- 1College of Veterinary Medicine (BK21 FOUR Program), Chungnam National University, Daejeon, Republic of Korea
- 2Jeonbuk Department of Inhalation Research, Korea Institute of Toxicology, Jeongeup, Republic of Korea
Introduction: The anti-inflammatory effect of green tea extract (GTE) has been confirmed in asthmatic mice, however, the pharmacological mechanism is not fully elucidated.
Methods: To investigate the therapeutic efficacy of GTE in asthma and identify specific pathways, murine model of allergic asthma was established by ovalbumin (OVA) sensitization and the challenge for 4 weeks, with oral treatment using GTE and dexamethasone (DEX). Inflammatory cell counts, cytokines, OVA-specific IgE, airway hyperreactivity, and antioxidant markers in the lung were evaluated. Also, pulmonary histopathological analysis and western blotting were performed. In vitro, we established the model by stimulating the human airway epithelial cell line NCI-H292 using lipopolysaccharide, and treating with GTE and mitogen-activated protein kinases (MAPKs) inhibitors.
Results: The GTE100 and GTE400 groups showed a decrease in airway hyperresponsiveness and the number of inflammatory cells in the bronchoalveolar lavage fluid (BALF) compared to the OVA group. GTE treatment also reduced interleukin (IL)‐13, IL-5, and IL‐4 levels in the BALF, and OVA-specific immunoglobulin E levels in the serum compared to those in the OVA group. GTE treatment decreased OVA-induced mucus secretion and airway inflammation. In addition, GTE suppressed the oxidative stress, and phosphorylation of MAPKs, which generally occurs after exposure to OVA. GTE administration also reduced matrix metalloproteinase‐9 activity and protein levels.
Conclusion: GTE effectively inhibited asthmatic respiratory inflammation and mucus hyperproduction induced by OVA inhalation. These results suggest that GTE has the potential to be used for the treatment of asthma.
Introduction
Allergic asthma is a chronic pulmonary disease characterized by mucus hyperproduction and inflammation, and its prevalence has been gradually increasing (1). T helper 2 (Th2) cells exert key roles in pathogenesis of asthma by secreting Th2 cytokines including interleukin (IL)-4, IL-13, and IL-5 (2). Th2 cytokines not only assemble eosinophils and induce their activation and infiltration but also activate B cells to produce allergen-specific immunoglobulin (Ig) E (3). Considering transduction of signaling molecules, oxidative stress plays crucial roles in the development of asthma. An imbalance between extracellular oxidative inducers and intracellular antioxidant responses produces reactive oxygen species (ROS), leading to the activation of inflammatory signaling. Of these, matrix metalloproteinase (MMP)‐9 and mitogen-activated protein kinases (MAPKs) play a pivotal role in allergic asthma development (4, 5). Previous studies have demonstrated that oxidative stress-driven MAPKs phosphorylation leads to the upregulation of MMP-9 expression, resulting in inflammation and airway remodeling both in vivo and in clinical trials (6–8). Therefore, inhibition of these signals may be an important therapeutic target for asthma.
Camellia sinensis (L.) Kuntze (green tea) is one of the most popular natural products with numerous health benefits (9). Green tea has antibacterial, antioxidant, anticancer, and anti-inflammatory properties (9, 10). According to previous studies, green tea reduced oxidative and inflammatory damage to the airways of guinea pigs and mediated the inhibition of IgE levels in patients with allergic asthma (11, 12). It contains catechins such as epicatechin, epigallocatechin (EGC), EGC-3-gallate (EGCG), and epicatechin-3-gallate (13). These catechins are the main antioxidants in green tea and exhibit a strong ability to reduce ROS and nitrogen species (13). EGCG, a major component of green tea extract (GTE), reduced mucus production and p38/MMP-9 protein levels in a mouse model of asthma (14). In addition, the protective effects of epicatechin gallate against ovalbumin (OVA)-induced asthma have been confirmed in several in vivo studies through the modulation of cytokine expression, T cell populations, and various molecular signaling pathways (15, 16). Based on these results, it is possible that green tea exerted a protective effect in an OVA-induced mouse model of asthma.
To investigate the therapeutic mechanism of GTE in allergic asthma, we examined the levels of mucus secretion, inflammatory cells, and cytokines. Additionally, we performed molecular biology analyses, focusing on the role of GTE in regulating the oxidative stress-mediated MAPKs/MMP-9 signaling pathway.
Materials and methods
GTE preparation
Dried green tea powder was purchased from DAJAYEON Co. Ltd (Sacheon, Gyeongnam, Republic of Korea). The source is leaves of C. sinensis (L.) Kuntze and the place of production is Sacheon. The plant name was confirmed using “The Plant List” (http://www.theplantlist.org) (Aug 25, 2023). GTE was prepared as described previously (10). The extraction yield was approximately 32%, and GTE contained 36%, 22%, and 7% of catechin, EGCG, and EGC, respectively, as confirmed by HPLC with a UV detector (10).
In vivo experiments
Animals and environmental conditions
All experimental procedures were approved by the Institutional Animal Care and Use Committee of Chungnam National University (Approval no. 202206A-CNU-121; June 29, 2022) and were conducted in accordance with the Guidelines for Animal Experiments of Chungnam National University. The research was conducted in accordance with internationally accepted principles for laboratory animal use and care. Male BALB/c mice (7 weeks old, body weight 20–22 g) were supplied by Orient Bio (Gyeonggi-do, Republic of Korea) and allowed to adapt for 8 days. Mice were housed in standard conditions (humidity 50 ± 10%, temperature 23 ± 3°C, 13–18 air changes/h, and 12 h light/dark cycles). Commercial rodent chow and tap water were provided ad libitum.
Experimental groups
The mice were divided into five groups (n = 8/group) as follows (1): normal control (NC), orally treated with phosphate-buffered saline (PBS) from day 16 to day 24 and PBS sensitization/challenge; (2) OVA: orally treated with PBS from day 16 to day 24 and OVA sensitization/challenge; (3) dexamethasone (DEX): orally treated with DEX (3 mg/kg/day) from day 16 to day 24 and OVA sensitization/challenge; and (4) GTE100 and GTE400: orally treated with GTE (100 or 400 mg/kg/day, respectively) from day 16 to day 24 and OVA sensitization/challenge. OVA challenge was conducted as described by earlier (17). All mice were sacrificed under anesthesia two days after the last treatment, and lung tissue and blood were harvested.
Airway hyperresponsiveness and evaluation of bronchoalveolar fluid
Airway hyperresponsiveness (AHR) of mice was evaluated by whole-body plethysmography (Allmedicus, Gyeonggi-do, Republic of Korea) at day 1 after the third OVA inhalation as described previously (17). The enhanced pause (Penh) unit was used to represent the data.
To obtain bronchoalveolar lavage fluid (BALF), the mice were euthanized by carbon dioxide inhalation two days after the last OVA inhalation. BALF sampling was performed as described previously (17). The Diff-Quik® staining reagent (Sysmex Corporation, Kobe, Japan) was used for inflammatory cell staining in BALF.
ROS levels in BALF cells were examined using a cellular ROS assay kit (Abcam, Cambridge, UK) in compliance with the manufacturer’s instructions. BALF cells were observed under a confocal microscope (Leica Microsystems, Wetzlar, Germany).
Enzyme-linked immunosorbent assay
The levels of IL-13, IL-5, and IL-4 in BALF were assessed using enzyme-linked immunosorbent assay (ELISA) kits (R&D Systems, Oxford, United Kingdom) in accordance with the manufacturer’s instructions. The absorbance was measured at 450 nm using a microplate reader (iMark™; Bio-Rad Laboratories, Hercules, CA).
ELISA kits (BioLegend, San Diego, CA) were used to measure the total IgE and OVA-specific IgE levels in the serum. After the assay procedures, the plates were washed with PBS, and 200 μL of o-phenylenediamine dihydrochloride (Sigma-Aldrich, St. Louis, MO) was added to each well. The absorbance was measured at 450 nm using a microplate reader (Bio-Rad Laboratories).
Histopathological/immunohistochemical examination
Lung tissues were processed as described previously (17). To evaluate mucus production and airway inflammation, lung tissue was stained with periodic acid-Schiff (PAS; IMEB Inc. San Marcos, CA) or hematoxylin and eosin (H&E; BBC Biochemical, Mount Vemon, WA). The score of mucus production was calculated as the percentage of purple (positive) stained cells in the total cell count. MMP-9 protein was stained using an immunohistochemistry (IHC) kit (Abcam) following the manufacturer’s instructions. A primary antibody against MMP-9 (1:300; Cell Signaling Technology, Danvers, MA) was used for IHC staining. Brown stained cells are positive for MMP-9. Quantitative analyses were conducted with a light microscope in a blinded manner (Leica Microsystems) at 10× and 20× objective lenses using the IMT i-Solution software (Vancouver, North Road Burnaby, Canada).
Western blot
The frozen liver tissues were homogenized with tissue lysis/extraction (Sigma-Aldrich) containing a protease/phosphatase inhibitor cocktail (Sigma-Aldrich) and centrifuged at 10,000 × g for 10 min at 4°C to isolate the proteins in the lysate. To examine the levels of proteins related to the MAPKs pathway, we conducted immunoblotting according as described previously (17). Antibodies and dilutions were as follows: total (t)-jun N-terminal kinase (JNK) (1:1,000 dilution), phospho (p)-JNK (1:1,000 dilution), t-p38 (1:1,000 dilution), p-p38 (1:1,000 dilution), t-extracellular signal-regulated kinase (ERK) (1:1,000 dilution), p-ERK (1:1,000 dilution), MMP-9 (1:1,000 dilution), and β-actin (1:2,000 dilution), all obtained from Abcam. Each protein band was photographed using ChemiDoc (Bio-Rad Laboratories).
Evaluation of MMP-9 activity in lung tissue
Lung samples were used to evaluate MMP-9 activity. Gelatin zymography was performed as described by (18). For electrophoresis, gels containing 1% gelatin and 10% sodium dodecyl sulfate–polyacrylamide gel electrophoresis were used as MMP substrates. The gels were then washed and incubated for 24 h at 37°C in a developing buffer. The gels were stained with coomassie brilliant blue and destained using 25% methanol and 8% acetic acid in distilled water. The white band on a blue background indicates the activity of MMP-9.
Determination of antioxidant markers in lung tissue
Malondialdehyde (MDA), glutathione reductase (GR), glutathione (GSH), superoxide dismutase (SOD), and catalase (CAT) were detected in the lung tissues of mice from all five groups. MDA, GSH, CAT, GR, and SOD assay kits were purchased from Cayman Chemicals (Ann Arbor, MI) and performed in strict accordance with the manufacturer’s protocols. Absorbance (450 nm) was measured using a microplate reader (Bio-Rad Laboratories).
In vitro experiments
Cell viability and assessment of cytotoxicity
The human airway epithelial cell line NCI-H292 was cultured in RPMI 1640 medium containing fetal bovine serum and antibiotics (5% CO2, 95% air, 37°C). An EZ-Cytox Cytotoxicity Assay Kit (DoGenBio Co., Ltd., Seoul, Republic of Korea) was used to measure cell viability in response to GTE. The NCI-H292 cells were cultured in 96-well plates (2.0 × 104 cells/well, 100 µL), and incubated overnight. The cells were incubated with GTE (between 5 μg/mL to 160 μg/mL, 2-fold increases) and EGCG (Sigma-Aldrich, between 5 μg/mL to 160 μg/mL, 2-fold increases) for 24 h. After GTE or EGCG treatment, the kit solution was inoculated into each well and the plate was incubated for 1 h. Cytotoxicity was evaluated by measuring absorbance (450 nm) using a microplate reader (Bio-Rad Laboratories). Cytotoxicity was normalized to that of control cells.
Evaluation of mRNA expression levels
The cells were incubated with 10, 20, and 40 μg/mL of GTE or 20 μg/mL of EGCG for 15 h, and then, inoculated with 0.5 μg/mL lipopolysaccharide (LPS) for 4 h. Total RNA was isolated utilizing the SmartGene Total RNA Extraction Kit (SJ Bioscience, Daejeon, Republic of Korea) according to manufacturer’s instruction. First-strand cDNA was synthesized from 1000 ng of total RNA using a Compact cDNA Synthesis Kit (SJ Bioscience). Quantitative PCR was performed using the CFXTM Connect Real-Time System (Bio-Rad Laboratories) and SYBR Green Q-PCR Master Mix with Low Rox (SJ Bioscience). The relative quantification values of the targets were normalized to those of the housekeeping and untreated controls.
Western blot
NCI-H292 cells were incubated with GTE (10, 20, and 40 μg/mL), EGCG (20 μg/mL), and MAPKs inhibitors, including SB2035880 (p38 inhibitor, 10 uM; Sigma-Aldrich), SP600125 (JNK inhibitor, 10 uM; Sigma-Aldrich), and PD098059 (ERK inhibitor, 10 uM; Sigma-Aldrich) for 13 h, followed by treatment with 0.5 μg/mL LPS for 1 day. Cellular proteins were extracted using a RIPA buffer (Sigma–Aldrich) containing a protease/phosphatase inhibitor (Sigma–Aldrich). The experimental methods and antibodies used were identical to in vivo experiments.
Statistical analysis
The results were expressed as the mean ± standard deviation. To calculate statistical significance, a one-way analysis of variance followed by post-hoc Tukey’s honest significant difference test was used. All calculations were performed using GraphPad InStat (version 3.0; GraphPad Software, Inc., CA). Significance was indicated by a p value less than 0.05 or 0.01.
Results
In vivo experiments
Effects of GTE on AHR in asthmatic mice
The Penh value of the OVA group was significantly higher than that of the NC group at all methylcholine concentrations (Figure 1A). The GTE100 and GTE400 groups treated with 30 mg/mL methylcholine, and the GTE400 group treated with 20 mg/mL methylcholine showed decreased Penh values compared with the OVA group (p < 0.01). The Penh values of the DEX group were significantly lower than those of the OVA group treated with 20 and 30 mg/mL methylcholine (p < 0.01).
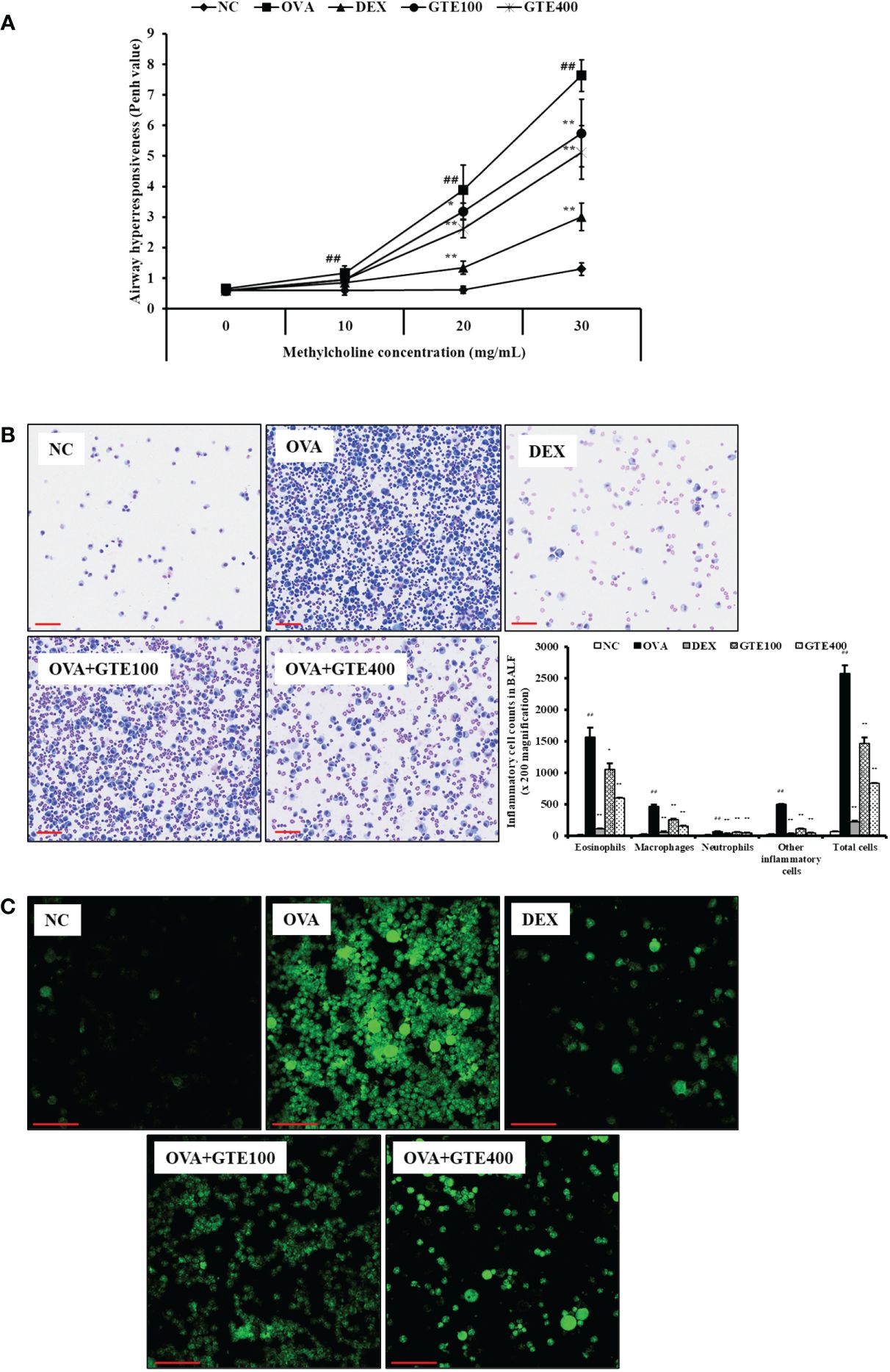
Figure 1 Green tea extract (GTE) decreases airway hyperresponsiveness (A), inflammatory cells in bronchoalveolar lavage fluid (B), and ROS production (C). Values are shown as means ± SD (n = 8). Significant differences: ##p < 0.01 vs. NC; *, **p < 0.05 and p < 0.01 vs. OVA, respectively. Scale bar = 100 µm.
Preventive effects of GTE on inflammatory cell counts and ROS production in BALF
To assess the effects of GTE on inflammatory cells in the BALF, neutrophils, lymphocytes, macrophages, and total cell counts were observed. As shown in Figure 1B, the inflammatory cell count of the OVA group was significantly higher than that of the NC group (p < 0.01). In contrast, the DEX group exhibited a marked decrease in the number of inflammatory cells compared to the OVA group (p < 0.01). The GTE-treated mice (100 and 400 mg/kg/day) had a reduced number of inflammatory cells compared to the OVA group (p < 0.01).
To evaluate the effect of GTE on ROS production, inflammatory cells were treated with DCFDA. As shown in Figure 1C, ROS level was markedly elevated in the OVA group compared with those in the NC group. In contrast, the GTE100 and GTE400 groups showed dose-dependently reduced ROS levels compared to the OVA group.
Effect of GTE on pulmonary inflammation and mucus production in lung tissue of asthmatic mice
As shown in Figure 2A, H&E-stained lung sections of asthmatic mice showed an accumulation of inflammatory cells in peribronchiolar lesions compared to the NC group (p < 0.01). The lung tissues of DEX-treated mice showed a reduction in inflammatory cell infiltration caused by OVA exposure (p < 0.01). Similar to DEX-treated mice, GTE-treated mice (100 and 400 mg/kg/day) showed dose-dependent inhibition of inflammatory cell infiltration (p < 0.05 and p < 0.01, respectively). As indicated in Figure 2B, mucus overproduction (positive: stained purple) was significantly increased in the airways of OVA mice compared to that in NC mice (p < 0.01), whereas lung sections from GTE (100 and 400 mg/kg/day) mice showed dose-dependently decreased mucus production (p < 0.01) compared to that in OVA mice. The suppression of mucus production was similar to that observed in the DEX group.
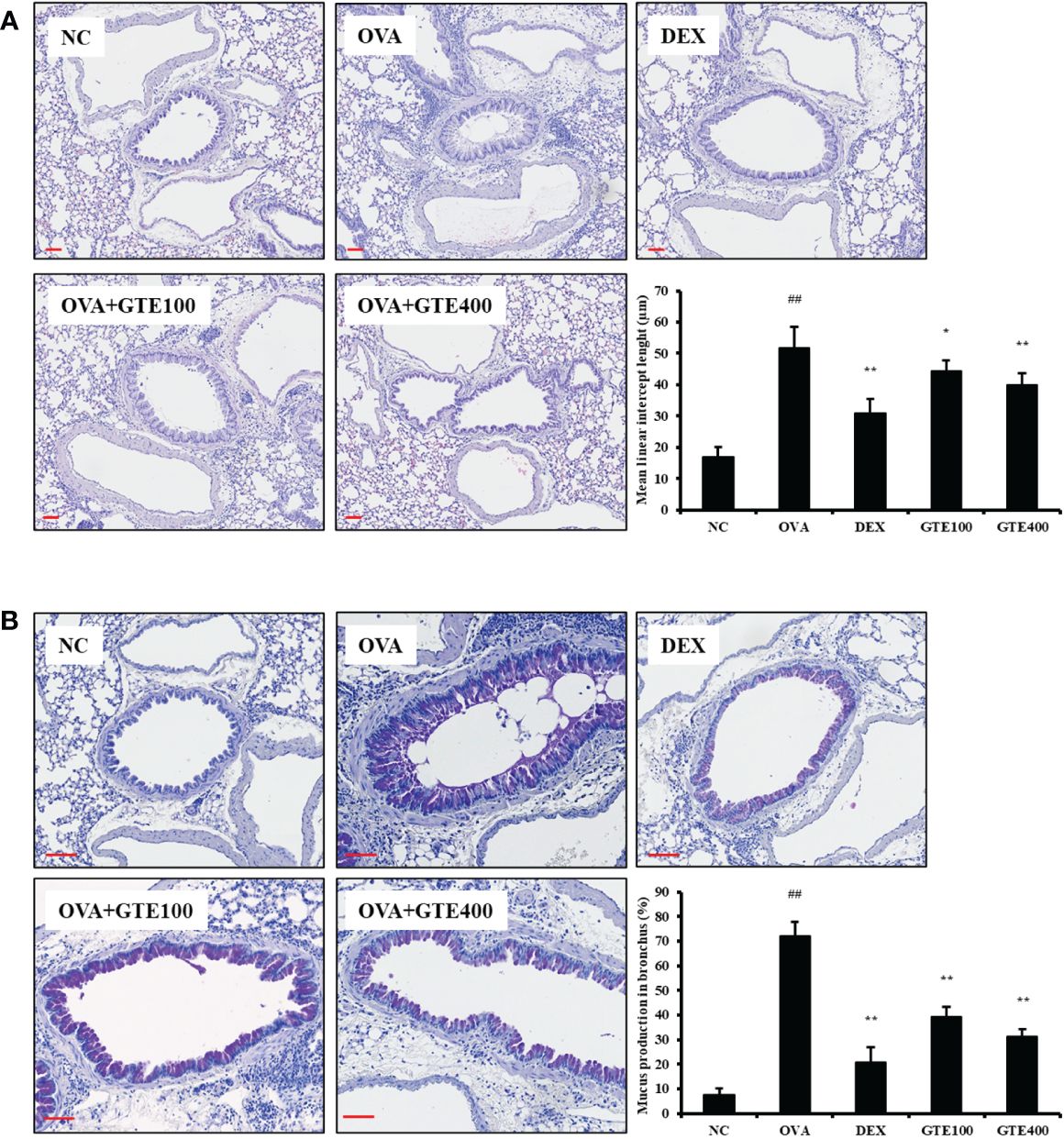
Figure 2 Green tea extract (GTE) inhibits the inflammation (A) and mucus overproduction (B) in lungs after ovalbumin (OVA) administration. Values are shown as means ± SD (n = 8). Significant differences: ##p < 0.01 vs. NC; *, **p < 0.05 and p < 0.01 vs. OVA, respectively. Scale bar = 100 µm.
Inhibitory effect of GTE on serum IgE and Th2 cytokines in BALF of OVA-challenged mice
IL-4, IL‐5, and IL‐13 levels in the OVA group were significantly higher than those in the NC group (Figures 3A–C, p < 0.01). In contrast, administration of DEX (p < 0.01) or GTE (400 mg/kg/day; p < 0.01) reduced the cytokine levels compared to those in the OVA group. Compared to the NC group, the OVA group showed a marked elevation in OVA-specific IgE and total IgE in serum (Figures 3D, E; p < 0.01). However, DEX (p < 0.01) and GTE (400 mg/kg/day; p < 0.01) administration significantly reduced OVA-specific IgE and total IgE levels in the serum in a dose-dependent manner compared with the OVA group.
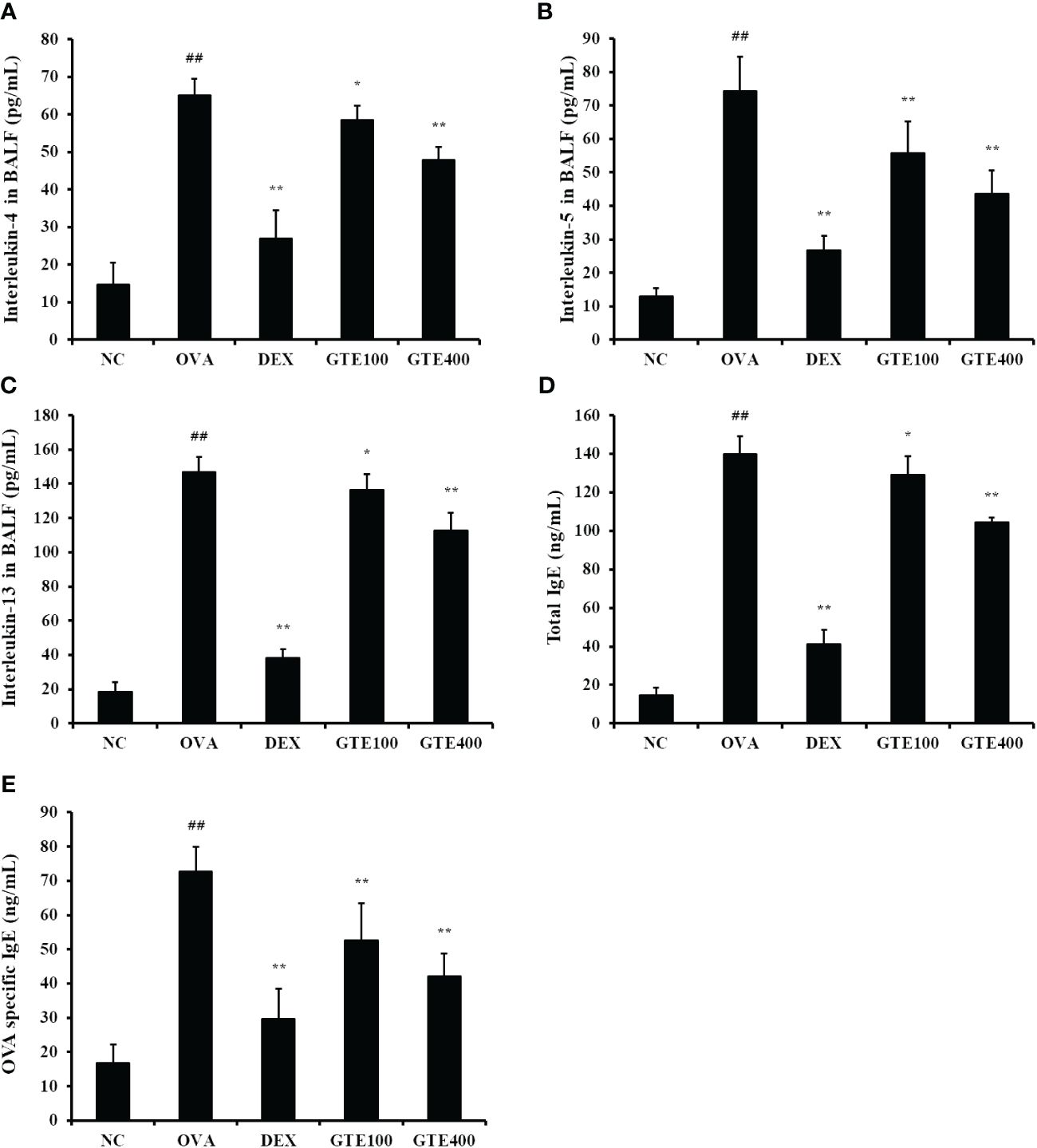
Figure 3 Green tea extract (GTE) inhibits the increased levels of interleukin (IL)-4 (A), IL-5 (B), IL-13 (C) in the bronchoalveolar lavage fluid (BALF), and total immunoglobulin (Ig) E (D) and ovalbumin (OVA)-specific IgE (E) in serum. Values are shown as means ± SD (n = 8). Significant differences: ##p < 0.01 vs. NC; *, **p < 0.05 and p < 0.01 vs. OVA, respectively.
Effects of GTE on MMP-9 expression in lung tissue of OVA-induced asthmatic mice
To evaluate MMP‐9 protein expression levels, IHC staining and western blotting of lung tissues were performed (Figures 4A, B). As shown in Figure 4B, OVA-induced asthmatic mice showed a significantly higher level of MMP‐9 (p < 0.01) than normal control mice. In contrast, the GTE-treated mice (100 and 400 mg/kg/day) exhibited significantly lower level of MMP‐9 (p < 0.05 and p < 0.01, respectively) than the OVA mice. Gelatin zymography was performed to assess the MMP-9 activity (Figure 4C). Similar to the IHC and western blot results, the OVA group exhibited significantly higher MMP-9 activity (p < 0.01) than the control mice. However, GTE-treated mice (100 and 400 mg/kg/day) exhibited dose-dependently decreased the activity of MMP‐9 (p < 0.05 and p < 0.01) compared to the OVA mice.
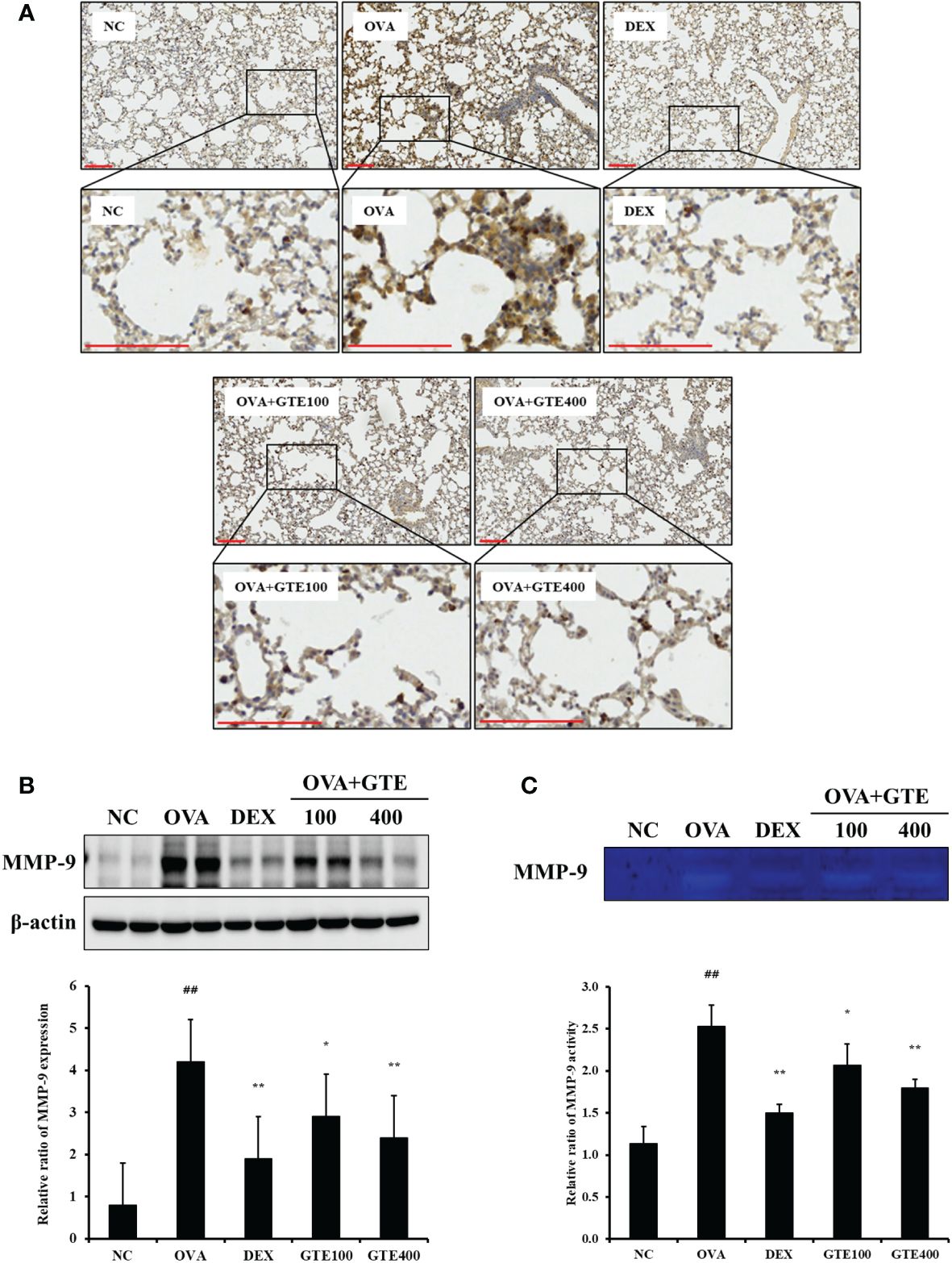
Figure 4 Green tea extract (GTE) reduces matrix metalloproteinase (MMP)-9 levels in lung tissue as confirmed by immunohistochemistry (A) and western blotting (B). GTE alleviated MMP-9 activity as confirmed by zymography (C). Values are shown as means ± SD (n = 8). Significant differences: ##p < 0.01 vs. NC; *, **p < 0.05 and p < 0.01 vs. OVA, respectively. Scale bar = 100 µm.
Effects of GTE on the MAPKs inflammatory pathway
As indicated in Figures 5A, B, the OVA mice showed significantly increased phosphorylation of ERK, JNK, and p38 compared to normal control mice (p < 0.01). In contrast, the elevated MAPKs phosphorylation was significantly inhibited by DEX and 400 mg/kg/day GTE (p < 0.01).
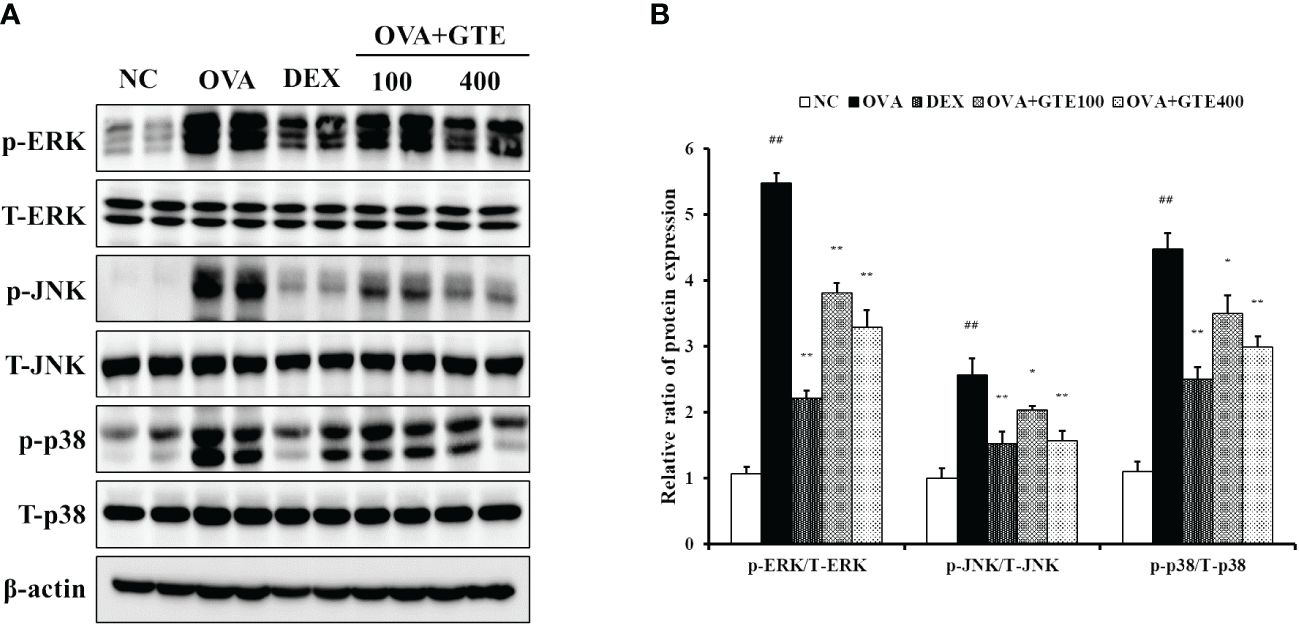
Figure 5 Green tea extract (GTE) suppresses ERK, JNK, and p38 phosphorylation (A). Densitometric values were calculated using ChemiDoc (B). Values are shown as means ± SD (n = 8). Significant differences: ##p < 0.01 vs. NC; *, **p < 0.05 and p < 0.01 vs. OVA, respectively.
Effect of GTE on antioxidant markers in the lung tissue of mice
As shown in Figures 6A–E, the mean activities of CAT, GR, and GSH, and the mean SOD level in the lung tissue homogenates of the OVA group were significantly lower than those in the control group. The GTE400 group showed significantly elevated CAT, GR, and SOD activities, and GSH level compared to the OVA group (p < 0.01). In contrast, elevated MDA level observed in the OVA group was dose-dependently decreased in the GTE100 and GTE400 groups, respectively (p < 0.05 and p < 0.01).
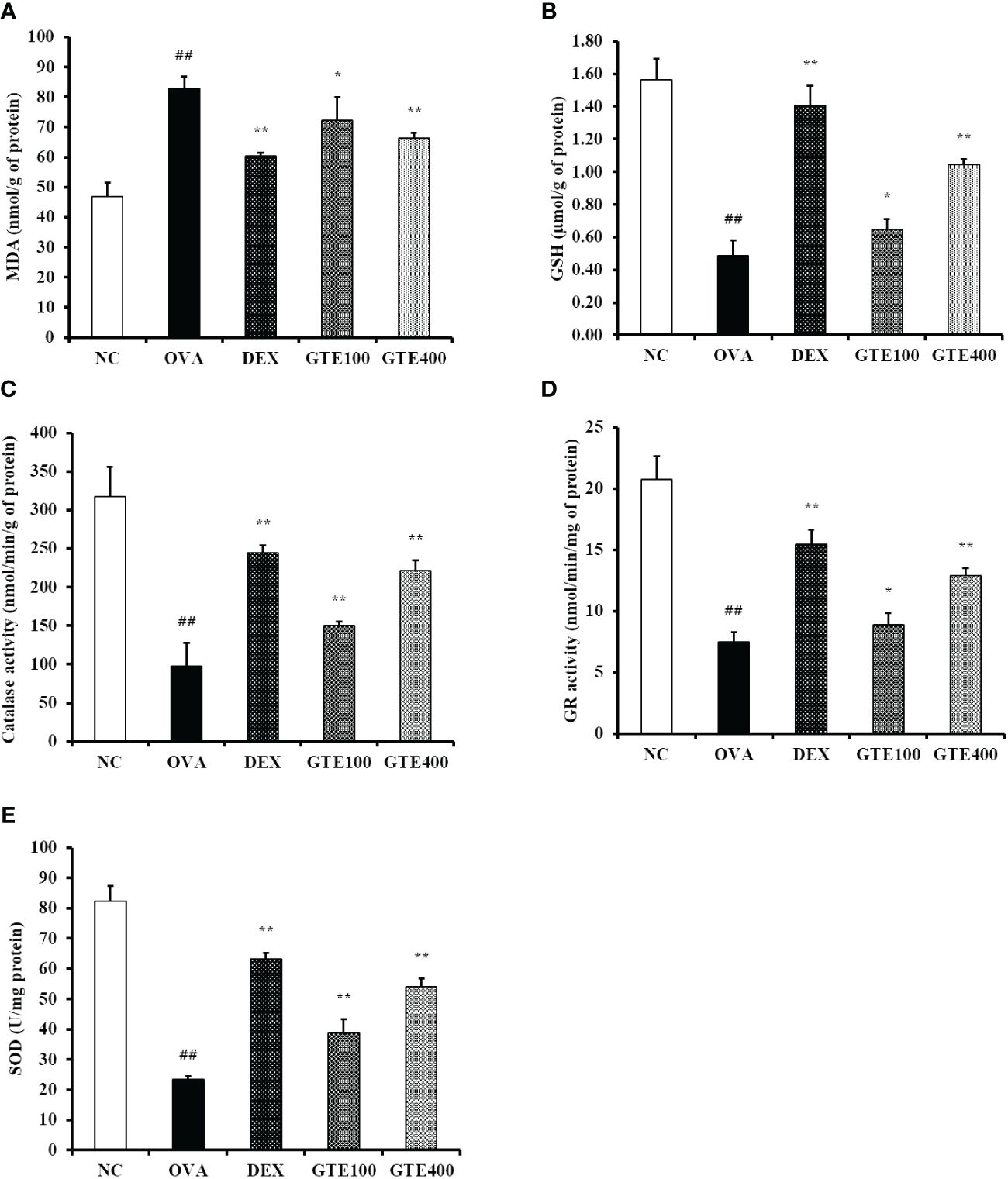
Figure 6 Green tea extract (GTE) reduces oxidative stress in lung tissue. (A) Malondialdehyde (MDA), (B) glutathione (GSH), (C) catalase (CAT), (D) glutathione reductase (GR), (E) superoxide dismutase (SOD) levels. Values are shown as means ± SD (n = 8). Significant differences: ##p < 0.01 vs. NC; *, **p < 0.05 and p < 0.01 vs. OVA, respectively.
In vitro experiments
Effect of GTE on mRNA expression of proinflammatory cytokines in LPS-stimulated NCI-H292 cells
GTE and EGCG had no cytotoxic effect to NCI-H292 cells at concentrations up to 80 μg/mL (Figures 7A, B). Therefore, up to 40 μg/mL of GTE and 20 μg/mL of EGCG were used in subsequent experiments. LPS-treated cells had statistically elevated mRNA levels of TNF-α, IL-1β, and IL-6 compared to untreated cells (Figures 7C–H, p < 0.01). However, LPS-stimulated mRNA expressions of these proinflammatory cytokines were dose-dependently decreased by treatment with GTE (Figures 7C–E). Also, LPS-stimulated mRNA expression of these proinflammatory cytokines was significantly decreased by treatment with 20 μg/mL of EGCG (Figures 7F–H, p < 0.01).
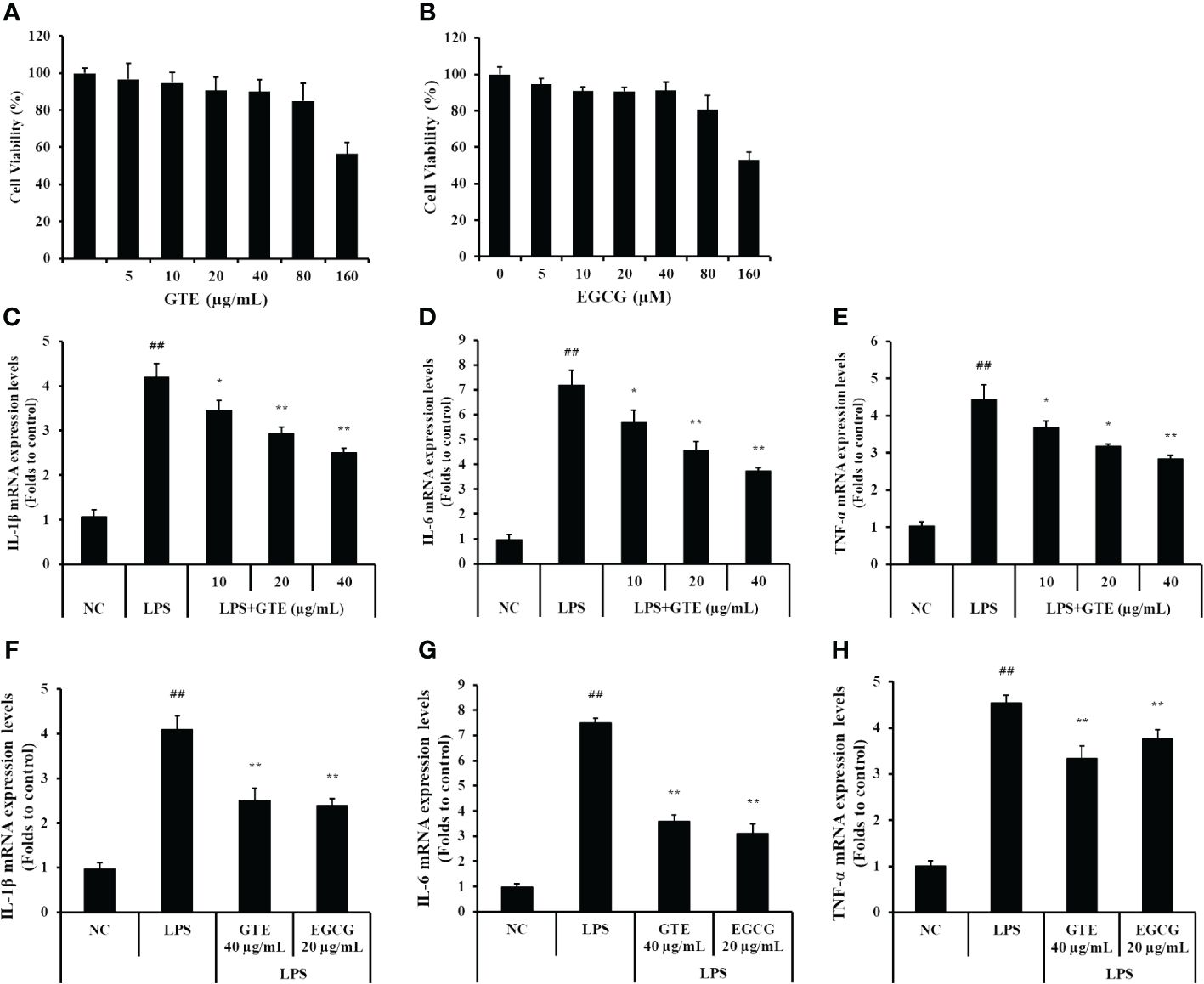
Figure 7 The concentration of (A) green tea extract (GTE) and (B) epigallocatechin gallate (EGCG) used in the experiment was determined based on the cytotoxicity results in NCI-H292 cells. GTE and EGCG reduced the increased levels of (C, F) interleukin (IL)-1β, (D, G) IL-6, (E, H) tumor necrosis factor (TNF)-α in the lipopolysaccharide (LPS)-stimulated cells. Cells were pretreated with 10, 20, and 40 µg/mL of GTE or 20 µg/mL of EGCG before LPS (0.5 µg/mL) treatment. Values are shown as means ± SD (n = 3). Significant differences: ##p < 0.01 vs. NC; *, **p < 0.05 and p < 0.01 vs. LPS, respectively.
Effects of GTE on the MAPKs pathway in LPS-treated NCI-H292 cells
LPS-treated cells showed elevated phosphorylation of MAPKs compared to untreated cells (Figures 8A, B). However, GTE-treated cells (40 μg/mL) showed a significant reduction of MAPKs phosphorylation compared with LPS-treated cells (p < 0.01). The expression pattern of MMP-9 was similar to that of MAPKs. LPS-treated cells showed a significant increase in MMP-9 expression, whereas GTE-treated cells showed a dose-dependent decrease compared to LPS-stimulated cells. GTE (40 μg/mL) and EGCG (20 μg/mL) showed similar results on the expression of MAPKs and MMP-9 (Figures 8C, D). Treatment of NCI-H292 cells with GTE and MAPKs inhibitors significantly reduced MAPKs phosphorylation compared to that in NCI-H292 cells treated with only MAPKs inhibitors (Figures 9A. B).
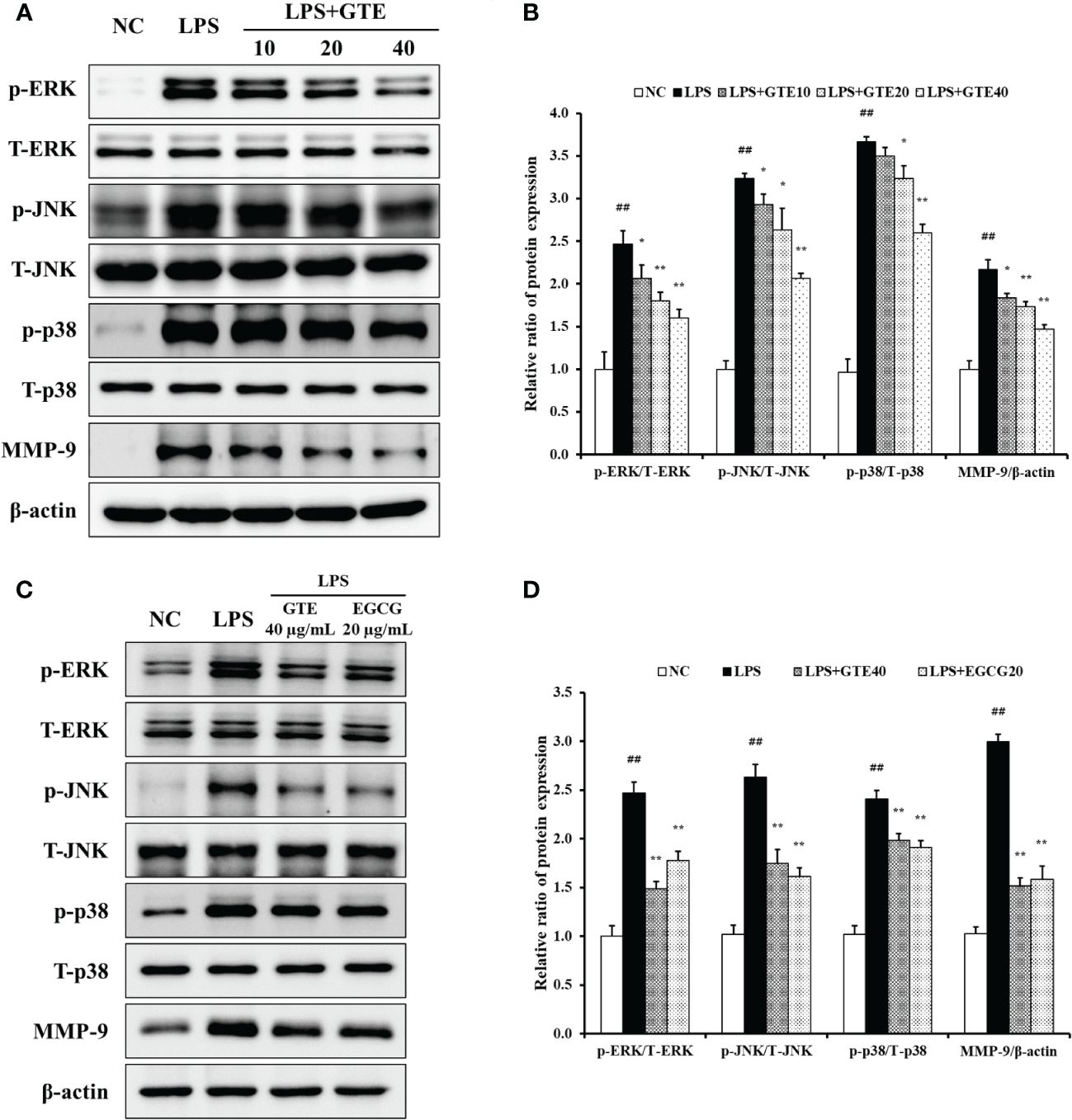
Figure 8 Green tea extract (GTE) inhibits dose-dependently increased ERK, JNK, and p38 phosphorylation and matrix metalloproteinase (MMP)-9 expression (A). GTE (40 µg/mL) and epigallocatechin gallate (EGCG, 20 μg/mL) suppress increased ERK, JNK, and p38 phosphorylation and matrix metalloproteinase (MMP)-9 expression (C). Densitometric values were calculated using ChemiDoc (B, D). Cells were pretreated with 10, 20, and 40 µg/mL of GTE before LPS (0.5 µg/mL) treatment. Values are shown as means ± SD (n = 3). Significant differences: ##p < 0.01 vs. NC; *, **p < 0.05 and p < 0.01 vs. LPS, respectively.
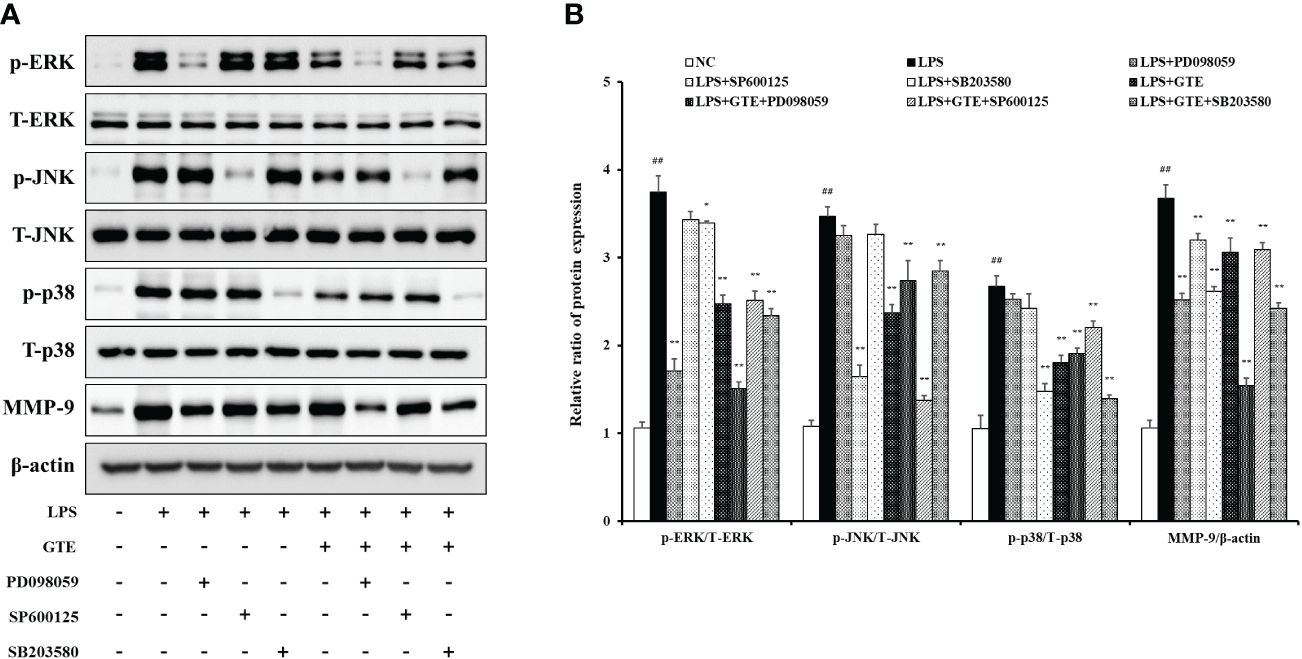
Figure 9 Effects of green tea extract (GTE) on the mitogen-activated protein kinase (MAPK) pathway in terms of matrix metalloproteinase (MMP)-9 production in lipopolysaccharide (LPS)-stimulated NCI-H292 cells. Cells were pretreated with 40 µg/mL of GTE, and 10 µM of PD098059, SP600125, and SB203680 before LPS (0.5 µg/mL) treatment (A). Densitometric values were calculated using ChemiDoc (B). Values are shown as means ± SD (n = 3). Significant differences: ##p < 0.01 vs. NC; *, **p < 0.05 and p < 0.01 vs. LPS, respectively.
Discussion
Asthma is a chronic pulmonary disease that causes various symptoms, such as respiratory inflammation, excessive mucus secretion, and AHR (19). In this study, we examined the therapeutic effects of GTE treatment in an OVA-induced mouse model of asthma, focusing on the oxidative stress-mediated inflammatory response and its underlying mechanisms. GTE effectively decreased proinflammatory cell counts and cytokine levels in the BALF and reduced OVA-specific IgE production in the serum. These preventive effects corresponded to a reduction in AHR and mucus production in the lungs of OVA-challenged mice. Furthermore, GTE treatment notably improved antioxidant enzyme activities followed by suppressed phosphorylation of JNK, ERK, p38 and expression of MMP‐9 increased by OVA inhalation.
Th2 cytokines are major contributors to the pathogenesis of allergic diseases, including asthma (20). Numerous clinical and preclinical studies have demonstrated a relationship between Th2 lymphocytes and the pathogenesis of asthma, including mucus hypersecretion and inflammation (3). In current study, GTE significantly suppressed the levels of Th2 cytokines, OVA‐specific IgE in serum, and inflammatory cell counts in BALF, and these effects of GTE corresponded with the histological findings in lung tissue, such as decreased inflammatory response and mucus secretion. These findings indicated that GTE significantly inhibited the pathogenesis of asthma by reducing Th2 cytokine levels.
The MAPKs pathway plays a key role in the downstream signaling events that lead to inflammation (21). Several studies have shown that phosphorylation of MAPKs triggers inflammatory and immune responses by regulating the production of TNF-α, IL-6, and other inflammatory factors in asthma (22). Previous studies have shown that ROS production causes cellular oxidative stress, leading to the activation of MAPKs. In particular, Bao and collaborators (23) reported that ozone-driven oxidative stress induced phosphorylation p-38 in an allergic asthma model, and Ma and collaborators (24) confirmed that oxidative stress activated MAPKs signaling in an OVA-challenged mouse model of asthma. These findings strongly support the hypothesis that the pathogenicity of asthma involves oxidative stress-driven MAPKs phosphorylation. In this study, administration of GTE elevated GSH levels and anti-oxidative enzyme activities, and reduced MDA levels, a marker of lipid peroxidation. These effects were followed by a dose-dependent reduction in MAPKs phosphorylation in OVA-induced asthmatic mice. Therefore, reduced oxidative stress and MAPKs signaling may be the mechanisms underlying the preventive effects of GTE on asthma development.
MMP‐9 belongs to the extracellular protease family and plays a key role in airway remodeling, including extracellular matrix reorganization, regulation of protein degradation, and cell migration (25, 26). The activation of MMP-9 is closely associated with the phosphorylation of MAPKs, which occurs in an asthmatic response (7). Lim and collaborators (27) reported that phosphorylation of MAPKs activated MMP-9 expression in OVA-challenged mice, and that MMP-9 levels were higher in samples from patients with allergic asthma, which were eliminated by MAPKs inhibitors (28). In the current study, the administration of GTE significantly suppressed the levels and activity of MMP‐9 as showed by IHC, western blotting, and zymography. These findings indicated that GTE reduced Th2 cytokine release and inhibited MMP-9 levels by decreasing MAPKs phosphorylation.
The protective effect of GTE in asthma is considered to be related with ROS production. Green tea has been studied for its antioxidant activity in various lung diseases (29–31). GTE reduces GSH, SOD and CAT levels in the lungs of a cigarette smoke-induced lung injury rat model (29, 30). Also, GTE suppresses paraquat-induced lung fibrosis in mouse via inhibiting nitric oxide production (31). Especially, polyphenols and catechins in GTE have a strong antioxidant effect, and polysaccharides also have a dose-dependent superoxide scavenging activity (32). These studies strongly support our results.
In conclusion, GTE treatment significantly suppressed Th2 cytokine production and inflammation in OVA-induced asthmatic mice and human lung epithelial cells. This effect of GTE was associated with the inhibition of oxidative stress-mediated MAPKs phosphorylation and MMP-9 inhibition. Therefore, the findings of this study suggest that GTE could be a potential therapeutic supplement for allergic asthma.
Data availability statement
The original contributions presented in the study are included in the article/Supplementary Material., further inquiries can be directed to the corresponding author/s.
Ethics statement
The animal study was approved by Animal Experiments of Chungnam National University. The study was conducted in accordance with the local legislation and institutional requirements.
Author contributions
J-WKi: Investigation, Writing – original draft. J-HK: Formal analysis, Writing – review & editing. J-SJ: Formal analysis, Writing – review & editing. C-YK: Formal analysis, Writing – review & editing. E-HC: Formal analysis, Writing – review & editing. E-JH: Writing – review & editing. H-JK: Writing – review & editing. S-HK: Writing – review & editing. J-WKo: Conceptualization, Funding acquisition, Supervision, Writing – review & editing. T-WK: Conceptualization, Funding acquisition, Supervision, Writing – review & editing.
Funding
The author(s) declare financial support was received for the research, authorship, and/or publication of this article. This work was supported by the research fund of Basic Science Research Program through the National Research Foundation of Korea (NRF) funded by the Ministry of Education (NRF-2021R1I1A3050864). This work was supported by a National Research Foundation of Korea (NRF) grant funded by the Korean government (MSIT) (2021R1A4A1033078).
Conflict of interest
The authors declare that the research was conducted in the absence of any commercial or financial relationships that could be construed as a potential conflict of interest.
Publisher’s note
All claims expressed in this article are solely those of the authors and do not necessarily represent those of their affiliated organizations, or those of the publisher, the editors and the reviewers. Any product that may be evaluated in this article, or claim that may be made by its manufacturer, is not guaranteed or endorsed by the publisher.
Supplementary material
The Supplementary Material for this article can be found online at: https://www.frontiersin.org/articles/10.3389/fimmu.2024.1362404/full#supplementary-material
References
1. Stern J, Pier J, Litonjua AA. Asthma epidemiology and risk factors. Semin Immunopathol. (2020) 42:5–15. doi: 10.1007/s00281-020-00785-1
2. Saeki M, Nishimura T, Kitamura N, Hiroi T, Mori A, Kaminuma O. Potential mechanisms of T cell-mediated and eosinophil-independent bronchial hyperresponsiveness. Int J Mol Sci. (2019) 20:2980. doi: 10.3390/ijms20122980
3. León B, Ballesteros-Tato A. Modulating Th2 cell immunity for the treatment of asthma. Front Immunol. (2021) 12:637948. doi: 10.3389/fimmu.2021.637948
4. Khorasanizadeh MH, Eskian M, Gelfand EW, Rezaei N. Mitogen-activated protein kinases as therapeutic targets for asthma. Pharmacol Ther. (2017) 174:112–26. doi: 10.1016/j.pharmthera.2017.02.024
5. Toujani S, Mehiri N, Hamzaoui K, Hadded H, Ben SN, Mjid M, et al. Role of metalloproteinases MMP-2 in asthma. Tunis Med. (2016) 94:167–71.
6. Lee YC, Lee HB, Rhee YK, Song CH. The involvement of matrix metalloproteinase-9 in airway inflammation of patients with acute asthma. Clin Exp Allergy. (2001) 31:1623–30. doi: 10.1046/j.1365-2222.2001.01211.x
7. Lee JI, Kil JH, Yu GH, Karadeniz F, Oh JH, Seo Y, et al. 3,5-Dicaffeoyl-epi-quinic acid inhibits the PMA-stimulated activation and expression of MMP-9 but not MMP-2 via downregulation of MAPK pathway. Z Naturforsch C J Biosci. (2020) 75:113–20. doi: 10.1515/znc-2019-0163
8. Kim CY, Kim JW, Kim JH, Jeong JS, Lim JO, Ko JW, et al. Inner shell of the chestnut (Castanea crenatta) suppresses inflammatory responses in ovalbumin-induced allergic asthma mouse model. Nutrients. (2022) 14:2067. doi: 10.3390/nu14102067
9. Reygaert WC. Green tea catechins: Their use in treating and preventing infectious diseases. BioMed Res Int. (2018) 2018:9105261. doi: 10.1155/2018/9105261
10. Kim JW, Kim CY, Kim JH, Jeong JS, Lim JO, Ko JW, et al. Prophylactic catechin-rich green tea extract treatment ameliorates pathogenic enterotoxic Escherichia coli-induced colitis. Pathogens. (2021) 10:1573. doi: 10.3390/pathogens10121573
11. Singh N, Kulkarni GT, Kumar Y. Montelukast sodium formulation containing green tea extract to reduce the oxidative stress in Guinea pig model of chronic allergic asthma. Recent Pat Drug Delivery Formul. (2018) 12:267–76. doi: 10.2174/1872211313666181211123903
12. Wu SY, Silverberg JI, Joks R, Durkin HG, Smith-Norowitz TA. Green tea (Camelia sinensis) mediated suppression of IgE production by peripheral blood mononuclear cells of allergic asthmatic humans. Scand J Immunol. (2012) 76:306–10. doi: 10.1111/j.1365-3083.2012.02729.x
13. Musial C, Kuban-Jankowska A, Gorska-Ponikowska M. Beneficial properties of green tea catechins. Int J Mol Sci. (2020) 21:1744. doi: 10.3390/IJMS21051744
14. Choi YS, Bae CH, Song SY, Kim YD. The effect of epigallocatechin-3-gallate in allergic airway inflammation. Rhinology. (2014) 52:1–7. doi: 10.4193/rhino13.189
15. Yang N, Shang YX. Epigallocatechin gallate ameliorates airway inflammation by regulating Treg/Th17 imbalance in an asthmatic mouse model. Int Immunopharmacol. (2019) 72:422–8. doi: 10.1016/j.intimp.2019.04.044
16. Yang N, Zhang H, Cai X, Shang Y. Epigallocatechin-3-gallate inhibits inflammation and epithelial-mesenchymal transition through the PI3K/AKT pathway via upregulation of PTEN in asthma. Int J Mol Med. (2018) 41:818–28. doi: 10.3892/ijmm.2017.3292
17. Kim JH, Kim JW, Kim CY, Jeong JS, Lim JO, Ko JW, et al. Korean red ginseng ameliorates allergic asthma through reduction of lung inflammation and oxidation. Antioxidants (Basel). (2022) 11:1422. doi: 10.3390/antiox11081422
18. Ko JW, Kwon HJ, Seo CS, Choi SJ, Shin NR, Kim SH, et al. 4-Hydroxycinnamic acid suppresses airway inflammation and mucus hypersecretion in allergic asthma induced by ovalbumin challenge. Phytother Res. (2020) 34:624–33. doi: 10.1002/ptr.6553
19. Hirose K, Iwata A, Tamachi T, Nakajima H. Allergic airway inflammation: key players beyond the Th2 cell pathway. Immunol Rev. (2017) 278:145–61. doi: 10.1111/imr.12540
20. Bosnjak B, Stelzmueller B, Erb KJ, Epstein MM. Treatment of allergic asthma: Modulation of Th2 cells and their responses. Respir Res. (2011) 12:1–17. doi: 10.1186/1465-9921-12-114
21. Thalhamer T, McGrath MA, Harnett MM. MAPKs and their relevance to arthritis and inflammation. Rheumatol (Oxford). (2008) 47:409–14. doi: 10.1093/rheumatology/kem297
22. Athari SS. Targeting cell signaling in allergic asthma. Signal Transduct Target Ther. (2019) 4:1–19. doi: 10.1038/s41392-019-0079-0
23. Bao A, Yang H, Ji J, Chen Y, Bao W, Li F, et al. Involvements of p38 MAPK and oxidative stress in the ozone-induced enhancement of AHR and pulmonary inflammation in an allergic asthma model. Respir Res. (2017) 18:1–12. doi: 10.1186/s12931-017-0697-4
24. Ma Y, Ge A, Zhu W, Liu YN, Ji NF, Zha WJ, et al. Morin attenuates ovalbumin-induced airway inflammation by modulating oxidative stress-responsive MAPK signaling. Oxid Med Cell Longev. (2016) 2016:5843672. doi: 10.1155/2016/5843672
25. Chauhan PS, Dash D, Singh R. Intranasal curcumin inhibits pulmonary fibrosis by modulating matrix metalloproteinase-9 (MMP-9) in ovalbumin-induced chronic asthma. Inflammation. (2017) 40:248–58. doi: 10.1007/s10753-016-0475-3
26. Melamed D, Messika O, Glass-Marmor L, Miller A. Modulation of matrix metalloproteinase-9 (MMP-9) secretion in B lymphopoiesis. Int Immunol. (2006) 18:1355–62. doi: 10.1093/intimm/dxl068
27. Lim JO, Kim YH, Lee IS, Il KW, SJ L, SW P, et al. Cinnamomum cassia (L.) J.Presl alleviates allergic responses in asthmatic mice via suppression of MAPKs and MMP-9. Front Pharmacol. (2022) 13:906916. doi: 10.3389/fphar.2022.906916
28. Ventura I, Vega A, Chacõn P, Chamorro C, Aroca R, Gõmez E, et al. Neutrophils from allergic asthmatic patients produce and release metalloproteinase-9 upon direct exposure to allergens. Allergy. (2014) 69:898–905. doi: 10.1111/all.12414
29. Al-Awaida W, Akash M, Aburubaiha Z, Talib WH, Shehadeh H. Chinese green tea consumption reduces oxidative stress, inflammation, and tissues damage in smoke exposed rats. Iran J Basic Med Sci. (2014) 17:740.
30. Chan KH, Ho SP, Yeung SC, So WHL, Cho CH, Koo MWL, et al. Chinese green tea ameliorates lung injury in cigarette smoke-exposed rats. Respir Med. (2009) 103:1746–54. doi: 10.1016/j.rmed.2009.04.027
31. Kim HR, Park BK, Oh YM, Lee YS, Lee DS, Kim HK, et al. Green tea extract inhibits paraquat-induced pulmonary fibrosis by suppression of oxidative stress and endothelin-l expression. Lung. (2006) 184:287–95. doi: 10.1007/s00408-005-2592-x
Keywords: asthma, green tea extract, inflammation, matrix metalloproteinase-9, mitogen-activated protein kinase signaling, oxidative stress
Citation: Kim J-W, Kim J-H, Jeong J-S, Kim C-Y, Chung E-H, Kim S-H, Hong E-J, Kwon H-J, Ko J-W and Kim T-W (2024) Green tea extract suppresses airway inflammation via oxidative stress-driven MAPKs/MMP-9 signaling in asthmatic mice and human airway epithelial cells. Front. Immunol. 15:1362404. doi: 10.3389/fimmu.2024.1362404
Received: 28 December 2023; Accepted: 18 April 2024;
Published: 30 April 2024.
Edited by:
Carmen Panaitescu, Victor Babes University of Medicine and Pharmacy, RomaniaReviewed by:
Renping Liu, Nanchang University, ChinaGeeta Devi Leishangthem, Guru Angad Dev Veterinary and Animal Sciences University, India
Copyright © 2024 Kim, Kim, Jeong, Kim, Chung, Kim, Hong, Kwon, Ko and Kim. This is an open-access article distributed under the terms of the Creative Commons Attribution License (CC BY). The use, distribution or reproduction in other forums is permitted, provided the original author(s) and the copyright owner(s) are credited and that the original publication in this journal is cited, in accordance with accepted academic practice. No use, distribution or reproduction is permitted which does not comply with these terms.
*Correspondence: Je-Won Ko, cmhlb2RhQGNudS5hYy5rcg==; Tae-Won Kim, dGFld29ua2ltQGNudS5hYy5rcg==