. Avadomide plus obinutuzumab in patients with relapsed or refractory B-cell non-Hodgkin lymphoma (CC-122-NHL-001): a multicentre, dose escalation and expansion phase 1 study. Lancet Haematol. (2020) 7:e649–59. doi: 10.1016/S2352-3026(20)30208-8
75. Cartron G, Watier H. Obinutuzumab: what is there to learn from clinical trials? Blood. (2017) 130:581–9. doi: 10.1182/blood-2017-03-771832
76. Witzig TE, Gordon LI, Cabanillas F, Czuczman MS, Emmanouilides C, Joyce R, et al. Randomized controlled trial of yttrium-90-labeled ibritumomab tiuxetan radioimmunotherapy versus rituximab immunotherapy for patients with relapsed or refractory low-grade, follicular, or transformed B-cell non-Hodgkin’s lymphoma. J Clin Oncol. (2002) 20:2453–63. doi: 10.1200/JCO.2002.11.076
77. Morschhauser F, Radford J, Van Hoof A, Vitolo U, Soubeyran P, Tilly H, et al. Phase III trial of consolidation therapy with yttrium-90-ibritumomab tiuxetan compared with no additional therapy after first remission in advanced follicular lymphoma. J Clin Oncol. (2008) 26:5156–64. doi: 10.1200/JCO.2008.17.2015
78. Sachpekidis C, Jackson DB, Soldatos TG. Radioimmunotherapy in non-Hodgkin’s lymphoma: retrospective adverse event profiling of Zevalin and Bexxar. Pharm (Basel). (2019) 12:141. doi: 10.3390/ph12040141
79. Kaminski MS, Tuck M, Estes J, Kolstad A, Ross CW, Zasadny K, et al. 131I-tositumomab therapy as initial treatment for follicular lymphoma. N Engl J Med. (2005) 352:441–9. doi: 10.1056/NEJMoa041511
80. Kaminski MS, Zelenetz AD, Press OW, Saleh M, Leonard J, Fehrenbacher L, et al. Pivotal study of iodine I 131 tositumomab for chemotherapy-refractory low-grade or transformed low-grade B-cell non-Hodgkin’s lymphomas. J Clin Oncol. (2001) 19:3918–28. doi: 10.1200/JCO.2001.19.19.3918
81. Iagaru A, Mittra ES, Ganjoo K, Knox SJ, Goris ML. 131I-Tositumomab (Bexxar) vs. 90Y-Ibritumomab (Zevalin) therapy of low-grade refractory/relapsed non-Hodgkin lymphoma. Mol Imaging Biol. (2010) 12:198–203. doi: 10.1007/s11307-009-0245-9
82. Green DJ, Press OW. Whither radioimmunotherapy: to be or not to be? Cancer Res. (2017) 77:2191–6. doi: 10.1158/0008-5472.CAN-16-2523
83. Chow VA, Rajendran JG, Fisher DR, Appelbaum FR, Cassaday RD, Martin PS, et al. A phase II trial evaluating the efficacy of high-dose Radioiodinated Tositumomab (Anti-CD20) antibody, etoposide and cyclophosphamide followed by autologous transplantation, for high-risk relapsed or refractory non-hodgkin lymphoma. Am J Hematol. (2020) 95:775–83. doi: 10.1002/ajh.25818
84. Shadman M, Li H, Rimsza L, Leonard JP, Kaminski MS, Braziel RM, et al. Continued excellent outcomes in previously untreated patients with follicular lymphoma after treatment with CHOP plus rituximab or CHOP plus 131I-tositumomab: long-term follow-up of phase III randomized study SWOG-S0016. J Clin Oncol. (2018) 36:697–703. doi: 10.1200/JCO.2017.74.5083
85. Schaefer NG, Huang P, Buchanan JW, Wahl RL. Radioimmunotherapy in non-Hodgkin lymphoma: opinions of nuclear medicine physicians and radiation oncologists. J Nucl Med. (2011) 52:830–8. doi: 10.2967/jnumed.110.085589
86. Chamarthy MR, Williams SC, Moadel RM. Radioimmunotherapy of non-Hodgkin’s lymphoma: from the “magic bullets” to “radioactive magic bullets.” Yale J Biol Med. (2011) 84:391–407.
87. Gazyvaro | European Medicines Agency (2023). Available online at: https://www.ema.europa.eu/en/medicines/human/EPAR/gazyvaro.
88. MabThera | European Medicines Agency (2023). Available online at: https://www.ema.europa.eu/en/medicines/human/EPAR/mabthera.
89. Lang DSP, Hagger C, Pearson A. Safety of rapid rituximab infusion in adult cancer patients: a systematic review. Int J Nurs Pract. (2011) 17:357–69. doi: 10.1111/j.1440-172X.2011.01950.x
90. Makatsori M, Kiani-Alikhan S, Manson AL, Verma N, Leandro M, Gurugama NP, et al. Hypogammaglobulinaemia after rituximab treatment-incidence and outcomes. QJM. (2014) 107:821–8. doi: 10.1093/qjmed/hcu094
91. Brinkmann U, Kontermann RE. The making of bispecific antibodies. mAbs. (2017) 9:182–212. doi: 10.1080/19420862.2016.1268307
92. Ma J, Mo Y, Tang M, Shen J, Qi Y, Zhao W, et al. Bispecific antibodies: from research to clinical application. Front Immunol. (2021) 12:626616. doi: 10.3389/fimmu.2021.626616
93. Sun Y, Yu X, Wang X, Yuan K, Wang G, Hu L, et al. Bispecific antibodies in cancer therapy: Target selection and regulatory requirements. Acta Pharm Sin B. (2023) 13:3583–97. doi: 10.1016/j.apsb.2023.05.023
94. Wu J, Fu J, Zhang M, Liu D. Blinatumomab: a bispecific T cell engager (BiTE) antibody against CD19/CD3 for refractory acute lymphoid leukemia. J Hematol Oncol. (2015) 8:104. doi: 10.1186/s13045-015-0195-4
95. Engelberts PJ, Hiemstra IH, de Jong B, Schuurhuis DH, Meesters J, Beltran Hernandez I, et al. DuoBody-CD3xCD20 induces potent T-cell-mediated killing of Malignant B cells in preclinical models and provides opportunities for subcutaneous dosing. EBioMedicine. (2020) 52:102625. doi: 10.1016/j.ebiom.2019.102625
96. van der Horst HJ, de Jonge AV, Hiemstra IH, Gelderloos AT, Berry DRAI, Hijmering NJ, et al. Epcoritamab induces potent anti-tumor activity against Malignant B-cells from patients with DLBCL, FL and MCL, irrespective of prior CD20 monoclonal antibody treatment. Blood Cancer J. (2021) 11:1–8. doi: 10.1038/s41408-021-00430-6
97. Mhibik M, Gaglione EM, Eik D, Herrick J, Le J, Ahn IE, et al. Cytotoxicity of the CD3×CD20 bispecific antibody epcoritamab in CLL is increased by concurrent BTK or BCL-2 targeting. Blood Advances. (2023) 7:4089–101. doi: 10.1182/bloodadvances.2022009517
98. Hutchings M, Mous R, Clausen MR, Johnson P, Linton KM, Chamuleau MED, et al. Dose escalation of subcutaneous epcoritamab in patients with relapsed or refractory B-cell non-Hodgkin lymphoma: an open-label, phase 1/2 study. Lancet. (2021) 398:1157–69. doi: 10.1016/S0140-6736(21)00889-8
99. Kater AP, Christensen JH, Bentzen HH, Niemann CU, Hutchings M, Chen J, et al. Subcutaneous epcoritamab in patients with relapsed/refractory chronic lymphocytic leukemia: preliminary results from the epcore CLL-1 trial. Blood. (2021) 138:2627. doi: 10.1182/blood-2021-146563
100. Research C for DE and. FDA grants accelerated approval to epcoritamab-bysp for relapsed or refractory diffuse large B-cell lymphoma and high-grade B-cell lymphoma. FDA (2023). Available at: https://www.fda.gov/drugs/drug-approvals-and-databases/fda-grants-accelerated-approval-epcoritamab-bysp-relapsed-or-refractory-diffuse-large-b-cell.
101. Tepkinly | European Medicines Agency (2023). Available online at: https://www.ema.europa.eu/en/medicines/human/EPAR/tepkinly.
102. Smith EJ, Olson K, Haber LJ, Varghese B, Duramad P, Tustian AD, et al. A novel, native-format bispecific antibody triggering T-cell killing of B-cells is robustly active in mouse tumor models and cynomolgus monkeys. Sci Rep. (2015) 5:17943. doi: 10.1038/srep17943
103. Zhu M, Olson K, Kirshner JR, Khaksar Toroghi M, Yan H, Haber L, et al. Translational findings for odronextamab: From preclinical research to a first-in-human study in patients with CD20+ B-cell Malignancies. Clin Trans Science. (2022) 15:954–66. doi: 10.1111/cts.13212
104. Bannerji R, Arnason JE, Advani RH, Brown JR, Allan JN, Ansell SM, et al. Odronextamab, a human CD20×CD3 bispecific antibody in patients with CD20-positive B-cell Malignancies (ELM-1): results from the relapsed or refractory non-Hodgkin lymphoma cohort in a single-arm, multicentre, phase 1 trial. Lancet Haematol. (2022) 9:e327–39. doi: 10.1016/S2352-3026(22)00072-2
105. Weinstock M, Elavalakanar P, Bright S, Ambati SR, Brouwer-Visser J, Pourpe S, et al. Complete responses to odronextamab in two patients with diffuse large B-cell lymphoma refractory to chimeric antigen receptor T-cell therapy. Br J Haematol. (2022) 199:366–70. doi: 10.1111/bjh.18383
106. Odronextamab BLA for Treatment of Relapsed/Refractory Follicular Lymphoma (FL) and Diffuse Large B-cell Lymphoma (DLBCL) Accepted for FDA Priority Review. Regeneron Pharmaceuticals Inc (2023). Available at: https://investor.regeneron.com/news-releases/news-release-details/odronextamab-bla-treatment-relapsedrefractory-follicular/.
107. Odronextamab Receives EMA Filing Acceptance for Treatment of Relapsed/Refractory Follicular Lymphoma and Diffuse Large B-cell Lymphoma. Regeneron Pharmaceuticals Inc (2023). Available at: https://investor.regeneron.com/news-releases/news-release-details/odronextamab-receives-ema-filing-acceptance-treatment/.
108. Sun LL, Ellerman D, Mathieu M, Hristopoulos M, Chen X, Li Y, et al. Anti-CD20/CD3 T cell–dependent bispecific antibody for the treatment of B cell Malignancies. Sci Trans Med. (2015) 7:287ra70–287ra70. doi: 10.1126/scitranslmed.aaa4802
109. Spiess C, Merchant M, Huang A, Zheng Z, Yang NY, Peng J, et al. Bispecific antibodies with natural architecture produced by co-culture of bacteria expressing two distinct half-antibodies. Nat Biotechnol. (2013) 31:753–8. doi: 10.1038/nbt.2621
110. Budde LE, Sehn LH, Matasar M, Schuster SJ, Assouline S, Giri P, et al. Safety and efficacy of mosunetuzumab, a bispecific antibody, in patients with relapsed or refractory follicular lymphoma: a single-arm, multicentre, phase 2 study. Lancet Oncol. (2022) 23:1055–65. doi: 10.1016/S1470-2045(22)00335-7
111. Bartlett NL, Assouline S, Giri P, Schuster SJ, Cheah CY, Matasar M, et al. Mosunetuzumab monotherapy is active and tolerable in patients with relapsed/refractory diffuse large B-cell lymphoma. Blood Advances. (2023) 7:4926–35. doi: 10.1182/bloodadvances.2022009260
112. Budde LE, Olszewski AJ, Assouline S, Lossos IS, Diefenbach C, Kamdar M, et al. Mosunetuzumab with polatuzumab vedotin in relapsed or refractory aggressive large B cell lymphoma: a phase 1b/2 trial. Nat Med. (2023) 30:229–39. doi: 10.1038/s41591-023-02726-5
113. Lunsumio | European Medicines Agency (2023). Available online at: https://www.ema.europa.eu/en/medicines/human/EPAR/lunsumio#ema-inpage-item-overview.
114. Research C for DE and. FDA D.I.S.C.O. Burst Edition: FDA approval of Lunsumio (mosunetuzumab-axgb) for adult patients with relapsed or refractory follicular lymphoma after two or more lines of systemic therapy. FDA (2023). Available at: https://www.fda.gov/drugs/resources-information-approved-drugs/fda-disco-burst-edition-fda-approval-lunsumio-mosunetuzumab-axgb-adult-patients-relapsed-or.
115. Bacac M, Colombetti S, Herter S, Sam J, Perro M, Chen S, et al. CD20-TCB with obinutuzumab pretreatment as next-generation treatment of hematologic Malignancies. Clin Cancer Res. (2018) 24:4785–97. doi: 10.1158/1078-0432.CCR-18-0455
116. Hutchings M, Morschhauser F, Iacoboni G, Carlo-Stella C, Offner FC, Sureda A, et al. Glofitamab, a novel, bivalent CD20-targeting T-cell–engaging bispecific antibody, induces durable complete remissions in relapsed or refractory B-cell lymphoma: A phase I trial. JCO. (2021) 39:1959–70. doi: 10.1200/JCO.20.03175
117. Dickinson MJ, Carlo-Stella C, Morschhauser F, Bachy E, Corradini P, Iacoboni G, et al. Glofitamab for relapsed or refractory diffuse large B-cell lymphoma. New Engl J Med. (2022) 387:2220–31. doi: 10.1056/NEJMoa2206913
118. Ferhanoglu B, Gulbas Z, Uzay A, Özcan M, Ozkalemkas F, Dal MS, et al. Glofitamab in relapsed/refractory diffuse large B cell lymphoma: real world data. Blood. (2022) 140:6704–5. doi: 10.1182/blood-2022-165385
119. Dickinson M, Viardot A, Marks R, Topp MS, Morschhauser F, Jacobs B, et al. Glofitamab + Pola-R-CHP in patients with previously untreated diffuse large B-cell lymphoma (DLBCL): Results from a phase Ib study. JCO. (2023) 41:7549–9. doi: 10.1200/JCO.2023.41.16_suppl.7549
120. Research C for DE and. FDA grants accelerated approval to glofitamab-gxbm for selected relapsed or refractory large B-cell lymphomas. FDA (2023). Available at: https://www.fda.gov/drugs/drug-approvals-and-databases/fda-grants-accelerated-approval-glofitamab-gxbm-selected-relapsed-or-refractory-large-b-cell.
121. Columvi | European Medicines Agency (2023). Available online at: https://www.ema.europa.eu/en/medicines/human/EPAR/columvi#ema.
122. Baliga R, Li K, Manlusoc M, Hinton P, Ng D, Tran M, et al. High avidity IgM-based CD20xCD3 bispecific antibody (IGM-2323) for enhanced T-cell dependent killing with minimal cytokine release. Blood. (2019) 134:1574. doi: 10.1182/blood-2019-131650
123. Hernandez GH, So J, Logronio KA, Kotturi MF, Kim WS, Armand P, et al. Pharmacodynamics and biomarker correlates of imvotamab (IGM-2323), the first-in-class CD20xCD3 bispecific igM antibody with dual mechanisms of action, in patients with advanced B cell Malignancies. Blood. (2022) 140:6436–8. doi: 10.1182/blood-2022-163235
124. Hart KC, Logronio K, Li M, Yakkundi P, Manlusoc M, Li K, et al. Abstract 4179: High valency of IGM-2323, a CD20xCD3 IgM bispecific T cell engager, displaces rituximab binding and induces potent B lymphoma cell killing. Cancer Res. (2022) 82:4179. doi: 10.1158/1538-7445.AM2022-4179
125. Logronio KA, Joglekar M, Oyasu M, Yakkundi P, Leabman MK, Hernandez G, et al. Combination of imvotamab and loncastuximab tesirine shows enhanced anti-tumor activity in a preclinical model of non-Hodgkin’s lymphoma. Hematological Oncol. (2023) 41:552–3. doi: 10.1002/hon.3164_413
126. Budde E, Gopal AK, Kim WS, Flinn IW, Cheah CYY, Nastoupil L, et al. A phase 1 dose escalation study of Igm-2323, a novel anti-CD20 x anti-CD3 IgM T cell engager (TCE) in patients with advanced B-cell Malignancies. Blood. (2021) 138:132. doi: 10.1182/blood-2021-153355
127. Chu SY, Lee SH, Rashid R, Chen H, Chan EW, Phung S, et al. Immunotherapy with long-lived anti-CD20 × Anti-CD3 bispecific antibodies stimulates potent T cell-mediated killing of human B cell lines and of circulating and lymphoid B cells in monkeys: A potential therapy for B cell lymphomas and leukemias. Blood. (2014) 124:3111. doi: 10.1182/blood.V124.21.3111.3111
128. Moore GL, Bernett MJ, Rashid R, Pong EW, Nguyen DHT, Jacinto J, et al. A robust heterodimeric Fc platform engineered for efficient development of bispecific antibodies of multiple formats. Methods. (2019) 154:38–50. doi: 10.1016/j.ymeth.2018.10.006
129. Patel K, Michot JM, Chanan-Khan A, Ghesquieres H, Bouabdallah K, Byrd JC, et al. Safety and anti-tumor activity of plamotamab (XmAb13676), an anti-CD20 x anti-CD3 bispecific antibody, in subjects with relapsed/refractory non-Hodgkin’s lymphoma. Blood. (2021) 138:2494. doi: 10.1182/blood-2021-144350
130. Patel K, Riedell PA, Tilly H, Ahmed S, Michot JM, Ghesquieres H, et al. A phase 1 study of plamotamab, an anti-CD20 x anti-CD3 bispecific antibody, in patients with relapsed/refractory non-Hodgkin’s lymphoma: recommended dose safety/efficacy update and escalation exposure-response analysis. Blood. (2022) 140:9470–2. doi: 10.1182/blood-2022-159586
131. Kellner C, Hallack D, Glorius P, Staudinger M, Mohseni Nodehi S, de Weers M, et al. Fusion proteins between ligands for NKG2D and CD20-directed single-chain variable fragments sensitize lymphoma cells for natural killer cell-mediated lysis and enhance antibody-dependent cellular cytotoxicity. Leukemia. (2012) 26:830–4. doi: 10.1038/leu.2011.288
132. Lutz S, Klausz K, Albici AM, Ebinger L, Sellmer L, Teipel H, et al. Novel NKG2D-directed bispecific antibodies enhance antibody-mediated killing of Malignant B cells by NK cells and T cells. Front Immunol. (2023) 14:1227572. doi: 10.3389/fimmu.2023.1227572
133. Nalivaiko K, Hofmann M, Kober K, Teichweyde N, Krammer PH, Rammensee HG, et al. A recombinant bispecific CD20×CD95 antibody with superior activity against normal and Malignant B-cells. Mol Ther. (2016) 24:298–305. doi: 10.1038/mt.2015.209
134. Hörner S, Moustafa-Oglou M, Teppert K, Hagelstein I, Kauer J, Pflügler M, et al. IgG-based bispecific anti-CD95 antibodies for the treatment of B cell-derived Malignancies and autoimmune diseases. Cancers. (2022) 14:3941. doi: 10.3390/cancers14163941
135. Brandl M, Große-Hovest L, Holler E, Kolb HJ, Jung G. Bispecific antibody fragments with CD20 × CD28 specificity allow effective autologous and allogeneic T-cell activation against Malignant cells in peripheral blood and bone marrow cultures from patients with B-cell lineage leukemia and lymphoma. Exp Hematol. (1999) 27:1264–70. doi: 10.1016/S0301-472X(99)00072-7
136. Otz T, Große-Hovest L, Hofmann M, Rammensee HG, Jung G. A bispecific single-chain antibody that mediates target cell-restricted, supra-agonistic CD28 stimulation and killing of lymphoma cells. Leukemia. (2009) 23:71–7. doi: 10.1038/leu.2008.271
137. Dickinson M, Carlo-Stella C, Morschhauser F, Bachy E, Corradini P, Iacoboni G, et al. Glofitamab in patients with relapsed/refractory (R/R) diffuse large B-cell lymphoma (DLBCL) and ≥ 2 prior therapies: Pivotal phase II expansion results. JCO. (2022) 40:7500–0. doi: 10.1200/JCO.2022.40.16_suppl.7500
138. Labrijn AF, Janmaat ML, Reichert JM, Parren PWHI. Bispecific antibodies: a mechanistic review of the pipeline. Nat Rev Drug Discovery. (2019) 18:585–608. doi: 10.1038/s41573-019-0028-1
139. Schuster SJ, Bartlett NL, Assouline S, Yoon SS, Bosch F, Sehn LH, et al. Mosunetuzumab induces complete remissions in poor prognosis non-Hodgkin lymphoma patients, including those who are resistant to or relapsing after chimeric antigen receptor T-cell (CAR-T) therapies, and is active in treatment through multiple lines. Blood. (2019) 134:6. doi: 10.1182/blood-2019-123742
140. Thieblemont C, Phillips T, Ghesquieres H, Cheah CY, Clausen MR, Cunningham D, et al. Epcoritamab, a novel, subcutaneous CD3xCD20 bispecific T-cell–engaging antibody, in relapsed or refractory large B-cell lymphoma: dose expansion in a phase I/II trial. JCO. (2023) 41:2238–47. doi: 10.1200/JCO.22.01725
141. Budde LE, Assouline S, Sehn LH, Schuster SJ, Yoon SS, Yoon DH, et al. Single-agent mosunetuzumab shows durable complete responses in patients with relapsed or refractory B-cell lymphomas: phase I dose-escalation study. JCO. (2022) 40:481–91. doi: 10.1200/JCO.21.00931
142. Budde LE, Olszewski AJ, Assouline S, Lossos IS, Diefenbach C, Kamdar M, et al. Mosunetuzumab plus polatuzumab vedotin demonstrates a favorable safety profile and efficacy in patients (Pts) with relapsed or refractory (R/R) large B-cell lymphoma (LBCL): primary analysis of a phase Ib/II study. Blood. (2023) 142:613. doi: 10.1182/blood-2023-174209
143. Falchi L, Carlo-Stella C, Morschhauser F, Hutchings M, Bachy E, Cartron G, et al. Glofitamab monotherapy in pts with relapsed/refractory (R/R) large B-cell lymphoma (LBCL): Extended follow-up and landmark analyses from a pivotal phase II study. JCO. (2023) 41:7550–0. doi: 10.1200/JCO.2023.41.16_suppl.7550
144. Marhelava K, Krawczyk M, Firczuk M, Fidyt K. CAR-T cells shoot for new targets: novel approaches to boost adoptive cell therapy for B cell-derived Malignancies. Cells. (2022) 11(11):1804. doi: 10.3390/cells11111804
145. Ruella M, Korell F, Porazzi P, Maus MV. Mechanisms of resistance to chimeric antigen receptor-T cells in haematological Malignancies. Nat Rev Drug Discovery. (2023) 22:976–95. doi: 10.1038/s41573-023-00807-1
146. Till BG, Jensen MC, Wang J, Qian X, Gopal AK, Maloney DG, et al. CD20-specific adoptive immunotherapy for lymphoma using a chimeric antigen receptor with both CD28 and 4-1BB domains: pilot clinical trial results. Blood. (2012) 119:3940–50. doi: 10.1182/blood-2011-10-387969
147. Majzner RG, Mackall CL. Tumor antigen escape from CAR T-cell therapy. Cancer Discovery. (2018) 8:1219–26. doi: 10.1158/2159-8290.CD-18-0442
148. Tan Su Yin E, Hu YX, Huang H. The breakthrough and the future: CD20 chimeric antigen receptor T-cell therapy for hematologic Malignancies. ImmunoMedicine. (2022) 2:e1039. doi: 10.1002/imed.1039
149. Schneider D, Xiong Y, Wu D, Nölle V, Schmitz S, Haso W, et al. A tandem CD19/CD20 CAR lentiviral vector drives on-target and off-target antigen modulation in leukemia cell lines. J Immunother Cancer. (2017) 5:42. doi: 10.1186/s40425-017-0246-1
150. Tong C, Zhang Y, Liu Y, Ji X, Zhang W, Guo Y, et al. Optimized tandem CD19/CD20 CAR-engineered T cells in refractory/relapsed B-cell lymphoma. Blood. (2020) 136:1632–44. doi: 10.1182/blood.2020005278
151. Zhang Y, Wang Y, Liu Y, Tong C, Wang C, Guo Y, et al. Long-term activity of tandem CD19/CD20 CAR therapy in refractory/relapsed B-cell lymphoma: a single-arm, phase 1-2 trial. Leukemia. (2022) 36:189–96. doi: 10.1038/s41375-021-01345-8
152. Larson SM, Walthers CM, Ji B, Ghafouri SN, Naparstek J, Trent J, et al. CD19/CD20 bispecific chimeric antigen receptor (CAR) in naive/memory T cells for the treatment of relapsed or refractory non-Hodgkin lymphoma. Cancer Discovery. (2023) 13:580–97. doi: 10.1158/2159-8290.CD-22-0964
153. Sang W, Shi M, Yang J, Cao J, Xu L, Yan D, et al. Phase II trial of co-administration of CD19- and CD20-targeted chimeric antigen receptor T cells for relapsed and refractory diffuse large B cell lymphoma. Cancer Med. (2020) 9:5827–38. doi: 10.1002/cam4.3259
154. Fousek K, Watanabe J, Joseph SK, George A, An X, Byrd TT, et al. CAR T-cells that target acute B-lineage leukemia irrespective of CD19 expression. Leukemia. (2021) 35:75–89. doi: 10.1038/s41375-020-0792-2
155. Brouwer-Visser J, Fiaschi N, Deering RP, Dhanik A, Cygan KJ, Zhang W, et al. Baseline biomarkers of T-cell function correlate with clinical responses to odronextamab (REGN1979), and loss of CD20 target antigen expression identified as a mechanism of treatment resistance. Blood. (2020) 136:10–1. doi: 10.1182/blood-2020-137499
156. Schuster SJ, Huw LY, Bolen CR, Assouline SE, Bartlett NL, Budde LE, et al. Characterization of CD20 expression loss as a mechanism of resistance to mosunetuzumab in patients with relapsed/refractory B-cell non-Hodgkin lymphomas. JCO. (2022) 40:7526–6. doi: 10.1200/JCO.2022.40.16_suppl.7526
157. Reinert J, Beitzen-Heineke A, Wethmar K, Stelljes M, Fiedler W, Schwartz S. Loss of CD22 expression and expansion of a CD22dim subpopulation in adults with relapsed/refractory B-lymphoblastic leukaemia after treatment with Inotuzumab-Ozogamicin. Ann Hematol. (2021) 100:2727–32. doi: 10.1007/s00277-021-04601-0
158. Liu SQ, Grantham A, Landry C, Granda B, Chopra R, Chakravarthy S, et al. A CRISPR screen reveals resistance mechanisms to CD3-bispecific antibody therapy. Cancer Immunol Res. (2021) 9:34–49. doi: 10.1158/2326-6066.CIR-20-0080
159. Beers SA, French RR, Chan HTC, Lim SH, Jarrett TC, Vidal RM, et al. Antigenic modulation limits the efficacy of anti-CD20 antibodies: implications for antibody selection. Blood. (2010) 115:5191–201. doi: 10.1182/blood-2010-01-263533
160. Beum PV, Peek EM, Lindorfer MA, Beurskens FJ, Engelberts PJ, Parren PWHI, et al. Loss of CD20 and bound CD20 antibody from opsonized B cells occurs more rapidly because of trogocytosis mediated by fc receptor-expressing effector cells than direct internalization by the B cells. J Immunol. (2011) 187:3438–47. doi: 10.4049/jimmunol.1101189
161. Taylor RP, Lindorfer MA. Fcγ-receptor–mediated trogocytosis impacts mAb-based therapies: historical precedence and recent developments. Blood. (2015) 125:762–6. doi: 10.1182/blood-2014-10-569244
162. Golay J, Zaffaroni L, Vaccari T, Lazzari M, Borleri GM, Bernasconi S, et al. Biologic response of B lymphoma cells to anti-CD20 monoclonal antibody rituximab in vitro: CD55 and CD59 regulate complement-mediated cell lysis. Blood. (2000) 95:3900–8. doi: 10.1182/blood.V95.12.3900
163. Li J, Zhu Z, Zhu Y, Li J, Li K, Zhong W. METTL3-mediated m6A methylation of C1qA regulates the Rituximab resistance of diffuse large B-cell lymphoma cells. Cell Death Discovery. (2023) 9:1–12. doi: 10.1038/s41420-023-01698-2
164. Lee SM, Min SW, Kwon HS, Bae GD, Jung JH, Park HI, et al. Effective clearance of rituximab-resistant tumor cells by breaking the mirror-symmetry of immunoglobulin G and simultaneous binding to CD55 and CD20. Sci Rep. (2023) 13:18275. doi: 10.1038/s41598-023-45491-8
165. Bowles JA, Weiner GJ. CD16 polymorphisms and NK activation induced by monoclonal antibody-coated target cells. J Immunol Methods. (2005) 304:88–99. doi: 10.1016/j.jim.2005.06.018
166. Cheney CM, Stephens DM, Mo X, Rafiq S, Butchar J, Flynn JM, et al. Ocaratuzumab, an Fc-engineered antibody demonstrates enhanced antibody-dependent cell-mediated cytotoxicity in chronic lymphocytic leukemia. MAbs. (2014) 6:749–55. doi: 10.4161/mabs.28282
167. Liu R, Oldham RJ, Teal E, Beers SA, Cragg MS. Fc-engineering for modulated effector functions-improving antibodies for cancer treatment. Antibodies (Basel). (2020) 9:64. doi: 10.3390/antib9040064
168. Arenas EJ, Martínez-Sabadell A, Rius Ruiz I, Román Alonso M, Escorihuela M, Luque A, et al. Acquired cancer cell resistance to T cell bispecific antibodies and CAR T targeting HER2 through JAK2 down-modulation. Nat Commun. (2021) 12:1237. doi: 10.1038/s41467-021-21445-4
169. Martínez-Sabadell A, Morancho B, Rius Ruiz I, Román Alonso M, Ovejero Romero P, Escorihuela M, et al. The target antigen determines the mechanism of acquired resistance to T cell-based therapies. Cell Rep. (2022) 41:111430. doi: 10.1016/j.celrep.2022.111430
170. Tie Y, Tang F, Wei Yq, Wei Xw. Immunosuppressive cells in cancer: mechanisms and potential therapeutic targets. J Hematol Oncol. (2022) 15:61. doi: 10.1186/s13045-022-01282-8
171. Silveira CRF, Corveloni AC, Caruso SR, Macêdo NA, Brussolo NM, Haddad F, et al. Cytokines as an important player in the context of CAR-T cell therapy for cancer: Their role in tumor immunomodulation, manufacture, and clinical implications. Front Immunol. (2022) 13:947648. doi: 10.3389/fimmu.2022.947648
172. Lykken JM, Horikawa M, Minard-Colin V, Kamata M, Miyagaki T, Poe JC, et al. Galectin-1 drives lymphoma CD20 immunotherapy resistance: validation of a preclinical system to identify resistance mechanisms. Blood. (2016) 127:1886–95. doi: 10.1182/blood-2015-11-681130
173. Xu-Monette ZY, Zhou J, Young KH. PD-1 expression and clinical PD-1 blockade in B-cell lymphomas. Blood. (2018) 131:68–83. doi: 10.1182/blood-2017-07-740993
174. Glover M, Avraamides S, Maher J. How can we engineer CAR T cells to overcome resistance? BTT. (2021) 15:175–98. doi: 10.2147/BTT.S252568
175. Mounier N, El Gnaoui T, Tilly H, Canioni D, Sebban C, Casasnovas RO, et al. Rituximab plus gemcitabine and oxaliplatin in patients with refractory/relapsed diffuse large B-cell lymphoma who are not candidates for high-dose therapy. A phase II Lymphoma Study Association trial. Haematologica. (2013) 98:1726–31. doi: 10.3324/haematol.2013.090597
176. Hertzberg M, Ku M, Catalani O, Althaus B, Simko S, Gregory GP. A phase III trial evaluating glofitamab in combination with gemcitabine plus oxaliplatin versus rituximab in combination with gemcitabine and oxaliplatin in patients with relapsed/refractory (R/R) diffuse large B-cell lymphoma (DLBCL). JCO. (2021) 39:TPS7575–TPS7575. doi: 10.1200/JCO.2021.39.15_suppl.TPS7575
177. Ansell SM, Tang H, Kurtin PJ, Koenig PA, Inwards DJ, Shah K, et al. Temsirolimus and rituximab in patients with relapsed or refractory mantle cell lymphoma: a phase 2 study. Lancet Oncol. (2011) 12:361–8. doi: 10.1016/S1470-2045(11)70062-6
178. Younes A, Burke JM, Cheson BD, Diefenbach CS, Ferrari S, Hahn UH, et al. Safety and efficacy of atezolizumab with rituximab and CHOP in previously untreated diffuse large B-cell lymphoma. Blood Adv. (2022) 7:1488–95. doi: 10.1182/bloodadvances.2022008344
179. Hutchings M, Gritti G, Sureda A, Terol MJ, Dyer MJ, Iacoboni G, et al. CD20-TCB, a novel T-cell-engaging bispecific antibody, can be safely combined with the anti-PD-L1 antibody atezolizumab in relapsed or refractory B-cell non-Hodgkin lymphoma. Blood. (2019) 134:2871. doi: 10.1182/blood-2019-123978
180. Nastoupil LJ, Chin CK, Westin JR, Fowler NH, Samaniego F, Cheng X, et al. Safety and activity of pembrolizumab in combination with rituximab in relapsed or refractory follicular lymphoma. Blood Adv. (2022) 6:1143–51. doi: 10.1182/bloodadvances.2021006240
181. Pavlasova G, Borsky M, Seda V, Cerna K, Osickova J, Doubek M, et al. Ibrutinib inhibits CD20 upregulation on CLL B cells mediated by the CXCR4/SDF-1 axis. Blood. (2016) 128:1609–13. doi: 10.1182/blood-2016-04-709519
182. Sandova V, Pavlasova GM, Seda V, Cerna KA, Sharma S, Palusova V, et al. IL4-STAT6 signaling induces CD20 in chronic lymphocytic leukemia and this axis is repressed by PI3Kδ inhibitor idelalisib. Haematologica. (2021) 106:2995–9. doi: 10.3324/haematol.2021.278644
183. Winiarska M, Bojarczuk K, Pyrzynska B, Bil J, Siernicka M, Dwojak M, et al. Inhibitors of SRC kinases impair antitumor activity of anti-CD20 monoclonal antibodies. MAbs. (2014) 6:1300–13. doi: 10.4161/mabs.32106
184. Bojarczuk K, Siernicka M, Dwojak M, Bobrowicz M, Pyrzynska B, Gaj P, et al. B-cell receptor pathway inhibitors affect CD20 levels and impair antitumor activity of anti-CD20 monoclonal antibodies. Leukemia. (2014) 28:1163–7. doi: 10.1038/leu.2014.12
185. Burger JA, Sivina M, Jain N, Kim E, Kadia T, Estrov Z, et al. Randomized trial of ibrutinib vs ibrutinib plus rituximab in patients with chronic lymphocytic leukemia. Blood. (2019) 133:1011–9. doi: 10.1182/blood-2018-10-879429
Keywords: CD20, B cell, leukemia, lymphoma, immunotherapy, monoclonal antibody, antibody-drug conjugate (ADC), CAR-T
Citation: Dabkowska A, Domka K and Firczuk M (2024) Advancements in cancer immunotherapies targeting CD20: from pioneering monoclonal antibodies to chimeric antigen receptor-modified T cells. Front. Immunol. 15:1363102. doi: 10.3389/fimmu.2024.1363102
Received: 29 December 2023; Accepted: 25 March 2024;
Published: 04 April 2024.
Edited by:
Alessandro Isidori, AORMN Hospital, ItalyReviewed by:
Gerardo Ferrer, Josep Carreras Leukaemia Research Institute (IJC), SpainRoman H. Khadka, University of Pennsylvania, United States
Copyright © 2024 Dabkowska, Domka and Firczuk. This is an open-access article distributed under the terms of the Creative Commons Attribution License (CC BY). The use, distribution or reproduction in other forums is permitted, provided the original author(s) and the copyright owner(s) are credited and that the original publication in this journal is cited, in accordance with accepted academic practice. No use, distribution or reproduction is permitted which does not comply with these terms.
*Correspondence: Malgorzata Firczuk, bWZpcmN6dWtAaW1kaWsucGFuLnBs