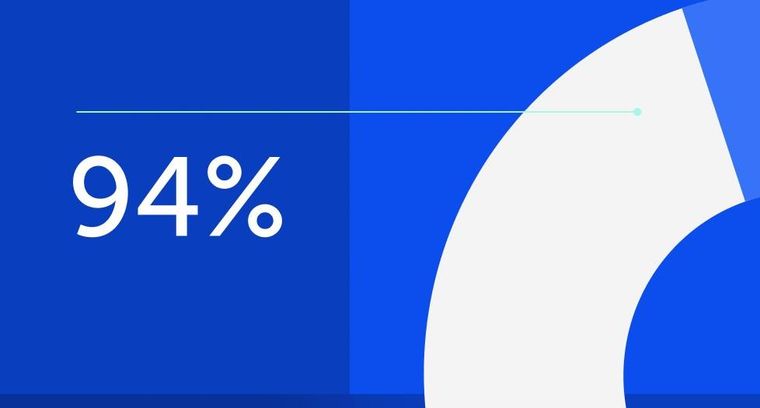
94% of researchers rate our articles as excellent or good
Learn more about the work of our research integrity team to safeguard the quality of each article we publish.
Find out more
MINI REVIEW article
Front. Immunol., 27 February 2024
Sec. Alloimmunity and Transplantation
Volume 15 - 2024 | https://doi.org/10.3389/fimmu.2024.1351717
This article is part of the Research TopicCommunity series in Progress of Allo- and Xeno-transplantation Facilitating the Initial Xeno-Kidney and Islet Clinical Trials, volume IIView all 12 articles
Combined islet and kidney xenotransplantation for the treatment of diabetic nephropathy represents a compelling and increasingly relevant therapeutic possibility for an ever-growing number of patients who would benefit from both durable renal replacement and cure of the underlying cause of their renal insufficiency: diabetes. Here we briefly review immune barriers to islet transplantation, highlight preclinical progress in the field, and summarize our experience with combined islet and kidney xenotransplantation, including both challenges with islet-kidney composite grafts as well as our recent success with sequential kidney followed by islet xenotransplantation in a pig-to-baboon model.
Diabetes is a leading cause of both cardiovascular disease and end stage renal disease (ESRD), and incidence is increasing across the country and across the globe (1). Human islet transplantation is an effective treatment for diabetic patients but requires lifelong immunosuppression: prospective islet transplant recipients must weigh the risks of immunosuppression against the short- and long-term complications of diabetes. Patients with diabetic nephropathy represent a unique – and growing – population that would benefit from both islet and kidney transplantation. Indeed, the favorable risk-to-benefit considerations of combined islet and kidney transplantation in this population inspired recent promising clinical studies in islet after kidney transplantation led by the Clinical Islet Transplantation (CIT) Consortium (2). However, at present these procedures are rare, due, in part, to a shortage of deceased donor organs (3). Xenotransplantation using organs derived from pigs may overcome this organ shortage and allow for broader application of combined islet and kidney transplantation.
The past several years have seen enormous progress in the field of xenotransplantation, with advances in gene-editing and immunosuppression leading to long-term survivals of both kidney and heart xenografts in pig-to-nonhuman primate (NHP) studies (4–7), as well as early studies (preclinical and clinical) in humans (8–10). Clinical translation of porcine islet transplantation predated these recent successes in solid organ xenotransplantation, with encouraging pig-to-NHP studies leading to several small clinical studies using porcine islets in humans (11–21). However, results of these early studies in clinical islet xenotransplantation have been mixed. While these differences between outcomes of preclinical and clinical xenogeneic islet transplantation may be partly explained by differences in the immunosuppression regimens used in the clinical trials – notably, CD40/CD40L costimulatory blockade, which has been critical to success in most preclinical studies, was not utilized – further trials have been limited by more recent consensus guidelines outlining an international framework to promote standardized clinical translation of pig-to-human islet transplantation from source pig development and manufacturing to patient monitoring (22).
Moreover, in the many years since these clinical studies in islet xenotransplantation were conducted, the landscape of diabetes management has changed. Patients with diabetes have other options for durable disease management. Innovations in glucose monitoring and the rapid development of hybrid closed-loop insulin delivery systems have improved quality of life for patients living with diabetes (23), and ongoing clinical trials of novel stem-cell derived islet cell therapy have published early and highly promising results (24). However, porcine islet xenotransplantation remains a compelling therapeutic possibility for patients with diabetic nephropathy who need both kidney and islet replacement. In these patients, there are minimal added risks associated with islet transplantation, as these patients are already on immunosuppression for their kidney grafts; in fact, islets may help protect against premature kidney graft loss associated with diabetes (25) as well as improve long-term vascular diabetic outcomes (26). Here, we will briefly highlight immunologic barriers in porcine islet transplantation, chronicle preclinical progress in the field, and summarize our own experience in combined islet and kidney transplantation, using both 1) vascularized islets in an islet-kidney composite graft, and 2) our more recent strategy of sequential kidney followed by islet xenotransplantation.
Porcine xenografts, including pig islets, elicit robust immune responses in humans. These responses involve both innate barriers (27) – including preformed natural antibodies (Nabs) and species incompatibilities in complement and coagulation systems leading to dysregulation – and adaptive immune components (reviewed in (28)). As with transplantation of solid organs, humoral immunity remains a key obstacle to long-term xenograft survival, and T cell-targeted immunosuppression strategies have been critical for prolonging islet survival (20, 29),. Unlike transplantation of other organs, however, transplanted islets also trigger an immediate inflammatory response, known as instant blood-mediated inflammatory reaction (IBMIR) (30, 31), related to expression of tissue factor on islets and leading to activation of innate responses that subsequently consume islets (32, 33). Although IBMIR is seen in auto- and allogeneic islet transplantation, greater immune barriers in xenotransplantation may lead to more pronounced islet losses (34, 35) as high as 70% in some studies (36).
Various strategies have been developed to overcome these short- and long-term immunologic hurdles, including islet encapsulation and source pig genetic modifications – both of which are intended to reduce the immunogenicity of the porcine islets.
Broadly, islet encapsulation technologies include microencapsulation of islets in alginate matrix, and macro-encapsulation of immobilized islets in bi-layered PTFE with a common oxygenation chamber (37). This microencapsulation technique successfully reversed diabetes for up to six months in preclinical studies of rhesus macaques (38), and was subsequently used in two nationally regulated clinical studies of porcine islet xenotransplantation in New Zealand and Argentina. Follow-up studies confirmed modest clinical benefit including reduction in HbA1c, hospitalization, and severe hypoglycemic and/or hyperglycemic events (21, 39) The key advantage of these technologies is that encapsulation may protect islets from the recipient immune system and obviate the need for immunosuppression; whereas islet transplantation alone is currently reserved for patients with hypoglycemic unawareness due to the morbidity of immunosuppression, transplantation of encapsulated islets without immunosuppression may tilt the risk-benefit ratio in favor of islet transplantation for cure of diabetes. Still, important hurdles remain in broader clinical application of this technology including variable recipient immune responses to the encapsulation material, which may lead to fibrosis of encapsulated grafts.
Source pig genetic modification is another strategy to overcome innate immune barriers and can be divided into two major categories: elimination of carbohydrate antigens that are targets of preformed antibodies, and correction of species incompatibilities. In solid organ xenotransplantation, preformed antibody binding leads to hyperacute rejection of graft; in free islet xenotransplantation, preformed antibody binding leads to an amplified IBMIR with islet loss (40). While elimination of targets of preformed Nabs (particularly elimination of α-gal with creation of α-1,3 Galactosyl transferase gene knockout or GalTKO source pigs) has been essential for successful pig-to-NHP heart and kidney xenotransplantation (4, 41, 42), the impact of using GalTKO source pigs on xenograft survival in islet transplantation is less conclusive, which may be a function of changes in α-gal expression with islet maturation (43–45). Similarly, correcting for species incompatibilities between porcine and primate complement regulatory systems through individual insertion of human complement regulatory proteins may not significantly reduce the incidence of IBMIR (44). However, combining carbohydrate antigen gene knockouts with complement regulatory transgenes proves additive: xenogeneic islets from GalTKO.hCD55.hCD59 and GalTKO.hCD39.hCD46 source pigs demonstrated reduced islet loss and attenuated IBMIR (15, 16). More recently, islets derived from neonatal GalTKO.hCD55.hCD59 source pigs demonstrated cure of diabetes with >1 year of insulin independence in the stringent pig-to-baboon model (46).
Marked improvements in diabetes management and emerging therapies have changed the risk-benefit calculus associated with islet transplantation more broadly, and porcine islet xenotransplantation in particular. As described in the preceding section, encapsulation technologies – which may allow for durable glucose control without immunosuppression – remain one relevant application for porcine islet xenotransplantation. Another relevant strategy is combining porcine islet xenotransplantation with solid organ xenotransplantation. This strategy has already been employed with success by the CIT consortium treating diabetic nephropathy with islet transplantation after kidney transplantation, but broader application is limited by the shortage of deceased donor organs. The following sections detail our preclinical experience with combined islet and kidney transplantation, including both composite islet-kidney transplantation as well as kidney-first sequential islet and kidney xenotransplantation.
As detailed above, xenogeneic islets are susceptible to destruction by both innate and adaptive mechanisms. The senior author of this review has demonstrated that transplanting pre-vascularized islets as part of a composite organ protects islets from innate immune destruction by circumventing the typical pathway that triggers IBMIR (47–49). We have previously reported successful preparation of composite islet-kidney (I-K) grafts which maintained normoglycemia and normal renal function after transplantation in pig-to-pig and nonhuman primate allogeneic transplantation models (Figure 1). Islets are isolated and pre-vascularized under autologous renal capsule, with subsequent transplantation of composite I-K graft (50). Preclinical allotransplantation studies in both pigs and in NHPs have demonstrated that this procedure preserves islets, likely by limiting innate immune destruction: diabetes is cured in animals who undergo composite I-K transplantation, while animals who undergo conventional free islet injection with the same islet equivalents (IEQs) remain insulin dependent (51, 52). Additional preclinical studies have demonstrated further improvements in islet yield and function with islet protective strategies including siRNA silencing of apoptotic genes (53). However, practical challenges have limited successful translation of this composite organ strategy for cure of diabetes and kidney failure in pig-to-NHP transplantation.
Figure 1 Schematic diagrams of preparation of a composite islet-kidney graft in donor and allogeneic composite islet-kidney transplantation in a recipient.
While I-K composite organs are ideally created in the same animal with autologous pig islets transplanted under autologous renal capsule, size constraints in our NHP recipient prevent successful I-K transplantation using a single source pig: a small (<30kg) source pig is needed for successful pig-to-NHP kidney transplantation, while a large (>60kg) source pig is needed in order to obtain sufficient islets for reversal of diabetes. This is a limitation primarily in our preclinical pig-to-NHP model, as larger kidneys from size matched >60kg pigs will likely be appropriate for human adult recipients. Nevertheless, overcoming this experimental constraint is critical to demonstration of composite I-K success. Our own attempts to isolate islets from juvenile source pig pancreases recapitulated the work of other investigators, confirming low islet-equivalent yield from juvenile pigs (54, 55). Accordingly, we elected to use two different source pigs for composite organ creation: large pigs would be used for islets, pre-vascularized prior to transplantation under the renal capsule of a smaller pig.
Using different source pigs for I-K composite organ creation introduced other challenges. Allogeneic islets from one source pig transplanted under the renal capsule of another source pig are vulnerable to recipient source pig immune responses, as with any allogeneic transplant. Strategies to mitigate these responses include 1) use of related pairs (cloned, inbred, or MHC-matched), and 2) minimization of the islet pre-vascularization period under allogeneic renal capsule.
Although the use of genetically identical (cloned) pairs for islet-kidney creation would be ideal, previous experiments have demonstrated long-term survival of skin and heart grafts without immunosuppression in highly inbred swine (56). Indeed, inbred animals, defined by co-ancestry >0.9, accepted allogeneic skin grafts for >340 days and accepted allogeneic heart grafts for >265 days without immunosuppression. MHC-matched pairs (co-ancestry >0.75) also allowed for acceptance of kidney grafts without immunosuppression, although hearts and islets were not accepted (50, 56, 57). Over the last three years, we have optimized composite I-K preparation using MHC-matched source pigs. As opposed to autologous I-K preparation, successful allogeneic preparation requires immunosuppression with both high dose tacrolimus and MMF. I-K preparation may be further optimized with reduction in pre-vascularization period from 6 weeks to 2 weeks. Still, it remains unclear whether I-K preparation in MHC-matched pairs preserves sufficient islets for reversal of diabetes in a xenogeneic recipient. We plan to revisit the composite I-K strategy in xenotransplantation when cloned pigs or highly inbred GalTKO pigs are available for these experiments.
Definitive evaluation of composite I-K transplantation in a pig-to-NHP model also requires a control: independent kidney and free islet transplantation. Negative controls were present in previous studies of composite IK technologies – in pig-to-pig, baboon-to-baboon, and macaque-to-macaque models – and demonstrated preservation of islets with composite I-K transplantation across allogeneic barriers. In the past year, due to lack of inbred or cloned source pigs, we tested an alternative strategy for combined islet and kidney transplantation across a xenogeneic barrier that would also serve as a control of the composite I-K strategy. This alternative approach involves delayed islet transplantation after kidney and vascularized thymus transplantation (role of vascularized thymus transplantation in the induction of tolerance across xenogeneic barriers reviewed in (28)), using a recipient size-matched kidney and thymus source pig, as well as a large source pig for islets. Notably this approach (without thymus co-transplantation) is also similar to recent work in human islet-after-kidney transplantation conducted by the CIT consortium. Although additional cases are required, we have achieved reversal of diabetes and life-supporting renal function for 180 days with this kidney-first sequential islet and kidney xenotransplantation (52). To our knowledge, this is the first demonstration of maintenance of durable normoglycemia and stable creatinine with porcine kidney and islets in a diabetic and life-supporting pig-to-baboon combined kidney, vascularized thymus and islet xenotransplantation model. These preliminary results were recently presented at the International Xenotransplantation Association Congress (San Diego, 2023), and are described in detail in the following sections:
In this experiment, we used two GalTKO.hCD55 source pigs from the National Swine Resource and Research Center (Sus scrofa domesticus, source: University of Missouri-Columbia, Columbia, MO) and one baboon recipient from the National Research and Resources Program (MD Anderson, Houston TX). Baboon recipient underwent B and T cell depletion with rituximab and rabbit anti-thymocyte globulin, followed by maintenance immunosuppression with anti-CD40 mAb (Nonhuman Primate Reagent Resource, University of Massachusetts Medical School, Worcester, MA). The baboon received kidney and vascularized thymic lobe grafts from a GalTKO.hCD55 (11.7kg) source pig on POD 0 with bilateral native nephrectomy. Diabetes was subsequently induced with streptozosin (STZ. 100mg/kg on POD 5, 50mg/kg on POD 9). After confirmation of diabetes, baboon underwent free islet transplantation into the portal vein, with islets isolated from unrelated GalTKO.hCD55 (95kg) source pig. Islet isolation was performed as previously described (58) and yielded 101K islet equivalents (IEQs) and 194K islet particle number (IPN). All animals were used in compliance with guidelines provided by the Animal Care and Use Committee at The Johns Hopkins University School of Medicine.
Both renal insufficiency and diabetes were cured with kidney-first sequential islet and kidney xenotransplantation. The baboon recipient maintained normal serum creatinine with no evidence of rejection for six months following kidney and islet transplant but was euthanized due to sepsis related to pyelonephritis in setting of stent occlusion on POD180. Immediately after islet transplantation, hyperglycemia was reversed with normalization of blood sugars from >250mg/dL (pre-transplant) to 80-110 mg/dL. Porcine islets functioned and maintained normal BG levels without any exogenous insulin treatment throughout the recipient’s postoperative course. Post-mortem evaluation of liver confirmed presence of insulin-staining islets.
As referenced above (see Preclinical progress in porcine islet xenotransplantation), investigators have recently achieved cure of diabetes in baboons using pooled islets from neonatal genetically modified pig donors (46). However, this required an average of 14 neonatal pancreases (70 piglets for 5 baboon recipients). In our model, we have achieved normoglycemia using islets derived from a single source adult pig with an administered islet mass of 12,500 IEQ/kg. Of note, this is within range though slightly less than was required in the recent clinical islet-after-kidney transplantation studies where successful islet transplants averaged >16,000 IEQ/kg (2).
One reason for the success of free islet transplantation in this model may be timing of sequential transplants: kidney-first transplantation promotes absorption of anti-pig antibodies, likely reducing IBMIR following islet transplantation, corresponding to reduced loss of islets. This may have enabled durable reversal of diabetes with fewer islet equivalents as compared with clinical islet-after-kidney transplantation. Indeed, the possible antibody absorption benefits of sequential transplant timing is less clear in the clinical islet-after-kidney studies, where islet transplantation occurred well after index kidney transplantation.
Lastly, adjunctive immunomodulatory strategies may also have played a role in the durable xenograft survival in this case. This animal received vascularized thymic lobe (VTL) graft co-transplantation from the kidney source pig, which has been shown to prolong xenograft survival in pig-to-baboon renal xenotransplantation (reviewed in (28)). Interferon gamma (IFN-γ) enzyme-linked immunosorbent spot (ELISpot) assay was performed to assess the potential immunomodulatory effect of VTL co-transplantation in this case. ELISpot assay at POD 180 demonstrated pig-specific unresponsiveness, suggesting that co-transplanted VTL graft may promote immunomodulatory effects. Further studies will clarify the mechanisms of in vitro unresponsiveness (59).
Additional cases are needed to replicate this work, but these encouraging results indicate that our negative control strategy, sequential kidney followed by islet xenotransplantation may reverse diabetes and renal insufficiency.
Porcine islet xenotransplantation is one promising strategy for cure of diabetes among an evolving landscape of emerging therapies in diabetes management. While islet-alone xenotransplantation strategies continue to show improvement with source pig genetic modifications and refinements to immunosuppression regimens, approaches like encapsulation which allow for reversal of diabetes without immunosuppression may be more clinically relevant. Porcine islet xenotransplantation, in conjunction with kidney xenotransplantation, remains a particularly compelling therapeutic possibility for patients with diabetic nephropathy who require both kidney and islet replacement, and who have already committed to immunosuppression for their kidney grafts. Composite islet-kidney transplantation has proven challenging in xenogeneic preclinical models; however, preliminary studies in islet-after-kidney xenotransplantation are promising and may point to a path forward with combined islet and kidney transplantation for diabetic nephropathy.
DE: Conceptualization, Formal Analysis, Investigation, Methodology, Writing – original draft. HI: Data curation, Formal Analysis, Investigation, Writing – review & editing. WLC: Formal Analysis, Methodology, Writing – review & editing. YH: Formal Analysis, Investigation, Writing – review & editing. WXC: Methodology, Writing – review & editing. MS: Formal Analysis, Investigation, Writing – review & editing. AS: Investigation, Writing – review & editing. DG: Methodology, Writing – review & editing. AM: Investigation, Writing – review & editing. KK: Methodology, Project administration, Writing – review & editing. ZS: Methodology, Supervision, Writing – review & editing. DW: Investigation, Methodology, Project administration, Writing – review & editing. KY: Conceptualization, Investigation, Methodology, Project administration, Supervision, Writing – review & editing.
The author(s) declare that no financial support was received for the research, authorship, and/or publication of this article.
The authors declare that the research was conducted in the absence of any commercial or financial relationships that could be construed as a potential conflict of interest.
All claims expressed in this article are solely those of the authors and do not necessarily represent those of their affiliated organizations, or those of the publisher, the editors and the reviewers. Any product that may be evaluated in this article, or claim that may be made by its manufacturer, is not guaranteed or endorsed by the publisher.
ELISpot, enzyme-linked immunosorbent spot assay; GalTKO, α-1,3 Galactosyl transferase gene knockout; hCD55, human CD55; hCD59, human CD59; hCD47, human CD47; IBMIR, instant blood-mediated inflammatory reaction; I-K, islet-kidney; IEQ, islet equivalents; IFN-γ, interferon gamma; IPN, islet particle number; mAb, monoclonal antibody; Nab, natural antibody; NHP, non-human primate; NK, natural killer; PTFE, PolyTetraFluoroEthylene; VTL, vascularized thymic lobe.
1. Divers J, Mayer-Davis EJ, Lawrence JM, Isom S, Dabelea D, Dolan L, et al. Trends in incidence of type 1 and type 2 diabetes among youths - selected counties and Indian reservations, United States, 2002-2015. MMWR Morb Mortal Wkly Rep. (2020) 69:161–5. doi: 10.15585/mmwr.mm6906a3
2. Markmann JF, Rickels MR, Eggerman TL, Bridges ND, Lafontant DE, Qidwai J, et al. Phase 3 trial of human islet-after-kidney transplantation in type 1 diabetes. Am J Transplant. (2021) 21:1477–92. doi: 10.1111/ajt.16174
3. Gamble A, Pepper AR, Bruni A, Shapiro AMJ. The journey of islet cell transplantation and future development. Islets. (2018) 10:80–94. doi: 10.1080/19382014.2018.1428511
4. Rivard CJ, Tanabe T, Lanaspa MA, Watanabe H, Nomura S, Andres-Hernando A, et al. Upregulation of CD80 on glomerular podocytes plays an important role in development of proteinuria following pig-to-baboon xeno-renal transplantation - an experimental study. Transpl Int. (2018) 31:1164–77. doi: 10.1111/tri.13273
5. Adams AB, Lovasik BP, Faber DA, Burlak C, Breeden C, Estrada JL, et al. Anti-C5 antibody tesidolumab reduces early antibody-mediated rejection and prolongs survival in renal xenotransplantation. Ann Surg. (2021) 274:473–80. doi: 10.1097/SLA.0000000000004996
6. Ma D, Hirose T, Lassiter G, Sasaki H, Rosales I, Coe TM, et al. Kidney transplantation from triple-knockout pigs expressing multiple human proteins in cynomolgus macaques. Am J Transplant. (2022) 22:46–57. doi: 10.1111/ajt.16780
7. Mohiuddin MM, Goerlich CE, Singh AK, Zhang T, Tatarov I, Lewis B, et al. Progressive genetic modifications of porcine cardiac xenografts extend survival to 9 months. Xenotransplantation. (2022) 29:e12744. doi: 10.1111/xen.12744
8. Griffith BP, Goerlich CE, Singh AK, Rothblatt M, Lau CL, Shah A, et al. Genetically modified porcine-to-human cardiac xenotransplantation. N Engl J Med. (2022) 387:35–44. doi: 10.1056/NEJMoa2201422
9. Montgomery RA, Stern JM, Lonze BE, Tatapudi VS, Mangiola M, Wu M, et al. Results of two cases of pig-to-human kidney xenotransplantation. N Engl J Med. (2022) 386:1889–98. doi: 10.1056/NEJMoa2120238
10. Locke JE, Kumar V, Anderson D, Porrett PM. Normal graft function after pig-to-human kidney xenotransplant. JAMA Surg. (2023). doi: 10.1001/jamasurg.2023.2774
11. Groth CG, Korsgren O, Tibell A, Tollemar J, Moller E, Bolinder J, et al. Transplantation of porcine fetal pancreas to diabetic patients. Lancet. (1994) 344:1402–4. doi: 10.1016/s0140-6736(94)90570-3
12. Valdes-Gonzalez R, Rodriguez-Ventura AL, White DJ, Bracho-Blanchet E, Castillo A, Ramirez-Gonzalez B, et al. Long-term follow-up of patients with type 1 diabetes transplanted with neonatal pig islets. Clin Exp Immunol. (2010) 162:537–42. doi: 10.1111/j.1365-2249.2010.04273.x
13. Valdes-Gonzalez RA, Dorantes LM, Garibay GN, Bracho-Blanchet E, Mendez AJ, Davila-Perez R, et al. Xenotransplantation of porcine neonatal islets of Langerhans and Sertoli cells: a 4-year study. Eur J Endocrinol. (2005) 153:419–27. doi: 10.1530/eje.1.01982
14. Rood PP, Bottino R, Balamurugan AN, Smetanka C, Ayares D, Groth CG, et al. Reduction of early graft loss after intraportal porcine islet transplantation in monkeys. Transplantation. (2007) 83:202–10. doi: 10.1097/01.tp.0000250680.36942.c6
15. Bottino R, Wijkstrom M, Windt der van DJ, Hara H, Ezzelarab M, Murase N, et al. Pig-to-monkey islet xenotransplantation using multi-transgenic pigs. Am J Transplant. (2014) 14:2275–87. doi: 10.1111/ajt.12868
16. Hawthorne WJ, Salvaris EJ, Phillips P, Hawkes J, Liuwantara D, Burns H, et al. Control of IBMIR in neonatal porcine islet xenotransplantation in baboons. Am J Transplant. (2014) 14:1300–9. doi: 10.1111/ajt.12722
17. Shin JS, Kim JM, Min BH, Yoon IH, Kim HJ, Kim JS, et al. Pre-clinical results in pig-to-non-human primate islet xenotransplantation using anti-CD40 antibody (2C10R4)-based immunosuppression. Xenotransplantation. (2018) 25. doi: 10.1111/xen.12356
18. Min BH, Shin JS, Kim JM, Kang SJ, Kim HJ, Yoon IH, et al. Delayed revascularization of islets after transplantation by IL-6 blockade in pig to non-human primate islet xenotransplantation model. Xenotransplantation. (2018) 25. doi: 10.1111/xen.12374
19. Cardona K, Korbutt GS, Milas Z, Lyon J, Cano J, Jiang W, et al. Long-term survival of neonatal porcine islets in nonhuman primates by targeting costimulation pathways. Nat Med. (2006) 12:304–6. doi: 10.1038/nm1375
20. Hering BJ, Wijkstrom M, Graham ML, Hardstedt M, Aasheim TC, Jie T, et al. Prolonged diabetes reversal after intraportal xenotransplantation of wild-type porcine islets in immunosuppressed nonhuman primates. Nat Med. (2006) 12:301–3. doi: 10.1038/nm1369
21. Matsumoto S, Abalovich A, Wechsler C, Wynyard S, Elliott RB. Clinical benefit of islet xenotransplantation for the treatment of type 1 diabetes. EBioMedicine. (2016) 12:255–62. doi: 10.1016/j.ebiom.2016.08.034
22. Hering BJ, Wijkstrom M, Graham ML, Hardstedt M, Aasheim TC, Jie T, et al. First update of the International Xenotransplantation Association consensus statement on conditions for undertaking clinical trials of porcine islet products in type 1 diabetes–Executive summary. Xenotransplantation. (2016) 23:3–13. doi: 10.1111/xen.12231
23. Templer S. Closed-loop insulin delivery systems: past, present, and future directions. Front Endocrinol (Lausanne). (2022) 13:919942. doi: 10.3389/fendo.2022.919942
24. Reichman TW, Ricordi C, Naji A, Markmann JF, Perkins BA, Wijkstrom M, et al. 836-P: glucose-dependent insulin production and insulin-independence in type 1 diabetes from stem cell–derived, fully differentiated islet cells—Updated data from the VX-880 clinical trial. Diabetes. (2023) 72. doi: 10.2337/db23-836-P
25. Larkins NG, Wong G, Johnson DW, Hawley C, Teixeira-Pinto A, Pleass H, et al. Early graft loss following transplantation from expanded criteria donors. Transplant Direct. (2021) 7:e783. doi: 10.1097/TXD.0000000000001235
26. Fiorina P, Folli F, Maffi P, Placidi C, Venturini M, Finzi G, et al. Islet transplantation improves vascular diabetic complications in patients with diabetes who underwent kidney transplantation: a comparison between kidney-pancreas and kidney-alone transplantation. Transplantation. (2003) 75:1296–301. doi: 10.1097/01.TP.0000061788.32639.D9
27. Mok D, Black M, Gupta N, Arefanian H, Tredget E, Rayat GR. Early immune mechanisms of neonatal porcine islet xenograft rejection. Xenotransplantation. (2019) 26:e12546. doi: 10.1111/xen.12546
28. Eisenson DL, Hisadome Y, Yamada K. Progress in xenotransplantation: immunologic barriers, advances in gene editing, and successful tolerance induction strategies in pig-to-primate transplantation. Front Immunol. (2022) 13:899657. doi: 10.3389/fimmu.2022.899657
29. Vadori M, Cozzi E. The immunological barriers to xenotransplantation. Tissue Antigens. (2015) 86:239–53. doi: 10.1111/tan.12669
30. Naziruddin B, Iwahashi S, Kanak MA, Takita M, Itoh T, Levy MF. Evidence for instant blood-mediated inflammatory reaction in clinical autologous islet transplantation. Am J Transplant. (2014) 14:428–37. doi: 10.1111/ajt.12558
31. Nilsson B, Ekdahl KN, Korsgren O. Control of instant blood-mediated inflammatory reaction to improve islets of Langerhans engraftment. Curr Opin Organ Transplant. (2011) 16:620–6. doi: 10.1097/MOT.0b013e32834c2393
32. Ji M, Yi S, Smith-Hurst H, Phillips P, Wu J, Hawthorne W, et al. The importance of tissue factor expression by porcine NICC in triggering IBMIR in the xenograft setting. Transplantation. (2011) 91:841–6. doi: 10.1097/TP.0b013e3182106091
33. Kourtzelis I, Magnusson PU, Kotlabova K, Lambris JD, Chavakis T. Regulation of instant blood mediated inflammatory reaction (IBMIR) in pancreatic islet xeno-transplantation: points for therapeutic interventions. Adv Exp Med Biol. (2015) 865:171–88. doi: 10.1007/978-3-319-18603-0_11
34. Bennet W, Sundberg B, Lundgren T, Tibell A, Groth CG, Richards A, et al. Damage to porcine islets of Langerhans after exposure to human blood in vitro, or after intraportal transplantation to cynomologus monkeys: protective effects of sCR1 and heparin. Transplantation. (2000) 69:711–9. doi: 10.1097/00007890-200003150-00007
35. Miyagawa S, Yamamoto A, Matsunami K, Wang D, Takama Y, Ueno T, et al. Complement regulation in the GalT KO era. Xenotransplantation. (2010) 17:11–25. doi: 10.1111/xen.2010.17.issue-1
36. Davalli AM, Ogawa Y, Scaglia L, Wu YJ, Hollister J, Bonner-Weir S, et al. Function, mass, and replication of porcine and rat islets transplanted into diabetic nude mice. Diabetes. (1995) 44:104–11. doi: 10.2337/diab.44.1.104
37. Liu Z, Hu W, He T, Dai Y, Hara H, Bottino R, et al. Pig-to-primate islet xenotransplantation: past, present, and future. Cell Transplant. (2017) 26:925–47. doi: 10.3727/096368917X694859
38. Dufrane D, Goebbels RM, Gianello P. Alginate macroencapsulation of pig islets allows correction of streptozotocin-induced diabetes in primates up to 6 months without immunosuppression. Transplantation. (2010) 90:1054–62. doi: 10.1097/TP.0b013e3181f6e267
39. Wynyard S, Nathu D, Garkavenko O, Denner J, Elliott R. Microbiological safety of the first clinical pig islet xenotransplantation trial in New Zealand. Xenotransplantation. (2014) 21:309–23. doi: 10.1111/xen.12102
40. Rayat GR, Rajotte RV, Elliott JF, Korbutt GS. Expression of Gal alpha(1,3)gal on neonatal porcine islet beta-cells and susceptibility to human antibody/complement lysis. Diabetes. (1998) 47:1406–11. doi: 10.2337/diabetes.47.9.1406
41. Yamada K, Yazawa K, Shimizu A, Iwanaga T, Hisashi Y, Nuhn M, et al. Marked prolongation of porcine renal xenograft survival in baboons through the use of alpha1,3-galactosyltransferase gene-knockout donors and the cotransplantation of vascularized thymic tissue. Nat Med. (2005) 11:32–4. doi: 10.1038/nm1172
42. Kuwaki K, Tseng YL, Dor FJ, Shimizu A, Houser SL, Sanderson TM, et al. Heart transplantation in baboons using alpha1,3-galactosyltransferase gene-knockout pigs as donors: initial experience. Nat Med. (2005) 11:29–31. doi: 10.1038/nm1171
43. Rayat GR, Rajotte RV, Hering BJ, Binette TM, Korbutt GS. In vitro and in vivo expression of Galalpha-(1,3)Gal on porcine islet cells is age dependent. J Endocrinol. (2003) 177:127–35. doi: 10.1677/joe.0.1770127
44. van der Windt DJ, Bottino R, Casu A, Campanile N, Smetanka C, He J, et al. Long-term controlled normoglycemia in diabetic non-human primates after transplantation with hCD46 transgenic porcine islets. Am J Transplant. (2009) 9:2716–26. doi: 10.1111/j.1600-6143.2009.02850.x
45. Thompson P, Badell IR, Lowe M, Cano J, Song M, Leopardi F, et al. Islet xenotransplantation using gal-deficient neonatal donors improves engraftment and function. Am J Transplant. (2011) 11:2593–602. doi: 10.1111/j.1600-6143.2011.03720.x
46. Hawthorne WJ, Salvaris EJ, Chew YV, Burns H, Hawkes J, Barlow H, et al. Xenotransplantation of genetically modified neonatal pig islets cures diabetes in baboons. Front Immunol. (2022) 13:898948. doi: 10.3389/fimmu.2022.898948
47. Yamada K, Shimizu A, Utsugi R, Ierino FL, Gargollo P, Haller GW, et al. Thymic transplantation in miniature swine. II. Induction of tolerance by transplantation of composite thymokidneys to thymectomized recipients. J Immunol. (2000) 164:3079–86. doi: 10.4049/jimmunol.164.6.3079
48. LaMattina JC, Kumagai N, Barth RN, Yamamoto S, Kitamura H, Moran SG, et al. Vascularized thymic lobe transplantation in miniature swine: I. Vascularized thymic lobe allografts support thymopoiesis. Transplantation. (2002) 73:826–31. doi: 10.1097/00007890-200203150-00032
49. Yamada K, Vagefi PA, Utsugi R, Kitamura H, Barth RN, LaMattina JC, et al. Thymic transplantation in miniature swine: III. Induction of tolerance by transplantation of composite thymokidneys across fully major histocompatibility complex-mismatched barriers. Transplantation. (2003) 76:530–6. doi: 10.1097/01.TP.0000080608.42480.E8
50. Kumagai N, LaMattina JC, Kamano C, Vagefi PA, Barth RN, JJ, et al. Vascularized islet cell transplantation in miniature Swine: islet-kidney allografts correct the diabetic hyperglycemia induced by total pancreatectomy. Diabetes. (2002) 51:3220–8. doi: 10.2337/diabetes.51.11.3220
51. Kumagai N, O’neil JJ, Barth RN, LaMattina JC, Utsugi R, Moran SG, et al. Vascularized islet-cell transplantation in miniature swine. I. Preparation of vascularized islet kidneys. Transplantation. (2002) 74:1223–30. doi: 10.1097/00007890-200211150-00005
52. Yamada K, Hirakata A, Tchipashvili V, Shimizu A, Iwaki H, Griesemer A, et al. Composite islet-kidneys from single baboon donors cure diabetes across fully allogenic barriers. Am J Transplant. (2011) 11:2603–12. doi: 10.1111/j.1600-6143.2011.03733.x
53. Pomposelli T, Wang P, Takeuchi K, Miyake K, Ariyoshi Y, Watanabe H, et al. Protection of pancreatic islets using theranostic silencing nanoparticles in a baboon model of islet transplantation. Diabetes. (2020) 69:2414–22. doi: 10.2337/db20-0517
54. Bottino R, Balamurugan AN, Smetanka C, Bertera S, He J, Rood PP, et al. Isolation outcome and functional characteristics of young and adult pig pancreatic islets for transplantation studies. Xenotransplantation. (2007) 14:74–82. doi: 10.1111/j.1399-3089.2006.00374.x
55. Kim JH, Kim HI, Lee KW, Yu JE, Kim SH, Park HS, et al. Influence of strain and age differences on the yields of porcine islet isolation: extremely high islet yields from SPF CMS miniature pigs. Xenotransplantation. (2007) 14:60–6. doi: 10.1111/j.1399-3089.2006.00364.x
56. Mezrich JD, Haller GW, Arn JS, Houser SL, Madsen JC, Sachs DH. Histocompatible miniature swine: an inbred large-animal model. Transplantation. (2003) 75:904–7. doi: 10.1097/01.TP.0000054839.43852.BF
57. Fuchimoto Y, Yamada K, Shimizu A, Yasumoto A, Sawada T, Huang CH, et al. Relationship between chimerism and tolerance in a kidney transplantation model. J Immunol. (1999) 162:5704–11. doi: 10.4049/jimmunol.162.10.5704.
58. Cui W, Gu Y, Miyamoto M, Tanaka M, Xu B, Imamura M, et al. Novel method for isolation of adult porcine pancreatic islets with two-stage digestion procedure. Cell Transplant. (1999) 8:391–8. doi: 10.1177/096368979900800408
Keywords: islet xenotransplantation, islet-kidney, xenogeneic immune response, tolerance, xenotransplantation
Citation: Eisenson DL, Iwase H, Chen W, Hisadome Y, Cui W, Santillan MR, Schulick AC, Gu D, Maxwell A, Koenig K, Sun Z, Warren D and Yamada K (2024) Combined islet and kidney xenotransplantation for diabetic nephropathy: an update in ongoing research for a clinically relevant application of porcine islet transplantation. Front. Immunol. 15:1351717. doi: 10.3389/fimmu.2024.1351717
Received: 06 December 2023; Accepted: 08 February 2024;
Published: 27 February 2024.
Edited by:
Burcin Ekser, Indiana University Bloomington, United StatesReviewed by:
Yi Wang, Second Affiliated Hospital of Hainan Medical University, ChinaCopyright © 2024 Eisenson, Iwase, Chen, Hisadome, Cui, Santillan, Schulick, Gu, Maxwell, Koenig, Sun, Warren and Yamada. This is an open-access article distributed under the terms of the Creative Commons Attribution License (CC BY). The use, distribution or reproduction in other forums is permitted, provided the original author(s) and the copyright owner(s) are credited and that the original publication in this journal is cited, in accordance with accepted academic practice. No use, distribution or reproduction is permitted which does not comply with these terms.
*Correspondence: Kazuhiko Yamada, a3lhbWFkYTZAamhtaS5lZHU=
Disclaimer: All claims expressed in this article are solely those of the authors and do not necessarily represent those of their affiliated organizations, or those of the publisher, the editors and the reviewers. Any product that may be evaluated in this article or claim that may be made by its manufacturer is not guaranteed or endorsed by the publisher.
Research integrity at Frontiers
Learn more about the work of our research integrity team to safeguard the quality of each article we publish.