- 1The First School of Clinical Medicine, Gannan Medical University, Ganzhou, China
- 2Laboratory Medicine, First Affiliated Hospital of Gannan Medical University, Ganzhou, China
Extracellular vesicles (EVs) are important cell-to-cell communication mediators. This paper focuses on the regulatory role of tumor-derived EVs on macrophages. It aims to investigate the causes of tumor progression and therapeutic directions. Tumor-derived EVs can cause macrophages to shift to M1 or M2 phenotypes. This indicates they can alter the M1/M2 cell ratio and have pro-tumor and anti-inflammatory effects. This paper discusses several key points: first, the factors that stimulate macrophage polarization and the cytokines released as a result; second, an overview of EVs and the methods used to isolate them; third, how EVs from various cancer cell sources, such as hepatocellular carcinoma, colorectal carcinoma, lung carcinoma, breast carcinoma, and glioblastoma cell sources carcinoma, promote tumor development by inducing M2 polarization in macrophages; and fourth, how EVs from breast carcinoma, pancreatic carcinoma, lungs carcinoma, and glioblastoma cell sources carcinoma also contribute to tumor development by promoting M2 polarization in macrophages. Modified or sourced EVs from breast, pancreatic, and colorectal cancer can repolarize M2 to M1 macrophages. This exhibits anti-tumor activities and offers novel approaches for tumor treatment. Therefore, we discovered that macrophage polarization to either M1 or M2 phenotypes can regulate tumor development. This is based on the description of altering macrophage phenotypes by vesicle contents.
1 Introduction
Most cells in the body can secrete extracellular vesicles (EVs), which are small lipid bilayer vesicles with a diameter of 30–2000 nm (1). Since most EV separation techniques are unable to concentrate on EVs produced by different mechanisms, the updated version of the MISEV guidelines in 2023 discourages the use of biologically-generated terms, such as exosomes, microvesicles, etc. The International Society for Extracellular Vesicles(ISEV) recommends use of the generic term ‘EV’ and operational extensions of this term. ISEV continues to encourage the use of these terms for the variety of EV subtypes that are separated based on size, density, cellular origin, and other characteristics. Generally, small extracellular vesicles(sEVs) refer to EVs <200 nm in diameter, and large extracellular vesicles(LEVs) refer to EVs >200 nm in diameter. EV mimetics produced by inducing cell rupture in the laboratory are named artificial cell-derived vesicles (ACDVs), and EV mimetics synthesized from molecular components are named synthetic vesicles(SVs) (2). This review will be named using sEVs or LEVs according to the extracellular vesicle size of the original authors, as well as ACDVs or SVs depending on how they were synthesized. EVs will be used uniformly for cases where the size and production methods of vesicles are not specified in the original text, or when some concepts are being described. EVs contain genetic materials like proteins and nucleic acids, playing various roles in disease processes. These roles involve causing changes in recipient cells, communicating between cells, transferring proteins and nucleic acids, impacting inflammation and immune regulation, and influencing angiogenesis and coagulation (3, 4).
EVs are crucial for studying disease pathogenesis, prognosis, and diagnostic due to their ability to carry biologically active cargo. Hematopoietic cells, including B cells, platelets, macrophages, and dendritic cells, produce sEVs (5). Additionally, non-hematopoietic cells, such as tumor cells, Schwann cells, astrocytes, etc., produce it (6–8). Several studies have demonstrated that sEVs alter the macrophage phenotype.
The active molecules that EVs contain determine the different phenotypes of macrophages. The complex junction of various cells that determine the tumor microenvironment (TME)—also referred to as the tumor stroma—is where cancer cells interact with surrounding cells. Immune cells, stromal cells, blood vessels, and extracellular matrix are characteristics of TMEs (9). Macrophages are found in all tissues and are essential components of the TME. As innate immune cells, macrophages can polarize in different directions based on their surrounding environment (10). Additionally, macrophages are found in all tissues. As a result, they are essential cellular components of TME (11). Macrophages play a role in the development and progression of tumors by promoting angiogenesis (12), facilitating tumor cell migration and invasion (13), and enhancing tumor drug resistance (14). Macrophages can undergo polarization and differentiate into M1 and M2 macrophages in response to alterations in the TME (15). When M1 macrophages are activated, they release pro-inflammatory factors like tumor necrosis factor-alpha (TNF-α), interleukin-6 (IL-6), and IL-12. Macrophage polarization is activated to the M1 phenotype through lipopolysaccharide (LPS) and interferon-gamma (16, 17). These factors also have anti-tumorigenic and pro-inflammatory properties. IL-4 and IL-13 promote M2 macrophages to polarize to the M2 phenotype, which releases several anti-inflammatory factors to reduce the inflammatory response (18, 19). M2 macrophages have anti-inflammatory and tumor-promoting effects. However, the relative roles of M1 and M2 macrophages significantly affect tumor development and therapy in the TME (20, 21). EVs produced from tumor cells are believed to facilitate communication between tumor cells and immune cells (22). Changes in immune cell phenotype in the TME can directly impact tumor progression (23). This paper focuses on how EVs from tumor cells influence macrophage polarization, affecting tumor progression.
2 Macrophage polarization
In addition to malignant cells, TME contains many normal cells, such as blood vessels, fibroblasts, and immune cells. Macrophages are significant immune cells in this milieu. In the TME, these malignant cells interact with the active factors secreted by normal cells (24). The primary characteristic of the TME is immunosuppression, which changes as the tumor progresses. For example, certain primary tumors release multiple molecules that contribute to cancer-promoting TME, facilitating tumor cell colonization and dissemination to distant organs (25). However, it is challenging to target tumors precisely because the TME has many commonly expressed cells that suppress the immune response. These are the reasons why TME-induced tumor treatment can be challenging. Although macrophages are the most abundant critical non-tumor immune cells in the TME, research into the mechanism of tumor-macrophage interaction may pave the way for novel approaches to tumor therapy (26).
Tumor-associated macrophages (TAMs) comprise 30% and 50% of the total tumor mass and are the most prevalent and significant non-tumorigenic cells in the TME (27). How macrophages polarize towards anti-inflammatory pro-tumorigenic M2 or pro-inflammatory anti-tumorigenic M1 determines whether the TME has anti-tumor or pro-tumorigenic effects. Additionally, the stimulation of TME influences the polarization of these macrophages. TAMs are essentially groups of macrophages with distinct phenotypes. When TAMs take on an M2 phenotype, they promote angiogenesis, immunosuppression, and invasive metastasis, contributing to tumor development (28). Strategies for treating TAMs-associated tumor immunotherapy include polarizing M2-like TAMs to M1 anti-tumor phenotype, reducing the survival of M2 phenotype TAMs, and inhibiting the recruitment of infiltrating macrophages (29, 30). The first of these approaches is the most straightforward.
Macrophages exhibit different phenotypes that are broadly classified into two types: classically activated M1-like macrophages and alternatively activated M2-like macrophages, depending on the TME (31, 32). In the early stages of the disease, macrophages often adopt a pro-inflammatory M1 phenotype; however, as the disease progresses, they adopt an anti-inflammatory M2 phenotype to promote tissue repair, regeneration, and fibrosis. Disease development is closely associated with the transition between the M1 and M2 phenotypes, and the two phenotypes each have unique roles that interact to regulate the development of the disease (Figure 1) (33).
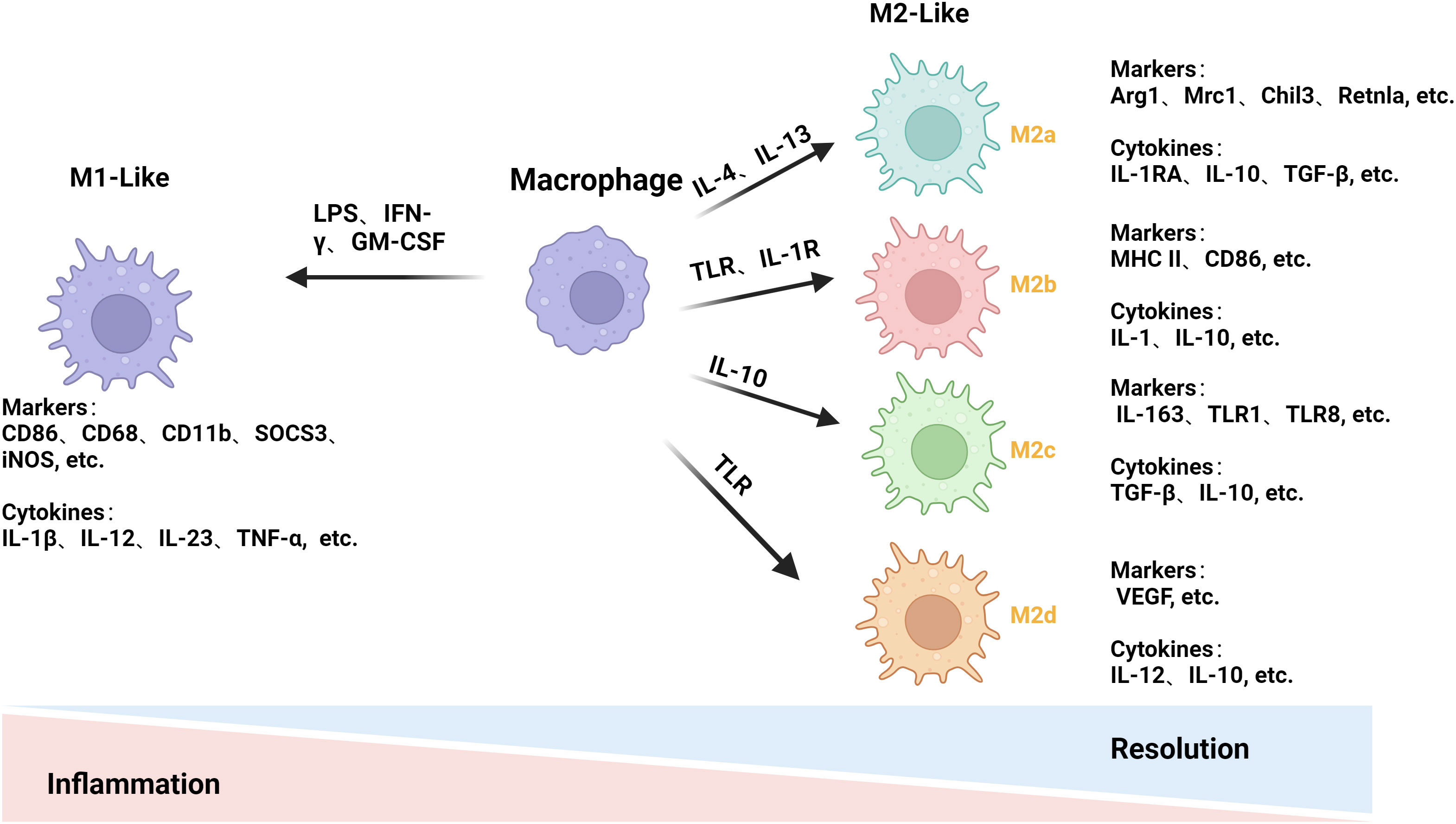
Figure 1 Mechanisms of macrophage polarization. LPS and others stimulate macrophage polarization to the M1 phenotype to release pro-inflammatory factors for pro-inflammatory and anti-tumor effects. IL-4 and others stimulate macrophages to differentiate into the M2 phenotype and release anti-inflammatory factors to achieve anti-inflammatory and pro-tumorigenic effects. The M2 phenotype is subdivided into four subtypes: M2a, M2b, M2c, and M2d. (This figure was created with Biorender.com.)
2.1 M1-like macrophages
M1 macrophages can present antigens and have pro-inflammatory effects (34). The M1 phenotype is induced by stimuli such as LPS, IFN-γ, and granulocyte monocyte colony-stimulating factor. This allows the M1 phenotype to play a significant role in the early stages of inflammation. Pro-inflammatory cytokines, such as IL-1β, IL-12, IL-23, and TNF-α, are released, further promoting inflammatory and cytotoxic responses (35, 36). To track changes in macrophage M1-like polarization, we often look for changes in relevant markers, including CD86, CD68, CD11b, suppressor of cytokine signaling 3, inducible nitric oxide synthase (iNOS), etc. (37, 38).
2.2 M2-like macrophages
M2 macrophages can be subdivided into M2a, M2b, M2c, and M2d. M2 macrophages have a low antigen-presenting ability and mostly release anti-inflammatory factors (39). IL-4 or IL-13 can effectively counteract inflammatory damage and promote tissue healing by stimulating macrophages to differentiate into M2a cells and release anti-inflammatory cytokines such as IL-1RA, IL-10, and transforming growth factor-beta (TGF-β) (40, 41) via the co-receptor IL-4Rα. Arg1, Mrc1, Chil3, and Retnla are their primary markers of detection (42). When TLR or IL-1R agonists are exposed to the body, they produce M2b macrophages that release cytokines, IL-10, IL-1, etc., to exert immunomodulatory effects. Major histocompatibility complex II (MHC II), CD86, etc., are their primary monitoring markers (41, 43). IL-10 helps macrophages become M2c. IL-10 helps macrophages become M2c, which has anti-inflammatory and anti-phagocytic effects, which stimulates macrophages to induce an M2c phenotype and produce cytokines like TGF-β and IL-10. This process also induces arginase-1 (Arg1) expression. CD163, TLR1, TLR8, etc., are the primary indicators for its detection (44, 45). TLR agonist-induced macrophages primarily release cytokines such as IL-10 and IL-12, which promote tumor progression and angiogenesis and are the primary source of M2d macrophages (46). Vascular endothelial growth factor and other primary monitoring markers (47). Different types of M2 macrophages have different roles, so it is crucial to study them separately.
3 Extracellular vesicles
3.1 Overview of extracellular vesicles
Sheep reticulocytes are the source of the EVs that Pan (48) and Johnstone (49) first discovered and dubbed “exosomes”. EVs are released by all types of cells, including blood, immune, cancer, and stem cells. Blood, urine, breast milk, ascites, amniotic fluid, saliva and cerebrospinal fluid are among the bodily fluids into which the stem cells created by these cells can be released (50). Proteins and nucleic acids (miRNA, mRNA, and lncRNA) are abundant in content found in EVs. Due to their genetic material carrying capacity, EVs are crucial in various diseases (51).
3.2 Techniques for isolation of extracellular vesicles
There is disagreement on the best extraction technique for EVs, although they are extracted using various techniques. Differential ultracentrifugation(dUC), size exclusion, immuno-capture, and precipitation are often employed techniques (52). When selecting an isolation approach, we must have a thorough grasp of the downstream needs of our research because each method has pros and cons of its own (53, 54). Our method for isolating EVs is selected based on our research needs for harvest rate and specificity. Due to its high harvest rate and low cost, dUC is the most commonly used method for isolating EVs. However, it has drawbacks, like being time-consuming and having limited specificity (55). Understanding that specificity and harvest rate have an inverse relationship is essential. As a result, no single technique can accomplish a high harvest rate and high specificity. The latest MISEV guidelines suggest that large volumes of source materials may require concentration before EVs can be separated from other extracellular particles (EPs), a move that may make some separation methods more efficient. Notably, the guidelines specifically note that precipitation methods may not be able to separate different types of EPs; for example, the Exosome Separation Kit relies heavily on polymer precipitation to separate EVs, and in fact these kits do not rigorously separate EVs, let alone other subtypes of EVs (2). The principles, advantages and disadvantages of various commonly used separation methods are mainly summarized below: (i) dUC is to apply increasing relative centrifugal forces to the EV‐containing fluid by centrifuging multiple times to separate vesicles and other EPs and by controlling the magnitude of centrifugal force and centrifugation time to distinguish between vesicles of different diameters. Typically we use low speed centrifugation to remove cellular debris and large vesicles (typically 10,000 to 2,000g) and ultra-high speed centrifugation for smaller vesicles (typically 100,000 to 200,000g). This method is the most commonly used and inexpensive, but it is very time-consuming, often requiring 60-120 min to extract the different subtypes of EVs needed, and has low specificity (53, 56). (ii) Density gradient centrifugation is a more rigorous form of ultra-centrifugation, where vesicles of different densities settle at different rates on a gradient. EV‐containing materials can be loaded beneath a gradient or onto the top of a gradient or cushion and then ultracentrifuged. After the EVs preparation is loaded below the gradient for a sufficiently long period of ultracentrifugation, the particles will eventually reach a density fraction corresponding to their buoyant density. Since smaller EVs run slower than larger ones, this method can be used to separate sEVs from LEVs. Density gradient method is also one of the more commonly used methods, which has high specificity for the extraction of EVs. However, the method has low yield and is very time-consuming, even requiring 16-48h to complete the whole experiment (57). (iii) Precipitation involves the use of chemicals such as polyethylene glycol to reduce the solubility of the EVs, thereby causing them to precipitate, followed by low-speed centrifugation to obtain sEVs. This method, although rapid in extraction, is particularly susceptible to the introduction of new contaminants such as polyethylene glycol and makes it difficult to distinguish between EPs and EVs (2). (iv) Size exclusion uses a column with a defined pore size to separate EVs of different particle sizes. Driven by gravity, larger EVs do not enter the pore and are quickly eluted through the column at an early stage, whereas the opposite is true for smaller EVs, so that different sizes of EVs can be collected by changing the substrate. This method allows for the rapid separation of EVs, but has a limited lifetime of the column, is costly to extract and often carries contaminating proteins (58). (v) Immunocapture involves the separation of vesicles coated with magnetic beads containing the target protein. The method is rapid but costly to extract (59). The above methods can be used to isolate EVs and different subtypes of EVs, especially LEVs and sEVs, can be isolated by changing the experimental conditions such as centrifugation speed, time, column pore size, etc. For characterization of the extracted EVs, the most recent guidelines state that there is no universal molecular marker for the identification of a specific subtype of EVs. In the current literature, studies of EVs have focused on smaller diameters, and commonly used identification tools include nanoflowmetry to detect the diameter of extracted vesicles, protein blotting to detect marker proteins of EVs (e.g., positive markers CD63, CD9, Alix, and negative marker GM130) (60), and transmission electron microscopy to observe the microscopic morphology of the vesicles (61).
4 Extracellular vesicles induce M2 polarization in macrophages
The development of tumors depends heavily on the TME. Macrophages are immune cells linked to the advancement of tumors (62). These macrophages are thought to be essential for the evolution of tumors because EVs promote polarization in macrophages, which can then be engaged in tumor proliferation, invasion, angiogenesis, and tissue inflammation through pertinent downstream pathways (63, 64). Macrophages activate tumor-associated fibroblasts, pro-angiogenic factors, and other components of the TME (65, 66).
Tumor-derived EVs can be absorbed by all immune cells and are essential in immunomodulation and TME (67). M1 and M2 macrophages can change in response to EVs, affecting the TME. According to studies, TAM is an M2 phenotype implicated in tumor angiogenesis, proliferation, and metastasis (68). Tumor-derived EVs have been proposed as a critical communication mediator between tumors and immune cells. Next, we will discuss how several tumor-derived EVs, enumerated in Table 1, contribute to macrophage M2 polarization and tumor growth.
4.1 Breast cancer-derived extracellular vesicles
Breast cancer is one of the most common malignant tumors in women globally, with a high rate of mortality and morbidity. Research has demonstrated that breast cancer cell-derived sEVs can induce M2 polarization in macrophages by the transfer of circ_0001142 and can also disrupt autophagy levels, promoting tumor proliferation, migration, invasion, and epithelial-mesenchymal transition (69). MiRNAs in tumor cells can regulate macrophage polarization using EVs vectors and circRNAs. The 3’-untranslated region of KDM6B was the target of increased production of miR-138-5p in EVs in breast cancer cell-derived EVs, which promoted M2 polarization by inhibiting the expression of KDM6B in macrophages. When Xun et al. transplanted mouse macrophages from EVs-treated breast cancer cells overexpressing miR-138-5p into mice for in vivo experiments, the experimental group displayed more lung metastases than those transplanted with mouse macrophages from control EVs (70). In sEVs derived from adriamycin-resistant breast cancers, Chen et al. found that miR-222 expression was significantly higher. They found that the levels of miR-222 were significantly higher in tumor tissues of patients resistant to chemotherapy compared to those sensitive to chemotherapy. This suggests that miR-222 could serve as a predictor of tumor progression. Resistant breast cancer-derived sEVs containing miR-222 promote macrophage M2 polarization and shift the role of macrophage against tumors from inhibition to promotion by activating the PTEN/AKT pathway. Additionally, they implanted a mixture of macrophages treated with adriamycin-resistant tumor cell sEVs subcutaneously in mice. They found that the subcutaneous tumors in the sensitive group were considerably smaller than those in the adriamycin-sensitive group (71).
4.2 Hepatocellular carcinoma-derived extracellular vesicles
HCC is the sixth most prevalent cancer globally and is third in terms of overall cancer mortality. Zhou et al. reported that EVs from liver cancer cells can be ingested by macrophages and cause them to upregulate TLR4 expression by inhibiting miR-372-3p. This is achieved by the EVs carrying PART1, a long-stranded noncoding RNA (lncRNA) that promotes M2 polarization in macrophages. Consequently, M2 macrophages significantly increased the tumorigenicity of HCC cells in nude mice, and lncRNA PART1 also promoted the proliferation, migration, invasion, and epithelial cell transformation (EMT) of tumors (72). MiR-452-5p and miR-200b-3p were found in high levels in EVs of HCC cells. They are linked to tumor progression, survival, and recurrence. Moreover, they promote the growth of hepatocellular carcinoma and can promote M2 polarization of macrophages. Following the injection of tumor cell-derived EV inhibitors or miRNA mimics into mice, the mice treated with EV mimics exhibited higher tumor volumes and more significant lung nodal metastases (73, 74). HCC cell-derived sEVs that express miR-21-5p aggregates in macrophages directly target RhoB to promote M2 polarization in macrophages and promote hepatocellular carcinoma proliferation (75).
4.3 Lung-cancer-derived extracellular vesicles
Lung cancer is among the world’s most common causes of cancer-related deaths, with its high aggressiveness and inferior prognosis. Regarding the pathogenesis of lung cancer, in addition to the microRNAs, such as miR-19b-3p, miR-138-5p, and miR-3153 that are carried by EVs originating from tumor cells and can trigger M2 polarization in macrophages and promote the development of lung cancer (70, 76, 77). Meanwhile, hypoxia can change the course of a tumor and is a common occurrence in the TME. Increasing research indicates that tumor cells may be stimulated to produce sEVs under hypoxic conditions to promote tumor progression. According to Jin et al., miR-21 expression was more abundant in hypoxic lung cancer-derived sEVs. These miR-21 were also found to be translocated to macrophages, where they bound to interferon regulatory factor 1 and inhibited its expression. This process can promote macrophage M2 polarization and upregulate tumor growth rate. In experiments with male nude mice, researchers injected a mix of H1299 cells and THP-1 cells under different conditions. Mice treated with sEVs from hypoxic H1299 cells showed higher levels of miR-21 expression and larger tumor volumes. Immunohistochemistry (IHC) analysis of the tumor tissues from mice in each group treated showed that the hypoxic sEVs-treated group had higher levels of CD163 expression in their tissues, suggesting a higher level of M2 macrophage infiltration (78). Gu et al. showed that hypoxic lung cancer cell-derived sEVs promote lung cancer by influencing macrophage polarization through increased miR-1290 levels. They measured tumor volume and weight in different groups using a mouse model and detected CD163 expression in the tissues using IHC. This showed that tumor tissues in mice treated with hypoxic cancer cells sEVs had increased in volume, weight, and CD163 expression (79).
4.4 Glioblastoma cell-derived extracellular vesicles
Although pilocytic astrocytomas, the least malignant of these, only have a 5–10 year survival rate, gliomas make up 80% of all primary tumors (80). Glioblastoma (GBM) is the most malignant type of glioma; it is highly aggressive and rapidly progressive and only gives patients a 15-month survival period after diagnosis (6.8%) (81). Following surgery, radiotherapy, and chemotherapy, patients with GBM eventually relapse, exhibiting a high degree of aggression and drug resistance. We suggest that immunosuppression may have a role in the poor response to GBM therapy (82). High levels of EVs released by GBM cells have been shown to serve as a diagnostic marker in the blood of patients with GBM (83). TAM cells originate from two primary sources: bone marrow-derived and microglia cells. In the GBM microenvironment, TAM cells are primarily derived from the latter. The crosstalk between GBM and microglia significantly influences tumor progression and therapeutic resistance. In a recent study by Zhang et al., microglia were found to absorb sEVs released by irradiated GBM cells, inducing them to undergo M2 polarization. This lowers the microglia’s ability to phagocytose microglia. The increased CCL2 expression in M2 microglia also notably supported the proliferation of irradiated GBM cells. Furthermore, the study observed an increase in circ_0012381 in sEVs derived from irradiated GBM cells, suggesting that these sEVs, containing circ_0012381, primarily induce M2 polarization in microglia. This offers a potential direction to improve the efficacy of radiotherapy. To confirm the findings of this experiment in vivo, they injected zebrafish embryos with a mixture of GBM cells and microglia and incubated them. Following that, they selected similarly sized fry to be exposed to radiation. At the end of the experiment, they used fluorescence microscopy to measure the tumor volume of the zebrafish. They found that the group of glioma tumor cells treated with the exosome inhibitor GW4869 ad notably reduced tumor weight and volume compared to the control group. This increased the efficacy of radiation therapy (84). Research has demonstrated that GBM cell-derived sEVs and GBM tissues have higher expression levels of miR-27a-3p. These microRNAs are transferred from sEVs to macrophages, inhibiting the zeste homolog 1 (EZH1) expression by targeting it. This process, mediated by miR-27a-3p, prompts M2 polarization in macrophages, which enhances proliferation, migration, and resistance to radiotherapy in GBM tumors. In addition to their contribution to tumor pathogenesis, they have the potential to serve as diagnostic markers for GBM (85).
4.5 Colorectal cancer-derived extracellular vesicles
CRC is the second most common cause of tumor-related deaths globally, with the third highest prevalence. Liver metastases from CRC account for a high number of deaths. In a study by Zhao et al., higher levels of miR-934 were found in the serum of CRC patients, with or without liver metastases, and in healthy individuals. This elevated expression of miR-934 may suggest the presence of liver metastases in CRC. Subsequently, they investigated the pathogenesis of this molecule in CRC cell-derived EVs. They discovered that via downregulating PTEN expression, miR-934 in EVs generated from CRC cells caused macrophage M2 polarization, CRC invasion, and metastasis (86). Pei et al. found that miR-203a-3p in sEVs from CRC cells act similarly to what was mentioned earlier by targeting PTEN to promote M2 polarization and assist in CRC hepatic metastasis. Additionally, they performed plasma analysis and discovered that CRC cell-derived sEVs containing miR-203a-3p might be used as a non-invasive fluid marker to identify liver metastasis (87). Therefore, we found that miRNAs in sEVs can strongly indicate diagnostic markers and trigger the onset of CRC, which could help with the subsequent CRC diagnosis and treatment. Of course, in addition to being a highly invasive and metastatic tumor in and of itself, CRC has a low survival rate and is a challenging case of chemoresistance. Aerobic fermentation produces ATP, which aids in rapid tumor growth and chemoresistance in malignant tumors. Wang et al. showed that tumor cell lines from oxaliplatin-resistant tumors can accelerate glycolysis and drug resistance by releasing sEVs containing ciRS-122, which upregulates ciRS-122 levels in sensitive cell lines through transcellular transfer. To separate the mice into experimental and control groups, they transplanted drug-resistant and sensitive tumor blocks into the right inguinal region of female nude mice. They transplanted SW480 tumor blocks of the same size into each inguinal region. They then injected oxaliplatin into the peritoneal cavity of each group of mice. They cut off the left side of the tumors to measure the volume, which was more significant than that of the control group. This study may provide directions for reversing chemoresistance in CRC (88). EMT increases the ability of cells to invade other cells and metastasize, which contributes to cancer development. According to Yang et al., there was an increase in the expression level of miR-106b-5p in the sEVs of EMT-CRC cells. This expression level is essential because it can target programmed cell death 4, activate the mammalian target of the rapamycin signaling pathway, and promote macrophage M2 polarization. Meanwhile, CRC cell migration, invasion, and metastasis mediated by EMT may be inhibited by activated M2 macrophages. Additionally, they looked at miR-106b expression in plasma sEVs and found that it was increased and correlated with CRC malignancy (89).
4.6 Pancreatic cancer-derived extracellular vesicles
Despite treatment, less than 5% of patients with pancreatic cancer survive for five years, making it one of the most dangerous cancers. The high rate of mortality and the advanced stage of the disease at the time of diagnosis are the primary causes of the high death rate among individuals with pancreatic cancer. Thus, research into the pathogenesis of pancreatic cancer and the development of new diagnostic markers are essential. An essential part of tumor metastasis is played by hypoxia and inflammatory cell infiltration. Wang et al. found that sEVs produced from pancreatic cancer cells could transfer miR-301a to macrophages by targeting ETS homologous factor. This promotes M2 polarization in macrophages by downregulating PTEN expression. They discovered miR-301a in serum sEVs and could be used as a diagnostic marker. They also proposed that miR-301a in sEVs may be exploited as a target for tumor immune escape (90). One of the main factors contributing to pancreatic cancer treatment challenges is immune evasion. Wang et al. discovered that EVs produced from pancreatic cancer cells could transfer miR-155-5p to macrophages through their targeting ETS homologous factor. This resulted in the activation of the Akt/NF-κB pathway, which enabled the macrophages to become M2 polarized and promoted tumor escape (91).
5 Extracellular vesicles induce M1 polarization in macrophages
M1 polarized macrophages have pro-inflammatory and anti-tumor properties (92). As nanocarriers, EVs can boost immune cell reactions against cancer. Thus, introducing EVs from donor cells into macrophages may cause M1 polarization in these cells, which has anti-tumor properties. Based on previous research, we discovered that proteins might be produced exogenously loaded onto EVs to drive them to particular target cell sites, in addition to the therapeutic benefits reported in naturally occurring EVs (28, 93). The pro-tumor phenotype M2 phenotype of macrophages is changed into an anti-tumor M1 phenotype by this EVs-based immunotherapy, which shows promise in slowing the growth of tumors (Table 1) (94).
5.1 Breast cancer-derived extracellular vesicles after artificial modification or treatment
Over the past 50 years, significant progress has been made in the diagnosis and treatment of breast cancer. Moradi-Chaleshtori et al. allegedly changed M2-polarized macrophages into M1 macrophages by electroporating sEVs from breast cancer 4T1 cell lines with miR-33 mimics during co-culturing with IL-4-induced M2-type macrophages. They concluded that macrophages could be reprogrammed for anti-tumor activities by introducing SVs expressing miR-33 (95). In a related study, they encapsulated miR-33 and miR-130 into sEVs to reprogram macrophages to produce more indicators of their M1 phenotype, which allowed them to exert anti-tumor activities. They injected mice with a mixture of 4T1 cells and macrophages from various treatment groups to observe how sEV-treated macrophages affected the development of breast cancer. The tumor volume was less, and patches of necrosis in the tumors of these mice were also visible for anti-tumor effects, as demonstrated by the eosin staining comparing the mice receiving miR-130 or miR-33-loaded SVs or both-treated macrophages to the control group (96). Finding more effective treatments is critical since triple-negative breast cancer makes up 10%–24% of all breast cancers in clinical practice and has a poor prognosis, significant drug resistance, and high metastasis. Ghalavand et al. treated the breast cancer 4T1 cell line with rapamycin and isolated sEVs to influence M2 macrophages produced by IL-4, repolarizing them towards M1 macrophages. This enhanced macrophage phagocytosis. These findings lift the immunosuppression of the tumor by promoting macrophage M1 polarization, which offers a fresh approach to clinical tumor therapy (97).
5.2 Colorectal cancer-derived extracellular vesicles after artificial modification or treatment
Reprogramming TAM cells to the M1 phenotype is one novel way to generate anti-tumor immunity for treating colorectal cancer. Numerous researchers have examined the correspondingly designed sEVs for this reason. A crucial molecule in macrophages going through M2 polarization is the signal transducer and activator of transcription 6 (STAT6). Kamerkar et al. created SVs that can be loaded with antisense oligonucleotides targeting STAT6. These SVs were extracted from HEK 293 cells after loading into the cells after design using the engEx platform. The modified sEVs can be absorbed and migrated by cells, and they can successfully inhibit the expression of STAT6 in macrophages, causing them to change from M2 to M1, which has anti-tumor effects (94). Short-course radiation therapy has been used in clinical settings to try and alter the ratio of M1 and M2 phenotypes. Stary et al. compared preoperative tissues with and without radiotherapy and isolated progenitor cells for ex vivo irradiation experiments. They found that short-course neoadjuvant radiotherapy in patients with rectal cancer led to a change in macrophages towards the M1 phenotype and an increase in the M1/M2 ratio. They isolated the EVs from irradiated or unirradiated DLD-1 cells and co-cultured them with M2 macrophages for 48 hours to investigate if the change in the TME by short-course radiation is linked to EV-mediated effects by irradiated cancer cells. They discovered that M2 macrophages tended to change to the M1 phenotype (98). The research suggests that treating colorectal cancer through macrophage transformation to M1 phenotype will be crucial.
5.3 Pancreatic cancer-derived extracellular vesicles after artificial modification or treatment
Approximately 80%–90% of pancreatic cancers are pancreatic ductal adenocarcinoma (PDAC), which has a 5-year survival rate of about 8%–25%. PDAC is a highly aggressive malignancy. Increasing its diagnostic sensitivity and treatment strategies is vital because it is typically found in advanced stages or has spread during diagnosis. Su et al. discovered that sEVs generated from pancreatic cancer Panc-1 cell line may change the polarization of macrophages from M1 to M2. This discovery prompted them to co-culture sEVs from Panc-1 cells containing miR-155 and miR-125b-2 with IL-4-induced M2 macrophages. They found higher levels of miR-155 and miR-125b-2 and increased IL-1β/Arg-1 and iNOS/Arg-1 ratios in macrophages. This confirms the shift of the M2 phenotype to M1 phenotype repolarization, which subsequently exerts anti-tumor invasion and metastasis effects (99).
5.4 Mesenchymal stem cell-derived extracellular vesicles
Mesenchymal stem cells (MSCs) are pluripotent stem cells extensively used in clinical settings and have the capacity for various differentiation and self-replenishment (100). MSCs come from various tissues, including the umbilical cord, bone marrow, muscle and adipose tissue (101). MSCs are extremely desired transporters with a significant affinity for inflammatory tissues and tumors. By secreting anti-inflammatory substances through autocrine and paracrine secretion, it can readily localize to the injury site and speed up the healing process of wounds (102, 103). MSCs-EVs exhibit significant advantages over typical MSC cell therapy regarding immunogenicity, safety and stability. They have also significantly progressed in treating certain diseases, such as cancer (104), Covid-19 (105), and neurological disorders (106).
The components carried by EVs, which have anti-tumor effects when released to convey anti-tumor mediators, make stem cell EVs therapy a double-edged sword (107). Chen et al. co-cultured HT-29 colorectal cancer cells and macrophages with human umbilical cord MSCs. Their sEV-borne miR-1827 inhibited SUCNR1 expression, preventing macrophage M2 polarization and colorectal cancer cell growth. The combination of MSCs-sEVs-miR-1827 decreased tumor size, reduced M2 polarization markers in macrophages within tumor tissues, and lowered the number of metastatic tumor lymph nodes in the liver of tumor-bearing mice. Concurrently, they conducted related in vivo experiments in nude mice. Stem cells generated from human umbilical cord mesenchymal stem cells have anti-tumor and anti-tumor liver metastatic properties (108). Liu et al. co-cultured MSCs-sEVs with macrophages, which inhibited the M2 polarization of macrophages. They then introduced the co-culture supernatant to glioma cell lines, which postponed cell proliferation and invasion. Additionally, they injected MSC-sEVs into mice harboring cancers and observed that the tumors grew lighter. When they dissected the tumor nodules in the lung tissue of the mice, they discovered that the MSC-injected mice had fewer lung tumor nodules overall. MSC-sEVs, therefore, possess anti-tumor and metastatic quality (109). However, stem cell-derived EVs can potentially have pro-tumorigenic effects, primarily associated with the source of the cargo or MSCs they transport. For instance, miR-21-5p in MSC EVs from human bone marrow targets PTEN in macrophages, leading to M2 polarization induction suppressing immune function in lung cancer. To inject MSC-EVs overexpressing miR-21-5p into mice using a nude mouse xenograft model, they increased the formation of tumors (110).
According to the studies above, tumor-derived EVs can also have anti-tumor effects following specific treatments. The human body has a variety of normal cells, including immune cells and stem cells, which are sources of EVs that can treat cancers. These many EV sources can be given to target cells more effectively and have the common benefit of not breaking down enzymatically in the body or causing first-pass metabolic effects (111). Unedited tumor-derived EVs are primarily utilized as therapeutic targets to increase therapeutic efficacy since they primarily control immune cells or transmit drug resistance. Tumor-derived EVs with contents altered through electroporation can prevent immunogenic reactions to cancer by directly reprogramming immune cells to produce anti-tumor effects. There are practical issues with this method of engineering EVs, and the host may reject the cargo-loaded EVs. Naturally, anticancer EVs are secreted by many normal immune cells, which have been altered to create innovative tumor vaccines. Nevertheless, EVs immune cells might be cytotoxic.
6 Conclusion
In conclusion, EVs show promise in treating and diagnosing several diseases, including oncological diseases. By transporting a range of genetic material, including DNA, RNA, and proteins, and transferring genetic material between donor and recipient cells, EVs can be involved in cellular communication. According to earlier research, EVs are crucial to the pathophysiology of various illnesses. Because EVs are difficult for enzymes to break down in bodily fluids—blood, urine, amniotic fluid, tear fluid, cerebrospinal fluid, etc.—they are found in a wide range of bodily fluids and are crucial for the early diagnosis of tumors. Urine EVs are early diagnostic markers for prostate cancer, while plasma EVs are specific diagnostic markers for lung adenocarcinoma. Tumor phenotype and metastatic potential are assessed by analyzing genetic material composition in tumor EVs from various samples. By targeting macrophages, the EVs released by tumor cells can change their phenotype, impacting the tumor microenvironment and controlling the tumor’s capacity to spread, invade, and metastasize. Thus, focusing on these EVs makes it easier to create innovative tumor-treating drugs. In terms of therapy, tumor-derived EVs can be altered to include cytokines that can activate macrophages to suppress cancer or even eradicate tumor cells, resulting in pro-inflammatory and anti-tumor outcomes that are summed up in Figure 2.
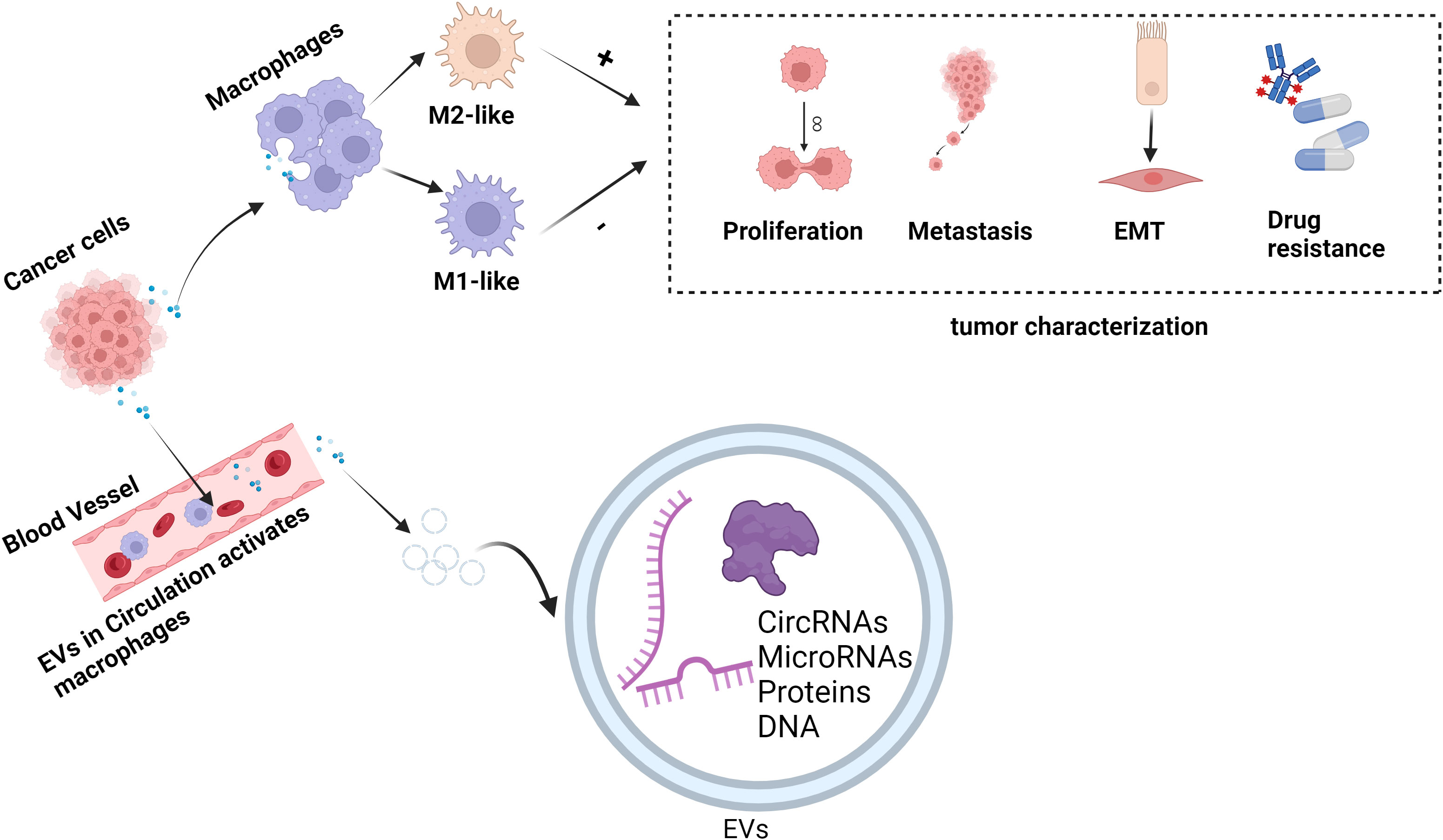
Figure 2 EVs regulate the role of macrophages in tumor pathogenesis. EVs exert anti-tumor or pro-tumor effects by carrying genetic material delivered to macrophages and regulating their polarization to M1 or M2 phenotypes. In the figure, “+” represents promotion and “-” represents inhibition. (This figure was created with Biorender.com.)
Apart from the previously discussed therapeutic approaches, several investigations have been conducted employing engineered EVs. These EVs carry essential anti-tumor drugs, creating a drug delivery system that effectively transports the active ingredients to the appropriate target cells, thus altering the TME for therapeutic effects. Of course, EVs differ from naturally EVs-modified drug carriers in a few ways, the primary ones being their affinity and lower toxicity. Furthermore, efficient anti-tumor vaccines such as dendritic cell-derived EVs have boosted anti-tumor immunity in breast cancer.
Controlling genes or proteins in EVs from tumor-associated cells provides new possibilities for developing anti-tumor solid agents. Macrophages absorb EVs derived from tumor cells and subsequently release growth factors that promote tumor growth. This allows for the artificial modification of EVs to produce cytotoxic chemokines that destroy tumors. Using EVs as a drug delivery system has several benefits, including the ability to bypass being metabolized by the liver, efficiently reach target cells and cross the blood-brain barrier. However, more research is required to increase the affinity, purity, and yield of EVs for this drug delivery method. The main focus of the study is how host immunity is absorbed by and reacts to the drug delivery system’s EVs. Hypersensitivity reactions happen, among other things, when the host develops an immunological response that rejects EVs. Therefore, more research on the routes or mechanisms mediated by EVs remains promising to optimize new tumor vaccines and targeted drugs.
Author contributions
LW: Software, Writing – original draft. WW: Software, Writing – original draft. DH: Supervision, Writing – review & editing. YL: Resources, Writing – original draft. ZL: Writing – review & editing. TZ: Resources, Supervision, Writing – review & editing. XW: Funding acquisition, Supervision, Writing – original draft, Writing – review & editing.
Funding
The author(s) declare financial support was received for the research, authorship, and/or publication of this article. This work was supported by the Science and Technology Plan of Jiangxi Provincial Health Commission (202210863), Municipal level scientific research plan project of Ganzhou Municipal Health Commission (2022-2-62), the Science and Technology Plan of Jiangxi Provincial Administration of Traditional Chinese Medicine (2022B495).
Acknowledgments
We would like to express our profound gratitude to all participants for their invaluable contributions to this research. Additionally, we appreciate the assistance of BioRender.com in creating the figures for this study.
Conflict of interest
The authors declare that the research was conducted in the absence of any commercial or financial relationships that could be construed as a potential conflict of interest.
Publisher’s note
All claims expressed in this article are solely those of the authors and do not necessarily represent those of their affiliated organizations, or those of the publisher, the editors and the reviewers. Any product that may be evaluated in this article, or claim that may be made by its manufacturer, is not guaranteed or endorsed by the publisher.
References
1. Huang D, Rao D, Xi X, Zhang Z, Zhong T. Application of extracellular vesicles proteins in cancer diagnosis. Front Cell Dev Biol. (2022) 10:1007360. doi: 10.3389/fcell.2022.1007360
2. Welsh JA, Goberdhan DCI, O’Driscoll L, Buzas EI, Blenkiron C, Bussolati B, et al. Minimal information for studies of extracellular vesicles (MISEV2023): From basic to advanced approaches. J Extracell Vesicles. (2024) 13:e12404. doi: 10.1002/jev2.12416
3. Ståhl AL, Johansson K, Mossberg M, Kahn R, Karpman D. Exosomes and microvesicles in normal physiology, pathophysiology, and renal diseases. Pediatr Nephrol. (2019) 34:11–30. doi: 10.1007/s00467-017-3816-z
4. Mas-Bargues C, Alique M. Extracellular vesicles as “Very important particles” (VIPs) in aging. Int J Mol Sci. (2023) 24:4250. doi: 10.3390/ijms24044250
5. Wang Y, Li C, Zhao R, Qiu Z, Shen C, Wang Z, et al. CircUbe3a from M2 macrophage-derived small extracellular vesicles mediates myocardial fibrosis after acute myocardial infarction. Theranostics. (2021) 11:6315–33. doi: 10.7150/thno.52843
6. Wang L, Chopp M, Szalad A, Lu X, Zhang Y, Wang X, et al. Exosomes derived from schwann cells ameliorate peripheral neuropathy in type 2 diabetic mice. Diabetes. (2020) 69:749–59. doi: 10.2337/db19-0432
7. Yuan X, Qian N, Ling S, Li Y, Sun W, Li J, et al. Breast cancer exosomes contribute to pre-metastatic niche formation and promote bone metastasis of tumor cells. Theranostics. (2021) 11:1429–45. doi: 10.7150/thno.45351
8. Pantazopoulou M, Lamprokostopoulou A, Karampela DS, Alexaki A, Delis A, Coens A, et al. Differential intracellular trafficking of extracellular vesicles in microglia and astrocytes. Cell Mol Life Sci. (2023) 80:193. doi: 10.1007/s00018-023-04841-5
9. Anderson NM, Simon MC. The tumor microenvironment. Curr Biol. (2020) 30:R921–r925. doi: 10.1016/j.cub.2020.06.081
10. Yunna C, Mengru H, Lei W, Weidong C. Macrophage M1/M2 polarization. Eur J Pharmacol. (2020) 877:173090. doi: 10.1016/j.ejphar.2020.173090
11. Li D, Zhang Q, Li L, Chen K, Yang J, Dixit D, et al. β2-microglobulin maintains glioblastoma stem cells and induces M2-like polarization of tumor-associated macrophages. Cancer Res. (2022) 82:3321–34. doi: 10.1158/0008-5472.CAN-22-0507
12. Han C, Yang Y, Sheng Y, Wang J, Li W, Zhou X, et al. The mechanism of lncRNA-CRNDE in regulating tumour-associated macrophage M2 polarization and promoting tumour angiogenesis. J Cell Mol Med. (2021) 25:4235–47. doi: 10.1111/jcmm.16477
13. Dan H, Liu S, Liu J, Liu D, Yin F, Wei Z, et al. RACK1 promotes cancer progression by increasing the M2/M1 macrophage ratio via the NF-κB pathway in oral squamous cell carcinoma. Mol Oncol. (2020) 14:795–807. doi: 10.1002/1878-0261.12644
14. Bagchi S, Yuan R, Engleman EG. Immune checkpoint inhibitors for the treatment of cancer: clinical impact and mechanisms of response and resistance. Annu Rev Pathol. (2021) 16:223–49. doi: 10.1146/annurev-pathol-042020-042741
15. Kerneur C, Cano CE, Olive D. Major pathways involved in macrophage polarization in cancer. Front Immunol. (2022) 13:1026954. doi: 10.3389/fimmu.2022.1026954
16. Xian X, Cai LL, Li Y, Wang RC, Xu YH, Chen YJ, et al. Neuron secrete exosomes containing miR-9-5p to promote polarization of M1 microglia in depression. J Nanobiotechnology. (2022) 20:122. doi: 10.1186/s12951-022-01332-w
17. Zou T, Gao S, Yu Z, Zhang F, Yao L, Xu M, et al. Salvianolic acid B inhibits RAW264.7 cell polarization towards the M1 phenotype by inhibiting NF-κB and Akt/mTOR pathway activation. Sci Rep. (2022) 12:13857. doi: 10.1038/s41598-022-18246-0
18. Wang S, Liu G, Li Y, Pan Y. Metabolic reprogramming induces macrophage polarization in the tumor microenvironment. Front Immunol. (2022) 13:840029. doi: 10.3389/fimmu.2022.840029
19. Dang B, Gao Q, Zhang L, Zhang J, Cai H, Zhu Y, et al. The glycolysis/HIF-1α axis defines the inflammatory role of IL-4-primed macrophages. Cell Rep. (2023) 42:112471. doi: 10.1016/j.celrep.2023.112471
20. Jiang Z, Lim SO, Yan M, Hsu JL, Yao J, Wei Y, et al. TYRO3 induces anti-PD-1/PD-L1 therapy resistance by limiting innate immunity and tumoral ferroptosis. J Clin Invest. (2021) 131:e139434. doi: 10.1172/JCI139434
21. Zhang M, Wang L, Chen Z. Research progress of extracellular vesicles in type 2 diabetes and its complications. Diabetes Med. (2022) 39:e14865. doi: 10.1111/dme.14865
22. Tkach M, Thalmensi J, Timperi E, Gueguen P, Névo N, Grisard E, et al. Extracellular vesicles from triple negative breast cancer promote pro-inflammatory macrophages associated with better clinical outcome. Proc Natl Acad Sci U.S.A. (2022) 119:e2107394119. doi: 10.1073/pnas.2107394119
23. Novizio N, Belvedere R, Pessolano E, Morello S, Tosco A, Campiglia P, et al. ANXA1 contained in EVs regulates macrophage polarization in tumor microenvironment and promotes pancreatic cancer progression and metastasis. Int J Mol Sci. (2021) 22:11018. doi: 10.3390/ijms222011018
24. Bilotta MT, Antignani A, Fitzgerald DJ. Managing the TME to improve the efficacy of cancer therapy. Front Immunol. (2022) 13:954992. doi: 10.3389/fimmu.2022.954992
25. Gong Z, Li Q, Shi J, Wei J, Li P, Chang CH, et al. Lung fibroblasts facilitate pre-metastatic niche formation by remodeling the local immune microenvironment. Immunity. (2022) 55:1483–1500.e1489.$
26. Peng C, Xu Y, Wu J, Wu D, Zhou L, Xia X. TME-related biomimetic strategies against cancer. Int J Nanomedicine. (2024) 19:109–35. doi: 10.2147/IJN.S441135
27. Poh AR, Ernst M. Targeting macrophages in cancer: from bench to bedside. Front Oncol. (2018) 8:49. doi: 10.3389/fonc.2018.00049
28. Cendrowicz E, Sas Z, Bremer E, Rygiel TP. The role of macrophages in cancer development and therapy. Cancers (Basel). (2021) 13:1946. doi: 10.3390/cancers13081946
29. Cianciaruso C, Beltraminelli T, Duval F, Nassiri S, Hamelin R, Mozes A, et al. Molecular profiling and functional analysis of macrophage-derived tumor extracellular vesicles. Cell Rep. (2019) 27:3062–3080.e3011. doi: 10.1016/j.celrep.2019.05.008
30. Li LG, Yang XX, Xu HZ, Yu TT, Li QR, Hu J, et al. A dihydroartemisinin-loaded nanoreactor motivates anti-cancer immunotherapy by synergy-induced ferroptosis to activate cgas/STING for reprogramming of macrophage. Adv Healthc Mater. (2023) 12:e2301561. doi: 10.1002/adhm.202301561
31. Liu J, Wan M, Lyon CJ, Hu TY. Nanomedicine therapies modulating Macrophage Dysfunction: a potential strategy to attenuate Cytokine Storms in severe infections. Theranostics. (2020) 10:9591–600. doi: 10.7150/thno.47982
32. Boutilier AJ, Elsawa SF. Macrophage polarization states in the tumor microenvironment. Int J Mol Sci. (2021) 22:6995. doi: 10.3390/ijms22136995
33. Liechty C, Hu J, Zhang L, Liechty KW, Xu J. Role of microRNA-21 and its underlying mechanisms in inflammatory responses in diabetic wounds. Int J Mol Sci. (2020) 21:3328. doi: 10.3390/ijms21093328
34. Xu L, Li B, Pi C, Zhu Z, Tao F, Xie K, et al. Targeting CD89 on tumor-associated macrophages overcomes resistance to immune checkpoint blockade. J Immunother Cancer. (2022) 10:e005447. doi: 10.1136/jitc-2022-005447
35. Castro-Dopico T, Fleming A, Dennison TW, Ferdinand JR, Harcourt K, Stewart BJ, et al. GM-CSF calibrates macrophage defense and wound healing programs during intestinal infection and inflammation. Cell Rep. (2020) 32:107857. doi: 10.1016/j.celrep.2020.107857
36. Wang WB, Li JT, Hui Y, Shi J, Wang XY, Yan SG. Combination of pseudoephedrine and emodin ameliorates LPS-induced acute lung injury by regulating macrophage M1/M2 polarization through the VIP/cAMP/PKA pathway. Chin Med. (2022) 17:19. doi: 10.1186/s13020-021-00562-8
37. Zhen H, Hu H, Rong G, Huang X, Tan C, Yu X. VitA or VitD ameliorates bronchopulmonary dysplasia by regulating the balance between M1 and M2 macrophages. BioMed Pharmacother. (2021) 141:111836. doi: 10.1016/j.biopha.2021.111836
38. Li SL, Wang ZM, Xu C, Che FH, Hu XF, Cao R, et al. Liraglutide attenuates hepatic ischemia-reperfusion injury by modulating macrophage polarization. Front Immunol. (2022) 13:869050. doi: 10.3389/fimmu.2022.869050
39. Chen C, Lin Z, Liu W, Hu Q, Wang J, Zhuang X, et al. Emodin accelerates diabetic wound healing by promoting anti-inflammatory macrophage polarization. Eur J Pharmacol. (2022) 936:175329. doi: 10.1016/j.ejphar.2022.175329
40. Hobby ARH, Berretta RM, Eaton DM, Kubo H, Feldsott E, Yang Y, et al. Cortical bone stem cells modify cardiac inflammation after myocardial infarction by inducing a novel macrophage phenotype. Am J Physiol Heart Circ Physiol. (2021) 321:H684–701. doi: 10.1152/ajpheart.00304.2021
41. Zhao S, Si M, Deng X, Wang D, Kong L, Zhang Q. HCV inhibits M2a, M2b and M2c macrophage polarization via HCV core protein engagement with Toll-like receptor 2. Exp Ther Med. (2022) 24:522. doi: 10.3892/etm
42. Gopalakrishnan A, Joseph J, Shirey KA, Keegan AD, Boukhvalova MS, Vogel SN, et al. Protection against influenza-induced Acute Lung Injury (ALI) by enhanced induction of M2a macrophages: possible role of PPARγ/RXR ligands in IL-4-induced M2a macrophage differentiation. Front Immunol. (2022) 13:968336. doi: 10.3389/fimmu.2022.968336
43. Zhang W, Xu W, Xiong S. Blockade of Notch1 signaling alleviates murine lupus via blunting macrophage activation and M2b polarization. J Immunol. (2010) 184:6465–78. doi: 10.4049/jimmunol.0904016
44. Lee J, French B, Morgan T, French SW. The liver is populated by a broad spectrum of markers for macrophages. In alcoholic hepatitis the macrophages are M1 and M2. Exp Mol Pathol. (2014) 96:118–25. doi: 10.1016/j.yexmp.2013.09.004
45. Miki S, Suzuki JI, Takashima M, Ishida M, Kokubo H, Yoshizumi M. S-1-Propenylcysteine promotes IL-10-induced M2c macrophage polarization through prolonged activation of IL-10R/STAT3 signaling. Sci Rep. (2021) 11:22469. doi: 10.1038/s41598-021-01866-3
46. Ordaz-Arias MA, Díaz-Alvarez L, Zúñiga J, Martinez-Sánchez ME, Balderas-Martínez YI. Cyclic attractors are critical for macrophage differentiation, heterogeneity, and plasticity. Front Mol Biosci. (2022) 9:807228. doi: 10.3389/fmolb.2022.807228
47. Hou Y, Shi J, Guo Y, Shi G. Inhibition of angiogenetic macrophages reduces disc degeneration-associated pain. Front Bioeng Biotechnol. (2022) 10:962155. doi: 10.3389/fbioe.2022.962155
48. Pan BT, Johnstone RM. Fate of the transferrin receptor during maturation of sheep reticulocytes in vitro: selective externalization of the receptor. Cell. (1983) 33:967–78. doi: 10.1016/0092-8674(83)90040-5
49. Harding CV, Heuser JE, Stahl PD. Exosomes: looking back three decades and into the future. J Cell Biol. (2013) 200:367–71. doi: 10.1083/jcb.201212113
50. Kalluri R, LeBleu VS. The biology, function, and biomedical applications of exosomes. Science. (2020) 367:eaau6977. doi: 10.1126/science.aau6977
51. Wei J, Wang Z, Han T, Chen J, Ou Y, Wei L, et al. Extracellular vesicle-mediated intercellular and interorgan crosstalk of pancreatic islet in health and diabetes. Front Endocrinol (Lausanne). (2023) 14:1170237. doi: 10.3389/fendo.2023.1170237
52. Lu Y, Wang L, Zhang M, Chen Z. Mesenchymal stem cell-derived small extracellular vesicles: A novel approach for kidney disease treatment. Int J Nanomedicine. (2022) 17:3603–18. doi: 10.2147/IJN.S372254
53. Théry C, Witwer KW, Aikawa E, Alcaraz MJ, Anderson JD, Andriantsitohaina R, et al. Minimal information for studies of extracellular vesicles 2018 (MISEV2018): a position statement of the International Society for Extracellular Vesicles and update of the MISEV2014 guidelines. J Extracell Vesicles. (2018) 7:1535750. doi: 10.1080/20013078.2018.1535750
54. Martellucci S, Orefice NS, Angelucci A, Luce A, Caraglia M, Zappavigna S. Extracellular vesicles: new endogenous shuttles for miRNAs in cancer diagnosis and therapy? Int J Mol Sci. (2020) 21:6486. doi: 10.3390/ijms21186486
55. Ciftci E, Bozbeyoglu N, Gursel I, Korkusuz F, Bakan Misirlioglu F, Korkusuz P. Comparative analysis of magnetically activated cell sorting and ultracentrifugation methods for exosome isolation. PloS One. (2023) 18:e0282238. doi: 10.1371/journal.pone.0282238
56. He T, Zhang Q, Xu P, Tao W, Lin F, Liu R, et al. Extracellular vesicle-circEHD2 promotes the progression of renal cell carcinoma by activating cancer-associated fibroblasts. Mol Cancer. (2023) 22:117. doi: 10.1186/s12943-023-01824-9
57. Xue Y, Huang X, Ou Z, Wu Y, Li Q, Huang X, et al. Isolation and purification of bacterial extracellular vesicles from human feces using density gradient centrifugation. J Vis Exp. (2023) 199:e65574. doi: 10.3791/65574
58. Visan KS, Lobb RJ, Ham S, Lima LG, Palma C, Edna CPZ, et al. Comparative analysis of tangential flow filtration and ultracentrifugation, both combined with subsequent size exclusion chromatography, for the isolation of small extracellular vesicles”. J Extracell Vesicles. (2022) 11:e12266. doi: 10.1002/jev2.12266
59. Prattichizzo F, De Nigris V, Sabbatinelli J, Giuliani A, Castaño C, Párrizas M, et al. CD31(+) extracellular vesicles from patients with type 2 diabetes shuttle a miRNA signature associated with cardiovascular complications. Diabetes. (2021) 70:240–54. doi: 10.2337/db20-0199
60. Weber B, Sturm R, Henrich D, Marzi I, Leppik L. CD44+ and CD31+ extracellular vesicles (EVs) are significantly reduced in polytraumatized patients with hemorrhagic shock - evaluation of their diagnostic and prognostic potential. Front Immunol. (2023) 14:1196241. doi: 10.3389/fimmu.2023.1196241
61. Sánchez-López CM, Manzaneque-López MC, Pérez-Bermúdez P, Soler C, Marcilla A. Characterization and bioactivity of extracellular vesicles isolated from pomegranate. Food Funct. (2022) 13:12870–82. doi: 10.1039/D2FO01806C
62. Dzobo K, Senthebane DA, Dandara C. The tumor microenvironment in tumorigenesis and therapy resistance revisited. Cancers (Basel). (2023) 15:376. doi: 10.3390/cancers15020376
63. Wu K, Lin K, Li X, Yuan X, Xu P, Ni P, et al. Redefining tumor-associated macrophage subpopulations and functions in the tumor microenvironment. Front Immunol. (2020) 11:1731. doi: 10.3389/fimmu.2020.01731
64. Zhu S, Yi M, Wu Y, Dong B, Wu K. Roles of tumor-associated macrophages in tumor progression: implications on therapeutic strategies. Exp Hematol Oncol. (2021) 10:60. doi: 10.1186/s40164-021-00252-z
65. Ireland LV, Mielgo A. Macrophages and fibroblasts, key players in cancer chemoresistance. Front Cell Dev Biol. (2018) 6:131. doi: 10.3389/fcell.2018.00131
66. Dallavalasa S, Beeraka NM, Basavaraju CG, Tulimilli SV, Sadhu SP, Rajesh K, et al. The role of tumor associated macrophages (TAMs) in cancer progression, chemoresistance, angiogenesis and metastasis - current status. Curr Med Chem. (2021) 28:8203–36. doi: 10.2174/0929867328666210720143721
67. Pucci M, Raimondo S, Urzì O, Moschetti M, Di Bella MA, Conigliaro A, et al. Tumor-derived small extracellular vesicles induce pro-inflammatory cytokine expression and PD-L1 regulation in M0 macrophages via IL-6/STAT3 and TLR4 signaling pathways. Int J Mol Sci. (2021) 22:12118. doi: 10.3390/ijms222212118
68. Lecoultre M, Dutoit V, Walker PR. Phagocytic function of tumor-associated macrophages as a key determinant of tumor progression control: a review. J Immunother Cancer. (2020) 8:e001408. doi: 10.1136/jitc-2020-001408
69. Lu C, Shi W, Hu W, Zhao Y, Zhao X, Dong F, et al. Endoplasmic reticulum stress promotes breast cancer cells to release exosomes circ_0001142 and induces M2 polarization of macrophages to regulate tumor progression. Pharmacol Res. (2022) 177:106098. doi: 10.1016/j.phrs.2022.106098
70. Xun J, Du L, Gao R, Shen L, Wang D, Kang L, et al. Cancer-derived exosomal miR-138-5p modulates polarization of tumor-associated macrophages through inhibition of KDM6B. Theranostics. (2021) 11:6847–59. doi: 10.7150/thno.51864
71. Chen WX, Wang DD, Zhu B, Zhu YZ, Zheng L, Feng ZQ, et al. Exosomal miR-222 from adriamycin-resistant MCF-7 breast cancer cells promote macrophages M2 polarization via PTEN/Akt to induce tumor progression. Aging (Albany NY). (2021) 13:10415–30. doi: 10.18632/aging.v13i7
72. Zhou J, Che J, Xu L, Yang W, Zhou W, Zhou C. Tumor-derived extracellular vesicles containing long noncoding RNA PART1 exert oncogenic effect in hepatocellular carcinoma by polarizing macrophages into M2. Dig Liver Dis. (2022) 54:543–53. doi: 10.1016/j.dld.2021.07.005
73. Zongqiang H, Jiapeng C, Yingpeng Z, Chuntao Y, Yiting W, Jiashun Z, et al. Exosomal miR-452-5p induce M2 macrophage polarization to accelerate hepatocellular carcinoma progression by targeting TIMP3. J Immunol Res. (2022) 2022:1032106. doi: 10.1155/2022/1032106
74. Xu Y, Luan G, Liu F, Zhang Y, Li Z, Liu Z, et al. Exosomal miR-200b-3p induce macrophage polarization by regulating transcriptional repressor ZEB1 in hepatocellular carcinoma. Hepatol Int. (2023) 17:889–903. doi: 10.1007/s12072-023-10507-y
75. Yu H, Pan J, Zheng S, Cai D, Luo A, Xia Z, et al. Hepatocellular carcinoma cell-derived exosomal miR-21-5p induces macrophage M2 polarization by targeting rhoB. Int J Mol Sci. (2023) 24:4593. doi: 10.3390/ijms24054593
76. Chen J, Zhang K, Zhi Y, Wu Y, Chen B, Bai J, et al. “Tumor-derived exosomal miR-19b-3p facilitates M2 macrophage polarization and exosomal LINC00273 secretion to promote lung adenocarcinoma metastasis via Hippo pathway”. Clin Transl Med. (2021) 11:e478. doi: 10.1002/ctm2.478
77. Xu L, Wang L, Yang R, Li T, Zhu X. Lung adenocarcinoma cell-derived exosomes promote M2 macrophage polarization through transmission of miR-3153 to activate the JNK signaling pathway. Hum Mol Genet. (2023) 32:2162–76. doi: 10.1093/hmg/ddad052
78. Jin J, Yu G. Hypoxic lung cancer cell-derived exosomal miR-21 mediates macrophage M2 polarization and promotes cancer cell proliferation through targeting IRF1. World J Surg Oncol. (2022) 20:241. doi: 10.1186/s12957-022-02706-y
79. Gu J, Yang S, Wang X, Wu Y, Wei J, Xu J. Hypoxic lung adenocarcinoma-derived exosomal miR-1290 induces M2 macrophage polarization by targeting SOCS3. Cancer Med. (2023) 12:12639–52. doi: 10.1002/cam4.5954
80. Bolcaen J, Kleynhans J, Nair S, Verhoeven J, Goethals I, Sathekge M, et al. “A perspective on the radiopharmaceutical requirements for imaging and therapy of glioblastoma”. Theranostics. (2021) 11:7911–47. doi: 10.7150/thno.56639
81. Louis DN, Perry A, Wesseling P, Brat DJ, Cree IA, Figarella-Branger D, et al. The 2021 WHO classification of tumors of the central nervous system: a summary. Neuro Oncol. (2021) 23:1231–51. doi: 10.1093/neuonc/noab106
82. Le Rhun E, Preusser M, Roth P, Reardon DA, van den Bent M, Wen P, et al. Molecular targeted therapy of glioblastoma. Cancer Treat Rev. (2019) 80:101896. doi: 10.1016/j.ctrv.2019.101896
83. Manda SV, Kataria Y, Tatireddy BR, Ramakrishnan B, Ratnam BG, Lath R, et al. Exosomes as a biomarker platform for detecting epidermal growth factor receptor-positive high-grade gliomas. J Neurosurg. (2018) 128:1091–101. doi: 10.3171/2016.11.JNS161187
84. Zhang C, Zhou Y, Gao Y, Zhu Z, Zeng X, Liang W, et al. Radiated glioblastoma cell-derived exosomal circ_0012381 induce M2 polarization of microglia to promote the growth of glioblastoma by CCL2/CCR2 axis. J Transl Med. (2022) 20:388. doi: 10.1186/s12967-022-03607-0
85. Zhao G, Yu H, Ding L, Wang W, Wang H, Hu Y, et al. microRNA-27a-3p delivered by extracellular vesicles from glioblastoma cells induces M2 macrophage polarization via the EZH1/KDM3A/CTGF axis. Cell Death Discovery. (2022) 8:260. doi: 10.1038/s41420-022-01035-z
86. Zhao S, Mi Y, Guan B, Zheng B, Wei P, Gu Y, et al. Tumor-derived exosomal miR-934 induces macrophage M2 polarization to promote liver metastasis of colorectal cancer. J Hematol Oncol. (2020) 13:156. doi: 10.1186/s13045-020-00991-2
87. Pei W, Wei K, Wu Y, Qiu Q, Zhu H, Mao L, et al. Colorectal cancer tumor cell-derived exosomal miR-203a-3p promotes CRC metastasis by targeting PTEN-induced macrophage polarization. Gene. (2023) 885:147692. doi: 10.1016/j.gene.2023.147692
88. Wang X, Zhang H, Yang H, Bai M, Ning T, Deng T, et al. Exosome-delivered circRNA promotes glycolysis to induce chemoresistance through the miR-122-PKM2 axis in colorectal cancer. Mol Oncol. (2020) 14:539–55. doi: 10.1002/1878-0261.12629
89. Yang C, Dou R, Wei C, Liu K, Shi D, Zhang C, et al. Tumor-derived exosomal microRNA-106b-5p activates EMT-cancer cell and M2-subtype TAM interaction to facilitate CRC metastasis. Mol Ther. (2021) 29:2088–107. doi: 10.1016/j.ymthe.2021.02.006
90. Wang X, Luo G, Zhang K, Cao J, Huang C, Jiang T, et al. Hypoxic Tumor-Derived Exosomal miR-301a Mediates M2 Macrophage Polarization via PTEN/PI3Kγ to Promote Pancreatic Cancer Metastasis. Cancer Res. (2018) 78:4586–98. doi: 10.1158/0008-5472.CAN-17-3841
91. Wang S, Gao Y. Pancreatic cancer cell-derived microRNA-155-5p-containing extracellular vesicles promote immune evasion by triggering EHF-dependent activation of Akt/NF-κB signaling pathway. Int Immunopharmacol. (2021) 100:107990. doi: 10.1016/j.intimp.2021.107990
92. Shan K, Feng N, Cui J, Wang S, Qu H, Fu G, et al. Resolvin D1 and D2 inhibit tumour growth and inflammation via modulating macrophage polarization. J Cell Mol Med. (2020) 24:8045–56. doi: 10.1111/jcmm.15436
93. Li R, Li D, Wang H, Chen K, Wang S, Xu J, et al. Exosomes from adipose-derived stem cells regulate M1/M2 macrophage phenotypic polarization to promote bone healing via miR-451a/MIF. Stem Cell Res Ther. (2022) 13:149. doi: 10.1186/s13287-022-02823-1
94. Kamerkar S, Leng C, Burenkova O, Jang SC, McCoy C, Zhang K, et al. Exosome-mediated genetic reprogramming of tumor-associated macrophages by exoASO-STAT6 leads to potent monotherapy antitumor activity. Sci Adv. (2022) 8:eabj7002. doi: 10.1126/sciadv.abj7002
95. Moradi-Chaleshtori M, Bandehpour M, Heidari N, Mohammadi-Yeganeh S, Mahmoud Hashemi S. Exosome-mediated miR-33 transfer induces M1 polarization in mouse macrophages and exerts antitumor effect in 4T1 breast cancer cell line. Int Immunopharmacol. (2021) 90:107198. doi: 10.1016/j.intimp.2020.107198
96. Moradi-Chaleshtori M, Bandehpour M, Soudi S, Mohammadi-Yeganeh S, Hashemi SM. In vitro and in vivo evaluation of anti-tumoral effect of M1 phenotype induction in macrophages by miR-130 and miR-33 containing exosomes. Cancer Immunol Immunother. (2021) 70:1323–39. doi: 10.1007/s00262-020-02762-x
97. Ghalavand M, Moradi-Chaleshtori M, Dorostkar R, Mohammadi-Yeganeh S, Hashemi SM. Exosomes derived from rapamycin-treated 4T1 breast cancer cells induced polarization of macrophages to M1 phenotype. Biotechnol Appl Biochem. (2023) 70:1754–71. doi: 10.1002/bab.2473
98. Stary V, Wolf B, Unterleuthner D, List J, Talic M, Laengle J, et al. Short-course radiotherapy promotes pro-inflammatory macrophages via extracellular vesicles in human rectal cancer. J Immunother Cancer. (2020) 8:e000667. doi: 10.1136/jitc-2020-000667
99. Su MJ, Aldawsari H, Amiji M. Pancreatic cancer cell exosome-mediated macrophage reprogramming and the role of microRNAs 155 and 125b2 transfection using nanoparticle delivery systems. Sci Rep. (2016) 6:30110. doi: 10.1038/srep30110
100. Batsali AK, Georgopoulou A, Mavroudi I, Matheakakis A, Pontikoglou CG, Papadaki HA. The role of bone marrow mesenchymal stem cell derived extracellular vesicles (MSC-EVs) in normal and abnormal hematopoiesis and their therapeutic potential. J Clin Med. (2020) 9:856. doi: 10.3390/jcm9030856
101. Nicodemou A, Bernátová S, Čeháková M, Danišovič Ľ. Emerging roles of mesenchymal stem/stromal-cell-derived extracellular vesicles in cancer therapy. Pharmaceutics. (2023) 15:1453. doi: 10.3390/pharmaceutics15051453
102. Sid-Otmane C, Perrault LP, Ly HQ. Mesenchymal stem cell mediates cardiac repair through autocrine, paracrine and endocrine axes. J Transl Med. (2020) 18:336. doi: 10.1186/s12967-020-02504-8
103. He J, Zhang N, Zhu Y, Jin R, Wu F. MSC spheroids-loaded collagen hydrogels simultaneously promote neuronal differentiation and suppress inflammatory reaction through PI3K-Akt signaling pathway. Biomaterials. (2021) 265:120448. doi: 10.1016/j.biomaterials.2020.120448
104. Cortés-Morales VA, Chávez-Sánchez L, Rocha-Zavaleta L, Espíndola-Garibay S, Monroy-García A, Castro-Manrreza ME, et al. Mesenchymal stem/stromal cells derived from cervical cancer promote M2 macrophage polarization. Cells. (2023) 12:1047. doi: 10.3390/cells12071047
105. Akbari A, Rezaie J. Potential therapeutic application of mesenchymal stem cell-derived exosomes in SARS-CoV-2 pneumonia. Stem Cell Res Ther. (2020) 11:356. doi: 10.1186/s13287-020-01866-6
106. Kang X, Jiang L, Chen X, Wang X, Gu S, Wang J, et al. Exosomes derived from hypoxic bone marrow mesenchymal stem cells rescue OGD-induced injury in neural cells by suppressing NLRP3 inflammasome-mediated pyroptosis. Exp Cell Res. (2021) 405:112635. doi: 10.1016/j.yexcr.2021.112635
107. Guo G, Tan Z, Liu Y, Shi F, She J. The therapeutic potential of stem cell-derived exosomes in the ulcerative colitis and colorectal cancer. Stem Cell Res Ther. (2022) 13:138. doi: 10.1186/s13287-022-02811-5
108. Chen J, Li Z, Yue C, Ma J, Cao L, Lin J, et al. “Human umbilical cord mesenchymal stem cell-derived exosomes carrying miR-1827 downregulate SUCNR1 to inhibit macrophage M2 polarization and prevent colorectal liver metastasis. Apoptosis. (2023) 28:549–65. doi: 10.1007/s10495-022-01798-x
109. Liu L, Cheng M, Zhang T, Chen Y, Wu Y, Wang Q. Mesenchymal stem cell-derived extracellular vesicles prevent glioma by blocking M2 polarization of macrophages through a miR-744-5p/TGFB1-dependent mechanism. Cell Biol Toxicol. (2022) 38:649–65. doi: 10.1007/s10565-021-09652-7
110. Ren W, Hou J, Yang C, Wang H, Wu S, Wu Y, et al. Extracellular vesicles secreted by hypoxia pre-challenged mesenchymal stem cells promote non-small cell lung cancer cell growth and mobility as well as macrophage M2 polarization via miR-21-5p delivery. J Exp Clin Cancer Res. (2019) 38:62. doi: 10.1186/s13046-019-1027-0
Keywords: extracellular vesicles, macrophages, M1, M2, colorectal cancer, glioblastoma
Citation: Wang L, Wang W, Hu D, Liang Y, Liu Z, Zhong T and Wang X (2024) Tumor-derived extracellular vesicles regulate macrophage polarization: role and therapeutic perspectives. Front. Immunol. 15:1346587. doi: 10.3389/fimmu.2024.1346587
Received: 29 November 2023; Accepted: 03 April 2024;
Published: 16 April 2024.
Edited by:
Zhirui Zeng, Guizhou Medical University, ChinaReviewed by:
Byungheon Lee, Kyungpook National University, Republic of KoreaLuciana Dini, Sapienza University of Rome, Italy
Copyright © 2024 Wang, Wang, Hu, Liang, Liu, Zhong and Wang. This is an open-access article distributed under the terms of the Creative Commons Attribution License (CC BY). The use, distribution or reproduction in other forums is permitted, provided the original author(s) and the copyright owner(s) are credited and that the original publication in this journal is cited, in accordance with accepted academic practice. No use, distribution or reproduction is permitted which does not comply with these terms.
*Correspondence: Tianyu Zhong, emhvbmd0aWFueXVAZ21haWwuY29t; Xiaoling Wang, d2FuZ3hpYW9saW5nMUBnbXUuY24=
†These authors have contributed equally to this work and share first authorship