- 1Department of Children Rehabilitation, Key Laboratory of Rehabilitation Medicine in Henan, The Fifth Affiliated Hospital of Zhengzhou University, Zhengzhou, Henan, China
- 2The American Psychiatric Association, Key Laboratory of Helicobacter pylori, Microbiota and Gastrointestinal Cancer of Henan Province, Marshall Medical Research Center, Fifth Affiliated Hospital of Zhengzhou University, Zhengzhou, China
Autism spectrum disorder (ASD) encompasses a range of neurodevelopmental conditions characterized by enduring impairments in social communication and interaction together with restricted repetitive behaviors, interests, and activities. No targeted pharmacological or physical interventions are currently available for ASD. However, emerging evidence has indicated a potential association between the development of ASD and dysregulation of the gut-brain axis. Repetitive transcranial magnetic stimulation (rTMS), a noninvasive diagnostic and therapeutic approach, has demonstrated positive outcomes in diverse psychiatric disorders; however, its efficacy in treating ASD and its accompanying gastrointestinal effects, particularly the effects on the gut–brain axis, remain unclear. Hence, this review aimed to thoroughly examine the existing research on the application of rTMS in the treatment of ASD. Additionally, the review explored the interplay between rTMS and the gut microbiota in children with ASD, focusing on the gut-brain axis. Furthermore, the review delved into the integration of rTMS and gut microbiota modulation as a targeted approach for ASD treatment based on recent literature. This review emphasizes the potential synergistic effects of rTMS and gut microbiota interventions, describes the underlying mechanisms, and proposes a potential therapeutic strategy for specific subsets of individuals with ASD.
1 Introduction
Autism spectrum disorder (ASD) is a profound neurodevelopmental condition that manifests in infants and young children (1). It is characterized by deficits in social and communication skills and a tendency to engage in repetitive behaviors (2). According to the Global Burden of Disease, Injury and Risk Factor Study 2016, the global prevalence of ASD is estimated at 62.2 million people (3). Moreover, its incidence has evidently increased over time (4). Consequently, it is imperative to prioritize theoretical investigations on and clinical interventions for ASD.
ASD is associated with a wide variety of comorbid conditions such as epilepsy, anxiety, melancholy, Tourette syndrome, and gastrointestinal (GI) disorders (5–7). Among the comorbidities commonly observed in children with ASD, GI issues, including stomach pain, constipation, and diarrhea, have been reported to affect 9%-70% or more individuals (8). The etiology of these GI issues might be associated with alterations in gut microorganisms. In recent years, there has been growing interest in investigating the role of the gut-brain axis in ASD, with studies demonstrating changes in the composition and function of gut microbiota in individuals with ASD. The gut-brain axis refers to the two-way communication between the central nervous system and the individual at large. A recent study indicated that changes in the gut microbiota can affect brain function and development (9). Meanwhile, repetitive transcranial magnetic stimulation (rTMS) has emerged as a noninvasive method of brain stimulation with the potential to ameliorate ASD-related symptoms (10). Research has indicated that rTMS significantly influences the composition and functionality of the gut microbiota (11). Consequently, comprehending the correlation and interplay between rTMS and the gut microbiota is of particular significance for ASD treatment. This article reviews the current literature on the role of rTMS in ASD treatment, underlining its effects on ASD-related gastrointestinal symptoms and exploring potential future implications in ASD treatment.
2 Role of rTMS in ASD processing
ASD affects approximately 1 in 59 children, but there are currently no biological treatments that address its underlying symptoms (12). According to preliminary research, rTMS can potentially alleviate the challenges faced by individuals with ASD (13). rTMS is a noninvasive brain stimulation method used to alter brain activity (14). In particular, rTMS under different stimulation modes can lead to long-term changes in activity in target brain regions that possibly outlast the effects of stimulation (15). Substantial research supports the potential efficacy of rTMS in treating the core symptoms of ASD (16).
Several previous studies have identified transcranial direct current stimulation (tDCS) as a promising therapeutic tool for modulating synaptic plasticity abnormalities and minimizing memory and learning deficits in many animal models of neuropsychiatric diseases. The accumulated studies suggest that tDCS modulates brain plasticity via synaptic modifications within the stimulated area. In these studies, changes in plasticity-related mechanisms were achieved through the induction of long-term potentiation (LTP) and upregulation of neuroplasticity-related proteins, such as c-Fos, brain-derived neurotrophic factor (BDNF), or N-methyl-D-aspartate receptors (NMDARs) (17). Moreover, three sessions of tDCS conducted at 1-3-week intervals significantly reduced the levels of several inflammatory cytokines in the brains of healthy rats. The tDCS-mediated reductions in inflammatory cytokine levels highlight its potential use as a countermeasure against inflammation and support the hypothesis that cytokines contribute to the modulation of synaptic plasticity (18). Excitation/inhibition (E/I) imbalance remains a widely discussed hypothesis in ASD. A previous study provided evidence in favor of the excitation/inhibition imbalance hypothesis in ASD and related neurodevelopmental disorders while revealing that its nature is region-specific with distinct pre- and postsynaptic mechanisms. In the hippocampus, disproportionate expression of gamma-aminobutyric acid (GABA)-A receptor dominates, whereas excessive GABA/glutamate ratios are the hallmark of changes in the prefrontal cortex and striatum (19).
2.1 rTMS could acted as an investigational tool in ASD
The ideal stimulation settings must be established to maximize the effectiveness and safety of rTMS for ASD treatment. Passing magnetic pulses, stimulating strength, pulse frequency, and interval time are variables in the stimulation process (20). The best stimulus environment that leads to neurological changes in the human body is the basis of the current rTMS program (21). Various TMS paradigms have been developed, including single-pulse TMS, pairing-pulse TMS, and rTMS, to improve lateral network excitation, inhibitory control, and plasticity. These techniques have been used to study neurological ASD, especially in individuals without intellectual barriers (Table 1) (15). The influence of rTMS on the brain and behavior is affected by factors such as the position, strength, frequency, quantity, and duration of stimulation and the specific pathophysiology of the disease being treated (28). The dorsolateral prefrontal cortex is a target region for stimulation to improve irritability, repetitive behaviors, and executive functioning (29). The main symptoms and supplementation of the sports cortex are also designed to improve exercise movement (30). The frontal pelt layer of the inner side improves intelligence, and simulation of the frontal cortex improves voice generation and the hand-eye coordination (31).
The Food and Drug Administration has approved two separate TMS devices, including high- and low-frequency rTMS systems, for the treatment of ASD (32). Both high-frequency (10-20 Hz) and low-frequency (1-5 Hz) rTMS devices have been examined for possible therapeutic effects against ASD. However, the differential frequency of rTMS resulted in different effects on the direction of long-term adaptation in neural excitability. rTMS at 20 Hz bilaterally increased regional cerebral blood flow in the frontal cortex, and surprisingly, 1-Hz rTMS decreased regional cerebral blood flow only in the contralateral prefrontal cortex (33). This raises the possibility of therapeutic applications that selectively activate or inhibit specific areas of the brain in different neuropsychiatric syndromes using this noninvasive procedure. Low- or high-frequency rTMS can be differentially used to reregulate dysfunctional circuits associated with ASD. To reduce ASD-associated excitement, low frequencies are applied to the left prefrontal cortex. This paradigm is used to study irritability and repeated behavior in patients with ASD, which has significantly improved, resulting in the expected defects of cortical suppression (34). Other studies used low-frequency rTMS to modify the operation of various lateral networks in the prefrontal cortex (35). In other studies, different lateral networks in the frontal cortex were differentially affected by low-frequency rTMS (36). Fecteau found that the 1-Hz rTMS can improve naming skills in individuals with ASD (37). When the object is named the left pars opercularis, it is enhanced when the left pars triangularis is applied. Enticott et al. observed improvement of movement-related cortical potentials following rTMS. A single cycle of stimulation of the primary motor cortex and supplementary motor area resulted in increased activity in these areas in subjects with ASD compared to the effects of sham treatment. There was no obvious change in exercise (38). To improve the excitement of the invalid pelt area and the ASD connection, some studies have adopted high-frequency rTMS technology. In double-blind, randomized, placebo-controlled trials, Enticott et al. provided rTMS or sham stimulation to the lateral prefrontal cortex (38) and found no change in the interpersonal response index but found significant improvement in the Ritvo Autism Asperger Diagnostic Scale (RAADS). Panerai et al. utilized high-frequency (8 Hz) stimulation in children with ASD and intellectual difficulties (39) and achieved significant improvement in eye coordination through providing training in behavior hand-fusion.
2.2 rTMS could acted as a therapeutic intervention in ASD
In recent years, guidelines for evaluating the therapeutic efficacy of rTMS in a variety of diseases have been published (Table 2). Four different types of studies were listed. A prospective, randomized, placebo-controlled clinical trial with concealed outcome assessment and a sample size of at least 25 actively treated patients was considered Class I if the following criteria were met: (a) well-explained dropouts and a sufficiently low number of crossovers to have a minimal likelihood of bias, (b) well-specified exclusion/inclusion criteria, (c) well-defined primary outcome, (d) appropriate consideration of dropouts and crossovers, and (e) the use of correlation analysis. Studies with smaller sample sizes (10-25) or randomized, placebo-controlled studies that did not meet at least one of the aforementioned criteria were considered type II studies. Category III included all other controlled trials. Type IV included uncontrolled studies, case series, and case reports. Although rTMS was the focus of 15 studies and case reports, most of these studies were categorized into Class III or IV. Consequently, the use of rTMS in ASD treatment was classified as Level C (possibly effective) (44).
Many knowledge gaps remain regarding the use of TMS for ASD treatment, including the stimulus intensity, optimal stimulus settings and application positions, possible clinical goals, and standards for selecting participants. Only a small number of clinical trials (n=4), three of which were randomized, double-blind, placebo-controlled studies (ClinicalTrials.gov IDs NCT02311751, NCT01388179, and NCT00808782), used rTMS as an intervention for different ASD symptom objectives. Before the off-label clinical use of rTMS in the treatment of ASD without an experimental device exemption and outside of an IRB-sanctioned research study can be considered, additional well-controlled trials with adequate power are needed.
3 Relationship between the gut microbiota and ASD
Numerous studies have revealed that GI issues, such as diarrhea, constipation, and abdominal pain, are common comorbidities in children with ASD and that they are associated with changes in the composition and diversity of the gut microbiota in those with ASD compared to typically developing individuals (Figure 1).
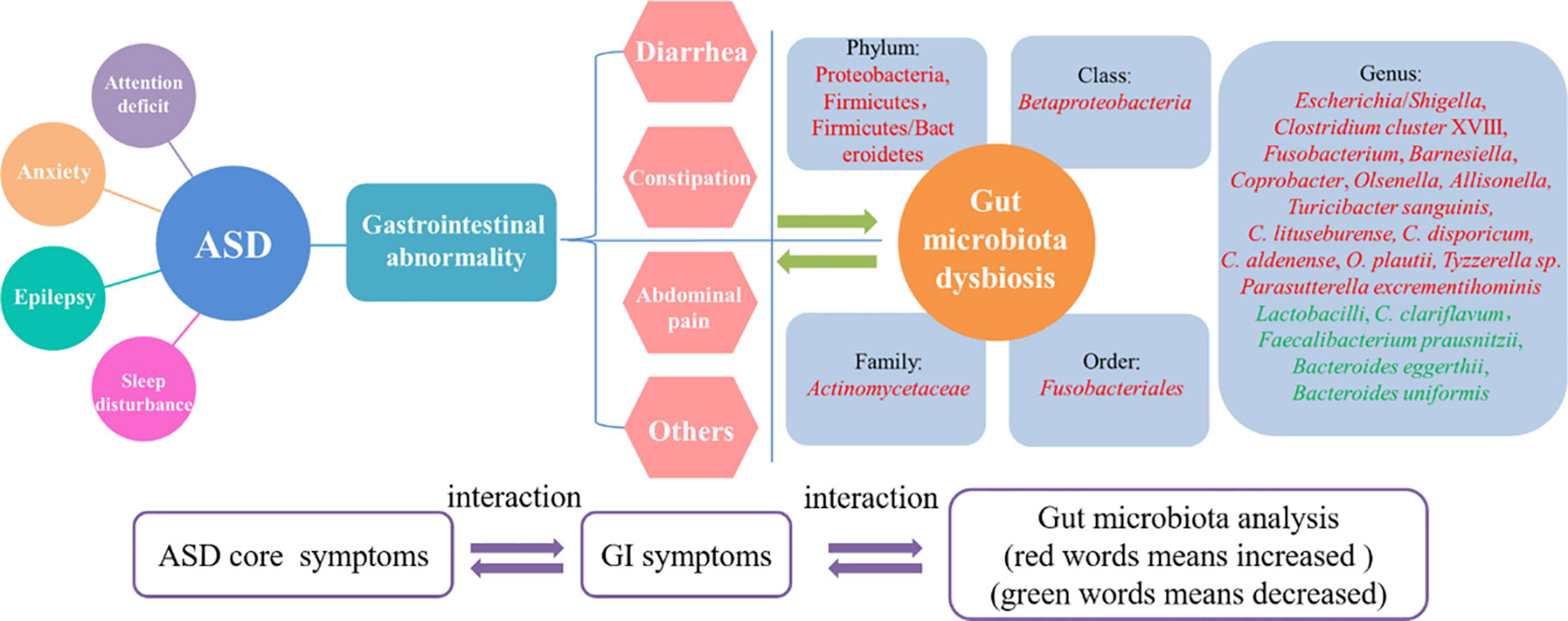
Figure 1 The interrelationship between core symptoms of ASD, gastrointestinal abnormities and gut microbiota dysbiosis. Gastrointestinal abnormities was recognized as one of the core symptoms of ASD as well as anxiety, epilepsy and attention deficit. The mainly features of the gastrointestinal abnormities including diarrhea, constipation and abdominal pain. All these features could resulted in gut microbiota dysbiosis, which showed some pathogenic genus like Shigella and Clostridium cluster XVIII was increased and some beneficial genus like Lactobacilli and Bacteroides eggerthii was decreased. The three parts of core symptoms, gastrointestinal symptoms and gut microbiota changes was interacted and mutual influence in ASD.
3.1 Gastrointestinal abnormality correlated with symptom severity of ASD
The occurrence of certain behavior problems is typically used to diagnose GI diseases (45). According to universal reports, there is a great correlation between the severity of ASD symptoms as measured by the Autism Treatment Evaluation Checklist and GI symptoms as measured by the 6-item Gastrointestinal Severity Index (46). The most common GI symptom in children with autism is constipation (47). Abdominal discomfort is significantly related to the severity of ASD core symptoms (48). The aforementioned research assumes that there is an association between GI tract abnormalities and behavior in ASD (49). Additionally, the abnormal GI tract is related to other ASD complications, including sleep problems, strange moods, and social deficiencies. GI comorbidities were found to be associated with sleep problems; mood abnormalities; argumentativeness; oppositional, defiant, or disruptive behavior; anxiety; sensory reactivity; rigidity; obsessive-compulsive behavior; self-mutilation, aggression; lack of expressive language; and social impairment (50).
3.2 Gut microbiota dysbiosis is associated with ASD-related gastrointestinal symptoms
Numerous studies revealed that the gut microbiota of children with ASD who experience constipation is significantly different from that of typically developing children. Substantial evidence has showed ASD children with constipation have higher relative abundances of Escherichia/Shigella and Clostridium cluster XVIII (51), the order Fusobacteriales, the family Actinomycetaceae, and the genera Fusobacterium, Barnesiella, Coprobacter, Olsenella and Allisonella (52), as well as lower Faecalibacterium prausnitzii, Bacteroides eggerthii, Bacteroides uniformis, Oscillospiraplautii, and Clostridium clariflavum amount (51). Additionally, although chronic constipation in healthy children is related to fatigue, low lactate levels (53) could explain constipation in patients with ASD (54). In the feces of patients with ASD and allergy, the relative abundance of Proteobacteria related to autoimmune diseases is higher (55). Furthermore, an increase in the rate of food allergies has been linked to the ratio of corporate/sterilization. There is an association between ileum and cecum richest company and cecal Proteobacteria counts (56). Accumulating evidence has revealed a relationship among feces, immune function, and the corporate/fungus ratio in children with ASD (55). Among children with ASD who report stomach discomfort, Clostridium aldenense and O. plautii counts are elevated. Some bacteria, such as C. aldenense and O. plautii, are related to ASD as well as constipation and other GI symptoms (57). Interestingly, among some young individuals with ASD, a Sanguinis related to GI issues was found (58). Parracho et al. proved that children with ASD exhibited higher levels of tissue-soluble toxin producers in their feces, although their levels were not higher than those observed in their healthy siblings (59). High-level scatterro bacteria is closely related to GI problems in individuals with ASD.
3.3 Gut microbiota dysbiosis leads to immune system dysregulation in ASD
Gut microbiota dysbiosis in ASD might contribute to immune dysregulation, GI symptoms, and abnormal neurotransmitter metabolism (60). Immune system abnormalities are often caused by gut microbiota dysbiosis in autism (61). A functioning immune system releases chemokines and cytokines including interleukin (IL)-1, IL-6, interferon, and tumor necrosis factor, which can cross the blood-brain barrier. These mediators bind to endothelial cells in the brain and induce immune responses (62). A previous study revealed that the plasma levels of IL-1, IL-6, and IL-8 were considerably higher in patients with ASD than in typically developing controls (63). Additionally, approximately 80% of the immune system is located in and around the gut mucosa (64).
Moreover, the gut microbiota alters brain functioning mostly via its special metabolites. Patients with ASD exhibit increased levels of metabolites including short-chain fatty acids (SCFAs), p-cresol, and ammonia in serum, urine, and feces. These compounds can cause behavioral symptoms in autism and symptoms resembling autism through the vagal pathway (65). Among these metabolites, SCFAs, including acetic acid, propionic acid, butyric acid, valeric acid, and caproic acid, have been identified as major signaling metabolites, playing critical roles in regulating catecholamine production throughout life and preserving the neurotransmitter phenotype after birth (66). These SCFAs were demonstrated by several studies to have importance in ASD (67).
4 Potential synergistic effects and mechanism of combined rTMS and gut microbiota modulation in ASD prevent and therapy
Poor social interaction, communication problems, and repetitive behaviors are hallmarks of ASD, which is a complex neurodevelopmental disorder (68). Numerous studies suggest that the development of autism depends on both hereditary and environmental factors (69). rTMS, as a noninvasive brain stimulation technology, has gained popularity and exhibited potential to treat ASD symptoms (70). Interest in the role of the gut-brain axis in ASD has increased recently because of studies demonstrating a relationship between gut microbiota composition and function in children with ASD. Thus, rTMS and gut microbiota modulation could be used as a combined treatment for ASD (Figure 2).
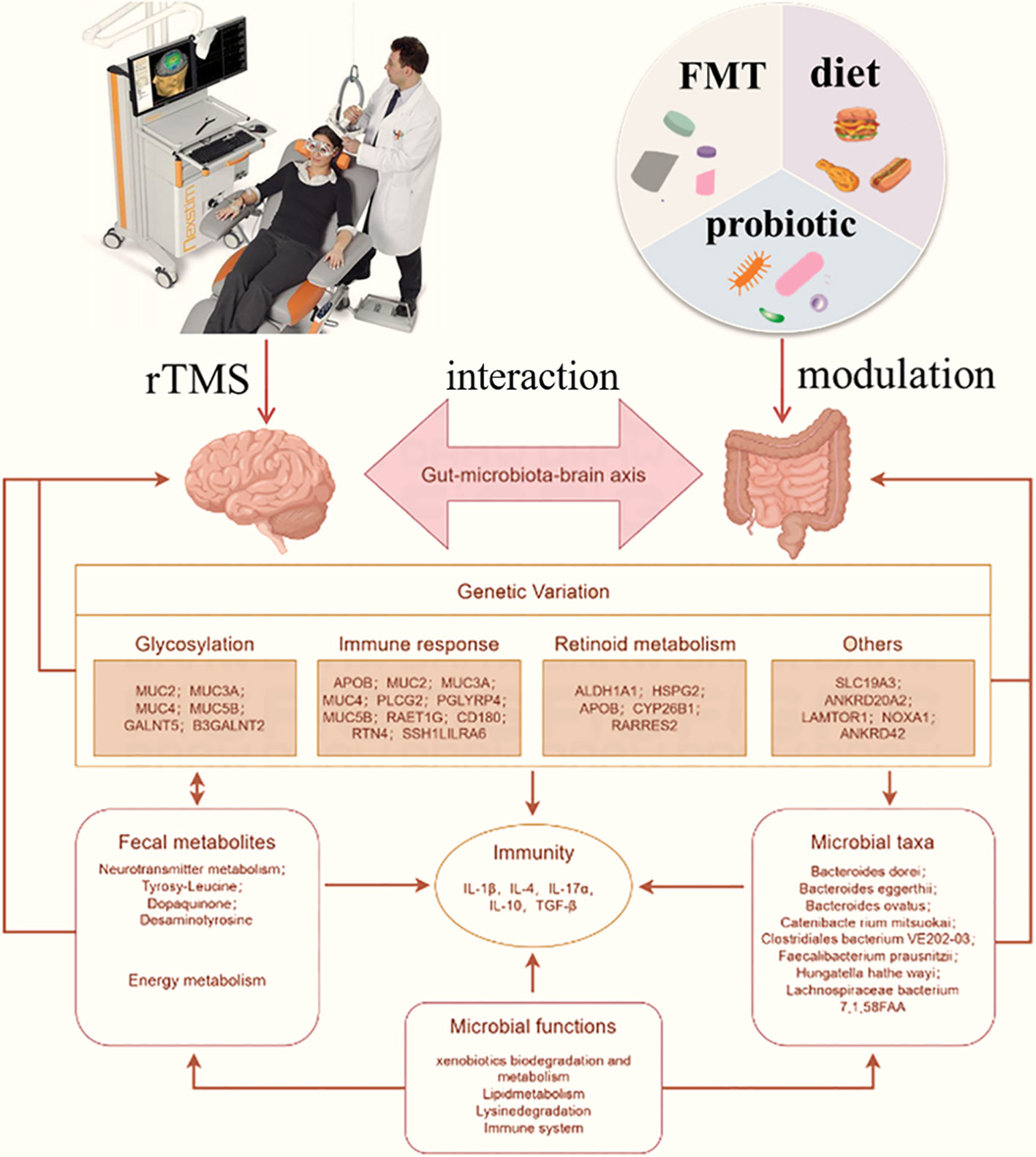
Figure 2 Potential treatment responses triggered by combined rTMS and gut microbiota modulation against ASD through interaction by gut-brain axis. rTMS can directly affect nervous function like glycosylation, immune response and retinoid metabolism to further affect brain performance. The fecal metabolites and microbial taxa was affected by gut microbiota modulation through diet, probiotics or FMT, which was also affect the immunity like IL-4, IL-10 and TGF-β. The interaction between microbial and nervous was achieved by gut microbiota-brain axis, which the intestinal function and brain function was mutual influence. Combination rTMS and gut microbiota modulation can play a double regulation that is contributed to the treatment of ASD.
A previous study demonstrated the existence of a sophisticated network of nervous, metabolic, immune, and other associations between the brain and the GI tract, known as the gut-brain-microbiome axis (71). rTMS influenced selected phyla of the gut microbiota, and the changes were associated with alterations in behavioral and metabolic parameters. Recent investigations suggest that alterations of the composition and diversity of gut microbiota caused by impaired energy homeostasis could play an important role in the development of neuropsychiatric and metabolic disorders (72). Furthermore, rTMS can reverse adverse effects on the gut microbiome, supporting the hypothesis of the existence of complex bidirectional communication between the brain and GI tract (gut-brain-microbiome axis). Several pathways, including those involving the vagus nerve, the immune and endocrine system, and the enteric nervous system, mediate the bidirectional communication between the brain and gut microbiota (73).
4.1 Combination of rTMS and gut microbiota modulation could modulate the nervous system
rTMS can enhance neuroplasticity by modulating neural activity and promoting synaptic plasticity (74). Some protocols appear to induce suppression or facilitation through Hebbian mechanisms of long-term depression and long-term potentiation across populations of neurons, whereas others induce these changes by modulating activity in GABAergic interneurons. The prominent role of inhibitory interneurons in the rTMS-induced modulation of cortical excitation is of importance in autism, similarly as the GABAergic system. rTMS as an investigational treatment partially supports the theories suggesting excitation/inhibition imbalance and aberrant plasticity mechanisms in ASD. Conversely, gut microbiota modulation can influence neuroplasticity through the production of neuroactive compounds and regulation of neurotransmitter metabolism (75). By combining these interventions, it is possible to enhance neuroplasticity mechanisms in the brain, leading to improved cognitive and behavioral outcomes in individuals with ASD. Additionally, the modulation of neurotransmitters is another potential pathway explaining the effects of the combined treatment. Both rTMS and gut microbiota modulation can influence neurotransmitter levels in the brain (76, 77). Gamma-aminobutyric acid (GABA) and glutamate are two neurotransmitters modulated by rTMS (78). Through the generation of metabolites including SCFAs and neurotransmitter precursors, gut microbiota modification can also affect neurotransmitter metabolism (79). By combining these interventions, it is possible to more comprehensively modulate neurotransmitter levels, which help alleviate ASD symptoms related to neurotransmitter dysregulation.
4.2 Combination of rTMS and gut microbiota modulation could regulate the immune response
rTMS and gut microbiota modulation have been implicated in the regulation of inflammation (80, 81). Inflammation is known to participate in the pathophysiology of ASD, and reducing inflammation can potentially alleviate symptoms associated with the disorder (82). rTMS can modulate the immune response and reduce pro-inflammatory cytokine levels (83). Gut microbiota modulation can also regulate inflammation through the production of anti-inflammatory factors and modulation of immune cells (83). By combining these interventions, it is possible to achieve synergistic anti-inflammatory effects, which might help alleviate ASD symptoms. Bidirectional communication between the gut and brain is achieved through the gut-brain axis, which plays a crucial role in the regulation of brain function and behavior. Both rTMS and gut microbiota modulation can influence the gut-brain axis (84, 85). rTMS can modulate gut microbiota composition and function through its effects on neurotransmitters and the immune system (86). Conversely, gut microbiota modulation can affect brain function and behavior through the production of neuroactive compounds, modulation of the immune system, and regulation of the intestinal barrier (87). By combining these interventions, it is possible to more comprehensively regulate the gut-brain axis, thereby improving ASD symptoms (Figure 3).
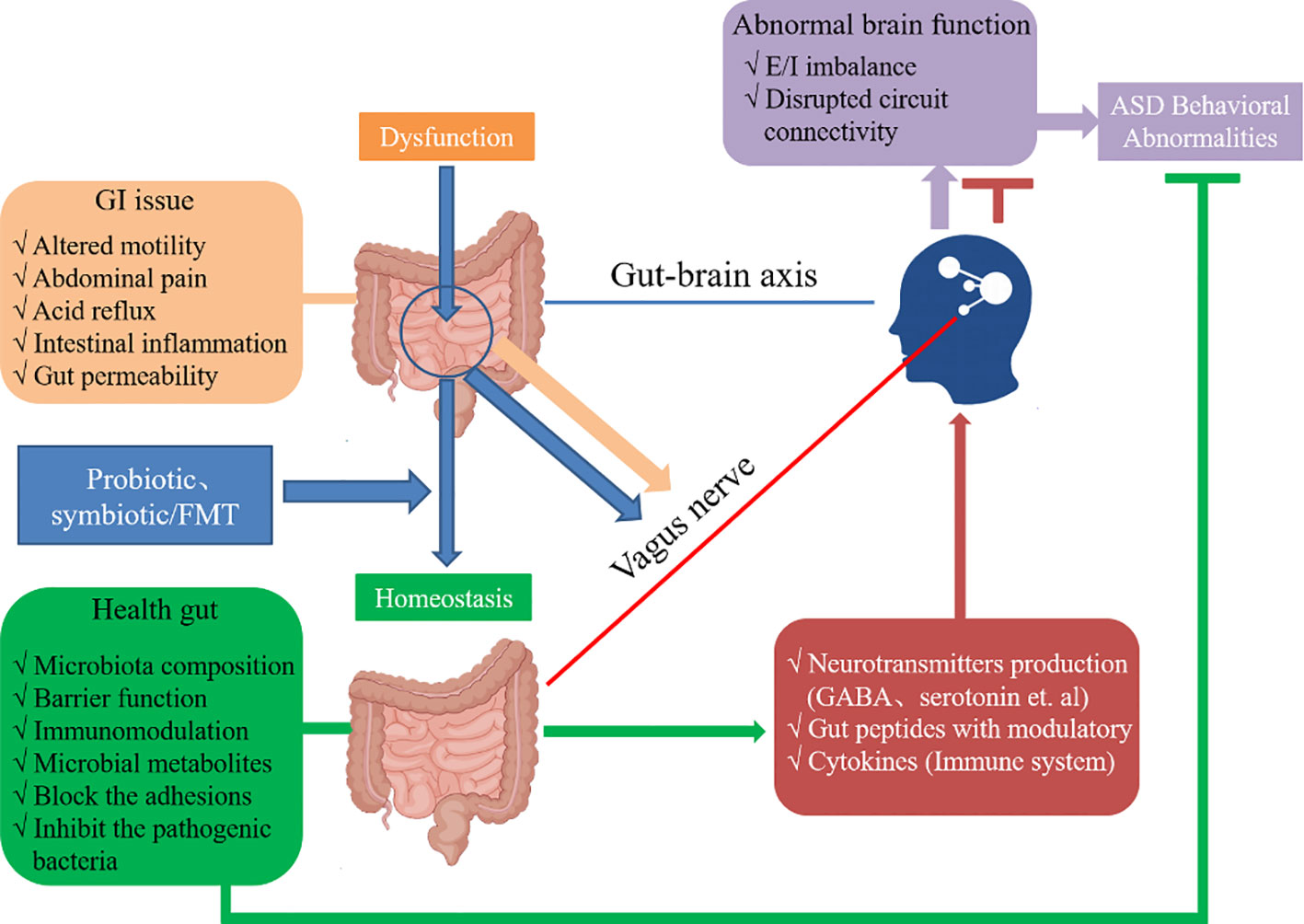
Figure 3 The ASD and dysregulation within the gut-brain axis and the appropriate mechanisms of interplay. The probiotics (e.g., Lactobacillus), symbiotics or FMT can enhance the homeostasis to maintain intestinal health. The microbiota composition and production of metabolites such as SCFAs can cross the “leaky gut” to affect brain function. Moreover, some microbiota can produce neuroactive compounds (e.g., GABA) that cross the “leaky gut” and influence brain function and induce abnormal behaviors. These neuroactive compounds can directly influence the HPA axis and increase circulating levels of cortisol. Metabolites, certain microbiota and neuroactive compounds can activate enteric neurons and affect brain function through the vagus nerve. Some microbiota and metabolites can activate gut immune cells, which can release cytokines into circulation.
4.3 Combination of rTMS and gut microbiota modulation could modulate psychological aspects
The potential synergistic effects of the combination of rTMS and gut microbiota modulation in ASD can be explored in other areas. GI symptoms, including abdominal pain, constipation, and diarrhea, are commonly reported in individuals with ASD (88). Gut microbiota modulation has been demonstrated to improve GI symptoms by restoring the balance of gut bacteria and reducing gut inflammation (89). rTMS, through its effects on the gut-brain axis, might also have a positive impact on GI symptoms. By combining these interventions, it is possible to target both the neurological and gastrointestinal aspects of ASD, leading to a more comprehensive improvement in overall well-being. Anxiety and depression are frequently observed in individuals with ASD (90). Both rTMS and gut microbiota modulation have proven antidepressant and anxiolytic effects. rTMS can modulate neural circuits involved in mood regulation (91), whereas gut microbiota modulation can influence the production of neurotransmitters and neuroactive compounds involved in mood regulation (92). By combining these interventions, it is possible to target both the neurological and psychological aspects of ASD, leading to reductions in anxiety and depression symptoms. Impairments in social communication and interaction are core features of ASD (93). rTMS has been revealed to modulate brain regions involved in social cognition and empathy, which are often impaired in individuals with ASD (94). Gut microbiota modulation can also influence social behavior through its effects on neurotransmitters and the immune system (95). By combining these interventions, it is possible to target the underlying neural mechanisms involved in social communication and interaction, leading to improvements in these domains.
5 Conclusion and future directions
In this review, we discussed the interrelationship between rTMS and the gut microbiota via the gut-brain axis in ASD. We also discussed the potential of combining rTMS with gut microbiota modulation as a novel therapeutic strategy for the prevention and treatment of ASD in children. However, further investigation is required to clarify the best treatment approaches, long-term outcomes, and safety profiles of these therapies. Additionally, to develop successful and focused therapies for individuals with ASD, deeper understanding of the underlying processes and the intricate relationships among rTMS, the gut microbiota, and the brain is essential. As we indicated, rTMS is believed to be safe when performed in compliance with current safety standards, particularly in pediatric populations. However, rTMS carries a small risk of unfavorable side effects. Therefore, before patients receive rTMS, several criteria, including their current medicines and medical history, must be evaluated. Additionally, the procedure’s risk-benefit ratio must be carefully considered.
Author contributions
PF: Conceptualization, Writing – original draft, Writing – review & editing. YYZ: Data curation, Software, Writing – review & editing. YHZ: Investigation, Writing – review & editing. PZ: Writing – review & editing. EL: Writing – review & editing.
Funding
The author(s) declare financial support was received for the research, authorship, and/or publication of this article. This work was supported by Zhengzhou Major Collaborative Innovation Project (No.18XTZX12003); Key projects of discipline construction in Zhengzhou University (No.XKZDJC202001); National Key Research and development program in China (No.2020YFC2006100); Natural Science Foundation of Henan Province (No.212300410399); Key Research and Development and Promotion Special Projects of Henan Province (No. 232102311068).
Conflict of interest
The authors declare that the research was conducted in the absence of any commercial or financial relationships that could be construed as a potential conflict of interest.
Publisher’s note
All claims expressed in this article are solely those of the authors and do not necessarily represent those of their affiliated organizations, or those of the publisher, the editors and the reviewers. Any product that may be evaluated in this article, or claim that may be made by its manufacturer, is not guaranteed or endorsed by the publisher.
Abbreviations
ASD, Autism spectrum disorder; rTMS, Repetitive transcranial magnetic stimulation; GI, Gastrointestinal; CNS, Central nervous system; DLPFC, Dorsal lateral prefrontal cortex; IRI, Interpersonal response index; dmPFC, Dorsomedial Prefrontal Cortex; ATEC, Autism treatment evaluation; IL-1, Interleukin-1; INF, Interferon; TNF, Tumor necrosis factor; SCFA, Short-chain fatty acid; GABA, Gamma-aminobutyric acid; tDCS, transcranial direct current stimulation; E/I, Excitation/inhibition.
References
1. Li Q, Zhou J-M. The microbiota–gut–brain axis and its potential therapeutic role in autis spectrum disorder. Neuroscience (2016) 324:131–9. doi: 10.1016/j.neuroscience.2016.03.013
2. A.P. Association. American Psychiatric Association: Diagnosti c and Statistical Manual of Mental Disorders Arlington: The American Psychiatric Association (2013). doi: 10.1176/appi.books.9780890425596
3. Vos T, Abajobir AA, Abate KH, Abbafati C, Abbas KM, Abd-Allah F, et al. Global, regional, and national incidence, prevalence, and years lived with disability for 328 diseases and injuries for 195 countries, 1990–2016: a systematic analysis for the Global Burden of Disease Study 2016. Lancet (2017) 390:1211–59. doi: 10.1016/S0140-6736(18)32279-7
4. Li Z, Liao Y, Zhou Q, Qu Q, Sheng M, Lv L, et al. Changes of gut microbiota in autism spectrum disorders and common probiotics & chinese herbal medicine therapeutic mechanisms: A review. Adv Neurodev Disord (2022) 6:1–14. doi: 10.1007/s41252-022-00266-6
5. Howes OD, Rogdaki M, Findon JL, Wichers RH, Charman T, King BH, et al. Autism spectrum disorder: Consensus guidelines on assessment, treatment and research from the British Association for Psychopharmacology. J Psychopharmacol (2018) 32:3–29. doi: 10.1177/0269881117741766
6. Chaidez V, Hansen RL, Hertz-Picciotto I. Gastrointestinal problems in children with autism, developmental delays or typical development. J Autism Dev Disord (2014) 44:1117–27. doi: 10.1007/s10803-013-1973-x
7. Autism and Developmental Disabilities Monitoring Network Surveillance Year 2008 Principal Investigators. Prevalence of autism spectrum disorders—autism and developmental disabilities monitoring network, 14 sites, United States, 2008. Morbidity Mortality Weekly Report: Surveillance Summaries (2012) 61:1–19.
8. Frye RE, Rossignol DA. Identification and treatment of pathophysiological comorbidities of autism spectrum disorder to achieve optimal outcomes. Clin Med Insights: Pediatr (2016) 10:CMPed.S38337. doi: 10.4137/CMPed.S38337
9. Wang H-X, Wang Y-P. Gut microbiota-brain axis. Chin Med J (2016) 129:2373–80. doi: 10.4103/0366-6999.190667
10. Bejenaru AM, Malhi NK. Use of repetitive transcranial magnetic stimulation in child psychiatry. Innov Clin Neurosci (2022) 19:11–22.
11. Korenblik V, Brouwer ME, Korosi A, Denys D, Bockting CLH, Brul S, et al. Are neuromodulation interventions associated with changes in the gut microbiota? A Systematic Review Neuropharmacol (2023) 223:109318. doi: 10.1016/j.neuropharm.2022.109318
12. Murphy CM, Wilson CE, Robertson DM, Ecker C, Daly EM, Hammond N, et al. Autism spectrum disorder in adults: diagnosis, management, and health services development. Neuropsychiatr Dis Treat (2016) 12:1669–86. doi: 10.2147/NDT.S65455
13. Gómez L, Vidal B, Maragoto C, Morales LM, Berrillo S, Vera Cuesta H, et al. Non-invasive brain stimulation for children with autism spectrum disorders: A short-term outcome study. Behav Sci (Basel Switzerland) (2017) 7:63. doi: 10.3390/bs7030063
14. Barker AT, Jalinous R, Freeston IL. Non-invasive magnetic stimulation of human motor cortex. Lancet. (1985) 325:1106–7. doi: 10.1016/s0140-6736(85)92413-4
15. Oberman LM, Enticott PG, Casanova MF, Rotenberg A, Pascual-Leone A, McCracken JT, et al. Transcranial magnetic stimulation in autism spectrum disorder: Challenges, promise, and roadmap for future research. Autism Res (2016) 9:184–203. doi: 10.1002/aur.1567
16. Khaleghi A, Zarafshan H. Effects of non-invasive neurostimulation on autism spectrum disorder: A systematic review. Clinical Psychopharmacology And Neuroscience (2020) 18:527–52. doi: 10.9758/cpn.2020.18.4.527
17. Cavaleiro C, Martins J, Gonçalves J, Castelo-Branco M. Memory and cognition-related neuroplasticity enhancement by transcranial direct current stimulation in rodents: A systematic review. Neural Plasticity (2020) 2020:1–23. doi: 10.1155/2020/4795267
18. Gonçalves J, Violante IR, Sereno J, Leitão RA, Cai Y, Abrunhosa A, et al. Testing the excitation/inhibition imbalance hypothesis in a mouse model of the autism spectrum disorder: in vivo neurospectroscopy and molecular evidence for regional phenotypes. Mol Autism (2017) 8. doi: 10.1186/s13229-017-0166-4
19. Ethridge VT, Gargas NM, Sonner MJ, Moore RJ, Romer SH, Hatcher-Solis C, et al. Effects of transcranial direct current stimulation on brain cytokine levels in rats. Front Neurosci (2022) 16:1069484. doi: 10.3389/fnins.2022.1069484
20. Schulze L, Feffer K, Lozano C, Giacobbe P, Daskalakis ZJ, Blumberger DM, et al. Number of pulses or number of sessions? An open-label study of trajectories of improvement for once-vs. twice-daily dorsomedial prefrontal rTMS in major depression. Brain Stimulation (2018) 11:327–36. doi: 10.1016/j.brs.2017.11.002
21. Chung SW, Rogasch NC, Hoy KE, Fitzgerald PB. Measuring brain stimulation induced changes in cortical properties using TMS-EEG. Brain Stimulation (2015) 8:1010–20. doi: 10.1016/j.brs.2015.07.029
22. Enticott PG, Kennedy HA, Rinehart NJ, Bradshaw JL, Tonge BJ, Daskalakis ZJ, et al. Interpersonal motor resonance in autism spectrum disorder: evidence against a global "mirror system" deficit. Front Hum Neurosci (2013) 7:218. doi: 10.3389/fnhum.2013.00218
23. Enticott PG, Kennedy HA, Rinehart NJ, Tonge BJ, Bradshaw JL, Fitzgerald PB. GABAergic activity in autism spectrum disorders: An investigation of cortical inhibition via transcranial magnetic stimulation. Neuropharmacology (2013) 68:202–9. doi: 10.1016/j.neuropharm.2012.06.017
24. Oberman LM, Pascual-Leone A, Rotenberg A. Modulation of corticospinal excitability by transcranial magnetic stimulation in children and adolescents with autism spectrum disorder. Front Hum Neurosci. (2014) 8:627. doi: 10.3389/fnhum.2014.00627
25. Oberman LM, Pascual-Leone A. Hyperplasticity in Autism Spectrum Disorder confers protection from Alzheimer's disease. Med Hypotheses (2014) 83:337–42. doi: 10.1016/j.mehy.2014.06.008
26. Enticott PG, Kennedy HA, Rinehart NJ, Tonge BJ, Bradshaw JL, Taffe JR, et al. Mirror Neuron Activity Associated with Social Impairments but not Age in Autism Spectrum Disorder. Biol Psychiatry (2012) 71:427–33. doi: 10.1016/j.biopsych.2011.09.001
27. Jung N, Biscaldi-Schäfer M, Münchau A, Mainberger F, Bäumer T, Rauh R, et al. Impaired long term potentiation (LTP)-like plasticity in patients with high functiong autism and Asperger syndrome (HFA/AS). Clinical Neurophysiology (2010) 155:99–106. doi: 10.1016/j.clinph.2023.07.011
28. Ibiricu MA, Morales G. [Transcranial magnetic stimulation]. Anales Del Sistema sanitario Navarra (2009) 32 Suppl 3:105–13. doi: 10.4321/S1137-66272009000600009.
29. Vahabzadeh A, McDougle CJ. Obsessive–compulsive disorder. In: McManus LM, Mitchell RN, editors. Pathobiology of Human Disease Academic Press, San Diego (2014). p. 2512–21. doi: 10.1038/s41572-019-0102-3
30. Harty PS, Cottet ML, Malloy JK, Kerksick CM. Nutritional and supplementation strategies to prevent and attenuate exercise-induced muscle damage: a brief review. Sports Medicine-open (2019) 5:1. doi: 10.1186/s40798-018-0176-6
31. Deary IJ, Cox SR, Hill WD, variation G. brain, and intelligence differences. Mol Psychiatry (2022) 27:335–53. doi: 10.1038/s41380-021-01027-y
32. Gustafsson L. Comment on "Disruption in the inhibitory architecture of the cell minicolumn: implications for autism". Neuroscientist Rev J Bringing Neurobiol Neurol Psychiatry (2004) 10:189–91. doi: 10.1177/1073858404263493
33. Speer AM, Kimbrell TA, Wassermann EM, Repella JD, Willis MW, Herscovitch P, et al. Opposite effects of high and low frequency rTMS on regional brain activity in depressed patients. Biol Psychiatry (2000) 48:1133–41. doi: 10.1016/j.jad.2008.10.006
34. Moxon-Emre I, Daskalakis ZJ, Blumberger DM, Croarkin PE, Lyon RE, Forde NJ, et al. Modulation of dorsolateral prefrontal cortex glutamate/glutamine levels following repetitive transcranial magnetic stimulation in young adults with autism. Front Neurosci (2021) 15:711542. doi: 10.3389/fnins.2021.711542
35. Xia X, Li Y, Wang Y, Xia J, Lin Y, Zhang X, et al. Functional role of dorsolateral prefrontal cortex in the modulation of cognitive bias. Psychophysiology (2021) 58:e13894. doi: 10.1111/psyp.13894
36. Pazzaglia C, Vollono C, Testani E, Coraci D, Granata G, Padua L, et al. Low-frequency rTMS of the primary motor area does not modify the response of the cerebral cortex to phasic nociceptive stimuli. Front Neurosci (2018) 12:878. doi: 10.3389/fnins.2018.00878
37. Fecteau S, Agosta S, Oberman L, Pascual-Leone A. Brain stimulation over Broca's area differentially modulates naming skills in neurotypical adults and individuals with Asperger's syndrome. Eur J Neurosci (2011) 34:158–64. doi: 10.1111/ejn.2011.34.issue-1
38. Enticott PG, Rinehart NJ, Tonge BJ, Bradshaw JL, Fitzgerald PB. Repetitive transcranial magnetic stimulation (rTMS) improves movement-related cortical potentials in autism spectrum disorders. Brain Stimulation (2012) 5:30–7. doi: 10.1016/j.brs.2011.02.001
39. Enticott PG, Fitzgibbon BM, Kennedy HA, Arnold SL, Elliot D, Peachey A, et al. randomized trial of deep repetitive transcranial magnetic stimulation (rTMS) for autism spectrum disorder. Brain Stimulation (2014) 7:206–11. doi: 10.1016/j.brs.2013.10.004
40. Casanova MF, Baruth JM, El-Baz A, Tasman A, Sears L, Sokhadze E. Repetitive transcranial magnetic stimulation (rTMS) modulates event-related potential (ERP) indices of attention in autism. Trans Neurosci (2012) 3:170–80. doi: 10.2478/s13380-012-0022-0
41. Niederhofer H. Effectiveness of the repetitive transcranical magnetic stimulation (rTMS) of 1 Hz for attention-deficit hyperactivity disorder (ADHD). Psychiatria Danubina (2008) 20:91–2.
42. Sokhadze E, Baruth J, Tasman A, Mansoor M, Ramaswamy R, Sears L, et al. Low-frequency repetitive transcranial magnetic stimulation (rTMS) affects event-related potential measures of novelty processing in autism. Appl Psychophysiol Biofeedback (2010) 35:147–61. doi: 10.1007/s10484-009-9121-2
43. Casanova MF, Hensley MK, Sokhadze EM, El-Baz AS, Wang Y, Li X, et al. Effects of weekly low-frequency rTMS on autonomic measures in children with autism spectrum disorder. Front Hum Neurosci (2014) 8:851. doi: 10.3389/fnhum.2014.00851
44. Lefaucheur JP, André-Obadia N, Antal A, Ayache SS, Baeken C, Benninger DH, et al. Evidence-based guidelines on the therapeutic use of repetitive transcranial magnetic stimulation (rTMS). Clin Neurophysiol Off J Int Fed Clin Neurophysiol (2014) 125:2150–206. doi: 10.1016/j.clinph.2019.11.002
45. Maenner MJ, Arneson CL, Levy SE, Kirby RS, Nicholas JS, Durkin MS. Brief report: Association between behavioral features and gastrointestinal problems among children with autism spectrum disorder. J Autism Dev Disord (2012) 42:1520–5. doi: 10.1007/s10803-011-1379-6
46. Adams JB, Johansen LJ, Powell LD, Quig D, Rubin RA. Gastrointestinal flora and gastrointestinal status in children with autism–comparisons to typical children and correlation with autism severity. BMC Gastroenterol (2011) 11:1–13. doi: 10.1186/1471-230X-11-22
47. Srikantha P, Mohajeri MH. The possible role of the microbiota-gut-brain-axis in autism spectrum disorder. Int J Mol Sci (2019) 20:2115. doi: 10.3390/ijms20092115
48. Ding HT, Taur Y, Walkup JT. Gut microbiota and autism: key concepts and findings. J Autism Dev Disord (2017) 47:480–9. doi: 10.1007/s10803-016-2960-9
49. Hsiao EY. Gastrointestinal issues in autism spectrum disorder. Harvard Rev Psychiatry (2014) 22:104–11. doi: 10.1016/j.chc.2020.02.005
50. Nikolov RN, Bearss KE, Lettinga J, Erickson C, Rodowski M, Aman MG, et al. Gastrointestinal symptoms in a sample of children with pervasive developmental disorders. J Autism Dev Disord (2009) 39:405–13. doi: 10.1007/s10803-008-0637-8
51. Strati F, Cavalieri D, Albanese D, De Felice C, Donati C, Hayek J, et al. New evidences on the altered gut microbiota in autism spectrum disorders. Microbiome (2017) 5:1–11. doi: 10.1186/s40168-017-0242-1
52. Liu S, Li E, Sun Z, Fu D, Duan G, Jiang M, et al. Altered gut microbiota and short chain fatty acids in Chinese children with autism spectrum disorder. Sci Rep (2019) 9:1–9. doi: 10.1038/s41598-018-36430-z
53. Iovene MR, Bombace F, Maresca R, Sapone A, Iardino P, Picardi A, et al. Intestinal dysbiosis and yeast isolation in stool of subjects with autism spectrum disorders. Mycopathologia (2017) 182:349–63. doi: 10.1007/s11046-016-0068-6
54. Kushak RI, Winter HS, Buie TM, Cox SB, Phillips CD, Ward NL. Analysis of the duodenal microbiome in autistic individuals: association with carbohydrate digestion. J Pediatr Gastroenterol Nutr (2017) 64:e110–6. doi: 10.1097/MPG.0000000000001458
55. Kong X, Liu J, Cetinbas M, Sadreyev R, Koh M, Huang H, et al. New and preliminary evidence on altered oral and gut microbiota in individuals with autism spectrum disorder (ASD): implications for ASD diagnosis and subtyping based on microbial biomarkers. Nutrients (2019) 11:2128. doi: 10.3390/nu11092128
56. Williams BL, Hornig M, Buie T, Bauman ML, Cho Paik M, Wick I, et al. Impaired carbohydrate digestion and transport and mucosal dysbiosis in the intestines of children with autism and gastrointestinal disturbances. PloS One (2011) 6:e24585. doi: 10.1371/journal.pone.0024585
57. Luna RA, Oezguen N, Balderas M, Venkatachalam A, Runge JK, Versalovic J, et al. Distinct microbiome-neuroimmune signatures correlate with functional abdominal pain in children with autism spectrum disorder. Cell Mol Gastroenterol Hepatol (2017) 3:218–30. doi: 10.1016/j.jcmgh.2016.11.008
58. Kang D-W, Park JG, Ilhan ZE, Wallstrom G, LaBaer J, Adams JB, et al. Reduced incidence of Prevotella and other fermenters in intestinal microflora of autistic children. PloS One (2013) 8:e68322. doi: 10.1371/journal.pone.0068322
59. Parracho HM, Bingham MO, Gibson GR, McCartney AL. Differences between the gut microflora of children with autistic spectrum disorders and that of healthy children. J Med Microbiol (2005) 54:987–91. doi: 10.1099/jmm.0.46101-0
60. Hughes HK, Rose D, Ashwood P. The gut microbiota and dysbiosis in autism spectrum disorders. Curr Neurol Neurosci Rep (2018) 18:81. doi: 10.1007/s11910-018-0887-6
61. Doenyas C. Gut microbiota, inflammation, and probiotics on neural development in autism spectrum disorder. Neuroscience (2018) 374:271–86. doi: 10.1016/j.neuroscience.2018.01.060
62. de Theije CG, Wu J, Da Silva SL, Kamphuis PJ, Garssen J, Korte SM, et al. Pathways underlying the gut-to-brain connection in autism spectrum disorders as future targets for disease management. Eur J Pharmacol (2011) 668:S70–80. doi: 10.1016/j.ejphar.2011.07.013
63. Ashwood P, Krakowiak P, Hertz-Picciotto I, Hansen R, Pessah I, Van de Water J. Elevated plasma cytokines in autism spectrum disorders provide evidence of immune dysfunction and are associated with impaired behavioral outcome. Brain Behav Immun (2011) 25:40–5. doi: 10.1016/j.bbi.2010.08.003
64. Critchfield JW, Van Hemert S, Ash M, Mulder L, Ashwood P. The potential role of probiotics in the management of childhood autism spectrum disorders. Gastroenterol Res Pract (2011) 2011. doi: 10.1155/2011/161358
65. Forsythe P, Bienenstock J, Kunze WA. Vagal pathways for microbiome-brain-gut axis communication. Microbial Endocrinol: Microbiota-gut-brain Axis Health Dis (2014) 2011:115–33. doi: 10.1007/978-1-4939-0897-4_5
66. Tengeler AC, Kozicz T, Kiliaan AJ. Relationship between diet, the gut microbiota, and brainfunction. Nutr Rev (2018) 76:603–17. doi: 10.1093/nutrit/nuy016
67. Wang L, Christophersen CT, Sorich MJ, Gerber JP, Angley MT, Conlon MA. Elevated fecal short chain fatty acid and ammonia concentrations in children with autism spectrum disorder. Digestive Dis Sci (2012) 57:2096–102. doi: 10.1007/s10620-012-2167-7
68. Mughal S, Faizy RM, Saadabadi A. Autism spectrum disorder. In: StatPearls. StatPearls Publishing, Treasure Island (FL (2023).
69. Cooper GS, Miller FW, Pandey JP. The role of genetic factors in autoimmune disease: implications for environmental research. Environ Health Perspect (1999) 107 Suppl 5:693–700. doi: 10.1289/ehp.99107s5693
70. Enticott PG, Barlow K. Repetitive transcranial magnetic stimulation (rTMS) in autism spectrum disorder: protocol for a multicentre randomised controlled clinical trial. Bmj Open (2021) 11:. doi: 10.1136/bmjopen-2020-046830
71. Ziomber-Lisiak A, Talaga-Ćwiertnia K, Sroka-Oleksiak A, Surówka AD, Juszczak K, Szczerbowska-Boruchowska M. Repetitive transcranial direct current stimulation modulates the brain-gut-microbiome axis in obese rodents. Pharmacol Rep PR (2022) 74:871–89. doi: 10.1007/s43440-022-00401-z
72. Detka J, Głombik K. Insights into a possible role of glucagon-like peptide-1 receptor agonists in the treatment of depression. Pharmacol Rep PR (2021) 73:1020–32. doi: 10.1007/s43440-021-00274-8
73. Artifon M, Schestatsky P, Griebler N, Tossi GM, Beraldo LM, Pietta-Dias C. Effects of transcranial direct current stimulation on the gut microbiome: A case report. Brain Stimulation (2020) 13:1451–2. doi: 10.1016/j.brs.2020.07.019
74. Pateraki G, Anargyros K, Aloizou AM, Siokas V, Bakirtzis C, Liampas I, et al. Therapeutic application of rTMS in neurodegenerative and movement disorders: A review. J Electromyography Kinesiol Off J Int Soc Electrophysiological Kinesiol (2022) 62:102622. doi: 10.1016/j.jelekin.2021.102622
75. Murciano-Brea J, Garcia-Montes M, Geuna S. Gut microbiota and neuroplasticity. Cells (2021) 10. doi: 10.3390/cells10082084
76. Strandwitz P. Neurotransmitter modulation by the gut microbiota. Brain Res (2018) 1693:128–33. doi: 10.1016/j.brainres.2018.03.015
77. Leblhuber F, Geisler S, Ehrlich D, Steiner K, Reibnegger G, Fuchs D. Repetitive transcranial magnetic stimulation in the treatment of resistant depression: changes of specific neurotransmitter precursor amino acids. Journal of Neural Transmission (2021) 128:1225–31. doi: 10.1007/s00702-021-02363-7
78. Zhang H, Huang X, Wang C, Liang K. Alteration of gamma-aminobutyric acid in the left dorsolateral prefrontal cortex of individuals with chronic insomnia: a combined transcranial magnetic stimulation-magnetic resonance spectroscopy study. Sleep Med (2022) 92:34–40. doi: 10.1016/j.sleep.2022.03.003
79. Chen Y, Xu J, Chen Y. Regulation of neurotransmitters by the gut microbiota and effects on cognition in neurological disorders. Nutrients (2021) 13:13. doi: 10.3390/nu13062099
80. Cruz CS, Ricci MF, Vieira AT. Gut microbiota modulation as a potential target for the treatment of lung infections. Front Pharmacol (2021) 12:724033. doi: 10.3389/fphar.2021.724033
81. Bai YW, Yang QH, Chen PJ, Wang XQ. Repetitive transcranial magnetic stimulation regulates neuroinflammation in neuropathic pain. Front Immunol (2023) 14:1172293. doi: 10.3389/fimmu.2023.1172293
82. Prata J, MaChado AS, von Doellinger O, Almeida MI, Barbosa MA, Coelho R, et al. The contribution of inflammation to autism spectrum disorders: recent clinical evidence. Methods Mol Biol (Clifton N.J.) (2019) 2011:493–510. doi: 10.1007/978-1-4939-9554-7_29
83. Wang Q, Zeng L, Hong W, Luo M, Zhao N, Hu X, et al. Inflammatory cytokines changed in patients with depression before and after repetitive transcranial magnetic stimulation treatment. Front Psychiatry (2022) 13:925007. doi: 10.3389/fpsyt.2022.925007
84. Morais LH, Schreiber HL, Mazmanian SK. The gut microbiota–brain axis in behaviour and brain disorders. Nat Rev Microbiol (2021) 19:241–55. doi: 10.1038/s41579-020-00460-0
85. Li R, Fu R, Cui Z-q, Guo L, Chen Y-h, Bai J, et al. Effects of low-frequency rTMS combined with risperidone on the gut microbiome in hospitalized patients with chronic schizophrenia. Brain Res (2023) 1819:148539. doi: 10.1016/j.brainres.2023.148539
86. Dicks LMT. Gut bacteria and neurotransmitters. Microorganisms (2022) 10:10. doi: 10.3390/microorganisms10091838
87. Mitra S, Dash R, Nishan AA, Habiba SU, Moon IS. Brain modulation by the gut microbiota:From disease to therapy. J Advanced Res (2022) 53:153–173. doi: 10.1016/j.jare.2022.12.001
88. Wasilewska J, Klukowski M. Gastrointestinal symptoms and autism spectrum disorder: links and risks - a possible new overlap syndrome. Pediatr Health Med Ther (2015) 6:153–66. doi: 10.2147/PHMT.S85717
89. Fong W, Li Q, Yu J. Gut microbiota modulation: a novel strategy for prevention and treatment of colorectal cancer. Oncogene (2020) 39:4925–43. doi: 10.1038/s41388-020-1341-1
90. Hollocks MJ, Lerh JW, Magiati I, Meiser-Stedman R, Brugha TS. Anxiety and depression in adults with autism spectrum disorder: a systematic review and meta-analysis. psychol Med (2019) 49:559–72. doi: 10.1017/S0033291718002283
91. Hutton TM, Aaronson ST, Carpenter LL, Pages K, West WS, Kraemer C, et al. The anxiolytic and antidepressant effects of transcranial magnetic stimulation in patients with anxious depression. J Clin Psychiatry (2023) 84:49. doi: 10.4088/JCP.22m14571
92. Simpson CA, Diaz-Arteche C, Eliby D, Schwartz OS, Simmons JG, Cowan CSM. The gut microbiota in anxiety and depression - A systematic review. Clin Psychol Rev (2021) 83:101943. doi: 10.1016/j.cpr.2020.101943
93. Watkins L, Kuhn M, Ledbetter-Cho K, Gevarter C, O'Reilly M. Evidence-based social communication interventions for children with autism spectrum disorder. Indian J Pediatr (2017) 84:68–75. doi: 10.1007/s12098-015-1938-5
94. Kim WJ, Rosselin C, Amatya B, Hafezi P, Khan F. Repetitive transcranial magnetic stimulation for management of post-stroke impairments: An overview of systematic reviews. J Rehabil Med (2020) 52:52. doi: 10.2340/16501977-2637
Keywords: autism spectrum disorder, rTMS, gut microbiota, gut-brain axis, immunology
Citation: Feng P, Zhang Y, Zhao Y, Zhao P and Li E (2024) Combined repetitive transcranial magnetic stimulation and gut microbiota modulation through the gut–brain axis for prevention and treatment of autism spectrum disorder. Front. Immunol. 15:1341404. doi: 10.3389/fimmu.2024.1341404
Received: 20 November 2023; Accepted: 07 February 2024;
Published: 22 February 2024.
Edited by:
Pradeep Kumar Shukla, University of Tennessee Health Science Center (UTHSC), United StatesReviewed by:
Fabrícia Lima Fontes-Dantas, Rio de Janeiro State University, BrazilChandra Shekhar, University of Tennessee Health Science Center (UTHSC), United States
Copyright © 2024 Feng, Zhang, Zhao, Zhao and Li. This is an open-access article distributed under the terms of the Creative Commons Attribution License (CC BY). The use, distribution or reproduction in other forums is permitted, provided the original author(s) and the copyright owner(s) are credited and that the original publication in this journal is cited, in accordance with accepted academic practice. No use, distribution or reproduction is permitted which does not comply with these terms.
*Correspondence: Enyao Li, MTM1MjY2NzY2NzZAMTI2LmNvbQ==; Pengya Feng, ZmVuZ3B5MTk5MkAxNjMuY29t