- 1Department of Hematology and Oncology, Dongzhimen Hospital, Beijing University of Chinese Medicine, Beijing, China
- 2Department of Pharmacology, Yale Medical School, New Haven, CT, United States
Lung cancer is a disease of global concern, and immunotherapy has brought lung cancer therapy to a new era. Besides promising effects in the clinical use of immune checkpoint inhibitors, immune-related adverse events (irAEs) and low response rates are problems unsolved. Natural products and traditional medicine with an immune-modulating nature have the property to influence immune checkpoint expression and can improve immunotherapy’s effect with relatively low toxicity. This review summarizes currently approved immunotherapy and the current mechanisms known to regulate immune checkpoint expression in lung cancer. It lists natural products and traditional medicine capable of influencing immune checkpoints or synergizing with immunotherapy in lung cancer, exploring both their effects and underlying mechanisms. Future research on immune checkpoint modulation and immunotherapy combination applying natural products and traditional medicine will be based on a deeper understanding of their mechanisms regulating immune checkpoints. Continued exploration of natural products and traditional medicine holds the potential to enhance the efficacy and reduce the adverse reactions of immunotherapy.
1 Introduction
Lung cancer is the leading cause of cancer death and ranks the second for the incidence rate worldwide, over 45% of lung cancer patients are diagnosed at late stage (1, 2). Chemotherapy and targeted therapy are widely used in advanced lung cancer depending on the status of actionable driver mutations (3). Besides directly killing tumor cells, clinicians are now concentrating on the tumor microenvironment. It is reported that immunosuppressive environment is related to poor treatment results (4). The tumor can evade immune surveillance in this environment and easily metastasize (5). Immune checkpoints are observed to be associated with immunosuppressive environment. Programmed cell death 1 (PD-1) and programmed death-ligand 1 (PD-L1), the most studied immune checkpoints, play essential roles in tumor progression (6, 7). Other immune checkpoints like IDO (Indoleamine-2,3-dioxygenase) and B7-H3 can also inhibit the immune reaction and promote lung cancer progression (8–10). Immune checkpoint inhibitors (ICIs) are now widely used for lung cancer treatment and have shown promising therapeutic activities (11, 12).
Nevertheless, only a few patients can benefit from this therapy, and the expression level of immune checkpoints such as PD-L1 is positively correlated to the effect of ICIs (13). Natural products are promising candidates to promote ICIs efficacy by upregulating immune checkpoints expression. On the other hand, ICIs directly blockade the interaction between immune checkpoints, while natural products can interrupt checkpoints interaction by inhibiting their expression via different mechanisms (14–16). Therefore, understanding the regulatory mechanisms of immune checkpoints in lung cancer and mechanisms of how natural products modulate immune checkpoint expression are extremely significant for exploring novel approaches to inhibit immune checkpoint expression and develop new drugs based on natural products. In this study, we would like to summarize currently known immune checkpoint regulation mechanisms in lung cancer and introduce the effect of natural products and traditional medicine that can influence immune checkpoints or promote immunotherapy in lung cancer.
2 Immunotherapy in lung cancer
Immune check point inhibitors pembrolizumab, atezolizumab, or cemiplimab can be used as first-line single-agent for metastasized non-small cell lung cancer (NSCLC) patients with PD-L1 expression level ≥50% and negative driver gene mutation that have recommended first-line targeted therapy according to the NCCN guideline (17). Furthermore, NCCN also recommends that metastatic NSCLC patients with PD-L1 levels of 1% to 49% and negative driver gene mutation to use pembrolizumab alone as a first-line therapy. Meanwhile, immune checkpoint inhibitors are not recommended as single-agents in small cell lung cancer (SCLC).
Besides being used as a single agent in advanced NSCLC, immune checkpoint inhibitors can combine with chemotherapy before or after surgery. CheckMate 816 is a phase 3 randomized trial that discovered neoadjuvant therapy with nivolumab plus platinum-doublet chemotherapy has a longer event-free survival time and higher pathologic complete response rate, major pathologic response rate, and overall response rate versus chemotherapy alone (18). Thus NCCN guidelines recommends nivolumab plus platinum-doublet chemotherapy as neoadjuvant therapy in resectable (tumors ≥4 cm or node positive) NSCLC (17). NCCN guidelines also recommends atezolizumab as an adjuvant therapy for patients who have previously received adjuvant chemotherapy with completely resected stage IIB to IIIA or high-risk stage IIA NSCLC and PD-L1 ≥1%, according to clinical trial results from another phase 3 randomized trial-IMpower010 which compared adjuvant therapy with atezolizumab versus best supportive care in patients with resected early-stage NSCLC (12). For extensive-stage SCLC, atezolizumab plus carboplatin plus etoposide followed by maintenance atezolizumab, or durvalumab plus etoposide plus (carboplatin or cisplatin) followed by maintenance durvalumab as first-line therapy based on the clinical trial data from two phase 3 randomized trials: IMpower133 or CASPIAN (11, 19–21). In SCLC, the NCCN guidelines recommend carboplatin plus etoposide plus atezolizumab as the first-line systemic therapy, followed by maintenance atezolizumab for patients with extensive-stage SCLC, based on the clinical outcomes of the IMpower133 trial (21–23). According to the results from the CASPIAN trial, the NCCN panel recommends durvalumab plus etoposide plus (carboplatin or cisplatin) as a first-line systemic therapy option, followed by maintenance durvalumab for patients with extensive-stage SCLC (20, 22) (11, 24, 25).
Although immunotherapy brings clinical benefits and gets involved in first-line therapy, a large number of patients do not respond to immune checkpoint inhibitors (26, 27). And for immune checkpoint inhibitors used in adjuvant and neoadjuvant therapy, there lacks a clearly defined best target population, which needs further exploration (28). Immune-related adverse events (irAEs) also need further research, the incidence of irAEs across agents and trials ranges from 15% to 90%, and approximately 0.5% to 13% of them happened severe irAEs which need immunosuppression or treatment discontinuation in monotherapy (29, 30). In brieg summary, there are still a lot of unsolved questions in immunotherapy requiring further exploration to bring patients better outcomes and fewer adverse events.
3 Immune checkpoints regulation in lung cancer
Most study of immune checkpoint regulation in lung cancer has focused on the regulation of PD-L1. Therefore, in this section, the regulatory mechanisms of PD-L1 are primarily discussed (Figure 1).
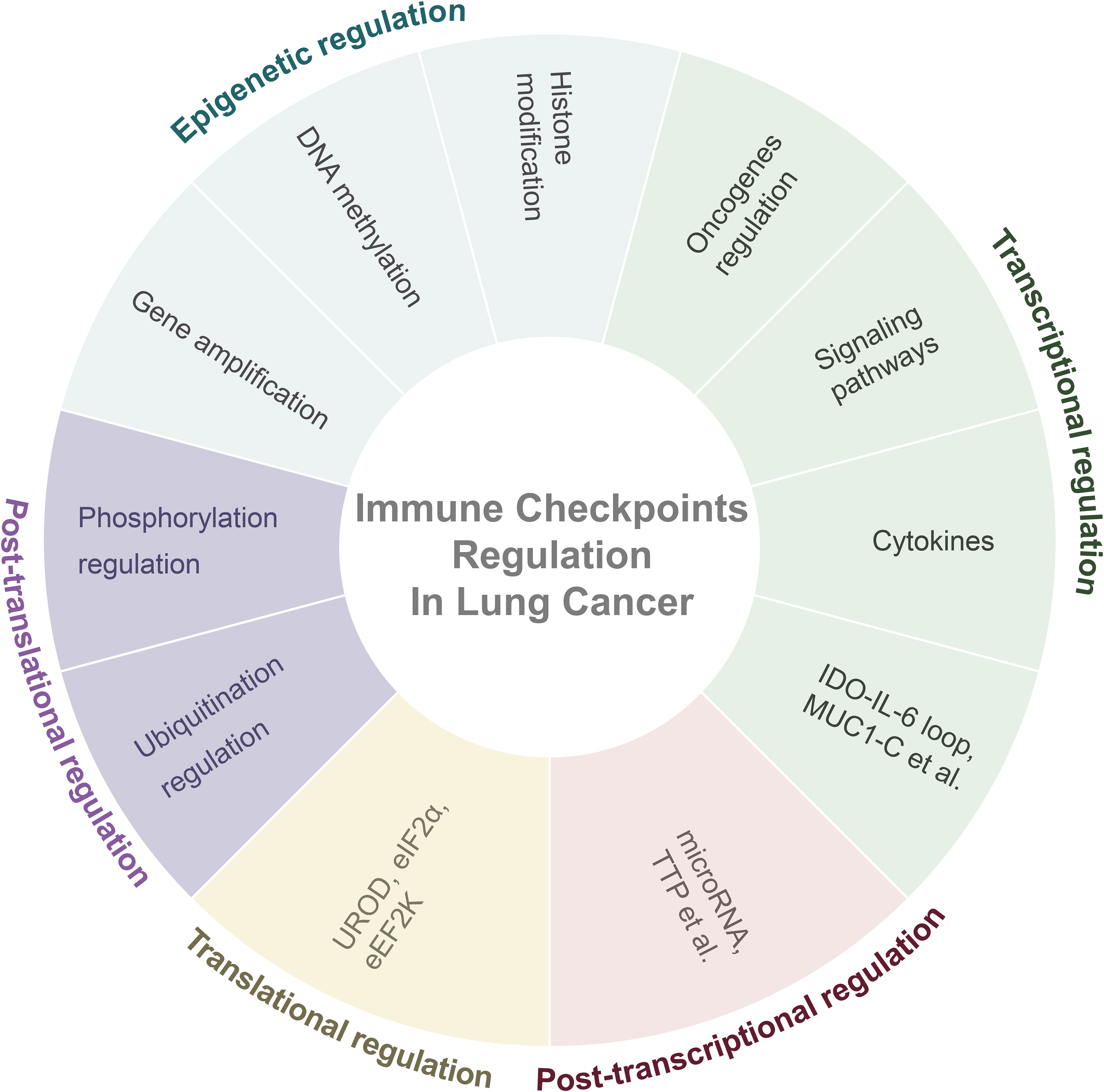
Figure 1 Overall regulatory mechanisms of immune checkpoints in lung cancer. Abbreviation: IDO= Indoleamine-2,3-dioxygenase; IL-6= Interleukin-6; MUC1-C= Mucin 1-C; TTP= AU-rich element-binding protein tristetraprolin; UROD= Uroporphyrinogen Decarboxylase; eEF2K= Eukaryotic Elongation Factor 2 Kinase.
3.1 PD-L1 regulation
3.1.1 Epigenetic regulation
Epigenetic regulation can alter chromatin structure and control the expression of PD-L1 by modulating the recognition and binding between transcription factors and DNA elements instead of altering the DNA sequence (31), for instance, epigenetic regulation of lung cancer PD-L1 involves gene amplification, DNA methylation, and histone modifications.
3.1.1.1 Gene amplification
In NSCLC, around 1.9% of patients have PD-L1 gene amplification and approximately 8.2% of them exhibit PD-L1 gene amplification at 9p24.1 which is associated with the JAK-STAT pathway and concurrent amplification of the JAK2 gene (32–34).
3.1.1.2 DNA methylation
DNA methylation involves the covalent modification of cytosine at the 5-position of nucleotides (35), regulates gene expression by controlling chromatin structure, DNA stability, and conformation (36). Study showed that TGF-β1 can inhibit the activation of DNMT1 (DNA methyltransferase-1), thereby demethylating the CD274 (PD-L1) promoter to upregulate PD-L1 expression (37). However, studies suggest that methylation negatively correlates with PD-L1 expression in NSCLC biopsies, yet the correlation is relatively weak, the role of methylation in PD-L1 expression remains contentious and the potential benefits of demethylation therapy combined with immunotherapy remain debatable (38).
3.1.1.3 Histone modification
Histone methylation plays a role in regulating the expression of PD-L1. Combined with histone methyltransferase, EZH2 (Enhancer of Zeste homolog2) can suppress PD-L1 expression by increasing trimethylation of histone H3K27 at the promoter, which is a regulatory mechanism that depends on HIF-1α in lung cancer (39).
Histone acetylation also plays a role in the regulation of PD-L1 expression. In drug-resistant A549/CDDP cell line, the downregulation of COP1 (E3 ligase constitutive photomorphogenesis protein 1) promotes the activation of the JNK/c-Jun pathway, subsequently inhibiting the expression of histone deacetylase 3 (HDAC3). This ultimately enhances the acetylation of histone H3 at the CD274 promoter resulting in the upregulation of PD-L1 expression (40). Also, a positive correlation has been observed between elevated PD-L1 expression in cisplatin-resistant NSCLC patients and c-Jun expression while a negative correlation exists with HDAC3 (40).
However, another study suggests that the application of HDAC inhibitors can downregulate PD-L1 expression levels in afatinib-resistant NSCLC patients (41). Another research observed a positive correlation between HDAC10 and PD-L1 expression levels in NSCLC patients through immunohistochemistry (42). These findings contradict results mentioned above, indicating the need for a further understanding of the role histone acetylation playing in PD-L1 regulation.
3.1.2 Transcriptional regulation
3.1.2.1 Oncogenes regulation
3.1.2.1.1 KRAS
KRAS mutations have been found to upregulate PD-L1 expression through the MAPK pathway, in which AP-1 family plays a vital role. Chen et al. found that the upregulation of PD-L1 in KRAS mutation lung adenocarcinoma is correlated with p-ERK regardless of p-AKT (43). Sumimoto et al. further discovered that KRAS mutations enhance PD-L1 transcription through the MAPK pathway by binding the downstream AP-1 component cJUN to the CD274 enhancer (44). KRAS mutations were identified as an independent factor inducing PD-L1 expression in the study of premalignant human bronchial epithelial cells, regulates PD-L1 expression through the MEK-ERK pathway and part of the MAPK pathway, with downstream involvement of the AP-1 family member FRA1 (FOS-related antigen 1) (45).
3.1.2.1.2 EML4-ALK
The EML4 (Echinoderm microtubule-associated protein like-4) - ALK (anaplastic lymphoma kinase) fusion has been detected in approximately 4-8.1% of NSCLC patients (46, 47). A study discovered lung adenocarcinoma patients with EML4-ALK fusion have higher levels of PD-L1 on tumor cells than patients without EML4-ALK fusion. The expression of PD-L1 of patients with the EML4-ALK fusion is positively correlated with p-ERK, p-STAT3, and p-AKT (48). Hong et al.’s research indicates that EML4-ALK fusion upregulates PD-L1 expression through the p-ERK1/2 and p-AKT signaling pathways, but it is not associated with the JAK3/STAT3 pathway (49). A study of lung adenocarcinoma demonstrated that EML4-ALK fusion can upregulate p-STAT3 levels, then p-STAT3 upregulates PD-L1 expression by binding to the CD274 promoter. Additionally, EML4-ALK fusion can upregulate HIF-1α under hypoxic conditions, and inhibit HIF-1α ubiquitination and degradation. Then HIF-1α binds to the CD274 promoter to upregulate PD-L1 expression. The authors also emphasized the synergistic role of HIF-1α and STAT3 in promoting PD-L1 expression under hypoxic conditions (50). MAPK, JAK-STAT, and PI3K-AKT pathways involved in EML4-ALK regulated PD-L1 expression, and HIF-1α effective at specific conditions.
3.1.2.1.3 EGFR
Akbay et al. initially mentioned a positive correlation between EGFR mutations and PD-L1 expression, however, their study did not further focus on EGFR mutations’ mechanisms regulating PD-L1 expression until subsequent research explored that (51). Studies suggested that EGFR mutations can upregulate PD-L1 expression through the AKT-STAT3 and p-ERK1/2/p-c-Jun pathways (52, 53). Okita et al. found that the baseline expression of PD-L1 in NSCLC was positively correlated, while EGFR levels negatively correlated with HER2 levels. However, EGF-induced PD-L1 expression was merely associated with EGFR levels. Both baseline and EGF-induced PD-L1 expression were partially associated with the PI3K/AKT and JAK/STAT pathways (54). In EGF-stimulated EGFR mutant NSCLC, EGF induced IL-6 secretion, and IL-6, subsequently induced PD-L1 expression through the JAK/STAT3 pathway (55). Guo et al.’s research suggested that EGFR mutations regulate PD-L1 expression through multiple pathways with interconnections among them, including PI3K-AKT-mTOR-HIF-1α, NF-κB, and the MAPK pathway (56). In lung adenocarcinoma with EGFR mutations, the EMT-related receptor tyrosine kinase AXL was positively correlated with PD-L1 expression. Inhibition of AXL kinase activity could downregulate PD-L1 mRNA (57, 58). Other studies put forward that in EGFR tyrosine kinase inhibitors (TKIs) resistant cells, PD-L1 levels were positively correlated with E-cadherin and EGFR phosphorylation levels, yet the exact mechanism remains unrevealed (59, 60). To summarize, EGFR mutation regulates PD-L1 mainly through JAK-STAT, PI3K-AKT, MAPK, and NF-κB pathways that interconnect with each other. However, negative correlation between PD-L1 expression and EGFR mutation levels are also proposed, so the regulation of PD-L1 by EGFR mutations requires further research (61).
PD-L1 expression in EGFR mutant lung cancer cells is generally higher than that in wild-type (62). However, research found that PD-L1 expression was associated with the STAT3, AKT, and ERK pathways in wild-type EGFR NSCLC, and ubiquitin ligases Cbl-b and c-Cbl could downregulate PD-L1 expression by inhibiting phosphorylation of STAT3/AKT/ERK pathways (63). The TUSC2 (also known as FUS1) gene can downregulate the activation of multiple tyrosine kinases, including EGFR (64, 65). Cao et al. found that TUSC2 in NSCLC could inhibit mTOR, thereby downregulating PD-L1 expression, and Dai et al. suggested that TUSC2 restoration in wild-type EGFR could downregulate PD-L1 expression through the mTOR pathway (66, 67). In wild-type EGFR cells, tumor cell-intrinsic CTLA4 could upregulate PD-L1 expression through the MEK-ERK pathway downstream of EGFR (68). Stutvoet et al. studied on non-EGFR mutant lung adenocarcinoma, found that EGF could induce IL-6 secretion in cells. IL-6, eventually induced PD-L1 expression through the STAT1 pathway. The authors also suggested that the MAPK pathway plays an essential part in regulating PD-L1 expression, as it can upregulate PD-L1 mRNA expression and increase the stability of PD-L1 mRNA (69). Generally speaking, PI3K-AKT, JAK-STAT, and MAPK are the pathways that participate in the regulation of PD-L1 in wild-type EGFR lung cancer.
Some studies have shown that EGFR TKIs can downregulate PD-L1 expression levels. EGFR combined with gefitinib can lower the expression of PD-L1 in EGFR mutant NSCLC through the NF-κB pathway (62, 70). However, Okita et al.’s research indicated that gefitinib cannot downregulate baseline PD-L1 expression which caused by EGFR mutations, but can inhibit EGF-induced upregulation of PD-L1. EGFR TKIs’ mechanism of regulating PD-L1 still remains unknown (54).
Although most studies suggested a positive correlation between EGFR mutations and PD-L1 expression, some studies indicated that EGFR mutant patients have a lower response to PD-1/PD-L1 treatment (71–74). Anti-PD-L1 immunotherapy and EGFR TKI treatment showed no synergistic effect but increasing toxicity in clinical studies, which suggests more exploration of the regulation of PD-L1 by EGFR in clinical applications (75, 76).
3.1.2.1.4 MYC
The MYC oncogene is overexpressed in 41% of NSCLC and is associated with the loss of cell differentiation (77). Studies have found a positive correlation between MYC expression levels and PD-L1 levels. MYC can directly bind to the PD-L1 gene promoter, then promote PD-L1 expression (78–80).
3.1.2.1.5 PTEN
PTEN (phosphatase and tensin homolog) is a gene that functions as a tumor suppressor by inhibiting the PI3K-AKT pathway. It is one of the most common mutated tumor suppressor genes (81, 82). In lung squamous cell carcinoma, approximately 15% of cases exhibit PTEN mutations (83). Studies have shown that PTEN loss in lung cancer is associated with high PD-L1 expression (84). The activation of the PI3K-AKT pathway, regulated by PTEN, is often linked to the upregulation of PD-L1 (4, 85). PTEN knockout significantly upregulates the expression of PI3K-AKT pathway (81). Therefore, current research suggests that the functional impairment of PTEN primarily upregulates PD-L1 expression through the PI3K-AKT pathway.
3.1.2.2 Signaling pathways
3.1.2.2.1 MAPK pathway
The MAPK pathway regulates PD-L1 expression in KRAS mutation NSCLC and EGFR mutation mentioned previously. Studies by Della Corte and Demuth have shown that the MAPK pathway can control PD-L1 expression at the transcriptional level by regulating PD-L1 mRNA levels (86, 87). It is found that MAPK can stimulate PD-L1 transcription through NF-κB as it can bind to the CD274 promoter to promote PD-L1 transcription, which suggests complex regulatory interactions among different pathways in PD-L1 regulation (86). The authors’ research further indicates that inhibiting key factors of the MAPK pathway, such as MEK, can increase the immunogenicity of tumor cells leading to the upregulation of MHC-I (major histocompatibility complex class-I) expression and increased mRNA levels of IFN-γ, IL-6, IL-1B, and TNF-α. Conversely, it downregulates the expression of other immune checkpoint molecules like CTLA-4, TIM-3, and LAG-3, thereby promoting various immune responses (86).
In addition to EGFR and KRAS mutations, MET (mesenchymal-epithelial transition factor) amplification positively correlates with PD-L1 expression. Inhibiting MET expression can downregulate PD-L1 levels (88, 89). MET also regulates PD-L1 expression through the MAPK pathway (87). Nevertheless, MET primarily regulates PD-L1 via the MAPK and PI3K/AKT pathways rather than the NF-κB pathway in EGFR-TKI-resistant NSCLC cells and whether NF-κB is involved in the MAPK pathway’s regulation of PD-L1 requires further investigation (90).
3.1.2.2.2 Hippo pathway
The Hippo pathway regulates tumor immunity highly associated with cancer (91). Several studies have indicated activation of the Hippo pathway proteins YAP (Yes-associated protein) and TAZ (WW domain-containing transcription regulator 1) can upregulate PD-L1 expression in NSCLC. Further research has shown that YAP and TAZ can form complexes with TEAD family proteins, bind to the CD274 promoter or enhancer, thus enhance PD-L1 expression (92–95). Other upstream factors like PKA (protein kinase A) and LATS (large tumor suppressor) also inhibit YAP and TAZ. In this regard, PD-L1 expression can be promoted by upregulating TAZ/YAP (92, 95).
3.1.2.2.3 ADORA1-ATF3 pathway
The ADORA1-ATF3 pathway also modulates the transcriptional regulation of PD-L1. Research by Liu et al. indicates that ADORA1 (adenosine A1 receptor) inhibition leads to increase in ATF3 (cAMP-dependent transcription factor 3) levels, which binds to the CD274 promoter and upregulates PD-L1 expression (96).
3.1.2.3 Cytokines
3.1.2.3.1 IFN-γ
The cytokine IFN-γ is considered as a primary inducer of PD-L1 expression in various tumors (97, 98). Several studies have suggested that IFN-γ promotes the expression of IRF-1 (interferon regulatory factor-1) by activating the JAK-STAT pathway in NSCLC. IRF-1 can bind to the CD274 promoter and facilitate PD-L1 transcription (99). Meanwhile, IRF-1 also plays a crucial role in both constitutive and IFN-γ-induced PD-L1 expression (100).
Lv et al. found that the JAK-STAT pathway activated by IFN-γ can promote the binding of TET1 (ten-eleven translocation methylcytosine dioxygenase 1) to IRF-1, regulating IRF-1 demethylation and thereby promoting PD-L1 expression (101). Lai et al. demonstrated a negative correlation between the methylation levels of IRF1/7 and PD-L1 expression and Decitabine demethylates IRF1/7 leading to the restoration of PD-L1 expression levels (102). In contrast to IRF-1, IRF-2 competitively binds to the CD274 promoter, inhibiting IRF-1’s ability to promote PD-L1 expression (103).
Gao et al. discovered that IFN-γ affects downstream IRF-1 to regulate PD-L1 expression through the JAK2-STAT1 pathway, and participates in regulation through the PI3K-AKT pathway. Further research indicated that the PI3K-AKT pathway also acts through STAT1 to control PD-L1 expression in IFN-γ-induced PD-L1 expression, emphasizing the primary role of the JAK-STAT pathway and it suggested that STAT3 does not participate in IFN-γ-mediated PD-L1 regulation (104). It is mentioned before in EGFR also demonstrated that IFN-γ can induce PD-L1 expression through STAT1, and inhibition of the EGFR pathway could partially affect IFN-γ-mediated PD-L1 expression (69). However, another research suggested that IFN-γ upregulates PD-L1 expression in lung adenocarcinoma cells through the PI3K/AKT and JAK/STAT3 pathways, which need for further research support to prove the potential of STAT3 in IFN-γ induced PD-L1 expression (105).
Besides IFN-γ, Morimoto et al. demonstrated that type I interferon IFN-β increases STAT1 mRNA levels and ultimately upregulates PD-L1 expression via IRF9 through the JAK1/2-STAT1 pathway and independently of the mTOR pathway (106).
3.1.2.3.2 HIF-1α
Hypoxia-inducible factor-1α (HIF-1α) is positively correlated with PD-L1 mRNA and protein expression in NSCLC (56, 107, 108). In myeloid-derived suppressor cells (MDSCs) of LLC (Lewis Lung Carcinoma) mice, HIF-1α can directly bind to the hypoxia-response element (HRE) in the CD274 promoter, promoting PD-L1 transcription (109). As mentioned earlier, it has also been observed that HIF-1α can bind to the CD274 promoter to upregulate PD-L1 expression in EML4-ALK fusion NSCLC (50).
3.1.2.3.3 TGF-β
TGF-β is known to promote tumor epithelial-mesenchymal transition (EMT) and inhibit anti-tumor immunity (110). It can upregulate the expression of DC cell immune checkpoints PD-L1 in an in vitro lung cancer microenvironment model (111). Researchers found that TGF-β and TGF-β1 can increase PD-L1 expression in NSCLC cells (112, 113).
David et al. further investigated how TGF-β1 upregulates PD-L1 expression. Their research indicates that TGF-β1 upregulates PD-L1 expression by enhancing PD-L1 transcription levels rather than increasing PD-L1 mRNA stability. This upregulation process might be dependent on Smad2. They found that the transcriptional start site of CD274 has Smad-binding elements, suggesting that Smad2 might upregulate PD-L1 expression by binding to the CD274 promoter, and further research is needed to validate these results (114). The study also suggests that in A549 cells, TGF-β1 may partially upregulate PD-L1 expression through the PI3K pathway.
Interestingly, the research also indicates that TGF-β1 can regulate microRNAs associated with PD-L1 mRNA regulation despite experimental results suggesting that TGF-β1 cannot increase the stability of PD-L1 mRNA (114). More research is needed to understand how TGF-β regulates PD-L1 expression. Conversely, PD-L1 can activate the TGF-β/Smad pathway and participate in primary resistance to EGFR-TKIs in EGFR mutant NSCLC cells (115).
Moreover, studies have shown that TGF-β can impede the efficacy of anti-PD-1/PD-L1 treatments, and the immunosuppressive effects of TGF-β and PD-L1 are independent and complementary (116, 117). Combining anti-TGF-β with anti-PD-1/PD-L1 therapies enhances the therapeutic effectiveness and overcomes treatment resistance (118–121). Therefore, the development of anti-TGF-β/PD-L1 bispecific antibodies, such as YM101, BiTP, and M7824, represents a valuable direction in anti-tumor research (122–126).
3.1.2.3.4 Other cytokines
Other cytokines, such as IL-27, have been shown to upregulate PD-L1 mRNA and cell surface protein levels in A549 cells (127). Nevertheless, not all cytokines positively regulate PD-L1 expression. For instance, research by Schalper et al. demonstrated that IL-10 does not impact PD-L1 expression, while Gao et al.’s study found that IL-10 can inhibit IFN-γ-induced STAT1 phosphorylation in lung adenocarcinoma, thereby suppressing IFN-γ-induced upregulation of PD-L1 (128, 129).
Other mechanisms in transcriptional regulation include MUC1, a frequently overexpressed transmembrane glycoprotein in NSCLC. Its subunit, MUC1-C, can form a complex with NF-κB’s p65 subunit and bind to the CD274 promoter, thereby enhancing PD-L1 expression (130).
3.1.3 Post-transcriptional regulation
Post-transcriptional regulation of PD-L1 is primarily achieved through binding to the 3’ untranslated region (3’ UTR) of PD-L1 mRNA. The factors involved in this regulation are primarily microRNAs (miRNAs) and a subset of other regulatory factors.
MicroRNAs (miRNAs) generally exert their inhibitory effects on target genes by binding to the 3’ UTR of mRNA, thereby promoting their degradation or inhibiting their transcription. Experimental evidence has demonstrated that microRNAs miR-34, miR-140, let-7, miR-200, and miR-200a-3p can bind to the 3’ UTR of PD-L1 mRNA, thereby downregulating the expression of PD-L1. miR-155-5p theoretically possesses binding sites in the 3’ UTR of PD-L1 mRNA but lacks experimental validation (131–137).
Other factors can regulate the expression of PD-L1 by modulating the levels of these miRNAs. For instance, wild-type p53 can bind to the promoter of miR-34, upregulating its expression (136). Long non-coding RNAs (lncRNAs) often act as molecular sponges, directly inhibiting miRNA function: Circ-CPA4 inhibits let-7 (132), MALAT1 inhibits miR-200a-3p (133), lncRNA LINC01140 can inhibit miR-377-3p and miR-155-5p, which are predicted to bind to the 3’ UTR of PD-L1 mRNA (138). MiR-142 has potential binding sites in PD-L1 mRNA and can downregulate PD-L1 expression, while lncRNA FGD5-AS1 acts as a molecular sponge directly interacting with miR-142 (139). One of Circular RNA (circRNA) named hsa_circRNA_002178 can also sequester miR-34 (140). Additionally, the non-RNA factor ZEB1 (zinc-finger E-box-binding homeobox 1) can relieve the inhibitory effect of miR-200 on PD-L1 expression (137).
Apart from miRNAs, other factors can directly act on PD-L1 mRNA. One such factor is TTP (AU-rich element-binding protein tristetraprolin), which can bind to the 3’ UTR of PD-L1 mRNA and inhibit PD-L1 expression (141). In addition, the AU-rich element-binding protein HuR, regulated by the Ang II (Angiotensin II)/AGTR1 pathway, can stabilize PD-L1 mRNA by binding to its 3’ UTR, thereby upregulating PD-L1 expression (142). Furthermore, research indicates that variant single nucleotide polymorphisms within the binding sites in the 3’ UTR of PD-L1 mRNA can disrupt this inhibitory effect (143).
Interestingly, miRNAs can not only directly regulate PD-L1 expression at the post-transcriptional level but can also indirectly influence PD-L1 by modulating related pathways. For instance, miR-135 can promote the phosphorylation of kinases in the JAK/STAT pathway, leading to an upregulation of PD-L1 expression (144). MiR-3127-5p has been found to increase PD-L1 expression by promoting STAT3 phosphorylation (145). MiR-181a and miR-940, by inhibiting the ubiquitin ligase Cbl-b and c-Cbl, activate the STAT3/AKT/ERK pathway, resulting in elevated PD-L1 levels (63). Additionally, miR-197 downregulates PD-L1 expression by inhibiting the CKS1B/STAT3 pathway (146).
In addition, the long non-coding RNA (lncRNA) NKX2-1-AS1 can inhibit the transcription of CD274 (PD-L1) by preventing the binding of NKX2-1 protein to the CD274 promoter (147). LncRNA SNHG12 enhances the stability and expression of PD-L1 and USP8 mRNA by binding to HuR. Simultaneously, USP8, through mediating deubiquitination, stabilizes PD-L1 protein (148).
3.1.4 Translational regulation
Currently, there is limited research on the translational regulation of NSCLC PD-L1. Suresh et al.’s study suggests that the deficiency of Uroporphyrinogen Decarboxylase (UROD) leads to a shortage of heme, which in turn activates an eIF2α kinase called Heme-Regulated Inhibitor (HRI). Then, phosphorylated eIF2α triggers a cascade of other kinases, inducing the integrated stress response (ISR) and global translation initiation inhibition. Under this stress, PD-L1 mRNA is preferentially translated. Moreover, eIF2α promotes the bypass of inhibitory upstream Open Reading Frames (uORFs), thereby facilitating PD-L1 translation (149). In another study, Wu et al. demonstrated that Eukaryotic Elongation Factor 2 Kinase (eEF2K) enhances the association between PD-L1 mRNA and active polysomes, leading to an upregulation of PD-L1 levels (150).
3.1.5 Post-translational regulation
Post-translational regulation of PD-L1 refers to the modulation of the PD-L1 protein. It primarily involves processes such as ubiquitination, phosphorylation, glycosylation, palmitoylation, acetylation, and others, all contributing to regulating PD-L1 at the protein level.
3.1.5.1 Ubiquitination regulation
Ubiquitination regulation encompasses both deubiquitination and ubiquitination of PD-L1. Deubiquitinase USP22 deubiquitinates PD-L1, preventing its proteasomal degradation (151). Furthermore, USP22 stabilizes CSN5, another deubiquitinase, through its deubiquitination activity. CSN5 has been shown in other tumors to stabilize PD-L1 protein through deubiquitination (152). And Wang et al. suggested that USP22 and CSN5 mutually enhance each other’s stabilizing effect on PD-L1 (151). Zhu et al.’s research revealed that deubiquitinases can upregulate PD-L1 protein levels in A549 cells. Their subsequent studies in other non-NSCLC cell lines found that deubiquitinase OTUB1 deubiquitinates PD-L1 by cleaving K48-linked poly-ubiquitin chains, stabilizing PD-L1. This study confirmed that OTUB1 can prevent the endoplasmic reticulum-associated degradation of PD-L1 (153).
However, not all deubiquitinases act to stabilize PD-L1. Xiong et al.’s research indicates that deubiquitinase USP8 downregulates PD-L1 protein levels by targeting K63-linked deubiquitination instead of K48-linked deubiquitination. More specifically, K63-linked deubiquitination antagonizes K48-linked deubiquitination, and deubiquitination of K63 leads to an increase in K48 ubiquitination. Therefore, USP8’s action is opposite to that of OTUB1, which deubiquitates PD-L1 at K48, promoting the degradation of PD-L1 protein (154). Nevertheless, USP8 mentioned above in lncRNA research suggests it can stabilize PD-L1 protein, so whether USP8 promotes or inhibits PD-L1 expression remains further explored (148).
Research on PD-L1 ubiquitination regulation brings up new points. The E3 ubiquitin ligase MARCH8 can interact with the N-terminal region of PD-L1 and ubiquitinate it, promoting the proteasomal degradation of PD-L1 (155). In other tumor types, members of the CMTM protein family have been shown to inhibit the ubiquitination of PD-L1 (156). In NSCLC-related research, CMTM6 has been found to co-localize with PD-L1, preventing PD-L1 from becoming a target of lysosome-mediated degradation (157). On the other hand, E3 ubiquitin ligase TRAF6 promotes K63 ubiquitination to stabilize PD-L1 (154).
3.1.5.2 Phosphorylation regulation
Phosphorylation and ubiquitination of the PD-L1 protein are closely interconnected. Wu et al. found that the EGFR inhibitor ES-072 can downregulate PD-L1 levels, and this process mainly depends on the ubiquitination of PD-L1 at K48 which leads to the proteasomal degradation of PD-L1. Further research revealed that ES-072 activates GSK3α through the AKT pathway. GSK3α phosphorylates PD-L1 at Ser279 and Ser283, and phosphorylation of PD-L1 promotes E3 ubiquitin ligase ARIH1 to ubiquitinate PD-L1 at K48. This demonstrates that phosphorylation and ubiquitination of PD-L1 act synergistically to promote its degradation (158).
Cha et al. discovered that AMPK can directly phosphorylate PD-L1 at Ser195. This induces abnormal endoplasmic reticulum mannose trimming during PD-L1 glycosylation, preventing PD-L1 from translocating to the cell membrane. Ultimately, this may lead to a reduction in PD-L1 expression through endoplasmic-reticulum-associated degradation (159).
The post-translational regulation of PD-L1 in NSCLC primarily involves ubiquitination and phosphorylation. There is also research indicating that mTOR can regulate PD-L1 expression by inhibiting its lysosomal protein degradation (160). In other types of cancer, there are additional modes of regulation, such as glycosylation, palmitoylation, and acetylation (161–163). The multifaceted regulation of PD-L1 post-translational regulation in NSCLC requires further investigation. The PD-L1 regulation mechanisms are summarized in Table 1.
3.2 IDO regulation
Indoleamine 2,3-dioxygenase (IDO) expression is identified as an independent negative prognostic factor in cancer (164, 165). IDO expression is associated with tumor-infiltrating forkhead box P3 positive regulatory T-cells (FoxP3+ Tregs) and is negatively associated with CD8+ cytotoxic T-cells (165). Currently, research on IDO regulation is rare. IL-27 can upregulate IDO mRNA levels and cell surface protein levels in A549 cells (127). IL-6 activates STAT3, which can bind to the IDO promoter, increasing IDO expression. The elevated IDO, in turn, promotes the transcription of IL-6 through AHR (aromatic hydrocarbon receptor), resulting in increased IL-6 secretion. This establishes an IDO–AHR–IL-6–STAT3 loop to sustain IDO expression (166). COX-2 (cyclooxygenase-2)/PGE2 (prostaglandin E2) pathway can support IDO1 constitutive expression in NSCLC; downstream β-catenin and ETV4 can bind to IDO1 promoter and promote IDO1 transcription (167).
3.3 GITRL regulation
Glucocorticoid-induced TNFR related protein (GITR) is expressed in various immune cells including T cells and natural killer cells. GITR can be activated by its ligand GITRL, leading to increased resistance to tumors and viral infections (168, 169). In an in vitro lung cancer microenvironment model, TGF-β has been shown to upregulate the expression of GITRL on DC cells (111). Further exploration is needed to command more specific details of mechanisms that can regulate GITR/GITRL in lung cancer.
4 Natural products targeting immune checkpoints or combined with immunotherapy
4.1 Natural products targeting the PD-1/PD-L1 axis
Most research focuses on natural products targeting the PD-1/PD-L1 axis in lung cancer. The strategy includes blocking the interaction between PD-1 and PD-L1 and influencing PD-1/PD-L1 expression (upregulation or downregulation).
4.1.1 Natural products block PD-1/PD-L1 interaction
The interaction between PD-1 and PD-L1 can suppress the proliferation, activation, and function of CD8+ T cells (170). The combination of PD-1 and PD-L1 is reversible and the competitive binding to PD-L1 or PD-1 can inhibit the interaction between these immune checkpoints (171). The blockade of PD-1/PD-L1 interaction further restores T cells’ anti-tumor function (171).
Quercetin, a flavonoid derived from various fruits and vegetables, can inhibit progression and enhance apoptosis in various types of cancer (172, 173). Quercetin can inhibit the interaction between His-HA-PD-1 and His-HA-PD-L1 with an IC50 value of 5μM in the ELISA assay (174). Quercetin interacts with PD-L1 more strongly because the dissociation constant of quercetin to PD-L1 is smaller than that to PD-1. It can enhance PMBC cells to kill PD-L1 high-expressed NCI-H460 cells. The researcher further proved that quercetin can inhibit PD-1/PD-L1 binding in HEK293 cells and suppress MDA-MB-231 xenografted mouse tumor growth by reactivating T cells. Nevertheless, the effect of quercetin inhibiting PD-1/PD-L1 in lung cancer needs further exploration.
4.1.2 Natural products downregulate tumor cell PD-L1 expression
Natural products can downregulate PD-L1 expression through different mechanisms. Most of them regulate PD-L1 at the transcriptional level, mainly through PI3K-AKT, JAK-STAT, NF-κB, and p53 signaling pathways. Some natural products can inhibit PD-L1 protein synthesis, promote PD-L1 protein degradation, and promote PD-L1 extracellular secretion to suppress PD-L1 expression (Table 2). All these natural products aim to inhibit tumor cells and activate immune cells.
Gallic acid, silibinin, and lycopene can inhibit PD-L1 expression through PI3K-AKT signaling. Gallic acid is derived from fruits, plants, and green tea (204, 205). It can prevent carcinogenesis, inhibit cancer cell proliferation, and induce cancer cell apoptosis (206, 207). Gallic acid can inhibit PD-L1 protein and mRNA levels in A549 and H292 cells. Further study proves that gallic acid binds to and therefore inhibits EGFR phosphorylation, binging about PI3K/AKT phosphorylation reduction and its downstream p53 protein and mRNA expression increase, and finally, through miR-34a to downregulate PD-L1 expression (175). Silibinin is extracted from Silybum marianum, a plant traditionally used to treat liver diseases; it can suppress tumorigenesis in various cancer types and inhibit lung cancer proliferation and angiogenesis (208, 209). Alexis et al. proved that silibinin inhibits NSCLC cell PD-L1 expression by suppressing PI3K/AKT molecular phosphorylation, and silibinin also influences PD-L1 expression through JAK2/STAT5 signaling (176). Lycopene, mainly present in tomatoes, can block AKT signaling by inhibiting AKT phosphorylation from downregulating IFN-γ induced PD-L1 protein and mRNA expression in LLC cells (177, 178). Epidermal growth factor receptor (EGFR) is the main receptor influencing PI3K-AKT signaling; gallic acid and silibinin can bind to EGFR and inhibit its phosphorylation, influencing the downstream signaling pathway (175, 176).
Fraxinellone, EGCG, luteolin, myricetin, oleic acid, silibinin, ursolic acid, butein, triptolide, and andrographolide can downregulate the PD-L1 expression through the JAK-STAT signaling pathway. D. dasycarpus is a traditional herb with various medical functions (210–212). Fraxinellone, a limonoid extracted from D. dasycarpus, can suppress the phosphorylation of JAK1 and JAK2 and then inhibit STAT3 transcription and phosphorylation, and it also inhibits HIF-1α protein synthesis; both pathways can reduce PD-L1 expression (179). (–)-epigallocatechin gallate (EGCG) is the main polyphenol in green tea whose cancer prevention effect has been widely reported (213). EGCG can reduce the p-STAT1 level, and further analysis showed that the reduction of p-STAT1 is highly correlated to the down-regulation of cell-surface PD-L1. EGCG can also influence the PI3K-AKT pathway, but this pathway failed to reduce surface PD-L1 (180). Luteolin can be discovered in various fruits and vegetables (181, 182). Luteolin and its derivative, apigenin, can potentially enhance chemotherapy (214, 215). Jiang et al. discovered that apigenin and luteolin inhibit cell surface PD-L1 and PD-L1 mRNA expression through downregulating STAT3 phosphorylation (183). Myricetin, also derived from various fruits and vegetables, possesses immune regulation and anti-tumor effects (216–221). Chen et al. discovered that myricetin inhibits PD-L1 protein and mRNA expression by inhibiting IFN-γ induced STAT1 and STAT3 phosphorylation (184). Nobiletin is from citrus peels that induce apoptosis function in lung cancer (222). Nobiletin downregulated the phosphorylated EGFR, JAK2, and STAT3 to suppress cell-surface protein and mRNA levels of PD-L1. Nobiletin can also promote miR-197 expression, inhibiting the STAT3 pathway (185). Oleic acid is an extract of olive oil and can be transformed in the gut and become oleoylethanolamide (OEA) (223, 224). Oleic acid has an anti-tumor effort, and oleic acid and OEA can block IFN-γ induced STAT1 phosphorylation to suppress PD-L1 protein and mRNA expression (186). Ursolic acid, derived from many fruits and herbs, inhibits cancer cell proliferation and autophagy (225–227). Ursolic acid can bind to EGFR and block its activation, suppressing downstream JAK2 and STAT3 phosphorylation. STAT3 can bind to the CD274 promoter and promote PD-L1 mRNA synthesis, thus upregulating PD-L1 protein expression (187). Butein is derived from Rhus verniciflua Stokes bark, and it can inhibit tumor angiogenesis, invasion, and metastasis (228, 229). Butein can inhibit STAT1 transcription and downregulate IFN-γ induced PD-L1 expression (188). Triptolide is extracted from Tripterygium wilfordii Hook. F., and Triptolide has been proven to regulate PD-L1 in other tumor cell lines, such as breast cancer and glioma (230–232). Triptolide can inhibit STAT3 to inhibit IFN-γ induced PD-L1 expression; it also inhibits JAK1 and JAK2 expression, STAT1 phosphorylation, and IRF1 protein level, resulting in PD-L1 transcriptional inhibition (189). Andrographolide (AD), extracted from Andrographis paniculate (Burm.f.) Nees (A. paniculate), was reported to possess anti-inflammatory and anti-cancer characteristics (233–235). AD can downregulate IFN-γ induced PD-L1 expression through downregulating the phosphorylation of STAT3 (190).
Ginsenoside Rg3 and Rk1 can downregulate PD-L1 expression through the NF-κB signaling pathway and Arsenic sulfide through the p53 signaling pathway. Panax Ginseng is widely used in traditional Chinese medicine to enhance immunity (236, 237). Ginsenoside Rg3 and Rk1 derived from Panax Ginseng can attenuate PD-L1 expression. Rg3 and Rk1 inhibit the phosphorylation of p65, while Rk1 also inhibits p65 expression and IKKα and IκBα phosphorylation, and Rg3 can also affect PI3K-Akt signaling (191, 192). Realgar derivative Arsenic sulfide (As4S4) has anti-tumor activities in several cancers (238, 239). It suppresses PD-L1 protein expression by upregulating p53 and miR-34a-5p levels (193).
Evodiamine and Nemania sp. EL006872 can downregulate PD-L1 expression through other mechanisms. Evodiamine is derived from Tetradium with anti-tumor activity (240, 241). MUC1-C can upregulate PD-L1 expression, and Jiang et al. discovered that evodiamine downregulates MUC1-C mRNA and protein expression and correlates with PD-L1 suppression (194). Nemania sp. EL006872 is an endolichenic fungi isolated from lichen Bryoria fuscescens (Gyelnik) Brodo and D. Hawksw; its secondary metabolites (mainly radianspenes C and D, and dahliane D) downregulate the aromatic hydrocarbon receptor (AHR) therefore decreasing surface PD-L1 expression in benzo[a]pyrene treated H1975 cells (195).
Licochalcone A, berberine, and SA-49 can influence protein synthesis or degradation of PD-L1. Licochalcone A (LCA) is derived from the root of Glycyrrhiza inflata Batalin. LCA induces apoptosis and decreases viability in lung cancer cells. That LCA abolishes PD-L1 protein synthesis induced by IFN-γ was proved by Yuan et al. using the Click-iT protein synthesis assay (196). Berberine is derived from the traditional Chinese medicine Coptis chinensis and has an anti-tumor effect with less cytotoxicity to normal cells (242, 243). Berberine can ubiquitinate PD-L1 and induce PD-L1 protein degradation without influencing PD-L1 transcription (197). Platycodin D (PD), a natural product isolated from Platycodon grandiflorum (244), decreases lung cancer cell surface PD-L1 levels by triggering PD-L1 extracellular release (200). Aloperine, derived from Sophora alopecuroides L., possesses an anti-tumor effect (245, 246). And its derivate, SA-49, was discovered can trigger PD-L1 degradation (199). Further exploration showed SA-49 inhibits GSK3β activation, inactivated GSK3β enhanced MITF transcription activity, and MITF finally promoted lysosome biogenesis, triggering PD-L1 lysosomal degradation (199).
Usnic acid derived from Usnea diffracta Vain and Lac water extract (active components laccaic acid A, B, C, and E) derived from lac insects can downregulate PD-L1 protein expression in lung cancer cells (201, 202, 247). While the researchers further explore related pathways in other tumor cells, such as Hela cells and melanoma cells, the mechanism of these natural products regulating PD-L1 expression in lung cancer needs further exploration. Acorus calamus L. Polysaccharide can suppress PD-L1 levels in LLC mice but also needs further exploration of the mechanisms (203).
4.1.3 Natural products upregulate tumor cell PD-L1 expression
PD-L1 expression in tumor cells is closely related to the effectiveness of anti-PD-L1 therapy; thus, some researchers sought to find natural products that increase PD-L1 expression (Table 3) (254).
Sophora alopecuroides Linn (SAL) is a Chinese herb with immunomodulating and anti-tumor functions (255). Sophocarpine (Sop) is one of the Alkaloids from SAL, and it can upregulate ADORA1 and ATF3 expression to increase the mRNA and protein level of PD-L1 (248).
Nagilactone E (NLE) is a diterpenoid derived from Podocarpus nagi seed oil—which can inhibit NSCLC cell migration and invasion (256, 257). NLE can promote the expression and phosphorylation of JNK to phosphorylate c-Jun and then upregulate mRNA, protein, and membrane PD-L1 expression (249).
Resveratrol is a polyphenol from fruits and vegetables. Studies have found that resveratrol possesses anti-proliferation, anti-migration, and enhanced chemotherapy properties. Resveratrol can inhibit Axin2 transcription, destabilize β-catenin, and promote β-catenin/TCF binding to CD274 promoter, thus upregulating PD-L1 expression (250).
Z-guggulsterone (Z-GS), extracted from the gumresin of the Commiphora mukul tree, can reduce growth and induce apoptosis in cancer cells (258, 259). Z-GS can inhibit the farnesoid X receptor, a transcription factor that inhibits PD-L1 expression, which is the partial reason Z-GS promotes PD-L1 expression (260). Z-GS can also activate PI3K/AKT (promote AKT phosphorylation) and MEK/Erk1/2 (promote ERK phosphorylation) pathways to induce PD-L1 expression (252).
Sponges extractive fascaplysin can upregulate PD-L1 expression and enhance anti-PD-1 immunotherapy in NSCLC cells and LLC tumor-bearing mice (253, 261). Fascaplysin combined with anti-PD-1 immunotherapy promoted CD4+ and CD8+ T cell infiltration. However, the mechanism fascaplysin influences PD-L1 remains unclear (253).
4.1.4 Natural products influence PD-1 or PD-L1 on immune cells
Some natural products can influence PD-1 or PD-L1 expression on immune cells, providing another anti-tumor method (Table 4).
Tumor-educated DCs can inhibit CD4(+) T cell proliferation and suppress T cell response, β-glucan can downregulate tumor-educated dendritic cells surface PD-L1 expression in LLC mice, which enhances priming of Th1 cells and CD8+ T cells while decreasing Treg cell differentiation (262, 267). Cordyceps militaris polysaccharide can inhibit M2 macrophage PD-L1 expression, induce polarization of M2 macrophages to M1, and reverse the immunosuppression effect of M2 macrophages on T lymphocytes (263). Tussilago farfara L. Polysaccharides can decrease peripheral CD274+ lymphocytes in LLC mice (264).
Platycodon grandiflorum (PG) is used as medicine for pulmonary and respiratory diseases in traditional Chinese medicine, and its immunomodulatory and anti-cancer effects were widely reported (244, 268–272). Unlike other natural products targeting PD-L1 expression on tumor cells, PG can reduce PD-1 expression on the CD8+ T cell surface. Yang et al. discovered that PG could reduce STAT3 phosphorylation and downregulate VEGF-A secretion in tumor cells. VEGF-A-VEGFR axis can upregulate PD-1 expression on CD8+ T cells; thus, PG indirectly inhibits CD8+ T cells PD-1 by inhibiting tumor VEGF-A secretion (265).
Artesunate is extracted from Artemisia carvifolia. Xing et al. discovered that Artesunate can upregulate PD-1 expression on peripheral CD8+ or CD4+ T cells. Moreover, they discovered that age over 60, lymphocytes>1.26×109/L, neutrophil to lymphocyte ratio (NLR)<5, chemotherapy, and monocytes between 0.29-0.95×109/L are positive factors that promote Artesunate induce CD8+ cell PD-1 expression. Lymphocytes ≤ 1.26×109/L, NLR<5, chemotherapy, monocytes between 0.29-0.95×109/L, and TNM stage≤III are positive factors that promote Artesunate inducing CD4+ cell PD-1 expression. Artesunate-induced PD-1 expression in CD8+ or CD4+ T cells may improve the prognosis of patients undergoing immune checkpoint inhibitor therapy (266). As mentioned, berberine also downregulates spleen T lymphocyte surface PD-1 expression and reverses the doxorubicin-induced immunosuppressive microenvironment (197).
4.2 Natural products targeting other immune checkpoints
Natural products that target other immune checkpoints are listed in Table 5. Astragaloside IV, erianin, dihydroartemisinin, calotropin, and Berbamine Hydrochloride can target other immune checkpoints besides PD-1 and PD-L1. Tumor-expressed IDO can limit the anti-tumor function of cytotoxic T cells (278). Astragalus membranaceus is a widely used herb in traditional Chinese medicine with strong immune modulatory properties (279, 280). Astragaloside IV extracted from Astragalus membranaceus can inhibit IDO expression in IDO-overexpressed lung cancer cells, upregulate co-cultured CD8+CD28+ cells, and downregulate Treg cells (273). Erianin extracted from the Dendrobium Chrysostom also can downregulate IDO mRNA and protein levels (274, 281). Dihydroartemisinin (DHA), derived from the antimalarial drug Artemisia annua, can downregulate B7-H3 expression (275, 282). Calotropin isolated from Asclepias curasavica L can repress CTLA-4 expression (276, 283). Berbamine Hydrochloride is derived from Coptis Chinensis and has an anti-tumor function (284–286). Berbamine Hydrochloride can activate Nox2 to resist lysosomal acidification and, therefore, inhibit lung cancer cell autophagy, which may potentiate the effect of chemotherapy (277).
Some other natural products that were described before targeting the PD-1/PD-L1 axis also affect other immune checkpoints: Nemania sp. EL006872 extract can downregulate GITRL and ICOSL protein expression (195); Licochalcone A can downregulate IDO1 protein expression (196).
4.3 Natural products combine with other therapies targeting immune checkpoints or combine with immunotherapy
Natural products can combine with other therapies like radiotherapy and chemotherapy and modulate immune checkpoint expression. Natural products can combine with immune checkpoint inhibitors to enhance the immunotherapy effect (Table 6).
Aconitum carmichaelii Debx, also called Fuzi in traditional Chinese medicine; previous studies proved that Fuzi inhibits tumor growth by improving immune responses (293, 294). Radiotherapy increases lung cancer tissue PD-L1 mRNA and protein levels, while Fuzi decreases it. Furthermore, when Fuzi combines with radiotherapy, it can increase serum immune-promoting cytokine (IL-2, IL-5, IL-6, IL-12) levels but decrease immune-suppressing cytokine (IL-10, TGF-β) levels (287).
B7-H3 is an immune checkpoint overexpressed on tumor cells and is positively related to poor prognosis and tumor progression (295, 296). Astragaloside IV sensitizes lung cancer cells to cisplatin by decreasing B7-H3 mRNA and protein levels (288).
Ginsenoside Rh2 and Rg3, both extracted from ginseng, can downregulate lung cancer cells PD-L1 expression induced by cisplatin: Ginsenoside Rh2 through PI3K/Akt pathway while Rg3 through PI3K/Akt and NF-κB pathway (191, 289). Moreover, Rh2 and Rg3 can attenuate cisplatin resistance and promote apoptosis in lung cancer cells.
Astragalus Polysaccharide Injection (PG2) is a prescription drug that alleviates cancer-related fatigue (297). Shih et al. treated lung cancer patients with immune checkpoint inhibitors (ICIs) and PG2 or ICIs alone and found out that PG2 can decrease the patient neutrophil-to-lymphocyte ratio (NLR). A low NLR is correlated with a better prognosis (291). Salvia miltiorrhiza Bunge (Danshen) extract cryptotanshinone, combined with anti-PD-L1 antibody, can make LLC-bearing mice tumor-free and increase CD45+ leukocytes, CD4+, and CD8+ T cells infiltration in tumors (292, 298). Some natural products, such as Platycodon grandiflorum and Sophocarpine, have been reported to enhance the efficacy of anti-PD-L1 antibodies, suppressing tumor growth (248, 265). Evodiamine has shown the ability to enhance the effectiveness of anti-PD-1 monoclonal antibodies (mAb), suppressing tumor growth and improving survival in LLC mice (194). Luteolin and apigenin have been observed to augment the impact of monoclonal antibodies, leading to tumor growth suppression, enhanced survival in LLC mice, and a notable increase in CD8+ T cells within the blood, spleen, and tumor (183). Nobiletin has demonstrated its ability to enhance PD-1 mAb treatment, promoting cytokine secretion in peripheral blood mononuclear cells (PBMC) and inducing greater tumor cell death (185). Lycopene has been shown to enhance the impact of PD-1 mAb therapy, resulting in increased tumor cell death, elevated production of anti-tumor cytokines (IL-2, IFNγ), and a higher CD4+/CD8+ ratio in the spleen (178). Gallic acid can potentially enhance the impact of PD-1 mAb treatment, leading to increased tumor cell death in a PBMC co-cultured system and elevated PBMC IFN-γ secretion (175). Andrographolide has been found to potentiate the anti-tumor effect of PD-1 mAb (190).
5 Traditional medicine targeting immune checkpoints or combined with immunotherapy
Traditional medicine can also influence immune checkpoints or combine with immunotherapy (Table 7, Supplementary Table 1). Bu Fei decoction is widely used in improving lung function, especially in the Qi deficient condition. Bu Fei decoction can reduce mRNA and protein levels of PD-L1 in tumor cells and reduce PD-L1 protein expression in xenograft mouse lung cancer cells (299). HYR-2 (Huoxue Yiqi recipe-2) is formed from the traditional Chinese medicine Ze Qi decoction. HYR-2 downregulates PD-L1 mRNA and protein expression in H1975 cells and LLC-xenograft mice, and further exploration shows HYR-2 inhibits PD-L1 through PI3K/Akt signaling (300). Qingfei Jiedu decoction is used as a decoction treating lung cancer (310). Pan et al. used Qingfei Jiedu decoction-containing serum to treat A549 cells and observed that it could reduce cell PD-L1 expression by modulating EGFR, HIF-1, JUN, and NF-κB expression. Moreover, Qingfei Jiedu decoction increases CD8+PD-1+ T cells, which are regarded as activated T cells, in LLC-bearing mice spleen (301). Qiyusanlong decoction can inhibit PD-L1 mRNA and protein expression in tumor tissue and PD-1 mRNA and protein expression in the spleen in LLC-bearing mice (302). The Feiji Recipe can reduce spleen Treg cells in mice-bearing LLC transfected with IDO and prolong mouse life span, and further research found that Feiji Recipe can downregulate IDO in LLC mice (303, 304). Yu-Ping-Feng (YPF), an ancient Chinese herbal formula, can inhibit IDO expression, increase NK cell tumor infiltration, and enhance NK cell cytotoxicity (305).
Lung Cancer Fang No. 1 was reported to improve immune function in SCLC patients. Lung Cancer Fang No. 1 combined with PD-1/PD-L1 inhibitor chemotherapy compared with using PD-1/PD-L1 inhibitor chemotherapy can decrease CYFRA21-1, CA125, and VGEF levels, increase CD4+, CD3+, and CD4+/CD8+ levels, and improve clinical symptoms (including cough, expectoration of sputum, hemoptysis, chest tightness, fatigue, chest pain, and fever) in SCLC patients (306). A clinical study on Jianpichuji Fang in combination with ICIs for the treatment of advanced NSCLC was conducted (307). The control group received sintilimab injection in combination with platinum-based combination chemotherapy, while the experimental group received Jianpichuji Fang in addition to the treatment used in the control group. There was no significant difference in disease control rates between the two groups after two treatment cycles. However, patients in the experimental group showed a greater improvement in KPS scores compared to the control group. Moreover, the incidence of digestive system nausea symptoms and leukopenia was lower in the experimental group than in the control group. In another advanced NSCLC clinical study, Yanghe Decoction was used in combination with ICIs (308). The control group received chemotherapy combined with immune checkpoint inhibitors (including pembrolizumab, camrelizumab, tislelizumab, and sintilimab), and the treatment group received Yanghe Decoction in addition to the treatment used in the control group for a total of four treatment cycles. The treatment group demonstrated better improvement in clinical symptoms (cough, sputum, hemoptysis, fatigue), stable KPS scores, and reduced levels of tumor markers CEA and CA19-9. In a study focused on NSCLC patients experiencing diarrhea after ICI treatment, the control group received oral prednisolone (1 mg/kg), while the treatment group received Warming Spleen and Kidney Fang for a total of 4 weeks (309). The results showed no significant difference in efficacy between the control and treatment groups. Nevertheless, the KPS level increased more in the treatment group, and there were fewer occurrences of common side effects associated with hormone treatment, such as nausea, vomiting, dizziness, headache, dry mouth, throat dryness, and infection.
6 Conclusion and outlook
This study reviewed immune checkpoints regulation mechanisms and summarized natural products and traditional Chinese medicine that influence immune checkpoints or immunotherapy in lung cancer. Immune checkpoint regulation in lung cancer includes epigenetic, transcriptional, post-transcriptional, translational, and post-translational regulation. Most research focuses on PD-L1 regulation. Furthermore, transcriptional regulation was mainly studied; oncogenes or cytokines through multiple signal pathways finally influence CD274 promoter or enhancer. Exploring the PD-L1 regulator provides a basis for investigating how natural products influence PD-L1. Therefore, most current research focuses on natural products that influence the PD-1/PD-L1 axis (Figure 2). Some natural products influence other immune checkpoints like IDO, B7-H3, CTLA-4, GITRL, ICOSL, and Nox2, but the underlying mechanisms need further elucidation. Natural products’ immune checkpoint regulation properties provide the potential to become new clinical immune checkpoint inhibitors or as a prodrug in research (199). Natural products and Traditional medicine can influence the expression of immune checkpoints through different signaling pathways, some of which can influence multiple pathways at the same time, such as silibinin, ginsenoside Rg3, fraxinellone, Z-guggulsterone, and Qingfei Jiedu decoction (176, 179, 191, 252, 301). Moreover, myricetin, Nemania sp. EL006872, and Licochalcone A can influence multiple immune checkpoints (184, 195, 196). When combined with immunotherapy, natural products and Traditional medicine can enhance the growth inhibition ability of immunotherapy and promote anti-tumor lymphocyte infiltration (178, 194, 253, 292, 306). Traditional medicine can also alleviate immune checkpoint-related adverse reaction and enhance patients’ physical well-being (306–309). Furthermore, some natural products can inhibit immunosuppressive factor secretion: IL-10, TGF-β; promote anti-tumor factor secretion: IL-2, IL-5, IL-6, IL-12; reduce immunosuppressive immune cells: Treg, M2 microphage; increase anti-tumor CD8+ T-cells (253, 262, 263, 265, 273, 287, 292, 303, 304). As a result, the combination of immune checkpoint inhibitors with low-toxicity natural products is a good solution to treat lung cancer.
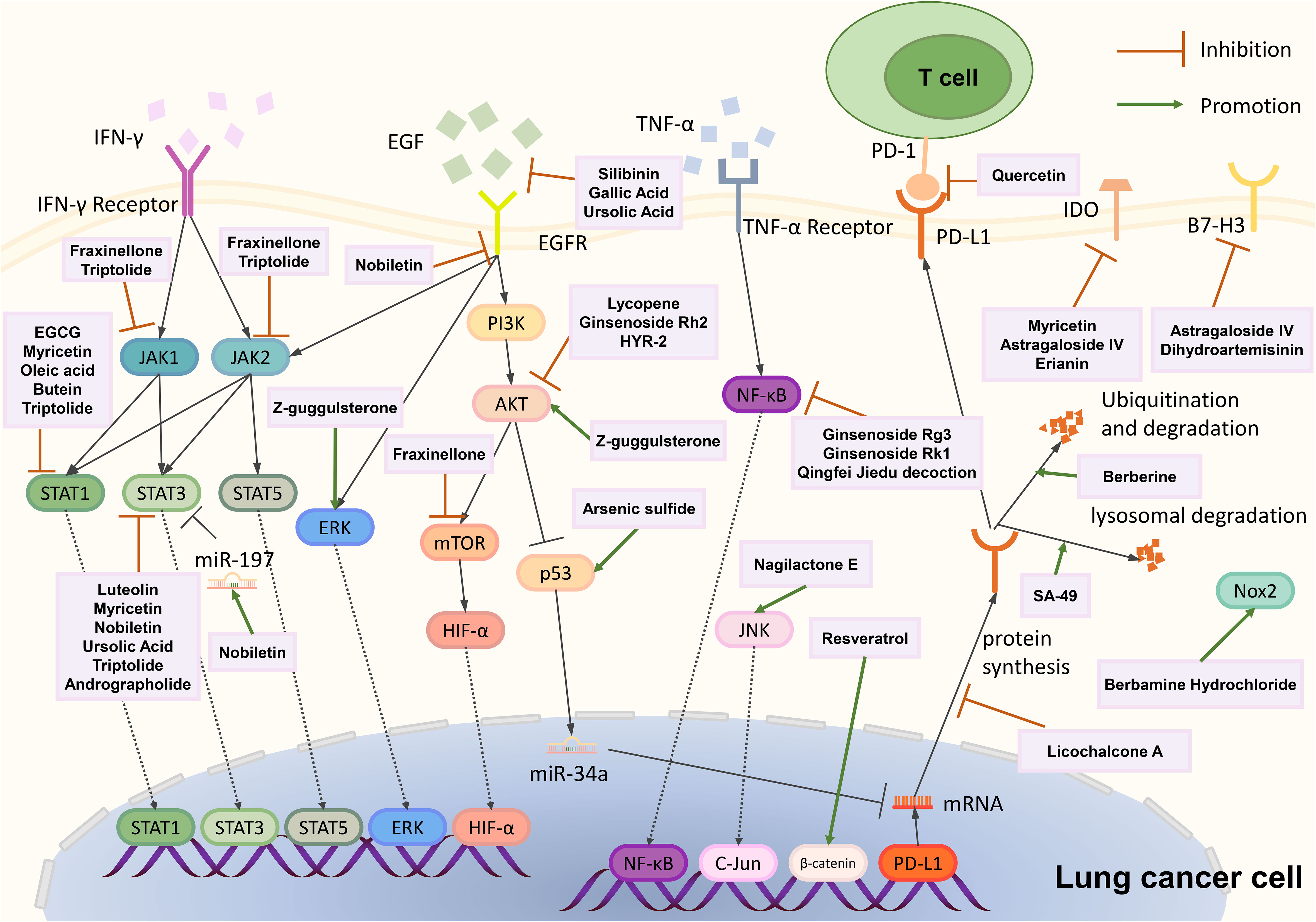
Figure 2 Schematic represents natural products that influence immune checkpoints in the lung cancer cell. Most natural products influence PD-L1 expression (upregulate or downregulate) through different pathways, and some natural products influence other immune checkpoints like IDO or B7-H3. Arrows indicate activations; blunt-ended lines indicate inhibitory effects; dotted arrows indicate translocations.
In the future, further exploring PD-L1 and other immune checkpoint regulation mechanisms and explaining the mechanisms of natural products influencing signaling pathways are needed: Silibinin alone can inhibit PD-L1 expression through both PI3K/AKT and JAK2/STAT5 signaling, while when it combines with crizotinib treating crizotinib-resistant cells, it inhibits PD-L1 expression through JAK-STAT3 signaling; Platycodon grandiflorum can reduce tumor cells downregulate VEGF-A secretion by inhibiting STAT3 phosphorylation, although it can inhibit STAT3 phosphorylation, the researcher did not report whether Platycodon grandiflorum can reduce tumor cell PD-L1 expression through this pathway (176, 265). Determining the synergistic effect among natural products influences different pathways is helpful for drug combinations.
Moreover, the low water solubility of some natural products will hinder their absorption and reduce their bioavailability in the body (311). Tumor heterogeneity is ubiquitous among the different pathological types of lung cancer, and the same natural products may have different effects on different types of lung cancer. Because of the above questions, no natural products are now used as single immune checkpoint inhibitors, and few clinical trials combine natural products with immune checkpoint inhibitors. Based on these unanswered questions, there is still a gap in the clinical use of natural products. The good news is that chemical or enzymatic modification of natural products may improve solubility and combine with a delivery system such as nanoparticles to improve drug bioavailability (312). Currently, there are numerous ongoing trials investigating natural products as lead compounds in anticancer formulations (313). Moreover, since gut microbiota can regulate the metabolism of oral natural products, understanding how gut microbiota affect each natural product also remains a critical question for the clinical use of natural products (314, 315). The extraction of natural products must adhere to principles of sustainability and cost-effectiveness. Some natural products, like ginsenoside Rk1, Rh2, and Rg3 derived from ginseng, have relatively high raw material costs and low extraction yields, limiting their widespread application. Therefore, improving the extraction yield of natural products or exploring chemical synthesis and semi-synthesis will be crucial directions for future research in this field (316).
Meanwhile, traditional medicine, a promising way for the clinical application of natural products, always combines many herbs to treat lung cancer based on clinical experience. Traditional medicine may have synergistic effects that can improve therapeutic efficiency, but the mechanism needs comprehensive exploration. Network pharmacology, through screening potential oncogenic pathways and targets between drug and tumor, is widely used to theoretically explore traditional medicine’s synergistic effects on lung cancer (317–319). Furthermore, further exploration of traditional medicine may need more understanding of signaling pathways and more in vivo and clinical experiments. Natural products widely exist in nature, a treasure house for searching for new tumor therapeutic drugs. They deserve our attention and need further study to enhance their therapeutic effect on lung cancer.
Author contributions
YZ: Writing – original draft. FW: Investigation, Writing – original draft. GL: Writing – review & editing. JX: Investigation, Writing – original draft. JZ: Investigation, Writing – original draft. EG: Writing – review & editing. JY: Writing – review & editing. JW: Conceptualization, Writing – original draft.
Funding
The author(s) declare financial support was received for the research, authorship, and/or publication of this article. This work was supported by The National Natural Science Foundation of China (Grant numbers 82172760).
Conflict of interest
The authors declare that the research was conducted in the absence of any commercial or financial relationships that could be construed as a potential conflict of interest.
Publisher’s note
All claims expressed in this article are solely those of the authors and do not necessarily represent those of their affiliated organizations, or those of the publisher, the editors and the reviewers. Any product that may be evaluated in this article, or claim that may be made by its manufacturer, is not guaranteed or endorsed by the publisher.
Supplementary material
The Supplementary Material for this article can be found online at: https://www.frontiersin.org/articles/10.3389/fimmu.2024.1340307/full#supplementary-material
Glossary
References
1. WHO. Trachea, bronchus and lung (C33-34) (2020). Available at: https://gco.iarc.fr/today/data/factsheets/cancers/15-Lung-fact-sheet.pdf.
2. Siegel RL, Miller KD, Fuchs HE, Jemal A. Cancer statistics, 2022. CA Cancer J Clin (2022) 72(1):7–33. doi: 10.3322/caac.21708
3. Feng RM, Zong YN, Cao SM, Xu RH. Current cancer situation in China: good or bad news from the 2018 Global Cancer Statistics? Cancer Commun (Lond) (2019) 39(1):22. doi: 10.1186/s40880-019-0368-6
4. Zhang P, Ma Y, Lv C, Huang M, Li M, Dong B, et al. Upregulation of programmed cell death ligand 1 promotes resistance response in non-small-cell lung cancer patients treated with neo-adjuvant chemotherapy. Cancer Sci (2016) 107(11):1563–71. doi: 10.1111/cas.13072
5. Turley SJ, Cremasco V, Astarita JL. Immunological hallmarks of stromal cells in the tumour microenvironment. Nat Rev Immunol (2015) 15(11):669–82. doi: 10.1038/nri3902
6. Guo L, Wei R, Lin Y, Kwok HF. Clinical and recent patents applications of PD-1/PD-L1 targeting immunotherapy in cancer treatment-current progress, strategy, and future perspective. Front Immunol (2020) 11:1508. doi: 10.3389/fimmu.2020.01508
7. Jiang X, Wang J, Deng X, Xiong F, Ge J, Xiang B, et al. Role of the tumor microenvironment in PD-L1/PD-1-mediated tumor immune escape. Mol Cancer (2019) 18(1):10. doi: 10.1186/s12943-018-0928-4
8. Hornyak L, Dobos N, Koncz G, Karanyi Z, Pall D, Szabo Z, et al. The role of indoleamine-2,3-dioxygenase in cancer development, diagnostics, and therapy. Front Immunol (2018) 9:151. doi: 10.3389/fimmu.2018.00151
9. Volaric A, Gentzler R, Hall R, Mehaffey JH, Stelow EB, Bullock TN, et al. Indoleamine-2,3-dioxygenase in non-small cell lung cancer: A targetable mechanism of immune resistance frequently coexpressed with PD-L1. Am J Surg Pathol (2018) 42(9):1216–23. doi: 10.1097/PAS.0000000000001099
10. Castellanos JR, Purvis IJ, Labak CM, Guda MR, Tsung AJ, Velpula KK, et al. B7-H3 role in the immune landscape of cancer. Am J Clin Exp Immunol (2017) 6(4):66–75.
11. Goldman JW, Dvorkin M, Chen Y, Reinmuth N, Hotta K, Trukhin D, et al. Durvalumab, with or without tremelimumab, plus platinum-etoposide versus platinum-etoposide alone in first-line treatment of extensive-stage small-cell lung cancer (CASPIAN): updated results from a randomised, controlled, open-label, phase 3 trial. Lancet Oncol (2021) 22(1):51–65. doi: 10.1016/S1470-2045(20)30539-8
12. Felip E, Altorki N, Zhou C, Csoszi T, Vynnychenko I, Goloborodko O, et al. Adjuvant atezolizumab after adjuvant chemotherapy in resected stage IB-IIIA non-small-cell lung cancer (IMpower010): a randomised, multicentre, open-label, phase 3 trial. Lancet (2021) 398(10308):1344–57. doi: 10.1016/S0140-6736(21)02098-5
13. Park S, Choi YD, Kim J, Kho BG, Park CK, Oh IJ, et al. Efficacy of immune checkpoint inhibitors according to PD-L1 tumor proportion scores in non-small cell lung cancer. Thorac Cancer (2020) 11(2):408–14. doi: 10.1111/1759-7714.13284
14. Lin X, Lu X, Luo G, Xiang H. Progress in PD-1/PD-L1 pathway inhibitors: From biomacromolecules to small molecules. Eur J Med Chem (2020) 186:111876. doi: 10.1016/j.ejmech.2019.111876
15. Shaabani S, Huizinga HPS, Butera R, Kouchi A, Guzik K, Magiera-Mularz K, et al. A patent review on PD-1/PD-L1 antagonists: small molecules, peptides, and macrocycles (2015-2018). Expert Opin Ther Pat (2018) 28(9):665–78. doi: 10.1080/13543776.2018.1512706
16. Zhu H, Bengsch F, Svoronos N, Rutkowski MR, Bitler BG, Allegrezza MJ, et al. BET bromodomain inhibition promotes anti-tumor immunity by suppressing PD-L1 expression. Cell Rep (2016) 16(11):2829–37. doi: 10.1016/j.celrep.2016.08.032
17. NCCN. Non-small cell lung cancer (Version: 6.2022). Plymouth Meeting, PA: National Comprehensive Cancer Network (2022).
18. Forde PM, Spicer J, Lu S, Provencio M, Mitsudomi T, Awad MM, et al. Neoadjuvant nivolumab plus chemotherapy in resectable lung cancer. N Engl J Med (2022) 386(21):1973–85. doi: 10.1056/NEJMoa2202170
19. NCCN. Small cell lung cancer (Version: 2.2023). Plymouth Meeting, PA: National Comprehensive Cancer Network (2022).
20. Paz-Ares L, Dvorkin M, Chen Y, Reinmuth N, Hotta K, Trukhin D, et al. Durvalumab plus platinum-etoposide versus platinum-etoposide in first-line treatment of extensive-stage small-cell lung cancer (CASPIAN): a randomised, controlled, open-label, phase 3 trial. Lancet (2019) 394(10212):1929–39. doi: 10.1016/S0140-6736(19)32222-6
21. Horn L, Mansfield AS, Szczesna A, Havel L, Krzakowski M, Hochmair MJ, et al. First-line atezolizumab plus chemotherapy in extensive-stage small-cell lung cancer. N Engl J Med (2018) 379(23):2220–9. doi: 10.1056/NEJMoa1809064
22. NCCN. Small cell lung cancer (Version: 2.2024). Plymouth Meeting, PA: National Comprehensive Cancer Network (2024).
23. Liu SV, Reck M, Mansfield AS, Mok T, Scherpereel A, Reinmuth N, et al. Updated overall survival and PD-L1 subgroup analysis of patients with extensive-stage small-cell lung cancer treated with atezolizumab, carboplatin, and etoposide (IMpower133). J Clin Oncol (2021) 39(6):619–30. doi: 10.1200/JCO.20.01055
24. Paz-Ares L, Chen Y, Reinmuth N, Hotta K, Trukhin D, Statsenko G, et al. Durvalumab, with or without tremelimumab, plus platinum-etoposide in first-line treatment of extensive-stage small-cell lung cancer: 3-year overall survival update from CASPIAN. ESMO Open (2022) 7(2):100408. doi: 10.1016/j.esmoop.2022.100408
25. Mathieu L, Shah S, Pai-Scherf L, Larkins E, Vallejo J, Li X, et al. FDA approval summary: atezolizumab and durvalumab in combination with platinum-based chemotherapy in extensive stage small cell lung cancer. Oncologist (2021) 26(5):433–8. doi: 10.1002/onco.13752
26. Dudnik E, Moskovitz M, Daher S, Shamai S, Hanovich E, Grubstein A, et al. Effectiveness and safety of nivolumab in advanced non-small cell lung cancer: The real-life data. Lung Cancer (2018) 126:217–23. doi: 10.1016/j.lungcan.2017.11.015
27. Schouten RD, Muller M, de Gooijer CJ, Baas P, van den Heuvel M. Real life experience with nivolumab for the treatment of non-small cell lung carcinoma: Data from the expanded access program and routine clinical care in a tertiary cancer centre-The Netherlands Cancer Institute. Lung Cancer (2018) 126:210–6. doi: 10.1016/j.lungcan.2017.11.012
28. Catania C, Muthusamy B, Spitaleri G, Del Signore E, Pennell NA. The new era of immune checkpoint inhibition and target therapy in early-stage non-small cell lung cancer. A Rev literature Clin Lung Cancer (2022) 23(2):108–15. doi: 10.1016/j.cllc.2021.11.003
29. Puzanov I, Diab A, Abdallah K, Bingham CO 3rd, Brogdon C, Dadu R, et al. Managing toxicities associated with immune checkpoint inhibitors: consensus recommendations from the Society for Immunotherapy of Cancer (SITC) Toxicity Management Working Group. J Immunother Cancer (2017) 5(1):95. doi: 10.1186/s40425-017-0300-z
30. Kumar V, Chaudhary N, Garg M, Floudas CS, Soni P, Chandra AB. Current diagnosis and management of immune related adverse events (irAEs) induced by immune checkpoint inhibitor therapy. Front Pharmacol (2017) 8:49. doi: 10.3389/fphar.2017.00049
31. Kumar S, Sharawat SK. Epigenetic regulators of programmed death-ligand 1 expression in human cancers. Transl Res (2018) 202:129–45. doi: 10.1016/j.trsl.2018.05.011
32. George J, Saito M, Tsuta K, Iwakawa R, Shiraishi K, Scheel AH, et al. Genomic amplification of CD274 (PD-L1) in small-cell lung cancer. Clin Cancer Res (2017) 23(5):1220–6. doi: 10.1158/1078-0432.CCR-16-1069
33. Clave S, Pijuan L, Casadevall D, Taus A, Gimeno J, Hernandez-Llodra S, et al. CD274 (PDL1) and JAK2 genomic amplifications in pulmonary squamous-cell and adenocarcinoma patients. Histopathology (2018) 72(2):259–69. doi: 10.1111/his.13339
34. Ikeda S, Okamoto T, Okano S, Umemoto Y, Tagawa T, Morodomi Y, et al. PD-L1 is upregulated by simultaneous amplification of the PD-L1 and JAK2 genes in non-small cell lung cancer. J Thorac Oncol (2016) 11(1):62–71. doi: 10.1016/j.jtho.2015.09.010
35. Feinberg AP. The key role of epigenetics in human disease prevention and mitigation. N Engl J Med (2018) 378(14):1323–34. doi: 10.1056/NEJMra1402513
36. Angeloni A, Bogdanovic O. Enhancer DNA methylation: implications for gene regulation. Essays Biochem (2019) 63(6):707–15. doi: 10.1042/EBC20190030
37. Asgarova A, Asgarov K, Godet Y, Peixoto P, Nadaradjane A, Boyer-Guittaut M, et al. PD-L1 expression is regulated by both DNA methylation and NF-kB during EMT signaling in non-small cell lung carcinoma. Oncoimmunology (2018) 7(5):e1423170. doi: 10.1080/2162402X.2017.1423170
38. Larsen TV, Dybdal N, Daugaard TF, Lade-Keller J, Lin L, Sorensen BS, et al. Examination of the functional relationship between PD-L1 DNA methylation and mRNA expression in non-small-cell lung cancer. Cancers (Basel) (2023) 15(6). doi: 10.3390/cancers15061909
39. Zhao Y, Wang XX, Wu W, Long H, Huang J, Wang Z, et al. EZH2 regulates PD-L1 expression via HIF-1α in non-small cell lung cancer cells. Biochem Biophys Res Commun (2019) 517(2):201–9. doi: 10.1016/j.bbrc.2019.07.039
40. Wang H, Fu C, Du J, Wang H, He R, Yin X, et al. Enhanced histone H3 acetylation of the PD-L1 promoter via the COP1/c-Jun/HDAC3 axis is required for PD-L1 expression in drug-resistant cancer cells. J Exp Clin Cancer Res (2020) 39(1):29. doi: 10.1186/s13046-020-1536-x
41. Booth L, Roberts JL, Poklepovic A, Avogadri-Connors F, Cutler RE, Lalani AS, et al. HDAC inhibitors enhance neratinib activity and when combined enhance the actions of an anti-PD-1 immunomodulatory antibody in vivo. Oncotarget (2017) 8(52):90262–77. doi: 10.18632/oncotarget.21660
42. Liu X, Wang Y, Zhang R, Jin T, Qu L, Jin Q, et al. HDAC10 is positively associated with PD-L1 expression and poor prognosis in patients with NSCLC. Front Oncol (2020) 10:485. doi: 10.3389/fonc.2020.00485
43. Chen N, Fang W, Lin Z, Peng P, Wang J, Zhan J, et al. KRAS mutation-induced upregulation of PD-L1 mediates immune escape in human lung adenocarcinoma. Cancer Immunol Immunother (2017) 66(9):1175–87. doi: 10.1007/s00262-017-2005-z
44. Sumimoto H, Takano A, Teramoto K, Daigo Y. RAS-mitogen-activated protein kinase signal is required for enhanced PD-L1 expression in human lung cancers. PloS One (2016) 11(11):e0166626. doi: 10.1371/journal.pone.0166626
45. Lee MH, Yanagawa J, Tran L, Walser TC, Bisht B, Fung E, et al. FRA1 contributes to MEK-ERK pathway-dependent PD-L1 upregulation by KRAS mutation in premalignant human bronchial epithelial cells. Am J Transl Res (2020) 12(2):409–27.
46. Pan Y, Zhang Y, Li Y, Hu H, Wang L, Li H, et al. ALK, ROS1 and RET fusions in 1139 lung adenocarcinomas: a comprehensive study of common and fusion pattern-specific clinicopathologic, histologic and cytologic features. Lung Cancer (2014) 84(2):121–6. doi: 10.1016/j.lungcan.2014.02.007
47. Hong S, Fang W, Hu Z, Zhou T, Yan Y, Qin T, et al. A large-scale cross-sectional study of ALK rearrangements and EGFR mutations in non-small-cell lung cancer in Chinese Han population. Sci Rep (2014) 4:7268. doi: 10.1038/srep07268
48. Ma L, Lv J, Dong Y, Zhang X, Li X, Zhang H, et al. PD-L1 expression and its regulation in lung adenocarcinoma with ALK translocation. Interdiscip Sci (2019) 11(2):266–72. doi: 10.1007/s12539-019-00331-0
49. Hong S, Chen N, Fang W, Zhan J, Liu Q, Kang S, et al. Upregulation of PD-L1 by EML4-ALK fusion protein mediates the immune escape in ALK positive NSCLC: Implication for optional anti-PD-1/PD-L1 immune therapy for ALK-TKIs sensitive and resistant NSCLC patients. Oncoimmunology (2016) 5(3):e1094598. doi: 10.1080/2162402X.2015.1094598
50. Koh J, Jang JY, Keam B, Kim S, Kim MY, Go H, et al. EML4-ALK enhances programmed cell death-ligand 1 expression in pulmonary adenocarcinoma via hypoxia-inducible factor (HIF)-1alpha and STAT3. Oncoimmunology (2016) 5(3):e1108514. doi: 10.1080/2162402X.2015.1108514
51. Akbay EA, Koyama S, Carretero J, Altabef A, Tchaicha JH, Christensen CL, et al. Activation of the PD-1 pathway contributes to immune escape in EGFR-driven lung tumors. Cancer Discovery (2013) 3(12):1355–63. doi: 10.1158/2159-8290.CD-13-0310
52. Abdelhamed S, Ogura K, Yokoyama S, Saiki I, Hayakawa Y. AKT-STAT3 pathway as a downstream target of EGFR signaling to regulate PD-L1 expression on NSCLC cells. J Cancer (2016) 7(12):1579–86. doi: 10.7150/jca.14713
53. Chen N, Fang W, Zhan J, Hong S, Tang Y, Kang S, et al. Upregulation of PD-L1 by EGFR activation mediates the immune escape in EGFR-driven NSCLC: implication for optional immune targeted therapy for NSCLC patients with EGFR mutation. J Thorac Oncol (2015) 10(6):910–23. doi: 10.1097/JTO.0000000000000500
54. Okita R, Maeda A, Shimizu K, Nojima Y, Saisho S, Nakata M. PD-L1 overexpression is partially regulated by EGFR/HER2 signaling and associated with poor prognosis in patients with non-small-cell lung cancer. Cancer Immunol Immunother (2017) 66(7):865–76. doi: 10.1007/s00262-017-1986-y
55. Zhang N, Zeng Y, Du W, Zhu J, Shen D, Liu Z, et al. The EGFR pathway is involved in the regulation of PD-L1 expression via the IL-6/JAK/STAT3 signaling pathway in EGFR-mutated non-small cell lung cancer. Int J Oncol (2016) 49(4):1360–8. doi: 10.3892/ijo.2016.3632
56. Guo R, Li Y, Wang Z, Bai H, Duan J, Wang S, et al. Hypoxia-inducible factor-1alpha and nuclear factor-kappaB play important roles in regulating programmed cell death ligand 1 expression by epidermal growth factor receptor mutants in non-small-cell lung cancer cells. Cancer Sci (2019) 110(5):1665–75. doi: 10.1111/cas.13989
57. Tieche CC, Gao Y, Buhrer ED, Hobi N, Berezowska SA, Wyler K, et al. Tumor initiation capacity and therapy resistance are differential features of EMT-related subpopulations in the NSCLC cell line A549. Neoplasia (2019) 21(2):185–96. doi: 10.1016/j.neo.2018.09.008
58. Tsukita Y, Fujino N, Miyauchi E, Saito R, Fujishima F, Itakura K, et al. Axl kinase drives immune checkpoint and chemokine signalling pathways in lung adenocarcinomas. Mol Cancer (2019) 18(1):24. doi: 10.1186/s12943-019-0953-y
59. Suda K, Rozeboom L, Rivard CJ, Yu H, Ellison K, Melnick MAC, et al. Therapy-induced E-cadherin downregulation alters expression of programmed death ligand-1 in lung cancer cells. Lung Cancer (2017) 109:1–8. doi: 10.1016/j.lungcan.2017.04.010
60. Suda K, Rozeboom L, Furugaki K, Yu H, Melnick MAC, Ellison K, et al. Increased EGFR phosphorylation correlates with higher programmed death ligand-1 expression: analysis of TKI-resistant lung cancer cell lines. BioMed Res Int (2017) 2017:7694202. doi: 10.1155/2017/7694202
61. Ji M, Liu Y, Li Q, Li X, Ning Z, Zhao W, et al. PD-1/PD-L1 expression in non-small-cell lung cancer and its correlation with EGFR/KRAS mutations. Cancer Biol Ther (2016) 17(4):407–13. doi: 10.1080/15384047.2016.1156256
62. Azuma K, Ota K, Kawahara A, Hattori S, Iwama E, Harada T, et al. Association of PD-L1 overexpression with activating EGFR mutations in surgically resected nonsmall-cell lung cancer. Ann Oncol (2014) 25(10):1935–40. doi: 10.1093/annonc/mdu242
63. Wang S, Xu L, Che X, Li C, Xu L, Hou K, et al. E3 ubiquitin ligases Cbl-b and c-Cbl downregulate PD-L1 in EGFR wild-type non-small cell lung cancer. FEBS Lett (2018) 592(4):621–30. doi: 10.1002/1873-3468.12985
64. Lin J, Sun T, Ji L, Deng W, Roth J, Minna J, et al. Oncogenic activation of c-Abl in non-small cell lung cancer cells lacking FUS1 expression: inhibition of c-Abl by the tumor suppressor gene product Fus1. Oncogene (2007) 26(49):6989–96. doi: 10.1038/sj.onc.1210500
65. Ji L, Roth JA. Tumor suppressor FUS1 signaling pathway. J Thorac Oncol (2008) 3(4):327–30. doi: 10.1097/JTO.0b013e31816bce65
66. Cao X, Zhao Y, Wang J, Dai B, Gentile E, Lin J, et al. TUSC2 downregulates PD-L1 expression in non-small cell lung cancer (NSCLC). Oncotarget (2017) 8(64):107621–9. doi: 10.18632/oncotarget.22581
67. Dai B, Yan S, Lara-Guerra H, Kawashima H, Sakai R, Jayachandran G, et al. Exogenous restoration of TUSC2 expression induces responsiveness to erlotinib in wildtype epidermal growth factor receptor (EGFR) lung cancer cells through context specific pathways resulting in enhanced therapeutic efficacy. PloS One (2015) 10(6):e0123967. doi: 10.1371/journal.pone.0123967
68. Zhang H, Dutta P, Liu J, Sabri N, Song Y, Li WX, et al. Tumour cell-intrinsic CTLA4 regulates PD-L1 expression in non-small cell lung cancer. J Cell Mol Med (2019) 23(1):535–42. doi: 10.1111/jcmm.13956
69. Stutvoet TS, Kol A, de Vries EG, de Bruyn M, Fehrmann RS, Terwisscha van Scheltinga AG, et al. MAPK pathway activity plays a key role in PD-L1 expression of lung adenocarcinoma cells. . J Pathol (2019) 249(1):52–64. doi: 10.1002/path.5280
70. Lin K, Cheng J, Yang T, Li Y, Zhu B. EGFR-TKI down-regulates PD-L1 in EGFR mutant NSCLC through inhibiting NF-κB. Biochem Biophys Res Commun (2015) 463(1-2):95–101. doi: 10.1016/j.bbrc.2015.05.030
71. To KKW, Fong W, Cho WCS. Immunotherapy in treating EGFR-mutant lung cancer: current challenges and new strategies. Front Oncol (2021) 11:635007. doi: 10.3389/fonc.2021.635007
72. Gainor JF, Shaw AT, Sequist LV, Fu X, Azzoli CG, Piotrowska Z, et al. EGFR mutations and ALK rearrangements are associated with low response rates to PD-1 pathway blockade in non-small cell lung cancer: A retrospective analysis. Clin Cancer Res (2016) 22(18):4585–93. doi: 10.1158/1078-0432.CCR-15-3101
73. Garassino MC, Gelibter AJ, Grossi F, Chiari R, Soto Parra H, Cascinu S, et al. Italian nivolumab expanded access program in nonsquamous non-small cell lung cancer patients: results in never-smokers and EGFR-mutant patients. J Thorac Oncol (2018) 13(8):1146–55. doi: 10.1016/j.jtho.2018.04.025
74. Garassino MC, Cho BC, Kim JH, Mazières J, Vansteenkiste J, Lena H, et al. Durvalumab as third-line or later treatment for advanced non-small-cell lung cancer (ATLANTIC): an open-label, single-arm, phase 2 study. Lancet Oncol (2018) 19(4):521–36. doi: 10.1016/S1470-2045(18)30144-X
75. Gavralidis A, Gainor JF. Immunotherapy in EGFR-mutant and ALK-positive lung cancer: implications for oncogene-driven lung cancer. Cancer J (2020) 26(6):517–24. doi: 10.1097/PPO.0000000000000491
76. Liang H, Liu X, Wang M. Immunotherapy combined with epidermal growth factor receptor-tyrosine kinase inhibitors in non-small-cell lung cancer treatment. Onco Targets Ther (2018) 11:6189–96. doi: 10.2147/OTT.S178497
77. Lorenz J, Friedberg T, Paulus R, Oesch F, Ferlinz R. Oncogene overexpression in non-small-cell lung cancer tissue: prevalence and clinicopathological significance. Clin Investig (1994) 72(2):156–63. doi: 10.1007/BF00184595
78. Wang J, Jia Y, Zhao S, Zhang X, Wang X, Han X, et al. BIN1 reverses PD-L1-mediated immune escape by inactivating the c-MYC and EGFR/MAPK signaling pathways in non-small cell lung cancer. Oncogene (2017) 36(45):6235–43. doi: 10.1038/onc.2017.217
79. Kim EY, Kim A, Kim SK, Chang YS. MYC expression correlates with PD-L1 expression in non-small cell lung cancer. Lung Cancer (2017) 110:63–7. doi: 10.1016/j.lungcan.2017.06.006
80. Casey SC, Tong L, Li Y, Do R, Walz S, Fitzgerald KN, et al. MYC regulates the antitumor immune response through CD47 and PD-L1. Science (2016) 352(6282):227–31. doi: 10.1126/science.aac9935
81. Xu C, Fillmore CM, Koyama S, Wu H, Zhao Y, Chen Z, et al. Loss of Lkb1 and Pten leads to lung squamous cell carcinoma with elevated PD-L1 expression. Cancer Cell (2014) 25(5):590–604. doi: 10.1016/j.ccr.2014.03.033
82. Salmena L, Carracedo A, Pandolfi PP. Tenets of PTEN tumor suppression. Cell (2008) 133(3):403–14. doi: 10.1016/j.cell.2008.04.013
83. Cancer Genome Atlas Research N. Comprehensive genomic characterization of squamous cell lung cancers. Nature (2012) 489(7417):519–25. doi: 10.1038/nature11404
84. Hlaing AM, Furusato B, Udo E, Kitamura Y, Souda M, Masutani M, et al. Expression of phosphatase and tensin homolog and programmed cell death ligand 1 in adenosquamous carcinoma of the lung. Biochem Biophys Res Commun (2018) 503(4):2764–9. doi: 10.1016/j.bbrc.2018.08.037
85. Ota K, Azuma K, Kawahara A, Hattori S, Iwama E, Tanizaki J, et al. Induction of PD-L1 expression by the EML4-ALK oncoprotein and downstream signaling pathways in non-small cell lung cancer. Clin Cancer Res (2015) 21(17):4014–21. doi: 10.1158/1078-0432.CCR-15-0016
86. Della Corte CM, Barra G, Ciaramella V, Di Liello R, Vicidomini G, Zappavigna S, et al. Antitumor activity of dual blockade of PD-L1 and MEK in NSCLC patients derived three-dimensional spheroid cultures. J Exp Clin Cancer Res (2019) 38(1):253. doi: 10.1186/s13046-019-1257-1
87. Demuth C, Andersen MN, Jakobsen KR, Madsen AT, Sørensen BS. Increased PD-L1 expression in erlotinib-resistant NSCLC cells with MET gene amplification is reversed upon MET-TKI treatment. Oncotarget (2017) 8(40):68221–9. doi: 10.18632/oncotarget.19920
88. Albitar M, Sudarsanam S, Ma W, Jiang S, Chen W, Funari V, et al. Correlation of MET gene amplification and TP53 mutation with PD-L1 expression in non-small cell lung cancer. Oncotarget (2018) 9(17):13682–93. doi: 10.18632/oncotarget.24455
89. Ahn HK, Kim S, Kwon D, Koh J, Kim YA, Kim K, et al. MET receptor tyrosine kinase regulates the expression of co-stimulatory and co-inhibitory molecules in tumor cells and contributes to PD-L1-mediated suppression of immune cell function. Int J Mol Sc (2019) 20(17). doi: 10.3390/ijms20174287
90. Peng S, Wang R, Zhang X, Ma Y, Zhong L, Li K, et al. EGFR-TKI resistance promotes immune escape in lung cancer via increased PD-L1 expression. Mol Cancer (2019) 18(1):165. doi: 10.1186/s12943-019-1073-4
91. Moroishi T, Hayashi T, Pan WW, Fujita Y, Holt MV, Qin J, et al. The hippo pathway kinases LATS1/2 suppress cancer immunity. Cell (2016) 167(6):1525–39 e17. doi: 10.1016/j.cell.2016.11.005
92. Janse van Rensburg HJ, Azad T, Ling M, Hao Y, Snetsinger B, Khanal P, et al. The hippo pathway component TAZ promotes immune evasion in human cancer through PD-L1. Cancer Res (2018) 78(6):1457–70. doi: 10.1158/0008-5472.CAN-17-3139
93. Miao J, Hsu PC, Yang YL, Xu Z, Dai Y, Wang Y, et al. YAP regulates PD-L1 expression in human NSCLC cells. Oncotarget (2017) 8(70):114576–87. doi: 10.18632/oncotarget.23051
94. Lee BS, Park DI, Lee DH, Lee JE, Yeo MK, Park YH, et al. Hippo effector YAP directly regulates the expression of PD-L1 transcripts in EGFR-TKI-resistant lung adenocarcinoma. Biochem Biophys Res Commun (2017) 491(2):493–9. doi: 10.1016/j.bbrc.2017.07.007
95. Feng J, Yang H, Zhang Y, Wei H, Zhu Z, Zhu B, et al. Tumor cell-derived lactate induces TAZ-dependent upregulation of PD-L1 through GPR81 in human lung cancer cells. Oncogene (2017) 36(42):5829–39. doi: 10.1038/onc.2017.188
96. Liu H, Kuang X, Zhang Y, Ye Y, Li J, Liang L, et al. ADORA1 inhibition promotes tumor immune evasion by regulating the ATF3-PD-L1 axis. Cancer Cell (2020) 37(3):324–39.e8. doi: 10.1016/j.ccell.2020.02.006
97. Chen S, Crabill GA, Pritchard TS, McMiller TL, Wei P, Pardoll DM, et al. Mechanisms regulating PD-L1 expression on tumor and immune cells. J Immunother Cancer (2019) 7(1):305. doi: 10.1186/s40425-019-0770-2
98. Zerdes I, Matikas A, Bergh J, Rassidakis GZ, Foukakis T. Genetic, transcriptional and post-translational regulation of the programmed death protein ligand 1 in cancer: biology and clinical correlations. Oncogene (2018) 37(34):4639–61. doi: 10.1038/s41388-018-0303-3
99. Garcia-Diaz A, Shin DS, Moreno BH, Saco J, Escuin-Ordinas H, Rodriguez GA, et al. Interferon receptor signaling pathways regulating PD-L1 and PD-L2 expression. Cell Rep (2017) 19(6):1189–201. doi: 10.1016/j.celrep.2017.04.031
100. Lee SJ, Jang BC, Lee SW, Yang YI, Suh SI, Park YM, et al. Interferon regulatory factor-1 is prerequisite to the constitutive expression and IFN-gamma-induced upregulation of B7-H1 (CD274). FEBS Lett (2006) 580(3):755–62. doi: 10.1016/j.febslet.2005.12.093
101. Lv H, Lv G, Chen C, Zong Q, Jiang G, Ye D, et al. NAD(+) metabolism maintains inducible PD-L1 expression to drive tumor immune evasion. Cell Metab (2021) 33(1):110–27 e5. doi: 10.1016/j.cmet.2020.10.021
102. Lai Q, Wang H, Li A, Xu Y, Tang L, Chen Q, et al. Decitibine improve the efficiency of anti-PD-1 therapy via activating the response to IFN/PD-L1 signal of lung cancer cells. Oncogene (2018) 37(17):2302–12. doi: 10.1038/s41388-018-0125-3
103. Wu A, Wu Q, Deng Y, Liu Y, Lu J, Liu L, et al. Loss of VGLL4 suppresses tumor PD-L1 expression and immune evasion. EMBO J (2019) 38(1). doi: 10.15252/embj.201899506
104. Gao Y, Yang J, Cai Y, Fu S, Zhang N, Fu X, et al. IFN-γ-mediated inhibition of lung cancer correlates with PD-L1 expression and is regulated by PI3K-AKT signaling. Int J Cancer (2018) 143(4):931–43. doi: 10.1002/ijc.31357
105. Zhang X, Zeng Y, Qu Q, Zhu J, Liu Z, Ning W, et al. PD-L1 induced by IFN-γ from tumor-associated macrophages via the JAK/STAT3 and PI3K/AKT signaling pathways promoted progression of lung cancer. Int J Clin Oncol (2017) 22(6):1026–33. doi: 10.1007/s10147-017-1161-7
106. Morimoto Y, Kishida T, Kotani SI, Takayama K, Mazda O. Interferon-β signal may up-regulate PD-L1 expression through IRF9-dependent and independent pathways in lung cancer cells. Biochem Biophys Res Commun (2018) 507(1-4):330–6. doi: 10.1016/j.bbrc.2018.11.035
107. Koh YW, Lee SJ, Han JH, Haam S, Jung J, Lee HW. PD-L1 protein expression in non-small-cell lung cancer and its relationship with the hypoxia-related signaling pathways: A study based on immunohistochemistry and RNA sequencing data. Lung Cancer (2019) 129:41–7. doi: 10.1016/j.lungcan.2019.01.004
108. Chang YL, Yang CY, Lin MW, Wu CT, Yang PC. High co-expression of PD-L1 and HIF-1α correlates with tumour necrosis in pulmonary pleomorphic carcinoma. Eur J Cancer (2016) 60:125–35. doi: 10.1016/j.ejca.2016.03.012
109. Noman MZ, Desantis G, Janji B, Hasmim M, Karray S, Dessen P, et al. PD-L1 is a novel direct target of HIF-1alpha, and its blockade under hypoxia enhanced MDSC-mediated T cell activation. J Exp Med (2014) 211(5):781–90. doi: 10.1084/jem.20131916
110. Pickup M, Novitskiy S, Moses HL. The roles of TGFbeta in the tumour microenvironment. Nat Rev Cancer (2013) 13(11):788–99. doi: 10.1038/nrc3603
111. Ni XY, Sui HX, Liu Y, Ke SZ, Wang YN, Gao FG. TGF-beta of lung cancer microenvironment upregulates B7H1 and GITRL expression in dendritic cells and is associated with regulatory T cell generation. Oncol Rep (2012) 28(2):615–21. doi: 10.3892/or.2012.1822
112. Funaki S, Shintani Y, Kawamura T, Kanzaki R, Minami M, Okumura M. Chemotherapy enhances programmed cell death 1/ligand 1 expression via TGF-β induced epithelial mesenchymal transition in non-small cell lung cancer. Oncol Rep (2017) 38(4):2277–84. doi: 10.3892/or.2017.5894
113. Evanno E, Godet J, Piccirilli N, Guilhot J, Milin S, Gombert JM, et al. Tri-methylation of H3K79 is decreased in TGF-beta1-induced epithelial-to-mesenchymal transition in lung cancer. Clin Epigenet (2017) 9:80. doi: 10.1186/s13148-017-0380-0
114. David JM, Dominguez C, McCampbell KK, Gulley JL, Schlom J, Palena C. A novel bifunctional anti-PD-L1/TGF-β Trap fusion protein (M7824) efficiently reverts mesenchymalization of human lung cancer cells. Oncoimmunology (2017) 6(10):e1349589. doi: 10.1080/2162402X.2017.1349589
115. Zhang Y, Zeng Y, Liu T, Du W, Zhu J, Liu Z, et al. The canonical TGF-β/Smad signalling pathway is involved in PD-L1-induced primary resistance to EGFR-TKIs in EGFR-mutant non-small-cell lung cancer. Respir Res (2019) 20(1):164. doi: 10.1186/s12931-019-1137-4
116. Mariathasan S, Turley SJ, Nickles D, Castiglioni A, Yuen K, Wang Y, et al. TGFβ attenuates tumour response to PD-L1 blockade by contributing to exclusion of T cells. Nature (2018) 554(7693):544–8. doi: 10.1038/nature25501
117. Yi M, Li T, Niu M, Wu Y, Zhao Z, Wu K. TGF-β: A novel predictor and target for anti-PD-1/PD-L1 therapy. Front Immunol (2022) 13. doi: 10.3389/fimmu.2022.1061394
118. Zhu S, Zhang T, Zheng L, Liu H, Song W, Liu D, et al. Combination strategies to maximize the benefits of cancer immunotherapy. J Hematol Oncol (2021) 14(1). doi: 10.1186/s13045-021-01164-5
119. Lind H, Gameiro SR, Jochems C, Donahue RN, Strauss J, Gulley JL, et al. Dual targeting of TGF-β and PD-L1 via a bifunctional anti-PD-L1/TGF-βRII agent: status of preclinical and clinical advances. J ImmunoTher Cancer (2020) 8(1):e000433. doi: 10.1136/jitc-2019-000433
120. Chen X, Wang L, Li P, Song M, Qin G, Gao Q, et al. Dual TGF-β and PD-1 blockade synergistically enhances MAGE-A3-specific CD8+ T cell response in esophageal squamous cell carcinoma. Int J Cancer (2018) 143(10):2561–74. doi: 10.1002/ijc.31730
121. Terabe M, Robertson FC, Clark K, De Ravin E, Bloom A, Venzon DJ, et al. Blockade of only TGF-β 1 and 2 is sufficient to enhance the efficacy of vaccine and PD-1 checkpoint blockade immunotherapy. OncoImmunology (2017) 6(5). doi: 10.1080/2162402X.2017.1308616
122. Yi M, Wu Y, Niu M, Zhu S, Zhang J, Yan Y, et al. Anti-TGF-β/PD-L1 bispecific antibody promotes T cell infiltration and exhibits enhanced antitumor activity in triple-negative breast cancer. J ImmunoTher Cancer (2022) 10(12). doi: 10.1136/jitc-2022-005543
123. Yi M, Niu M, Wu Y, Ge H, Jiao D, Zhu S, et al. Combination of oral STING agonist MSA-2 and anti-TGF-β/PD-L1 bispecific antibody YM101: a novel immune cocktail therapy for non-inflamed tumors. J Hematol Oncol (2022) 15(1). doi: 10.1186/s13045-022-01363-8
124. Yi M, Zhang J, Li A, Niu M, Yan Y, Jiao Y, et al. The construction, expression, and enhanced anti-tumor activity of YM101: a bispecific antibody simultaneously targeting TGF-β and PD-L1. J Hematol Oncol (2021) 14(1). doi: 10.1186/s13045-021-01045-x
125. Yi M, Niu M, Zhang J, Li S, Zhu S, Yan Y, et al. Combine and conquer: manganese synergizing anti-TGF-β/PD-L1 bispecific antibody YM101 to overcome immunotherapy resistance in non-inflamed cancers. J Hematol Oncol (2021) 14(1). doi: 10.1186/s13045-021-01155-6
126. Strauss J, Heery CR, Schlom J, Madan RA, Cao L, Kang Z, et al. Phase I trial of M7824 (MSB0011359C), a bifunctional fusion protein targeting PD-L1 and TGFβ, in advanced solid tumors. Clin Cancer Res (2018) 24(6):1287–95. doi: 10.1158/1078-0432.CCR-17-2653
127. Carbotti G, Barisione G, Airoldi I, Mezzanzanica D, Bagnoli M, Ferrero S, et al. IL-27 induces the expression of IDO and PD-L1 in human cancer cells. Oncotarget (2015) 6(41):43267–80. doi: 10.18632/oncotarget.6530
128. Schalper KA, Carvajal-Hausdorf D, McLaughlin J, Altan M, Velcheti V, Gaule P, et al. Differential expression and significance of PD-L1, IDO-1, and B7-H4 in human lung cancer. Clin Cancer Res (2017) 23(2):370–8. doi: 10.1158/1078-0432.CCR-16-0150
129. Gao Y, Lu J, Zeng C, Yang J, Huang B, Zhang N, et al. IL-10 suppresses IFN-gamma-mediated signaling in lung adenocarcinoma. Clin Exp Med (2020) 20(3):449–59. doi: 10.1007/s10238-020-00626-3
130. Bouillez A, Rajabi H, Jin C, Samur M, Tagde A, Alam M, et al. MUC1-C integrates PD-L1 induction with repression of immune effectors in non-small-cell lung cancer. Oncogene (2017) 36(28):4037–46. doi: 10.1038/onc.2017.47
131. Huang J, Weng Q, Shi Y, Mao W, Zhao Z, Wu R, et al. MicroRNA-155-5p suppresses PD-L1 expression in lung adenocarcinoma. FEBS Open Bio (2020) 10(6):1065–71. doi: 10.1002/2211-5463.12853
132. Hong W, Xue M, Jiang J, Zhang Y, Gao X. Circular RNA circ-CPA4/ let-7 miRNA/PD-L1 axis regulates cell growth, stemness, drug resistance and immune evasion in non-small cell lung cancer (NSCLC). J Exp Clin Cancer Res (2020) 39(1):149. doi: 10.1186/s13046-020-01648-1
133. Wei S, Wang K, Huang X, Zhao Z, Zhao Z. LncRNA MALAT1 contributes to non-small cell lung cancer progression via modulating miR-200a-3p/programmed death-ligand 1 axis. Int J Immunopathol Pharmacol (2019) 33. doi: 10.1177/2058738419859699
134. Xie WB, Liang LH, Wu KG, Wang LX, He X, Song C, et al. MiR-140 expression regulates cell proliferation and targets PD-L1 in NSCLC. Cell Physiol Biochem (2018) 46(2):654–63. doi: 10.1159/000488634
135. Cortez MA, Ivan C, Valdecanas D, Wang X, Peltier HJ, Ye Y, et al. PDL1 Regulation by p53 via miR-34. J Natl Cancer Inst (2016) 108(1). doi: 10.1093/jnci/djv303
136. Braun MW, Iwakuma T. Regulation of cytotoxic T-cell responses by p53 in cancer. Trans Cancer Res (2016) 5(6):692–7. doi: 10.21037/tcr.2016.11.76
137. Chen L, Gibbons DL, Goswami S, Cortez MA, Ahn Y-H, Byers LA, et al. Metastasis is regulated via microRNA-200/ZEB1 axis control of tumour cell PD-L1 expression and intratumoral immunosuppression. Nat Commun (2014) 5(1). doi: 10.1038/ncomms6241
138. Xia R, Geng G, Yu X, Xu Z, Guo J, Liu H, et al. LINC01140 promotes the progression and tumor immune escape in lung cancer by sponging multiple microRNAs. J ImmunoTher Cancer (2021) 9(8). doi: 10.1136/jitc-2021-002746
139. Zhu F, Niu R, Shao X, Shao X. FGD5−AS1 promotes cisplatin resistance of human lung adenocarcinoma cell via the miR−142−5p/PD−L1 axis. Int J Mol Med (2020) 47(2):523–32. doi: 10.3892/ijmm.2020.4816
140. Wang J, Zhao X, Wang Y, Ren F, Sun D, Yan Y, et al. circRNA-002178 act as a ceRNA to promote PDL1/PD1 expression in lung adenocarcinoma. Cell Death Dis (2020) 11(1). doi: 10.1038/s41419-020-2230-9
141. Coelho MA, de Carné Trécesson S, Rana S, Zecchin D, Moore C, Molina-Arcas M, et al. Oncogenic RAS signaling promotes tumor immunoresistance by stabilizing PD-L1 mRNA. Immunity (2017) 47(6):1083–99.e6. doi: 10.1016/j.immuni.2017.11.016
142. Yang K, Zhou J, Chen Y, Chen Y, Chen L, Zhang P, et al. Angiotensin II contributes to intratumoral immunosuppressionvia induction of PD-L1 expression in non-small cell lung carcinoma. Int Immunopharmacol (2020) 84:106507. doi: 10.1016/j.intimp.2020.106507
143. Du W, Zhu J, Chen Y, Zeng Y, Shen D, Zhang N, et al. Variant SNPs at the microRNA complementary site in the B7-H1 3′-untranslated region increase the risk of non-small cell lung cancer. Mol Med Rep (2017) 16(3):2682–90. doi: 10.3892/mmr.2017.6902
144. Wang N, Zhang T. Downregulation of microRNA-135 promotes sensitivity of non-small cell lung cancer to gefitinib by targeting TRIM16. Oncol Res Featuring Preclinical Clin Cancer Ther (2018) 26(7):1005–14. doi: 10.3727/096504017X15144755633680
145. Tang D, Zhao D, Wu Y, Yao R, Zhou L, Lu L, et al. The miR-3127-5p/p-STAT3 axis up-regulates PD-L1 inducing chemoresistance in non-small-cell lung cancer. J Cell Mol Med (2018) 22(8):3847–56. doi: 10.1111/jcmm.13657
146. Fujita Y, Yagishita S, Hagiwara K, Yoshioka Y, Kosaka N, Takeshita F, et al. The clinical relevance of the miR-197/CKS1B/STAT3-mediated PD-L1 network in chemoresistant non-small-cell lung cancer. Mol Ther (2015) 23(4):717–27. doi: 10.1038/mt.2015.10
147. Kathuria H, Millien G, McNally L, Gower AC, Tagne JB, Cao Y, et al. NKX2-1-AS1 negatively regulates CD274/PD-L1, cell-cell interaction genes, and limits human lung carcinoma cell migration. Sci Rep (2018) 8(1):14418. doi: 10.1038/s41598-018-32793-5
148. Huang Y, Xia L, Tan X, Zhang J, Zeng W, Tan B, et al. Molecular mechanism of lncRNA SNHG12 in immune escape of non-small cell lung cancer through the HuR/PD-L1/USP8 axis. Cell Mol Biol Lett (2022) 27(1):43. doi: 10.1186/s11658-022-00343-7
149. Suresh S, Chen B, Zhu J, Golden RJ, Lu C, Evers BM, et al. eIF5B drives integrated stress response-dependent translation of PD-L1 in lung cancer. Nat Cancer (2020) 1(5):533–45. doi: 10.1038/s43018-020-0056-0
150. Wu Y, Xie J, Jin X, Lenchine RV, Wang X, Fang DM, et al. eEF2K enhances expression of PD-L1 by promoting the translation of its mRNA. Biochem J (2020) 477(22):4367–81. doi: 10.1042/BCJ20200697
151. Wang Y, Sun Q, Mu N, Sun X, Wang Y, Fan S, et al. The deubiquitinase USP22 regulates PD-L1 degradation in human cancer cells. Cell Commun Signal (2020) 18(1):112. doi: 10.1186/s12964-020-00612-y
152. Lim SO, Li CW, Xia W, Cha JH, Chan LC, Wu Y, et al. Deubiquitination and stabilization of PD-L1 by CSN5. Cancer Cell (2016) 30(6):925–39. doi: 10.1016/j.ccell.2016.10.010
153. Zhu D, Xu R, Huang X, Tang Z, Tian Y, Zhang J, et al. Deubiquitinating enzyme OTUB1 promotes cancer cell immunosuppression via preventing ER-associated degradation of immune checkpoint protein PD-L1. Cell Death Differ (2021) 28(6):1773–89. doi: 10.1038/s41418-020-00700-z
154. Xiong W, Gao X, Zhang T, Jiang B, Hu MM, Bu X, et al. USP8 inhibition reshapes an inflamed tumor microenvironment that potentiates the immunotherapy. Nat Commun (2022) 13(1):1700. doi: 10.1038/s41467-022-29401-6
155. Qian G, Guo J, Vallega KA, Hu C, Chen Z, Deng Y, et al. Membrane-associated RING-CH 8 functions as a novel PD-L1 E3 ligase to mediate PD-L1 degradation induced by EGFR inhibitors. Mol Cancer Res (2021) 19(10):1622–34. doi: 10.1158/1541-7786.MCR-21-0147
156. Mezzadra R, Sun C, Jae LT, Gomez-Eerland R, de Vries E, Wu W, et al. Identification of CMTM6 and CMTM4 as PD-L1 protein regulators. Nature (2017) 549(7670):106–10. doi: 10.1038/nature23669
157. Burr ML, Sparbier CE, Chan YC, Williamson JC, Woods K, Beavis PA, et al. CMTM6 maintains the expression of PD-L1 and regulates anti-tumour immunity. Nature (2017) 549(7670):101–5. doi: 10.1038/nature23643
158. Wu Y, Zhang C, Liu X, He Z, Shan B, Zeng Q, et al. ARIH1 signaling promotes anti-tumor immunity by targeting PD-L1 for proteasomal degradation. Nat Commun (2021) 12(1). doi: 10.1038/s41467-021-22467-8
159. Cha JH, Yang WH, Xia W, Wei Y, Chan LC, Lim SO, et al. Metformin promotes antitumor immunity via endoplasmic-reticulum-associated degradation of PD-L1. Mol Cell (2018) 71(4):606–20 e7. doi: 10.1016/j.molcel.2018.07.030
160. Lastwika KJ, Wilson W 3rd, Li QK, Norris J, Xu H, Ghazarian SR, et al. Control of PD-L1 expression by oncogenic activation of the AKT-mTOR pathway in non-small cell lung cancer. Cancer Res (2016) 76(2):227–38. doi: 10.1158/0008-5472.CAN-14-3362
161. Gao Y, Nihira NT, Bu X, Chu C, Zhang J, Kolodziejczyk A, et al. Acetylation-dependent regulation of PD-L1 nuclear translocation dictates the efficacy of anti-PD-1 immunotherapy. Nat Cell Biol (2020) 22(9):1064–75. doi: 10.1038/s41556-020-0562-4
162. Yang Y, Hsu JM, Sun L, Chan LC, Li CW, Hsu JL, et al. Palmitoylation stabilizes PD-L1 to promote breast tumor growth. Cell Res (2019) 29(1):83–6. doi: 10.1038/s41422-018-0124-5
163. Veillon L, Fakih C, Abou-El-Hassan H, Kobeissy F, Mechref Y. Glycosylation changes in brain cancer. ACS Chem Neurosci (2018) 9(1):51–72. doi: 10.1021/acschemneuro.7b00271
164. Brochez L, Chevolet I, Kruse V. The rationale of indoleamine 2,3-dioxygenase inhibition for cancer therapy. Eur J Cancer (2017) 76:167–82. doi: 10.1016/j.ejca.2017.01.011
165. Meireson A, Devos M, Brochez L. IDO expression in cancer: different compartment, different functionality? Front Immunol (2020) 11. doi: 10.3389/fimmu.2020.531491
166. Litzenburger UM, Opitz CA, Sahm F, Rauschenbach KJ, Trump S, Winter M, et al. Constitutive IDO expression in human cancer is sustained by an autocrine signaling loop involving IL-6, STAT3 and the AHR. Oncotarget (2014) 5(4):1038–51. doi: 10.18632/oncotarget.1637
167. Hennequart M, Pilotte L, Cane S, Hoffmann D, Stroobant V, Plaen E, et al. Constitutive IDO1 expression in human tumors is driven by cyclooxygenase-2 and mediates intrinsic immune resistance. Cancer Immunol Res (2017) 5(8):695–709. doi: 10.1158/2326-6066.CIR-16-0400
168. Tian J, Zhang B, Rui K, Wang S. The role of GITR/GITRL interaction in autoimmune diseases. Front Immunol (2020) 11:588682. doi: 10.3389/fimmu.2020.588682
169. Nocentini G, Riccardi C. GITR: a multifaceted regulator of immunity belonging to the tumor necrosis factor receptor superfamily. Eur J Immunol (2005) 35(4):1016–22. doi: 10.1002/eji.200425818
170. Andrews LP, Yano H, Vignali DAA. Inhibitory receptors and ligands beyond PD-1, PD-L1 and CTLA-4: breakthroughs or backups. Nat Immunol (2019) 20(11):1425–34. doi: 10.1038/s41590-019-0512-0
171. Iwai Y, Ishida M, Tanaka Y, Okazaki T, Honjo T, Minato N. Involvement of PD-L1 on tumor cells in the escape from host immune system and tumor immunotherapy by PD-L1 blockade. Proc Natl Acad Sci U.S.A. (2002) 99(19):12293–7. doi: 10.1073/pnas.192461099
172. Tang SM, Deng XT, Zhou J, Li QP, Ge XX, Miao L. Pharmacological basis and new insights of quercetin action in respect to its anti-cancer effects. BioMed Pharmacother (2020) 121:109604. doi: 10.1016/j.biopha.2019.109604
173. Kedhari Sundaram M, Raina R, Afroze N, Bajbouj K, Hamad M, Haque S, et al. Quercetin modulates signaling pathways and induces apoptosis in cervical cancer cells. Biosci Rep (2019) 39(8). doi: 10.1042/BSR20190720
174. Jing L, Lin J, Yang Y, Tao L, Li Y, Liu Z, et al. Quercetin inhibiting the PD-1/PD-L1 interaction for immune-enhancing cancer chemopreventive agent. Phytother Res (2021) 35(11):6441–51. doi: 10.1002/ptr.7297
175. Kang DY, Sp N, Jo ES, Rugamba A, Hong DY, Lee HG, et al. The inhibitory mechanisms of tumor PD-L1 expression by natural bioactive gallic acid in non-small-cell lung cancer (NSCLC) cells. Cancers (Basel) (2020) 12(3). doi: 10.3390/cancers12030727
176. Rugamba A, Kang DY, Sp N, Jo ES, Lee JM, Bae SW, et al. Silibinin regulates tumor progression and tumorsphere formation by suppressing PD-L1 expression in non-small cell lung cancer (NSCLC) cells. Cells (2021) 10(7). doi: 10.3390/cells10071632
177. Imran M, Ghorat F, Ul-Haq I, Ur-Rehman H, Aslam F, Heydari M, et al. Lycopene as a natural antioxidant used to prevent human health disorders. Antioxid (Basel) (2020) 9(8). doi: 10.3390/antiox9080706
178. Jiang X, Wu H, Zhao W, Ding X, You Q, Zhu F, et al. Lycopene improves the efficiency of anti-PD-1 therapy via activating IFN signaling of lung cancer cells. Cancer Cell Int (2019) 19:68. doi: 10.1186/s12935-019-0789-y
179. Xing Y, Mi C, Wang Z, Zhang ZH, Li MY, Zuo HX, et al. Fraxinellone has anticancer activity in vivo by inhibiting programmed cell death-ligand 1 expression by reducing hypoxia-inducible factor-1α and STAT3. Pharmacol Res (2018) 135:166–80. doi: 10.1016/j.phrs.2018.08.004
180. Rawangkan A, Wongsirisin P, Namiki K, Iida K, Kobayashi Y, Shimizu Y, et al. Green tea catechin is an alternative immune checkpoint inhibitor that inhibits PD-L1 expression and lung tumor growth. Molecules (2018) 23(8). doi: 10.3390/molecules23082071
181. Lopez-Lazaro M. Distribution and biological activities of the flavonoid luteolin. Mini Rev Med Chem (2009) 9(1):31–59. doi: 10.2174/138955709787001712
182. Miean KH, Mohamed S. Flavonoid (myricetin, quercetin, kaempferol, luteolin, and apigenin) content of edible tropical plants. J Agric Food Chem (2001) 49(6):3106–12. doi: 10.1021/jf000892m
183. Jiang ZB, Wang WJ, Xu C, Xie YJ, Wang XR, Zhang YZ, et al. Luteolin and its derivative apigenin suppress the inducible PD-L1 expression to improve anti-tumor immunity in KRAS-mutant lung cancer. Cancer Lett (2021) 515:36–48. doi: 10.1016/j.canlet.2021.05.019
184. Chen YC, He XL, Qi L, Shi W, Yuan LW, Huang MY, et al. Myricetin inhibits interferon-γ-induced PD-L1 and IDO1 expression in lung cancer cells. Biochem Pharmacol (2022) 197:114940. doi: 10.1016/j.bcp.2022.114940
185. Sp N, Kang DY, Lee JM, Jang KJ. Mechanistic Insights of Anti-Immune Evasion by Nobiletin through Regulating miR-197/STAT3/PD-L1 Signaling in Non-Small Cell Lung Cancer (NSCLC) Cells. Int J Mol Sci (2021) 22(18). doi: 10.3390/ijms22189843
186. Yamagata K, Uzu E, Yoshigai Y, Kato C, Tagami M. Oleic acid and oleoylethanolamide decrease interferon-γ-induced expression of PD-L1 and induce apoptosis in human lung carcinoma cells. Eur J Pharmacol (2021) 903:174116. doi: 10.1016/j.ejphar.2021.174116
187. Kang DY, Sp N, Lee JM, Jang KJ. Antitumor effects of ursolic acid through mediating the inhibition of STAT3/PD-L1 signaling in non-small cell lung cancer cells. Biomedicines (2021) 9(3). doi: 10.3390/biomedicines9030297
188. Zhao L, Zhang W, Luan F, Chen X, Wu H, He Q, et al. Butein suppresses PD-L1 expression via downregulating STAT1 in non-small cell lung cancer. BioMed Pharmacother (2023) 157:114030. doi: 10.1016/j.biopha.2022.114030
189. Xie Y, Ding J, Gao J, Zhang J, Cen S, Zhou J. Triptolide reduces PD-L1 through the EGFR and IFN-γ/IRF1 dual signaling pathways. Int Immunopharmacol (2023) 118:109993. doi: 10.1016/j.intimp.2023.109993
190. Wang XR, Jiang ZB, Xu C, Meng WY, Liu P, Zhang YZ, et al. Andrographolide suppresses non-small-cell lung cancer progression through induction of autophagy and antitumor immune response. Pharmacol Res (2022) 179:106198. doi: 10.1016/j.phrs.2022.106198
191. Jiang Z, Yang Y, Yang Y, Zhang Y, Yue Z, Pan Z, et al. Ginsenoside Rg3 attenuates cisplatin resistance in lung cancer by downregulating PD-L1 and resuming immune. BioMed Pharmacother (2017) 96:378–83. doi: 10.1016/j.biopha.2017.09.129
192. Hu M, Yang J, Qu L, Deng X, Duan Z, Fu R, et al. Ginsenoside Rk1 induces apoptosis and downregulates the expression of PD-L1 by targeting the NF-κB pathway in lung adenocarcinoma. Food Funct (2020) 11(1):456–71. doi: 10.1039/C9FO02166C
193. Tian W, Sun Y, Cheng Y, Ma X, Du W, Shi W, et al. Arsenic sulfide reverses cisplatin resistance in non-small cell lung cancer in vitro and in vivo through targeting PD-L1. Thorac Cancer (2021) 12(19):2551–63. doi: 10.1111/1759-7714.14136
194. Jiang ZB, Huang JM, Xie YJ, Zhang YZ, Chang C, Lai HL, et al. Evodiamine suppresses non-small cell lung cancer by elevating CD8(+) T cells and downregulating the MUC1-C/PD-L1 axis. J Exp Clin Cancer Res (2020) 39(1):249. doi: 10.1186/s13046-020-01741-5
195. Varlı M, Pham HT, Kim SM, Taş İ, Gamage CDB, Zhou R, et al. An acetonic extract and secondary metabolites from the endolichenic fungus Nemania sp. EL006872 exhibit immune checkpoint inhibitory activity in lung cancer cell. Front Pharmacol (2022) 13:986946. doi: 10.3389/fphar.2022.986946
196. Yuan LW, Jiang XM, Xu YL, Huang MY, Chen YC, Yu WB, et al. Licochalcone A inhibits interferon-gamma-induced programmed death-ligand 1 in lung cancer cells. Phytomedicine (2021) 80:153394. doi: 10.1016/j.phymed.2020.153394
197. Liu Y, Liu X, Zhang N, Yin M, Dong J, Zeng Q, et al. Berberine diminishes cancer cell PD-L1 expression and facilitates antitumor immunity via inhibiting the deubiquitination activity of CSN5. Acta Pharm Sin B (2020) 10(12):2299–312. doi: 10.1016/j.apsb.2020.06.014
198. Zhang S, Zhou L, Zhang M, Wang Y, Wang M, Du J, et al. Berberine maintains the neutrophil N1 phenotype to reverse cancer cell resistance to doxorubicin. Front Pharmacol (2019) 10:1658. doi: 10.3389/fphar.2019.01658
199. Zhang N, Dou Y, Liu L, Zhang X, Liu X, Zeng Q, et al. SA-49, a novel aloperine derivative, induces MITF-dependent lysosomal degradation of PD-L1. EBioMedicine (2019) 40:151–62. doi: 10.1016/j.ebiom.2019.01.054
200. Huang MY, Jiang XM, Xu YL, Yuan LW, Chen YC, Cui G, et al. Platycodin D triggers the extracellular release of programed death Ligand-1 in lung cancer cells. Food Chem Toxicol (2019) 131:110537. doi: 10.1016/j.fct.2019.05.045
201. Sun TX, Li MY, Zhang ZH, Wang JY, Xing Y, Ri M, et al. Usnic acid suppresses cervical cancer cell proliferation by inhibiting PD-L1 expression and enhancing T-lymphocyte tumor-killing activity. Phytother Res (2021) 35(7):3916–35. doi: 10.1002/ptr.7103
202. Li L, Yokoyama S, Han N, Hayakawa Y. Lac water extract inhibits IFN-γ signaling through JAK2-STAT1-IRF1 axis in human melanoma. RSC Adv (2018) 8(38):21534–40. doi: 10.1039/C8RA02955E
203. Lopatina KA, Safonova EA, Nevskaya KV, Stakheeva MN, Gur'ev AM, Zueva EP, et al. Effect of acorus calamus L. Polysaccharide on CD274 and CD326 expression by lewis lung carcinoma cells in mice. Bull Exp Biol Med (2017) 164(1):102–5. doi: 10.1007/s10517-017-3934-4
204. Abdelwahed A, Bouhlel I, Skandrani I, Valenti K, Kadri M, Guiraud P, et al. Study of antimutagenic and antioxidant activities of gallic acid and 1,2,3,4,6-pentagalloylglucose from Pistacia lentiscus. Confirmation by microarray expression profiling. Chem Biol Interact (2007) 165(1):1–13. doi: 10.1016/j.cbi.2006.10.003
205. Shahrzad S, Aoyagi K, Winter A, Koyama A, Bitsch I. Pharmacokinetics of gallic acid and its relative bioavailability from tea in healthy humans. J Nutr (2001) 131(4):1207–10. doi: 10.1093/jn/131.4.1207
206. Liao CC, Chen SC, Huang HP, Wang CJ. Gallic acid inhibits bladder cancer cell proliferation and migration via regulating fatty acid synthase (FAS). J Food Drug Anal (2018) 26(2):620–7. doi: 10.1016/j.jfda.2017.06.006
207. Phan AN, Hua TN, Kim MK, Vo VT, Choi JW, Kim HW, et al. Gallic acid inhibition of Src-Stat3 signaling overcomes acquired resistance to EGF receptor tyrosine kinase inhibitors in advanced non-small cell lung cancer. Oncotarget (2016) 7(34):54702–13. doi: 10.18632/oncotarget.10581
208. Cheung CW, Gibbons N, Johnson DW, Nicol DL. Silibinin–a promising new treatment for cancer. Anticancer Agents Med Chem (2010) 10(3):186–95. doi: 10.2174/1871520611009030186
209. Mateen S, Raina K, Agarwal R. Chemopreventive and anti-cancer efficacy of silibinin against growth and progression of lung cancer. Nutr Cancer (2013) 65 Suppl 1(0 1):3–11. doi: 10.1080/01635581.2013.785004
210. Yang S, Li Z, Wang J, Ruan J, Zheng C, Huang P, et al. Eudesmane-type sesquiterpene glycosides from dictamnus dasycarpus turcz. Molecules (2018) 23(3). doi: 10.3390/molecules23030642
211. Wu XF, Ouyang ZJ, Feng LL, Chen G, Guo WJ, Shen Y, et al. Suppression of NF-kappaB signaling and NLRP3 inflammasome activation in macrophages is responsible for the amelioration of experimental murine colitis by the natural compound fraxinellone. Toxicol Appl Pharmacol (2014) 281(1):146–56. doi: 10.1016/j.taap.2014.10.002
212. Kim JH, Park YM, Shin JS, Park SJ, Choi JH, Jung HJ, et al. Fraxinellone inhibits lipopolysaccharide-induced inducible nitric oxide synthase and cyclooxygenase-2 expression by negatively regulating nuclear factor-kappa B in RAW 264.7 macrophages cells. Biol Pharm Bull (2009) 32(6):1062–8. doi: 10.1248/bpb.32.1062
213. Fujiki H, Watanabe T, Sueoka E, Rawangkan A, Suganuma M. Cancer prevention with green tea and its principal constituent, EGCG: from early investigations to current focus on human cancer stem cells. Mol Cells (2018) 41(2):73–82. doi: 10.14348/molcells.2018.2227
214. Sudhakaran M, Sardesai S, Doseff AI. Flavonoids: new frontier for immuno-regulation and breast cancer control. Antioxid (Basel) (2019) 8(4). doi: 10.3390/antiox8040103
215. Yan X, Qi M, Li P, Zhan Y, Shao H. Apigenin in cancer therapy: anti-cancer effects and mechanisms of action. Cell Biosci (2017) 7:50. doi: 10.1186/s13578-017-0179-x
216. Semwal DK, Semwal RB, Combrinck S, Viljoen A. Myricetin: A dietary molecule with diverse biological activities. Nutrients (2016) 8(2):90. doi: 10.3390/nu8020090
217. Zhang Y, Ma JN, Ma CL, Qi Z, Ma CM. Simultaneous quantification of ten constituents of Xanthoceras sorbifolia Bunge using UHPLC-MS methods and evaluation of their radical scavenging, DNA scission protective, and alpha-glucosidase inhibitory activities. Chin J Nat Med (2015) 13(11):873–80. doi: 10.1016/S1875-5364(15)30092-3
218. Zhong S, Kong Y, Zhou L, Zhou C, Zhang X, Wang Y. Efficient conversion of myricetin from Ampelopsis grossedentata extracts and its purification by MIP-SPE. J Chromatogr B Analyt Technol BioMed Life Sci (2014) 945-946:39–45. doi: 10.1016/j.jchromb.2013.11.036
219. Song X, Tan L, Wang M, Ren C, Guo C, Yang B, et al. Myricetin: A review of the most recent research. BioMed Pharmacother (2021) 134:111017. doi: 10.1016/j.biopha.2020.111017
220. Jiang M, Zhu M, Wang L, Yu S. Anti-tumor effects and associated molecular mechanisms of myricetin. BioMed Pharmacother (2019) 120:109506. doi: 10.1016/j.biopha.2019.109506
221. Shih YW, Wu PF, Lee YC, Shi MD, Chiang TA. Myricetin suppresses invasion and migration of human lung adenocarcinoma A549 cells: possible mediation by blocking the ERK signaling pathway. J Agric Food Chem (2009) 57(9):3490–9. doi: 10.1021/jf900124r
222. Luo G, Guan X, Zhou L. Apoptotic effect of citrus fruit extract nobiletin on lung cancer cell line A549 in vitro and in vivo. Cancer Biol Ther (2008) 7(6):966–73. doi: 10.4161/cbt.7.6.5967
223. Battino M, Forbes-Hernandez TY, Gasparrini M, Afrin S, Cianciosi D, Zhang J, et al. Relevance of functional foods in the Mediterranean diet: the role of olive oil, berries and honey in the prevention of cancer and cardiovascular diseases. Crit Rev Food Sci Nutr (2019) 59(6):893–920. doi: 10.1080/10408398.2018.1526165
224. Fu J, Gaetani S, Oveisi F, Lo Verme J, Serrano A, Rodriguez De Fonseca F, et al. Oleylethanolamide regulates feeding and body weight through activation of the nuclear receptor PPAR-alpha. Nature (2003) 425(6953):90–3. doi: 10.1038/nature01921
225. Feng XM, Su XL. Anticancer effect of ursolic acid via mitochondria-dependent pathways. Oncol Lett (2019) 17(6):4761–7. doi: 10.3892/ol.2019.10171
226. Wang M, Yu H, Wu R, Chen ZY, Hu Q, Zhang YF, et al. Autophagy inhibition enhances the inhibitory effects of ursolic acid on lung cancer cells. Int J Mol Med (2020) 46(5):1816–26. doi: 10.3892/ijmm.2020.4714
227. Wang X, Zhang F, Yang L, Mei Y, Long H, Zhang X, et al. Ursolic acid inhibits proliferation and induces apoptosis of cancer cells in vitro and in vivo. J BioMed Biotechnol (2011) 2011:419343. doi: 10.1155/2011/419343
228. Padmavathi G, Roy NK, Bordoloi D, Arfuso F, Mishra S, Sethi G, et al. Butein in health and disease: A comprehensive review. Phytomedicine (2017) 25:118–27. doi: 10.1016/j.phymed.2016.12.002
229. Padmavathi G, Rathnakaram SR, Monisha J, Bordoloi D, Roy NK, Kunnumakkara AB. Potential of butein, a tetrahydroxychalcone to obliterate cancer. Phytomedicine (2015) 22(13):1163–71. doi: 10.1016/j.phymed.2015.08.015
230. Reno TA, Kim JY, Raz DJ. Triptolide inhibits lung cancer cell migration, invasion, and metastasis. Ann Thorac Surg (2015) 100(5):1817–24. doi: 10.1016/j.athoracsur.2015.05.074
231. Zhang L, Yu JS. Triptolide reverses helper T cell inhibition and down-regulates IFN-gamma induced PD-L1 expression in glioma cell lines. J Neurooncol (2019) 143(3):429–36. doi: 10.1007/s11060-019-03193-0
232. Liang M, Fu J. Triptolide inhibits interferon-gamma-induced programmed death-1-ligand 1 surface expression in breast cancer cells. Cancer Lett (2008) 270(2):337–41. doi: 10.1016/j.canlet.2008.05.025
233. Kandanur SGS, Tamang N, Golakoti NR, Nanduri S. Andrographolide: A natural product template for the generation of structurally and biologically diverse diterpenes. Eur J Med Chem (2019) 176:513–33. doi: 10.1016/j.ejmech.2019.05.022
234. He W, Sun J, Zhang Q, Li Y, Fu Y, Zheng Y, et al. Andrographolide exerts anti-inflammatory effects in Mycobacterium tuberculosis-infected macrophages by regulating the Notch1/Akt/NF-kappaB axis. J Leukoc Biol (2020) 108(6):1747–64. doi: 10.1002/JLB.3MA1119-584RRR
235. Varma A, Padh H, Shrivastava N. Andrographolide: a new plant-derived antineoplastic entity on horizon. Evid Based Complement Alternat Med (2011) 2011:815390. doi: 10.1093/ecam/nep135
237. Liu T, Zhao L, Zhang Y, Chen W, Liu D, Hou H, et al. Ginsenoside 20(S)-Rg3 targets HIF-1alpha to block hypoxia-induced epithelial-mesenchymal transition in ovarian cancer cells. PloS One (2014) 9(9):e103887. doi: 10.1371/journal.pone.0103887
238. Lu DP, Qiu JY, Jiang B, Wang Q, Liu KY, Liu YR, et al. Tetra-arsenic tetra-sulfide for the treatment of acute promyelocytic leukemia: a pilot report. Blood (2002) 99(9):3136–43. doi: 10.1182/blood.V99.9.3136
239. Pastorek M, Gronesova P, Cholujova D, Hunakova L, Bujnakova Z, Balaz P, et al. Realgar (As4S4) nanoparticles and arsenic trioxide (As2O3) induced autophagy and apoptosis in human melanoma cells in vitro. Neoplasma (2014) 61(6):700–9. doi: 10.4149/neo_2014_085
240. Mohan V, Agarwal R, Singh RP. A novel alkaloid, evodiamine causes nuclear localization of cytochrome-c and induces apoptosis independent of p53 in human lung cancer cells. Biochem Biophys Res Commun (2016) 477(4):1065–71. doi: 10.1016/j.bbrc.2016.07.037
241. Yang S, Chen J, Tan T, Wang N, Huang Y, Wang Y, et al. Evodiamine exerts anticancer effects against 143B and MG63 cells through the wnt/beta-catenin signaling pathway. Cancer Manag Res (2020) 12:2875–88. doi: 10.2147/CMAR.S238093
242. Ni WJ, Ding HH, Tang LQ. Berberine as a promising anti-diabetic nephropathy drug: An analysis of its effects and mechanisms. Eur J Pharmacol (2015) 760:103–12. doi: 10.1016/j.ejphar.2015.04.017
243. Liu B, Wang G, Yang J, Pan X, Yang Z, Zang L. Berberine inhibits human hepatoma cell invasion without cytotoxicity in healthy hepatocytes. PloS One (2011) 6(6):e21416. doi: 10.1371/journal.pone.0021416
244. Zhang L, Wang Y, Yang D, Zhang C, Zhang N, Li M, et al. Platycodon grandiflorus - an ethnopharmacological, phytochemical and pharmacological review. J Ethnopharmacol (2015) 164:147–61. doi: 10.1016/j.jep.2015.01.052
245. Wang H, Yang S, Zhou H, Sun M, Du L, Wei M, et al. Aloperine executes antitumor effects against multiple myeloma through dual apoptotic mechanisms. J Hematol Oncol (2015) 8(1). doi: 10.1186/s13045-015-0120-x
246. Zhang LI, Zheng Y, Deng H, Liang LEI, Peng J. Aloperine induces G2/M phase cell cycle arrest and apoptosis in HCT116 human colon cancer cells. Int J Mol Med (2014) 33(6):1613–20. doi: 10.3892/ijmm.2014.1718
247. Ingolfsdottir K. Usnic acid. Phytochemistry (2002) 61(7):729–36. doi: 10.1016/S0031-9422(02)00383-7
248. Chen S, Ma S, Wang H, Shao X, Ding B, Guo Z, et al. Unraveling the mechanism of alkaloids from Sophora alopecuroides Linn combined with immune checkpoint blockade in the treatment of non-small cell lung cancer based on systems pharmacology. Bioorg Med Chem (2022) 64:116724. doi: 10.1016/j.bmc.2022.116724
249. Chen YC, Huang MY, Zhang LL, Feng ZL, Jiang XM, Yuan LW, et al. Nagilactone E increases PD-L1 expression through activation of c-Jun in lung cancer cells. Chin J Nat Med (2020) 18(7):517–25. doi: 10.1016/S1875-5364(20)30062-5
250. Yang M, Li Z, Tao J, Hu H, Li Z, Zhang Z, et al. Resveratrol induces PD-L1 expression through snail-driven activation of Wnt pathway in lung cancer cells. J Cancer Res Clin Oncol (2021) 147(4):1101–13. doi: 10.1007/s00432-021-03510-z
251. Maj E, Maj B, Bobak K, Gos M, Chodyński M, Kutner A, et al. Differential response of lung cancer cells, with various driver mutations, to plant polyphenol resveratrol and vitamin D active metabolite PRI-2191. Int J Mol Sci (2021) 22(5). doi: 10.3390/ijms22052354
252. Tian H, Gui Y, Wei Y, Shang B, Sun J, Ma S, et al. Z-guggulsterone induces PD-L1 upregulation partly mediated by FXR, Akt and Erk1/2 signaling pathways in non-small cell lung cancer. Int Immunopharmacol (2021) 93:107395. doi: 10.1016/j.intimp.2021.107395
253. Luo L, Xu G. Fascaplysin induces apoptosis and ferroptosis, and enhances anti-PD-1 immunotherapy in non-small cell lung cancer (NSCLC) by promoting PD-L1 expression. Int J Mol Sci (2022) 23(22). doi: 10.3390/ijms232213774
254. Topalian SL, Hodi FS, Brahmer JR, Gettinger SN, Smith DC, McDermott DF, et al. Safety, activity, and immune correlates of anti-PD-1 antibody in cancer. N Engl J Med (2012) 366(26):2443–54. doi: 10.1056/NEJMoa1200690
255. Liu H, Zou M, Li P, Wang H, Lin X, Ye J. Oxymatrine−mediated maturation of dendritic cells leads to activation of FOXP3+/CD4+ Treg cells and reversal of cisplatin−resistance in lung cancer cells. Mol Med Rep (2019) 19(5):4081–90. doi: 10.3892/mmr.2019.10064
256. Feng ZL, Zhang LL, Zheng YD, Liu QY, Liu JX, Feng L, et al. Norditerpenoids and dinorditerpenoids from the seeds of podocarpus nagi as cytotoxic agents and autophagy inducers. J Nat Prod (2017) 80(7):2110–7. doi: 10.1021/acs.jnatprod.7b00347
257. Zhang LL, Jiang XM, Huang MY, Feng ZL, Chen X, Wang Y, et al. Nagilactone E suppresses TGF-beta1-induced epithelial-mesenchymal transition, migration and invasion in non-small cell lung cancer cells. Phytomedicine (2019) 52:32–9. doi: 10.1016/j.phymed.2018.09.222
258. Urizar NL, Moore DD. GUGULIPID: a natural cholesterol-lowering agent. Annu Rev Nutr (2003) 23:303–13. doi: 10.1146/annurev.nutr.23.011702.073102
259. An MJ, Cheon JH, Kim SW, Kim ES, Kim TI, Kim WH. Guggulsterone induces apoptosis in colon cancer cells and inhibits tumor growth in murine colorectal cancer xenografts. Cancer Lett (2009) 279(1):93–100. doi: 10.1016/j.canlet.2009.01.026
260. You W, Li L, Sun D, Liu X, Xia Z, Xue S, et al. Farnesoid X receptor constructs an immunosuppressive microenvironment and sensitizes FXR(high)PD-L1(low) NSCLC to anti-PD-1 immunotherapy. Cancer Immunol Res (2019) 7(6):990–1000. doi: 10.1158/2326-6066.CIR-17-0672
261. Soni R, Muller L, Furet P, Schoepfer J, Stephan C, Zumstein-Mecker S, et al. Inhibition of cyclin-dependent kinase 4 (Cdk4) by fascaplysin, a marine natural product. Biochem Biophys Res Commun (2000) 275(3):877–84. doi: 10.1006/bbrc.2000.3349
262. Ning Y, Xu D, Zhang X, Bai Y, Ding J, Feng T, et al. β-glucan restores tumor-educated dendritic cell maturation to enhance antitumor immune responses. Int J Cancer (2016) 138(11):2713–23. doi: 10.1002/ijc.30002
263. Bi S, Huang W, Chen S, Huang C, Li C, Guo Z, et al. Cordyceps militaris polysaccharide converts immunosuppressive macrophages into M1-like phenotype and activates T lymphocytes by inhibiting the PD-L1/PD-1 axis between TAMs and T lymphocytes. Int J Biol Macromol (2020) 150:261–80. doi: 10.1016/j.ijbiomac.2020.02.050
264. Safonova EA, Lopatina KA, Razina TG, Zueva EP, Gur'ev AM, Belousov MV. Effects of tussilago farfara L. Polysaccharides on the expression of PD-1 (CD279) and PD-L1 (CD274) in peripheral blood and tumor tissue lymphocytes in mice with lewis lung carcinoma. Bull Exp Biol Med (2020) 169(3):378–82. doi: 10.1007/s10517-020-04891-w
265. Yang R, Pei T, Huang R, Xiao Y, Yan J, Zhu J, et al. Platycodon grandiflorum triggers antitumor immunity by restricting PD-1 expression of CD8(+) T cells in local tumor microenvironment. Front Pharmacol (2022) 13:774440. doi: 10.3389/fphar.2022.774440
266. Xing Y, Gui S, Hu W, Su Y, Zhang W, Hu C, et al. Artesunate upregulates the expression of CD39, CD279 and granzyme B in CD4(+) and CD8(+) T lymphocytes of patients with lung cancer. Xi Bao Yu Fen Zi Mian Yi Xue Za Zhi (2022) 38(4):338–46. doi: 10.13423/j.cnki.cjcmi.009362
267. Liu Q, Zhang C, Sun A, Zheng Y, Wang L, Cao X. Tumor-educated CD11bhighIalow regulatory dendritic cells suppress T cell response through arginase I. J Immunol (2009) 182(10):6207–16. doi: 10.4049/jimmunol.0803926
268. Li T, Chen X, Chen X, Ma DL, Leung CH, Lu JJ. Platycodin D potentiates proliferation inhibition and apoptosis induction upon AKT inhibition via feedback blockade in non-small cell lung cancer cells. Sci Rep (2016) 6:37997. doi: 10.1038/srep37997
269. Zhao R, Chen M, Jiang Z, Zhao F, Xi B, Zhang X, et al. Platycodin-D induced autophagy in non-small cell lung cancer cells via PI3K/akt/mTOR and MAPK signaling pathways. J Cancer (2015) 6(7):623–31. doi: 10.7150/jca.11291
270. Park MJ, Ryu HS, Kim JS, Lee HK, Kang JS, Yun J, et al. Platycodon grandiflorum polysaccharide induces dendritic cell maturation via TLR4 signaling. Food Chem Toxicol (2014) 72:212–20. doi: 10.1016/j.fct.2014.07.011
271. Han EH, Park JH, Kim JY, Chung YC, Jeong HG. Inhibitory mechanism of saponins derived from roots of Platycodon grandiflorum on anaphylactic reaction and IgE-mediated allergic response in mast cells. Food Chem Toxicol (2009) 47(6):1069–75. doi: 10.1016/j.fct.2009.01.041
272. Wang C, Schuller Levis GB, Lee EB, Levis WR, Lee DW, Kim BS, et al. Platycodin D and D3 isolated from the root of Platycodon grandiflorum modulate the production of nitric oxide and secretion of TNF-alpha in activated RAW 264. 7 Cells Int Immunopharmacol (2004) 4(8):1039–49. doi: 10.1016/j.intimp.2004.04.005
273. Zhang A, Zheng Y, Que Z, Zhang L, Lin S, Le V, et al. Astragaloside IV inhibits progression of lung cancer by mediating immune function of Tregs and CTLs by interfering with IDO. J Cancer Res Clin Oncol (2014) 140(11):1883–90. doi: 10.1007/s00432-014-1744-x
274. Su C, Zhang P, Liu J, Cao Y. Erianin inhibits indoleamine 2, 3-dioxygenase -induced tumor angiogenesis. BioMed Pharmacother (2017) 88:521–8. doi: 10.1016/j.biopha.2017.01.090
275. Hu BQ, Huang JF, Niu K, Zhou J, Wang NN, Liu Y, et al. B7-H3 but not PD-L1 is involved in the antitumor effects of Dihydroartemisinin in non-small cell lung cancer. Eur J Pharmacol (2023) 950:175746. doi: 10.1016/j.ejphar.2023.175746
276. Tian L, Xie XH, Zhu ZH. Calotropin regulates the apoptosis of non−small cell cancer by regulating the cytotoxic T−lymphocyte associated antigen 4−mediated TGF−β/ERK signaling pathway. Mol Med Rep (2018) 17(6):7683–91. doi: 10.3892/mmr.2018.8853
277. Zhan Y, Chen Q, Song Y, Wei X, Zhao T, Chen B, et al. Berbamine Hydrochloride inhibits lysosomal acidification by activating Nox2 to potentiate chemotherapy-induced apoptosis via the ROS-MAPK pathway in human lung carcinoma cells. Cell Biol Toxicol (2022) 39:1297–317. doi: 10.1007/s10565-022-09756-8
278. Prendergast GC. Immune escape as a fundamental trait of cancer: focus on IDO. Oncogene (2008) 27(28):3889–900. doi: 10.1038/onc.2008.35
279. Cao J, Chen Z, Zhu Y, Li Y, Guo C, Gao K, et al. Huangqi-Honghua combination and its main components ameliorate cerebral infarction with Qi deficiency and blood stasis syndrome by antioxidant action in rats. J Ethnopharmacol (2014) 155(2):1053–60. doi: 10.1016/j.jep.2014.05.061
280. Li J, Zhong Y, Li H, Zhang N, Ma W, Cheng G, et al. Enhancement of Astragalus polysaccharide on the immune responses in pigs inoculated with foot-and-mouth disease virus vaccine. Int J Biol Macromol (2011) 49(3):362–8. doi: 10.1016/j.ijbiomac.2011.05.015
281. Ng TB, Liu F, Wang ZT. Antioxidative activity of natural products from plants. Life Sci (2000) 66(8):709–23. doi: 10.1016/S0024-3205(99)00642-6
282. Dai X, Zhang X, Chen W, Chen Y, Zhang Q, Mo S, et al. Dihydroartemisinin: A potential natural anticancer drug. Int J Biol Sci (2021) 17(2):603–22. doi: 10.7150/ijbs.50364
283. Mo EP, Zhang RR, Xu J, Zhang H, Wang XX, Tan QT, et al. Calotropin from Asclepias curasavica induces cell cycle arrest and apoptosis in cisplatin-resistant lung cancer cells. Biochem Biophys Res Commun (2016) 478(2):710–5. doi: 10.1016/j.bbrc.2016.08.011
284. Xie J, Ma T, Gu Y, Zhang X, Qiu X, Zhang L, et al. Berbamine derivatives: a novel class of compounds for anti-leukemia activity. Eur J Med Chem (2009) 44(8):3293–8. doi: 10.1016/j.ejmech.2009.02.018
285. Zhang H, Zhu T, Fu R, Peng Y, Jing P, Xu W, et al. Combination of detoxified pneumolysin derivative deltaA146Ply and berbamine as a treatment approach for breast cancer. Mol Ther Oncol (2020) 18:247–61. doi: 10.1016/j.omto.2020.06.015
286. Li W, Li Y, Tian W, Han X, Zhao J, Xin Z, et al. 2-methylbenzoyl berbamine, a multi-targeted inhibitor, suppresses the growth of human osteosarcoma through disabling NF-kappaB, ERK and AKT signaling networks. Aging (Albany NY) (2020) 12(14):15037–49. doi: 10.18632/aging.103565
287. Zhang Q, Chen X, Luo Y, Ren H, Qiao T. Fuzi enhances anti-tumor efficacy of radiotherapy on lung cancer. J Cancer (2017) 8(19):3945–51. doi: 10.7150/jca.22162
288. He CS, Liu YC, Xu ZP, Dai PC, Chen XW, Jin DH. Astragaloside IV enhances cisplatin chemosensitivity in non-small cell lung cancer cells through inhibition of B7-H3. Cell Physiol Biochem (2016) 40(5):1221–9. doi: 10.1159/000453175
289. Chen Y, Zhang Y, Song W, Zhang Y, Dong X, Tan M. Ginsenoside rh2 improves the cisplatin anti-tumor effect in lung adenocarcinoma A549 cells via superoxide and PD-L1. Anticancer Agents Med Chem (2020) 20(4):495–503. doi: 10.2174/1871520619666191209091230
290. Cuyàs E, Pérez-Sánchez A, Micol V, Menendez JA, Bosch-Barrera J. STAT3-targeted treatment with silibinin overcomes the acquired resistance to crizotinib in ALK-rearranged lung cancer. Cell Cycle (2016) 15(24):3413–8. doi: 10.1080/15384101.2016.1245249
291. Tsao SM, Wu TC, Chen J, Chang F, Tsao T. Astragalus polysaccharide injection (PG2) normalizes the neutrophil-to-lymphocyte ratio in patients with advanced lung cancer receiving immunotherapy. Integr Cancer Ther (2021) 20:1534735421995256. doi: 10.1177/1534735421995256
292. Liu S, Han Z, Trivett AL, Lin H, Hannifin S, Yang D, et al. Cryptotanshinone has curative dual anti-proliferative and immunotherapeutic effects on mouse Lewis lung carcinoma. Cancer Immunol Immunother (2019) 68(7):1059–71. doi: 10.1007/s00262-019-02326-8
293. Liou SS, Liu IM, Lai MC, Cheng JT. Comparison of the antinociceptive action of crude Fuzei, the root of Aconitum, and its processed products. J Ethnopharmacol (2005) 99(3):379–83. doi: 10.1016/j.jep.2005.02.014
294. Li H, Sun M, Xu J, Li H, Zang M, Cui Y. Immunological response in H22 transplanted mice undergoing Aconitum coreanum polysaccharide treatment. Int J Biol Macromol (2013) 55:295–300. doi: 10.1016/j.ijbiomac.2013.01.011
295. Sun J, Mao Y, Zhang YQ, Guo YD, Mu CY, Fu FQ, et al. Clinical significance of the induction of macrophage differentiation by the costimulatory molecule B7-H3 in human non-small cell lung cancer. Oncol Lett (2013) 6(5):1253–60. doi: 10.3892/ol.2013.1586
296. Flies DB, Chen L. The new B7s: playing a pivotal role in tumor immunity. J Immunother (2007) 30(3):251–60. doi: 10.1097/CJI.0b013e31802e085a
297. Chung-Kuan W, Ping-Hsiu W, Hung-Chih L. Impressive response of advanced hepatocellular carcinoma to cisplatin combined with sorafenib, nivolumab, and PG2 immunomodulatory injection: A case report. J Clin Case Rep (2018) 08(08). doi: 10.4172/2165-7920.10001161
298. Chen W, Lu Y, Chen G, Huang S. Molecular evidence of cryptotanshinone for treatment and prevention of human cancer. Anticancer Agents Med Chem (2013) 13(7):979–87. doi: 10.2174/18715206113139990115
299. Pang L, Han S, Jiao Y, Jiang S, He X, Li P. Bu Fei Decoction attenuates the tumor associated macrophage stimulated proliferation, migration, invasion and immunosuppression of non-small cell lung cancer, partially via IL-10 and PD-L1 regulation. Int J Oncol (2017) 51(1):25–38. doi: 10.3892/ijo.2017.4014
300. Teng L, Wang K, Chen W, Wang YS, Bi L. HYR-2 plays an anti-lung cancer role by regulating PD-L1 and Akkermansia muciniphila. Pharmacol Res (2020) 160:105086. doi: 10.1016/j.phrs.2020.105086
301. Pan J, Yang H, Zhu L, Lou Y, Jin B. Qingfei Jiedu decoction inhibits PD-L1 expression in lung adenocarcinoma based on network pharmacology analysis, molecular docking and experimental verification. Front Pharmacol (2022) 13:897966. doi: 10.3389/fphar.2022.897966
302. Zhang X, Tong J, Li Z. Qiyusanlong decoction inhibits the level of PD-1/PD-L1 in mice bearing Lewis lung carcinoma. Xi Bao Yu Fen Zi Mian Yi Xue Za Zhi (2016) 32(6):770–4. doi: 10.13423/j.cnki.cjcmi.007785
303. Bi L, Jin S, Zheng Z, Wang Q, Jiao Y, You J, et al. Inhibitory effect of feiji recipe on IDO induced immune escape on the murine model of lewis lung carcinoma. Zhongguo Zhong Xi Yi Jie He Za Zhi (2016) 36(1):69–74.
304. Luo B, Que ZJ, Zhou ZY, Wang Q, Dong CS, Jiang Y, et al. Feiji Recipe inhibits the growth of lung cancer by modulating T-cell immunity through indoleamine-2,3-dioxygenase pathway in an orthotopic implantation model. J Integr Med (2018) 16(4):283–9. doi: 10.1016/j.joim.2018.04.008
305. Luo Y, Wu J, Zhu X, Gong C, Yao C, Ni Z, et al. NK cell-dependent growth inhibition of lewis lung cancer by Yu-Ping-Feng, an ancient Chinese herbal formula. Mediators Inflammation (2016) 2016:3541283. doi: 10.1155/2016/3541283
306. Wang L, Lei X, Wang X. Efficacy and safety of PD-1/PD-L1 inhibitor chemotherapy combined with lung cancer fang no. 1 in relapsed and refractory SCLC: A retrospective observational study. Comput Math Methods Med (2022) 2022:2848220. doi: 10.1155/2022/2848220
307. Zhang Z. Clinical Observation of Jianpichuji Fang Combined with Immune-checkpoint inhibitors in the treatment of advanced non-small cell lung cancer. Cangzhou, Hebei, China: Chengde Medical University (2023).
308. Yang H. The clinical research of the Treatment On NSCLC patients with Yanghe Decotion combined with immune checkpoint inhibitors. Nanchang, Jiangxi, China: Jiangxi University of Chinese Medicine (2023).
309. Yang B, Lu X, Liu H, Liu N, Wang H. Clinical observation of warming spleen and kidney to improve lung cancer immune checkpoint inhibitor associated diarrhea. Jilin J Traditional Chin Med (2022) 42(07):792–5. doi: 10.13463/j.cnki.jlzyy.2022.07.013
310. Pan J, Zhu L, Song K, Yang H, Lou Y, Shi Y, et al. Exploration medication rule of Song Kang applying traditional Chinese medicine to assist gefitinib in syndrome differentiation and treatment of lung adenocarcinoma based on R language. J Zhejiang Chin Med Univ (2020) 44(05):419–25. doi: 10.16466/j.issn1005-5509.2020.05.003
311. Rathaur P, RJ. Metabolism and pharmacokinetics of phytochemicals in the human body. Curr Drug Metab (2019) 20(14):1085–102. doi: 10.2174/1389200221666200103090757
312. McClements DJ. Advances in nanoparticle and microparticle delivery systems for increasing the dispersibility, stability, and bioactivity of phytochemicals. Biotechnol Adv (2020) 38:107287. doi: 10.1016/j.biotechadv.2018.08.004
313. Mondal S, Bandyopadhyay S, Ghosh MK, Mukhopadhyay S, Roy S, Mandal C. Natural products: promising resources for cancer drug discovery. Anticancer Agents Med Chem (2012) 12(1):49–75. doi: 10.2174/187152012798764697
314. Martin AM, Sun EW, Rogers GB, Keating DJ. The influence of the gut microbiome on host metabolism through the regulation of gut hormone release. Front Physiol (2019) 10:428. doi: 10.3389/fphys.2019.00428
315. Carrera-Quintanar L, Lopez Roa RI, Quintero-Fabian S, Sanchez-Sanchez MA, Vizmanos B, Ortuno-Sahagun D. Phytochemicals that influence gut microbiota as prophylactics and for the treatment of obesity and inflammatory diseases. Mediators Inflammation (2018) 2018:9734845. doi: 10.1155/2018/9734845
316. Atanasov AG, Zotchev SB, Dirsch VM, Supuran CT. Natural products in drug discovery: advances and opportunities. Nat Rev Drug Discovery (2021) 20(3):200–16. doi: 10.1038/s41573-020-00114-z
317. Zhang Y, Yao Y, Fu Y, Yuan Z, Wu X, Wang T, et al. Inhibition effect of oxyepiberberine isolated from Coptis chinensis franch. On non-small cell lung cancer based on a network pharmacology approach and experimental validation. J Ethnopharmacol (2021) 278:114267. doi: 10.1016/j.jep.2021.114267
318. Yuan C, Wang MH, Wang F, Chen PY, Ke XG, Yu B, et al. Network pharmacology and molecular docking reveal the mechanism of Scopoletin against non-small cell lung cancer. Life Sci (2021) 270:119105. doi: 10.1016/j.lfs.2021.119105
Keywords: natural product, traditional medicine, lung cancer, immune checkpoint, immunotherapy
Citation: Zhou Y, Wang F, Li G, Xu J, Zhang J, Gullen E, Yang J and Wang J (2024) From immune checkpoints to therapies: understanding immune checkpoint regulation and the influence of natural products and traditional medicine on immune checkpoint and immunotherapy in lung cancer. Front. Immunol. 15:1340307. doi: 10.3389/fimmu.2024.1340307
Received: 17 November 2023; Accepted: 29 January 2024;
Published: 15 February 2024.
Edited by:
Michael R. Shurin, University of Pittsburgh Medical Center, United StatesReviewed by:
Ming Yi, Zhejiang University, ChinaSaurabh Srivastav, Rice University, United States
Wei Wang, Shanxi Zhendong Pharmaceutical, China
Songxiao Xu, University of Chinese Academy of Sciences, China
Copyright © 2024 Zhou, Wang, Li, Xu, Zhang, Gullen, Yang and Wang. This is an open-access article distributed under the terms of the Creative Commons Attribution License (CC BY). The use, distribution or reproduction in other forums is permitted, provided the original author(s) and the copyright owner(s) are credited and that the original publication in this journal is cited, in accordance with accepted academic practice. No use, distribution or reproduction is permitted which does not comply with these terms.
*Correspondence: Jing Wang, andhbmcyOTM2QDEyNi5jb20=
†ORCID: Jing Wang, orcid.org/0000-0002-2735-7283