- 1Department of Gynecology, The Second Affiliated Hospital, Zhejiang University School of Medicine, Hangzhou, China
- 2Zhejiang Provincial Clinical Research Center for Obstetrics and Gynecology, Hangzhou, China
- 3Department of Oncology, Tongji Hospital of Tongji Medical College, Huazhong University of Science and Technology, Wuhan, China
- 4Department of Radiation Oncology, Hubei Cancer Hospital, Tongji Medical College, Huazhong University of Science and Technology, Wuhan, China
- 5Department of Breast Surgery, The First Affiliated Hospital, College of Medicine, Zhejiang University, Hangzhou, China
Natural Killer (NK) cells, intrinsic to the innate immune system, are pivotal in combating cancer due to their independent cytotoxic capabilities in antitumor immune response. Unlike predominant treatments that target T cell immunity, the limited success of T cell immunotherapy emphasizes the urgency for innovative approaches, with a spotlight on harnessing the potential of NK cells. Despite tumors adapting mechanisms to evade NK cell-induced cytotoxicity, there is optimism surrounding Chimeric Antigen Receptor (CAR) NK cells. This comprehensive review delves into the foundational features and recent breakthroughs in comprehending the dynamics of NK cells within the tumor microenvironment. It critically evaluates the potential applications and challenges associated with emerging CAR-NK cell therapeutic strategies, positioning them as promising tools in the evolving landscape of precision medicine. As research progresses, the unique attributes of CAR-NK cells offer a new avenue for therapeutic interventions, paving the way for a more effective and precise approach to cancer treatment.
1 Background
Natural Killer (NK) cells are essential contributors to the immune response, demonstrating formidable cytotoxic capabilities towards infected, stressed, transformed, and foreign cells (1). Belonging to the innate lymphoid cells (ILCs) family, NK/cells actively engage in the initial stages of host defense. They originate from CD34high hematopoietic stem cells (HSCs) in the bone marrow (2). The developmental stages involve differentiation from HSCs toward common lymphoid progenitors, progressing to NK cell progenitors. Although the bone marrow serves as the primary site for NK cell development, extramedullary maturation takes place in secondary lymphoid tissues, including the thymus and lymph nodes. Mature NK cells originating in the bone marrow migrate towards secondary lymphoid tissues and peripheral organs (3). The maturation process is marked by changes in surface markers, with CD56 expression indicating distinct functional properties (4). CD56bright NK cells are primarily cytokine releasers, evolving into cytotoxic CD56dim NK cells with further maturation, acquiring type III Fcγ receptor CD16 and killer-cell immunoglobulin-like receptors (KIRs) (5). Memory-like features in NK cells are observed following exposure to cytokines like IL-12, IL-15, and IL-18. These memory-like NK cells exhibit improved activation upon re-exposure to stimulation, contributing to adaptive immune responses (6–10).
Mature NK cells undergo orchestrated migration from the bone marrow to SLTs and various peripheral non-lymphoid organs. This migration is regulated by a myriad of molecules, such as chemokines, integrins, and selectins. The receptors S1P5 and CX3CR1, along with downregulated CXCR4, facilitate NK cell exit from bone marrow into the bloodstream (11). Specific receptors and ligands dictate NK cell homing to organs. For example, CCR7 engagement with CCL19 and CCL21 is crucial for NK cell homing to lymph nodes (12). NK cells are found in various organs, each exhibiting distinct trafficking patterns. The liver, lungs, intestine, uterus, and even the central nervous system (CNS) are infiltrated by NK cells, contributing to immune surveillance (13, 14). The local environment of liver involves both resident and recruited NK cells, promoting tolerance to avoid chronic inflammation. Chemokine receptors like CXCR6 play an important role in NK cell retention in the liver, and similar mechanisms guide NK cell infiltration in other organs, including the decidua and lungs (15–17).
NK cells contribute significantly to the defense mechanisms of the host by directly targeting and eliminating infected and transformed cells (18, 19). Additionally, they release cytokines that coordinate the actions of various immune subsets. Importantly, the killing mechanism employed by NK cells is distinct from that of adaptive T lymphocytes, as it operates without being constrained by human leukocyte antigen (HLA) restriction (20). The balance between suppressive and activating receptors on NK cells determines their status, with inhibitory receptors recognizing major histocompatibility complex (MHC) class I molecules on target cells (21). In the context of cancer biology, NK cells exhibit anti-tumor activity, targeting cells with low MHC-I expression and recognizing stress-induced ligands. Inhibitory receptors, including killer cell immunoglobulin-like receptors (KIRs) and CD94/NKG2A, contribute to NK cell education, ensuring self-tolerance (22). Activating receptors, such as NK gene 2D (NKG2D) and natural cytotoxicity receptors (NCRs), play crucial roles in NK cell responses against cancer cells (23, 24). NK cells can also contribute to cancer therapy through antibody-dependent cell-mediated cytotoxicity (ADCC) effects by CD16 receptors (25–27). The activity of NK cells is subject to intricate regulation by a balance of inhibitory and activating receptors, which together play a crucial role in the immune response of NK cells against tumors and foreign pathogens. This delicate equilibrium ensures that NK cells can discriminate between healthy cells and those presenting abnormalities, such as infected or malignantly transformed cells, contributing significantly to the body’s natural immune surveillance mechanisms. This comprehensive overview provides a nuanced understanding of NK cell development, particularly in cancer progression and therapeutic interventions. The integrated perspective highlights the multifaceted roles of NK cells in immune surveillance and their potential as therapeutic targets in cancer treatment.
2 The role of NK cells in cancer progression
NK cells, play a crucial role in the complex landscape of cancer development (28). Specifically implicated in hematopoietic tumors, these cells engage in direct interactions with tumor cells, marking them as promising candidates for therapeutic interventions in the battle against cancers (29). The significance of NK cells in cancer immunotherapy is underscored by their unique ability to recognize and eliminate abnormal cells without prior sensitization, a feature that sets them apart from other immune cells (29). Despite their potential, the practical application of NK cells in cancer therapy faces a myriad of challenges. One prominent hurdle is the limited infiltration of NK cells into solid tumors, a phenomenon that stands in stark contrast to the robust infiltration of cytotoxic T lymphocytes (CTLs) (30, 31). This discrepancy raises questions about the mechanisms that impede the effective interaction between NK cells and the tumor microenvironments (TME). Understanding these impediments is crucial for optimizing NK cell-based therapeutic strategies.
A multifaceted obstacle to NK cell infiltration lies in the intricate architecture of the TME. The solid-tumoral contact and subsequent infiltration of NK cells are thwarted by various factors, contributing to a significantly lower density of infiltrated NK cells when compared to CTLs (31). The hypoxic conditions within the TME, characterized by increased levels of hypoxia-inducible factor 1 alpha (HIF-1α), emerge as a significant player in blunting NK cell activity against solid tumors (32). HIF-1α not only contributes to the immunosuppressive milieu but also hampers the efficacy of NK cell-mediated anti-tumor responses (32). Adding another layer of complexity to the interaction between NK cells and tumors, recent research has provided insights into the role of cancer-derived exosomes in undermining NK cell function (33). These extracellular vesicles, secreted by cancer cells, carry bioactive molecules that can modulate the immune response. The impact of cancer-derived exosomes on NK cells further emphasizes the need for a comprehensive understanding of the intricate crosstalk within the TME (33).
NK cells face the challenge of overcoming these obstacles and successfully infiltrate solid tumors. First, they must extravasate from the bloodstream, navigating through the stiff extracellular matrix (ECM) and tumor stroma. This process involves the secretion of enzymes such as urokinase plasminogen activator, matrix metalloproteinases, and serine dipeptidyl peptidase IV (34). Additionally, heparinase plays a crucial role in this process, emphasizing the complexity of the microenvironment that NK cells must navigate (35). However, even when NK cells overcome these physical barriers and reach the tumor site, their journey is far from over (36, 37). The TME, molded by various immunosuppressive factors, transforms arriving NK cells into a state of immunosuppression. This transformation involves exposure to a plethora of immunosuppressive cytokines, including transforming growth factor-beta (TGF-β), activin-A, adenosine, IL-10, and prostaglandin E2 (PGE2) (38–43). Additionally, the TME harbors inhibitory immune cells that further hamper the functions of NK cell within solid tumors (44–47).
Despite these challenges, the infiltration of NK cells into the TME is associated with a relatively favorable and promising prognosis in numerous cancers (48–50). This observation highlights the role of NK cells in cancer initiation and progression, while facing formidable barriers, their presence within the TME signifies a potential positive outcome. Mouse models provide further evidence of anti-tumor capabilities, revealing that NK cells actively suppress tumor occurrence through immunosurveillance (51–53). Understanding the mechanism of NK cell activation is fundamental to unraveling their role in tumor occurrence and development. The activation process within the TME involves a complex interplay of activating and inhibiting receptors, along with various cytokines and their corresponding ligands or receptors (54–56). These receptors allow NK cells to specifically recognize biomarkers presented on the cancer cell membrane, setting the stage for either tumor surveillance or the promotion of tumor immune escape.
The activation of NK cells culminates in their ability to eliminate tumor cells through multiple mechanisms. Direct release of perforin and granzymes, as well as the induction of apoptosis through ADCC effects, Fas ligand (FasL), or TNF-related apoptosis-inducing ligand (TRAIL), represents the tumor-killing toolkit of activated NK cells. Furthermore, the secretion of lymphokines, including IFN-γ and TNF-α, contributes to retard tumor growth across various cancer types (28). An intriguing facet of NK cell-mediated anti-tumor responses is the release of neoantigens upon the destruction of tumor cells. These neoantigens act as beacons, prompting an adaptive immune response that extends beyond the immediate actions of NK cells. This communicative bridge between innate and adaptive immunity involves dendritic cells (DCs), which play crucial roles in tumor immune responses. NK cells, in their activated state, promote the recruitment of conventional DCs to the TME, further enhancing the immune elimination of tumor cells (57).
While hurdles exist in their efficient infiltration into solid tumors and the subsequent immunosuppressive shaping by the TME, the potential for NK cells to act as potent tumor suppressors cannot be overlooked (58–60). Advancements in understanding the intricacies of NK cell activation, the complex TME, and innovative approaches like iPSC-derived NK cells collectively pave the way for novel therapeutic strategies (61–65). Harnessing the full potential of NK cells holds the promise of revolutionizing cancer treatment, particularly in the context of “cold” tumors that lack neoantigens, thereby surpassing conventional cancer therapies and ushering in a new era of precision immunotherapy (66).
3 Chimeric antigen receptor-engineered natural killer cells in cancer immunotherapy
In the realm of cancer treatment, immunotherapy has emerged as a revolutionary approach, particularly with the advent of Chimeric Antigen Receptor (CAR) technology. The emergence of CAR-NK cell therapy represents a promising shift in the landscape of cancer immunotherapy, offering potential solutions to the challenges faced by CAR-T cell therapy (67). While CAR-T cells have demonstrated clinical benefit in some specific hematological cancers, the field has encountered obstacles, including the time-intensive generation of therapeutic doses and the difficulty in obtaining sufficient autologous T cells from heavily pre-treated cancer patients (68–70). In response to these challenges, CAR-NK cell therapy emerges as an attractive alternative, showcasing distinct advantages (71).
One of the primary strengths of CAR-NK cells lies in their use of allogeneic NK sources, addressing concerns related to GVHD. Unlike CAR-T cells, CAR-NK cells can utilize an unlimited allogeneic NK source without triggering GVHD, providing a considerable safety advantage (72). Furthermore, CAR-NK therapy introduces the potential for “off-the-shelf” products, leveraging NK cell lines or iPSC-NK, resulting in a substantially shortened production time (73, 74). This efficiency is crucial for treating patients with rapidly progressing diseases, a limitation often encountered in CAR-T therapy. The versatility of CAR-NK cells is evident in their ability to target a broad range of tumor antigens in both hematological and solid malignancies (61, 75–82). The antigen recognition domain, typically consisting of a single-chain fragment derived from a monoclonal antibody, facilitates targeted therapy. While CD19/CD20/CD33 remain major targets for hematological cancers, CAR-NK cells have demonstrated efficacy against solid cancer targets, such as Her2, EpCAM, and EGFR, offering a broader spectrum of applicability (82–85).
Like CAR-T cells, CAR-NK cells undergo genetic modification to express CARs designed to recognize specific antigens present on target cells (Figure 1). The pre-clinical studies have employed various delivery methods, such as lentiviral or retroviral-based transduction, transposon systems, and electroporation of mRNA, highlighting the adaptability of CAR-NK technology (86). The signaling domains of CAR-NK cells closely resemble those of CAR-T cells, incorporating TCR co-stimulatory molecules, such as CD28, 4-1BB, NKG2D, 2B4, and DNAM1 (86). Notably, CAR-NK cells not only direct cytotoxicity against tumor cells by targeting specific antigens but also exhibit potential in eliminating immunosuppressive cells within the TME. This includes targeting myeloid-derived suppressor cells (MDSCs) and M2-like tumor-associated macrophages (TAMs), indicating a broader therapeutic impact beyond direct tumor cell killing (87, 88). The proposed combination of CAR-NK cells with T cell-based therapies for solid tumors further underscores their potential in reshaping cancer immunotherapy strategies. The safety profile of CAR-NK cells is a significant advantage, with allogeneic haploidentical NK cells demonstrating safety for adoptive cell therapy by reducing the risk of GVHD (89). Unlike CAR-T cells, CAR-NK cells exhibit fewer safety concerns, including on-target/off-tumor effects, cytokine release syndrome (CRS), and tumor lysis syndrome. The unique ability of CAR-NK cells to detect MHC class I-negative tumor cells further extends their applicability (90).
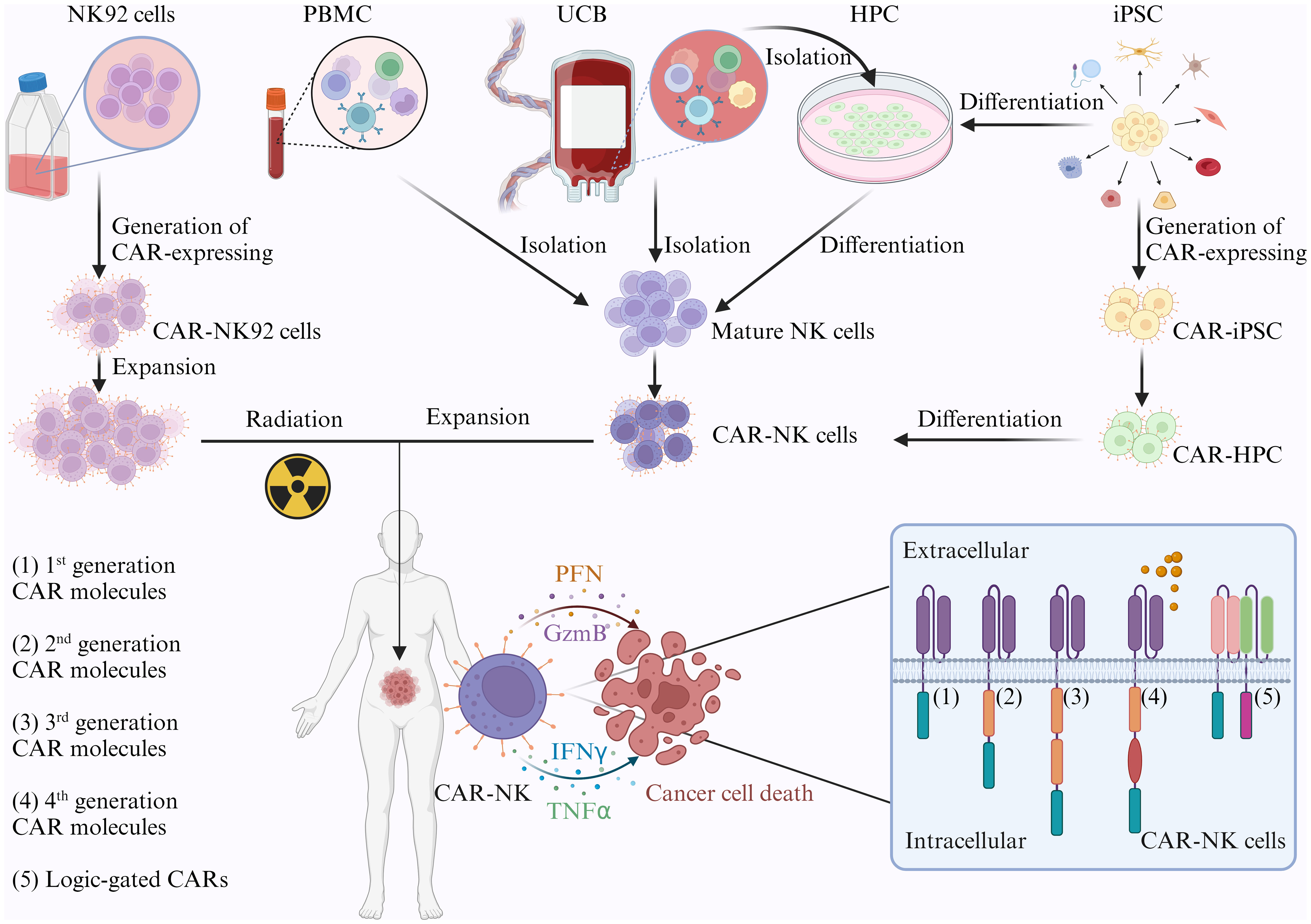
Figure 1 The development of CAR-NK cells. The NK92 cell line is commonly used due to its ability to indefinitely expand in vitro. Primary NK cells can be directly isolated from peripheral blood mononuclear cells (PBMCs) or umbilical cord blood (UCB) using a NK cell isolation kit. These cells are then activated, genetically modified with CAR-expressing vectors, and expanded in NK cell-specific media with cytokines for clinical use. CD34+ hematopoietic progenitor cells (HPCs) can be differentiated into NK cells with a cytokine cocktail, and these cells are engineered with CAR before in vitro expansion and infusion. Induced pluripotent stem cells (iPSCs) have emerged as a promising “off-the-shelf” source for CAR-NK cells, given their unlimited proliferative capacity. iPSCs can differentiate into CD34+ HPCs, then into NK cells. Importantly, CAR-expressing vectors can be introduced into iPSCs, leading to CAR-iPSCs, which can further differentiate into CAR-HPCs and CAR-NK cells (Created with Biorender).
CAR-NK cell-mediated immunotherapy has rapidly emerged as a compelling alternative for patients facing metastatic malignancies, showcasing considerable promise in the realm of cancer immunotherapy (91). Despite significant exploration of CAR-NK cells in preclinical studies, their applications in various tumor models are predominantly at the preclinical stage, showing both potential and the need for further investigation. Notably, clinically approved second-generation CAR-NK cells, incorporating the CD3ζ domain and a 4-1BB or CD28 co-stimulatory domain, have primarily targeted CD19+ lymphoid-derived hematologic malignancies (92).
Initially designed to combat hematological malignancies such as lymphoma, myeloma, and leukemia, CAR-NK cells have demonstrated efficacy in preclinical settings. CD19-CAR-NK cells, in particular, have exhibited superior efficiency in treating lymphoid malignancies compared to CAR-T-based cellular immunotherapy, showcasing their potential advantages (92). For instance, the phase 1/2 study NCT03056339 explored the efficacy of anti-CD19 CAR-NK cells, derived from cord blood, in 11 patients with relapsed or refractory CD19+ hematologic cancers (93). The CAR-NK cells, modified with a safety switch, were administered in varying doses after lymphodepleting chemotherapy (93). Results showed that CAR-NK cell treatment was well-tolerated, with no major toxic effects such as cytokine release syndrome or neurotoxicity (93). Of the 11 patients, 73% responded positively to the treatment, with 7 achieving complete remission (93). Responses were rapid, observed within 30 days, and CAR-NK cells persisted for at least 12 months. The study suggests the potential effectiveness and safety of CAR-NK cell therapy in CD19+ cancers (93).
Beyond hematological malignancies, CAR-NK cells hold promise for addressing metastatic solid tumors, where CAR-T cell therapy faces substantial limitations (94). With intrinsic advantages such as substantial cytolytic ability, non-MHC-restricted recognition, natural tumor tissue infiltration, and minimal untoward effects, CAR-NK cells present a viable therapeutic option for solid tumor management (94). Preclinical studies have demonstrated their efficacy against diverse solid tumors (95, 96). However, clinical data on CAR-NK cells in solid tumor treatment remains limited, with ongoing phase I/II trials showing feasibility and potential efficacy. Continued exploration and clinical trials are essential to unravel the safety and efficacy of CAR-NK cells in the dynamic landscape of cancer therapy. Additionally, Generally, CAR-NK cell therapy stands out as a promising alternative to CAR-T therapy, presenting a myriad of advantages, including safety, versatility, and efficiency. Current clinical trials, examining the safety and effectiveness of CAR-NK cell therapy in both hematological and solid malignances, underscore its potential to transform the landscape of cancer treatment (Table 1). Further research and clinical exploration are crucial to fully understand and harness the transformative power of CAR-NK cells in the realm of cancer immunotherapy.
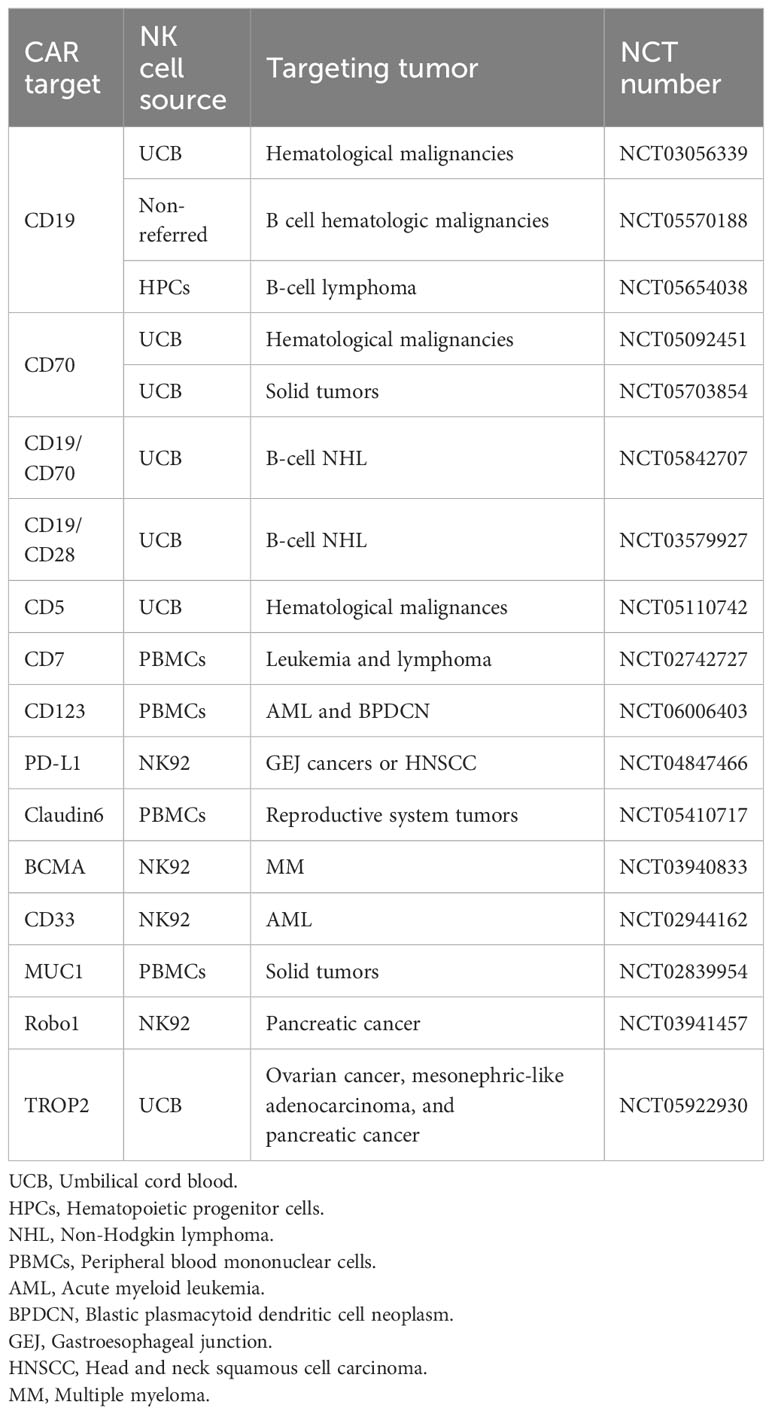
Table 1 The ongoing clinical trials of CAR-NK in cancer immunotherapy, which have progressed beyond phase 1 thus far, are documented.
There are some other cancer immunotherapy paradigms except CAR strategy, such as oncolytic viruses and immune checkpoint inhibitors (97, 98). CAR-NK cells, oncolytic viruses, and immune checkpoint inhibitors represent cutting-edge cancer therapies with distinct mechanisms and applications. CAR-NK cells, engineered to target specific cancer antigens, are notable for their safety and specificity, particularly in hematologic malignancies. Oncolytic viruses selectively infect and kill cancer cells, showing promise in solid tumors; however, their effectiveness varies (99). Immune checkpoint inhibitors, enhancing the immune system’s ability to fight cancer, have been successful across various cancer types but can cause significant immune-related side effects. While CAR-NK cells are in early research stages and involve complex manufacturing, oncolytic viruses require careful genetic engineering for effectiveness. Immune checkpoint inhibitors, widely applicable, face challenges in cost and accessibility. Each therapy offers unique benefits and limitations, shaping individual treatment plans based on cancer type, stage, and patient health. The evolving landscape of these therapies continues to advance cancer treatment options.
4 Perspective and conclusion
In conclusion, the intricate interplay between NK cells and the formidable challenges posed by the TME highlights the dualistic nature of these immune actors in the context of cancer. While obstacles such as limited infiltration into solid tumors and the immunosuppressive milieu within the TME impede the full realization of NK cell potential, their presence within tumors is associated with a relatively favorable prognosis. Understanding the complex activation processes involving key receptors and cytokines is pivotal for unraveling their role in tumor occurrence and development. Advancements, particularly in iPSC-derived NK cells, offer new dimensions to NK cell-based immunotherapy, potentially revolutionizing cancer treatment by enhancing tumor surveillance, elimination functions, and the recruitment of T cells to the TME.
Simultaneously, the emergence of CAR-NK cell therapy presents a dynamic and promising alternative in the realm of cancer immunotherapy. The unique advantages of CAR-NK cells, including allogeneic sourcing, reduced risk of GVHD, and innate anti-tumor capabilities, position them as a versatile tool in the fight against cancer. While preclinical successes demonstrate their efficacy against hematological and solid malignancies, addressing challenges such as low cell persistence and efficient trafficking to tumor sites is imperative for successful clinical integration. CAR-NK cell therapy, additionally, faces challenges including limited NK cell persistence and difficulties in targeting and infiltrating solid tumors due to the complex tumor microenvironment. These factors impact CAR-NK cells’ effectiveness, particularly in solid tumor treatments. The ongoing clinical trials targeting various antigens underscore the commitment to realizing the therapeutic potential of CAR-NK cells, marking a critical phase in reshaping the landscape of cancer treatment.
In navigating the future of cancer immunotherapy, the convergence of NK cells and CAR-NK cells offers a transformative outlook. The intricate mechanisms underlying NK cell activation and the adaptability of CAR-NK cells in recognizing diverse tumor antigens illuminate the depth of research required for their successful integration into mainstream oncology. As these cellular therapies progress from scientific promise to clinical reality, they hold the potential to redefine precision immunotherapy, providing renewed hope and improved outcomes for patients facing challenging malignancies. In this evolving landscape, NK cells and CAR-NK cells stand as promising agents, guiding us toward a future where cancer treatment is not just a battle but a personalized, targeted, and effective therapeutic strategy.
Author contributions
MY: Conceptualization, Funding acquisition, Supervision, Writing – review & editing. TL: Writing – original draft. MN: Writing – review & editing. WZ: Writing – review & editing. SQ: Writing – review & editing. JZ: Supervision, Writing – review & editing.
Funding
The author(s) declare financial support was received for the research, authorship, and/or publication of this article. This work was supported by Natural Science Foundation of Zhejiang Province (LQ24H160007), China Postdoctoral Science Foundation (No. 2022M722766 and 2023M743016), and Postdoctoral Fellowship Program of CPSF (No. GZB20230642).
Conflict of interest
The authors declare that the research was conducted in the absence of any commercial or financial relationships that could be construed as a potential conflict of interest.
The author(s) declared that they were an editorial board member of Frontiers, at the time of submission. This had no impact on the peer review process and the final decision.
Publisher’s note
All claims expressed in this article are solely those of the authors and do not necessarily represent those of their affiliated organizations, or those of the publisher, the editors and the reviewers. Any product that may be evaluated in this article, or claim that may be made by its manufacturer, is not guaranteed or endorsed by the publisher.
Abbreviations
NK, Natural Killer cell; ILC, innate lymphoid cell; HSC, hematopoietic stem cell; KIR, killer-cell immunoglobulin-like receptor; CNS, central nervous system; HLA, human leukocyte antigen; MHC, major histocompatibility complex; iPSC, Induced pluripotent stem cell; KIR, killer cell immunoglobulin-like receptor; NKG2D, NK gene 2D; NCR, natural cytotoxicity receptor; ADCC, antibody-dependent cell-mediated cytotoxicity; CTL, cytotoxic T lymphocyte; TME, the tumor microenvironment; HIF-1α, hypoxia-inducible factor 1 alpha; ECM, extracellular matrix; TGF-β, transforming growth factor-beta; GE2, prostaglandin E2; FasL, Fas ligand; TRAIL, TNF-related apoptosis-inducing ligand; DC, dendritic cell; CAR, Chimeric Antigen Receptor; GVHD, graft versus host disease; CRS, cytokine release syndrome.
References
1. Chiossone L, Dumas PY, Vienne M, Vivier E. Natural killer cells and other innate lymphoid cells in cancer. Nat Rev Immunol (2018) 18(11):671–88. doi: 10.1038/s41577-018-0061-z
2. Freud AG, Caligiuri MA. Human natural killer cell development. Immunol Rev (2006) 214:56–72. doi: 10.1111/j.1600-065X.2006.00451.x
3. Stokic-Trtica V, Diefenbach A, Klose CSN. NK cell development in times of innate lymphoid cell diversity. Front Immunol (2020) 11:813. doi: 10.3389/fimmu.2020.00813
4. Abel AM, Yang C, Thakar MS, Malarkannan S. Natural killer cells: development, maturation, and clinical utilization. Front Immunol (2018) 9:1869. doi: 10.3389/fimmu.2018.01869
5. Jacobs R, Hintzen G, Kemper A, Beul K, Kempf S, Behrens G, et al. CD56bright cells differ in their KIR repertoire and cytotoxic features from CD56dim NK cells. Eur J Immunol (2001) 31(10):3121–7. doi: 10.1002/1521-4141(2001010)31:10<3121::aid-immu3121>3.0.co;2-4
6. Shin E, Bak SH, Park T, Kim JW, Yoon SR, Jung H, et al. Understanding NK cell biology for harnessing NK cell therapies: targeting cancer and beyond. Front Immunol (2023) 14:1192907. doi: 10.3389/fimmu.2023.1192907
7. Romee R, Schneider SE, Leong JW, Chase JM, Keppel CR, Sullivan RP, et al. Cytokine activation induces human memory-like NK cells. Blood (2012) 120(24):4751–60. doi: 10.1182/blood-2012-04-419283
8. O’Leary JG, Goodarzi M, Drayton DL, von Andrian UH. T cell- and B cell-independent adaptive immunity mediated by natural killer cells. Nat Immunol (2006) 7(5):507–16. doi: 10.1038/ni1332
9. Sun JC, Beilke JN, Lanier LL. Adaptive immune features of natural killer cells. Nature (2009) 457(7229):557–61. doi: 10.1038/nature07665
10. Cooper MA, Elliott JM, Keyel PA, Yang L, Carrero JA, Yokoyama WM. Cytokine-induced memory-like natural killer cells. Proc Natl Acad Sci U.S.A. (2009) 106(6):1915–9. doi: 10.1073/pnas.0813192106
11. Ran GH, Lin YQ, Tian L, Zhang T, Yan DM, Yu JH, et al. Natural killer cell homing and trafficking in tissues and tumors: from biology to application. Signal Transduct Target Ther (2022) 7(1):205. doi: 10.1038/s41392-022-01058-z
12. Pesce S, Moretta L, Moretta A, Marcenaro E. Human NK cell subsets redistribution in pathological conditions: A role for CCR7 receptor. Front Immunol (2016) 7:414. doi: 10.3389/fimmu.2016.00414
13. Moroso V, Famili F, Papazian N, Cupedo T, van der Laan LJ, Kazemier G, et al. NK cells can generate from precursors in the adult human liver. Eur J Immunol (2011) 41(11):3340–50. doi: 10.1002/eji.201141760
14. Vacca P, Mingari MC, Moretta L. Natural killer cells in human pregnancy. J Reprod Immunol (2013) 97(1):14–9. doi: 10.1016/j.jri.2012.10.008
15. Hudspeth K, Donadon M, Cimino M, Pontarini E, Tentorio P, Preti M, et al. Human liver-resident CD56(bright)/CD16(neg) NK cells are retained within hepatic sinusoids via the engagement of CCR5 and CXCR6 pathways. J Autoimmun (2016) 66:40–50. doi: 10.1016/j.jaut.2015.08.011
16. Carlino C, Stabile H, Morrone S, Bulla R, Soriani A, Agostinis C, et al. Recruitment of circulating NK cells through decidual tissues: a possible mechanism controlling NK cell accumulation in the uterus during early pregnancy. Blood (2008) 111(6):3108–15. doi: 10.1182/blood-2007-08-105965
17. Cong J, Wei H. Natural killer cells in the lungs. Front Immunol (2019) 10:1416. doi: 10.3389/fimmu.2019.01416
18. Carlsten M, Järås M. Natural killer cells in myeloid Malignancies: immune surveillance, NK cell dysfunction, and pharmacological opportunities to bolster the endogenous NK cells. Front Immunol (2019) 10:2357. doi: 10.3389/fimmu.2019.02357
19. Russo E, Laffranchi M, Tomaipitinca L, Del Prete A, Santoni A, Sozzani S, et al. NK cell anti-tumor surveillance in a myeloid cell-shaped environment. Front Immunol (2021) 12:787116. doi: 10.3389/fimmu.2021.787116
20. Kim S, Poursine-Laurent J, Truscott SM, Lybarger L, Song YJ, Yang L, et al. Licensing of natural killer cells by host major histocompatibility complex class I molecules. Nature (2005) 436(7051):709–13. doi: 10.1038/nature03847
21. Lanier LL. Up on the tightrope: natural killer cell activation and inhibition. Nat Immunol (2008) 9(5):495–502. doi: 10.1038/ni1581
22. Yi M, Li T, Niu M, Mei Q, Zhao B, Chu Q, et al. Exploiting innate immunity for cancer immunotherapy. Mol Cancer (2023) 22(1):187. doi: 10.1186/s12943-023-01885-w
23. Lanier LL. NKG2D receptor and its ligands in host defense. Cancer Immunol Res (2015) 3(6):575–82. doi: 10.1158/2326-6066.Cir-15-0098
24. Sivori S, Vacca P, Del Zotto G, Munari E, Mingari MC, Moretta L. Human NK cells: surface receptors, inhibitory checkpoints, and translational applications. Cell Mol Immunol (2019) 16(5):430–41. doi: 10.1038/s41423-019-0206-4
25. Capuano C, Pighi C, Battella S, De Federicis D, Galandrini R, Palmieri G. Harnessing CD16-mediated NK cell functions to enhance therapeutic efficacy of tumor-targeting mAbs. Cancers (Basel) (2021) 13(10):2500. doi: 10.3390/cancers13102500
26. Chin DS, Lim CSY, Nordin F, Arifin N, Jun TG. Antibody-dependent cell-mediated cytotoxicity through natural killer (NK) cells: unlocking NK cells for future immunotherapy. Curr Pharm Biotechnol (2022) 23(4):552–78. doi: 10.2174/1389201022666210820093608
27. Muntasell A, Ochoa MC, Cordeiro L, Berraondo P, López-Díaz de Cerio A, Cabo M, et al. Targeting NK-cell checkpoints for cancer immunotherapy. Curr Opin Immunol (2017) 45:73–81. doi: 10.1016/j.coi.2017.01.003
28. Bald T, Krummel MF, Smyth MJ, Barry KC. The NK cell-cancer cycle: advances and new challenges in NK cell-based immunotherapies. Nat Immunol (2020) 21(8):835–47. doi: 10.1038/s41590-020-0728-z
29. Shimasaki N, Jain A, Campana D. NK cells for cancer immunotherapy. Nat Rev Drug Discovery (2020) 19(3):200–18. doi: 10.1038/s41573-019-0052-1
30. Russick J, Torset C, Hemery E, Cremer I. NK cells in the tumor microenvironment: Prognostic and theranostic impact. Recent advances and trends. Semin Immunol (2020) 48:101407. doi: 10.1016/j.smim.2020.101407
31. Cózar B, Greppi M, Carpentier S, Narni-Mancinelli E, Chiossone L, Vivier E. Tumor-infiltrating natural killer cells. Cancer Discovery (2021) 11(1):34–44. doi: 10.1158/2159-8290.Cd-20-0655
32. Ni J, Wang X, Stojanovic A, Zhang Q, Wincher M, Bühler L, et al. Single-cell RNA sequencing of tumor-infiltrating NK cells reveals that inhibition of transcription factor HIF-1α Unleashes NK cell activity. Immunity (2020) 52(6):1075–1087.e1078. doi: 10.1016/j.immuni.2020.05.001
33. Hosseini R, Sarvnaz H, Arabpour M, Ramshe SM, Asef-Kabiri L, Yousefi H, et al. Cancer exosomes and natural killer cells dysfunction: biological roles, clinical significance and implications for immunotherapy. Mol Cancer (2022) 21(1):15. doi: 10.1186/s12943-021-01492-7
34. Edsparr K, Basse PH, Goldfarb RH, Albertsson P. Matrix metalloproteinases in cytotoxic lymphocytes impact on tumour infiltration and immunomodulation. Cancer Microenviron (2011) 4(3):351–60. doi: 10.1007/s12307-010-0057-0
35. Putz EM, Mayfosh AJ, Kos K, Barkauskas DS, Nakamura K, Town L, et al. NK cell heparanase controls tumor invasion and immune surveillance. J Clin Invest (2017) 127(7):2777–88. doi: 10.1172/jci92958
36. Dong W, Wu X, Ma S, Wang Y, Nalin AP, Zhu Z, et al. The mechanism of anti-PD-L1 antibody efficacy against PD-L1-negative tumors identifies NK cells expressing PD-L1 as a cytolytic effector. Cancer Discovery (2019) 9(10):1422–37. doi: 10.1158/2159-8290.Cd-18-1259
37. Benson DM Jr., Bakan CE, Mishra A, Hofmeister CC, Efebera Y, Becknell B, et al. The PD-1/PD-L1 axis modulates the natural killer cell versus multiple myeloma effect: a therapeutic target for CT-011, a novel monoclonal anti-PD-1 antibody. Blood (2010) 116(13):2286–94. doi: 10.1182/blood-2010-02-271874
38. Melaiu O, Lucarini V, Cifaldi L, Fruci D. Influence of the tumor microenvironment on NK cell function in solid tumors. Front Immunol (2019) 10:3038. doi: 10.3389/fimmu.2019.03038
39. Yi M, Wu Y, Niu M, Zhu S, Zhang J, Yan Y, et al. Anti-TGF-β/PD-L1 bispecific antibody promotes T cell infiltration and exhibits enhanced antitumor activity in triple-negative breast cancer. J Immunother Cancer (2022) 10(12):e005543. doi: 10.1136/jitc-2022-005543
40. Yi M, Zhang J, Li A, Niu M, Yan Y, Jiao Y, et al. The construction, expression, and enhanced anti-tumor activity of YM101: a bispecific antibody simultaneously targeting TGF-β and PD-L1. J Hematol Oncol (2021) 14(1):27. doi: 10.1186/s13045-021-01045-x
41. Robson NC, Wei H, McAlpine T, Kirkpatrick N, Cebon J, Maraskovsky E. Activin-A attenuates several human natural killer cell functions. Blood (2009) 113(14):3218–25. doi: 10.1182/blood-2008-07-166926
42. Cekic C, Day YJ, Sag D, Linden J. Myeloid expression of adenosine A2A receptor suppresses T and NK cell responses in the solid tumor microenvironment. Cancer Res (2014) 74(24):7250–9. doi: 10.1158/0008-5472.Can-13-3583
43. Yu P, Wang Y, Yuan D, Sun Y, Qin S, Li T. Vascular normalization: reshaping the tumor microenvironment and augmenting antitumor immunity for ovarian cancer. Front Immunol (2023) 14:1276694. doi: 10.3389/fimmu.2023.1276694
44. Tong L, Jiménez-Cortegana C, Tay AHM, Wickström S, Galluzzi L, Lundqvist A. NK cells and solid tumors: therapeutic potential and persisting obstacles. Mol Cancer (2022) 21(1):206. doi: 10.1186/s12943-022-01672-z
45. Tumino N, Di Pace AL, Besi F, Quatrini L, Vacca P, Moretta L. Interaction between MDSC and NK cells in solid and hematological Malignancies: impact on HSCT. Front Immunol (2021) 12:638841. doi: 10.3389/fimmu.2021.638841
46. Law AMK, Valdes-Mora F, Gallego-Ortega D. Myeloid-derived suppressor cells as a therapeutic target for cancer. Cells (2020) 9(3):561. doi: 10.3390/cells9030561
47. Zimmer J, Andrès E, Hentges F. NK cells and Treg cells: a fascinating dance cheek to cheek. Eur J Immunol (2008) 38(11):2942–5. doi: 10.1002/eji.200838813
48. Smyth MJ, Crowe NY, Godfrey DI. NK cells and NKT cells collaborate in host protection from methylcholanthrene-induced fibrosarcoma. Int Immunol (2001) 13(4):459–63. doi: 10.1093/intimm/13.4.459
49. Smyth MJ, Thia KY, Street SE, Cretney E, Trapani JA, Taniguchi M, et al. Differential tumor surveillance by natural killer (NK) and NKT cells. J Exp Med (2000) 191(4):661–8. doi: 10.1084/jem.191.4.661
50. Lee H, Quek C, Silva I, Tasker A, Batten M, Rizos H, et al. Integrated molecular and immunophenotypic analysis of NK cells in anti-PD-1 treated metastatic melanoma patients. Oncoimmunology (2019) 8(2):e1537581. doi: 10.1080/2162402x.2018.1537581
51. Guerra N, Tan YX, Joncker NT, Choy A, Gallardo F, Xiong N, et al. NKG2D-deficient mice are defective in tumor surveillance in models of spontaneous Malignancy. Immunity (2008) 28(4):571–80. doi: 10.1016/j.immuni.2008.02.016
52. Takeda K, Hayakawa Y, Smyth MJ, Kayagaki N, Yamaguchi N, Kakuta S, et al. Involvement of tumor necrosis factor-related apoptosis-inducing ligand in surveillance of tumor metastasis by liver natural killer cells. Nat Med (2001) 7(1):94–100. doi: 10.1038/83416
53. Zheng X, Qian Y, Fu B, Jiao D, Jiang Y, Chen P, et al. Mitochondrial fragmentation limits NK cell-based tumor immunosurveillance. Nat Immunol (2019) 20(12):1656–67. doi: 10.1038/s41590-019-0511-1
54. Huntington ND, Cursons J, Rautela J. The cancer-natural killer cell immunity cycle. Nat Rev Cancer (2020) 20(8):437–54. doi: 10.1038/s41568-020-0272-z
55. Khan M, Arooj S, Wang H. NK cell-based immune checkpoint inhibition. Front Immunol (2020) 11:167. doi: 10.3389/fimmu.2020.00167
56. Hansen ML, Woetmann A, Krejsgaard T, Kopp KL, Søkilde R, Litman T, et al. IFN-α primes T- and NK-cells for IL-15-mediated signaling and cytotoxicity. Mol Immunol (2011) 48(15-16):2087–93. doi: 10.1016/j.molimm.2011.07.008
57. Böttcher JP, Bonavita E, Chakravarty P, Blees H, Cabeza-Cabrerizo M, Sammicheli S, et al. NK Cells Stimulate Recruitment of cDC1 into the Tumor Microenvironment Promoting Cancer Immune Control. Cell (2018) 172(5):1022–1037.e1014. doi: 10.1016/j.cell.2018.01.004
58. Myers JA, Miller JS. Exploring the NK cell platform for cancer immunotherapy. Nat Rev Clin Oncol (2021) 18(2):85–100. doi: 10.1038/s41571-020-0426-7
59. Sivori S, Pende D, Quatrini L, Pietra G, Della Chiesa M, Vacca P, et al. NK cells and ILCs in tumor immunotherapy. Mol Aspects Med (2021) 80:100870. doi: 10.1016/j.mam.2020.100870
60. Hodgins JJ, Khan ST, Park MM, Auer RC, Ardolino M. Killers 2.0: NK cell therapies at the forefront of cancer control. J Clin Invest (2019) 129(9):3499–510. doi: 10.1172/jci129338
61. Li Y, Hermanson DL, Moriarity BS, Kaufman DS. Human iPSC-derived natural killer cells engineered with chimeric antigen receptors enhance anti-tumor activity. Cell Stem Cell (2018) 23(2):181–192.e185. doi: 10.1016/j.stem.2018.06.002
62. Lin X, Sun Y, Dong X, Liu Z, Sugimura R, Xie G. IPSC-derived CAR-NK cells for cancer immunotherapy. BioMed Pharmacother (2023) 165:115123. doi: 10.1016/j.biopha.2023.115123
63. Woan KV, Kim H, Bjordahl R, Davis ZB, Gaidarova S, Goulding J, et al. Harnessing features of adaptive NK cells to generate iPSC-derived NK cells for enhanced immunotherapy. Cell Stem Cell (2021) 28(12):2062–2075.e2065. doi: 10.1016/j.stem.2021.08.013
64. Cichocki F, Bjordahl R, Gaidarova S, Mahmood S, Abujarour R, Wang H, et al. iPSC-derived NK cells maintain high cytotoxicity and enhance in vivo tumor control in concert with T cells and anti-PD-1 therapy. Sci Transl Med (2020) 12(568):eaaz5618. doi: 10.1126/scitranslmed.aaz5618
65. Goldenson BH, Hor P, Kaufman DS. iPSC-derived natural killer cell therapies - expansion and targeting. Front Immunol (2022) 13:841107. doi: 10.3389/fimmu.2022.841107
66. Guillerey C, Huntington ND, Smyth MJ. Targeting natural killer cells in cancer immunotherapy. Nat Immunol (2016) 17(9):1025–36. doi: 10.1038/ni.3518
67. Xie G, Dong H, Liang Y, Ham JD, Rizwan R, Chen J. CAR-NK cells: A promising cellular immunotherapy for cancer. EBioMedicine (2020) 59:102975. doi: 10.1016/j.ebiom.2020.102975
68. Sterner RC, Sterner RM. CAR-T cell therapy: current limitations and potential strategies. Blood Cancer J (2021) 11(4):69. doi: 10.1038/s41408-021-00459-7
69. Zhang X, Zhu L, Zhang H, Chen S, Xiao Y. CAR-T cell therapy in hematological Malignancies: current opportunities and challenges. Front Immunol (2022) 13:927153. doi: 10.3389/fimmu.2022.927153
70. Jogalekar MP, Rajendran RL, Khan F, Dmello C, Gangadaran P, Ahn BC. CAR T-Cell-Based gene therapy for cancers: new perspectives, challenges, and clinical developments. Front Immunol (2022) 13:925985. doi: 10.3389/fimmu.2022.925985
71. Maalej KM, Merhi M, Inchakalody VP, Mestiri S, Alam M, Maccalli C, et al. CAR-cell therapy in the era of solid tumor treatment: current challenges and emerging therapeutic advances. Mol Cancer (2023) 22(1):20. doi: 10.1186/s12943-023-01723-z
72. Zhang L, Meng Y, Feng X, Han Z. CAR-NK cells for cancer immunotherapy: from bench to bedside. biomark Res (2022) 10(1):12. doi: 10.1186/s40364-022-00364-6
73. Heipertz EL, Zynda ER, Stav-Noraas TE, Hungler AD, Boucher SE, Kaur N, et al. Current perspectives on “Off-the-shelf” Allogeneic NK and CAR-NK cell therapies. Front Immunol (2021) 12:732135. doi: 10.3389/fimmu.2021.732135
74. Caruso S, De Angelis B, Del Bufalo F, Ciccone R, Donsante S, Volpe G, et al. Safe and effective off-the-shelf immunotherapy based on CAR.CD123-NK cells for the treatment of acute myeloid leukaemia. J Hematol Oncol (2022) 15(1):163. doi: 10.1186/s13045-022-01376-3
75. Liu E, Tong Y, Dotti G, Shaim H, Savoldo B, Mukherjee M, et al. Cord blood NK cells engineered to express IL-15 and a CD19-targeted CAR show long-term persistence and potent antitumor activity. Leukemia (2018) 32(2):520–31. doi: 10.1038/leu.2017.226
76. Wang J, Lupo KB, Chambers AM, Matosevic S. Purinergic targeting enhances immunotherapy of CD73(+) solid tumors with piggyBac-engineered chimeric antigen receptor natural killer cells. J Immunother Cancer (2018) 6(1):136. doi: 10.1186/s40425-018-0441-8
77. Xu Y, Liu Q, Zhong M, Wang Z, Chen Z, Zhang Y, et al. 2B4 costimulatory domain enhancing cytotoxic ability of anti-CD5 chimeric antigen receptor engineered natural killer cells against T cell Malignancies. J Hematol Oncol (2019) 12(1):49. doi: 10.1186/s13045-019-0732-7
78. Li Y, Basar R, Wang G, Liu E, Moyes JS, Li L, et al. KIR-based inhibitory CARs overcome CAR-NK cell trogocytosis-mediated fratricide and tumor escape. Nat Med (2022) 28(10):2133–44. doi: 10.1038/s41591-022-02003-x
79. Gang M, Marin ND, Wong P, Neal CC, Marsala L, Foster M, et al. CAR-modified memory-like NK cells exhibit potent responses to NK-resistant lymphomas. Blood (2020) 136(20):2308–18. doi: 10.1182/blood.2020006619
80. Albinger N, Pfeifer R, Nitsche M, Mertlitz S, Campe J, Stein K, et al. Primary CD33-targeting CAR-NK cells for the treatment of acute myeloid leukemia. Blood Cancer J (2022) 12(4):61. doi: 10.1038/s41408-022-00660-2
81. Dong H, Ham JD, Hu G, Xie G, Vergara J, Liang Y, et al. Memory-like NK cells armed with a neoepitope-specific CAR exhibit potent activity against NPM1 mutated acute myeloid leukemia. Proc Natl Acad Sci U.S.A. (2022) 119(25):e2122379119. doi: 10.1073/pnas.2122379119
82. Ma R, Lu T, Li Z, Teng KY, Mansour AG, Yu M, et al. An oncolytic virus expressing IL15/IL15Rα Combined with off-the-shelf EGFR-CAR NK cells targets glioblastoma. Cancer Res (2021) 81(13):3635–48. doi: 10.1158/0008-5472.Can-21-0035
83. Heim C, Moser LM, Kreyenberg H, Bonig HB, Tonn T, Wels WS, et al. ErbB2 (HER2)-CAR-NK-92 cells for enhanced immunotherapy of metastatic fusion-driven alveolar rhabdomyosarcoma. Front Immunol (2023) 14:1228894. doi: 10.3389/fimmu.2023.1228894
84. Zhang Q, Zhang H, Ding J, Liu H, Li H, Li H, et al. Combination therapy with epCAM-CAR-NK-92 cells and regorafenib against human colorectal cancer models. J Immunol Res (2018) 2018:4263520. doi: 10.1155/2018/4263520
85. Schnalzger TE, de Groot MH, Zhang C, Mosa MH, Michels BE, Röder J, et al. 3D model for CAR-mediated cytotoxicity using patient-derived colorectal cancer organoids. EMBO J (2019) 38(12):e100928. doi: 10.15252/embj.2018100928
86. Liu S, Galat V, Galat Y, Lee YKA, Wainwright D, Wu J. NK cell-based cancer immunotherapy: from basic biology to clinical development. J Hematol Oncol (2021) 14(1):7. doi: 10.1186/s13045-020-01014-w
87. Parihar R, Rivas C, Huynh M, Omer B, Lapteva N, Metelitsa LS, et al. NK cells expressing a chimeric activating receptor eliminate MDSCs and rescue impaired CAR-T cell activity against solid tumors. Cancer Immunol Res (2019) 7(3):363–75. doi: 10.1158/2326-6066.Cir-18-0572
88. Zhang P, Zhao S, Wu C, Li J, Li Z, Wen C, et al. Effects of CSF1R-targeted chimeric antigen receptor-modified NK92MI & T cells on tumor-associated macrophages. Immunotherapy (2018) 10(11):935–49. doi: 10.2217/imt-2018-0012
89. Hermanson DL, Kaufman DS. Utilizing chimeric antigen receptors to direct natural killer cell activity. Front Immunol (2015) 6:195. doi: 10.3389/fimmu.2015.00195
90. Pan K, Farrukh H, Chittepu V, Xu H, Pan CX, Zhu Z. CAR race to cancer immunotherapy: from CAR T, CAR NK to CAR macrophage therapy. J Exp Clin Cancer Res (2022) 41(1):119. doi: 10.1186/s13046-022-02327-z
91. Xiao L, Cen D, Gan H, Sun Y, Huang N, Xiong H, et al. Adoptive transfer of NKG2D CAR mRNA-engineered natural killer cells in colorectal cancer patients. Mol Ther (2019) 27(6):1114–25. doi: 10.1016/j.ymthe.2019.03.011
92. Morgan MA, Büning H, Sauer M, Schambach A. Use of cell and genome modification technologies to generate improved “Off-the-shelf” CAR T and CAR NK cells. Front Immunol (2020) 11:1965. doi: 10.3389/fimmu.2020.01965
93. Liu E, Marin D, Banerjee P, Macapinlac HA, Thompson P, Basar R, et al. Use of CAR-transduced natural killer cells in CD19-positive lymphoid tumors. N Engl J Med (2020) 382(6):545–53. doi: 10.1056/NEJMoa1910607
94. Marofi F, Rahman HS, Thangavelu L, Dorofeev A, Bayas-Morejón F, Shirafkan N, et al. Renaissance of armored immune effector cells, CAR-NK cells, brings the higher hope for successful cancer therapy. Stem Cell Res Ther (2021) 12(1):200. doi: 10.1186/s13287-021-02251-7
95. Zhang Q, Bi J, Zheng X, Chen Y, Wang H, Wu W, et al. Blockade of the checkpoint receptor TIGIT prevents NK cell exhaustion and elicits potent anti-tumor immunity. Nat Immunol (2018) 19(7):723–32. doi: 10.1038/s41590-018-0132-0
96. Lee MY, Robbins Y, Sievers C, Friedman J, Abdul Sater H, Clavijo PE, et al. Chimeric antigen receptor engineered NK cellular immunotherapy overcomes the selection of T-cell escape variant cancer cells. J Immunother Cancer (2021) 9(3):e002128. doi: 10.1136/jitc-2020-002128
97. Mondal M, Guo J, He P, Zhou D. Recent advances of oncolytic virus in cancer therapy. Hum Vaccin Immunother (2020) 16(10):2389–402. doi: 10.1080/21645515.2020.1723363
98. Bagchi S, Yuan R, Engleman EG. Immune checkpoint inhibitors for the treatment of cancer: clinical impact and mechanisms of response and resistance. Annu Rev Pathol (2021) 16:223–49. doi: 10.1146/annurev-pathol-042020-042741
Keywords: natural killer cell, CAR-NK, cancer immunotherapy, the tumor microenvironment, adoptive cell transfer
Citation: Li T, Niu M, Zhang W, Qin S, Zhou J and Yi M (2024) CAR-NK cells for cancer immunotherapy: recent advances and future directions. Front. Immunol. 15:1361194. doi: 10.3389/fimmu.2024.1361194
Received: 25 December 2023; Accepted: 29 January 2024;
Published: 09 February 2024.
Edited by:
Yingfeng Wan, University of Michigan, United StatesReviewed by:
Dmitry Aleksandrovich Zinovkin, Gomel State Medical University, BelarusQingling Hua, First Affiliated Hospital of Anhui Medical University, China
Guo Yaping, Zhengzhou University, China
Copyright © 2024 Li, Niu, Zhang, Qin, Zhou and Yi. This is an open-access article distributed under the terms of the Creative Commons Attribution License (CC BY). The use, distribution or reproduction in other forums is permitted, provided the original author(s) and the copyright owner(s) are credited and that the original publication in this journal is cited, in accordance with accepted academic practice. No use, distribution or reproduction is permitted which does not comply with these terms.
*Correspondence: Ming Yi, mingyi_onco@outlook.com; 1322068@zju.edu.cn; Jianwei Zhou, jianwei-zhou@zju.edu.cn