- 1Department of Immunology, University Medical Center Hamburg-Eppendorf, Hamburg, Germany
- 2I. Department of Medicine, University Medical Center Hamburg-Eppendorf, Hamburg, Germany
- 3Department of General, Visceral and Thoracic Surgery, University Medical Center Hamburg-Eppendorf, Hamburg, Germany
An increase in the extracellular concentration of ATP as a consequence of cellular stress or cell death results in the activation of immune cells. To prevent inflammation, extracellular ATP is rapidly metabolized to adenosine, which deploys an anti-inflammatory signaling cascade upon binding to P1 receptors on immune cells. The ectonucleotidases necessary for the degradation of ATP and generation of adenosine are present on the cell membrane of many immune cells, and their expression is tightly regulated under conditions of inflammation. The discovery that extracellular vesicles (EVs) carry purinergic enzyme activity has brought forward the concept of EVs as a new player in immune regulation. Adenosine-generating EVs derived from cancer cells suppress the anti-tumor response, while EVs derived from immune or mesenchymal stem cells contribute to the restoration of homeostasis after infection. Here we will review the existing knowledge on EVs containing purinergic enzymes and molecules, and discuss the relevance of these EVs in immune modulation and their potential for therapy.
1 Introduction
The adaptive immune system reacts to infected and tumor cells by mounting a tailored response that involves the activation of cytotoxicity mechanisms for the killing of target cells, and the release of inflammatory cytokines that will recruit and activate other immune cells. The aftermath of this response can lead to inflammation, and activated effector cells are tightly controlled by regulatory T cells (Tregs), and by processes such as activation-induced cell death or fratricide. Inflammation, cellular stress and cell death promote the release of adenosine triphosphate (ATP) into the extracellular space, drastically increasing the concentration by several orders of magnitude to the 100 µM range. Extracellular ATP further stimulates immune cells by binding and activating P2 receptors, but can also be metabolized by purinergic enzymes to generate immune suppressive adenosine. The purinergic enzymes involved in the “canonical” degradation of ATP to adenosine are the ectonucleotidases CD39 and CD73. CD39 degrades ATP first to ADP and then further to AMP, and CD73 hydrolyzes AMP to adenosine, which then exerts anti-inflammatory effects by binding to and activating A2A adenosine receptors on immune cells (1). Also the concerted action of other enzymes, such as ENPP1 and alkaline phosphatases (AP) metabolize ATP to adenosine, while adenosine deaminase (ADA) reduces adenosine-mediated signaling by degrading adenosine to inosine (Figure 1). The immediate effects upon engagement of purinergic receptors together with the swift activity of the ectoenzymes degrading ATP and generating and degrading adenosine procures a highly efficient system for the regulation of ongoing immune responses.
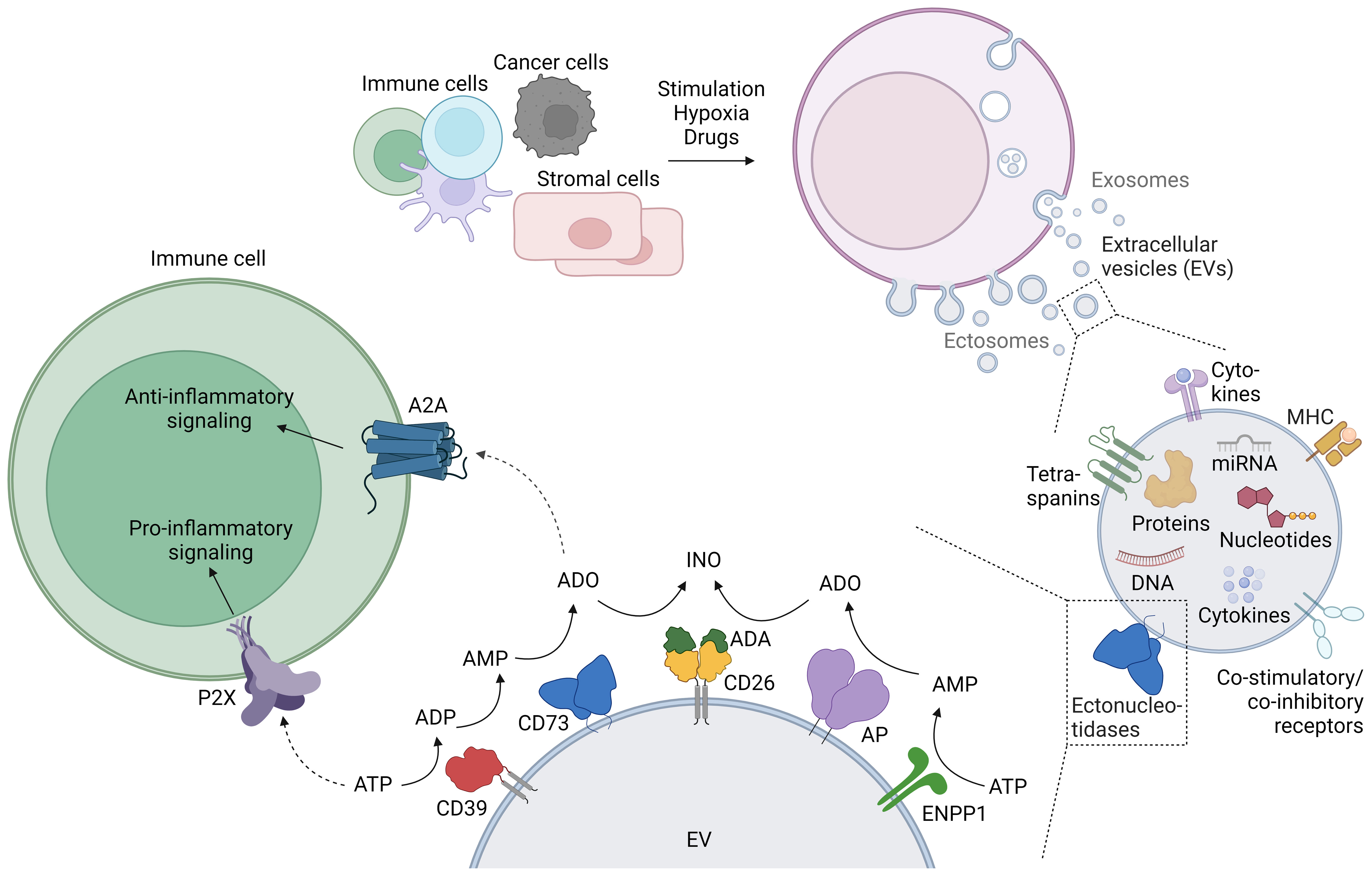
Figure 1 Generation of extracellular vesicles (EVs), their purinergic cargo, and the effect of EV-bound purinergic molecules on immune cells. The release of EVs is physiologic in most cells, and diverse stimuli such as activation or hypoxia enhance EV release. EVs contain a variety of cargo molecules, including purinergic enzymes (ectonucleotidases) at the membrane, and nucleotides enclosed within the lipid bilayer. The enzymatic activity of EV-bound ectonucleotidases can alter the concentration of extracellular adenine nucleotides, which can be sensed by immune cells. This figure was created with BioRender.com.
With a vast range of surface molecules and bioactivities as cargo, extracellular vesicles (EVs) constitute a powerful means of intercellular communication. EVs carry a broad variety of proteins, DNA, RNA and nucleotides on their surface or inside the EV lipid bilayer. This cargo can be transported and exert its function on cells that are located at significant distances from the donor cells. Importantly, inflammation and the tumor environment foster the release of EVs and influence the mechanism of vesicular release and their cargo. Consequently, it is not surprising that EVs can modulate the immune response by playing both pro-inflammatory and anti-inflammatory roles.
Many purinergic molecules are found in EVs. To name a few, the ectonucleotidases CD39, CD73, and ENPP1, APs, and also ADA and its ‘membrane anchor’ CD26. Moreover, both ATP and adenosine were found in EVs. In this review, we will exclusively focus on the immunoregulatory role of EVs containing molecules of the purinergic pathway. For the interested reader, excellent reviews covering the role of EVs in the immune system (2) and the interplay between purinergic signaling and EVs (3) have been recently published.
2 Purinergic enzymes and metabolites in extracellular vesicles
EVs are small lipid bilayer particles released by almost all cell types under physiological and pathological conditions. The term EVs comprises different types of vesicles, broadly subdivided into exosomes and ectosomes (Figure 1). Exosomes are usually small vesicles (30 – 150 nm) of endosomal origin. They are generated by inward budding of the cell membrane, subsequent formation of multivesicular bodies (MVBs), and release through fusion with the plasma membrane. Ectosome is the generic term for EVs generated by vesicle budding from the plasma membrane. Ectosomes include a variety of vesicles, e.g. microvesicles and apoptotic bodies, and consequently vary greatly in size (100 nm – 2 µm). There are many more types of vesicles, sometimes named after their cell of origin (e.g. oncosomes are ectosomes derived from tumor cells), or their location of release (e.g. trans-synaptic vesicles are released during the formation of the immune synapse), reflecting the huge diversity of EVs (2, 4). Membrane or membrane-associated proteins such as tetraspanins (CD9, CD63, CD81) and Flotillin are typical markers for EVs, although not specific for a certain type of EV. LAMP-1, TSG-101, and Alix are rather related to vesicles derived from the endosomal pathway, and can be used to distinguish exosomes from ectosomes (5). Although EV production by cells occurs under steady state conditions, cell stimulation massively increases EV release: Immune cells release EVs upon activation (6, 7) or light exposure (8). Cancer cells release EVs under hypoxic conditions, photodynamic treatment, chemotherapy, high cytosolic Ca2+ concentrations, treatment with cytokines, and stimulation with ATP (9–11). Cytokines, hypoxia and drugs trigger EV release by other cells (e.g. adipocytes, HEK cells) (12). In summary, the release of EVs with different sizes and origins is a physiological occurrence that is enhanced under activation and cellular stress.
The membrane proteins and molecular cargo of the EVs often mirror their cells of origin. In addition to the omnipresent tetraspanins, proteins decorating the surface of the EVs include adhesion molecules, major histocompatibility complex (MHC) proteins (13), immune receptors (14), cytokines (15) and enzymes, among them ectonucleotidases and proteases (2). These proteins often form functional units such as the synaptosome (16) or antigen presentation modules capable of eliciting T cell responses (13). Inside the lipid bilayer, EVs contain cytokines and growth factors (17), cytoskeleton components and enzymes, RNA (including micro RNA and long non-coding RNA) (18, 19), DNA (20), telomeres (7), and nucleotides (21–23) (Figure 1).
In 2007, the finding of CD39 and CD73 on the cell membrane of murine Tregs and the generation of immune suppressive adenosine led Deaglio and colleagues to propose a novel mechanism of T cell suppression by Tregs (24). Subsequent reports of tumor- and Treg-derived exosomes expressing CD39 and CD73, which were capable of generating adenosine and immune suppression pioneered research on EVs and purinergic enzymes (25, 26).
The AMPase CD73 is often present in modulatory EVs (Table 1). The nature of CD73 as lipid raft-associated, GPI-anchored protein seems to favor a release in vesicles, as EV formation is associated with lipid rafts (53). Therefore, CD73 is even mentioned as EV marker in the “Minimal Information for Studies of Extracellular Vesicles” (MISEV) guidelines (54). Mesenchymal stem cells (MSC) and tumor cells express high levels of CD73, and this is reflected in the corresponding EVs. CD39 is found in EVs derived from acute myeloid leukemia (AML), myeloma, and some solid tumors. Murine Treg-derived EVs harbor both CD39 and CD73, while human Tregs, with very low CD73 on their cell surface, produce EVs with CD39, but no CD73. Because both enzymes are required for the generation of adenosine, it is often the case that one of the enzymatic activities is provided in trans, by neighboring cells. For instance, CD73+ EVs rely on the presence of CD39 on the membrane of activated (and sometimes exhausted) T cells to generate adenosine (33), and the suppressive function of human Tregs is lower if responder cells are CD73-negative (31).
Beyond CD39 and CD73, other ectonucleotidases such as CD38, ectonucleotide pyrophosphatase/phosphodiesterase 1 (ENPP1/CD203a), alkaline phosphatases (AP), and CD26, as well as purinergic P2 receptors are present on EVs (Table 1). CD38 degrades nicotinamide adenine dinucleotide (NAD+) to the second messengers ADP-ribose, cyclic ADP-ribose, and NAADP, which modulate the immune response by intracellular Ca2+ mobilization from the endoplasmic reticulum (55, 56). CD38 was found on EVs derived from the plasma or bone marrow of patients with multiple myeloma and neuroblastoma, and on EVs from lymphoblastoid B cells (49, 50, 57, 58). ADP-ribose, generated by CD38, can be further metabolized to AMP by ENPP1 (59). In addition, ENPP1 degrades ATP and the STING agonist 2’3’-cGAMP, which is why ENPP1 is considered a modulator of the type I interferon pathway (60). ENPP1-containing EVs were isolated from the bone marrow of patients with multiple myeloma (49). Alkaline phosphatases (APs) are a class of enzymes with broad substrate specificity that dephosphorylate a variety of targets including ATP, ADP and AMP. AP-containing EVs have been found for example in the blood and placenta of pregnant women (61, 62). CD26 is a membrane-bound protein with own enzymatic activity (dipeptidylpeptidase) that serves as an anchor for ADA, the enzyme degrading adenosine to inosine. Its presence on EVs, as shown on tubular epithelial cell-derived EVs after ischemia-reperfusion injury (63), could ‘catch’ soluble ADA and bring it in proximity to target cells. A potential immune modulatory effect of EVs containing these ‘non-canonical’ ectonucleotidases has only rarely been investigated. Notably, stimulation of the P2X7 receptor with ATP induces the release of EVs from dendritic cells (DCs), microglia and tumor cells (52, 64–66). ATP-induced EVs from DCs contain IL-1β as well as the P2X7 receptor itself. Subsequent exposure of the vesicles to ATP results in a P2X7-dependent lysis of the vesicles and the release of cytokines (52).
There are reports of ´soluble´ ENPP1 (67), CD26 (68), CD73, AP, ADA (69, 70), or corresponding enzymatic activities in different body fluids without having specifically differentiated between EVs and the soluble forms of these proteins. Because the EV purification methodology has only recently been standardized, the immune regulatory potential of EVs carrying purinergic enzymes and metabolites is likely higher than the current state of research might suggest. Future studies need to clarify the origin of ´soluble´ purinergic molecules and their role in inflammation.
3 Extracellular vesicles as modifiers of cell functions
EVs may be taken up by the target cell by endocytosis or phagocytosis and their content released intracellularly. The molecules delivered by the EVs can modulate cellular functions. Until now, it is not clear to which extent recipient cells take up the cargo of EVs, and how relevant this is for the function of the recipient cell. For many years, the transfer of miRNA and subsequent gene suppression through EVs were seen as a main feature of EV-cell interaction, however, recent publications show that EV uptake is rather a low yield process (71), and that also the transfer of miRNA is low (72). In contrast, vesicular telomere transfer in the immunological synapse occurs very efficiently upon interaction of T cells and antigen-presenting cells, leading to the elongation of telomeres in the T cells and promoting long-term immunological memory (7). It is not yet clear whether the transfer of EV cargo is targeted. In the case of miRNAs, distinct nucleotide motifs determine either secretion in EVs or cellular retention. This indicates an ‘active’ decision of miRNA sorting into EVs (73). By analogy, ATP or adenosine contained in EVs may be released into the cytoplasm of the target cell. As the intracellular concentration of these nucleotides is much higher than in the extracellular space, EV-derived nucleotides will unlikely alter the concentration significantly. Alternatively, adenine nucleotides can be released from EVs in proximity to cells, i.e. adenosine released by cancer EVs reduces cytotoxic T cell perforin secretion, potentially contributing to tumor growth (21).
For EV-bound ectonucleotidases and other membrane proteins that have their active site facing the extracellular space, fusion of the EVs with the target cells is not necessary to exert their function. Proteins in the EV membrane can interact with the target cell, modulating cellular functions. For example, PD-L1 on tumor-derived EVs decreases the proliferation of PD-1-expressing tumor-infiltrating CD8 T cells (74). Additionally, EVs can present antigens, which leads to the activation of immune cells (13, 75). Ectonucleotidases such as CD39, CD73, ENPP1, and APs on EVs metabolize adenine nucleotides in proximity to a target cell without requiring a direct EV-cell contact, i.e. enabling the activation of adenosine receptor signaling on T cells. Considering the extremely short half-life of adenosine of only five to ten seconds (76), the enzymatic activity of EV-bound ectonucleotidases might be essential for immune regulation as it facilitates the production of anti-inflammatory adenosine in proximity to immune cells lacking e.g. CD73 expression. In contrast to soluble enzymes, EV-bound ectonucleotidases could be “attracted” to target cells by EV-cell-interactions and may be more stable, which allows a longer time in circulation. Research to determine the stability and longevity of EVs in vivo is still ongoing: When injecting EVs into mice, EVs still could be found after nine days in the lung, liver, and spleen (77), while in another study the EVs were eliminated after six hours (78). In a mouse model with a continuous generation of labeled tumor-EVs in vivo, EVs were found 35 days after tumor implantation in almost all organs (79), and breast milk-derived EVs survive circulation through the gastrointestinal tract and reached target organs with miRNA cargo (80). These data show that EVs can travel in the body and that their life span is long enough to reach different organs in the mouse.
4 Control of the immune response by extracellular vesicles with purinergic activity
Adenosine binding to the A2A receptor on immune cells modulates antigen presentation, inhibits the activation of T and B cells, suppresses cytokine production, and promotes the generation of Tregs. Such immune regulatory mechanisms help to prevent excessive or prolonged immune responses, which, if not controlled, could lead to tissue damage and autoimmunity. This a priori beneficial immune modulatory function has the downside of a potentially premature termination of an immune response against persistent viral antigens and tumors (81).
Adenosine has an extremely short half-life of under 10 seconds. Therefore, the availability of extracellular adenosine depends on the activity of the purinergic enzymes that metabolize ATP to AMP and adenosine. In T cells, both CD39 and CD73 are upregulated shortly after activation, however, while CD39 remains on the cell membrane of activated cells, CD73 is released from the membrane, mostly in EVs (31). In this way, effector cells are able to survive in an ATP-rich environment at the same time that they are not suppressed by pericellular adenosine. EVs containing purinergic enzymes can move away from the cell of origin and exert their enzymatic activity in a paracrine fashion in the vicinity of cells lacking the full machinery for adenosine production. Indeed, human Tregs and Treg-derived EVs are competent in degrading ATP to AMP, but much less efficient in generating adenosine. CD73 can be provided by other cells, either cell-bound or in form of EVs (31, 39). The source of CD73-decorated EVs includes for example recently-activated T cells and stromal cells at sites of inflammation (31, 33), and tumor cells (25) (Table 1). In contrast to human Tregs, EVs derived from murine Tregs contain both CD39 and CD73, and are able to generate adenosine by themselves (26).
Hypoxia in the tumor microenvironment triggers the upregulation of CD39 and CD73 in cancer cells (82), which metabolize ATP, generating sufficient adenosine to cause immune suppression (83). Hypoxia also promotes the release of EVs from cancer cells, and these EVs exhibit potent enzymatic activity to degrade ATP and AMP (25) (Table 1), suppressing T cell function. B cell-derived EVs carrying CD39 and CD73 further exacerbate the immune suppressive environment within the tumor (45). Both ATP and adenosine have been found in tumor cell-derived EVs (23), and perforin secreted by activated cytotoxic T cells can disrupt the EV membrane and lead to adenosine release that will in turn inhibit cytotoxic responses (21). Antibody-mediated blockade of CD73 enzymatic activity revokes immune suppression by tumor-derived EVs in vitro, highlighting the importance of the purinergic pathway in immune suppression in the context of tumors (29). EVs with purinergic enzyme activity travel far beyond the tumor and are detected in peripheral blood, where their presence correlates with cancer progression (30, 45, 49).
In summary, EVs containing purinergic enzyme activity or nucleotides are potent immune modulators whose activity is not restricted to the vicinity of their cells of origin.
5 Translational application of extracellular vesicles targeting the purinergic pathway
EVs are promising biomarkers for diagnostics, monitoring of disease course and response to therapy, and already regarded as therapeutic agents. Changes in EV concentration or EV cargo are used as predictive biomarkers for autoimmunity, cancer, ischemia and neurodegeneration. In the field of cancer, the increasing interest in liquid biopsies for the detection of circulating tumor cells provides an excellent platform to test the feasibility of using EVs as predictive biomarkers. Given the high expression of CD73 in tumor cells and its role in immune suppression, especially the presence of CD73 on EVs could help to predict response to immunotherapy (27).
In inflammation, CD73-containing EVs have a beneficial effect by suppressing effector mechanisms or by promoting M2 macrophage polarization and tissue regeneration (35, 47). The advantages of EVs from human cells or cell lines as therapeutic agents are the potential to cross biological barriers, cell-specific function, and their low immunogenicity because they do not display exogenous factors (84, 85). The in vitro generation and modulation of mesenchymal stem cell-derived EVs for Covid-19 treatment and autoimmune diseases is currently tested in clinical and pre-clinical studies (86, 87), with no severe adverse effects described so far. In preclinical models, MSC- or Treg-derived EVs have been successfully used in an animal model of rheumatoid arthritis (88, 89). Even though the role of CD73 or adenosine in the modulatory function was not addressed, it is noteworthy that both EV-donor cell types express CD73, and a synergism to the proposed mechanism is plausible.
In order to use EVs for therapy, it is critical to further understand EV biology. Research on EVs has long been hampered due to technical challenges and the lack of standardization protocols for EV isolation, storage and characterization methods. The International Society for Extracellular Vesicles (ISEV) has proposed and updated the “Minimal Information for Studies of Extracellular Vesicles” (MISEV), to promote reproducibility in this field of research (54, 90). EVs can be isolated with increasing purity and recovery yield from various kinds of biological fluids, conditioned cell culture media and tissues (91), facilitating proteomics and transcriptomic analyses (92, 93), as well as enzymatic activity and functional assays (31). Analyses at single-vesicle level are limited due to the small size and high level of heterogeneity of EVs. Nanoscale flow cytometry is promising, but still challenging for surface phenotyping of EVs, as the small size limits the detection and antibody binding (94). While characterization methods are improving, in vivo EV tracking remains difficult. Deciphering the physiological stability, biodistribution and interactions of EVs with cells in in vivo settings is essential for assessing the (patho)physiological role of EVs and their potential use for therapy. Recent progress in real-time non-invasive imaging, high-resolution microscopy and labelling techniques should pave the way for better understanding of the EV biology.
In conclusion, an increasing number of publications describe the presence of several purinergic enzymes (CD39, CD73, ENPP1, CD26, ADA and APs) as well as of adenine nucleotides (ATP and adenosine) in EVs. Especially on cancer-derived EVs, but also on EVs from immune cells, the adenine nucleotide-metabolizing function of ectonucleotidases has immunomodulatory effects. With advancing technologies, future EV studies will give further insights into the biological role and clinical application of EV-mediated purinergic activity.
Author contributions
RW: Conceptualization, Writing – original draft, Writing – review & editing. DN: Writing – original draft, Writing – review & editing. FS: Writing – review & editing. FC: Writing – review & editing. NG: Writing – review & editing, Funding acquisition. ET: Writing – review & editing, Conceptualization, Funding acquisition, Supervision, Writing – original draft.
Funding
The author(s) declare financial support was received for the research, authorship, and/or publication of this article. Work on the purinergic system in our research groups is supported by the German Research Council SFB 1328 (Project-ID: 335447717 to ET and NG, subproject A14) and FOR 2879 ImmunoStroke (Project-ID: 405358801 to ET, subproject C1). We acknowledge financial support from the Open Access Publication Fund of UKE - Universitätsklinikum Hamburg-Eppendorf and DFG – German Research Foundation
Acknowledgments
We thank our colleagues in the Department of Immunology for continuous discussions over the topic of purinergic signaling.
Conflict of interest
NG reports financial support from Roche, not involving the work presented here.
The remaining authors declare that the research was conducted in the absence of any commercial or financial relationships that could be construed as a potential conflict of interest.
Publisher’s note
All claims expressed in this article are solely those of the authors and do not necessarily represent those of their affiliated organizations, or those of the publisher, the editors and the reviewers. Any product that may be evaluated in this article, or claim that may be made by its manufacturer, is not guaranteed or endorsed by the publisher.
References
1. Linden J, Cekic C. Regulation of lymphocyte function by adenosine. Arterioscler Thromb Vasc Biol (2012) 32:2097–103. doi: 10.1161/ATVBAHA.111.226837
2. Buzas EI. The roles of extracellular vesicles in the immune system. Nat Rev Immunol (2022) 23:1–15. doi: 10.1038/s41577-022-00763-8
3. Carotti V, Rigalli JP, van Asbeck-van der Wijst J, Hoenderop JGJ. Interplay between purinergic signalling and extracellular vesicles in health and disease. Biochem Pharmacol (2022) 203:115192. doi: 10.1016/J.BCP.2022.115192
4. Céspedes PF, Jainarayanan A, Fernández-Messina L, Valvo S, Saliba DG, Kurz E, et al. T-cell trans-synaptic vesicles are distinct and carry greater effector content than constitutive extracellular vesicles. Nat Commun (2022) 13:1–18. doi: 10.1038/s41467-022-31160-3
5. Mathieu M, Névo N, Jouve M, Valenzuela JI, Maurin M, Verweij FJ, et al. Specificities of exosome versus small ectosome secretion revealed by live intracellular tracking of CD63 and CD9. Nat Commun (2021) 12:1–18. doi: 10.1038/s41467-021-24384-2
6. van der Vlist EJ, Arkesteijn GJA, van de Lest CHA, Stoorvogel W, Nolte-'t Hoen EN, Wauben MHM. CD4+ T cell activation promotes the differential release of distinct populations of nanosized vesicles. J Extracell Vesicles (2012) 1. doi: 10.3402/jev.v1i0.18364
7. Lanna A, Vaz B, D’Ambra C, Valvo S, Vuotto C, Chiurchiù V, et al. An intercellular transfer of telomeres rescues T cells from senescence and promotes long-term immunological memory. Nat Cell Biol (2022) 24:1461–74. doi: 10.1038/s41556-022-00991-z
8. Ruan S, Erwin N, He M. Light-induced high-efficient cellular production of immune functional extracellular vesicles. J Extracell Vesicles (2022) 11. doi: 10.1002/jev2.12194
9. Hariharan H, Kesavan Y, Raja NS. Impact of native and external factors on exosome release: understanding reactive exosome secretion and its biogenesis. Mol Biol Rep (2021) 48:7559–73. doi: 10.1007/s11033-021-06733-y
10. Aubertin K, Silva AKA, Luciani N, Espinosa A, Djemat A, Charue D, et al. Massive release of extracellular vesicles from cancer cells after photodynamic treatment or chemotherapy. Sci Rep (2016) 6:1–11. doi: 10.1038/srep35376
11. Kao Y-C, Chang Y-W, Lai CP, Chang N-W, Huang C-H, Chen C-S, et al. Ectopic ATP synthase stimulates the secretion of extracellular vesicles in cancer cells. Commun Biol (2023) 6:642. doi: 10.1038/s42003-023-05008-5
12. Muñiz-García A, Romero M, Falcόn-Perez JM, Murray P, Zorzano A, Mora S. Hypoxia-induced HIF1α activation regulates small extracellular vesicle release in human embryonic kidney cells. Sci Rep (2022) 12:1–14. doi: 10.1038/s41598-022-05161-7
13. Raposo G, Nijman HW, Stoorvogel W, Leijendekker R, Harding CV, Melief CJM, et al. B lymphocytes secrete antigen-presenting vesicles. J Exp Med (1996) 183:1161–72. doi: 10.1084/JEM.183.3.1161
14. Choudhuri K, Llodrá J, Roth EW, Tsai J, Gordo S, Wucherpfennig KW, et al. Polarized release of T-cell-receptor-enriched microvesicles at the immunological synapse. Nature (2014) 507:118–23. doi: 10.1038/nature12951
15. Lima LG, Ham S, Shin H, Chai EPZ, Lek ESH, Lobb RJ, et al. Tumor microenvironmental cytokines bound to cancer exosomes determine uptake by cytokine receptor-expressing cells and biodistribution. Nat Commun (2021) 12:3543. doi: 10.1038/s41467-021-23946-8
16. Kim H-R, Mun Y, Lee K-S, Park Y-J, Park J-S, Park J-H, et al. T cell microvilli constitute immunological synaptosomes that carry messages to antigen-presenting cells. Nat Commun (2018) 9:3630. doi: 10.1038/s41467-018-06090-8
17. Fitzgerald W, Freeman ML, Lederman MM, Vasilieva E, Romero R, Margolis L. A system of cytokines encapsulated in extraCellular vesicles. Sci Rep (2018) 8:1–11. doi: 10.1038/s41598-018-27190-x
18. Wozniak AL, Adams A, King KE, Dunn W, Christenson LK, Hung W-T, et al. The RNA binding protein FMR1 controls selective exosomal miRNA cargo loading during inflammation. J Cell Biol (2020) 219:e201912074. doi: 10.1083/jcb.201912074
19. Gezer U, Özgür E, Cetinkaya M, Isin M, Dalay N. Long non-coding RNAs with low expression levels in cells are enriched in secreted exosomes. Cell Biol Int (2014) 38:1076–9. doi: 10.1002/cbin.10301
20. Bitto NJ, Cheng L, Johnston EL, Pathirana R, Phan TK, Poon IKH, et al. Staphylococcus aureus membrane vesicles contain immunostimulatory DNA, RNA and peptidoglycan that activate innate immune receptors and induce autophagy. J Extracell Vesicles (2021) 10:e12080. doi: 10.1002/jev2.12080
21. Tadokoro H, Hirayama A, Kudo R, Hasebe M, Yoshioka Y, Matsuzaki J, et al. Adenosine leakage from perforin-burst extracellular vesicles inhibits perforin secretion by cytotoxic T-lymphocytes. PloS One (2020) 15:e0231430. doi: 10.1371/journal.pone.0231430
22. Ludwig N, Gillespie DG, Reichert TE, Jackson EK, Whiteside TL. Purine metabolites in tumor-derived exosomes may facilitate immune escape of head and neck squamous cell carcinoma. Cancers (Basel) (2020) 12:1602. doi: 10.3390/cancers12061602
23. Vultaggio-Poma V, Falzoni S, Chiozzi P, Sarti AC, Adinolfi E, Giuliani AL, et al. Extracellular ATP is increased by release of ATP-loaded microparticles triggered by nutrient deprivation. Theranostics (2022) 12:859–74. doi: 10.7150/thno.66274
24. Deaglio S, Dwyer KM, Gao W, Friedman D, Usheva A, Erat A, et al. Adenosine generation catalyzed by CD39 and CD73 expressed on regulatory T cells mediates immune suppression. J Exp Med (2007) 204:1257–65. doi: 10.1084/jem.20062512
25. Clayton A, Al-Taei S, Webber J, Mason MD, Tabi Z. Cancer exosomes express CD39 and CD73, which suppress T cells through adenosine production. J Immunol (2011) 187:676–83. doi: 10.4049/jimmunol.1003884
26. Smyth LA, Ratnasothy K, Tsang JYS, Boardman D, Warley A, Lechler R, et al. CD73 expression on extracellular vesicles derived from CD4+CD25+Foxp3+ T cells contributes to their regulatory function. Eur J Immunol (2013) 43:2430–40. doi: 10.1002/eji.201242909
27. Lu T, Zhang Z, Zhang J, Pan X, Zhu X, Wang X, et al. CD73 in small extracellular vesicles derived from HNSCC defines tumour-associated immunosuppression mediated by macrophages in the microenvironment. J Extracell Vesicles (2022) 11:e12218. doi: 10.1002/jev2.12218
28. Wang M, Jia J, Cui Y, Peng Y, Jiang Y. CD73-positive extracellular vesicles promote glioblastoma immunosuppression by inhibiting T-cell clonal expansion. Cell Death Dis (2021) 12:1065. doi: 10.1038/s41419-021-04359-3
29. Ploeg EM, Ke X, Britsch I, Hendriks MAJM, van der Zant FA, Kruijff S, et al. Bispecific antibody CD73xEpCAM selectively inhibits the adenosine-mediated immunosuppressive activity of carcinoma-derived extracellular vesicles. Cancer Lett (2021) 521:109–18. doi: 10.1016/j.canlet.2021.08.037
30. Turiello R, Capone M, Morretta E, Monti MC, Madonna G, Azzaro R, et al. Exosomal CD73 from serum of patients with melanoma suppresses lymphocyte functions and is associated with therapy resistance to anti-PD-1 agents. J Immunother Cancer (2022) 10:e004043. doi: 10.1136/JITC-2021-004043
31. Schneider E, Winzer R, Rissiek A, Ricklefs I, Meyer-Schwesinger C, Ricklefs FL, et al. CD73-mediated adenosine production by CD8 T cell-derived extracellular vesicles constitutes an intrinsic mechanism of immune suppression. Nat Commun (2021) 12:5911. doi: 10.1038/S41467-021-26134-W
32. Ravichandran R, Itabashi Y, Fleming T, Bansal S, Bowen S, Poulson C, et al. Low-dose IL-2 prevents murine chronic cardiac allograft rejection: Role for IL-2-induced T regulatory cells and exosomes with PD-L1 and CD73. Am J Transplant (2022) 22:2180–94. doi: 10.1111/ajt.17101
33. Kerkelä E, Laitinen A, Räbinä J, Valkonen S, Takatalo M, Larjo A, et al. Adenosinergic immunosuppression by human mesenchymal stromal cells requires co-operation with T cells. Stem Cells (2016) 34:781–90. doi: 10.1002/stem.2280
34. Amarnath S, Foley JE, Farthing DE, Gress RE, Laurence A, Eckhaus MA, et al. Bone marrow-derived mesenchymal stromal cells harness purinergenic signaling to tolerize human th1 cells. vivo. Stem Cells (2015) 33:1200–12. doi: 10.1002/stem.1934
35. Watanabe Y, Fukuda T, Hayashi C, Nakao Y, Toyoda M, Kawakami K, et al. Extracellular vesicles derived from GMSCs stimulated with TNF-α and IFN-α promote M2 macrophage polarization via enhanced CD73 and CD5L expression. Sci Rep (2022) 12:13344. doi: 10.1038/s41598-022-17692-0
36. Nakao Y, Fukuda T, Zhang Q, Sanui T, Shinjo T, Kou X, et al. Exosomes from TNF-α-treated human gingiva-derived MSCs enhance M2 macrophage polarization and inhibit periodontal bone loss. Acta Biomater (2021) 122:306–24. doi: 10.1016/j.actbio.2020.12.046
37. Teo KYW, Zhang S, Loh JT, Lai RC, Hey HWD, Lam KP, et al. Mesenchymal stromal cell exosomes mediate M2-like macrophage polarization through CD73/ecto-5′-nucleotidase activity. Pharmaceutics (2023) 15:1489. doi: 10.3390/PHARMACEUTICS15051489/S1
38. Bauer FN, Tertel T, Stambouli O, Wang C, Dittrich R, Staubach S, et al. CD73 activity of mesenchymal stromal cell-derived extracellular vesicle preparations is detergent-resistant and does not correlate with immunomodulatory capabilities. Cytotherapy (2023) 25:138–47. doi: 10.1016/j.jcyt.2022.09.006
39. Tung SL, Fanelli G, Matthews RI, Bazoer J, Letizia M, Vizcay-Barrena G, et al. Regulatory T cell extracellular vesicles modify T-effector cell cytokine production and protect against human skin allograft damage. Front Cell Dev Biol (2020) 8:317. doi: 10.3389/fcell.2020.00317
40. Wang Y, Liu M, Zhang L, Liu X, Ji H, Wang Y, et al. Cancer CD39 drives metabolic adaption and mal-differentiation of CD4+ T cells in patients with non-small-cell lung cancer. Cell Death Dis (2023) 14:804. doi: 10.1038/s41419-023-06336-4
41. Hong C-S, Sharma P, Yerneni SS, Simms P, Jackson EK, Whiteside TL, et al. Circulating exosomes carrying an immunosuppressive cargo interfere with cellular immunotherapy in acute myeloid leukemia. Sci Rep (2017) 7:14684. doi: 10.1038/s41598-017-14661-w
42. Schuler PJ, Saze Z, Hong C-S, Muller L, Gillespie DG, Cheng D, et al. Human CD4+ CD39+ regulatory T cells produce adenosine upon co-expression of surface CD73 or contact with CD73+ exosomes or CD73+ cells. Clin Exp Immunol (2014) 177:531–43. doi: 10.1111/cei.12354
43. Azambuja JH, Ludwig N, Yerneni S, Rao A, Braganhol E, Whiteside TL. Molecular profiles and immunomodulatory activities of glioblastoma-derived exosomes. Neuro-Oncology Adv (2020) 2. doi: 10.1093/NOAJNL/VDAA056
44. Scholl JN, de Fraga Dias A, Pizzato PR, Lopes DV, Moritz CEJ, Jandrey EHF, et al. Characterization and antiproliferative activity of glioma-derived extracellular vesicles. Nanomedicine (Lond) (2020) 15:1001–18. doi: 10.2217/nnm-2019-0431
45. Zhang F, Li R, Yang Y, Shi C, Shen Y, Lu C, et al. Specific decrease in B-cell-derived extracellular vesicles enhances post-chemotherapeutic CD8+ T cell responses. Immunity (2019) 50:738–750.e7. doi: 10.1016/j.immuni.2019.01.010
46. Mezzasoma L, Bellezza I, Orvietani P, Manni G, Gargaro M, Sagini K, et al. Amniotic fluid stem cell-derived extracellular vesicles are independent metabolic units capable of modulating inflammasome activation in THP-1 cells. FASEB J (2022) 36:e22218. doi: 10.1096/fj.202101657R
47. Ludwig N, Yerneni SS, Azambuja JH, Gillespie DG, Menshikova EV, Jackson EK, et al. Tumor-derived exosomes promote angiogenesis via adenosine A2B receptor signaling. Angiogenesis (2020) 23:599–610. doi: 10.1007/s10456-020-09728-8
48. Theodoraki MN, Hoffmann TK, Jackson EK, Whiteside TL. Exosomes in HNSCC plasma as surrogate markers of tumour progression and immune competence. Clin Exp Immunol (2018) 194:67–78. doi: 10.1111/cei.13157
49. Morandi F, Marimpietri D, Horenstein AL, Bolzoni M, Toscani D, Costa F, et al. Microvesicles released from multiple myeloma cells are equipped with ectoenzymes belonging to canonical and non-canonical adenosinergic pathways and produce adenosine from ATP and NAD+. Oncoimmunology (2018) 7:e1458809. doi: 10.1080/2162402X.2018.1458809
50. Morandi F, Marimpietri D, Horenstein AL, Corrias MV, Malavasi F. Microvesicles expressing adenosinergic ectoenzymes and their potential role in modulating bone marrow infiltration by neuroblastoma cells. Oncoimmunology (2019) 8:e1574198. doi: 10.1080/2162402X.2019.1574198
51. Jiang ZG, Wu Y, Csizmadia E, Feldbrügge L, Enjyoji K, Tigges J, et al. Characterization of circulating microparticle-associated CD39 family ecto-nucleotidases in human plasma. Purinergic Signal (2014) 10:611–8. doi: 10.1007/s11302-014-9423-6
52. Pizzirani C, Ferrari D, Chiozzi P, Adinolfi E, Sandonà D, Savaglio E, et al. Stimulation of P2 receptors causes release of IL-1beta-loaded microvesicles from human dendritic cells. Blood (2007) 109:3856–64. doi: 10.1182/BLOOD-2005-06-031377
53. De Gassart A, Géminard C, Février B, Raposo G, Vidal M. Lipid raft-associated protein sorting in exosomes. Blood (2003) 102:4336–44. doi: 10.1182/blood-2003-03-0871
54. Théry C, Witwer KW, Aikawa E, Alcaraz MJ, Anderson JD, Andriantsitohaina R, et al. Minimal information for studies of extracellular vesicles 2018 (MISEV2018): A position statement of the International Society for Extracellular Vesicles and update of the MISEV2014 guidelines. J Extracell Vesicles (2018) 7. doi: 10.1080/20013078.2018.1535750
55. Lee HC. Cyclic ADP-ribose and nicotinic acid adenine dinucleotide phosphate (NAADP) as messengers for calcium mobilization. J Biol Chem (2012) 287:31633–40. doi: 10.1074/jbc.R112.349464
56. Guse AH. Calcium mobilizing second messengers derived from NAD. Biochim Biophys Acta - Proteins Proteomics (2015) 1854:1132–7. doi: 10.1016/j.bbapap.2014.12.015
57. Zumaquero E, Muñoz P, Cobo M, Lucena G, Pavón EJ, Martín A, et al. Exosomes from human lymphoblastoid B cells express enzymatically active CD38 that is associated with signaling complexes containing CD81, Hsc-70 and Lyn. Exp Cell Res (2010) 316:2692–706. doi: 10.1016/j.yexcr.2010.05.032
58. Brennan K, Iversen KF, Blanco-Fernández A, Lund T, Plesner T, Mc Gee MM. Extracellular vesicles isolated from plasma of multiple myeloma patients treated with daratumumab express CD38, PD-L1, and the complement inhibitory proteins CD55 and CD59. Cells (2022) 11:3365. doi: 10.3390/cells11213365
59. Horenstein AL, Chillemi A, Zaccarello G, Bruzzone S, Quarona V, Zito A, et al. A CD38/CD203a/CD73 ectoenzymatic pathway independent of CD39 drives a novel adenosinergic loop in human T lymphocytes. Oncoimmunology (2013) 2:e26246. doi: 10.4161/onci.26246
60. Carozza JA, Cordova AF, Brown JA, AlSaif Y, Bohnert V, Cao X, et al. ENPP1’s regulation of extracellular cGAMP is a ubiquitous mechanism of attenuating STING signaling. Proc Natl Acad Sci U.S.A. (2022) 119:e2119189119. doi: 10.1073/pnas.2119189119
61. Tersigni C, Redman CW, Dragovic R, Tannetta D, Scambia G, Di Simone N, et al. HLA-DR is aberrantly expressed at feto-maternal interface in pre-eclampsia. J Reprod Immunol (2018) 129:48–52. doi: 10.1016/j.jri.2018.06.024
62. Parveen A, Mishra S, Srivastava M, Chaudhary DK, Kapoor D, Gupta A, et al. Circulating placental alkaline phosphatase expressing exosomes in maternal blood showed temporal regulation of placental genes. Front Med (2021) 8:758971/BIBTEX. doi: 10.3389/FMED.2021.758971/BIBTEX
63. Du J, Sun Q, Wang Z, Wang F, Chen F, Wang H, et al. Tubular epithelial cells derived-exosomes containing CD26 protects mice against renal ischemia/reperfusion injury by maintaining proliferation and dissipating inflammation. Biochem Biophys Res Commun (2021) 553:134–40. doi: 10.1016/j.bbrc.2021.03.057
64. Bianco F, Pravettoni E, Colombo A, Schenk U, Möller T, Matteoli M, et al. Astrocyte-derived ATP induces vesicle shedding and IL-1 beta release from microglia. J Immunol (2005) 174:7268–77. doi: 10.4049/JIMMUNOL.174.11.7268
65. Pegoraro A, De Marchi E, Ferracin M, Orioli E, Zanoni M, Bassi C, et al. P2X7 promotes metastatic spreading and triggers release of miRNA-containing exosomes and microvesicles from melanoma cells. Cell Death Dis (2021) 12. doi: 10.1038/S41419-021-04378-0
66. Adinolfi E, De Marchi E, Grignolo M, Szymczak B, Pegoraro A. The P2X7 receptor in oncogenesis and metastatic dissemination: New insights on vesicular release and adenosinergic crosstalk. Int J Mol Sci (2023) 24. doi: 10.3390/IJMS241813906
67. Aresta Branco MSL, Gutierrez Cruz A, Dayton J, Perrino BA, Mutafova-Yambolieva VN. Mechanosensitive hydrolysis of ATP and ADP in lamina propria of the murine bladder by membrane-bound and soluble nucleotidases. Front Physiol (2022) 13:918100. doi: 10.3389/fphys.2022.918100
68. Cordero OJ, Salgado FJ, Mera-Varela A, Nogueira M. Serum interleukin-12, interleukin-15, soluble CD26, and adenosine deaminase in patients with rheumatoid arthritis. Rheumatol Int (2001) 21:69–74. doi: 10.1007/s002960100134
69. Pettengill M, Robson S, Tresenriter M, Millán JL, Usheva A, Bingham T, et al. Soluble ecto-5′-nucleotidase (5′-NT), alkaline phosphatase, and adenosine deaminase (ADA1) activities in neonatal blood favor elevated extracellular adenosine. J Biol Chem (2013) 288:27315–26. doi: 10.1074/jbc.M113.484212
70. Zeiner J, Loukovaara S, Losenkova K, Zuccarini M, Korhonen AM, Lehti K, et al. Soluble and membrane-bound adenylate kinase and nucleotidases augment ATP-mediated inflammation in diabetic retinopathy eyes with vitreous hemorrhage. J Mol Med (2019) 97:341–54. doi: 10.1007/s00109-018-01734-0
71. Bonsergent E, Grisard E, Buchrieser J, Schwartz O, Théry C, Lavieu G. Quantitative characterization of extracellular vesicle uptake and content delivery within mammalian cells. Nat Commun (2021) 12:1–11. doi: 10.1038/s41467-021-22126-y
72. Albanese M, Chen YFA, Hüls C, Gärtner K, Tagawa T, Mejias-Perez E, et al. MicroRNAs are minor constituents of extracellular vesicles that are rarely delivered to target cells. PloS Genet (2021) 17:e1009951. doi: 10.1371/journal.pgen.1009951
73. Garcia-Martin R, Wang G, Brandão BB, Zanotto TM, Shah S, Kumar Patel S, et al. MicroRNA sequence codes for small extracellular vesicle release and cellular retention. Nature (2022) 601:446–51. doi: 10.1038/s41586-021-04234-3
74. Chen G, Huang AC, Zhang W, Zhang G, Wu M, Xu W, et al. Exosomal PD-L1 contributes to immunosuppression and is associated with anti-PD-1 response. Nature (2018) 560:382–6. doi: 10.1038/S41586-018-0392-8
75. Théry C, Duban L, Segura E, Væron P, Lantz O, Amigorena S. Indirect activation of naïve CD4+ T cells by dendritic cell-derived exosomes. Nat Immunol (2002) 3:1156–62. doi: 10.1038/ni854
76. Klabunde RE. Dipyridamole inhibition of adenosine metabolism in human blood. Eur J Pharmacol (1983) 93:21–6. doi: 10.1016/0014-2999(83)90026-2
77. Gangadaran P, Li XJ, Lee HW, Oh JM, Kalimuthu S, Rajendran RL, et al. A new bioluminescent reporter system to study the biodistribution of systematically injected tumor-derived bioluminescent extracellular vesicles in mice. Oncotarget (2017) 8:109894. doi: 10.18632/ONCOTARGET.22493
78. Lai CP, Mardini O, Ericsson M, Prabhakar S, Maguire CA, Chen JW, et al. Dynamic biodistribution of extracellular vesicles in vivo using a multimodal imaging reporter. ACS Nano (2014) 8:483–94. doi: 10.1021/nn404945r
79. Hikita T, Miyata M, Watanabe R, Oneyama C. In vivo imaging of long-term accumulation of cancer-derived exosomes using a BRET-based reporter. Sci Rep (2020) 10. doi: 10.1038/S41598-020-73580-5
80. López de Las Hazas MC, Del Pozo-Acebo L, Hansen MS, Gil-Zamorano J, Mantilla-Escalante DC, Gómez-Coronado D, et al. Dietary bovine milk miRNAs transported in extracellular vesicles are partially stable during GI digestion, are bioavailable and reach target tissues but need a minimum dose to impact on gene expression. Eur J Nutr (2022) 61:1043–56. doi: 10.1007/s00394-021-02720-y
81. Ohta A, Gorelik E, Prasad SJ, Ronchese F, Lukashev D, Wong MKK, et al. A2A adenosine receptor protects tumors from antitumor T cells. Proc Natl Acad Sci U.S.A. (2006) 103:13132–7. doi: 10.1073/pnas.0605251103
82. Allard B, Longhi MS, Robson SC, Stagg J. The ectonucleotidases CD39 and CD73: Novel checkpoint inhibitor targets. Immunol Rev (2017) 276:121–44. doi: 10.1111/imr.12528
83. Blay J, White TD, Hoskin DW. The extracellular fluid of solid carcinomas contains immunosuppressive concentrations of adenosine. Cancer Res (1997) 57:2602–5.
84. Arifin DR, Witwer KW, Bulte JWM. Non-Invasive imaging of extracellular vesicles: Quo vaditis. vivo? J Extracell vesicles (2022) 11:e12241. doi: 10.1002/jev2.12241
85. Cecchin R, Troyer Z, Witwer K, Morris KV. Extracellular vesicles: The next generation in gene therapy delivery. Mol Ther (2023) 31:1225–30. doi: 10.1016/j.ymthe.2023.01.021
86. Martinez-Arroyo O, Ortega A, Forner MJ, Cortes R. Mesenchymal stem cell-derived extracellular vesicles as non-coding RNA therapeutic vehicles in autoimmune diseases. Pharmaceutics (2022) 14. doi: 10.3390/PHARMACEUTICS14040733
87. Huang Y, Li X, Yang L. Mesenchymal stem cells and their derived small extracellular vesicles for COVID-19 treatment. Stem Cell Res Ther (2022) 13:1–12. doi: 10.1186/S13287-022-03034-4/TABLES/2
88. Xu K, Ma D, Zhang G, Gao J, Su Y, Liu S, et al. Human umbilical cord mesenchymal stem cell-derived small extracellular vesicles ameliorate collagen-induced arthritis via immunomodulatory T lymphocytes. Mol Immunol (2021) 135:36–44. doi: 10.1016/J.MOLIMM.2021.04.001
89. Chen J, Huang F, Hou Y, Lin X, Liang R, Hu X, et al. TGF-β-induced CD4+ FoxP3+ regulatory T cell-derived extracellular vesicles modulate Notch1 signaling through miR-449a and prevent collagen-induced arthritis in a murine model. Cell Mol Immunol (2021) 18:2516–29. doi: 10.1038/S41423-021-00764-Y
90. Witwer KW, Goberdhan DCI, O’Driscoll L, Théry C, Welsh JA, Blenkiron C, et al. Updating MISEV: Evolving the minimal requirements for studies of extracellular vesicles. J Extracell Vesicles (2021) 10. doi: 10.1002/jev2.12182
91. Crescitelli R, Lässer C, Lötvall J. Isolation and characterization of extracellular vesicle subpopulations from tissues. Nat Protoc (2021) 16:1548–80. doi: 10.1038/s41596-020-00466-1
92. Poh QH, Rai A, Salamonsen LA, Greening DW. Omics insights into extracellular vesicles in embryo implantation and their therapeutic utility. Proteomics (2023) 23:2200107. doi: 10.1002/PMIC.202200107
93. Lischnig A, Bergqvist M, Ochiya T, Lässer C. Quantitative proteomics identifies proteins enriched in large and small extracellular vesicles. Mol Cell Proteomics (2022) 21:100273. doi: 10.1016/j.mcpro.2022.100273
Keywords: extracellular vesicles, purinergic signaling, CD73, adenosine, immune regulation, tumor microenvironment
Citation: Winzer R, Nguyen DH, Schoppmeier F, Cortesi F, Gagliani N and Tolosa E (2024) Purinergic enzymes on extracellular vesicles: immune modulation on the go. Front. Immunol. 15:1362996. doi: 10.3389/fimmu.2024.1362996
Received: 29 December 2023; Accepted: 30 January 2024;
Published: 15 February 2024.
Edited by:
Santina Bruzzone, University of Genoa, ItalyReviewed by:
Elena Adinolfi, University of Ferrara, ItalyCopyright © 2024 Winzer, Nguyen, Schoppmeier, Cortesi, Gagliani and Tolosa. This is an open-access article distributed under the terms of the Creative Commons Attribution License (CC BY). The use, distribution or reproduction in other forums is permitted, provided the original author(s) and the copyright owner(s) are credited and that the original publication in this journal is cited, in accordance with accepted academic practice. No use, distribution or reproduction is permitted which does not comply with these terms.
*Correspondence: Eva Tolosa, ZXRvbG9zYUB1a2UuZGU=
†These authors have contributed equally to this work