- 1Key Laboratory for Quality Evaluation of Bulk Herbs of Hunan Province, School of Pharmacy, Hunan University of Chinese Medicine, Changsha, China
- 2Institute of Chinese Materia Medica, China Academy of Chinese Medical Sciences, Beijing, China
Inflammation has been shown to trigger a wide range of chronic diseases, particularly inflammatory diseases. As a result, the focus of research has been on anti-inflammatory drugs and foods. In recent years, the field of medicinal and edible homology (MEH) has developed rapidly in both medical and food sciences, with 95% of MEH being associated with plants. Phenolic acids are a crucial group of natural bioactive substances found in medicinal and edible homologous plants (MEHPs). Their anti-inflammatory activity is significant as they play a vital role in treating several inflammatory diseases. These compounds possess enormous potential for developing anti-inflammatory drugs and functional foods. However, their development is far from satisfactory due to their diverse structure and intricate anti-inflammatory mechanisms. In this review, we summarize the various types, structures, and distribution of MEHP phenolic acids that have been identified as of 2023. We also analyze their anti-inflammatory activity and molecular mechanisms in inflammatory diseases through NF-κB, MAPK, NLRP3, Nrf2, TLRs, and IL-17 pathways. Additionally, we investigate their impact on regulating the composition of the gut microbiota and immune responses. This analysis lays the groundwork for further exploration of the anti-inflammatory structure-activity relationship of MEHP phenolic acids, aiming to inspire structural optimization and deepen our understanding of their mechanism, and provides valuable insights for future research and development in this field.
1 Introduction
Inflammatory diseases can trigger abnormal reactions in various body systems, leading to tissue damage and dysfunction, which seriously affects human health, and inflammation is the basis of inflammatory diseases. Inflammation is a cascade of chemical signals triggered by viral and bacterial infections, toxic compound stimulation, and tissue damage, which can activate white blood cells to produce and release inflammatory cytokines. Chronic inflammation can contribute to the development of various chronic diseases such as inflammatory diseases, autoimmune diseases, tumors, neurogenic diseases, diabetes, cardiovascular diseases, and tissue fibrosis (1, 2). Therefore, anti-inflammatory drugs and foods have always been a hot topic of research. Medicinal and edible homology (MEH) refers to natural resources offering edible and medicinal value. Being safe and healthy options with medicinal functions, MEH-based research and product development are receiving increasing attention (3, 4). In 2002, a list of items that function as both food and medicine (the catalog of “Medicinal and edible homologous” sources) was released by the former Chinese Ministry of health. As of now, there are 110 Chinese medicinal materials that have been included (3), and 102 of these are plants, accounting for nearly 95%.
Medicinal and edible homologous plants (MEHPs) are characterized by the presence of a variety of active ingredients. Phenolic acids are one of the most representative ingredients of MEHPs. Phenolic acids are a class of organic acids with directly linked phenolic groups to aromatic rings, are abundant in plants, encompassing a broad spectrum of medicinal and edible varieties, and constitute vital secondary metabolites. Currently, phenolic acids hold broad applications across various sectors, including the food industry, medicine, health supplements, and cosmetics. They are integrated into food products as natural preservatives and antioxidants, enhancing shelf life (5). Within the pharmaceutical domain, phenolic acids serve as therapeutic agents or adjuvants for combating inflammatory conditions and select cancers (6). As dietary supplements, they contribute to health promotion and disease prevention (7). Additionally, phenolic acids are leveraged in skincare for their potent antioxidant and anti-inflammatory benefits, particularly in anti-aging and protective formulations (8). The structural skeleton of phenolic acids is mainly composed of a carboxyl group and one or more hydroxyl groups bound to aromatic rings. Phenolic acids can be divided into three classes: hydroxybenzoic acids, hydroxyphenylacetic acids, and hydroxycinnamic acids, all having anti-inflammatory, anti-oxidant, anti-bacterial, and anti-viral activities (9–12). Bioactivity is closely related to the structure of MEHP phenolic acids; hence, an understanding of the varied structures of these compounds is important.
Anti-inflammatory activity is one of the main features of MEHP phenolic acids and plays an important role in the prevention and treatment of numerous inflammatory diseases (13–16). Although the pathogenesis of these diseases is different, the regulation of inflammatory signaling pathways is similar. Therefore, it is essential to elucidate the anti-inflammatory mechanisms of MEHP phenolic acids for intensive research on their anti-inflammatory diseases’ activity.
We performed a comprehensive database search of PubMed, Web of Science, and Science Direct for entries up to November 2023, to systematically review the types, structures, anti-inflammatory activities, and molecular mechanisms of MEHP phenolic acids. The objective is to provide scientific basis for in-depth research and comprehensive development of the anti-inflammatory activities of MEHP phenolic acids.
2 Structure and distribution of MEHP phenolic acids
Upon conducting a thorough literature review, we discovered that 68 types of MEHP were reported to contain a comprehensive collection of 167 phenolic acids. Among these, there are 45 hydroxybenzoic acids, 113 hydroxycinnamic acids, 8 hydroxyphenylacetic acids, and 1 other phenolic acid. The 68 MEHPs belong to 35 families with 6 species from Rosaceae or Lamiaceae, 5 from Zingiberaceae, 4 from Caprifoliaceae or Compositae, and 3 from Moraceae, Rutaceae, Leguminosae, or Campanulacea.
2.1 Hydroxybenzoic acids
Hydroxybenzoic acids are based on a hydroxybenzoic acid skeleton. The hydroxybenzoic acids can be divided into simple hydroxybenzoic acids, polyhydroxybenzoic acids, hydroxybenzoates, and hydroxybenzoate glycosides. According to reports, there are 18 types of simple hydroxybenzoic acids, 6 types of polyhydroxybenzoic acids, 12 types of hydroxybenzoates, and 9 types of hydroxybenzoate glycosides. Simple hydroxybenzoic acids are the most widely distributed (including vanillic acid, gallic acid, syringic acid, salicylic acid, protocatechuic acid, p-hydroxybenzoic acid, etc.) among which vanillic acid, gallic acid, and syringic acid are distributed in 28, 25, and 24 MEHPs, respectively. Details are shown in Table 1 and the structure is shown in Figure 1.
2.2 Hydroxycinnamic acids
Hydroxycinnamic acids are the most abundant and widely distributed phenolic acids. According to structure, they can be divided into simple hydroxycinnamic acids, hydrogenated hydroxycinnamic acids, polyhydroxycinnamic acids, hydroxycinnamates, hydroxycinnamate glycosides, and hydroxycinnamate salts. Among the reported MEHP phenolic acids, there are 10 simple hydroxycinnamic acids, 7 hydrogenated hydroxycinnamic acids, 46 polyhydroxycinnamic acids, 26 hydroxycinnamates, 21 hydroxycinnamate glycosides, and 1 hydroxycinnamate salt. Among these, simple hydroxycinnamic acids and polyhydroxycinnamic acids are the most diverse. The most widely distributed simple hydroxycinnamic acids include caffeic acid, ferulic acid, and p-coumaric acid, which are distributed in 39, 31, and 28 MEHPs, respectively. Most polyhydroxycinnamic acids have caffeic acid as the parent core, including caffeoylquinic acids which combine caffeic acid and quinic acid (chlorogenic acid) and rosmarinic acid which is a combination of caffeic acid and danshensu. Chlorogenic acid is the phenolic acid with the largest reported distribution in 43 MEHPs. In addition, the reported hydroxycinnamate salt (caffeic acid 3-sulfonate) was only distributed in Piper nigrum L. Detailed information is shown in Table 2 and the structure is shown in Figure 2.
2.3 Hydroxyphenylacetic acids and other acids
In contrast, hydroxyphenylacetic acids are the least abundant phenolic acids. Only 8 hydroxyphenylacetic acids have been reported in MEHPs, including 5 simple hydroxyphenylacetic acids, 2 hydroxyphenylacetates, and 1 hydroxyphenylacetate glycoside. There are only 10 MEHPs reported. In addition, another type of phenolic acid was found in Piper nigrum L.: 5-(3’,4’-dihydroxyphenyl)-valeric acid. Details are shown in Table 3 and the structure is shown in Figure 3.
We found that 5 MEHPs contain more than 20 phenolic acids: Morus alba L. (fruit) (26), Piper nigrum L (23), Prunella vulgaris L (23), Sesamum indicum L (22), Perilla frutescens (L.) Britt. (leaf) (20). Among these, Prunella vulgaris L. and Perilla frutescens (L.) Britt (leaf) belong to Lamiaceae, indicating that phenolic acids may be the main active compounds in Lamiaceae plants. Among the 167 identified MEHP phenolic acids, hydroxycinnamic acids were the most numerous and widely distributed, with chlorogenic acid present in 43 MEHPs, highlighting its accessibility and potential for development.
3 Anti-inflammatory activity and mechanism of MEHP phenolic acids
Recognizing the pivotal role of inflammatory response in inflammatory diseases, anti-inflammatory drugs occupy a central position in their management and treatment. The intricate relationship between the anti-inflammatory mechanism and inflammatory diseases underscores their interconnectedness. Presently, the anti-inflammatory drugs available in the market primarily function through various pathways, including nuclear factor- kappa B (NF-κB), mitogen activated protein kinase (MAPK), NOD-like receptor protein 3(NLRP3), nuclear factor E2-related factor 2 (Nrf2), toll-like receptors (TLRs), and interleukin-17 (IL-17). Additionally, the regulation of gut microbiota and immune response mechanisms contribute significantly to their effectiveness. Notably, MEHPs phenolic acids exhibit remarkable anti-inflammatory activity, as evidenced in numerous studies on inflammatory diseases. Their diverse anti-inflammatory mechanisms of action offer promising potential for further development.
3.1 NF-κB pathway
Nuclear factor- kappa B (NF-κB) is an important nuclear transcription factor in cells, formed by dimerization of Rel proteins (p50, p52, p65, c-Ral, and RalB). NF-κB pathway consists of canonical and non-canonical pathways. (1) Canonical: NF-κB (p65/p50) and inhibitor of NF-κB (IκBα) are bound in the cytoplasm with an inactive dimer. When subjected to reactive-oxygen species (ROS), toll-like receptors (TLRs), interleukin 1β (IL-1β) and tumor necrosis factor α (TNF-α), inhibitor of κB kinase (IKKβ) is activated, then IκB is degraded, and p65/p50 dimer is dissociated. Subsequently, p65 is phosphorylated and translocated to the nucleus to activate the target genes, inducing the transcription of TNF-α, IL-1β, and interleukin 6 (IL-6) (143). (2) Noncanonical: RalB binds to p100 as inactive dimer in the cytoplasm. Lymphotoxin β(LTβ), B cell activating factor (BAFF), and tumor necrosis factor receptor superfamily member 5(CD40) stimulate the accumulation of NF-κB-inducing kinase (NIK) and activate IKKα. Then, p100 is degraded to p52, and the RalB/p52 dimer is translocated to the nucleus, to induce the transcription of related genes (144). MEHP phenolic acids can inhibit the NF-κB pathway by inhibiting canonical and non-canonical pathways (Figure 4).
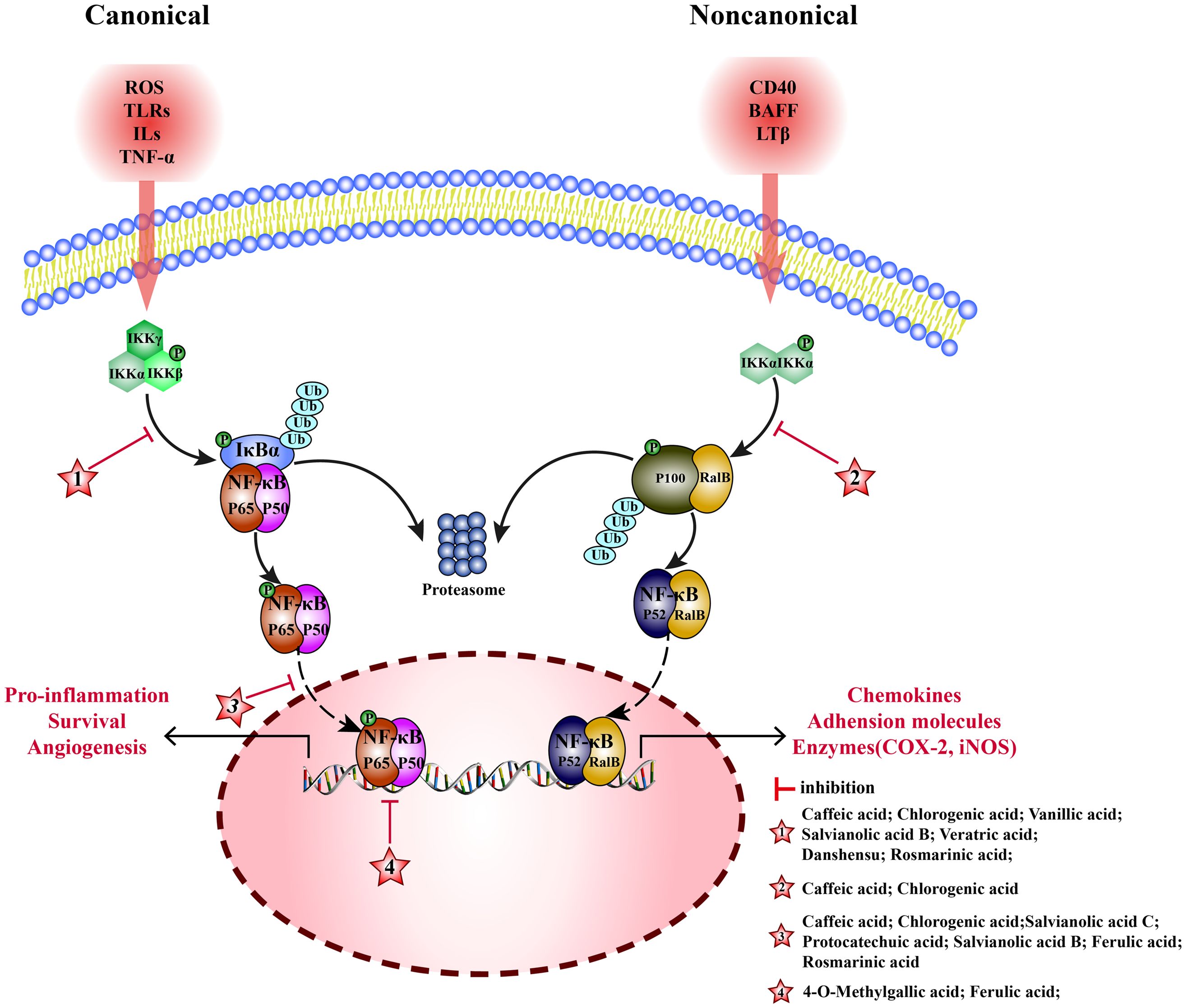
Figure 4 Molecular mechanism of the anti-inflammatory activity of phenolic acids from medicinal and edible homologous plants against the NF-κB pathway.
3.1.1 Inhibiting the canonical pathway
In canonical pathway, MEHP phenolic acid can inhibit the NF-kB pathway by suppressing the activation and nuclear translocation of NF-kB and blocking the binding of NF-kB to target genes.
Various hydroxycinnamic acids with caffeic acid as the parent nucleus, such as caffeic acid, chlorogenic acid, salvianolic acid B, and rosmarinic acid, have inhibitory effects on multiple links of canonical NF-kB pathway. Caffeic acid inhibits phosphorylation of IKKα/β and IκBα to inhibit activation of NF-κB, playing a key role in anti-rheumatoid arthritis (145), it can also inhibit nuclear translocation of p-p65, and alleviating inflammation to protect ischemia/reperfution(I/R)-injury in rats (25). Chlorogenic acid inhibits phosphorylation of IκBα and the p65 protein levels to interfere with NF-κB pathway, showing anti-arthritis (146) and anti-mastitis (147) effects. Meanwhile, it inhibits nuclear translocation of p-p65 to block NF-κB signaling pathway alleviating LPS-induced inflammation in Caco-2 (148), RAW 264.7 (149), and rat hepatic stellate cells (150). salvianolic acid B inhibits activation of NF-κB pathway by inhibiting the phosphorylation of p65 (151), alleviating inflammation of arthritis mouse, it can also reduce the release of TNF-α, IL-1β, and IL-6 by inhibiting nuclear translocation of p65 producing anti-atherosclerotic effect (152). In an inflammatory model of human skin fibroblasts (HSF) induced by TNF-α, rosmarinic acid has been shown to inhibit the phosphorylation and degradation of IκBα and the activation of NF-κB (153). Additionally, rosmarinic acid can alleviate acute pancreatitis induced by sodium taurocholate by inhibiting the nuclear translocation of p65 (154). In LPS-induced acute kidney injury in mice, ferulic acid can inhibit inflammation by inhibiting nuclear translocation of p65 (155). Moreover, ferulic acid cuts off the combination between p-NF-κB and the transcription factor cAMP-response element binding protein(CREB), inhibiting NF-κB binding to DNA, helping repair acute liver injury induced by cecal ligation perforation (CLP) in mice (156).
Furthermore, two additional hydroxycinnamic acids have also been reported to have inhibitory effects on the canonical NF-kB pathway. Danshensu reduces expression of p-IKKα/β, p-IκBα, and p-p65, and upregulates expression of IκBα to alleviate chronic kidney disease in mice (157). Salvianolic acid C blocks NF-κB signaling pathway by inhibiting nuclear translocation of p-p65 and suppresses inflammation in BV2 cells induced by LPS (158).
Four hydroxybenzoic acids also possess inhibitory effects on the canonical NF-kB pathway. Vanillic acid inhibits phosphorylation IκBα, alleviating inflammation of chondrocytes in patients with arthritis (159). In acute lung injury mice, veratric acid inhibits the phosphorylation of IκB and p65, regulating the NF-κB signaling pathway to alleviate inflammatory damage induced by LPS (160). Protocatechuic acid inhibits nuclear translocation of p65, protein and mRNA expression of TNF- α, IL-1 β, and IL-6 in SH-SY5Y cells, and promotes repair after cerebral hemorrhage in mice (161). 4-O-methylgallic acid can modify the DNA binding domain of NF-κB to directly block NF-κB binding with DNA in the nucleus, thereby inhibiting leukocyte adhesion to endothelial cells and preventing vascular inflammation (162).
3.1.2 Inhibiting the noncanonical pathway
In noncanonical pathways, an accumulation of NIK promotes phosphorylation of IKKα, activating NF-κB (RalB/p52); therefore, NIK is a key kinase. Two hydroxycinnamic acids belonging to the caffeic acid category have been found to exert inhibitory effects on the noncanonical NF-kB pathway. Caffeic acid inhibits phosphorylation of NIK and IKK and the activation of noncanonical NF-κB pathway, alleviating inflammation in endothelial cells (163). Chlorogenic acid inhibits the expression of RalB and p52 to exert anti- liver cancer effects (164).
In comparison to non-canonical pathways, MEHP phenolic acids exert a more pronounced inhibitory effect on canonical pathways. Seven hydroxycinnamic acids and four hydroxybenzoic acids possess inhibitory effects on the non-pharmacological pathway, demonstrated across various models and conditions. Notably, four caffeic acid-like hydroxycinnamic acids are capable of simultaneously targeting diverse stages of the canonical pathway to alleviate inflammatory conditions, including NF-kB activation, nuclear translocation, and binding to target genes. Furthermore, two types of hydroxycinnamic acid, both belonging to the caffeic acid category, can inhibit the non-pharmacological pathway.
3.2 MAPK pathway
Mitogen activated protein kinase (MAPK) is a serine threonine protein kinase. The MAPK pathway is composed of a tertiary kinase pattern, including mitogen-activated protein kinase kinase kinase (MKKKs), mitogen-activated protein kinase kinases (MKKs), and MAPKs. MAPKs comprises four subfamilies: extracellular regulated protein kinases (ERK), mitogen-activated protein kinase p38 (p38), c-Jun N-terminal kinase (JNK), and extracellular regulated protein kinases 5 (ERK5), and these pathways are named accordingly. Among them, ERK, p38, and JNK are the three canonical MAPK pathways, which are closely associated with inflammation. MEHP phenolic acids can inhibit the MAPK pathway and exert anti-inflammatory activity by inhibiting the activation of kinases (Figure 5).
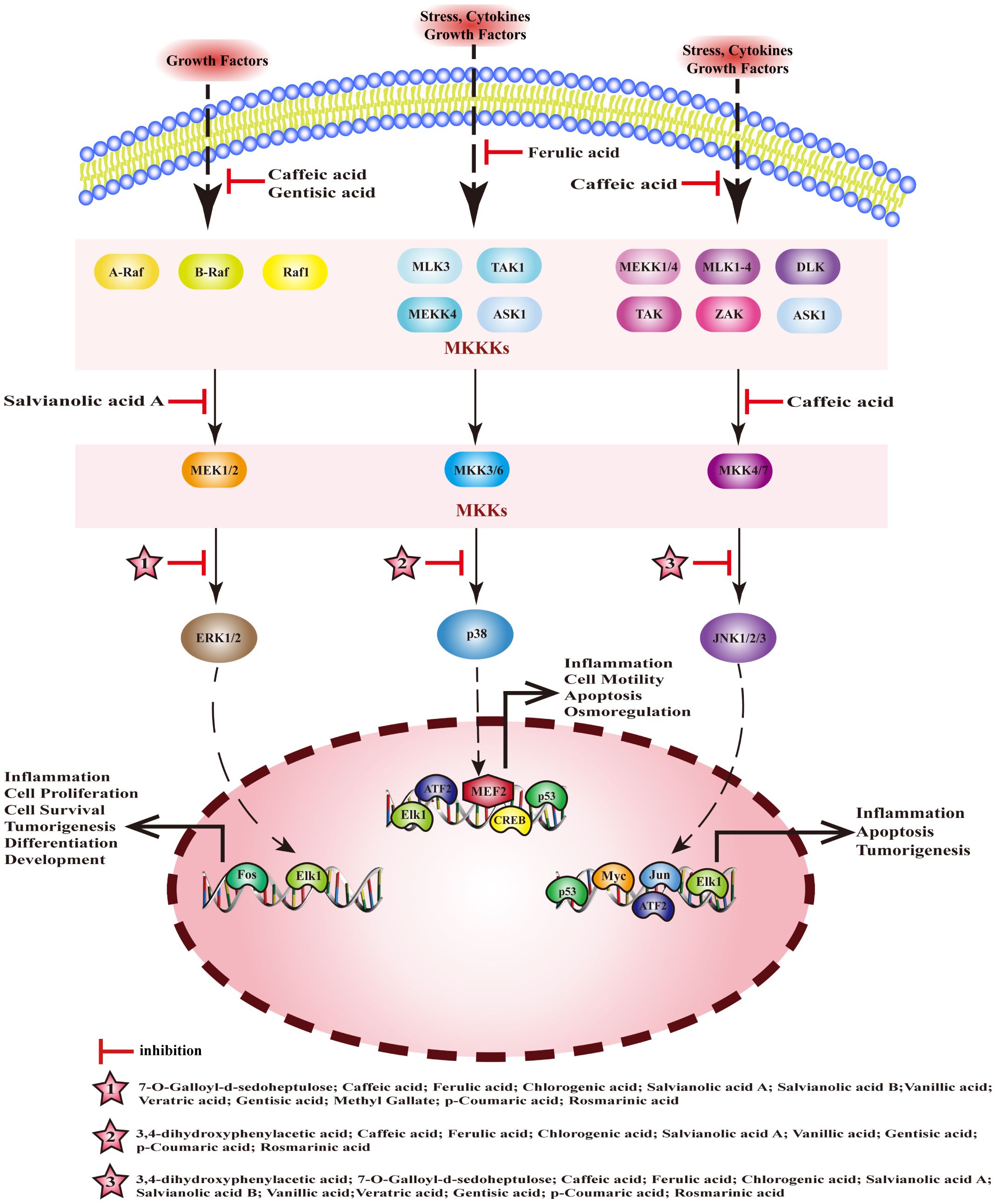
Figure 5 Molecular mechanism of the anti-inflammatory activity of phenolic acids from medicinal and edible homologous plants against the MAPK pathway.
3.2.1 Inhibiting MKKKs
The MKKKs family of Serine/threonine-protein kinase (Raf), encompassing A-Raf, B-Raf, and Raf1, plays a pivotal role in the activation of the ERK pathway. Concurrently, MKKKs such as serine/threonine-protein kinase RIM15 (TAK1), mitogen-activated protein kinase kinase kinase (MEKK), and mitogen-activated protein kinase kinase kinase 5 (ASK1) are instrumental in triggering the p38 and JNK pathways. Gentisic acid inhibits the expression of Raf in ankle and knee tissues and regulates Raf/ERK signaling, thus alleviating rheumatoid arthritis in rats (165). Caffeic acid exerts anti-gastritis effects by inhibiting interleukin-1 receptor-associated kinase 1 (IRAK1), interleukin-1 receptor-associated kinase 4 (IRAK4), and TAK1 by interfering with the JNK/MAPK pathway (166). Caffeic acid can inhibit the phosphorylation of c-Raf and the activation of ERK1/2, reduce the release of inflammatory factors, and exert a detoxifying effect on liver toxicity induced by acetaminophen(APAP) in mice (167). Ferulic acid alleviates LPS-induced inflammation of RAW 264.7 cells by inhibiting the phosphorylation of TAK1, interfering with the p38/MAPK pathway to inhibit the activation of NF-κB (168).
3.2.2 Inhibiting MKKs
The three canonical MAPK pathways correspond to distinct MKKs, the ERK pathway is associated with MEK, the p38 pathway aligns with MKK3/6, and the JNK pathway is linked to MKK4/7. Two hydroxycinnamic acids, with caffeic acid serving as their central component, exert a significant inhibitory influence on MKKs. Caffeic acid can exert anti-gastritis effects by inhibiting MKK4/7 to inhibit the JNK/MAPK pathway (166). Salvianolic acid A can effectively mitigate the inflammatory response in the lungs of patients suffering from acute lung injury by suppressing LPS-induced phosphorylation of MEK, and ERK within the lung tissue (169).
3.2.3 Inhibiting MAPKs
In mice with colitis, chlorogenic acid reduces the expression ERK1/2, p-ERK, p38, p-p38, JNK, p-JNK, p-IκB, and p-p65 in tissues, blocks the ERK/JNK pathway, and reduces symptoms of colitis (170). In rats with arthritis, p-coumaric acid promotes the inactivation of MAPK pathway, inhibits inflammation, cartilage degeneration, and osteoclast formation by downregulating the expression of JNK, p-JNK, and ERK1/2[187]; it can also inhibit the expression of p-p38/pJNK/pERK and p-IKKβ/p-IκB/NF-κB, block caspase-1/MAPK/NF-κB signaling cascade to inhibit the inflammation of activated mast cell and splenocyte[188].Ferulic acid can inhibit NF-κB pathway by reducing the phosphorylation of p38 and JNK, thereby preventing endometritis (171). Salvianolic acid A inhibits the activation of p38, JNK, and ERK, blocking the activation of MAPK pathways, and exerts anti-inflammatory effects in mice with arthritis (172). 3,4-dihydroxyphenylacetic acid inhibits inflammation and repairs the intestinal barrier dysfunction in mice with type 2 diabetes by inhibiting the activation of JNK and p38 (173). 7-O-galloyl-d-sedoheptulose can inhibit the activation of NF-κB and AP-1 and plays a key role in liver protection in type 2 diabetes by inhibiting phosphorylation of ERK1/2 and JNK (174). Caffeic acid significantly inhibits the expression of p-p38, regulates inflammation and apoptosis through p53 and p38/MAPK signaling pathways, and prevents atherosclerosis (175). In addition, it can inhibit the phosphorylation of JNK, p38, and c-Jun in a dose-dependent manner, and block phosphorylation of ERK1/2 to alleviate LPS-induced inflammation in bovine mammary epithelial cells (bMECs) (176). Salvianolic acid B can downregulate the expression of p-ERK and p-JNK, inhibit the transcription of inflammatory factors, and produce anti-pneumonia effects (177). Vanillic acid significantly reduces the levels of pERK, pJNK, and p-p38, regulates the NF-κB/MAPKs signaling pathway to alleviate the allergic inflammation of HMC-1 (178). Methyl gatellate can inhibit LPS-induced inflammation in mouse macrophages by inhibiting the activation of ERK (179). Rosmarinic acid can inhibit the activation of ERK, JNK, and p38, block MAPK/NF-κB signaling pathway to improve LPS-induced inflammation in vascular smooth muscle cells (180).
When it comes to inhibiting the MAPK pathway, MEHP phenolic acids demonstrate the most profound inhibitory effect on MAPKs. Among the compounds tested, seven hydroxycinnamic acids, three hydroxybenzoic acids, and one hydroxyphenylacetic acid all exhibit inhibitory effects on MAPKs, with most of them capable of suppressing multiple types of MAPKs. In terms of inhibiting MKKKs, one hydroxybenzoic acid and two hydroxycinnamic acids are effective, while two caffeic acid-like hydroxycinnamic acids specifically demonstrate an inhibitory effect on MKKs.
3.3 NLRP3 pathway
NOD-like receptor protein 3(NLRP3) is an inflammasome sensor protein, and the activation of NLRP3 can generate an oligomer complex “Inflammamasone”, which includes apoptosis-associated speck-like protein containing CARD(ASC) and caspase-1. The activation of the typical NLRP3 inflammasome pathway requires two stages: Signal 1 (priming): upregulation of the protein expressions related to inflammasomes (including inflammasome sensor proteins, IL-1β, and IL-18) by upregulating the transcriptional activity of NF-κB. Signal 2 (activation): NLRP3 interacts with pro-caspase-1 after assembly with ASC, then produces a large amount of caspase-1, which catalyzes the dissociation of pro-IL-1β and pro-IL-18 and initiates inflammatory response. MEHP phenolic acids can exert anti-inflammatory activity by inhibiting the NLRP3 pathway; the mechanism is shown in Figure 6.
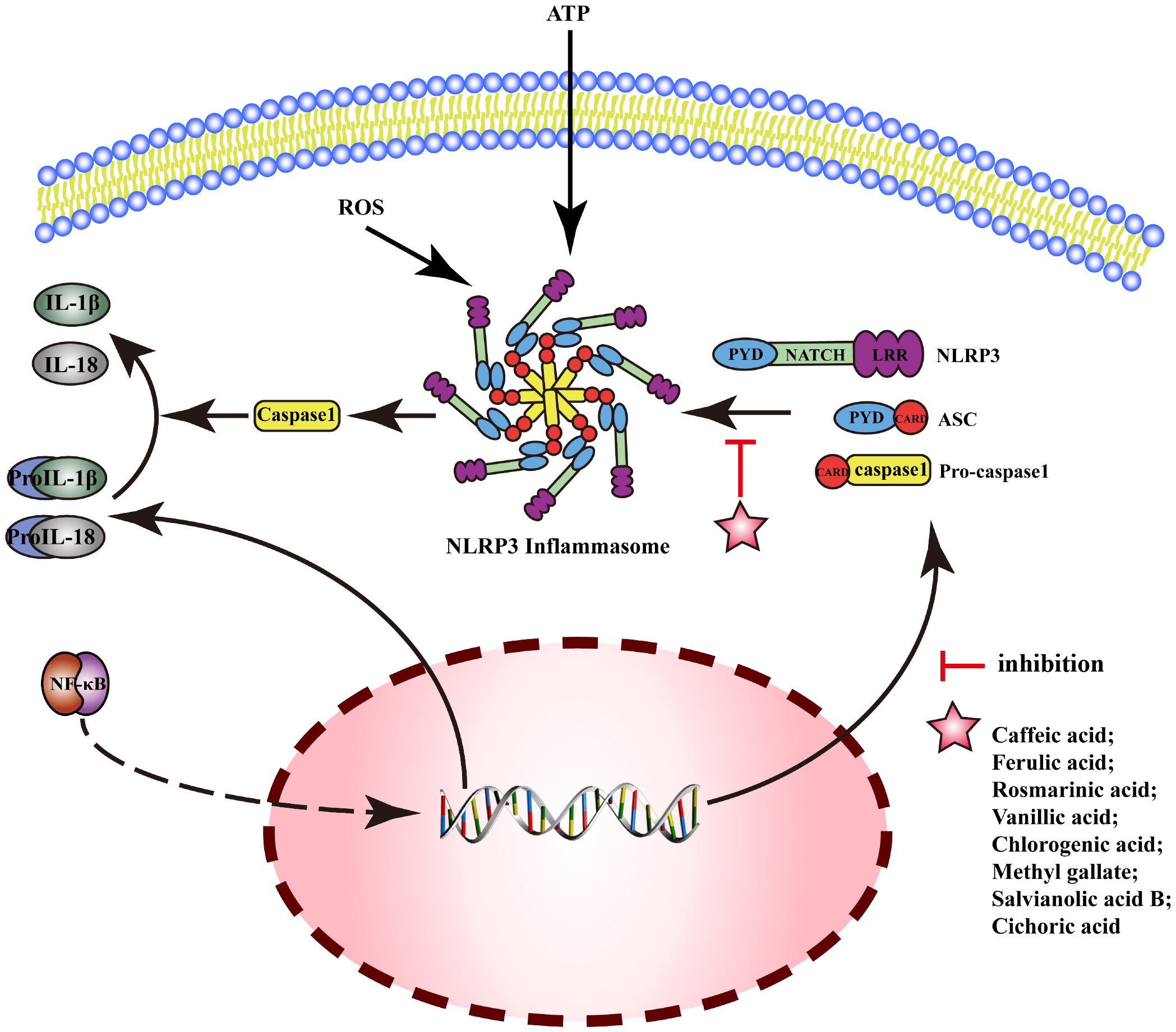
Figure 6 Molecular mechanism of the anti-inflammatory activity of phenolic acids from medicinal and edible homologous plants against the NLRP3 pathway.
In acute gouty arthritis, ferulic acid can exert anti-inflammatory effects by inhibiting the activation of NLRP3 inflammasomes (181). Caffeic acid downregulates mRNA expression of IL-1β and IL-18 to reduce inflammatory reaction of human umbilical vein endothelial cell (HUVEC) induced by advanced glycation end products (AGEs) (182). Rosmarinic acid exerts anti-inflammatory effects by inhibiting the activation and assembly of NLRP3 inflammasomes in psoriasis (183), liver injury (184), and neuroinflammation (185). Vanillic acid can inhibit the activation of NLRP3 inflammasomes and the expression of IL-18 and IL-1β to alleviate arthritis in rats by downregulating the expression of caspase-1, ASC, and NLRP3 (186). Chlorogenic acid improves pneumonia induced by Klebsiella pneumoniae (187), and inhibits periodontal disease (188) by inhibiting activation of NLRP3 inflammasome. Methyl gallate can inhibit the assembly of NLRP3 inflammasome by blocking oligomerization of NLRP3 to alleviate the inflammatory response in mice with hyperuricemic nephropathy (189). Salvianolic acid B attenuates cell death mediated by endoplasmic reticulum stress, by inhibiting NLRP3 inflammasome and reducing the secretion of caspase-1, IL-1β, and IL-18 (190). Cichoric acid decreases the levels of NLRP3, IL-1β, caspase-1, ASC oligomer, and ASC monomer and the release of IL-1β and TNF-α, inhibiting the inflammation in THP-1-derived macrophages (THP-Ms) induced by monosodium urate (MSU) (191).
Reports indicate that two hydroxybenzoic acids and six hydroxycinnamic acids possess the ability to suppress the NLRP3 pathway. Notably, five of these hydroxycinnamic acids share caffeic acid as their common backbone, suggesting that caffeic acid-derived phenolic acids exert the most pronounced inhibitory effect on the NLRP3 pathway.
3.4 Nrf2 pathway
Nuclear factor E2-related factor 2 (Nrf2) is a key transcription factor, that normally, binds to kelch-like ECH-associated protein 1(Keap1) in the cytoplasm, rapidly degrading under the action of ubiquitin proteasome pathway. When cells are stimulated by ROS or other nucleophilic agents, Nrf2 uncouples with Keap1 and is activated by phosphorylation. It is then transported into the nucleus where it competes with p65/p50 to activate the transcription factor CBP, inhibits the binding of p65/p50 to target genes and reduce the transcription of TNF-α, IL-1β, and IL-6 to inhibit the inflammatory response. Therefore, activation of Nrf2 and nuclear translocation of Nrf2 are key links in regulating Nrf2 pathway. The mechanism of MEHP phenolic acids exerting anti-inflammatory activity through Nrf2 pathway is shown in Figure 7.
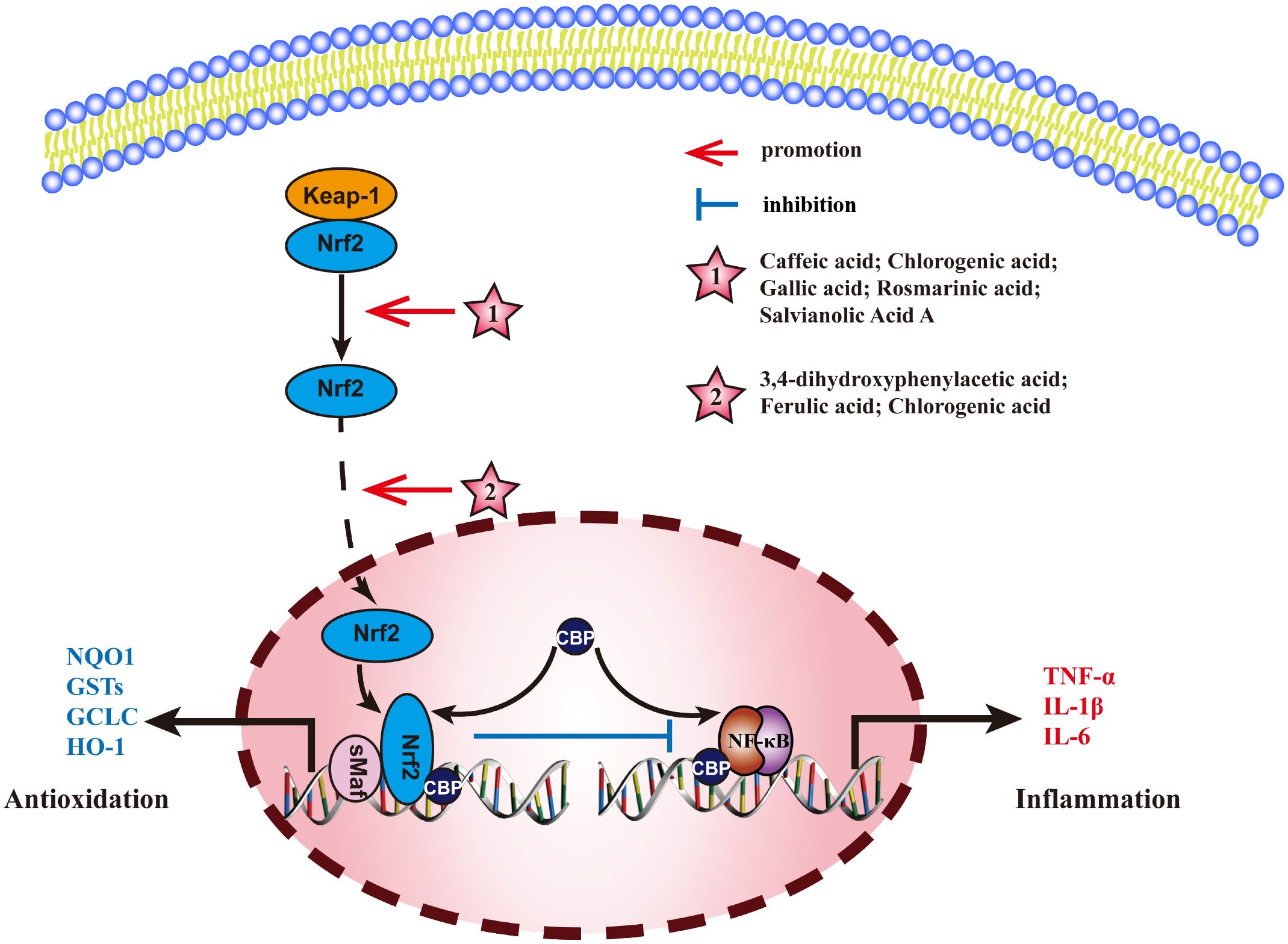
Figure 7 Molecular mechanism of the anti-inflammatory activity of phenolic acids from medicinal and edible homologous plants against the Nrf2 pathway.
3.4.1 Promoting the activation of Nrf2
Caffeic acid exerts an anti-hepatitis effect by upregulating the expression and phosphorylation of P62 (an autophagy substrate), promoting its binding and degradation with Keap1, inducing an increase in Nrf2 expression (192). Chlorogenic acid activates Nrf2/HO-1 pathway to alleviate oxidative stress and inflammatory response, repairs intestinal barrier, and effectively improves DSS-induced colitis (193). Gallic acid inhibits NF-κB pathway by binding to Keap1 and mediating Nrf2 activation, thus exerting anti-pneumonia effect (194). Rosmarinic acid can bind to Keap1, blocking the association between Keap1 and Nrf2 and activating Nrf2, thereby relieving bacterial pneumonia (195). Salvianolic acid A can directly bind to Keap1, promote the activation of Nrf2, and alleviate the inflammatory response in Schwann cells induced by high glucose (196).
3.4.2 Promoting nuclear translocation of Nrf2
3,4-dihydroxyphenylacetic acid can inhibit ethanol-induced hepatotoxicity by increasing Nrf2 protein expression and nuclear translocation (197). Ferulic acid increases the nuclear translocation of Nrf2 to inhibit LPS-induced inflammation in bMECs (198). Chlorogenic acid can improve ischemic brain injury (199), relieve endometritis (200), and regulate blood sugar (201) by increasing nuclear translocation of Nrf2 and inhibiting NF-κB pathway.
Among all the MEHP phenolic acids, hydroxycinnamic acid stands out for its remarkable promoting effect on the Nrf2 pathway. Specifically, five hydroxycinnamic acids, all belonging to the caffeic acid family, can enhance the activation of Nrf2. Furthermore, gallic acid, a hydroxybenzoic acid, also demonstrates a similar effect. Additionally, two hydroxycinnamic acids and one hydroxyphenylacetic acid contribute to the nuclear translocation of Nrf2. Notably, chlorogenic acid is unique in its ability to concurrently promote both the activation and nuclear translocation of Nrf2, thereby exerting significant anti-inflammatory effects.
3.5 TLRs pathway
Toll-like receptors (TLRs) are pattern recognition receptors (PRRs) that recognize microorganisms when they invade the body and activate immune responses. In general, TLRs mainly transduce signals through myeloid differentiation factor 88(MyD88) or TIR-domain containing adaptor inducing interferon-β(TRIF) pathways. MyD88 signals induce the production of inflammatory factors (such as TNF, IL-6, IL-1β) and chemokines (such as C-C motif ligand 4, CCL4). MyD88 binds to TLRs and recruits IRAK4 and IRAK1/2 to Myddosome, which activates (TNF receptor-associated factor 6) TRAF6, induces the activation of NF-κB and MAPK pathways and the expression of proinflammatory cytokines (202). MEHP phenolic acids exert anti-inflammatory effects mainly by interfering with the TLRs/MyD88 pathway (Figure 8).
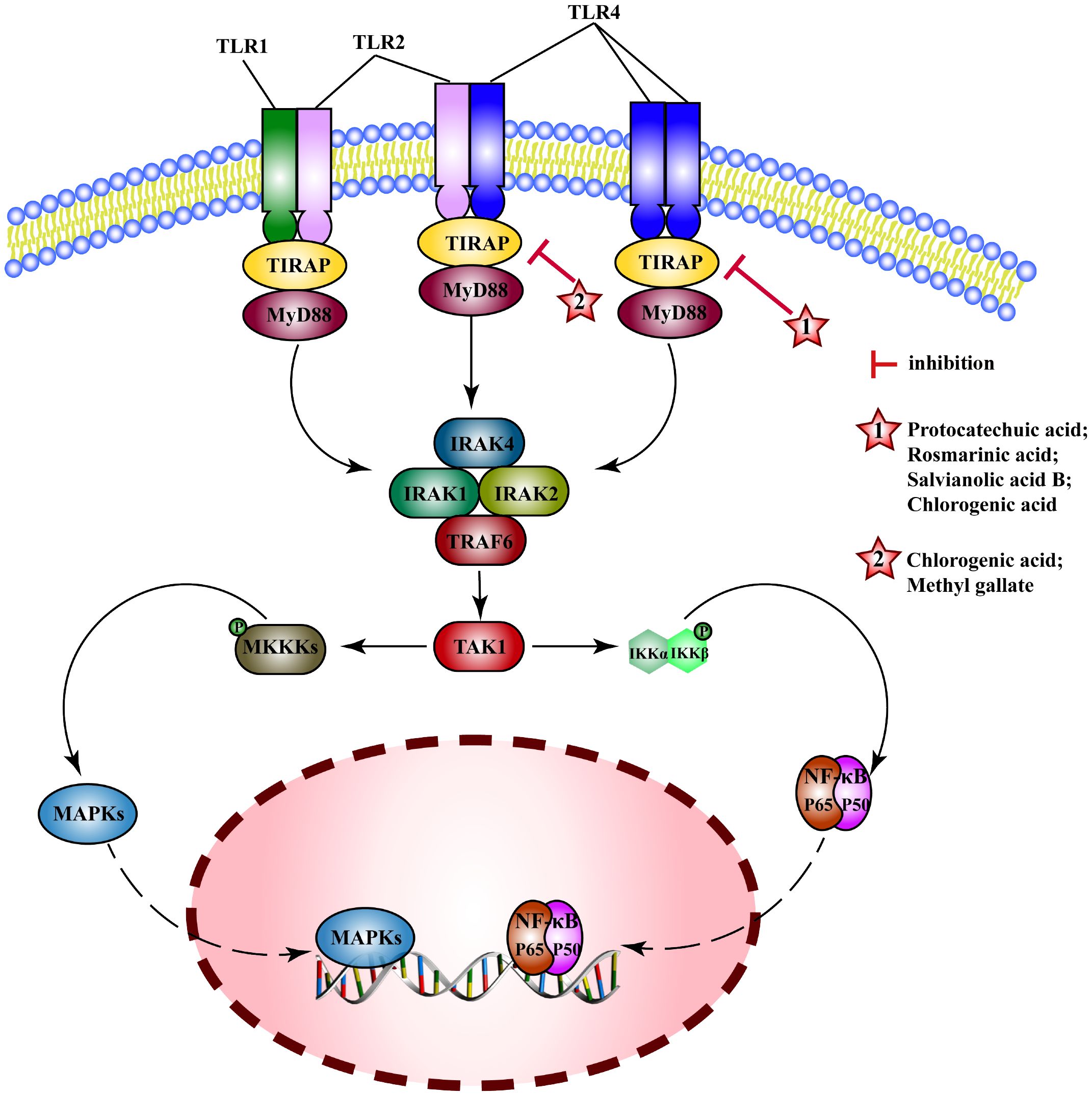
Figure 8 Molecular mechanism of the anti-inflammatory activity of phenolic acids from medicinal and edible homologous plants against the TLRs pathway.
For influenza A in mice (203), acute pancreatitis in rats (204), protocatechuic acid alleviates inflammatory response by reducing the activation of TLR4 and inhibiting NF-κB pathway. Rosmarinic acid can improve neuroinflammation after spinal cord injury (205), relieve hyperlipidemia (206), and inhibit mastitis (207) by inhibiting TLR4/MyD88-NF-κB pathway. Salvianolic acid B attenuates PM 2.5-induced tracheitis in mice by inhibiting TLR4, MyD88, and TRAF6, interfering MAPK pathway and blocking NRLP3 activation (208). Chlorogenic acid inhibits expression of TLR4 and MyD88, interferes with their downstream pathways to improve the intestinal barrier damage in weaned piglets (209), alleviates hepatitis in mice (210) and alcoholic hepatitis in rats (211), and reduces inflammation in mouse glial cells (BV2) (212) and human gingival fibroblasts (HGFs) (213) induced by LPS, and Escherichia coli-induced inflammation in sheep endometrial epithelial cells (SEECs) (214). Chlorogenic acid may also exert anti-inflammatory effects by interfering with other TLRs such as, by inhibiting TLR2/TLR9-Myd88 signaling pathway to attenuate the inflammatory response in herpes encephalitis (215), down-regulating expression of TLR2/4 to decrease activity of NF-κB signaling pathway in epidermal cells, and inhibiting skin inflammation in mice (216). Methyl gallate can inhibit the activation of TLR2 to inhibit NF-κB and MAPK pathway and alleviate toe swelling in mice (217).
Researchers have identified the anti-inflammatory potential of MEHP phenolic acids, primarily by modulating the TLR/MyD88 pathway, showcasing their efficacy in various inflammatory models. Two hydroxybenzoic acids and three caffeic acid based hydroxycinnamic acids exhibit inhibitory effects on the TLRs pathway. Specifically, protocatechuic acid, rosmarinic acid, and salvianolic acid B can suppress the TLR4 pathway, whereas methyl gallate demonstrates inhibitory action towards the TLR2 pathway. Remarkably, chlorogenic acid possesses the ability to simultaneously inhibit the TLR2, TLR4, and TLR9 pathways, thereby exerting anti-inflammatory effects in a diverse range of diseases. These findings signified a wide spectrum of potential MEHP phenolic acid-mediated therapeutic interventions targeting the TLR-mediated inflammatory pathways.
3.6 IL-17 pathway
Interleukin-17 (IL-17) is a potent pro-inflammatory cytokine, which binds to its receptor IL-17R and activates TRAF6 through Act1, leading to the triggering of NF-kB and MAPK pathways.
There are few reported MEHP phenolic acids that can regulate the IL-17 pathway, only three of which are hydroxycinnamic acid. Caffeic acid inhibits expression of IL-17 mRNA in intestinal tissue and alleviates DSS-induced colitis in mice (218). Ferulic acid inhibits secretion of IL-17 and blocks the combination of IL-17A and IL-17RA, thus improving skin inflammation in psoriatic mice (219). Rosmarinic acid can alleviate psoriasis-like dermatitis in mice by decreasing the differentiation of Th17 cells and inhibiting the expression of IL-17A (220) (Figure 9).
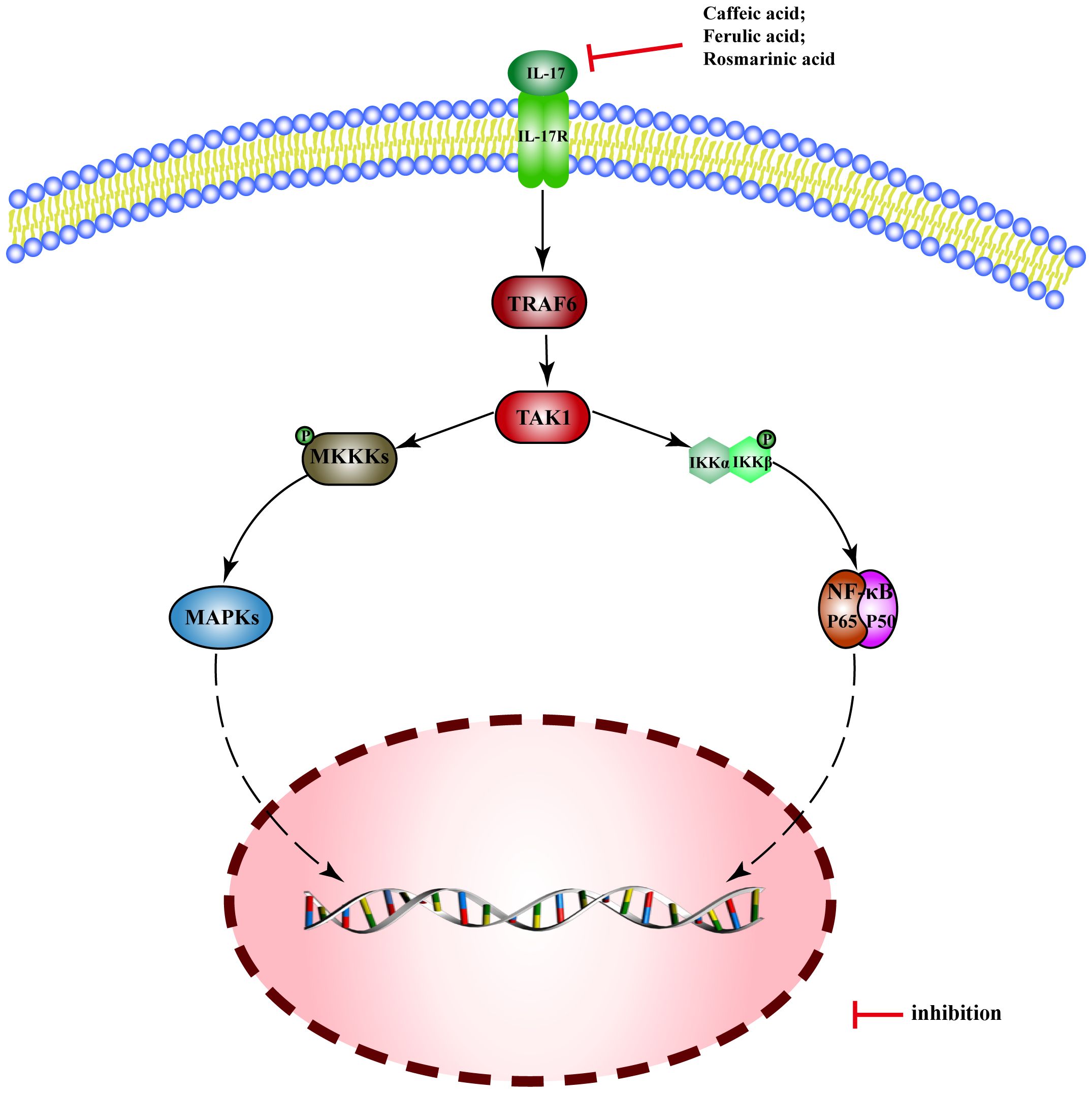
Figure 9 Molecular mechanism of the anti-inflammatory activity of phenolic acids from medicinal and edible homologous plants against the IL-17 pathway.
3.7 Regulating intestinal microflora
Numerous studies show that intestinal microbial species are closely related to anti-inflammatory effects and the by-products of bacterial metabolism, including some short-chain fatty acids (SCFA), can play a role in inhibiting inflammation. Ferulic acid significantly increases intestinal SCFA producing bacteria, as Olsenella, Eisenbergiella, Dubosiella, Clostridiales_unclassified, and Faecalibaculum, reduces endotoxin-producing and obesity-related bacteria, and inhibits the intestinal barrier functional damage induced by a high-fat diet in mice (221). Chlorogenic acid increases the abundance of SCFA-producing bacteria, such as Dubosiella, Romboutsia, Mucispirillum, and Faecalibaculum, as well as Akkermansia, enhanced the integrity of the intestinal barrier, while successfully preventing glucose metabolic disorders and endotoxemia (222).
Gut microbiota abundance and richness are closely associated with inflammation. The increase of Firmicutes and the decrease of Bacteroidetes could inhibit the development of inflammation (223). Protocatechuic acid can enhance the diversity of cecal microbiota, decrease the occurrence of Bacteroidota, Proteobacteria, and Escherichia Shigella, while promoting the abundance of Firmicutes and Lactobacillus, and mitigating Salmonella Typhimurium-induced intestinal barrier damage and inflammatory response in yellow chickens (224). Syringic acid enriches the abundance of Alistipes and norank_f_norank_o_Gastranaerophilales in mice, improving intestinal inflammation (225). Caffeic acid modulates the composition of the gut microbiome by reducing the relative abundance of Bacteroides and Turicibacter, while simultaneously increasing the relative abundance of Alistipes and Dubosiella, enhancing the abundance of Dubosiella and Akkermansia, effectively alleviating DSS-induced colitis in mice (226). Vanillic acid improves LPS-induced intestinal inflammation in weaned piglets by increasing the proportion of Firmicutes/Bacteroidetes, reducing the abundance of Prevotellaceae, and increasing the abundance of Lachoiraceaea, Lachnospira, Eubacterium eligens, and Eubacterium (227). Chlorogenic acid can alleviate colitis induced by a high fat diet in obese rats by reducing the abundance of Blautia, Sutterella, and Akkermansia bacteria and increasing the abundance of Ruminococcus (228).
The gut microbiota boasts a rich and diverse composition, and MEHP phenolic acids can exert anti-inflammatory effects by enhancing its diversity and modulating its richness. Notably, two hydroxybenzoic acids and four hydroxycinnamic acids possess significant effects, with ferulic acid and chlorogenic acid can increase the abundance of bacteria responsible for producing short-chain fatty acids (SCFAs), thereby promoting their production and exerting anti-inflammatory benefits.
3.8 Regulating immune responses
Immune response is a self-protective function of the body, where the appropriate immune response can clear pathogens, but excessive immune response can cause harm to the body; inflammation is a result of a severe immune response. Five distinct types of caffeic acid based hydroxycinnamic acid exhibit outstanding immune responses regulatory effects. Ethyl caffeate can alleviate collagen-induced arthritis in mice by inhibiting Th1 immune response and IFNγ-related signaling pathways (229). Salvianolic acid A regulates the immune response of dermis and inhibits the immune response of Th2/Th17/Th1 to alleviate atopic dermatitis in mice (230). Salvianolic acid B increases the percentage of CD3+CD4+/CD3+CD8+, restores balance of Th1 and Th2 type cytokines to inhibit the inflammatory response induced by a high fat diet (231). Rosmarinic acid inhibits production of IFN-γ and IL-4 from activated CD4+ cells, reduces the infiltration of CD4+, CD8+, and mast cells, slowing down the development of mouse atopic dermatitis (232). Chlorogenic acid can inhibit microglial polarization toward the M1 phenotype and improve neuroinflammation (233).
Collectively, these findings underscore the potent immune-regulatory capabilities of caffeic acid-based hydroxycinnamic acids in various inflammatory and autoimmune conditions, highlighting their potential for therapeutic applications in immune-mediated diseases.
4 Conclusion and future prospects
MEHP phenolic acids exhibit strong and varied anti-inflammatory mechanisms, highlighting their potential for therapeutic innovations. Their action in crucial pathways like NF-κB, MAPK, NLRP, Nrf2, TLRs, and IL-17, along with the regulation of gut microbiota and immune responses, amplifies their effectiveness.
Overall, hydroxycinnamic acid displays the most potent anti-inflammatory activity among MEHP phenolic acids, likely due to its carboxyl group’s adjacent double bond. The number, position, and types of substituents on hydroxyl groups significantly affect the anti-inflammatory effects. Compounds like protocatechuic acid, 4-O-methylgallic acid, 3,4-dihydroxyphenylacetic acid, gentic acid, gallic acid, danshensu, caffeic acid, etc with two or more hydroxyl groups, mainly have hydroxyl substitutions in the para position. Phenolic acid molecules with alkoxy (e.g., methoxy) or alkyl (e.g., methyl) substituents might enhance their compatibility with biomolecules (like enzymes or receptors) by increasing their lipid solubility or by stabilizing hydroxyl radicals, thus amplifying their anti-inflammatory potential. This is observed in compounds such as vanillic acid, ferulic acid, etc. Specifically, phenolic acids with catechol-like configurations, exhibiting two adjacent hydroxyl groups, are characterized by their robust antioxidant capabilities, enabling them to effectively neutralize free radicals and display pronounced anti-inflammatory properties. This is exemplified by Protocatechuic acid among all coffee acid derivatives. Caffeic acids, including caffeic acid, rosmarinic acid, chlorogenic acid, etc stand out due to their structural benefits, playing pivotal roles across varied anti-inflammatory pathways. This highlights a promising strategy for the structural refinement and enhancement of phenolic acids to bolster their therapeutic outcomes.
While advancements have been noted in the research of MEHP phenolic acids, several hurdles remain for their clinical utilization. A significant challenge is pinpointing the dosage that is both efficacious and safe, given the potential for toxicity at elevated levels. Moreover, the interplay between phenolic acids and other medications could potentially influence their therapeutic efficacy. Furthermore, variability across batches of MEHP phenolic acids demands stringent standardization and quality control measures. Additionally, the long-term safety and any adverse effects of phenolic acids are subjects that warrant further investigation. Lastly, there is an evident need for more clinical trials to substantiate the therapeutic efficacy and safety of phenolic acids in managing inflammatory conditions.
To meaningfully tackle these challenges, considerable research on MEHP phenolic acids remains to be conducted. Firstly, delving into the correlation between the molecular structure of phenolic acids and their biological activity is essential, enabling the design and development of more potent phenolic acid derivatives. Secondly, the innovation of drug delivery systems should be prioritized to enhance the bioavailability and stability of these compounds. Personalization of phenolic acid therapy, tailored to an individual’s genetic and metabolic profile, presents a promising avenue for exploration. Moreover, investigating the synergistic use of phenolic acids with other pharmaceuticals or therapeutic approaches could potentially amplify their therapeutic impact. Notably, unraveling the intricate molecular mechanisms of phenolic acids, particularly their influence on cellular signaling pathways, is also a critical area for further research. Lastly, an increased number of clinical trials are imperative to yield conclusive evidence regarding the efficacy and safety of phenolic acids in combating inflammatory diseases.
In essence, MEHP phenolic acids possess significant commercial potential as both “anti-inflammatory drugs” and “ anti-inflammatory functional foods,” thereby fostering a healthier future for all.
Author contributions
JX: Writing – original draft. SX: Writing – original draft. YL: Writing – review & editing. BX: Writing – review & editing. ML: Writing – review & editing. ZZ: Writing – review & editing. ZS: Writing – review & editing. QP: Writing – review & editing. CL: Writing – review & editing. DL: Conceptualization, Project administration, Writing – review & editing. LL: Conceptualization, Project administration, Writing – review & editing.
Funding
The author(s) declare financial support was received for the research, authorship, and/or publication of this article. This work was supported by Hunan Province Science and Technology Innovation Leading Talent Project (No. 2021RC4034, China), Hunan Science and Technology Innovation Team Project (No. 2021RC4064, China), Hunan Provincial Natural Science Foundation (No. 2022JJ80085, No. 2022JJ80020, China), Changsha Municipal Science Foundation (No. kq2202268, China), and the Key Discipline Project on Chinese Pharmacology of Hunan University of Chinese Medicine (No. 202302, China), Research and Innovation Project for Graduate Students in Hunan Province (No. CX20230789).
Conflict of interest
The authors declare that the research was conducted in the absence of any commercial or financial relationships that could be construed as a potential conflict of interest.
Publisher’s note
All claims expressed in this article are solely those of the authors and do not necessarily represent those of their affiliated organizations, or those of the publisher, the editors and the reviewers. Any product that may be evaluated in this article, or claim that may be made by its manufacturer, is not guaranteed or endorsed by the publisher.
References
1. Lu Q, Li R, Yang Y, Zhang Y, Zhao Q, Li J. Ingredients with anti-inflammatory effect from medicine food homology plants. Food Chem. (2022) 368:130610. doi: 10.1016/j.foodchem.2021.130610
2. Anna LK. Inflammation in focus: the beginning and the end. Pathol Oncol Res. (2021) 27:1610136. doi: 10.3389/pore.2021.1610136
3. Xue H, Wang W, Bian J, Gao Y, Hao Z, Tan J. Recent advances in medicinal and edible homologous polysaccharides: Extraction, purification, structure, modification, and biological activities. Int J Biol Macromol. (2022) 222:1110–26. doi: 10.1016/j.ijbiomac.2022.09.227
4. Lu Q, Xie Y, Luo J, Gong Q, Li C. Natural flavones from edible and medicinal plants exhibit enormous potential to treat ulcerative colitis. Front Pharmacol. (2023) 14:1168990. doi: 10.3389/fphar.2023.1168990
5. Galanakis CM. Phenols recovered from olive mill wastewater as additives in meat products. Trends Food Sci Technology. (2018) 79:98–105. doi: 10.1016/j.tifs.2018.07.010
6. Yahfoufi N, Alsadi N, Jambi M, Matar C. The immunomodulatory and anti-inflammatory role of polyphenols. Nutrients. (2018) 10:1618. doi: 10.3390/nu10111618
7. Sun W, Shahrajabian MH. Therapeutic potential of phenolic compounds in medicinal plants—Natural health products for human health. Molecules. (2023) 28:1845. doi: 10.3390/molecules28041845
8. Ekiert H, Klimek-Szczykutowicz M, Rzepiela A, Klin P, Szopa A. Artemisia species with high biological values as a potential source of medicinal and cosmetic raw materials. Molecules. (2022) 27:6427. doi: 10.3390/molecules27196427
9. Valanciene E, Jonuskiene I, Syrpas M, Augustiniene E, Matulis P, Simonavicius A, et al. Advances and prospects of phenolic acids production, biorefinery and analysis. Biomolecules. (2020) 10:874. doi: 10.3390/biom10060874
10. Malarz J, Yudina YV, Stojakowska A. Hairy root cultures as a source of phenolic antioxidants: simple phenolics, phenolic acids, phenylethanoids, and hydroxycinnamates. Int J Mol Sci. (2023) 24:6920. doi: 10.3390/ijms24086920
11. Oluwole O, Fernando WB, Lumanlan J, Ademuyiwa O, Jayasena V. Role of phenolic acid, tannins, stilbenes, lignans and flavonoids in human health – a review. Int J Food Sci Tech. (2022) 57:6326–35. doi: 10.1111/ijfs.15936
12. Rashmi HB, Negi PS. Phenolic acids from vegetables: A review on processing stability and health benefits. Food Res Int. (2020) 136:109298. doi: 10.1016/j.foodres.2020.109298
13. Pan X, Ma X, Jiang Y, Wen J, Yang L, Chen D, et al. A comprehensive review of natural products against liver fibrosis: flavonoids, quinones, lignans, phenols, and acids. Evid Based Complement Alternat Med. (2020) 2020:7171498. doi: 10.1155/2020/7171498
14. Hazafa A, Iqbal MO, Javaid U, Tareen MBK, Amna D, Ramzan A, et al. Inhibitory effect of polyphenols (phenolic acids, lignans, and stilbenes) on cancer by regulating signal transduction pathways: a review. Clin Transl Oncol. (2021) 24:432–45. doi: 10.1007/s12094–021-02709–3
15. Kiokias S, Oreopoulou V. A review of the health protective effects of phenolic acids against a range of severe pathologic conditions (Including coronavirus-based infections). Molecules. (2021) 26:5405. doi: 10.3390/molecules26175405
16. Cordeiro M, Martins V, Silva A, Rocha HAO, Rachetti V, Scortecci KC. Phenolic acids as antidepressant agents. Nutrients. (2022) 14:4309. doi: 10.3390/nu14204309
17. Magiera S, Zaręba M. Chromatographic determination of phenolic acids and flavonoids in lycium barbarum L. and evaluation of antioxidant activity. Food Anal Methods. (2015) 8:2665–74. doi: 10.1007/s12161-015-0166-y
18. Dossou SSK, Xu F, Cui X, Sheng C, Zhou R, You J, et al. Comparative metabolomics analysis of different sesame (Sesamum indicum L.) tissues reveals a tissue-specific accumulation of metabolites. BMC Plant Biol. (2021) 21:352. doi: 10.1186/s12870–021-03132–0
19. Menghini L, Leporini L, Vecchiotti G, Locatelli M, Carradori S, Ferrante C, et al. Crocus sativus L. stigmas and byproducts: Qualitative fingerprint, antioxidant potentials and enzyme inhibitory activities. Food Res Int. (2018) 109:91–8. doi: 10.1016/j.foodres.2018.04.028
20. Jin JH, Cheng ZH, Chen DF. Two new compounds and anti-complementary constituents from amomum tsao-ko. Nat Prod Commun. (2013) 8:1715–8. doi: 10.1177/1934578x1300801214
21. Khalaf HA, El-Saadani RM, El-Desouky AI, Abdeldaiem MH, Elmehy ME. Antioxidant and antimicrobial activity of gamma-irradiated chicory (Cichorium intybus L.) leaves and roots. Food Measure. (2018) 12:1843–51. doi: 10.1007/s11694–018-9798–0
22. Tkacz K, Wojdyło A, Turkiewicz IP, Nowicka P. Triterpenoids, phenolic compounds, macro- and microelements in anatomical parts of sea buckthorn (Hippophaë rhamnoides L.) berries, branches and leaves. J Food Compos Anal. (2021) 103:104107. doi: 10.1016/j.jfca.2021.104107
23. Ahmed HM, Mohan Al-Zubaidy A, Othman-Qadir G. Biological investigations on macro-morphological characteristics, polyphenolic acids, antioxidant activity of Perilla frutescens (L) Britt. grown under open field. Saudi J Biol Sci. (2022) 29:3213–22. doi: 10.1016/j.sjbs.2022.01.059
24. Jiang R, Xu XH, Wang K, Yang XZ, Bi YF, Yan Y, et al. Ethyl acetate extract from Panax ginseng C.A. Meyer and its main constituents inhibit alpha-melanocyte-stimulating hormone-induced melanogenesis by suppressing oxidative stress in B16 mouse melanoma cells. J Ethnopharmacol. (2017) 208:149–56. doi: 10.1016/j.jep.2017.07.004
25. Esmaeili N, Ebrahimzadeh H, Abdi K, Safarian S. Determination of some phenolic compounds in Crocus sativus L. corms and its antioxidant activities study. Pharmacogn Mag. (2011) 7:74–80. doi: 10.4103/0973–1296.75906
26. Pal K, Chowdhury S, Dutta SK, Chakraborty S, Chakraborty M. Pandit GK, et al. Analysis of rhizome colour content, bioactive compound profiling and ex-situ conservation of turmeric genotypes (Curcuma longa L.) from sub-Himalayan terai region of India. Ind Crops Prod. (2020) 150:112401. doi: 10.1016/j.indcrop.2020.112401
27. He CN, Gao WW, Yang JX, Bi W, Zhang XS, Zhao YJ. Identification of autotoxic compounds from fibrous roots of Panax quinquefolium L. Plant Soil. (2008) 318:63–72. doi: 10.1007/s11104–008-9817–8
28. Zadernowski R, Naczk M, Czaplicki S, Rubinskiene M, Sza´lkiewicz M. Composition of phenolic acids in sea buckthorn (Hippophae rhamnoides L.) berries. J Am Oil Chem Soc. (2005) 82:175–9. doi: 10.1007/s11746–005-5169–1
29. Ma Y, Wang P, Chen Z, Gu Z, Yang R. NaCl stress on physio-biochemical metabolism and antioxidant capacity in germinated hulless barley (Hordeum vulgare L.). J Sci Food Agric. (2019) 99:1755–64. doi: 10.1002/jsfa.9365
30. Shen P, Gu Y, Zhang C, Sun C, Qin L, Yu C, et al. Metabolomic Approach for Characterization of Polyphenolic Compounds in Laminaria japonica, Undaria pinnatifida, Sargassum fusiforme and Ascophyllum nodosum. Foods. (2021) 10:192. doi: 10.3390/foods10010192
31. Kumnerdkhonkaen P, Saenglee S, Asgar MA, Senawong G, Khongsukwiwat K, Senawong T. Antiproliferative activities and phenolic acid content of water and ethanolic extracts of the powdered formula of Houttuynia cordata Thunb. fermented broth and Phyllanthus emblica Linn. fruit. BMC Complement Altern Med. (2018) 18:130. doi: 10.1186/s12906-018-2185-x
32. Tohma H, Gülçin İ, Bursal E, Gören AC, Alwasel SH, Köksal E. Antioxidant activity and phenolic compounds of ginger (Zingiber officinale Rosc.) determined by HPLC-MS/MS. Food Measure. (2016) 11:556–66. doi: 10.1007/s11694-016-9423-z
33. Oppong MB, Zhang B-Y, Fang S-M, Qiu F. Secondary metabolites from Sterculia lychnophora Hance (Pangdahai). Biochem Systematics Ecology. (2020) 92:104125. doi: 10.1016/j.bse.2020.104125
34. Sánchez-Salcedo EM, Mena P, García-Viguera C, Martínez JJ, Hernández F. Phytochemical evaluation of white (Morus alba L.) and black (Morus nigra L.) mulberry fruits, a starting point for the assessment of their beneficial properties. J Funct Foods. (2015) 12:399–408. doi: 10.1016/j.jff.2014.12.010
35. Rho T, Yoon KD. Chemical constituents of nelumbo nucifera seeds. Natural Product Sci. (2017) 23:253–7. doi: 10.20307/nps.2017.23.4.253
36. Limwachiranon J, Huang H, Shi Z, Li L, Luo Z. Lotus flavonoids and phenolic acids: health promotion and safe consumption dosages. Compr Rev Food Sci Food Saf. (2018) 17:458–71. doi: 10.1111/1541–4337.12333
37. Zeb A, Haq A, Murkovic M. Effects of microwave cooking on carotenoids, phenolic compounds and antioxidant activity of Cichorium intybus L. (chicory) Leaves Eur Food Res Technol. (2018) 245:365–74. doi: 10.1007/s00217–018-3168–3
38. Jun HI, Kim BT, Song GS, Kim YS. Structural characterization of phenolic antioxidants from purple perilla (Perilla frutescens var. acuta) leaves. Food Chem. (2014) 148:367–72. doi: 10.1016/j.foodchem.2013.10.028
39. Wei WL, Zeng R, Gu CM, Qu Y, Huang LF. Angelica sinensis in China-A review of botanical profile, ethnopharmacology, phytochemistry and chemical analysis. J Ethnopharmacol. (2016) 190:116–41. doi: 10.1016/j.jep.2016.05.023
40. Wang SY, Zhao H, Xu HT, Han XD, Wu YS, Xu FF, et al. Kaempferia galanga L.: progresses in phytochemistry, pharmacology, toxicology and ethnomedicinal uses. Front Pharmacol. (2021) 12:675350. doi: 10.3389/fphar.2021.675350
41. Abu-Izneid T, Rauf A, Khalil AA, Olatunde A, Khalid A, Alhumaydhi FA, et al. Nutritional and health beneficial properties of saffron (Crocus sativus L): a comprehensive review. Comput Math Methods Med. (2022) 62:2683–706. doi: 10.1080/10408398.2020.1857682
42. Jeon JS, Kim J, Park S, Ryou C, Kim CY. Preparative purification of plasmin activity stimulating phenolic derivatives from Gastrodia elata using centrifugal partition chromatography. BioMed Chromatogr. (2016) 30:976–82. doi: 10.1002/bmc.3640
43. Feng Y, Dunshea FR, Suleria HAR. LC-ESI-QTOF/MS characterization of bioactive compounds from black spices and their potential antioxidant activities. J Food Sci Technol. (2020) 57:4671–87. doi: 10.1007/s13197–020-04504–4
44. Chung IM, Lim JJ, Ahn MS, Jeong HN, An TJ, Kim SH. Comparative phenolic compound profiles and antioxidative activity of the fruit, leaves, and roots of Korean ginseng (Panax ginseng Meyer) according to cultivation years. J Ginseng Res. (2016) 40:68–75. doi: 10.1016/j.jgr.2015.05.006
45. Palmieri S, Pellegrini M, Ricci A, Compagnone D, Lo Sterzo C. Chemical composition and antioxidant activity of thyme, hemp and coriander extracts: A comparison study of maceration, soxhlet, UAE and RSLDE techniques. Foods. (2020) 9:1221. doi: 10.3390/foods9091221
46. Ramakrishna R, Sarkar D, Manduri A, Iyer SG, Shetty K. Improving phenolic bioactive-linked anti-hyperglycemic functions of dark germinated barley sprouts (Hordeum vulgare L.) using seed elicitation strategy. J Food Sci Technol. (2017) 54:3666–78. doi: 10.1007/s13197–017-2828–9
47. Chen SN, Xie RP, Li J, Fan YW, Liu XR, Zhang B, et al. Alteration on phenolic acids and the appearance of lotus (Nelumbo nucifera Gaertn) seeds dealt with antistaling agents during storage. Int J Food Prop. (2018) 21:1481–94. doi: 10.1080/10942912.2018.1489834
48. Yang DJ, Chang YY, Hsu CL, Liu CW, Lin YL, Lin YH, et al. Antiobesity and hypolipidemic effects of polyphenol-rich longan (Dimocarpus longans Lour.) flower water extract in hypercaloric-dietary rats. J Agric Food Chem. (2010) 58:2020–7. doi: 10.1021/jf903355q
49. Olech M, Nowak R, Załuski D, Kapusta I, Amarowicz R, Oleszek W. Hyaluronidase, acetylcholinesterase inhibiting potential, antioxidant activity, and LC-ESI-MS/MS analysis of polyphenolics of rose (Rosa rugosa Thunb.) teas and tinctures. Int J Food Prop. (2017) 20:S16–25. doi: 10.1080/10942912.2017.1287722
50. Li X, Li X, Zhou B, Man S, Gao W, Jing S. Study on the Bioactive Constituents and in vitro Antioxidant and in vivo Antiinflammatory Activities of Extracts from the Fruits of Ziziphus Jujuba Mill. cv. Jinsixiaozao Hort. Food Sci Technol Res. (2017) 23:417–26. doi: 10.3136/fstr.23.417
51. Saravanakumar K, Park S, Sathiyaseelan A, Kim KN, Cho SH, Mariadoss AVA, et al. Metabolite profiling of methanolic extract of gardenia jaminoides by LC-MS/MS and GC-MS and its anti-diabetic, and anti-oxidant activities. Pharm (Basel). (2021) 14:102. doi: 10.3390/ph14020102
52. Wang X, Cheng K, Liu Z, Sun Y, Zhou L, Xu M, et al. Bioactive constituents of Mosla chinensis-cv. Jiangxiangru ameliorate inflammation through MAPK signaling pathways and modify intestinal microbiota in DSS-induced colitis mice. Phytomedicine. (2021) 93:153804. doi: 10.1016/j.phymed.2021.153804
53. Sanchez-Salcedo EM, Tassotti M, Del Rio D, Hernandez F, Martinez JJ, Mena P. (Poly)phenolic fingerprint and chemometric analysis of white (Morus alba L.) and black (Morus nigra L.) mulberry leaves by using a non-targeted UHPLC-MS approach. Food Chem. (2016) 212:250–5. doi: 10.1016/j.foodchem.2016.05.121
54. An LJ, Guan S, Shi GF, Bao YM, Duan YL, Jiang B. Protocatechuic acid from Alpinia oxyphylla against MPP+-induced neurotoxicity in PC12 cells. Food Chem Toxicol. (2006) 44:436–43. doi: 10.1016/j.fct.2005.08.017
55. Sahin S, Demir C, Malyer H. Determination of phenolic compounds in Prunella L. by liquid chromatography-diode array detection. J Pharm BioMed Anal. (2011) 55:1227–30. doi: 10.1016/j.jpba.2011.03.016
56. Czerwinska ME, Melzig MF. Cornus mas and cornus officinalis-analogies and differences of two medicinal plants traditionally used. Front Pharmacol. (2018) 9:894. doi: 10.3389/fphar.2018.00894
57. Ren X-M, Han Z-Z, Song L-X, Lv Z-Y, Yang Y-B, Xiao Y, et al. Four new phenolic compounds from the tender leaves of Eucommia ulmoides Oliv. and their anti-inflammatory activities. Phytochem Lett. (2021) 44:173–7. doi: 10.1016/j.phytol.2021.06.020
58. Doan LP, Nguyen TT, Pham MQ, Tran QT, Pham QL, Tran DQ, et al. Extraction process, identification of fatty acids, tocopherols, sterols and phenolic constituents, and antioxidant evaluation of seed oils from five fabaceae species. Processes. (2019) 7:456. doi: 10.3390/pr7070456
59. Shao F, Gu L, Chen H, Liu R, Huang H, Chen L, et al. Evaluation of hypolipidemic and antioxidant effects in phenolrich fraction of crataegus pinnatifida fruit in hyperlipidemia rats and identification of chemical composition by ultra-performance liquid chromatography coupled with quadropole time-of-flight mass spectrometry. Pharmacogn Mag. (2017) 13:725–31. doi: 10.4103/pm.pm_402_16
60. Zompra AA, Chasapi SA, Karagkouni EC, Karamouzi E, Panopoulos P, Spyroulias GA. Metabolite and bioactive compounds profiling of meteora sea buckthorn berries through high-resolution NMR analysis. Metabolites. (2021) 11:822. doi: 10.3390/metabo11120822
61. Choi CY, Cho SS, Yoon IS. Hot-water extract of the branches of Hovenia dulcis Thunb. (Rhamnaceae) ameliorates low-fiber diet-induced constipation in rats. Drug Des Devel Ther. (2018) 12:695–703. doi: 10.2147/DDDT.S150284
62. Li W, Yang HJ. Phenolic constituents from platycodon grandiflorum root and their anti-inflammatory activity. Molecules. (2021) 26:4530. doi: 10.3390/molecules26154530
63. Ghimire BK, Yu CY, Chung IM. Assessment of the phenolic profile, antimicrobial activity and oxidative stability of transgenic Perilla frutescens L.overexpressing tocopherol methyltransferase (gamma-tmt) gene. Plant Physiol Biochem. (2017) 118:77–87. doi: 10.1016/j.plaphy.2017.06.006
64. Zhou XJ, Yan LL, Yin PP, Shi LL, Zhang JH, Liu YJ, et al. Structural characterisation and antioxidant activity evaluation of phenolic compounds from cold-pressed Perilla frutescens var. arguta seed flour. Food Chem. (2014) 164:150–7. doi: 10.1016/j.foodchem.2014.05.062
65. Liu J, Xu X, Jiang R, Sun L, Zhao D. Vanillic acid in Panax ginseng root extract inhibits melanogenesis in B16F10 cells via inhibition of the NO/PKG signaling pathway. Biosci Biotechnol Biochem. (2019) 83:1205–15. doi: 10.1080/09168451.2019.1606694
66. Jia W, Bi Q, Jiang S, Tao J, Liu L, Yue H, et al. Hypoglycemic activity of Codonopsis pilosula (Franch.) Nannf. in vitro and in vivo and its chemical composition identification by UPLC-Triple-TOF-MS/MS. Food Funct. (2022) 13:2456–64. doi: 10.1039/D1FO03761G
67. Ye Z, Dai JR, Zhang CG, Lu Y, Wu LL, Gong AGW, et al. Chemical Differentiation of Dendrobium officinale and Dendrobium devonianum by Using HPLC Fingerprints, HPLC-ESI-MS, and HPTLC Analyses. Evid Based Complement Alternat Med. (2017) 2017:8647212. doi: 10.1155/2017/8647212
68. Li Z, Wang Y, Ouyang H, Lu Y, Qiu Y, Feng Y, et al. A novel dereplication strategy for the identification of two new trace compounds in the extract of Gastrodia elata using UHPLC/Q-TOF-MS/MS. J Chromatogr B. (2015) 988:45–52. doi: 10.1016/j.jchromb.2015.02.020
69. Habib HM, Theuri SW, Kheadr EE, Mohamed FE. Functional, bioactive, biochemical, and physicochemical properties of the Dolichos lablab bean. Food Funct. (2017) 8:872–80. doi: 10.1039/C6FO01162D
70. Fukalova Fukalova T, Garcia-Martinez MD, Raigon MD. Nutritional Composition, Bioactive Compounds, and Volatiles Profile Characterization of Two Edible Undervalued Plants: Portulaca oleracea L. and Porophyllum ruderale (Jacq.) Cass. Plants (Basel). (2022) 11:377. doi: 10.3390/plants11030377
71. Wu M, Cai J, Fang Z, Li S, Huang Z, Tang Z, et al. The composition and anti-aging activities of polyphenol extract from phyllanthus emblica L. Fruit. Nutrients. (2022) 14:857. doi: 10.3390/nu14040857
72. Wang E, Li Y, Maguy BL, Lou Z, Wang H, Zhao W, et al. Separation and enrichment of phenolics improved the antibiofilm and antibacterial activity of the fractions from Citrus medica L. var. sarcodactylis in vitro and in tofu. Food Chem. (2019) 294:533–8. doi: 10.1016/j.foodchem.2019.05.038
73. Xue X, Zhao A, Wang Y, Ren H, Du J, Li D, et al. Composition and content of phenolic acids and flavonoids among the different varieties, development stages, and tissues of Chinese Jujube (Ziziphus jujuba Mill.). PloS One. (2021) 16:e0254058. doi: 10.1371/journal.pone.0254058
74. Pedro AC, Maurer JBB, Zawadzki-Baggio SF, Ávila S, Maciel GM, Haminiuk CWI. Bioactive compounds of organic goji berry ( Lycium barbarum L.) prevents oxidative deterioration of soybean oil. Ind Crops Prod. (2018) 112:90–7. doi: 10.1016/j.indcrop.2017.10.052
75. Butkhup L, Samappito W, Samappito S. Phenolic composition and antioxidant activity of white mulberry (Morus albaL.) fruits. Int J Food Sci Tech. (2013) 48:934–40. doi: 10.1111/ijfs.12044
76. Polumackanycz M, Wesolowski M, Viapiana A. Morus alba L. and Morus nigra L. Leaves as a Promising Food Source of Phenolic Compounds with Antioxidant Activity. Plant Foods Hum Nutr. (2021) 76:458–65. doi: 10.1007/s11130–021-00922–7
77. Costanzo G, Vitale E, Iesce MR, Naviglio D, Amoresano A, Fontanarosa C, et al. Antioxidant Properties of Pulp, Peel and Seeds of Phlegrean Mandarin (Citrus reticulata Blanco) at Different Stages of Fruit Ripening. Antioxidants (Basel). (2022) 11:187. doi: 10.3390/antiox11020187
78. Filipiak-Szok A, Kurzawa M, Szlyk E, Twaruzek M, Blajet-Kosicka A, Grajewski J. Determination of mycotoxins, alkaloids, phytochemicals, antioxidants and cytotoxicity in Asiatic ginseng (Ashwagandha, Dong quai, Panax ginseng). Chem Zvesti. (2017) 71:1073–82. doi: 10.1007/s11696–016-0028–0
79. Dong Y, Feng ZL, Chen HB, Wang FS, Lu JH. Corni Fructus: a review of chemical constituents and pharmacological activities. Chin Med. (2018) 13:34. doi: 10.1186/s13020-018-0191-z
80. Sdouga D, Branca F, Kabtni S, Di Bella MC, Trifi-Farah N, Marghali S. Morphological traits and phenolic compounds in Tunisian wild populations and cultivated varieties of portulaca oleracea L. Agronomy. (2020) 10:948. doi: 10.3390/agronomy10070948
81. Alonso-Esteban JI, Pinela J, Ciric A, Calhelha RC, Sokovic M, Ferreira I, et al. Chemical composition and biological activities of whole and dehulled hemp (Cannabis sativa L.) seeds. Food Chem. (2022) 374:131754. doi: 10.1016/j.foodchem.2021.131754
82. Li F, Zhang B, Chen G, Fu X. The novel contributors of anti-diabetic potential in mulberry polyphenols revealed by UHPLC-HR-ESI-TOF-MS/MS. Food Res Int. (2017) 100:873–84. doi: 10.1016/j.foodres.2017.06.052
83. Zou W, Gong L, Zhou F, Long Y, Li Z, Xiao Z, et al. Anti-inflammatory effect of traditional Chinese medicine preparation Penyanling on pelvic inflammatory disease. J Ethnopharmacol. (2021) 266:113405. doi: 10.1016/j.jep.2020.113405
84. Ai Z, Zhang Y, Li X, Sun W, Liu Y. Widely targeted metabolomics analysis to reveal transformation mechanism of cistanche deserticola active compounds during steaming and drying processes. Front Nutr. (2021) 8:742511. doi: 10.3389/fnut.2021.742511
85. Wang Y, Xiang L, Wang C, Tang C, He X. Antidiabetic and antioxidant effects and phytochemicals of mulberry fruit (Morus alba L.) polyphenol enhanced extract. PloS One. (2013) 8:e71144. doi: 10.1371/journal.pone.0071144
86. Mondal M, Saha S, Sarkar C, Hossen MS, Hossain MS, Khalipha ABR, et al. Role of citrus medica L. Fruits extract in combatting the hematological and hepatic toxic effects of carbofuran. Chem Res Toxicol. (2021) 34:1890–902. doi: 10.1021/acs.chemrestox.1c00166
87. Bai Y, Xia B, Xie W, Zhou Y, Xie J, Li H, et al. Phytochemistry and pharmacological activities of the genus Prunella. Food Chem. (2016) 204:483–96. doi: 10.1016/j.foodchem.2016.02.047
88. Gundogdu M, Muradoglu F, Sensoy RIG, Yilmaz H. Determination of fruit chemical properties of Morus nigra L., Morus alba L. and Morus rubra L. by HPLC. Sci Hortic. (2011) 132:37–41. doi: 10.1016/j.scienta.2011.09.035
89. Horinishi A, Osaki S, Masuda T, Nomura E, Tanaka Y. Nakamura Y-i, et al. Proanthocyanidin in the fruit of Japanese apricot (Prunus mume Sieb. et Zucc.) and their structural estimation by HPLC-ESI-MS/MS. J Food Compos Anal. (2021) 103:104039. doi: 10.1016/j.jfca.2021.104039
90. Ganzon JG, Chen LG, Wang CC. 4-O-Caffeoylquinic acid as an antioxidant marker for mulberry leaves rich in phenolic compounds. J Food Drug Anal. (2018) 26:985–93. doi: 10.1016/j.jfda.2017.11.011
91. Ying L, Wang D, Du G. Analysis of bioactive components in the fruit, roots, and leaves of alpinia oxyphylla by UPLC-MS/MS. Evid Based Complement Alternat Med. (2021) 2021:5592518. doi: 10.1155/2021/5592518
92. Liu W, Zhu DW, Liu DH, Geng MJ, Yang TW, Wang X. Influence of P Deficiency on Major Secondary Metabolism in Flavonoids Synthesis Pathway ofChrysanthemum morifoliumRamat. J Plant Nutr. (2014) 38:868–85. doi: 10.1080/01904167.2014.957396
93. Li K, Zeng M, Li Q, Zhou B. Identification of polyphenolic composition in the fruits of Rubus chingii Hu and its antioxidant and antiproliferative activity on human bladder cancer T24 cells. Food Measure. (2018) 13:51–60. doi: 10.1007/s11694-018-9918-x
94. Barros L, Dueñas M, Dias MI, Sousa MJ, Santos-Buelga C, Ferreira ICFR. Phenolic profiles of in vivo and in vitro grown Coriandrum sativum L. Food Chem. (2012) 132:841–8. doi: 10.1016/j.foodchem.2011.11.048
95. Cheung HY, Zhang QF. Enhanced analysis of triterpenes, flavonoids and phenolic compounds in Prunella vulgaris L. by capillary zone electrophoresis with the addition of running buffer modifiers. J Chromatogr A. (2008) 1213:231–8. doi: 10.1016/j.chroma.2008.10.033
96. Criste A, Urcan AC, Bunea A, Pripon Furtuna FR, Olah NK, Madden RH, et al. Phytochemical composition and biological activity of berries and leaves from four Romanian sea buckthorn (Hippophae rhamnoides L.) varieties. Molecules. (2020) 25:1170. doi: 10.3390/molecules25051170
97. Lu Y, Song W, Liang X, Wei D, Zhou X. Chemical fingerprint and quantitative analysis of cirsium setosum by LC. Chromatographia. (2009) 70:125–31. doi: 10.1365/s10337-009-1114-z
98. Jia Q, Zhang S, Zhang H, Yang X, Cui X, Su Z, et al. A comparative study on polyphenolic composition of berries from the tibetan plateau by UPLC-Q-orbitrap MS system. Chem Biodivers. (2020) 17:e2000033. doi: 10.1002/cbdv.202000033
99. Kong D, Li Y, Bai M, Deng Y, Liang G, Wu H. A comparative study of the dynamic accumulation of polyphenol components and the changes in their antioxidant activities in diploid and tetraploid Lonicera japonica. Plant Physiol Biochem. (2017) 112:87–96. doi: 10.1016/j.plaphy.2016.12.027
100. Ferreira SS, Silva AM, Nunes FM. Citrus reticulata Blanco peels as a source of antioxidant and anti-proliferative phenolic compounds. Ind Crops Prod. (2018) 111:141–8. doi: 10.1016/j.indcrop.2017.10.009
101. Fu Q, Wang H, Lan Y, Li S, Hashi Y, Chen S. High-throughput and sensitive screening of compounds with deoxyribonucleic acid-binding activity by a high-performance liquid chromatography-tandem mass spectrometry-fluorescence detection technique using palmatine as a fluorescence probe. J Chromatogr A. (2014) 1323:123–34. doi: 10.1016/j.chroma.2013.11.015
102. Gong J, Chu B, Gong L, Fang Z, Zhang X, Qiu S, et al. Comparison of Phenolic Compounds and the Antioxidant Activities of Fifteen Chrysanthemum morifolium Ramat cv. 'Hangbaiju' in China. Antioxidants (Basel). (2019) 8:325. doi: 10.3390/antiox8080325
103. Lee YH, Kim B, Kim S, Kim MS, Kim H, Hwang SR, et al. Characterization of metabolite profiles from the leaves of green perilla (Perilla frutescens) by ultra high performance liquid chromatography coupled with electrospray ionization quadrupole time-of-flight mass spectrometry and screening for their antioxidant properties. J Food Drug Anal. (2017) 25:776–88. doi: 10.1016/j.jfda.2016.09.003
104. Duan L, Zhang C, Zhao Y, Chang Y, Guo L. Comparison of bioactive phenolic compounds and antioxidant activities of different parts of taraxacum mongolicum. Molecules. (2020) 25:3260. doi: 10.3390/molecules25143260
105. Dong W, Ni Y, Kokot S. Differentiation of mint (Mentha haplocalyx Briq.) from different regions in China using gas and liquid chromatography. J Sep Sci. (2015) 38:402–9. doi: 10.1002/jssc.201401130
106. Pferschy-Wenzig EM, Ortmann S, Atanasov AG, Hellauer K, Hartler J, Kunert O, et al. Characterization of constituents with potential anti-inflammatory activity in chinese lonicera species by UHPLC-HRMS based metabolite profiling. Metabolites. (2022) 12:288. doi: 10.3390/metabo12040288
107. Liu R-y, Deng J, Lin X-L, Li Y-m, Lin Y, Xia B-h, et al. Metabolomics Reveals Distinct Metabolites between Lonicera japonica and Lonicera macranthoides Based on GC-MS. J Chem. (2020) 2020:6738571. doi: 10.1155/2020/6738571
108. Liu J, Feng L, Gu J, Wang R, Zhang M, Jiang J, et al. Simultaneous determination of ten characteristic antioxidant compounds for inhibiting cancer cell proliferation in Prunella vulgaris L. from different regions using HPLC-UV coupled with MS identification. Anal Methods. (2014) 6:3139–46. doi: 10.1039/c3ay41754a
109. Li J, Wang G, Qin Y, Zhang X, Wang HF, Liu HW, et al. Neuroprotective constituents from the aerial parts of Cannabis sativa L. subsp. sativa. RSC Adv. (2020) 10:32043–9. doi: 10.1039/D0RA04565A
110. Yao C-Y, Song Z-J, Ruan L-J, Yan B-X, Wu Q-H, He L-L, et al. A new methoxylated flavone from Lonicera hypoglauca and its chemotaxonomic significance. Biochem Systematics Ecology. (2021) 97:104279. doi: 10.1016/j.bse.2021.104279
111. Engels C, Schieber A, Gänzle MG. Sinapic acid derivatives in defatted Oriental mustard (Brassica juncea L.) seed meal extracts using UHPLC-DAD-ESI-MS n and identification of compounds with antibacterial activity. Eur Food Res Technol. (2012) 234:535–42. doi: 10.1007/s00217-012-1669-z
112. Su L, Wang Y-m, Zhong K-r, Tu G-z, Jiang Y-y, Liu B. Chemical constituents of mentha haplocalyx. Chem Nat Compd. (2019) 55:351–3. doi: 10.1007/s10600–019-02688–6
113. Lin TF, Qiu JN, Zhang S, Zhang Y, Zhang Y, Sun M, et al. Screening out the anti-insomnia components from Prunella vulgaris L. based on plasma pharmacochemistry combined with pharmacodynamic experiments and UPLC-MS/MS analysis. J Ethnopharmacol. (2021) 279:114373. doi: 10.1016/j.jep.2021.114373
114. Tang H, Zhao T, Sheng Y, Zheng T, Fu L, Zhang Y. Dendrobium officinale Kimura et Migo: A Review on Its Ethnopharmacology, Phytochemistry, Pharmacology, and Industrialization. Evid Based Complement Alternat Med. (2017) 2017:7436259. doi: 10.1155/2017/7436259
115. Lin L-Z, Harnly JM. Identification of the phenolic components of chrysanthemum flower (Chrysanthemum morifolium Ramat). Food Chem. (2010) 120:319–26. doi: 10.1016/j.foodchem.2009.09.083
116. Dugo P, Donato P, Cacciola F, Germano MP, Rapisarda A, Mondello L. Characterization of the polyphenolic fraction of Morus alba leaves extracts by HPLC coupled to a hybrid IT-TOF MS system. J Sep Sci. (2009) 32:3627–34. doi: 10.1002/jssc.200900348
117. Tian BM, Xie XM, Shen PP, Wu J, Wang J. Comparison of the antioxidant activities and the chemical compositions of the antioxidants of different polarity crude extracts from the fruits of Chaenomeles speciosa(Sweet) Nakai. JPC-J Planar Chromat Mod TLC. (2015) 28:443–7. doi: 10.1556/1006.2015.28.6.4
118. Gao L, Gou N, Yuan E, Ren J. Bioactivity-oriented purification of polyphenols from cinnamomum cassia presl. with anti-proliferation effects on colorectal cancer cells. Plant Foods Hum Nutr. (2020) 75:561–8. doi: 10.1007/s11130–020-00846–8
119. Han ZP, Liu RL, Cui HY, Zhang ZQ. Microwave-assisted extraction and lc/ms analysis of phenolic antioxidants in sweet apricot (Prunus Armeniaca L.) kernel skins. J Liq Chromatogr Relat Technol. (2013) 36:2182–95. doi: 10.1080/10826076.2012.717057
120. Yu L, Wu W, Pan Y, Wang W, Sun L, Liu Y, et al. Quality evaluation of different varieties of Zanthoxylum bungeanum Maxim. peels based on phenolic profiles, bioactivity, and HPLC fingerprint. J Food Sci. (2020) 85:1090–7. doi: 10.1111/1750–3841.15095
121. Meng J, Leung KS, Jiang Z, Dong X, Zhao Z, Xu L. Establishment of HPLC-DAD-MS fingerprint of fresh houttuynia cordata. Chem Pharm Bull (Tokyo). (2005) 53:1604–9. doi: 10.1248/cpb.53.1604
122. Liu X, Wang Y, Ge W, Cai G, Guo Y, Gong J. Spectrum-effect relationship between ultra-high-performance liquid chromatography fingerprints and antioxidant activities of Lophatherum gracile Brongn. Food Sci Nutr. (2022) 10:1592–601. doi: 10.1002/fsn3.2782
123. Dalar A, Konczak I. Cichorium intybus from Eastern Anatolia: Phenolic composition, antioxidant and enzyme inhibitory activities. Ind Crops Prod. (2014) 60:79–85. doi: 10.1016/j.indcrop.2014.05.043
124. Tang D, Li H, Li P, Wen X, Qian Z. Interaction of bioactive components caffeoylquinic acid derivatives in chinese medicines with bovine serum albumin. Chem Pharm Bull (Tokyo). (2008) 56:360–5. doi: 10.1248/cpb.56.360
125. Li Y, Guo S, Zhu Y, Yan H, Qian DW, Wang HQ, et al. Comparative analysis of twenty-five compounds in different parts of Astragalus membranaceus var. mongholicus and Astragalus membranaceus by UPLC-MS/MS. J Pharm Anal. (2019) 9:392–9. doi: 10.1016/j.jpha.2019.06.002
126. Mena P, Sánchez-Salcedo EM, Tassotti M, Martínez JJ, Hernández F, Del Rio D. Phytochemical evaluation of eight white (Morus alba L.) and black (Morus nigra L.) mulberry clones grown in Spain based on UHPLC-ESI-MSn metabolomic profiles. Food Res Int. (2016) 89:1116–22. doi: 10.1016/j.foodres.2016.06.012
127. Ahmed HM. Ethnomedicinal, phytochemical and pharmacological investigations of perilla frutescens (L.) britt. Molecules. (2018) 24:102. doi: 10.3390/molecules24010102
128. Liu F, Deng C, Cao W, Zeng G, Deng X, Zhou Y. Phytochemicals of Pogostemon Cablin (Blanco) Benth. aqueous extract: Their xanthine oxidase inhibitory activities. BioMed Pharmacother. (2017) 89:544–8. doi: 10.1016/j.biopha.2017.01.040
129. Innocenti M, Gallori S, Giaccherini C, Ieri F, Vincieri FF, Mulinacci N. Evaluation of the phenolic content in the aerial parts of different varieties of cichorium intybus L. J Agric Food Chem. (2005) 53:6497–502. doi: 10.1021/jf050541d
130. Zheng YF, Li DY, Sun J, Cheng JM, Chai C, Zhang L, et al. Comprehensive comparison of two color varieties of perillae folium using rapid resolution liquid chromatography coupled with quadruple-time-of-flight mass spectrometry (RRLC-Q/TOF-MS)-based metabolic profile and in vivo/in vitro anti-oxidative activity. J Agric Food Chem. (2020) 68:14684–97. doi: 10.1021/acs.jafc.0c05407
131. Hameed S, Imran A, Nisa Mu, Arshad MS, Saeed F, Arshad MU, et al. Characterization of extracted phenolics from black cumin (Nigella sativa linn), coriander seed (Coriandrum sativum L.), and fenugreek seed (Trigonella foenum-graecum). Int J Food Prop. (2019) 22:714–26. doi: 10.1080/10942912.2019.1599390
132. Kesavan K, Gnanasekaran J, Gurunagarajan S, Nayagam AAJ. Microscopic, physicochemical and phytochemical analysis of gardenia jasminoides (Ellis). Int J Pharm Pharm Sci. (2018) 10:97–102. doi: 10.22159/ijpps.2018v10i1.21665
133. Ye J, Su J, Chen K, Liu H, Yang X, He Y, et al. Comparative investigation on chemical constituents of flower bud, stem and leaf of Lonicera japonica Thunb. by HPLC-DAD-ESI-MS/MS n and GC-MS. J Anal Chem. (2014) 69:777–84. doi: 10.1134/s1061934814080036
134. Naowaratwattana W, De-Eknamkul W, Mejia EGD. Phenolic-containing organic extracts of mulberry (Morus alba L.) leaves inhibit hepG2 hepatoma cells through G2/M phase arrest, induction of apoptosis, and inhibition of topoisomerase IIa activity. J Med Food. (2010) 13:1045–56. doi: 10.1089/jmf.2010.1021
135. Dietz BM, Liu D, Hagos GK, Yao P, Schinkovitz A, Pro SM, et al. Angelica sinensis and its alkylphthalides induce the detoxification enzyme NAD(P)H: quinone oxidoreductase 1 by alkylating keap1. Chem Res Toxicol. (2008) 21:1939–48. doi: 10.1021/tx8001274
136. Yao F, Huang Y, Wang Y, He X. Anti-inflammatory diarylheptanoids and phenolics from the rhizomes of kencur (Kaempferia galanga L.). Ind Crops Prod. (2018) 125:454–61. doi: 10.1016/j.indcrop.2018.09.026
137. Wang X, Wei Y, Tian WY, Sakharkar MK, Liu Q, Yang X, et al. Characterization of Nine Compounds Isolated from the Acid Hydrolysate of Lonicera fulvotomentosa Hsu et S. C. Cheng and Evaluation of Their In Vitro Activity towards HIV Protease. Molecules. (2019) 24:4526. doi: 10.3390/molecules24244526
138. Chen W, Lu J, Zhang J, Wu J, Yu L, Qin L, et al. Traditional Uses, Phytochemistry, Pharmacology, and Quality Control of Dendrobium officinale Kimura et. Migo. Front Pharmacol. (2021) 12:726528. doi: 10.3389/fphar.2021.726528
139. Li J, Deng Z, He Y, Fan Y, Dong H, Chen R, et al. Differential specificities of polyphenol oxidase from lotus seeds (Nelumbo nucifera Gaertn.) toward stereoisomers, (–)-epicatechin and (+)-catechin: Insights from comparative molecular docking studies. LWT Food Sci Technol. (2021) 148:111728. doi: 10.1016/j.lwt.2021.111728
140. Tsai T, Lin L. Phenolic glycosides and pyrrolidine alkaloids from codonopsis tangshen. Chem Pharm Bull (Tokyo). (2008) 56:1546–50. doi: 10.1248/cpb.56.1546
141. Chen L, Yang YY, Zhou RR, Fang LZ, Zhao D, Cai P, et al. The extraction of phenolic acids and polysaccharides from Lilium lancifolium Thunb. using deep eutectic solvent. Anal Methods. (2021) 13:1226–31. doi: 10.1039/D0AY02352C
142. Xia B, Yan D, Bai Y, Xie J, Cao Y, Liao D, et al. Determination of phenolic acids in Prunella vulgaris L.: a safe and green extraction method using alcohol-based deep eutectic solvents. Anal Methods. (2015) 7:9354–64. doi: 10.1039/C5AY02035B
143. Yu H, Lin L, Zhang Z, Zhang H, Hu H. Targeting NF-kappaB pathway for the therapy of diseases: mechanism and clinical study. Signal Transduct Target Ther. (2020) 5:209. doi: 10.1038/s41392–020-00312–6
144. Mockenhaupt K, Gonsiewski A, Kordula T. RelB and neuroinflammation. Cells. (2021) 10:1609. doi: 10.3390/cells10071609
145. Wang W, Sun W, Jin L. Caffeic acid alleviates inflammatory response in rheumatoid arthritis fibroblast-like synoviocytes by inhibiting phosphorylation of IkappaB kinase alpha/beta and IkappaBalpha. Int Immunopharmacol. (2017) 48:61–6. doi: 10.1016/j.intimp.2017.04.025
146. Fu X, Lyu X, Liu H, Zhong D, Xu Z, He F, et al. Chlorogenic acid inhibits BAFF expression in collagen-induced arthritis and human synoviocyte MH7A cells by modulating the activation of the NF-kappaB signaling pathway. J Immunol Res. (2019) 2019:8042097. doi: 10.1155/2019/8042097
147. Gao R, Yang H, Jing S, Liu B, Wei M, He P, et al. Protective effect of chlorogenic acid on lipopolysaccharide-induced inflammatory response in dairy mammary epithelial cells. Microb Pathog. (2018) 124:178–82. doi: 10.1016/j.micpath.2018.07.030
148. Yu LM, Mao LQ, Wu CY, Ye W, Wang X. Chlorogenic acid improves intestinal barrier function by downregulating CD14 to inhibit the NF-κB signaling pathway. J Funct Foods. (2021) 85:104640. doi: 10.1016/j.jff.2021.104640
149. Hwang SJ, Kim YW, Park Y, Lee HJ, Kim KW. Anti-inflammatory effects of chlorogenic acid in lipopolysaccharide-stimulated RAW 264.7 cells. Inflammation Res. (2014) 63:81–90. doi: 10.1007/s00011–013-0674–4
150. Shi H, Dong L, Dang X, Liu Y, Jiang J, Wang Y, et al. Effect of chlorogenic acid on LPS-induced proinflammatory signaling in hepatic stellate cells. Inflammation Res. (2013) 62:581–7. doi: 10.1007/s00011–013-0610–7
151. Lou Y, Wang C, Zheng W, Tang Q, Chen Y, Zhang X, et al. Salvianolic acid B inhibits IL-1beta-induced inflammatory cytokine production in human osteoarthritis chondrocytes and has a protective effect in a mouse osteoarthritis model. Int Immunopharmacol. (2017) 46:31–7. doi: 10.1016/j.intimp.2017.02.021
152. Yang Y, Pei K, Zhang Q, Wang D, Feng H, Du Z, et al. Salvianolic acid B ameliorates atherosclerosis via inhibiting YAP/TAZ/JNK signaling pathway in endothelial cells and pericytes. Biochim Biophys Acta Mol Cell Biol Lipids. (2020) 1865:158779. doi: 10.1016/j.bbalip.2020.158779
153. Lee J, Jung E, Kim Y, Lee J, Park J, Hong S, et al. Rosmarinic acid as a downstream inhibitor of IKK-beta in TNF-alpha-induced upregulation of CCL11 and CCR3. Br J Pharmacol. (2006) 148:366–75. doi: 10.1038/sj.bjp.0706728
154. Fan YT, Yin GJ, Xiao WQ, Qiu L, Yu G, Hu YL, et al. Rosmarinic acid attenuates sodium taurocholate-induced acute pancreatitis in rats by inhibiting nuclear factor-kappaB activation. Am J Chin Med. (2015) 43:1117–35. doi: 10.1142/S0192415X15500640
155. Mir SM, Ravuri HG, Pradhan RK, Narra S, Kumar JM, Kuncha M, et al. Ferulic acid protects lipopolysaccharide-induced acute kidney injury by suppressing inflammatory events and upregulating antioxidant defenses in Balb/c mice. BioMed Pharmacother. (2018) 100:304–15. doi: 10.1016/j.biopha.2018.01.169
156. Cao L, Li Z, Yang Z, Wang M, Zhang W, Ren Y, et al. Ferulic acid positively modulates the inflammatory response to septic liver injury through the GSK-3beta/NF-kappaB/CREB pathway. Life Sci. (2021) 277:119584. doi: 10.1016/j.lfs.2021.119584
157. Zhang HF, Wang YL, Gao C, Gu YT, Huang J, Wang JH, et al. Salvianolic acid A attenuates kidney injury and inflammation by inhibiting NF-kappaB and p38 MAPK signaling pathways in 5/6 nephrectomized rats. Acta Pharmacol Sin. (2018) 39:1855–64. doi: 10.1038/s41401–018-0026–6
158. Song J, Zhang W, Wang J, Yang H, Zhao X, Zhou Q, et al. Activation of Nrf2 signaling by salvianolic acid C attenuates NF−kappaB mediated inflammatory response both in vivo and in vitro. Int Immunopharmacol. (2018) 63:299–310. doi: 10.1016/j.intimp.2018.08.004
159. Ziadlou R, Barbero A, Martin I, Wang X, Qin L, Alini M, et al. Anti-inflammatory and chondroprotective effects of vanillic acid and epimedin C in human osteoarthritic chondrocytes. Biomolecules. (2020) 10:932. doi: 10.3390/biom10060932
160. Ran X, Chao S, Jun-Gang Z, Yun H, Kuan-Bing C, Wen-Jun S. Protective effect of veratric acid on lipopolysaccharide-induced acute lung injury in mice. Eur J Pharmacol. (2014) 740:227–32. doi: 10.1016/j.ejphar.2014.07.006
161. Xi Z, Hu X, Chen X, Yang Y, Ren J, Wang B, et al. Protocatechuic acid exerts protective effects via suppression of the P38/JNK- NF-kappaB signalling pathway in an experimental mouse model of intracerebral haemorrhage. Eur J Pharmacol. (2019) 854:128–38. doi: 10.1016/j.ejphar.2019.03.008
162. Lee G, Na HJ, Namkoong S, Jeong Kwon H, Han S, Ha KS, et al. 4-O-methylgallic acid down-regulates endothelial adhesion molecule expression by inhibiting NF-kappaB-DNA-binding activity. Eur J Pharmacol. (2006) 551:143–51. doi: 10.1016/j.ejphar.2006.08.061
163. Kim SR, Jung YR, Kim DH, An HJ, Kim MK, Kim ND, et al. Caffeic acid regulates LPS-induced NF-kappaB activation through NIK/IKK and c-Src/ERK signaling pathways in endothelial cells. Arch Pharm Res. (2014) 37:539–47. doi: 10.1007/s12272–013-0211–6
164. Jiang Y, Nan H, Shi N, Hao W, Dong J, Chen H. Chlorogenic acid inhibits proliferation in human hepatoma cells by suppressing noncanonical NF-kappaB signaling pathway and triggering mitochondrial apoptosis. Mol Biol Rep. (2021) 48:2351–64. doi: 10.1007/s11033–021-06267–3
165. Dong X, Zhang Q, Zeng F, Cai M, Ding D. The protective effect of gentisic acid on rheumatoid arthritis via the RAF/ERK signaling pathway. J Orthop Surg Res. (2022) 17:109. doi: 10.1186/s13018–022-03006–7
166. Yang WS, Jeong D, Yi YS, Park JG, Seo H, Moh SH, et al. IRAK1/4-targeted anti-inflammatory action of caffeic acid. Mediators Inflamm. (2013) 2013:518183. doi: 10.1155/2013/518183
167. Pang C, Shi L, Sheng Y, Zheng Z, Wei H, Wang Z, et al. Caffeic acid attenuated acetaminophen-induced hepatotoxicity by inhibiting ERK1/2-mediated early growth response-1 transcriptional activation. Chem Biol Interact. (2016) 260:186–95. doi: 10.1016/j.cbi.2016.10.009
168. Lampiasi N, Montana G. An in vitro inflammation model to study the Nrf2 and NF-kappaB crosstalk in presence of ferulic acid as modulator. Immunobiology. (2018) 223:349–55. doi: 10.1016/j.imbio.2017.10.046
169. Liu Q, Zhu C-l, Li H-r, Xie J, Guo Y, Li P, et al. Salvianolic acid A protects against lipopolysaccharide-induced acute lung injury by inhibiting neutrophil NETosis. Oxid Med Cell Longevity. (2022) 2022:1–15. doi: 10.1155/2022/7411824
170. Gao W, Wang C, Yu L, Sheng T, Wu Z, Wang X, et al. Chlorogenic acid attenuates dextran sodium sulfate-induced ulcerative colitis in mice through MAPK/ERK/JNK pathway. BioMed Res Int. (2019) 2019:6769789. doi: 10.1155/2019/6769789
171. Yin P, Zhang Z, Li J, Shi Y, Jin N, Zou W, et al. Ferulic acid inhibits bovine endometrial epithelial cells against LPS-induced inflammation via suppressing NK-kappaB and MAPK pathway. Res Vet Sci. (2019) 126:164–9. doi: 10.1016/j.rvsc.2019.08.018
172. Wu Y, Wang Z, Lin Z, Fu X, Zhan J, Yu K. Salvianolic acid A has anti-osteoarthritis effect in vitro and in vivo. Front Pharmacol. (2020) 11:682. doi: 10.3389/fphar.2020.00682
173. Liu M, Wang L, Huang B, Lu Q, Liu R. 3,4-Dihydroxyphenylacetic acid ameliorates gut barrier dysfunction via regulation of MAPK-MLCK pathway in type 2 diabetes mice. Life Sci. (2022) 305:120742. doi: 10.1016/j.lfs.2022.120742
174. Park CH, Noh JS, Tanaka T, Roh SS, Lee JC, Yokozawa T. Polyphenol isolated from Corni Fructus, 7-O-galloyl-D-sedoheptulose, modulates advanced glycation endproduct-related pathway in type 2 diabetic db/db mice. Arch Pharm Res. (2015) 38:1270–80. doi: 10.1007/s12272–014-0457–7
175. Wang Y, Wang Y, Li J, Hua L, Han B, Zhang Y, et al. Effects of caffeic acid on learning deficits in a model of Alzheimer's disease. Int J Mol Med. (2016) 38:869–75. doi: 10.3892/ijmm.2016.2683
176. Liu M, Fang G, Yin S, Zhao X, Zhang C, Li J, et al. Caffeic acid prevented LPS-induced injury of primary bovine mammary epithelial cells through inhibiting NF-kappaB and MAPK activation. Mediators Inflamm. (2019) 2019:1897820. doi: 10.1155/2019/1897820
177. Liu Q, Shi X, Tang L, Xu W, Jiang S, Ding W, et al. Salvianolic acid B attenuates experimental pulmonary inflammation by protecting endothelial cells against oxidative stress injury. Eur J Pharmacol. (2018) 840:9–19. doi: 10.1016/j.ejphar.2018.09.030
178. Jeong HJ, Nam SY, Kim HY, Jin MH, Kim MH, Roh SS, et al. Anti-allergic inflammatory effect of vanillic acid through regulating thymic stromal lymphopoietin secretion from activated mast cells. Nat Prod Res. (2018) 32:2945–9. doi: 10.1080/14786419.2017.1389938
179. Chae HS, Kang OH, Choi JG, Oh YC, Lee YS, Brice OO, et al. Methyl gallate inhibits the production of interleukin-6 and nitric oxide via down-regulation of extracellular-signal regulated protein kinase in RAW 264.7 cells. Am J Chin Med. (2010) 38:973–83. doi: 10.1142/S0192415X10008391
180. Chen CP, Lin YC, Peng YH, Chen HM, Lin JT, Kao SH. Rosmarinic acid attenuates the lipopolysaccharide-provoked inflammatory response of vascular smooth muscle cell via inhibition of MAPK/NF-kappaB cascade. Pharm (Basel). (2022) 15:437. doi: 10.3390/ph15040437
181. Doss HM, Dey C, Sudandiradoss C, Rasool MK. Targeting inflammatory mediators with ferulic acid, a dietary polyphenol, for the suppression of monosodium urate crystal-induced inflammation in rats. Life Sci. (2016) 148:201–10. doi: 10.1016/j.lfs.2016.02.004
182. Cao X, Xia Y, Zeng M, Wang W, He Y, Liu J. Caffeic acid inhibits the formation of advanced glycation end products (AGEs) and mitigates the AGEs-induced oxidative stress and inflammation reaction in human umbilical vein endothelial cells (HUVECs). Chem Biodivers. (2019) 16:e1900174. doi: 10.1002/cbdv.201900174
183. Zhou MW, Jiang RH, Kim KD, Lee JH, Kim CD, Yin WT, et al. Rosmarinic acid inhibits poly(I:C)-induced inflammatory reaction of epidermal keratinocytes. Life Sci. (2016) 155:189–94. doi: 10.1016/j.lfs.2016.05.023
184. Yao Y, Li R, Liu D, Long L, He N. Rosmarinic acid alleviates acetaminophen-induced hepatotoxicity by targeting Nrf2 and NEK7-NLRP3 signaling pathway. Ecotoxicol Environ Saf. (2022) 241:113773. doi: 10.1016/j.ecoenv.2022.113773
185. Wei Y, Chen J, Hu Y, Lu W, Zhang X, Wang R, et al. Rosmarinic acid mitigates lipopolysaccharide-induced neuroinflammatory responses through the inhibition of TLR4 and CD14 expression and NF-kappaB and NLRP3 inflammasome activation. Inflammation. (2018) 41:732–40. doi: 10.1007/s10753–017-0728–9
186. Ma Z, Huang Z, Zhang L, Li X, Xu B, Xiao Y, et al. Vanillic acid reduces pain-related behavior in knee osteoarthritis rats through the inhibition of NLRP3 inflammasome-related synovitis. Front Pharmacol. (2020) 11:599022. doi: 10.3389/fphar.2020.599022
187. Zeng J, Wan X, Liu T, Xiong Y, Xiang G, Peng Y, et al. Chlorogenic acid ameliorates Klebsiella pneumoniae-induced pneumonia in immunosuppressed mice via inhibiting the activation of NLRP3 inflammasomes. Food Funct. (2021) 12:9466–75. doi: 10.1039/D0FO03185B
188. Huang X, Liu Y, Shen H, Fu T, Guo Y, Qiu S. Chlorogenic acid attenuates inflammation in LPS-induced Human gingival fibroblasts via CysLT1R/Nrf2/NLRP3 signaling. Int Immunopharmacol. (2022) 107:108706. doi: 10.1016/j.intimp.2022.108706
189. Liu P, Wang W, Li Q, Hu X, Xu B, Wu C, et al. Methyl gallate improves hyperuricemia nephropathy mice through inhibiting NLRP3 pathway. Front Pharmacol. (2021) 12:759040. doi: 10.3389/fphar.2021.759040
190. Tang Y, Wa Q, Peng L, Zheng Y, Chen J, Chen X, et al. Salvianolic acid B suppresses ER stress-induced NLRP3 inflammasome and pyroptosis via the AMPK/foxO4 and syndecan-4/rac1 signaling pathways in human endothelial progenitor cells. Oxid Med Cell Longev. (2022) 2022:8332825. doi: 10.1155/2022/8332825
191. Wang Q, Lin B, Li Z, Su J, Feng Y. Cichoric acid ameliorates monosodium urate-induced inflammatory response by reducing NLRP3 inflammasome activation via inhibition of NF-kB signaling pathway. Evid Based Complement Alternat Med. (2021) 2021:8868527. doi: 10.1155/2021/8868527
192. Shen J, Wang G, Zuo J. Caffeic acid inhibits HCV replication via induction of IFNalpha antiviral response through p62-mediated Keap1/Nrf2 signaling pathway. Antiviral Res. (2018) 154:166–73. doi: 10.1016/j.antiviral.2018.04.008
193. Wan F, Cai X, Wang M, Chen L, Zhong R, Liu L, et al. Chlorogenic acid supplementation alleviates dextran sulfate sodium (DSS)-induced colitis via inhibiting inflammatory responses and oxidative stress, improving gut barrier integrity and Nrf-2/HO-1 pathway. J Funct Foods. (2021) 87:104808. doi: 10.1016/j.jff.2021.104808
194. Singla E, Puri G, Dharwal V, Naura AS. Gallic acid ameliorates COPD-associated exacerbation in mice. Mol Cell Biochem. (2021) 476:293–302. doi: 10.1007/s11010–020-03905–5
195. Albarakati AJA. Protocatechuic acid counteracts oxidative stress and inflammation in carrageenan-induced paw edema in mice. Environ Sci pollut Res Int. (2022) 29:56393–402. doi: 10.1007/s11356–022-19688–9
196. Xu C, Hou B, He P, Ma P, Yang X, Yang X, et al. Neuroprotective Effect of Salvianolic Acid A against Diabetic Peripheral Neuropathy through Modulation of Nrf2. Oxid Med Cell Longev. (2020) 2020:6431459. doi: 10.1155/2020/6431459
197. Liu Y, Kurita A, Nakashima S, Zhu B, Munemasa S, Nakamura T, et al. 3,4-Dihydroxyphenylacetic acid is a potential aldehyde dehydrogenase inducer in murine hepatoma Hepa1c1c7 cells. Biosci Biotechnol Biochem. (2017) 81:1978–83. doi: 10.1080/09168451.2017.1361809
198. Liu M, Zhang C, Xu X, Zhao X, Han Z, Liu D, et al. Ferulic acid inhibits LPS-induced apoptosis in bovine mammary epithelial cells by regulating the NF-kappaB and Nrf2 signalling pathways to restore mitochondrial dynamics and ROS generation. Vet Res. (2021) 52:104. doi: 10.1186/s13567–021-00973–3
199. Zheng Y, Li L, Chen B, Fang Y, Lin W, Zhang T, et al. Chlorogenic acid exerts neuroprotective effect against hypoxia-ischemia brain injury in neonatal rats by activating Sirt1 to regulate the Nrf2-NF-kappaB signaling pathway. Cell Commun Signal. (2022) 20:84. doi: 10.1186/s12964–022-00860–0
200. Gao F, Fu K, Li H, Feng Y, Tian W, Cao R. Chlorogenic acid ameliorates mice clinical endometritis by activating Keap1/Nrf2 and inhibiting NFkappaB signalling pathway. J Pharm Pharmacol. (2021) 73:785–95. doi: 10.1093/jpp/rgab020
201. Bao L, Li J, Zha D, Zhang L, Gao P, Yao T, et al. Chlorogenic acid prevents diabetic nephropathy by inhibiting oxidative stress and inflammation through modulation of the Nrf2/HO-1 and NF-kB pathways. Int Immunopharmacol. (2018) 54:245–53. doi: 10.1016/j.intimp.2017.11.021
202. Balka KR, De Nardo D. Understanding early TLR signaling through the Myddosome. J Leukoc Biol. (2019) 105:339–51. doi: 10.1002/JLB.MR0318-096R
203. Wang Q, Ren X, Wu J, Li H, Yang L, Zhang Y, et al. Protocatechuic acid protects mice from influenza A virus infection. Eur J Clin Microbiol Infect Dis. (2022) 41:589–96. doi: 10.1007/s10096-022-04401-y
204. Abdelmageed ME, Nader MA, Zaghloul MS. Targeting HMGB1/TLR4/NF-kappaB signaling pathway by protocatechuic acid protects against l-arginine induced acute pancreatitis and multiple organs injury in rats. Eur J Pharmacol. (2021) 906:174279. doi: 10.1016/j.ejphar.2021.174279
205. Ma Z, Lu Y, Yang F, Li S, He X, Gao Y, et al. Rosmarinic acid exerts a neuroprotective effect on spinal cord injury by suppressing oxidative stress and inflammation via modulating the Nrf2/HO-1 and TLR4/NF-kappaB pathways. Toxicol Appl Pharmacol. (2020) 397:115014. doi: 10.1016/j.taap.2020.115014
206. Ham JR, Lee HI, Choi RY, Sim MO, Seo KI, Lee MK. Anti-steatotic and anti-inflammatory roles of syringic acid in high-fat diet-induced obese mice. Food Funct. (2016) 7:689–97. doi: 10.1039/C5FO01329A
207. Jiang K, Ma X, Guo S, Zhang T, Zhao G, Wu H, et al. Anti-inflammatory effects of rosmarinic acid in lipopolysaccharide-induced mastitis in mice. Inflammation. (2018) 41:437–48. doi: 10.1007/s10753–017-0700–8
208. Li L, Kan L, Xie Q. Inhalation of salvianolic acid B prevents fine particulate matter-induced acute airway inflammation and oxidative stress by downregulating the LTR4/myD88/NLRP3 pathway. Oxid Med Cell Longev. (2021) 2022:5044356. doi: 10.1155/2022/5044356
209. Chen J, Yu B, Chen D, Huang Z, Mao X, Zheng P, et al. Chlorogenic acid improves intestinal barrier functions by suppressing mucosa inflammation and improving antioxidant capacity in weaned pigs. J Nutr Biochem. (2018) 59:84–92. doi: 10.1016/j.jnutbio.2018.06.005
210. Yuan Y, Gong X, Zhang L, Jiang R, Yang J, Wang B, et al. Chlorogenic acid ameliorated concanavalin A-induced hepatitis by suppression of Toll-like receptor 4 signaling in mice. Int Immunopharmacol. (2017) 44:97–104. doi: 10.1016/j.intimp.2017.01.017
211. Buko V, Zavodnik I, Budryn G, Zaklos-Szyda M, Belonovskaya E, Kirko S, et al. Chlorogenic Acid Protects against Advanced Alcoholic Steatohepatitis in Rats via Modulation of Redox Homeostasis, Inflammation, and Lipogenesis. Nutrients. (2021) 13:4155. doi: 10.3390/nu13114155
212. Xu W, Luo T, Chai J, Jing P, Xiong L. Chlorogenic acid alleviates the inflammatory stress of LPS-induced BV2 cell via interacting with TLR4-mediated downstream pathway. Comput Math Methods Med. (2022) 2022:6282167. doi: 10.1155/2022/6282167
213. Park CM, Yoon HS. Chlorogenic acid as a positive regulator in LPS-PG-induced inflammation via TLR4/myD88-mediated NF-kappaB and PI3K/MAPK signaling cascades in human gingival fibroblasts. Mediators Inflammation. (2022) 2022:2127642. doi: 10.1155/2022/2127642
214. Hu X, Wang M, Pan Y, Xie Y, Han J, Zhang X, et al. Anti-inflammatory effect of astragalin and chlorogenic acid on escherichia coli-induced inflammation of sheep endometrial epithelium cells. Front Vet Sci. (2020) 7:201. doi: 10.3389/fvets.2020.00201
215. Guo YJ, Luo T, Wu F, Mei YW, Peng J, Liu H, et al. Involvement of TLR2 and TLR9 in the anti-inflammatory effects of chlorogenic acid in HSV-1-infected microglia. Life Sci. (2015) 127:12–8. doi: 10.1016/j.lfs.2015.01.036
216. Luo J, He W, Li X, Ji X, Liu J. Anti-acne vulgaris effects of chlorogenic acid by anti-inflammatory activity and lipogenesis inhibition. Exp Dermatol. (2021) 30:865–71. doi: 10.1111/exd.14277
217. Correa LB, Seito LN, Manchope MF, Verri WA Jr., Cunha TM, Henriques MG, et al. Methyl gallate attenuates inflammation induced by Toll-like receptor ligands by inhibiting MAPK and NF-Kappab signaling pathways. Inflammation Res. (2020) 69:1257–70. doi: 10.1007/s00011–020-01407–0
218. Ye Z, Liu Z, Henderson A, Lee K, Hostetter J, Wannemuehler M, et al. Increased CYP4B1 mRNA is associated with the inhibition of dextran sulfate sodium-induced colitis by caffeic acid in mice. Exp Biol Med (Maywood). (2009) 234:605–16. doi: 10.3181/0901-RM-1
219. Lo HY, Li CC, Cheng HM, Liu IC, Ho TY, Hsiang CY. Ferulic acid altered IL-17A/IL-17RA interaction and protected against imiquimod-induced psoriasis-like skin injury in mice. Food Chem Toxicol. (2019) 129:365–75. doi: 10.1016/j.fct.2019.04.060
220. Zhang M, Li N, Cai R, Gu J, Xie F, Wei H, et al. Rosmarinic acid protects mice from imiquimod induced psoriasis-like skin lesions by inhibiting the IL-23/Th17 axis via regulating Jak2/Stat3 signaling pathway. Phytother Res. (2021) 35:4526–37. doi: 10.1002/ptr.7155
221. Tian B, Geng Y, Wang P, Cai M, Neng J, Hu J, et al. Ferulic acid improves intestinal barrier function through altering gut microbiota composition in high-fat diet-induced mice. Eur J Nutr. (2022) 61:3767–83. doi: 10.1007/s00394–022-02927–7
222. Ye X, Liu Y, Hu J, Gao Y, Ma Y, Wen D. Chlorogenic acid-induced gut microbiota improves metabolic endotoxemia. Front Endocrinol. (2021) 12:762691. doi: 10.3389/fendo.2021.762691
223. Tai WC, Yao CC, Tsai YC. Changes in gut microbiota of patients with inflammatory bowel disease receiving biologic therapy. J Crohn's Colitis. (2024) 18:i2153. doi: 10.1093/ecco-jcc/jjad212.1349
224. Cui X, Zhang S, Jiang S, Gou Z, Wang Y. Dietary protocatechuic acid ameliorates ileal mucosal barrier injury and inflammatory response and improves intestinal microbiota composition in Yellow chickens challenged with Salmonella typhimurium. Poultry Sci. (2023) 102:102496. doi: 10.1016/j.psj.2023.102496
225. Luo Q, Gong P, Shi R, Chen W, Wang C, Zhang C, et al. Syringic acid alleviates dextran sulfate sodium-induced colitis in mice by modulating gut microbiota. J Agric Food Chem. (2023) 71:8458–70. doi: 10.1021/acs.jafc.3c02441
226. Wan F, Zhong R, Wang M, Zhou Y, Chen Y, Yi B, et al. Caffeic acid supplement alleviates colonic inflammation and oxidative stress potentially through improved gut microbiota community in mice. Front Microbiol. (2021) 12:784211. doi: 10.3389/fmicb.2021.784211
227. Hu R, Wu S, Li B, Tan J, Yan J, Wang Y, et al. Dietary ferulic acid and vanillic acid on inflammation, gut barrier function and growth performance in lipopolysaccharide-challenged piglets. Anim Nutr. (2022) 8:144–52. doi: 10.1016/j.aninu.2021.06.009
228. Xie MG, Fei YQ, Wang Y, Wang WY, Wang Z. Chlorogenic acid alleviates colon mucosal damage induced by a high-fat diet via gut microflora adjustment to increase short-chain fatty acid accumulation in rats. Oxid Med Cell Longev. (2021) 2021:3456542. doi: 10.1155/2021/3456542
229. Xu S, Zuo A, Guo Z, Wan C. Ethyl caffeate ameliorates collagen-induced arthritis by suppressing th1 immune response. . J Immunol Res. (2017) 2017:7416792. doi: 10.1155/2017/7416792
230. Jeon WJ, Lee JH, Lee JE, Son SE, Okajima F, Chung KW, et al. Salvianolic acid A suppresses DNCB-induced atopic dermatitis-like symptoms in BALB/c mice. Evid Based Complement Alternat Med. (2021) 2021:7902592. doi: 10.1155/2021/7902592
231. Wang B, Sun J, Shi Y, Le G. Salvianolic acid B inhibits high-fat diet-induced inflammation by activating the Nrf2 pathway. J Food Sci. (2017) 82:1953–60. doi: 10.1111/1750–3841.13808
232. Jang AH, Kim TH, Kim GD, Kim JE, Kim HJ, Kim SS, et al. Rosmarinic acid attenuates 2,4-dinitrofluorobenzene-induced atopic dermatitis in NC/Nga mice. Int Immunopharmacol. (2011) 11:1271–7. doi: 10.1016/j.intimp.2011.04.007
Keywords: medicinal and edible homology, plant sources, structure and distribution, phenolic acids, anti-inflammatory, inflammatory diseases, mechanism, pathway
Citation: Xie J, Xiong S, Li Y, Xia B, Li M, Zhang Z, Shi Z, Peng Q, Li C, Lin L and Liao D (2024) Phenolic acids from medicinal and edible homologous plants: a potential anti-inflammatory agent for inflammatory diseases. Front. Immunol. 15:1345002. doi: 10.3389/fimmu.2024.1345002
Received: 27 November 2023; Accepted: 10 June 2024;
Published: 21 June 2024.
Edited by:
Mohamed El-Shazly, Ain Shams University, EgyptReviewed by:
Kun-Ping Li, Guangdong Pharmaceutical University, ChinaTao Yi, Hong Kong Baptist University, Hong Kong SAR, China
Copyright © 2024 Xie, Xiong, Li, Xia, Li, Zhang, Shi, Peng, Li, Lin and Liao. This is an open-access article distributed under the terms of the Creative Commons Attribution License (CC BY). The use, distribution or reproduction in other forums is permitted, provided the original author(s) and the copyright owner(s) are credited and that the original publication in this journal is cited, in accordance with accepted academic practice. No use, distribution or reproduction is permitted which does not comply with these terms.
*Correspondence: Limei Lin, bGl6YXNtaWxlQDE2My5jb20=; Duanfang Liao, ZGZsaWFvQGhudWNtLmVkdS5jbg==
†These authors have contributed equally to this work and share first authorship
‡These authors have contributed equally to this work and share last authorship