- 1Department of Biochemistry, All India Institute of Medical Sciences, New Delhi, India
- 2Department of Immunology and Microbiology, The Scripps Research Institute, La Jolla, CA, United States
- 3Center for HIV/AIDS Vaccine Immunology and Immunogen Discovery, The Scripps Research Institute, La Jolla, CA, United States
- 4Center for Viral Systems Biology, The Scripps Research Institute, La Jolla, CA, United States
- 5International AIDS Vaccine Initiative (IAVI) Neutralizing Antibody Center, The Scripps Research Institute, La Jolla, CA, United States
- 6International Centre for Genetic Engineering and Biotechnology (ICGEB)-Emory Vaccine Center, International Center for Genetic Engineering and Biotechnology, New Delhi, India
- 7Department of Pediatrics, All India Institute of Medical Sciences, New Delhi, India
- 8Multi-omics Vaccine Evaluation Consortium, The Scripps Research Institute, La Jolla, CA, United States
Introduction: A limited subset of HIV-1 infected adult individuals typically after at least 2-3 years of chronic infection, develop broadly neutralizing antibodies (bnAbs), suggesting that highly conserved neutralizing epitopes on the HIV-1 envelope glycoprotein are difficult for B cell receptors to effectively target, during natural infection. Recent studies have shown the evolution of bnAbs in HIV-1 infected infants.
Methods: We used bulk BCR sequencing (BCR-seq) to profile the B cell receptors from longitudinal samples (3 time points) collected from a rare pair of antiretroviralnaïve, HIV-1 infected pediatric monozygotic twins (AIIMS_329 and AIIMS_330) who displayed elite plasma neutralizing activity against HIV-1.
Results: BCR-seq of both twins revealed convergent antibody characteristics including V-gene use, CDRH3 lengths and somatic hypermutation (SHM). Further, antibody clonotypes with genetic features similar to highly potent bnAbs isolated from adults showed ongoing development in donor AIIMS_330 but not in AIIMS_329, corroborating our earlier findings based on plasma bnAbs responses. An increase in SHM was observed in sequences of the IgA isotype from AIIMS_330.
Discussion: This study suggests that children living with chronic HIV-1 can develop clonotypes of HIV-1 bnAbs against multiple envelope epitopes similar to those isolated from adults, highlighting that such B cells could be steered to elicit bnAbs responses through vaccines aimed to induce bnAbs against HIV-1 in a broad range of people including children.
Introduction
Human immunodeficiency virus type 1 (HIV-1) infections are a global health problem that has affected an estimated 38.4 million people worldwide including children (1). The integration of virus into the host cell genome and tremendous level of viral mutation observed in individuals living with HIV-1 are major obstacles to developing effective therapeutics and vaccines against HIV-1 (2, 3). During HIV-1 infection, neutralizing antibodies (nAbs) and non-nAbs are elicited against multiple epitopes of the HIV-1 envelope glycoprotein (4–6). In the past 15 years, the invention of high-throughput technologies for antibody generation i.e. single B cell sorting, high-throughput antibody screening by micro-neutralization methods and single cell sequencing, have led to the discovery and molecular characterization of highly potent second-generation HIV-1 broadly neutralizing antibodies (bnAbs) both from adults and children living with HIV-1 (5–7). Presently, researchers around the globe are leveraging our understanding of the genetic, functional, and structural properties of these bnAbs to develop HIV-1 vaccines that reliably induce broad and potently neutralizing antibody responses (8–10).
The characteristic features of HIV-1 bnAbs targeting multiple envelope epitopes that are isolated from adult donors have been extensively studied and are well-known (5, 6). However, the understanding of genetic and molecular features of HIV-1 bnAbs from children is still limited. So far, only two HIV-1 pediatric bnAbs have been identified, BF520.1 by Simonich et.al (11). and AIIMS-P01 by us (Kumar et. al.) (12). Further insights into the pediatric B cell repertoire are urgently needed to support next-generation vaccine design and vaccination strategies aiming to elicit protective bnAbs responses in a wide range of individuals including children. It has been reported in multiple studies that children living with HIV-1 showed broader and more potent bnAbs responses with multiple epitope specificities as compared to bnAbs from adults even within one-year of age, suggesting that bnAb responses in children are developed from different maturation mechanisms/pathways (11–22). This is supported by the discovery of BF520.1 and AIIMS-P01 pediatric bnAbs which exhibit comparable HIV-1 neutralization breadth and potency to adult bnAbs but with limited somatic hypermutation (SHM) (11, 12).
In the past 15 years, we have established a rare cohort of HIV-1 clade C chronically infected pediatric donors including infants (12, 15, 17, 18, 22–26). Recently, we reported the identification of infant and adolescent pediatric elite-neutralizers (AIIMS_329 and AIIMS_330) from characterization of their plasma HIV-1 bnAbs responses at a single and longitudinal time points, respectively (15, 22). We observed the development of bnAbs targeting the V1/V2 apex, glycan supersite, andCD4 binding site (CD4bs) in AIIMS_330. We also noted V1/V2 apex- and glycan supersite-dependent bnAbs in AIIMS_329, but at a lower potency and breadth in comparison to AIIMS_330 (22). Herein, we performed deep sequencing of the bulk B cell repertoires (BCR-seq) of two monozygotic twin HIV-1 pediatric elite-neutralizers (AIIMS_329 and AIIMS_330). BCR-seq of both AIIMS_329 and AIIMS_330 showed convergent antibody characteristics including Variable gene use, heavy chain complementarity determining region (HCDR3) lengths, SHM frequency. Mapping BCR-seq data to known HIV-1 bnAbs, allowed us to identify antibody clonotypes with similar features to potent HIV-1 bnAbs in AIIMS_330 but not in AIIMS_329, corroborating with our previous serological findings. This study is an important step toward defining specific features of the antibody repertoire and shared clonotype maturation that are associated with the development of HIV-1 elite-neutralizing activity. Some of the shared lineages identified in this pair of identical twins, who had acquired HIV-1 infection by vertical transmission, may have been elicited by exposure to common HIV-1 antigens. Defining the sequences of such shared clonotypes in a large number of children can shed light on the role of specific B cell receptor features in the response to HIV-1 infection.
Materials and methods
Patients characteristics and ethics statement
Monozygotic twin antiretroviral naïve HIV-1 clade C pediatric elite-neutralizers (AIIMS_329 and AIIMS_330) were recruited from the Outdoor Patient Department of the Department of Pediatrics, All India Institute of Medical Sciences (AIIMS), New Delhi, India for this study at the age of 9 years and were followed for a total period of 60 months. Blood was drawn in 5-ml EDTA vials, and plasma was aliquoted and stored for plasma antibody-based HIV-1 neutralization assays, viral RNA isolation, and viral load determinations.
Next-generation sequencing of HIV-1 pediatric B cell antibody repertoires
The deep sequencing of bulk BCR was performed using primers and protocols as described previously (27). Briefly, total RNA from 2 or 3 million peripheral blood mononuclear cells (PBMCs) was extracted (RNeasy Maxi Kit, Qiagen) from each time point (2015 (112 months p.i), 2016 (117 months p.i) and 2018 (138 months p.i)) and antibody sequences were amplified using methods and primers as previously described. The PCR product sizes were verified on agarose gel (E-Gel EX; Invitrogen) and quantified with fluorometry (Qubit; Life Technologies), pooled at approximately equimolar concentrations and each sample pool was re-quantified before sequencing on an Illumina MiSeq (MiSeq Reagent Kit v3, 600-cycle).
Processing of next-generation sequencing data
The Abstar analysis pipeline was used as previously described to quality trim, remove adapters and merge paired sequences (27). Sequences were then annotated with Abstar in combination with UMI based error correction by AbCorrect (https://github.com/briney/abtools/). For comparison of frequencies, read counts were scaled for each repertoire as previously described due to the large differences in the number of reads between each group. Somatic hypermutation (SHM) was calculated using observed mutations function in R package Shazam (28) which calculates observed number of mutations in each input nucleotide sequence compared to aligned germline sequences. Only the V-region was used for calculating the SHM.
Clonotype analysis
Clonotype analysis was performed using Immcantation pipeline (28, 29). To get comparable results between the groups, sequences from 330_2018 timepoint were downsampled from 4,000,000 to 400,000 sequences (Supplementary Table 1). The downsampling was performed by randomly selecting 40,000 sequences from the total sequences in multiple iterations. Each subsample was analyzed to evaluate the probability of that subsample belonging to the original distribution. The subsample with the highest probability was selected for further analysis. Sequences were grouped into clonotypes based on nucleotide hamming distance of 0.16 calculated based on bimodal distribution of distance of each sequence with its nearest neighbor. Alternatively, sequences were also clustered into clonal groups using an in-house script. The criteria used for clonal assignment was sequences having same V and J gene usage, same CDRH3 length and at least 80% CDRH3 amino acid identity. Germline V(D)J sequence was reconstructed using IMGT-gapped reference V, D and J sequences.
Deep mapping of HIV-1 mAbs to B cell repertoire sequencing data
List of HIV-1mAbs which have been reported previously was compiled from CATNAP database and literature (5–7, 30). Antibodies for which the gene usage information and CDRH3 amino acid sequence was available were selected for downstream analysis. The list of selected mAbs is given in Supplementary Table 7. Each mAb was mapped to all the sequences to identify the ones which have the same V and J gene usage, same CDRH3 length and at least 50% identity in CDRH3 amino acid sequence.
Alignment and phylogeny
Alignment was performed using R package MSA. Sequence logos were made using R package ggseqlogo. Distance between sequences were calculated using the neighbor joining method. Phylogenetic tree was constructed using R package ape.
Statistical analysis
All analysis was performed using R programming language (version 4.1.0). Statistical significance between groups was estimated using Welch’s t-test. Chi-square test was used for pairwise comparison between the groups. For each V-gene, a 2x2 contingency table of counts of the V-gene present in the repertoire vs the counts of other V-genes not-present in the repertoire was created and chi-square test was performed. The following packages were used for the analyses: scatterpie (0.1.7), nortest (1.0-4), scales (1.1.1), ggrepel (0.9.1), stringr (1.4.0), tigger (1.0.0), ComplexUpset (1.3.0), ggpubr (0.4.0), alakazam (1.1.0), shazam (1.1.0), patchwork (1.1.1), writexl (1.4.0), readxl (1.3.1), dplyr (1.0.6), reshape2 (1.4.4) and ggplot2 (3.3.5).
Data and code availability statement
Raw sequence data that support the findings in this study are available at the NCBI Sequencing Read Archive (www.ncbi.nlm.nih.gov/sra) under BioProjectnumber: PRJNA999025. Processed datasets are available at https://github.com/prashantbajpai/HIV_BCR_Analysis. All bash and R scripts required for reproducing the data can be found on github repository (https://github.com/prashantbajpai/HIV_BCR_Analysis). Raw data used for generating the figures are available at the github page or in the Supplementary Files.
Results
Deep BCR sequencing of HIV-1 pediatric elite-neutralizers
AIIMS_329 and AIIMS_330 are monozygotic twins, and both display elite HIV-1 plasma neutralization. Longitudinal samples were obtained from both twins prior to their initiation of combined antiretroviral therapy (cART) in 2018. The total number of sequences obtained for each subject after sequencing and annotation ranged from approximately 1.0x105 to 4.0x105 sequences (Supplementary Table 1). Immunogenetic analysis of heavy chain Variable (VH) genes identified IGHV3-21, IGHV3-23, and IGHV-34 to be predominant gene usages in both AIIMS_329 and AIIMS_330 groups in the three timepoints of their sample collection in the years 2015 (112 months p.i.), 2016 (117 months p.i.), and 2018 (138 months p.i.). The IGHV3-21 gene frequency, within the BCR repertoire from the three time points of AIIMS_330 (median frequency 5.87%) was significantly higher than in AIIMS_329 (median frequency 4.01%, p-value < 0.0001). Maximum IGHV3-21 gene frequency was observed in the 330_2015 BCR repertoire (Figure 1A, Supplementary Tables 2A–C). The frequency of all other major V-genes, IGHV3-23, IGHV4-34, IGHV3-7, and IGHV4-39 were also found to be significantly different between AIIMS_329 and AIIMS_330 at all three timepoints. (Figure 1A, Supplementary Table 2D). Consistent with a previous study (31), from the human antibody repertoire, IGHD3-22, IGHD3-10, IGHD6-13 were found to be major D genes and IGHJ4 was found to be predominant heavy J gene. No noteworthy differences were observed in the frequencies of heavy chain Diversity (D)or Joining (J) genes (Figures 1B, C). In the light chain, IGKV4-1 and IGKV3-20 were the predominant gene usages. We observed a trend toward higher frequency of IGKV4-1 in AIIMS_330 compared to AIIMS_329 (14.54%, 20.35%, 14.16% in 330_2015, 330_2016 and 330_2018 compared to 8.89%, 10.27%, 12.99% in 329_2015, 329_2016 and 329_2018). We also observed frequency of IGLV2-14 to be lower in AIIMS_330 compared to AIIMS_329 (2%, 2.09%, 2.13% in 330_2015, 330_2016 and 330_2018 compared to 4.63%, 7.91%, 5.09% in 329_2015, 329_2016 and 329_2018) (Figure 1D, Supplementary Table 3). We also observed marked differences in the light chain J gene use between the two groups (Figure 1E). Gene usage IGKJ2 showed a trend toward higher frequency in AIIMS_330 at three timepoints. Further, the frequency of IGLJ3 was markedly lower in AIIMS_330 compared to AIIMS_329. Overall, though heavy chain V, D, and J gene frequencies were similar in AIIMS_329 and AIIMS_330, we observed marked differences in lambda and kappa light chains between the two groups at all timepoints.
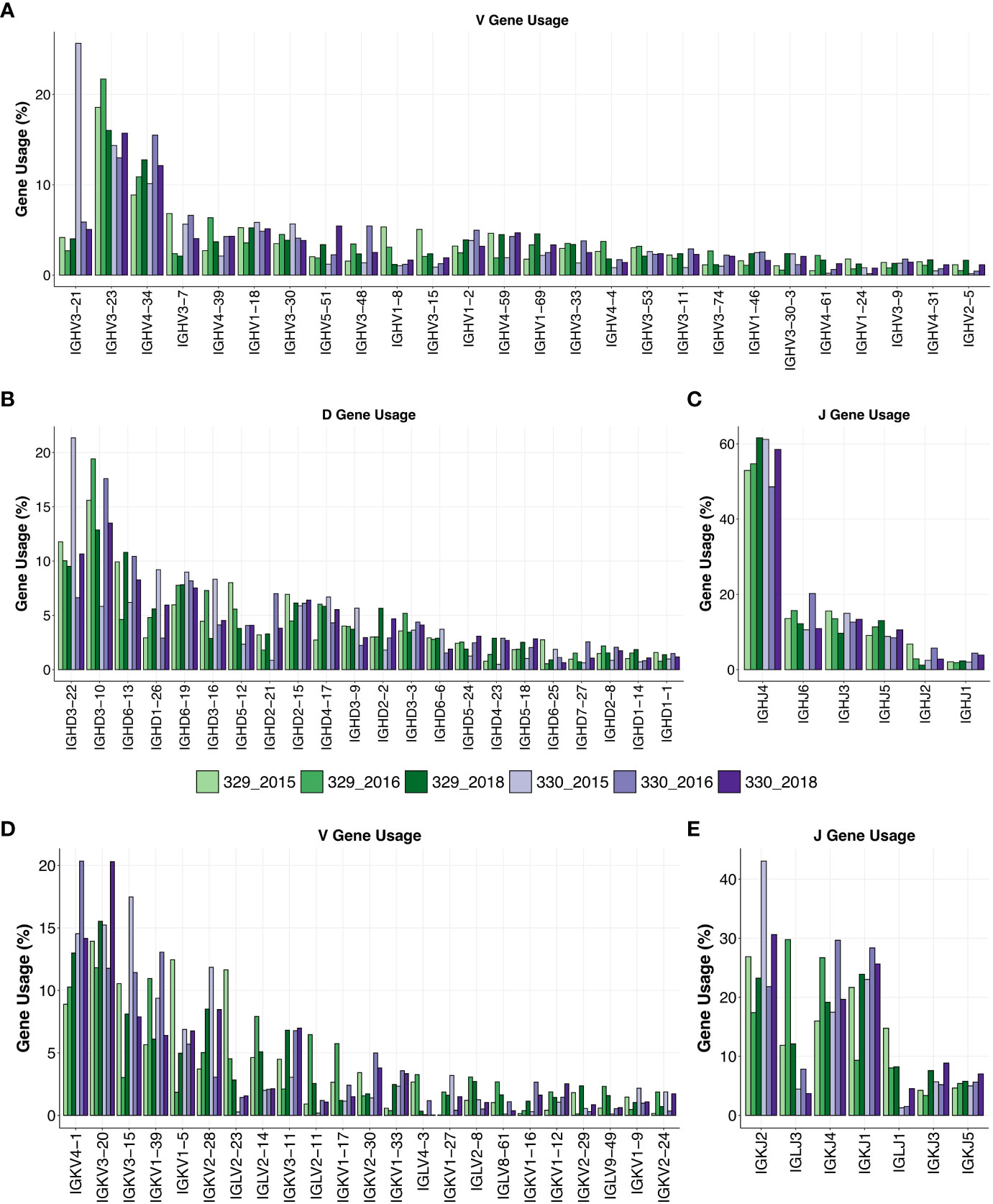
Figure 1 Gene usage of heavy and light chains. V-gene (A), D-gene (B) and J-gene (C) usage of heavy chains of genes shown as percentage of total sequences and arranged by descending frequency. V-gene (D) and D-gene (E) of light chains are shown.
Shared immunogenetic features between AIIMS_329 and AIIMS_330
The CDRH3 length distributions were similar for AIIMS_329 and AIIMS_330 at all time points, with mean CDHR3 length being lower in the AIIMS_330(12.91, 13.22 and 12.66 in 330_2015, 330_2016, and 330_2018, respectively, compared to 12.97, 13.37, and 12.97 in 329_2015, 329_2016, and 329_2018) (Figure 2A, Supplementary Table 4). A similar trend was observed in the distribution of light chain CDR3 lengths (Supplementary Figure 1). The SHM frequency was higher in 330_2016 and 330_2018 compared to 329_2016 and 329_2018 respectively. In the 2018 timepoint, median SHM in AIIMS_330 was 2.42% compared to 0.41% in AIIMS_329 (Figure 2B, Supplementary Table 5). Interestingly, when the sequences were segregated into isotypes, sequences from IgA isotype were observed to have significantly higher SHM in AIIMS_330 compared to AIIMS_329. The median SHM in IgA sequences was found to be 11.74%, 6.97%, and 6.94% in 330_2015, 330_2016, and 330_2018, respectively, compared to 7.63%, 6.05%, and 4.72% in 329_2015, 329_2016, and 329_2018. No significant changes were observed in other isotypes (Figure 2C, Supplementary Table 6).
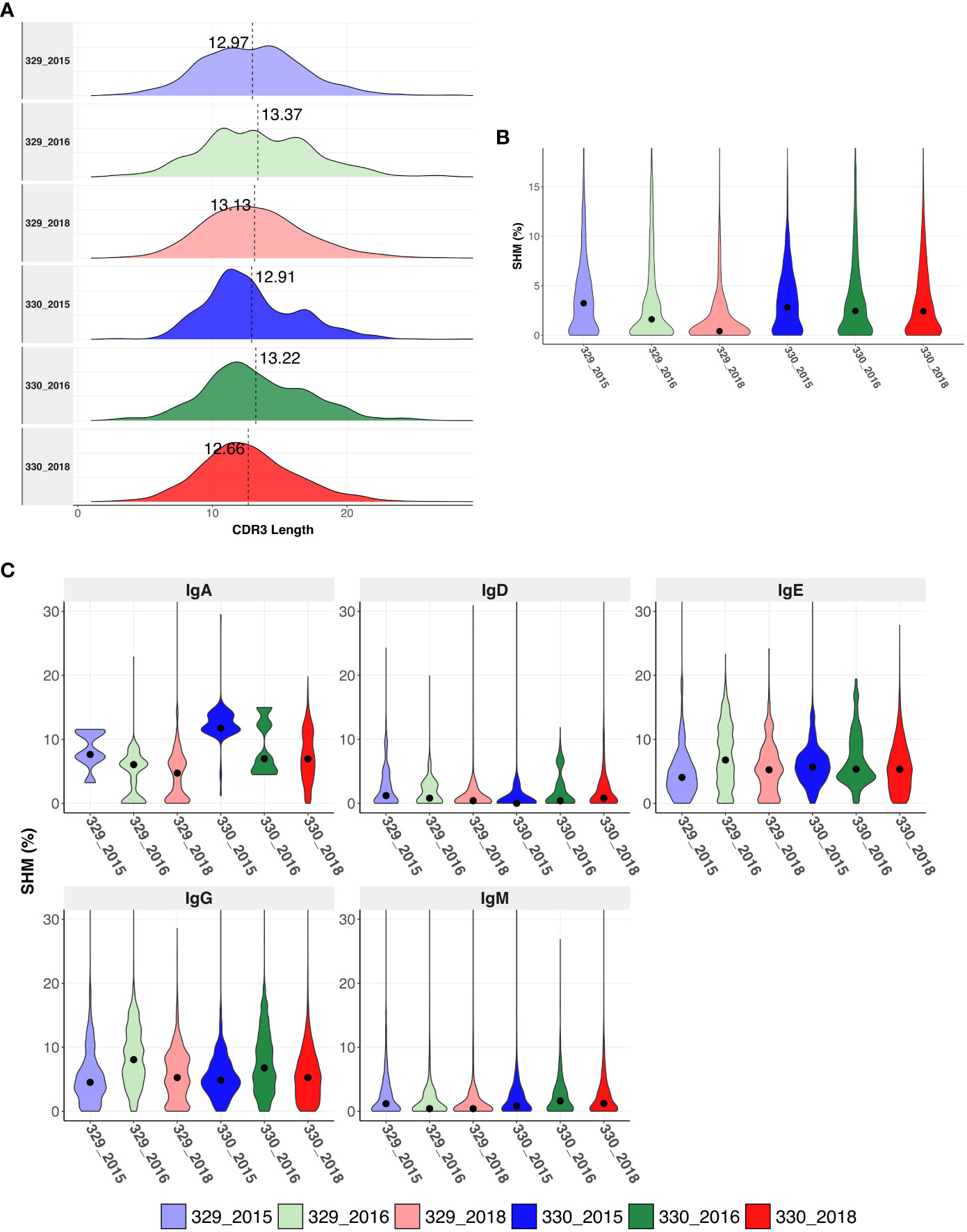
Figure 2 Immunogenetics characteristics of heavy chain sequences. (A) CDRH3 length distribution of heavy chains in each group. The dotted horizontal line shows the median of each group. (B) Violin plot shows somatic hypermutation (SHM) in each group. The dot represents the median in each group. (C) Violin plot shows the SHM in each group segregated by isotype. SHM were calculated using total number of nucleotide mutations in the V-gene. The dot represents the mean in each group.
Shared clonotype analysis identified distinct features between AIIMS_330 and AIIMS_329
Sequences were subsampled before performing the clonotype analysis (more detail of the subsetting approach can be found in the methods section). Frequency of total shared clonotypes between any two groups was found to be less than 0.07% (76 shared clonotypes across 10918 total clonotypes, Figure 3A bottom panel). The frequency of shared clonotypes between the monozygotic twins were observed to be lower than frequency of shared clonotypes between two random HIV seronegative individuals (Supplementary Figure 2). The mean frequency of shared clonotypes between the samples represented in Briney et.al. (27), were found to be 1.86% (Supplementary Table 8). However, the frequency of shared clonotypes observed between the monozygotic twins was higher compared to shared clonotype frequency of synthetic sequences generated in Briney et.al., was 0.0032% (27). Of all the clonotypes identified in each group, clones in 330_2018 timepoint had the highest SHM compared to others. Most of the clonotypes in 330_2018 timepoint had mean SHM >10%. We also observed higher expansion in 2018 timepoint in AIIMS_330 compared to AIIMS_329 as observed from lower number of clonotypes and higher number of sequences in each clonotype. Interestingly, the overall clonotypes in 2018 timepoint in both groups were higher compared to 2015 and 2016 timepoints (Figure 3A middle panels). No notable differences were observed in the V-family distribution of shared clonotypes (Figure 3A top panel). We also analyzed the clones shared between each sample and timepoints. We did not observe any clonotypes shared between all the groups or even between AIIMS_330 and AIIMS_329 at all three timepoints. Interestingly, the highest number of shared clonotypes were identified between 330_2016 and 330_2018 timepoints (39 shared clonotypes) but not between any timepoints in AIIMS_329. Of these 39 clonotypes, only 3 had mean SHM greater than 10%. However, those 3 clonotypes did not have any significant differences in their CDR3 amino acid (Figure 3B). We identified 12 clonotypes that were shared between AIIMS_330 and AIIMS_329 at 2016 timepoint of which 5 clonotypes had mean SHM greater than 10%. These 5 clonotypes were found to have accumulated higher mutations in CDR3 compared to the 3 clonotypes shared between 330_2016 and 330_2018 (Figure 3C). None of these shared clonotypes were predicted to encode sequence insertions or deletions which are frequently found in HIV bnAbs and thought to be difficult to elicit by vaccination. Such BCRs could plausibly define a more efficient path for bnAb maturation that can be exploited by rational HIV vaccine immunogens. We also observed 21 clonotypes in common between 2018 timepoint between AIIMS_330 and AIIMS_329. Of these, only one clonotype had >10% SHM. CDR3 of sequences from these clonotypes were observed to harbor higher number of mutations in 330_2018 timepoint compared to 329_2018 (Figure 3D).
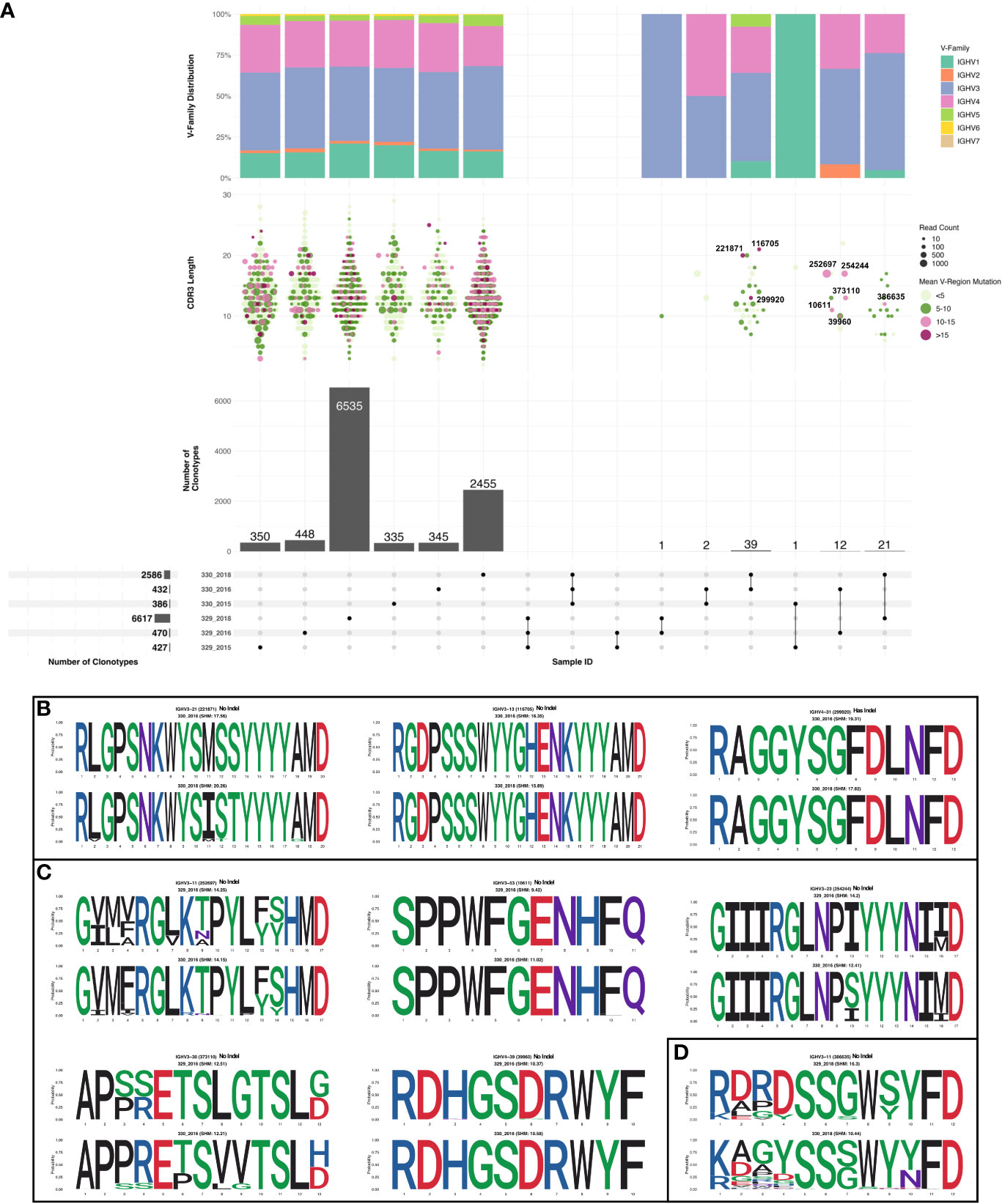
Figure 3 Shared clonotypes between the groups. (A) UpSet plot illustrating the intersection of clonotypes between the subject 329 and 330 at the three time points (2015, 2016 and 2018). Only the clonotypes which were not singlets with more than one sequences were selected for the analysis. Each vertical bar shows the number of clonotypes present in each group indicated by the intersection matrix below it (where a single dot in the matrix is a single group). The number above the bars show the number of common clonotypes between the groups of intersecting sets of clonotypes marked below the bars. For each intersection the dot plot above indicates the CDRH3 length distribution for each clonotype. Size of each dot indicates the number of sequences present in each clonotype and the color shows the somatic hypermutation (SHM) frequency. The vertical bar above it shows the distribution of VH genes for each intersection. Within the shared clonotypes, the clones with SHM >= 10% are labeled and shown. (B) Sequence logo representing alignment of CDRH3 region of clones common between 2016 and 2018 timepoint of subject 330 with >= 10% SHM. (C) Sequence logo representing alignment of CDRH3 region of clones common between 2016 and subject 329 and 330 with >= 10% SHM. (D) Sequence logo representing alignment of CDRH3 region of clones common between 2018 timepoint of subject 329 and 330 with >= 10% SHM.
Mapping of sequences to published adult HIV bnAbs identified 330_2018 to be capable of producing bnAbs with characteristics of adult bnAbs
To identify if either of the two children could generate known HIV-1 bnAbs at any timepoint, we mapped the sequences from each group to known HIV mAbs compiled from HIV neutralizing antibody database (CATNAP) and published studies. A total of 347 mAbs were compiled (Supplementary Table 7) and mapped to sequences from each group. The criteria for calling a sequence a successful hit is described in the methods. Of the total 347 mAbs, 78 mAbs mapped to at least one of the sequences in at least one of the groups (% of sequences mapped: 2.83%). Surprisingly, majority of the sequences that mapped to these mAbs were from 330_2018 timepoint but no other timepoints. Moreover, we observed the expansion/more frequency of the following HIV-1 bnAbs like sequences in AIIMS_330:VRC38.01 (V2 apex) (32), VRC34.01 (fusion peptide) (33), HGN194 (V3) (34), HK20 (gp41 HR) (35), DH270 (glycan supersite) (36), BG505.m27 (V3) (37), 8ANC131 (CD4bs) (38), 2558 (V3) (39), and VRC33.01 (CD4bs) (40) (Figure 4A). Strikingly, except DH511.1, DH511.6 (MPER) (41)and BG505.m27 HIV-1 bnAbs, no other mapped sequences from AIIMS_330 only showed SHM >10%, however, such high SHMs were not observed for any of the mapped sequences from AIIMS_329. The 78 mAbs that were mapped to heavy chain sequences were also mapped to the light chain sequences. Using the same mapping criteria, it was found that 28 of the mAbs also mapped to light chain sequences and sequences from AIIMS_330 showed higher SHM compared to other timepoints (Figures 4B, C). We also mapped the sequences from HIV seronegative subjects from Briney et. al., to known HIV mAbs (27). Of the total 2,298,309 sequences tested across 7 HIV seronegative subjects, only 1804 sequences mapped to 42 HIV mAbs. The frequency of mapped sequences was 0.078% which was 36-fold lower compared to frequency of mapped sequences from the monozygotic twins (2.83%, Supplementary Table 8). In summary, we show that both heavy and light chain sequences mapped to similar set of mAbs and showed higher SHM and number in AIIMS_330.
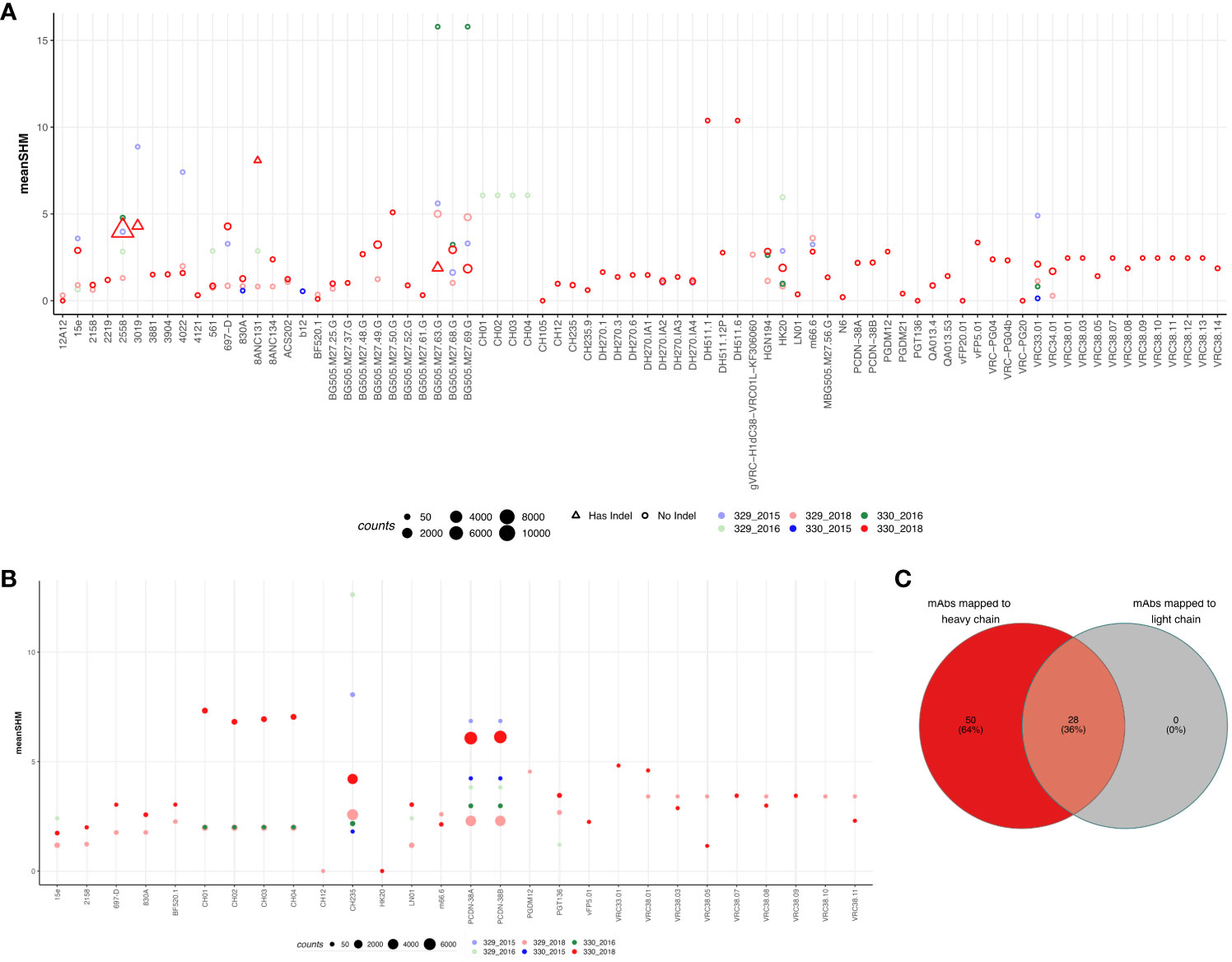
Figure 4 Sequences shared with publicly available HIV bnAbs. Publicly available mAbs mapped to the heavy chain (A) and light chain (B) sequences are shown. Each HIV mAb is shown on the x-axis while and each dot represents the number of sequences mapped to the respective mAb. The criteria for calling mAb a hit was same V and J gene, more that 50% identity in the CDHR3 amino acid and same CDRH3 length. The size of the dot represents the number of sequences mapped and color represents the group. On y-axis mean somatic hypermutation (SHM) is shown. (C) Venn diagram showing the overlap of mAbs that mapped in both heavy and light chain sequences.
Discussion
An understanding of pediatric HIV-1 bnAb responses elicited during chronic infection can provide critical insights for the design and development of effective universal HIV-1 vaccines for both adults and children capable of eliciting potent HIV-1 bnAb responses (42, 43). Human antibody repertoire comprises a whole group of antibody genes generated in an individual since birth, which can be specific to an antigen during an infection/disease and vaccination. BCR-seq is a powerful method that has enabled the in-depth analysis of genetic features of antibody responses and tracking of antibody evolution during an infection/vaccination (27). Studying such antibody repertoires could reveal important information that include germline antibody genes, junctional diversity, SHM, clonotypes and allows identification of rare antibody lineage genes specific to a particular antigen.
One of our previous study based on longitudinal characterization of plasma samples we reported the identification of adolescent pediatric elite-neutralizers who were HAART-naïve and living with chronic HIV-1 clade-C infection for more than 11 years (22). We evaluated the V1/V2 apex, glycan supersite, and CD4bs specific responses in AIIMS_329 and AIIMS_330, using ELISA and point mutated HIV-1 pseudoviruses based neutralization assays (22). We showed a longitudinal development of HIV-1 bnAbs targeting V1V2, N332 and CD4bs in AIIMS_330, whereas V1V2 and N332-supersite dependent HIV-1 bnAbs in AIIMS_329, but at a lower potency and breadth in comparison to AIIMS_330 (22). We further generated autologous viruses between year 2015 and 2018 sample time points from the contemporaneous (2015 timepoint), at which we isolated the bnAb AIIMS-P01; and evolving viruses (2016 timepoint) and contemporaneous (2018 timepoint) for the recent identification of 44m bnAb from AIIMS_330 (44). AIIMS-P01 bnAb from AIIMS_330 was observed to neutralize its coexisting and evolving autologous viruses (12, 22). The 44m bnAb isolated at 2018 time point demonstrated improved HIV-1 neutralization as compared to AIIMS-P01, showing the maturation of HIV-1 bnAbs in AIIMS_330 (44). We extensively characterized the plasma HIV-1 neutralizing breadth, successfully amplified, and characterized HIV-1 Envs from longitudinal three timepoints of AIIMS_329 and AIIMS_330 sample collection in the years 2015 (112 months p.i.), 2016 (117 months p.i.), and 2018 (138 months p.i.) (22). We observed that the plasma nAbs of AIIMS_330, the donor with coevolving plasma neutralizing activity and a mixture of sensitive and resistant viruses, showed high potency, a high GMT, and multiple antibody specificities in the plasma, suggesting that the coevolution of viruses and nAbs favored the development of plasma breadth and potency (12, 22).
Herein, we performed bulk BCR-seq of these two monozygotic twins HIV-1 pediatric elite-neutralizers (AIIMS_329 and AIIMS_330) to understand their BCR repertoire. Analysis of three time points (112, 117 and 138 months p.i.) from these pediatric elite-neutralizers showed high convergence of antibody gene-usage, CDRH3 lengths, SHM, and clonotypes. Though a higher IgA SHM was observed in AIIMS_330, no other isotype showed differential mutation patterns. The development of HIV-specific IgA responses with affinity maturation have been shown to in association with anti-gp41 IgA antibodies that occurs to a greater extent in elite controllers than in individuals on HAART (45), suggesting IgA in AIIMS_330 could be associated with lower viral load as compared to AIIMS_329.
Current common strategies to generate antigen specific antibodies and evaluating humoral vaccine responses are: 1) high-throughput sorting antigen-specific B cells followed by paired heavy and light chain gene amplification and sequencing, 2) barcoded antigen approaches like LIBRA-seq which leverage single cell microfluidics to link antibody genetics and binding specificity in high throughput, and 3) activation and culture of primary B cells followed by functional supernatant screening to identify B cell clones with desirable functional profiles. Such methods are labor-and time-intensive ways of identifying antigen-specific antibodies. Mapping sequences to well-known established antibody CDR3 information can provide templates or blueprints to identify important antibody genes in a distinct set of individual populations, antibody discovery, and vaccine response evaluation. Such strategies have been used to identify potent antibodies against Dengue, HIV-1, SARS-CoV-2, and influenza (46).
Here, we mapped BCR sequencing data against datasets of known HIV-1 bnAbs (30). Interestingly, this analysis led to the identification of multiple HIV-1 specific antibody clonotypes isolated from both adults and children in our BCR-seq data. AIIMS_330 showed clonotypes similar to several HIV-1 bnAbs identified in adults which are known to target multiple epitopes including MPER, CD4bs, N332, FP and V1V2, suggesting that antibody data mining based on CDRH3 sequences could help in bnAbs lineage identification to a particular antigen, as recently observed by an AI-based pipeline developed by Wu et.al (46). for COVID-19 and Flu antibody genes and DENV specific mAbs by Durham et.al (47). Our analysis of these bnAb lineages showed the evolution/more frequency (Figure 4) of these antibodies in AIIMS_330 donor, which corroborates with our previous findings based on plasma characterization and evolution of multi-epitope specific antibodies including CD4bs bnAbs in AIIMS_330 than AIIMS_329 (22). Overall, the observation of some shared lineages in this pair of identical pediatric twins, who had acquired HIV-1 infection by vertical transmission, suggest that they could evolve in response to common antigenic stimulus. Defining the sequences of such shared clonotypes, in a large number of children living with chronic HIV-1 infection worldwide, can shed light in understanding the role of specific B cell receptor repertoires in HIV-1 infection. The lack of availability of further plasma and PBMCs samples from these pediatric twins for in depth viral sequencing or making individual mAbs from different timepoints is a limitation for the present and for future studies on these pediatric twins’ samples.
Our study provides insight into the BCR repertoires of pediatric twins capable of exceptionally broad and potent HIV-1 neutralization. We noted the presence of clonotypes with genetic similarity to known adults’ HIV-1 bnAbs targeting multiple epitope specificities in children, which corroborated the results of our previous study which characterized the plasma neutralization breadth, potency, and epitope specificity from the same pair of donors (22). We anticipate this data will be useful for the design and development of effective vaccine candidates and strategies for both adults and children to combat HIV-1. Our BCR-seq findings from this unique pair of pediatric elite-neutralizers suggests that multivalent HIV-1 vaccine development strategies focused on inducing a diverse range of HIV-1 bnAb specificities is likely superior to approaches that focus solely on a single epitope.
Data availability statement
The datasets presented in this study can be found in online repositories. The names of the repository/repositories and accession number(s) can be found below: PRJNA999025 (SRA).
Ethics statement
This study involved human participants/samples. It was approved by the Institute Ethics Committee (IEC/59/08.01.16, IEC/NP-536/04.11.2013, IEC/NP-295/2011 and RP-15/2011) of All India Institute of Medical Sciences (AIIMS), New Delhi, India. This study was conducted in accordance with the local legislation and institutional requirements. Written informed consent for participation in this study was provided by the participants’ legal guardians/next of kin.
Author contributions
SK: Conceptualization, Formal Analysis, Funding acquisition, Investigation, Methodology, Project administration, Visualization, Writing – original draft. PB: Data curation, Formal Analysis, Software, Visualization, Writing – original draft. CJ: Data curation, Investigation, Methodology, Software, Writing – review & editing. SKK: Resources, Writing – review & editing. RL: Resources, Writing – review & editing. DB: Resources, Supervision, Writing – review & editing. BB: Conceptualization, Data curation, Methodology, Software, Supervision, Validation, Writing – review & editing. KL: Conceptualization, Funding acquisition, Supervision, Writing – review & editing.
Funding
The author(s) declare financial support was received for the research, authorship, and/or publication of this article. We are thankful to the SERB, India (CRG/2021/003984), and Department of Biotechnology (DBT) (BT/PR 24520/MED/29/1222/2017) for the funding provided to KL. This study is supported in part by ViiV Healthcare and the HIV Research Trust Scholarship 2019 awarded to SK as part of his Graduate (Ph.D.) work training and gaining expertise in Next Generation Sequencing based technologies to sequence single B cell and bulk B cell repertoire sequencing. We are thankful to the National Institute of Allergy and Infectious Diseases (NIAID), National Institutes of Health (NIH), for the fundings provided to BB (P01 AI177683, P30 AI036214, and R35 GM133682), and the funding provided to DRB (UM1 AI144462). SK is supported by the DBT/Wellcome Trust India Alliance Early Career Fellowship (IA/E/18/1/504307). The funders had no role in the decision to publish or the preparation of the manuscript.
Conflict of interest
The authors declare that the research was conducted in the absence of any commercial or financial relationships that could be construed as a potential conflict of interest.
The author(s) declared that they were an editorial board member of Frontiers, at the time of submission. This had no impact on the peer review process and the final decision.
Publisher’s note
All claims expressed in this article are solely those of the authors and do not necessarily represent those of their affiliated organizations, or those of the publisher, the editors and the reviewers. Any product that may be evaluated in this article, or claim that may be made by its manufacturer, is not guaranteed or endorsed by the publisher.
Supplementary material
The Supplementary Material for this article can be found online at: https://www.frontiersin.org/articles/10.3389/fimmu.2024.1272493/full#supplementary-material
Supplementary Figure 1 | Immunogenetics characteristics of light chain sequences. (A) CDRL3 length distribution of kappa and lambda chains in each group. The dotted horizontal line shows the median of each group.
Supplementary Figure 2 | Shared clonotypes between HIV seronegative subjects from Briney et al. Venn diagram shows the overlap of clones between 7 individuals data taken from Briney et. al (27). Percentage of overlapping clones are shown for each intersection. The names represent the subject names from the original paper.
References
1. UNAIDS data 2022. Available online at: https://www.unaids.org/en/resources/documents/2023/2022_unaids_data.
2. Cuevas JM, Geller R, Garijo R, López-Aldeguer J, Sanjuán R. Extremely high mutation rate of HIV-1 in vivo. PloS Biol. (2015) 13:e1002251. doi: 10.1371/journal.pbio.1002251
3. Cohn LB, Chomont N, Deeks SG. The biology of the HIV-1 latent reservoir and implications for cure strategies. Cell Host Microbe. (2020) 27:519–30. doi: 10.1016/j.chom.2020.03.014
4. Pantophlet R, Burton DR. GP120: target for neutralizing HIV-1 antibodies. Annu Rev Immunol. (2006) 24:739–69. doi: 10.1146/annurev.immunol.24.021605.090557
5. Sok D, Burton DR. Recent progress in broadly neutralizing antibodies to HIV. Nat Immunol. (2018) 19:1179–88. doi: 10.1038/s41590-018-0235-7
6. Kumar S, Singh S, Luthra K. An overview of human anti-HIV-1 neutralizing antibodies against diverse epitopes of HIV-1. ACS Omega. (2023) 8:7252–61. doi: 10.1021/acsomega.2c07933
7. Burton DR, Hangartner L. Broadly neutralizing antibodies to HIV and their role in vaccine design. Annu Rev Immunol. (2016) 20:34:635–59. doi: 10.1146/annurev-immunol-041015-055515
8. del Moral-Sánchez I, Russell RA, Schermer EE, Cottrell CA, Allen JD, Torrents de la Peña A, et al. High thermostability improves neutralizing antibody responses induced by native-like HIV-1 envelope trimers. NPJ Vaccines. (2022) 7:1–12. doi: 10.1038/s41541-022-00446-4
9. Sanders RW, Derking R, Cupo A, Julien JP, Yasmeen A, de Val N, et al. A next-generation cleaved, soluble HIV-1 Env trimer, BG505 SOSIP.664 gp140, expresses multiple epitopes for broadly neutralizing but not non-neutralizing antibodies. PloS Pathog. (2013) 9:e1003618. doi: 10.1371/journal.ppat.1003618
10. del Moral-Sánchez I, Wee EG, Xian Y, Lee WH, Allen JD, de la Peña AT, et al. Triple tandem trimer immunogens for HIV-1 and influenza nucleic acid-based vaccines. bioRxiv. (2023). doi: 10.1101/2023.08.27.554987v1
11. Simonich CA, Williams KL, Verkerke HP, Williams JA, Nduati R, Lee KK, et al. HIV-1 neutralizing antibodies with limited hypermutation from an infant. Cell. (2016) 166:77–87. doi: 10.1016/j.cell.2016.05.055
12. Kumar S, Panda H, Makhdoomi MA, Mishra N, Safdari HA, Chawla H, et al. An HIV-1 broadly neutralizing antibody from a clade C-infected pediatric elite neutralizer potently neutralizes the contemporaneous and autologous evolving viruses. J Virol. (2019) 93. doi: 10.1128/JVI.01495-18
13. Goo L, Chohan V, Nduati R, Overbaugh J. Early development of broadly neutralizing antibodies in HIV-1-infected infants. Nat Med. (2014) 20:655–8. doi: 10.1038/nm.3565
14. Kumar S, Batra H, Singh S, Chawla H, Singh R, Katpara S, et al. Effect of combination antiretroviral therapy on human immunodeficiency virus 1 specific antibody responses in subtype-C infected children. J Gen Virol. (2020) 101:1289–99. doi: 10.1099/jgv.0.001480
15. Mishra N, Sharma S, Dobhal A, Kumar S, Chawla H, Singh R, et al. Broadly neutralizing plasma antibodies effective against autologous circulating viruses in infants with multivariant HIV-1 infection. Nat Commun. (2020) 11:4409. doi: 10.1038/s41467-020-18225-x
16. Mishra N, Sharma S, Dobhal A, Kumar S, Chawla H, Singh R, et al. A rare mutation in an infant-derived HIV-1 envelope glycoprotein alters interprotomer stability and susceptibility to broadly neutralizing antibodies targeting the trimer apex. J Virol. (2020) 94:e00814-20. doi: 10.1128/JVI.00814-20
17. Aggarwal H, Khan L, Chaudhary O, Kumar S, Makhdoomi MA, Singh R, et al. Alterations in B cell compartment correlate with poor neutralization response and disease progression in HIV-1 infected children. Front Immunol. (2017) 8:1697. doi: 10.3389/fimmu.2017.01697
18. Makhdoomi MA, Khan L, Kumar S, Aggarwal H, Singh R, Lodha R, et al. Evolution of cross-neutralizing antibodies and mapping epitope specificity in plasma of chronic HIV-1-infected antiretroviral therapy-naïve children from India. J Gen Virol. (2017) 98:1879–91. doi: 10.1099/jgv.0.000824
19. Ditse Z, Muenchhoff M, Adland E, Jooste P, Goulder P, Moore PL, et al. HIV-1 subtype C infected children with exceptional neutralization breadth exhibit polyclonal responses targeting known epitopes. J Virol (2018) 92:17. doi: 10.1128/JVI.00878-18
20. Muenchhoff M, Adland E, Karimanzira O, Crowther C, Pace M, Csala A, et al. Nonprogressing HIV-infected children share fundamental immunological features of nonpathogenic SIV infection. Sci Transl Med. (2016) 8:358ra125. doi: 10.1126/scitranslmed.aag1048
21. Muenchhoff M, Prendergast AJ, Goulder PJR. Immunity to HIV in early life. Front Immunol. (2014) 5:391. doi: 10.3389/fimmu.2014.00391
22. Mishra N, Makhdoomi MA, Sharma S, Kumar S, Dobhal A, Kumar D, et al. Viral characteristics associated with maintenance of elite neutralizing activity in chronically HIV-1 clade C infected monozygotic pediatric twins. J Virol. (2019) 93(17):e00654-19. doi: 10.1128/JVI.00654-19
23. Khan L, Makhdoomi MA, Kumar S, Nair A, Andrabi R, Clark BE, et al. Identification of CD4-binding site dependent plasma neutralizing antibodies in an HIV-1 infected Indian individual. PloS One. (2015) 10:e0125575. doi: 10.1371/journal.pone.0125575
24. Kumar S, Kumar R, Khan L, Makhdoomi MA, Thiruvengadam R, Mohata M, et al. CD4-binding site directed cross-neutralizing scFv monoclonals from HIV-1 subtype C infected Indian children. Front Immunol. (2017) 8:1568. doi: 10.3389/fimmu.2017.01568
25. Khan L, Kumar R, Thiruvengadam R, Parray HA, Makhdoomi MA, Kumar S, et al. Cross-neutralizing anti-HIV-1 human single chain variable fragments(scFvs) against CD4 binding site and N332 glycan identified from a recombinant phage library. Sci Rep. (2017) 7:45163. doi: 10.1038/srep45163
26. Kumar S, Kumar R, Makhdoomi M, Khan L, Prakash S, Thiruvengadam R, et al. Production of cross neutralizing single chain fragment variables (scFv) from HIV-1 infected Indian children. BMC Infect Dis. (2014) 14:E25. doi: 10.1186/1471-2334-14-S3-E25
27. Briney B, Inderbitzin A, Joyce C, Burton DR. Commonality despite exceptional diversity in the baseline human antibody repertoire. Nature. (2019) 566:393–7. doi: 10.1038/s41586-019-0879-y
28. Gupta NT, Vander Heiden JA, Uduman M, Gadala-Maria D, Yaari G, Kleinstein SH. Change-O: a toolkit for analyzing large-scale B cell immunoglobulin repertoire sequencing data. Bioinformatics. (2015) 31:3356–8. doi: 10.1093/bioinformatics/btv359
29. Vander Heiden JA, Yaari G, Uduman M, Stern JNH, O’Connor KC, Hafler DA, et al. pRESTO: a toolkit for processing high-throughput sequencing raw reads of lymphocyte receptor repertoires. Bioinformatics. (2014) 30:1930–2. doi: 10.1093/bioinformatics/btu138
30. Yoon H, Macke J, West AP Jr, Foley B, Bjorkman PJ, Korber B, et al. CATNAP: a tool to compile, analyze and tally neutralizing antibody panels. Nucleic Acids Res. (2015) 43:W213–9. doi: 10.1093/nar/gkv404
31. Shi B, Dong X, Ma Q, Sun S, Ma L, Yu J, et al. The usage of human IGHJ genes follows a particular non-random selection: the recombination signal sequence may affect the usage of human IGHJ genes. Front Genet. (2020) 11:524413. doi: 10.3389/fgene.2020.524413
32. Cale EM, Gorman J, Radakovich NA, Crooks ET, Osawa K, Tong T, et al. Virus-like particles identify an HIV V1V2 apex-binding neutralizing antibody that lacks a protruding loop. Immunity. (2017) 46:777–791.e10. doi: 10.1016/j.immuni.2017.04.011
33. Shen CH, DeKosky BJ, Guo Y, Xu K, Gu Y, Kilam D, et al. VRC34-antibody lineage development reveals how a required rare mutation shapes the maturation of a broad HIV-neutralizing lineage. Cell Host Microbe. (2020) 27:531–543.e6. doi: 10.1016/j.chom.2020.01.027
34. Watkins JD, Siddappa NB, Lakhashe SK, Humbert M, Sholukh A, Hemashettar G, et al. An anti-HIV-1 V3 loop antibody fully protects cross-clade and elicits T-cell immunity in macaques mucosally challenged with an R5 clade C SHIV. PloS One. (2011) 6:e18207. doi: 10.1371/journal.pone.0018207
35. Sabin C, Corti D, Buzon V, Seaman MS, Lutje Hulsik D, Hinz A, et al. Crystal structure and size-dependent neutralization properties of HK20, a human monoclonal antibody binding to the highly conserved heptad repeat 1 of gp41. PloS Pathog. (2010) 6:e1001195. doi: 10.1371/journal.ppat.1001195
36. Bonsignori M, Kreider EF, Fera D, Meyerhoff RR, Bradley T, Wiehe K, et al. Staged induction of HIV-1 glycan-dependent broadly neutralizing antibodies. Sci Transl Med. (2017) 9. doi: 10.1126/scitranslmed.aai7514
37. Simonich C, Shipley MM, Doepker L, Gobillot T, Garrett M, Cale EM, et al. A diverse collection of B cells responded to HIV infection in infant BG505. Cell Rep Med. (2021) 2:100314. doi: 10.1016/j.xcrm.2021.100314
38. Bonsignori M, Zhou T, Sheng Z, Chen L, Gao F, Joyce MG, et al. Maturation pathway from germline to broad HIV-1 neutralizer of a CD4-mimic antibody. Cell. (2016) 165:449–63. doi: 10.1016/j.cell.2016.02.022
39. Andrabi R, Williams C, Wang XH, Li L, Choudhary AK, Wig N, et al. Cross-neutralizing activity of human anti-V3 monoclonal antibodies derived from non-B clade HIV-1 infected individuals. Virology. (2013) 439:81–8. doi: 10.1016/j.virol.2012.12.010
40. Cottrell CA, Manne K, Kong R, Wang S, Zhou T, Chuang GY, et al. Structural basis of glycan276-dependent recognition by HIV-1 broadly neutralizing antibodies. Cell Rep. (2021) 37:109922. doi: 10.1016/j.celrep.2021.109922
41. Williams LD, Ofek G, Schätzle S, McDaniel JR, Lu X, Nicely NI, et al. Potent and broad HIV-neutralizing antibodies in memory B cells and plasma. Sci Immunol. (2017) 2:eaal2200. doi: 10.1126/sciimmunol.aal2200
42. Andrabi R, Bhiman JN, Burton DR. Strategies for a multi-stage neutralizing antibody-based HIV vaccine. Curr Opin Immunol. (2018) 53:143–51. doi: 10.1016/j.coi.2018.04.025
43. Burton DR. What are the most powerful immunogen design vaccine strategies? Reverse vaccinology 2.0 shows great promise. Cold Spring Harb Perspect Biol. (2017) 9. doi: 10.1101/cshperspect.a030262
44. Kumar S, Singh S, Chatterjee A, Bajpai P, Sharma S, Katpara S, et al. Recognition determinants of improved HIV-1 neutralization by a heavy chain matured pediatric antibody. iScience. 26(9):107579. doi: 10.1016/j.isci.2023.107579
45. Nabi R, Moldoveanu Z, Wei Q, Golub ET, Durkin HG, Greenblatt RM, et al. Differences in serum IgA responses to HIV-1 gp41 in elite controllers compared to viral suppressors on highly active antiretroviral therapy. PloS One. (2017) 12:e0180245. doi: 10.1371/journal.pone.0180245
46. Wang Y, Yuan M, Lv H, Peng J, Wilson IA, Wu NC. A large-scale systematic survey reveals recurring molecular features of public antibody responses to SARS-CoV-2. Immunity. (2022) 55(6):1105–17. doi: 10.1101/2021.11.26.470157
Keywords: HIV-1, children, elite-neutralizers, B cell repertoire sequencing, bnAbs, clonotypes
Citation: Kumar S, Bajpai P, Joyce C, Kabra SK, Lodha R, Burton DR, Briney B and Luthra K (2024) B cell repertoire sequencing of HIV-1 pediatric elite-neutralizers identifies multiple broadly neutralizing antibody clonotypes. Front. Immunol. 15:1272493. doi: 10.3389/fimmu.2024.1272493
Received: 04 August 2023; Accepted: 02 February 2024;
Published: 16 February 2024.
Edited by:
Yiming Shao, Chinese Center for Disease Control and Prevention, ChinaReviewed by:
Suresh D. Sharma, United States Department of Health and Human Services, United StatesKevin Wiehe, Duke University, United States
David Easterhoff, Moderna Therapeutics, United States
Copyright © 2024 Kumar, Bajpai, Joyce, Kabra, Lodha, Burton, Briney and Luthra. This is an open-access article distributed under the terms of the Creative Commons Attribution License (CC BY). The use, distribution or reproduction in other forums is permitted, provided the original author(s) and the copyright owner(s) are credited and that the original publication in this journal is cited, in accordance with accepted academic practice. No use, distribution or reproduction is permitted which does not comply with these terms.
*Correspondence: Kalpana Luthra, kalpanaluthra@gmail.com; Bryan Briney, briney@scripps.edu
†These authors have contributed equally to this work