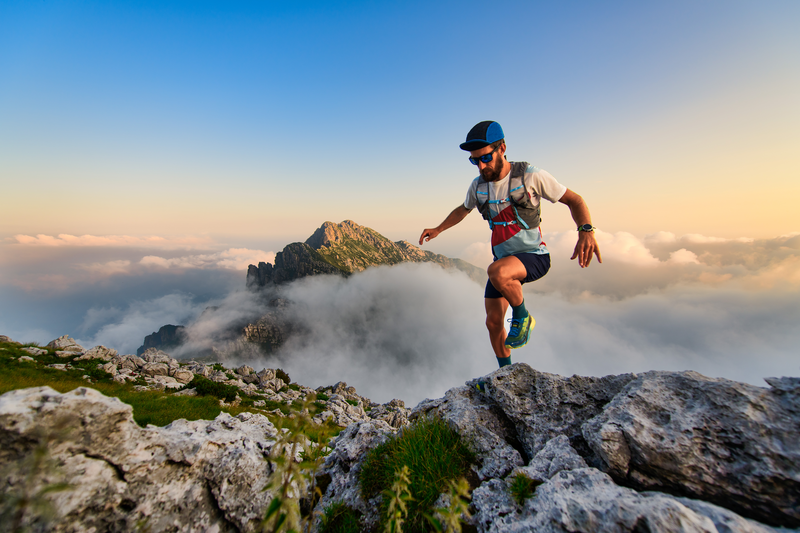
94% of researchers rate our articles as excellent or good
Learn more about the work of our research integrity team to safeguard the quality of each article we publish.
Find out more
REVIEW article
Front. Immunol. , 28 March 2024
Sec. Cancer Immunity and Immunotherapy
Volume 15 - 2024 | https://doi.org/10.3389/fimmu.2024.1359532
This article is part of the Research Topic Beyond PD-1: novel checkpoint receptors and ligands as targets for immunotherapy View all 18 articles
Immunotherapy has revolutionized cancer treatment, with the anti-PD-1/PD-L1 axis therapy demonstrating significant clinical efficacy across various tumor types. However, it should be noted that this therapy is not universally effective for all PD-L1-positive patients, highlighting the need to expedite research on the second ligand of PD-1, known as Programmed Cell Death Receptor Ligand 2 (PD-L2). As an immune checkpoint molecule, PD-L2 was reported to be associated with patient’s prognosis and plays a pivotal role in cancer cell immune escape. An in-depth understanding of the regulatory process of PD-L2 expression may stratify patients to benefit from anti-PD-1 immunotherapy. Our review focuses on exploring PD-L2 expression in different tumors, its correlation with prognosis, regulatory factors, and the interplay between PD-L2 and tumor treatment, which may provide a notable avenue in developing immune combination therapy and improving the clinical efficacy of anti-PD-1 therapies.
The threat of cancer to human health continues, highlighting the vital importance of early detection, diagnosis and treatment in tumor management. However, the challenge in early diagnosis and treatment arises from the inconspicuous symptoms and lack of specificity in early-stage tumors. The current surgery, chemoradiotherapy, targeted therapy, and combined treatment in a variety of ways have greatly improved the therapeutic effect of tumors, however, due to the severe side effects and drug resistance, their efficacy remains unsatisfactory (1, 2). Hence, there is an imperative to innovate and develop novel treatments that can overcome these limitations. The advent of immunotherapy has revolutionized cancer treatment, owing much of its success to the breakthroughs in immune checkpoint blockade.
Programmed cell death-1(PD-1) is a crucial immunosuppressive molecule with two known ligands, programmed cell death-ligand 1(PD-L1) and PD-L2. The interaction between PD-1 and its ligands results in two opposing effects. One effect is the inhibition of T-cell immunity and the reduction of unnecessary immune responses, contributing to the prevention of autoimmune diseases. Another is the inhibition of the immune system’s ability to monitor and clear tumor cells, thereby allowing the occurrence and development of tumors (3). At present, many studies have been carried out on the PD-1/PD-L1 axis. Certain drugs, including Nivolumab and Pembrolizumab, are employed in the treatment of malignant tumors. However, it has been observed during treatment that not all PD-L1-positive patients exhibit a discernible response to anti-PD-1/PD-L1 axis treatment. Conversely, some PD-L1-negative patients show a positive response to the anti-PD-1/PD-L1 axis treatment, indicating that there may be other molecules or receptors that interact with PD-1 (4).
As the second known ligand of PD-1, whether PD-L2 can be used as another target for tumor therapy has attracted the attention of many researchers. As research progresses, potential interactions and relationships between PD-L1 and PD-L2 emerge. Simultaneous or separate expression of these two ligands can yield varied outcomes in terms of tumor development and prognosis. In a study involving 172 patients with head and neck tumors treated with pembrolizumab, the overall response rate (ORR) was two times higher in both PD-L1 and PD-L2 positive patients than in patients positive for PD-L1 alone. When PD-L2 expression was confined to tumor cells, the ORR was 26.5% in PD-L2 positive patients compared to 16.7% in PD-L2 negative patients (4). These findings indicate a potential relationship between PD-L1 and PD-L2, highlighting the need for investigating anti-PD-1/PD-L2 axis therapy. This may enhance the therapeutic efficacy of tumors when combined with anti-PD-1/PD-L1 axis therapy.
The binding affinity of PD-1 with PD-L1 and PD-1 with PD-L2 is quite different. While both ligands exhibit binding affinities to PD-1, structural analyses reveal significant differences in their interaction mechanisms. The binding of PD-1 and PD-L1 induces complex molecular configuration changes(Figure 1A), whereas the interaction between PD-1 and PD-L2 is simpler (5). Surface plasmon resonance analyses highlight that PD-L2 demonstrates 2 to 6 times higher affinity for PD-1 compared to PD-L1 (6), and in some cases, the affinity is reported to be 30 times higher (7). PD-L1 and PD-L2 not only bind to PD-1, but also have their own second binding sites. PD-L1 binds to CD80 (8) while PD-L2 binds to RGMb (9). CD80 functions as a co-stimulatory factor during T lymphocyte activation when CD86 activates, playing a pivotal role in autoimmune surveillance, humoral immune response, and transplantation reactions. The interaction between CD80 and PD-L1 results in heterodimerization, thereby impeding the interaction between PD-L1 and PD-1 (Figure 1B). However, the interaction of PD-L2 with PD-1 is not negatively regulated by the level of CD80 (10). Therefore, this also explains to some extent that anti-PD-L1 therapy is not effective for all PD-L1 positive patients, which further highlights the necessity and importance of studying PD-L2 and anti-PD-L2 drugs. This heightened affinity has implications for the development of small-molecule compound drugs and the PD-1/PD-L2 axis.
Figure 1 A shows the process of combining PD-L1 and PD-L2 with PD-1 respectively. B.(A) Besides PD-1, another binding site of PD-L1 and PD-L2 is PD-L2-RGMB and PD-L1-CD80. (B) CD80 can heterodimerize with PD-L1, which prevents the interaction between PD-L1 and PD-1.
This paper reviews the current status of PD-L2 research in tumors, encompassing the expression of PD-L2 in various common tumors, the association between PD-L2 expression and prognosis, regulatory factors, and the interplay between PD-L2 and tumor treatment.
Studies have identified the expression of PD-L2 in various common tumors, including but not limited to lung cancer, colorectal cancer, gastric cancer, esophageal cancer, head and neck cancer, breast cancer, cervical cancer, and liver cancer. High expression of PD-L2 tends to be associated with poor prognosis (Table 1).
In tumor cells, the PD-L2 can be regulated by multiple signaling pathways, playing an important role in the occurrence and development of tumors. Therefore, it is imperative to devote sufficient attention to these signaling pathways to enhance our comprehension of the role of PD-L2 in tumorigenesis.
The JAK/STAT pathway, formally recognized as the Janus kinase/signal transducer and transcriptional activator signaling pathway, stands as a pivotal communication hub in cellular function. An increasing body of evidence suggests that aberrations in the JAK/STAT pathway are linked to various cancers and autoimmune diseases (35). Studies have reported that the JAK/STAT pathway can trigger the expression of PD-L2 in tumor-related macrophages in lung adenocarcinoma. Additionally, soluble factors derived from cancer cells can induce the overexpression of PD-L2 in macrophages (36), hereby promoting tumor initiation and progression. Overexpression of PD-L1/PD-L2 in some malignant lymphomas is further linked to the activation or abnormalities of the JAK/STAT pathway, often accompanied by alterations in chromosome 9p24.1 (37, 38). These findings substantiate the role of the JAK/STAT pathway in regulating PD-L2 expression.
WNT (Wingless/Integrated) signaling serves as a crucial molecular regulator guiding a spectrum of physiological processes, encompassing embryonic development, adult stems cell homeostasis, and tissue regeneration (39, 40). Aberrations in the WNT signaling pathway are associated with the development and dysfunction of immune cells, as well as the promotion of immune escape (41). In tumor cells exhibiting high PD-L2 expression, a concomitant reduction in immune infiltration was often observed. In genetic identification, overexpression of genes within the WNT signaling pathway significantly correlated with the lack of immune infiltration (32). In melanoma, PD-L2 expression is also significantly increased in tumor cells with high phosphorylated β-catenin(pS1-βcat protein) expression downstream of the WNT/β-catenin signaling pathway (42), and the strong correlation between the high expression of this downstream protein and PD-L2 may offer insights for future tumor treatments targeting the signaling pathway.
The transcription factor Nuclear factor-κB(NF-κB) plays a pivotal role in inflammation, oncogenesis, and tumor progression, being aberrantly activated in the majority of cancers, thereby contributing to tumorigenesis and progression (43). In a mouse liver cancer model, prolonged indomethacin use was observed to upregulate the expression of PD-L1 and PD-L2 through the TRIF/NF-κB and the JAK/STAT1 pathway. This effect ultimately led to a poor prognosis for hepatocellular carcinoma (HCC) by suppressing Tumor necrosis factor-α(TNF-α) and Interferon gamma-γ(IFN-γ) in liver cancer cells (44). Similarly, an investigation into the pathogenesis of systemic lupus erythematosus identified two crucial pathways, Toll-like receptor and type I interferon, which play a significant role in the development of Systemic lupus erythematosus. These pathways regulate the expression of PD-1 and its ligands (PD-L 2, PD-L1) through the activation of NF-κB, thereby promoting the occurrence and development of systemic lupus erythematosus (45). Furthermore, genetic analysis of clear cell renal carcinoma also revealed an association between the expression of PD-L2 and mRNA levels of NF-κB p65 (46).
Protein kinase B/mammalian target of rapamycin (PI3K/Akt/mTOR) signaling pathway is a fundamental regulator of key physiological processes, including cell growth, migration, survival, and metabolism. Its dysregulation, a prevalent anomaly in tumor patients, significantly contributes to the initiation and progression of tumors (47). In a study focused on gene mutations in meningiomas, heightened PD-L2 expression was observed in patients with mutations in the AKT/mTOR signaling pathway when compared to other immune checkpoint proteins (48). In addition, Irina et al. underscored the pivotal role of PD-L2 in metastatic tumors, emphasizing its significance in tumor progression. They found that the level of the VHL protein, a tumor suppressor gene, determined the invasion capacity of tumors. By strategically targeting VHL through pathological means, they induced changes in the transcriptional and AKT/mTOR regulatory pathways, thereby influencing the expression of PD-L2. This intricate interplay ultimately fuels the initiation and progression of tumors (46, 49).
Known as Toll-like receptor 9, is a key receptor for DNA recognition in cells, activating both innate and acquired immune responses and playing a crucial role in the immune system (50). Ongoing reports and clinical studies suggest promising potential for TLR9 agonists in cancer treatment (51). Baruah et al. reported that high expression of PD-L1/PD-L2 in fibroblasts from HPV-positive head and neck tumor patients is mediated by TLR9, which reduces the expression of PD-L1/PD-L2 when using the TLR9 specific antagonist, ODNTTAGGG (52). This finding opens a new path for targeted TLR9 to regulate immune checkpoint PD-1 and its ligands, which may be of important significance for the treatment of tumors. Moreover, this observation hints at a potential role for HPV in the intricate interplay of TLR9 and immune checkpoint modulation.
At the level of genome regulation, the amplification, deletion, or mutation of PD-L2-related genes will change the expression of PD-L2 at the source. The amplification of 9p24.1 will increase the transcription of the PD-L2 gene through Janus kinase 2(JAK2)/signal transduction and transcription activator 1(STAT1) signaling pathway (53). PD-L1-L2-SE, a super-enhancer located between the genes of CD274 and CD273, can induce and activate the transcription of PD-L2 (54). Methylation of the PD-L2 gene reduces the production of PD-L2 protein by inhibiting transcription. Histone acetylation will change the contact between histone and DNA and increase the transcription of the PD-L2 gene. Myc and HOXC10 are transcription-stimulating factors, and combining with the promoter region of PD-L2 gene will significantly increase the transcription level of PD-L2. Ganetespib, located upstream of Myc, suppresses the activity of heat shock protein 90 (HSP90), resulting in an alteration of PD-L2 expression (55). E26 transformation-specific variant transcription factor (ETV4) belongs to the ETS transcription factor family, which enhances PD-L2 transcription by binding to the PD-L1-L2-SE region. When octamer binding protein 2 (OCT2) binds to PD-L2 intron, OCT2 can increase the localization of PD-L2 on the B cell membrane. These regulatory sites may become potential targets for tumor immunotherapy (56).
The majority of RNA transcribed from human genes cannot encode proteins, constituting what is known as non-coding RNAs. Despite their inability to code for proteins, these non-coding RNAs, including microRNA(miRNA), Long non-coding RNA(LncRNA), intron RNA, and repetitive RNA, exert significant influence on normal gene expression and contribute to various aspects of disease development. This study delves into the intricate impact of non-coding RNAs on the PD-L2, providing insights that highlight their potential as therapeutic targets for anti-tumor drugs (57). within this context, particular emphasis is placed on summarizing and elucidating the nuanced effects of miRNA and lncRNA on PD-L2 (Figure 2).
MicroRNA, a subtype of non-coding RNA with a compact length ranging from 19 to 25 nucleotides, exerts its functional impact through post-transcriptional silencing of target genes. Functionally, miRNA plays a pivotal role in post-transcriptional silencing of target genes (58). This discussion succinctly summarizes the current understanding of miRNAs, focusing specifically on their roles in regulating the PD-L2, as detailed in Table 2.
MiR-194-5p: Plays a crucial role in the onset and advancement of various malignant tumors, encompassing HCC, renal cell carcinoma, melanoma, and pancreatic carcinoma (66–68). Mechanistically, miR-194-5p predominantly targets the RNA transcripts of PD-L1/PD-L2, hindering the translation of PD-L2 mRNA and consequently diminishing the protein content of PD-L2. Experimental evidence presented by Fan et al. empha sizes that hsa-mir-194-5p has the ability to interact simultaneously with the RNA transcripts of both PD-L1 and PD-L2. Through the use of miR-194-5p mi mics and inhibitors, it was observed that inhibiting miR-194-5p led to an increase in the protein levels of PD-L1/PD-L2, while miR-194-5p mimics resulted in a decrease in the protein levels of PD-L1/PD-L2. These findings distinctly indicate that miR-194-5p exerts targeted action on PD-Ls, inhibiting the translation of PD-1 ligand proteins. Consequently, in cases of abnormal function or absence of miR-194-5p, an overproduction of PD-L2 protein occurs. The interplay between PD-1 and PD-L2 induces immunosuppression in hepatocellular carcinoma, thereby fostering the initiation and progression of the tumor (61).
MiR-100-5p and miR-138-5p: Studies have shown that miR-100-5p and miR-138-5p are related to mitogen-activated protein kinase (MAPK) signaling pathway, apoptosis, and tumor necrosis factor (TNF) pathway, all of which are the regulators of cancer development, progression, and immune escape (62). In a study conducted by EI Ahanidi et al., the investigation into the relationship between miR-100-5p, miR-138-5p, and the TERT/PDL1/PD-L2 axis demonstrated that miR-138-5p exhibited elevated expression in normal bladder tissue, displaying a consistent downward trend from low-grade to high-grade tumors and from early-stage to progressive tumors. Notably, both miRNAs showed a negative correlation with tumor staging in bladder cell lines, and this negative correlation extended to the expression of PD-L1/PD-L2 protein (62). These results indicated that the combination of miR-100-5p and miR-138-5p to target genes inhibited their mRNA translation, resulting in the decrease of the expression level of PD-L1/PD-L2 protein, and finally induced immunosuppression of the tumor, further promoting the malignant progression and poor prognosis of the tumor.
Long non-coding RNA, defined by a length exceeding 200 nucleotides, plays a pivotal role in diverse biological processes. As a central focus in genetic research, LncRNA has been increasingly recognized as a critical regulatory factor for protein-encoding genes in various diseases, particularly cancer, where it significantly contributes to both the initiation and progression of tumor (69). Recent studies delving into the intricate network between lncRNA, and microRNA have revealed their regulatory role in immune checkpoint gene expression in breast cancer. This suggests that LncRNA may influence tumor progression by modulating immune checkpoint pathways (70).
PCED1B-AS1: Has been implicated in the pathogenesis of various human cancers, including gastric cancer, colorectal cancer, pancreatic ductal cancer, and renal clear cell carcinoma (71–74). Recent investigations highlight the role of lncRNA in regulating multiple target genes by acting as a miRNA sponge, effectively inhibiting miRNA function. Given the crucial role of PD-L1/PD-L2 in mediating tumor immunosuppression, Fan et al. reported that the long-chain non-coding RNA PCED1B-AS1 may function as a cytoplasmic sponge, modulating the expression of PD-Ls by sequestering miR-194-5p. Knocking down PCED1B-AS1 using lentivirus shRNA in a liver cancer cell line resulted in a simultaneous reduction in the protein levels of PD-L1 and PD-L2. Conversely, lentivirus overexpression of PCED1B-AS1 increased the protein levels of PD-L1 and PD-L2. These findings suggest that PCED1B-AS1 exerts control over the expression of PD-L1/PD-L2 in hepatoma cells by inhibiting miRNA function. Aberrations in PCED1B-AS1 may induce immunosuppression in hepatocellular carcinoma, ultimately contributing to tumor deterioration and progression (61).
AC099850.3: lncRNA-AC099850.3 emerges as a novel player with abnormal expression across various tumor types, including lung adenocarcinoma and hepatocellular carcinoma, showing associations with tumor staging, poor prognosis, and immune infiltration (75, 76). In HCC, the knockdown of AC099850.3 significantly impairs the in vitro proliferation and invasion capabilities of HCC and inhibits tumor cell growth in vivo. Notably, AC099850.3 exhibits a marked positive correlation with PD-L1 and PD-L2, key immune checkpoints in vivo. High expression of PD-L1/PD-L2 is generally linked to poor tumor prognosis, indicating that AC099850.3 functions as an immune-related gene, regulating the expression of critical immune checkpoints in HCC. This, in turn, induces immunosuppression in HCC. Furthermore, studies have revealed that AC099850.3 promotes the malignant progression of hepatocellular carcinoma through the AKT signaling pathway. Spirina et al. reported that the AKT signaling pathway exerts a regulatory effect on PD-L2 (49).
TLC6: T-cell leukemia/lymphoma 6, a novel regulator implicated in HCC and renal clear cell carcinoma progression, associated with a poor prognosis (77, 78). In breast cancer, low TCL6 expression independently predicts an adverse outcome for PR (estrogen and progesterone receptor)-negative patients, while also correlating with immune infiltrating cells, including B cells, CD4+ T cells, and CD8+ T cells. Furthermore, TCL6 shows a significant positive correlation with PD1, PD-L1, and PD-L2 (63). However, the pathway or mechanism by which TCL6 acts on the expression of PD-L1/PD-L2 still needs further study (20). TCL6 presents a promising avenue for exploration in cancer therapeutics.
A large number of studies have shown that microorganisms in the intestinal tract and other parts may promote the occurrence and development of cancer, and affect cancer immune surveillance and response to immunotherapy (79). Studies have reported that intestinal microflora can down-regulate the expression of PD-L2 in CD11c+DCs (dendritic cells) in the intestine and tumor drainage lymph nodes and can promote anti-tumor immunity by combining with partner repulsion guiding molecule B (RGMB). Park et al. discovered an intestinal flora-C. cateniformis, which can induce the above-mentioned effects. The combination of PD-L2 and RGMB plays a role in inhibiting anti-cancer T cells. Blocking the interaction between PD-L2 and RGMB with antibodies can further relieve the inhibition of T cells, which is beneficial for T cells to clear cancer cells. This indicates that PD-L2 not only inhibits T cells through PD-1 but also exerts inhibitory effects through RGMB (64, 65). This also confirmed a new strategy of cancer immunotherapy-blocking the interaction between PD-L2 and RGMB to enhance the response to cancer immunotherapy.
Because the affinity of PD-1 to PD-L1 and PD-1 to PD-L2 is quite different. These small molecules hold the potential to penetrate the tumor microenvironment more effectively than traditional anti-PD-1/PD-L1 axis drugs, such as pabolizumab, potentially enhancing therapeutic efficacy (80). One notable molecular targeted drug in this context is JQ1, a BET-bromine domain inhibitor. JQ1 inhibits the binding of the BET-bromine domain and histone, suppressing the transcription of target genes. In studies conducted by Liu et al., JQ1 treatment led to a reduction in PD-L2 mRNA levels in renal cell carcinoma, and prostate, liver, and lung cancer cell lines (81). Therefore, JQ1 may be a potential molecular drug for tumor treatment. Table 3 summarizes the current new tumor treatment strategies for PD-L2. Although some of them are still in the research stage, they represent the new direction of tumor treatment in the future.
The so-called PD-L2 vaccine is to introduce PD-L2 antigen or proteins or cells carrying the restricted epitopes of human leukocyte antigen (HLA) expressed by PD-L2 into patients, thus inducing the activation of PD-L2 specific T cells. PD-L2-specific T cells, naturally produced in cancer patients, play a dual role in supporting anti-tumor immunity. These T cells can directly kill target cells and indirectly contribute to anti-tumor immunity by releasing pro-inflammatory factors in the tumor microenvironment. In clinical trials, researchers aim to enhance the immune response against PD-L2 by administering the PD-L2 vaccine, hoping to complement other forms of immunotherapy. While the treatment’s effectiveness hasn’t shown significant improvement in patients, it holds potential as a target for cancer vaccine and immune checkpoint-blocking treatments (85).
As a vital component of tumor treatment, the potential of radiotherapy interactions with immune checkpoint inhibitors are also reported. A study revealed a significant increase in PD-L1 and PD-L2 expression in MCF-120 (human breast cancer cells) after hyperthermia (HT) and radiotherapy RT (2×5 Gy) treatment at any temperature (86). Considering the elevated expression of immune checkpoint molecules, incorporating immune checkpoint inhibitors into the combined tumor hyperthermia and radiotherapy approach may optimize therapeutic outcomes. In addition, radiotherapy is previously reported to be able to locally activate the immune system, potentially inducing a systemic immune response and targeting distant metastatic tumors (87). Previous research demonstrated increased efficacy of paolizumab in lung cancer patients who underwent prioradiotherapy (88). And the activation of immune system by radiotherapy radiation may be optimized and improved in tumors with more inhibition of immune system by PD-L2 at baseline. However, understanding the pathway through which radiotherapy affects PD-L1/PD-L2 and investigating the potential relationship between the immune response induced by local radiotherapy require further exploration (31).
Previous studies have shown that transplantation of fecal flora from patients with good response to PD-1 therapy can reduce the drug resistance of melanoma patients to PD-1 therapy, thus improving the tumor treatment effect. Gram-positive anaerobic bacteria in human intestinal microorganisms can promote anti-tumor immune response by down-regulating the expression of PD-L2 and binding RGMB to some extent (65). This will further expand the starting point of tumor treatment. On the one hand, it inspires us to fully strengthen the study of intestinal flora, clarify the types of intestinal microorganisms for tumor treatment, and bring a new dawn to tumor patients who have poor responses to immunotherapy through targeted flora transplantation. On the other hand, using intestinal microorganisms as a discovery platform to identify a new target of cancer immunotherapy-PD-L2-RGMB, and blocking the interaction between PD-L2 and RGMB can enhance the response to cancer immunotherapy. Small molecule drugs targeting specific intestinal microorganisms can be further developed to enhance the effect of cancer immunotherapy.
In conclusion, PD-L2 stands as a key player in various human cancers, showing significant promise as a therapeutic target. Currently, there exist numerous constraints, notably the incomplete comprehension of PD-L2 expression and its regulatory mechanism, as well as the insufficient exploration of related signal pathways. Moreover, the clinical approval of anti-PD-L2 antibodies for tumor treatment remains elusive, necessitating further investigation and discovery. However, the high affinity of PD-L2 for PD-1, the new insight that intestinal microorganisms regulate PD-1 pathway, and the richness of PD-L2 related therapies all indicate that cancer immunotherapy is expected to make a breakthrough. The discovery of new pathways such as PD-L2-RGMB has further expanded the scope of targeted therapy. The continuous exploration of clinical application of PD-L2 has brought exciting prospects for promoting cancer treatment. The full names of abbreviations in this article are displayed in Table 4.
YY: Writing – original draft, Writing – review & editing. XY: Writing – original draft, Writing – review & editing. XB: Conceptualization, Writing – review & editing. JY: Investigation, Writing – review & editing. JS: Writing – original draft, Writing – review & editing.
The author(s) declare that no financial support was received for the research, authorship, and/or publication of this article.
The authors declare that the research was conducted in the absence of any commercial or financial relationships that could be construed as a potential conflict of interest.
All claims expressed in this article are solely those of the authors and do not necessarily represent those of their affiliated organizations, or those of the publisher, the editors and the reviewers. Any product that may be evaluated in this article, or claim that may be made by its manufacturer, is not guaranteed or endorsed by the publisher.
1. Wang J, Seebacher N, Shi H, Kan Q, Duan Z. Novel strategies to prevent the development of multidrug resistance (MDR) in cancer. Oncotarget. (2017) 8:84559–71. doi: 10.18632/oncotarget.v8i48
2. Hanna A, Shevde LA. Hedgehog signaling: modulation of cancer properies and tumor mircroenvironment. Mol Cancer. (2016) 15:24. doi: 10.1186/s12943-016-0509-3
3. Salmaninejad A, Khoramshahi V, Azani A, Soltaninejad E, Aslani S, Zamani MR, et al. PD-1 and cancer: molecular mechanisms and polymorphisms. Immunogenetics. (2018) 70:73–86. doi: 10.1007/s00251-017-1015-5
4. Yearley JH, Gibson C, Yu N, Moon C, Murphy E, Juco J, et al. PD-L2 expression in human tumors: relevance to anti-PD-1 therapy in cancer. Clin Cancer Res. (2017) 23:3158–67. doi: 10.1158/1078-0432.CCR-16-1761
5. Ghiotto M, Gauthier L, Serriari N, Pastor S, Truneh A, Nunès J, et al. PD-L1 and PD-L2 differ in their molecular mechanisms of interaction with PD-1. Int Immunol. (2010) 22:651–60. doi: 10.1093/intimm/dxq049
6. Youngnak P, Kozono Y, Kozono H, Iwai H, Otsuki N, Jin H, et al. Differential binding properties of B7-H1 and B7-DC to programmed death-1. Biochem Biophys Res Commun. (2003) 307:672–7. doi: 10.1016/S0006-291X(03)01257-9
7. Lázár-Molnár E, Scandiuzzi L, Basu I, Quinn T, Sylvestre E, Palmieri E, et al. Structure-guided development of a high-affinity human Programmed Cell Death-1: Implications for tumor immunotherapy. EBioMedicine. (2017) 17:30–44. doi: 10.1016/j.ebiom.2017.02.004
8. Butte MJ, Keir ME, Phamduy TB, Sharpe AH, Freeman GJ. Programmed death-1 ligand 1 interacts specifically with the B7-1 costimulatory molecule to inhibit T cell responses. Immunity. (2007) 27:111–22. doi: 10.1016/j.immuni.2007.05.016
9. Xiao Y, Yu S, Zhu B, Bedoret D, Bu X, Francisco LM, et al. RGMb is a novel binding partner for PD-L2 and its engagement with PD-L2 promotes respiratory tolerance. J Exp Med. (2014) 211:943–59. doi: 10.1084/jem.20130790
10. Burke KP, Chaudhri A, Freeman GJ, Sharpe AH. The B7:CD28 family and friends: Unraveling coinhibitory interactions. Immunity. (2024) 57:223–44. doi: 10.1016/j.immuni.2024.01.013
11. Ludovini V, Bianconi F, Siggillino A, Vannucci J, Baglivo S, Berti V, et al. High PD-L1/IDO-2 and PD-L2/IDO-1 co-expression levels are associated with worse overall survival in resected non-small cell lung cancer patients. Genes. (2021) 12(2):273. doi: 10.3390/genes12020273
12. Takamori S, Takada K, Azuma K, Jogo T, Shimokawa M, Toyokawa G, et al. Prognostic impact of programmed death-ligand 2 expression in primary lung adenocarcinoma patients. Ann Surg Oncol. (2019) 26:1916–24. doi: 10.1245/s10434-019-07231-z
13. Huang KCY, Chiang SF, Chen TW, Chen WTL, Yang PC, Ke TW, et al. Prognostic relevance of programmed cell death 1 ligand 2 (PDCD1LG2/PD-L2) in patients with advanced stage colon carcinoma treated with chemotherapy. Sci Rep. (2020) 10:22330. doi: 10.1038/s41598-020-79419-3
14. Zengin M, Zergeroğlu S, Okcu O, Benek S. PD-1 and PD-L2 expression predict relapse risk and poor survival in patients with stage III colorectal cancer. Cell Oncol. (2021) 44:423–32. doi: 10.1007/s13402-020-00579-5
15. Shan ZG, Zhao YL, Zhang JY, Yan ZB, Wang TT, Mao FY, et al. FasL+ PD-L2+ Identifies a novel immunosuppressive neutrophil population in human gastric cancer that promotes disease progression. Adv Sci Weinh Baden-Wurtt Ger. (2022) 9:e2103543. doi: 10.1002/advs.202103543
16. Nakayama Y, Mimura K, Kua LF, Okayama H, Min AKT, Saito K, et al. Immune suppression caused by PD-L2 expression on tumor cells in gastric cancer. Gastric Cancer. (2020) 23:961–73. doi: 10.1007/s10120-020-01079-z
17. Tanaka K, Miyata H, Sugimura K, Kanemura T, Hamada-Uematsu M, Mizote Y, et al. Negative influence of programmed death-1-ligands on the survival of esophageal cancer patients treated with chemotherapy. Cancer Sci. (2016) 107:726–33. doi: 10.1111/cas.12938
18. Zhao JJ, Zhou ZQ, Wang P, Chen CL, Liu Y, Pan QZ, et al. Orchestration of immune checkpoints in tumor immune contexture and their prognostic significance in esophageal squamous cell carcinoma. Cancer Manag Res. (2018) 10:6457–68. doi: 10.2147/CMAR
19. Duchnowska R, Pęksa R, Radecka B, Mandat T, Trojanowski T, Jarosz B, et al. Immune response in breast cancer brain metastases and their microenvironment: the role of the PD-1/PD-L axis. Breast Cancer Res BCR. (2016) 18:43. doi: 10.1186/s13058-016-0702-8
20. Baptista MZ, Sarian LO, Derchain SFM, Pinto GA, Vassallo J. Prognostic significance of PD-L1 and PD-L2 in breast cancer. Hum Pathol. (2016) 47:78–84. doi: 10.1016/j.humpath.2015.09.006
21. Chervoneva I, Peck AR, Sun Y, Yi M, Udhane SS, Langenheim JF, et al. High PD-L2 predicts early recurrence of ER-positive breast cancer. JCO Precis Oncol. (2023) 7:e2100498. doi: 10.1200/PO.21.00498
22. Solinas C, Garaud S, De Silva P, Boisson A, Van den Eynden G, de Wind A, et al. Immune checkpoint molecules on tumor-infiltrating lymphocytes and their association with tertiary lymphoid structures in human breast cancer. Front Immunol. (2017) 8:1412. doi: 10.3389/fimmu.2017.01412
23. Montoyo-Pujol YG, García-Escolano M, Ponce JJ, Delgado-García S, Martín TA, Ballester H, et al. Variable intrinsic expression of immunoregulatory biomarkers in breast cancer cell lines, mammospheres, and co-cultures. Int J Mol Sci. (2023) 24:4478. doi: 10.3390/ijms24054478
24. Rotman J, den Otter LAS, Bleeker MCG, Samuels SS, Heeren AM, Roemer MGM, et al. PD-L1 and PD-L2 expression in cervical cancer: regulation and biomarker potential. Front Immunol. (2020) 11:596825. doi: 10.3389/fimmu.2020.596825
25. Xue C, Zhu D, Chen L, Xu Y, Xu B, Zhang D, et al. Expression and prognostic value of PD-L1 and PD-L2 in ovarian cancer. Transl Cancer Res. (2019) 8:111–9. doi: 10.21037/tcr
26. Batman S, Matsuo K, Mhawech-Fauceglia P, Munro E, Weisenberger M, Allen A, et al. Intersection of DNA repair pathways and the immune landscape identifies PD-L2 as a prognostic marker in epithelial ovarian cancer. Cancers. (2021) 13:1972. doi: 10.3390/cancers13081972
27. Jung HI, Jeong D, Ji S, Ahn TS, Bae SH, Chin S, et al. Overexpression of PD-L1 and PD-L2 is associated with poor prognosis in patients with hepatocellular carcinoma. Cancer Res Treat Off J Korean Cancer Assoc. (2017) 49:246–54. doi: 10.4143/crt.2016.066
28. Yang H, Zhou X, Sun L, Mao Y. Correlation between PD-L2 expression and clinical outcome in solid cancer patients: A meta-analysis. Front Oncol. (2019) 9:47. doi: 10.3389/fonc.2019.00047
29. Shrestha R, Bridle KR, Crawford DHG, Jayachandran A. TNF−α−mediated epithelial−to−mesenchymal transition regulates expression of immune checkpoint molecules in hepatocellular carcinoma. Mol Med Rep. (2020) 21:1849–60. doi: 10.3892/mmr
30. Tao YY, Shi Y, Gong XQ, Li L, Li ZM, Yang L, et al. Radiomic analysis based on magnetic resonance imaging for predicting PD-L2 expression in hepatocellular carcinoma. Cancers. (2023) 15:365. doi: 10.3390/cancers15020365
31. Zhao SG, Lehrer J, Chang SL, Das R, Erho N, Liu Y, et al. The immune landscape of prostate cancer and nomination of PD-L2 as a potential therapeutic target. J Natl Cancer Inst. (2019) 111:301–10. doi: 10.1093/jnci/djy141
32. Sridharan V, Gjini E, Liao X, Chau NG, Haddad RI, Severgnini M, et al. Immune profiling of adenoid cystic carcinoma: PD-L2 expression and associations with tumor-infiltrating lymphocytes. Cancer Immunol Res. (2016) 4:679–87. doi: 10.1158/2326-6066.CIR-16-0031
33. Zhang Y, Xu J, Hua J, Liu J, Liang C, Meng Q, et al. A PD-L2-based immune marker signature helps to predict survival in resected pancreatic ductal adenocarcinoma. J Immunother Cancer. (2019) 7:233. doi: 10.1186/s40425-019-0703-0
34. Obeid JM, Erdag G, Smolkin ME, Deacon DH, Patterson JW, Chen L, et al. PD-L1, PD-L2 and PD-1 expression in metastatic melanoma: Correlation with tumor-infiltrating immune cells and clinical outcome. OncoImmunology. (2016) 5:e1235107. doi: 10.1080/2162402X.2016.1235107
35. Hu X, li J, Fu M, Zhao X, Wang W. The JAK/STAT signaling pathway: from bench to clinic. Signal Transduct Target Ther. (2021) 6:402. doi: 10.1038/s41392-021-00791-1
36. Matsubara E, Shinchi Y, Komohara Y, Yano H, Pan C, Fujiwara Y, et al. PD-L2 overexpression on tumor-associated macrophages is one of the predictors for better prognosis in lung adenocarcinoma. Med Mol Morphol. (2023) 56(4):250–6. doi: 10.1007/s00795-023-00361-0
37. Xie W, Medeiros LJ, Li S, Tang G, Fan G, Xu J. PD-1/PD-L1 pathway: A therapeutic target in CD30+ Large cell lymphomas. Biomedicines. (2022) 10:1587. doi: 10.3390/biomedicines10071587
38. Ansell SM, Lesokhin AM, Borrello I, Halwani A, Scott EC, Gutierrez M, et al. PD-1 blockade with nivolumab in relapsed or refractory Hodgkin’s lymphoma. N Engl J Med. (2015) 372:311–9. doi: 10.1056/NEJMoa1411087
39. Harb J, Lin PJ, Hao J. Recent development of Wnt signaling pathway inhibitors for cancer therapeutics. Curr Oncol Rep. (2019) 21:12. doi: 10.1007/s11912-019-0763-9
40. Parsons MJ, Tammela T, Dow LE. WNT as a driver and dependency in cancer. Cancer Discov. (2021) 11:2413–29. doi: 10.1158/2159-8290.CD-21-0190
41. Galluzzi L, Spranger S, Fuchs E, López-Soto A. WNT signaling in cancer immunosurveillance. Trends Cell Biol. (2019) 29:44–65. doi: 10.1016/j.tcb.2018.08.005
42. Pinczewski J, Obeng RC, Slingluff CL, Engelhard VH. Phospho-β-catenin expression in primary and metastatic melanomas and in tumor-free visceral tissues, and associations with expression of PD-L1 and PD-L2. Pathol Res Pract. (2021) 224:153527. doi: 10.1016/j.prp.2021.153527
43. Lu T, Stark GR. NF-κB: regulation by methylation. Cancer Res. (2015) 75:3692–5. doi: 10.1158/0008-5472.CAN-15-1022
44. Xu P, Sun Z, Wang Y, Miao C. Long-term use of indomethacin leads to poor prognoses through promoting the expression of PD-1 and PD-L2 via TRIF/NF-κB pathway and JAK/STAT3 pathway to inhibit TNF-α and IFN-γ in hepatocellular carcinoma. Exp Cell Res. (2015) 337:53–60. doi: 10.1016/j.yexcr.2015.07.007
45. Curran CS, Gupta S, Sanz I, Sharon E. PD-1 immunobiology in systemic lupus erythematosus. J Autoimmun. (2019) 97:1–9. doi: 10.1016/j.jaut.2018.10.025
46. Spirina L, Yurmazov Z, Usynin E, Kondakova I, Ladutko E, Choynzonov E. Regulation of immunity in clear cell renal carcinoma: role of PD-1, PD-L1, and PD-L2. Curr Issues Mol Biol. (2021) 43:1072–80. doi: 10.3390/cimb43020076
47. Yu L, Wei J, Liu P. Attacking the PI3K/Akt/mTOR signaling pathway for targeted therapeutic treatment in human cancer. Semin Cancer Biol. (2022) 85:69–94. doi: 10.1016/j.semcancer.2021.06.019
48. Proctor DT, Patel Z, Lama S, Resch L, van Marle G, Sutherland GR. Identification of PD-L2, B7-H3 and CTLA-4 immune checkpoint proteins in genetic subtypes of meningioma. Oncoimmunology. (2019) 8:e1512943. doi: 10.1080/2162402X.2018.1512943
49. Spirina L, Avgustinovich A, Afanas’ev S, Volkov M, Dobrodeev A, Cheremisina O, et al. PD-L1 status in gastric cancers, association with the transcriptional, growth factors, AKT/mTOR components change, and autophagy initiation. Int J Mol Sci. (2021) 22:11176. doi: 10.3390/ijms222011176
50. Kumagai Y, Takeuchi O, Akira S. TLR9 as a key receptor for the recognition of DNA. Adv Drug Deliv Rev. (2008) 60:795–804. doi: 10.1016/j.addr.2007.12.004
51. Krieg AM. Toll-like receptor 9 (TLR9) agonists in the treatment of cancer. Oncogene. (2008) 27:161–7. doi: 10.1038/sj.onc.1210911
52. Baruah P, Bullenkamp J, Wilson POG, Lee M, Kaski JC, Dumitriu IE. TLR9 mediated tumor-stroma interactions in human papilloma virus (HPV)-positive head and neck squamous cell carcinoma up-regulate PD-L1 and PD-L2. Front Immunol. (2019) 10:1644. doi: 10.3389/fimmu.2019.01644
53. Green MR, Monti S, Rodig SJ, Juszczynski P, Currie T, O’Donnell E, et al. Integrative analysis reveals selective 9p24.1 amplification, increased PD-1 ligand expression, and further induction via JAK2 in nodular sclerosing Hodgkin lymphoma and primary mediastinal large B-cell lymphoma. Blood. (2010) 116:3268–77. doi: 10.1182/blood-2010-05-282780
54. Xu Y, Wu Y, Zhang S, Ma P, Jin X, Wang Z, et al. A tumor-specific super-enhancer drives immune evasion by guiding synchronous expression of PD-L1 and PD-L2. Cell Rep. (2019) 29:3435–47.e4. doi: 10.1016/j.celrep.2019.10.093
55. Tang YC, Hsiao JR, Jiang SS, Chang JY, Chu PY, Liu KJ, et al. c-MYC-directed NRF2 drives Malignant progression of head and neck cancer via glucose-6-phosphate dehydrogenase and transketolase activation. Theranostics. (2021) 11:5232–47. doi: 10.7150/thno.53417
56. Wang Y, Du J, Gao Z, Sun H, Mei M, Wang Y, et al. Evolving landscape of PD-L2: bring new light to checkpoint immunotherapy. Br J Cancer. (2023) 128:1196–207. doi: 10.1038/s41416-022-02084-y
57. Matsui M, Corey DR. Non-coding RNAs as drug targets. Nat Rev Drug Discov. (2017) 16:167–79. doi: 10.1038/nrd.2016.117
58. Lu TX, Rothenberg ME. MicroRNA. J Allergy Clin Immunol. (2018) 141:1202–7. doi: 10.1016/j.jaci.2017.08.034
59. Zavareh RB, Spangenberg SH, Woods A, Martínez-Peña F, Lairson LL. HSP90 inhibition enhances cancer immunotherapy by modulating the surface expression of multiple immune checkpoint proteins. Cell Chem Biol. (2021) 28:158–68.e5. doi: 10.1016/j.chembiol.2020.10.005
60. Li S, Zhang W, Wu C, Gao H, Yu J, Wang X, et al. HOXC10 promotes proliferation and invasion and induces immunosuppressive gene expression in glioma. FEBS J. (2018) 285:2278–91. doi: 10.1111/febs.14476
61. Fan F, Chen K, Lu X, Li A, Liu C, Wu B. Dual targeting of PD-L1 and PD-L2 by PCED1B-AS1 via sponging hsa-miR-194-5p induces immunosuppression in hepatocellular carcinoma. Hepatol Int. (2021) 15:444–58. doi: 10.1007/s12072-020-10101-6
62. El Ahanidi H, El Azzouzi M, Hafidi Alaoui C, Tetou M, Bensaid M, Chaoui I, et al. Immune checkpoint and telomerase crosstalk is mediated by miRNA-138 in bladder cancer. Front Oncol. (2021) 11:795242. doi: 10.3389/fonc.2021.795242
63. Zhang Y, Li Z, Chen M, Chen H, Zhong Q, Liang L, et al. lncRNA TCL6 correlates with immune cell infiltration and indicates worse survival in breast cancer. Breast Cancer Tokyo Jpn. (2020) 27:573–85. doi: 10.1007/s12282-020-01048-5
64. Fidelle M, Lebhar I, Zitvogel L, Kroemer G. Microbiota-associated immunotherapy resistance caused by deficient PD-L2 - RGMb signaling. Oncoimmunology. (2023) 12:2224679. doi: 10.1080/2162402X.2023.2224679
65. Park JS, Gazzaniga FS, Wu M, Luthens AK, Gillis J, Zheng W, et al. Targeting PD-L2-RGMb overcomes microbiome-related immunotherapy resistance. Nature. (2023) 617:377–85. doi: 10.1038/s41586-023-06026-3
66. Jiao M, Guo H, Chen Y, Li L, Zhang L. DARS-AS1 promotes clear cell renal cell carcinoma by sequestering miR-194-5p to up-regulate DARS. BioMed Pharmacother. (2020) 128:110323. doi: 10.1016/j.biopha.2020.110323
67. Liebig JK, Kuphal S, Bosserhoff AK. HuRdling senescence: huR breaks BRAF-induced senescence in melanocytes and supports melanoma growth. Cancers. (2020) 12:1299. doi: 10.3390/cancers12051299
68. Wang C, Li X, Zhang L, Chen Y, Dong R, Zhang J, et al. miR-194-5p down-regulates tumor cell PD-L1 expression and promotes anti-tumor immunity in pancreatic cancer. Int Immunopharmacol. (2021) 97:107822. doi: 10.1016/j.intimp.2021.107822
69. Quinn JJ, Chang HY. Unique features of long non-coding RNA biogenesis and function. Nat Rev Genet. (2016) 17:47–62. doi: 10.1038/nrg.2015.10
70. Xu S, Wang Q, Kang Y, Liu J, Yin Y, Liu L, et al. Long noncoding RNAs control the modulation of immune checkpoint molecules in cancer. Cancer Immunol Res. (2020) 8:937–51. doi: 10.1158/2326-6066.CIR-19-0696
71. Liu J, Qian J, Mo Q, Tang L, Xu Q. Long non-coding RNA PCED1B-AS1 promotes the proliferation of colorectal adenocarcinoma through regulating the miR-633/HOXA9 axis. Bioengineered. (2022) 13(3):5407–20. doi: 10.1080/21655979.2022.2037225
72. Ren J, Xu N, Zhou R, Huang F, Zhang H, Li W. Long non-coding RNA PCED1B antisense RNA 1 promotes gastric cancer progression via modulating microRNA-215-3p / C-X-C motif chemokine receptor 1 axis. Bioengineered. (2021) 12:6083–95. doi: 10.1080/21655979.2021.1971503
73. Zhang Y, Ma H, Chen C. Long non−coding RNA PCED1B−AS1 promotes pancreatic ductal adenocarcinoma progression by regulating the miR−411−3p/HIF−1α axis. Oncol Rep. (2021) 46:134. doi: 10.3892/or
74. Qin J, Zhu T, Wu W, Chen H, He Y. Long non-coding RNA PCED1B-AS1 promotes the progression of clear cell renal cell carcinoma through miR-484/ZEB1 axis. OncoTargets Ther. (2021) 14:393–402. doi: 10.2147/OTT.S270149
75. Chen X, Guo J, Zhou F, Ren W, Pu J, Mutti L, et al. Over-expression of long non-coding RNA-AC099850.3 correlates with tumor progression and poor prognosis in lung adenocarcinoma. Front Oncol. (2022) 12:895708. doi: 10.3389/fonc.2022.895708
76. Wu F, Wei H, Liu G, Zhang Y. Bioinformatics profiling of five immune-related lncRNAs for a prognostic model of hepatocellular carcinoma. Front Oncol. (2021) 11:667904. doi: 10.3389/fonc.2021.667904
77. Kulkarni P, Dasgupta P, Hashimoto Y, Shiina M, Shahryari V, Tabatabai ZL, et al. A lncRNA TCL6-miR-155 interaction regulates the Src-Akt-EMT network to mediate kidney cancer progression and metastasis. Cancer Res. (2021) 81:1500–12. doi: 10.1158/0008-5472.CAN-20-0832
78. Luo LH, Jin M, Wang LQ, Xu GJ, Lin ZY, Yu DD, et al. Long noncoding RNA TCL6 binds to miR-106a-5p to regulate hepatocellular carcinoma cells through PI3K/AKT signaling pathway. J Cell Physiol. (2020) 235:6154–66. doi: 10.1002/jcp.29544
79. Park EM, Chelvanambi M, Bhutiani N, Kroemer G, Zitvogel L, Wargo JA. Targeting the gut and tumor microbiota in cancer. Nat Med. (2022) 28:690–703. doi: 10.1038/s41591-022-01779-2
80. Lv J, Jiang Z, Yuan J, Zhuang M, Guan X, Liu H, et al. Pan-cancer analysis identifies PD-L2 as a tumor promotor in the tumor microenvironment. Front Immunol. (2023) 14:1093716. doi: 10.3389/fimmu.2023.1093716
81. Liu K, Zhou Z, Gao H, Yang F, Qian Y, Jin H, et al. JQ1, a BET-bromodomain inhibitor, inhibits human cancer growth and suppresses PD-L1 expression. Cell Biol Int. (2019) 43:642–50. doi: 10.1002/cbin.11139
82. Tang S, Kim PS. A high-affinity human PD-1/PD-L2 complex informs avenues for small-molecule immune checkpoint drug discovery. Proc Natl Acad Sci USA. (2019) 116:24500–6. doi: 10.1073/pnas.1916916116
83. Skalniak L, Zak KM, Guzik K, Magiera K, Musielak B, Pachota M, et al. Small-molecule inhibitors of PD-1/PD-L1 immune checkpoint alleviate the PD-L1-induced exhaustion of T-cells. Oncotarget. (2017) 8:72167–81. doi: 10.18632/oncotarget.v8i42
84. Oaknin A, Gilbert L, Tinker AV, Brown J, Mathews C, Press J, et al. Safety and antitumor activity of dostarlimab in patients with advanced or recurrent DNA mismatch repair deficient/microsatellite instability-high (dMMR/MSI-H) or proficient/stable (MMRp/MSS) endometrial cancer: interim results from GARNET-a phase I, single-arm study. J Immunother Cancer. (2022) 10:e003777. doi: 10.1136/jitc-2021-003777
85. Ahmad SM, Martinenaite E, Holmström M, Jørgensen MA, Met Ö, Nastasi C, et al. The inhibitory checkpoint, PD-L2, is a target for effector T cells: Novel possibilities for immune therapy. Oncoimmunology. (2017) 7:e1390641. doi: 10.1080/2162402X.2017.1390641
86. Sengedorj A, Hader M, Heger L, Frey B, Dudziak D, Fietkau R, et al. The effect of hyperthermia and radiotherapy sequence on cancer cell death and the immune phenotype of breast cancer cells. Cancers. (2022) 14:2050. doi: 10.3390/cancers14092050
87. Park B, Yee C, Lee KM. The effect of radiation on the immune response to cancers. Int J Mol Sci. (2014) 15:927–43. doi: 10.3390/ijms15010927
88. Shaverdian N, Lisberg AE, Bornazyan K, Veruttipong D, Goldman JW, Formenti SC, et al. Previous radiotherapy and the clinical activity and toxicity of pembrolizumab in the treatment of non-small-cell lung cancer: a secondary analysis of the KEYNOTE-001 phase 1 trial. Lancet Oncol. (2017) 18:895–903. doi: 10.1016/S1470-2045(17)30380-7
Keywords: PD-1, PD-L2, cancer, immunity, combination therapy
Citation: Yang Y, Yan X, Bai X, Yang J and Song J (2024) Programmed cell death-ligand 2: new insights in cancer. Front. Immunol. 15:1359532. doi: 10.3389/fimmu.2024.1359532
Received: 21 December 2023; Accepted: 18 March 2024;
Published: 28 March 2024.
Edited by:
Dallas Flies, NextCure, Inc., United StatesReviewed by:
Wenzheng Guo, University of Kentucky, United StatesCopyright © 2024 Yang, Yan, Bai, Yang and Song. This is an open-access article distributed under the terms of the Creative Commons Attribution License (CC BY). The use, distribution or reproduction in other forums is permitted, provided the original author(s) and the copyright owner(s) are credited and that the original publication in this journal is cited, in accordance with accepted academic practice. No use, distribution or reproduction is permitted which does not comply with these terms.
*Correspondence: Jianbo Song, amlhbmJvMjYxMUBzeG11LmVkdS5jbg==
†These authors have contributed equally to this work
Disclaimer: All claims expressed in this article are solely those of the authors and do not necessarily represent those of their affiliated organizations, or those of the publisher, the editors and the reviewers. Any product that may be evaluated in this article or claim that may be made by its manufacturer is not guaranteed or endorsed by the publisher.
Research integrity at Frontiers
Learn more about the work of our research integrity team to safeguard the quality of each article we publish.