- Division of Hematologic Malignancies, Department of Medical Oncology, Dana-Farber Cancer Institute, Harvard Medical School, Boston, MA, United States
Allogeneic hematopoietic stem cell transplantation (HSCT) is a potentially curative therapy for many hematologic malignancies as well as non-malignant conditions. Part of the curative basis underlying HSCT for hematologic malignancies relies upon induction of the graft versus leukemia (GVL) effect in which donor immune cells recognize and eliminate residual malignant cells within the recipient, thereby maintaining remission. GVL is a clinically evident phenomenon; however, specific cell types responsible for inducing this effect and molecular mechanisms involved remain largely undefined. One of the best examples of GVL is observed after donor lymphocyte infusions (DLI), an established therapy for relapsed disease or incipient/anticipated relapse. DLI involves infusion of peripheral blood lymphocytes from the original HSCT donor into the recipient. Sustained remission can be observed in 20-80% of patients treated with DLI depending upon the underlying disease and the intrinsic burden of targeted cells. In this review, we will discuss current knowledge about mechanisms of GVL after DLI, experimental strategies for augmenting GVL by manipulation of DLI (e.g. neoantigen vaccination, specific cell type selection/depletion) and research outlook for improving DLI and cellular immunotherapies for hematologic malignancies through better molecular definition of the GVL effect.
1 Introduction
The graft versus leukemia (GVL) effect of allogeneic hematopoietic stem cell transplantation (HSCT) is a clinically well-established phenomenon reliant upon the donor immune system recognizing and eliminating cancerous cells. Effective GVL is a key component of the curative potential of HSCT for hematologic malignancies, yet the biological basis for GVL remains poorly understood, thus limiting the ability to leverage this phenomenon for therapeutic benefit. Anti-cancer immune reactions were clearly observed in animal models of leukemia as early as the 1960s (1), though early immunotherapy trials in humans in the 1980s were underwhelming (2). GVL reactions in humans first gained support in 1990 when Horowitz et al. demonstrated that recipients of allogeneic stem cell grafts depleted of T cells had a higher incidence of relapse (3). This study also found that recipients of syngeneic grafts (i.e. stem cells from an identical twin) had a higher relapse rate compared to recipients of allografts (3). Both lines of evidence support the notion that the allogeneic donor immune system helps to provide immunologic control of disease.
In the modern era, GVL activity is clearly observed in two clinical scenarios of incipient relapse (e.g. recurrence of disease-associated mutations, decreasing cell counts, loss of donor chimerism) or overt relapse. These can be addressed by tapering of immune suppression (IST) and/or donor lymphocyte infusions (DLI). For patients with relapsing disease, immunosuppressive medications for prevention of graft versus host disease (GVHD) can be rapidly tapered, thereby engendering enhanced donor immune activation, primarily T cell alloreactivity. In approximately 20% of patients, IST alone is sufficient to reinduce sustained remission (4). DLI on the other hand involves reinfusion of peripheral blood lymphocytes from the original donor into the HSCT recipient. DLI was first shown to be successful for reinducing remission in patients with relapsed hematologic malignancy in 1990 (5) and since that time has become an established mode of adoptive cellular immunotherapy.
In this review, we will discuss the establishment of DLI as the archetype of effective GVL, its role and efficacy in different hematologic malignancies, clinical applications and combination therapies for enhancing the GVL effect of DLI, and recent biological insights into the mechanisms underlying the success of this therapy.
2 Section 1 – historical context of GVL and role of DLI
One year before E. Donnall Thomas’s seminal work describing the first successful bone marrow transplantation in humans (6), Barnes and Loutit observed the first evidence of GVL activity in rodents (7). Mice treated with radiation doses insufficient to eradicate leukemia cells had disease recurrence if reconstituted with bone marrow stem cells from the same strain but maintained remission if given stem cells from a different strain, thus implicating the donor immune system in elimination of residual leukemia and maintenance of remission. As bone marrow transplantation gained traction for its potential as a curative therapy, the presence of GVL activity was inferred by the observation, beginning in the 1970s, that patients who developed graft versus host disease (GVHD), particularly chronic GVHD, were relatively protected from disease relapse and had improved survival compared to patients without chronic GVHD (8, 9).
GVHD is a common and sometimes fatal complication of HSCT wherein donor immune cell alloreactivity against host tissues causes inflammation, leading to tissue injury (10). In its acute form, typically observed in the first 100 days after HSCT, the most common manifestations include diarrhea, rash, and liver function abnormalities (11). The chronic form of GVHD, usually developing or persisting after 100 days post-HSCT, has many protean manifestations but most commonly affects the skin, eyes, mouth, liver, fascia, and lungs (12). The pathogenesis of GVHD is incompletely understood (12, 13), and, although beyond the scope of the current review, has been well-reviewed elsewhere (14–21). As a major cause of treatment related morbidity and mortality, adequate prevention of GVHD is a key component of HSCT, and numerous strategies have been developed for GVHD prophylaxis (22). Since acute GVHD is thought to be driven largely by donor T cell alloreactivity (23–25), one early and highly effective strategy for GVHD prevention is T cell depletion (TCD) of allografts (26–31). While efficacious for preventing GVHD, many studies have found that TCD is associated with higher relapse rates, consistent with the role of T cells in driving both GVHD and GVL (3, 32–37). Thus donor immune cell activity (predominantly T cell alloreactivity) driving both GVHD and GVL are closely linked phenomena.
Recognition of the relationship of GVHD with GVL prompted early trials of adoptive cellular therapy for relapse prevention. In 1989, Sullivan et al. hypothesized that post-HSCT infusion of donor buffy coat (lymphocytes) to induce GVHD would likewise offer protection from relapse (38). Patients were randomized to receive standard GVHD prophylaxis alone or in combination with buffy coat lymphocytes from the stem cell donor, with the hypothesis that patients receiving the buffy coat lymphocytes may have reduced relapse risk through induction of GVHD. The incidence and severity of acute GVHD was much higher in the patients receiving buffy coat, as was non-relapse mortality (driven largely by infections complicating GVHD); however, 5-year relapse rates did not differ between the two cohorts. Curiously, this study did not find an increased incidence of chronic GVHD among recipients of buffy coat lymphocytes, perhaps explaining the absence of protection from relapse since other studies have consistently found a greater effect of chronic rather than acute GVHD associated with reduced relapse risk (39–42).
The first successful demonstration of DLI for treatment of relapsed disease was reported in 1990 by Kolb et al. (5) In this study, three patients with relapsed chronic myelogenous leukemia (CML) after HSCT were treated with DLI, resulting in complete hematologic and molecular remissions for all patients. Two of the patients developed GVHD. This report marked a turning point in the ability to leverage adoptive cellular immunotherapy for treatment of post-HSCT relapsed hematologic malignancies. A subsequent case report of one patient treated with DLI for post-HSCT relapsed CML demonstrated achievement of molecular remission (e.g. elimination of detectable Philadelphia chromosome BCR-ABL by polymerase chain reaction [PCR]) and return of full donor chimerism in the bone marrow; however, this was only achieved after onset of GVHD, underscoring the relationship between these phenomena and successful DLI (43).
3 Section 2 – clinical applications of DLI
3.1 DLI in CML and other diseases
Prior to 2000 and the subsequent approval of imatinib for treatment of CML, this disease was a common indication for HSCT; thus, many of the early studies of successful DLI were established in CML (5, 44). After 2000, CML became a less common indication for HSCT while the frequency of HSCT for AML increased (45). Several studies have established that post-HSCT relapsed CML is highly sensitive to the GVL effects of DLI, with complete response rates between 60-80% (46–49). DLI has less efficacy in other hematologic diseases, with response rates of approximately 20-30% for other diseases including acute myeloid leukemia (AML), acute lymphocytic leukemia (ALL), multiple myeloma (MM), and non-Hodgkin lymphomas (NHL) (46, 50, 51). Furthermore, CML patients who achieve complete molecular remission after DLI have highly durable responses, sometimes lasting several years and thus resulting in effective cures (52, 53).
Why CML harbors such exquisite sensitivity to the GVL effect of DLI is unclear. Yet this sensitivity to anti-leukemic alloreactivity receives support from several other lines of evidence. First, CML patients are most sensitive to the increased risk of relapse associated with T cell depleted HSCT; whereas T cell depleted HSCT for other diseases does not appear to impact relapse rate to the same degree (3, 34–36, 54, 55). Second, the relationship of development of chronic GVHD with reduced relapse risk appears to be stronger for CML compared to other diseases including AML (56, 57). Curiously, DLI appears to be even more effective for post-HSCT relapsed CML than imatinib (58), The relative homogeneity of stable phase CML compared to other hematologic malignancies could underlie differences in biologic responsivity to GVL effects of donor T cells. Interestingly, more blast phase CML, a more advanced phase biologically more similar to acute leukemia, is relatively insensitive to the GVL effect of DLI, further underscoring the so far undefined contribution of disease biology to success or failure of GVL (46, 59, 60). Yet the basis for these observations require further study which may illuminate opportunities to optimize DLI for other diseases through better understanding of the molecular mechanisms responsible for response to DLI in CML.
3.2 Dosing and interval of DLI
The initial paper reporting success of DLI administered total nucleated cells at a dose of approximately 4x108 cells per kilogram (kg) of body weight, and while this strategy was successful in achieving remission, some patients developed GVHD (5). Subsequent studies sought to determine the optimal dosing for preservation of GVL while minimizing GVHD. One such study published in 1995 evaluated 22 patients with relapsed CML after HSCT who received DLI at 8 dose levels between 1 x 105 and 5 x 108 CD3+ T cells/kg in 4 to 33 week intervals (61). Eight patients achieved remission at doses as low as 1 x 107 CD3+ cells/kg, while 11 additional patients required escalating doses between 5 x 107 CD3+ cells/kg to 5 x 108 CD3+ cells/kg. This group concluded that doses of 1 x 107 cells/kg were a reasonable starting point, with the option of escalating doses with subsequent infusions to achieve remission. A later case report demonstrated achievement of remission in a patient with extensive GVHD with DLI doses of 1 x 106 and 5 x 106 CD3+ cells/kg (62). A larger study of 40 patients with relapsed CML treated with one of three different doses of CD4+ (i.e. CD8+ depleted) DLI: 0.3, 1.0, or 1.5 x 108 cells/kg (63). Patients who failed to achieve remission at the first dose level could receive escalating doses in an attempt to achieve response. All four patients at the highest dose level achieved response, compared to 10 of 14 patients at the lowest dose level; however, the higher dose level had double the incidence of GVHD compared to the lower dose. Subsequent studies have confirmed these findings and suggested that DLI doses of 1 x 107 cells/kg are sufficient to induce response with an acceptable rate of GVHD, while higher doses do not meaningfully increase response rates but do drastically increase the risk of toxicity (64).In the current era, DLI is most commonly given at a starting dose of 1 x 107 cells/kg with an interval of approximately four weeks between infusions to allow for development of GVHD, per EMBT guidelines (65). Up to three or four total infusions may be administered. For prophylactic or pre-emptive indications, lower starting doses, such as 1 x 105 or 1 x 106 cells/kg may be considered.
3.3 Timing of DLI: prophylactic vs. preemptive vs. therapeutic
While DLI was initially developed as a therapy for relapsed disease (46), multiple studies have demonstrated across different diseases that DLI is most successful in reinstating remission in patients with lower disease burden (51, 66, 67). To improve likelihood of response, differences in timing of DLI have been explored.
3.3.1 - Prophylactic DLI
Since relapse remains the leading cause of mortality (68), strategies for reducing relapse are paramount for improving overall outcomes for patients undergoing HSCT. Thus, administering DLI prophylactically, particularly for patients at higher than average risk of relapse and thereby capitalizing upon the additional GVL effects of adoptive cellular therapy presents an attractive option. Patients for whom prophylactic DLI is administered are typically without any signs of impending or molecular relapse (e.g. full donor chimerism, no decreasing blood cell counts or return of disease-defining mutations) and are typically treated within the first six months following HSCT. A registry study comparing matched pairs of patients who either did or did not receive prophylactic DLI showed that there was no difference in relapse rate across the entire cohort, patients with high-risk AML (i.e. unfavorable cytogenetic risk category or transplant in second complete remission or beyond) had improved overall survival if they received prophylactic DLI (Table 1) (69). Another retrospective analysis demonstrated a similar improvement in relapse rate for recipients of prophylactic DLI (22%) compared to the control group (53%) (70). Unsurprisingly, the major potential adverse event after DLI is development of GVHD (71, 74), which has been reported as being higher in prophylactic DLI compared to preemptive or therapeutic DLI (71). Several studies have also leveraged G-CSF priming of prophylactic DLI products, which may enhance GVL activity while mitigating GVHD through induction of immunomodulatory effects by G-CSF (72, 73, 75).
3.3.2 - Pre-emptive DLI
Hematologic or morphologic relapse of leukemia, defined as reappearance of greater than or equal to 5% bone marrow or peripheral blood blasts, can often be preceded by molecular relapse (i.e. recurrence of cytogenetic or molecular abnormalities associated with underlying disease) or other indications of impending relapse, including decreasing donor chimerism (76). Furthermore, presence of both mixed chimerism and measurable residual disease (MRD) after HSCT are both well-established predictors of later relapse, particularly for recipients of reduced intensity conditioning (RIC) HSCT (77–81). Therefore, administration of DLI at the first sign of impending or molecular relapse, termed preemptive DLI, has been evaluated as a therapy for leveraging GVL and re-establishing remission. Overall, preemptive DLI has a higher success rate compared to DLI for overt disease relapse, particularly for AML, with some studies reporting as high as 70% and five-year overall survival approaching 70% for AML (Table 2) (82–85). Advances in quantification of MRD before and after HSCT provide an opportunity to leverage GVL effects of preemptive DLI in this subset of patients at higher risk of relapse (91, 92). One early example of this was a study that utilized measurement of the Wilms tumor 1 gene (WT1), a commonly expressed AML-associated antigen, as a readout of MRD positivity post-HSCT (86). Patients who were MRD-positive for WT1 received either preemptive DLI or low dose IL-2, with those receiving preemptive DLI having improved overall survival and lower incidence of relapse compared to the IL-2 group (86). A more recent study found a similar benefit of preemptive DLI on longer term clinical outcomes but also noted positive outcomes among patients receiving low dose IL-2 rather than DLI, suggesting this as a viable alternative for patients in whom DLI may be contraindicated (e.g. presence of GVHD) or infeasible (e.g. donor availability) (93). Aside from MRD positivity, the main indication for preemptive DLI is low or decreasing donor chimerism post-HSCT, which is a well-established predictive marker of later relapse, particularly for recipients of RIC regimens (77). Multiple studies have established that preemptive DLI is an effective strategy for improving donor chimerism post-HSCT, and that attaining full donor chimerism is linked to improved longer term overall survival and reduced relapse (87–90, 94).
3.3.3 - Therapeutic DLI
Therapeutic DLI, given for morphologic/hematologic relapse, is the most studied and commonly employed strategy. However, in sharp contrast to preemptive DLI, the success rates of therapeutic DLI for most diseases is quite poor, with the exception of CML (46). A nationwide Japanese study found that while patients given preemptive DLI for cytogenetic/molecular relapse (mostly AML) had a response rate of 57%, patients treated with therapeutic DLI after hematologic/morphologic relapse only had a 20% response rate, likely related to higher tumor burden and/or higher risk cytogenetic/mutational profiles, which is in line with response rates reported by other groups for DLI given in the setting of hematologic relapse (Table 3) (95, 98). Further, development of GVHD after DLI is linked to response, but in at least one study did not impact overall survival (63, 99). Some small studies, particularly in the CML setting have shown efficacy of a lower CD3+ starting dose for initial DLI infusion, which in some patients is sufficient to induce remission, later followed by escalating DLI doses, in an attempt to mitigate development of GVHD (96, 97, 100–102). The majority of this review will focus on applications of therapeutic DLI for relapsed disease.
3.3.4 - DLI versus second HSCT
For patients with overt relapse of disease after first HSCT, there is no established therapy with superior response rates, though options include additional therapy (chemotherapy, hypomethylating agents), DLI, second HSCT, or therapy followed by DLI or second HSCT. There is clinical equipoise surrounding the question of whether DLI or second HSCT is the superior strategy. A large EBMT registry study of 418 adults with relapsed AML assessed outcomes after DLI compared to second HSCT. No difference in overall survival was observed between the two treatment groups; rather, outcomes were better, irrespective of cellular therapy approach, for patients who relapsed >6 months after first HSCT compared to those who relapsed early (<6 months) after HSCT (103). Another smaller retrospective study analyzed outcomes for 89 patients with relapse or graft failure who received either DLI or second HSCT and found a trend toward improved overall survival for recipients of second HSCT. Only selected patients may be candidates for either strategy. In general, patients must have no or minimal GVHD present for consideration of DLI or second HSCT. Additionally, older and less fit patients may be recommended for DLI rather than HSCT if they are not deemed to be sufficiently fit to tolerate conditioning therapy ahead of a second HSCT. Thus, rigorous comparison of these two interventions is difficult, and the decision of which strategy to employ remains a highly individualized process, dependent upon the specific patient, disease, and toxicity characteristics in each case (104).
3.4 Manipulations to DLI
3.4.1 - CD8 depletion
Various strategies have been employed to optimize GVL response of DLI and/or minimize toxicities of associated GVHD through manipulation of the DLI product (Table 4). One early strategy hypothesized that, since some evidence implicated CD8+ T cells in the pathogenesis of GVHD (131), perhaps infusion of CD4+ T cells alone would result in effective GVL without GVHD (63). Patients with relapsed CML, MM, or other diseases were treated with defined doses of CD8-depleted CD4+ T cells with clinical responses in ~80% of patients and GVHD developing in about one-third of patients [compared to a ~75% GVHD incidence with conventional DLI (46)]. With this encouraging result, several additional studies evaluated CD8-depletion as a strategy for preserving GVL while reducing GVHD for not only DLI for disease relapse (30, 46, 105), but also as a prophylactic strategy for patients receiving RIC HSCT (132) or those with mixed chimerism (MC) or persistent disease immediately following HSCT (106). However, this latter study did still find a high incidence of grade II-IV acute GVHD (5 of 22 patients, 23%), suggesting that CD8 T cells are not the sole drivers of GVHD. Indeed, subsequent work has drawn a clear link between CD4+ T cell activity and GVHD development. These studies all demonstrated encouraging data for GVHD incidence with preserved GVL activity, including sustained remission for the majority of patients (133). Studies in mice have demonstrated that naive CD4+ T cells alone are capable of inducing GVHD, while mice receiving memory CD4+ T cells are protected from GVHD (134–137). Several murine studies have established the ability of CD4+ T cells to promote GVHD, for example through major histocompatibiltiy complex (MHC) II presentation of intestinal microbiome antigens or other non-hematopoietic recipient antigens to promote GVHD (138–142).
3.4.2 - Naive T cell depletion
Several groups have posited that GVHD pathogenesis is driven largely by alloreactive naive T cells and hypothesized that selective depletion of CD45RA+ naive T cells may reduce GVHD while retaining GVL, a strategy that has been applied to HSCT with encouraging results (107, 143–145). To this end, several groups have piloted methods for efficiently depleting CD45RA+ cells from HSCT followed by prophylactic DLI with T cells depleted of the naive compartment for reduction in GVHD with the aim to preserve GVL (108–111). These relatively small studies have indicated safety and efficacy of this approach. One study of 15 patients has applied this approach for therapeutic DLI for treatment of relapsed disease with donor-derived CD8+ memory T cells, showing safety and low incidence of GVHD (112).
3.4.3 - Treg depletion
There is considerable evidence in both mice and humans that prevalence of CD4+CD25+ regulatory T cells (Tregs) is associated with protection from GVHD, and these cells may play an important role in mitigating GVHD pathogenesis through control of effector T cell alloreactivity (146–151). It has been hypothesized that Tregs may also dampen GVL through a similar mechanism (152). This idea received some support from the finding that patients who achieved durable remission after DLI for treatment of relapsed disease had lower proportion of Tregs in the DLI product compared to patients who did not respond (153). Two independent groups subsequently sought to explore this concept further in clinical trials testing the safety and efficacy of DLI depleted of Tregs (113, 114) Maury et al. studied 17 patients with relapsed disease who had failed to respond conventional DLI. Two patients experienced disease response to a single infusion of Treg-depleted DLI, while an additional four patients responded after a second infusion of DLI. All responders experienced GVHD (114). Longer term follow up demonstrated that three of the six responders remained in remission at 5 years following Treg-depleted DLI, while three patients who initially had a response ultimately relapsed with the original malignancy and succumbed to disease within three years of treatment (154). The Dana-Farber group conducted a phase I trial of 21 patients treated with CD25+ Treg-depleted DLI at two different dose levels, of whom four were in complete remission at the time of DLI after treatment with cytoreductive therapy for relapse (113). At the lower dose (1x107 CD3+ cells/kg), one of six patients responded, while at the higher dose (3x107 CD3+ cells/kg), 60% experienced complete or partial response, with a one year overall survival rate in that cohort of 53%. Seven patients developed GVHD, one of whom died as a result of this complication. Comparison to a contemporaneous cohort of conventional DLI therapy suggested improved response rates with Treg depletion (113).
3.4.4 - Alloanergization
Alloanergization is an approach to prevent GVHD by tolerizing alloreactive donor T cells to the recipient through chronic antigen stimulation with concurrent co-stimulatory blockade, thus leading to T cell anergy and hyporesponsiveness to alloantigens (155). Initially developed as a strategy to expand the pool of potential donors beyond full HLA-match, alloanergization has been shown to be an effective method of administering CD34+-selected haploidentical HSCT, followed by DLI, with in vitro studies suggesting intact GVL (115, 156, 157).
3.4.5 - Activation of donor cells
Early experiments in mouse models of leukemia and HSCT suggested that activation of alloreactive donor T cells could prevent or treat relapse through enhanced GVL (158). These observations prompted the hypothesis that promoting T cell activation with cytokines such as IL-2 ex vivo or in vivo prior to DLI could improve outcomes (159, 160). A case report of a patient with relapsed CML after HSCT lended support for this approach, when the patient achieved remission after three sequential DLI treatments, along with subcutaneous injections of recombinant IL-2 (161). A subsequent larger phase I study in patients with relapsed acute or chronic leukemia (ALL, MDS/AML, or CML) further supported this notion, wherein more than half of the patients demonstrated response to combination therapy (116). Interestingly, in this study, six of the 10 responding patients developed GVHD, while in four patients GVL was independent of GVHD. Only one of the seven nonresponder patients developed GVHD, underscoring the close relationship between these immunologic phenomena. Another strategy for is using ex vivo cytokine treatment to activate donor T cells, thereby priming their killing efficacy (cytokine-induced killer [CIK] cells) (117). Two groups have recently tested the efficacy of CIK cells to carry out GVL activity. The first group administered a single infusion of CIK to patients who did not achieve full donor chimerism after HSCT, since these patients are at higher risk of relapse (119). CIK infusion did not appear to substantially impact donor chimerism at day +90 after HSCT (~55-70 days after CIK), and outcomes including survival and relapse rates were similar to historical cohorts. The second group tested conventional DLI compared to CIK infusion in patients with molecular or hematologic relapse after HSCT (118). In this study, all patients with hematologic relapse progressed after DLI or CIK; however, among patients with molecular relapse only, complete remissions were induced in 53% of patients treated with CIK versus 29% with conventional DLI. This promising result suggests that CIK improves the GVL activity of donor cells over conventional DLI and may be an effective therapy for molecular relapse of disease.
3.4.6 – Leukemia antigen vaccination
Other studies have questioned whether the GVL effect of DLI can be promoted by activation of alloreactive T cells in an antigen specific way through individualized vaccination strategies. While vaccination has been employed for solid tumors including melanoma and renal cell carcinoma with some degree of success (162–164), hematologic malignancies generally are characterized by lower tumor neoantigen burden, thus decreasing the likelihood of antigen-specific T cell responses (165). Nevertheless, other potential antigens including leukemia-associated antigens or minor histocompatibility antigens (mhAgs) in the post-HSCT setting may be targets (166, 167). To this end, a phase I clinical trial tested whether infusion of donor-derived dendritic cells (DCs) cultured in the presence of GM-CSF and IL-4 could enhance the GVL effect of DLI (123). The study found that this was a safe and feasible approach for modifying DLI, and that four of the patients achieved a durable remission lasting greater than 5 years. A similar vaccination strategy was tested at the time of HSCT (rather than for post-HSCT relapsed disease) wherein personalized vaccines were created for patients with AML undergoing HSCT (124). In this study, donor-derived DCs generated from PBMCs were cultured with GM-CSF and IL-4 along with AML cells derived from the patient’s original diagnosis. Patients were vaccinated serially after HSCT, which was generally well tolerated and resulted in 70% overall survival at nearly 5 years post-HSCT. Correlative analysis demonstrated rise and persistence of circulating T cells reactive to whole AML cells and leukemia-associated antigens. Despite these encouraging results, a subsequent phase II randomized study of inoculation with similarly generated leukemia-reactive DC vaccines compared to placebo showed no difference in overall survival, progression-free survival, or relapse (168). Altogether, antigen-specific activation of alloreactive donor T cells is an attractive possibility for enhancing GVL, though the optimal strategy requires further study.
3.4.7 Interferon to stimulate GVL
Treatment with interferon alpha (IFNɑ) has been investigated, either alone or in combination with DLI, as a potential method for promoting GVL activity. The basis for this hypothesis lies in the potential ability of IFNɑ to stimulate expression of cell surface molecules on leukemia cells, thereby sensitizing leukemia cells to alloimmune activity, while IFNɣ contributes to regulation of T cell differentiation and function (120, 122). IFNɑ may also aid in preventing GVHD by inhibiting CD4 proliferation (121). One early study treated 18 patients with IFNɑ prior to DLI and found that four of 14 assessable patients successfully engrafted with donor cells (169). A subsequent small comparative study assessed response to DLI alone in 3 patients versus DLI with IFNɑ in 10 patients, where 9 of 10 patients treated with DLI and IFNɑ achieved complete molecular remission whereas all of the patients treated with DLI alone experienced disease progression (170). DLI with interferon appears to be effective in CML (66), though small studies in MDS/AML have shown promise, though this strategy has not been evaluated in larger randomized clinical trials (171, 172). Interferon gamma (IFNɣ) has also been studied as a strategy to promote GVL, since T cell secretion of IFNɣ can have anti-tumor effects (173). Murine studies of IFNɣ wild-type or knockout mice have shown that IFNɣ deletion leads to augmentation of GVHD in an allogeneic transplant model, with GVL effects inversely correlated with GVHD (174). A subsequent study demonstrated the ability of IFNɣ to promote GVL in the context of interferon gamma receptor knockout leukemia cells, indicating that the anti-leukemic properties of IFNɣ did not require direct interaction with the leukemia cells themselves. Though a direct anti-leukemic function of IFN may not be required for effective GVL, IFNɣ has been shown to upregulate expression of MHC class II genes in AML and blast crisis CML cells, whereas chronic phase CML cells were sensitive to GVL effects independent of IFNɣ (175). A recent phase I clinical trial in humans have leveraged this effect by combining IFNɣ treatment with DLI, with promising safety data as well as evidence of increased MHC class II expression and improvement in T cell chimerism in four patients with relapsed MDS/AML (176, 177). Subsequent larger studies are needed to determine the clinical efficacy of this approach.
3.5 Clinical trials of DLI and combination therapy
Since patients have the greatest likelihood of responding to DLI when disease burden is lowest (51), several studies have attempted combination or sequential cytoreductive or targeted therapies with DLI to improve response rates. One prospective study evaluated 65 patients with relapsed myeloid malignancy who were treated with cytarabine-based chemotherapy followed by DLI (178). Approximately half of the 57 evaluable patients experienced response to therapy; however, treatment related mortality was high (23%), and 2-year overall survival was 19%. Among patients who experienced complete response after combination therapy, 2 year overall survival was 41%, indicating that achieving a response with chemoimmunotherapy is capable of leading to durable remissions.
More recently, several groups have evaluated the efficacy of hypomethylating agents, often azacitidine (Aza), in combination with DLI for treatment of relapse after HSCT (Table 5). A 2014 study examined outcomes of 31 patients with relapsed myeloid malignancy after HSCT treated with Aza salvage therapy, of whom 11 received at least one DLI in addition (125). Response rate was 35% (14% complete response, 21% stable disease), but addition of DLI did not appear to influence likelihood of response. This study supported the idea of Aza as effective salvage therapy for relapsed myeloid malignancy, leading for subsequent larger studies, though the additive benefit of DLI remained unclear. A 2015 retrospective study from the German Cooperative Transplant Study Group evaluated outcomes of 154 patients with post-HSCT relapsed myeloid disease (AML, myelodysplastic syndrome [MDS], or myeloproliferative neoplasm [MPN]) of whom 105 received Aza followed by DLI while 49 received Aza alone. Thirty-three percent of patients had a response (27% with complete response, 6% with partial response), and patients with molecular-only relapse or MDS were more likely to be responders (126). Based on data from mouse studies demonstrating that azacitidine administration preserved GVL activity while preventing GVHD through immunomodulation of T cells (179, 180), a small phase I dose escalation study tested the safety and efficacy of DLI followed by Aza in the first 10 days, based on studies in mice that this strategy increased Treg numbers to provide protection from GVHD without loss of GVL (127). Six of the eight patients responded to treatment with no development of grade III-IV acute GVHD. A more recent study retrospectively analyzed 77 patients with high risk for relapse (54 with AML, 23 with MDS; high risk defined by genetic mutations or disease status at HSCT [>2nd complete remission or refractory disease]) who were treated with prophylactic low-dose Aza (up to 12 cycles if tolerated) followed by up to 3 doses of DLI after HSCT (129). Seventy-nine percent of patients ultimately received combination therapy, and overall survival at 2 years was 70%. Historial outcomes for patients with similarly high-risk disease demonstrate a ~20-53% 2-year overall survival rate (181–183). Another 2021 study measured expression of the MDS/AML-associated antigen WT1 as an indicator of MRD and therefore molecular relapse in 35 patients and then treated these patients with Aza and DLI (130). More than half of patients responded (37% MRD negative complete response, 20% MRD positive complete response, and overall survival at 2 years was 35%, supporting Aza and DLI combination therapy for treatment of early relapse. More recently, Aza and DLI, particularly for pre-emptive treatment of relapse, have received further experimental support. A multi-center retrospective analysis of 71 patients with myeloid neoplasms were treated with Aza alone or Aza and DLI (128). The overall response rate was 49%, with 38% of patients achieving complete response. This study reported that addition of DLI to Aza enhanced overall survival and event free survival; however, the main reason that patients may not proceed to DLI after Aza was disease progression (17 of 31 patients receiving Aza alone), possibly confounding the interpretation of the additive benefit of DLI to Aza. Nevertheless, combination of Aza and DLI appears to be a feasible strategy for leveraging enhanced GVL for pre-emptive treatment of MDS/AML relapse and may be a reasonable salvage treatment option for patients with hematologic relapse.
There is considerable interest in utilizing other strategies to enhance the immunologic effects of GVL for hematologic diseases, particularly MDS/AML, for example with immune checkpoint blockade (ICB), yet success of these approaches has been modest thus far (184). A recent phase I study demonstrated marked response of relapsed myeloid malignancies to ICB with the CTLA-4 inhibitor ipilimumab (185). Response was associated with enhanced cytotoxic CD8 T cell infiltration into leukemic tissues, with decreased Treg activation, and expansion of effector T cells in the blood of these patients, suggesting enhanced GVL activity. Subsequent molecular analysis of responding patient samples demonstrated increased expression of T cell activation and proinflammatory cytokines (186). This raises the interesting hypothesis that ipilimumab functions to restore T cell GVL activity and perhaps combination of ipilimumab with DLI could further amplify the GVL effect of DLI. A phase I trial is currently underway to test this hypothesis (NCT03912064).
3.6 Other cell types with putative GVL activity
Several nonclassical T cell subsets have also been implicated in GVL activity of DLI. Mouse studies have demonstrated that HSCT performed with donors lacking ɣδ T cells have impaired GVL activity and worsened GVHD (187). Studies of human primary cells in vitro have demonstrated a direct cytotoxic effect of ɣδ T cells on AML cells (188). One case report has implicated persistence of a donor-derived ɣδ T cell clone with prolonged survival after DLI for T-ALL (189). Invariant NKT cells (iNKT) are a rare CD1d-restricted T cell subset with phenotypic and functional characteristics shared between NK and T cells (190). In vitro studies using both leukemia cell lines and primary human AML blasts has demonstrated direct killing of leukemia cells in a CD1d-dependent manner by iNKT cells, an intriguing finding that warrants further mechanistic investigation (191).
Though the GVL effect of DLI is thought to be predominantly driven by alloreactive donor T cells, other immune cell types may play important roles in recognizing and eradicating malignant cells. NK cells are a population of innate lymphoid cells that normally function to eliminate viruses but may also have anticancer functions (192). NK cells treated with a cocktail of cytokines have been shown to acquire a memory-like state, endowing them with the ability to proliferative and carry out cytotoxic functions (193). These cytokine-induced memory (CIML) NK cells have been shown to have reactivity and cytotoxicity against myeloid leukemia cells in both mice and humans (194). An ongoing phase I trial aims to test infusion of CIML NK cells for treatment of relapsed myeloid disease after HSCT (NCT04024761). Preliminary correlative analysis of patient samples from this trial indicate that CIML NK cells rapidly expand and persist for months after infusion (195). Further study is needed to confirm the safety and efficacy of CIML NK adoptive cellular immunotherapy.
B cells have also been linked to GVL responses after DLI. One study identified the presence of antibodies that recognized CML-associated epitopes in the sera of patients who responded to DLI, and antibody titers were temporally associated with response (196, 197). A subsequent study of patients with CLL who received DLI for relapsed disease post-HSCT identified high titers of antibodies directed against CLL-associated antigens (198). Antibodies directed to Y chromosome antigens (H-Y antigens) in the setting of sex-mismatched HSCT have previously been implicated in both GVHD and GVL (199, 200). One study identified a coordinated B- and T-cell response directed toward the DBY antigen on the Y chromosome in a male HSCT recipient from a female donor (201). While the exact role of B cells in promoting the GVL effect remains unknown, these findings support the potential for B cell mediated cellular therapies or antibody therapy to enhance GVL.
4 Section 4 - mechanisms of response and relapse
4.1 - Immune escape leading to relapse
Relapse remains the leading cause of morbidity and mortality for patients post-HSCT, particularly for AML. In recent years, mechanisms of relapse, and in particular, mechanisms of immune escape from donor immune cell-mediated GvL are increasingly being recognized. A 2009 study of relapse after haploidentical HSCT identified loss of the mismatched HLA locus in leukemic cells in 5 patients after relapse because of uniparental disomy of chromosome 6p (202). This group demonstrated that donor-derived T cells were unable to recognize the relapsed mutant leukemia cells lacking the mismatched HLA haplotype, though donor T cells maintained the ability to recognize and mediate cytotoxicity toward leukemia cells from the original diagnosis that retained the mismatched HLA. This and other studies established HLA loss and uniparental disomy as an important mechanism of immune escape leading to leukemia relapse and resistance to maintenance of GvL (202, 203). In the HLA-matched setting, recurrent HLA loss and downregulation of MHC class II genes have also been identified as a mechanism of leukemic escape and relapse, likely due to loss of leukemia-associated antigens and/or mHAgs (204, 205). Subsequent studies have identified immunologic signatures associated with leukemia escape and relapse, including deregulation of co-stimulatory ligands (e.g. CD80, PD-L1), loss of response to GVL (IFNɣ) and tumor necrosis factor-ɑ (TNF-ɑ), and IL-2/STAT5 signaling (202, 206, 207). Strategies for employing DLI or other immune mediated GvL strategies will need to account for HLA-loss and the likelihood of effective GvL for each treatment strategy in light of these immune escape mechanisms sometimes employed by leukemic cells.
4.2 - GVL mechanisms of DLI
Relapse of hematologic malignancy after HSCT may be at least in part attributable to development of T cell dysfunction due to exhaustion and therefore loss of the protective GVL effect endowed by HSCT. T cell exhaustion is well documented in viral infections and various cancer settings and is hypothesized to arise at least in part due to chronic antigen stimulation, though the pathways leading to exhaustion, particularly in the cancer setting, remain incompletely defined (208). ICB is thought to be effective as cancer therapy due to the ability to reverse T cell exhaustion, perhaps in specific “progenitor-exhausted” subsets, defined by expression of TCF1 (209, 210). More recently, single cell analysis of patients with post-HSCT relapsed CML treated with DLI demonstrate expansion of progenitor exhausted T cells among responding patients after DLI (211). Correlative studies of bone marrow samples from patients who received DLI for relapsed CML have identified enhanced infiltration of CD8 T cells and reversal of exhaustion in patients with effective GVL after DLI, in a mechanism similar to that seen in other tumor settings with ICB (212). Prior studies of patients with relapsed myeloid malignancy treated on a clinical trial with ipilimumab demonstrated similar CD8 T cell infiltration and exhaustion reversal in disease biopsies (185, 186). It has been hypothesized that CTLA-4 inhibition with ipilimumab may act on CD4 T cells, dendritic cells, or even perhaps AML leukemia cells themselves to contribute to reversal of exhaustion in CD8 effector T cells (185, 186, 213). T cell exhaustion reversal has also been implicated in AML responders to DLI (214). These data support a central role for reversal of exhaustion in the leukemic microenvironment as necessary for effective GVL after DLI. Further work is needed to clarify the mechanisms of T cell exhaustion reversal associated with effective GVL to better leverage this for improved future therapies (Figure 1).
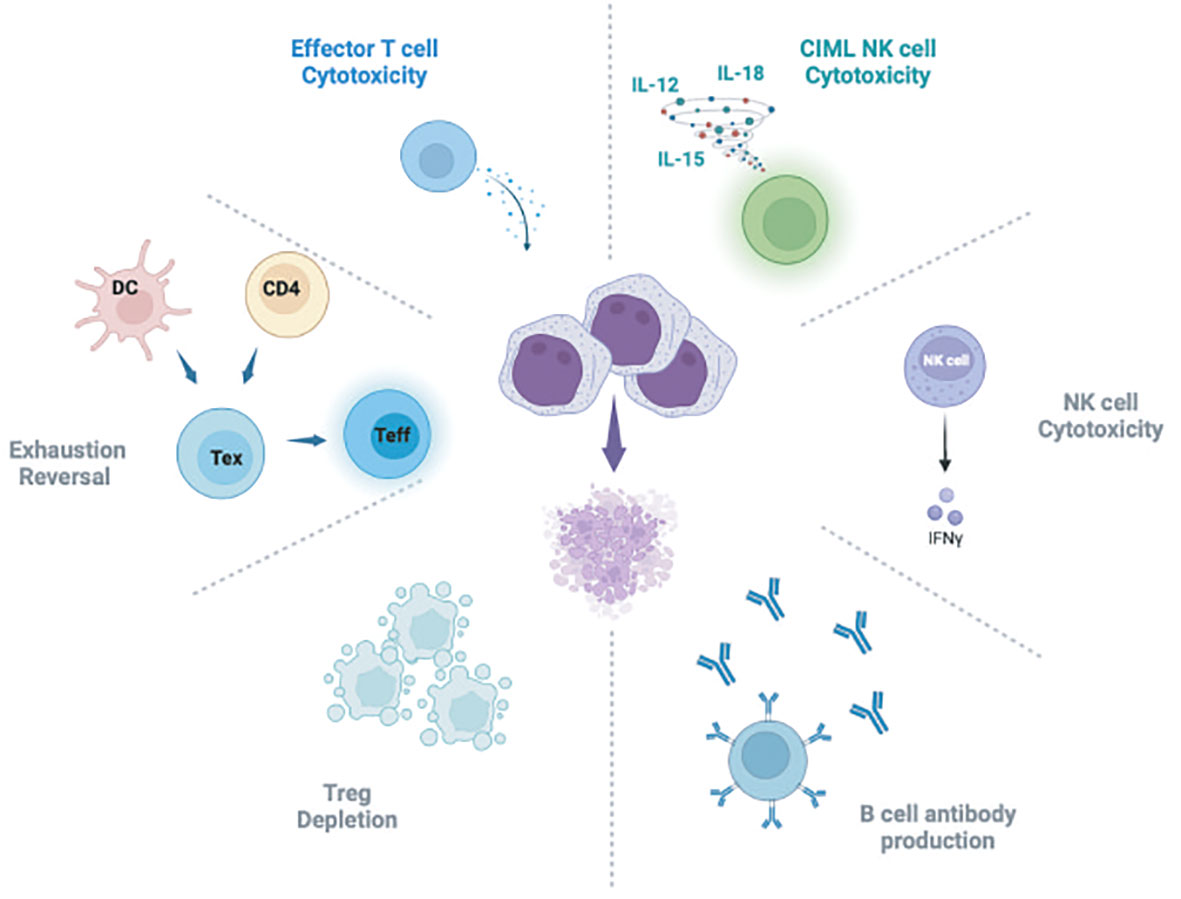
Figure 1 Schematic of hypothesized mechanisms of GVL activity attributed to DLI. DC, Dendritic cell; Tex, T exhausted cells; Teff, T effector cells; CIML NK, Cytokine induced memory-like NK cells. Figure generated using BioRender software.
As discussed above, many studies implicate immune responses to mHAgs in the GVL immune response (166, 215, 216). Several specific alloantigens have been identified that are capable of eliciting either CD4 or CD8 GVL immune responses (217–220). One correlative study of effector T cells derived from CML patients experiencing effective GVL after DLI demonstrated enhanced interferon gamma production from CD8 effector T cells, including cytotoxic T cells specific to patient-derived mhAgs (221). Though the precise antigens and mechanisms of GVL activity remain incompletely defined, some potential activation ligands have been identified. For example, stimulation of toll-like receptors (TLR) has been shown to be associated with GVL response after DLI (222, 223). In another murine model of HSCT, production of interleukin 12 (IL-12) by plasmacytoid dendritic cells (pDCs) was found to be associated with more effective GVL compared to mice receiving unmanipulated marrow enriched for conventional myeloid dendritic cells (224). The precise cellular and molecular mechanisms underlying effective GVL are likely complex and multifactorial. While major advances in molecular understanding have been made with the advent of more sensitive analytic modalities, additional research is needed to better define the key players and strategies for optimizing the GVL activity of DLI.
5 Section 5: concluding remarks
The GVL effect associated with DLI for relapsed hematologic disease after HSCT is a clinically well-established phenomenon; yet, response rates for most diseases remain low. Future studies are critically necessary for determining optimal timing of DLI as well as strategies for enhancing GVL activity. While newer combination therapies with hypomethylating agents such as Aza or immune checkpoint inhibitors like ipilimumab have shown promise, further experimental evidence is needed to improve response rates and prevent or treat relapsed disease. Further, an improved molecular understanding of the precise cellular subsets and mechanisms underlying effective GVL as well as mechanisms of resistance to therapy will support development of rationally designed and more targeted therapies to sharpen the GVL effect of DLI while mitigating toxicities.
Author contributions
KM: Conceptualization, Writing – original draft, Writing – review & editing. JA: Conceptualization, Writing – original draft, Writing – review & editing.
Funding
The author(s) declare that financial support was received for the research, authorship, and/or publication of this article. The authors acknowledge the National Cancer Institute, National Institutes of Health (P01CA229092) and the Jock and Bunny Adams Research and Education Endowment for support for this work. KM is supported by the Richard K. Lubin Family Foundation Fellowship.
Conflict of interest
The authors declare that the research was conducted in the absence of any commercial or financial relationships that could be construed as a potential conflict of interest.
Publisher’s note
All claims expressed in this article are solely those of the authors and do not necessarily represent those of their affiliated organizations, or those of the publisher, the editors and the reviewers. Any product that may be evaluated in this article, or claim that may be made by its manufacturer, is not guaranteed or endorsed by the publisher.
References
1. Cole WH, Everson TC. Spontaneous regression of cancer: preliminary report. Ann Surg. (1956) 144(3):366–83. doi: 10.1097/00000658-195609000-00007
2. Foon KA, Smalley RV, Riggs CW, Gale RP. The role of immunotherapy in acute myelogenous leukemia. Arch Intern Med. (1983) 143:1726–31. doi: 10.1001/archinte.143.9.1726
3. Horowitz MM, Gale RP, Sondel PM, Goldman JM, Kersey J, Kolb HJ, et al. Graft-versus-leukemia reactions after bone marrow transplantation. Blood. (1990) 75:555–62. doi: 10.1182/blood.V75.3.555.bloodjournal753555
4. Kekre N, Kim HT, Thanarajasingam G, Armand P, Antin JH, Cutler C, et al. Efficacy of immune suppression tapering in treating relapse after reduced intensity allogeneic stem cell transplantation. Haematologica. (2015) 100:1222–7. doi: 10.3324/haematol.2015.129650
5. Kolb HJ, Mittermüller J, Clemm C, Holler E, Ledderose G, Brehm G, et al. Donor leukocyte transfusions for treatment of recurrent chronic myelogenous leukemia in marrow transplant patients. Blood. (1990) 76:2462–5. doi: 10.1182/blood.V76.12.2462.2462
6. Thomas ED, Lochte HL Jr, Lu WC, Ferrebee JW. Intravenous infusion of bone marrow in patients receiving radiation and chemotherapy. N Engl J Med. (1957) 257:491–6. doi: 10.1056/NEJM195709122571102
7. Barnes DW, Corp MJ, Loutit JF, Neal FE. Treatment of murine leukaemia with X rays and homologous bone marrow; preliminary communication. Br Med J. (1956) 2:626–7. doi: 10.1136/bmj.2.4993.626
8. Weiden PL, Flournoy N, Thomas ED, Prentice R, Fefer A, Buckner CD, et al. Antileukemic effect of graft-versus-host disease in human recipients of allogeneic-marrow grafts. N Engl J Med. (1979) 300:1068–73. doi: 10.1056/NEJM197905103001902
9. Weiden PL, Sullivan KM, Flournoy N, Storb R, Thomas ED, Seattle Marrow Transplant Team. Antileukemic effect of chronic graft-versus-host disease: contribution to improved survival after allogeneic marrow transplantation. N Engl J Med. (1981) 304:1529–33. doi: 10.1056/NEJM198106183042507
10. Zeiser R. Advances in understanding the pathogenesis of graft-versus-host disease. Br J Haematol. (2019) 187:563–72. doi: 10.1111/bjh.16190
11. Zeiser R. Introduction to a review series on pathophysiology and treatment of acute GVHD. Blood. (2020) 136:375–6. doi: 10.1182/blood.2019004271
12. Filipovich AH, Weisdorf D, Pavletic S, Socie G, Wingard JR, Lee SJ, et al. National Institutes of Health consensus development project on criteria for clinical trials in chronic graft-versus-host disease: I Diagnosis and staging working group report. Biol Blood Marrow Transplant. (2005) 11:945–56. doi: 10.1016/j.bbmt.2005.09.004
13. Buxbaum NP, Socié G, Hill GR, MacDonald KPA, Tkachev V, Teshima T, et al. Chronic GvHD NIH Consensus Project Biology Task Force: evolving path to personalized treatment of chronic GvHD. Blood Adv. (2023) 7:4886–902. doi: 10.1182/bloodadvances.2022007611
14. Socié G, Kean LS, Zeiser R, Blazar BR. Insights from integrating clinical and preclinical studies advance understanding of graft-versus-host disease. J Clin Invest. (2021) 131(12):e149296. doi: 10.1172/JCI149296
15. Blazar BR, Hill GR, Murphy WJ. Dissecting the biology of allogeneic HSCT to enhance the GvT effect whilst minimizing GvHD. Nat Rev Clin Oncol. (2020) 17:475–92. doi: 10.1038/s41571-020-0356-4
16. Thangavelu G, Blazar BR. Achievement of tolerance induction to prevent acute graft-vs.-host disease. Front Immunol. (2019) 10:309. doi: 10.3389/fimmu.2019.00309
17. Jaglowski SM, Blazar BR. How ibrutinib, a B-cell Malignancy drug, became an FDA-approved second-line therapy for steroid-resistant chronic GVHD. Blood Adv. (2018) 2:2012–9. doi: 10.1182/bloodadvances.2018013060
18. Zeiser R, Socié G, Blazar BR. Pathogenesis of acute graft-versus-host disease: from intestinal microbiota alterations to donor T cell activation. Br J Haematol. (2016) 175:191–207. doi: 10.1111/bjh.14295
19. Blazar BR, Murphy WJ, Abedi M. Advances in graft-versus-host disease biology and therapy. Nat Rev Immunol. (2012) 12:443–58. doi: 10.1038/nri3212
20. Wu S-R, Reddy P. Tissue tolerance: a distinct concept to control acute GVHD severity. Blood. (2017) 129:1747–52. doi: 10.1182/blood-2016-09-740431
21. Teshima T, Reddy P, Zeiser R. Acute graft-versus-host disease: novel biological insights. Biol Blood Marrow Transplant. (2016) 22:11–6. doi: 10.1016/j.bbmt.2015.10.001
22. Farhan S, Holtan SG. Graft-versus-host disease: teaching old drugs new tricks at less cost. Front Immunol. (2023) 14:1225748. doi: 10.3389/fimmu.2023.1225748
23. Lohmeyer JK, Hirai T, Turkoz M, Buhler S, Lopes Ramos T, Köhler N, et al. Analysis of the T-cell repertoire and transcriptome identifies mechanisms of regulatory T-cell suppression of GVHD. Blood. (2023) 141:1755–67. doi: 10.1182/blood.2022017982
24. Sacirbegovic F, Günther M, Greco A, Zhao D, Wang X, Zhou M, et al. Graft-versus-host disease is locally maintained in target tissues by resident progenitor-like T cells. Immunity. (2023) 56:369–385.e6. doi: 10.1016/j.immuni.2023.01.003
25. Furlan SN, Watkins B, Tkachev V, Cooley S, Panoskaltsis-Mortari A, Betz K, et al. Systems analysis uncovers inflammatory Th/Tc17-driven modules during acute GVHD in monkey and human T cells. Blood. (2016) 128:2568–79. doi: 10.1182/blood-2016-07-726547
26. Soiffer RJ. Naïve T-cell depletion to prevent GVHD: searching for a better mousetrap. J Clin Oncol. (2022) 40:1139–41. doi: 10.1200/JCO.22.00105
27. Soiffer RJ, Kim HT, McGuirk J, Horwitz ME, Johnston L, Patnaik MM, et al. Prospective, randomized, double-blind, phase III clinical trial of anti-T-lymphocyte globulin to assess impact on chronic graft-versus-host disease-free survival in patients undergoing HLA-matched unrelated myeloablative hematopoietic cell transplantation. J Clin Oncol. (2017) 35:4003–11. doi: 10.1200/JCO.2017.75.8177
28. Luznik L, Pasquini MC, Logan B, Soiffer RJ, Wu J, Devine SM, et al. Randomized phase III BMT CTN trial of calcineurin inhibitor-free chronic graft-versus-host disease interventions in myeloablative hematopoietic cell transplantation for hematologic Malignancies. J Clin Oncol. (2022) 40:356–68. doi: 10.1200/JCO.21.02293
29. Antin JH. T-cell depletion in GVHD: less is more? Blood. (2011) 117:6061–2. doi: 10.1182/blood-2011-04-348409
30. Soiffer RJ, Alyea EP, Hochberg E, Wu C, Canning C, Parikh B, et al. Randomized trial of CD8+ T-cell depletion in the prevention of graft-versus-host disease associated with donor lymphocyte infusion. Biol Blood Marrow Transplant. (2002) 8:625–32. doi: 10.1053/bbmt.2002.v8.abbmt080625
31. Antin JH, Bierer BE, Smith BR, Ferrara J, Guinan EC, Sieff C, et al. Selective depletion of bone marrow T lymphocytes with anti-CD5 monoclonal antibodies: effective prophylaxis for graft-versus-host disease in patients with hematologic Malignancies. Blood. (1991) 78:2139–49. doi: 10.1182/blood.V78.8.2139.bloodjournal7882139
32. Ho VT, Soiffer RJ. The history and future of T-cell depletion as graft-versus-host disease prophylaxis for allogeneic hematopoietic stem cell transplantation. Blood. (2001) 98:3192–204. doi: 10.1182/blood.V98.12.3192
33. Mitsuyasu RT, Champlin RE, Gale RP, Ho WG, Lenarsky C, Winston D, et al. Treatment of donor bone marrow with monoclonal anti-T-cell antibody and complement for the prevention of graft-versus-host disease A prospective, randomized, double-blind trial. Ann Intern Med. (1986) 105:20–6. doi: 10.7326/0003-4819-105-1-20
34. Marmont AM, Horowitz MM, Gale RP, Sobocinski K, Ash RC, van Bekkum DW, et al. -cell depletion of HLA-identical transplants in leukemia. T Blood. (1991) 78:2120–30. doi: 10.1182/blood.V78.8.2120.bloodjournal7882120
35. Apperley JF, Mauro FR, Goldman JM, Gregory W, Arthur CK, Hows J, et al. Bone marrow transplantation for chronic myeloid leukaemia in first chronic phase: importance of a graft-versus-leukaemia effect. Br J Haematol. (1988) 69:239–45. doi: 10.1111/j.1365-2141.1988.tb07628.x
36. Goldman JM, Gale RP, Horowitz MM, Biggs JC, Champlin RE, Gluckman E, et al. Bone marrow transplantation for chronic myelogenous leukemia in chronic phase. Increased risk for relapse associated with T-cell depletion. Ann Intern Med. (1988) 108:806–14. doi: 10.7326/0003-4819-108-6-806
37. Forcade E, Chevret S, Finke J, Ehninger G, Ayuk F, Beelen D, et al. Impact of in vivo T-cell depletion in patients with myelodysplastic syndromes undergoing allogeneic hematopoietic stem cell transplant: a registry study from the Chronic Malignancies Working Party of the EBMT. Bone Marrow Transplant. (2022) 57:768–74. doi: 10.1038/s41409-022-01620-x
38. Sullivan KM, Storb R, Buckner CD, Fefer A, Fisher L, Weiden PL, et al. Graft-versus-host disease as adoptive immunotherapy in patients with advanced hematologic neoplasms. N Engl J Med. (1989) 320:828–34. doi: 10.1056/NEJM198903303201303
39. Weisdorf DJ, Nesbit ME, Ramsay NK, Woods WG, Goldman AI, Kim TH, et al. Allogeneic bone marrow transplantation for acute lymphoblastic leukemia in remission: prolonged survival associated with acute graft-versus-host disease. J Clin Oncol. (1987) 5:1348–55. doi: 10.1200/JCO.1987.5.9.1348
40. Porter DL, Roth MS, McGarigle C, Ferrara JL, Antin JH. Induction of graft-versus-host disease as immunotherapy for relapsed chronic myeloid leukemia. N Engl J Med. (1994) 330:100–6. doi: 10.1056/NEJM199401133300204
41. Kato M, Kurata M, Kanda J, Kato K, Tomizawa D, Kudo K, et al. Impact of graft-versus-host disease on relapse and survival after allogeneic stem cell transplantation for pediatric leukemia. Bone Marrow Transplant. (2019) 54:68–75. doi: 10.1038/s41409-018-0221-6
42. Negrin RS. Graft-versus-host disease versus graft-versus-leukemia. Hematol Am Soc Hematol Educ Program. (2015) 2015:225–30. doi: 10.1182/asheducation-2015.1.225
43. Drobyski WR, Roth MS, Thibodeau SN, Gottschall JL. Molecular remission occurring after donor leukocyte infusions for the treatment of relapsed chronic myelogenous leukemia after allogeneic bone marrow transplantation. Bone Marrow Transplant. (1992) 10(3):301–4.
44. Kolb HJ, Schattenberg A, Goldman JM, Hertenstein B, Jacobsen N, Arcese W, et al. Graft-versus-leukemia effect of donor lymphocyte transfusions in marrow grafted patients. Blood. (1995) 86:2041–50. doi: 10.1182/blood.V86.5.2041.bloodjournal8652041
45. Vang D. HCT presentation slides . Available online at: https://bethematchclinical.org/medical-education-and-research/hct-presentation-slides/#/ (Accessed September 26, 2023).
46. Collins RH Jr, Shpilberg O, Drobyski WR, Porter DL, Giralt S, Champlin R, et al. Donor leukocyte infusions in 140 patients with relapsed Malignancy after allogeneic bone marrow transplantation. J Clin Oncol. (1997) 15:433–44. doi: 10.1200/JCO.1997.15.2.433
47. Soiffer RJ, Alyea EP, Ritz J. Immunomodulatory effects of donor lymphocyte infusions following allogeneic bone marrow transplantation. J Clin Apher. (1995) 10:139–43. doi: 10.1002/jca.2920100308
48. Guglielmi C, Arcese W, Dazzi F, Brand R, Bunjes D, Verdonck LF, et al. Donor lymphocyte infusion for relapsed chronic myelogenous leukemia: prognostic relevance of the initial cell dose. Blood. (2002) 100:397–405. doi: 10.1182/blood.V100.2.397
49. Michallet AS, Nicolini F, Fürst S, Le QH, Dubois V, Hayette S, et al. Outcome and long-term follow-up of alloreactive donor lymphocyte infusions given for relapse after myeloablative allogeneic hematopoietic stem cell transplantations (HSCT). Bone Marrow Transplant. (2005) 35:601–8. doi: 10.1038/sj.bmt.1704807
50. Huff CA, Fuchs EJ, Smith BD, Blackford A, Garrett-Mayer E, Brodsky RA, et al. Graft-versus-host reactions and the effectiveness of donor lymphocyte infusions. Biol Blood Marrow Transplant. (2006) 12:414–21. doi: 10.1016/j.bbmt.2005.11.520
51. Schmid C, Labopin M, Nagler A, Bornhäuser M, Finke J, Fassas A, et al. Donor lymphocyte infusion in the treatment of first hematological relapse after allogeneic stem-cell transplantation in adults with acute myeloid leukemia: a retrospective risk factors analysis and comparison with other strategies by the EBMT Acute Leukemia Working Party. J Clin Oncol. (2007) 25:4938–45. doi: 10.1200/JCO.2007.11.6053
52. Dazzi F, Szydlo RM, Cross NC, Craddock C, Kaeda J, Kanfer E, et al. Durability of responses following donor lymphocyte infusions for patients who relapse after allogeneic stem cell transplantation for chronic myeloid leukemia. Blood. (2000) 96:2712–6. doi: 10.1182/blood.V96.8.2712
53. Schattenberg A, Preijers F, Mensink E, Bär B, Schaap N, Geurts van Kessel A, et al. Survival in first or second remission after lymphocyte-depleted transplantation for Philadelphia chromosome-positive CML in first chronic phase. Bone Marrow Transplant. (1997) 19:1205–12. doi: 10.1038/sj.bmt.1700824
54. Martin PJ, Clift RA, Fisher LD, Buckner CD, Hansen JA, Appelbaum FR, et al. HLA-identical marrow transplantation during accelerated-phase chronic myelogenous leukemia: analysis of survival and remission duration. Blood. (1988) 72:1978–84. doi: 10.1182/blood.V72.6.1978.bloodjournal7261978
55. Sehn LH, Alyea EP, Weller E, Canning C, Lee S, Ritz J, et al. Comparative outcomes of T-cell-depleted and non-T-cell-depleted allogeneic bone marrow transplantation for chronic myelogenous leukemia: impact of donor lymphocyte infusion. J Clin Oncol. (1999) 17:561–8. doi: 10.1200/JCO.1999.17.2.561
56. Boyiadzis M, Arora M, Klein JP, Hassebroek A, Hemmer M, Urbano-Ispizua A, et al. Impact of chronic graft-versus-host disease on late relapse and survival on 7,489 patients after myeloablative allogeneic hematopoietic cell transplantation for leukemia. Clin Cancer Res. (2015) 21:2020–8. doi: 10.1158/1078-0432.CCR-14-0586
57. Enright H, Davies SM, DeFor T, Shu X, Weisdorf D, Miller W, et al. Relapse after non-T-cell-depleted allogeneic bone marrow transplantation for chronic myelogenous leukemia: early transplantation, use of an unrelated donor, and chronic graft-versus-host disease are protective. Blood. (1996) 88:714–20. doi: 10.1182/blood.V88.2.714.bloodjournal882714
58. Weisser M, Tischer J, Schnittger S, Schoch C, Ledderose G, Kolb HJ. A comparison of donor lymphocyte infusions or imatinib mesylate for patients with chronic myelogenous leukemia who have relapsed after allogeneic stem cell transplantation. Haematologica. (2006) 91:663–6.
59. van Rhee F, Lin F, Cullis JO, Spencer A, Cross NC, Chase A, et al. Relapse of chronic myeloid leukemia after allogeneic bone marrow transplant: the case for giving donor leukocyte transfusions before the onset of hematologic relapse. Blood. (1994) 83:3377–83. doi: 10.1182/blood.V83.11.3377.bloodjournal83113377
60. Kolb HJ, Schattenberg A, Goldman JM, Hertenstein B, Jacobsen N, Arcese W, et al. Graft-versus-leukemia effect of donor lymphocyte transfusions in marrow grafted patients. European Group for Blood and Marrow Transplantation Working Party Chronic Leukemia [see comments]. Blood. (1995) 86:2041–50. doi: 10.1182/blood.V86.5.2041.bloodjournal8652041
61. Mackinnon S, Papadopoulos EB, Carabasi MH, Reich L, Collins NH, Boulad F, et al. Adoptive immunotherapy evaluating escalating doses of donor leukocytes for relapse of chronic myeloid leukemia after bone marrow transplantation: separation of graft-versus-leukemia responses from graft-versus-host disease. Blood. (1995) 86:1261–8. doi: 10.1182/blood.V86.4.1261.bloodjournal8641261
62. Rahman SL, Mahendra P, Nacheva E, Sinclair P, Arno J, Marcus RE. Achievement of complete cytogenetic remission after two very low-dose donor leucocyte infusions in a patient with extensive cGVHD relapsing in accelerated phase post allogeneic BMT for CML. Bone Marrow Transplant. (1998) 21:955–6. doi: 10.1038/sj.bmt.1701195
63. Alyea EP, Soiffer RJ, Canning C, Neuberg D, Schlossman R, Pickett C, et al. Toxicity and efficacy of defined doses of CD4+ donor lymphocytes for treatment of relapse after allogeneic bone marrow transplant. Blood. (1998) 91:3671–80. doi: 10.1182/blood.V91.10.3671.3671_3671_3680
64. Bar M, Sandmaier BM, Inamoto Y, Bruno B, Hari P, Chauncey T, et al. Donor lymphocyte infusion for relapsed hematological Malignancies after allogeneic hematopoietic cell transplantation: prognostic relevance of the initial CD3+ T cell dose. Biol Blood Marrow Transplant. (2013) 19:949–57. doi: 10.1016/j.bbmt.2013.03.001
65. Carreras E, Dufour C, Mohty M, Kröger N. The EBMT handbook: hematopoietic stem cell transplantation and cellular therapies [Internet]. 7th ed. (2019) Cham (CH): Springer.
66. Posthuma EFM, Marijt EWAF, Barge RMY, van Soest RA, Baas IO, Starrenburg CWJI, et al. Alpha-interferon with very-low-dose donor lymphocyte infusion for hematologic or cytogenetic relapse of chronic myeloid leukemia induces rapid and durable complete remissions and is associated with acceptable graft-versus-host disease. Biol Blood Marrow Transplant. (2004) 10:204–12. doi: 10.1016/j.bbmt.2003.11.003
67. Takami A, Yano S, Yokoyama H, Kuwatsuka Y, Yamaguchi T, Kanda Y, et al. Donor lymphocyte infusion for the treatment of relapsed acute myeloid leukemia after allogeneic hematopoietic stem cell transplantation: a retrospective analysis by the Adult Acute Myeloid Leukemia Working Group of the Japan Society for Hematopoietic Cell Transplantation. Biol Blood Marrow Transplant. (2014) 20:1785–90. doi: 10.1016/j.bbmt.2014.07.010
68. Styczyński J, Tridello G, Koster L, Iacobelli S, van Biezen A, van der Werf S, et al. Death after hematopoietic stem cell transplantation: changes over calendar year time, infections and associated factors. Bone Marrow Transplant. (2020) 55:126–36. doi: 10.1038/s41409-019-0624-z
69. Schmid C, Labopin M, Schaap N, Veelken H, Schleuning M, Stadler M, et al. Prophylactic donor lymphocyte infusion after allogeneic stem cell transplantation in acute leukaemia - a matched pair analysis by the Acute Leukaemia Working Party of EBMT. Br J Haematol. (2019) 184:782–7. doi: 10.1111/bjh.15691
70. Jedlickova Z, Schmid C, Koenecke C, Hertenstein B, Baurmann H, Schwerdtfeger R, et al. Long-term results of adjuvant donor lymphocyte transfusion in AML after allogeneic stem cell transplantation. Bone Marrow Transplant. (2016) 51:663–7. doi: 10.1038/bmt.2015.234
71. Santoro N, Mooyaart JE, Devillier R, Koc Y, Vydra J, Castagna L, et al. Donor lymphocyte infusions after haploidentical stem cell transplantation with PTCY: A study on behalf of the EBMT cellular therapy & immunobiology working party. Bone Marrow Transplant. (2023) 58:54–60. doi: 10.1038/s41409-022-01839-8
72. Wang Y, Liu D-H, Fan Z-P, Sun J, Wu X-J, Ma X, et al. Prevention of relapse using DLI can increase survival following HLA-identical transplantation in patients with advanced-stage acute leukemia: a multi-center study. Clin Transplant. (2012) 26:635–43. doi: 10.1111/j.1399-0012.2012.01626.x
73. Schneidawind C, Jahnke S, Schober-Melms I, Schumm M, Handgretinger R, Faul C, et al. G-CSF administration prior to donor lymphocyte apheresis promotes anti-leukaemic effects in allogeneic HCT patients. Br J Haematol. (2019) 186:60–71. doi: 10.1111/bjh.15881
74. Scarisbrick JJ, Dignan FL, Tulpule S, Gupta ED, Kolade S, Shaw B, et al. A multicentre UK study of GVHD following DLI: rates of GVHD are high but mortality from GVHD is infrequent. Bone Marrow Transplant. (2015) 50:62–7. doi: 10.1038/bmt.2014.227
75. Huang X-J, Chang YJ, Zhao XY. Maintaining hyporesponsiveness and polarization potential of T cells after in vitro mixture of G-CSF mobilized peripheral blood grafts and G-CSF primed bone marrow grafts in different proportions. Transpl Immunol. (2007) 17:193–7. doi: 10.1016/j.trim.2006.10.002
76. Cheson BD, Bennett JM, Kopecky KJ, Büchner T, Willman CL, Estey EH, et al. Revised recommendations of the international working group for diagnosis, standardization of response criteria, treatment outcomes, and reporting standards for therapeutic trials in acute myeloid leukemia. J Clin Oncol. (2003) 21:4642–9. doi: 10.1200/JCO.2003.04.036
77. Koreth J, Kim HT, Nikiforow S, Milford EL, Armand P, Cutler C, et al. Donor chimerism early after reduced-intensity conditioning hematopoietic stem cell transplantation predicts relapse and survival. Biol Blood Marrow Transplant. (2014) 20:1516–21. doi: 10.1016/j.bbmt.2014.05.025
78. Thol F, Gabdoulline R, Liebich A, Klement P, Schiller J, Kandziora C, et al. Measurable residual disease monitoring by NGS before allogeneic hematopoietic cell transplantation in AML. Blood. (2018) 132:1703–13. doi: 10.1182/blood-2018-02-829911
79. Murdock HM, Kim HT, Denlinger N, Vachhani P, Hambley B, Manning BS, et al. Impact of diagnostic genetics on remission MRD and transplantation outcomes in older patients with AML. Blood. (2022) 139:3546–57. doi: 10.1182/blood.2021014520
80. Paras G, Morsink LM, Othus M, Milano F, Sandmaier BM, Zarling LC, et al. Conditioning intensity and peritransplant flow cytometric MRD dynamics in adult AML. Blood. (2022) 139:1694–706. doi: 10.1182/blood.2021014804
81. Dillon R, Hills R, Freeman S, Potter N, Jovanovic J, Ivey A, et al. Molecular MRD status and outcome after transplantation in NPM1-mutated AML. Blood. (2020) 135:680–8. doi: 10.1182/blood.2019002959
82. Krishnamurthy P, Potter VT, Barber LD, Kulasekararaj AG, Lim ZY, Pearce RM, et al. Outcome of donor lymphocyte infusion after T cell-depleted allogeneic hematopoietic stem cell transplantation for acute myelogenous leukemia and myelodysplastic syndromes. Biol Blood Marrow Transplant. (2013) 19:562–8. doi: 10.1016/j.bbmt.2012.12.013
83. Schmid C, Labopin M, Schaap N, Veelken H, Brecht A, Stadler M, et al. Long-term results and GvHD after prophylactic and preemptive donor lymphocyte infusion after allogeneic stem cell transplantation for acute leukemia. Bone Marrow Transplant. (2022) 57:215–23. doi: 10.1038/s41409-021-01515-3
84. Tan Y, Du K, Luo Y, Shi J, Cao L, Zheng Y, et al. Superiority of preemptive donor lymphocyte infusion based on minimal residual disease in acute leukemia patients after allogeneic hematopoietic stem cell transplantation. Transfusion. (2014) 54:1493–500. doi: 10.1111/trf.12524
85. Beitinjaneh AM, Saliba R, Bashir Q, Shah N, Parmar S, Hosing C, et al. Durable responses after donor lymphocyte infusion for patients with residual multiple myeloma following non-myeloablative allogeneic stem cell transplant. Leuk Lymphoma. (2012) 53:1525–9. doi: 10.3109/10428194.2012.656635
86. Yan C-H, Liu D-H, Liu K-Y, Xu L-P, Liu Y-R, Chen H, et al. Risk stratification-directed donor lymphocyte infusion could reduce relapse of standard-risk acute leukemia patients after allogeneic hematopoietic stem cell transplantation. Blood. (2012) 119:3256–62. doi: 10.1182/blood-2011-09-380386
87. Solomon SR, Sizemore CA, Zhang X, Brown S, Holland HK, Morris LE, et al. Preemptive DLI without withdrawal of immunosuppression to promote complete donor T-cell chimerism results in favorable outcomes for high-risk older recipients of alemtuzumab-containing reduced-intensity unrelated donor allogeneic transplant: a prospective phase II trial. Bone Marrow Transplant. (2014) 49:616–21. doi: 10.1038/bmt.2014.2
88. Stadler M, Venturini L, Bünting I, Dammann E, Weissinger EM, Schwarzer A, et al. Navigating preemptive and therapeutic donor lymphocyte infusions in advanced myeloid Malignancies by high-sensitivity chimerism analysis. Front Oncol. (2022) 12:867356. doi: 10.3389/fonc.2022.867356
89. Caldemeyer LE, Akard LP, Edwards JR, Tandra A, Wagenknecht DR, Dugan MJ. Donor Lymphocyte Infusions Used to Treat Mixed-Chimeric and High-Risk Patient Populations in the Relapsed and Nonrelapsed Settings after Allogeneic Transplantation for Hematologic Malignancies Are Associated with High Five-Year Survival if Persistent Full Donor Chimerism Is Obtained or Maintained. Biol Blood Marrow Transplant. (2017) 23:1989–97. doi: 10.1016/j.bbmt.2017.07.007
90. Feliu J, Potter V, Grimaldi F, Clay J, Floro L, Saha C, et al. Full donor chimerism without graft-versus-host disease: the key factor for maximum benefit of pre-emptive donor lymphocyte infusions (pDLI). Bone Marrow Transplant. (2020) 55:562–9. doi: 10.1038/s41409-019-0695-x
91. Jentzsch M, Bischof L, Ussmann J, Backhaus D, Brauer D, Metzeler KH, et al. Prognostic impact of the AML ELN2022 risk classification in patients undergoing allogeneic stem cell transplantation. Blood Cancer J. (2022) 12:170. doi: 10.1038/s41408-022-00764-9
92. Jentzsch M, Bischof L, Backhaus D, Brauer D, Schulz J, Franke G-N, et al. Impact of MRD status in patients with AML undergoing allogeneic stem cell transplantation in the first vs the second remission. Blood Adv. (2022) 6:4570–80. doi: 10.1182/bloodadvances.2022007168
93. Yuan X-L, Tan Y-M, Shi J-M, Zhao Y-M, Yu J, Lai X-Y, et al. Preemptive low-dose interleukin-2 or DLI for late-onset minimal residual disease in acute leukemia or myelodysplastic syndrome after allogeneic hematopoietic stem cell transplantation. Ann Hematol. (2021) 100:517–27. doi: 10.1007/s00277-020-04326-6
94. Bar M, Flowers MED, Storer BE, Chauncey TR, Pulsipher MA, Thakar MS, et al. Reversal of low donor chimerism after hematopoietic cell transplantation using pentostatin and donor lymphocyte infusion: A prospective phase II multicenter trial. Biol Blood Marrow Transplant. (2018) 24:308–13. doi: 10.1016/j.bbmt.2017.10.016
95. Miyamoto T, Fukuda T, Nakashima M, Henzan T, Kusakabe S, Kobayashi N, et al. Donor lymphocyte infusion for relapsed hematological Malignancies after unrelated allogeneic bone marrow transplantation facilitated by the Japan marrow donor program. Biol Blood Marrow Transplant. (2017) 23:938–44. doi: 10.1016/j.bbmt.2017.02.012
96. Dazzi F, Szydlo RM, Craddock C, Cross NC, Kaeda J, Chase A, et al. Comparison of single-dose and escalating-dose regimens of donor lymphocyte infusion for relapse after allografting for chronic myeloid leukemia. Blood. (2000) 95:67–71. doi: 10.1182/blood.V95.1.67
97. Peggs KS, Thomson K, Hart DP, Geary J, Morris EC, Yong K, et al. Dose-escalated donor lymphocyte infusions following reduced intensity transplantation: toxicity, chimerism, and disease responses. Blood. (2004) 103:1548–56. doi: 10.1182/blood-2003-05-1513
98. Schmid C, Labopin M, Nagler A, Niederwieser D, Castagna L, Tabrizi R, et al. Treatment, risk factors, and outcome of adults with relapsed AML after reduced intensity conditioning for allogeneic stem cell transplantation. Blood. (2012) 119:1599–606. doi: 10.1182/blood-2011-08-375840
99. Porter D, Levine JE. Graft-versus-host disease and graft-versus-leukemia after donor leukocyte infusion. Semin Hematol. (2006) 43:53–61. doi: 10.1053/j.seminhematol.2005.09.005
100. El-Cheikh J, Crocchiolo R, Furst S, Ladaique P, Castagna L, Faucher C, et al. Donor CD3(+) lymphocyte infusion after reduced intensity conditioning allogeneic stem cell transplantation: single-center experience. Exp Hematol. (2013) 41:17–27. doi: 10.1016/j.exphem.2012.09.008
101. Bacigalupo A, Soracco M, Vassallo F, Abate M, Van Lint MT, Gualandi F, et al. Donor lymphocyte infusions (DLI) in patients with chronic myeloid leukemia following allogeneic bone marrow transplantation. Bone Marrow Transplant. (1997) 19:927–32. doi: 10.1038/sj.bmt.1700762
102. Ali T, Behfar M, Mohseni R, Salajegheh P, Kheder M, Abou-Fakher F, et al. Escalated dose donor lymphocyte infusion treatment in patients with primary immune deficiencies after HSCT with reduced-intensity conditioning regimen. Hematol Oncol Stem Cell Ther. (2022) 15:272–8. doi: 10.1016/j.hemonc.2021.06.002
103. Kharfan-Dabaja MA, Labopin M, Polge E, Nishihori T, Bazarbachi A, Finke J, et al. Association of second allogeneic hematopoietic cell transplant vs donor lymphocyte infusion with overall survival in patients with acute myeloid leukemia relapse. JAMA Oncol. (2018) 4:1245–53. doi: 10.1001/jamaoncol.2018.2091
104. Al-Shaibani E, Bautista R, Lipton JH, Kim DD, Viswabandya A, Kumar R, et al. Comparison of outcomes after second allogeneic hematopoietic cell transplantation versus donor lymphocyte infusion in allogeneic hematopoietic cell transplant patients. Clin Lymphoma Myeloma Leuk. (2022) 22:e327–34. doi: 10.1016/j.clml.2021.11.004
105. Alyea EP, Canning C, Neuberg D, Daley H, Houde H, Giralt S, et al. CD8+ cell depletion of donor lymphocyte infusions using cd8 monoclonal antibody-coated high-density microparticles (CD8-HDM) after allogeneic hematopoietic stem cell transplantation: a pilot study. Bone Marrow Transplant. (2004) 34:123–8. doi: 10.1038/sj.bmt.1704536
106. Orti G, Lowdell M, Fielding A, Samuel E, Pang K, Kottaridis P, et al. Phase I study of high-stringency CD8 depletion of donor leukocyte infusions after allogeneic hematopoietic stem cell transplantation. Transplantation. (2009) 88:1312–8. doi: 10.1097/TP.0b013e3181bbf382
107. Bleakley M, Sehgal A, Seropian S, Biernacki MA, Krakow EF, Dahlberg A, et al. Naive T-cell depletion to prevent chronic graft-versus-host disease. J Clin Oncol. (2022) 40:1174–85. doi: 10.1200/JCO.21.01755
108. Maschan M, Blagov S, Shelikhova L, Shekhovtsova Z, Balashov D, Starichkova J, et al. Low-dose donor memory T-cell infusion after TCR alpha/beta depleted unrelated and haploidentical transplantation: results of a pilot trial. Bone Marrow Transplant. (2018) 53:264–73. doi: 10.1038/s41409-017-0035-y
109. Dunaikina M, Zhekhovtsova Z, Shelikhova L, Glushkova S, Nikolaev R, Blagov S, et al. Safety and efficacy of the low-dose memory (CD45RA-depleted) donor lymphocyte infusion in recipients of αβ T cell-depleted haploidentical grafts: results of a prospective randomized trial in high-risk childhood leukemia. Bone Marrow Transplant. (2021) 56:1614–24. doi: 10.1038/s41409-021-01232-x
110. Maung KK, Chen BJ, Barak I, Li Z, Rizzieri DA, Gasparetto C, et al. Phase I dose escalation study of naive T-cell depleted donor lymphocyte infusion following allogeneic stem cell transplantation. Bone Marrow Transplant. (2021) 56:137–43. doi: 10.1038/s41409-020-0991-5
111. Castagna L, Valli V, Timofeeva I, Capizzuto R, Bramanti S, Mariotti J, et al. Feasibility and efficacy of CD45RA+ Depleted donor lymphocytes infusion after haploidentical transplantation with post-transplantation cyclophosphamide in patients with hematological Malignancies. Transplant Cell Ther. (2021) 27:478.e1–5. doi: 10.1016/j.jtct.2021.03.010
112. Muffly L, Sheehan K, Armstrong R, Jensen K, Tate K, Rezvani AR, et al. Infusion of donor-derived CD8 memory T cells for relapse following allogeneic hematopoietic cell transplantation. Blood Adv. (2018) 2:681–90. doi: 10.1182/bloodadvances.2017012104
113. Nikiforow S, Kim HT, Daley H, Reynolds C, Jones KT, Armand P, et al. A phase I study of CD25/regulatory T-cell-depleted donor lymphocyte infusion for relapse after allogeneic stem cell transplantation. Haematologica. (2016) 101:1251–9. doi: 10.3324/haematol.2015.141176
114. Maury S, Lemoine FM, Hicheri Y, Rosenzwajg M, Badoual C, Cheraï M, et al. CD4+CD25+ regulatory T cell depletion improves the graft-versus-tumor effect of donor lymphocytes after allogeneic hematopoietic stem cell transplantation. Sci Transl Med. (2010) 2:41ra52. doi: 10.1126/scitranslmed.3001302
115. Davies JK, Brennan LL, Wingard JR, Cogle CR, Kapoor N, Shah AJ, et al. Infusion of alloanergized donor lymphocytes after CD34-selected haploidentical myeloablative hematopoietic stem cell transplantation. Clin Cancer Res. (2018) 24:4098–109. doi: 10.1158/1078-0432.CCR-18-0449
116. Slavin S, Naparstek E, Nagler A, Ackerstein A, Samuel S, Kapelushnik J, et al. Allogeneic cell therapy with donor peripheral blood cells and recombinant human interleukin-2 to treat leukemia relapse after allogeneic bone marrow transplantation. Blood. (1996) 87:2195–204. doi: 10.1182/blood.V87.6.2195.bloodjournal8762195
117. Laport GG, Sheehan K, Baker J, Armstrong R, Wong RM, Lowsky R, et al. Adoptive immunotherapy with cytokine-induced killer cells for patients with relapsed hematologic Malignancies after allogeneic hematopoietic cell transplantation. Biol Blood Marrow Transplant. (2011) 17:1679–87. doi: 10.1016/j.bbmt.2011.05.012
118. Merker M, Salzmann-Manrique E, Katzki V, Huenecke S, Bremm M, Bakhtiar S, et al. Clearance of hematologic Malignancies by allogeneic cytokine-induced killer cell or donor lymphocyte infusions. Biol Blood Marrow Transplant. (2019) 25:1281–92. doi: 10.1016/j.bbmt.2019.03.004
119. Narayan R, Benjamin JE, Shah O, Tian L, Tate K, Armstrong R, et al. Donor-derived cytokine-induced killer cell infusion as consolidation after nonmyeloablative allogeneic transplantation for myeloid neoplasms. Biol Blood Marrow Transplant. (2019) 25:1293–303. doi: 10.1016/j.bbmt.2019.03.027
120. Upadhyaya G, Guba SC, Sih SA, Feinberg AP, Talpaz M, Kantarjian HM, et al. Interferon-alpha restores the deficient expression of the cytoadhesion molecule lymphocyte function antigen-3 by chronic myelogenous leukemia progenitor cells. J Clin Invest. (1991) 88:2131–6. doi: 10.1172/JCI115543
121. Robb RJ, Kreijveld E, Kuns RD, Wilson YA, Olver SD, Don ALJ, et al. Type I-IFNs control GVHD and GVL responses after transplantation. Blood. (2011) 118:3399–409. doi: 10.1182/blood-2010-12-325746
122. Zhao C, Zhang Y, Zheng H. The effects of interferons on allogeneic T cell response in GVHD: the multifaced biology and epigenetic regulations. Front Immunol. (2021) 12:717540. doi: 10.3389/fimmu.2021.717540
123. Ho VT, Kim HT, Kao G, Cutler C, Levine J, Rosenblatt J, et al. Sequential infusion of donor-derived dendritic cells with donor lymphocyte infusion for relapsed hematologic cancers after allogeneic hematopoietic stem cell transplantation. Am J Hematol. (2014) 89:1092–6. doi: 10.1002/ajh.23825
124. Rosenblatt J, Stone RM, Uhl L, Neuberg D, Joyce R, Levine JD, et al. Individualized vaccination of AML patients in remission is associated with induction of antileukemia immunity and prolonged remissions. Sci Transl Med. (2016) 8:368ra171. doi: 10.1126/scitranslmed.aag1298
125. Tessoulin B, Delaunay J, Chevallier P, Loirat M, Ayari S, Peterlin P, et al. Azacitidine salvage therapy for relapse of myeloid Malignancies following allogeneic hematopoietic SCT. Bone Marrow Transplant. (2014) 49:567–71. doi: 10.1038/bmt.2013.233
126. Schroeder T, Rachlis E, Bug G, Stelljes M, Klein S, Steckel NK, et al. Treatment of acute myeloid leukemia or myelodysplastic syndrome relapse after allogeneic stem cell transplantation with azacitidine and donor lymphocyte infusions–a retrospective multicenter analysis from the German Cooperative Transplant Study Group. Biol Blood Marrow Transplant. (2015) 21:653–60. doi: 10.1016/j.bbmt.2014.12.016
127. Ghobadi A, Choi J, Fiala MA, Fletcher T, Liu J, Eissenberg LG, et al. Phase I study of azacitidine following donor lymphocyte infusion for relapsed acute myeloid leukemia post allogeneic stem cell transplantation. Leuk Res. (2016) 49:1–6. doi: 10.1016/j.leukres.2016.07.010
128. Liberatore C, Stanghellini MTL, Lorentino F, Vago L, Carrabba MG, Greco R, et al. Azacitidine and donor lymphocytes infusions in acute myeloid leukemia and myelodysplastic syndrome relapsed after allogeneic hematopoietic stem cell transplantation from alternative donors. Ther Adv Hematol. (2022) 13:20406207221090882. doi: 10.1177/20406207221090882
129. Guillaume T, Thépot S, Peterlin P, Ceballos P, Bourgeois AL, Garnier A, et al. Prophylactic or preemptive low-dose azacitidine and donor lymphocyte infusion to prevent disease relapse following allogeneic transplantation in patients with high-risk acute myelogenous leukemia or myelodysplastic syndrome. Transplant Cell Ther. (2021) 27:839.e1–6. doi: 10.1016/j.jtct.2021.06.029
130. Rautenberg C, Bergmann A, Pechtel S, Fischermanns C, Haas R, Germing U, et al. Wilm’s Tumor 1-guided preemptive treatment with hypomethylating agents for molecular relapse of AML and MDS after allogeneic transplantation. Bone Marrow Transplant. (2021) 56:442–50. doi: 10.1038/s41409-020-01039-2
131. Soiffer RJ, Gonin R, Murray C, Robertson MJ, Cochran K, Chartier S, et al. Prediction of graft-versus-host disease by phenotypic analysis of early immune reconstitution after CD6-depleted allogeneic bone marrow transplantation. Blood. (1993) 82:2216–23. doi: 10.1182/blood.V82.7.2216.bloodjournal8272216
132. Meyer RG, Britten CM, Wehler D, Bender K, Hess G, Konur A, et al. Prophylactic transfer of CD8-depleted donor lymphocytes after T-cell-depleted reduced-intensity transplantation. Blood. (2007) 109:374–82. doi: 10.1182/blood-2006-03-005769
133. Shimoni A, Gajewski JA, Donato M, Martin T, O’Brien S, Talpaz M, et al. Long-Term follow-up of recipients of CD8-depleted donor lymphocyte infusions for the treatment of chronic myelogenous leukemia relapsing after allogeneic progenitor cell transplantation. Biol Blood Marrow Transplant. (2001) 7:568–75. doi: 10.1016/S1083-8791(01)70017-1
134. Anderson BE, McNiff J, Yan J, Doyle H, Mamula M, Shlomchik MJ, et al. Memory CD4+ T cells do not induce graft-versus-host disease. J Clin Invest. (2003) 112:101–8. doi: 10.1172/JCI200317601
135. Chen BJ, Cui X, Sempowski GD, Liu C, Chao NJ. Transfer of allogeneic CD62L- memory T cells without graft-versus-host disease. Blood. (2004) 103:1534–41. doi: 10.1182/blood-2003-08-2987
136. Xystrakis E, Bernard I, Dejean AS, Alsaati T, Druet P, Saoudi A. Alloreactive CD4 T lymphocytes responsible for acute and chronic graft-versus-host disease are contained within the CD45RChigh but not the CD45RClow subset. Eur J Immunol. (2004) 34:408–17. doi: 10.1002/eji.200324528
137. Zheng H, Matte-Martone C, Li H, Anderson BE, Venketesan S, Sheng Tan H, et al. Effector memory CD4+ T cells mediate graft-versus-leukemia without inducing graft-versus-host disease. Blood. (2008) 111:2476–84. doi: 10.1182/blood-2007-08-109678
138. Koyama M, Mukhopadhyay P, Schuster IS, Henden AS, Hülsdünker J, Varelias A, et al. MHC class II antigen presentation by the intestinal epithelium initiates graft-versus-host disease and is influenced by the microbiota. Immunity. (2019) 51:885–898.e7. doi: 10.1016/j.immuni.2019.08.011
139. Koyama M, Hippe DS, Srinivasan S, Proll SC, Miltiadous O, Li N, et al. Intestinal microbiota controls graft-versus-host disease independent of donor-host genetic disparity. Immunity. (2023) 56:1876–1893.e8. doi: 10.1016/j.immuni.2023.06.024
140. Jones SC, Murphy GF, Friedman TM, Korngold R. Importance of minor histocompatibility antigen expression by nonhematopoietic tissues in a CD4+ T cell-mediated graft-versus-host disease model. J Clin Invest. (2003) 112:1880–6. doi: 10.1172/JCI200319427
141. Dwyer GK, Mathews LR, Villegas JA, Lucas A, Gonzalez de Peredo A, Blazar BR, et al. IL-33 acts as a costimulatory signal to generate alloreactive Th1 cells in graft-versus-host disease. J Clin Invest. (2022) 132(12):e150927. doi: 10.1172/JCI150927
142. Yeh AC, Koyama M, Waltner O, Minnie SA, Boiko J, Shabaneh TB, et al. Microbiota-specific T cells contribute to graft-versus-host disease after allogeneic stem cell transplantation. Blood. (2023) 142:345–5. doi: 10.1182/blood-2023-178773
143. Chérel M, Choufi B, Trauet J, Cracco P, Dessaint J-P, Yakoub-Agha I, et al. Naïve subset develops the most important alloreactive response among human CD4+ T lymphocytes in human leukocyte antigen-identical related setting. Eur J Haematol. (2014) 92:491–6. doi: 10.1111/ejh.12283
144. Distler E, Bloetz A, Albrecht J, Asdufan S, Hohberger A, Frey M, et al. Alloreactive and leukemia-reactive T cells are preferentially derived from naive precursors in healthy donors: implications for immunotherapy with memory T cells. Haematologica. (2011) 96:1024–32. doi: 10.3324/haematol.2010.037481
145. Shlomchik WD. Graft-versus-host disease. Nat Rev Immunol. (2007) 7:340–52. doi: 10.1038/nri2000
146. Cohen JL, Boyer O. The role of CD4+CD25hi regulatory T cells in the physiopathogeny of graft-versus-host disease. Curr Opin Immunol. (2006) 18:580–5. doi: 10.1016/j.coi.2006.07.007
147. Zorn E, Kim HT, Lee SJ, Floyd BH, Litsa D, Arumugarajah S, et al. Reduced frequency of FOXP3+ CD4+CD25+ regulatory T cells in patients with chronic graft-versus-host disease. Blood. (2005) 106:2903–11. doi: 10.1182/blood-2005-03-1257
148. Rezvani K, Mielke S, Ahmadzadeh M, Kilical Y, Savani BN, Zeilah J, et al. High donor FOXP3-positive regulatory T-cell (Treg) content is associated with a low risk of GVHD following HLA-matched allogeneic SCT. Blood. (2006) 108:1291–7. doi: 10.1182/blood-2006-02-003996
149. Robb RJ, Lineburg KE, Kuns RD, Wilson YA, Raffelt NC, Olver SD, et al. Identification and expansion of highly suppressive CD8(+)FoxP3(+) regulatory T cells after experimental allogeneic bone marrow transplantation. Blood. (2012) 119:5898–908. doi: 10.1182/blood-2011-12-396119
150. Zheng J, Liu Y, Liu Y, Liu M, Xiang Z, Lam K-T, et al. Human CD8+ regulatory T cells inhibit GVHD and preserve general immunity in humanized mice. Sci Transl Med. (2013) 5:168ra9. doi: 10.1126/scitranslmed.3004943
151. Mielke S, Rezvani K, Savani BN, Nunes R, Yong ASM, Schindler J, et al. Reconstitution of FOXP3+ regulatory T cells (Tregs) after CD25-depleted allotransplantation in elderly patients and association with acute graft-versus-host disease. Blood. (2007) 110:1689–97. doi: 10.1182/blood-2007-03-079160
152. Zhou Q, Bucher C, Munger ME, Highfill SL, Tolar J, Munn DH, et al. Depletion of endogenous tumor-associated regulatory T cells improves the efficacy of adoptive cytotoxic T-cell immunotherapy in murine acute myeloid leukemia. Blood. (2009) 114:3793–802. doi: 10.1182/blood-2009-03-208181
153. Hicheri Y, Bouchekioua A, Hamel Y, Henry A, Rouard H, Pautas C, et al. Donor regulatory T cells identified by FoxP3 expression but also by the membranous CD4+CD127low/neg phenotype influence graft-versus-tumor effect after donor lymphocyte infusion. J Immunother. (2008) 31:806–11. doi: 10.1097/CJI.0b013e318184908d
154. Maury S, Redjoul R, Cabanne L, Vigouroux S, Legros L, Cohen JL. Regulatory T-cell depletion in donor lymphocyte infusions for haematological Malignancies: long-term outcomes from a prospective study. Br J Haematol. (2014) 166:452–5. doi: 10.1111/bjh.12856
155. Gribben JG, Guinan EC, Boussiotis VA, Ke XY, Linsley L, Sieff C, et al. Complete blockade of B7 family-mediated costimulation is necessary to induce human alloantigen-specific anergy: a method to ameliorate graft-versus-host disease and extend the donor pool. Blood. (1996) 87:4887–93. doi: 10.1182/blood.V87.11.4887.bloodjournal87114887
156. Davies JK, Yuk D, Nadler LM, Guinan EC. Induction of alloanergy in human donor T cells without loss of pathogen or tumor immunity. Transplantation. (2008) 86:854–64. doi: 10.1097/TP.0b013e3181861b6c
157. Davies JK, Barbon CM, Voskertchian AR, Nadler LM, Guinan EC. Induction of alloantigen-specific anergy in human peripheral blood mononuclear cells by alloantigen stimulation with co-stimulatory signal blockade. J Vis Exp. (2011) (49):2673. doi: 10.3791/2673
158. Weiss L, Reich S, Slavin S. Use of recombinant human interleukin-2 in conjunction with bone marrow transplantation as a model for control of minimal residual disease in Malignant hematological disorders: I Treatment of murine leukemia in conjunction with allogeneic bone marrow transplantation and IL-2-activated cell-mediated immunotherapy. Cancer Invest. (1992) 10:19–26. doi: 10.3109/07357909209032785
159. Slavin S, Ackerstein A, Weiss L, Nagler A, Or R, Naparstek E. Immunotherapy of minimal residual disease by immunocompetent lymphocytes and their activation by cytokines. Cancer Invest. (1992) 10:221–7. doi: 10.3109/07357909209032764
160. Slavin S, Ackerstein A, Kedar E, Weiss L. IL-2 activated cell-mediated immunotherapy: control of minimal residual disease in Malignant disorders by allogeneic lymphocytes and IL-2. Bone Marrow Transplant. (1990) 6 Suppl 1:86–90.
161. Kapelushnik J, Nagler A, Or R, Naparstek E, Ackerstein A, Samuel S, et al. Activated allogeneic cell therapy (allo-ACT) for relapsed chronic myelogenous leukemia (CML) refractory to buffy coat transfusions post-allogeneic bone marrow transplantation. Bone Marrow Transplant. (1996) 18:1153–6.
162. Hu Z, Leet DE, Allesøe RL, Oliveira G, Li S, Luoma AM, et al. Personal neoantigen vaccines induce persistent memory T cell responses and epitope spreading in patients with melanoma. Nat Med. (2021) 27:515–25. doi: 10.1038/s41591-020-01206-4
163. Nestle FO, Alijagic S, Gilliet M, Sun Y, Grabbe S, Dummer R, et al. Vaccination of melanoma patients with peptide- or tumor lysate-pulsed dendritic cells. Nat Med. (1998) 4:328–32. doi: 10.1038/nm0398-328
164. Thurnher M, Rieser C, Höltl L, Papesh C, Ramoner R, Bartsch G. Dendritic cell-based immunotherapy of renal cell carcinoma. Urol Int. (1998) 61:67–71. doi: 10.1159/000030291
165. Saini SK, Holmberg-Thydén S, Bjerregaard A-M, Unnikrishnan A, Dorfmüller S, Platzbecker U, et al. Neoantigen reactive T cells correlate with the low mutational burden in hematological Malignancies. Leukemia. (2022) 36:2734–8. doi: 10.1038/s41375-022-01705-y
166. Robert S, Albring JC, Frebel K, Opitz C, Urh J, Wolf C, et al. Alloantigen expression on Malignant cells and healthy host tissue influences graft-versus-tumor reactions after allogeneic hematopoietic stem cell transplantation. Bone Marrow Transplant. (2018) 53:807–19. doi: 10.1038/s41409-017-0071-7
167. Warren EH, Fujii N, Akatsuka Y, Chaney CN, Mito JK, Loeb KR, et al. Therapy of relapsed leukemia after allogeneic hematopoietic cell transplantation with T cells specific for minor histocompatibility antigens. Blood. (2010) 115:3869–78. doi: 10.1182/blood-2009-10-248997
168. Ho VT, Kim HT, Brock J, Galinsky I, Daley H, Reynolds C, et al. GM-CSF secreting leukemia cell vaccination for MDS/AML after allogeneic HSCT: a randomized, double-blinded, phase 2 trial. Blood Adv. (2022) 6:2183–94. doi: 10.1182/bloodadvances.2021006255
169. Porter DL, Connors JM, Van Deerlin VM, Duffy KM, McGarigle C, Saidman SL, et al. Graft-versus-tumor induction with donor leukocyte infusions as primary therapy for patients with Malignancies. J Clin Oncol. (1999) 17:1234. doi: 10.1200/JCO.1999.17.4.1234
170. Moravcová J, Nádvorníková S, Zmeková V, Michalová K, Brezinová J, Lukásová M, et al. Molecular monitoring of responses to DLI and DLI + IFN treatment of post-SCT relapses in patients with CML. Leuk Res. (2003) 27:719–29. doi: 10.1016/S0145-2126(02)00280-1
171. Mo X-D, Zhang X-H, Xu L-P, Wang Y, Yan C-H, Chen H, et al. Interferon-α: A potentially effective treatment for minimal residual disease in acute leukemia/myelodysplastic syndrome after allogeneic hematopoietic stem cell transplantation. Biol Blood Marrow Transplant. (2015) 21:1939–47. doi: 10.1016/j.bbmt.2015.06.014
172. Henden AS, Varelias A, Leach J, Sturgeon E, Avery J, Kelly J, et al. Pegylated interferon-2α invokes graft-versus-leukemia effects in patients relapsing after allogeneic stem cell transplantation. Blood Adv. (2019) 3:3013–9. doi: 10.1182/bloodadvances.2019000453
173. Lu Y, Waller EK. Dichotomous role of interferon-gamma in allogeneic bone marrow transplant. Biol Blood Marrow Transplant. (2009) 15:1347–53. doi: 10.1016/j.bbmt.2009.07.015
174. Yang Y-G, Qi J, Wang M-G, Sykes M. Donor-derived interferon gamma separates graft-versus-leukemia effects and graft-versus-host disease induced by donor CD8 T cells. Blood. (2002) 99:4207–15. doi: 10.1182/blood.V99.11.4207
175. Matte-Martone C, Liu J, Zhou M, Chikina M, Green DR, Harty JT, et al. Differential requirements for myeloid leukemia IFN-γ conditioning determine graft-versus-leukemia resistance and sensitivity. J Clin Invest. (2017) 127:2765–76. doi: 10.1172/JCI85736
176. Ito S, Krakow EF, Ventura K, Rodger A, Geramita E, Moore E, et al. Pilot trial of IFN-γ and donor lymphocyte infusion to treat relapsed AML and MDS after allogeneic hematopoietic stem cell transplantation. Blood. (2022) 140:7678–9. doi: 10.1182/blood-2022-157054
177. Geramita E, Ventura K, Bhise S, Moore E, Krakow EF, Hill GR, et al. Pilot Trial of Interferon-γ and Donor Lymphocyte Infusion to Treat Relapsed Myeloblastic Malignancies after Allogeneic Hematopoietic Stem Cell Transplantation, in: (2023). Available online at: https://tandem.confex.com/tandem/2023/meetingapp.cgi/Paper/21033 (Accessed January 29, 2024).
178. Levine JE, Braun T, Penza SL, Beatty P, Cornetta K, Martino R, et al. Prospective trial of chemotherapy and donor leukocyte infusions for relapse of advanced myeloid Malignancies after allogeneic stem-cell transplantation. J Clin Oncol. (2002) 20:405–12. doi: 10.1200/JCO.2002.20.2.405
179. Sánchez-Abarca LI, Gutierrez-Cosio S, Santamaría C, Caballero-Velazquez T, Blanco B, Herrero-Sánchez C, et al. Immunomodulatory effect of 5-azacytidine (5-azaC): potential role in the transplantation setting. Blood. (2010) 115:107–21. doi: 10.1182/blood-2009-03-210393
180. Choi J, Ritchey J, Prior JL, Holt M, Shannon WD, Deych E, et al. In vivo administration of hypomethylating agents mitigate graft-versus-host disease without sacrificing graft-versus-leukemia. Blood. (2010) 116:129–39. doi: 10.1182/blood-2009-12-257253
181. Fang M, Storer B, Estey E, Othus M, Zhang L, Sandmaier BM, et al. Outcome of patients with acute myeloid leukemia with monosomal karyotype who undergo hematopoietic cell transplantation. Blood. (2011) 118:1490–4. doi: 10.1182/blood-2011-02-339721
182. Middeke JM, Fang M, Cornelissen JJ, Mohr B, Appelbaum FR, Stadler M, et al. Outcome of patients with abnl(17p) acute myeloid leukemia after allogeneic hematopoietic stem cell transplantation. Blood. (2014) 123:2960–7. doi: 10.1182/blood-2013-12-544957
183. Jimenez Jimenez AM, De Lima M, Komanduri KV, Wang TP, Zhang M-J, Chen K, et al. An adapted European LeukemiaNet genetic risk stratification for acute myeloid leukemia patients undergoing allogeneic hematopoietic cell transplant. A CIBMTR analysis. Bone Marrow Transplant. (2021) 56:3068–77. doi: 10.1038/s41409-021-01450-3
184. Stahl M, Goldberg AD. Immune checkpoint inhibitors in acute myeloid leukemia: novel combinations and therapeutic targets. Curr Oncol Rep. (2019) 21:37. doi: 10.1007/s11912-019-0781-7
185. Davids MS, Kim HT, Bachireddy P, Costello C, Liguori R, Savell A, et al. Ipilimumab for patients with relapse after allogeneic transplantation. N Engl J Med. (2016) 375:143–53. doi: 10.1056/NEJMoa1601202
186. Penter L, Zhang Y, Savell A, Huang T, Cieri N, Thrash EM, et al. Molecular and cellular features of CTLA-4 blockade for relapsed myeloid Malignancies after transplantation. Blood. (2021) 137:3212–7. doi: 10.1182/blood.2021010867
187. Song Y, Zhu Y, Hu B, Liu Y, Lin D, Jin Z, et al. Donor γδT cells promote GVL effect and mitigate aGVHD in allogeneic hematopoietic stem cell transplantation. Front Immunol. (2020) 11:558143. doi: 10.3389/fimmu.2020.558143
188. Dolstra H, Fredrix H, van der Meer A, de Witte T, Figdor C, van de Wiel-van Kemenade E. TCR gamma delta cytotoxic T lymphocytes expressing the killer cell-inhibitory receptor p58.2 (CD158b) selectively lyse acute myeloid leukemia cells. Bone Marrow Transplant. (2001) 27:1087–93. doi: 10.1038/sj.bmt.1703043
189. Xu L, Weng J, Huang X, Zeng C, Chen S, Geng S, et al. Persistent donor derived Vδ4 T cell clones may improve survival for recurrent T cell acute lymphoblastic leukemia after HSCT and DLI. Oncotarget. (2016) 7:42943–52. doi: 10.18632/oncotarget.v7i28
190. Krovi SH, Gapin L. Invariant natural killer T cell subsets-more than just developmental intermediates. Front Immunol. (2018) 9:1393. doi: 10.3389/fimmu.2018.01393
191. Jahnke S, Schmid H, Secker K-A, Einhaus J, Duerr-Stoerzer S, Keppeler H, et al. Invariant NKT cells from donor lymphocyte infusions (DLI-iNKTs) promote lysis of leukemic blasts in a CD1d-dependent manner. Front Immunol. (2019) 10:1542. doi: 10.3389/fimmu.2019.01542
192. Laskowski TJ, Biederstädt A, Rezvani K. Natural killer cells in antitumour adoptive cell immunotherapy. Nat Rev Cancer. (2022) 22:557–75. doi: 10.1038/s41568-022-00491-0
193. Leong JW, Chase JM, Romee R, Schneider SE, Sullivan RP, Cooper MA, et al. Preactivation with IL-12, IL-15, and IL-18 induces CD25 and a functional high-affinity IL-2 receptor on human cytokine-induced memory-like natural killer cells. Biol Blood Marrow Transplant. (2014) 20:463–73. doi: 10.1016/j.bbmt.2014.01.006
194. Romee R, Rosario M, Berrien-Elliott MM, Wagner JA, Jewell BA, Schappe T, et al. Cytokine-induced memory-like natural killer cells exhibit enhanced responses against myeloid leukemia. Sci Transl Med. (2016) 8:357ra123. doi: 10.1126/scitranslmed.aaf2341
195. Shapiro RM, Birch GC, Hu G, Vergara Cadavid J, Nikiforow S, Baginska J, et al. Expansion, persistence, and efficacy of donor memory-like NK cells infused for posttransplant relapse. J Clin Invest. (2022) 132(11):e154334. doi: 10.1172/JCI154334
196. Wu CJ, Yang XF, McLaughlin S, Neuberg D, Canning C, Stein B, et al. Detection of a potent humoral response associated with immune-induced remission of chronic myelogenous leukemia. J Clin Invest. (2000) 106:705–14. doi: 10.1172/JCI10196
197. Zhang W, Choi J, Zeng W, Rogers SA, Alyea EP, Rheinwald JG, et al. Graft-versus-leukemia antigen CML66 elicits coordinated B-cell and T-cell immunity after donor lymphocyte infusion. Clin Cancer Res. (2010) 16:2729–39. doi: 10.1158/1078-0432.CCR-10-0415
198. Marina O, Hainz U, Biernacki MA, Zhang W, Cai A, Duke-Cohan JS, et al. Serologic markers of effective tumor immunity against chronic lymphocytic leukemia include nonmutated B-cell antigens. Cancer Res. (2010) 70:1344–55. doi: 10.1158/0008-5472.CAN-09-3143
199. Miklos DB, Kim HT, Zorn E, Hochberg EP, Guo L, Mattes-Ritz A, et al. Antibody response to DBY minor histocompatibility antigen is induced after allogeneic stem cell transplantation and in healthy female donors. Blood. (2004) 103:353–9. doi: 10.1182/blood-2003-03-0984
200. Miklos DB, Kim HT, Miller KH, Guo L, Zorn E, Lee SJ, et al. Antibody responses to H-Y minor histocompatibility antigens correlate with chronic graft-versus-host disease and disease remission. Blood. (2005) 105:2973–8. doi: 10.1182/blood-2004-09-3660
201. Zorn E, Miklos DB, Floyd BH, Mattes-Ritz A, Guo L, Soiffer RJ, et al. Minor histocompatibility antigen DBY elicits a coordinated B and T cell response after allogeneic stem cell transplantation. J Exp Med. (2004) 199:1133–42. doi: 10.1084/jem.20031560
202. Vago L, Perna SK, Zanussi M, Mazzi B, Barlassina C, Stanghellini MTL, et al. Loss of mismatched HLA in leukemia after stem-cell transplantation. N Engl J Med. (2009) 361:478–88. doi: 10.1056/NEJMoa0811036
203. Villalobos IB, Takahashi Y, Akatsuka Y, Muramatsu H, Nishio N, Hama A, et al. Relapse of leukemia with loss of mismatched HLA resulting from uniparental disomy after haploidentical hematopoietic stem cell transplantation. Blood. (2010) 115:3158–61. doi: 10.1182/blood-2009-11-254284
204. Jan M, Leventhal MJ, Morgan EA, Wengrod JC, Nag A, Drinan SD, et al. Recurrent genetic HLA loss in AML relapsed after matched unrelated allogeneic hematopoietic cell transplantation. Blood Adv. (2019) 3:2199–204. doi: 10.1182/bloodadvances.2019000445
205. Christopher MJ, Petti AA, Rettig MP, Miller CA, Chendamarai E, Duncavage EJ, et al. Immune escape of relapsed AML cells after allogeneic transplantation. N Engl J Med. (2018) 379:2330–41. doi: 10.1056/NEJMoa1808777
206. Shahid S, Ceglia N, Le Luduec J-B, McPherson A, Spitzer B, Kontopoulos T, et al. Immune profiling after allogeneic hematopoietic cell transplantation in pediatric acute myeloid leukemia. Blood Adv. (2023) 7:5069–81. doi: 10.1182/bloodadvances.2022009468
207. Toffalori C, Zito L, Gambacorta V, Riba M, Oliveira G, Bucci G, et al. Immune signature drives leukemia escape and relapse after hematopoietic cell transplantation. Nat Med. (2019) 25:603–11. doi: 10.1038/s41591-019-0400-z
209. Blank CU, Haining WN, Held W, Hogan PG, Kallies A, Lugli E, et al. Defining “T cell exhaustion.”Nat Rev Immunol. (2019) 19:665–74. doi: 10.1038/s41577-019-0221-9
210. Harris R, Mammadli M, Hiner S, Suo L, Yang Q, Sen JM, et al. TCF-1 regulates NKG2D expression on CD8 T cells during anti-tumor responses. Cancer Immunol Immunother. (2023) 72:1581–601. doi: 10.1007/s00262-022-03323-0
211. Bachireddy P, Azizi E, Burdziak C, Nguyen VN, Ennis CS, Maurer K, et al. Mapping the evolution of T cell states during response and resistance to adoptive cellular therapy. Cell Rep. (2021) 37:109992. doi: 10.1016/j.celrep.2021.109992
212. Bachireddy P, Hainz U, Rooney M, Pozdnyakova O, Aldridge J, Zhang W, et al. Reversal of in situ T-cell exhaustion during effective human antileukemia responses to donor lymphocyte infusion. Blood. (2014) 123:1412–21. doi: 10.1182/blood-2013-08-523001
213. Probst HC, McCoy K, Okazaki T, Honjo T, van den Broek M. Resting dendritic cells induce peripheral CD8+ T cell tolerance through PD-1 and CTLA-4. Nat Immunol. (2005) 6:280–6. doi: 10.1038/ni1165
214. Liu L, Chang Y-J, Xu L-P, Zhang X-H, Wang Y, Liu K-Y, et al. Reversal of T cell exhaustion by the first donor lymphocyte infusion is associated with the persistently effective antileukemic responses in patients with relapsed AML after allo-HSCT. Biol Blood Marrow Transplant. (2018) 24:1350–9. doi: 10.1016/j.bbmt.2018.03.030
215. Akatsuka Y, Morishima Y, Kuzushima K, Kodera Y, Takahashi T. Minor histocompatibility antigens as targets for immunotherapy using allogeneic immune reactions. Cancer Sci. (2007) 98:1139–46. doi: 10.1111/j.1349-7006.2007.00521.x
216. Dickinson AM, Wang X-N, Sviland L, Vyth-Dreese FA, Jackson GH, Schumacher TNM, et al. In situ dissection of the graft-versus-host activities of cytotoxic T cells specific for minor histocompatibility antigens. Nat Med. (2002) 8:410–4. doi: 10.1038/nm0402-410
217. Griffioen M, van der Meijden ED, Slager EH, Honders MW, Rutten CE, van Luxemburg-Heijs SAP, et al. Identification of phosphatidylinositol 4-kinase type II beta as HLA class II-restricted target in graft versus leukemia reactivity. Proc Natl Acad Sci U.S.A. (2008) 105:3837–42. doi: 10.1073/pnas.0712250105
218. Kircher B, Stevanovic S, Urbanek M, Mitterschiffthaler A, Rammensee H-G, Grünewald K, et al. Induction of HA-1-specific cytotoxic T-cell clones parallels the therapeutic effect of donor lymphocyte infusion. Br J Haematol. (2002) 117:935–9. doi: 10.1046/j.1365-2141.2002.03536.x
219. van Balen P, van Bergen CAM, van Luxemburg-Heijs SAP, de Klerk W, van Egmond EHM, Veld SAJ, et al. CD4 donor lymphocyte infusion can cause conversion of chimerism without GVHD by inducing immune responses targeting minor histocompatibility antigens in HLA class II. Front Immunol. (2018) 9:3016. doi: 10.3389/fimmu.2018.03016
220. Laghmouchi A, Hoogstraten C, van Balen P, Falkenburg JHF, Jedema I. The allogeneic HLA-DP-restricted T-cell repertoire provoked by allogeneic dendritic cells contains T cells that show restricted recognition of hematopoietic cells including primary Malignant cells. Haematologica. (2019) 104:197–206. doi: 10.3324/haematol.2018.193680
221. Zorn E, Wang KS, Hochberg EP, Canning C, Alyea EP, Soiffer RJ, et al. Infusion of CD4+ donor lymphocytes induces the expansion of CD8+ donor T cells with cytolytic activity directed against recipient hematopoietic cells. Clin Cancer Res. (2002) 8:2052–60.
222. Lin Y, Zhang L, Cai AX, Lee M, Zhang W, Neuberg D, et al. Effective posttransplant antitumor immunity is associated with TLR-stimulating nucleic acid-immunoglobulin complexes in humans. J Clin Invest. (2011) 121:1574–84. doi: 10.1172/JCI44581
223. Durakovic N, Radojcic V, Skarica M, Bezak KB, Powell JD, Fuchs EJ, et al. Factors governing the activation of adoptively transferred donor T cells infused after allogeneic bone marrow transplantation in the mouse. Blood. (2007) 109:4564–74. doi: 10.1182/blood-2006-09-048124
224. Darlak KA, Wang Y, Li J-M, Harris WAC, Owens LM, Waller EK. Enrichment of IL-12-producing plasmacytoid dendritic cells in donor bone marrow grafts enhances graft-versus-leukemia activity in allogeneic hematopoietic stem cell transplantation. Biol Blood Marrow Transplant. (2013) 19:1331–9. doi: 10.1016/j.bbmt.2013.06.016
Keywords: GVL, graft versus leukemia, DLI, donor lymphocyte infusion, cellular therapy
Citation: Maurer K and Antin JH (2024) The graft versus leukemia effect: donor lymphocyte infusions and cellular therapy. Front. Immunol. 15:1328858. doi: 10.3389/fimmu.2024.1328858
Received: 27 October 2023; Accepted: 28 February 2024;
Published: 15 March 2024.
Edited by:
Motoko Koyama, Fred Hutchinson Cancer Center, United StatesReviewed by:
Marie Bleakley, Fred Hutchinson Cancer Center, United StatesDaigo Hashimoto, Hokkaido University, Japan
Copyright © 2024 Maurer and Antin. This is an open-access article distributed under the terms of the Creative Commons Attribution License (CC BY). The use, distribution or reproduction in other forums is permitted, provided the original author(s) and the copyright owner(s) are credited and that the original publication in this journal is cited, in accordance with accepted academic practice. No use, distribution or reproduction is permitted which does not comply with these terms.
*Correspondence: Joseph H. Antin, Sm9zZXBoX0FudGluQGRmY2kuaGFydmFyZC5lZHU=