- 1Univ Paris Est-Creteil (UPEC), Institut National de la Santé et de la Recherche Médicale (INSERM), Institut Mondor de la Recherche Biomédicale (IMRB), Creteil, France
- 2Etablissement Français du Sang (EFS), Ivry-sur-Seine, France
- 3Laboratory of Excellence, Biogénèse et Pathologies du Globule Rouge (GR-Ex), Paris, France
- 4Service de Maladies Infectieuses et Immunologie Clinique, Centre Hospitalier Universitaire Henri-Mondor, Assistance Publique-Hôpitaux de Paris (AP-HP), Université Paris-Est Créteil (UPEC), Créteil, France
- 5Institut Cochin, Inserm U1016, Centre National de la Recherche Scientifique (CNRS) UMR8104, Université Paris-Cité, Paris, France
- 6Faculté de Médecine and Centre de Recherche ARThrite, Université Laval, Québec, QC, Canada
- 7Centre de Recherche du Centre Hospitalier Universitaire de Québec, Université Laval, Québec, QC, Canada
- 8Etablissement Français du Sang Auvergne-Rhône-Alpes, Saint-Etienne, France
- 9SAINBIOSE, INSERM, U1059, University of Lyon, Saint-Etienne, France
- 10Division of Infectious Diseases, Queen’s University, Kingston, ON, Canada
Introduction: People living with HIV (PLWH) now benefit from combined antiviral treatments that durably control viral replication. These antiretroviral treatments decrease mortality and improve quality of life in PLWH, but do not completely control the excessive non-specific activation of the immune system in PLWH. This chronic immune activation is a key element of HIV immunopathology that contributes to the pathophysiology of inflammatory comorbid conditions, such as cardiovascular disorders, cancer and autoimmune diseases. Circulating non-exosomal extracellular vesicles, also known as microparticles (MPs) are detected in these diseases and have been linked to immune activation. The objective of this study was to characterize the MPs present in PLWH and to assess their association with chronic immune activation.
Methods: We performed flow cytometry for the complete phenotypic characterization of MPs from fresh plasma from PLWH and from people without HIV as the control group. The absolute number, size and cellular origin of MPs were evaluated. The immunoregulatory profile was determined by cell origin, for MPs derived from platelets (PMPs), monocytes (MMPs) and T lymphocytes (LMPs).
Results: PLWH had significantly more circulating MPs than controls, for MPs of all sizes originating from T lymphocytes, red blood cells, neutrophils, dendritic cells, B lymphocytes and endothelial cells. PMPs and MMPs were not more numerous in PLWH, but the immunoregulatory phenotypes of these MPs differed between PLWH and controls. These differences in immunoregulatory molecule expression profile were also observed for LMPs. PDL1, ICOSL, CCR5, TGFβ1, MHC classes I and II, TRAIL, CXCR4, OX40, DC-SIGN, CTLA4 and PDL2 were more strongly expressed on the surface of MPs from PLWH than on those from controls.
Conclusion: MPs are an important element in intercellular communication, making it possible to transfer phenotypes and functions to immune cells. The significantly higher numbers of MPs expressing diverse immunomodulatory molecules in PLWH may make a major contribution to the maintenance and/or the development of immune-cell activation in these individuals.
Introduction
Antiretroviral treatment (ART) controls viral replication in people living with HIV (PLWH), thereby increasing life expectancy, but successful treatment requires high levels of adherence and ART can have multiple adverse effects in long-term use. PLWH on ART have a higher life expectancy due to a decrease in the incidence of opportunistic diseases associated with immune recovery, but they may experience other non-infectious diseases, such as cardiovascular diseases and cancer, which may be favored by excessive non-specific chronic activation of the immune system. This chronic activation contributes an immune aging process, constitutes a significant barrier to the development of immune therapy and is a key factor in the immunopathology of HIV (1).
The extracellular vesicles (EVs) secreted by cells can be classified into several subgroups, including exosomes, apoptotic bodies, and non-exosomal extracellular vesicles, which are also known as microparticles (MPs), microvesicles, or ectosomes (2–4). MPs are generally larger (200-900 nm) than exosomes and are generated by budding of the plasma membrane (5). These vesicles are present in the blood and carry membrane and cytoplasmic elements, such as membrane receptors, cytokines and nucleic acids, from the cell of origin (5–7).
MPs are involved in intercellular communication and can modulate the immune system through interactions with many immune cells (conventional CD4+ TL, Tfh, Th17, Treg, monocytes, B lymphocytes, dendritic cells) (8–15). The underlying mechanisms remain incompletely understood, but these interactions may involve immune ligands/receptors present on the surface of the MPs. We recently showed that CD27+ and CD70+ MPs can transfer these receptors to CD4+ TLs, thereby increasing activation and lymphoproliferation (11).
MPs are also linked to the pathogenesis of viral infections, through roles in viral dissemination, inflammation, and modulation of the immune system (12, 16, 17). EVs have been shown to play an important role in the diffusion of HIV infection via the transfer of coreceptors, notably CCR5 and CXCR4 (18, 19). They may also reduce HIV-associated humoral responses by inhibiting the in vitro production of immunoglobulins G and A by memory B cells (20). However, almost all studies on EVs and HIV have focused exclusively on the total population of EVs (21–23). As a result, it is not possible to draw any firm conclusions on the role of a specific subset of EVs, such as MPs, in HIV infection and the modulation of immune responses. Nevertheless, we know that the concentration of circulating MPs increases in PLWH (20, 24–26) and that the MP phenotype may be altered in PLWH, which may have an even greater impact on the activation state of immune cells and the regulation of their response.
We used flow cytometry for extensive characterization of the phenotypes of MPs from fresh plasma obtained from PLWH, comparing the results for these individuals with those for people without HIV. We determined the absolute number, size, and cellular origin of MPs. We also characterized the proteins expressed on the membrane of these MPs with a panel of cellular activation and immune checkpoint markers potentially involved in chronic immune cell activation. The immunoregulatory profile of MPs by cell origin was also specified for those with the strongest immunoregulatory potential: MPs derived from platelets (PMPs), monocytes (MMPs) and T lymphocytes (LMPs) (8, 9, 15, 27, 28).
Materials and methods
Study population
We used fresh blood samples from patients followed in the infectious diseases and clinical immunology department of Henri-Mondor University Hospital (Creteil, France). The patients included had at least five years of follow-up for HIV infection. They were on active antiretroviral therapy, had an undetectable viral load (< 50 copies/mL) and had a CD4/CD8 ratio greater than 0.5. Demographics, clinical and treatment characteristics, and virological outcomes were collected. Healthy blood donors were enrolled as a control group for comparison with the PLWH, with whom they were matched for sex, ethnicity, and body mass index (BMI) (Supplementary Tables S1, S2). Blood samples from the control group were provided by the French national blood bank (Etablissement Français du Sang, EFS). None of the controls had had an infection (bacterial, viral, fungal, yeast) or had been vaccinated in the 30 days preceding inclusion. The study was approved by the local Ethics Committee, and all participants gave written informed consent.
MP-enriched EV preparation
MP-enriched EVs were isolated as previously described (9, 10, 29), by differential centrifugation at an initial speed of 3,000 x g at 4°C for 10 minutes. The supernatant was then centrifuged at 13,000 x g at 4°C for 10 minutes for the preparation of a platelet-free supernatant. MP-enriched EVs were concentrated by centrifuging the platelet-free supernatant for 1 hour at 100,000 x g at 4°C. MP-enriched EVs were resuspended in filtered PBS (filtration through a 0.1 μm pore-size PES membrane) and MPs were characterized by flow cytometry detection.
MP phenotyping
MPs were labeled as previously described (10, 11, 29), with fluorochrome-conjugated monoclonal antibodies. MPs were labeled with five different panels from the antibodies described in Supplemental Table S3. Fluorescence was assessed with a 20-parameter LSR Fortessa flow cytometer with a small-particle option (BD Biosciences) based on photomultiplier (PMT)-coupled forward scatter (FSC) detection. This mode of detection was used to ensure the optimal detection of MPs with diameters of 200 to 900 nm. The performance of the flow cytometer was checked before each assay. Megamix-Plus FSC and SSC beads (BioCytex, Marseille, France) of known dimensions (with mean diameters of 200 nm, 500 nm and 900 nm) were used for the standardization of FSC-PMT parameters and definition of the MP gate. MPs were acquired at low speed and quantified in Trucount tubes (BD Biosciences).
Flow cytometry analysis
Flow cytometry data were analyzed with FlowJo software (v.10.7.1, Ashland, OR).
Statistical analysis
All analyses were performed with Prism 6.07 software (GraphPad Software, La Jolla, CA). Only significant differences between groups (P<0.05) are indicated on the data plots.
Results
Characterization of MPs in PLWH
We compared the profiles of MPs from fresh plasma between PLWH (n = 42) and controls (n = 21) (Figure 1). The MP gate extended from just below the 200 nm bead signal up to the 900 nm bead signal on the side scatter (SSC) and photomultiplier (PMT)-forward scatter (FSC) dot plot (Figure 1A). The number and size distribution of MPs were determined by considering three size classes: small (< 300 nm), medium (300-500 nm), and large (> 500 nm) MPs (Figures 1A–C).
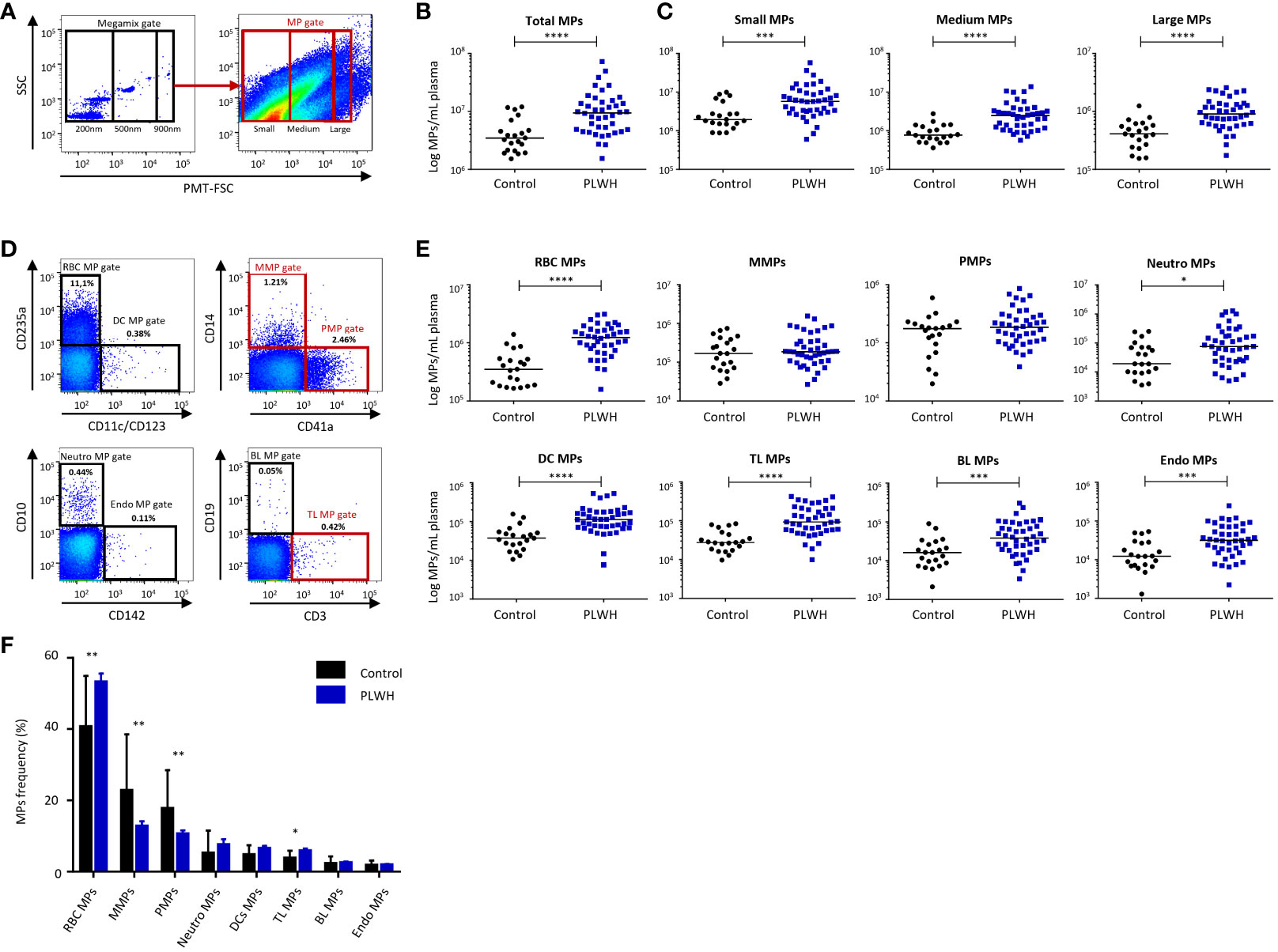
Figure 1 Distribution of MPs by size and cellular origin: comparison of PLWH and controls. (A) Example of the gating strategy used for MP phenotyping by flow cytometry for the control group (n = 21) and PLWH (n = 42) (21 experiments with 2 patients and 1 control per experiment). On the left, a dot plot showing the settings based on fluorescent beads for the differentiation of three particle sizes: 200, 500 and 900 nm in diameter. On the right, dot plot for MP acquisition on a Fortessa flow cytometer for one representative PLWH. (B) Total MPs and (C) MPs of various sizes were quantified by flow cytometry and their concentrations were determined with Trucount tubes. (D) Example of the gating strategy used for the cellular origin phenotyping of MPs by flow cytometry for the control group (n = 21) and PLWH (n = 42) (21 experiments with 2 patients and 1 control per experiment). RBC MPs, MMPs, PMPs, Neutro MPs, DC MPs, TL MPs, BL MPs and Endo MPs were acquired in the MP gate. The indicated percentages represent the cell subpopulations of the MPs out of the total population. Representative FACS plots for each cellular subtype of MPs are shown. (E) Each cellular subtype of MPs was quantified by flow cytometry and MP concentrations were determined with Trucount tubes. The cellular markers are arranged in descending order of the number of MPs/mL expressing them in plasma. Horizontal bars indicate the median values. (F) The frequencies of CD235a+ RBC MP, CD14+ MMPs, CD41a+ PMPs, CD10+ Neutro MPs, CD11c+, CD123+ DC MPs, CD3+ TL MPs, CD19+ BL MPs and CD142+ Endo MPs were determined by flow cytometry for the control group (n = 21) and PLWH (n = 42) (21 experiments, with 2 patients and 1 control per experiment). The percentages were reported on the totality of MPs of cellular origin studied. P values (P<0.05 considered significant) were obtained in Mann-Whitney and post hoc tests. **** P<0.0001, *** P<0.001, ** P<0.01, * P<0.05.
PLWH had significantly more circulating MPs than the controls (13.1x106 ± 2.1x106 vs. 4.7x106 ± 0.7x106 MPs/ml plasma, P<0.0001) (Figure 1B). This large increase in the number of MPs concerned MPs of all sizes: small MPs, 8.8x106 ± 1.6x106 vs. 3.2x106 ± 0.6x106 MPs/ml plasma (P=0.0003); medium-sized MPs, 3.2x106 ± 0.5x106 vs. 1.0x106 ± 0.1x106 MPs/ml plasma (P<0.0001); large MPs, 1.1x106 ± 0.1x106 vs. 0.5x106 ± 0.1x106 MPs/ml plasma (P<0.0001) (Figure 1C).
Cellular origin of MPs in PLWH
We analyzed subpopulations of MPs defined on the basis of their cellular origin, as determined by evaluating the expression of surface proteins specific to red blood cells (CD235a+ RBC MPs), platelets (CD41a+ PMPs), monocytes (CD14+ MMPs), neutrophils (CD10+ Neutro MPs), dendritic cells (CD11c+, CD123+ DC MPs), T lymphocytes (CD3+ TL MPs), B lymphocytes (CD19+ BL MPs) and endothelial cells (CD142+ Endo MPs) (Figures 1D–F).
The concentrations of MPs of all cell origins other than platelets or monocytes (PMPs or MMPs) were significantly higher in PLWH than in controls: for RBC MPs, 1.3x106 ± 0.1x106 vs. 0.4x106 ± 0.1x106 MPs/ml (P<0.0001); for DC MPs, 14.5x104 ± 1.9x104 vs. 4.7x104 ± 0.8x104 MPs/ml (P<0.0001); for Neutro MPs, 20.6x104 ± 4.9x104 vs. 5.8x104 ± 1.6x104 MPs/ml (P=0.0101); for TL MPs, 13.6x104 ± 1.7x104 vs. 3.6x104 ± 0.5x104 MPs/ml (P<0.0001); for BL MPs, 5.8x104 ± 0.9x104 vs. 2.2x104 ± 0.5x104 MPs/ml (P=0.0007); for Endo MPs, 4.7x104 ± 0.7x104 vs. 1.8x104 ± 0.3x104 MPs/ml (P=0.0008) (Figure 1E).
We also compared the frequencies of each subtype of MPs based on cell origin between PLWH and controls (Figure 1F). RBC MPs, PMPs and MMPs were each present at frequencies of more than 10% and together accounted for about 80% of the MPs studied in blood (Figure 1F). The frequencies of RBC MPs and TL MPs were significantly higher in PLWH than in controls (RBC MPs, 53.2% ± 2.4% vs. 40.7% ± 3.1%, P=0.004); TL MPs, 5.9% ± 0.6% vs. 3.8% ± 0.5%, P=0.034). However, the frequencies of MMPs and PMPs were significantly lower in PLWH than in controls (MMPs, 12.8% ± 1.4% vs. 22.8% ± 3.4%, P=0.008; PMPs, 10.6% ± 1.0% vs. 17.7% ± 2.3%, P=0.006) (Figure 1F). We found no correlation between cellular and MP phenotypes, either in PLWH or in the control group (data not shown).
Immunoregulatory profile of total MPs in PLWH
We characterized the immunoregulatory profile of total MPs from PLWH and controls. We performed flow cytometry to assess the membrane expression of 23 cellular activation and immune checkpoint markers potentially involved in chronic immune activation (PD1, ICOSL, OX40L, CD40L, LAG3, TGF-β1, PDL1, PDL2, HLA-ABC, CD86, DC-SIGN, CLEC2, CXCR4, CCR5, CD40, OX40, FASL, TIM3, HLA-DR, TRAIL, CTLA4, CD27 and CD39) (Supplementary Figures 1, 2, Figure 2).
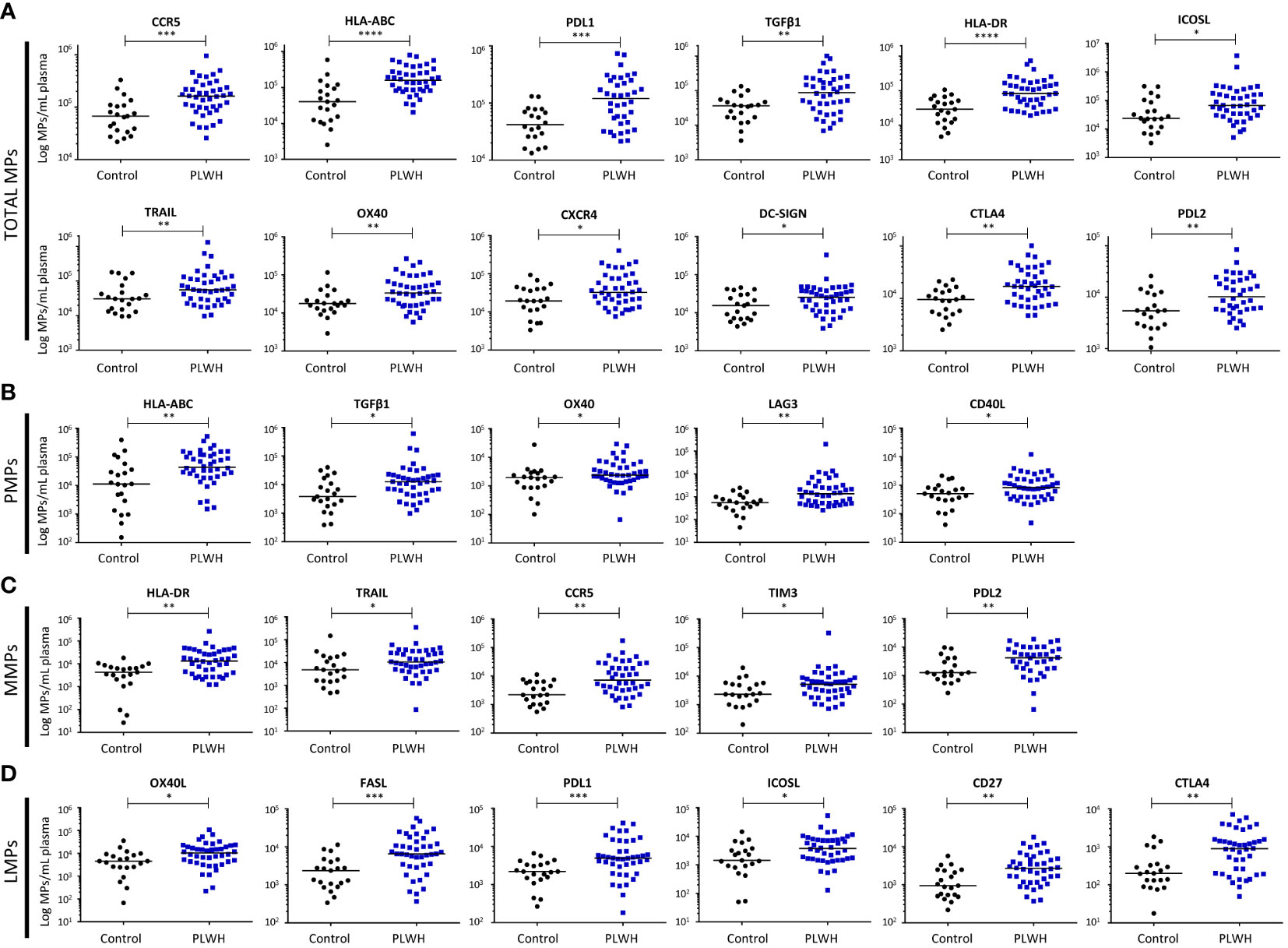
Figure 2 Immunoregulatory molecule phenotypes of MPs from PLWH and the control group. The expression of immunoregulatory markers on the surface of total MPs (A), CD41a+ PMPs (B), CD14+ MMPs (C) and CD3+ LMPs (D) was quantified by flow cytometry on fresh plasma samples from the control group (n = 21) and PLWH (n = 42) (21 experiments, with 2 patients and 1 control per experiment). The concentrations of MPs expressing each of the markers were determined with Trucount tubes. The immunoregulatory markers are arranged in descending order of the number of MPs/mL of plasma expressing them. Horizontal bars indicate the median values. P values (P<0.05 considered significant) were obtained in Mann-Whitney and post hoc tests. **** P<0.0001, *** P<0.001, ** P<0.01, * P<0.05.
We identified 12 immunoregulatory molecules among the markers tested that were more strongly expressed by the MPs of PLWH than by those of controls (Figure 2A). The markers on total MPs that did not differ significantly between groups are presented in Supplementary Figure 2.
For ligands of PD1, the differences between PLWH and the control group were as follows: for PDL1+ MPs, 1.7x105 ± 0.3x105 vs. 0.5x105 ± 0.1x105 MPs/ml, (P=0.0006), and for PDL2+ MPs, 1.6x104 ± 0.3x104 vs. 0.7x104 ± 0.1x104 MPs/ml (P=0.003).
For MHC I and II molecules, the differences in expression on MPs between PLWH and controls were as follows: for HLA-ABC+ MPs, 2.2x105 ± 0.3x105 vs. 0.8x105 ± 0.3x105 MPs/ml (P<0.0001), and for HLA-DR+ MPs, 1.3x105 ± 0.2x105 vs. 0.4x105 ± 0.1x105 MPs/ml (P<0.0001).
For the costimulation molecules ICOSL and OX40, the differences between PLWH and controls were as follows: for ICOSL+ MPs, 2.2x105 ± 0.9x105 vs. 0.7x105 ± 0.2x105 MPs/ml (P=0.016), and for OX40+ MPs, 5.5x104 ± 0.1x104 vs. 2.4x104 ± 0.1x104 MPs/ml (P=0.008).
For the other costimulation molecules considered, TGF-β1, TRAIL and CTLA4, the differences between PLWH controls were as follows: for TGF-β1+ MPs, 1.6x105 ± 0.3x105 vs. 0.4x105 ± 0.1x105 MPs/ml (P=0.002); for TRAIL+ MPs, 1.3x105 ± 0.3x105 vs. 0.5x105 ± 0.1x105 MPs/ml (P=0.0241), and for CTLA4+ MPs, 2.3x104 ± 0.3x104 vs. 1.0x104 ± 0.1x104 MPs/ml (P=0.002).
Chemokine receptors were also more strongly expressed on the MPs of PLWH than on those of controls: for CCR5+ MPs, 2.0x105 ± 0.3x105 vs. 0.9x105 ± 0.2x105 MPs/ml plasma (P=0.0003), and for CXCR4+ MPs, 6.3x104 ± 0.1x104 vs. 2.8x104 ± 0.5x104 MPs/ml plasma (P=0.039).
DC-SIGN was also more strongly expressed on the MPs from PLWH than on those of controls: 3.4x104 ± 0.8x104 vs. 1.9x104 ± 0.3x104 MPs/ml plasma (P=0.04).
Immunoregulatory profiles of PMPs, MMPs and LMPs in PLWH
We then evaluated the immunoregulatory profile of MPs according to their cell type of origin, focusing particularly on PMPs, MMPs and LMPs, which are derived from cell subsets known to be involved in immunoregulation (8–11, 13, 14).
CD41a+ PMPs
MPs from platelets can account for up to 32% of the MPs circulating in the blood of PLWH (Figure 1F) and are therefore a potentially important factor in innate and adaptive immunity. The immunoregulatory molecule expression profile of the PMPs of PLWH was characterized by flow cytometry based on 15 surface markers: HLA-ABC, TGFβ1, CXCR4, ICOSL, CLEC2, DC-SIGN, CD40, CD86, OX40, OX40L, CCR5, CD39, CD40L, LAG3, PD1 (Supplementary Figure 3, Figure 2B). We observed a significant overexpression of five immunoregulatory molecules on the PMPs of PLWH relative to controls: HLA-ABC, 8.6x104 ± 1.6x104 vs. 4.8x104 ± 2.0x104 MPs/ml (P=0.004); TGF-β1, 3.6x104 ± 1.5x104 vs. 0.9x104 ± 0.2x104 MPs/ml (P=0.014); OX40, 4.7x103 ± 0.9x103 vs. 3.0x103 ± 1.3x103 MPs/ml (P=0.04); CD40L, 1.4x103 ± 0.3x103 vs. 0.7x103 ± 0.1x103 MPs/ml (P=0.03) and LAG3, 7.4x103 ± 4.8x103 vs. 0.7x103 ± 0.1x103 MPs/ml (P=0.002) (Figure 2B). The markers on PMPs that did not differ significantly between groups are presented in Supplementary Figure 3.
CD14+ MMPs
MPs derived from monocytes can account for up to 38% of the circulating MPs in the blood of PLWH (Figure 1F) and may have effects on inflammation, oxidative stress and cell apoptosis. We performed flow cytometry to characterize the immunoregulatory molecule profiles of MMPs for 16 markers: PDL1, HLA-DR, TRAIL, OX40L, ICOSL, FASL, CD86, CCR5, TIM3, CXCR4, CD39, PDL2, TGFβ1, LAG3, CD40L, PD1 (Supplementary Figure 4, Figure 2C). Five immunoregulatory molecules were significantly overexpressed on MMPs from PLWH relative to MMPs from the control group: HLA-DR, 2.5x104 ± 0.7x104 vs. 0.5x104 ± 0.1x104 MPs/ml (P=0.001); TRAIL, 2.5x104 ± 0.8x104 vs. 1.5x104 ± 0.7x104 MPs/ml (P=0.022); CCR5, 1.8x104 ± 0.4x104 vs. 0.4x104 ± 0.1x104 MPs/ml (P=0.001); TIM3, 1.4x104 ± 0.8x104 vs. 0.4x104 ± 0.1x104 MPs/ml (P=0.044), and PDL2, 5.8x103 ± 0.8x103 vs. 2.5x103 ± 0.6x103 MPs/ml (P=0.005) (Figure 2C). The markers on MMPs that did not differ significantly between groups are presented in Supplementary Figure 4.
CD3+ LMPs
MPs derived from lymphocytes can account for up to 18% of the MPs circulating in the blood of PLWH (Figure 1F). The mean frequency of LMPs among total circulating MPs is only 6%, but even small numbers of LMPs can exert immunomodulatory effects, particularly on lymphocytes. The surface immunoregulatory molecule profile of the LMPs was characterized by flow cytometry with 12 markers: OX40L, CD39, ICOSL, FASL, PDL1, CD27, LAG3, TGFβ1, TRAIL, CD40L, PD1 and CTLA-4 (Supplementary Figure 5, Figure 2D). We found that six immunomodulatory molecules were more strongly expressed on LMPs from PLWH than on LMPs from controls: OX40L, 1.6x104 ± 0.3x104 vs. 0.7x104 ± 0.2x104 MPs/ml (P=0.01); ICOSL, 6.2x103 ± 1.3x103 vs. 2.9x103 ± 0.7x103 MPs/ml (P=0.01); FASL, 11.3x103 ± 1.9x103 vs. 3.0x103 ± 0.6x103 MPs/ml (P=0.0002); PDL1, 8.8x103 ± 1.6x103 vs. 2.5x103 ± 0.7x103 MPs/ml (P=0.0009); CD27, 3.9x103 ± 0.6x103 vs. 1.5x103 ± 0.3x103 MPs/ml (P=0.001), and CTLA4, 14.7x102 ± 2.6x102 vs. 4.0x102 ± 1.1x102 MPs/ml (P=0.001). (Figure 2D). The markers on LMPs that did not differ significantly between groups are presented in Supplementary Figure 5.
Finally, despite the identical activation profiles of PLWH and control MPs (Supplementary Figure 6), the number of immunomodulatory MPs was greater in PLWH than controls.
Discussion
We found that PLWH on ART had significantly larger numbers of MPs in their plasma than controls. This increase in MP numbers, which points to cellular activation, is potentially important because MPs can have various effects, depending on their phenotype and numbers (9–11). Many factors, including aging, can influence the production of MPs even in people without HIV (30). However, we observed no correlation between age and the number of MPs in either PLWH or controls (data not shown).
We found that the increase in the number of MPs in PLWH was not dependent on MP size, with all size classes, including the largest (900 nm), affected. This finding is important, because the largest MPs may contain organelles, such as mitochondria, which may participate in the production of anti-cardiolipin antibodies (12). These antibodies play an important role in anti-phospholipid antibody syndrome and are responsible for the development of a state of thrombophilia. Other MPs may contain proteasomes, which can contribute to antigen presentation through class I MHC (14).
Moreover, the phenotypic characteristics of MPs are key to identification of the cellular targets of MPs in the immune system. Indeed, the interactions of MPs with immune cells differ according to the cellular origin of the MPs and their immunoregulatory phenotype (9, 11). The interaction between MPs and immune cells is not random and involves molecules present on the surface of MPs (11). This interaction can result in a transfer of molecules from the MP to the immune cell, with immunomodulatory consequences. We show here that the increase in MP numbers in PLWH concerns not only MPs from red blood cells and endothelial cells, but also other MPs derived from immune system cells, such as neutrophils, dendritic cells and T and B lymphocytes. This increase cannot be explained by an increase in the number of cells because we have never observed any correlation between cellular expression and MPs, in either controls (9) or PLWH (data not shown). Furthermore, we have recently shown that the role of MPs depends on their quantity (9). We have shown that LMPs, even if present at only very low levels, can facilitate both cellular and humoral responses. These findings support the possible maintenance of an inflammatory endothelial environment, and immune activation through specific interactions of MPs with the immune system (11).
Only MPs derived from monocytes and platelets were present in similar numbers in both the PLWH and control groups. However, the phenotype of these MPs differed between PLWH and controls. Indeed, the analysis of immunomodulatory molecule expression on the surface of PMPs indicated that class I MHC molecules (HLA-ABC), TGFβ1, OX40 (CD134), LAG3 (CD223) and CD40L (CD154) were more strongly expressed on the PMPs of PLWH than on those of controls. These data confirm the state of chronic platelet activation described in PLWH patients (31) and also clearly suggest a role for these MPs in immune activation (9).
Differences in the immunomodulatory molecules expressed on the surface were also found for MMPs, but for the molecules concerned were different, with an overexpression of molecules capable of modulating immune activation, such as class II MHC (HLA-DR), TRAIL (CD253), TIM3 (CD366) and PDL2 (CD273) in PLWH relative to controls. The MMPs of PLWH also had higher levels of CCR5 expression on their surface. MMPs could, thus, play a key role in the spread of the virus and the maintenance of virus reservoirs (14, 18).
Similarly, LMPs from PLWH had significantly higher levels of expression for OX40L (CD252), ICOSL (CD275), FASL (CD178), PDL1 (CD274), CTLA4 (CD152) and CD27 than those from controls. These findings clearly suggest that these MPs could play a role in the transfer or maintenance of T-lymphocyte immune activation, as reported in our previous studies (11). Indeed, CD27+ MPs bind only to cells that already express CD27. This binding results partly from the co-expression of CD70 by these CD27+ MPs (11). These data testify to the ability of MPs to reprogram cells via marker transfer, thereby maintaining an immune phenotype.
We also observed much stronger DC-SIGN and CXCR4 expression on MPs from PLWH than on those from controls. The cellular origin of the MPs overexpressing these two markers remains unclear, but we suspect that they are derived from dendritic cells and/or B lymphocytes and can participate in the maintenance and dissemination of the virus. Furthermore, it has been known since the early 2000s that CXCR4 and CCR5 are present on MPs and can transfer a capacity for infection to cells lacking these receptors (18, 19). This ability of MPs to transfer markers suggests that DC-SIGN and CXCR4 MPs may be associated with the maintenance of a viral load in reservoirs.
Despite the careful application of selection criteria during the recruitment of participants (HIV infection, patient on treatment with subsequent immune reconstitution), phenotyping results for MPs were highly heterogeneous, particularly for PDL1, TGFβ1 and CTLA4. This variability suggests that comorbid conditions in these patients may drive the characteristic expression of immunomodulatory markers on the surface of MPs. Indeed, these MPs with particular phenotypes may be associated with comorbid conditions and chronic activation of the immune system.
Other chronic co-infections and the resulting activation of the immune system may also explain these higher levels of MPs, but we found no link between the number of MPs and chronic co-infection with HBV or HCV (data not shown).
Finally, we hypothesize that antiviral treatments may direct the production of MPs. We found no correlation between the frequency or phenotype of MPs and ART treatment (data not shown), but we believe that this lack of correlation may result from treatment heterogeneity, which might also account for the occurrence of comorbidities as a function of the MPs induced. The only information available to support this hypothesis comes from studies on the effects on RBCs of ART. Indeed, Peltenburg et al. have shown that abacavir treatment is associated with an increase in cardiovascular risk, whereas no such increase in risk is observed for tenofovir or didanosine (32, 33). However, it remains unclear whether this risk is associated with RBC-MPs. There are also indirect links, such as the impact on cholesterol and, hence, on MP survival (34), and the effect of nucleoside reverse transcriptase inhibitors on RBC (35). Dolutegravir is also directly associated with the apoptosis of RBCs (36) or other cell types (37), inflammation (38), and platelet activation correlated with an increase in the risk of cardiometabolic comorbidities (39). This last point links platelet activation and the production of PMPs (12).
The data obtained here are therefore of potential value for the development of new treatments for reducing the impact of these MPs, whether or not they are linked to infection. Indeed, new therapeutic or vaccination strategies based on the specific transfer of functions from MPs could be envisaged (11). However, as we recently showed with CD27+ MPs, it is important to take the contribution of immunoregulatory MPs into account, particularly in patients treated with immunotherapies based on monoclonal antibodies (11).
Data availability statement
The original contributions presented in the study are included in the article/Supplementary Material. Further inquiries can be directed to the corresponding author.
Ethics statement
The studies involving humans were approved by Comite de Protection des Personnes - Ile-de-France IX. The studies were conducted in accordance with the local legislation and institutional requirements. The participants provided their written informed consent to participate in this study.
Author contributions
DN-L: Formal analysis, Investigation, Writing – original draft. MT: Writing – review & editing. RB: Writing – review & editing. SM: Writing – review & editing, Investigation. PV: Writing – review & editing, Investigation. JL: Writing – review & editing, Investigation. AD: Writing – review & editing. MA: Writing – review & editing. EB: Writing – review & editing. FC: Writing – review & editing. HH-C: Writing – review & editing. SP: Writing – review & editing. J-DL: Writing – review & editing. FP: Writing – review & editing. SG: Writing – review & editing. BV: Conceptualization, Supervision, Validation, Writing – original draft.
Funding
The author(s) declare financial support was received for the research, authorship, and/or publication of this article. This work was supported by the ANRS, Etablissement Français du Sang, INSERM and Université Paris-Est Créteil.
Acknowledgments
We are particularly grateful to the healthy blood donors who participated in this study, and the EFS team responsible for collecting blood donations.
Conflict of interest
The authors declare that the research was conducted in the absence of any commercial or financial relationships that could be construed as a potential conflict of interest.
Publisher’s note
All claims expressed in this article are solely those of the authors and do not necessarily represent those of their affiliated organizations, or those of the publisher, the editors and the reviewers. Any product that may be evaluated in this article, or claim that may be made by its manufacturer, is not guaranteed or endorsed by the publisher.
Supplementary material
The Supplementary Material for this article can be found online at: https://www.frontiersin.org/articles/10.3389/fimmu.2024.1354065/full#supplementary-material
Abbreviations
PLWH, people living with HIV; ART, antiretroviral treatment; EV, extracellular vesicles; MPs, microvesicles; PMPs, platelet microvesicles; MMPs, monocyte microvesicles; LMPs, lymphocyte microvesicles; TL, T lymphocyte; BL, B lymphocyte; RBC, red blood cell; Neutro, neutrophil; DCs, dendritic cells; Endo, endothelial cells.
References
1. Appay V, Kelleher A. Immune activation and immune aging in HIV infection. Curr Opin HIV AIDS. (2016) 11:242–9. doi: 10.1097/COH.0000000000000240
2. Dias M, Costa C, daSilva L. The ambiguous roles of extracellular vesicles in HIV replication and pathogenesis. Front Microbiol. (2018) 9:2411. doi: 10.3389/fmicb.2018.02411
3. Colombo M, Raposo G, Théry C. Biogenesis, secretion, and intercellular interactions of exosomes and other extracellular vesicles. Annu Rev Cell Dev Biol. (2014) 30:255–89. doi: 10.1146/annurev-cellbio-101512-122326
4. van Niel G, D’Angelo G, Raposo G. Shedding light on the cell biology of extracellular vesicles. Nat Rev Mol Cell Biol. (2018) 19:213–28. doi: 10.1038/nrm.2017.125
5. Théry C, Ostrowski M, Segura E. Membrane vesicles as conveyors of immune responses. Nat Rev Immunol. (2009) 9:581–93. doi: 10.1038/nri2567
6. Dieudé M, Bell C, Turgeon J, Beillevaire D, Pomerleau L, Yang B, et al. The 20S proteasome core, active within apoptotic exosome-like vesicles, induces autoantibody production and accelerates rejection. Sci Trans Med. (2015) 7:200. doi: 10.1126/scitranslmed.aac9816
7. Boudreau L, Duchez A, Cloutier N, Soulet D, Martin N, Bollinger J, et al. Platelets release mitochondria serving as substrate for bactericidal group IIA-secreted phospholipase A2 to promote inflammation. Blood. (2014) 124:2173–83. doi: 10.1182/blood-2014-05-573543
8. Danesh A, Inglis H, Jackman R, Wu S, Deng X, Muench M, et al. Exosomes from red blood cell units bind to monocytes and induce proinflammatory cytokines, boosting T-cell responses in vitro. Blood. (2014) 123:687–96. doi: 10.1182/blood-2013-10-530469
9. Pinheiro M, Tamagne M, Elayeb R, Andrieu M, Pirenne F, Vingert B. Blood microparticles are a component of immune modulation in red blood cell transfusion. Eur J Immunol. (2020) 50:1237–40. doi: 10.1002/eji.201948481
10. Neyrinck-Leglantier D, Tamagne M, L’honoré S, Cagnet L, Pakdaman S, Marchand A, et al. Autologous blood extracellular vesicles and specific CD4+ T-cell co-activation. Front Immunol. (2022) 13:992483. doi: 10.3389/fimmu.2022.992483
11. Cagnet L, Neyrinck-Leglantier D, Tamagne M, Berradhia L, Khelfa M, Cleophax S, et al. CD27+ microparticle interactions and immunoregulation of CD4+ T lymphocytes. Front Immunol. (2023) 14:1043255. doi: 10.3389/fimmu.2023.1043255
12. Melki I, Tessandier N, Zufferey A, Boilard E. Platelet microvesicles in health and disease. Platelets. (2017) 28:214–21. doi: 10.1080/09537104.2016.1265924
13. Dinkla S, van Cranenbroek B, van der Heijden W, He X, Wallbrecher R, Dumitriu I, et al. Platelet microparticles inhibit IL-17 production by regulatory T cells through P-selectin. Blood. (2016) 127:1976–86. doi: 10.1182/blood-2015-04-640300
14. Marcoux G, Laroche A, Hasse S, Bellio M, Mbarik M, Tamagne M, et al. Platelet EVs contain an active proteasome involved in protein processing for antigen presentation via MHC-I molecules. Blood. (2021) 138:2607–20. doi: 10.1182/blood.2020009957
15. Sadallah S, Eken C, Martin P, Schifferli J. Microparticles (ectosomes) shed by stored human platelets downregulate macrophages and modify the development of dendritic cells. J Immunol. (2011) 186:6543–52. doi: 10.4049/jimmunol.1002788
16. Caobi A, Nair M, Raymond A. Extracellular vesicles in the pathogenesis of viral infections in humans. Viruses. (2020) 12:1200. doi: 10.3390/v12101200
17. Al Sharif S, Pinto D, Mensah G, Dehbandi F, Khatkar P, Kim Y, et al. Extracellular vesicles in HTLV-1 communication: the story of an invisible messenger. Viruses. (2020) 12:1422. doi: 10.3390/v12121422
18. Mack M, Kleinschmidt A, Brühl H, Klier C, Nelson P, Cihak J, et al. Transfer of the chemokine receptor CCR5 between cells by membrane-derived microparticles: a mechanism for cellular human immunodeficiency virus 1 infection. Nat Med. (2000) 6:769–75. doi: 10.1038/77498
19. Rozmyslowicz T, Majka M, Kijowski J, Murphy S, Conover D, Poncz M, et al. Platelet- and megakaryocyte-derived microparticles transfer CXCR4 receptor to CXCR4-null cells and make them susceptible to infection by X4-HIV. AIDS (London England). (2003) 17:33–42. doi: 10.1097/00002030-200301030-00006
20. Gasper-Smith N, Crossman D, Whitesides J, Mensali N, Ottinger J, Plonk S, et al. Induction of plasma (TRAIL), TNFR-2, Fas ligand, and plasma microparticles after human immunodeficiency virus type 1 (HIV-1) transmission: implications for HIV-1 vaccine design. J virology. (2008) 82:7700–10. doi: 10.1128/JVI.00605-08
21. Ruiz-de-León M, Jiménez-Sousa M, Moreno S, García M, Gutiérrez-Rivas M, León A, et al. Lower expression of plasma-derived exosome miR-21 levels in HIV-1 elite controllers with decreasing CD4 T cell count. J microbiology immunology infection = Wei mian yu gan ran za zhi. (2019) 52:667–71. doi: 10.1016/j.jmii.2018.07.007
22. Chettimada S, Lorenz D, Misra V, Wolinsky S, Gabuzda D. Small RNA sequencing of extracellular vesicles identifies circulating miRNAs related to inflammation and oxidative stress in HIV patients. BMC Immunol. (2020) 21:57. doi: 10.1186/s12865-020-00386-5
23. DeMarino C, Pleet M, Cowen M, Barclay R, Akpamagbo Y, Erickson J, et al. Antiretroviral drugs alter the content of extracellular vesicles from HIV-1-infected cells. Sci Rep. (2018) 8:7653. doi: 10.1038/s41598-018-25943-2
24. Mayne E, Funderburg N, Sieg S, Asaad R, Kalinowska M, Rodriguez B, et al. Increased platelet and microparticle activation in HIV infection: upregulation of P-selectin and tissue factor expression. J acquired Immune deficiency syndromes (1999). (2012) 59:340–6. doi: 10.1097/QAI.0b013e3182439355
25. Hijmans J, Stockelman K, Garcia V, Levy M, Brewster L, Bammert T, et al. Circulating microparticles are elevated in treated HIV -1 infection and are deleterious to endothelial cell function. J Am Heart Assoc. (2019) 8:e011134. doi: 10.1161/JAHA.118.011134
26. Marques de Menezes E, Deng X, Liu J, Bowler S, Shikuma C, Stone M, et al. Plasma CD16 + Extracellular vesicles associate with carotid artery intima-media thickness in HIV + Adults on combination antiretroviral therapy. mBio. (2022) 13. doi: 10.1128/mbio.03005-21
27. Sadallah S, Amicarella F, Eken C, Iezzi G, Schifferli J. Ectosomes released by platelets induce differentiation of CD4+T cells into T regulatory cells. Thromb haemostasis. (2014) 112:1219–29. doi: 10.1160/TH14-03-0281
28. Marques de Menezes E, Ramallho J, Bucovsky M, Shane E, Yin M, Norris P. Serum extracellular vesicles expressing bone activity markers associate with bone loss after HIV antiretroviral therapy. AIDS (London England). (2020) 34:351–61. doi: 10.1097/QAD.0000000000002430
29. Pannetier L, Tamagne M, Bocquet T, Pirenne F, Ansart-Pirenne H, Vingert B. HLA molecule expression on the surface of cells and microparticles in platelet concentrates. Transfusion. (2021) 61:1023–8. doi: 10.1111/trf.16201
30. Yin Y, Chen H, Wang Y, Zhang L, Wang X. Roles of extracellular vesicles in the aging microenvironment and age-related diseases. J extracellular vesicles. (2021) 10:e12154. doi: 10.1002/jev2.12154
31. Damien P, Cognasse F, Lucht F, Suy F, Pozzetto B, Garraud O, et al. Highly active antiretroviral therapy alters inflammation linked to platelet cytokines in HIV-1-infected patients. J Infect diseases. (2013) 208:868–70. doi: 10.1093/infdis/jit260
32. Peltenburg N, Bierau J, Bakker J, Schippers J, Lowe S, Paulussen A, et al. Erythrocyte Inosine triphosphatase activity: A potential biomarker for adverse events during combination antiretroviral therapy for HIV. PloS One. (2018) 13:e0191069. doi: 10.1371/journal.pone.0191069
33. Peltenburg N, Bierau J, Schippers J, Lowe S, Paulussen A, van den Bosch B, et al. Metabolic events in HIV-infected patients using abacavir are associated with erythrocyte inosine triphosphatase activity. J antimicrobial chemotherapy. (2019) 74:157–64. doi: 10.1093/jac/dky383
34. Latham V, Stebbing J, Mandalia S, Michailidis C, Davies E, Bower M, et al. Adherence to trizivir and tenofovir as a simplified salvage regimen is associated with suppression of viraemia and a decreased cholesterol. J antimicrobial chemotherapy. (2005) 56:186–9. doi: 10.1093/jac/dki170
35. Durand-Gasselin L, Da Silva D, Benech H, Pruvost A, Grassi J. Evidence and possible consequences of the phosphorylation of nucleoside reverse transcriptase inhibitors in human red blood cells. Antimicrobial Agents chemotherapy. (2007) 51:21055–2111. doi: 10.1128/AAC.00831-06
36. Al Mamun Bhuyan A, Signoretto E, Bissinger R, Lang F. Enhanced eryptosis following exposure to dolutegravir. Cell Physiol Biochem. (2016) 39:639–50. doi: 10.1159/000445655
37. Wang W, Mao L, Lai H, Wang Y, Jiang Z, Li W, et al. Dolutegravir derivative inhibits proliferation and induces apoptosis of non-small cell lung cancer cells via calcium signaling pathway. Pharmacol Res. (2020) 161:105129. doi: 10.1016/j.phrs.2020.105129
38. Theron A, Anderson R, Madzime M, Rossouw T, Steel H, Meyer P, et al. Pro-inflammatory interactions of dolutegravir with human neutrophils in an in vitro study. Molecules (Basel Switzerland). (2022) 27:9057. doi: 10.3390/molecules27249057
Keywords: immune activation (IA), extracellular vesicle (EV), people living with HIV (PLWH), microparticles (MPs), immunoregulatory molecules, chronic immune activation
Citation: Neyrinck-Leglantier D, Tamagne M, Ben Rayana R, Many S, Vingert P, LeGagneux J, Delorme AS, Andrieu M, Boilard E, Cognasse F, Hamzeh-Cognasse H, Perez-Patrigeon S, Lelievre J-D, Pirenne F, Gallien S and Vingert B (2024) Immunoregulatory molecule expression on extracellular microvesicles in people living with HIV. Front. Immunol. 15:1354065. doi: 10.3389/fimmu.2024.1354065
Received: 11 December 2023; Accepted: 20 February 2024;
Published: 04 March 2024.
Edited by:
Mario Clerici, University of Milan, ItalyReviewed by:
Caroline Subra, Henry M. Jackson Foundation for the Advancement of Military Medicine (HJF), United StatesAikaterini Alexaki, Centre Hospitalier Universitaire Vaudois (CHUV), Switzerland
Copyright © 2024 Neyrinck-Leglantier, Tamagne, Ben Rayana, Many, Vingert, LeGagneux, Delorme, Andrieu, Boilard, Cognasse, Hamzeh-Cognasse, Perez-Patrigeon, Lelievre, Pirenne, Gallien and Vingert. This is an open-access article distributed under the terms of the Creative Commons Attribution License (CC BY). The use, distribution or reproduction in other forums is permitted, provided the original author(s) and the copyright owner(s) are credited and that the original publication in this journal is cited, in accordance with accepted academic practice. No use, distribution or reproduction is permitted which does not comply with these terms.
*Correspondence: Benoît Vingert, YmVub2l0LnZpbmdlcnRAaW5zZXJtLmZy