- 1Singapore Immunology Network (SIgN), Agency for Science, Technology and Research (ASTAR), Singapore, Singapore
- 2Lymphoma Translational Research Laboratory, Division of Cellular and Molecular Research, National Cancer Centre Singapore, Singapore, Singapore
- 3Oncology-Academic Clinical Programme (ONCO-ACP), Duke-National University of Singapore (NUS) Medical School, Singapore, Singapore
- 4Division of Medical Oncology, National Cancer Centre Singapore, Singapore, Singapore
- 5Clinic for Lymphoma, Myeloma and Blood Disorders, Mount Elizabeth Hospital Novena Specialist Centre, Singapore, Singapore
- 6Cancer Science Institute of Singapore, National University of Singapore, Singapore, Singapore
- 7Department of Medicine, Yong Loo Lin School of Medicine, National University of Singapore, Singapore, Singapore
- 8Department of Hematology-Oncology, National University Cancer Institute of Singapore, National University Health System, Singapore, Singapore
- 9Director’s Office, National Cancer Centre Singapore, Singapore, Singapore
- 10Office of Education, Duke-National University of Singapore (NUS) Medical School, Singapore, Singapore
- 11Cancer and Stem Cell Biology, Duke-National University of Singapore (NUS) Graduate Medical School, Singapore, Singapore
- 12School of Biological Sciences, Nanyang Technological University, Singapore, Singapore
Introduction: Natural killer/T cell lymphoma (NKTL) is an aggressive malignancy associated with poor prognosis. This is largely due to limited treatment options, especially for relapsed patients. Immunotherapies like immune checkpoint inhibitors (ICI) and anti-CD38 therapies have shown promising but variable clinical efficacies. Combining these therapies has been suggested to enhance efficacy.
Methods: We conducted a case study on a relapsed NKTL patient treated sequentially with anti-CD38 followed by ICI (anti-PD1) using cytometry analyses.
Results and Discussion: Our analysis showed an expected depletion of peripheral CD38+ B cells following anti-CD38 treatment. Further analysis indicated that circulating anti-CD38 retained their function for up to 13 weeks post-administration. Anti-PD1 treatment triggered re-activation and upregulation of CD38 on the T cells. Consequently, these anti-PD1-activated T cells were depleted by residual circulating anti-CD38, rendering the ICI treatment ineffective. Finally, a meta-analysis confirmed this counterproductive effect, showing a reduced efficacy in patients undergoing combination therapy. In conclusion, our findings demonstrate that sequential anti-CD38 followed by anti-PD1 therapy leads to a counterproductive outcome in NKTL patients. This suggests that the treatment sequence is antithetic and warrants re-evaluation for optimizing cancer immunotherapy strategies.
1 Introduction
NK/T-cell lymphoma (NKTL) is a rare and aggressive form of an Epstein Barr Virus (EBV)-associated cancer with a predilection for Asian and South American populations (1). Early stage NKTL patients are typically treated with a combination of radiotherapy and chemotherapy, while L-asparaginase based regimens such as SMILE (steroid, methotrexate, ifosfamide, L-asparaginase and etoposide) are given at late stages or relapsed patients (2). However, these chemotherapy regimens typically give rise to adverse events such as high grade lymphopenia and increased infection risks, leaving SMILE refractory NKTL patients with a poor prognosis (3).
In recent years, advances in immunotherapy provide a new avenue for the treatment of cancer patients. By harnessing the body’s natural defense to fight cancer cells, this approach has proven to be effective for the treatment of many cancers (4–8). One of these strategies is to destroy cancer cells by injecting depleting antibodies directed against tumor-specific surface markers. Daratumumab (anti-CD38) was found to effectively deplete multiple myeloma (MM) cells as these cells express high levels of CD38 (9). A case report showed that NKTL tumors also express CD38 and suggested that Daratumumab monotherapy may be efficacious in NKTL patients (10). A recent clinical trial however reported only limited clinical benefit for NKTL patients, casting some doubt on the usefulness of Daratumumab for this type of cancer (10, 11). More importantly, CD38 expression is not restricted to the transformed tissue. It is present on various immune subsets including B cells (12) and, as a common activation marker on NK cells (13, 14) and T cells (15–17). Thus, we hypothesize that the use of Daratumumab may thus also have some compromising effect on the immune system.
Another promising approach appears to be immune checkpoint inhibition (ICI). ICI therapies disrupt the sensing of inhibitory receptor signals delivered from the transformed tissue by tumor-specific T or NK cells to reinvigorate their cytotoxic capacity (18). Currently the most promising target is the PD1/PDL1 axis. PD1 is an immunoinhibitory molecule expressed on activated lymphocytes including CD8+ T cells (19). The ligand (PDL1) is commonly found on antigen-presenting cells and often expressed by tumor cells (20, 21). Ligation of PD1 with PDL1 inhibits T cell cytotoxicity (21, 22) and the use of anti-PD1 perturbing this receptor/ligand interaction can unleash the suppressed cytotoxicity of T cells to kill the tumor cells (23). In patients with relapsed/refractory NKTL the efficacy of ICI therapy was demonstrated with anti-PD1 antibodies such as Pembrolizumab or Nivolumab, which reportedly achieved complete response (CR) in around 30-70% of the patients (24–28).
In spite of the apparent effectiveness of ICI-based immunotherapies, they often work only in a subset of patients (24–27). A common strategy to increase treatment efficiency is through the combination with other (immune-) therapies to synergize their effects (29). For NKTL, it had been suggested that the combined use of anti-PD1 and anti-CD38 therapy may help to improve the low efficacy rates of these monotherapies (30). However, here we show that a combination of these two treatments is in fact counterproductive: the residual depleting anti-CD38 antibody (Daratumumab) effectively eliminates the effector CD8+ T cell populations that were (re-)activated by the anti-PD1 treatment and was associated with a strong upregulation of CD38. The counteracting effect of Daratumumab on checkpoint inhibition was evident more than 6 weeks after completing Daratumumab therapy. Residual levels of Daratumumab were still sufficient to deplete all effector memory CD8+ T cells, typically induced by the anti-PD1 treatment.
2 Materials and methods
2.1 Ethics approval and consent to participate
Fresh blood samples were obtained from healthy volunteers and patients in accordance to the Helsinki declaration. Healthy donor samples were collected under the SingHealth Centralised Institutional Review Board (CIRB Ref: 2017/2806) and HSA “Residual Blood Samples for Research” project titled “Blood biomarkers of immune-related diseases” (Ref: #201306-05). Patient samples were obtained under SingHealth CIRB Ref: 2004/407/F. Written informed consent was obtained from all donors prior to sample collection.
2.2 Search strategy and selection criteria for meta-analysis
The databases used to retrieve relevant articles include National Center for Biotechnology Information (NCBI) Pubmed and ClinicalTrials.gov. The keywords used are “anti-CD38 therapy, anti-PD1 therapy, anti-PDL1 therapy, combination therapy trials, cancer trials, Daratumumab, Pembrolizumab and Nivolumab”. All studies with cancer patient receiving either anti-PDL1/anti-PD1 alone or in combination with anti-CD38 were included. Subsequently, trials in which patients received anti-CD38 or ICI in combination with another agent were excluded. Incomplete trials or trials with insufficient information were also not included. Anti-CD38 monotherapy studies which consists of only multiple myeloma patients were excluded from analysis since CD38 acts directly on the myeloma cells and not within the scope of this study (9). The Preferred Reporting Items for Systemic Reviews and Meta-Analyses (PRISMA) flow diagram can be found in Supplementary Figure 6.
2.3 Quantitation of genomic EBV DNA levels in patient plasma samples
Patient plasma samples were split into two fractions and treated either with Buffer RDD (Qiagen) or DNAse I (Qiagen). DNA detected from the DNAse I-treated fraction represents only virion-encapsidated DNA, while mock-treated DNA (by Buffer RDD) comprises the total DNA in the patient’s plasma (cell-free host DNA, virion-encapsidated DNA and free-floating viral DNA). An equal volume of cold phenol-saturated Tris-EDTA (10 mM Tris, pH 8.0 with 1 mM EDTA) was added to each tube followed by vigorous shaking. Samples were then centrifuged at 13,000 relative centrifugal force (rcf) at 4°C for 15 mins. An additional 100 μL of Buffer EB (Qiagen) was gently added to facilitate aspiration. The aqueous phase of DNA was extracted and stored at -20°C until use. Standard ethanol precipitation using glycogen as carrier was performed to concentrate the DNA. Buffer EB (Qiagen) was used to resuspend the purified DNA. Virus DNA quantification was performed in accordance to published qPCR protocol using 1 μL template DNA (31). Standard curve was generated using DNA from the Namalwa EBV-positive Burkitt’s lymphoma cell line (32).
2.4 Anti-EBV viral capsid antigen IgG and total IgG quantification
Quantification of total IgG was performed according to manufacturer’s protocol using Human IgG ELISA quantification set (Bethyl Laboratories) with heat inactivated human plasma samples diluted 1:50,000. Quantification of anti-EBV VCA IgG was performed according to manufacturer’s protocol using EBV-VCA IgG ELISA kit (Calbiotech) using heat inactivated human plasma samples.
2.5 Competitive anti-CD38 staining
NK92 cells were first stained using the Fixable Aqua Dead Cell Kit (Thermofisher), followed by incubation with either with titrated amounts of Darzalex (Daratumumab) (Johnson & Johnson), or plasma from patients or healthy control and incubated for 10 mins at room temperature. The cells were then washed using MACS buffer (0.5% BSA + 2mM EDTA in PBS) followed by staining with different clones of anti-CD38: LS198-4-2 (Beckman Coulter), JK36 (Beckman Coulter) and HIT2 (BD Biosciences) for 10 mins in the dark at room temperature. The cells were then washed and analyzed using BD LSRFortessa™ cell analyzer. Data analyses were performed using Flowjo V10.5.3 (BD).
2.6 Immunophenotyping by flow cytometry
PBMCs were isolated using Ficoll-Paque density gradient centrifugation, frozen in freezing medium [10% DMSO, 90% fetal bovine serum (FBS)] and stored at -80°C until use. For staining, PBMCs were thawed using complete RPMI (RPMI+10%FBS) and washed with PBS. The cells were first stained using Fixable Aqua Dead Cell Kit (Thermofisher), followed by staining with anti-CD3 (UCHT1) (Biolegend), anti-CD4 (RPA-T4) (BD Biosciences), anti-CD8 (SK1) (Biolegend), anti-CD19 (HIB19) (BD Biosciences), anti-CD27 (O323) (Biolegend), anti-CD56 (B159) (BD Biosciences), anti-CD45RA (2H4) (Beckman Coulter), anti-CD38 (HIT2) (BD Biosciences) and anti-CD38 (JK36) (Beckman Coulter). The cells were washed and analyzed using BD LSRFortessa™ cell analyzer. Data analyses were performed using Flowjo V10.5.3 (BD)
2.7 Immunophenotyping by mass cytometry
Frozen PBMCs were thawed using complete RPMI (RPMI+10%FBS) and washed with PBS. The cells were then treated with cisplatin for 5 mins, followed by incubation with metal-conjugated surface antibodies cocktail (Table 1) for 30 mins at 37°C. Cells were washed twice with CyFACS buffer (PBS with 4% FBS, 0.05% sodium azide), followed by primary antibody staining for 30 mins on ice. Subsequently, cells were washed twice with CyFACS buffer, followed by permeabilization and fixation with Foxp3 fix/perm solution (eBioscience) for 30 mins on ice. Following this, cells were washed with permeabilization buffer (Biolegend) and then stained with intracellular antibody cocktail (Table 1) for 30 mins on ice, wash with Biolegend permeabilization buffer then stained with metal-conjugated streptavidin for 10 mins on ice. Finally, cells were washed with PBS and fixed overnight using 2% PFA made in PBS. The next day, cells were barcoded and stained with Cell-ID Intercalator-Ir (Fluidigm) in PBS for 20 mins at room temperature. Cells were washed twice with CyFACS buffer followed by a final wash using MiliQ water and passed through size filter. Filtered cells were analyzed using Helios mass cytometer (Fluidigm) with CyTOF software version 7.0.8493. Data analyses were performed using Flowjo V10.5.3 (BD) and Cytofkit2 (33).
2.8 Complement dependent cytotoxicity
NK92 cells were incubated with either Daratumumab, plasma from healthy control (HC) or combination therapy patient for 10 mins at room temperature. The cells were then washed using MACS buffer followed by incubating with serum from HC for 3hours at 37°C. Heat inactivated serum was included as negative control. After incubation, the cells were stained with Fixable Aqua Dead Cell Kit (Thermofisher). The cells were then washed and analyzed using BD LSRFortessa™ cell analyzer. Data analyses were performed using Flowjo V10.5.3 (BD)
3 Results
3.1 Treatment regime of the NKTL patient receiving combination therapy
To study the potential outcome of combining anti-CD38 (Daratumumab) and ICI treatments, we analysed the blood samples from a relapsed/refractory patient with NKTL who was treated sequentially with Daratumumab followed by ICI (combination therapy patient). The patient did not respond to conventional SMILE (steroid, methotrexate, ifosfamide, L-asparaginase and etoposide) therapy. He was then enrolled in a Daratumumab trial (ClinicalTrials.gov ID: NCT02927925) where he received 2 cycles of 16mg/kg Daratumumab (Jassen) over a period of 4 weeks (Figure 1A). As NKTL cells release EBV DNA, the tumor burden could be directly associated with the amount of EBV DNA circulating in the blood (34). The increasing levels of circulating EBV-DNA levels indicated that the patient did not respond to the Daratumumab treatment (Figure 1B) and thus the treatment was stopped. Three weeks post Daratumumab treatment (3wpD), the patient was started on 3 cycles of off-label escalating dose of anti-PD1 (Nivolumab) (Bristol Myers Squibb). Patient was given 140mg (first cycle), 180mg (second cycle) and 200mg (third cycle), with 2 weeks interval between each cycle. However, the patient also responded poorly to this treatment (Figure 1B). He developed secondary leukocytosis around 7 wpD treatment before passing on at 14wpD (Figures 1A, B). Blood samples were collected at 2wpD (tp0), 7wpD (tp1) and 13wpD (tp2), with the latter two being taken 4 and 10 weeks post anti-PD1 (wpP), respectively (Figure 1).
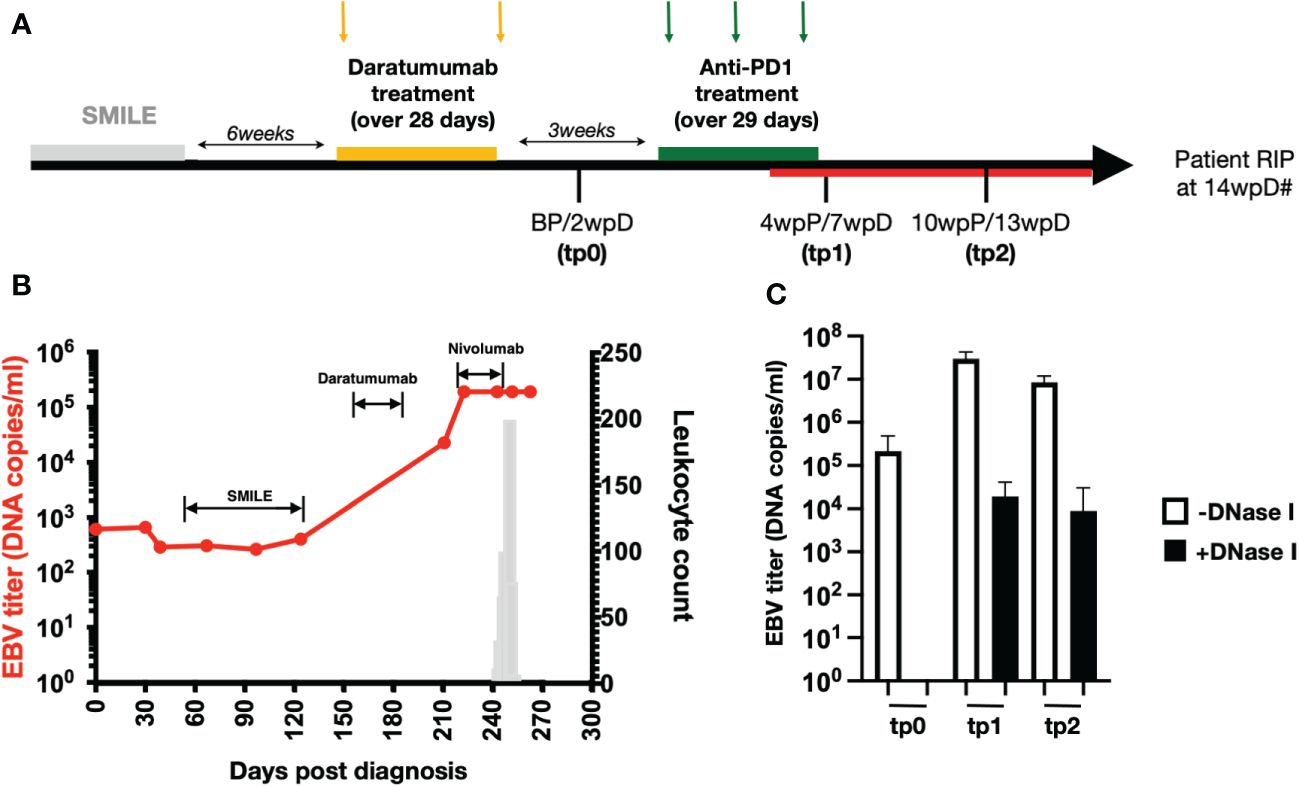
Figure 1 High levels of EBV load in NKTL patient who underwent combination therapy (A) Treatment timeline of the relapsing NKTL patient that underwent Daratumumab and anti-PD1 combination therapy (combination therapy patient). Patient was given SMILE therapy but did not respond to treatment. Six weeks after the end of SMILE therapy, patient was given 2 doses of Daratumumab over a period of 28 days. Two weeks after the last Daratumumab (2wpD) and before anti-PD1 (BP) treatment, plasma and PBMCs were collected (tp0). At 3wpD, patient was put on 3 doses of anti-PD1 (Nivolumab) treatment, spaced 15 days apart over a period of 29 days. Plasma and PBMCs were collected again at 7wpD (tp1) and 13wpD (tp2) respectively. Tp1 and tp2 also coincide with 4 weeks and 10 weeks post start of anti-PD1 (wpP) treatment. Combination therapy patient developed transient leukocytosis (indicated by red line on timeline) during anti-PD1 treatment. Hash (#) indicates demise of combination therapy patient at 14wpD. (B) Line graph (in red) showing plasma EBV load of the patient (left y-axis) over time after she was diagnosed with stage IVB NKTL. Labels on the chart indicate the treatment administered to the patient during that period as indicated in (A). Bar chart (in grey) shows leukocyte count (right y-axis) in the patient as she develops secondary leukemia during the course of the disease. (C) Bar chart showing plasma EBV load in the patient at BP, tp1 and tp2 with and without DNaseI digestion prior to DNA extraction.
Both NKTL patient 1 and 2 were ICI (Pembrolizumab) (Merck) treatment responder and included as reference for ICI monotherapy.
3.2 Increased EBV viremia and reduced levels of EBV-specific IgG
Anti-PD1 treatment did not reduce the level of circulating EBV DNA, indicating that tumor burden did not decrease (Figures 1B, C). Resistance to DNase digestion indicated that a fraction of the measured viral DNA was derived from virions, suggesting re-activation of the associated-virus from the lymphoma (Figure 1C). Analysis of the plasma EBV virus capsid antigen (VCA)-specific IgG levels further showed that the patient undergoing combination therapy had only negligible levels of EBV-specific IgG in his plasma as compared to other NKTL patients who are not treated with Daratumumab, indicating a compromised humoral anti-EBV response (Supplementary Figure 1).
3.3 Effective in vivo depletion of CD38+ lymphocytes by Daratumumab
Daratumumab is a depleting antibody known to eliminate CD38-expressing B cells (35). This subset includes plasma cells, plasmablasts and memory B cells (36). As expected, we noted a complete absence of CD38+ B cells in the patient after the Daratumumab treatment (Figure 2A) (12). In order to confirm the observation is not due to epitope competition between anti-CD38 staining antibody (HIT2 clone) and Daratumumab bound on the cell surface, we repeated the staining using JK36, an anti-CD38 nanobody reported not to share a common epitope with Daratumumab (Supplementary Figure 2) (37, 38). Flow cytometry analysis confirmed that the CD38+ B cell population is indeed missing (Supplementary Figure 2), which may explain the compromised humoral anti-viral response by the patient (Supplementary Figure 1).
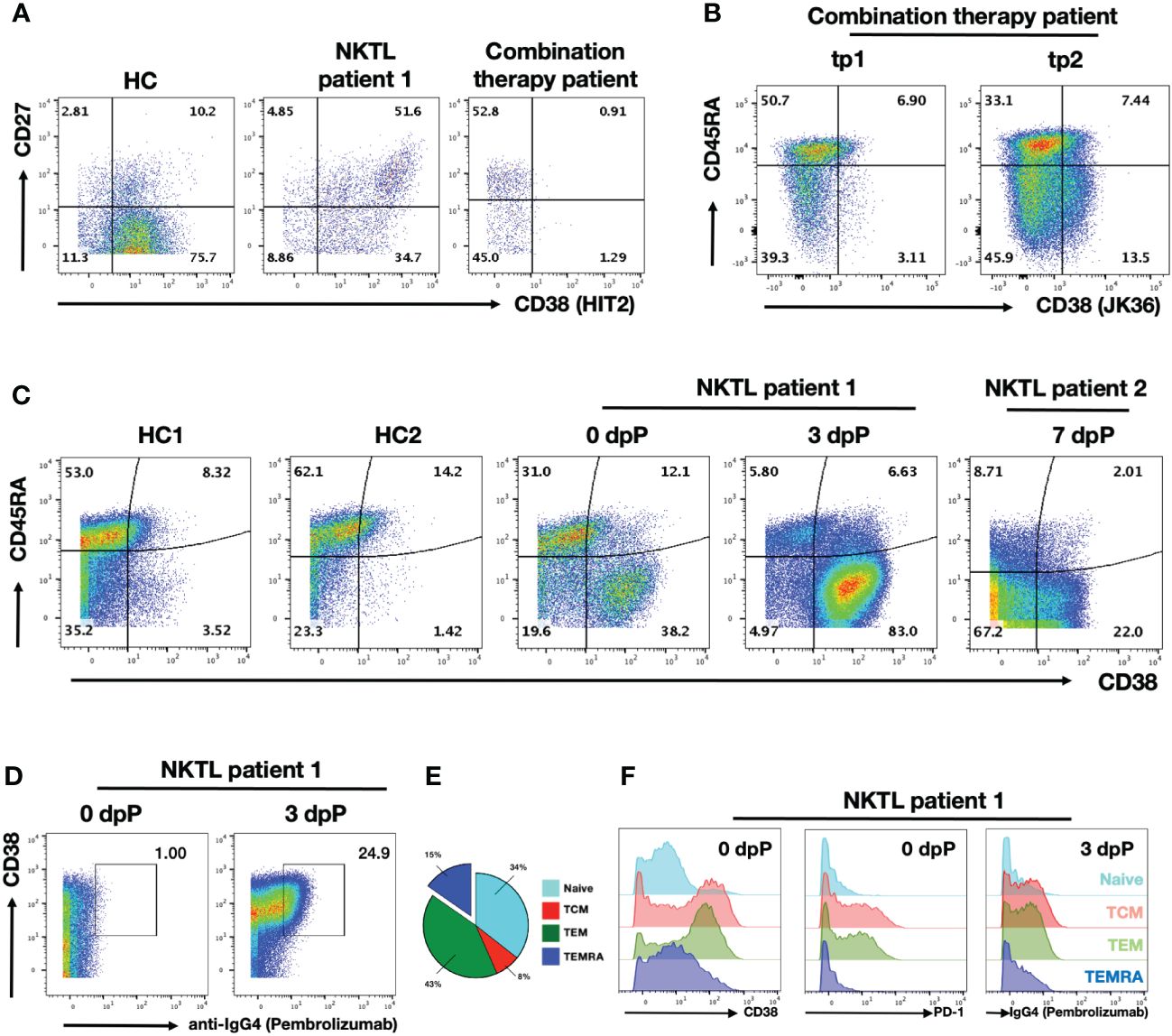
Figure 2 Daratumumab deplete B and activated T cells in NKTL patient undergoing combination therapy PBMC samples were obtained from healthy control (HC), NKTL patient control (NKTL patient 1) and combination therapy patient were analysed using flow cytometry. (A) Scatterplots showing CD27 and CD38 (HIT2 clone) expression on gated B cell subsets. Numbers in the scatterplot show the percentage of respective population within each quadrant of total B cells. (B) Scatterplots showing CD45RA and CD38 (JK36 clone) expressing CD8+ T cells in combination therapy patient from tp1 and tp2. Numbers in the scatterplot show the percentage of respective population within each quadrant of total CD8+ T cells. (C) Scatterplots showing CD45RA and CD38 (HIT2 clone) expressing CD8+ T cells in HCs and anti-PD1 treated NKTL patients (NKTL patient 1 samples obtained on 0dpP and 3dpP; NKTL patient 2 on 7dpP). Numbers in the scatterplot show the percentage of respective population within each quadrant of total CD8+ T cells. (D) Scatterplots showing CD38 and anti-IgG4 staining on CD8+ T cells in anti-PD1 treated NKTL patient 1 from 0dpP and 3dpP. Anti-IgG4 is used as a proxy to detect for binding of Pembrolizumab on the cells. Boxed up region shows proportion of IgG4+ cells. (E) Pie chart showing segregation of CD8+ T cell subsets from NKTL patient 1 into naïve (CCR7+CD45RA+), TCM (CCR7+CD45RA-), TEM (CCR7-CD45RA-) and TEMRA (CCR7-CD45RA+) based on their CCR7 and CD45RA expression prior to anti-PD1 treatment. (F) Histogram plots showing CD38 (left) and PD1 (middle) expression the respective on CD8+ T cells subsets in NKTL patient 1 prior to anti-PD1 treatment. Histogram plots (right) showing anti-IgG4 staining (targeting Pembrolizumab) on the respective CD8+ T cell subsets 3 days after anti-PD1 treatment.
While depletion of B cells and loss of antibodies is a reported side-effect of Daratumumab treatment, we also observed strong depletion of CD38+ CD8+ T cell subsets that was previously unreported (Figure 2B) (35). Interestingly, earlier report of Daratumumab depletion on T cell subsets was limited to only CD38+ Tregs (13). Anti-PD1 treatment aims to reinvigorate exhausted tumor-specific CD8+ T cells and this (re-)activation apparently triggers a substantial increase in the proportion of CD38+ CD8+ T cells (39, 40). We detected less than 10% of CD38+ CD8+ T cells at tp1 (7wpD/4wpP) (Figure 2B). The fraction of CD38+ CD8+ T cells started increasing at tp2 (10wpD/13wpP), indicating that the depleting capacity of the anti-CD38 antibody was diminishing (Figure 2B). Notably, the analysis of anti-PD1-treated NKTL patient revealed that the (re-)activation of T cells by anti-PD1 is associated with a strong upregulation of CD38 (Figure 2C). While a substantial fraction of CD38+ CD8+ T cells were already detected before the start of the therapy (day 0), the proportion of CD38+ CD8+ T cells increased substantially from 50% to 90% at day 3 after anti-PD1 treatment (Pembrolizumab) in NKTL patient 2 (Figure 2C). A similar activation was also observed in NKTL patient 1 at 7dpP (Figure 2C). Based on the CD45RA negative (CD45RA-) phenotype, these CD38+ activated cells mostly represented memory T cells (Figure 2C).
In order to confirm that these CD38+ T cells are re-activated by the binding of Pembrolizumab, we stained the cells using anti-IgG4. As Pembrolizumab is an IgG4 isotype, anti-IgG4 staining serves as a proxy for Pembrolizumab binding (41). Anti-IgG4 stained almost exclusively the CD38+ CD8+ T cells that were sampled 3 days after Pembrolizumab administration, confirming that anti-PD1 therapy is mediated through this subset (Figure 2D).
As it was previously noted that CD38+ CD8+ T cell subsets are mainly CD45RA-, we further segregated the CD8+ T cells into 4 major subsets to determine which subset Pembrolizumab is binding to. Separation of the CD8+ T cell populations into the 4 major subsets of naïve T cells (CCR7+CD45RA+), effector memory T cells (TEM, CCR7-CD45RA-), central memory T cells (TCM, CCR7+CD45RA-) and TEM expressing RA cells (TEMRA, CCR7-CD45RA+) subsets indicated that TEM and TCM comprise about half of the total CD8+ T cell population in NKTL patient 1 (Figure 2E) (42). Further characterization of these subsets confirmed that a large fraction TCM and TEM CD8+ T cells express CD38 (Figure 2F, left panel). The two subsets also expressed the highest levels of PD1 prior to the Pembrolizumab treatment indicating that these two subsets are the primary targets of ICI treatment (Figure 2F, middle panel). This was confirmed by the strong anti-IgG4 staining on both TCM and TEM 3 days after anti-PD1 treatment (Figure 2F, right panel). Notably, there were only 15% TEMRA CD8+ T cells in this patient.
3.4 Long-term persistence of active Daratumumab in circulation
In order to avoid potential side effects of the anti-CD38 therapy on ICI, the infusion of Daratumumab was stopped for 3 weeks prior to the start of the anti-PD1 treatment. However, it was unclear how long Daratumumab can remain functional in circulation. Earlier reports mentioned Daratumumab has a half-life of 21 days and around 11.5 ug/ml of circulating Daratumumab was detected at 6 weeks post infusion (38, 43). To determine the amount of circulating Daratumumab in the plasma of NKTL patient 1, we established an in vitro assay based on epitope competition. The anti-CD38 clone LS198-4-2 was found to be blocked by Daratumumab in a dose-dependent matter (Supplementary Figure 3). Using this antibody to stain the CD38-expressing cell line NK92 allowed us to quantify the amount of Daratumumab to concentrations as low as 500 ng/ml (Supplementary Figure 4). Using this assay to analyze plasma samples from NKTL patient 1, we estimated the amount of circulating Daratumumab in this patient to be 625, 25 and 2.5 ug/ml at 2 weeks (tp0), 7 weeks (tp1) and 13 wpD treatment (tp2) respectively. This is in line with what was previously reported (38, 43).
In order to determine whether circulating Daratumumab in the combination therapy patient is functional, we performed an in vitro complement dependent cytotoxicity (CDC) assay with NK92 target cells (44). Titration of Daratumumab showed effective CDC on NK92 at >400 ng/ml (Supplementary Figure 4B). The plasma samples collected at tp0, tp1 and tp2 were well above this threshold. Incubation of NK92 with these plasma samples resulted in CDC, confirming that circulating Daratumumab retains its lytic function up to 13 wpD (Figure 3B).
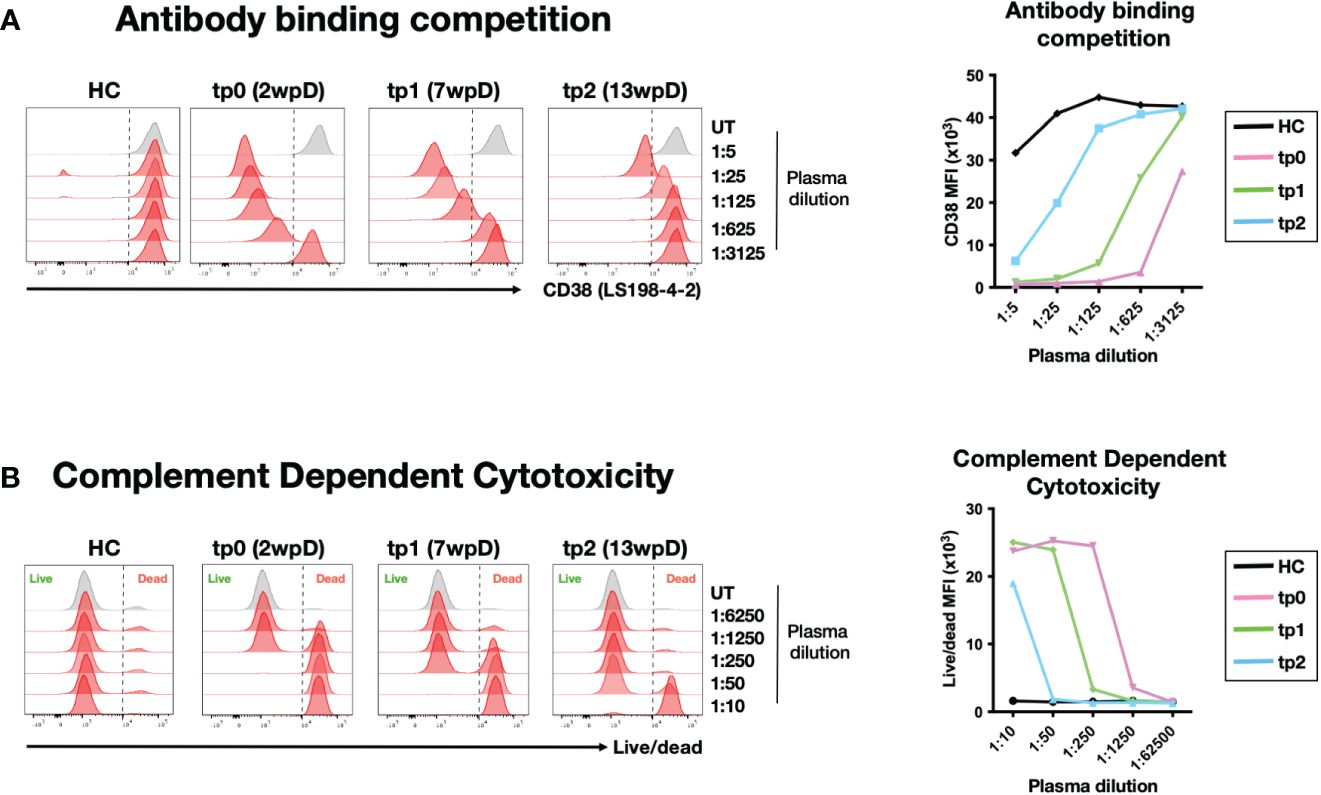
Figure 3 Circulating Daratumumab is functional up to 13 weeks post administration (A) Indirect measurement of circulating Daratumumab in combination therapy patient plasma samples. CD38-high NK92 cell line was pre-incubated with different dilution of combination therapy-treated plasma samples from tp0, tp1 and tp2 or HC. Histograms (left) and line graph showing interference of circulating Daratumumab on CD38 (clone LS198-4-2) binding using flow cytometry. Line graph (right) shows CD38 mean fluorescence intensity (MFI) on NK92 pre-incubated with combination therapy patient plasma samples. (B) NK92 cell line pre-treated with different dilutions of combination therapy patient plasma samples were incubated with pooled HC sera for 2-3 hours for complement dependent cytotoxicity (CDC). Cell viability was determined using live/dead staining and analysed using flow cytometry. Histogram plot (left) and line graph (right) shows live/dead MFI on NK92 after CDC was performed.
3.5 Anti-CD38 therapy enriches for (exhausted) PD1- TEMRA cells
Our earlier analysis on anti-PD1-treated NKTL patients suggests CD38+ TEM and TCM are most susceptible to depletion by Daratumumab treatment (Figure 2). In line with this, we found TCM and TEM to be strikingly underrepresented at tp0 (2wpD) in the combination therapy patient (Figure 4A). Almost three quarters of the CD8+ T cells from this patient were TEMRAs, which are senescent T cells with poor proliferative potential associated with poor survival in cancer patients (45).
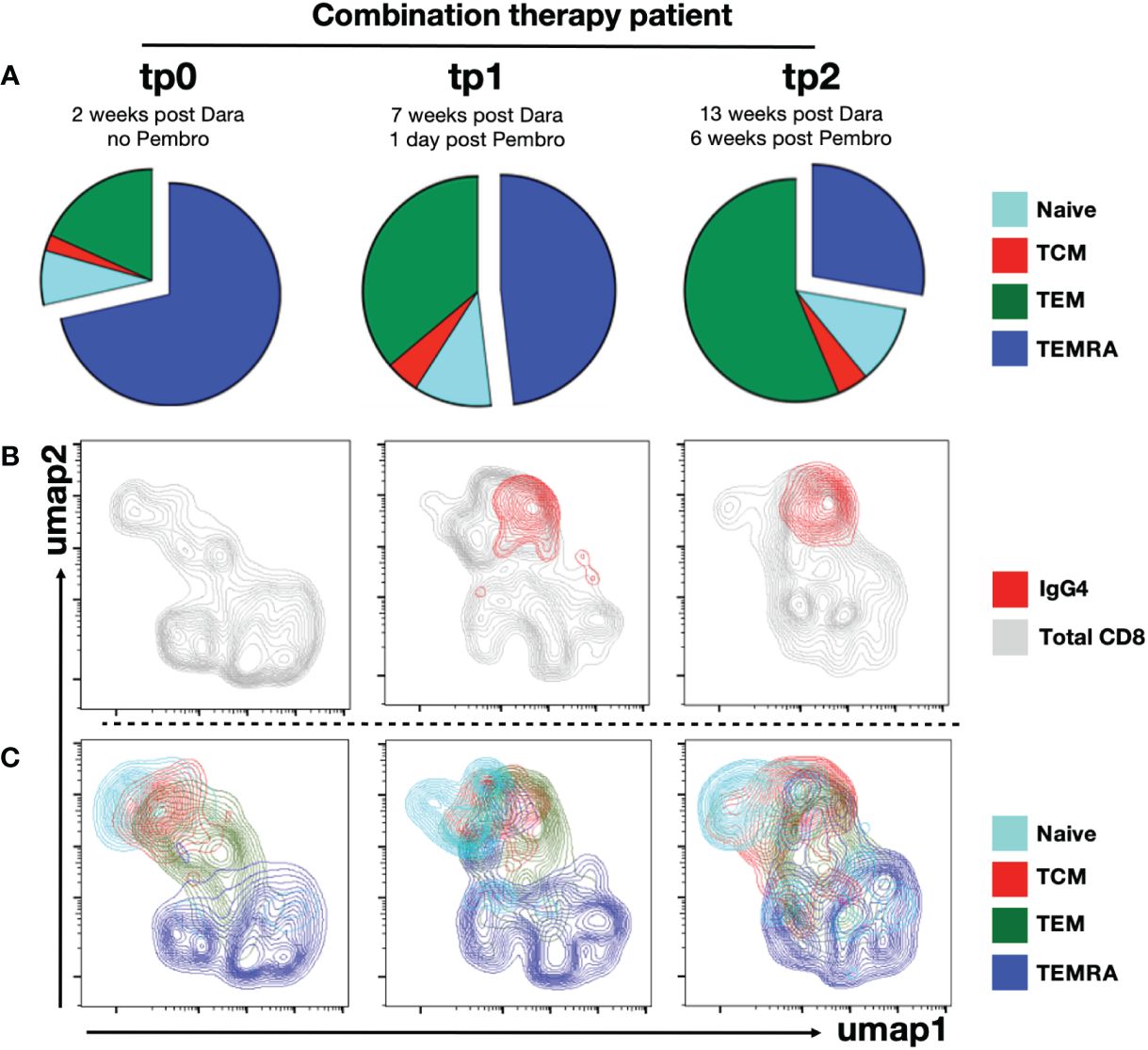
Figure 4 Anti-PD1 targets mainly effector and central memory CD8+ T cell subsets. PBMCs from combination therapy patient were immunophenotyped using CyTOF. CD8+ T cells were gated. (A) Piecharts showing distribution of into naïve, TCM, TEM and TEMRA CD8+ T cell subsets from combination therapy patient at tp0, tp1 and tp2. UMAP clustering of CD8+ T cells from combination therapy patient at tp0, tp1 and tp2 were performed. (B) Umap clusters of total CD8+ T cells (grey) were overlaid with anti-IgG4+ CD8+ T cells (red) or (C) segregated into naïve, TCM, TEM and TEMRA subsets.
A gradual re-emergence of TEM and proportionate reduction of TEMRA was evident at tp1 and tp2 (Figure 4A; Supplementary Figure 5). The re-emergence of TEM was also accompanied by recovery of CD38+ population at tp2 in the combination therapy patient, likely due to the waning of circulating Daratumumab at tp2 (Figures 2B, 3A). This was further confirmed by ex vivo staining with anti-IgG4 staining, indicating that the binding of the anti-PD1 antibody (Nivolumab) was restricted to TCM and TCM, while the bulk of TEMRA cells in this patient were not targeted by the treatment (Figures 4B, C).
3.6 Anti-CD38/ICI combination therapy is ineffective in a variety of cancer types
To assess the efficacy of combining anti-CD38 and PD1/PDL1-directed ICI in more generalizable scenario, we did a comprehensive literature search and compared the objective response rate (ORR) of small scale- and clinical trial-studies that administer either a combination of anti-CD38/ICI or ICI alone in various forms of cancer (Table 2). Taking into account all the published data of these studies, we noted a significant reduction in the ORR of patients undergoing combination (46, 62, 66) therapy as compared to ICI alone (24, 25, 27, 46–61, 63–65, 67–76) (Figure 5). This was independent of the type of PD1/PDL1 interference, as no significant difference in ORR was observed whether anti-PD1 or anti-PDL1 monotherapy was applied (Supplementary Figure 7). In our case study as well as these published combination therapies, anti-CD38 was given prior to the application of the PD1-based ICI. Thus, a combination of these two therapies in this order seem to be counter-productive (46, 62, 66).
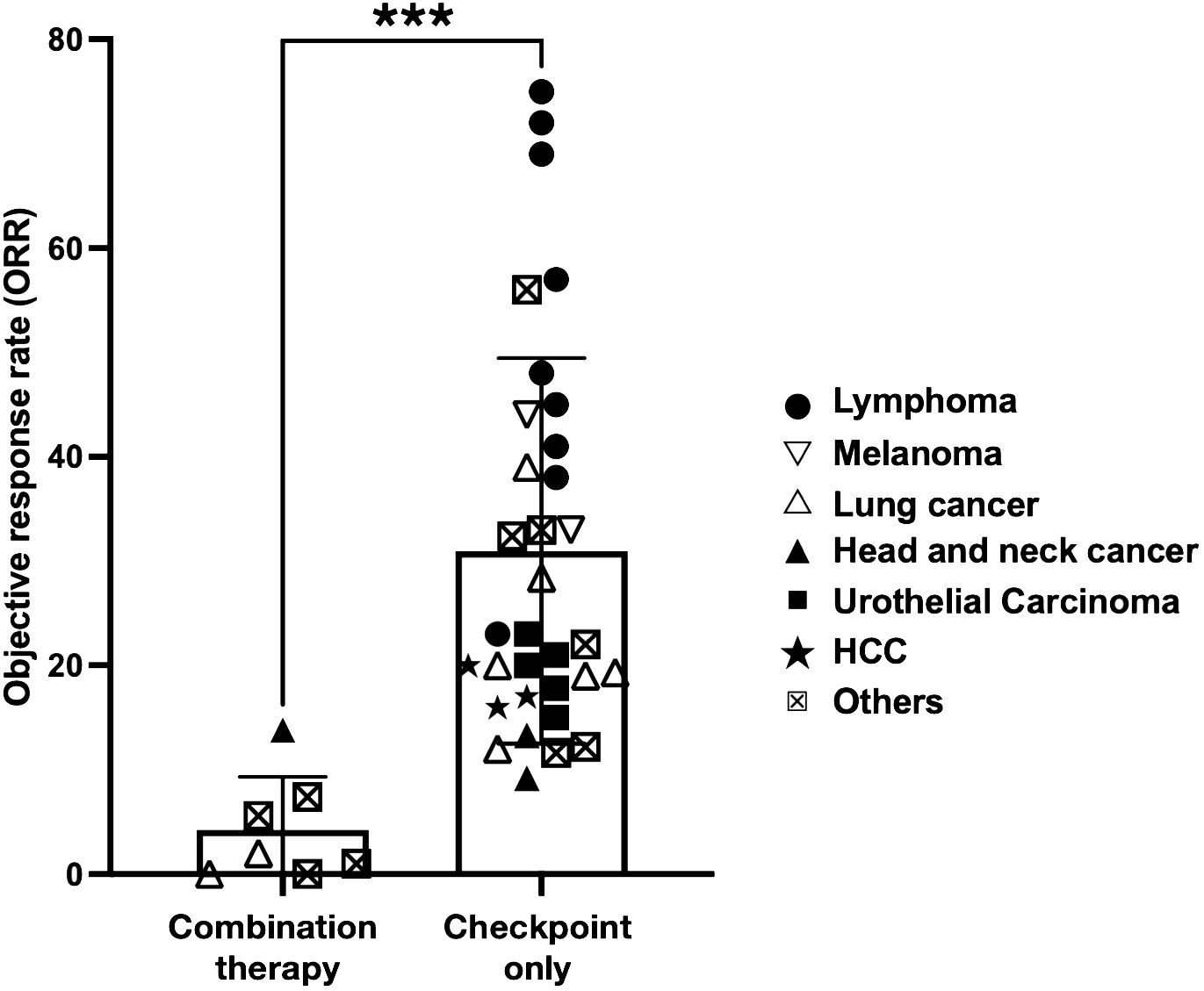
Figure 5 Cancer patients that received combination therapy fare significantly worse. Scatterplot comparing ORR of clinical trials performed in cancer patients that received combination therapy (anti-CD38 and immune checkpoint inhibitor (ICI) or ICI monotherapy only. ICI includes either anti-PD1 or anti-PDL1 therapy. The symbols indicate type of cancer that is being treated in the trial. Statistical analysis was performed using two-tailed Mann-Whitney U test. A p-value of less than 0.05 is considered statistically significant. (***p<0.0001).
4 Discussion
NKTL patients that failed L-asparaginase chemotherapy are usually faced with a dismal outcome (77). While ICI has shown to be a promising treatment for relapsing NKTL patients, the ORR ranges from 37-54% (11, 24, 25, 27, 61). As ICI and anti-CD38 therapy function through different mechanisms, the possibility of combining ICI and anti-CD38 to improve patient outcome was raised (30). Here we present data from a relapsed NKTL patient who underwent combination therapy to demonstrate that the combined use of anti-CD38 and anti-PD1 produced antithetic effects. This was confirmed in a meta-study based on data from published cancer trials showing that ORR from combination therapies is significantly reduced as compared to ICI monotherapies.
ICI monotherapy is reportedly effective in a subset of refractory NKTL patients (24–27). As shown in the anti-PD1 treated NKTL patients, anti-PD1 treatment triggered a strong activation and expansion of the CD45RA- memory cells TCM and TEM. The two subsets expressed the highest levels of PD1 resulting in a strong binding of the anti-PD1 antibody (Pembrolizumab). As this reactivation is associated with an upregulation of CD38, these subsets were depleted by Daratumumab, leaving mostly TEMRA cells as the predominant CD8+ T cell subset in patient. TEMRAs are defined as terminally differentiated senescent memory cells with low replicative potential and typically are associated with poor anti-tumor response (42, 78).
Daratumumab is used for treating multiple myeloma (MM), a malignancy arising from CD38 high plasma cells (9, 12). It is thus unsurprising to find the patient to be severely depleted of CD38+ B cells such as plasma cells (35). Depletion of these cells likely accounted for the low anti-EBV IgG titer. The loss of antibody titer and B cell responses can also cause patients to be more susceptible to infections (79), which could be a contributing factor to the uncontrollable increase in EBV viremia and small burst of virion production as evidenced by the detection of encapsidated viral DNA.
On top of this, a more important factor associated with the failure of the combination therapy is likely the depletion of (reactivated) CD38+ T cells. Upregulation of CD38+ on activated CD8+ T cells has been widely used as a prognostic marker for various diseases, including HIV infection (15, 17) and chronic lymphocytic leukemia (80). The importance of these CD38+ leukocytes was observed in hepatocarcinoma patients, where patients with higher levels of infiltrating CD38+ cells were found to respond better to anti-PD1 treatment (81). A recent study in non-small cell lung carcinoma (NSCLC) patients also showed that a higher level of tumor-infiltrating CD38+ CD8+ T cells is correlated with better survival outcomes. In line with our own observation, their data further suggest that CD38+ CD8+ T cells are the prime target for ICI reinvigoration (82) and that CD38 was upregulated on CD8+ T cells after anti-PD1 therapy (40, 83). In agreement with these data, we also noted that Pembrolizumab was predominantly detected on CD38-expressing CD8+ T cells.
Daratumumab shows potent depleting effect. At 7 wpD treatment, we found only negligible level of CD38+ CD8+ T cells in the patient blood. High levels of circulating Daratumumab were shown to be fully functional up to 13wpD through in vitro CDC assay, suggesting that any activated CD38+ CD8+ T cells induced by the anti-PD1 treatment are likely being depleted by the circulating Daratumumab. This notion is supported by the re-emergence of CD38+ CD8+ T cells coinciding with waning levels of circulating Daratumumab at 13wpD. Further analysis of the re-emerging CD38+ CD8+ subsets revealed that these are CD45RA- TCM and TEM populations, the same subsets expressing PD1 and hence are targeted by anti-PD1 therapy. While the depletion of NK cells (13, 14) and B cells has been previously reported, activated CD8+ T cells are also apparently effectively removed by this antibody.
Taken together, our results show that Daratumumab can persist in the circulation for up to 13 weeks without losing its lytic function. As the activation of CD8+ T cells by the anti-PD1 treatment results in the upregulation of CD38, these reinvigorated cells become an immediate target for Daratumumab-mediated lysis, thus abrogating the effectiveness of anti-PD1 treatment. Notably, clinical trial involving the use of Daratumumab and Atezolizumab (anti-PDL1) (NCT03023423) was also halted due to the lack of clinical efficacy (46).
5 Conclusions
Despite the small sample size, this study has important clinical implications in the treatment of NKTL patients. Our results provided strong evidence to caution against the combined use of anti-PD1 and anti-CD38 agents, especially in the sequential order of anti-CD38 followed by anti-PD1.
Data availability statement
The original contributions presented in the study are included in the article/Supplementary Material. Further inquiries can be directed to the corresponding authors.
Ethics statement
Healthy donor samples were collected under the SingHealth Centralised Institutional Review Board (CIRB Ref: 2017/2806). Patient samples were obtained under SingHealth CIRB Ref: 2004/407/F. Written informed consent was obtained from all donors prior to sample collection. Written informed consent was obtained from the individual(s) for the publication of any potentially identifiable images or data included in this article.
Author contributions
WL: Writing – review & editing, Writing – original draft, Validation, Supervision, Project administration, Methodology, Investigation, Formal Analysis, Data curation, Conceptualization. JQL: Writing – review & editing, Writing – original draft, Data curation. TT: Writing – review & editing, Resources, Conceptualization. DT: Writing – review & editing, Resources, Methodology. SK: Writing – review & editing, Writing – original draft, Project administration, Investigation, Data curation. KP: Writing – review & editing, Writing – original draft, Supervision, Project administration, Methodology. LW: Writing – review & editing, Writing – original draft, Supervision, Project administration, Methodology. JL: Writing – review & editing, Writing – original draft, Supervision, Project administration, Methodology. KT: Writing – review & editing, Writing – original draft, Validation, Project administration, Methodology. WC: Writing – review & editing, Validation, Supervision. SL: Writing – review & editing, Resources, Funding acquisition. CO: Writing – review & editing, Resources, Methodology, Investigation, Funding acquisition, Data curation. OR: Writing – review & editing, Writing – original draft, Supervision, Resources, Funding acquisition, Formal Analysis, Conceptualization.
Funding
The author(s) declare that financial support was received for the research, authorship, and/or publication of this article. The study was supported by the Biomedical Research Council, A*STAR and Singapore Ministry of Health’s National Medical Research Council (NMRC-OFLCG-18May0028). OR and JWL grants are supported by A*STAR IAF-PP H22J2a0043 and A*STAR JCO (Grant ID: 222D89) respectively. LW and JQL are supported by MOH- OFYIRG19nov-0013 and MOH-OFYIRG22jul-0017 respectively.
Acknowledgments
We would like to thank the A*STAR SIgN Flow Cytometry and mass cytometry platforms for enabling the cytometry works for this publication. A*STAR’s SIgN Flow Cytometry platform is supported by research grants including the Biomedical Research Council (BMRC) grant and the National Research Foundation (NRF), Immunomonitoring Service Platform (Ref: ISP: NRF2017_SISFP09) grant. We also thank all patients and healthy donors for making this study possible.
Conflict of interest
The authors declare that the research was conducted in the absence of any commercial or financial relationships that could be construed as a potential conflict of interest.
Publisher’s note
All claims expressed in this article are solely those of the authors and do not necessarily represent those of their affiliated organizations, or those of the publisher, the editors and the reviewers. Any product that may be evaluated in this article, or claim that may be made by its manufacturer, is not guaranteed or endorsed by the publisher.
Supplementary material
The Supplementary Material for this article can be found online at: https://www.frontiersin.org/articles/10.3389/fimmu.2024.1346178/full#supplementary-material
References
1. Morton LM, Wang SS, Devesa SS, Hartge P, Weisenburger DD, Linet MS. Lymphoma incidence patterns by WHO subtype in the United States, 1992-2001. Blood. (2006) 107:265–76. doi: 10.1182/blood-2005-06-2508
2. Isobe K, Uno T, J-i T, Kawakami H, Ueno N, Wakita H, et al. Extranodal natural killer/T-cell lymphoma, nasal type. Cancer. (2006) 106:609–15. doi: 10.1002/cncr.21656
3. Yamaguchi M, Kwong YL, Kim WS, Maeda Y, Hashimoto C, Suh C, et al. Phase II study of SMILE chemotherapy for newly diagnosed stage IV, relapsed, or refractory extranodal natural killer (NK)/T-cell lymphoma, nasal type: the NK-Cell Tumor Study Group study. J Clin Oncol. (2011) 29:4410–6. doi: 10.1200/JCO.2011.35.6287
4. Chow LQM, Haddad R, Gupta S, Mahipal A, Mehra R, Tahara M, et al. Antitumor activity of pembrolizumab in biomarker-unselected patients with recurrent and/or metastatic head and neck squamous cell carcinoma: Results from the phase ib KEYNOTE-012 expansion cohort. J Clin Oncol. (2016) 34:3838–45. doi: 10.1200/JCO.2016.68.1478
5. Powles T, Eder JP, Fine GD, Braiteh FS, Loriot Y, Cruz C, et al. MPDL3280A (anti-PD-L1) treatment leads to clinical activity in metastatic bladder cancer. Nature. (2014) 515:558–62. doi: 10.1038/nature13904
6. Rizvi NA, Hellmann MD, Snyder A, Kvistborg P, Makarov V, Havel JJ, et al. Cancer immunology. Mutational landscape determines sensitivity to PD-1 blockade in non-small cell lung cancer. Sci (New York NY). (2015) 348:124–8. doi: 10.1126/science.aaa1348
7. Brahmer JR, Tykodi SS, Chow LQ, Hwu WJ, Topalian SL, Hwu P, et al. Safety and activity of anti-PD-L1 antibody in patients with advanced cancer. N Engl J Med. (2012) 366:2455–65. doi: 10.1056/NEJMoa1200694
8. Ansell SM, Lesokhin AM, Borrello I, Halwani A, Scott EC, Gutierrez M, et al. PD-1 blockade with nivolumab in relapsed or refractory hodgkin's lymphoma. New Engl J Med. (2014) 372:311–9. doi: 10.1056/NEJMoa1411087
9. Lokhorst HM, Plesner T, Laubach JP, Nahi H, Gimsing P, Hansson M, et al. Targeting CD38 with daratumumab monotherapy in multiple myeloma. N Engl J Med. (2015) 373:1207–19. doi: 10.1056/NEJMoa1506348
10. Hari P, Raj RV, Olteanu H. Targeting CD38 in refractory extranodal natural killer cell-T-cell lymphoma. N Engl J Med. (2016) 375:1501–2. doi: 10.1056/NEJMc1605684
11. Huang H, Zhu J, Yao M, Kim TM, Yoon DH, Cho S-G, et al. Daratumumab monotherapy for patients with relapsed or refractory natural killer/T-cell lymphoma, nasal type: an open-label, single-arm, multicenter, phase 2 study. J Hematol Oncol. (2021) 14:25. doi: 10.1186/s13045-020-01020-y
12. Sanz I, Wei C, Jenks SA, Cashman KS, Tipton C, Woodruff MC, et al. Challenges and opportunities for consistent classification of human B cell and plasma cell populations. Front Immunol. (2019) 10:2458–. doi: 10.3389/fimmu.2019.02458
13. Krejcik J, Casneuf T, Nijhof IS, Verbist B, Bald J, Plesner T, et al. Daratumumab depletes CD38+ immune regulatory cells, promotes T-cell expansion, and skews T-cell repertoire in multiple myeloma. Blood. (2016) 128:384–94. doi: 10.1182/blood-2015-12-687749
14. Zambello R, Barilà G, Manni S, Piazza F, Semenzato G. NK cells and CD38: implication for (Immuno)Therapy in plasma cell dyscrasias. Cells. (2020) 9:768. doi: 10.3390/cells9030768
15. Bofill M, Mocroft A, Lipman M, Medina E, Borthwick NJ, Sabin CA, et al. Increased numbers of primed activated CD8+CD38+CD45RO+ T cells predict the decline of CD4+ T cells in HIV-1-infected patients. Aids. (1996) 10:827–34. doi: 10.1097/00002030-199607000-00005
16. Sandoval-Montes C, Santos-Argumedo L. CD38 is expressed selectively during the activation of a subset of mature T cells with reduced proliferation but improved potential to produce cytokines. J Leukocyte Biol. (2005) 77:513–21. doi: 10.1189/jlb.0404262
17. Liu Z, Cumberland WG, Hultin LE, Prince HE, Detels R, Giorgi JV. Elevated CD38 antigen expression on CD8+ T cells is a stronger marker for the risk of chronic HIV disease progression to AIDS and death in the Multicenter AIDS Cohort Study than CD4+ cell count, soluble immune activation markers, or combinations of HLA-DR and CD38 expression. J Acquir Immune Defic Syndr Hum Retrovirol. (1997) 16:83–92. doi: 10.1097/00042560-199710010-00003
18. Jiang T, Shi T, Zhang H, Hu J, Song Y, Wei J, et al. Tumor neoantigens: from basic research to clinical applications. J Hematol Oncol. (2019) 12:93. doi: 10.1186/s13045-019-0787-5
19. Petrovas C, Casazza JP, Brenchley JM, Price DA, Gostick E, Adams WC, et al. PD-1 is a regulator of virus-specific CD8+ T cell survival in HIV infection. J Exp Med. (2006) 203:2281–92. doi: 10.1084/jem.20061496
20. Dong H, Strome SE, Salomao DR, Tamura H, Hirano F, Flies DB, et al. Tumor-associated B7-H1 promotes T-cell apoptosis: a potential mechanism of immune evasion. Nat Med. (2002) 8:793–800. doi: 10.1038/nm730
21. Freeman GJ, Long AJ, Iwai Y, Bourque K, Chernova T, Nishimura H, et al. Engagement of the pd-1 immunoinhibitory receptor by a novel B7 family member leads to negative regulation of lymphocyte activation. J Exp Med. (2000) 192:1027–34. doi: 10.1084/jem.192.7.1027
22. Parry RV, Chemnitz JM, Frauwirth KA, Lanfranco AR, Braunstein I, Kobayashi SV, et al. CTLA-4 and PD-1 receptors inhibit T-cell activation by distinct mechanisms. Mol Cell Biol. (2005) 25:9543–53. doi: 10.1128/MCB.25.21.9543-9553.2005
23. Garon EB, Rizvi NA, Hui R, Leighl N, Balmanoukian AS, Eder JP, et al. Pembrolizumab for the treatment of non–small-cell lung cancer. New Engl J Med. (2015) 372:2018–28. doi: 10.1056/NEJMoa1501824
24. Li X, Cheng Y, Zhang M, Yan J, Li L, Fu X, et al. Activity of pembrolizumab in relapsed/refractory NK/T-cell lymphoma. J Hematol Oncol. (2018) 11:15. doi: 10.1186/s13045-018-0559-7
25. Kwong Y-L, Chan TSY, Tan D, Kim SJ, Poon L-M, Mow B, et al. PD1 blockade with pembrolizumab is highly effective in relapsed or refractory NK/T-cell lymphoma failing l-asparaginase. Blood. (2017) 129:2437–42. doi: 10.1182/blood-2016-12-756841
26. Lim JQ, Huang D, Tang T, Tan D, Laurensia Y, Peng R-J, et al. Whole-genome sequencing identifies responders to Pembrolizumab in relapse/refractory natural-killer/T cell lymphoma. Leukemia. (2020) 34:3413–9. doi: 10.1038/s41375-020-1000-0
27. Kim SJ, Lim JQ, Laurensia Y, Cho J, Yoon SE, Lee JY, et al. Avelumab for the treatment of relapsed or refractory extranodal NK/T-cell lymphoma: an open-label phase 2 study. Blood. (2020) 136:2754–63. doi: 10.1182/blood.2020007247
28. Cai J, Liu P, Huang H, Li Y, Ma S, Zhou H, et al. Combination of anti-PD-1 antibody with P-GEMOX as a potentially effective immunochemotherapy for advanced natural killer/T cell lymphoma. Signal Transduct Target Ther. (2020) 5:289. doi: 10.1038/s41392-020-00331-3
29. Mokhtari RB, Homayouni TS, Baluch N, Morgatskaya E, Kumar S, Das B, et al. Combination therapy in combating cancer. Oncotarget. (2017) 8:38022–43. doi: 10.18632/oncotarget.v8i23
30. Jaccard A, Hermine O. A major turning point in NK/T-cell lymphoma? Blood. (2017) 129:2342–3. doi: 10.1182/blood-2017-03-769075
31. Lo YM, Chan LY, Lo KW, Leung SF, Zhang J, Chan AT, et al. Quantitative analysis of cell-free Epstein-Barr virus DNA in plasma of patients with nasopharyngeal carcinoma. Cancer Res. (1999) 59:1188–91. doi: 10.1158/1078-0432.ccr-0991-3
32. Lawrence JB, Villnave CA, Singer RH. Sensitive, high-resolution chromatin and chromosome mapping in situ: presence and orientation of two closely integrated copies of EBV in a lymphoma line. Cell. (1988) 52:51–61. doi: 10.1016/0092-8674(88)90530-2
33. Chen H, Lau MC, Wong MT, Newell EW, Poidinger M, Chen J. Cytofkit: A bioconductor package for an integrated mass cytometry data analysis pipeline. PLoS Comput Biol. (2016) 12:e1005112. doi: 10.1371/journal.pcbi.1005112
34. Lei KIK, Chan LYS, Chan W-Y, Johnson PJ, Lo YMD. Diagnostic and prognostic implications of circulating cell-free epstein-barr virus DNA in natural killer/T-cell lymphoma. Clin Cancer Res. (2002) 8:29–34.
35. Frerichs KA, Bosman PWC, van Velzen JF, Fraaij PLA, Koopmans MPG, Rimmelzwaan GF, et al. Effect of daratumumab on normal plasma cells, polyclonal immunoglobulin levels, and vaccination responses in extensively pre-treated multiple myeloma patients. Haematologica. (2020) 105:e302–e6. doi: 10.3324/haematol.2019.231860
36. Fu Y, Zhang Z, Yang Z, Jiang Y, Han X, Xu J, et al. CD27–CD38+ B cells accumulated in early HIV infection exhibit transitional profile and promote HIV disease progression. Cell Rep. (2021) 36:109344. doi: 10.1016/j.celrep.2021.109344
37. Lacaille V, Kapinsky M. The Nanobody JK36 circumvents the epitope overlapping commonly observed between therapeutic anti-CD38 antibodies and commercially available analytical mouse antibodies. J Immunol. (2021) 206:59.24–4. doi: 10.4049/jimmunol.206.Supp.59.24
38. Oberle A, Brandt A, Alawi M, Langebrake C, Janjetovic S, Wolschke C, et al. Long-term CD38 saturation by daratumumab interferes with diagnostic myeloma cell detection. Haematologica. (2017) 102:e368–e70. doi: 10.3324/haematol.2017.169235
39. Barber DL, Wherry EJ, Masopust D, Zhu B, Allison JP, Sharpe AH, et al. Restoring function in exhausted CD8 T cells during chronic viral infection. Nature. (2006) 439:682–7. doi: 10.1038/nature04444
40. Huang AC, Postow MA, Orlowski RJ, Mick R, Bengsch B, Manne S, et al. T-cell invigoration to tumor burden ratio associated with anti-PD-1 response. Nature. (2017) 545:60–5. doi: 10.1038/nature22079
41. Scapin G, Yang X, Prosise WW, McCoy M, Reichert P, Johnston JM, et al. Structure of full-length human anti-PD1 therapeutic IgG4 antibody pembrolizumab. Nat Struct Mol Biol. (2015) 22:953–8. doi: 10.1038/nsmb.3129
42. Larbi A, Fulop T. From “truly naïve” to “exhausted senescent” T cells: When markers predict functionality. Cytometry Part A. (2014) 85:25–35. doi: 10.1002/cyto.a.22351
43. Frerichs KA, Minnema MC, Levin MD, Broijl A, Bos GMJ, Kersten MJ, et al. Efficacy and safety of daratumumab combined with all-trans retinoic acid in relapsed/refractory multiple myeloma. Blood Adv. (2021) 5:5128–39. doi: 10.1182/bloodadvances.2021005220
44. Sanchez L, Wang Y, Siegel DS, Wang ML. Daratumumab: a first-in-class CD38 monoclonal antibody for the treatment of multiple myeloma. J Hematol Oncol. (2016) 9:51–. doi: 10.1186/s13045-016-0283-0
45. Zhang J, He T, Xue L, Guo H. Senescent T cells: a potential biomarker and target for cancer therapy. eBioMedicine. (2021) 68. doi: 10.1016/j.ebiom.2021.103409
46. Pillai RN, Ramalingam SS, Thayu M, Lorenzini P, Alvarez Arias DA, Moy C, et al. Daratumumab plus atezolizumab in previously treated advanced or metastatic NSCLC: Brief report on a randomized, open-label, phase 1b/2 study (LUC2001 JNJ-54767414). JTO Clin Res Rep. (2021) 2:100104. doi: 10.1016/j.jtocrr.2020.100104
47. Armand P, Rodig S, Melnichenko V, Thieblemont C, Bouabdallah K, Tumyan G, et al. Pembrolizumab in relapsed or refractory primary mediastinal large B-cell lymphoma. J Clin Oncol. (2019) 37:3291–9. doi: 10.1200/JCO.19.01389
48. Chung HC, Ros W, Delord J-P, Perets R, Italiano A, Shapira-Frommer R, et al. Efficacy and safety of pembrolizumab in previously treated advanced cervical cancer: Results from the phase II KEYNOTE-158 study. J Clin Oncol. (2019) 37:1470–8. doi: 10.1200/JCO.18.01265
49. Overman MJ, McDermott R, Leach JL, Lonardi S, Lenz HJ, Morse MA, et al. Nivolumab in patients with metastatic DNA mismatch repair-deficient or microsatellite instability-high colorectal cancer (CheckMate 142): an open-label, multicentre, phase 2 study. Lancet Oncol. (2017) 18:1182–91. doi: 10.1016/S1470-2045(17)30422-9
50. Fuchs CS, Doi T, Jang RW, Muro K, Satoh T, MaChado M, et al. Safety and efficacy of pembrolizumab monotherapy in patients with previously treated advanced gastric and gastroesophageal junction cancer: Phase 2 clinical KEYNOTE-059 trial. JAMA Oncol. (2018) 4:e180013. doi: 10.1001/jamaoncol.2018.0013
51. Zhu AX, Finn RS, Edeline J, Cattan S, Ogasawara S, Palmer D, et al. Pembrolizumab in patients with advanced hepatocellular carcinoma previously treated with sorafenib (KEYNOTE-224): a non-randomised, open-label phase 2 trial. Lancet Oncol. (2018) 19:940–52. doi: 10.1016/S1470-2045(18)30351-6
52. Verset G, Borbath I, Karwal M, Verslype C, Van Vlierberghe H, Kardosh A, et al. Pembrolizumab monotherapy for previously untreated advanced hepatocellular carcinoma: Data from the open-label, phase II KEYNOTE-224 trial. Clin Cancer Res. (2022) 28:2547–54. doi: 10.1158/1078-0432.CCR-21-3807
53. El-Khoueiry AB, Sangro B, Yau T, Crocenzi TS, Kudo M, Hsu C, et al. Nivolumab in patients with advanced hepatocellular carcinoma (CheckMate 040): an open-label, non-comparative, phase 1/2 dose escalation and expansion trial. Lancet. (2017) 389:2492–502. doi: 10.1016/S0140-6736(17)31046-2
54. Ferris RL, Blumenschein G, Fayette J, Guigay J, Colevas AD, Licitra L, et al. Nivolumab for recurrent squamous-cell carcinoma of the head and neck. New Engl J Med. (2016) 375:1856–67. doi: 10.1056/NEJMoa1602252
55. Guigay J, Lee K-W, Patel MR, Daste A, Wong DJ, Goel S, et al. Avelumab for platinum-ineligible/refractory recurrent and/or metastatic squamous cell carcinoma of the head and neck: phase Ib results from the JAVELIN Solid Tumor trial. J ImmunoTherapy Cancer. (2021) 9:e002998. doi: 10.1136/jitc-2021-002998
56. Chen R, Zinzani PL, Fanale MA, Armand P, Johnson NA, Brice P, et al. Phase II study of the efficacy and safety of pembrolizumab for relapsed/refractory classic hodgkin lymphoma. J Clin Oncol. (2017) 35:2125–32. doi: 10.1200/JCO.2016.72.1316
57. Wolchok JD, Chiarion-Sileni V, Gonzalez R, Rutkowski P, Grob J-J, Cowey CL, et al. Overall survival with combined nivolumab and ipilimumab in advanced melanoma. New Engl J Med. (2017) 377:1345–56. doi: 10.1056/NEJMoa1709684
58. Robert C, Ribas A, Schachter J, Arance A, Grob JJ, Mortier L, et al. Pembrolizumab versus ipilimumab in advanced melanoma (KEYNOTE-006): post-hoc 5-year results from an open-label, multicentre, randomised, controlled, phase 3 study. Lancet Oncol. (2019) 20:1239–51. doi: 10.1016/S1470-2045(19)30388-2
59. Nghiem P, Bhatia S, Lipson EJ, Sharfman WH, KudChadkar RR, Brohl AS, et al. Durable tumor regression and overall survival in patients with advanced merkel cell carcinoma receiving pembrolizumab as first-line therapy. J Clin Oncol. (2019) 37:693–702. doi: 10.1200/JCO.18.01896
60. Kaufman HL, Russell JS, Hamid O, Bhatia S, Terheyden P, D’Angelo SP, et al. Updated efficacy of avelumab in patients with previously treated metastatic Merkel cell carcinoma after ≥1 year of follow-up: JAVELIN Merkel 200, a phase 2 clinical trial. J ImmunoTherapy Cancer. (2018) 6:7. doi: 10.1186/s40425-017-0310-x
61. Tao R, Fan L, Song Y, Hu Y, Zhang W, Wang Y, et al. Sintilimab for relapsed/refractory extranodal NK/T cell lymphoma: a multicenter, single-arm, phase 2 trial (ORIENT-4). Signal Transduction Targeted Ther. (2021) 6:365. doi: 10.1038/s41392-021-00768-0
62. Simonelli M, Garralda E, Eskens F, Gil-Martin M, Yen CJ, Obermannova R, et al. Isatuximab plus atezolizumab in patients with advanced solid tumors: results from a phase I/II, open-label, multicenter study. ESMO Open. (2022) 7:100562. doi: 10.1016/j.esmoop.2022.100562
63. Huang HQ, Tao R, Zou L, Cen H, Guo Y, Huang Y, et al. Preliminary results from a multicenter, single-arm, phase 2 study of CS1001, an anti-programmed death-ligand 1 (PD-L1) human monoclonal antibody (mAb), in patients (pts) with relapsed or refractory extranodal natural killer/T cell lymphoma (rr-ENKTL). Blood. (2019) 134:2833. doi: 10.1182/blood-2019-121865
64. Kim SJ, Hyeon J, Cho I, Ko YH, Kim WS. Comparison of efficacy of pembrolizumab between epstein-barr virus−Positive and −Negative relapsed or refractory non-hodgkin lymphomas. Cancer Res Treat Off J Korean Cancer Assoc. (2019) 51:611 – 22. doi: 10.4143/crt.2018.191
65. Balar AV, Galsky MD, Rosenberg JE, Powles T, Petrylak DP, Bellmunt J, et al. Atezolizumab as first-line treatment in cisplatin-ineligible patients with locally advanced and metastatic urothelial carcinoma: a single-arm, multicentre, phase 2 trial. Lancet. (2017) 389:67–76. doi: 10.1016/S0140-6736(16)32455-2
66. Zucali PA, Lin C-C, Carthon BC, Bauer TM, Tucci M, Italiano A, et al. Targeting CD38 and PD-1 with isatuximab plus cemiplimab in patients with advanced solid Malignancies: results from a phase I/II open-label, multicenter study. J ImmunoTherapy Cancer. (2022) 10:e003697. doi: 10.1136/jitc-2021-003697
67. Mok TSK, Wu Y-L, Kudaba I, Kowalski DM, Cho BC, Turna HZ, et al. Pembrolizumab versus chemotherapy for previously untreated, PD-L1-expressing, locally advanced or metastatic non-small-cell lung cancer (KEYNOTE-042): a randomised, open-label, controlled, phase 3 trial. Lancet. (2019) 393:1819–30. doi: 10.1016/S0140-6736(18)32409-7
68. Vokes EE, Ready N, Felip E, Horn L, Burgio MA, Antonia SJ, et al. Nivolumab versus docetaxel in previously treated advanced non-small-cell lung cancer (CheckMate 017 and CheckMate 057): 3-year update and outcomes in patients with liver metastases. Ann Oncol. (2018) 29:959–65. doi: 10.1093/annonc/mdy041
69. Chung HC, Piha-Paul SA, Lopez-Martin J, Schellens JHM, Kao S, Miller WH Jr., et al. Pembrolizumab after two or more lines of previous therapy in patients with recurrent or metastatic SCLC: Results from the KEYNOTE-028 and KEYNOTE-158 studies. J Thorac Oncol. (2020) 15:618–27. doi: 10.1016/j.jtho.2019.12.109
70. Ready NE, Ott PA, Hellmann MD, Zugazagoitia J, Hann CL, de Braud F, et al. Nivolumab monotherapy and nivolumab plus ipilimumab in recurrent small cell lung cancer: Results from the checkMate 032 randomized cohort. J Thorac Oncol. (2020) 15:426–35. doi: 10.1016/j.jtho.2019.10.004
71. Kojima T, Shah MA, Muro K, Francois E, Adenis A, Hsu CH, et al. Randomized phase III KEYNOTE-181 study of pembrolizumab versus chemotherapy in advanced esophageal cancer. J Clin Oncol. (2020) 38:4138–48. doi: 10.1200/JCO.20.01888
72. Bellmunt J, de Wit R, Vaughn DJ, Fradet Y, Lee JL, Fong L, et al. Pembrolizumab as second-line therapy for advanced urothelial carcinoma. N Engl J Med. (2017) 376:1015–26. doi: 10.1056/NEJMoa1613683
73. Galsky MD, Saci A, Szabo PM, Han GC, Grossfeld G, Collette S, et al. Nivolumab in patients with advanced platinum-resistant urothelial carcinoma: Efficacy, safety, and biomarker analyses with extended follow-up from checkMate 275. Clin Cancer Res. (2020) 26:5120–8. doi: 10.1158/1078-0432.CCR-19-4162
74. Rosenberg JE, Hoffman-Censits J, Powles T, van der Heijden MS, Balar AV, Necchi A, et al. Atezolizumab in patients with locally advanced and metastatic urothelial carcinoma who have progressed following treatment with platinum-based chemotherapy: a single-arm, multicentre, phase 2 trial. Lancet. (2016) 387:1909–20. doi: 10.1016/S0140-6736(16)00561-4
75. Powles T, O'Donnell PH, Massard C, Arkenau HT, Friedlander TW, Hoimes CJ, et al. Efficacy and safety of durvalumab in locally advanced or metastatic urothelial carcinoma: Updated results from a phase 1/2 open-label study. JAMA Oncol. (2017) 3:e172411. doi: 10.1001/jamaoncol.2017.2411
76. Antonia SJ, Villegas A, Daniel D, Vicente D, Murakami S, Hui R, et al. Durvalumab after chemoradiotherapy in stage III non–small-cell lung cancer. New Engl J Med. (2017) 377:1919–29. doi: 10.1056/NEJMoa1709937
77. Kim SJ, Yoon DH, Jaccard A, Chng WJ, Lim ST, Hong H, et al. A prognostic index for natural killer cell lymphoma after non-anthracycline-based treatment: a multicentre, retrospective analysis. Lancet Oncol. (2016) 17:389–400. doi: 10.1016/S1470-2045(15)00533-1
78. Zhao Y, Shao Q, Peng G. Exhaustion and senescence: two crucial dysfunctional states of T cells in the tumor microenvironment. Cell Mol Immunol. (2020) 17:27–35. doi: 10.1038/s41423-019-0344-8
79. Johnsrud AJ, Johnsrud JJ, Susanibar SA, Kamimoto JJ, Kothari A, Burgess M, et al. Infectious and immunological sequelae of daratumumab in multiple myeloma. Br J Haematology. (2019) 185:187–9. doi: 10.1111/bjh.15433
80. Malavasi F, Deaglio S, Damle R, Cutrona G, Ferrarini M, Chiorazzi N. CD38 and chronic lymphocytic leukemia: a decade later. Blood. (2011) 118:3470–8. doi: 10.1182/blood-2011-06-275610
81. Ng HHM, Lee RY, Goh S, Tay ISY, Lim X, Lee B, et al. Immunohistochemical scoring of CD38 in the tumor microenvironment predicts responsiveness to anti-PD-1/PD-L1 immunotherapy in hepatocellular carcinoma. J Immunother Cancer. 2020 8(2). doi: 10.1136/jitc-2020-000987
82. Wu P, Zhao L, Chen Y, Xin Z, Lin M, Hao Z, et al. CD38 identifies pre-activated CD8+ T cells which can be reinvigorated by anti-PD-1 blockade in human lung cancer. Cancer Immunology Immunother. (2021) 70:3603–16. doi: 10.1007/s00262-021-02949-w
Keywords: immunotherapy, lymphoma, T cell activation, checkpoint inhibition, combination therapy
Citation: Lee WWL, Lim JQ, Tang TPL, Tan D, Koh SM, Puan KJ, Wang LW, Lim J, Tan KP, Chng WJ, Lim ST, Ong CK and Rotzschke O (2024) Counterproductive effects of anti-CD38 and checkpoint inhibitor for the treatment of NK/T cell lymphoma. Front. Immunol. 15:1346178. doi: 10.3389/fimmu.2024.1346178
Received: 29 November 2023; Accepted: 27 March 2024;
Published: 12 April 2024.
Edited by:
Gaël Roué, Josep Carreras Leukaemia Research Institute (IJC), SpainReviewed by:
Zihang Chen, Sichuan University, ChinaVladimir Otasevic, University Clinical Center of Serbia, Serbia
Qingqing Cai, First Affiliated Hospital of Sun Yat-sen University, China
Govind babu Kanakasetty, HCG Cancer Hospital, India
Copyright © 2024 Lee, Lim, Tang, Tan, Koh, Puan, Wang, Lim, Tan, Chng, Lim, Ong and Rotzschke. This is an open-access article distributed under the terms of the Creative Commons Attribution License (CC BY). The use, distribution or reproduction in other forums is permitted, provided the original author(s) and the copyright owner(s) are credited and that the original publication in this journal is cited, in accordance with accepted academic practice. No use, distribution or reproduction is permitted which does not comply with these terms.
*Correspondence: Olaf Rotzschke, b2xhZl9yb3R6c2Noa2VAaW1tdW5vbC5hLXN0YXIuZWR1LnNn; Choon Kiat Ong, Y21yb2NrQG5jY3MuY29tLnNn
†These authors have contributed equally to this work and share last authorship