- 1Department of Acupuncture and Moxibustion, Shenzhen Luohu Hospital of Traditional Chinese Medicine (Shenzhen Hospital of Shanghai University of Traditional Chinese Medicine), Shenzhen, China
- 2The Brain Cognition and Brain Disease Institute (BCBDI), Shenzhen Institute of Advanced Technology, Chinese Academy of Sciences, Shenzhen, China
- 3Shenzhen-Hong Kong Institute of Brain Science-Shenzhen Fundamental Research Institutions, Shenzhen, China
- 4Institute of Acupuncture and Moxibustion, China Academy of Chinese Medical Science, Beijing, China
Ischemic stroke (IS) is one of the leading causes of death and disability. Complicated mechanisms are involved in the pathogenesis of IS. Immunomodulatory mechanisms are crucial to IS. Acupuncture is a traditional non-drug treatment that has been extensively used to treat IS. The exploration of neuroimmune modulation will broaden the understanding of the mechanisms underlying acupuncture treatment. This review summarizes the immune response of immune cells, immune cytokines, and immune organs after an IS. The immunomodulatory mechanisms of acupuncture treatment on the central nervous system and peripheral immunity, as well as the factors that influence the effects of acupuncture treatment, were summarized. We suggest prospects and future directions for research on immunomodulatory mechanisms of acupuncture treatment for IS based on current progress, and we hope that these will provide inspiration for researchers. Additionally, acupuncture has shown favorable outcomes in the treatment of immune-based nervous system diseases, generating new directions for research on possible targets and treatments for immune-based nervous system diseases.
1 Introduction
Ischemic stroke (IS) is an acute cerebrovascular disease in which blood cannot be supplied to the brain due to a sudden blockage of cerebral vessels (1). IS continues to be the third-leading cause of mortality and disability worldwide, accounting for 5.7% of all deaths and 11.6% of all disabilities worldwide (2). IS survivors often suffer from disabilities that cause a poor quality of life and a heavy economic burden (3). In addition, several comorbidities caused by IS, such as melancholy, epilepsy, exhaustion, and pain, further impair the quality of life of patients (4–7).
An increasing number of studies have shown that the immune response is a double-edged sword in the pathophysiology of IS and has become a breakthrough target in treatment strategies (8). IS induces a series of peripheral and central immune reactions that are crucial to the pathophysiology of development, acute damage cascades, and the chronic course after an IS (9). The acute and chronic phases of IS and the long-term effects of stroke are greatly affected by the immune system (10). The immune response after IS involves innate and adaptive immunity in the central and peripheral nervous systems (11). Targeting regulatory immunity is a promising approach for reducing stroke-related dysfunction.
Acupuncture is a traditional non-pharmacological treatment that dates back thousands of years. It uses needles to penetrate the skin at specific acupoints on the body, head, and ears. Patients with IS receive acupuncture as a complementary medical treatment in various countries, including China, Korea, and the USA (12, 13). Numerous lines of evidence indicated that acupuncture was effective and safe for IS and its complications (14, 15). In addition, acupuncture has been shown to modulate immune responses, particularly neuroimmune responses (16, 17). However, no review has outlined the immunomodulatory mechanisms underlying the effects of acupuncture in the treatment of IS.
In this review, we summarize the immune response following an IS, as well as the immunomodulatory effects of acupuncture in the treatment of IS and its consequences. In addition, we propose some new considerations for the future research direction of acupuncture for IS to provide a new strategy for stroke rehabilitation based on neuroimmune.
2 Immune responses after an ischemic stroke
2.1 Immune cell response after an ischemic stroke
Immune cells are the primary components of the central immune system. Immune cells play a vital role in regulating the progression of IS. These immune cells include microglia, astrocytes, oligodendrocytes (OLs), neutrophils, T cells, B cells, dendritic cells, and macrophages.
2.1.1 Microglia—the first line of defense
Microglia are frequently found as resident immune cells in the central nervous system (CNS) and affect pathological processes such as neuronal healing, antigen presentation, and poststroke inflammation (18). The functions of microglia and monocytes/macrophages in cleaning debris, triggering neuroinflammation, causing tissue damage, and encouraging tissue recovery are believed to be a double-edged sword (19). After an IS, with the release of damage-associated molecular patterns (DAMPs) and cytokines, the microglia are activated and react. The activation of microglia from the brain and macrophages from the blood composes the first line of defense, which is the innate immune response (20). The two main types of microglia, M1-like and M2-like, can be distinguished based on their biological role and the cytokines and chemokines they secrete (21). Two types of microglia play different roles. Clearing cellular debris, secreting anti-inflammatory substances, promoting angiogenesis, enhancing angiogenesis, and synaptic remodeling are performed by M2-like microglia (22, 23). M2-like microglia inhibited inflammatory damage to protect the blood–brain barrier (BBB) by releasing interleukin (IL)-4, IL-10, and transforming growth factor-β1 (TGF-β1) (24). Ischemic neurons cause M1-like microglia to become polarized, and M1-like microglia release proinflammatory substances that wreak havoc in the nervous system and harm neurons (25). M1-like microglia play a hazardous role after IS, such as damaging the BBB and aggravating the harmful inflammation response (26). Most of the activated microglia in the subacute and chronic phases of stroke are M1-like, which is detrimental to brain regeneration, while M2-like microglia predominate in the first 24 h following stroke (27, 28). Some research has verified that the regulation of microglial polarization can be a promising target to treat IS (29, 30).
2.1.2 Astrocytes—double-edged sword for modulation
The most prevalent glial cell type in the CNS, astrocytes, has an essential function (31). Similar to microglia, damaged brain tissues with the secretion of cytokines and DAMPs stimulated the receptors of astrocytes to differentiate phenotype (32). Astrocytes are actived into two polarization states after an IS, named A1 and A2, facilitating the understanding of the reactive state of astrocytes. Numerous processes are related to astrocytes, including homeostasis, neuronal and synaptic development, control of cerebral blood flow, and vascular remodeling and repair (33, 34). A2-subtype astrocytes secrete substances that facilitate the recovery of neurons, including neurotrophic factors, glutamic acid, homocysteine, and cholesterol (35). Nevertheless, A1-subtype astrocyte development is detrimental to neurons, as it prevents effective axonal regeneration (36). M1-like microglia can promote astrocyte activation (34). More in vivo and in vitro research should be conducted to explore astrocyte pathways in the case of IS.
2.1.3 Oligodendrocytes—for preserving axonal integrity
OLs, which create myelin, are discovered in white matter (37). When OLs are affected by ischemic damage, OLs produce tissue demyelination and axonal instability, resulting in various neurological deficits (38). Damaged OLs were replaced to restore saltatory conduction and relieve motor function after an IS. Increasing myelin production, augmenting synaptogenesis, promoting oligodendrogenesis, promoting OLs maturation, and suppressing remyelination are the strategies to target OLs for the treatment of IS (39).
2.1.4 Neutrophils—infiltration from blood
Neutrophils are the first immune cells transported to the CNS from the peripheral immune system after stroke (40). The enhancement in neutrophils results in the disruption of cerebral edema, brain injury, and the BBB after an IS (41). Neutrophils also impact thrombosis and atherosclerosis, which are high risk factors of IS (42, 43). Neutrophils invade the brain during the hyperacute, acute, and subacute phases (44). Importantly, circulating neutrophils are related to stroke severity, stroke outcomes, and stroke prognosis (45). The responses of neutrophils after an IS include three parts: (1) neutrophil activation and recruitment, (2) neutrophil adhesion to endothelial cells, and (3) neutrophil transmigration and neurovascular interactions (46). Cytokines, chemokines, and DAMPs act on neutrophils, which cause the recruitment of neutrophils to the site of injury and the delivery of adhesion molecules (47). Neutrophils are key modulators of IS injury. Thus, researchers show a great interest in neutrophils as treatment targets to prevent IS and relieve ischemic brain injury. The processes of neutrophil recruitment and transmigration can also be potential targets to influence stroke severity in detail (48).
2.1.5 T lymphocytes—their conflicting roles in ischemic stroke
T lymphocytes, which are adaptive immune cells, exacerbate ischemic–reperfusion injury in acute IS, in both an antigen-dependent and an antigen-independent manner (49). T lymphocytes release interferon-gamma (IFN-γ), IL-21, and IL-17, which cause harmful effects in IS (50). On the basis of cell functions, T cells are divided into multiple types marked by CD3 expression, including CD8+ cytotoxic T lymphocytes, CD4+ T helper cells, regulatory T cells (Tregs), and γδ T cells (51). CD4+ T cells are the main effector T cells, which modulate brain inflammation through releasing cytokines after an IS (52). CD4+ T cells play a double-edged role in inflammation regulation after a stroke, with anti-inflammatory and proinflammatory effects. CD8+ T cells play a cytotoxic role and promote cell apoptosis by recognizing antigens on T-cell receptors and releasing granzyme and perforin subsequently after an IS (53). Tregs are a type of T cell that influences immune responses after an IS (54). Tregs secrete anti-inflammatory cytokines, inhibit proinflammatory cytokines, induce cell lysis, promote neural regeneration, and modulate microglial and macrophage polarization after an IS (54). It is currently uncertain if Tregs are helpful or harmful in IS, as well as how they affect IS at different phases. Peripheral γδ T cells infiltrated the lesion site after an IS and aggravated BBB injury (55). Numerous γδ T cells infiltrate the ischemic penumbra, and knockout of γδ T cells relieves motor dysfunction and significantly reduces infarction and BBB damage (55). Though the major function of T cells has already been discovered, their specific role and the mechanism of T cells after a stroke need more attention.
In conclusion, the summary of the type and function of immune cells in IS is shown in Table 1.
2.2 Immune cytokines’ responses after an ischemic stroke
Immune cytokines secreted by immune cells are promising prognostic and predictive biomarkers for predicting stroke outcomes and therapeutic targets in patients with IS (56). They affect infarct and edema volumes (57). The classification of immune cytokines includes proinflammatory and anti-inflammatory cytokines on the basis of their function.
2.2.1 Proinflammatory cytokines
The proinflammatory cytokines mediate brain tissue destruction after an IS. Tumor necrosis factor (TNF), IL-1β, IL-6, IFN-γ, and IL-17 are classical and well-known proinflammatory cytokines (58). TNF-α is produced by various immune cell types and is related to various physiological events, including endothelial necroptosis, breakdown of the BBB, and the results of stroke (26). After a stroke, the expression of TNF-α, IL-1β, and IL-6 increased, aggravating the brain injury (59). IFN-γ, which is a proinflammatory cytokine, is pivotal for activating microglia into neurotoxic phenotypes that induce oxidative stress, severe dysfunction, and neuron death (60). The level of IL-17 increased in patients with IS. IL-17 accelerates neutrophil recruitment to the ischemic hemisphere (61).
2.2.2 Anti-inflammatory cytokines
IL-10, TGF-β1, and IL-4 are classical anti-inflammatory cytokines that mediate neuroprotection. IL-10 has a role in clear vascular endothelial protective properties and neuronal protection after brain injury (62). Once the level of TGF-β1 was upregulated, the apoptosis of neurons was suppressed (63). IL-4 modulates the proliferation of B cells and T cells, and the differentiation of B cells. Enhancing signaling through the IL-4 receptor may alleviate inflammation after ischemia and attenuate sensorimotor and cognitive deficits (64).
2.2.3 Multiple effects cytokines
The current study considers that IL-21 is an anti-inflammatory cytokine after an IS. IL-21R-deficient mice had reduced collateral vascular connections and increased brain infarct volume after ischemic brain injury. Moreover, the IL-21 receptor exerts neuroprotective effects in IS mice (65). However, IL-21 promotes the proinflammatory effects of macrophages during respiratory infection (66).
2.3 Immune organ responses after an ischemic stroke
Immune-related organs include the spleen, bone marrow, thymus, lymph nodes, lungs, liver, adrenal glands, and gut (67). The bone marrow exudes plenty of proinflammatory cells and cytokines, resulting in exacerbating peripheral inflammation (68). The gut changes intestinal dysbiosis to affect peripheral inflammation via regulating the gut–brain axis (69). The liver metabolism takes part in immunosuppression, inflammation, and oxidative stress after an IS (70). IS causes pulmonary damage, harmful inflammation, and reduction of alveolar macrophage phagocytic capability, which is related to the brain–lung axis (71). Most studies focus on the changes in the spleen and thymus after an IS, but the research depth is insufficient. Additionally, the function and change of bone marrow, lymph glands, lungs, and intestines after an IS should receive more attention.
2.3.1 The spleen
Spleen weight and size dramatically decreased in middle cerebral artery occlusion (MCAO) rats and mice, and spleen atrophy and cell death occurred after ischemia (72–74). The number of serum leukocytes is adversely linked to changes in spleen size in patients (75). At 24 h after ischemia, MCAO rats with splenectomies had lower levels of proinflammatory cytokines, fewer T cells, neutrophils, and macrophages in the brain tissue, and higher levels of anti-inflammatory factor IL-10, resulting in a smaller infarct size in the brain (76).
2.3.2 The thymus
At 3, 7, and 13 days after reperfusion, the number of CD8α+ T cells in the spleen and thymus of 90-min ischemia–reperfusion (I/R) rats decreased significantly, the TUNEL+ apoptotic cells in the spleen increased significantly, and the numbers of Iba1+ macrophages, CD68+ macrophages, and Ki67+ proliferating cells decreased significantly in the spleen and thymus of 90-min I/R rats and increased in brain tissue (77). The number of CD3+ T cells in the spleen and thymus of 30-min I/R mice was enhanced 24 h after stroke (78). The number of microglia, the level of TNF-α, and the inflammatory microbiota in the spleen and thymus of 60-min I/R rats increased significantly (79).
3 The mechanism of acupuncture treatment regulating immunity in ischemic stroke
In this review, acupuncture treatment includes electroacupuncture (EA) and manual acupuncture (MA). Acupuncture treatment stimulates acupoints to modulate the neuroendocrine–immune network (80). When dealing with various pathological conditions, acupuncture treatment can modulate immune system function, immune cell motivation and phenotypic metamorphosis, and immune cytokine expression. In this section, the mechanism of acupuncture treatment on immunity after an IS was extracted and summarized. As shown in Tables 2, 3, authors, animal model, acupoint selection, acupuncture method, stimulation parameter, treatment course, and neuroimmune molecular and cellular results were extracted and summarized (Tables 2, 3).
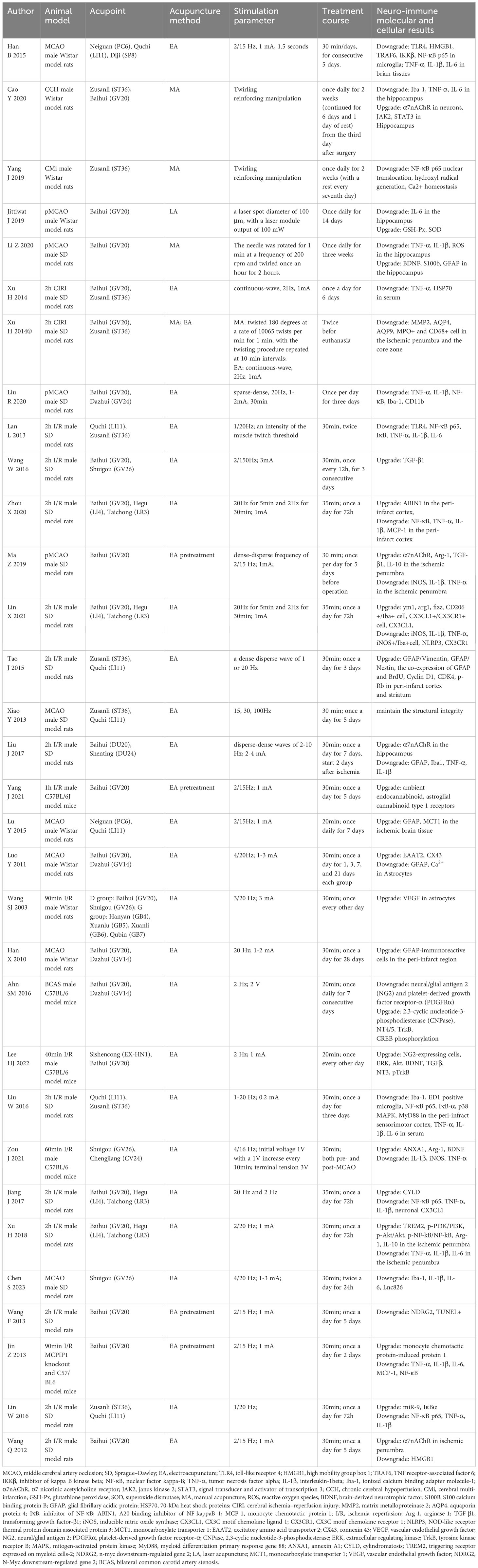
Table 2 Summary of studies exploring the effects of acupuncture treatment on ischemic stroke by modulating the central nervous system.
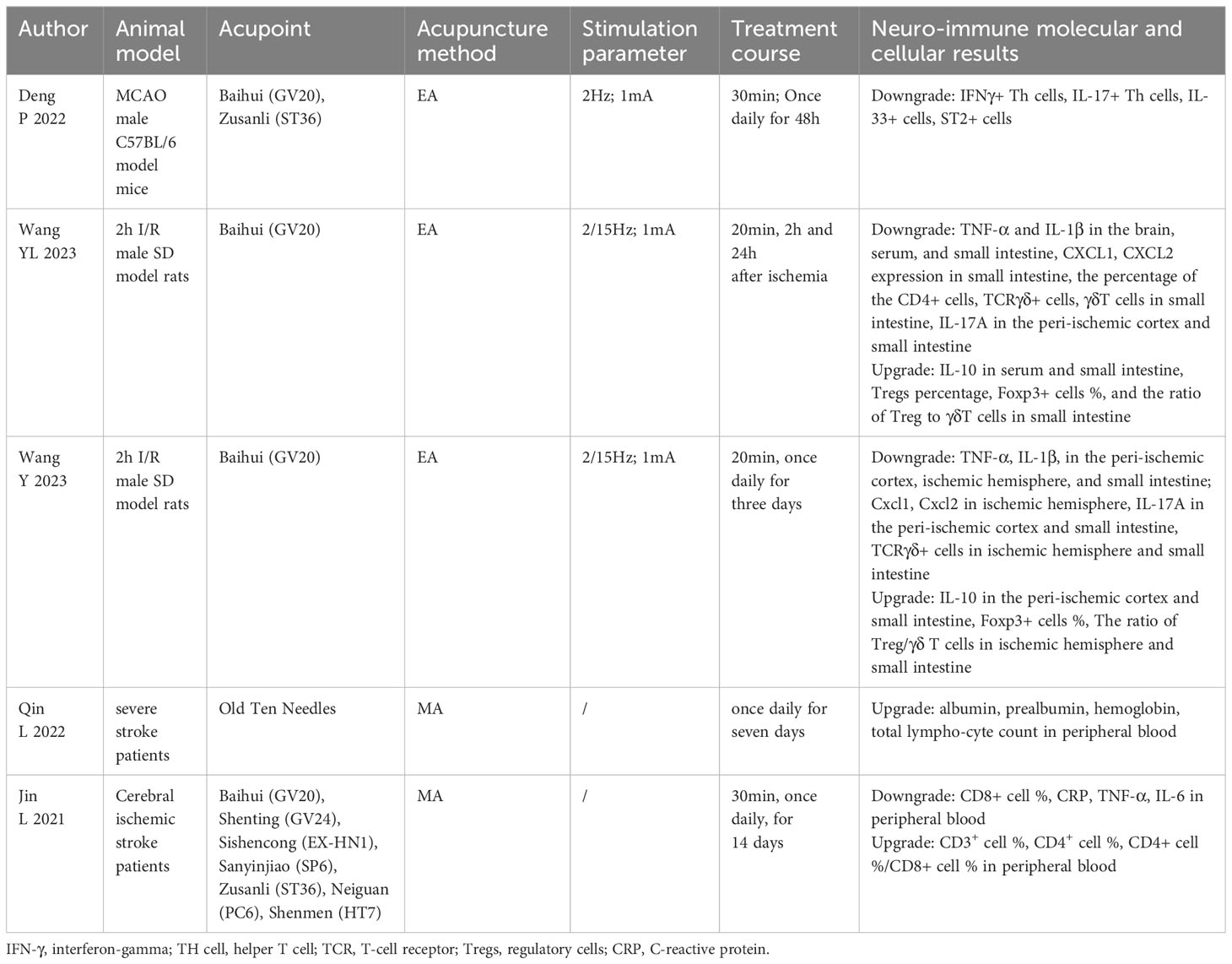
Table 3 Summary of studies exploring the effects of acupuncture treatment on ischemic stroke by modulating peripheral immunity.
3.1 The immunomodulatory effect of acupuncture treatment on the central nervous system
3.1.1 The immunomodulatory effect of acupuncture treatment on immunocytes
3.1.1.1 Microglia
Acupuncture improves stroke by modulating microglial polarization and morphology. Moreover, acupuncture treatment regulates microglial markers, receptors, and signaling pathways. A study showed that EA at GV26 and CV24 relieved neurological deficits by enhancing the expression of annexin A1 and its receptor formyl peptide receptor, promoting M2-like microglia polarization and the levels of arginase-1 (Arg-1) and brain-derived neurotrophic factor (BDNF), and reducing the levels of IL-1β, inducible nitric oxide synthase (iNOS), and TNF-α in the cerebral cortex (81). The nuclear factor kappa-B (NF-κB) is a key nuclear transcription factor, which plays a crucial role in the inflammation and immune responses of cells (82). EA at GV20 and GV24 regulated brain injury-induced inflammation by inhibiting NF-κB activation and decreasing the expression of ionized calcium binding adapter molecule-1 (Iba-1), CD11b, TNF-α, and IL-1β, indicating that EA suppressed the activation of microglia and the inflammatory response in brain tissues (83). EA at LI11 and ST36 reduced infarct volume by attenuating the overactivation of Iba-1 and ED1-positive microglia in the peri-infract sensorimotor cortex, decreasing the level of TNF-α, IL-1β, and IL-6 in serum, and preventing the nucleus translocation of NF-κB p65 and the expression of p38 mitogen-activated protein kinase (p38 MAPK) and myeloid differentiation primary response gene 88 (MyD88) in the peri-infract sensorimotor cortex. These outcomes indicated that EA recovered motor impairment by inhibiting microglia-mediated neuroinflammation (84). EA at PC6, LI11, and SP8 in MCAO rats suppressed the toll-like receptor 4/NF-κB (TLR4/NF-κB) signaling pathway to alleviate microglia activation and inflammation in brain tissues (85). EA at GV20, LI4, and LR3 inhibits neuroinflammatory damage by increasing the A20-binding inhibitor of NF-kappaB 1 (ABIN1) and suppressing NF-κB activation in the peri-infarct cortex, mediating the modulation of microglial polarization (86). EA activated the microglia-specific receptor triggering receptor expressed in myeloid cells 2 (TREM2) through PI3K/AKT and NF-κB signaling pathways to decrease inflammation in the CNS (87).
The cholinergic-anti-inflammatory pathway is the efferent arm of the inflammatory reflex, which mediates the prevention of systemic inflammation by stimulating the vagus nerve (88). The cholinergic anti-inflammatory system involves neuroimmune interactions that produce systemic and anti-inflammatory effects through the α7 nicotinic acetylcholine receptor (α7nAChR). EA pretreatment reduced infarct volume and improved neurological deficits by activating the α7nAChR-mediated phenotypic conversion of microglia, thus reducing the inflammatory response in the ischemic penumbra (89). MA at ST36 and GV20 promoted cognitive function and protected neurons in CCH rats via downregulating the expression of TNF-α and IL-6 and reducing the number of microglia in the hippocampus by activating the janus kinase 2/signal transducer and activator of transcription 3 (JAK2/STAT3) pathway by targeting α7nAChR (90). EA pretreatment inhibits high mobility group box 1 (HMGB1) release by α7nAChR activation in I/R rats (91).
Crosstalk between microglia and neurons is a potential target for neuroimmune regulation (92). EA at GV20, LI4, and LR3 plays anti-inflammatory and neuroprotective roles by upregulating cylindromatosis (CYLD) and CX3C motif chemokine ligand 1 (CX3CL1) expression and downregulating CX3C motif chemokine receptor 1 (CX3CR1) expression, suggesting that the crosstalk between microglia and neurons is regulated. Therefore, M1-like microglial polarization is suppressed, and M2-like microglia polarization is prompted (93). Jiang et al. found that EA at GV20, LI4, and LR3 relieved neurological deficits by upregulating CYLD in neurons, preventing the nucleus translocation of NF-κB p65 in neurons and microglia polarization, and downregulating the expression of TNF-α, IL-1β, and neuronal CX3CL1 in the peri-ischemic area (94). EA at GV26 modulates microglial polarization through the Lnc826-mediated hippo/YAP pathway in the cortex (95).
Above all, M1-like microglia is suppressed, M2-like microglia is prompted, and the crosstalk between microglia and neurons is regulated by acupuncture treatment stimulation. In addition, acupuncture treatment inhibits the proinflammatory pathway and activates the anti-inflammatory pathway to modulate the phenotype and morphology of microglia and microglial markers and receptors. Subsequently, the proinflammatory cytokines secreted by microglia decreased and the anti-inflammatory cytokines secreted by microglia increased. The regulation of microglia in the CNS by acupuncture treatment for the treatment of IS through multiple targets is shown in Figure 1.
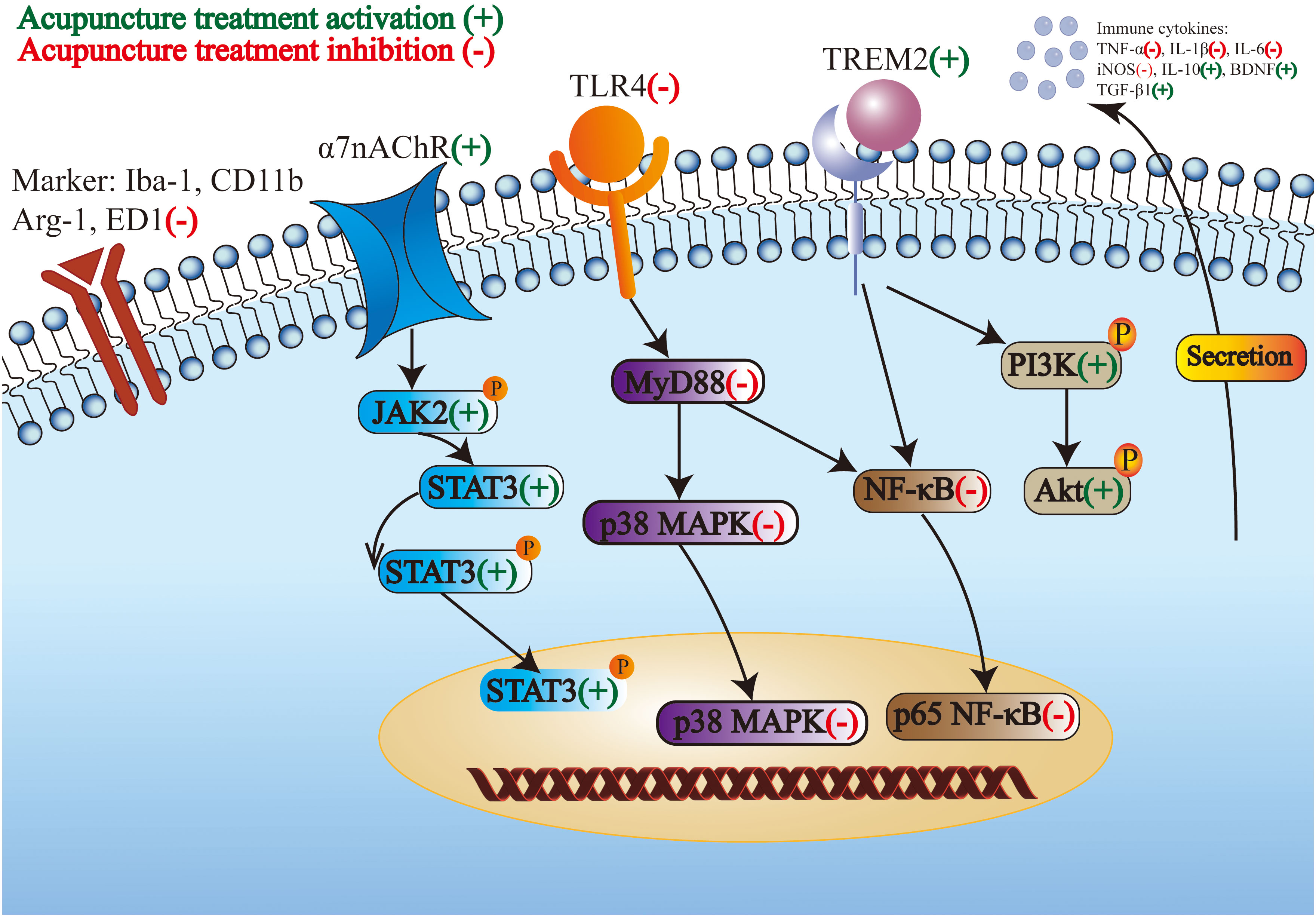
Figure 1 The regulation of microglia in the central nervous system by acupuncture treatment for the treatment of ischemic stroke through multiple targets.
3.1.1.2 Astrocytes
EA improves stroke by regulating the proliferation of astrocytes and the cytokines secreted by astrocytes. EA at ST36 and LI11 maintains the structural integrity of astrocytes in brain tissue (96). Owing to the special double-edged sword function of astrocytes, two opposing results were observed. EA at GV20 and GV14 recovered post-ischemic behavioral dysfunction by activating astrocytes and preventing excess reactive gliosis (97). EA at ST36 and LI11 exerted neuroprotective effects by enhancing the proliferation of astrocytes and increasing the secretion of BDNF from reactive astrocytes in the peri-infarct cortex and striatum (98). Therefore, more research should be conducted to explore the conditions required for astrocyte activation or inhibition. EA at DU20 and DU24 reduced the neuroinflammatory response by activating α7nAChR and decreasing the number of astrocytes (99). EA pretreatment at GV20 upregulated ambient endocannabinoid (eCB) expression and activated ischemic penumbral astroglial cannabinoid type 1 receptors (CB1R) in the ischemic penumbra, protecting neurons from ischemia (100). EA at PC6 and LI11 activated lactate metabolism in resident astrocytes for the use of injured neurons around the ischemic area by enhancing lactate transporter (monocarboxylate transporter 1, MCT1) expression (101). EA at GV20 and GV14 regulated the activation of astrocytes, increased the expression of excitatory amino acid transporter-2 (EAAT2) and connexin 43 (CX43), decreased Ca2+ in astrocytes, and promoted beneficial interactions between astrocytes and synapses in brain tissues (102). EA at GB4, GB5, GB6, and GB7 enhanced the induction of vascular endothelial growth factor in astrocytes of the peri-infarct area after reperfusion (103). EA pretreatment at GV20 attenuated the expression and cellular translocation of the N-Myc downstream-regulated gene 2 (NDRG2) in astrocytes in the ischemic penumbra (104).
In summary, the proliferation and structural integrity of astrocytes were enhanced, excess reactive gliosis is prevented, and the release of beneficial neurotransmitters secreted by astrocytes increased through acupuncture treatment stimulation. Receptors and pathways in astrocytes play an important anti-inflammatory role in these effects.
3.1.1.3 Neutrophils, monocytes/macrophages, and oligodendrocytes
The following studies focused on the regulation of neutrophils, monocytes, macrophages, and OLs. EA and MA at GV20 and ST36 exerted neuroprotective actions in MCAO rats by decreasing the number of immune cells, including neutrophils and monocytes/macrophages (105). EA pretreatment at GV20 reduced inflammatory cytokine production and leukocyte infiltration by inducing the expression of monocyte chemotactic protein-induced protein 1 (MCPIP1) in monocytes in brain tissues (106).
EA at EX-HN1 increased the percentage of CC1-positive cells, indicating that EA promotes the survival and differentiation of OLs (107). EA at GV20 and GV14 ameliorated memory impairment by strengthening OL differentiation from OL precursor cells and by upregulating the Neurotrophin 4/5-tyrosine kinase receptor B (NT4/5-TrkB) pathway in OLs (108).
Few studies focused on the effects of neutrophils, monocytes, macrophages, and OLs after an IS stimulated by acupuncture treatment. These immune cells were believed to be promising targets for the treatment of IS. Hence, these immune cells deserve further study in the future.
3.1.2 Acupuncture treatment effects on immune cytokines
Studies have shown that cytokines are the core mediators of neuroimmune regulatory mechanisms (109, 110). Acupuncture bidirectionally modulates the release of immune cytokines, forming a complex network with multiple effects on IS. Acupuncture regulates immune cytokines from immune cells to control multiple immune responses after an IS. The scalp acupuncture at GV20 was determined to counteract ischemic brain injury through downregulation of TNF-α and IL-1b in the hippocampi (111). EA at GV20 and ST36 exerted neuroprotective properties in CIRI rats by reducing TNF-α and 70-kDa heat shock proteins (HSP70) (112). EA at GV20 and GV26 improved neurological function by increasing the serum level of TGF-β1 (113). LA at GV20 alleviated cognitive and motor deficits by reducing the expression of IL-6 and improving glutathione peroxidase (GSH-Px) and superoxide dismutase (SOD) activity in the hippocampus (114).
The NF-κB signaling pathway is a well-known nuclear transcription factor and the most studied proinflammatory biomarker and target of IS. MA at ST36 protected cognitive function in cerebral multi-infarction rats by suppressing NF-κB p65 nuclear translocation in hippocampal tissues (115). EA at ST36 and LI11 prevented neuroinflammation by regulating the miR-9-mediated NF-κB signaling pathway in the ischemic cortex (116). EA at LI11 and ST36 on the paralyzed limb showed neuroprotective activity by exhibiting the TLR4/NF-κB pathway (117).
Hence, proinflammatory cytokines were reduced, anti-inflammatory cytokines were upregulated, and the proinflammatory signaling pathway was suppressed by acupuncture treatment stimulation.
3.2 The immunomodulatory effect of acupuncture treatment on peripheral immunity
Acupuncture treatment regulates peripheral immunity, especially immune cells in the peripheral blood and immune cells in the spleen. EA at GV20 and ST36 improved motor function and brain damage in MCAO rats by decreasing IFNγ+ Th cells and IL-17+ Th cells in spleen tissues and peripheral blood (118). EA at GV20 alleviated cerebral injury by reducing the level of TNF-α and IL-1β in the brain, serum, and small intestine; reducing IL-17A, CXCL1, and CXCL2 expression, and the percentage of CD4+ cells, TCRγδ+ cells, and γδT cells in the small intestine; increasing the level of IL-10 in the serum and small intestine; and increasing Tregs percentage and the ratio of Treg to γδT cells in the small intestine (119, 120). Those studies indicated that EA regulated the differentiation of T-cell subsets in the small intestine and promoted the balance of Treg/γδ T cells toward Tregs. MA increased the total lymphocyte count in IS patient’s peripheral blood (121). MA increased IS patient’s CD8+ cell percentage and the level of CRP, TNF-α, and IL-6 in peripheral blood, and decreased CD3+ cell percentage, CD4+ cell percentage, the ratio of CD4+ cell percentage, and CD8+ cell percentage in peripheral blood (122). Therefore, major studies focus on suppressing harmful T cells in spleen tissues and peripheral blood, decreasing the expression of proinflammatory cytokines in serum and the small intestine, increasing the expression of anti-inflammatory cytokines in serum and the small intestine, and increasing the beneficial T cells in peripheral blood.
Above all, the central and peripheral immune modulation by acupuncture treatment for the treatment of IS is shown in Figure 2.
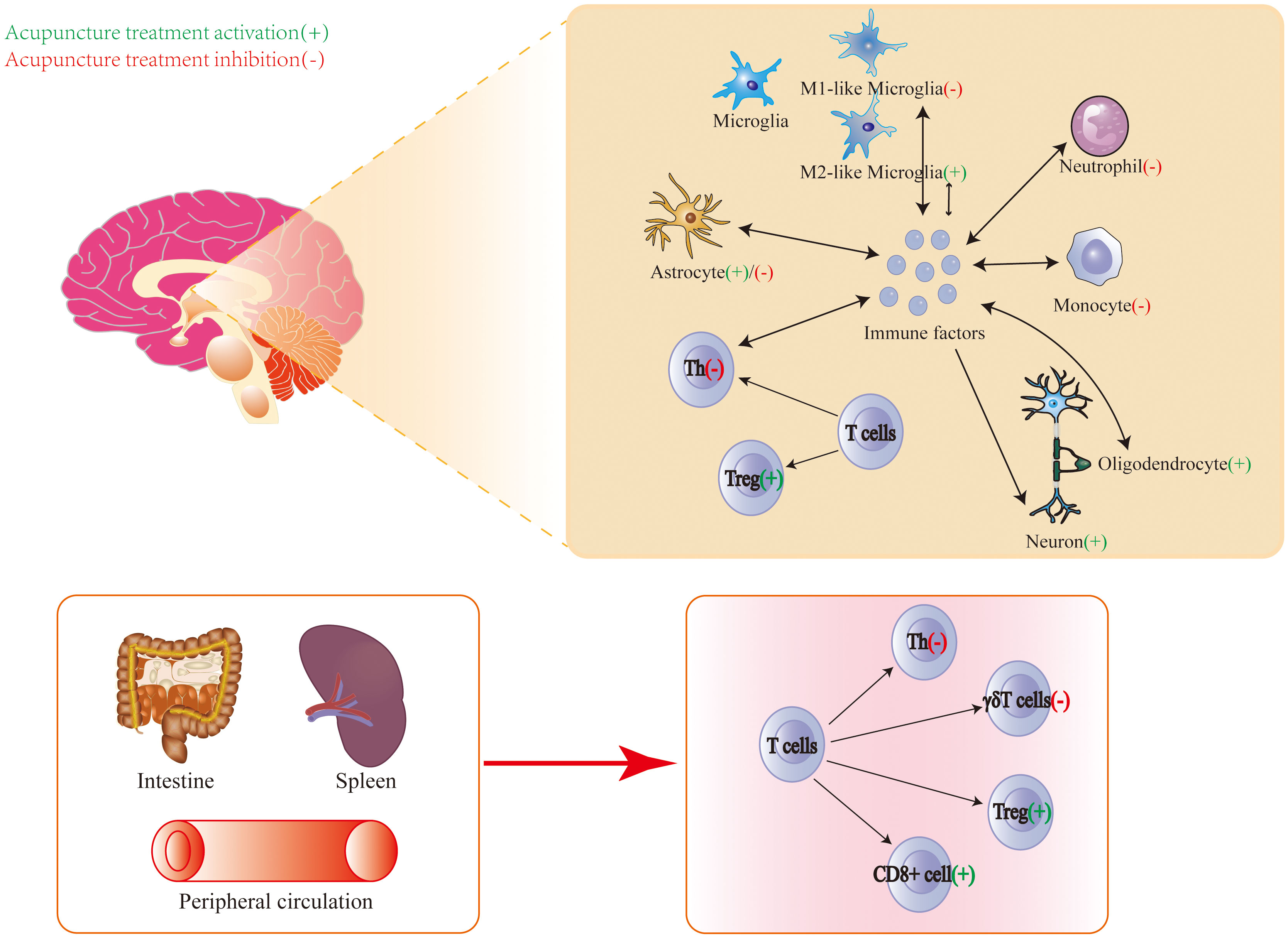
Figure 2 Central and peripheral immune modulation by acupuncture treatment for the treatment of ischemic stroke.
4 Factors influencing acupuncture treatment effect
The operation time of acupuncture is an important factor that influences the efficacy of acupuncture treatment. Gao et al. found that for stroke patients, acupuncture was more effective in the morning than in the afternoon (123). The effect of EA at GV20 and GV14 in the subacute phase was better than that of EA in the acute phase (124). Needle retention time had a significant effect on the efficacy of acupuncture treatment. He et al. considered the time–effect relationship between needle retention time and acupuncture efficacy for patients with IS (125). The efficacy of the 60-min needle retention time was better than that of the 40-min and 20-min needle retention times (125). A similar conclusion was reached in MCAO rats. When comparing neural function recovery, neurobehavioral scores were greater in the 48-h group than in the 12-h group and in the 24-h group (126). The output frequency of the EA instrument affects its efficacy. The efficacy of EA at 15 and 30 Hz was better than the efficacy of EA at 100 Hz in MCAO rats (96). The perceived stimulation intensity of MA had a differential effect on cerebral activation and cardiovascular reflex response (127). Additionally, EA and MA have different efficacy for IS. The efficacy of EA is better than the efficacy of MA for recovering nerve defects, improving quality of life (128), and strengthening limb motor function (129).
Acupoints are associated with the specificity of the acupuncture effect, and acupoint application is one of the factors that influence efficacy. MA at Neiguan (PC6) for 60 s was more effective than that at 5 s and 180 s in MCAO rats (130). The EA at Hanyan (GB4), Xuanlu (GB5), Xuanli (GB6), and Qubin (GB7) showed more significant recovery than at Baihui (GV20) and Shuigou (GV26) (103). Another study showed that acupuncture at Neiguan (PC6) was more effective than acupuncture at Chize (LU5) and Shuigou (GV26) (131). Zhang et al. used cluster analysis to illustrate that the Neiguan (PC6), Weizhong (BL40), Chize (LU5), Sanyinjiao (SP6), and Shuigou (GV26) groups had the most valid and suitable acupuncture parameters (132). Acupoint selection in the current study was unsystematic, because little attention was paid for the accurate stimulation of neurons. More research is needed to explore the most effective acupoints to accurately stimulate the corresponding neural signaling pathways.
Overall, different types of acupuncture treatment, duration, operation time, retention, output frequency, and acupoint selection will affect the effect of acupuncture treatment.
5 Prospect and future direction
Immune responses are complicated cascade reactions after an IS. However, current studies about immune organs, cells, and cytokine responses remain shallow. The immune mechanisms of IS are appealing and deserve to be explored. For example, myeloid cells that take on a foamy appearance are a key driver of the inflammatory response after an IS. Targeting lipid accumulation in foam cells may be a promising strategy for accelerating recovery from IS (133).
A phase III trial indicated that the tumor necrosis factor receptor-immunoglobulin G1, which controls a single inflammatory cytokine, was ineffective in the incidence or resolution of organ dysfunction (134). Programmed cell death protein 1 (PD-1) inhibitors, which are immune checkpoint inhibitors, can regulate cellular immunity in patients with cancer. However, PD-1 inhibitors cause endocrine autoimmune diseases, gastrointestinal discomfort, hepatotoxicity, rashes, and thyroid dysfunction at the same time (135). The inflammatory cascade reaction after ischemia is produced by multiple cytokines and not by a single cytokine; therefore, successful treatment of IS requires inhibition of multiple cytokines. Acupuncture improves disease by modulating the release of multiple biomolecules into the microenvironment, where they can activate the neuroendocrine–immune network to achieve holistic modulation (136). Therefore, acupuncture is a promising potential treatment for IS through the regulation of the immune network. The underlying mechanisms of acupuncture in IS require further exploration.
The vagus nerve and hypothalamic–pituitary–adrenal (HPA) axis are important components of neuroimmune interactions because they control immune function and inflammatory responses. The hypothesis of acupuncture neuroimmune regulation in the IS model by eliciting the vagal–adrenal and spinal–sympathetic axes has been raised. Ma et al. confirmed the neuroanatomy of EA at ST36 with low intensity driving the vagal–adrenal axis to produce anti-inflammatory effects (137), while EA at ST25 with high intensity activates NPY splenic noradrenergic neurons via the spinal–sympathetic axis (138). In particular, the current intensity is critical. EA at ST36 improved inflammatory arthritis in Lyme disease-susceptible C3H mice by stimulating the sciatic–vagal nerve (139). Since current studies on the neuroimmune regulation mechanism in acupuncture treatment for IS have not focused on the vagus nerve, HPA axis, or splenic sympathetic nerve, they will be a promising target.
Natural killer (NK) cells are cytotoxic lymphocytes of the innate immune system. Acupuncture also regulates NK cells by modulating the expression of the NK cell receptor CD94, protein tyrosine kinase, adhesion molecule vascular cell adhesion molecule-1 (VCAM-1), and protein tyrosine phosphatase (140). There has been no research on the mechanism of acupuncture that focuses on the modulation of NK cells. Therefore, NK cells are a promising target for exploration.
Immunoregulators can be used to treat IS, offering a new therapeutic approach to IS treatment. Acupuncture aims to restore and maintain the dynamic equilibrium of the immune system instead of strengthening or inhibiting the immune system. These evidences show that acupuncture is a potential adjunct to immune regulation in IS, which has not been previously proposed in published reviews.
6 Conclusion
Acupuncture treatment is an ancient non-drug treatment, and it shows fewer side effects and beneficial therapeutic effects. This review summarizes the progress in understanding the immunomodulatory mechanisms of acupuncture treatment for IS and provides a theoretical framework for further mechanism research. In summary, the above evidences suggest that acupuncture has the potential to modulate the immune response induced by IS, which is related to its neuroprotective effects. The exploration of the mechanisms of acupuncture promotes its global impact.
Author contributions
HK: Conceptualization, Investigation, Writing – original draft. XZ: Funding acquisition, Methodology, Supervision, Writing – review & editing. HC: Supervision, Writing – review & editing. HT: Data curation, Writing – original draft. HZ: Funding acquisition, Investigation, Methodology, Writing – review & editing.
Funding
The author(s) declare financial support was received for the research, authorship, and/or publication of this article. This study was funded by the Shenzhen Science and Technology Program (Grant Numbers JCYJ20220818100803007 and JCYJ20210324120804012).
Acknowledgments
We would like to thank Editage (www.editage.cn) for English language editing.
Conflict of interest
The authors declare that the research was conducted in the absence of any commercial or financial relationships that could be construed as a potential conflict of interest.
Publisher’s note
All claims expressed in this article are solely those of the authors and do not necessarily represent those of their affiliated organizations, or those of the publisher, the editors and the reviewers. Any product that may be evaluated in this article, or claim that may be made by its manufacturer, is not guaranteed or endorsed by the publisher.
References
2. GBD 2019 Stroke Collaborators. Global, regional, and national burden of stroke and its risk factors, 1990-2019: a systematic analysis for the Global Burden of Disease Study 2019. Lancet Neurol. (2021) 20:795–820. doi: 10.1016/s1474-4422(21)00252-0
3. Cerniauskaite M, Quintas R, Koutsogeorgou E, Meucci P, Sattin D, Leonardi M, et al. Quality-of-life and disability in patients with stroke. Am J Phys Med rehabilitation. (2012) 91:S39–47. doi: 10.1097/PHM.0b013e31823d4df7
4. De Doncker W, Dantzer R, Ormstad H, Kuppuswamy A. Mechanisms of poststroke fatigue. J neurology neurosurgery Psychiatry. (2018) 89:287–93. doi: 10.1136/jnnp-2017-316007
6. Altman K, Shavit-Stein E, Maggio N. Post stroke seizures and epilepsy: from proteases to maladaptive plasticity. Front Cell Neurosci. (2019) 13:397. doi: 10.3389/fncel.2019.00397
7. Medeiros GC, Roy D, Kontos N, Beach SR. Post-stroke depression: A 2020 updated review. Gen Hosp Psychiatry. (2020) 66:70–80. doi: 10.1016/j.genhosppsych.2020.06.011
8. Chauhan C, Kaundal RK. The role of cGAS-STING signaling in ischemic stroke: From immune response to therapeutic targeting. Drug Discovery Today. (2023) 28:103792. doi: 10.1016/j.drudis.2023.103792
9. Endres M, Moro MA, Nolte CH, Dames C, Buckwalter MS, Meisel A. Immune pathways in etiology, acute phase, and chronic sequelae of ischemic stroke. Circ Res. (2022) 130:1167–86. doi: 10.1161/circresaha.121.319994
10. Iadecola C, Buckwalter MS, Anrather J. Immune responses to stroke: mechanisms, modulation, and therapeutic potential. J Clin Invest. (2020) 130:2777–88. doi: 10.1172/jci135530
11. Shi SX, Vodovoz SJ, Xiu Y, Liu N, Jiang Y, Katakam PVG, et al. T-lymphocyte interactions with the neurovascular unit: implications in intracerebral hemorrhage. Cells. (2022) 11. doi: 10.3390/cells11132011
12. Chang H, Kwon YD, Yoon SS. Use of acupuncture therapy as a supplement to conventional medical treatments for acute ischaemic stroke patients in an academic medical centre in Korea. Complementary therapies Med. (2011) 19:256–63. doi: 10.1016/j.ctim.2011.07.003
13. Gong CZ, Liu W. Acupuncture and the opioid epidemic in america. Chin J Integr Med. (2018) 24:323–7. doi: 10.1007/s11655-018-2989-z
14. Zhang S, Wu B, Liu M, Li N, Zeng X, Liu H, et al. Acupuncture efficacy on ischemic stroke recovery: multicenter randomized controlled trial in China. Stroke. (2015) 46:1301–6. doi: 10.1161/strokeaha.114.007659
15. Jiang C, Yang S, Tao J, Huang J, Li Y, Ye H, et al. Clinical efficacy of acupuncture treatment in combination with rehaCom cognitive training for improving cognitive function in stroke: A 2 × 2 factorial design randomized controlled trial. J Am Med Directors Assoc. (2016) 17:1114–22. doi: 10.1016/j.jamda.2016.07.021
16. Gong Y, Li N, Lv Z, Zhang K, Zhang Y, Yang T, et al. The neuro-immune microenvironment of acupoints-initiation of acupuncture effectiveness. J leukocyte Biol. (2020) 108:189–98. doi: 10.1002/jlb.3ab0420-361rr
17. Li N, Guo Y, Gong Y, Zhang Y, Fan W, Yao K, et al. The anti-inflammatory actions and mechanisms of acupuncture from acupoint to target organs via neuro-immune regulation. J Inflammation Res. (2021) 14:7191–224. doi: 10.2147/jir.S341581
18. Arcuri C, Mecca C, Bianchi R, Giambanco I, Donato R. The pathophysiological role of microglia in dynamic surveillance, phagocytosis and structural remodeling of the developing CNS. Front Mol Neurosci. (2017) 10:191. doi: 10.3389/fnmol.2017.00191
19. Dudvarski Stankovic N, Teodorczyk M, Ploen R, Zipp F, Schmidt MHH. Microglia-blood vessel interactions: a double-edged sword in brain pathologies. Acta neuropathologica. (2016) 131:347–63. doi: 10.1007/s00401-015-1524-y
20. Xiong XY, Liu L, Yang QW. Functions and mechanisms of microglia/macrophages in neuroinflammation and neurogenesis after stroke. Prog neurobiology. (2016) 142:23–44. doi: 10.1016/j.pneurobio.2016.05.001
21. Xu S, Lu J, Shao A, Zhang JH, Zhang J. Glial cells: role of the immune response in ischemic stroke. Front Immunol. (2020) 11:294. doi: 10.3389/fimmu.2020.00294
22. Wang J, Xing H, Wan L, Jiang X, Wang C, Wu Y. Treatment targets for M2 microglia polarization in ischemic stroke. Biomedicine pharmacotherapy = Biomedecine pharmacotherapie. (2018) 105:518–25. doi: 10.1016/j.biopha.2018.05.143
23. Var SR, Shetty AV, Grande AW, Low WC, Cheeran MC. Microglia and macrophages in neuroprotection, neurogenesis, and emerging therapies for stroke. Cells. (2021) 10. doi: 10.3390/cells10123555
24. Candelario-Jalil E, Dijkhuizen RM, Magnus T. Neuroinflammation, stroke, blood-brain barrier dysfunction, and imaging modalities. Stroke. (2022) 53:1473–86. doi: 10.1161/strokeaha.122.036946
25. Jurga AM, Paleczna M, Kuter KZ. Overview of general and discriminating markers of differential microglia phenotypes. Front Cell Neurosci. (2020) 14:198. doi: 10.3389/fncel.2020.00198
26. Chen AQ, Fang Z, Chen XL, Yang S, Zhou YF, Mao L, et al. Microglia-derived TNF-α mediates endothelial necroptosis aggravating blood brain-barrier disruption after ischemic stroke. Cell Death disease. (2019) 10:487. doi: 10.1038/s41419-019-1716-9
27. Cherry JD, Olschowka JA, O'Banion MK. Neuroinflammation and M2 microglia: the good, the bad, and the inflamed. J neuroinflammation. (2014) 11:98. doi: 10.1186/1742-2094-11-98
28. Kolosowska N, Keuters MH, Wojciechowski S, Keksa-Goldsteine V, Laine M, Malm T, et al. Peripheral administration of IL-13 induces anti-inflammatory microglial/macrophage responses and provides neuroprotection in ischemic stroke. Neurother J Am Soc Exp NeuroTherapeutics. (2019) 16:1304–19. doi: 10.1007/s13311-019-00761-0
29. Zhang W, Xu H, Li C, Han B, Zhang Y. Exploring Chinese herbal medicine for ischemic stroke: insights into microglia and signaling pathways. Front Pharmacol. (2024) 15:1333006. doi: 10.3389/fphar.2024.1333006
30. Zhang W, Tian T, Gong SX, Huang WQ, Zhou QY, Wang AP, et al. Microglia-associated neuroinflammation is a potential therapeutic target for ischemic stroke. Neural regeneration Res. (2021) 16:6–11. doi: 10.4103/1673-5374.286954
31. Sofroniew MV, Vinters HV. Astrocytes: biology and pathology. Acta neuropathologica. (2010) 119:7–35. doi: 10.1007/s00401-009-0619-8
32. Fan YY, Huo J. A1/A2 astrocytes in central nervous system injuries and diseases: Angels or devils? Neurochemistry Int. (2021) 148:105080. doi: 10.1016/j.neuint.2021.105080
33. Williamson MR, Fuertes CJA, Dunn AK, Drew MR, Jones TA. Reactive astrocytes facilitate vascular repair and remodeling after stroke. Cell Rep. (2021) 35:109048. doi: 10.1016/j.celrep.2021.109048
34. Shen XY, Gao ZK, Han Y, Yuan M, Guo YS, Bi X. Activation and role of astrocytes in ischemic stroke. Front Cell Neurosci. (2021) 15:755955. doi: 10.3389/fncel.2021.755955
35. Allen NJ, Eroglu C. Cell biology of astrocyte-synapse interactions. Neuron. (2017) 96:697–708. doi: 10.1016/j.neuron.2017.09.056
36. Sims NR, Yew WP. Reactive astrogliosis in stroke: Contributions of astrocytes to recovery of neurological function. Neurochemistry Int. (2017) 107:88–103. doi: 10.1016/j.neuint.2016.12.016
37. Hernández IH, Villa-González M, Martín G, Soto M, Pérez-Álvarez MJ. Glial cells as therapeutic approaches in brain ischemia-reperfusion injury. Cells. (2021) 10. doi: 10.3390/cells10071639
38. Dewar D, Underhill SM, Goldberg MP. Oligodendrocytes and ischemic brain injury. J Cereb Blood Flow Metab. (2003) 23:263–74. doi: 10.1097/01.Wcb.0000053472.41007.F9
39. Huang S, Ren C, Luo Y, Ding Y, Ji X, Li S. New insights into the roles of oligodendrocytes regulation in ischemic stroke recovery. Neurobiol disease. (2023) 184:106200. doi: 10.1016/j.nbd.2023.106200
40. Wanrooy BJ, Wen SW, Wong CH. Dynamic roles of neutrophils in post-stroke neuroinflammation. Immunol Cell Biol. (2021) 99:924–35. doi: 10.1111/imcb.12463
41. Ansari J, Gavins FNE. Neutrophils and platelets: immune soldiers fighting together in stroke pathophysiology. Biomedicines. (2021) 9. doi: 10.3390/biomedicines9121945
42. Kapoor S, Opneja A, Nayak L. The role of neutrophils in thrombosis. Thromb Res. (2018) 170:87–96. doi: 10.1016/j.thromres.2018.08.005
43. Döring Y, Drechsler M, Soehnlein O, Weber C. Neutrophils in atherosclerosis: from mice to man. Arteriosclerosis thrombosis Vasc Biol. (2015) 35:288–95. doi: 10.1161/atvbaha.114.303564
44. Beuker C, Strecker JK, Rawal R, Schmidt-Pogoda A, Ruck T, Wiendl H, et al. Immune cell infiltration into the brain after ischemic stroke in humans compared to mice and rats: a systematic review and meta-analysis. Trans stroke Res. (2021) 12:976–90. doi: 10.1007/s12975-021-00887-4
45. El Amki M, Glück C, Binder N, Middleham W, Wyss MT, Weiss T, et al. Neutrophils obstructing brain capillaries are a major cause of no-reflow in ischemic stroke. Cell Rep. (2020) 33:108260. doi: 10.1016/j.celrep.2020.108260
46. Dimasi D, Sun WY, Bonder CS. Neutrophil interactions with the vascular endothelium. Int immunopharmacology. (2013) 17:1167–75. doi: 10.1016/j.intimp.2013.05.034
47. Metzemaekers M, Gouwy M, Proost P. Neutrophil chemoattractant receptors in health and disease: double-edged swords. Cell Mol Immunol. (2020) 17:433–50. doi: 10.1038/s41423-020-0412-0
48. Jickling GC, Liu D, Ander BP, Stamova B, Zhan X, Sharp FR. Targeting neutrophils in ischemic stroke: translational insights from experimental studies. J Cereb Blood Flow Metab. (2015) 35:888–901. doi: 10.1038/jcbfm.2015.45
49. Stoll G, Nieswandt B. Thrombo-inflammation in acute ischaemic stroke - implications for treatment. Nat Rev Neurology. (2019) 15:473–81. doi: 10.1038/s41582-019-0221-1
50. Rayasam A, Kijak JA, Kissel L, Choi YH, Kim T, Hsu M, et al. CXCL13 expressed on inflamed cerebral blood vessels recruit IL-21 producing T(FH) cells to damage neurons following stroke. J neuroinflammation. (2022) 19:125. doi: 10.1186/s12974-022-02490-2
51. Lei TY, Ye YZ, Zhu XQ, Smerin D, Gu LJ, Xiong XX, et al. The immune response of T cells and therapeutic targets related to regulating the levels of T helper cells after ischaemic stroke. J neuroinflammation. (2021) 18:25. doi: 10.1186/s12974-020-02057-z
52. Liu Y, Dong J, Zhang Z, Liu Y, Wang Y. Regulatory T cells: A suppressor arm in post-stroke immune homeostasis. Neurobiol disease. (2023) 189:106350. doi: 10.1016/j.nbd.2023.106350
53. Cai W, Shi L, Zhao J, Xu F, Dufort C, Ye Q, et al. Neuroprotection against ischemic stroke requires a specific class of early responder T cells in mice. J Clin Invest. (2022) 132. doi: 10.1172/jci157678
54. Wang H, Wang Z, Wu Q, Yuan Y, Cao W, Zhang X. Regulatory T cells in ischemic stroke. CNS Neurosci Ther. (2021) 27:643–51. doi: 10.1111/cns.13611
55. Dong X, Zhang X, Li C, Chen J, Xia S, Bao X, et al. γδ T cells aggravate blood-brain-barrier injury via IL-17A in experimental ischemic stroke. Neurosci letters. (2022) 776:136563. doi: 10.1016/j.neulet.2022.136563
56. Doll DN, Barr TL, Simpkins JW. Cytokines: their role in stroke and potential use as biomarkers and therapeutic targets. Aging disease. (2014) 5:294–306. doi: 10.14336/ad.2014.0500294
57. Martha SR, Cheng Q, Fraser JF, Gong L, Collier LA, Davis SM, et al. Expression of cytokines and chemokines as predictors of stroke outcomes in acute ischemic stroke. Front neurology. (2019) 10:1391. doi: 10.3389/fneur.2019.01391
58. Zhu H, Hu S, Li Y, Sun Y, Xiong X, Hu X, et al. Interleukins and ischemic stroke. Front Immunol. (2022) 13:828447. doi: 10.3389/fimmu.2022.828447
59. Mo ZT, Zheng J, Liao YL. Icariin inhibits the expression of IL-1β, IL-6 and TNF-α induced by OGD/R through the IRE1/XBP1s pathway in microglia. Pharm Biol. (2021) 59:1473–9. doi: 10.1080/13880209.2021.1991959
60. Kann O, Almouhanna F, Chausse B. Interferon γ: a master cytokine in microglia-mediated neural network dysfunction and neurodegeneration. Trends neurosciences. (2022) 45:913–27. doi: 10.1016/j.tins.2022.10.007
61. Backes FN, de Souza A, Bianchin MM. IL-23 and IL-17 in acute ischemic stroke: Correlation with stroke scales and prognostic value. Clin Biochem. (2021) 98:29–34. doi: 10.1016/j.clinbiochem.2021.09.003
62. Garcia JM, Stillings SA, Leclerc JL, Phillips H, Edwards NJ, Robicsek SA, et al. Role of interleukin-10 in acute brain injuries. Front neurology. (2017) 8:244. doi: 10.3389/fneur.2017.00244
63. Zhu H, Gui Q, Hui X, Wang X, Jiang J, Ding L, et al. TGF-β1/smad3 signaling pathway suppresses cell apoptosis in cerebral ischemic stroke rats. Med Sci monitor Int Med J Exp Clin Res. (2017) 23:366–76. doi: 10.12659/msm.899195
64. Huang Y, Li K, Dai Q, Pang H, Xu Z, Luo X, et al. SCH 644343 alleviates ischemic stroke-induced neuroinflammation by promoting microglial polarization via the IL-4/SREBP-1 signaling pathway. Eur J Pharmacol. (2023) 958:176033. doi: 10.1016/j.ejphar.2023.176033
65. Lee HK, Keum S, Sheng H, Warner DS, Lo DC, Marchuk DA. Natural allelic variation of the IL-21 receptor modulates ischemic stroke infarct volume. J Clin Invest. (2016) 126:2827–38. doi: 10.1172/jci84491
66. Yang S, Zeng J, Hao W, Sun R, Tuo Y, Tan L, et al. IL-21/IL-21R Promotes the Pro-Inflammatory Effects of Macrophages during C. muridarum Respiratory Infection. Int J Mol Sci. (2023) 24. doi: 10.3390/ijms241612557
67. Godinho-Silva C, Cardoso F, Veiga-Fernandes H. Neuro-immune cell units: A new paradigm in physiology. Annu Rev Immunol. (2019) 37:19–46. doi: 10.1146/annurev-immunol-042718-041812
68. Courties G, Herisson F, Sager HB, Heidt T, Ye Y, Wei Y, et al. Ischemic stroke activates hematopoietic bone marrow stem cells. Circ Res. (2015) 116:407–17. doi: 10.1161/circresaha.116.305207
69. Xie X, Wang L, Dong S, Ge S, Zhu T. Immune regulation of the gut-brain axis and lung-brain axis involved in ischemic stroke. Neural regeneration Res. (2024) 19:519–28. doi: 10.4103/1673-5374.380869
70. Inderhees J, Schwaninger M. Liver metabolism in ischemic stroke. Neuroscience. (2024). doi: 10.1016/j.neuroscience.2023.12.013
71. Samary CS, Ramos AB, Maia LA, Rocha NN, Santos CL, Magalhães RF, et al. Focal ischemic stroke leads to lung injury and reduces alveolar macrophage phagocytic capability in rats. Crit Care (London England). (2018) 22:249. doi: 10.1186/s13054-018-2164-0
72. Ajmo CT Jr., Collier LA, Leonardo CC, Hall AA, Green SM, Womble TA, et al. Blockade of adrenoreceptors inhibits the splenic response to stroke. Exp neurology. (2009) 218:47–55. doi: 10.1016/j.expneurol.2009.03.044
73. Jin R, Zhu X, Liu L, Nanda A, Granger DN, Li G. Simvastatin attenuates stroke-induced splenic atrophy and lung susceptibility to spontaneous bacterial infection in mice. Stroke. (2013) 44:1135–43. doi: 10.1161/strokeaha.111.000633
74. Yu H, Cai Y, Zhong A, Zhang Y, Zhang J, Xu S. The "Dialogue" Between central and peripheral immunity after ischemic stroke: focus on spleen. Front Immunol. (2021) 12:792522. doi: 10.3389/fimmu.2021.792522
75. Sahota P, Vahidy F, Nguyen C, Bui TT, Yang B, Parsha K, et al. Changes in spleen size in patients with acute ischemic stroke: a pilot observational study. Int J stroke. (2013) 8:60–7. doi: 10.1111/ijs.12022
76. Zhang BJ, Men XJ, Lu ZQ, Li HY, Qiu W, Hu XQ. Splenectomy protects experimental rats from cerebral damage after stroke due to anti-inflammatory effects. Chin Med J (Engl). (2013) 126:2354–60.
77. Tan C, Wang Z, Zheng M, Zhao S, Shichinohe H, Houkin K. Responses of immune organs after cerebral ischemic stroke. J Nippon Med School = Nippon Ika Daigaku zasshi. (2021) 88:228–37. doi: 10.1272/jnms.JNMS.2021_88-308
78. Glab JA, Puthalakath H, Zhang SR, Vinh A, Drummond GR, Sobey CG, et al. Bim deletion reduces functional deficits following ischemic stroke in association with modulation of apoptosis and inflammation. Neuromolecular Med. (2022) 24:405–14. doi: 10.1007/s12017-022-08703-4
79. Kingsbury C, Shear A, Heyck M, Sadanandan N, Zhang H, Gonzales-Portillo B, et al. Inflammation-relevant microbiome signature of the stroke brain, gut, spleen, and thymus and the impact of exercise. J Cereb Blood Flow Metab. (2021) 41:3200–12. doi: 10.1177/0271678x211039598
80. Ding SS, Hong SH, Wang C, Guo Y, Wang ZK, Xu Y. Acupuncture modulates the neuro-endocrine-immune network. QJM monthly J Assoc Physicians. (2014) 107:341–5. doi: 10.1093/qjmed/hct196
81. Zou J, Huang GF, Xia Q, Li X, Shi J, Sun N. Electroacupuncture promotes microglial M2 polarization in ischemic stroke via annexin A1. Acupunct Med. (2022) 40:258–67. doi: 10.1177/09645284211057570
82. Lawrence T. The nuclear factor NF-kappaB pathway in inflammation. Cold Spring Harbor Perspect Biol. (2009) 1:a001651. doi: 10.1101/cshperspect.a001651
83. Liu R, Xu NG, Yi W, Ji C. Electroacupuncture attenuates inflammation after ischemic stroke by inhibiting NF-κB-mediated activation of microglia. Evidence-Based complementary Altern Med eCAM. (2020) 2020:8163052. doi: 10.1155/2020/8163052
84. Liu W, Wang X, Yang S, Huang J, Xue X, Zheng Y, et al. Electroacupunctre improves motor impairment via inhibition of microglia-mediated neuroinflammation in the sensorimotor cortex after ischemic stroke. Life Sci. (2016) 151:313–22. doi: 10.1016/j.lfs.2016.01.045
85. Han B, Lu Y, Zhao H, Wang Y, Li L, Wang T. Electroacupuncture modulated the inflammatory reaction in MCAO rats via inhibiting the TLR4/NF-κB signaling pathway in microglia. Int J Clin Exp Pathol. (2015) 8:11199–205.
86. Zhou X, Lu W, Wang Y, Li J, Luo Y. A20-binding inhibitor of NF-κB 1 ameliorates neuroinflammation and mediates antineuroinflammatory effect of electroacupuncture in cerebral ischemia/reperfusion rats. Evidence-Based complementary Altern Med eCAM. (2020) 2020:6980398. doi: 10.1155/2020/6980398
87. Xu H, Mu S, Qin W. Microglia TREM2 is required for electroacupuncture to attenuate neuroinflammation in focal cerebral ischemia/reperfusion rats. Biochem Biophys Res Commun. (2018) 503:3225–34. doi: 10.1016/j.bbrc.2018.08.130
88. Okusa MD, Rosin DL, Tracey KJ. Targeting neural reflex circuits in immunity to treat kidney disease. Nat Rev Nephrology. (2017) 13:669–80. doi: 10.1038/nrneph.2017.132
89. Ma Z, Zhang Z, Bai F, Jiang T, Yan C, Wang Q. Electroacupuncture pretreatment alleviates cerebral ischemic injury through α7 nicotinic acetylcholine receptor-mediated phenotypic conversion of microglia. Front Cell Neurosci. (2019) 13:537. doi: 10.3389/fncel.2019.00537
90. Cao Y, Wang L, Lin LT, Wang XR, Ma SM, Yang NN, et al. Acupuncture attenuates cognitive deficits through α7nAChR mediated anti-inflammatory pathway in chronic cerebral hypoperfusion rats. Life Sci. (2021) 266:118732. doi: 10.1016/j.lfs.2020.118732
91. Wang Q, Wang F, Li X, Yang Q, Li X, Xu N, et al. Electroacupuncture pretreatment attenuates cerebral ischemic injury through α7 nicotinic acetylcholine receptor-mediated inhibition of high-mobility group box 1 release in rats. J neuroinflammation. (2012) 9:24. doi: 10.1186/1742-2094-9-24
92. Haidar MA, Ibeh S, Shakkour Z, Reslan MA, Nwaiwu J, Moqidem YA, et al. Crosstalk between microglia and neurons in neurotrauma: an overview of the underlying mechanisms. Curr neuropharmacology. (2022) 20:2050–65. doi: 10.2174/1570159x19666211202123322
93. Lin X, Zhan J, Jiang J, Ren Y. Upregulation of neuronal cylindromatosis expression is essential for electroacupuncture-mediated alleviation of neuroinflammatory injury by regulating microglial polarization in rats subjected to focal cerebral ischemia/reperfusion. J Inflammation Res. (2021) 14:2061–78. doi: 10.2147/jir.S307841
94. Jiang J, Luo Y, Qin W, Ma H, Li Q, Zhan J, et al. Electroacupuncture suppresses the NF-κB signaling pathway by upregulating cylindromatosis to alleviate inflammatory injury in cerebral ischemia/reperfusion rats. Front Mol Neurosci. (2017) 10:363. doi: 10.3389/fnmol.2017.00363
95. Chen S, Wang L, Yuan Y, Wen Y, Shu S. Electroacupuncture regulates microglia polarization via lncRNA-mediated hippo pathway after ischemic stroke. Biotechnol Genet Eng Rev. (2023) 9:1–17. doi: 10.1080/02648725.2023.2177046
96. Xiao Y, Wu X, Deng X, Huang L, Zhou Y, Yang X. Optimal electroacupuncture frequency for maintaining astrocyte structural integrity in cerebral ischemia. Neural regeneration Res. (2013) 8:1122–31. doi: 10.3969/j.issn.1673-5374.2013.12.007
97. Han X, Huang X, Wang Y, Chen H. A study of astrocyte activation in the periinfarct region after cerebral ischemia with electroacupuncture. Brain injury. (2010) 24:773–9. doi: 10.3109/02699051003610482
98. Tao J, Zheng Y, Liu W, Yang S, Huang J, Xue X, et al. Electro-acupuncture at LI11 and ST36 acupoints exerts neuroprotective effects via reactive astrocyte proliferation after ischemia and reperfusion injury in rats. Brain Res bulletin. (2016) 120:14–24. doi: 10.1016/j.brainresbull.2015.10.011
99. Liu J, Li C, Peng H, Yu K, Tao J, Lin R, et al. Electroacupuncture attenuates learning and memory impairment via activation of α7nAChR-mediated anti-inflammatory activity in focal cerebral ischemia/reperfusion injured rats. Exp Ther Med. (2017) 14:939–46. doi: 10.3892/etm.2017.4622
100. Yang C, Liu J, Wang J, Yin A, Jiang Z, Ye S, et al. Activation of astroglial CB1R mediates cerebral ischemic tolerance induced by electroacupuncture. J Cereb Blood Flow Metab. (2021) 41:2295–310. doi: 10.1177/0271678x21994395
101. Lu Y, Zhao H, Wang Y, Han B, Wang T, Zhao H, et al. Electro-acupuncture up-regulates astrocytic MCT1 expression to improve neurological deficit in middle cerebral artery occlusion rats. Life Sci. (2015) 134:68–72. doi: 10.1016/j.lfs.2015.05.014
102. Luo Y, Xu NG, Yi W, Yu T, Yang ZH. Study on the correlation between synaptic reconstruction and astrocyte after ischemia and the influence of electroacupuncture on rats. Chin J Integr Med. (2011) 17:750–7. doi: 10.1007/s11655-011-0754-7
103. Wang SJ, Omori N, Li F, Jin G, Hamakawa Y, Sato K, et al. Functional improvement by electro-acupuncture after transient middle cerebral artery occlusion in rats. Neurological Res. (2003) 25:516–21. doi: 10.1179/016164103101201751
104. Wang F, Gao Z, Li X, Li Y, Li X, Zhong H, et al. NDRG2 is involved in anti-apoptosis induced by electroacupuncture pretreatment after focal cerebral ischemia in rats. Neurological Res. (2013) 35:406–14. doi: 10.1179/1743132813y.0000000159
105. Xu H, Zhang Y, Sun H, Chen S, Wang F. Effects of acupuncture at GV20 and ST36 on the expression of matrix metalloproteinase 2, aquaporin 4, and aquaporin 9 in rats subjected to cerebral ischemia/reperfusion injury. PloS One. (2014) 9:e97488. doi: 10.1371/journal.pone.0097488
106. Jin Z, Liang J, Wang J, Kolattukudy PE. Delayed brain ischemia tolerance induced by electroacupuncture pretreatment is mediated via MCP-induced protein 1. J neuroinflammation. (2013) 10:63. doi: 10.1186/1742-2094-10-63
107. Lee HJ, Jung DH, Kim NK, Shin HK, Choi BT. Effects of electroacupuncture on the functionality of NG2-expressing cells in perilesional brain tissue of mice following ischemic stroke. Neural regeneration Res. (2022) 17:1556–65. doi: 10.4103/1673-5374.330611
108. Ahn SM, Kim YR, Kim HN, Shin YI, Shin HK, Choi BT. Electroacupuncture ameliorates memory impairments by enhancing oligodendrocyte regeneration in a mouse model of prolonged cerebral hypoperfusion. Sci Rep. (2016) 6:28646. doi: 10.1038/srep28646
109. Stone TW, Clanchy FIL, Huang YS, Chiang NY, Darlington LG, Williams RO. An integrated cytokine and kynurenine network as the basis of neuroimmune communication. Front Neurosci. (2022) 16:1002004. doi: 10.3389/fnins.2022.1002004
110. Loftis JM, Huckans M, Morasco BJ. Neuroimmune mechanisms of cytokine-induced depression: current theories and novel treatment strategies. Neurobiol disease. (2010) 37:519–33. doi: 10.1016/j.nbd.2009.11.015
111. Li Z, Meng X, Ren M, Shao M. Combination of scalp acupuncture with exercise therapy effectively counteracts ischemic brain injury in rats. J stroke cerebrovascular Dis. (2020) 29:105286. doi: 10.1016/j.jstrokecerebrovasdis.2020.105286
112. Xu H, Sun H, Chen SH, Zhang YM, Piao YL, Gao Y. Effects of acupuncture at Baihui (DU20) and Zusanli (ST36) on the expression of heat shock protein 70 and tumor necrosis factor α in the peripheral serum of cerebral ischemia-reperfusion-injured rats. Chin J Integr Med. (2014) 20:369–74. doi: 10.1007/s11655-014-1800-z
113. Wang WB, Yang LF, He QS, Li T, Ma YY, Zhang P, et al. Mechanisms of electroacupuncture effects on acute cerebral ischemia/reperfusion injury: possible association with upregulation of transforming growth factor beta 1. Neural regeneration Res. (2016) 11:1099–101. doi: 10.4103/1673-5374.187042
114. Jittiwat J. Baihui point laser acupuncture ameliorates cognitive impairment, motor deficit, and neuronal loss partly via antioxidant and anti-inflammatory effects in an animal model of focal ischemic stroke. Evidence-Based complementary Altern Med eCAM. (2019) 2019:1204709. doi: 10.1155/2019/1204709
115. Yang JW, Wang XR, Ma SM, Yang NN, Li QQ, Liu CZ. Acupuncture attenuates cognitive impairment, oxidative stress and NF-κB activation in cerebral multi-infarct rats. Acupunct Med. (2019) 37:283–91. doi: 10.1136/acupmed-2017-011491
116. Liu W, Wang X, Zheng Y, Shang G, Huang J, Tao J, et al. Electroacupuncture inhibits inflammatory injury by targeting the miR-9-mediated NF-κB signaling pathway following ischemic stroke. Mol Med Rep. (2016) 13:1618–26. doi: 10.3892/mmr.2015.4745
117. Lan L, Tao J, Chen A, Xie G, Huang J, Lin J, et al. Electroacupuncture exerts anti-inflammatory effects in cerebral ischemia-reperfusion injured rats via suppression of the TLR4/NF-κB pathway. Int J Mol Med. (2013) 31:75–80. doi: 10.3892/ijmm.2012.1184
118. Deng P, Wang L, Zhang Q, Chen S, Zhang Y, Xu H, et al. Therapeutic potential of a combination of electroacupuncture and human iPSC-derived small extracellular vesicles for ischemic stroke. Cells. (2022) 11. doi: 10.3390/cells11050820
119. Wang Y, Chen Y, Meng L, Wu B, Ouyang L, Peng R, et al. Electro-acupuncture treatment inhibits the inflammatory response by regulating γδ T and Treg cells in ischemic stroke. Exp neurology. (2023) 17:114324. doi: 10.1016/j.expneurol.2023.114324
120. Wang YL, Meng LL, Chen YL, OuYang L, Wu BF, Peng R, et al. Electro-acupuncture treatment ameliorates intestinal inflammatory injury in cerebral ischemia-reperfusion rats via regulating the balance of Treg / γδ T cells. Brain Res. (2023) 6:148233. doi: 10.1016/j.brainres.2023.148233
121. Qin L, Zhang XX, Jin X, Cui CH, Tang CZ. The effect of acupuncture on enteral nutrition and gastrointestinal dynamics in patients who have suffered a severe stroke. Curr neurovascular Res. (2022) 19:275–81. doi: 10.2174/1567202619666220822123023
122. Jin L, Han C. Effects of acupuncture on clinical outcome and helper T cell distribution and abundance in patients with convalescent ischemic stroke. Am J Trans Res. (2021) 13:8118–25.
123. Gao Y, Lin Z, Tao J, Yang S, Chen R, Jiang C, et al. Evidence of timing effects on acupuncture: A functional magnetic resonance imaging study. Exp Ther Med. (2015) 9:59–64. doi: 10.3892/etm.2014.2056
124. Young-Wook P, Gi Yoon H, Min Jae K, Seo-Yeon L, Byung Tae C, Hwa Kyoung S. Subacute electroacupuncture at Baihui (GV 20) and Dazhui (GV 14) promotes post-stroke functional recovery via neurogenesis and astrogliosis in a photothrombotic stroke mouse model. J Tradit Chin Med. (2019) 39:833–41.
125. He Y, Han B, Hu J, Yuan L, Chen Z, Li J, et al. An observation on the acupuncture treatment of ischemic wind-stroke with different lengths of needle-retaining time. J Tradit Chin Med. (2006) 26:83–7.
126. Chang XB, Fan XN, Wang S, Yang S, Yang X, Zhang YN, et al. Influence of acupuncture on neural movement function in rats with middle cerebral artery occlusion–a randomized controlled trial. J Tradit Chin Med. (2012) 32:105–9. doi: 10.1016/s0254-6272(12)60041-7
127. Bäcker M, Hammes MG, Valet M, Deppe M, Conrad B, Tölle TR, et al. Different modes of manual acupuncture stimulation differentially modulate cerebral blood flow velocity, arterial blood pressure and heart rate in human subjects. Neurosci letters. (2002) 333:203–6. doi: 10.1016/s0304-3940(02)01109-6
128. Tian L, Du X, Wang J, Sun R, Zhang Z, Yuan B, et al. [Comparative study on the effects between manual acupuncture and electroacupuncture for hemiplegia after acute ischemic stroke]. Zhongguo Zhen Jiu. (2016) 36:1121–5. doi: 10.13703/j.0255-2930.2016.11.001
129. Yang JS, Gao X, Sun R, Wang X, Wang H, Zhang JC, et al. [Effect of electroacupuncture intervention on rehabilitation of upper limb motor function in patients with ischemic stroke]. Zhen Ci Yan Jiu. (2015) 40:489–92. doi: 10.13702/j.1000-0607.2015.06.012
130. Zhang C, Wen Y, Fan XN, Tian G, Zhou XY, Deng SZ, et al. Therapeutic effects of different durations of acupuncture on rats with middle cerebral artery occlusion. Neural regeneration Res. (2015) 10:159–64. doi: 10.4103/1673-5374.150727
131. Chang XB, Fan XN, Wang S, Meng ZH, Yang X, Shi XM. Cluster analysis for acupoint specificity of acupuncture effect based on cerebral infarction rat model. Chin J Integr Med. (2013) 19:853–8. doi: 10.1007/s11655-013-1530-7
132. Chang XB, Wang S, Meng ZH, Fan XN, Yang X, Shi XM. Study on acupuncture parameters impacting on the acupuncture effect using cluster analysis in a rat model with middle cerebral artery occlusion. Chin J Integr Med. (2014) 20:130–5. doi: 10.1007/s11655-013-1584-6
133. Zbesko JC, Stokes J, Becktel DA, Doyle KP. Targeting foam cell formation to improve recovery from ischemic stroke. Neurobiol disease. (2023) 181:106130. doi: 10.1016/j.nbd.2023.106130
134. Abraham E, Laterre PF, Garbino J, Pingleton S, Butler T, Dugernier T, et al. Lenercept (p55 tumor necrosis factor receptor fusion protein) in severe sepsis and early septic shock: a randomized, double-blind, placebo-controlled, multicenter phase III trial with 1,342 patients. Crit Care Med. (2001) 29:503–10. doi: 10.1097/00003246-200103000-00006
135. Alsaab HO, Sau S, Alzhrani R, Tatiparti K, Bhise K, Kashaw SK, et al. PD-1 and PD-L1 checkpoint signaling inhibition for cancer immunotherapy: mechanism, combinations, and clinical outcome. Front Pharmacol. (2017) 8:561. doi: 10.3389/fphar.2017.00561
136. Cui J, Song W, Jin Y, Xu H, Fan K, Lin D, et al. Research progress on the mechanism of the acupuncture regulating neuro-endocrine-immune network system. Veterinary Sci. (2021) 8. doi: 10.3390/vetsci8080149
137. Liu S, Wang Z, Su Y, Qi L, Yang W, Fu M, et al. A neuroanatomical basis for electroacupuncture to drive the vagal-adrenal axis. Nature. (2021) 598:641–5. doi: 10.1038/s41586-021-04001-4
138. Liu S, Wang ZF, Su YS, Ray RS, Jing XH, Wang YQ, et al. Somatotopic organization and intensity dependence in driving distinct NPY-expressing sympathetic pathways by electroacupuncture. Neuron. (2020) 108:436–450.e7. doi: 10.1016/j.neuron.2020.07.015
139. Akoolo L, Djokic V, Rocha SC, Ulloa L, Parveen N. Sciatic-vagal nerve stimulation by electroacupuncture alleviates inflammatory arthritis in lyme disease-susceptible C3H mice. Front Immunol. (2022) 13:930287. doi: 10.3389/fimmu.2022.930287
Keywords: immunomodulatory mechanism, acupuncture treatment, ischemic stroke, research progress, future direction, prospects
Citation: Kuang H, Zhu X, Chen H, Tang H and Zhao H (2024) The immunomodulatory mechanism of acupuncture treatment for ischemic stroke: research progress, prospects, and future direction. Front. Immunol. 15:1319863. doi: 10.3389/fimmu.2024.1319863
Received: 11 October 2023; Accepted: 03 April 2024;
Published: 02 May 2024.
Edited by:
Yong Huang, Southern Medical University, ChinaReviewed by:
Yong Tang, Chengdu University of Traditional Chinese Medicine, ChinaYi Liu, Dalian Municipal Central Hospital, China
Haiming Zhang, Huazhong University of Science and Technology, China
Copyright © 2024 Kuang, Zhu, Chen, Tang and Zhao. This is an open-access article distributed under the terms of the Creative Commons Attribution License (CC BY). The use, distribution or reproduction in other forums is permitted, provided the original author(s) and the copyright owner(s) are credited and that the original publication in this journal is cited, in accordance with accepted academic practice. No use, distribution or reproduction is permitted which does not comply with these terms.
*Correspondence: Hong Zhao, hongzhao2005@aliyun.com