- 1Department of Hematology, The First Affiliated Hospital of Guangzhou University of Chinese Medicine, Guangzhou, China
- 2Department of Hematology, Dongzhimen Hospital of Beijing University of Chinese Medicine, Beijing, China
- 3Department of Rehabilitation Medicine, Southern Theater General Hospital, Guangzhou, China
- 4The First Clinical Medical College, Guangzhou University of Chinese Medicine, Guangzhou, China
- 5Department of Respiratory and Critical Care Medicine, Yantai Yuhuangding Hospital, Yantai, China
- 6Department of Hematology, Shenzhen Qianhai Shekou Pilot Free Trade Zone Hospital, Shenzhen, China
During the COVID-19 pandemic, elderly patients with underlying condition, such as tumors, had poor prognoses after progressing to severe pneumonia and often had poor response to standard treatment. Mesenchymal stem cells (MSCs) may be a promising treatment for patients with severe pneumonia, but MSCs are rarely used for patients with carcinoma. Here, we reported a 67-year-old female patient with lung adenocarcinoma who underwent osimertinib and radiotherapy and suffered from radiation pneumonitis. Unfortunately, she contracted COVID-19 and that rapidly progressed to severe pneumonia. She responded poorly to frontline treatment and was in danger. Subsequently, she received a salvage treatment with four doses of MSCs, and her symptoms surprisingly improved quickly. After a lung CT scan that presented with a significantly improved infection, she was discharged eventually. Her primary disease was stable after 6 months of follow-up, and no tumor recurrence or progression was observed. MSCs may be an effective treatment for hyperactive inflammation due to their ability related to immunomodulation and tissue repair. Our case suggests a potential value of MSCs for severe pneumonia that is unresponsive to conventional therapy after a COVID-19 infection. However, unless the situation is urgent, it needs to be considered with caution for patients with tumors. The safety in tumor patients still needs to be observed.
1 Introduction
Since the winter of 2019, the pandemic of coronavirus disease 2019 (COVID-19), caused by severe acute respiratory syndrome coronavirus 2 (SARS-CoV-2), has threatened global public health. COVID-19 is a self-limiting disease for most people. However, approximately 22.97% of cases of COVID-19 developed into acute respiratory distress syndrome (ARDS), and 9.68% of patients needed admission to intensive care unit, with a mortality rate of 1.28% in 2020 (1). The virulence of SARS-CoV-2 has significantly weakened nowadays (2). However, people with old age or frail condition still face a high risk of progression to severe or critical disease of COVID-19. The risk factors associated with severe disease include age more than 60 years old, smoking, being unvaccinated against COVID-19, HIV infection, and underlying noncommunicable illness (1, 3). Any kind of malignancy history was an independent risk factor for severe illness (4) and mortality (5). The estimated pooled mortality rate was 5.6% for the whole population. Nevertheless, it significantly increased to 22.4% for all malignancies, and 32.9% for lung cancer (6). Therefore, lung cancer patients were at a greater risk of death than other types of carcinoma in the pandemic of COVID-19 (7).
As a self-limiting disease, supportive care is essential for all patients (3). Furthermore, the frontline drugs strongly recommended by WHO are nirmatrelvir/ritonavir for non-severe disease and corticosteroids, interleukin-6 (IL-6) receptor blockers, and baricitinib for severe or critical illness (8). The conditional treatments include ruxolitinib, tofacitinib, convalescent plasma, molnupiravir, remdesivir, and so on (8). Nirmatrelvir/ritonavir can significantly reduce the 30-day risk of hospitalization or death by approximately 11.16 per 1000 patients. There are patients (approximately 2.30%) who did not respond to this drug, which include 0.25% who needed ICU admission, 0.083% under mechanical ventilation, and 0.125% who died (9). It is believed that tissue damage co-exists with cytokine storms caused by hyper-activating the immunity system in severe and critical disease (10). On the other hand, baricitinib and tocilizumab, two widely used inflammatory factor inhibitors, also improve the survival of patients with severe or critical COVID-19. However, approximately 18.0% of patients treated with baricitinib and 24% of patients treated with tocilizumab did not respond to these drugs, among whom 56.0% and 46.7%, respectively, progressed to death (11, 12). Thus, the therapy that possesses the ability of inflammatory modulation and tissue repair might be the ideal choice for those who do not respond to frontline treatment. Mesenchymal stem cells (MSCs) have been reported to possess multi-potency (13), which leads to MSCs being widely used in regenerative medicine and tissue engineering. Nowadays, MSCs’ ability related to immunomodulation is proven as well (14). Therefore, MSCs can theoretically be used to treat severe and critical COVID-19 patients who do not respond to frontline drugs, and the efficacy has been proven by many clinical trials (15–35). However, MSCs are rarely used in cancer patients due to concerns about safety and neoplasm recurrence. Hence, the safety and efficacy of MSCs for cancer patients are still unclear. Here, we report a patient with lung cancer. She suffered from severe pneumonia caused by COVID-19 following radiation pneumonitis and was finally successfully salvaged by treatment with MSCs after the failure of the first-line treatment, which brings in hopes of offering clinicians evidence and confidence in MSCs utilization.
2 Case presentation
2.1 Recent medical history
A 67-year-old female patient had suffered from a recurring pain in the neck, shoulder, and left upper limb for more than 3 months since August 2021, so she visited the Yantai Yuhuangding Hospital on November 26, 2021. The laboratory tests indicated that carcinoembryonic antigen (CEA) was at 34.5 ng/mL and neuron-specific enolase (NSE) was at 19.1 ng/mL, and a BI-RADS 4a focal and bilateral axilla lymphadenectasis were detected by color ultrasonic scan. The CT scan suggested a large focal in the left lung superior lobe. No evidence proved the existence of an infectious disease, and therefore she was hospitalized and went through a CT-guided percutaneous core needle biopsy of the lung on December 2, 2021. The pathological and molecular biological results supported the diagnosis of non-small cell lung cancer (adenocarcinoma) with epidermal growth factor receptor (EGFR) and TP53 mutation. She was finally diagnosed with lung adenocarcinoma of the left superior lobe (cT3NxM1c, with hilar, mediastinum, neck vertebra, and lumbar vertebra metastasis and EGFR and TP53 mutation). She visited the oncology department and received oral EGFR inhibitor osimertinib based on the doctor’s recommendations and the National Comprehensive Cancer Network (NCCN) guidelines. The periodic lung CT examination after oral osimertinib showed a significant reduction of lung lesions. Then, she underwent irradiation therapy from June to August 2022. In November, she began coughing, with phlegm, and later developed shortness of breath, with no fever, and had chest pain, and she was hospitalized in Yantaishan Hospital. She was diagnosed with tuberculosis, aspergillus, and pneumocystis jirovecii infection and treated with isoniazid, pyrazinamide, ethambutol, quinolone antibiotics, sulfamethoxazole/trimethoprim, voriconazole, and glucocorticoid. The symptoms were significantly alleviated after the anti-infective therapy, but she still had cough and phlegm sometimes. The respiratory symptoms were exacerbated again on December 18, 2022, so the patient visited the respiratory department of Yantai Yuhuangding Hospital on December 19, 2022 and was hospitalized. She felt dyspnea and had no fever at admission. The test for SARS-CoV-2 RNA performed 1 day before hospitalization yielded a negative result.
2.2 Medical history
The patient had a history of hypertension stage 2 and coronary atherosclerotic cardiopathy (CAD) for approximately 20 years, but recently, her blood pressure was normal and she had no sign of CAD without any intervention.
2.3 SARS-CoV-2 vaccination history
The patient was vaccinated with a first dose of inactivated anti-SARS-CoV-2 vaccine (Vero cell) on May 20, 2021, and the second dose was received on June 17, 2021.
2.4 Physical examination
At admission, her body temperature was 36.7°C, her pulse rate was 97/min, her respiratory rate was 23/min, and her blood pressure was 146/79 mmHg. SpO2 was 99% with a 3 L/min of nasal oxygen supply. Other physical examinations revealed no specific signs, but the Velcro rale was auscultated.
2.5 Laboratory test at hospitalization
The emergency laboratory test results were as follows: blood routine—white blood cell count (WBC), 8.02 × 109/L; neutrophil count (NEU), 7.53 × 109/L; hemoglobin (HGB), 99 g/L; platelet (PLT), 176 × 109/L; lymphocyte count (LYM), 0.22 × 109/L; D-dimer (DDi), 2.24 mg/L; procalcitonin (PCT), 0.0916 ng/mL; and high sensitivity C-reactive protein (hs-CRP), 42.65 mg/L. ProBNP, cardiac biomarkers, hepatic and kidney function tests, and electrolyte panel were not significantly abnormal.
2.6 Therapeutic intervention and outcome
2.6.1 Frontline treatment and outcome
Given the patient’s poor lung condition caused by radiation therapy and suppressed immunity caused by anti-tumor agents, the patient was at a high risk of opportunistic infection. Sulfamethoxazole/trimethoprim (SMZ/TMP, 0.96 g q6h), moxifloxacin (400 mg qd), piperacillin-tazobactam (4.5 g q8h), ganciclovir (0.25 g q12h), methylprednisolone (80 mg qd), and supportive therapy were empirically administrated on the first day. However, the patient’s symptoms were not significantly alleviated after 2 days of treatment. The heart rate accelerated and SpO2 were decreased, which meant a more serious anoxia than before. The test results for SARS-CoV-2 RNA (swab), (1,3)-β-D-glucan (peripheral blood), and galactomannan (peripheral blood) were negative. A chest X-ray tomography was arranged on December 22, 2022, and it indicated a multiple ground-glass shadow of the lung. Considering the poor immunity result from a previous carcinoma history, intravenous immunoglobulin (10 g qd) was used from December 21 to 27, 2022. Moxifloxacin and piperacillin–tazobactam were ceased, and voriconazole (200 mg q12h) was added by December 22, 2022, given her history of aspergillus and high risk of aspergillus re-infection, and we reduced methylprednisolone as well (40 mg qd) (Figure 1).
After observing the abovementioned treatment for another week, the patient’s condition was still unsatisfactory. Although the body temperature and blood pressure were normal, the patient often coughed with phlegm, had dyspnea, and was fatigued. The SpO2 fluctuated between 84% and 94% with nasal oxygen supply. On December 29, 2022 the COVID-19 RNA test was positive. Chest computerized tomography suggested new bilateral interstitial pneumonia (Figure 2). Ganciclovir was ceased, and paxlovid was administrated from December 29, 2022. Cefoperazone–sulbactam (3 g q8h) was also added starting December 30, 2022.
However, no alleviation of the patient’s symptoms was observed after the 5-day treatment including nirmatrelvir/ritonavir. Although we tried the addition of linezolid (200 mg q12h) since January 3, 2023, the symptoms were still serious. Therefore, we advised the utilization of MSCs for salvage therapy.
2.6.2 Salvage treatment by MSCs
2.6.2.1 MSCs preparation and ethical consideration
Clinical-grade human umbilical cord mesenchymal stem cells (hUC-MSCs) were supplied by the umbilical cord blood bank of Shandong Province. The preparation was completed in a GMP laboratory. Samples of human umbilical cords were collected and cut into 2- to 3-mm pieces to be processed for MSCs isolation. Small cylindrical fragments (d = 2 mm) were removed from the mucous connective tissue (Wharton’s jelly matrix), avoiding blood vessels and amniotic epithelium. They were transferred to culture dishes without any blood serum for adherent culture. Wharton’s jelly fragments were incubated in human MSCs growth medium at 37°C in a humidified incubator under a 21% O2 and 5% CO2 atmosphere. After 7–10 days of culture, the first colonies of WJ-MSCs were observed. Then, the cells were pursued until subconfluence; the non-adherent cells were removed, and the stromal cells were detached (0.05% trypsin-ethylenediaminetetraacetic acid (trypsin-EDTA)) and then transferred into 25-cm3 flasks at an initial density of 5 × 103/cm3 and cultured up to 70%–80% confluence before collection for subsequent passages. Cells at passages P5 were used and had the International Society for Cell & Gene Therapy (ISCT)-recommended cell surface characteristics of MSCs, including expression (95%) of clusters of differentiation 73 (CD73), CD90, and CD105 and lack of cell surface presentation (<2%) of CD34, CD45, CD14 or CD11b, CD79α or CD19, and human leukocyte antigen-DR (HLA-DR).
Intravenous administration was used. Before the intravenous drip, the hUC-MSCs were suspended in 100 mL of normal saline, and the total number of transplanted cells was calculated as 1 × 106 cells/kg. The MSCs were infused through the patient’s right cubital veins for approximately 1 h (35 drops/min).
It had received approval from the Ethics Committee of Yantai Yuhuangding Hospital for compassionate use. Informed consent was obtained from the patient and her family members.
2.6.2.2 Efficacy of MSCs
The first dose (4.93 × 107 cells) of MSCs was intravenously infused on January 4, 2023. Her difficulty in breathing was slightly alleviated. The second dose (4.88 × 107 cells) was infused on January 8, which led to an advancing improvement. SpO2 was higher than before and fluctuated between 95% and 99% after the second dose of MSCs. However, on January 14, 2023, the chest CT indicated a slightly extending bilateral pneumonia and novel pneumothorax of the left lung. Serum interleukin-6 (IL-6) was 34.17 pg/mL (Figure 3), which was believed to be relative to severe COVID-19 (36). PCT was 0.0785 ng/mL and hs-CRP was 9.68 mg/L. We were concerned that the patient’s condition would be exacerbated again, so the third dose (4.94 × 107 cells) of MSCs was arranged in the morning of January 16, 2023. Although the patient had six times of hemoptysis at night, it was quickly controlled the next day and the condition became much better 3 days later. Cefoperazone–sulbactam was changed into ceftizoxime (2 g q12h) in January 14 as well because of limited evidence of serious bacterial infection (low level of PCT detected and normal count of WBC and NEU on weekly surveillance). The fourth and last dose (4.95 × 107 cells) of MSCs for consolidation therapy was administrated on January 24, 2023. The patient no longer relied on middle to high oxygen concentration since January 25, 2023. A lung X-ray suggested an improvement of right pneumonia and absorption of the left pneumothorax. All symptoms were alleviated significantly, and her performance status recovered. We prescribed oral antibiotics since February 2, 2023, and the patient was eventually discharged 2 days later. The patient is still alive since the last follow-up on July 15, 2023.
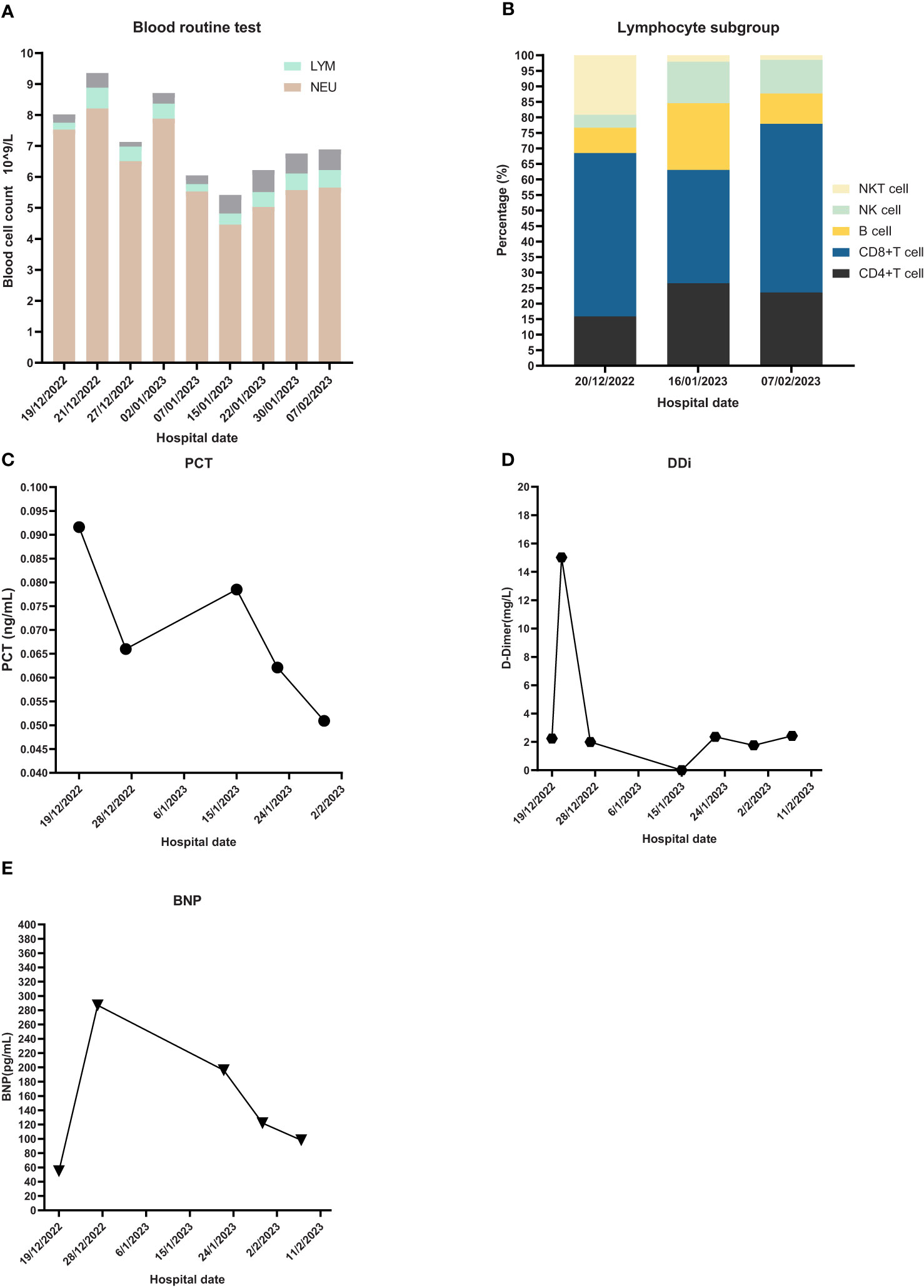
Figure 3 Laboratory Inspection. (A) Blood routine test and proportion of different blood cells. NEU: neutrophil, LYM: lymphocyte. (B) Lymphocyte subgroup analysis. (C) PCT: Procalcitonin. (D) DDi: D-Dimer. (E) BNP: B-type natriuretic peptide.
3 Discussion
We report a patient with a poor lung condition due to carcinoma and radiotherapy. Complex infections, including bacteria, fungus, and COVID-19, further impaired the lung. Nevertheless, bacteria and fungus might not be the vital pathogen in this case because of continuously low levels of PCT, WBC, NEU, and (1,3)-β-D-glucan and the characteristics of chest CT. The utilization of broad-spectrum antibiotics and antifungal agents did not effectively reverse the pneumonia. The patient received nirmatrelvir/ritonavir immediately when the SARS-CoV-2 RNA test was positive. It was suggested that the hospital admission or death rate of patients who were given nirmatrelvir/ritonavir was only 2.1% (37). However, she developed a severe disease in a short time. The mortality of all lung cancer patients with COVID-19 was 31.0% in a multi-country pooled analysis (38). It increased to 58.4% when patients developed severe disease (39). Therefore, lung cancer patients are at higher risk of death, and the risk would incredibly increase when patients progress to severe COVID-19. We believed that the patient’s condition was less likely to alleviate without advance treatment, and it was urgent and necessary at that time to find a suitable treatment as soon as possible to reverse the disease quickly. The lung injury due to inflammation and former radiation pneumonitis (RP) were a concern, and she needed a treatment option that had a function of immunomodulation and injury repair. The frontline inflammatory inhibitor baricitinib (JAK inhibitor) and tocilizumab (IL-6 inhibitor) could be choices, but they were both hard to acquire at that time and had no evidence of tissue repair effect. In addition, remdesivir is also an option. However, the Chinese Food and Drug Administration did not approve the marketing of remdesivir in China, which may be because, according to the results of several randomized controlled trials, Chinese patients may not benefit from the drug. These trials showed that there was no significant difference in the recovery rate and the risk of progression to severe disease between remdesivir and the control group (40–42). The patient received four doses of MSCs infusion and finally achieved a surprisingly effective outcome. The case indicates that patients with a poor condition of the lung and serious inflammation may benefit from MSCs.
3.1 Potential mechanism of MSCs
ARDS or acute lung injury caused by SARS-CoV-2 induces cytokine storm characterized by severe hypoxemia, high-permeability pulmonary edema, and reduced compliance of the integrated respiratory system as a result of widespread compressive atelectasis and fluid-filled alveoli (43). Moreover, the virus triggers alveolar and interstitial fibrin deposition, endothelial dysfunction, and pulmonary intravascular coagulation, which may also contribute to the persistence of abnormal inflammatory and disease progress. Recent studies revealed that the biological mechanism of MSCs for the treatment of infectious lung injury might involve multiple pathways. However, immunomodulation and tissue repair might be both crucial.
3.1.1 Immunomodulation
SARS-CoV-2 enters cells, and cells’ capillary permeability increases, allowing more viruses to transfer into cells, resulting in ARDS. The virus activates an immune response involving natural killer (NK) cells, CD4+T cells, CD8+T cells, and B cells in COVID-19 patients, causing the abnormal production of cytokines (44). The abnormal cytokine profile results in excessively activated but dysfunctional immunity reactions in patients with severe COVID-19, which presents a delayed or failed elimination of virus. It has been proven that MSCs affect both innate and acquired immunity to modulate the abnormal immune reaction in patients.
Induction of inflammatory cytokines from macrophages by the nucleocapsid (N) protein of SARS-CoV-2 has been proven. N-protein could promote tumor necrosis factor (TNF), interleukin-1β (IL-1β), interleukin-6 (IL-6), and monocyte chemoattractant protein-1 (MCP-1) secretion of macrophages, which was associated with the occurrence of severe COVID-19 (45). MSCs affect macrophages through contact-dependent interaction, paracrine-mediated mechanisms, autophagy, mitophagy, and oxidative stress (46). With MSCs’ existence, pro-inflammatory macrophages secrete anti-inflammatory factors and pro-inflammatory cytokines were downregulated, consequently resulting in the enhancement of phagocytic properties and macrophage type switch (47). In an experiment in mice, a significant reduction in inflammatory cell and alveolar hemorrhage were observed, and the total number of macrophages and neutrophils, respectively, were remarkably decreased (48).
In patients with severe COVID-19, NK cell was activated by activating ligands or pro-inflammatory cytokines produced by NK cell itself (49) or other cells. However, activating receptors on NK cells and transcription factor T-bet were both downregulated, and transforming growth factor-β (TGFβ) inhibited the function of NK cells. This resulted in NK cell exhaustion, impairment, disability to degranulate, and cytotoxicity (50). The immunological profile in patients with COVID-19 is characterized by the contraction of immature CD56bright and expansion of mature CD57+FcϵRlγneg adaptive NK cells, which might lead to hyperactive inflammation (51). Several studies have proven that CD107α expression of NK cells was observed when NK cells were co-cultured with MSCs, which suggested that MSCs could effectively assist in NK cell degranulation (52).
Dendritic cells (DCs) are major antigen-presenting cells of innate immunity and bridge adaptive immunity. SARS-CoV-2 promotes the expression of major histocompatibility complex (MHC) molecules and co-stimulatory receptors of DCs that represent its maturation. Additionally, the nuclear factor-κB (NF-κB) pathway, which is believed to be vital to the signal responsible for the expression and secretion of pro-inflammatory cytokines, is activated when DCs interact with virus protein (53, 54). MSCs have the capacity to inhibit DC maturity and induce mature DC (mDC) differentiation into regulatory DC by many pathways involving notch-1/Jagged-1 signal (55–57), Akt signal (58), C-C motif chemokine receptor 7 (CCR7) gene degradation (59), T helper 17 (Th17) cell inhibition (60), and so on.
At the aspect of T cell, the virus-specified T cell and bystander T cell are both activated in the early stage of infection (61). However, the unresolved inflammation due to high exposure dose or rapid replication of virus, age, and deficient immunity may result in prolonging the activation of bystander T cells, which can produce pro-inflammatory cytokines continuously (62). Those long-lasting T cells in severe COVID-19 are dysfunctional and pathological, and massive activation-induced cell death is observed (63, 64). MSCs demonstrate an immunomodulatory effect on CD4+ T cells’ subgroup balance and an immunosuppressive effect on CD3+ T cells. MSCs inhibited Th17 and induced Treg differentiation, which led to the attenuation of lung injury caused by the virus (65). On the other hand, when MSCs were injected in mice with viral pneumonia, attenuated proliferation of both CD3+, CD4+ and CD4+, CD8+ T cells was observed, resulting in the significant suppression of pro-inflammation factor level and the protection of mice alveolar epithelial cells from inflammatory injury similar to severe COVID-19 (66).
The antibody against SARS-CoV-2 is secreted by B cells. In patients with a severe disease, an impaired germinal center response and a robust extrafollicular response were observed compared to those with a mild infection, which might be associated with an elevated level of antibody (perhaps low-mutation IgG1) and pro-inflammatory cytokines. The abnormal activation of extrafollicular B cells in severe COVID-19 is similar to autoimmune response and correlated to an increasing level of inflammation hallmarks and death (67–69). When co-cultured with MSCs, most proteins of the pathway activating B cells were significantly reduced, resulting in the reduction of cell proliferation and transcription gene expression involving terminal differentiation into plasma cell of B cell (70). Regulatory B cell (Breg) is a new subgroup recently defined with a function similar to Treg, and Breg is increased by MSCs. IL-10 secreted by Breg was increased, and immunoglobulin production was further inhibited (71). MSCs were believed to ameliorate lung tissue injury by inhibition of chemotaxis gene and immunoglobulin expression at the aspect of humoral immunity (72).
In summary, MSCs are believed to be effective for severe COVID-19 by the immunity pathways of reducing pro-inflammatory factor through multiple signal pathways, consequently promoting macrophage phenotype switch, NK cell degranulation, regulatory DC generation, Treg differentiation, and B cell activation inhibition.
3.1.2 Tissue repair
There are two types of lung damage relative to severe COVID-19: one induced by inflammation, which possibly induces ARDS and the other connected with fibrosis, also known as post-COVID pulmonary fibrosis. At first, in the acute phase, the virus causes diffuse and severe alveolar damage, including critical endothelial injury, microangiopathy, and obstruction of the alveolar capillaries. After this, the pulmonary surfactant becomes dysfunctional and deficient, contributing to edema and fibrosis. In severe cases, the abovementioned process is continuous and transforms normal lung tissue into fibrous tissue eventually (73).
MSCs demonstrate multi-potency and high proliferation ability in many studies, which make MSCs an ideal option for regenerative medicine. The extracellular vesicles (EVs) from MSCs are released and contact with the target recipient cell. Afterward, the damaged lung tissue is protected from inflammatory injury and renewed (74, 75). When MSCs were co-cultured with injured lung tissue in vitro, 44 kinds of proteins were secreted to accelerate airway epithelium repair by stimulating migration, proliferation, and differentiation. MSCs enhanced anti-apoptosis signal pathways, restored matrix metalloproteinases function, and reduced the percentage of type II alveolar epithelial cells (76–78). An analysis of EVs’ miRNA indicated that miR-223-3p, which is associated with the alleviation of pulmonary fibrosis, was abundant in EVs, and it could attenuate the deposition of fibrosis-related factors. In vitro, EVs restricted the activation and proliferation of fibroblasts (79). Additionally, miR-214-3p and miR-466f-3p derived from MSCs mediated prevention from radiation injury and inhibited lung fibrosis by downregulating the ATM/P53/P21 signaling and inhibiting the AKT/GSK3β pathway, respectively (80, 81).
Therefore, MSCs repair injured lung tissue caused by severe COVID-19 disease probably through promoting molecules involved in airway epithelium proliferation and differentiation, activation of anti-apoptosis signal pathways, and inhibition of fibroblasts.
3.2 Clinical use and research
3.2.1 Case reports
MSCs have been tried as a salvage treatment for critically ill pneumonia patients after the outbreak of the pandemic. In 2020, Chinese physicians successively shared several cases of severe pneumonia treated with MSCs (82–88), and then similar cases began to be reported in other countries and regions (87–96). The dose range for an intravenous drip of MSCs was (1–3) × 106 kg in most of the cases (82–90, 96). It is also important to note that none of these cases had a history of cancer, except for three cases reported in Sweden in 2022 (96) (Table 1).
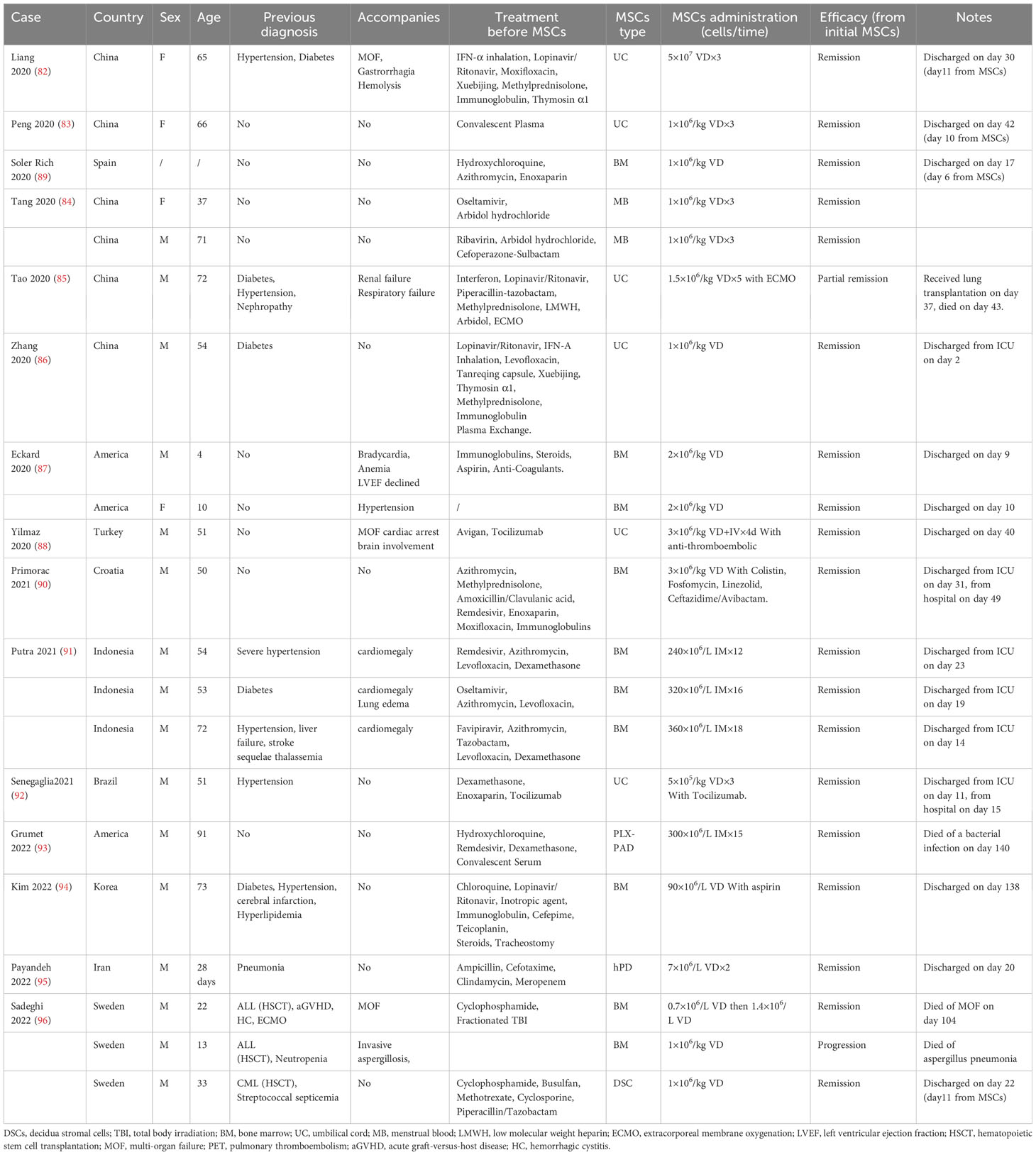
Table 1 Characteristics of public case reports of mesenchymal stem cells (MSCs) for patients with severe pneumonia.
3.2.2 Clinical trials
Many trials have been performed to explore the efficacy of MSCs for patients with severe pneumonia. The common dose of MSCs for intravenous injection was 0.5 to 3 × 106 cell/kg each time for one to three administrations. However, the clinical outcome was varied.
In some small-sample single-arm trials, MSCs demonstrated ideal efficacy with a survival rate of more than 80% (15, 18, 19), while it was just almost 60% in a large-sample single-arm trial (17). A significantly better survival and time to improvement (24) were likewise observed in some cohort studies (23, 24), but MSCs therapy did not dramatically shorten hospital duration (22, 24, 25). In many small-sample randomized controlled trials, MSCs therapy could significantly decrease mortality (27–29, 31), shorten hospital time, and increase the rate of clinical improvement (26, 27, 30, 31, 97). However, the diagnosis of all eligible subjects was COVID-19 pneumonia, without malignancy under treatment.
A long-time follow-up was described in only one trial, but the risk of secondary cancer was not mentioned (97). Limited by a small sample, all other trials also did not explore the risk of secondary malignancy in a long time after MSCs administration (Table 2).
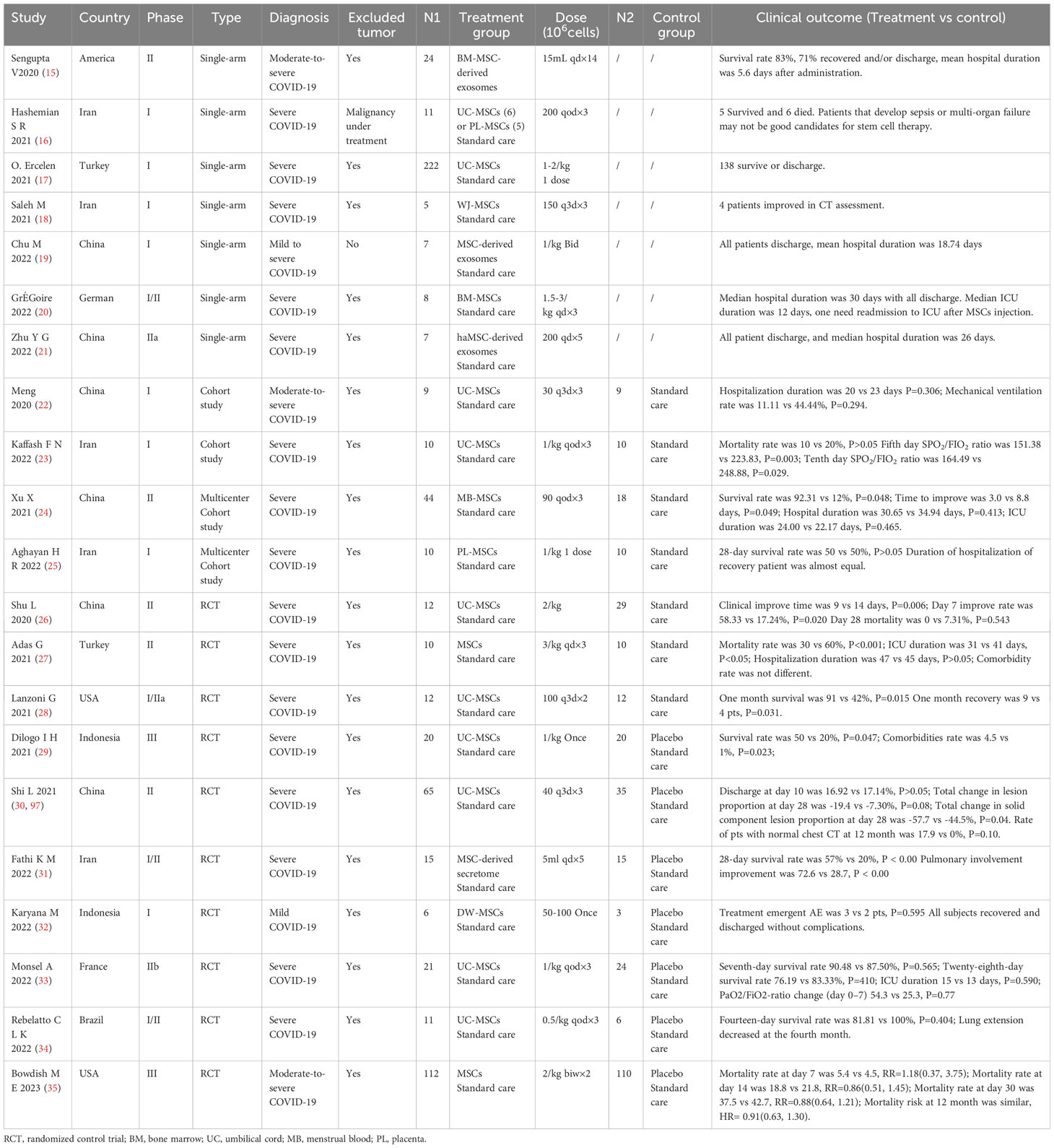
Table 2 Characteristics of public clinical trials of mesenchymal stem cells (MSCs) for patients with severe pneumonia.
3.3 Drawbacks and risk of MSCs
The most controversial drawback is its uncertain effect on tumor generation. Some laboratory studies indicate that MSCs might promote cancer progression and therapeutic resistance. MSCs may secrete prostaglandin E2 (PGE2), IFN-γ, IL-4, TGF-β1, and VEGF to inhibit anti-tumor immune response (98), stimulate angiogenesis (99), promote cancer cells metastasis (100) and proliferation (101), and protect them from apoptosis (102), thereby promoting tumor growth. Furthermore, MSCs protect chronic myeloid leukemia cells and ovarian cancer cells against anti-tumor drugs by downregulation of caspase 3 via CXCL12/CXCR4 axis (103, 104). A similar phenomenon of drug resistance associated with MSCs was also observed in multiple myeloma and colorectal cancer via the production of CXCL13 (105) and IL-6 (106), respectively. Moreover, the multipotential of MSCs brings about concerns that MSCs themselves might be the potential cancer stem cells when MSCs are cultured for the long term (107).
Clinically, caution needs to be taken regarding some adverse events (AEs) of MSCs. A meta-analysis summarized the AEs of MSCs application over the past 15 years. The most frequent major AEs were fever and administration site conditions, and meaningfully common minor AEs were constipation, fatigue, and insomnia (108). No increasing risk of tumor generation had been observed (109). However, given the ethics principle, MSCs’ risk of promoting tumor relapse is still unclear because patients with cancer have been excluded from almost all clinical trials.
4 Conclusion
This is a rare case, with lung cancer and radiation pneumonitis, who suffered from severe infection and was successfully benefit from MSCs infusion. MSCs perhaps reverse the clinical outcomes by immunomodulation and tissue repair. Our case fills the gap of MSCs application for patients with carcinoma accompanied with severe pneumonia. MSCs may be an option for a refractory case in such a period without sufficient and effective drugs.
Data availability statement
The original contributions presented in the study are included in the article/supplementary material. Further inquiries can be directed to the corresponding author.
Ethics statement
The studies involving humans were approved by Ethics Committee of Yantai Yuhuangding Hospital. The studies were conducted in accordance with the local legislation and institutional requirements. The participants provided their written informed consent to participate in this study. Written informed consent was obtained from the individual(s) for the publication of any potentially identifiable images or data included in this article.
Author contributions
XH: Investigation, Writing – original draft. XT: Investigation, Writing – original draft. XX: Investigation, Writing – original draft. TJ: Conceptualization, Writing – review & editing. YX: Supervision, Validation, Writing – review & editing. ZL: Conceptualization, Supervision, Validation, Writing – review & editing.
Funding
The author(s) declare financial support was received for the research, authorship, and/or publication of this article. This work was supported by funding from the National Natural Science Foundation of China (project no. 81903973, project no. 81873426), Shenzhen Science and Technology Plan Project (project no. JCYJ20220530151613030), Shenzhen Nanshan District Technology R&D and Creative Design Project Sub-funded Health Science and Technology Project (project no. NS2022004).
Conflict of interest
The authors declare that the research was conducted in the absence of any commercial or financial relationships that could be construed as a potential conflict of interest.
Publisher’s note
All claims expressed in this article are solely those of the authors and do not necessarily represent those of their affiliated organizations, or those of the publisher, the editors and the reviewers. Any product that may be evaluated in this article, or claim that may be made by its manufacturer, is not guaranteed or endorsed by the publisher.
References
1. Jutzeler CR, Bourguignon L, Weis CV, Tong B, Wong C, Rieck B, et al. Comorbidities, clinical signs and symptoms, laboratory findings, imaging features, treatment strategies, and outcomes in adult and pediatric patients with COVID-19: A systematic review and meta-analysis. Travel Med Infect Dis (2020) 37:101825. doi: 10.1016/j.tmaid.2020.101825
2. DeGrasse DC, Black SD. The rise of SARS-CoV-2 (COVID-19) omicron subvariant pathogenicity. Cureus (2023) 15:e40148. doi: 10.7759/cureus.40148
3. World Health Organization. Clinical management of COVID-19: Living guideline, 13 January 2023 (2023). Available at: https://app.magicapp.org/#/guideline/j1WBYn.
4. Li J, Huang DQ, Zou B, Yang H, Hui WZ, Rui F, et al. Epidemiology of COVID-19: A systematic review and meta-analysis of clinical characteristics, risk factors, and outcomes. J Med Virol (2021) 93:1449–58. doi: 10.1002/jmv.26424
5. Taylor EH, Marson EJ, Elhadi M, Macleod KDM, Yu YC, Davids R, et al. Factors associated with mortality in patients with COVID-19 admitted to intensive care: A systematic review and meta-analysis. Anaesthesia (2021) 76:1224–32. doi: 10.1111/anae.15532
6. Zhang H, Han H, He T, Labbe KE, Hernandez AV, Chen H, et al. Clinical characteristics and outcomes of COVID-19-infected cancer patients: A systematic review and meta-analysis. J Natl Cancer Institute (2021) 113:371–80. doi: 10.1093/jnci/djaa168
7. Khoury E, Nevitt S, Madsen WR, Turtle L, Davies G, Palmieri C. Differences in outcomes and factors associated with mortality among patients with SARS-CoV-2 infection and cancer compared with those without cancer: A systematic review and meta-analysis. JAMA Netw Open (2022) 5:e2210880. doi: 10.1001/jamanetworkopen.2022.10880
8. World Health Organization. Therapeutics and COVID-19: Living guideline, 13 January 2023 (2023). Available at: https://app.magicapp.org/#/guideline/nBkO1E.
9. Bajema KL, Berry K, Streja E, Rajeevan N, Li Y, Mutalik P, et al. Effectiveness of COVID-19 treatment with nirmatrelvir-ritonavir or molnupiravir among u.s. Veterans: Target trial emulation studies with one-month and six-month outcomes. Ann Internal Med (2023) 176:807–16. doi: 10.7326/M22-3565
10. Singh DD, Han I, Choi E-H, Yadav DK. A clinical update on SARS-CoV-2: Pathology and development of potential inhibitors. Curr Issues Mol Biol (2023) 45:400–33. doi: 10.3390/cimb45010028
11. Trøseid M, Arribas JR, Assoumou L, Holten AR, Poissy J, Terzić V, et al. Efficacy and safety of baricitinib in hospitalized adults with severe or critical COVID-19 (bari-SolidAct): A randomised, double-blind, placebo-controlled phase 3 trial. Crit Care (London England) (2023) 27:9. doi: 10.1186/s13054-022-04205-8
12. Hermine O, Mariette X, Tharaux P-L, Resche-Rigon M, Porcher R, Ravaud P, et al. Effect of tocilizumab vs usual care in adults hospitalized with COVID-19 and moderate or severe pneumonia: A randomized clinical trial. JAMA Internal Med (2021) 181:32–40. doi: 10.1001/jamainternmed.2020.6820
13. Dzobo K. Recent trends in multipotent human mesenchymal stem/stromal cells: Learning from history and advancing clinical applications. Omics: J Integr Biol (2021) 25:342–57. doi: 10.1089/omi.2021.0049
14. Li J-P, Wu K-H, Chao W-R, Lee Y-J, Yang S-F, Chao Y-H. Immunomodulation of mesenchymal stem cells in acute lung injury: From preclinical animal models to treatment of severe COVID-19. Int J Mol Sci (2022) 23:8196. doi: 10.3390/ijms23158196
15. Sengupta V, Sengupta S, Lazo A, Woods P, Nolan A, Bremer N. Exosomes derived from bone marrow mesenchymal stem cells as treatment for severe COVID-19. Stem Cells Dev (2020) 29:747–54. doi: 10.1089/scd.2020.0080
16. Hashemian SR, Aliannejad R, Zarrabi M, Soleimani M, Vosough M, Hosseini SE, et al. Mesenchymal stem cells derived from perinatal tissues for treatment of critically ill COVID-19-induced ARDS patients: A case series. Stem Cell Res Ther (2021) 12:91. doi: 10.1186/s13287-021-02165-4
17. OE N, Pekkoc-Uyanik KC, Alpaydin N, Gulay GR, Simsek M. Clinical experience on umbilical cord mesenchymal stem cell treatment in 210 severe and critical COVID-19 cases in Turkey. Stem Cell Rev Rep (2021) 17:1917–25. doi: 10.1007/s12015-021-10214-x
18. Saleh M, Vaezi AA, Aliannejad R, Sohrabpour AA, Kiaei SZF, Shadnoush M, et al. Cell therapy in patients with COVID-19 using wharton’s jelly mesenchymal stem cells: A phase 1 clinical trial. Stem Cell Res Ther (2021) 12:410. doi: 10.1186/s13287-021-02483-7
19. Chu M, Wang H, Bian L, Huang J, Wu D, Zhang R, et al. Nebulization therapy with umbilical cord mesenchymal stem cell-derived exosomes for COVID-19 pneumonia. Stem Cell Rev Rep (2022) 18:2152–63. doi: 10.1007/s12015-022-10398-w
20. Grégoire C, Layios N, Lambermont B, Lechanteur C, Briquet A, Bettonville V, et al. Bone marrow-derived mesenchymal stromal cell therapy in severe COVID-19: Preliminary results of a phase i/II clinical trial. Front Immunol (2022) 13:932360. doi: 10.3389/fimmu.2022.932360
21. Zhu YG, Shi MM, Monsel A, Dai CX, Dong X, Shen H, et al. Nebulized exosomes derived from allogenic adipose tissue mesenchymal stromal cells in patients with severe COVID-19: A pilot study. Stem Cell Res Ther (2022) 13:220. doi: 10.1186/s13287-022-02900-5
22. Meng F, Xu R, Wang S, Xu Z, Zhang C, Li Y, et al. Human umbilical cord-derived mesenchymal stem cell therapy in patients with COVID-19: A phase 1 clinical trial. Signal Transduct Target Ther (2020) 5:172. doi: 10.1038/s41392-020-00286-5
23. Kaffash Farkhad N, Sedaghat A, Reihani H, Adhami Moghadam A, Bagheri Moghadam A, Khadem Ghaebi N, et al. Mesenchymal stromal cell therapy for COVID-19-induced ARDS patients: A successful phase 1, control-placebo group, clinical trial. Stem Cell Res Ther (2022) 13:283. doi: 10.1186/s13287-022-02920-1
24. Xu X, Jiang W, Chen L, Xu Z, Zhang Q, Zhu M, et al. Evaluation of the safety and efficacy of using human menstrual blood-derived mesenchymal stromal cells in treating severe and critically ill COVID-19 patients: An exploratory clinical trial. Clin Transl Med (2021) 11:e297. doi: 10.1002/ctm2.297
25. Aghayan HR, Salimian F, Abedini A, Fattah Ghazi S, Yunesian M, Alavi-Moghadam S, et al. Human placenta-derived mesenchymal stem cells transplantation in patients with acute respiratory distress syndrome (ARDS) caused by COVID-19 (phase i clinical trial): Safety profile assessment. Stem Cell Res Ther (2022) 13:365. doi: 10.1186/s13287-022-02953-6
26. Shu L, Niu C, Li R, Huang T, Wang Y, Huang M, et al. Treatment of severe COVID-19 with human umbilical cord mesenchymal stem cells. Stem Cell Res Ther (2020) 11:361. doi: 10.1186/s13287-020-01875-5
27. Adas G, Cukurova Z, Yasar KK, Yilmaz R, Isiksacan N, Kasapoglu P, et al. The systematic effect of mesenchymal stem cell therapy in critical COVID-19 patients: A prospective double controlled trial. Cell Transplant (2021) 30:9636897211024942. doi: 10.1177/09636897211024942
28. Lanzoni G, Linetsky E, Correa D, Messinger Cayetano S, Alvarez RA, Kouroupis D, et al. Umbilical cord mesenchymal stem cells for COVID-19 acute respiratory distress syndrome: A double-blind, phase 1/2a, randomized controlled trial. Stem Cells Transl Med (2021) 10:660–73. doi: 10.1002/sctm.20-0472
29. Dilogo IH, Aditianingsih D, Sugiarto A, Burhan E, Damayanti T, Sitompul PA, et al. Umbilical cord mesenchymal stromal cells as critical COVID-19 adjuvant therapy: A randomized controlled trial. Stem Cells Transl Med (2021) 10:1279–87. doi: 10.1002/sctm.21-0046
30. Shi L, Huang H, Lu X, Yan X, Jiang X, Xu R, et al. Effect of human umbilical cord-derived mesenchymal stem cells on lung damage in severe COVID-19 patients: A randomized, double-blind, placebo-controlled phase 2 trial. Signal Transduct Target Ther (2021) 6:58. doi: 10.1038/s41392-021-00488-5
31. Fathi-Kazerooni M, Fattah-Ghazi S, Darzi M, Makarem J, Nasiri R, Salahshour F, et al. Safety and efficacy study of allogeneic human menstrual blood stromal cells secretome to treat severe COVID-19 patients: Clinical trial phase i & II. Stem Cell Res Ther (2022) 13:96. doi: 10.1186/s13287-022-02771-w
32. Karyana M, Djaharuddin I, Rif’ati L, Arif M, Choi MK, Angginy N, et al. Safety of DW-MSC infusion in patients with low clinical risk COVID-19 infection: A randomized, double-blind, placebo-controlled trial. Stem Cell Res Ther (2022) 13:134. doi: 10.1186/s13287-022-02812-4
33. Monsel A, Hauw-Berlemont C, Mebarki M, Heming N, Mayaux J, Nguekap Tchoumba O, et al. Treatment of COVID-19-associated ARDS with mesenchymal stromal cells: A multicenter randomized double-blind trial. Crit Care (2022) 26:48. doi: 10.1186/s13054-022-03930-4
34. Rebelatto CLK, Senegaglia AC, Franck CL, Daga DR, Shigunov P, Stimamiglio MA, et al. Safety and long-term improvement of mesenchymal stromal cell infusion in critically COVID-19 patients: A randomized clinical trial. Stem Cell Res Ther (2022) 13:122. doi: 10.1186/s13287-022-02796-1
35. Bowdish ME, Barkauskas CE, Overbey JR, Gottlieb RL, Osman K, Duggal A, et al. A randomized trial of mesenchymal stromal cells for moderate to severe acute respiratory distress syndrome from COVID-19. Am J Respir Crit Care Med (2023) 207:261–70. doi: 10.1164/rccm.202201-0157OC
36. Liu F, Li L, Xu M, Wu J, Luo D, Zhu Y, et al. Prognostic value of interleukin-6, c-reactive protein, and procalcitonin in patients with COVID-19. J Clin Virol (2020) 127:104370. doi: 10.1016/j.jcv.2020.104370
37. Schwartz KL, Wang J, Tadrous M, Langford BJ, Daneman N, Leung V, et al. Population-based evaluation of the effectiveness of nirmatrelvir-ritonavir for reducing hospital admissions and mortality from COVID-19. CMAJ: Can Med Assoc J (2023) 195:E220–6. doi: 10.1503/cmaj.221608
38. Wu M, Liu S, Wang C, Wu Y, Liu J. Risk factors for mortality among lung cancer patients with covid-19 infection: A systematic review and meta-analysis. PloS One (2023) 18:e0291178. doi: 10.1371/journal.pone.0291178
39. Nadkarni AR, Vijayakumaran SC, Gupta S, Divatia JV. Mortality in cancer patients with COVID-19 who are admitted to an ICU or who have severe COVID-19: A systematic review and meta-analysis. JCO Global Oncol (2021) 7:1286–305. doi: 10.1200/GO.21.00072
40. Wang Y, Zhang D, Du G, Du R, Zhao J, Jin Y, et al. Remdesivir in adults with severe COVID-19: A randomised, double-blind, placebo-controlled, multicentre trial. Lancet (London England) (2020) 395:1569–78. doi: 10.1016/S0140-6736(20)31022-9
41. Beigel JH, Tomashek KM, Dodd LE, Mehta AK, Zingman BS, Kalil AC, et al. Remdesivir for the treatment of covid-19 - final report. N Engl J Med (2020) 383:1813–26. doi: 10.1056/NEJMoa2007764
42. Gottlieb RL, Vaca CE, Paredes R, Mera J, Webb BJ, Perez G, et al. Early remdesivir to prevent progression to severe covid-19 in outpatients. N Engl J Med (2022) 386:305–15. doi: 10.1056/NEJMoa2116846
43. Selickman J, Vrettou CS, Mentzelopoulos SD, Marini JJ. COVID-19-related ARDS: Key mechanistic features and treatments. J Clin Med (2022) 11:4896. doi: 10.3390/jcm11164896
44. Zheng J, Miao J, Guo R, Guo J, Fan Z, Kong X, et al. Mechanism of COVID-19 causing ARDS: Exploring the possibility of preventing and treating SARS-CoV-2. Front Cell Infect Microbiol (2022) 12:931061. doi: 10.3389/fcimb.2022.931061
45. Lai D, Zhu K, Li S, Xiao Y, Xu Q, Sun Y, et al. SARS-CoV-2 n protein triggers acute lung injury via modulating macrophage activation and infiltration in in vitro and in vivo. J Inflammation Res (2023) 16:1867–77. doi: 10.2147/JIR.S405722
46. Stevens HY, Bowles AC, Yeago C, Roy K. Molecular crosstalk between macrophages and mesenchymal stromal cells. Front Cell Dev Biol (2020) 8:600160. doi: 10.3389/fcell.2020.600160
47. Jerkic M, Szaszi K, Laffey JG, Rotstein O, Zhang H. Key role of mesenchymal stromal cell interaction with macrophages in promoting repair of lung injury. Int J Mol Sci (2023) 24:3376. doi: 10.3390/ijms24043376
48. Hezam K, Wang C, Fu E, Zhou M, Liu Y, Wang H, et al. Superior protective effects of PGE2 priming mesenchymal stem cells against LPS-induced acute lung injury (ALI) through macrophage immunomodulation. Stem Cell Res Ther (2023) 14:48. doi: 10.1186/s13287-023-03277-9
49. Gotthardt D, Trifinopoulos J, Sexl V, Putz EM. JAK/STAT cytokine signaling at the crossroad of NK cell development and maturation. Front Immunol (2019) 10:2590. doi: 10.3389/fimmu.2019.02590
50. Lee MJ, Blish CA. Defining the role of natural killer cells in COVID-19. Nat Immunol (2023) 24:1628–38. doi: 10.1038/s41590-023-01560-8
51. Varchetta S, Mele D, Oliviero B, Mantovani S, Ludovisi S, Cerino A, et al. Unique immunological profile in patients with COVID-19. Cell Mol Immunol (2021) 18:604–12. doi: 10.1038/s41423-020-00557-9
52. Najar M, Fayyad-Kazan M, Merimi M, Burny A, Bron D, Fayyad-Kazan H, et al. Mesenchymal stromal cells and natural killer cells: A complex story of love and hate. Curr Stem Cell Res Ther (2019) 14:14–21. doi: 10.2174/1574888x13666180912125736
53. Sebastião AI, Mateus D, Carrascal MA, Sousa C, Cortes L, Bachmann MF, et al. CuMV VLPs containing the RBM from SARS-CoV-2 spike protein drive dendritic cell activation and Th1 polarization. Pharmaceutics (2023) 15:825. doi: 10.3390/pharmaceutics15030825
54. Liu X-Q, Peng Y-Q, Huang L-X, Li C-G, Kuang P-P, Chen D-H, et al. Dendritic cells mediated by small extracellular vesicles derived from MSCs attenuated the ILC2 activity via PGE2 in patients with allergic rhinitis. Stem Cell Res Ther (2023) 14:180. doi: 10.1186/s13287-023-03408-2
55. Xu L-L, Fu H-X, Zhang J-M, Feng F-E, Wang Q-M, Zhu X-L, et al. Impaired function of bone marrow mesenchymal stem cells from immune thrombocytopenia patients in inducing regulatory dendritic cell differentiation through the notch-1/jagged-1 signaling pathway. Stem Cells Dev (2017) 26:1648–61. doi: 10.1089/scd.2017.0078
56. Li X, Dong Y, Yin H, Qi Z, Wang D, Ren S. Mesenchymal stem cells induced regulatory dendritic cells from hemopoietic progenitor cells through notch pathway and TGF-β synergistically. Immunol Lett (2020) 222:49–57. doi: 10.1016/j.imlet.2020.03.005
57. Lu Z, Meng S, Chang W, Fan S, Xie J, Guo F, et al. Mesenchymal stem cells activate notch signaling to induce regulatory dendritic cells in LPS-induced acute lung injury. J Transl Med (2020) 18:241. doi: 10.1186/s12967-020-02410-z
58. Lu Z, Chang W, Meng S, Xu X, Xie J, Guo F, et al. Mesenchymal stem cells induce dendritic cell immune tolerance via paracrine hepatocyte growth factor to alleviate acute lung injury. Stem Cell Res Ther (2019) 10:372. doi: 10.1186/s13287-019-1488-2
59. Reis M, Mavin E, Nicholson L, Green K, Dickinson AM, Wang XN. Mesenchymal stromal cell-derived extracellular vesicles attenuate dendritic cell maturation and function. Front Immunol (2018) 9:2538. doi: 10.3389/fimmu.2018.02538
60. Guo R, Liang Q, He Y, Wang C, Jiang J, Chen T, et al. Mesenchymal stromal cells-derived extracellular vesicles regulate dendritic cell functions in dry eye disease. Cells (2022) 12:33. doi: 10.3390/cells12010033
61. Lee H, Jeong S, Shin E-C. Significance of bystander t cell activation in microbial infection. Nat Immunol (2022) 23:13–22. doi: 10.1038/s41590-021-00985-3
62. Bergamaschi L, Mescia F, Turner L, Hanson AL, Kotagiri P, Dunmore BJ, et al. Longitudinal analysis reveals that delayed bystander CD8+ t cell activation and early immune pathology distinguish severe COVID-19 from mild disease. Immunity (2021) 54:1257–1275.e8. doi: 10.1016/j.immuni.2021.05.010
63. Shakiba MH, Gemünd I, Beyer M, Bonaguro L. Lung t cell response in COVID-19. Front Immunol (2023) 14:1108716. doi: 10.3389/fimmu.2023.1108716
64. Yunis J, Short KR, Yu D. Severe respiratory viral infections: T-cell functions diverging from immunity to inflammation. Trends Microbiol (2023) 31:644–56. doi: 10.1016/j.tim.2022.12.008
65. Chen J, Zhang X, Xie J, Xue M, Liu L, Yang Y, et al. Overexpression of TGFβ1 in murine mesenchymal stem cells improves lung inflammation by impacting the Th17/treg balance in LPS-induced ARDS mice. Stem Cell Res Ther (2020) 11:311. doi: 10.1186/s13287-020-01826-0
66. Liao Y, Fu Z, Huang Y, Wu S, Wang Z, Ye S, et al. Interleukin-18-primed human umbilical cord-mesenchymal stem cells achieve superior therapeutic efficacy for severe viral pneumonia via enhancing t-cell immunosuppression. Cell Death Dis (2023) 14:66. doi: 10.1038/s41419-023-05597-3
67. Laidlaw BJ, Ellebedy AH. The germinal centre b cell response to SARS-CoV-2. Nat Rev Immunol (2021) 22:7–18. doi: 10.1038/s41577-021-00657-1
68. Woodruff MC, Ramonell RP, Nguyen DC, Cashman KS, Saini AS, Haddad NS, et al. Extrafollicular b cell responses correlate with neutralizing antibodies and morbidity in COVID-19. Nat Immunol (2020) 21:1506–16. doi: 10.1038/s41590-020-00814-z
69. Woodruff MC, Ramonell RP, Haddad NS, Anam FA, Rudolph ME, Walker TA, et al. Dysregulated naive b cells and de novo autoreactivity in severe COVID-19. Nature (2022) 611:139–47. doi: 10.1038/s41586-022-05273-0
70. Magatti M, Masserdotti A, Bonassi Signoroni P, Vertua E, Stefani FR, Silini AR, et al. B lymphocytes as targets of the immunomodulatory properties of human amniotic mesenchymal stromal cells. Front Immunol (2020) 11:1156. doi: 10.3389/fimmu.2020.01156
71. Liu J, Liu Q, Chen X. The immunomodulatory effects of mesenchymal stem cells on regulatory b cells. Front Immunol (2020) 11:1843. doi: 10.3389/fimmu.2020.01843
72. Feng B, Zhu J, Xu Y, Chen W, Sheng X, Feng X, et al. Immunosuppressive effects of mesenchymal stem cells on lung b cell gene expression in LPS-induced acute lung injury. Stem Cell Res Ther (2020) 11:418. doi: 10.1186/s13287-020-01934-x
73. Krygier A, Szmajda-Krygier D, Świechowski R, Pietrzak J, Wosiak A, Wodziński D, et al. Molecular pathogenesis of fibrosis, thrombosis and surfactant dysfunction in the lungs of severe COVID-19 patients. Biomolecules (2022) 12:1845. doi: 10.3390/biom12121845
74. Williams T, Salmanian G, Burns M, Maldonado V, Smith E, Porter RM, et al. Versatility of mesenchymal stem cell-derived extracellular vesicles in tissue repair and regenerative applications. Biochimie (2023) 207:33–48. doi: 10.1016/j.biochi.2022.11.011
75. Kim J, Kim EH, Lee H, Sung JH, Bang OY. Clinical-scale mesenchymal stem cell-derived extracellular vesicle therapy for wound healing. Int J Mol Sci (2023) 24:33–48. doi: 10.3390/ijms24054273
76. Halim NS, Aizat WM, Yahaya BH. The effect of mesenchymal stem cell-secreted factors on airway epithelial repair. Regener Med (2019) 14:15–31. doi: 10.2217/rme-2018-0020
77. Shologu N, Scully M, Laffey JG, O’Toole D. Human mesenchymal stem cell secretome from bone marrow or adipose-derived tissue sources for treatment of hypoxia-induced pulmonary epithelial injury. Int J Mol Sci (2018) 19:2996. doi: 10.3390/ijms19102996
78. Yang W, Huang C, Wang W, Zhang B, Chen Y, Xie X. Bone mesenchymal stem cell-derived exosomes prevent hyperoxia-induced apoptosis of primary type II alveolar epithelial cells in vitro. PeerJ (2022) 10:e13692. doi: 10.7717/peerj.13692
79. Hou L, Zhu Z, Jiang F, Zhao J, Jia Q, Jiang Q, et al. Human umbilical cord mesenchymal stem cell-derived extracellular vesicles alleviated silica induced lung inflammation and fibrosis in mice via circPWWP2A/miR-223-3p/NLRP3 axis. Ecotoxicol Environ Saf (2023) 251:114537. doi: 10.1016/j.ecoenv.2023.114537
80. Lei X, He N, Zhu L, Zhou M, Zhang K, Wang C, et al. Mesenchymal stem cell-derived extracellular vesicles attenuate radiation-induced lung injury via miRNA-214-3p. Antioxid Redox Signal (2021) 35:849–62. doi: 10.1089/ars.2019.7965
81. Li Y, Shen Z, Jiang X, Wang Y, Yang Z, Mao Y, et al. Mouse mesenchymal stem cell-derived exosomal miR-466f-3p reverses EMT process through inhibiting AKT/GSK3β pathway via c-MET in radiation-induced lung injury. J Exp Clin Cancer Res: CR (2022) 41:128. doi: 10.1186/s13046-022-02351-z
82. Liang B, Chen J, Li T, Wu H, Yang W, Li Y, et al. Clinical remission of a critically ill COVID-19 patient treated by human umbilical cord mesenchymal stem cells: A case report. Med (Baltimore) (2020) 99:e21429. doi: 10.1097/md.0000000000021429
83. Peng H, Gong T, Huang X, Sun X, Luo H, Wang W, et al. A synergistic role of convalescent plasma and mesenchymal stem cells in the treatment of severely ill COVID-19 patients: A clinical case report. Stem Cell Res Ther (2020) 11:291. doi: 10.1186/s13287-020-01802-8
84. Tang L, Jiang Y, Zhu M, Chen L, Zhou X, Zhou C, et al. Clinical study using mesenchymal stem cells for the treatment of patients with severe COVID-19. Front Med (2020) 14:664–73. doi: 10.1007/s11684-020-0810-9
85. Tao J, Nie Y, Wu H, Cheng L, Qiu Y, Fu J, et al. Umbilical cord blood-derived mesenchymal stem cells in treating a critically ill COVID-19 patient. J Infect Dev Ctries (2020) 14:1138–45. doi: 10.3855/jidc.13081
86. Zhang Y, Ding J, Ren S, Wang W, Yang Y, Li S, et al. Intravenous infusion of human umbilical cord wharton’s jelly-derived mesenchymal stem cells as a potential treatment for patients with COVID-19 pneumonia. Stem Cell Res Ther (2020) 11:207. doi: 10.1186/s13287-020-01725-4
87. Eckard AR, Borow KM, Mack EH, Burke E, Atz AM. Remestemcel-l therapy for COVID-19-associated multisystem inflammatory syndrome in children. Pediatrics (2021) 147:e2020046573. doi: 10.1542/peds.2020-046573
88. Yilmaz R, Adas G, Cukurova Z, Kart Yasar K, Isiksacan N, Oztel ON, et al. Mesenchymal stem cells treatment in COVID-19 patient with multi-organ involvement. Bratisl Lek Listy (2020) 121:847–52. doi: 10.4149/BLL_2020_139
89. Soler Rich R, Rius Tarruella J, Melgosa Camarero MT. Expanded mesenchymal stem cells: A novel therapeutic approach of SARS-CoV-2 pneumonia (COVID-19). Concepts regarding a first case in Spain. Med Clin (Barc) (2020) 155:318–9. doi: 10.1016/j.medcli.2020.06.018
90. Primorac D, Stojanovic Stipic S, Strbad M, Girandon L, Barlic A, Frankic M, et al. Compassionate mesenchymal stem cell treatment in a severe COVID-19 patient: A case report. Croat Med J (2021) 62:288–96. doi: 10.3325/cmj.2021.62.288
91. Putra A, Widyatmoko A, Ibrahim S, Amansyah F, Amansyah F, Berlian MA, et al. Case series of the first three severe COVID-19 patients treated with the secretome of hypoxia-mesenchymal stem cells in Indonesia. F1000Res (2021) 10:228. doi: 10.12688/f1000research.51191.2
92. Senegaglia AC, Rebelatto CLK, Franck CL, Lima JS, Boldrini-Leite LM, Daga DR, et al. Combined use of tocilizumab and mesenchymal stromal cells in the treatment of severe COVID-19: Case report. Cell Transplant (2021) 30:9636897211021008. doi: 10.1177/09636897211021008
93. Grumet M, Sherman J, Dorf BS. Efficacy of MSC in patients with severe COVID-19: Analysis of the literature and a case study. Stem Cells Transl Med (2022) 11:1103–12. doi: 10.1093/stcltm/szac067
94. Kim K, Bae KS, Kim HS, Lee WY. Effectiveness of mesenchymal stem cell therapy for COVID-19-induced ARDS patients: A case report. Medicina (Kaunas) (2022) 58:1698. doi: 10.3390/medicina58121698
95. Payandeh M, Habibi R, Norooznezhad AH, Hoseinkhani Z, Mansouri F, Yarani R, et al. Human placenta-derived mesenchymal stromal cells transfusion in a critically ill infant diagnosed with coronavirus disease 2019 (COVID-19): A case report. Transfus Apher Sci (2022) 61:103454. doi: 10.1016/j.transci.2022.103454
96. Sadeghi B, Ringden O, Gustafsson B, Castegren M. Mesenchymal stromal cells as treatment for acute respiratory distress syndrome. Case reports following hematopoietic cell transplantation and a review. Front Immunol (2022) 13:963445. doi: 10.3389/fimmu.2022.963445
97. Shi L, Yuan X, Yao W, Wang S, Zhang C, Zhang B, et al. Human mesenchymal stem cells treatment for severe COVID-19: 1-year follow-up results of a randomized, double-blind, placebo-controlled trial. EBioMedicine (2022) 75:103789. doi: 10.1016/j.ebiom.2021.103789
98. Rivera-Cruz CM, Shearer JJ, Figueiredo Neto M, Figueiredo ML. The immunomodulatory effects of mesenchymal stem cell polarization within the tumor microenvironment niche. Stem Cells Int (2017) 2017:4015039. doi: 10.1155/2017/4015039
99. Li G-C, Zhang H-W, Zhao Q-C, Sun LI, Yang J-J, Hong L, et al. Mesenchymal stem cells promote tumor angiogenesis via the action of transforming growth factor β1. Oncol Lett (2016) 11:1089–94. doi: 10.3892/ol.2015.3997
100. Martin FT, Dwyer RM, Kelly J, Khan S, Murphy JM, Curran C, et al. Potential role of mesenchymal stem cells (MSCs) in the breast tumour microenvironment: Stimulation of epithelial to mesenchymal transition (EMT). Breast Cancer Res Treat (2010) 124:317–26. doi: 10.1007/s10549-010-0734-1
101. Liu S, Ginestier C, Ou SJ, Clouthier SG, Patel SH, Monville F, et al. Breast cancer stem cells are regulated by mesenchymal stem cells through cytokine networks. Cancer Res (2011) 71:614–24. doi: 10.1158/0008-5472.CAN-10-0538
102. Xuan X, Tian C, Zhao M, Sun Y, Huang C. Mesenchymal stem cells in cancer progression and anticancer therapeutic resistance. Cancer Cell Int (2021) 21:595. doi: 10.1186/s12935-021-02300-4
103. Vianello F, Villanova F, Tisato V, Lymperi S, Ho K-K, Gomes AR, et al. Bone marrow mesenchymal stromal cells non-selectively protect chronic myeloid leukemia cells from imatinib-induced apoptosis via the CXCR4/CXCL12 axis. Haematologica (2010) 95:1081–9. doi: 10.3324/haematol.2009.017178
104. Lis R, Touboul C, Mirshahi P, Ali F, Mathew S, Nolan DJ, et al. Tumor associated mesenchymal stem cells protects ovarian cancer cells from hyperthermia through CXCL12. Int J Cancer (2011) 128:715–25. doi: 10.1002/ijc.25619
105. Zhang G, Miao F, Xu J, Wang R. Mesenchymal stem cells from bone marrow regulate invasion and drug resistance of multiple myeloma cells by secreting chemokine CXCL13. Bosnian J Basic Med Sci (2020) 20:209–17. doi: 10.17305/bjbms.2019.4344
106. Li H-J, Reinhardt F, Herschman HR, Weinberg RA. Cancer-stimulated mesenchymal stem cells create a carcinoma stem cell niche via prostaglandin E2 signaling. Cancer Discov (2012) 2:840–55. doi: 10.1158/2159-8290.CD-12-0101
107. Røsland GV, Svendsen A, Torsvik A, Sobala E, McCormack E, Immervoll H, et al. Long-term cultures of bone marrow-derived human mesenchymal stem cells frequently undergo spontaneous Malignant transformation. Cancer Res (2009) 69:5331–9. doi: 10.1158/0008-5472.CAN-08-4630
108. Wang Y, Yi H, Song Y. The safety of MSC therapy over the past 15 years: A meta-analysis. Stem Cell Res Ther (2021) 12:545. doi: 10.1186/s13287-021-02609-x
Keywords: severe pneumonia, COVID-19, mesenchymal stem cells, lung cancer, immune modulation, tissue repairment
Citation: Huang X, Tan X, Xie X, Jiang T, Xiao Y and Liu Z (2024) Successful salvage of a severe COVID-19 patient previously with lung cancer and radiation pneumonitis by mesenchymal stem cells: a case report and literature review. Front. Immunol. 15:1321236. doi: 10.3389/fimmu.2024.1321236
Received: 13 October 2023; Accepted: 04 January 2024;
Published: 06 February 2024.
Edited by:
Michal Holub, Charles University, CzechiaCopyright © 2024 Huang, Tan, Xie, Jiang, Xiao and Liu. This is an open-access article distributed under the terms of the Creative Commons Attribution License (CC BY). The use, distribution or reproduction in other forums is permitted, provided the original author(s) and the copyright owner(s) are credited and that the original publication in this journal is cited, in accordance with accepted academic practice. No use, distribution or reproduction is permitted which does not comply with these terms.
*Correspondence: Zenghui Liu, MTAzMDQyNTRAcXEuY29t
†These authors have contributed equally to this work and share first authorship