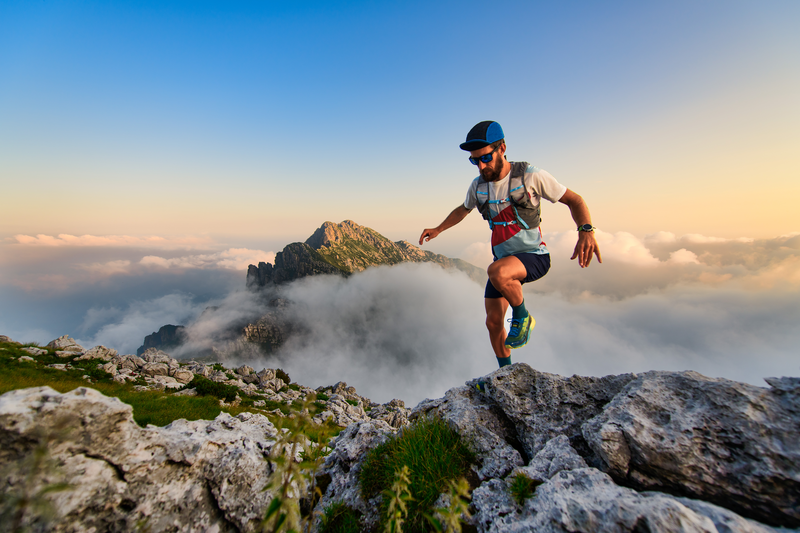
95% of researchers rate our articles as excellent or good
Learn more about the work of our research integrity team to safeguard the quality of each article we publish.
Find out more
REVIEW article
Front. Immunol. , 01 March 2024
Sec. Cancer Immunity and Immunotherapy
Volume 15 - 2024 | https://doi.org/10.3389/fimmu.2024.1355812
This article is part of the Research Topic New Approaches to Neoadjuvant Therapy and Translational Resection in Hepatobiliary Malignancies View all 9 articles
Surgical resection and liver transplant remain the only curative therapies for most patients with hepatocellular carcinoma (HCC). Systemic therapy options have typically been ineffective, but recent advances, such as the combination of immune checkpoint inhibitors and targeted therapies, have shown great promise. Neoadjuvant systemic therapy in resectable or locally advanced HCC is under active investigation with encouraging results in small, early-phase trials. Many of these completed and ongoing trials include combinations of systemic therapy (e.g. immune checkpoint inhibitors, tyrosine kinase inhibitors), transarterial therapies, and radiation. Despite early successes, larger trials with evaluation of long-term oncologic outcomes are needed to determine the role of neoadjuvant systemic therapy in patients with HCC who may be eligible for curative intent surgery or transplant.
Hepatocellular carcinoma (HCC) is an aggressive primary liver tumor that most commonly arises in the setting of chronic liver inflammation or cirrhosis (1). Surgical resection or transplant remain the most effective treatments for patients with HCC. Patients who are not surgical candidates due to co-morbidities, liver dysfunction, or advanced disease rely on alternative options like locoregional therapies (e.g. transarterial therapies, percutaneous ablation) and/or systemic therapy. Additionally, many patients develop local or distant recurrence and will need systemic therapy (2). Sorafenib, a multi-targeted tyrosine kinase inhibitor (TKI), was the first effective systemic therapy for HCC and had remained the only systemic option for patients with advanced disease for over a decade until the REFLECT trial demonstrated noninferiority versus lenvatinib (3–5). Unfortunately, sorafenib and lenvatinib still only demonstrate an average survival of 12-14 months among patients with unresectable HCC. As such, there has been intense interest in identifying new systemic therapies to improve long term outcomes.
Immune checkpoint inhibitors (ICI) have proven to be effective in many solid tumors. Through interaction at immune checkpoints, cancer cells can inhibit T cells and evade the immune system, which can contribute to local progression or metastasis. ICIs block this interaction and restore the normal function of T cells (6, 7). The unique immune microenvironment of the liver plays a significant role in tumorigenesis and has been the focus of research efforts to evaluate the use of ICIs for HCC (8, 9). In the landmark IMbrave150 trial, patients with advanced HCC had improved survival with atezolizumab (PD-L1 inhibitor) and bevacizumab (vascular endothelial growth factor receptor (VEGFR) inhibitor) compared with sorafenib (10). This combination is now recommended as first-line therapy in the advanced setting (11). Additionally, the HIMALAYA trial demonstrated the efficacy of tremelimumab and durvalumab for patients with advanced HCC; in turn, this combination has also been approved as first-line therapy (12).
Given the success of ICI in the setting of advanced and metastatic HCC, studies are actively evaluating their use in the neoadjuvant and adjuvant setting. Currently, data on adjuvant or neoadjuvant options are relatively limited among patients with HCC (2). Recently, the combination of atezolizumab and bevacizumab demonstrated improved recurrence-free survival (RFS) in the adjuvant setting (13). In the neoadjuvant setting, ICI may allow for patients to be downstaged to be eligible for surgery or transplantation (14–17). Additionally, there is growing evidence that neoadjuvant immunotherapy may prime the immune system and prevent local or distant recurrence (18–23). The Barcelona Clinic Liver Cancer (BCLC) guidelines provide a logically designed, validated algorithm for initial management strategies across the spectrum of presentation of HCC (Figure 1) (11). Despite the recent success of systemic therapy combinations in advanced HCC, the optimal strategy for incorporating these therapies into the treatment paradigm for resectable and potentially resectable HCC has not been established. We herein review the published literature, as well as ongoing clinical trials focused on neoadjuvant systemic therapy for HCC.
Figure 1 BCLC staging and first-line treatment algorithm. Liver function is evaluated using the Child–Pugh staging. AFP, alpha–fetoprotein; ALBI, albumin–bilirubin; BSC, best supportive care; ECOG-PS, Eastern Cooperative Oncology Group-performance status; LT, liver transplantation; MELD, model of end-stage liver disease; TACE, transarterial chemoembolization. This figure was reprinted with permission from reference 9. Appropriate copyright permission was obtained.
The tumor microenvironment (TME) in hepato-pancreatico-biliary (HPB) cancers is a disordered inflammatory state characterized by infiltration of immune cells with paradoxically immunosuppressive phenotypes, such as regulatory T cells, exhausted CD4+ and CD8+ T cells, M2 macrophages, myeloid-derived suppressor cells (MDSC), and cancer-associated fibroblasts (CAF) (9). The T cells within the TME, referred to as tumor-infiltrating lymphocytes (TIL), are the primary target of immune checkpoint inhibitors (Figure 2). Many of these agents act on either the programmed death receptor 1 (PD-1) located on host T cells or the programmed death ligand 1 (PD-L1), which can be expressed by macrophages, some activated T cells, B cells, as well as certain tumor cells (24). Other ICI act on cytotoxic lymphocyte antigen 4 (CTLA-4), a receptor on host T cells that interacts with CD80/86 and inhibits MHC-II-dependent T cell receptor signaling (25). A third immune checkpoint that has been more recently described is LAG3, which is also a T cell surface receptor (26). Monoclonal antibodies targeting these checkpoints were initially used in immunogenic cancers such as melanoma, renal cell carcinoma, and non-small cell lung cancer, but are being widely studied in a variety of cancer types. These agents may be most applicable to cancers with PD-L1 expression, high tumor mutational burden, or DNA mismatch repair deficiency (27). However, the inherently tolerogenic microenvironment in the liver may represent another TME phenotype in which ICI may be effective (28). With established efficacy in the advanced setting, there is growing interest in the use of ICI in the neoadjuvant setting (29).
Figure 2 Schematic depicting various immune checkpoint ligands and corresponding receptors. CTLA-4, cytotoxic T lymphocyte antigen 4; CD, cluster of differentiation; TCR, T cell receptor; PD-1, programmed death receptor 1; MHC, major histocompatibility complex. This figure was reprinted from an open access publication (6).
Cemiplimab is a relatively newer monoclonal antibody against PD-1 and is currently used in advanced cutaneous squamous cell carcinoma (30). Marron et al. reported results from a single-arm, open-label, multicenter phase II study of neoadjuvant cemiplimab for patients with resectable HCC (31). This is part of a larger study of neoadjuvant cemiplimab for resectable HCC, non-small cell lung cancer (NSCLC), and head and neck squamous cell carcinoma (NCT03916627). This study enrolled 21 patients with resectable HCC between November 2019 and August 2020. Participants received two doses of preoperative cemiplimab, curative intent partial hepatectomy, and eight cycles of postoperative cemiplimab. The primary endpoint was significant tumor necrosis, defined as >70% necrosis on surgical pathology. Most patients (95%) underwent hepatectomy; one patient had surgery delayed due to pneumonitis. Among those individuals who underwent surgery, 4 (20%) had major pathologic response, defined as >70% necrosis, and three patients had 50-75% tumor necrosis (Table 1). Previous studies have demonstrated that the utility of RECIST 1.1 criteria is limited in patients on immunotherapy regimens (32). In line with these findings, this trial noted that on-treatment MRI was a better predictor of tumor necrosis than RECIST 1.1 criteria (32). This trial is currently ongoing and long term outcomes have not yet been reported.
There are three trials examining neoadjuvant single-agent ICI in HCC (Table 2). One (NCT05471674) has been completed; this study evaluated neoadjuvant nivolumab in borderline resectable HCC with a primary outcome of pathologic tumor response. Secondary endpoints include RFS, OS, short-term surgical outcomes, and safety. Results have not yet been reported. Two studies of neoadjuvant pembrolizumab in resectable HCC are ongoing, with primary outcomes including RFS and TIL from resected specimens.
Table 2 Neoadjuvant immunotherapy trials currently registered with clinicaltrials.gov.
Combining checkpoint inhibitors with complementary mechanisms of action has been well established in other disease sites. Kaseb et al. conducted an open-label phase II trial comparing perioperative nivolumab (anti-PD-1) alone to nivolumab and ipilimumab (anti-CTLA-4) in patients with resectable HCC (33). The primary endpoint was safety and tolerability, with secondary endpoints including response rate, as well as pathologic and immunologic correlates of response. Among 27 treated patients, 20 underwent surgical resection. Of note, none of the surgical cancellations were due to toxicity, but rather to disease progression (n=4), concern for insufficient future liver remnant (n=2), and prohibitive adhesive disease (n=1). As seen in trials of combination ipilimumab and nivolumab for other disease sites, immune-related adverse events were higher with combination therapy compared with monotherapy (34–36). Major pathologic response rate was 27% versus 33% for combination therapy versus monotherapy cohorts, respectively (33). However, long-term outcomes were improved with combination therapy, with median progression-free survival (PFS) 19.4 months for the combination therapy cohort versus 9.4 months for nivolumab alone (Table 1) (33).
The PRIME-HCC trial of preoperative nivolumab with ipilimumab is ongoing, but results from an interim analysis were presented at the American Society of Clinical Oncology (ASCO) annual meeting in 2022 (37). Among a safety report of 15 participants, one (7%) had a grade 3 or higher treatment-related adverse event. There were 13 patients with RECIST-evaluable preoperative imaging; objective response rate was 23%, with a disease control rate of 92%. Nine patients had evaluable pathology specimens, of which 78% had a pathologic response; pathologic complete response was seen in two (22%) (37). The full published results of this trial are eagerly anticipated, particularly in terms of long-term recurrence-free and overall survival (38).
Recently, a meta-analysis of neoadjuvant immune checkpoint inhibitors in HCC was published (39). This systematic review and meta-analysis included four published studies (31, 33, 40, 41), as well as five studies that were available only as conference abstracts (including PRIME-HCC described above) (37). In the pooled analysis, neoadjuvant ICI in HCC was beneficial in terms of pathologic response and recurrence-free survival, with decreased odds [odds ratio (OR) 0.17 (95% confidence interval (CI) 0.10-0.30)] of a pathologic complete response, and major pathologic response [OR 0.38 (95% CI 0.21-0.69)] in the absence of neoadjuvant ICI (39). The peer-reviewed published studies were weighted more heavily; however 34.6% of the weighted data related to pathologic complete response were based on data from conference abstracts only, which raises concerns about possible publication bias in the pooled results in the meta-analysis (39, 42). Many of the included studies also did not include a comparison group, which limited generalizability of the results in favor of neoadjuvant ICI (39).
In addition to PRIME-HCC, there are four other ongoing studies examining neoadjuvant combination immunotherapy for patients with HCC (Table 2). These studies include the NEOTOMA trial, which is evaluating tremelimumab and durvalumab in resectable HCC, and a different trial of a LAG3 inhibitor in addition to nivolumab among patients with potentially resectable HCC. Another trial combines nivolumab with a novel agent targeting the chemokines CCR2/CCR5, which may mitigate the effect of myeloid-derived suppressor cells (MDSC) in the TME (43). MDSC are immature host immune cells that tend to accumulate in the TME and suppress antitumor immunity through a variety of mechanisms (44). The prevalence of MDSC in the TME has been associated with poor outcomes among patients with HPB cancers (45). However, targeting MDSC by manipulating their chemotaxis can increase the immunogenicity of an otherwise immunologically cold TME (46). This unique immunotherapy combination is representative of a larger trend within cancer immunotherapy trials in which established agents such as checkpoint inhibitors are combined with other immune-modulating agents with distinct mechanisms.
Cancer vaccines have been studied in solid tumors for many years, but have generally had low efficacy in most solid tumors, especially in the advanced setting (47, 48). Few trials have examined neoadjuvant vaccine strategies in HPB cancers with a few notable exceptions (49, 50). As monotherapy, these vaccines are generally well-tolerated, but have more efficacy when combined with ICI (51). Developed either as a personalized vaccine against the individual’s unique tumor-associated antigens (TAA) or with a prespecified TAA, vaccines are usually combined with an immune adjuvant. For example, among patients with HCC, heat shock protein 70 (HSP70) is a commonly utilized tumor antigen and the glypican-3 (GPC3) domain of carcinoembryonic antigen (CEA) has been proposed as a biomarker (52).
Nakajima et al. published results from the YCP02 trial, a phase I trial of a vaccine that combined HSP70, GPC3, a LAG3 antibody, and the immune adjuvant poly-ICLC. This vaccine was designed to elicit a T cell response to HSP70 and GPC3, using LAG3 checkpoint inhibition as a counter-regulatory mechanism; it was first demonstrated to be safe in a dose-escalation study among patients with metastatic gastrointestinal cancers (53). In a subsequent phase I trial, subjects with resectable HCC received 6 weekly vaccine doses preoperatively and 10 doses postoperatively (54). Vaccination was well tolerated (Table 1). Analysis of surgical pathology specimens demonstrated that 60% of participants (n=12) had infiltration of the tumor with CD8+ T cells specific for HSP70 and/or GPC3 (54). Further investigation is needed to determine whether this vaccine may prevent recurrence or prolong survival.
There has been increased interest in combining different modalities with immune therapy, particularly in the neoadjuvant setting. Trans-arterial therapies, ICI, and TKI have been studied in the neoadjuvant setting with the goals of local downstaging and preventing recurrence. Combining ICI with other modalities may increase the efficacy of ICI by increasing immunogenicity in the neoadjuvant setting; this approach has been validated in preclinical studies in HPB cancers and in immunogenic solid tumors such as melanoma (20, 55, 56).
In a phase II trial, combination of immunotherapy with trans-arterial chemoembolization (TACE) was evaluated in the neoadjuvant setting for patients with HCC (57). Drug-eluting bead TACE (DEB-TACE) was combined with sintilimab, a PD-1 inhibitor, with the rationale that TACE will cause the release of neoantigens and subsequently make HCC more immunogenic. In turn, this process may increase the efficacy of ICI therapy. Sixty patients with BCLC A or B outside the Milan criteria were treated with this combination. The majority of participants were male (85%) and had cirrhosis secondary to hepatitis B infection (84%). At a median follow-up of 26 months, median PFS was 30.5 months, with a 12-month PFS estimate of 75%. Among all treated patients, objective response rate was 62%. Ultimately 51 patients proceeded to surgical resection and 7 (14%) had a pathologic complete response (Table 1). These patients also had a corresponding decrease in tumor markers, including AFP and PIVKA-II (57).
The combination of ICI with tyrosine kinase inhibitors has gained increasing interest in the neoadjuvant setting in HCC. Nivolumab with cabozantinib, a multi-kinase inhibitor with activity against VEGFR2, was studied in the neoadjuvant setting with the goal of converting unresectable HCC to resectable and increasing antitumor immunity (40). In this single-center phase 1b trial, 15 patients with borderline resectable or locally advanced HCC were given 8 weeks of neoadjuvant therapy. Surgery was attempted in 13 of 15 patients and completed in 12; 5 patients had major pathologic response (Table 1). This study met its primary endpoint by demonstrating feasibility of this neoadjuvant therapy regimen (40).
Xia et al. reported a single-arm, open-label, phase II trial evaluating camrelizumab (anti-PD-1) and apatinib (a VEGFR2 inhibitor) in the preoperative setting for patients with resectable HCC (41). This combination was well tolerated among the 18 enrolled participants, with only 16% experiencing a grade 3 or 4 adverse event. Major pathologic response rate, defined as >90% tumor necrosis, occurred in 17.6% of participants. RFS at one year was 53.9% (Table 1). Exploratory analyses of circulating tumor DNA (ctDNA) suggested a correlation between decrease in ctDNA levels and response to neoadjuvant therapy, whereas rising ctDNA during adjuvant therapy was associated with recurrence (41).
There are many ongoing trials involving combination NAT (Table 3). Of the 34 trials identified, 28 (82.3%) involve at least one immune checkpoint inhibitor, and 26 (76.5%) involve at least one tyrosine kinase inhibitor. There were 10 studies (29.4%) utilizing three or more modalities, including ICI, TKI, transarterial therapies, hepatic arterial infusion chemotherapy (HAIC), systemic chemotherapy, and SBRT. The majority of these studies (n=44, 88%) give NAT prior to curative intent resection, with the remainder (n=6, 12%) prior to transplant.
Table 3 Combination neoadjuvant therapy trials currently registered with clinicaltrials.gov.
Neoadjuvant systemic therapy in HCC has demonstrated early success in clinical trials. These early phase neoadjuvant trials have laid the groundwork for further expansion in future clinical trials focused on combination neoadjuvant therapy. Future efforts will likely aim to incorporate targeted therapies, immunotherapies, and locoregional therapies to prime the immune system and downstage locally advanced tumors.
Preclinical and correlative studies bolster the theory that NAT will lead to a local tumor response and prevent recurrence by altering the TME; this process has been observed both with immune therapies and other agents (58). The TME of HCC warrants special consideration given the liver’s complex immune system. In a healthy liver, the immune microenvironment is tightly regulated due to constant exposure to both self and non-self antigens (e.g. dietary and bacterial products) (28). In the setting of chronic inflammation, the microenvironment is altered and reflects a state of immune cell exhaustion. This process makes the liver more susceptible to the growth and expansion of malignant cells. As such, HCC commonly arises in patients with cirrhosis, which is due to chronic inflammation from various etiologies (e.g. alcohol or fat consumption, chronic hepatitis) (59). When cancer cells evade the immune system through manipulation of immune checkpoints, the underlying problem can be exacerbated. As such, ICIs can disrupt the HCC TME to restore immune cell function against cancer cells (28).
Several synergistic mechanisms for the efficacy of neoadjuvant immunotherapy in HCC have been described. One consistent finding with these trials and in other disease sites is the formation of tertiary lymphoid structures (TLS), which serve as the locus for generating T cell memory and are associated with improved survival (60–63). Importantly, examination of TLS was one of the correlative analyses in the aforementioned study of cabozantinib and nivolumab, in which responders had a higher density of TLS as well as more tumor-specific CD4+ and CD8+ T cells (40). A further analysis of these patients was conducted using spatial transcriptomics, which identified cancer-associated fibroblasts, intratumor inflammatory markers, and modulation of the extracellular matrix as predictors of response (58). Additionally, the co-localization of PAX5, a regulator of B cell maturation, with areas of increased B cell gene expression suggests an important role for these B cells in response to immunotherapy (58). Knowledge of the role of B cells in the tumor microenvironment is evolving, as most of the focus on the role of TIL in the TME has been on tumor infiltrating T lymphocytes. These so-called TIL-Bs play an important role in supporting the development of tumor-specific T cells as well as promoting innate antitumor immunity by recruiting and reprogramming natural killer cells and macrophages (64).
These immunologic correlates of response to ICI are well described in the setting of surgical resection, yet there are unanswered questions about the feasibility of ICI prior to transplant. For example, it has yet to be established whether preoperative immunotherapy is compatible with postoperative immunosuppression, and whether this approach affects oncologic outcomes, risk of rejection, or immunosuppression-related infections or cancers (65).
While there are many future directions for studying neoadjuvant systemic therapy in HCC, perhaps the two most exciting opportunities involve priming the immune system to prevent recurrence and downstaging locally advanced tumors to allow for resection or transplant. While the cabozantinib and nivolumab study examined both topics, it was a small phase 1b trial and larger trials are needed to confirm these results. There are other studies of combination therapies that have enrolled patients with advanced disease, such as portal vein invasion or individuals otherwise ineligible for resection or transplant; these studies have reported impressive results with downstaging. For example, a phase II trial of lenvatinib and toripalimab with hepatic artery infusion chemotherapy with 5-fluorouracil and oxaliplatin (FOLFOX) demonstrated an ORR of 66.7%; perhaps more interestingly, 22.2% of participants became eligible for resection or transplant despite having locally advanced disease at presentation (66).
Adaptive trial design may be a more efficient strategy to evaluate whether neoadjuvant systemic therapy with or without local therapies may be able to downstage locally advanced HCC to allow for resection or transplant. Eligibility for resection or transplant, response to neoadjuvant therapy, and/or relevant biomarkers may be able to stratify participants into different treatment arms within the same trial. An example of adaptive trial design in neoadjuvant therapy is the OPRA trial in rectal cancer, where participants were assigned to a surgical treatment arm only after post-NAT restaging (67). Another example is in pancreatic cancer, where the effect of NAT on resectability is a crucial and dynamic component of treatment selection and sequencing; therefore neoadjuvant trials such as SWOG S-1505 are designed in a way to allow reassessment of resectability after NAT (16, 23, 68). In HCC, determination of resectability may be improved by dynamic assessment over time. Thus, patients with locally advanced HCC may be a population in which NAT will become the most useful. To further this research, trials must thoroughly investigate biomarkers including immunologic correlates and ctDNA in addition to long term survival outcomes. In turn, this approach will allow for better selection of patient populations who will benefit the most from NAT.
There may be safety considerations with implementing NAT prior to major liver resection or transplant. In particular, many of these combinations contain TKIs; those agents with the most efficacy in HCC either preferentially (sorafenib, lenvatinib) or selectively (apatinib) inhibit angiogenesis and related growth factor signaling. Interestingly, these agents seem to have a safer perioperative safety profile than bevacizumab, which has been shown to increase the risk of post-operative morbidity and mortality (69, 70). The effect of ICI alone on surgical outcomes is less clear. The PRIME-HCC trial using both nivolumab and ipilimumab will provide interesting insight from this standpoint (38). While it is unlikely that surgical complications would increase as a direct result of ICI, immune-related adverse events such as endocrinopathies, pneumonitis, or even hepatitis may have important implications for perioperative outcomes (71).
Some limitations common to the currently available evidence for NAT in HCC include small sample size, heterogeneous inclusion criteria, and non-uniform outcome measures, such as varying definitions of “major pathologic response.” Ultimately, large, multi-center trials with long-term oncologic outcomes are needed to demonstrate the utility of neoadjuvant systemic therapy in patients with HCC. These trials should evaluate long-term outcomes such as overall survival and RFS, surrogate markers such as pathologic response and local downstaging, and immunologic and tumor-intrinsic biomarkers.
RC: Writing – original draft. SR: Writing – review & editing. TP: Writing – review & editing.
The author(s) declare that no financial support was received for the research, authorship, and/or publication of this article.
The authors declare that the research was conducted in the absence of any commercial or financial relationships that could be construed as a potential conflict of interest.
All claims expressed in this article are solely those of the authors and do not necessarily represent those of their affiliated organizations, or those of the publisher, the editors and the reviewers. Any product that may be evaluated in this article, or claim that may be made by its manufacturer, is not guaranteed or endorsed by the publisher.
1. Siegel RL, Miller KD, Jemal A. Cancer statistics, 2020. CA: A Cancer J Clin. (2020) 70(1):7–30. doi: 10.3322/caac.21590
2. Benson AB, D'Angelica MI, Abbott DE, Anaya DA, Anders R, Are C, et al. Hepatobiliary cancers, version 2.2021, NCCN clinical practice guidelines in oncology. J Natl Compr Canc Netw. (2021) 19(5):541–65. doi: 10.6004/jnccn.2021.0022
3. Llovet JM, Ricci S, Mazzaferro V, Hilgard P, Gane E, Blanc JF, et al. Sorafenib in advanced hepatocellular carcinoma. N Engl J Med. (2008) 359(4):378–90. doi: 10.1056/NEJMoa0708857
4. Bruix J, Takayama T, Mazzaferro V, Chau GY, Yang J, Kudo M, et al. Adjuvant sorafenib for hepatocellular carcinoma after resection or ablation (STORM): a phase 3, randomised, double-blind, placebo-controlled trial. Lancet Oncol. (2015) 16(13):1344–54. doi: 10.1016/S1470-2045(15)00198-9
5. Kudo M, Finn RS, Qin S, Han KH, Ikeda K, Piscaglia F, et al. Lenvatinib versus sorafenib in first-line treatment of patients with unresectable hepatocellular carcinoma: a randomised phase 3 non-inferiority trial. Lancet. (2018) 391(10126):1163–73. doi: 10.1016/S0140-6736(18)30207-1
6. Wei Y, Li Z. LAG3-PD-1 combo overcome the disadvantage of drug resistance. Front Oncol. (2022) 12:831407. doi: 10.3389/fonc.2022.831407
7. Alsaab HO, Sau S, Alzhrani R, Tatiparti K, Bhise K, Kashaw SK, et al. PD-1 and PD-L1 checkpoint signaling inhibition for cancer immunotherapy: Mechanism, combinations, and clinical outcome. Front Pharmacol. (2017) 8:561. doi: 10.3389/fphar.2017.00561
8. Robinson MW, Harmon C, O’Farrelly C. Liver immunology and its role in inflammation and homeostasis. Cell Mol Immunol. (2016) 13(3):267–76. doi: 10.1038/cmi.2016.3
9. Ruff SM, Shannon AH, Beane JD, Pawlik TM. Highlighting novel targets in immunotherapy for liver cancer. Expert Rev Gastroenterol Hepatol. (2022) 16(11-12):1029–41. doi: 10.1080/17474124.2022.2150841
10. Finn RS, Qin S, Ikeda M, Galle PR, Ducreux M, Kim TY, et al. Atezolizumab plus bevacizumab in unresectable hepatocellular carcinoma. N Engl J Med. (2020) 382(20):1894–905. doi: 10.1056/NEJMoa1915745
11. Reig M, Forner A, Rimola J, Ferrer-Fàbrega J, Burrel M, Garcia-Criado Á, et al. BCLC strategy for prognosis prediction and treatment recommendation: The 2022 update. J Hepatol. (2022) 76(3):681–93. doi: 10.1016/j.jhep.2021.11.018
12. Abou-Alfa GK, Lau G, Kudo M, Chan SL, Kelley RK, Furuse J, et al. Tremelimumab plus durvalumab in unresectable hepatocellular carcinoma. NEJM Evidence. (2022) 1(8). doi: 10.1056/evidoa2100070
13. Kudo M. Adjuvant atezolizumab-bevacizumab after curative therapy for hepatocellular carcinoma. Hepatobiliary Surg Nutr. (2023) 12(3):435–9. doi: 10.21037/hbsn-23-203
14. Laschtowitz A, Roderburg C, Tacke F, Mohr R. Preoperative immunotherapy in hepatocellular carcinoma: Current state of the art. J Hepatocell Carcinoma. (2023) 10:181–91. doi: 10.2147/JHC.S347944
15. Heimbach JK, Gores GJ, Nagorney DM, Rosen CB. Liver transplantation for perihilar cholangiocarcinoma after aggressive neoadjuvant therapy: a new paradigm for liver and biliary malignancies? Surgery. (2006) 140(3):331–4. doi: 10.1016/j.surg.2006.01.010
16. Brown ZJ, Heh V, Labiner HE, Brock GN, Ejaz A, Dillhoff M, et al. Surgical resection rates after neoadjuvant therapy for localized pancreatic ductal adenocarcinoma: meta-analysis. Br J Surg. (2022) 110(1):34–42. doi: 10.1093/bjs/znac354
17. Cloyd JM, Prakash L, Vauthey JN, Aloia TA, Chun YS, Tzeng CW, et al. The role of preoperative therapy prior to pancreatoduodenectomy for distal cholangiocarcinoma. Am J Surg. (2019) 218(1):145–50. doi: 10.1016/j.amjsurg.2018.08.024
18. Robert C. Is earlier better for melanoma checkpoint blockade? Nat Med. (2018) 24(11):1645–8. doi: 10.1038/s41591-018-0250-0
19. Amaria RN, Reddy SM, Tawbi HA, Davies MA, Ross MI, Glitza IC, et al. Neoadjuvant immune checkpoint blockade in high-risk resectable melanoma. Nat Med. (2018) 24(11):1649–54. doi: 10.1038/s41591-018-0197-1
20. Reijers I, Rozeman EA, Menzies AM, Wiel BAVD, Eriksson H, Suijkerbuijk K, et al. Personalized response-driven adjuvant therapy after combination ipilimumab and nivolumab in high-risk resectable stage III melanoma: PRADO trial. J Clin Oncol. (2019) 37(15_suppl):TPS9605–TPS. doi: 10.1200/JCO.2019.37.15_suppl.TPS9605
21. Petricevic B, Kabiljo J, Zirnbauer R, Walczak H, Laengle J, Bergmann M. Neoadjuvant immunotherapy in gastrointestinal cancers – the new standard of care? Semin Cancer Biol. (2022) 86:834–50. doi: 10.1016/j.semcancer.2022.05.015
22. Wang H, Li S, Liu T, Chen J, Dang J. Neoadjuvant immune checkpoint inhibitor in combination with chemotherapy or chemoradiotherapy in resectable esophageal cancer: A systematic review and meta-analysis. Front Immunol. (2022) 13:998620. doi: 10.3389/fimmu.2022.998620
23. Chick RC, Gunderson AJ, Rahman S, Cloyd JM. Neoadjuvant immunotherapy for localized pancreatic cancer: Challenges and early results. Cancers (Basel). (2023) 15(15). doi: 10.3390/cancers15153967
24. McLoughlin KC, Brown ZJ, Shukla Y, Shukla V. Promise and pitfalls of immune checkpoint inhibitors in hepato-pancreato-biliary malignancies. Discovery Med. (2018) 26(142):85–92.
25. Rotte A. Combination of CTLA-4 and PD-1 blockers for treatment of cancer. J Exp Clin Cancer Res. (2019) 38(1):255. doi: 10.1186/s13046-019-1259-z
26. Li R, Qiu J, Zhang Z, Qu C, Tang Z, Yu W, et al. Prognostic significance of lymphocyte-activation gene 3 (LAG3) in patients with solid tumors: a systematic review, meta-analysis and pan-cancer analysis. Cancer Cell Int. (2023) 23(1):306. doi: 10.1186/s12935-023-03157-5
27. Marabelle A, Le DT, Ascierto PA, Di Giacomo AM, De Jesus-Acosta A, Delord JP, et al. Efficacy of pembrolizumab in patients with noncolorectal high microsatellite Instability/Mismatch repair-deficient cancer: Results from the phase II KEYNOTE-158 study. J Clin Oncol. (2020) 38(1):1–10. doi: 10.1200/JCO.19.02105
28. Brown ZJ, Ruff SM, Pawlik TM. The effect of liver disease on hepatic microenvironment and implications for immune therapy. Front Pharmacol. (2023) 14:1225821. doi: 10.3389/fphar.2023.1225821
29. Ruff SM, Manne A, Cloyd JM, Dillhoff M, Ejaz A, Pawlik TM. Current landscape of immune checkpoint inhibitor therapy for hepatocellular carcinoma. Curr Oncol. (2023) 30(6):5863–75. doi: 10.3390/curroncol30060439
30. Migden MR, Khushalani NI, Chang ALS, Lewis KD, Schmults CD, Hernandez-Aya L, et al. Cemiplimab in locally advanced cutaneous squamous cell carcinoma: results from an open-label, phase 2, single-arm trial. Lancet Oncol. (2020) 21(2):294–305. doi: 10.1016/S1470-2045(19)30728-4
31. Marron TU, Fiel MI, Hamon P, Fiaschi N, Kim E, Ward SC, et al. Neoadjuvant cemiplimab for resectable hepatocellular carcinoma: a single-arm, open-label, phase 2 trial. Lancet Gastroenterol Hepatol. (2022) 7(3):219–29. doi: 10.1016/S2468-1253(21)00385-X
32. Wolchok JD, Hoos A, O'Day S, Weber JS, Hamid O, Lebbe C, et al. Guidelines for the evaluation of immune therapy activity in solid tumors: immune-related response criteria. Clin Cancer Res. (2009) 15(23):7412–20. doi: 10.1158/1078-0432.ccr-09-1624
33. Kaseb AO, Hasanov E, Cao HST, Xiao L, Vauthey JN, Lee SS, et al. Perioperative nivolumab monotherapy versus nivolumab plus ipilimumab in resectable hepatocellular carcinoma: a randomised, open-label, phase 2 trial. Lancet Gastroenterol Hepatol. (2022) 7(3):208–18. doi: 10.1016/S2468-1253(21)00427-1
34. Wolchok JD, Chiarion-Sileni V, Gonzalez R, Rutkowski P, Grob J-J, Cowey CL, et al. Overall survival with combined nivolumab and ipilimumab in advanced melanoma. New Engl J Med. (2017) 377(14):1345–56. doi: 10.1056/nejmoa1709684
35. Schadendorf D, Hassel J, Fluck M, Eigentler T, Loquai C, Berneburg M, et al. Adjuvant immunotherapy with nivolumab (NIVO) alone or in combination with ipilimumab (IPI) versus placebo in stage IV melanoma patients with no evidence of disease (NED): a randomized, double-blind phase 2 trial (IMMUNED). Ann Oncol. (2019) 30:v851–934.
36. Rozeman EA, Menzies AM, van Akkooi ACJ, Adhikari C, Bierman C, van de Wiel BA, et al. Identification of the optimal combination dosing schedule of neoadjuvant ipilimumab plus nivolumab in macroscopic stage III melanoma (OpACIN-neo): a multicentre, phase 2, randomised, controlled trial. Lancet Oncol. (2019) 20(7):948–60. doi: 10.1016/S1470-2045(19)30151-2
37. D'Alessio A, Pai M, Spalding D, Rajagopal P, Talbot T, Goldin R, et al. Preliminary results from a phase ib study of neoadjuvant ipilimumab plus nivolumab prior to liver resection for hepatocellular carcinoma: The PRIME-HCC trial. J Clin Oncol. (2022) 40(16_suppl):4093–3. doi: 10.1200/JCO.2022.40.16_suppl.4093
38. Pinato DJ, Cortellini A, Sukumaran A, Cole T, Pai M, Habib N, et al. PRIME-HCC: phase ib study of neoadjuvant ipilimumab and nivolumab prior to liver resection for hepatocellular carcinoma. BMC Cancer. (2021) 21(1). doi: 10.1186/s12885-021-08033-x
39. Zhao M, Chen S, Li C, Du Y, Li P. Neoadjuvant immune checkpoint inhibitors for resectable hepatocellular carcinoma: A systematic review and meta-analysis. Cancers. (2023) 15(3):600. doi: 10.3390/cancers15030600
40. Ho WJ, Zhu Q, Durham J, Popovic A, Xavier S, Leatherman J, et al. Neoadjuvant cabozantinib and nivolumab converts locally advanced HCC into resectable disease with enhanced antitumor immunity. Nat Cancer. (2021) 2(9):891–903. doi: 10.1038/s43018-021-00234-4
41. Xia Y, Tang W, Qian X, Li X, Cheng F, Wang K, et al. Efficacy and safety of camrelizumab plus apatinib during the perioperative period in resectable hepatocellular carcinoma: a single-arm, open label, phase II clinical trial. J Immunother Cancer. (2022) 10(4). doi: 10.1136/jitc-2022-004656
42. Scherer RW, Saldanha IJ. How should systematic reviewers handle conference abstracts? a view from the trenches. Syst Rev. (2019) 8(1):264. doi: 10.1186/s13643-019-1188-0
43. Nywening TM, Wang-Gillam A, Sanford DE, Belt BA, Panni RZ, Cusworth BM, et al. Targeting tumour-associated macrophages with CCR2 inhibition in combination with FOLFIRINOX in patients with borderline resectable and locally advanced pancreatic cancer: a single-centre, open-label, dose-finding, non-randomised, phase 1b trial. Lancet Oncol. (2016) 17(5):651–62. doi: 10.1016/S1470-2045(16)00078-4
44. Ostrand-Rosenberg S, Sinha P, Beury DW, Clements VK. Cross-talk between myeloid-derived suppressor cells (MDSC), macrophages, and dendritic cells enhances tumor-induced immune suppression. Semin Cancer Biol. (2012) 22(4):275–81. doi: 10.1016/j.semcancer.2012.01.011
45. Groth C, Hu X, Weber R, Fleming V, Altevogt P, Utikal J, et al. Immunosuppression mediated by myeloid-derived suppressor cells (MDSCs) during tumour progression. Br J Cancer. (2019) 120(1):16–25. doi: 10.1038/s41416-018-0333-1
46. Sarhan D, Eisinger S, He F, Bergsland M, Pelicano C, Driescher C, et al. Targeting myeloid suppressive cells revives cytotoxic anti-tumor responses in pancreatic cancer. iScience. (2022) 25(11):105317. doi: 10.1016/j.isci.2022.105317
47. Clifton GT, Kohrt HE, Peoples GE. Critical issues in cancer vaccine trial design. Vaccine. (2015) 33(51):7386–92. doi: 10.1016/j.vaccine.2015.09.019
48. Hale DF, Vreeland TJ, Peoples GE. Arming the immune system through vaccination to prevent cancer recurrence. Am Soc Clin Oncol Educ Book. (2016) 35:e159–67. doi: 10.14694/edbk_158946
49. Li K, Tandurella JA, Gai J, Zhu Q, Lim SJ, Thomas DL, et al. Multi-omic analyses of changes in the tumor microenvironment of pancreatic adenocarcinoma following neoadjuvant treatment with anti-PD-1 therapy. Cancer Cell. (2022) 40(11). doi: 10.1016/j.ccell.2022.10.001
50. Heumann T, Judkins C, Li K, Lim SJ, Hoare J, Parkinson R, et al. A platform trial of neoadjuvant and adjuvant antitumor vaccination alone or in combination with PD-1 antagonist and CD137 agonist antibodies in patients with resectable pancreatic adenocarcinoma. Nat Commun. (2023) 14(1):3650. doi: 10.1038/s41467-023-39196-9
51. Vreeland TJ, Clifton GT, Herbert GS, Hale DF, Jackson DO, Berry JS, et al. Gaining ground on a cure through synergy: combining checkpoint inhibitors with cancer vaccines. Expert Rev Clin Immunol. (2016) 12(12):1347–57. doi: 10.1080/1744666x.2016.1202114
52. Nakatsura T, Yoshitake Y, Senju S, Monji M, Komori H, Motomura Y, et al. Glypican-3, overexpressed specifically in human hepatocellular carcinoma, is a novel tumor marker. Biochem Biophys Res Commun. (2003) 306(1):16–25. doi: 10.1016/s0006-291x(03)00908-2
53. Nakajima M, Hazama S, Tamada K, Udaka K, Kouki Y, Uematsu T, et al. A phase i study of multi-HLA-binding peptides derived from heat shock protein 70/glypican-3 and a novel combination adjuvant of hLAG-3Ig and poly-ICLC for patients with metastatic gastrointestinal cancers: YNP01 trial. Cancer Immunology Immunother. (2020) 69(8):1651–62. doi: 10.1007/s00262-020-02518-7
54. Nakajima M, Hazama S, Tokumitsu Y, Shindo Y, Matsui H, Matsukuma S, et al. Phase i study of a novel therapeutic vaccine as perioperative treatment for patients with surgically resectable hepatocellular carcinoma: The YCP02 trial. Hepatol Res. (2023) 53(7):649–60. doi: 10.1111/hepr.13900
55. Zhang H, Ye L, Yu X, Jin K, Wu W. Neoadjuvant therapy alters the immune microenvironment in pancreatic cancer. Front Immunol. (2022) 13:956984. doi: 10.3389/fimmu.2022.956984
56. Michelakos T, Cai L, Villani V, Sabbatino F, Kontos F, Fernández-Del Castillo C, et al. Tumor microenvironment immune response in pancreatic ductal adenocarcinoma patients treated with neoadjuvant therapy. JNCI: J Natl Cancer Institute. (2021) 113(2):182–91. doi: 10.1093/jnci/djaa073
57. Guo C, Zhang J, Huang X, Chen Y, Sheng J, Sun J, et al. Preoperative sintilimab plus transarterial chemoembolization for hepatocellular carcinoma exceeding the milan criteria: A phase II trial. Hepatol Commun. (2023) 7(3):e0054. doi: 10.1097/HC9.0000000000000054
58. Zhang S, Yuan L, Danilova L, Mo G, Zhu Q, Deshpande A, et al. Spatial transcriptomics analysis of neoadjuvant cabozantinib and nivolumab in advanced hepatocellular carcinoma identifies independent mechanisms of resistance and recurrence. Genome Med. (2023) 15(1). doi: 10.1186/s13073-023-01218-y
59. Sara A, Ruff SM, Noonan AM, Pawlik TM. Real-world use of immunotherapy for hepatocellular carcinoma. Pragmat Obs Res. (2023) 14:63–74. doi: 10.2147/POR.S397972
60. Shu DH, Ho WJ, Kagohara LT, Girgis A, Shin SM, Danilova L, et al. Immune landscape of tertiary lymphoid structures in hepatocellular carcinoma (HCC) treated with neoadjuvant immune checkpoint blockade. bioRxiv. (2023). doi: 10.1101/2023.10.16.562104
61. Helmink BA, Reddy SM, Gao J, Zhang S, Basar R, Thakur R, et al. B cells and tertiary lymphoid structures promote immunotherapy response. Nature. (2020) 577(7791):549–55. doi: 10.1038/s41586-019-1922-8
62. Gunderson AJ, Rajamanickam V, Bui C, Bernard B, Pucilowska J, Ballesteros-Merino C, et al. Germinal center reactions in tertiary lymphoid structures associate with neoantigen burden, humoral immunity and long-term survivorship in pancreatic cancer. OncoImmunology. (2021) 10(1):1900635. doi: 10.1080/2162402x.2021.1900635
63. Lynch KT, Young SJ, Meneveau MO, Wages NA, Engelhard VH, Slingluff CL Jr, et al. Heterogeneity in tertiary lymphoid structure b-cells correlates with patient survival in metastatic melanoma. J ImmunoTherapy Cancer. (2021) 9(6):e002273. doi: 10.1136/jitc-2020-002273
64. Laumont CM, Nelson BH. B cells in the tumor microenvironment: Multi-faceted organizers, regulators, and effectors of anti-tumor immunity. Cancer Cell. (2023) 41(3):466–89. doi: 10.1016/j.ccell.2023.02.017
65. Katariya NN, Lizaola-Mayo BC, Chascsa DM, Giorgakis E, Aqel BA, Moss AA, et al. Immune checkpoint inhibitors as therapy to down-stage hepatocellular carcinoma prior to liver transplantation. Cancers (Basel). (2022) 14(9). doi: 10.3390/cancers14092056
66. He M, Ming S, Lai Z, Li Q. A phase II trial of lenvatinib plus toripalimab and hepatic arterial infusion chemotherapy as a first-line treatment for advanced hepatocellular carcinoma (LTHAIC study). J Clin Oncol. (2021) 39(15_suppl):4083–3. doi: 10.1200/JCO.2021.39.15_suppl.4083
67. Smith JJ, Strombom P, Chow OS, Roxburgh CS, Lynn P, Eaton A, et al. Assessment of a watch-and-Wait strategy for rectal cancer in patients with a complete response after neoadjuvant therapy. JAMA Oncol. (2019) 5(4):e185896. doi: 10.1001/jamaoncol.2018.5896
68. Ahmad SA, Duong M, Sohal DPS, Gandhi NS, Beg MS, Wang-Gillam A, et al. Surgical outcome results from SWOG S1505: A randomized clinical trial of mFOLFIRINOX versus Gemcitabine/Nab-paclitaxel for perioperative treatment of resectable pancreatic ductal adenocarcinoma. Ann Surg. (2020) 272(3):481–6. doi: 10.1097/SLA.0000000000004155
69. Shah DR, Dholakia S, Shah RR. Effect of tyrosine kinase inhibitors on wound healing and tissue repair: implications for surgery in cancer patients. Drug Saf. (2014) 37(3):135–49. doi: 10.1007/s40264-014-0139-x
70. Gordon CR, Rojavin Y, Patel M, Zins JE, Grana G, Kann B, et al. A review on bevacizumab and surgical wound healing: an important warning to all surgeons. Ann Plast Surg. (2009) 62(6):707–9. doi: 10.1097/SAP.0b013e3181828141
Keywords: hepatocellular carcinoma, neoadjuvant, immunotherapy, targeted therapy, immune checkpoint inhibitor
Citation: Chick RC, Ruff SM and Pawlik TM (2024) Neoadjuvant systemic therapy for hepatocellular carcinoma. Front. Immunol. 15:1355812. doi: 10.3389/fimmu.2024.1355812
Received: 14 December 2023; Accepted: 19 February 2024;
Published: 01 March 2024.
Edited by:
Alfred Wei Chieh Kow, National University of Singapore, SingaporeReviewed by:
Arjun Khunger, Peacehealth Sacred Heart Medical center at Riverbend, United StatesCopyright © 2024 Chick, Ruff and Pawlik. This is an open-access article distributed under the terms of the Creative Commons Attribution License (CC BY). The use, distribution or reproduction in other forums is permitted, provided the original author(s) and the copyright owner(s) are credited and that the original publication in this journal is cited, in accordance with accepted academic practice. No use, distribution or reproduction is permitted which does not comply with these terms.
*Correspondence: Timothy M. Pawlik, dGltLnBhd2xpa0Bvc3VtYy5lZHU=
Disclaimer: All claims expressed in this article are solely those of the authors and do not necessarily represent those of their affiliated organizations, or those of the publisher, the editors and the reviewers. Any product that may be evaluated in this article or claim that may be made by its manufacturer is not guaranteed or endorsed by the publisher.
Research integrity at Frontiers
Learn more about the work of our research integrity team to safeguard the quality of each article we publish.