- 1Department of Traditional Chinese Medicine, Jinan Maternity and Child Care Hospital Affiliated to Shandong First Medical University, Jinan, China
- 2Institute of Chinese Materia Medica, China Academy of Chinese Medical Sciences, Beijing, China
- 3Department of Nursing, The First Affiliated Hospital of Shandong First Medical University (Shandong Provincial Qianfoshan Hospital), Jinan, China
Lung cancer (LC) produces some of the most malignant tumors in the world, with high morbidity and mortality. Tumor immune microenvironment (TIME), a component of the tumor microenvironment (TME), are critical in tumor development, immune escape, and drug resistance. The TIME is composed of various immune cells, immune cytokines, etc, which are important biological characteristics and determinants of tumor progression and outcomes. In this paper, we reviewed the recently published literature and discussed the potential uses of natural products in regulating TIME. We observed that a total of 37 natural compounds have been reported to exert anti-cancer effects by targeting the TIME. In different classes of natural products, terpenoids are the most frequently mentioned compounds. TAMs are one of the most investigated immune cells about therapies with natural products in TIME, with 9 natural products acting through it. 17 natural products exhibit anti-cancer properties in LC by modulating PD-1 and PD-L1 protein activity. These natural products have been extensively evaluated in animal and cellular LC models, but their clinical trials in LC patients are lacking. Based on the current review, we have revealed that the mechanisms of LC can be treated with natural products through TIME intervention, resulting in a new perspective and potential therapeutic drugs.
1 Introduction
Cancer poses a significant clinical, social, and economic burden, and lung cancer(LC) is one of the leading culprits. LC is a highly prevalent disease and remains the major cause of global cancer-associated mortality. In 2020, LC is predicted that there were around 2.21 million newly diagnosed instances of cancer, accounting for approximately 11.4% of all reported cancer cases. Additionally, there were approximately 1.80 million fatalities attributed to cancer, representing approximately 18% of all reported cancer-related deaths (1). LC can be classified into two main histological categories: non-small-cell lung carcinoma (NSCLC) and small-cell lung carcinoma (SCLC). More than 80 to 85% of LC cases are NSCLC, which is more common than SCLC (2). Treating LC has always been a tricky and complex process. Managing LC includes surgery, chemotherapy, radiation therapy, biological therapies, immunotherapies, and targeted therapies. Since the turn of the century, the cancer treatment landscape has dramatically changed, leading to substantial improvements in outcomes for patients (3). Immunotherapy has shown promising efficacy in regenerating and repairing tumor-specific immune responses by targeting both the activation and recovery of immune cell function. Immunotherapy has thus brought new treatment options for cancer treatment, including LC. With the rapid advancement of tumor immunotherapy, LC treatment has gradually transitioned into a new era of immunotherapy.
In the process of transforming normal cells into tumor cells, the internal and external environment of tumor cells is closely related to the course of the disease. This highly structured and closely related complex ecosystem is called the tumor microenvironment (TME). TME includes both cancerous and non-cancerous cells. Non-cancerous cells (such as immune cells, endothelial cells, fat cells, etc.) and extracellular matrix in TME have extremely important effects on the biological behavior of cancerous cells. Since immune cells represent a large fraction of the TME, they play a key role in mediating pro- and antitumor immune responses (4). The tumor immune microenvironment (TIME) is often mentioned and studied independently. The TIME includes tumor cells, immune cells, immune cytokines, etc. The TME contains different types, in which TIME is like a battlefield between the tumor and immunity, mostly caused by the interaction of diverse tumor cells with immune cells. The characteristics of the TIME are intrinsically related to the efficacy of immunotherapy. Identifying targets that modulate immune metabolism helps to maximize the efficacy of cancer therapies (5). Immunotherapy alters the TIME and restores the ability of anti-tumor immune cells to kill tumors (6). Tumors can evade immune destruction by constructing an immunosuppressive microenvironment.
Natural products with novel structures, multi-targets, multi-pathways, few toxic and side effects, and diversified biological activities have numerous kinds of impacts on the treatment of diseases and are some of the most important sources of new drug research and development. Natural compounds and their derivatives show strong anti-tumor activity in many types of cancer, and their effects are being confirmed through various experimental studies, including on lung, breast, colon, and prostate cancer (7). Natural products have a significant impact on cancer treatment by modulating TME and different signaling pathways (8). The investigation of novel natural-product-derived compounds with the potential to modulate tumor immunotherapy has emerged as a prominent and globally recognized area of research (9). Many natural products have immune-modulating properties that could revolutionize the LC landscape. This work provides a comprehensive review of the processes by which natural compounds are regulated during the TIME of LC. Natural compounds will undoubtedly be essential to the control of TIME in LC treatment. This investigation provides a theoretical basis for natural compounds that regulate the TIME in LC treatments and presents innovative research ideas for exploring and developing new anticancer drugs.
2 Overview of tumor immune microenvironment and lung cancer
The interaction and co-evolution between tumor cells and their microenvironments causes LC to occur and develop. The crucial involvement of the TME in the onset and advancement of LC has been widely recognized during the past decade (10). The TIME is the “battlefield” where tumour cells and immune cells interact. Certain immune cells can detect and eliminate tumor cells, whereas tumor cells can also influence their microenvironment by producing chemicals that signal other cells and cause immunological tolerance. It is well established that neighboring immune cells and interactions across cell types influence the biological phenotypes and behaviors of malignant T cells. This complex tumor ecosystem is collectively known as the TIME (11). The immune system and tumor cells have complex interactions throughout the initiation and development of cancer (12). Different immune cells possess distinct surface markers, secretory factors, and corresponding immune functions. Immune cells are the primary players in TIME, affecting and controlling the occurrence and development of tumors in a complex and precise manner. Based on the various immune cell types identified in the TIME, this study examines the function of LC-associated immune cell types.
2.1 Helper T cells
Helper T (Th) cells are the largest subpopulation of T cells and important components of the TIME. The cluster of differentiation (CD) is a nomenclature system that identifies and classifies cell surface molecules present in leukocytes. CD is a common cellular marker for immune antigen recognition, with CD4 (markers for Th cells) and CD8 (markers for cytotoxic T cells) being the most common. Th cells and regulatory T cells (Tregs) are commonly known as CD4 T cells due to the presence of CD4 molecules on their cell surfaces. The activation of quiescent naive CD4+ T cells (Th0) is assisted by their identification of antigen-major histocompatibility complex molecules and appropriate co-stimulation (13). Naive CD4+ T cells differentiate into one of several Th cell subsets, depending on specific antigens and cytokines in the immune microenvironment. Th cells play a pivotal role in regulating adaptive immune responses at epithelial sites by the secretion of a diverse range of cytokines, which serve to attract and modulate the function of various other immune cells (14). Different subtypes of Th cells produce different cytokines and perform different immune functions. In 1986, Mosmann discovered the mysterious veil of the Th1 and Th2 subsets (15), and since then, other cell subpopulations have also been discovered, such as Th3, Th9, Th17, Th22, Tfh, etc. In the TIME of LC, Th1, Th2, Th9, and Th17 tend to be of higher concern.
Th1 cells are primarily responsible for regulating cellular immune responses; secrete immune cytokines; and promote the activation and proliferation of macrophages, cytotoxic T cells (CTLs), and natural killer (NK) cells. Th1 responses are overrepresented in LC (16). When tumor necrosis factor (TNF)-α and interferon (IFN)-γ levels are high, IL-2 levels are low, and these are Th1-biased cytokines in LC. The higher expression of TNF-α is associated with a low risk of LC progression (17). IFN-γ can cause tumor cells to evade immune surveillance in LC (18). IL-2 is considered an effective treatment option for activating the anti-tumor immune response. The validation of the therapeutic efficacy of increasing the activity of NK cells and T cells through IL-2 signaling has been confirmed as a novel approach for SCLC (19). In fact, the role of Th1 in lung cancer is mainly summarized in three aspects: the secretion of regulatory cytokines, the regulation of the immune response of other immune cells and the homeostasis of Th1/Th2 cells.
Th2 cells are associated with pro-tumor activity, mediating this function by producing various cytokines. IL-6, IL-10, and IL-4 are considered the main Th2 immune cytokines, mainly encouraging tumor growth by hindering the host’s immune system. IL-4 influences tumor-associated macrophage polarization in LC. Signal Transducer and Activator of Transcription 6 (STAT6) deficit leads to a decrease in IL-4 levels, therefore resulting in a diminished differentiation of macrophages into M2 macrophages (20). An increase in IL-6 levels can lead to molecular targeted drug resistance in LC, and these levels might be useful as a prognostic marker in patients with NSCLC (21). LC with mutations in the epidermal growth factor receptor (EGFR) exhibits a deficiency in IL-10, resulting in the inadequate induction of CD39 expression in CD8+ T cells, commonly referred to as cytotoxic CD8+ T lymphocytes or CTLs. Furthermore, IL-10 plays a crucial role in enhancing CD8+ T-cell-mediated cytotoxic functions, which depends on the presence of CD39 (22). Th1 cells are involved in the promotion of the anti-tumor immune response, while Th2 cells counteract the activity of Th1 cells. Tumorigenesis and relapse may depend on alterations to Th1/Th2 cytokine homeostasis. Accordingly, in patients with LC, it can be found that Th2 cytokine levels increase, whereas Th1 levels decrease (23).
Th9 cells are characterized by the secretion of IL-10 and IL-9. Th9 cells mainly secrete cytokine IL-9 to act on other cells to play anti-tumor immunity. It has been reported to be effective in eliminating solid tumors, and exhibit remarkable anti-tumor properties compared with Th1 and Th17 cells (24). In addition, it has been reported that Th9 cells induce EMT in LC, thereby promoting migration and metastasis (25). According to the reports mentioned above, Th9 cells have a dual role in tumorigenesis, as they can play both anti-tumor and pro-tumor functions. Therefore, the inducing effect of drugs on Th9 cell function is a promising research direction.
Th17 cells and related immune cytokines can promote either tumorigenesis or tumor suppression, but the relevant mechanism is still unknown. The interaction between Th17 cells and Tregs is crucial in regulating autoimmunity and cancer (26). It is abundantly clear that the balance between Th17 cells and Tregs is significantly disturbed in NSCLC (27). In fact, the intricate relationships between immune cells often play an unexpected role in TIME, but they are often mysterious and waiting to be revealed.
2.2 Cytotoxic T lymphocytes
It is generally recognized that CTLs, as the primary effector cells of cellular immunity in the human body, can detect and eliminate malignancies, making them an essential part of the adaptive immune system. The number of CTLs within the TME is a pivotal prognostic marker of cancer, and high-CTL tumors are more likely to respond to immunotherapy than low-CTL tumors (28). CD3 is a common surface marker of T cells, marking all T cells. CD8+ is used as a specific marker in CTLs, so it is often called the CD8+ T-cell. CD8+ T cells constitute a highly heterogeneous cell population among patients with LC (29). The mechanism by which CTLs kill tumor cells involves the following three aspects (1): the granular exocytosis pathway, via the synaptic exocytosis of cytotoxic granules that contain perforin and granzymes into the target, results in tumour cell destruction (2); the cytotoxic cytokine pathway, secretes cytokines, including IFN-γ and TNF-α (3); and CTLs are capable of eliminating target T cells via a chain reaction that results in apoptosis via the FAS ligand (FASLG) molecule (30, 31). Tumor heterogeneity determines that even the same immune cells play different roles at different TIME. The significance of CTLs in SCLC has been explored more thoroughly than in NSCLC. Insufficient anti-tumor immune responses are caused by a lack of pre-existing tumor-infiltrating T lymphocytes (TITLs), particularly CTLs, in the SCLC TME (32). In addition, SCLC cells have an immune-provoking impact on cytotoxic T lymphocytes, which upregulate the co-inhibitory receptors of CTLs, thus inducing T-cell exhaustion upon prolonged activation (33). As multicellular organisms, cell-cell interactions (CCIs) play an important role in maintaining homeostasis and coordinating physiological functions. CCIs between immunizations plays an irreplaceable role in TIME, although it is complex and mysterious.
2.3 Regulatory T cells
Tregs are a special subgroup of CD4+ T cells with immunosuppressive, which represent 5−10% of peripheral CD4+ T cells (34). Tregs represent an important immunosuppressive cell type in TIME, which inhibit the activation of other immune cells and maintain the immune system’s homeostasis. Tregs can inhibit anti-tumor immunity through two stages, hindering immunosurveillance against cancer development, and hampering effective anti-tumor immune responses in hosts (35). Tregs are the “culprit” that helps tumor cells evade the body’s immune surveillance. Tumor cells can use Tregs to achieve immune escape. In addition, Tregs are therapeutic targets and biomarkers that can predict the LC’s survival length and recurrence, particularly in circulation or regional lymph nodes (36). In NSCLC, Tregs are associated with tumor staging, therapeutic efficacy, and prognosis, infiltrating tissues. Tregs attenuate immunologic anticancer effects in NSCLC patients (37). Their inhibition mechanisms include the following: suppressive cytokines; IL-2 consumption; the antigen-presenting cell (APC) mediated pathway via cytotoxic T-lymphocyte-associated protein 4 (CTLA-4); the T-cell immunoreceptor with immunoglobulin and the ITIM domain (TIGIT); the metabolite-related mechanism via CD39/CD73; lymphocyte activation gene 3 (LAG3); contact-dependent suppression via programmed death ligand 1 (PD-L1); and other mechanisms (38).
2.4 Natural killer cells
NK cells belong to large granular lymphocytes and are the third largest lymphocyte population after T cells and B cells. They serve as the primary effector cells regarding innate immunity and are highly heterogeneous in the TIME. The main role of NK cells can be summarized in two aspects: One acts as an important part of tumor immune surveillance. Many receptors on NK cells tightly regulate their activation and allow them to distinguish between ‘normal’ and ‘dangerous’ cells (39). The second is directly lysing tumor cells in a similar manner to activated CTLs. NK cells possess two primary cytotoxic mechanisms: granulocyte apoptosis mediated by perforin and granzyme and antibody-dependent cell-mediated cytotoxicity (ADCC) (40). Furthermore, as cytotoxic innate lymphoid cells, NK cells generate inflammatory cytokines and chemokines, including TNF-α and IFN-γ (41). The involvement of NK cells in LC is not fixed but rather exhibits alterations throughout the progression of the disease. The anti-tumor effect of NK cells varies according to the stage of LC. In the early stages of tumor development, NK cells with high cytotoxicity and survival can efficiently eliminate tumor cells. The NK cells involved in tumor promotion enter a mildly dysfunctional condition and reach a state of equilibrium with the tumor cells. Tumor cells resist NK cell-mediated immunosurveillance and attempt to escape from them as the tumor develops (42). The interaction between NK cells and LC is reciprocal. The colony-forming level and cytotoxicity of NK are significantly negatively correlated with LC, and the colony-formation and cytotoxicity of NK cells in LC patients were significantly lower than those in healthy people (43). There is increasing evidence suggesting that improving NK cell functioning may induce tumor regression, and immunotherapy targeting NK cells may be an effective strategy in LC treatment (44, 45).
2.5 Tumor-associated macrophages
Tumor-associated macrophages (TAMs) are significant constituents of the TIME, exhibiting a wide range of supporting and inhibitory influences on the growth, advancement, and metastasis of LC (46). In LC, TAMs are involved in participating in the growth, angiogenesis, metastasis, and invasion of cancer cells. Thus, TAMs are regarded as a potentially effective therapeutic target for LC (47). According to a recent study, in LC and LC-related disorders, cytokines and chemokines that are released through interactions between TAMs and tumor cells substantially stimulate antiapoptotic, hyperproliferative, and metastatic responses (48). TAMs can be divided into three subsets: the classical subtype M0 (non-polarized or neutral), M1 (anti-tumor), and M2 (pro-tumor) macrophage. TAMs have the opposite functional potential, which may depend on the cytokine environment in the TIME. The M1- and M2-like gene signature expressions are not mutually exclusive in early LC, as both gene expression profiles can be displayed simultaneously by the TAMs (49). However, TAMs in early-stage lung adenocarcinoma appear to express genes promoting tumorigenesis, and they are not known to have distinct M1 and M2 macrophage polarization (50). The value of TAMs in LC TIME is certain, but their specific mechanism remains to be fully explored and studied.
2.6 Cancer-associated fibroblasts
Over the past decade, the idea that Cancer-associated fibroblasts (CAFs) are immunosuppressive cells has been widely accepted. CAFs, as a most prominent and abundant cell population, are a major component of the TME and account for nearly 70% of cells in tumor tissues (51). CAFs perform a wide range of functions, such as the secretion of inflammatory ligands, growth factors, and extracellular matrix (ECM) proteins that enhance drug resistance, immune antagonistic effects, and tumor development (52). In addition, CAFs are actively involved in cancer progression, in the way that through complex interactions with other cell types in the TIME (53). As prospective therapeutic targets, T-cell exclusion in NSCLC is governed by the distinct ECM profiles and spatial distribution of CAFs (54). Furthermore, CAFs serve as a prognostic indicator for immunotherapy efficacy in non-small cell lung cancer (55). The ‘Reverse Warburg Effect’ in LC is supported by research, but it is limited to certain tumor cell lines and can be modified by different CAFs (56). The complex role and differential efficacy of CAFs in the TIME are certainly fully reflected in LC, but this needs to be further explored.
2.7 Tumor-infiltrating B cells
Although T cells have been the primary focus of tumor immune response studies in recent years, B cells also play an important role in tumor immunity. B cells, which continuously comprise a significant cellular component of the TME, might play an essential function in tumor immunity (57). B-cell density is considered a new prognostic biomarker of NSCLC patient survival (58). LC progression is significantly regulated by tumor-infiltrating B (TIL-B) cells. However, the role of TIL-B cells in human cancer, that is, whether they are anti-tumor or pro-tumor, depends on the tumor type. In patients with NSCLC, there is evidence that TIL-B cells have an anti-tumor effect. Anti-tumor immunity is mediated through the maintenance of structure and function of tertiary lymphoid structures (TLSs), the production of tumor-specific antibodies by TIL-B cells, and the promotion of T-cell responses; all of these mechanisms are associated with favorable outcomes in LC (59). Given their ability to efficiently deliver antigens to CD4+ TILs and modify the CD4+ TIL phenotype, TIL-B cells represent a promising therapeutic target in SCLC (60).
2.8 Dendritic cells
Dendritic cells (DCs) are a heterogeneous leukocyte population comprising distinct subsets, and their strong ability to initiate and regulate adaptive immune responses is the basis for successful anti-tumor immune responses (61). Tumor-specific immune responses are initiated, programmed, and regulated by DCs. DCs possess a distinctive ability to initiate immunological responses by capturing antigens and subsequently processing them into peptides. These peptides are then presented to naive T cells in lymphoid tissues via major histocompatibility complex (MHC) molecules (62). DCs play a critical role in protecting against LC, and clinical trials have shown that their function decreases in LC patients (63). According to research, patients diagnosed with advanced NSCLC have not responded well to vaccination with DCs or DC/cytokine-induced killer (CIK) cells (64). Modulating DCs at the early stage of LC seems to be a feasible immunoanticancer strategy, as suggested by the above studies.
2.9 Mast cells
Mast cells (MCs) are unique tissue-resident immune cells of the myeloid lineage, that secretes a variety of cytokines involved in immune regulation. MCs are recognized as critically shaping tumor cell and TME behavior (65). MCs play a complex role in the TME by modulating different tumor biological events, such as cell proliferation and survival, invasiveness, angiogenesis, and metastasis. Furthermore, tumor-associated MCs can influence TME through their interactions with other tumor-infiltrating cells (66), such as Th cells. The value of MCs in different types of LC continues to be studied. Some studies suggest that a constant decrease in MCs may be implicated in the whole invasive process of lung adenocarcinoma (LUAD) (67). Moreover, research confirms that high MC abundance correlates with prolonged survival in early-stage LC patients (68). The expression of the four genes linked to resting MCs (RMCRGs) and resting MCs infiltration in NSCLC are positively correlated; the greater the risk score, the lower the expression of immune checkpoint inhibitor (ICI) and resting MCs infiltration (69).
2.10 Myeloid-derived suppressor cells
Myeloid-derived suppressor cells (MDSCs) are a diverse population of pathologically activated cells that have strong immunosuppressive properties (70). In the context of cancer, MDSCs are abnormally produced and recruited into the TME to aid in establishing an immunosuppressive microenvironment that facilitates tumor immune escape (71). MDSCs are closely connected to the prognosis of patients with LC (72). There is increasing evidence that MDSCs are involved in the development of LC and may be used to predict the efficacy of immune checkpoint blockade (ICB) treatments (73). MDSCs can be further studied as immunosuppressive regulators and therapeutic targets in LC.
The critical role of TIME in promoting tumor growth and metastasis is strongly established. The TIME has been utilized to analyze particular interactions between tumor cells and immune cells of various types, and it was found that changes in the TIME, represented by immune cells, affect the progression and metastasis of LC. Although the anticancer and pro-cancer effects of some immune cells are still controversial, the role of TIME in LC is well established. This also makes TIME a potential target for improved immunotherapy. Overall, TIME is a novel field facing major challenges and deserves further exploration.
3 Anticancer effects of natural products when targeting tumor immune microenvironments
Natural compounds play an important role in preventing and treating various cancers and have great research value and development prospects. The therapeutic role of natural compounds in cancer is complex and varied, and discoveries are constantly being updated. A wide range of natural compounds have been found to possess anticancer properties, demonstrating various actions such as inhibiting cell proliferation, promoting apoptosis, preventing metastasis and angiogenesis, regulating autophagy, reversing multidrug resistance, modulating immune responses, and enhancing the efficacy of chemotherapy both in vitro and in vivo. Natural compounds have an impact on tumor cells as well as TIME. Natural compounds are crucial to LC immunotherapy (74, 75). A total of 37 natural compounds have been reported to exert anti-cancer effects by targeting the TIME, Figure 1 presents them.
3.1 Terpenoids
Terpenoids are a class of biologically active natural compounds that exhibit a wide range of pharmacological properties, rendering them a valuable reservoir of potential compounds for drug discovery (76). Recent efforts to study and produce terpenoids for their anticancer properties have shown promise and could lead to new possibilities for treating cancer. Many terpenoids are effective anti-LC agents that can delay the invasion and metastasis of lung carcinoma cells (77). Tumor-infiltrating immune cells and associated cytokines are crucial in facilitating or impeding tumor growth, a factor that has become an important focus in the treatment of LC due to the complexity of its development. This study found that 13 terpenoids exert their anti-LC effects by modulating the TIME. Table 1 provides the basic information and mechanisms of these 13 terpenoids derived from recent studies on LC, while Figure 2 presents their chemical structures.
3.1.1 Astragaloside-IV
Astragaloside-IV(AS-IV) is a cycloartane triterpene glycoside chemical and one of the bioactive ingredients isolated from Astragalus membranaceus Bunge. Astragalus membranaceus Bunge, also known as “HuangQi”, is widely used to treat various cancers in Traditional Chinese medicine (TCM). AS-IV, a well-investigated and significant natural compound in TCM, has exhibited its anti-tumor properties by impeding the growth, invasion, and metastasis of tumor cells in multiple cancer types (93). TAMs are key regulators of the complex interplay between cancer and the TIME (94) and a significant therapeutic target in LC. The M2 macrophage subpopulation is a cancer-promoting immune cell in TAMs, and M2 macrophage polarization invasions of tumor sites are frequently associated with tumor development and poor prognosis. By partially inhibiting the AMPK signaling pathway-mediated polarization of TAM M2 macrophages, AS-IV decreases the invasion, angiogenesis, and migration of tumor cells in LC (78). Tryptophan (Trp) is primarily catabolized by the enzyme indoleamine 2,3-dioxygenase (IDO) (95). IDO can degrade Trp, an important amino acid, into kynurenine and other downstream metabolites, which can decrease effector T-cell function and promote Treg differentiation. AS-IV exhibits a potent anticancer effect in vivo and can increase the immune response by lowering Tregs and raising CTLs activity, which may be linked to its ability to suppress IDO expression (79). Astragaloside demonstrates potential as a TIME intervention agent in LC, capable of enhancing the immune response and inhibiting tumor immune evasion through the regulation of various immune cells(include TAMs, CTLs and Tregs mentioned above).
3.1.2 Ginsenosides (Rg3 and Rh2)
Ginsenosides are effective components of terpenoids extracted from Panax ginseng C. A. Mey. (it is also known as Renshen in TCM) that can inhibit tumors and enhance body immune functions. Ginsenosides are a class of tetracyclic saponins that can be categorized into two groups, namely dammarane and oleanane, based on the structure of their aglycones. Within the dammarane group, ginsenosides can be further classified into four types: protopanaxatriol (PPT), protopanaxadiol (PPD), oleanane (OA), and ocotillol (OCT). Ginsenoside Rg3 and ginsenoside Rh2 are both the PPD type. A large number of ginsenosides are effective in LC intervention, but only Ginsenosides Rg3 and Rh2 have been reported on regarding LC immunotherapy.
Although ginsenoside Rg3 and ginsenoside Rh2 compounds are similar in structure, the structure of ginsenoside Rg3 is more complex and well-studied. A meta-analysis of 12 studies confirmed that ginsenoside Rg3 has some efficacy advantages in improving immune function in NSCLC patients compared with other saponins (96). A monomer preparation of ginsenoside Rg3 was approved by the National Medical Products Administration in Chinese and is frequently used for various cancers, particularly in NSCLC, which can improve the survival rate and objective response rate in combination with chemotherapy in NSCLC patients (80).
Tumor cells can become immunogenic by changing from non-immunogenic to immunogenic in response to external stimuli, which is known as immunogenic cell death (ICD). Ginsenoside Rg3 can induce ICD in lung carcinoma cells via the mediated induction of apoptosis and a subsequent increase in the expression of the chaperone protein calreticulin (CRT) and heat shock proteins (HSPs) on the surface of lung carcinoma cells. In addition, Rg3 induces the secretion of TNF-α or TGF-β and promotes IFN-γ production by tumor cells (81). Weakening the tumor’s chemotherapy resistance through immune regulation is also an important part of anticancer research into natural products. PD-L1 is overexpressed in lung carcinoma cells/cisplatin-resistance (CR) cells compared with lung carcinoma cells, and ginsenoside Rg3 can suppress the PD-L1 expression and resume T-cell functions (82). The degree and success rate of anti-tumor immune response are determined by the synergistic effect of multiple cytokines in TIME, and TNF-α, TGF-β and IFN-γ are the cytokines that are often concerned. TNF-α is a central cytokine that contributes to malignant tumor progression in TIME. TNF-α upregulation has also been observed in ginsenoside Rh2-treated A549 tumour cells (97). Ginsenosides have garnered greater attention in the context of their regulatory effects on immune cytokines, in comparison to other natural products.
3.1.3 Atractylenolides (Atractylenolides II and III)
Atractylenolides are sesquiterpenoids produced from the rhizomes of the plant Atractylodes Rhizoma (it is also known as Baizhu in TCM). These compounds have anti-tumor activity both in vitro and in vivo, making them an attractive option for treating a variety of cancers (98). Atractylenolides exhibit a diverse array of pharmacological properties, and their role in the treatment of LC has been proven (99). Atractylenolides mainly include Atractylenolide I, II, and III and Atracylon. Their pharmacological action is somewhat similar, possibly because of their shared tricyclic structure. In atractylenolides, atractylenolides II and III have been reported to be able to exert anti-cancer effects by intervening in TIME.
The promotion of tumor proliferation, angiogenesis, and metastasis is greatly influenced by M2 macrophage. By efficiently preventing M2 macrophage polarization, atractylenolide II prevents tumor cell metastasis in vivo as well as in vitro. The efficient inhibition of M2 macrophage polarization by Atractylenolide II mostly occurs through the activation of the STAT6 signaling pathway by inhibiting IL-4/IL-13 (83).
IDO has been recognized as a crucial protein checkpoint involved in the modulation of the TIME, hence facilitating tumor progression. By directly binding to the Jak3 protein, Atractylenolide III has demonstrated remarkable efficacy in blocking IFN-γ driven Jak3/STAT3 signaling pathway-dependent IDO activation (84).
3.1.4 Cannabinoids
Cannabinoids are terpenophenolic compounds derived from the Cannabis sativa L. plant. Cannabidiol is a non-psychoactive cannabinoid, and clinical studies have reported that cannabidiol may cause surprising reactions in LC patients (100).
The expression of Intercellular Adhesion Molecule-1 (ICAM-1), a transmembrane glycoprotein belonging to the immunoglobulin superfamily (IgSF), is observed in tumor cells. ICAM-1 can impact tumor development by promoting adhesive between tumor and immune cells (101). Cannabidiol can increase lung tumour cell lysis caused by lymphokine-activated killer cells by upregulating ICAM-1 (85). Cannabinoid receptors are key targets for cannabinoid action and are expressed in tumour cells and TIME cells. The expression of the cannabinoid receptor has an impact on the development of cancer in different types of tumors. TME-derived Cannabinoid2 s NSCLC model (102). This provides a new path to discovering LC treatments using cannabinoids to intervene in the TIME.
3.1.5 Triptolide
Triptolide is a diterpenoid of the abietane class that was extracted from Tripterygium wilfordii Hook.(also known as Leigongteng in TCM) Triptolide is the key ingredient of Tripterygium wilfordii Hook against cancers, it demonstrates substantial anticancer properties. The IL-6/STAT3 signaling pathway acts to drive the malignant progression of tumors, while strongly suppressing the antitumour immune response. Thus, target IL-6/STAT3 signaling pathway are poised to provide therapeutic benefit by stimulating antitumour immunity. The IL-6/STAT3 signaling pathway may play an essential role in the TIME; IL-6-mediated STAT3 activation in the TME inhibits the functional maturation of DCs, thus activating effector T cells and blocking the emergence of anti-tumor immunity in cancers (103). Triptolide can exert anti-tumor effects on lung carcinoma cells of NSCLC by inhibiting the activation of the IL-6/STAT3 axis (86).
3.1.6 Oridonin
Oridonin is a biologically active diterpenoid molecule derived from the plant species Rabdosia rubescens. Multiple studies have provided evidence supporting the inhibitory effects of oridonin on angiogenesis in diverse cancer types, including LC (104). Oridonin exhibits potential as an immunostimulatory drug for NK cells, thereby positioning it as a prospective candidate for the treatment of LC. The administration of Oridonin has been observed to improve the cellular cytotoxicity of NK-92MI cells towards tumor cells through the stimulation of degranulation markers and cytotoxic effector molecules. Additionally, it can enhance the upregulation of activation markers on NK-92MI cells, as well as the expression of ligands associated with these indicators in LC cells (87).
3.1.7 Lycopene
Lycopene is a dark-red carotenoid belonging to the tetraterpenoid family and is widely found in a variety of plants, especially ripe red fruits and vegetables such as tomatoes. From an anticancer perspective, people often look to lycopene as a dietary supplement that may help to prevent the occurrence of cancer. Lycopene’s immunomodulatory effects could make it an anticancer agent, as it modulates immune cells to suppress tumor growth and progression (105). Lycopene treatment increases the CD4+/CD8+ ratio in the spleen and promotes IFNγ-expressing CD8+ T cells in tumor tissues. Furthermore, lycopene can assist anti-PD-1 by elevating the IL-1 and IFN-γ levels while also reducing the IL-4 and IL-10 levels in the spleens of mice injected with LLC cells. Upon IFN-γ stimulation, lycopene diminishes PD-L1 expression by activating JAK and repressing AKT phosphorylation (88).
3.1.8 Lupeol
Lupeol is a triterpenoid found in various vegetables and fruits, as well as medicinal plants such as olives, mangos, elms, and Aloe vera (L.) Burm. f., Lupinus micranthus Guss., etc. Lupeol is an effective inhibitor of proliferating tumour cells and has a powerful anticancer effect against various neoplasms (colorectal, lung, and liver) (106). Plasminogen activator inhibitor-1 (PAI-1) is differentially highly expressed in various types of tumor types, which involved in cancer progression. The lupeol demonstrates inhibitory effects on the synthesis of PAI-1, hence impeding the recruitment of THP-1 macrophages (THP-1 cells that have undergone differentiation into macrophages) towards LC cells. Furthermore, it has been observed that lupeol exhibits the ability to inhibit the polarization of M2 macrophages, resulting in a decrease in the migratory capacity of Lewis LC cells (89).
3.1.9 Paeoniflorin
Paeoniflorin is a monoterpene glycoside isolated from Paeonia lactiflora Pall(also known as Baishao in TCM). Paeoniflorin is reported to possess a wide spectrum of anti-tumor effects, including for LC (107). Paeoniflorin can induce tumor cell apoptosis and inhibit tumor proliferation, invasion and metastasis through different mechanisms, and immune regulation be one of the keys to playing these roles.The polarization state and infiltration degree of TAMs in the TME are significantly correlated with cancer treatment and prognosis. Paeoniflorin can affect the cell cycle progression, viability, and migration of lung carcinoma cells by inhibiting the M2 macrophage polarization of TAMs (90).
3.1.10 Bakuchiol
Bakuchiol is a natural meroterpenoid extracted from Cullen corylifolium (L.) Medik. Bakuchiol is considered a potential anticancer compound, and its anti-tumor activity against LC has been confirmed in vitro (108). Bakuchiol treatment increases the population of cytotoxic immune cells (i.e., M1 macrophages and CD8+ T cells) while also decreasing pro-tumor immune cells (i.e., CD3+ T cells, Tregs, and M2 macrophages). Bakuchiol stimulates the production of anti-inflammatory cytokines, such as IL1-α, IL2, IFN-γ, TNF-α, IL4, and IL10. PD-L1 expression in the tumor is also lowered by Bakuchiol. AKT and STAT3 signaling is inhibited by Bakuchiol (91).
3.1.11 Platycodin D
Platycodin D is a triterpenoid saponin extracted from Platycodon grandiflorus (Jacq.) A. DC.(it is also known as Jiegeng in TCM). Jiegeng is a herb that has been used TCM in China for thousands of years and is commonly found in various tumor treatment TCM formula(Fufang). By binding PD-L1 to PD-1 on the surface of T cells, tumor cells can inhibit the immune effect of T cells, leading to immune escape. Jiegeng has a role in regulating TIME and can trigger anti-tumor immunity by limiting PD-1 expression in CD8+ T cells (109). Platycodin D decreases the protein level of PD-L1, triggers the extracellular release of PD-L1 in lung carcinoma cells, and enhances IL-2 secretion in T cells (92).
3.2 Flavonoids
Flavonoids are a large class of natural products that have a variety of quantities and complex structures In chemical structure, the basic skeleton of flavonoids is composed of three rings (C6-C3-C6) (110). Many preclinical studies have demonstrated that flavonoids inhibit LC development and can target signaling pathways (111). Flavonoids have diverse and extensive immunomodulatory and anticancer activities (112). This study found that 10 flavonoids exert anti-lung-cancer effects by modulating the TIME. Table 2 provides the basic information and mechanisms of these 10 flavonoids derived from recent studies on LC, while Figure 3 presents their chemical structures.
3.2.1 Rocaglamide
Rocaglamide is a flavonoid extract obtained from Aglaia odorata and Aglaia duperreana belonging to the cyclopenta [b]-tetrahydrobenzofurans chemical class. In vivo and in vitro studies have shown that rocaglamide is a new candidate drug for the treatment of cancer (123). Concerning the structure-specific postulated biogenetic origin of rocamide, it is possible that the cycloaddition of a flavonoid nucleus and a cinnamic acid amide moiety result in the formation of cyclopenta [bc]benzopyran ring system (124).
Rocaglamide can play an anticancer role in NSCLC by promoting NK cell infiltration. There are two main mechanisms by which rocaglamide regulates NK cells, including the autophagy inhibition pathway and the non-autophagy inhibition pathway. UNC-51-like kinase 1 (ULK1), a serine/threonine kinase, plays a key role as an autophagic initiator. In the first pathway, rocaglamide enhances the NK-cell-mediated killing of NSCLC cells by inhibiting autophagy, which is achieved by targeting ULK1 (113). The cyclic GMP-AMP synthase (cGAS) and its downstream signalling effector stimulator of interferon genes (STING) play a crucial role in cancer development. The cGAS/STING signaling pathways has been widely concerned and has become the forefront and hot spot of current research. Rocaglamide activates cGAS/STING signaling pathways in NSCLC cells, leading to the upregulation of CCL5 and CXCL10 and the enhanced infiltration of NK cells (114).
3.2.2 Puerarin
An isoflavone glycoside known as puerarin was extracted from the root of the Pueraria lobata (Willd.) Ohwi and is known as Gegen in TCM. Puerarin is a naturally occurring medicinal substance that exhibits a range of biological activities, including antioxidative, anti-inflammatory, anti-tumor, immunomodulatory, and neuroprotective properties (125). It is one of the key components of puerarin’s anti-tumor effect, regulating immune cells and cytokines. Puerarin, a negative metastasis regulator of NSCLC, can induce anti-tumor effects by skewing macrophage populations back into the M1 subpopulation. Moreover, puerarin decreases the level of pro-tumor cytokines (IL-10, TGF-β, and IL-4) and increases the expression of anti-tumor cytokines (IFN-γ, IL-12, and TNF-α) (115).
3.2.3 Nobiletin
Nobiletin is a main flavone compound of the peels of citrus fruits, such as Citrus depressa, Citrus sinensis, Citrus reticulata, Citrus limon, etc. Numerous pharmacological effects attributed to nobiletin have been researched recently, including NOB’s anti-tumor activity (126). PD-1 is an essential component of the TIME and functions as a receptor that impedes T-cell activation. The EGFR phosphorylation can activates Janus kinase-2 (JAK2) and signal transduction, thereby activating STAT3 to results in the regulation of PD-L1 expression. Nobiletin inhibits the expression of PD-L1 by EGFR/JAK2/STAT3 signaling pathway, thereby enhancing anti-tumor immunity (116).
3.2.4 (−)-Epigallocatechin gallate
The compound (−)-epigallocatechin gallate (EGCG), classified as a flavonoid, is a polyphenolic catechin that constitutes approximately 59% of the overall catechins present in green tea leaves (127). EGCG partially restores T cell activity by inhibiting PD-L1/PD-1 signaling pathway, leading to the inhibition of lung carcinoma cell growth. The mechanism of EGCG-inhibited PD-L1 expression is induced by both IFN-γ and epidermal growth factor (EGF) (117). The role of IFN-γ in tumor immunotherapy is full of paradoxes. IFN-γ can enhance immune function, but it can also accelerate T cell failure by up-regulating PD-L1. The regulation of IFN-γ by EGCG requires further exploration due to its diverse role.
3.2.5 Myricetin
Myricetin is a flavonol compound widely found in many plants. The families Myricaceae, Polygonaceae, Primulaceae, Pinaceae, and Anacardiaceae are the richest sources of myricetin (128). IFN-γ is a major modulator of the TIME and performs a critical function in TIME. Myricetin reverses the effects of IFN-γ-treated LC cells on the survival, CD69 expression, proliferation, and IL-2 production of Jurkat-PD-1 T cells. An essential mechanism underlying the aforementioned therapeutic effects is that IFN-γ induces transcriptional upregulation of PD-L1 and IDO1 via the JAK/STAT/IRF1 axis, which is targeted and inhibited by myricetin (118).
3.2.6 Licochalcone A
Licochalcone A is a flavonoid extracted from Glycyrrhiza uralensis Fisch.(also known as Gancao in TCM) and presents a wide range of pharmacological effects, including anticancer. The excessive secretion of IFN-γ in the TIME can induce the expression of PD-L1 in tumor cells and promote the immune escape of tumor cells. Licochalcone A abrogates IFN-γ-induced PD-L1 expression via reactive oxygen species (ROS) generation (119).
3.2.7 Silibinin
Silibinin is a natural flavonol isolated from Silybum marianum (L.) Gaertn. Silibinin reportedly possesses strong anticancer properties, and immune regulation is one aspect of its anticancer role. NSCLC with anaplastic lymphoma kinase (ALK) gene rearrangement is a specific type of NSCLC. Immune evasion in ALK-positive NSCLC may be facilitated by PD-L1. Silibin reverse acquires resistance and restores sensitivity to crizotinib-resistant tumor cells. Silibinin treatments significantly inhibit the upregulation of the PD-L1 immune checkpoint regulator in crizotinib-refractory LC cells (120).
3.2.8 Isovitexin
Isovitexin is an isomer of vitexin extracted from Vitex negundo var. cannabifolia (Siebold and Zucc.) Hand.-Mazz. Isovitexin has a variety of biological activities, including anti-cancer. Isovitexin promotes lipopolysaccharide (LPS)- and lectin-stimulated splenocyte proliferation and enhances CTLs and NK cell activities, as well as the secretion of IL-2 and TNF-α (121).
3.2.9 Luteolin (Apigenin)
Luteolin is a flavonoid that was originally isolated from Reseda odorata L. It is found in vegetables, medicinal herbs, and fruits. Apigenin is mainly derived from Apium graveolens L., but it is also found in other plants such as Perilla frutescens (L.) Britton, Verbena officinalis L., and Melissa axillaris (Benth.) Bakh. f. Luteolin and apigenin can block interactions between PD-1 in T cells and PD-L1 in tumor cells, inhibiting IFN-γ-induced PD-L1 expression (122).
3.3 Alkaloids
Alkaloids are a large group of naturally occurring organic compounds that contain a nitrogen atom or nitrogen atoms in their structures, which cause alkalinity. The acidic tumor microenvironment (ATME) greatly limits the activity of immune cells, which are not conducive to anti-tumor immune responses. Previous studies have shown that low pH in tumors inhibits anticancer immune effectors. In addition, the ATME can contribute to immune evasion (129). Alkaloids can play a therapeutic role by neutralizing the ATME. Alkaloids also serve as a rich resource for drug discovery, and numerous alkaloids screened from medicinal plants have shown antiproliferative and anticancer effects in a wide variety of cancers both in vitro and in vivo (130). This study found that seven alkaloids exert anti-lung-cancer effects by modulating the TIME. Table 3 provides the basic information and mechanisms of these seven alkaloids derived from recent studies on LC, while Figure 4 presents their chemical structures.
3.3.1 Matrine
Matrine is a key tetracycloquinolizindine alkaloid isolated from Sophora flavescens Aiton(also known as Kushen in TCM). Many studies have been conducted on its anticancer activities against different types of tumour cells, and the results are reasonably acceptable (140).
Toll-like receptors (TLRs) are present and play multiple functions in various immune cell types involved in tumor immunity. TLRS can be expressed on macrophages, neutrophils, DCs, NK cells and mast cells. Matrine exerts an anti-tumor effect by the modulation of the TLR signaling pathway, facilitating the release of immune cytokines, increasing the efficiency of immune cells, and promoting an immunological response. It mainly includes the following aspects: First, matrine interferes with the secretion of immune cytokines, which might decrease the secretion of IL-10 and increase that of IL-6, TNF-α, and IL-12. The second feature is that matrine increases the expression of MHC-II, CD54, CD80, and CD86 on the surface of DCs, which promotes DC differentiation and maturation. In addition, matrine can cause autologous mixed T lymphocytes to produce DC-activated killer (DAK) cells (131). The phosphatidylinositol-3-kinase (PI3K)/protein kinase B (AKT or PKB) signaling pathway serves as a crucial regulator in macrophage survival, migration and proliferation. The anti-cancer and anti-metastasis effects of matrine inhibit EMT induced by M2-like macrophages via the AKT/PI3K signaling pathways, boosting T-cell-mediated anti-tumor immunity by targeting TAMs (132).
3.3.2 Sanguinarine
Sanguinarine, a benzophenanthridine alkaloid obtained from the root of Sanguinaria canadensis, exhibits significant potential in the field of cancer treatment due to its potent anticancer properties (141). MDSCs play a key role in inhibiting tumor immune effector cell function, preventing cancer from being attacked by the patient’s immune system. Sanguinarine reduces the MDSC accumulation, weakens the immunosuppressive ability of MDSCs, promotes the differentiation of MDSCs (differentiated into macrophages and DC), and increases the anti-lung-cancer immune response (133). In addition, sanguinarine has the potential to act as a novel regulator of the Wnt/β-catenin signaling pathway, modulating M2 macrophage polarization and inhibiting angiogenesis, both of which have potential application values in LC immunotherapy therapy (134).
3.3.3 Homoharringtonine
Homoharringtonine is a cytotoxic alkaloid that was originally isolated from Cephalotaxus hainanensis. It is a unique agent with a long history of research development. Homotaxine, an approved medication by the Food and Drug Administration (FDA) for the treatment of chronic myeloid leukemia, has been found to exhibit notable inhibitory effects on the expression of NRF2 and ARE-dependent genes in human LC A549 cells. Furthermore, homotaxine has demonstrated its potential as an anti-LC agent, as indicated by numerous studies (142). Homoharringtonine may be a potent immunotherapy drug, against LC associated with Kirsten rats arcomaviral oncogene homolog (KRAS) mutations by directly killing tumour cells and indirectly influencing the TIME. Its influence on the TIME includes two aspects: one promotes the activation of TIL-B, resulting in its anti-tumor activity; the second suppresses the expression of tumor suppressors (RB and p21) and oncogenic proteins (Kras, Akt, ERK, STAT3, CDK6, and CDK4) (135).
3.3.4 Oxymatrine
Oxymatrine, a quinolizidine alkaloid, is a prominent member of the matrine-type alkaloids derived from Sophora flavescens Aiton. Numerous investigations have shown that OMT has numerous useful pharmacological qualities, including anticancer effects (143). Oxymatrine may increase the apoptosis of drug-resistant tumor cells by activating DC differentiation and function, thereby enhancing the anti-tumor immune response. Oxymatrine may promote DC maturation and mediate the differentiation of T cells into Tregs (136).
3.3.5 Morphine
Morphine is used as an opioid analgesic to treat acute and chronic moderate-to-severe pain. First discovered in 1806, Serturner isolated the monomer compound morphine from opium poppies, pioneering and promoting a new branch of science that came to be known as alkaloid chemistry (144). Morphine, a common analgesic in clinical practice, is favored for alleviating cancer pain, but it can also control the immune response to tumors. Morphine regulates immune factors (IL-2, IL-10, TGF-β, and PD-L1), thereby promoting tumor immune escape (137).
3.3.6 Evodiamine
Evodiamine is one of the main alkaloid components extracted from Tetradium ruticarpum (A. Juss.) T. G. Hartley and has been reported to have anti-tumor activity in human tumour cells (145). MUC1-C is an oncogenic protein that is excessively expressed in cancer. By increasing CD8+ T cells and decreasing the MUC1-C/PD-L1 axis, evodiamine is primarily utilized in the treatment of NSCLC (138).
3.3.7 Sophocarpine
Sophocarpine is one of the representative constituents of quinolizidine alkaloids extracted from Sophora alopecuroides Linn. Sophorine is a quinolizidine alkaloid, which has many pharmacological effects, mainly showing strong anti-tumor activity and anti-inflammatory effect. Adenosine A1 receptors (ADORA1) play a role in promoting tumor growth in cancer. Activated transcription factor 3 (ATF3) is abnormally expressed in a variety of cancers and is involved in tumorigenesis. By stimulating the ADORA1/ATF3 axis, sophocarpine increases PD-L1 expression, thereby enhancing the efficacy of PD-L1 inhibitory therapy (139).
3.4 Other natural products
Other natural products include polyphenols, quinones, steroids, and phenylpropanoids, which are not classified separately given their small quantities, but they are introduced by merging related natural products. This study found that seven other natural products exert anti-lung-cancer effects by modulating the TIME. Table 4 provides the basic information and mechanisms of these seven additional natural compounds derived from recent studies on LC, while Figure 5 shows their chemical structures.
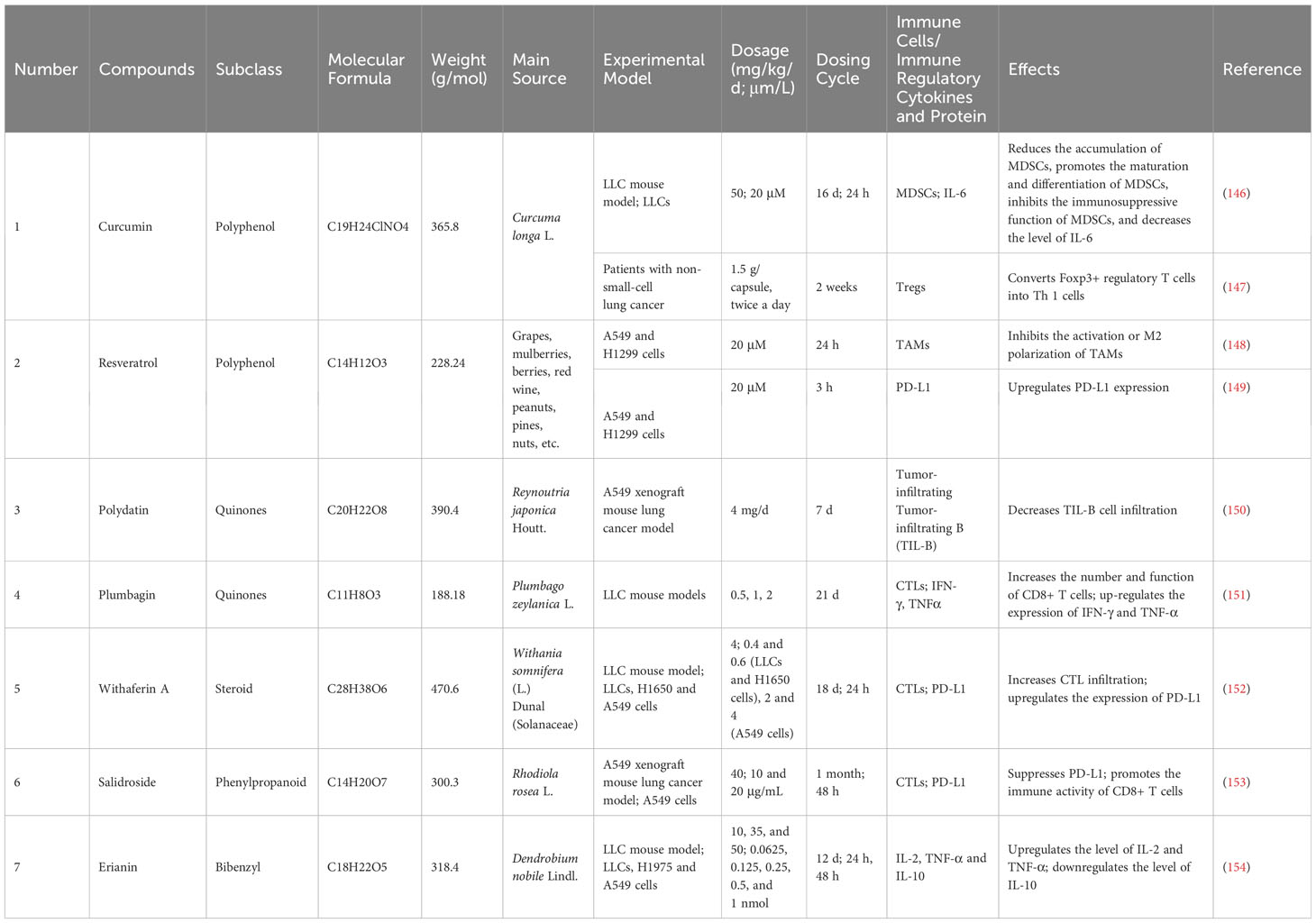
Table 4 Basic information and mechanisms of 7 additional natural compounds derived from recent studies.
3.4.1 Curcumin
Curcumin is a lipophilic polyphenol derived from Curcuma longa L. that has been documented to have promising anticancer activities, and it is well tolerated in humans (155). MDSCs have a significant role in the TIME as potent immune-suppressive cells. Curcumin has been observed to decrease the accumulation of MDSCs and facilitate the development and differentiation of MDSCs within tumor tissue. Moreover, curcumin also inhibits the immunosuppressive function of MDSCs and decreases the level of IL-6 (146). Curcumin therapy inhibits Tregs and increases Th1 in the peripheral system of LC patients by repressing the gene transcription of Foxp3 and increasing the expression of iIFN-γ (147). Furthermore, curcumin could improve the immune system in LC by inducing efficient T-cell-mediated anti-tumor immunological responses (156).
3.4.2 Resveratrol
Resveratrol, a non-flavonoid polyphenol, was initially identified in the roots of white hellebore (Veratrum grandiflorum O. Loes) in 1940, and subsequently isolated from the roots of Polygonum cuspidatum in 1963 (157). Resveratrol is mainly found in grapes, mulberries, berries, red wine, peanuts, pines, and nuts. Although resveratrol is a phytochemical known for its antioxidant properties, its anticancer potential has been researched worldwide.
Inhibiting the activation or M2 macrophage polarization of TAMs is an effective cancer therapy. By preventing TAMs from polarizing M2-like, resveratrol suppresses the proliferation of LC (148). Resveratrol inhibits anti-tumor immunity primarily by regulating PD-L1 expression via the Wnt signaling pathway activation pathway (149). In addition, resveratrol can increase TNF-α, IFN-γ, IL-12, and IL-2 expression and increase the cytotoxic effects of CD8+ T-cell expressions in lung squamous cell carcinoma (158).
3.4.3 Polydatin
Polydatin is an anthraquinone component extracted from Reynoutria japonica Houtt. and has a variety of biological roles. The therapeutic role of polydatin in LC intervention is positive, and studies have confirmed that it may be a potential therapeutic candidate for treating NSCLC, playing a role by inhibiting proliferation and metastasis (159). Polydatin can improve sensitivity and reduce the adverse effects of radiotherapy through immune regulation. TIL-B cells are found in all phases of LC, implying that B-cells play an important role in the course of the disease. Polydatin significantly decreases radiotherapy-induced tumor B-cell infiltration (150).
3.4.4 Plumbagin
Plumbagin is a plant-derived naphthoquinone mainly obtained from three families, including Plumbaginaceae, Droseraceae, and Ebenaceae (160). The number of CD8+ T cells that infiltrate tumors is shown to rise in mice treated with plumbagin. Furthermore, these cells exhibit promising effector capabilities. Specifically, CD8+ T cells effectively activate phenotypes and increase the effector function of CD8+ T cells by upregulating the production of TNF-α, IFN-γ, and granzyme B (GrzmB). In addition, the proportion of MHC-2/DC cells in mice with tumors treated with plumbagin was increased (151).
3.4.5 Withaferin A
Withaferin A is a pivotal steroidal lactone extracted from Withania somnifera (L.) Dunal (Solanaceae), also known as Ashwagandha, was one of the pivotal prehistoric remedies in Ayurveda. Withaferin A is receiving growing attention as a promising anticancer phytochemical, because of its polypharmaceutical medicinal effects, suppressing tumor cell survival, proliferation, motility, metastasis, angiogenesis, and chemosensitization (161).
Withaferin A has been found to trigger ICD in NSCLC cells, leading to an upregulation of PD-L1 expression. Furthermore, withaferin A targets immunosuppressive cells and increases CTL infiltration to raise LC tumor susceptibility to α-PD-L1, which in turn triggers an anti-tumor immune response (152).
3.4.6 Salidroside
Salidroside is a phenylpropanoid glycoside isolated from Rhodiola rosea L.(also known as Hongjingtian in TCM), which has been used for a long time as adaptogens in TCM (162). Salidroside promotes the immune activity of CD8+ T cells by inhibiting the expression of PD-L1, thereby preventing tumor immune escape in LUAD cells. However, the PD-L1 pathway mediated by circ_0009624 is the key to the above effect (153).
3.4.7 Erianin
Erianin is a natural bibenzyl compound isolated from Dendrobium chrysotoxum Lindl and has been reported to be a prospective natural agent for LC treatment (163). Erianin is abundant in TCM Shihu, Shihu is widely used in the treatment of lung diseases. Administering erianin substantially upregulates IL-2 and TNF-α levels, decreases IL-10 levels, and enhances immune function (154).
4 Discussion and conclusions
LC is still the leading cause of cancer-related mortality. Surgery, chemotherapy, and radiation therapy are the main conventional treatments commonly used for LC. Cancer immunotherapy has many advantages over chemotherapy and radiation therapy, and immunotherapy has received particular attention because of its favorable efficacy, low-risk ratio, and long-lasting activity (164). The TIME is an important target and pathway for immunotherapy. A variety of immune cells, stromal cells and cytokines within the TME can affect responses to immunotherapy (165). Changes in immune cells, cytokines, and associated receptors in the TIME can affect the development and deterioration of tumors. The anti-tumor effects of natural products are constantly being explored, and they may have value in treating tumors through TIME intervention. A total of 37 natural compounds have been reported to exert immunotherapeutic effects by modulating immune cells, cytokines, or related receptors in the TIME. Many of the natural compounds discussed in this paper can affect the TIME of LC, can improve immune surveillance, have cancer-cell-killing abilities, and have great potential as adjuvant therapies for LC. Tumors can resist chemotherapeutic drugs through various mechanisms, and TIME is crucial to this process. The combining natural compounds and chemotherapeutic drugs has a synergistic effect, which can solve the problem of chemotherapy drug resistance.
Targeting TIME for the treatment of LC has certain regularities, with terpenoids being the most prevalent natural compounds, and 13 species of terpenoids exist. The extensive quantity and diverse array of terpenoids could contribute to this observed phenomenon. In the study of the chemical structure of natural products, we found that there are great differences in the chemical structure of various natural products, and we have not found any obvious similarity and regularity. The sample size currently used is not large enough to support the analysis, which may be one reason for this. Therefore, it is crucial to further augment the sample size in order to comprehensively illuminate the chemical structural attributes of natural products. In the main sources of natural products, some content worth researching and exploring has been found. It is found that many natural products targeting TIME therapy for LC are derived from Chinese Herbal Medicine (CHM). Moreover, these CHM are consistent in TCM classification, that is, most of them belong to the CHM tonic (Buyi, Shihu, Hongjingtian, etc.). class, such as Renshen, Huangqi, Baizhu, Gancao, Baishao, Shihu Hongjingtian, etc. The CHM exerts its efficacy in cancer treatment by hindering tumor progression and enhancing the immune system of the host organism (166). The tumor elimination mechanism through the restoration of the body’s immune function aligns with the TCM concept of “nourishing positive accumulation and eliminating cancer by itself” (167). These perspectives appear to offer a guiding explanation for the management of LC utilizing TCM.
Tumor-bearing experimental animals are crucial for the preclinical development of cancer drugs. A broad range of tumor models is available, the subcutaneous tumor model is the most frequently utilized model (168). The LLC mouse model and A549 xenograft mouse LC model are the two most common animal models of LC in preclinical studies of natural products against LC. The LLC cell line was first derived from a C57BL mouse that had a tumor in its lung due to the implantation of primary LLC. LLC is highly representative of the human adenocarcinoma subtype of LC and widely used as a model for LC. Human lung adenocarcinoma cells were used to develop the A549 cell line, which is commonly employed to generate xenograft LC models, particularly in NSCLC. Most in vitro experiments are also carried out around the above two cell lines, using mature animal or cell models, which also provides more reliable evidence for the effectiveness of using natural products to intervene in LC.
The study on the common/classical signaling pathway for regulating natural products of TIME is a hot topic, but unfortunately, there are no obvious rules to be found. STAT proteins are known as “potential therapeutic targets for altering metabolic rate”. Among them, STAT3-related signaling pathways may have certain developmental value in TIME regulation. During the intervention of triptolide, atractylenolide III,nobiletin and bakuchiol, STAT3-related signaling pathways were all mentioned. The Wnt signaling pathway was mentioned in studies on the regulation of immune cells and immune checkpoints by resveratrol and sanguinarine. But the reference to a generic/classical signaling pathway in the above study seems to be more accidental. Perhaps there is not yet enough content of natural products that regulate TIME-related signaling pathways to provide useful references and enlightening perspectives. Immunotherapies that target PD-1/PD-L1 axis have shown unprecedented success in a wide variety of human cancers (169). Of course, PD-1/PD-L1 axis may be the most concerned common/classical axis in the natural product intervention TIME, and we will introduce it in the next paragraph.
As for the relevant research on using natural products to intervene in the TIME, we found that the target research has concentration characteristics. TAMs are one of the most investigated immune cells about therapies with natural products in TIME. At least ten different natural compounds have been identified to have a role in LC treatment via TAMs. TAMs are crucial components of the TIME and are involved in the progression, metastasis, and proliferation of tumors. The recent advancements in natural compounds in immunomodulation have highlighted their significant potential against cancer (170). In addition, 6 natural products were reported to have anti-cancer effects by regulating CTLs. However, there are fewer studies on natural products targeting other immune cells, so they are not introduced in detail. PD-1 acts as a crucial factor in impeding immune responses and fostering self-tolerance through its regulation of T-cell activity, activation of antigen-specific T-cell apoptosis, and inhibition of Tregs’ apoptosis (171). PD-L1, serving as the primary ligand for PD-1, generates a co-inhibitory signal within activated T cells, hence facilitating T-cell apoptosis, impaired reactivity, and compromised functional capacity (172). The proteins PD-1 and PD-L1 have emerged as significant subjects of study in the field of cancer immune regulation. Researchers have identified at least 17 natural products that exhibit anticancer properties in LC via modulating the activity of these proteins. IFN-γ is a cytokine that is predominantly produced by immune system cells. It plays crucial functions in maintaining tissue equilibrium, as well as in immunological responses and the surveillance of tumors (173). Macrophages are the main physiological targets of IFN-γ, and natural products interfere with the TME by regulating IFN-γ to play an anticancer role. IFN-γ can induce PD-L1 expression on tumor cells, a phenomenon called “adaptive resistance” (174). Many of natural products play an anticancer role by inhibiting IFN-γ secretion/expression and thereby affecting PD-L1. Research has revealed that TNF-α induces diverse oncogenic and tumor-suppressive effects in TME and that dynamic changes in TME could influence the pharmacological action of PD-1/PD-L1 blockers (175). Many natural products have been shown to regulate the secretion of TNF-α, but the subsequent role of TNF-α regulation remains to be further revealed. Attempts to discover a signaling pathway in natural product regulation of TIME have received much attention, but unfortunately have not. The Stat3-related pathway may have some development value, and it has been mentioned in the intervention process of triptolide, atractylenolide III,nobiletin and bakuchiol.
The TIME is a complex system. It is regulated by various signaling pathways, necessitates the interaction of different cell types, and modulates a broad spectrum of cellular responses. Although the numerous studies described in this review have examined the involvement of natural products in cancer mechanisms through TIME interventions, there is still a lack of research on the complex regulatory networks in the TIME formed by multiple pathways. Most natural products work by interfering with one type of immune cell or cytokine, but the incremental effect or crosstalk reaction of different immune cells or cytokines has yet to be revealed. Most studies on TIME intervention using natural products have focused on experiments involving cell lines and subcutaneous tumor mice, so there is a lack of human clinical observation data. The complex structure of natural products can provide effective reference and inspiration for drug development. In addition, the generation of structural analogues to explore structure–activity relationships and optimize natural product leads can be challenging (176). Given a series of factors, including the limited number of studies included in this work and the complexity of the chemical structures of natural products, this study only summarizes the structural commonalities of natural products in the treatment of LC through immune regulation, a subject that needs further exploration. anti-tumor immunotherapy has shown good application prospects in the clinical treatment of LC, but most natural products still need to be taken from their original discovery in basic research to clinical diagnosis and treatment. The use of natural compounds as candidate drugs for new tumor immunotherapies has great development prospects, and it is a path and direction worth exploring in tumor research.
Author contributions
PY: Writing – original draft. SL: Writing – review & editing. ZL: Writing – original draft. CX: Writing – review & editing.
Funding
The author(s) declare that no financial support was received for the research, authorship, and/or publication of this article.
Conflict of interest
The authors declare that the research was conducted in the absence of any commercial or financial relationships that could be construed as a potential conflict of interest.
Publisher’s note
All claims expressed in this article are solely those of the authors and do not necessarily represent those of their affiliated organizations, or those of the publisher, the editors and the reviewers. Any product that may be evaluated in this article, or claim that may be made by its manufacturer, is not guaranteed or endorsed by the publisher.
Glossary
References
1. Ferlay J, Colombet M, Soerjomataram I, Parkin DM, Piñeros M, Znaor A, et al. Cancer statistics for the year 2020: An overview. Int J Cancer (2021) 149:778–89. doi: 10.1002/ijc.33588
2. Schabath MB, Cote ML. Cancer progress and priorities: lung cancer. Cancer Epidemiol biomark Prev (2019) 28:1563–79. doi: 10.1158/1055-9965.EPI-19-0221
3. Scott EC, Baines AC, Gong YT, Moore R, Pamuk GE, Saber H, et al. Trends in the approval of cancer therapies by the FDA in the twenty-first century. Nat Rev Drug Discovery (2023) 22:625–40. doi: 10.1038/s41573-023-00723-4
4. Pansy K, Uhl B, Krstic J, Szmyra M, Fechter K, Santiso A, et al. Immune regulatory processes of the tumor microenvironment under Malignant conditions. Int J Mol Sci (2021) 22:13311. doi: 10.3390/ijms222413311
5. Lv BZ, Wang YP, Ma DJ, Cheng W, Liu J, Yong T, et al. Immunotherapy: Reshape the tumor immune microenvironment. Front Immunol (2022) 13:844142. doi: 10.3389/fimmu.2022.844142
6. Bader JE, Voss K, Rathmell JC. Targeting metabolism to improve the tumor microenvironment for cancer immunotherapy. Mol Cell (2020) 78:1019–33. doi: 10.1016/j.molcel.2020.05.034
7. Kim A, Ha J, Kim J, Cho Y, Ahn J, Cheon C, et al. Natural products for pancreatic cancer treatment: From traditional medicine to modern drug discovery. Nutrients (2021) 13:3801. doi: 10.3390/nu13113801
8. Hashem S, Ali TA, Akhtar S, Nisar S, Sageena G, Ali S, et al. Targeting cancer signaling pathways by natural products: Exploring promising anti-cancer agents. BioMed Pharmacother (2022) 150:113054. doi: 10.1016/j.biopha.2022.113054
9. Deng LJ, Qi M, Li N, Lei YH, Zhang DM, Chen JX. Natural products and their derivatives: Promising modulators of tumor immunotherapy. J Leukoc Biol (2020) 108:493–508. doi: 10.1002/JLB.3MR0320-444R
10. Altorki NK, Markowitz GJ, Gao DC, Port JL, Saxena A, Stiles B, et al. The lung microenvironment: An important regulator of tumour growth and metastasis. Nat Rev Cancer (2019) 19:9–31. doi: 10.1038/s41568-018-0081-9
11. Park EG, Pyo SJ, Cui YX, Yoon SH, Nam JW. Tumor immune microenvironment lncRNAs. Brief Bioinform (2022) 23:bbab504. doi: 10.1093/bib/bbab504
12. Yu WN, Lei QY, Yang L, Qin GH, Liu SS, Wang D, et al. Contradictory roles of lipid metabolism in immune response within the tumor microenvironment. J Hematol Oncol (2021) 14:187. doi: 10.1186/s13045-021-01200-4
13. Saravia J, Chapman NM, Chi HB. Helper T cell differentiation. Cell Mol Immunol (2019) 16:634–43. doi: 10.1038/s41423-019-0220-6
14. Boieri M, Malishkevich A, Guennoun R, Marchese E, Kroon S, Trerice KE, et al. CD4+ T helper 2 cells suppress breast cancer by inducing terminal differentiation. J Exp Med (2022) 219:e20201963. doi: 10.1084/jem.20201963
15. Mosmann TR, Cherwinski H, Bond MW, Giedlin MA, Coffman RL. Two types of murine helper T cell clone. I. Definition according to profiles of lymphokine activities and secreted proteins. J Immunol (1986) 136:2348–57.
16. Ma QY, Huang DY, Zhang HJ, Wang SH, Chen XF. Upregulation of bacterial-specific Th1 and Th17 responses that are enriched in CXCR5+CD4+ T cells in non-small cell lung cancer. Int Immunopharmacol (2017) 52:305–9. doi: 10.1016/j.intimp.2017.09.024
17. Pal S, Yadav P, Sainis KB, Shankar BS. TNF-α and IGF-1 differentially modulate ionizing radiation responses of lung cancer cell lines. Cytokine (2018) 101:89–98. doi: 10.1016/j.cyto.2016.06.015
18. Zhang XH, Zeng YY, Qu QX, Zhu JJ, Liu ZY, Ning WW, et al. PD-L1 induced by IFN-γ from tumor-associated macrophages via the JAK/STAT3 and PI3K/AKT signaling pathways promoted progression of lung cancer. Int J Clin Oncol (2017) 22:1026–33. doi: 10.1007/s10147-017-1161-7
19. Pan YW, Hao Y, Han H, Chen T, Ding HL, Labbe KE, et al. Nemvaleukin alfa, a novel engineered IL-2 fusion protein, drives antitumor immunity and inhibits tumor growth in small cell lung cancer. J Immunother Cancer (2022) 10:e004913. doi: 10.1136/jitc-2022-004913
20. Fu CP, Jiang LY, Hao SY, Liu ZL, Ding SL, Zhang WW, et al. Activation of the IL-4/STAT6 signaling pathway promotes lung cancer progression by increasing M2 myeloid cells. Front Immunol (2019) 10:2638. doi: 10.3389/fimmu.2019.02638
21. Liu W, Wang HX, Bai FX, Ding L, Huang YY, Lu CC, et al. IL-6 promotes metastasis of non-small-cell lung cancer by up-regulating TIM-4 via, NF-κB. Cell Prolif (2020) 53:e12776. doi: 10.1111/cpr.12776
22. Qiao M, Zhou F, Liu XY, Jiang T, Wang HW, Jia YJ, et al. Interleukin-10 induces expression of CD39 on CD8+T cells to potentiate anti-PD1 efficacy in EGFR-mutated non-small cell lung cancer. J Immunother Cancer (2022) 10:e005436. doi: 10.1136/jitc-2022-005436
23. Mateu-Jimenez M, Curull V, Pijuan L, Sánchez-Font A, Rivera-Ramos H, Rodríguez-Fuster A, et al. Systemic and tumor Th1 and Th2 inflammatory profile and macrophages in lung cancer: Influence of underlying chronic respiratory disease. J Thorac Oncol (2017) 12:235–48. doi: 10.1016/j.jtho.2016.09.137
24. Chen T, Guo JF, Cai ZH, Li BH, Sun LL, Shen YY, et al. Th9 cell differentiation and its dual effects in tumor development. Front Immunol (2020) 11:1026. doi: 10.3389/fimmu.2020.01026
25. Salazar Y, Zheng X, Brunn D, Raifer H, Picard F, Zhang YJ, et al. Microenvironmental Th9 and Th17 lymphocytes induce metastatic spreading in lung cancer. J Clin Investig (2020) 130:3560–75. doi: 10.1172/JCI124037
26. Knochelmann HM, Dwyer CJ, Bailey SR, Amaya SM, Elston DM, Mazza-McCrann JM, et al. When worlds collide: Th17 and Treg cells in cancer and autoimmunity. Cell Mol Immunol (2018) 15:458–69. doi: 10.1038/s41423-018-0004-4
27. Joerger M, Finn SP, Cuffe S, Byrne AT, Gray SG. The IL-17-Th1/Th17 pathway: An attractive target for lung cancer therapy? Expert Opin Ther Targets (2016) 20:1339–56. doi: 10.1080/14728222.2016.1206891
28. Farhood B, Najafi M, Mortezaee K. CD8+ cytotoxic T lymphocytes in cancer immunotherapy: A review. J Cell Physiol (2019) 234:8509–21. doi: 10.1002/jcp.27782
29. Simoni Y, Becht E, Fehlings M, Loh CY, Koo SL, Teng KWW, et al. Bystander CD8+ T cells are abundant and phenotypically distinct in human tumour infiltrates. Nature (2018) 557:575–9. doi: 10.1038/s41586-018-0130-2
30. Durgeau A, Virk Y, Corgnac S, Mami-Chouaib F. Recent advances in targeting CD8 T-cell immunity for more effective cancer immunotherapy. Front Immunol (2018) 9:14. doi: 10.3389/fimmu.2018.00014
31. Kamiya M, Mizoguchi F, Kawahata K, Wang DL, Nishibori M, Day J, et al. Targeting necroptosis in muscle fibers ameliorates inflammatory myopathies. Nat Commun (2022) 13:166. doi: 10.1038/s41467-021-27875-4
32. Kursunel MA, Taskiran EZ, Tavukcuoglu E, Yanik H, Demirag F, Karaosmanoglu B, et al. Small cell lung cancer stem cells display mesenchymal properties and exploit immune checkpoint pathways in activated cytotoxic T lymphocytes. Cancer Immunol Immunother (2021) 71:445–59. doi: 10.1007/s00262-021-02998-1
33. Chen YM, Jin Y, Hu X, Chen M. Infiltrating T lymphocytes in the tumor microenvironment of small cell lung cancer: A state of knowledge review. J Cancer Res Clin Oncol (2022) 148:881–95. doi: 10.1007/s00432-021-03895-x
34. Liu Y, Zhang L, Wang BS, Yang ZJ, Xu GQ, Ma AH, et al. Requirement for POH1 in differentiation and maintenance of regulatory T cells. Cell Death Differ (2019) 26:751–62. doi: 10.1038/s41418-018-0162-z
35. Togashi Y, Shitara K, Nishikawa H. Regulatory T cells in cancer immunosuppression—Implications for anticancer therapy. Nat Rev Clin Oncol (2019) 16:356–71. doi: 10.1038/s41571-019-0175-7
36. Zhang D, Chen ZH, Wang DC, Wang XD. Regulatory T cells and potential inmmunotherapeutic targets in lung cancer. Cancer Metastasis Rev (2015) 34:277–90. doi: 10.1007/s10555-015-9566-0
37. Liang JQ, Bi GS, Shan GY, Jin X, Bian YY, Wang Q. Tumor-associated regulatory T cells in non-small-cell lung cancer: Current advances and future perspectives. J Immunol Res (2022) 2022:4355386. doi: 10.1155/2022/4355386
38. Haruna M, Ueyama A, Yamamoto Y, Hirata M, Goto K, Yoshida H, et al. The impact of CCR8+ regulatory T cells on cytotoxic T cell function in human lung cancer. Sci Rep (2022) 12:5377. doi: 10.1038/s41598-022-09458-5
39. Hodgins JJ, Khan ST, Park MM, Auer RC, Ardolino M. Killers 2.0: NK cell therapies at the forefront of cancer control. J Clin Investig (2019) 129:3499–510. doi: 10.1172/JCI129338
40. Franks SE, Wolfson B, Hodge JW. Natural born killers: NK cells in cancer therapy. Cancers (2020) 12:2131. doi: 10.3390/cancers12082131
41. Wolf NK, Kissiov DU, Raulet DH. Roles of natural killer cells in immunity to cancer, and applications to immunotherapy. Nat Rev Immunol (2023) 23:90–105. doi: 10.1038/s41577-022-00732-1
42. Cong JJ, Wang XW, Zheng XH, Wang D, Fu BQ, Sun R, et al. Dysfunction of natural killer cells by FBP1-induced inhibition of glycolysis during lung cancer progression. Cell Metab (2018) 28:243–55.e5. doi: 10.1016/j.cmet.2018.06.021
43. Li Q, Cai SN, Li MJ, Zhou XJ, Wu G, Kang K, et al. Natural killer cell exhaustion in lung cancer. Int Immunopharmacol (2021) 96:107764. doi: 10.1016/j.intimp.2021.107764
44. Aktaş ON, Öztürk AB, Erman B, Erus S, Tanju S, Dilege Ş. Role of natural killer cells in lung cancer. J Cancer Res Clin Oncol (2018) 144:997–1003. doi: 10.1007/s00432-018-2635-3
45. Zeng YQ, Lv XZ, Du J. Natural killer cell-based immunotherapy for lung cancer: Challenges and perspectives (Review). Oncol Rep (2021) 46:232. doi: 10.3892/or.2021.8183
46. Sedighzadeh SS, Khoshbin AP, Razi S, Keshavarz-Fathi M, Rezaei N. A narrative review of tumor-associated macrophages in lung cancer: Regulation of macrophage polarization and therapeutic implications. Transl Lung Cancer Res (2021) 10:1889–916. doi: 10.21037/tlcr-20-1241
47. Xu F, Wei Y, Tang Z, Liu BJ, Dong JC. Tumor-associated macrophages in lung cancer: Friend or foe? (Review). Mol Med Rep (2020) 22:4107–15. doi: 10.3892/mmr.2020.11518
48. Sarode P, Zheng X, Giotopoulou GA, Weigert A, Kuenne C, Günther S, et al. Reprogramming of tumor-associated macrophages by targeting β-catenin/FOSL2/ARID5A signaling: A potential treatment of lung cancer. Sci Adv (2020) 6:eaaz6105. doi: 10.1126/sciadv.aaz6105
49. Garrido-Martin EM, Mellows TWP, Clarke J, Ganesan AP, Wood O, Cazaly A, et al. M1hot tumor-associated macrophages boost tissue-resident memory T cells infiltration and survival in human lung cancer. J Immunother Cancer (2020) 8:e000778. doi: 10.1136/jitc-2020-000778
50. He D, Wang D, Lu P, Yang N, Xue ZG, Zhu XM, et al. Single-cell RNA sequencing reveals heterogeneous tumor and immune cell populations in early-stage lung adenocarcinomas harboring EGFR mutations. Oncogene (2020) 40:355–68. doi: 10.1038/s41388-020-01528-0
51. Zhang HF, Jiang H, Zhu LC, Li JW, Ma SL. Cancer-associated fibroblasts in non-small cell lung cancer: Recent advances and future perspectives. Cancer Lett (2021) 514:38–47. doi: 10.1016/j.canlet.2021.05.009
52. Biffi G, Tuveson DA. Diversity and biology of cancer-associated fibroblasts. Physiol Rev (2020) 101:147–76. doi: 10.1152/physrev.00048.2019
53. Chen Y, McAndrews KM, Kalluri R. Clinical and therapeutic relevance of cancer-associated fibroblasts. Nat Rev Clin Oncol (2021) 18:792–804. doi: 10.1038/s41571-021-00546-5
54. Grout JA, Sirven P, Leader AM, Maskey S, Hector E, Puisieux I, et al. Spatial positioning and matrix programs of cancer-associated fibroblasts promote T-cell exclusion in human lung tumors. Cancer Discovery (2022) 12:2606–25. doi: 10.1158/2159-8290.CD-21-1714
55. Wang S, Fan G, Li L, He Y, Lou N, Xie T, et al. Integrative analyses of bulk and single-cell RNA-seq identified cancer-associated fibroblasts-related signature as a prognostic factor for immunotherapy in NSCLC. Cancer Immunol Immunother (2023) 72:2423–42. doi: 10.1007/s00262-023-03428-0
56. Cruz-Bermúdez A, Laza-Briviesca R, Vicente-Blanco RJ, García-Grande A, Coronado MJ, Laine-Menéndez S, et al. Cancer-associated fibroblasts modify lung cancer metabolism involving ROS and TGF-β signaling. Free Radic Biol Med (2019) 130:163–73. doi: 10.1016/j.freeradbiomed.2018.10.450
57. Aizik L, Dror Y, Taussig D, Barzel A, Carmi Y, Wine Y. Antibody repertoire analysis of tumor-infiltrating B cells reveals distinct signatures and distributions across tissues. Front Immunol (2021) 12:705381. doi: 10.3389/fimmu.2021.705381
58. Germain C, Gnjatic S, Tamzalit F, Knockaert S, Remark R, Goc J, et al. Presence of B cells in tertiary lymphoid structures is associated with a protective immunity in patients with lung cancer. Am J Respir Crit Care Med (2014) 189:832–44. doi: 10.1164/rccm.201309-1611OC
59. Wang SS, Liu W, Ly D, Xu H, Qu LM, Zhang L. Tumor-infiltrating B cells: Their role and application in anti-tumor immunity in lung cancer. Cell Mol Immunol (2019) 16:6–18. doi: 10.1038/s41423-018-0027-x
60. Bruno TC, Ebner PJ, Moore BL, Squalls OG, Waugh KA, Eruslanov EB, et al. Antigen-presenting intratumoral B cells affect CD4+ TIL phenotypes in non-small cell lung cancer patients. Cancer Immunol Res (2017) 5:898–907. doi: 10.1158/2326-6066.CIR-17-0075
61. Lee YS, Radford KJ. The role of dendritic cells in cancer. Int Rev Cell Mol Biol (2019) 348:123–78. doi: 10.1016/bs.ircmb.2019.07.006
62. Stevens D, Ingels J, Van Lint S, Vandekerckhove B, Vermaelen K. Dendritic cell-based immunotherapy in lung cancer. Front Immunol (2021) 11:620374. doi: 10.3389/fimmu.2020.620374
63. Wang JB, Huang X, Li FR. Impaired dendritic cell functions in lung cancer: A review of recent advances and future perspectives. Cancer Commun (2019) 39:43. doi: 10.1186/s40880-019-0387-3
64. Mohsenzadegan M, Peng RW, Roudi R. Dendritic cell/cytokine-induced killer cell-based immunotherapy in lung cancer: What we know and future landscape. J Cell Physiol (2020) 235:74–86. doi: 10.1002/jcp.28977
65. Lichterman JN, Reddy SM. Mast cells: A new frontier for cancer immunotherapy. Cells (2021) 10:1270. doi: 10.3390/cells10061270
66. Aponte-López A, Muñoz-Cruz S.“Mast cells in the tumor microenvironment. In: Birbrair A, editor. Tumor microenvironment. Cham: Springer (2020). p. 159–73. doi: 10.1007/978-3-030-49270-0_9
67. Zhu JF, Fan Y, Xiong YL, Wang WC, Chen JK, Xia YM, et al. Delineating the dynamic evolution from preneoplasia to invasive lung adenocarcinoma by integrating single-cell RNA sequencing and spatial transcriptomics. Exp Mol Med (2022) 54:2060–76. doi: 10.1038/s12276-022-00896-9
68. Bao XW, Shi R, Zhao TY, Wang YF. Mast cell-based molecular subtypes and signature associated with clinical outcome in early-stage lung adenocarcinoma. Mol Oncol (2020) 14:917–32. doi: 10.1002/1878-0261.12670
69. Yang Y, Qian WW, Zhou J, Fan XM. A mast cell-related prognostic model for non-small cell lung cancer. J Thorac Dis (2023) 15:1948–57. doi: 10.21037/jtd-23-362
70. Jiménez-Cortegana C, Galassi C, Klapp V, Gabrilovich DI, Galluzzi L. Myeloid-derived suppressor cells and radiotherapy. Cancer Immunol Res (2022) 10:545–57. doi: 10.1158/2326-6066.CIR-21-1105
71. Dysthe M, Parihar R. Myeloid-derived suppressor cells in the tumor microenvironment. In: Birbrair A, editor. Tumor microenvironment. Cham: Springer (2020). p. 117–40. doi: 10.1007/978-3-030-35723-8_8
72. Liu YF, Han YK, Zhang YH, Lv TT, Peng XC, Huang JB. LncRNAs has been identified as regulators of Myeloid-derived suppressor cells in lung cancer. Front Immunol (2023) 14:1067520. doi: 10.3389/fimmu.2023.1067520
73. Yang ZZ, Guo JC, Weng LL, Tang WX, Jin SL, Ma W. Myeloid-derived suppressor cells-new and exciting players in lung cancer. J Hematol Oncol (2020) 13:10. doi: 10.1186/s13045-020-0843-1
74. Luo H, Vong CT, Chen HB, Gao Y, Lyu P, Qiu L, et al. Naturally occurring anti-cancer compounds: Shining from Chinese herbal medicine. Chin Med (2019) 14:48. doi: 10.1186/s13020-019-0270-9
75. Zhou XT, Wang XM, Sun Q, Zhang WF, Liu C, Ma WZ, et al. Natural compounds: A new perspective on targeting polarization and infiltration of tumor-associated macrophages in lung cancer. BioMed Pharmacother (2022) 151:113096. doi: 10.1016/j.biopha.2022.113096
76. Yao PY, Liu YJ. Terpenoids: Natural compounds for Non-Alcoholic Fatty Liver Disease (NAFLD) therapy. Molecules (2022) 28:272. doi: 10.3390/molecules28010272
77. Huang M, Lu JJ, Huang MQ, Bao JL, Chen XP, Wang YT. Terpenoids: Natural products for cancer therapy. Expert Opin Investig Drugs (2012) 21:1801–18. doi: 10.1517/13543784.2012.727395
78. Xu F, Cui WQ, Wei Y, Cui J, Qiu J, Hu LL, et al. Astragaloside IV inhibits lung cancer progression and metastasis by modulating macrophage polarization through AMPK signaling. J Exp Clin Cancer Res (2018) 37:207. doi: 10.1186/s13046-018-0878-0
79. Zhang AL, Zheng YH, Que ZJ, Zhang LL, Lin SC, Le V, et al. Astragaloside IV inhibits rogression of lung cancer by mediating immune function of Tregs and CTLs by interfering with IDO. J Cancer Res Clin Oncol (2014) 140:1883–90. doi: 10.1007/s00432-014-1744-x
80. Zhuang ZJ, Chen QY, Zhong XY, Chen HQ, Yu RJ, Tang Y. Ginsenoside Rg3, a promising agent for NSCLC patients in the pandemic: A large-scale data mining and systemic biological analysis. J Ginseng Res (2023) 47:291–301. doi: 10.1016/j.jgr.2022.09.006
81. Son KJ, Choi KR, Lee SJ, Lee H. Immunogenic cell death induced by ginsenoside Rg3: Significance in dendritic cell-based anti-tumor immunotherapy. Immune Netw (2016) 16:75–84. doi: 10.4110/in.2016.16.1.75
82. Jiang ZS, Yang YF, Yang YL, Zhang Y, Yue ZS, Pan ZY, et al. Ginsenoside Rg3 attenuates cisplatin resistance in lung cancer by downregulating PD-L1 and resuming immune. BioMed Pharmacother (2017) 96:378–83. doi: 10.1016/j.biopha.2017.09.129
83. Zhang YT, Liu YX, Wang JG, Jiang ZY, Zhang L, Cui Y, et al. Atractylenolide II inhibits tumor-associated macrophages (TAMs)-induced lung cancer cell metastasis. Immunopharmacol Immunotoxicol (2022) 44:227–37. doi: 10.1080/08923973.2022.2037629
84. Liu JB, Chen D, Bao TT, Fan FT, Yu C. The anticancer effects of atractylenolide III associate with the downregulation of Jak3/Stat3-dependent IDO expression. Front Pharmacol (2020) 10:1505. doi: 10.3389/fphar.2019.01505
85. Haustein M, Ramer R, Linnebacher M, Manda K, Hinz B. Cannabinoids increase lung cancer cell lysis by lymphokine-activated killer cells via upregulation of ICAM-1. Biochem Pharmacol (2014) 92:312–25. doi: 10.1016/j.bcp.2014.07.014
86. Huang Y, Chen Z, Wang Y, Ba X, Huang Y, Shen P, et al. Triptolide exerts an anti-tumor effect on non-small cell lung cancer cells by inhibiting activation of the IL-6/STAT3 axis. Int J Mol Med (2019) 44:291–300. doi: 10.3892/ijmm.2019.4197
87. Hwang TL, Chang CH. Oridonin enhances cytotoxic activity of natural killer cells against lung cancer. Int Immunopharmacol (2023) 122:110669. doi: 10.1016/j.intimp.2023.110669
88. Jiang XF, Wu H, Zhao W, Ding X, You Q, Zhu F, et al. Lycopene improves the efficiency of anti-PD-1 therapy via activating IFN signaling of lung cancer cells. Cancer Cell Int (2019) 19:68. doi: 10.1186/s12935-019-0789-y
89. Park HJ, Chi GY, Choi YH, Park SH. Lupeol suppresses plasminogen activator inhibitor-1-mediated macrophage recruitment and attenuates M2 macrophage polarization. Biochem Biophys Res Commun (2020) 527:889–95. doi: 10.1016/j.bbrc.2020.04.160
90. Wu Q, Chen GL, Li YJ, Chen Y, Lin FZ. Paeoniflorin inhibits macrophage-mediated lung cancer metastasis. Chin J Nat Med (2015) 13:925–32. doi: 10.1016/S1875-5364(15)30098-4
91. Lin MX, Xu Q, Luo Y, Liu GH, Hou PF. Bakuchiol inhibits lung cancer by modulating tumor microenvironment and the expression of PD-L1. J Biochem Mol Toxicol (2023) 37:e23401. doi: 10.1002/jbt.23401
92. Huang MY, Jiang XM, Xu YL, Yuan LW, Chen YC, Cui GZ, et al. Platycodin D triggers the extracellular release of programed death Ligand-1 in lung cancer cells. Food Chem Toxicol (2019) 131:110537. doi: 10.1016/j.fct.2019.05.045
93. Zhou LX, Li MP, Chai ZB, Zhang JL, Cao K, Deng L, et al. Anticancer effects and mechanisms of astragaloside-IV (Review). Oncol Rep (2023) 49:5. doi: 10.3892/or.2022.8442
94. Huang CM, Ou RZ, Chen XN, Zhang YX, Li JX, Liang YH, et al. Tumor cell-derived SPON2 promotes M2-polarized tumor-associated macrophage infiltration and cancer progression by activating PYK2 in CRC. J Exp Clin Cancer Res (2021) 40:304. doi: 10.1186/s13046-021-02108-0
95. Fujiwara Y, Kato S, Nesline MK, Conroy JM, DePietro P, Pabla S, et al. Indoleamine 2,3-dioxygenase (IDO) inhibitors and cancer immunotherapy. Cancer Treat Rev (2022) 110:102461. doi: 10.1016/j.ctrv.2022.102461
96. Gao S, Fang CC, Wang TC, Lu WJ, Wang N, Sun LF, et al. The effect of ginsenoside Rg3 combined with chemotherapy on immune function in non-small cell lung cancer: A systematic review and meta-analysis of randomized controlled trials. Medicine (2023) 102:e33463. doi: 10.1097/MD.0000000000033463
97. Huang JJ, Peng KJ, Wang LH, Wen B, Zhou L, Luo T, et al. Ginsenoside Rh2 inhibits proliferation and induces apoptosis in human leukemia cells via TNF-α signaling pathway. Acta Biochim Biophys Sin (2016) 48:750–5. doi: 10.1093/abbs/gmw049
98. Jiang Y, Guo KB, Wang PP, Zhu Y, Huang JQ, Ruan SM. The antitumor properties of atractylenolides: Molecular mechanisms and signaling pathways. BioMed Pharmacother (2022) 155:113699. doi: 10.1016/j.biopha.2022.113699
99. Deng M, Chen HJ, Long JY, Song JW, Xie L, Li XF. Atractylenolides (I, II, and III): A review of their pharmacology and pharmacokinetics. Arch Pharmacal Res (2021) 44:633–54. doi: 10.1007/s12272-021-01342-6
100. Sulé-Suso J, Watson NA, van Pittius DG, Jegannathen A. Striking lung cancer response to self-administration of cannabidiol: A case report and literature review. SAGE Open Med Case Rep (2019) 7:2050313X19832160. doi: 10.1177/2050313X19832160
101. Bui TM, Wiesolek HL, Sumagin R. ICAM-1: A master regulator of cellular responses in inflammation, injury resolution, and tumorigenesis. J Leukoc Biol (2020) 108:787–99. doi: 10.1002/JLB.2MR0220-549R
102. Sarsembayeva A, Kienzl M, Gruden E, Ristic D, Maitz K, Valadez-Cosmes P, et al. Cannabinoid receptor 2 plays a pro-tumorigenic role in non-small cell lung cancer by limiting anti-tumor activity of CD8+ T and NK cells. Front Immunol (2023) 13:997115. doi: 10.3389/fimmu.2022.997115
103. Kitamura H, Ohno Y, Toyoshima Y, Ohtake J, Homma S, Kawamura H, et al. Interleukin-6/STAT3 signaling as a promising target to improve the efficacy of cancer immunotherapy. Cancer Sci (2017) 108:1947–52. doi: 10.1111/cas.13332
104. Abdullah NA, Md Hashim NF, Ammar A, Muhamad Zakuan N. An insight into the anti-angiogenic and anti-metastatic effects of oridonin: Current knowledge and future potential. Molecules (2021) 26:775. doi: 10.3390/molecules26040775
105. Puah BP, Jalil J, Attiq A, Kamisah Y. New insights into molecular mechanism behind anti-cancer activities of lycopene. Molecules (2021) 26:3888. doi: 10.3390/molecules26133888
106. Gunasekaran M, Ravi R, Subramanian K. Molecular docking analysis of lupeol with different cancer targets. Bioinformation (2022) 18:134–40. doi: 10.6026/97320630018134
107. Wang XZ, Xia L, Zhang XY, Chen Q, Li X, Mou Y, et al. The multifaceted mechanisms of Paeoniflorin in the treatment of tumors: State-of-the-Art. BioMed Pharmacother (2022) 149:112800. doi: 10.1016/j.biopha.2022.112800
108. Chopra B, Dhingra AK, Grewal AS, Jain A, Chaudhary J, Grover P. Bakuchiol: A potential anticancer compound from Psoralea corylifolia linn. Anti-Cancer Agents Med Chem (2023) 23:989–98. doi: 10.2174/1871520623666230130102910
109. Yang RJ, Pei TL, Huang RF, Xiao Y, Yan JN, Zhu JL, et al. Platycodon grandiflorum triggers antitumor immunity by restricting PD-1 expression of CD8+ T cells in local tumor microenvironment. Front Pharmacol (2022) 13:774440. doi: 10.3389/fphar.2022.774440
110. Shen N, Wang TF, Gan Q, Liu S, Wang L, Jin B. Plant flavonoids: Classification, distribution, biosynthesis, and antioxidant activity. Food Chem (2022) 383:132531. doi: 10.1016/j.foodchem.2022.132531
111. Zanoaga O, Braicu C, Jurj A, Rusu A, Buiga R, Berindan-Neagoe I. Progress in research on the role of flavonoids in lung cancer. Int J Mol Sci (2019) 20:4291. doi: 10.3390/ijms20174291
112. Sun Q, Liu Q, Zhou XT, Wang XM, Li HY, Zhang WF, et al. Flavonoids regulate tumor-associated macrophages—From structure-activity relationship to clinical potential (Review). Pharmacol Res (2022) 184:106419. doi: 10.1016/j.phrs.2022.106419
113. Yao C, Ni ZY, Gong CY, Zhu XW, Wang LX, Xu ZH, et al. Rocaglamide enhances NK cell-mediated killing of non-small cell lung cancer cells by inhibiting autophagy. Autophagy (2018) 14:1831–44. doi: 10.1080/15548627.2018.1489946
114. Yan XW, Yao C, Fang C, Han M, Gong CY, Hu D, et al. Rocaglamide promotes the infiltration and antitumor immunity of NK cells by activating cGAS-STING signaling in non-small cell lung cancer. Int J Biol Sci (2022) 18:585–98. doi: 10.7150/ijbs.65019
115. Kang HG, Zhang J, Wang BZ, Liu MR, Zhao J, Yang MX, et al. Puerarin inhibits M2 polarization and metastasis of tumor-associated macrophages from NSCLC xenograft model via inactivating MEK/ERK 1/2 pathway. Int J Oncol (2017) 50:545–54. doi: 10.3892/ijo.2017.3841
116. Sp N, Kang DY, Lee JM, Jang KJ. Mechanistic insights of anti-immune evasion by nobiletin through regulating miR-197/STAT3/PD-L1 signaling in Non-Small Cell Lung Cancer (NSCLC) cells. Int J Mol Sci (2021) 22:9843. doi: 10.3390/ijms22189843
117. Rawangkan A, Wongsirisin P, Namiki K, Iida K, Kobayashi Y, Shimizu Y, et al. Green tea catechin is an alternative immune checkpoint inhibitor that inhibits PD-L1 expression and lung tumor growth. Molecules (2018) 23:2071. doi: 10.3390/molecules23082071
118. Chen YC, He XL, Qi L, Shi W, Yuan LW, Huang MY, et al. Myricetin inhibits interferon-γ-induced PD-L1 and IDO1 expression in lung cancer cells. Biochem Pharmacol (2022) 197:114940. doi: 10.1016/j.bcp.2022.114940
119. Yuan LW, Jiang XM, Xu YL, Huang MY, Chen YC, Yu WB, et al. Licochalcone A inhibits interferon-gamma-induced programmed death-ligand 1 in lung cancer cells. Phytomedicine (2021) 80:153394. doi: 10.1016/j.phymed.2020.153394
120. Cuyàs E, Pérez-Sánchez A, Micol V, Menendez JA, Bosch-Barrera J. STAT3-targeted treatment with silibinin overcomes the acquired resistance to crizotinib in ALK-rearranged lung cancer. Cell Cycle (2016) 15:3413–8. doi: 10.1080/15384101.2016.1245249
121. Chen RL, Wang Z, Huang P, Sun CH, Yu WY, Zhang HH, et al. Isovitexin potentiated the antitumor activity of cisplatin by inhibiting the glucose metabolism of lung cancer cells and reduced cisplatin-induced immunotoxicity in mice. Int Immunopharmacol (2021) 94:107357. doi: 10.1016/j.intimp.2020.107357
122. Jiang ZB, Wang WJ, Xu C, Xie YJ, Wang XR, Zhang YZ, et al. Luteolin and its derivative apigenin suppress the inducible PD-L1 expression to improve anti-tumor immunity in KRAS-mutant lung cancer. Cancer Lett (2021) 515:36–48. doi: 10.1016/j.canlet.2021.05.019
123. Dai C, Zhou X, Wang L, Tan RM, Wang W, Yang B, et al. Rocaglamide prolonged allograft survival by inhibiting differentiation of Th1/Th17 cells in cardiac transplantation. Oxid Med Cell Longev (2022) 2022:2048095. doi: 10.1155/2022/2048095
124. Pan L, Woodard JL, Lucas DM, Fuchs JR, Kinghorn AD. Rocaglamide, silvestrol and structurally related bioactive compounds from Aglaia species. Nat Prod Rep (2014) 31:924–39. doi: 10.1039/c4np00006d
125. Wang D, Bu T, Li YQ, He YY, Yang F, Zou L. Pharmacological activity, pharmacokinetics, and clinical research progress of puerarin. Antioxidants (2022) 11:2121. doi: 10.3390/antiox11112121
126. Ashrafizadeh M, Zarrabi A, Saberifar S, Hashemi F, Hushmandi K, Hashemi F, et al. Nobiletin in cancer therapy: How this plant derived-natural compound targets various oncogene and onco-suppressor pathways. Biomedicines (2020) 8:110. doi: 10.3390/biomedicines8050110
127. Almatroodi SA, Almatroudi A, Khan AA, Alhumaydhi FA, Alsahli MA, Rahmani AH. Potential therapeutic targets of Epigallocatechin Gallate (EGCG), the most abundant catechin in green tea, and its role in the therapy of various types of cancer. Molecules (2020) 25:3146. doi: 10.3390/molecules25143146
128. Imran M, Saeed F, Hussain G, Imran A, Mehmood Z, Gondal TA, et al. Myricetin: A comprehensive review on its biological potentials. Food Sci Nutr (2021) 9:5854–68. doi: 10.1002/fsn3.2513
129. Boedtkjer E, Pedersen SF. The acidic tumor microenvironment as a driver of cancer. Annu Rev Physiol (2020) 82:103–26. doi: 10.1146/annurev-physiol-021119-034627
130. Mondal A, Gandhi A, Fimognari C, Atanasov AG, Bishayee A. Alkaloids for cancer prevention and therapy: Current progress and future perspectives. Eur J Pharmacol (2019) 858:172472. doi: 10.1016/j.ejphar.2019.172472
131. Wang JK, Zhao BS, Wang M, Liu CY, Li YQ, Ma QT, et al. Anti-tumor and phenotypic regulation effect of matrine on dendritic cells through regulating TLRs pathway. Chin J Integr Med (2021) 27:520–6. doi: 10.1007/s11655-020-3433-8
132. Zhao B, Hui XD, Wang J, Zeng HR, Yan Y, Hu Q, et al. Matrine suppresses lung cancer metastasis via targeting M2-like tumour-associated-macrophages polarization. Am J Cancer Res (2021) 11:4308–28.
133. Li B, Luo YB, Zhou YX, Wu JC, Fang ZH, Li Y. Role of sanguinarine in regulating immunosuppression in a Lewis lung cancer mouse model. Int Immunopharmacol (2022) 110:108964. doi: 10.1016/j.intimp.2022.108964
134. Cui YJ, Luo YB, Qian QH, Tian J, Fang Z, Wang X, et al. Sanguinarine regulates tumor-associated macrophages to prevent lung cancer angiogenesis through the WNT/β-catenin pathway. Front Oncol (2022) 12:732860. doi: 10.3389/fonc.2022.732860
135. Weng TY, Wu HF, Li CY, Hung YH, Chang YW, Chen YL, et al. Homoharringtonine induced immune alteration for an Efficient Anti-tumor Response in Mouse Models of Non-small Cell Lung Adenocarcinoma Expressing Kras Mutation. Sci Rep (2018) 8:8216. doi: 10.1038/s41598-018-26454-w
136. Liu H, Zou MM, Li P, Wang HF, Lin XJ, Ye J. Oxymatrine-mediated maturation of dendritic cells leads to activation of FOXP3+/CD4+ Treg cells and reversal of cisplatin-resistance in lung cancer cells. Mol Med Rep (2019) 19:4081–90. doi: 10.3892/mmr.2019.10064
137. Wang QC, Liu ZF, Tang SH, Wu Z. Morphine suppresses the immune function of lung cancer by up-regulating MAEL expression. BMC Pharmacol Toxicol (2022) 23:92. doi: 10.1186/s40360-022-00632-z
138. Jiang ZB, Huang JM, Xie YJ, Zhang YZ, Chang C, Lai HL, et al. Evodiamine suppresses non-small cell lung cancer by elevating CD8+ T cells and downregulating the MUC1-C/PD-L1 axis. J Exp Clin Cancer Res (2020) 39:249. doi: 10.1186/s13046-020-01741-5
139. Chen S, Ma SX, Wang HQ, Shao XX, Ding BJ, Guo ZH, et al. Unraveling the mechanism of alkaloids from Sophora alopecuroides Linn combined with immune checkpoint blockade in the treatment of non-small cell lung cancer based on systems pharmacology. Bioorganic Med Chem (2022) 64:116724. doi: 10.1016/j.bmc.2022.116724
140. Rashid HU, Xu YM, Muhammad Y, Wang LS, Jiang J. Research advances on anticancer activities of matrine and its derivatives: An updated overview. Eur J Med Chem (2019) 161:205–38. doi: 10.1016/j.ejmech.2018.10.037
141. Lou GH, Wang J, Hu J, Gan QX, Peng CY, Xiong HJ, et al. Sanguinarine: A double-edged sword of anticancer and carcinogenesis and its future application prospect. Anti-Cancer Agents Med Chem (2021) 21:2100–10. doi: 10.2174/1871520621666210126091512
142. Kang JS, Lee J, Nam LB, Yoo OK, Pham KT, Duong TH, et al. Homoharringtonine stabilizes secondary structure of guanine-rich sequence existing in the 5’-untranslated region of Nrf2. Bioorganic Med Chem Lett (2019) 29:2189–96. doi: 10.1016/j.bmcl.2019.06.049
143. Huan DQ, Hop NQ, Son NT. Oxymatrine: A current overview of its health benefits. Fitoterapia (2023) 168:105565. doi: 10.1016/j.fitote.2023.105565
144. Krishnamurti C, Rao SC. The isolation of morphine by Serturner. Indian J Anaesth (2016) 60:861–2. doi: 10.4103/0019-5049.193696
145. Lu XM, Zhang WJ, Liu Y, Liu MM. Evodiamine exerts inhibitory roles in non-small cell lung cancer cell A549 and its sub-population of stem-like cells. Exp Ther Med (2022) 24:746. doi: 10.3892/etm.2022.11682
146. Liu D, You M, Xu YJ, Li FL, Zhang DY, Li XJ, et al. Inhibition of curcumin on myeloid-derived suppressor cells is requisite for controlling lung cancer. Int Immunopharmacol (2016) 39:265–72. doi: 10.1016/j.intimp.2016.07.035
147. Zou JY, Su CH, Luo HH, Lei YY, Zeng B, Zhu HS, et al. Curcumin converts Foxp3+ regulatory T cells to T helper 1 cells in patients with lung cancer. J Cell Biochem (2018) 119:1420–8. doi: 10.1002/jcb.26302
148. Sun LW, Chen BN, Jiang R, Li JD, Wang B. Resveratrol inhibits lung cancer growth by suppressing M2-like polarization of tumor associated macrophages. Cell Immunol (2017) 311:86–93. doi: 10.1016/j.cellimm.2016.11.002
149. Yang MM, Li ZY, Tao JP, Hu H, Li ZL, Zhang ZJ, et al. Resveratrol induces PD-L1 expression through snail-driven activation of Wnt pathway in lung cancer cells. J Cancer Res Clin Oncol (2021) 147:1101–13. doi: 10.1007/s00432-021-03510-z
150. Guo JM, Ding W, Cai SL, Ren P, Chen FX, Wang JW, et al. Polydatin radiosensitizes lung cancer while preventing radiation injuries by modulating tumor-infiltrating B cells. J Cancer Res Clin Oncol (2023) 149:9529–42. doi: 10.1007/s00432-023-04762-7
151. Jiang ZB, Xu C, Wang WJ, Zhang YZ, Huang JM, Xie YJ, et al. Plumbagin suppresses non-small cell lung cancer progression through downregulating ARF1 and by elevating CD8+ T cells. Pharmacol Res (2021) 169:105656. doi: 10.1016/j.phrs.2021.105656
152. Khalil R, Green RJ, Sivakumar K, Varandani P, Bharadwaj S, Mohapatra SS, et al. Withaferin a increases the effectiveness of immune checkpoint blocker for the treatment of non-small cell lung cancer. Cancers (2023) 15:3089. doi: 10.3390/cancers15123089
153. Jin GL, Ma M, Yang CY, Zhen L, Feng MK. Salidroside suppresses the multiple oncogenic activates and immune escape of lung adenocarcinoma through the circ_0009624-mediated PD-L1 pathway. Thorac Cancer (2023) 14:2493–503. doi: 10.1111/1759-7714.15034
154. Zhang HQ, Xie XF, Li GM, Chen JR, Li MT, Xu X, et al. Erianin inhibits human lung cancer cell growth via PI3K/Akt/mTOR pathway in vitro and in vivo. Phytother Res (2021) 35:4511–25. doi: 10.1002/ptr.7154
155. Lelli D, Pedone C, Majeed M, Sahebkar A. Curcumin and lung cancer: The role of microRNAs. Curr Pharm Des (2017) 23:3440–4. doi: 10.2174/1381612823666170109144818
156. Luo FF, Song X, Zhang Y, Chu YW. Low-dose curcumin leads to the inhibition of tumor growth via enhancing CTL-mediated antitumor immunity. Int Immunopharmacol (2011) 11:1234–40. doi: 10.1016/j.intimp.2011.04.002
157. Kaur A, Tiwari R, Tiwari G, Ramachandran V. Resveratrol: A vital therapeutic agent with multiple health benefits. Drug Res (2022) 72:5–17. doi: 10.1055/a-1555-2919
158. Gao S, Kang MC, Wang JZ, Zhao R, Zhang GJ, Zheng J, et al. Resveratrol improves the cytotoxic effect of CD8 +T cells in the tumor microenvironment by regulating HMMR/Ferroptosis in lung squamous cell carcinoma. J Pharm BioMed Anal (2023) 229:115346. doi: 10.1016/j.jpba.2023.115346
159. Zou J, Yang Y, Yang Y, Liu XR. Polydatin suppresses proliferation and metastasis of non-small cell lung cancer cells by inhibiting NLRP3 inflammasome activation via NF-κB pathway. BioMed Pharmacother (2018) 108:130–6. doi: 10.1016/j.biopha.2018.09.051
160. Panichayupakaranant P, Ahmad MI. Plumbagin and its role in chronic diseases. In: Gupta SC, Prasad S, Aggarwal BB, editors. Drug discovery from mother nature, vol. . p . Cham: Springer (2016). p. 229–46. doi: 10.1007/978-3-319-41342-6_10
161. Chirumamilla CS, Pérez-Novo C, Van Ostade X, Vanden Berghe W. Molecular insights into cancer therapeutic effects of the dietary medicinal phytochemical withaferin A. Proc Nutr Soc (2017) 76:96–105. doi: 10.1017/S0029665116002937
162. Zhang XM, Xie L, Long JY, Xie QX, Zheng Y, Liu K, et al. Salidroside: A review of its recent advances in synthetic pathways and pharmacological properties. Chem Interact (2021) 339:109268. doi: 10.1016/j.cbi.2020.109268
163. Chen P, Wu QB, Feng J, Yan LL, Sun YT, Liu SP, et al. Erianin, a novel dibenzyl compound in Dendrobium extract, inhibits lung cancer cell growth and migration via calcium/calmodulin-dependent ferroptosis. Signal Transduct Target Ther (2020) 5:51. doi: 10.1038/s41392-020-0149-3
164. Aldarouish M, Wang C. Trends and advances in tumor immunology and lung cancer immunotherapy. J Exp Clin Cancer Res (2016) 35:157. doi: 10.1186/s13046-016-0439-3
165. Kinoshita T, Terai H, Yaguchi T. Clinical efficacy and future prospects of immunotherapy in lung cancer. Life (2021) 11:1029. doi: 10.3390/life11101029
166. Wang S, Long S, Deng Z, Wu W. Positive role of chinese herbal medicine in cancer immune regulation. Am J Chin Med (2020) 48:1577–92. doi: 10.1142/S0192415X20500780
167. Zhang Y, Lou Y, Wang J, Yu C, Shen W. Research status and molecular mechanism of the traditional chinese medicine and antitumor therapy combined strategy based on tumor microenvironment. Front Immunol (2021) 11:609705. doi: 10.3389/fimmu.2020.609705
168. Stribbling SM, Ryan AJ. The cell-line-derived subcutaneous tumor model in preclinical cancer research. Nat Protoc (2022) 17:2108–28. doi: 10.1038/s41596-022-00709-3
169. Yu LN, Jin Y, Song MJ, Zhao Y, Zhang HQ. When natural compounds meet nanotechnology: Nature-inspired nanomedicines for cancer immunotherapy. Pharmaceutics (2022) 14:1589. doi: 10.3390/pharmaceutics14081589
170. Ai L, Xu A, Xu J. Roles of PD-1/PD-L1 pathway: signaling, cancer, and beyond. Adv Exp Med Biol (2020) 1248:33–59. doi: 10.1007/978-981-15-3266-5_3
171. Han YY, Liu DD, Li LH. PD-1/PD-L1 pathway: Current researches in cancer. Am J Cancer Res (2020) 10:727–42.
172. Shi L, Chen SH, Yang LJ, Li YQ. The role of PD-1 and PD-L1 in T-cell immune suppression in patients with hematological Malignancies. J Hematol Oncol (2013) 6:74. doi: 10.1186/1756-8722-6-74
173. Ivashkiv LB. IFNγ: Signalling, epigenetics and roles in immunity, metabolism, disease and cancer immunotherapy. Nat Rev Immunol (2018) 18:545–58. doi: 10.1038/s41577-018-0029-z
174. Gao Y, Yang J, Cai Y, Fu S, Zhang N, Fu X, et al. IFN-γ-mediated inhibition of lung cancer correlates with PD-L1 expression and is regulated by PI3K-AKT signaling. Int J Cancer (2018) 143(4):931–43. doi: 10.1002/ijc.31357
175. Huang H, Yu H, Li X, Li Y, Zhu G, Su L, et al. Genomic analysis of TNF-related genes with prognosis and characterization of the tumor immune microenvironment in lung adenocarcinoma. Front Immunol (2022) 13:993890. doi: 10.3389/fimmu.2022.993890
Keywords: natural compounds, lung cancer, tumor immune microenvironments, immune cells, immune cytokines, review
Citation: Yao P, Liang S, Liu Z and Xu C (2024) A review of natural products targeting tumor immune microenvironments for the treatment of lung cancer. Front. Immunol. 15:1343316. doi: 10.3389/fimmu.2024.1343316
Received: 23 November 2023; Accepted: 18 January 2024;
Published: 01 February 2024.
Edited by:
Yuan Li, Shandong University, ChinaReviewed by:
Zebo Jiang, Macau University of Science and Technology, ChinaXingkang Wu, Shanxi University, China
Junyan Wang, Guangzhou University of Chinese Medicine, China
Copyright © 2024 Yao, Liang, Liu and Xu. This is an open-access article distributed under the terms of the Creative Commons Attribution License (CC BY). The use, distribution or reproduction in other forums is permitted, provided the original author(s) and the copyright owner(s) are credited and that the original publication in this journal is cited, in accordance with accepted academic practice. No use, distribution or reproduction is permitted which does not comply with these terms.
*Correspondence: Su Liang, hongyu94@sohu.com; Cuiping Xu, xucuiping775@sohu.com