- 1Graduate School, Beijing University of Chinese Medicine, Beijing, China
- 2Traditional Chinese Medicine Department of Rheumatism, China-Japan Friendship Hospital, Beijing, China
- 3Traditional Chinese Medicine Department, Peking University Third Hospital, Beijing, China
- 4Beijing Key Laboratory of Immune Inflammatory Disease, China-Japan Friendship Hospital, Beijing, China
Sjögren’s syndrome (SS) is a chronic systemic autoimmune disease that typically presents with lymphocyte, dendritic cell, and macrophage infiltration of exocrine gland ducts and the formation of ectopic germinal centers. The interactions of lymphocyte homing receptors and addressins and chemokines and their receptors, such as α4β7/MAdCAM-1, LFA-1/ICAM-1, CXCL13/CXCR5, CCL25/CCR9, CX3CL1/CX3CR1, play important roles in the migration of inflammatory cells to the focal glands and the promotion of ectopic germinal center formation in SS. A variety of molecules have been shown to be involved in lymphocyte homing, including tumor necrosis factor-α, interferon (IFN)-α, IFN-β, and B cell activating factor. This process mainly involves the Janus kinase-signal transducer and activator of transcription signaling pathway, lymphotoxin-β receptor pathway, and nuclear factor-κB signaling pathway. These findings have led to the development of antibodies to cell adhesion molecules, antagonists of chemokines and their receptors, compounds interfering with chemokine receptor signaling, and gene therapies targeting chemokines and their receptors, providing new targets for the treatment of SS in humans. The aim of this study was to explore the relationship between lymphocyte homing and the pathogenesis of SS, and to provide a review of recent studies addressing lymphocyte homing in targeted therapy for SS.
1 Introduction
Sjögren syndrome (SS) is a common chronic autoimmune disease, associated with other systemic autoimmune diseases such as systemic lupus erythematosus (SLE) and rheumatoid arthritis (RA), and may be comorbid with them (1). SS mainly affects the exocrine glands, especially salivary glands and lacrimal glands, and primarily presents with dry mouth and eyes (2). In severe cases, patients may show damage to extra-glandular organs and systems, including the dermatologic, musculoskeletal, pulmonary, gastrointestinal, hepatobiliary, hematologic, renal, or neurologic systems (3). The more serious aspect of SS is the considerably higher risk of developing malignant non-Hodgkin’s lymphoma (NHL). It occurs in about 2%-5% cases, and it is the main cause of a decreased survival in SS (4). Pathological manifestations of SS exocrine gland tissue include infiltration of CD4+ T and B lymphocytes, altered follicular structure and fibrosis, hypoplasia or loss of glandular function (5, 6). In addition, approximately 25 ± 5% of SS salivary gland will have the formation of ectopic germinal centers (GCs), a specialized site for B cell activation and antibody maturation in non-lymphoid organs (7, 8).
Chemokines and their receptors play regulatory roles in the inflammatory response, and abnormal expression of chemokines has been shown to be associated with the pathogenesis of various autoimmune diseases, including RA, SLE, and Behcet disease. However, the association between chemokines and the pathogenesis of SS has received less attention (9, 10). Chemokines and their receptors have been recently shown to be involved in the lymphocyte homing process in SS, and are closely related to the generation of ectopic lymphoid structures (ELSs), a specialized microenvironment that forms in response to persistent antigenic and inflammatory stimuli in non-lymphoid organs (11, 12). Expression of chemokines promotes the recruitment of lymphoid tissue inducer cells, causing crosstalk between cells and inducing GCs (13). GCs have been shown to be predictive of an increased risk of lymphoma in SS patients. Investigation of the role of chemokines might give clues for a better understanding of the pathophysiology of mechanisms of SS and of SS-associated lymphomagenesis, leading to the identification of new treatment targets in SS. Therefore, a literature review was performed on SS and homing mechanisms; a total of 168 papers were selected for discussion. The aim of this review is to provide an update concerning the targets of lymphocyte homing in SS.
2 Lymphocyte migration molecules
Lymphocyte migration is a form of lymphocyte homing that primarily involves the migration of lymphocytes from the bloodstream to various tissues and organs and is mediated by a series of molecules (14). The chemokines secreted by the cells of various organs in the organism bind to the chemokine receptors expressed by the lymphocytes and promote the binding of the homing receptors on the surface of the lymphocytes to specific addressins, which in turn facilitates the migration of the lymphocytes to the specific tissues and organs. The homing process involves a cascade of migratory molecules, including lymphocyte homing receptors such as selectins/integrins, addressins, chemokines, and their receptors (15). Lymphocyte homing receptors are a class of molecules expressed on the surface of lymphocytes, and include L-selectin, P-selectin, and E-selectin of the selectin family, α4β7 of the integrin family, very late activation antigen VLA-4 (α4β1), and the lymphocyte function-associated antigen LFA-1 (αLβ2), whose interactions with the corresponding addressins mediate the processes of adhesion and efflux between lymphocytes and endothelial cells (16, 17). Addressins are adhesion molecules expressed by vascular endothelial cells, including the immunoglobulin superfamily of mucosal addressin cell adhesion molecule-1 (MadCAM-1), intercellular cell adhesion molecule-1 (ICAM-1), and vascular cell adhesion molecule (VCAM-1), E calreticulin of the calreticulin family, and a number of unclassified adhesion molecules such as mucosal vascular addressin (Mad) and peripheral node addressin (PNAD) (18, 19).
Chemokines are a series of small molecular mass proteins that play important roles in the generation of lymphoid organs under physiological and pathological conditions, as well as in the differentiation, homing, and recirculation of lymphocyte subpopulations in the immune response (20). Many chemokines are associated with lymphocyte homing, and they vary from one tissue site to another. Chemokines are classified into four subclasses, C, CC, CXC, and CX3C, according to the order of the two conserved cysteines at their amino-terminal end, of which CC and CXC are considered to be the two major chemokine subclasses (21). Chemokines can be expressed constitutively and inducibly. The vast majority of constitutive chemokines have specific receptors, whereas inducible chemokines show shared receptors. Each subpopulation of immune cells shows a different pattern of chemokine receptor expression, which allows them to respond differently to chemokines and migrate according to the specific needs of each environment (22). Chemokines and their receptors constitute a complex network widely involved in cellular immunity, growth and development, and inflammation, playing diverse roles in chronic infectious diseases, autoimmune diseases, and tumors (23).
3 Bioassays for the determination of chemokines
In recent years, saliva and tears have emerged as promising fluids for the important diagnosis of SS through the detection of chemokine biomarkers therein, which has had a tremendous impact on the development of multiple assay platforms (24). However, bioassays specifically designed to selectively determine one or more chemokines are scarce. Methods to determine chemokine levels are mostly based on immunoassays such as ELISA (25). Western blotting assays have also been used to study the expression of various chemokines in biological fluids (26). However, these techniques have limited sensitivity in detecting very small amounts of target compounds. Most of the immunoassays require long assay times, do not allow real-time study of chemokine secretion, and are also dependent on specific and sensitive immunoreagents, thus not allowing high-throughput analysis of protein expression in tissues (27). Proteomics is a promising tool, and tear proteomics has made relatively less progress than saliva proteomics for technical reasons (28). Salivary proteomics not only identifies chemokine biomarkers, but also helps to elucidate the molecular pathways behind disease pathogenesis, which allows for better stratification of patients and potentially opens up new avenues for targeted therapies (29). However, limitations regarding the necessary technologies for saliva collection and storage and different protocols for proteomic and molecular analysis need to be urgently addressed (30). In addition, electrochemical and optical biosensors have been applied for chemokine determination. Although they are highly sensitive and selective, they are more susceptible to physical damage and environmental interferences, and are more expensive to perform and analyze (31).
4 Lymphocytic infiltration of exocrine glands in SS
The histologic features of exocrine glands in SS include the presence of lymphocytic infiltration around ductal cells, with a minority of other immune cells such as macrophages, dendritic cells (DCs), and natural killer (NK) cells (32). Inflammatory lesions usually show focal dense aggregates, and the composition of the lesion varies with the severity of the infiltrate, with T cells predominating in mild infiltrates and B cells in severe infiltrates (33). CD4+ T cells are the main infiltrating T cells, with helper T cell (Th)1, Th2, Th17, and follicular helper T (Tfh) cells (34). Th1 cytokines are represented by interferon (IFN) and tumor necrosis factor (TNF), whose expression has been associated with glandular tissue damage; Th2 cells play a key role in maintaining B cell function; Th17 cells mainly secrete interleukin (IL)-17, which can induce the expression of a variety of cytokines, such as pro-inflammatory cytokines, chemokines, and matrix metalloproteins (MMPs); and Tfh cells are essential for GC formation and B cell activation (35–37). Although CD4+ T cells are a key factor in the immunopathogenesis of SS, various studies have shown that CD8+ T lymphocytes contribute to vesicular damage in exocrine glands (38). CD27+ memory B cells, marginal zone B cells, and plasma cells are all key B cell subpopulations involved in the pathogenesis of SS, and play a role in promoting SS pathogenesis in terms of antigen presentation and cytokines (39).
In summary, the pathological changes in SS are lymphocytic infiltration of exocrine glands, whereas chemokines and their receptors are involved in causing aggregation of lymphoid cells and induction of the chronic inflammatory response. The levels of the chemokines CCL19, CXCL8, CXCL9, CXCL10, CXCL11, and CXCL17 have been shown to be significantly increased in the saliva or tears of SS patients, and those of the chemokines CCL5, CCL17, CCL21, CCL22, CCL25, CXCL1, and CXCL12 have been shown to be upregulated in the salivary glands of SS patients. In addition, both CXCL13 and CX3CL1 levels were elevated in the serum of SS patients. Thus, aberrantly expressed chemokines in SS patients selectively induce and regulate lymphoid cell subsets, which regulate the circulation of lymphocytes between target tissues, blood, and secondary lymphoid organs to form ectopic GCs and participate in their disease processes.
5 Lymphocyte homing and SS
Multiple chronic inflammatory diseases and autoimmune diseases such as SS, RA, multiple sclerosis, and Crohn’s disease involve the entry of lymphocytes in the blood into the lymphoid tissues or inflammatory sites. Selectins mediate initial and rolling adhesion is the first step in the process: the mobile lymphocytes initially tether to the vascular wall, and then roll along the wall surface of the blood vessel. After activation by chemokines, the stimulation of cell surface integrins is the second step. Integrins mediate stable adhesion and migration is the third step: activated α4β7, VLA-4, and LFA-1 on the surface of lymphocytes bind tightly to the adhesion molecules MAdCAM-1, VCAM-1, and ICAM-1, respectively, expressed on endothelial cells, which reduces the rolling speed of lymphocytes and promotes the occurrence of firm adhesion, eventually resulting in migration to specific tissues.
5.1 Abnormal expression of lymphocyte homing receptor and addressin
5.1.1 L-selectin/PNAD
L-selectin/PNAD L-selectin is a cell adhesion molecule expressed on the surface of naïve and central memory T cells, and is involved in the initial attachment of lymphocytes to high endothelial venules (HEVs) in lymph nodes. PNAD, a sulfated and fucosylated glycoprotein, is expressed on HEVs, and facilitates the entry of naïve T cells into lymphoid organs at non-mucosal tissue sites. L-selectin/PNAD is involved in the tethering and rolling of lymphocytes along the HEV in lymphoid tissues. The salivary levels of soluble L-selectin and IL-7 have been shown to be higher in Indian patients with SS (40). SS patients with higher serum concentrations of circulating L-selectin are more likely to have Raynaud’s phenomenon, autoimmune thyroiditis, and elevated levels of rheumatoid factor (RF) (41).
5.1.2 α4β7/MAdCAM-1
α4β7 belongs to the integrin family, and is expressed on initial T and B lymphocytes, NK cells, monocytes, macrophages, and eosinophils. MAdCAM-1, the predominant ligand for α4β7, is expressed by mucosal endothelial cells of mesenteric lymph nodes, Peyer’s patches, and associated lymphoid tissues (42). α4β7/MAdCAM-1 mediates rolling adhesion of lymphocytes to the capillary lumen on mucosal tissues, followed by stable adhesion to endothelial cells. One study revealed a significant increase in the α4β7-negative cell population in IL-17-expressing cells in the salivary glands, peripheral blood, and spleen of aged NOD mice, and the marked increase in α4β7-negative IL-17-expressing cells in the salivary glands may be involved in the onset and development of SS (43).
5.1.3 VLA-4/VCAM-1
VLA-4 is a heterodimer of α4 and β1, also known as leukocyte integrins or CD49d/CD29, which is expressed on the surface of most lymphocytes, monocytes, eosinophils, and basophils, while VCAM-1 is a member of the immunoglobulin superfamily that is expressed widely in macrophages, DCs, myeloid fibroblasts, and myocytes. VLA-4 selectively promotes stable adhesion of lymphocytes through the specific binding of VCAM-1. Animal experiments verified that the vascular endothelium in the inflamed region of the lacrimal gland in nonobese diabetic (NOD) mice express VCAM-1 and PAND, and that the majority of lymphocytes in the inflamed glands express a4 integrin, L-selectin, and LFA-1 (44). VCAM-1 staining indicated that VCAM-1 may be more important in determining the distribution of B than T lymphocytes in lymphocytic infiltration of non-lymphoid tissue in SS patients, and thus GCs may form by immigration of B cells via VCAM-1+ vessels at the center of T cell aggregates (45).
5.1.4 LFA-1/ICAM-1
LFA-1 is composed of α-chain CD11a and β-chain CD18, which are distributed on the surface of T and B lymphocytes, monocytes, macrophages, and neutrophils, playing important roles in lymphocyte passage through the vascular endothelium. ICAM-1 also belongs to the immunoglobulin superfamily, and is distributed on the surface of fibroblasts, vascular endothelial cells, and activated lymphocytes. LFA-1/ICAM-1 mainly mediates the migration of lymphocytes toward the transvascular endothelium, and their eventual passage through the vascular endothelium to the site of injury. It has shown that in the lacrimal gland of NOD mice, LFA-1 and ICAM-1 are upregulated in lacrimal acinar cells and infiltrating lymphocytes (46). Previous study has also reported that ICAM-1 expression on epithelial cells is moderately upregulated in the salivary gland microenvironment of SS patients (47). Tissue biopsies of salivary glands from SS patients have suggested that cytokine-mediated upregulation of VCAM-1 and ICAM-1 promotes the recruitment of T cells expressing VLA-4 and LFA-1 (48). Further in vitro experiments revealed that TNF-induced apoptotic human salivary gland cells significantly upregulated ICAM-1 and CCL20 expression, and ultimately triggered apoptosis and tissue destruction (49).
5.2 Abnormal expression of chemokines and their receptors
5.2.1 CXCL13/CXCR5
CXCL13, a member of the CXC chemokine family, also known as B cell-attracting chemokine-1 (BCA-1) or B lymphocyte chemoattractant (BLC), is the major active chemokine on mature B cells. CXCL13 is constitutively expressed in secondary lymphoid organs such as the spleen and lymph nodes. CXCR5 is the corresponding receptor for CXCL13, and is predominantly expressed on the surface of B and Tfh cells. CXCL13 and CXCR5 are associated with B lymphocytes, Tfh cell homing, and the formation of B cell follicular zones in lymphoid organs. CXCL13 is recognized as a biomarker of SS severity and correlates with disease activity, including clinical parameters. Its expression in human serum and saliva has been proven to increase with disease progression (50), with a marked increase in the number of CXCR5-expressing cells infiltrating focal salivary gland and interstitium (51). CXCL13 has also been identified as a biomarker for histological involvement in SS. Recent studies have shown that elevated serum levels rather than saliva levels of CXCL13 in SS patients are correlated with the histomorphometric features of the salivary glands, including the size of aggregates and the formation of GCs (52, 53), as well as with the risk and incidence of NHL (54, 55). CXCL13 plays a crucial role in lymphocyte neogenesis, maintenance of secondary lymphoid tissue structure, and immune response (56). It is also involved in the initiation and organization of ELSs. In inflammatory tissues, heightened CXCL13 expression regulates the infiltration and localization of B cells, as well as their movement within ectopic GCs (57). Furthermore, CXCL13 can attract Tfh cells towards B cells in ELSs, facilitating B-cell help (58). Collectively, CXCL13 is currently the most extensively studied and most promising chemokine in SS, and may serve as a reliable biomarker for monitoring and diagnosis of SS.
5.2.2 CXCL12/CXCR4
CXCL12 was originally discovered as a pre-B cell growth factor, and is indispensable in lymphangiogenesis. It can be secreted by bone marrow stromal cells, and is therefore also named stromal cell-derived factor-1 (SDF-1). Human CXCL12 is chemotactic for CD4+ T lymphocytes, monocytes, neutrophils and DCs, all of which also express CXCR4. CXCL12/CXCR4 is an important chemokine for the aggregation of B cells into lymphoid follicles, and for the survival of malignant B cells in the salivary glands of mucosa-associated lymphoid tissue (MALT) lymphoma (56). CXCL12 was predominantly observed in ducts and malignant B cells infiltrating the salivary glands of SS patients with MALT lymphoma; levels of CXCL12 were elevated in MALT lymphoma and isolated tumor cells (56). Upregulation of CXCL12, along with elevated CXCR4 expression on CD4+ effector memory T (TEM) cells, has been observed in epithelial cells adjacent to lymphocyte-infiltrated areas of salivary and lacrimal glands in alymphoplasia (aly)/aly mice (59). An in vitro Transwell migration assay verified that the migration of (aly)/aly mice CD4+ TEM cells was significantly elevated in response to CXCL12, and the migration response increased with increasing CXCL12 concentration (60).
5.2.3 CCL21/CCR7
CCL21, also known as exodus-2 and secondary lymphoid chemokine (SLC), is a small homeostatic cytokine belonging to the CC chemokine family. Human CCL21 is highly expressed in secondary lymphoid tissues such as the lymph nodes, appendix, and spleen. CCR7 is expressed predominantly on the surface of T cells, B cells, activated NK cells, and DCs, where is it frequently involved in promoting migration. CCL21 binding to a specific receptor, CCR7, synergistically regulates the initiation of immune response and induction of immune tolerance. High levels of CCL21/CCR7 expression in the salivary glands of SS patients were associated with elevated erythrocyte sedimentation rate (ESR), IgG and RF levels, anti-Ro/SSA and La/SSB antibody titers, and a higher focus score and European League Against Rheumatism SS disease activity index (ESSDAI) value on biopsy (61, 62). In patients with extra-glandular manifestations of SS, the prevalence of lymphadenopathy increased with elevated CCL21 levels (63). It has been shown that a strong up-regulation of CCL21 takes place in correlation with the presence of a high number of activated T cells infiltrating the glands, which suggested a prominent role for CCL21 in the SS-associated lymphomagenesis by recruiting and chronically activating T cells, which, once stimulated, provide a stimulus for the expansion and survival of B cells within the reaction zone (56). Hence, CCL21 is directly involved in the construction of ectopic reactive lymphoid tissue.
5.2.4 CCL25/CCR9
CCL25, the thymus-expressed chemokine (TECK), is expressed primarily in the thymus and intestinal epithelial cells, but can also be produced by parenchymal cells, and migrates immature T cells to the thymus for maturation and release. CCL25 is found in the oral mucosa of patients with SS, and is primarily produced by epithelial cells (64). CCL25 binds to the chemokine receptor CCR9, which is expressed on the cell membranes of lymphocytes, monocytes, DCs, and neutrophils. CCR9-expressing cells were observed in the vicinity of epithelial cells in mucosal tissues (65). A study investigating the local and systemic CCL25/CCR9 axis in SS patients found that the levels of CCR9-expressing Th cells and their ligand CCL25 were increased in the salivary glands of SS patients; enhanced CCL25 expression attracted circulating CCR9-expressing Th cells (66). These CCR9-expressing Th cells were highly responsive to IL-7; secreted high levels of IFN-γ, IL-21, and IL-17; and effectively stimulated B cells, confirming that the CCL25/CCR9 axis plays an important role in the immunopathology of SS (65).
5.2.5 CX3CL1/CX3CR1
CX3CL1 is the only member of the CX3C subfamily of chemokines, and its specific endogenous receptor is CX3CR1. CX3CL1 exists in a membrane-bound and a shed form, and the membrane-bound form can be expressed on epithelial cells and vascular endothelial cells (67). It was shown that CX3CL1 induction, cleavage and recruitment of CX3CR1-expressing immune cells were associated with cathepsin S activity, which was significantly and specifically increased in SS patient tears (68). Soluble CX3CL1, which is cleaved from membrane-bound CX3CL1 by proteases including cathepsin S, mediates chemotaxis of CX3CR1 expressing cells. CX3CR1 is predominantly expressed on monocytes, macrophages, neutrophils, T cells, and activated NK cells. CX3CL1/CX3CR1 is closely associated with leukocyte capture and adhesion and chemotaxis of inactivated peripheral CD8+ T cells, NK cells, and monocytes. CX3CL1 levels were found to be significantly elevated in lacrimal gland acinar cells, and the levels of CX3CR1-expressing T cells and macrophages were significantly increased in the lacrimal glands of NOD mice (68). In addition, CX3CL1 protein levels were elevated in the serum of SS patients; transcriptional profiling of peripheral B cells showed upregulation of CX3CR1 expression (69); and histological assessments showed upregulation of the mRNA levels of CX3CL1 and CX3CR1 in the salivary glands of SS patients (70). Moreover, serum CX3CL1 levels were significantly higher in SS patients with extra-glandular manifestations than in those without extra-glandular manifestations (71). As a contributor to the formation of ectopic GC in SS, CX3CL1/CX3CR1 may become a new tool for the evaluation and diagnosis of SS.
In addition to these chemokines and their receptors, salivary gland ductal cells and inflammatory cells of SS patients showed reduced expression of CCL28 mRNA and increased expression of CCL21/CCR7, CCL5/CCR1 and CXCL1/CXCR2, accompanied by more severe histopathologic damage (72, 73). SS patients showed low levels of CCL2, but markedly high levels of CXCL17 and CXCL8 in their tears or saliva, with CCL2 associated with positive ocular tests (74–76). Another immunohistochemical study demonstrated that the ratio of CCL2 and CCL12 mRNA transcripts in the lacrimal gland lesion cells of MRL/MpJ mice increased with age and disease extent (77). Furthermore, the expression levels of CXCL9, CXCL10, CXCL11, and CXCR3 also increased in the tear film and ocular surface of SS patients, with the levels of CXCL11 and CXCL10 correlating with worsening ocular symptoms (78). CCL22 and CCL17 were detected in human salivary gland ductal epithelial cells by immunohistochemical analysis, and CCR4 was detected on lymphocytes heavily infiltrated in the glands (79). CCL19/CCR7 expression was found to be up-regulated in the salivary glands of SS patients compared to non-SS (61). Subsequently, in SS model mice, CCL22 was found to enhance the migratory activity of CD4+ T cells by increasing the expression of CCR4 on T cells to enhance salivary gland T cell responses (80). CXCL9 and CCL19 expression were significantly upregulated in the lacrimal glands of NOD mice, and their expression was regulated by IFN signaling (81). The chemokines and their receptors mentioned in the available literature and their main findings in SS patients (Table 1) and murine models (Table 2) are listed.
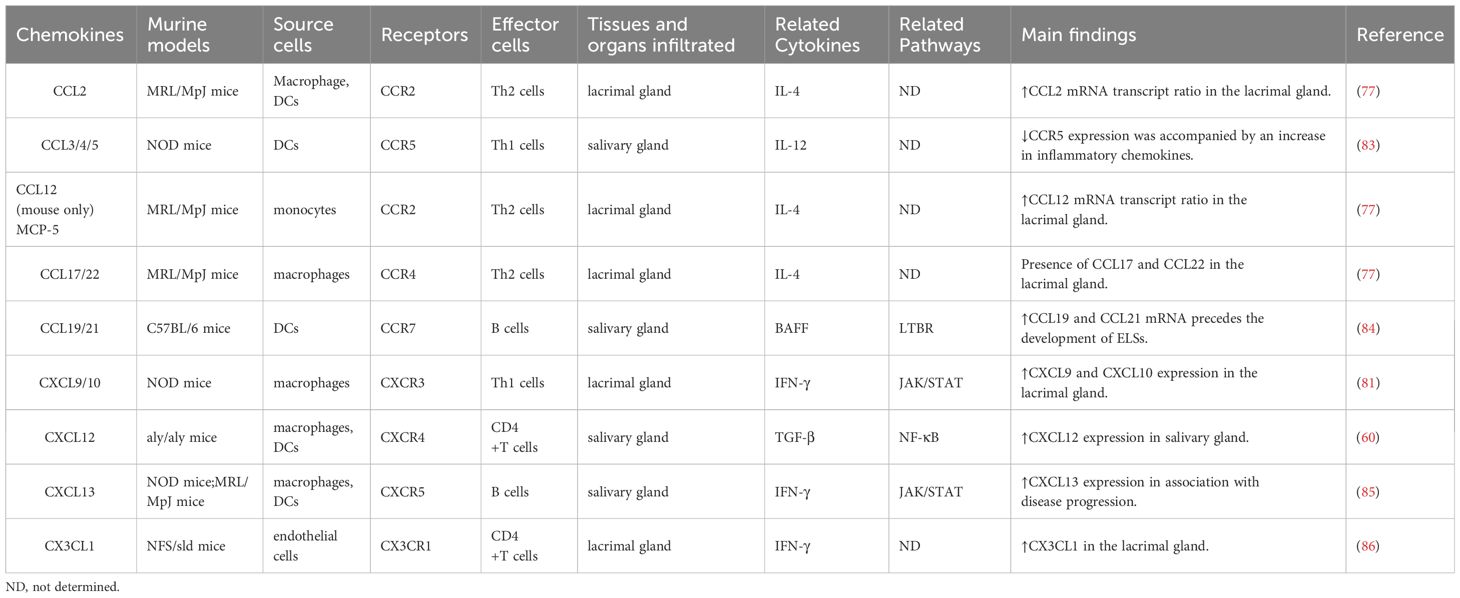
Table 2 Chemokines associated with murine models of Sjögren’s-like disease and their chemotactic effects.
6 Lymphocyte homing and SS extra-glandular damage
SS is a diffuse connective tissue disease that can involve all systems of the body, and may present with extra-glandular organ involvement, such as interstitial lung disease (ILD), interstitial nephritis, annular erythema, primary biliary cirrhosis, and arthritis. This extra-glandular organ involvement may be related to lymphocyte leakage to the corresponding tissues and organs. Bronchus-associated lymphoid tissue is common in patients with SS lung complications, and the development of this tissue is associated with increased expression of lymphoid tissue chemokines such as CXCL13 and CCL21 (87). In mice injected with the stimulator of interferon gene agonist that showed lymphocytic infiltration in the peri-bronchial regions, the lungs showed increased expression of multiple chemokines (88). The atypical chemokine receptor CCX-CKR regulates the bioavailability of CCL19, CCL21, and CCL25. Lymphocyte infiltration has been observed in the salivary glands and liver of CCX-CKR gene-deficient mice with an increased incidence of SS-like liver lesions (89). SS patients with annular erythema showed strong expression of LFA-1/ICAM-1 on endothelial cells and monocytes, which may explain why most lymphocytes are localized around blood vessels (90). Additionally, LFA-1 and ICAM-1 showed higher expression in activated T cells in the joint fluid of patients with SS-associated arthritis (91). SS patients often present with reduced corneal innervation and corneal epithelial cell proliferation. Corneal epithelial cell proliferation in SS model mice has been reported to be associated with increased expression of CXCL1 in corneal epithelial mRNA (92). Another case-control study noted that female patients with SS showed elevated levels of CXCL10 in endocervical swab samples and increased lymphocytic infiltration in the vagina, which may account for vaginal dryness (93).
7 Lymphocyte homing targeted therapy in SS
Recent advancements in the field of lymphocyte homing have provided new insights into the pathogenesis of SS. Consequently, antibodies to cell adhesion molecules, antagonists of chemokines and their receptors, compounds that interfere with chemokine receptor signaling, and gene therapies targeting chemokines and their receptors have been gradually identified, providing new targets for human treatment of SS.
Retinoic acid is an important factor in maintaining intestinal homeostasis through direct regulation of effector cytokines (94). Inhibition or upregulation of retinoic acid leads to differential expression of CCR9 and α4β7 in Th17 cells (95). There are data suggesting that SS patients have lower retinoic acid concentrations and higher IL-17 expression levels in their serum compared to healthy controls, and NOD mice treated with retinoic acid showed reduced numbers of α4β7-negative Th17 cells and lower levels of IL-17 in vivo (43). These findings demonstrate the therapeutic potential of retinoic acid in SS, but more clinical validation is needed.
7.1 Antibodies to cell adhesion molecules
A study evaluating the efficacy of blocking cell adhesion molecules in vivo found that the combination of the ICAM-1 antibody with the anti-LFA-1 antibody prevented autoimmune lacrimal gland disease in SS model mice (96). Thus, the ICAM-1/LFA-1 pathway may play a key role in the development of T-cell-mediated inflammation in the lacrimal gland. On the basis of this finding, another study introduced a new tool, the multivalent biopolymeric nanoparticle (IBP-SI), which can inhibit the adhesion of lymphocytes to cells expressing high levels of ICAM-1 in vitro, disrupt the interaction between ICAM-1 and LFA-1, and can be utilized as an in vivo assessment tool or a potential therapeutic agent (46).
7.2 Anti-TNF-α treatment
TNF-α is a pro-inflammatory cytokine that is secreted in high amounts in many autoimmune or inflammatory diseases such as RA, Crohn’s disease, and SS (97). TNF-α promotes the expression of pro-inflammatory cytokines such as CXCR3, and promotes lymphocyte infiltration into the target organs, leading to the development of these diseases (98). TNF-α also destroys glands by inducing apoptosis of human salivary gland cells either alone or in combination with other inflammatory cytokines (49). Among patients with SS, TNF-α expression is elevated in salivary glands and serum (99). TNF-α-dependent activation of the exogenous apoptotic pathway was found to lead to upregulation of ICAM-1 and CCL20 in human salivary gland endothelial cells in vitro, which in turn promoted the infiltration of tissues by immune cells, mainly CD8+ lymphocytes (49). A study using SS model mice showed that TNF-α expression in the submandibular gland increased with the spontaneous development of SS-like exocrinopathy in NOD mice, and was accompanied by major infiltration of T and B cells (100). Thus, TNF-α may be an important pathogenic mediator in the pathogenesis of SS. Laboratory studies suggest that anti-TNF-α treatment may have a therapeutic effect on SS (100, 101). NOD mice administered neutralizing anti-TNF-α antibodies during the pre-morbid phase of the disease showed a marked improvement in salivary secretion, suggesting that the clinical signs of SS were alleviated (102). Another study in NOD mice revealed that TNF-α blockers could reduce the number of infiltrating T and B cells in the submandibular gland by downregulating the local expression of CXCL9 and CXCL13, and thereby alleviate glandular injury (100). Nevertheless, the findings from clinical trials investigating the efficacy of anti-TNF-α treatment in SS have been disheartening. Several randomized, double-blind, placebo-controlled studies examining the effectiveness of anti-TNF drugs such as infliximab and etanercept have failed to demonstrate any evidence of their efficacy in SS (103, 104). Consequently, further investigation is warranted to ascertain the potential usefulness of anti-TNF therapy in the clinical management of SS.
7.3 Inhibition of JAK/STAT signaling: baricitinib, CP-25
Type I interferon (IFN-α and IFN-β) and type II interferon (IFN-γ) target genes are upregulated in the peripheral blood and salivary glands of SS patients, and play central roles in SS pathogenesis (105). In the salivary glands of SS patients, DCs are the main source of IFN-α, whereas CD4+ T cells and NK cells are the main producers of IFN-γ (106). Recent studies have shown that IFN-α and IFN-γ are involved in the aggregation of infiltrating immune cells in the salivary glands of SS patients through the Janus kinase (JAK)-signal transducer and activator of transcription (STAT) signaling pathway by promoting the production of CXCL13 and CXCL10, respectively (107, 108). The JAK family of cytoplasmic protein tyrosine kinases includes JAK1, JAK2, JAK3, and tyrosine kinase 2. JAK binds to type 1 and type 2 cytokine receptors and transmits extracellular cytokine signals to activate STAT proteins, which translocate to the nucleus and regulate the transcription of effector genes (109). JAK-STAT signaling is initiated by over 50 cytokines binding to their respective receptors on the cell surface (110). Therefore, JAK is an excellent target for the treatment of various cytokine-mediated diseases. Baricitinib is a selective JAK1/2 inhibitor approved for the treatment of moderate-to-severely active RA (111). Baricitinib has been shown to inhibit IFN-γ-induced CXCL10 expression and attenuate immune cell chemotaxis through inhibition of JAK/STAT signaling (112). Very recently, the efficacy and safety of baricitinib for active SS patients have been explored in a pilot non-controlled trial. Baricitinib contributes to improving disease activity and main clinical manifestations, promising for the treatment of SS (113). The main active ingredient of total paeoniflorin is paeoniflorin (Pae), which has been used clinically for the treatment of autoimmune diseases (114). Paeoniflorin-6’-O-benzene sulfonate (CP-25) is a drug obtained by esterification of Pae that shows better lipid solubility and bioavailability than Pae (115). In vitro studies have revealed that CP-25 can negatively regulate the JAK1-STAT1/2 pathway, counteracting CXCL13 secretion and downregulating CXCL13 expression, and impede the migration of lymphocytes to the salivary glands (116, 117). These findings provide an experimental basis for the evaluation of CP-25 as a potential drug for the treatment of SS.
7.4 LTBR antagonists: baminercept, Rituximab
B cell-activating factor (BAFF), also known as B lymphocyte-stimulating factor, is involved in B cell survival and humoral immune response, and plays a key role in B cell homeostasis (118). Elevated levels of BAFF have been observed in the serum, saliva and salivary glands of SS patients (119). In addition, higher levels of BAFF have been observed in the salivary glands of patients with GCs (120). In vivo experiments confirmed that NOD mice treated with soluble BAFF receptor (BAFFR) and anti-CXCL13 antibody do not develop salivary dysfunction, and that blocking BAFFR attenuates SS disease progression and may be an effective therapeutic strategy for SS (121). The lymphotoxin-beta receptor (LTBR) pathway has been associated with the appearance of ELSs at sites of chronic inflammation in a variety of autoimmune diseases, and has been shown to regulate the expression levels of CXCL13, CCL19, and PNAd (122, 123). Evidence from animal models and SS patients suggests that CXCL13 and the LTBR receptor pathway are required for the development of ELSs and may be effective therapeutic targets for SS (124). Blockade of LTBR reduced CXCL13 protein expression in the lacrimal gland of NOD mice, and CCL19 expression was significantly inhibited in the infiltration zone of salivary glands (125). LTBR antagonists inhibited HEV addressin expression and lymphocyte infiltration in the diseased glands, with beneficial effects on tear and saliva secretion and the integrity of the ocular surface (126). Baminercept is a lymphotoxin-beta receptor IgG fusion protein (LTBR-Ig). A multicenter trial suggested that baminercept significantly reduced the plasma levels of CXCL13 and altered the number of circulating B and T cells in SS patients, but failed to significantly improve glandular and extra-glandular disease in SS patients (127). Further observations with expanded samples are needed in future studies. Rituximab (RTX), a chimeric humanized monoclonal anti-CD20 antibody, inhibits B cell activation, proliferation and differentiation, and acts as a B cell scavenger. A prospective, multicenter, follow-up study demonstrated that B cell depletion therapy with RTX could restore B cell disorders by decreasing salivary gland LTB mRNA levels, modulating CXCL13/CXCR5 interactions, eliminating ELSs, and decreasing immune infiltration and lymphoid organization in target tissues (128). However, a double blind study has also demonstrated that RTX does not yield a substantial improvement in SS-related symptoms and immunological parameters of SS, and that the association is primarily limited to fatigue (129). Hence, further researches are justified to clarify the therapeutic efficacy of RTX in the treatment of SS.
7.5 Blockade of NF-κB pathway: AMD3100, A20
NF-κB plays a key role in the regulation of many inflammatory processes of immune cells (130). Two NF-κB signaling pathways exist in the immune cells: a classical pathway initiated by the NF-κB1 complex and an alternative nonclassical pathway initiated by the NF-κB2 complex (131). A previous study showed that activation of the NF-κB2 pathway in (aly)/aly mice negatively regulates TGF-β signaling, whereas TGF-β upregulates the expression of CXCR4 and regulates the migration of T cells toward autoimmune targets, leading to damage similar to that seen in the early histopathological stages of SS (132). The CXCR4 antagonist AMD3100 inhibits autoimmune lesions in (aly)/aly mice by reducing TEM cell infiltration (60). Knockdown of the NF-κB pathway inhibitor A20 in mouse submandibular gland epithelial cells resulted in upregulation of CXCR4 expression, triggering T lymphocyte infiltration and formation of immune foci (133). NF-κB2 controls the migratory activity of TEM by regulating the expression of CXCR4, which may be a potential therapeutic target for the treatment of SS.
Notably, progress in the field of lymphocyte homing has led to the development of novel targets and drugs with therapeutic potential. For example, abatacept treatment reduces the number of activated circulating Tfh cells, thereby contributing to the attenuation of Tfh cell-dependent B cell hyperactivity in SS (134); disintegrin and metalloproteinase-17 (ADAM17) inhibitors abrogate CXCL1/CXCR2 interactions to block the inflammatory response in SS (135); miR-744-5p may be a potential therapeutic target to ameliorate ocular inflammation in SS patients with dry eye (136); and poly (ADP-ribose) polymerase family member 9 (PARP-9) is a regulator of immune cell infiltration during SS progression (137). Nevertheless, most of these candidates are still in the preclinical stage, and more research efforts are needed to obtain evidence for their use in SS treatment. In summary, the development of new targets that can modulate lymphocyte migration molecules is a promising strategy for the treatment of SS (Figure 1).
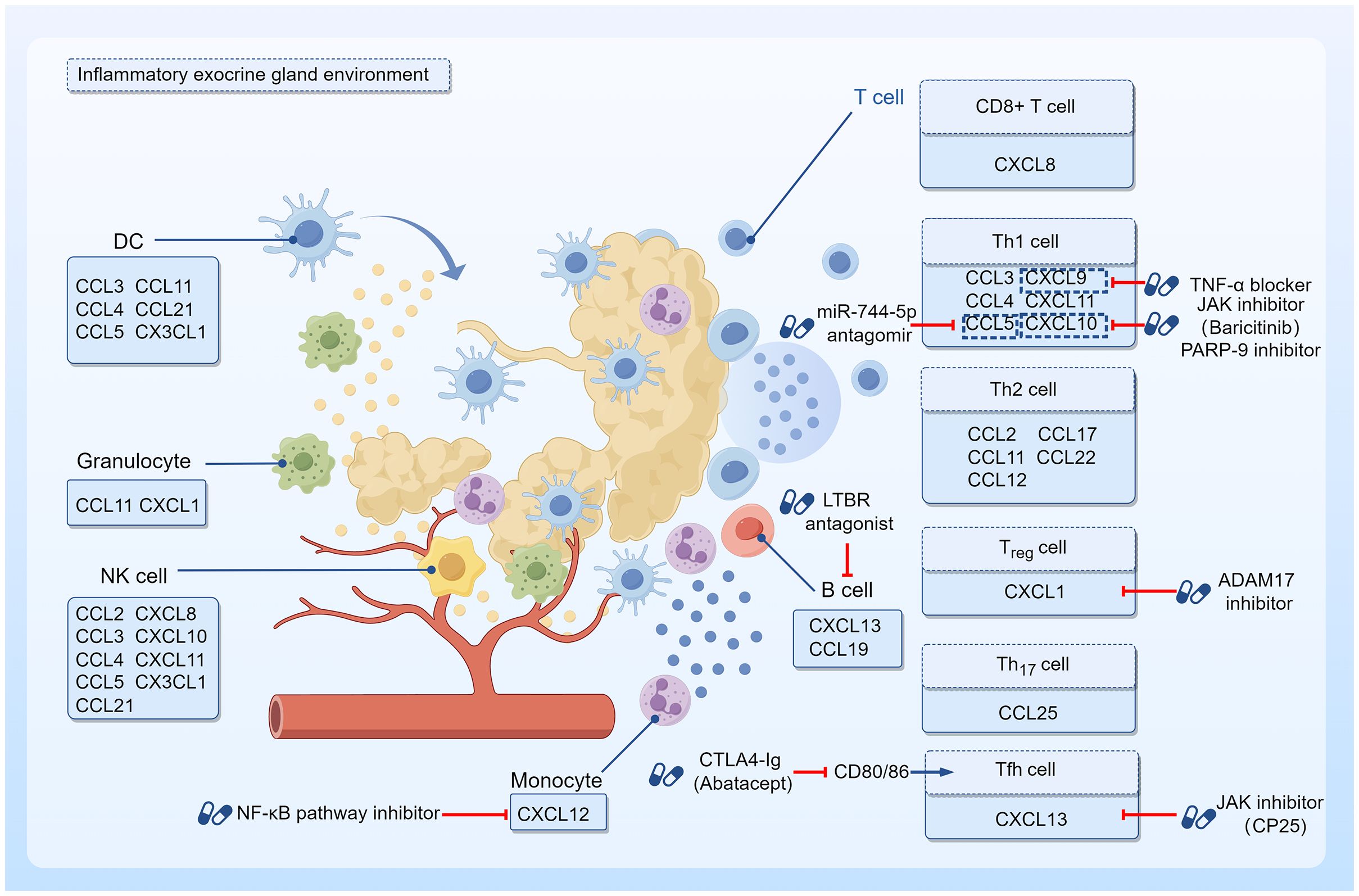
Figure 1 Targeted therapy with chemokines and their effector cells. The inflammatory environment of SS exocrine glands shows infiltration of T and B lymphocytes around ductal cells with a few other immune cells such as monocytes, granulocytes, DCs, and NK cells. As chemokine effector cells, they are able to express specific chemokine receptors that bind to the corresponding chemokines, thus showing chemotactic properties. Drugs with the potential to prevent chemokine effector cells from infiltrating into tissues and organs have been investigated on this basis. Jak inhibitor CP-25 could downregulate CXCL13 expression, and impede the migration of lymphocytes to the salivary gland. Baricitinib, another Jak inhibitor, suppressed IFN-γ-induced CXCL10 expression and attenuated immune cell chemotaxis. PARP-9 inhibitor also downregulated CXCL10 and reduced immune cell infiltration during SS progression. CTLA4-Ig treatment inhibited CD80/86 and reduced the number of activated circulating Tfh cells. LTBR antagonists reduced CXCL13 protein expression. NF-κB pathway inhibitors downregulated CXCL12 receptor expression in monocytes. TNF-α blockers could downregulate the local expression of CXCL9. The use of a miR-744-5p antagomir decreased the levels of the CCL5 in the inflammatory milieu. ADAM17 inhibitors abrogated CXCL1/CXCR2 interactions to block the inflammatory response.
8 Conclusions
Lymphocyte homing is a complex process involving multiple molecules, and the migration associated with this process is extensive and multisite. SS pathogenesis is mainly related to the abnormal infiltration of lymphocytes into exocrine glands, and SS treatments targeting lymphocyte homing are a hotspot of current research. In the pathogenesis of SS, the interaction of lymphocyte homing receptors and addressins such as L-selectin/PNAD, α4β7/MAdCAM-1, VLA-4/VCAM-1, LFA-1/ICAM-1, and chemokines and their receptors such as CXCL13/CXCR5, CXCL12/CXCR4, CCL21/CCR7, CCL25/CCR9, CX3CL1/CX3CR1 regulate lymphocyte migration to the corresponding tissues and organs. Studies have shown that a variety of molecules are involved in lymphocyte homing, including TNF-α, IFN-α, IFN-β, and BAFF, and the process mainly involves the JAK-STAT signaling pathway, the LTBR pathway, and the NF-κB signaling pathway. Blocking lymphocyte homing can alleviate the disease, but considering the complexity of this process, the specific molecular mechanisms need to be explored more deeply to identify more specific molecules to provide targeted therapy for SS treatment.
Author contributions
JHL: Data curation, Writing – original draft. X-BY: Writing – original draft. ZH: Writing – original draft. QH: Writing – original draft. JY: Writing – original draft. YZ: Writing – original draft. JC: Writing – review & editing. WS: Writing – review & editing. JL: Conceptualization, Writing – review & editing. QT: Conceptualization, Writing – review & editing.
Funding
The author(s) declare financial support was received for the research, authorship, and/or publication of this article. This work was supported by Capital's Funds for Health Improvement and Research (2024-1-4065), Rheumatology Branch of China Association of Chinese Medicine Youth Pei Ying Project (No.202327-007), National High Level Hospital Clinical Research Funding (2022-NHLHCRF-LX-02-0103), Elite Medical Professionals project of the China-Japan Friendship Hospital (ZRJY2021-QM14), and Beijing TCM science and technology development fund project (BJZYQN-2023-04).
Conflict of interest
The authors declare that the research was conducted in the absence of any commercial or financial relationships that could be construed as a potential conflict of interest.
Publisher’s note
All claims expressed in this article are solely those of the authors and do not necessarily represent those of their affiliated organizations, or those of the publisher, the editors and the reviewers. Any product that may be evaluated in this article, or claim that may be made by its manufacturer, is not guaranteed or endorsed by the publisher.
Supplementary material
The Supplementary Material for this article can be found online at: https://www.frontiersin.org/articles/10.3389/fimmu.2024.1345381/full#supplementary-material
References
1. Kollert F, Fisher BA. Equal rights in autoimmunity: is sjögren’s syndrome ever 'Secondary'? Rheumatol (Oxford). (2020) 59:1218–25. doi: 10.1093/rheumatology/keaa009
2. Negrini S, Emmi G, Greco M, Borro M, Sardanelli F, Murdaca G, et al. Sjögren’s syndrome: A systemic autoimmune disease. Clin Exp Med. (2022) 22:9–25. doi: 10.1007/s10238-021-00728-6
3. Cafaro G, Bursi R, Chatzis LG, Fulvio G, Ferro F, Bartoloni E, et al. One year in review 2021: sjögren’s syndrome. Clin Exp Rheumatol. (2021) 39 Suppl 133:3–13. doi: 10.55563/clinexprheumatol/eojaol
4. Bruno D, Tolusso B, Lugli G, Di Mario C, Petricca L, Perniola S, et al. B-cell activation biomarkers in salivary glands are related to lymphomagenesis in primary sjögren’s disease: A pilot monocentric exploratory study. Int J Mol Sci. (2024) 25:3259. doi: 10.3390/ijms25063259
5. Fasano S, Mauro D, Macaluso F, Xiao F, Zhao Y, Lu L, et al. Pathogenesis of primary sjögren’s syndrome beyond B lymphocytes. Clin Exp Rheumatol. (2020) 38 Suppl 126:315–23.
6. Tian Y, Yang H, Liu N, Li Y, Chen J. Advances in pathogenesis of sjögren’s syndrome. J Immunol Res. (2021) 2021:5928232. doi: 10.1155/2021/5928232
7. Sène D, Ismael S, Forien M, Charlotte F, Kaci R, Cacoub P, et al. Ectopic germinal center-like structures in minor salivary gland biopsy tissue predict lymphoma occurrence in patients with primary sjögren’s syndrome. Arthritis Rheumatol. (2018) 70:1481–8. doi: 10.1002/art.40528
8. He J, Jin Y, Zhang X, Zhou Y, Li R, Dai Y, et al. Characteristics of germinal center-like structures in patients with sjögren’s syndrome. Int J Rheum Dis. (2017) 20:245–51. doi: 10.1111/1756-185X.12856
9. Ghafouri-Fard S, Shahir M, Taheri M, Salimi A. A review on the role of chemokines in the pathogenesis of systemic lupus erythematosus. Cytokine. (2021) 146:155640. doi: 10.1016/j.cyto.2021.155640
10. Murayama MA, Shimizu J, Miyabe C, Yudo K, Miyabe Y. Chemokines and chemokine receptors as promising targets in rheumatoid arthritis. Front In Immunol. (2023) 14:1100869. doi: 10.3389/fimmu.2023.1100869
11. Soret P, Le Dantec C, Desvaux E, Foulquier N, Chassagnol B, Hubert S, et al. A new molecular classification to drive precision treatment strategies in primary sjögren’s syndrome. Nat Commun. (2021) 12:3523. doi: 10.1038/s41467-021-23472-7
12. Blokland SLM, Flessa C-M, van Roon JAG, Mavragani CP. Emerging roles for chemokines and cytokines as orchestrators of immunopathology in sjögren’s syndrome. Rheumatol (Oxford). (2021) 60:3072–87. doi: 10.1093/rheumatology/key438
13. Nocturne G, Mariette X. Sjögren syndrome-associated lymphomas: an update on pathogenesis and management. Br J Haematol. (2015) 168:317–27. doi: 10.1111/bjh.13192
14. Bombardieri M, Pitzalis C. Ectopic lymphoid neogenesis and lymphoid chemokines in sjogren’s syndrome: at the interplay between chronic inflammation, autoimmunity and lymphomagenesis. Curr Pharm Biotechnol. (2012) 13:1989–96. doi: 10.2174/138920112802273209
15. Tzioufas AG, Kapsogeorgou EK, Moutsopoulos HM. Pathogenesis of sjögren’s syndrome: what we know and what we should learn. J Autoimmun. (2012) 39:4–8. doi: 10.1016/j.jaut.2012.01.002
16. Ivetic A, Hoskins Green HL, Hart SJ. L-selectin: A major regulator of leukocyte adhesion, migration and signaling. Front In Immunol. (2019) 10:1068. doi: 10.3389/fimmu.2019.01068
17. Gérard A, Cope AP, Kemper C, Alon R, Köchl R. Lfa-1 in T cell priming, differentiation, and effector functions. Trends Immunol. (2021) 42:706–22. doi: 10.1016/j.it.2021.06.004
18. Bui TM, Wiesolek HL, Sumagin R. Icam-1: A master regulator of cellular responses in inflammation, injury resolution, and tumorigenesis. J Leukoc Biol. (2020) 108:787–99. doi: 10.1002/JLB.2MR0220-549R
19. Singh M, Thakur M, Mishra M, Yadav M, Vibhuti R, Menon AM, et al. Gene regulation of intracellular adhesion molecule-1 (Icam-1): A molecule with multiple functions. Immunol Lett. (2021) 240:123–36. doi: 10.1016/j.imlet.2021.10.007
20. Khan MA, Khurana N, Ahmed RS, Umar S, Md G Sarwar AH, Alam Q, et al. Chemokines: A potential therapeutic target to suppress autoimmune arthritis. Curr Pharm Des. (2019) 25:2937–46. doi: 10.2174/1381612825666190709205028
21. Ridiandries A, Tan JTM, Bursill CA. The role of cc-chemokines in the regulation of angiogenesis. Int J Mol Sci. (2016) 17:1856. doi: 10.3390/ijms17111856
22. Laufer JM, Legler DF. Beyond migration-chemokines in lymphocyte priming, differentiation, and modulating effector functions. J Leukoc Biol. (2018) 104:301–12. doi: 10.1002/JLB.2MR1217-494R
23. CecChinato V, Uguccioni M. Insight on the regulation of chemokine activities. J Leukoc Biol. (2018) 104:295–300. doi: 10.1002/JLB.3MR0118-014R
24. Baldini C, Cecchettini A, Gallo A, Bombardieri S. Updates on sjögren’s syndrome: from proteomics to protein biomarkers. Expert Rev Proteomics. (2017) 14:491–8. doi: 10.1080/14789450.2017.1333904
25. Mahajan SD, Schwartz SA, Nair MPN. Immunological assays for chemokine detection in in-vitro culture of cns cells. Biol Proced Online. (2003) 5:90–102. doi: 10.1251/bpo50
26. Chen X, Chen R, Jin R, Huang Z. The role of cxcl chemokine family in the development and progression of gastric cancer. Int J Clin Exp Pathol. (2020) 13:484–92.
27. McDonough AA, Veiras LC, Minas JN, Ralph DL. Considerations when quantitating protein abundance by immunoblot. Am J Physiol Cell Physiol. (2015) 308:C426–C33. doi: 10.1152/ajpcell.00400.2014
28. von Thun Und Hohenstein-Blaul N, Funke S, Grus FH. Tears as a source of biomarkers for ocular and systemic diseases. Exp Eye Res. (2013) 117:126–37. doi: 10.1016/j.exer.2013.07.015
29. Giusti L, Baldini C, Bazzichi L, Bombardieri S, Lucacchini A. Proteomic diagnosis of sjögren’s syndrome. Expert Rev Proteomics. (2007) 4:757–67. doi: 10.1586/14789450.4.6.757
30. Vissink A, Spijkervet FK, Van Nieuw Amerongen A. Aging and saliva: A review of the literature. Spec Care Dentist. (1996) 16:95–103. doi: 10.1111/j.1754-4505.1996.tb00842.x
31. Sánchez-Tirado E, Agüí L, González-Cortés A, Yáñez-Sedeño P, Pingarrón JM. Biodetection techniques for quantification of chemokines. Chemosensors. (2022) 10:294. doi: 10.3390/chemosensors10080294
32. Rischmueller M, Tieu J, Lester S. Primary sjögren’s syndrome. Best Pract Res Clin Rheumatol. (2016) 30:189–220. doi: 10.1016/j.berh.2016.04.003
33. Kapsogeorgou EK, Christodoulou MI, Panagiotakos DB, Paikos S, Tassidou A, Tzioufas AG, et al. Minor salivary gland inflammatory lesions in sjögren syndrome: do they evolve? J Rheumatol. (2013) 40:1566–71. doi: 10.3899/jrheum.130256
34. Hong X, Meng S, Tang D, Wang T, Ding L, Yu H, et al. Single-cell rna sequencing reveals the expansion of cytotoxic cd4+ T lymphocytes and a landscape of immune cells in primary sjögren’s syndrome. Front In Immunol. (2020) 11:594658. doi: 10.3389/fimmu.2020.594658
35. Verstappen GM, Kroese FGM, Bootsma H. T cells in primary sjögren’s syndrome: targets for early intervention. Rheumatol (Oxford). (2021) 60:3088–98. doi: 10.1093/rheumatology/kez004
36. Shi L, Wang J, Guo H-X, Han X-L, Tang Y-P, Liu G-Y. Circulating th2 cell reduction and th1/th2 imbalance are correlated with primary sjogren’s syndrome-associated interstitial lung disease. Arthritis Res Ther. (2022) 24:121. doi: 10.1186/s13075-022-02811-z
37. Verstappen GM, Corneth OBJ, Bootsma H, Kroese FGM. Th17 cells in primary sjögren’s syndrome: pathogenicity and plasticity. J Autoimmun. (2018) 87:16–25. doi: 10.1016/j.jaut.2017.11.003
38. Yao Y, Ma J-F, Chang C, Xu T, Gao C-Y, Gershwin ME, et al. Immunobiology of T cells in sjögren’s syndrome. Clin Rev Allergy Immunol. (2021) 60:111–31. doi: 10.1007/s12016-020-08793-7
39. Ibrahem HM. B cell dysregulation in primary sjögren’s syndrome: A review. Jpn Dent Sci Rev. (2019) 55:139–44. doi: 10.1016/j.jdsr.2019.09.006
40. Kabeerdoss J, Sandhya P, Mandal SK, Gowri M, Danda D. High salivary soluble L-selectin and interleukin-7 levels in asian Indian patients with primary sjögren’s syndrome. Clin Rheumatol. (2016) 35:3063–7. doi: 10.1007/s10067-016-3406-7
41. García-Carrasco M, Pizcueta P, Cervera R, Ramos-Casals M, Sisó A, de la Red G, et al. Circulating concentrations of soluble L-selectin (Cd62l) in patients with primary sjögren’s syndrome. Ann Rheum Dis. (2000) 59:297–9. doi: 10.1136/ard.59.4.297
42. Kuhbandner K, Hammer A, Haase S, Terbrack E, Hoffmann A, Schippers A, et al. Madcam-1-mediated intestinal lymphocyte homing is critical for the development of active experimental autoimmune encephalomyelitis. Front In Immunol. (2019) 10:903. doi: 10.3389/fimmu.2019.00903
43. Hwang S-H, Woo JS, Moon J, Yang S, Park J-S, Lee J, et al. Il-17 and ccr9+Α4β7- th17 cells promote salivary gland inflammation, dysfunction, and cell death in sjögren’s syndrome. Front In Immunol. (2021) 12:721453. doi: 10.3389/fimmu.2021.721453
44. Mikulowska-Mennis A, Xu B, Berberian JM, Michie SA. Lymphocyte migration to inflamed lacrimal glands is mediated by vascular cell adhesion molecule-1/alpha(4)Beta(1) integrin, peripheral node addressin/L-selectin, and lymphocyte function-associated antigen-1 adhesion pathways. Am J Pathol. (2001) 159:671–81. doi: 10.1016/S0002-9440(10)61738-5
45. Edwards JC, Wilkinson LS, Speight P, Isenberg DA. Vascular cell adhesion molecule 1 and alpha 4 and beta 1 integrins in lymphocyte aggregates in sjögren’s syndrome and rheumatoid arthritis. Ann Rheum Dis. (1993) 52:806–11. doi: 10.1136/ard.52.11.806
46. Hsueh P-Y, Ju Y, Vega A, Edman MC, MacKay JA, Hamm-Alvarez SF. A multivalent icam-1 binding nanoparticle which inhibits icam-1 and lfa-1 interaction represents a new tool for the investigation of autoimmune-mediated dry eye. Int J Mol Sci. (2020) 21:2758. doi: 10.3390/ijms21082758
47. St Clair EW, Angellilo JC, Singer KH. Expression of cell-adhesion molecules in the salivary gland microenvironment of sjögren’s syndrome. Arthritis Rheum. (1992) 35:62–6. doi: 10.1002/art.1780350110
48. Saito I, Terauchi K, Shimuta M, Nishiimura S, Yoshino K, Takeuchi T, et al. Expression of cell adhesion molecules in the salivary and lacrimal glands of sjogren’s syndrome. J Clin Lab Anal. (1993) 7:180–7. doi: 10.1002/jcla.1860070309
49. Wang Y, Shnyra A, Africa C, Warholic C, McArthur C. Activation of the extrinsic apoptotic pathway by tnf-alpha in human salivary gland (Hsg) cells in vitro, suggests a role for the tnf receptor (Tnf-R) and intercellular adhesion molecule-1 (Icam-1) in sjögren’s syndrome-associated autoimmune sialadenitis. Arch Oral Biol. (2009) 54:986–96. doi: 10.1016/j.archoralbio.2009.07.011
50. Zhu T, Pan Z, Zhang N. Elevated cxcl13 in primary sjögren’s syndrome and its correlation with disease activity: A systematic review and meta-analysis. Clin Rheumatol. (2022) 41:2791–802. doi: 10.1007/s10067-022-06210-2
51. Aqrawi LA, Ivanchenko M, Björk A, Ramírez Sepúlveda JI, Imgenberg-Kreuz J, Kvarnström M, et al. Diminished cxcr5 expression in peripheral blood of patients with sjögren’s syndrome may relate to both genotype and salivary gland homing. Clin Exp Immunol. (2018) 192:259–70. doi: 10.1111/cei.13118
52. ColaFrancesco S, Priori R, Smith CG, Minniti A, Iannizzotto V, Pipi E, et al. Cxcl13 as biomarker for histological involvement in sjögren’s syndrome. Rheumatol (Oxford). (2020) 59:165–70. doi: 10.1093/rheumatology/kez255
53. Nocturne G, Seror R, Fogel O, Belkhir R, Boudaoud S, Saraux A, et al. Cxcl13 and ccl11 serum levels and lymphoma and disease activity in primary sjögren’s syndrome. Arthritis Rheumatol. (2015) 67:3226–33. doi: 10.1002/art.39315
54. Traianos EY, Locke J, Lendrem D, Bowman S, Hargreaves B, Macrae V, et al. Serum cxcl13 levels are associated with lymphoma risk and lymphoma occurrence in primary sjögren’s syndrome. Rheumatol Int. (2020) 40:541–8. doi: 10.1007/s00296-020-04524-5
55. Loureiro-Amigo J, Franco-Jarava C, Perurena-Prieto J, Palacio C, Martínez-Valle F, Soláns-Laqué R. Serum cxcl13, baff, il-21 and il-22 levels are related to disease activity and lymphocyte profile in primary sjögren’s syndrome. Clin Exp Rheumatol. (2021) 39 Suppl 133:131–9. doi: 10.55563/clinexprheumatol/fp741f
56. Barone F, Bombardieri M, Rosado MM, Morgan PR, Challacombe SJ, De Vita S, et al. Cxcl13, ccl21, and cxcl12 expression in salivary glands of patients with sjogren’s syndrome and malt lymphoma: association with reactive and Malignant areas of lymphoid organization. J Immunol. (2008) 180:5130–40. doi: 10.4049/jimmunol.180.7.5130
57. Corsiero E, Nerviani A, Bombardieri M, Pitzalis C. Ectopic lymphoid structures: powerhouse of autoimmunity. Front In Immunol. (2016) 7:430. doi: 10.3389/fimmu.2016.00430
58. Bombardieri M, Lewis M, Pitzalis C. Ectopic lymphoid neogenesis in rheumatic autoimmune diseases. Nat Rev Rheumatol. (2017) 13:141–54. doi: 10.1038/nrrheum.2016.217
59. Sánchez-Martín L, Sánchez-Mateos P, Cabañas C. Cxcr7 impact on cxcl12 biology and disease. Trends Mol Med. (2013) 19:12–22. doi: 10.1016/j.molmed.2012.10.004
60. Kurosawa M, Arakaki R, Yamada A, Tsunematsu T, Kudo Y, Sprent J, et al. Nf-Κb2 controls the migratory activity of memory T cells by regulating expression of cxcr4 in a mouse model of sjögren’s syndrome. Arthritis Rheumatol. (2017) 69:2193–202. doi: 10.1002/art.40230
61. Liu Z, Li F, Pan A, Xue H, Jiang S, Zhu C, et al. Elevated ccl19/ccr7 expression during the disease process of primary sjgren’s syndrome. other. (2019) 10:795. doi: 10.3389/fimmu.2019.00795
62. Lee K-E, Kang J-H, Yim Y-R, Kim J-E, Lee J-W, Wen L, et al. Predictive significance of ccl21 and cxcl13 levels in the minor salivary glands of patients with sjögren’s syndrome. Clin Exp Rheumatol. (2017) 35:234–40.
63. Barone F, Bombardieri M, Manzo A, Blades MC, Morgan PR, Challacombe SJ, et al. Association of cxcl13 and ccl21 expression with the progressive organization of lymphoid-like structures in sjögren’s syndrome. Arthritis Rheum. (2005) 52:1773–84. doi: 10.1002/art.21062
64. Kunkel EJ, Campbell JJ, Haraldsen G, Pan J, Boisvert J, Roberts AI, et al. Lymphocyte cc chemokine receptor 9 and epithelial thymus-expressed chemokine (Teck) expression distinguish the small intestinal immune compartment: epithelial expression of tissue-specific chemokines as an organizing principle in regional immunity. J Exp Med. (2000) 192:761–8. doi: 10.1084/jem.192.5.761
65. Blokland SLM, Hillen MR, Kruize AA, Meller S, Homey B, Smithson GM, et al. Increased ccl25 and T helper cells expressing ccr9 in the salivary glands of patients with primary sjögren'’s syndrome: potential new axis in lymphoid neogenesis. Arthritis Rheumatol. (2017) 69:2038–51. doi: 10.1002/art.40182
66. Blokland SLM, Kislat A, Homey B, Smithson GM, Kruize AA, Radstake TRDJ, et al. Decreased circulating cxcr3 + ccr9+T helper cells are associated with elevated levels of their ligands cxcl10 and ccl25 in the salivary gland of patients with sjögren’s syndrome to facilitate their concerted migration. Scand J Immunol. (2020) 91:e12852. doi: 10.1111/sji.12852
67. Pokharel J, Shryki I, Zwijnenburg AJ, Sandu I, Krumm L, Bekiari C, et al. The Cellular Microenvironment Regulates Cx3cr1 Expression on Cd8+ T cells and the Maintenance of Cx3cr1+ Cd8+ T cells. Eur J Immunol. (2023) 54:e2350658. doi: 10.1002/eji.202350658
68. Fu R, Guo H, Janga S, Choi M, Klinngam W, Edman MC, et al. Cathepsin S activation contributes to elevated cx3cl1 (Fractalkine) levels in tears of a sjögren’s syndrome murine model. Sci Rep. (2020) 10:1455. doi: 10.1038/s41598-020-58337-4
69. Imgenberg-Kreuz J, Sandling JK, Björk A, Nordlund J, Kvarnström M, Eloranta ML, et al. Transcription profiling of peripheral B cells in antibody-positive primary sjögren’s syndrome reveals upregulated expression of cx3cr1 and a type I and type ii interferon signature. Scand J Immunol. (2018) 87:e12662. doi: 10.1111/sji.12662
70. Astorri E, Scrivo R, Bombardieri M, Picarelli G, Pecorella I, Porzia A, et al. Cx3cl1 and cx3cr1 expression in tertiary lymphoid structures in salivary gland infiltrates: fractalkine contribution to lymphoid neogenesis in sjogren's syndrome. Rheumatol (Oxford). (2014) 53:611–20. doi: 10.1093/rheumatology/ket401
71. Lee JH, Kwok S-K, Jung SM, Lee J, Lee J-S, Baek SY, et al. Role of fractalkine in the pathogenesis of primary sjögren syndrome: increased serum levels of fractalkine, its expression in labial salivary glands, and the association with clinical manifestations. J Rheumatol. (2014) 41:2425–38. doi: 10.3899/jrheum.130892
72. Yu X, Zhu F, Yu X, Wang J, Wu B, Li C. Serum ccl28 as a biomarker for diagnosis and evaluation of sjögren’s syndrome. Scand J Rheumatol. (2023) 52:200–7. doi: 10.1080/03009742.2021.2001930
73. Lisi S, Sisto M, Lofrumento DD, D'Amore M, De Lucro R, Ribatti D. A potential role of the gro-Α/cxcr2 system in sjögren’s syndrome: regulatory effects of pro-inflammatory cytokines. Histochem Cell Biol. (2013) 139:371–9. doi: 10.1007/s00418-012-1035-z
74. Hernández-Molina G, Ruiz-Quintero N, Lima G, Hernández-Ramírez D, Llorente-Chávez A, Saavedra-González V, et al. Chemokine tear levels in primary sjögren’s syndrome and their relationship with symptoms. Int Ophthalmol. (2022) 42:2355–61. doi: 10.1007/s10792-022-02233-5
75. Lee YJ, Scofield RH, Hyon JY, Yun P-Y, Lee H-J, Lee EY, et al. Salivary chemokine levels in patients with primary sjogren's syndrome. Rheumatol (Oxford). (2010) 49:1747–52. doi: 10.1093/rheumatology/keq121
76. Hernández-Ruiz M, Zlotnik A, Llorente L, Hernandez-Molina G. Markedly high salivary and lacrimal cxcl17 levels in primary sjögren’s syndrome. Joint Bone Spine. (2018) 85:379–80. doi: 10.1016/j.jbspin.2017.05.014
77. Akpek EK, Jabs DA, Gérard HC, Prendergast RA, Hudson AP, Lee B, et al. Chemokines in autoimmune lacrimal gland disease in mrl/mpj mice. Invest Ophthalmol Visual Sci. (2004) 45:185–90. doi: 10.1167/iovs.03-0812
78. Yoon K-C, Park C-S, You I-C, Choi H-J, Lee K-H, Im S-K, et al. Expression of cxcl9, -10, -11, and cxcr3 in the tear film and ocular surface of patients with dry eye syndrome. Invest Ophthalmol Visual Sci. (2010) 51:643–50. doi: 10.1167/iovs.09-3425
79. Moriyama M, Hayashida JN, Toyoshima T, Ohyama Y, Shinozaki S, Tanaka A, et al. Cytokine/chemokine profiles contribute to understanding the pathogenesis and diagnosis of primary sjögren’s syndrome. Clin Exp Immunol. (2012) 169:17–26. doi: 10.1111/j.1365-2249.2012.04587.x
80. Ushio A, Arakaki R, Otsuka K, Yamada A, Tsunematsu T, Kudo Y, et al. Ccl22-producing resident macrophages enhance T cell response in sjögren’s syndrome. Front In Immunol. (2018) 9:2594. doi: 10.3389/fimmu.2018.02594
81. Chaly Y, Barr JY, Sullivan DA, Thomas HE, Brodnicki TC, Lieberman SM. Type I interferon signaling is required for dacryoadenitis in the nonobese diabetic mouse model of sjögren syndrome. Int J Mol Sci. (2018) 19:3259. doi: 10.3390/ijms19103259
82. Hernández-Molina G, Michel-Peregrina M, Hernández-Ramírez DF, Sánchez-Guerrero J, Llorente L. Chemokine saliva levels in patients with primary sjögren’s syndrome, associated sjögren’s syndrome, pre-clinical sjögren’s syndrome and systemic autoimmune diseases. Rheumatol (Oxford). (2011) 50:1288–92. doi: 10.1093/rheumatology/ker019
83. Wildenberg ME, van Helden-Meeuwsen CG, van de Merwe JP, Moreno C, Drexhage HA, Versnel MA. Lack of ccr5 on dendritic cells promotes a proinflammatory environment in submandibular glands of the nod mouse. J Leukoc Biol. (2008) 83:1194–200. doi: 10.1189/jlb.1107794
84. Bombardieri M, Barone F, Lucchesi D, Nayar S, van den Berg WB, Proctor G, et al. Inducible tertiary lymphoid structures, autoimmunity, and exocrine dysfunction in a novel model of salivary gland inflammation in C57bl/6 mice. J Immunol. (2012) 189:3767–76. doi: 10.4049/jimmunol.1201216
85. Kramer JM, Klimatcheva E, Rothstein TL. Cxcl13 is elevated in sjögren’s syndrome in mice and humans and is implicated in disease pathogenesis. J Leukoc Biol. (2013) 94:1079–89. doi: 10.1189/jlb.0113036
86. Tsubota K, Nishiyama T, Mishima K, Inoue H, Doi T, Hattori Y, et al. The role of fractalkine as an accelerating factor on the autoimmune exocrinopathy in mice. Invest Ophthalmol Visual Sci. (2009) 50:4753–60. doi: 10.1167/iovs.08-2596
87. Rangel-Moreno J, Hartson L, Navarro C, Gaxiola M, Selman M, Randall TD. Inducible bronchus-associated lymphoid tissue (Ibalt) in patients with pulmonary complications of rheumatoid arthritis. J Clin Invest. (2006) 116:3183–94. doi: 10.1172/JCI28756
88. Papinska J, Bagavant H, Gmyrek GB, Deshmukh US. Pulmonary involvement in a mouse model of sjögren’s syndrome induced by sting activation. Int J Mol Sci. (2020) 21:4512. doi: 10.3390/ijms21124512
89. Bunting MD, Comerford I, Seach N, Hammett MV, Asquith DL, Körner H, et al. Ccx-ckr deficiency alters thymic stroma impairing thymocyte development and promoting autoimmunity. Blood. (2013) 121:118–28. doi: 10.1182/blood-2012-06-434886
90. Katayama I, Asai T, Nishiyama S, Nishioka K. Icam-1 and lfa-1 expressions in the lesional skin of annular erythema associated with sjögren syndrome. J Dermatol. (1990) 17:719–23. doi: 10.1111/j.1346-8138.1990.tb03019.x
91. Ichikawa Y, Shimizu H, Yoshida M, Takaya M, Arimori S. Accessory molecules expressed on the peripheral blood or synovial fluid T lymphocytes from patients with sjögren’s syndrome or rheumatoid arthritis. Clin Exp Rheumatol. (1992) 10:447–54.
92. Stepp MA, Pal-Ghosh S, Tadvalkar G, Williams AR, Pflugfelder SC, De Paiva CS. Reduced corneal innervation in the cd25 null model of sjögren syndrome. Int J Mol Sci. (2018) 19:3821. doi: 10.3390/ijms19123821
93. van Nimwegen JF, van der Tuuk K, Liefers SC, Verstappen GM, Visser A, Wijnsma RF, et al. Vaginal dryness in primary sjögren’s syndrome: A histopathological case-control study. Rheumatol (Oxford). (2020) 59:2806–15. doi: 10.1093/rheumatology/keaa017
94. Abdelhamid L, Luo XM. Retinoic acid, leaky gut, and autoimmune diseases. Nutrients. (2018) 10:1016. doi: 10.3390/nu10081016
95. Chatterjee A, Gogolak P, Blottière HM, Rajnavölgyi É. The impact of atra on shaping human myeloid cell responses to epithelial cell-derived stimuli and on T-lymphocyte polarization. Mediators Inflammation. (2015) 2015:579830. doi: 10.1155/2015/579830
96. Takahashi M, Mimura Y, Hayashi Y. Role of the icam-1/lfa-1 pathway during the development of autoimmune dacryoadenitis in an animal model for sjögren’s syndrome. Pathobiology. (1996) 64:269–74. doi: 10.1159/000164058
97. Kaistha A, Levine J. Inflammatory bowel disease: the classic gastrointestinal autoimmune disease. Curr Probl Pediatr Adolesc Health Care. (2014) 44:328–34. doi: 10.1016/j.cppeds.2014.10.003
98. Algood HMS, Lin PL, Yankura D, Jones A, Chan J, Flynn JL. Tnf influences chemokine expression of macrophages in vitro and that of cd11b+ Cells in vivo during mycobacterium tuberculosis infection1. J Immunol. (2004) 172:6846–57. doi: 10.4049/jimmunol.172.11.6846
99. Kang EH, Lee YJ, Hyon JY, Yun PY, Song YW. Salivary cytokine profiles in primary sjögren’s syndrome differ from those in non-sjögren sicca in terms of tnf-Α Levels and th-1/th-2 ratios. Clin Exp Rheumatol. (2011) 29:970–6.
100. Zhou J, Kawai T, Yu Q. Pathogenic role of endogenous tnf-Α in the development of sjögren’s-like sialadenitis and secretory dysfunction in non-obese diabetic mice. Lab Invest. (2017) 97:458–67. doi: 10.1038/labinvest.2016.141
101. Yamamura Y, Motegi K, Kani K, Takano H, Momota Y, Aota K, et al. Tnf-Α Inhibits aquaporin 5 expression in human salivary gland acinar cells via suppression of histone H4 acetylation. J Cell Mol Med. (2012) 16:1766–75. doi: 10.1111/j.1582-4934.2011.01456.x
102. An Q, Zhao J, Zhu X, Yang B, Wu Z, Su Y, et al. Exploiting the role of T cells in the pathogenesis of sjögren’s syndrome for therapeutic treatment. Front In Immunol. (2022) 13:995895. doi: 10.3389/fimmu.2022.995895
103. Mariette X, Ravaud P, Steinfeld S, Baron G, Goetz J, Hachulla E, et al. Inefficacy of infliximab in primary sjögren’s syndrome: results of the randomized, controlled trial of remicade in primary sjögren’s syndrome (Tripss). Arthritis Rheum. (2004) 50:1270–6. doi: 10.1002/art.20146
104. Sankar V, Brennan MT, Kok MR, Leakan RA, Smith JA, Manny J, et al. Etanercept in sjögren’s syndrome: A twelve-week randomized, double-blind, placebo-controlled pilot clinical trial. Arthritis Rheum. (2004) 50:2240–5. doi: 10.1002/art.20299
105. Brkic Z, Maria NI, van Helden-Meeuwsen CG, van de Merwe JP, van Daele PL, Dalm VA, et al. Prevalence of interferon type I signature in cd14 monocytes of patients with sjogren's syndrome and association with disease activity and baff gene expression. Ann Rheum Dis. (2013) 72:728–35. doi: 10.1136/annrheumdis-2012-201381
106. Imgenberg-Kreuz J, Sandling JK, Almlöf JC, Nordlund J, Signér L, Norheim KB, et al. Genome-wide DNA methylation analysis in multiple tissues in primary sjögren’s syndrome reveals regulatory effects at interferon-induced genes. Ann Rheum Dis. (2016) 75:2029–36. doi: 10.1136/annrheumdis-2015-208659
107. Aota K, Kani K, Yamanoi T, Nakashiro K-I, Ishimaru N, Azuma M. Distinct regulation of cxcl10 production by cytokines in human salivary gland ductal and acinar cells. Inflammation. (2018) 41:1172–81. doi: 10.1007/s10753-018-0764-0
108. Zhao J, Kubo S, Nakayamada S, Shimajiri S, Zhang X, Yamaoka K, et al. Association of plasmacytoid dendritic cells with B cell infiltration in minor salivary glands in patients with sjögren’s syndrome. Mod Rheumatol. (2016) 26:716–24. doi: 10.3109/14397595.2015.1129694
109. Leonard WJ, O'Shea JJ. Jaks and stats: biological implications. Annu Rev Immunol. (1998) 16:293–322. doi: 10.1146/annurev.immunol.16.1.293
110. Pringle S, Wang X, Bootsma H, Spijkervet FKL, Vissink A, Kroese FGM. Small-molecule inhibitors and the salivary gland epithelium in sjögren’s syndrome. Expert Opin Investig Drugs. (2019) 28:605–16. doi: 10.1080/13543784.2019.1631796
111. Urits I, Israel J, Hakobyan H, Yusin G, Lassiter G, Fackler N, et al. Baricitinib for the treatment of rheumatoid arthritis. Reumatologia. (2020) 58:407–15. doi: 10.5114/reum.2020.102006
112. Aota K, Yamanoi T, Kani K, Ono S, Momota Y, Azuma M. Inhibition of jak-stat signaling by baricitinib reduces interferon-Γ-induced cxcl10 production in human salivary gland ductal cells. Inflammation. (2021) 44:206–16. doi: 10.1007/s10753-020-01322-w
113. Bai W, Liu H, Dou L, Yang Y, Leng X, Li M, et al. Pilot study of baricitinib for active sjogren's syndrome. Ann Rheum Dis. (2022) 81:1050–2. doi: 10.1136/annrheumdis-2021-222053
114. Luo J, Jin D-E, Yang G-Y, Zhang Y-Z, Wang J-M, Kong W-P, et al. Total glucosides of paeony for rheumatoid arthritis: A systematic review of randomized controlled trials. Complement Ther Med. (2017) 34:46–56. doi: 10.1016/j.ctim.2017.07.010
115. Wang C, Yuan J, Zhang LL, Wei W. Pharmacokinetic comparisons of paeoniflorin and paeoniflorin-6'o-benzene sulfonate in rats via different routes of administration. Xenobiotica. (2016) 46:1142–50. doi: 10.3109/00498254.2016.1149633
116. Wu H, Chen X, Gu F, Zhang P, Xu S, Liu Q, et al. Cp-25 alleviates antigen-induced experimental sjögren’s syndrome in mice by inhibiting jak1-stat1/2-cxcl13 signaling and interfering with B-cell migration. Lab Invest. (2021) 101:1084–97. doi: 10.1038/s41374-020-0453-0
117. Gu F, Xu S, Zhang P, Chen X, Wu Y, Wang C, et al. Cp-25 alleviates experimental sjögren’s syndrome features in nod/ltj mice and modulates T lymphocyte subsets. Basic Clin Pharmacol Toxicol. (2018) 123:423–34. doi: 10.1111/bcpt.13025
118. Moisini I, Davidson A. Baff: A local and systemic target in autoimmune diseases. Clin Exp Immunol. (2009) 158:155–63. doi: 10.1111/j.1365-2249.2009.04007.x
119. Lavie F, Miceli-Richard C, Quillard J, Roux S, Leclerc P, Mariette X. Expression of baff (Blys) in T cells infiltrating labial salivary glands from patients with sjögren’s syndrome. J Pathol. (2004) 202:496–502. doi: 10.1002/path.1533
120. Szodoray P, Alex P, Jonsson MV, Knowlton N, Dozmorov I, Nakken B, et al. Distinct profiles of sjögren’s syndrome patients with ectopic salivary gland germinal centers revealed by serum cytokines and baff. Clin Immunol. (2005) 117:168–76. doi: 10.1016/j.clim.2005.06.016
121. Sharma A, Kiripolsky J, Klimatcheva E, Howell A, Fereidouni F, Levenson R, et al. Early baff receptor blockade mitigates murine sjögren’s syndrome: concomitant targeting of cxcl13 and the baff receptor prevents salivary hypofunction. Clin Immunol. (2016) 164:85–94. doi: 10.1016/j.clim.2016.01.015
122. Gatumu MK, Skarstein K, Papandile A, Browning JL, Fava RA, Bolstad AI. Blockade of lymphotoxin-beta receptor signaling reduces aspects of sjögren’s syndrome in salivary glands of non-obese diabetic mice. Arthritis Res Ther. (2009) 11:R24. doi: 10.1186/ar2617
123. Pitzalis C, Jones GW, Bombardieri M, Jones SA. Ectopic lymphoid-like structures in infection, cancer and autoimmunity. Nat Rev Immunol. (2014) 14:447–62. doi: 10.1038/nri3700
124. Fava RA, Browning JL, Gatumu M, Skarstein K, Bolstad A-I. Ltbr-pathway in sjogren's syndrome: cxcl13 levels and B-cell-enriched ectopic lymphoid aggregates in nod mouse lacrimal glands are dependent on ltbr. Adv Exp Med Biol. (2011) 691:383–90. doi: 10.1007/978-1-4419-6612-4_39
125. Browning JL. Inhibition of the lymphotoxin pathway as a therapy for autoimmune disease. Immunol Rev. (2008) 223:202–20. doi: 10.1111/j.1600-065X.2008.00633.x
126. Fava RA, Kennedy SM, Wood SG, Bolstad AI, Bienkowska J, Papandile A, et al. Lymphotoxin-beta receptor blockade reduces cxcl13 in lacrimal glands and improves corneal integrity in the nod model of sjögren’s syndrome. Arthritis Res Ther. (2011) 13:R182. doi: 10.1186/ar3507
127. St Clair EW, Baer AN, Wei C, Noaiseh G, Parke A, Coca A, et al. Clinical efficacy and safety of baminercept, a lymphotoxin Β Receptor fusion protein, in primary sjögren’s syndrome: results from a phase ii randomized, double-blind, placebo-controlled trial. Arthritis Rheumatol. (2018) 70:1470–80. doi: 10.1002/art.40513
128. Carubbi F, Cipriani P, Marrelli A, Benedetto P, Ruscitti P, Berardicurti O, et al. Efficacy and safety of rituximab treatment in early primary sjögren’s syndrome: A prospective, multi-center, follow-up study. Arthritis Res Ther. (2013) 15:R172. doi: 10.1186/ar4359
129. Dass S, Bowman SJ, Vital EM, Ikeda K, Pease CT, Hamburger J, et al. Reduction of fatigue in sjögren syndrome with rituximab: results of a randomised, double-blind, placebo-controlled pilot study. Ann Rheum Dis. (2008) 67:1541–4. doi: 10.1136/ard.2007.083865
130. Gerondakis S, Fulford TS, Messina NL, Grumont RJ. Nf-Κb control of T cell development. Nat Immunol. (2014) 15:15–25. doi: 10.1038/ni.2785
131. Oeckinghaus A, Hayden MS, Ghosh S. Crosstalk in nf-Κb signaling pathways. Nat Immunol. (2011) 12:695–708. doi: 10.1038/ni.2065
132. Ishimaru N, Kishimoto H, Hayashi Y, Sprent J. Regulation of naive T cell function by the nf-kappab2 pathway. Nat Immunol. (2006) 7:763–72. doi: 10.1038/ni1351
133. Wang X, Shaalan A, Liefers S, Coudenys J, Elewaut D, Proctor GB, et al. Dysregulation of nf-kb in glandular epithelial cells results in sjögren’s-like features. PloS One. (2018) 13:e0200212. doi: 10.1371/journal.pone.0200212
134. Verstappen GM, Meiners PM, Corneth OBJ, Visser A, Arends S, Abdulahad WH, et al. Attenuation of follicular helper T cell-dependent B cell hyperactivity by abatacept treatment in primary sjögren’s syndrome. Arthritis Rheumatol. (2017) 69:1850–61. doi: 10.1002/art.40165
135. Sisto M, Lisi S. New insights into adams regulation of the gro-Α/cxcr2 system: focus on sjögren’s syndrome. Int Rev Immunol. (2015) 34:486–99. doi: 10.3109/08830185.2014.975892
136. Pilson Q, Smith S, Jefferies CA, Ní Gabhann-Dromgoole J, Murphy CC. Mir-744-5p contributes to ocular inflammation in patients with primary sjogrens syndrome. Sci Rep. (2020) 10:7484. doi: 10.1038/s41598-020-64422-5
137. Tian Q, Zhao H, Ling H, Sun L, Xiao C, Yin G, et al. Poly(Adp-Ribose) Polymerase Enhances Infiltration of Mononuclear Cells in Primary sjögren’s Syndrome through Interferon-Induced Protein with Tetratricopeptide Repeats 1-Mediated up-Regulation of Cxcl10. Arthritis Rheumatol. (2020) 72:1003–12. doi: 10.1002/art.41195
Keywords: Sjögren’s syndrome, lymphocyte homing, chemokines, addressins, targeting therapy
Citation: Liao J, Yu X, Huang Z, He Q, Yang J, Zhang Y, Chen J, Song W, Luo J and Tao Q (2024) Chemokines and lymphocyte homing in Sjögren’s syndrome. Front. Immunol. 15:1345381. doi: 10.3389/fimmu.2024.1345381
Received: 27 November 2023; Accepted: 15 April 2024;
Published: 26 April 2024.
Edited by:
Remo Castro Russo, Federal University of Minas Gerais, BrazilReviewed by:
Jillian M. Richmond, University of Massachusetts Medical School, United StatesLoukas Chatzis, Laiko General Hospital of Athens, Greece
Copyright © 2024 Liao, Yu, Huang, He, Yang, Zhang, Chen, Song, Luo and Tao. This is an open-access article distributed under the terms of the Creative Commons Attribution License (CC BY). The use, distribution or reproduction in other forums is permitted, provided the original author(s) and the copyright owner(s) are credited and that the original publication in this journal is cited, in accordance with accepted academic practice. No use, distribution or reproduction is permitted which does not comply with these terms.
*Correspondence: Jing Luo, luojinggg@sina.com; Qingwen Tao, taoqg1@sina.com