- 1Department of Medicine, University of Wisconsin-Madison, Madison, WI, United States
- 2William S. Middleton Memorial Veteran’s Hospital, Madison, WI, United States
Introduction: Anti-citrullinated protein antibodies (ACPAs) are a hallmark of rheumatoid arthritis, but the sources of citrullinated antigens as well as which peptidylarginine deiminases (PADs) are required for their production remain incompletely defined. Here, we investigated if macrophage extracellular traps (METs) could be a source of citrullinated proteins bound by APCAs, and if their formation requires PAD2 or PAD4.
Methods: Thioglycolate-induced peritoneal macrophages from wild-type, PAD2-/-, and PAD4-/- mice or human peripheral blood-derived M1 macrophages were activated with a variety of stimulants, then fixed and stained with DAPI and either anti-citrullinated histone H4 (citH4) antibody or sera from ACPA+ or ACPA- rheumatoid arthritis subjects. METs were visualized by immunofluorescence, confirmed to be extracellular using DNase, and quantified.
Results: We found that ionomycin and monosodium urate crystals reliably induced murine citH4+ METs, which were reduced in the absence of PAD2 and lost in the absence of PAD4. Also, IgG from ACPA+, but not ACPA-, rheumatoid arthritis sera bound to murine METs, and in the absence of PAD2 or PAD4, ACPA-bound METs were lost. Finally, ionomycin induced human METs that are citH4+ and ACPA-bound.
Discussion: Thus, METs may contribute to the pool of citrullinated antigens bound by ACPAs in a PAD2- and PAD4-dependent manner, providing new insights into the targets of immune tolerance loss in rheumatoid arthritis.
Introduction
Citrullinated proteins, formed by the post-translational modification of arginines to citrullines, are targeted by anti-citrullinated protein antibodies (ACPAs) in ~75% of people with rheumatoid arthritis (RA), a chronic autoimmune disease (1). There are five isoforms of human peptidylarginine deiminases (PADs) that catalyze citrullination, but primarily PAD2 and PAD4 have been implicated in RA due to their presence in immune cells (2) and RA synovial tissue (3), their contributions to murine inflammatory arthritis (4, 5), and the presence of RA-associated single nucleotide polymorphisms in both PADI2 and PADI4 (6, 7). However, the mechanisms by which these two PADs contribute to the high levels of citrullinated proteins in RA (8) that are bound by APCAs remain incompletely defined.
PAD4 is required for histone citrullination and the formation of citrullinated neutrophil extracellular traps (NETs) (9, 10), extracellular structures composed of decondensed chromatin and cellular proteins that are important in an antimicrobial response but also increased in RA and bound by ACPAs (11–13). Thus PAD4, via NETs, may provide citrullinated antigens, driving autoimmune responses in RA. However, despite being required for the formation of citrullinated NETs, PAD4 was not required for increased citrullination in murine inflammatory arthritis (4, 5). In contrast, PAD2 was required for joint citrullination in murine inflammatory arthritis but not NET production (5, 10). Thus, there may be additional sources of citrullinated antigens beyond NETs.
Macrophages also produce extracellular traps (METs) (14–16), but their role in RA is only emerging. RA synovial macrophage numbers correlate with increased articular destruction (17), while depletion reduces arthritis severity, splenic citrullination, and anti-cyclic citrullinated peptide (CCP) titers in murine inflammatory arthritis (18, 19). Further, citrullinated proteins, PAD4, and PAD2 appear to colocalize with macrophages in inflammatory tissue, and activated murine and RA synovial macrophages generate citrullinated METs (19, 20). However, it remains unknown if PAD4 or PAD2 is required for MET formation and if METs can make citrullinated proteins bound by ACPAs.
In this study we investigate the requirement of PAD4 and PAD2 in the production of METs that could be a source of citrullinated proteins bound by ACPAs.
Materials and methods
Mice
Similar numbers of male and female age- and sex-matched adult wild-type, PAD4-/- (9, 21) and PAD2-/- (22) mice back-crossed 12 generations to the C57BL/6 background were used. Animals were housed in a specific pathogen-free facility and experiments were approved by the University of Wisconsin Institutional Animal Care and Use Committee.
Human subjects
Human subjects research was approved by the University of Wisconsin Institutional Review Board and complied with the Helsinki Declaration. Sera from five RA subjects with anti–CCP and rheumatoid factor levels >2x the upper limit of normal were combined to generate the ACPA+ serum pool. Sera from five RA subjects with negative anti-CCP and rheumatoid factor test results were combined for the ACPA- serum pool. Samples were obtained from the University of Wisconsin Rheumatology Biorepository (23).
Murine macrophage isolation
Four days after intraperitoneal injection with aged 4% thioglycolate (Hardy Diagnostics), mice were euthanized for peritoneal lavage with 6-7 ml of cold RPMI with 2% heat-inactivated fetal bovine serum (FBS). The peritoneal fluid was centrifuged at 500xg for 5 minutes and the cell pellet resuspended in RPMI with L-glutamine, 2% FBS, and penicillin-streptomycin (macrophage media). The cell suspension was incubated in non-tissue culture treated plastic dishes at 37°C with 5% CO2 in a humidified incubator. The next day, the supernatant was aspirated, and the wells washed with RPMI and then phosphate buffered saline (PBS) prior to incubation with cold 2mM EDTA on ice for 30 minutes. Detached cells were collected, centrifuged at 500xg for 5 minutes, and resuspended in fresh macrophage media. By flow cytometry, cells were on average 86% F4/80+ macrophages and 0.17% Ly6G+Ly6C+ neutrophils with the remainder of cells primarily B cells and monocytes.
Human peripheral blood-derived M1 macrophage preparation
Frozen human peripheral blood-derived M1 macrophages (polarized with lipopolysaccharide [LPS] and interferon-gamma) from three independent healthy donors (StemCell Technologies) were thawed at 37°C until a small frozen cell pellet remained. The cell suspension was gently brought to a final volume of 20 ml of 37°C RPMI with 10% FBS, followed by centrifugation at 300xg for 10 minutes, gentle resuspension with 15 ml of 10% FBS in RPMI, and repeat centrifugation at 300xg for 10 minutes. Cells were resuspended in macrophage media and confirmed to be 99% CD14+CD80+CD68+ macrophages with no CD11b+CD15+CD14- neutrophils by flow cytometry.
Macrophage stimulation
Murine macrophages (3-6x104 cells/ml) were seeded in 500 µl of macrophage media in 24-well tissue culture plates containing acid washed glass coverslips, followed by incubation at 37°C with 5% CO2 for 30-60 minutes. After optimization of stimulant doses, cells received no treatment or were treated with 5x106 heat-killed Candida albicans (InvivoGen), 20 μM ionomycin (MilliporeSigma), 30 μg/mL LPS from E. coli (InvivoGen), 1.2 mg/mL monosodium urate (MSU) crystals (InvivoGen), 10 μg/mL platelet activating factor (PAF, MilliporeSigma), 50 nM phorbol myristate acetate (PMA, MP Biomedicals), or 400 ng/mL tumor necrosis factor alpha (TNFα, R&D Systems) followed by incubation for 20 hours at 37°C with 5% CO2.
Human macrophages (8x104 cells/ml) were seeded as above and incubated at 37°C with 5% CO2 for 4 hours. After optimization of stimulant doses, cells received no treatment or were treated with 6.5 μM ionomycin (MP Biomedicals) or 25 ng/mL TNFα (R&D Systems) followed by the above incubation parameters. The following concentrations of MSU were tested: 1.2 mg/ml, 400 µg/ml, and 130 µg/ml.
DNase experiments
After incubation with stimulants for 20 hours, coverslips were washed with PBS and one coverslip from each condition (per experiment) was incubated with 20 U/ml of DNase I in 1x DNase buffer (New England Biolabs) in PBS at 37°C with 5% CO2 for 20 minutes, while paired coverslips were incubated with DNase buffer in PBS without DNase I.
Immunofluorescence
Cells were prepared for immunofluorescence as previously described (10). For histone citrullination experiments, coverslips were washed with PBS, then treated with a solution of 4% paraformaldehyde, 1% NP-40, and 0.5% Triton X-100 in PBS for 30 minutes at 4°C, washed, blocked for 1 hour at room temperature with antibody blocking solution (2.5% bovine serum albumin, 5% goat serum, and 0.5% Tween-20 in PBS), incubated overnight at 4°C in a humidified chamber with rabbit anti-mouse histone H4 (citrulline 3) IgG (MilliporeSigma) diluted 1:100 in antibody blocking solution, washed, and incubated for 1 hour at room temperature with a 1:200 dilution of donkey anti-rabbit IgG conjugated to TRITC (Jackson Laboratories) and a 1:1000 dilution of 4′,6-diamidino-2-phenylindole (DAPI) in antibody blocking solution. Coverslips were washed and mounted on glass microscope slides using Prolong Diamond Antifade (Molecular Probes).
For ACPA binding experiments, coverslips were fixed with 4% paraformaldehyde in PBS for 30 minutes at 4°C, washed, and then blocked in either murine ACPA blocking solution (2.5% bovine serum albumin and 5% goat serum in PBS) for 3 hours at 4°C or human ACPA blocking solution (5% bovine serum albumin and 10% goat serum) with human IgG1 Fc block (BD Biosciences) in PBS overnight at 4°C, before incubation with ACPA+ or ACPA- sera diluted 1:75 in respective blocking solution overnight at 4°C in a humidified chamber. Coverslips were then washed, permeabilized with 1% NP-40, 0.5% Triton X-100, and 0.5% Tween-20 in PBS for 30 minutes at 4°C, washed, incubated with 1:200 goat IgG anti-human IgG conjugated to TRITC (SouthernBiotech) and 1:1000 DAPI in respective blocking solution with 0.5% Tween-20 for 1 hour at room temperature followed by washing and mounting as above.
Quantification of METs
For murine experiments, a Leica fluorescence microscope with Image Pro-Plus v.6.3 (Media Cybernetics) was used to image five pre-determined areas on each coverslip at 400x. For human experiments, images were acquired on a Leica DMi8 inverted microscope with Leica Application Suite X at 200X. For each image, total cells, MET-like structures (defined as decondensed or diffuse DNA structures), and non-METs (defined as condensed nuclei) were counted by eye in a blinded manner (DNase treatment, stimulant, genotype, and ACPA presence unknown to counters) using ImageJ.
Confocal imaging
Confocal images of coverslips were acquired with a Nikon A1-RS scanning confocal microscope with a 40x oil objective. Images were prepared with NIS-Elements software.
Statistical analysis
Data were analyzed by paired t-test using Prism (GraphPad) with p<0.05 considered significant.
Results
To evaluate stimuli that induce METs and engage PADs to produce citrullinated METs in mice, we treated murine peritoneal macrophages in vitro with seven stimuli previously shown to induce extracellular traps in neutrophils and/or macrophages (5, 10, 16, 19, 24). After fixation and permeabilization, cells were stained with DAPI and anti-citrullinated histone H4 (citH4, Figure 1A), and the percentage of cells that formed citH4+ or citH4- MET-like structures (defined by decondensed or diffuse chromatin with or without citrullinated histones) was quantified. To ensure that the MET-like structures were extracellular, cells were treated with DNase prior to permeabilization (Figure 1B) and MET percentages compared with non-DNase treated cells. As quantified in Figure 1C, Candida, ionomycin, MSU, and PAF induced MET-like structures, but only the MET-like structures induced by ionomycin, MSU, and PAF were reduced with DNase treatment, indicating true extracellular structures. Further, only ionomycin, MSU, and PAF significantly induced citH4+ MET-like structures, which were lost with DNase treatment (Figure 1D). Interestingly, Candida, LPS, and TNFα appeared to induce citH4- MET-like structures (Figure 1E). However, the MET-like structures induced by Candida and LPS were not reduced with DNase treatment, suggesting that they were not truly METs. Finally, although the percentage of MET-like structures was small, combining the data from Figures 1C, E, TNFα appeared to induce citH4- MET-like structures that were degraded by DNase and thus would be citH4- METs. Of note, no difference was observed in METs between male and female mice (Supplementary Figure 1). Together, these data suggest that ionomycin, MSU, and PAF are activators of DNase-accessible, true citH4+ METs in murine peritoneal macrophages. Thus, moving forwards, we use the term “MET” and not “MET-like structure”.
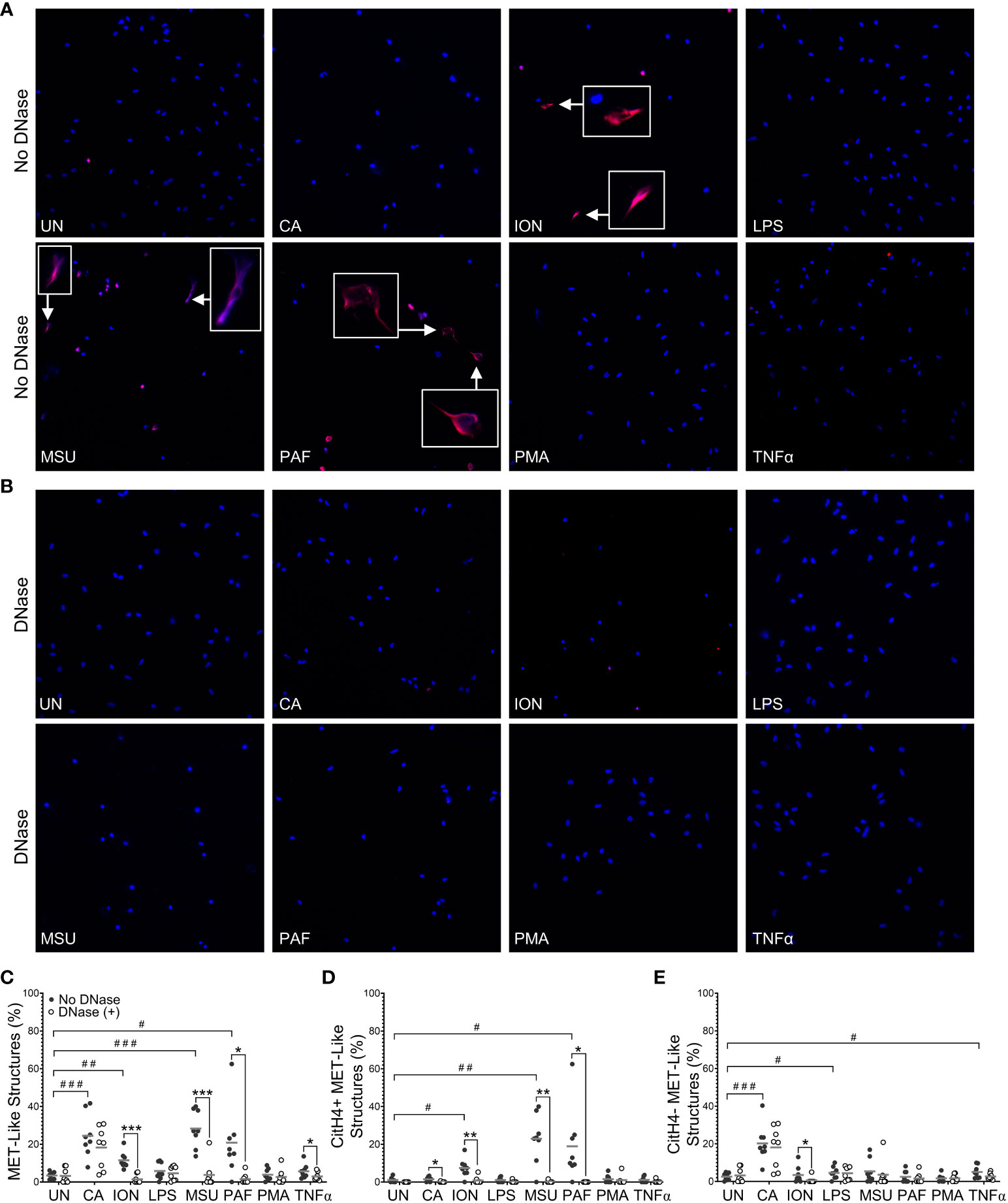
Figure 1 Murine macrophages produce METs in response to specific stimuli. Murine peritoneal macrophages were left untreated (UN) or were treated with heat-killed Candida albicans (CA), ionomycin (ION), lipopolysaccharide (LPS), monosodium urate (MSU) crystals, platelet activating factor (PAF), phorbol myristate acetate (PMA), or tumor necrosis factor alpha (TNFα) followed by treatment with no DNase or DNase, then fixation and permeabilization, staining with DAPI (blue) and anti-citrullinated histone H4 (citH4) antibody (pink), imaging, and quantification of MET-like structures. Representative images at 400x of no DNase (A) or DNase (B) treated macrophages. Arrows indicate citH4+ MET-like structures and insets are enlarged 3x. Graphs depict the means and individual values for the percent of macrophages that formed total (C), citH4+ (D), or citH4- (E) MET-like structures for each stimulant compared to untreated for the non-DNase treated samples (#p<0.05, ##p<0.01, ###p<0.001) and for DNase compared to no DNase for each stimulant (*p<0.05, **p<0.01, ***p<0.001) by paired t-test (n=8).
To determine if PAD4 is required to produce citH4+ METs, we performed similar experiments as above with macrophages from PAD4+/+ and PAD4-/- mice stimulated with ionomycin and MSU (Figure 2). Ionomycin mimics the calcium flux that occurs in activated immune cells, including macrophages (25), and MSU induces IL-1ß via the NLRP3 inflammasome, a pathogenic structure in RA macrophages (26, 27). Thus, both stimuli are highly relevant for RA. Because PAF-induced METosis could be variable, it was not selected for further use. As shown in Figure 2B, PAD4-/- macrophages formed significantly fewer METs than PAD4+/+ macrophages in response to ionomycin and MSU. Further, PAD4-/- macrophages did not produce any citH4+ METs in response to stimuli (Figure 2C). The small number of METs produced in the absence of PAD4 appeared to be citH4- (Figure 2D). In addition to quantifying citH4+ METs, we also quantified citH4+ non-MET macrophages, i.e., macrophages with condensed nuclei. CitH4+ non-MET macrophages were also nonexistent in the absence of PAD4 (Figure 2E). Thus, PAD4 is required for histone H4 citrullination in stimulated macrophages and for the production of citH4+ METs.
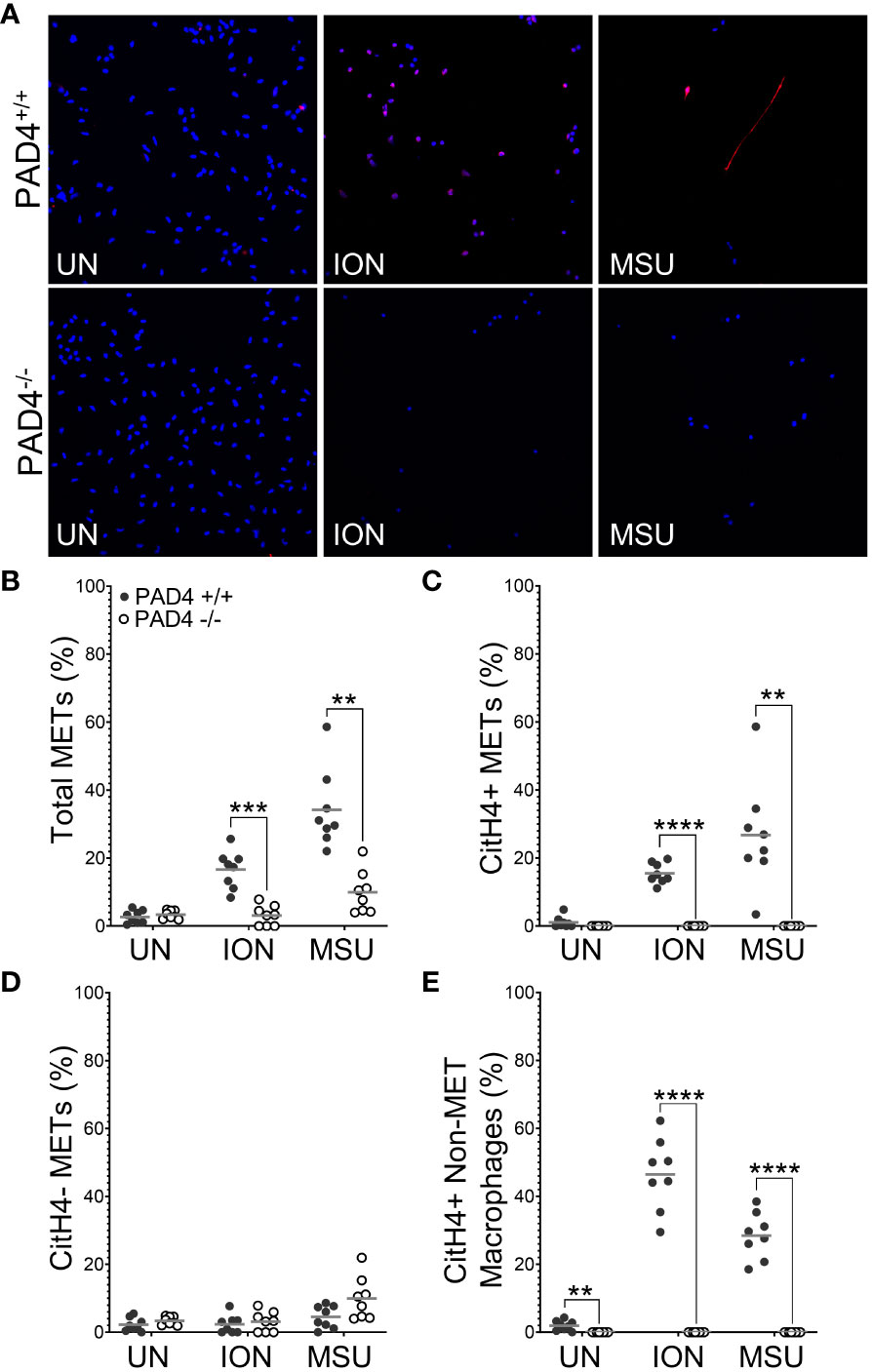
Figure 2 Citrullinated histone-containing murine METs and non-MET macrophages are lost in the absence of PAD4. Peritoneal macrophages from PAD4+/+ and PAD4-/- mice were left untreated (UN) or were treated with ionomycin (ION) or monosodium urate (MSU) crystals and stained with DAPI (blue) and anti-citrullinated histone H4 (citH4) antibody (pink). (A) Representative images at 400x. Graphs depict the means and individual values for the percentage of macrophages that were total METs (B), citH4+ METs (C), citH4- METs (D), and citH4+ non-MET macrophages (E) for each condition. For all panels: PAD4+/+ and PAD4-/- were compared by paired t-test (**p<0.01, ***p<0.001, ****p<0.0001) and n=8.
We then used identical methods and PAD2+/+ and PAD2-/- mice to determine if PAD2 is required for MET production. As shown in Figure 3B, total METs were reduced for PAD2-/- macrophages in response to ionomycin and MSU, similar to PAD4-/- macrophages. However, lack of PAD2 led to a reduction, but not complete absence, of citH4+ METs (Figure 3C). Similar to PAD4-/- macrophages, citH4- METs were not reduced in the absence of PAD2. Finally, the number of citH4+ non-MET macrophages was only minimally reduced and only for MSU stimulation in the absence of PAD2, as opposed to a complete absence of citH4+ non-MET macrophages for the PAD4 knockout. Together these data suggest that both PAD4 and, to a lesser extent, PAD2 are required to produce citH4+ METs.
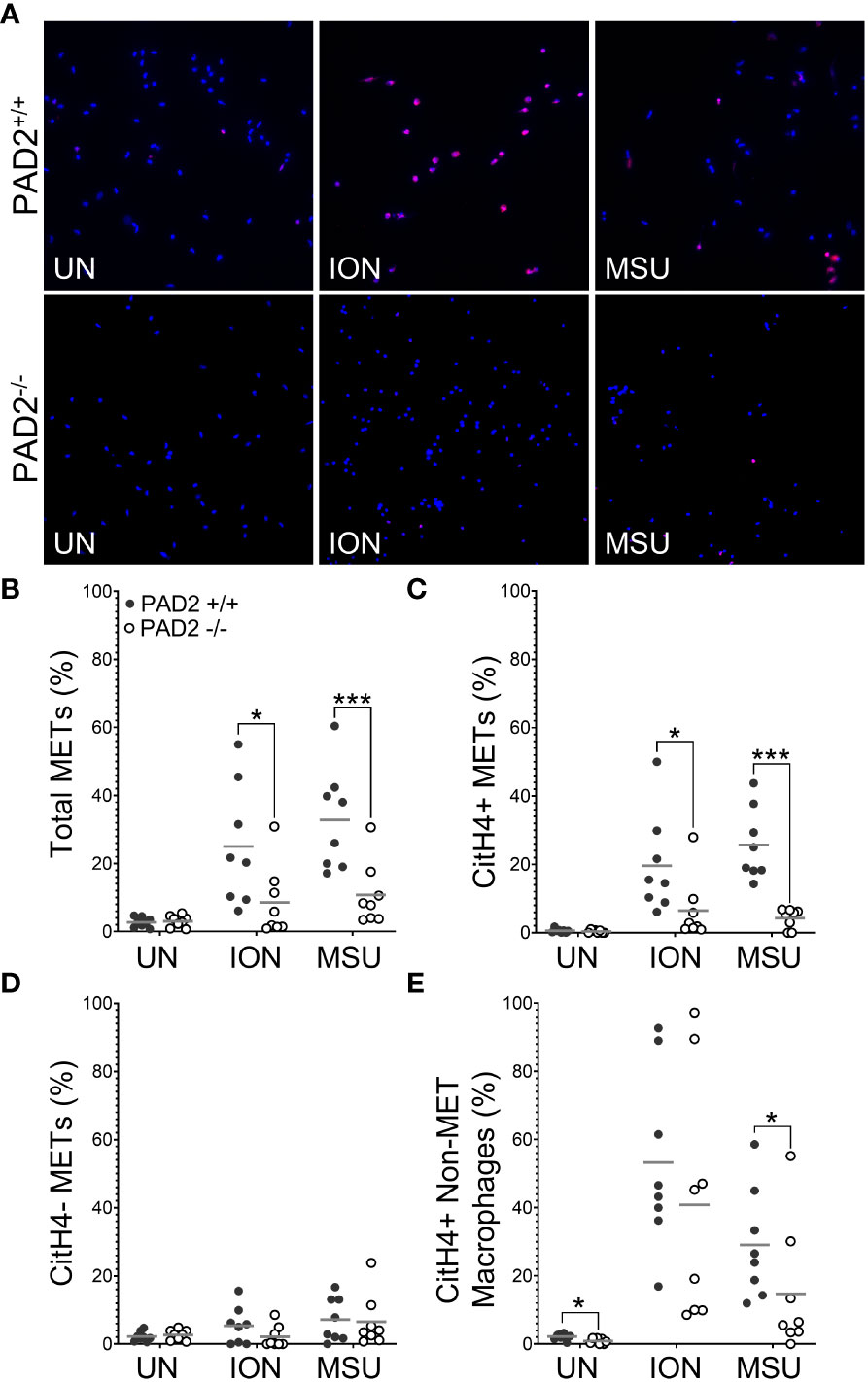
Figure 3 Citrullinated histone-containing murine METs are reduced in the absence of PAD2. Peritoneal macrophages from PAD2+/+ and PAD2-/- mice were left untreated (UN) or were treated with ionomycin (ION) or monosodium urate (MSU) crystals and stained with DAPI (blue) and anti-citrullinated histone H4 (citH4) antibody (pink). (A) Representative images at 400x. Graphs depict the means and individual values for the percentage of total METs (B), citH4+ METs (C), citH4- METs (D), and citH4+ non-MET macrophages (E) for each condition. For all panels: PAD2+/+ and PAD2-/- were compared by paired t-test (*p<0.05, ***p<0.001) and n=8.
Next, we determined if the citrullinated antigens present in murine METs or activated macrophages could be bound by ACPAs. We repeated the above experiments with wild-type mice and two modifications: (1) incubating macrophages with human sera pooled from ACPA+ or ACPA- RA subjects instead of anti-citH4 antibody and (2) permeabilization after incubation with sera, instead of before, to better mimic the endogenous biology as opposed to the goal of detecting histone citrullination in all cells. As shown in Figures 4A, B, essentially no binding of IgG from ACPA- RA sera to METs was detected, whereas IgG from ACPA+ RA sera bound to ionomycin- and MSU-activated METs. Binding of IgG from ACPA+ sera to METs was similar in males and females (Supplementary Figure 2). We also detected IgG binding for APCA+ sera, but not ACPA- RA sera, to non-MET macrophages after stimulation with ionomycin or MSU (Figure 4C). Since the cells were not permeabilized prior to incubation with ACPA+ sera, this observation suggested either binding to citrullinated proteins on the cell membrane or activation-induced membrane permeabilization, allowing ACPAs access to intracellular antigens. To differentiate between the two possibilities, we used confocal imaging. We observed intracellular staining with ACPA+ sera (Figure 4D), which is consistent with membrane permeabilization. Finally, we compared ACPA-bound METs and non-MET macrophages with and without DNase treatment (Supplementary Figure 3). As expected, all METs were lost upon DNase treatment. In contrast, the ACPA-bound non-MET macrophages were unaffected by DNase, suggesting that citrullinated proteins in the cytoplasm of activated macrophages are accessible to ACPAs, while the DNA in the nucleus is not accessible to DNase.
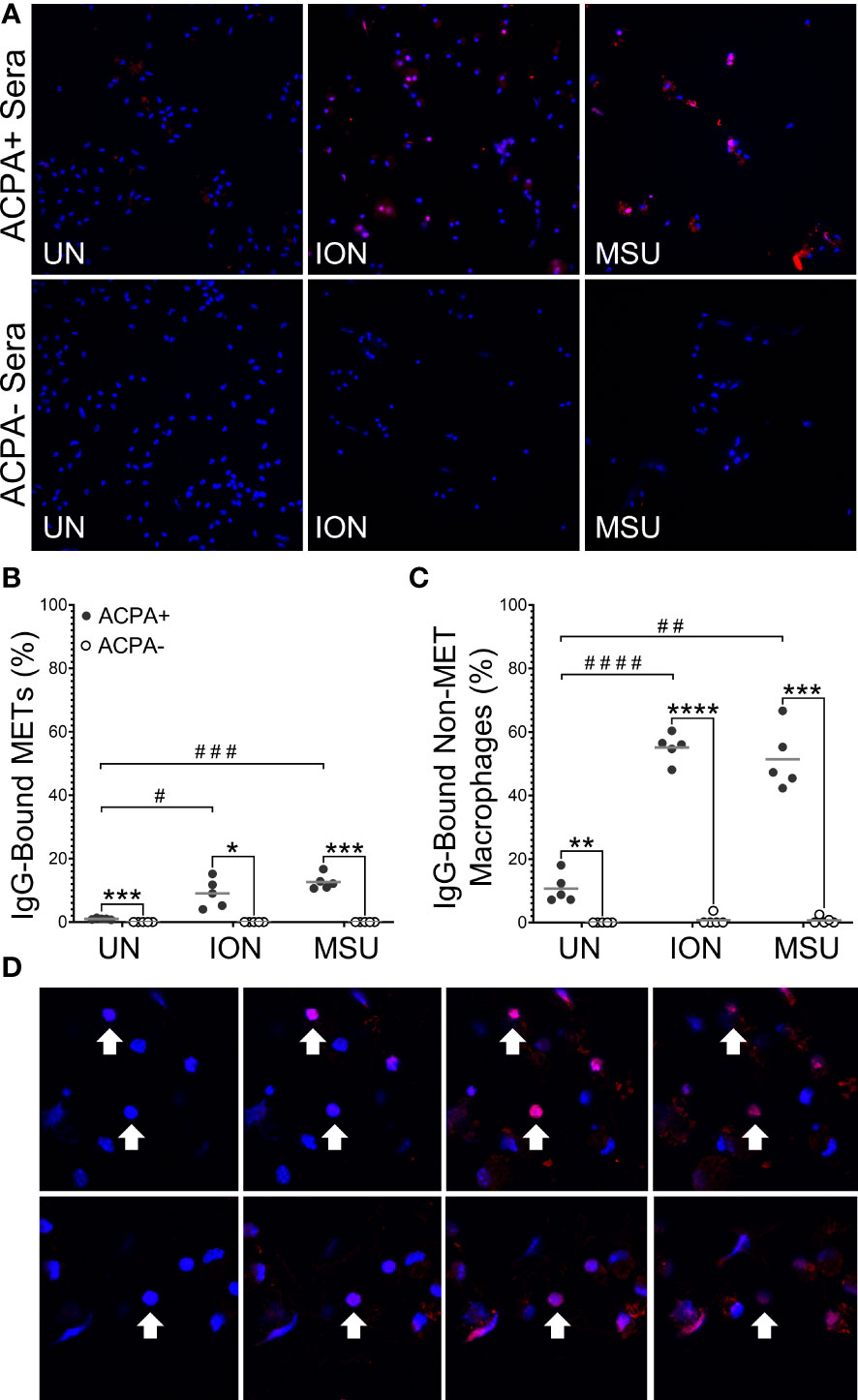
Figure 4 Anti-citrullinated protein antibodies (ACPAs) bind to murine METs and activated macrophages. Untreated (UN), ionomycin (ION)-treated or monosodium urate (MSU) crystal-treated macrophages were fixed, incubated with sera from ACPA+ or ACPA- rheumatoid arthritis subjects, then permeabilized and stained with DAPI (blue) and anti-human IgG antibody (pink). (A) Representative images at 400x. Graphs depict the means and individual values for the percentage of macrophages that were IgG-bound METs (B) or IgG-bound non-MET macrophages (C) for each stimulant compared to the untreated control for ACPA+ sera (paired t-test; #p<0.05, ##p<0.01, ###p<0.001, #### p<0.0001) and for ACPA+ versus ACPA- for each condition (paired t-test; *p<0.05, **p<0.01, ***p<0.001, ****p<0.0001); n=5. (D) Representative 1.5 μm z section intervals obtained by confocal microscopy of three representative non-MET macrophages (arrows) that had been incubated with ACPA+ sera.
Then, we determined if PAD4 or PAD2 is required to generate murine METs or activated macrophages that can be bound by ACPAs. We repeated the above ACPA experiments using wild-type, PAD4-/-, and PAD2-/- mice. We found that in the absence of PAD4 (Figure 5) or PAD2 (Figure 6), there was a dramatic reduction in ACPA-bound METs and a lesser reduction in ACPA-bound non-MET macrophages. Interestingly, METs that were not ACPA-bound also were reduced in the absence of PAD2 or PAD4, potentially highlighting overall reduced binding by ACPA+ sera as compared to the anti-citH4 antibodies. Of note, we also performed the same experiment using each ACPA+ serum sample independently with overall similar results and some differences in staining patterns (Supplementary Figures 4, 5). Taken together, our findings suggest that activated murine macrophages and METs have citrullinated proteins that are ACPA targets, and the generation of those citrullinated proteins is dependent upon both PAD4 and PAD2.
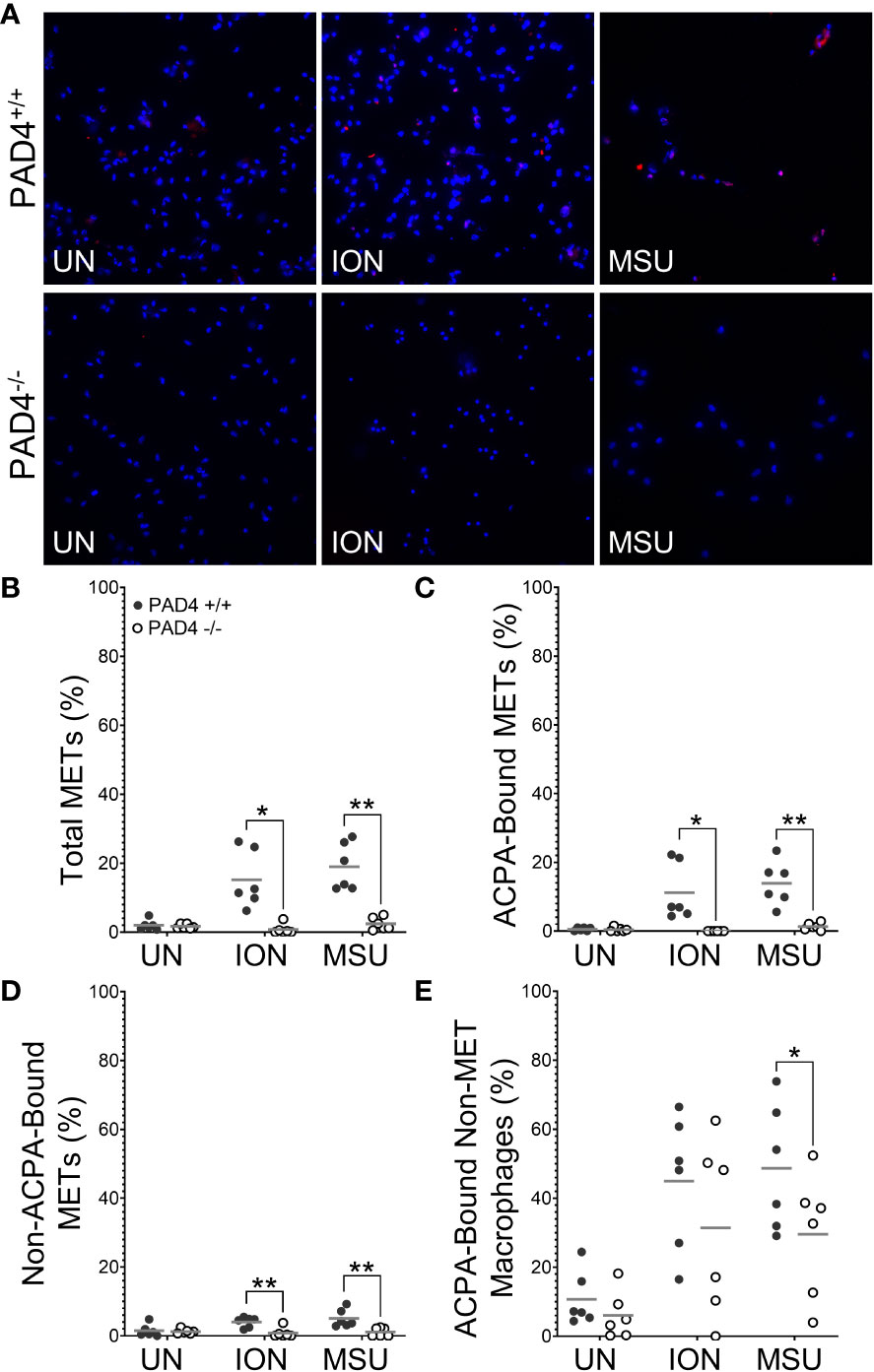
Figure 5 ACPA-bound murine METs are lost in the absence of PAD4. Peritoneal macrophages from PAD4+/+ and PAD4-/- mice were left untreated (UN) or were treated with ionomycin (ION) or monosodium urate (MSU) crystals, then fixed, incubated with ACPA+ rheumatoid arthritis sera, permeabilized, and stained with DAPI (blue) and anti-human IgG antibody (pink). (A) Representative images at 400x. Graphs depict the means and individual values for the percentage of macrophages that are total METs (B), ACPA-bound METs (C), METs not bound by ACPAs (D), or ACPA-bound non-MET macrophages (E). For all panels: PAD4+/+ and PAD4-/- were compared by paired t-test (*p<0.05, **p<0.01) and n=6.
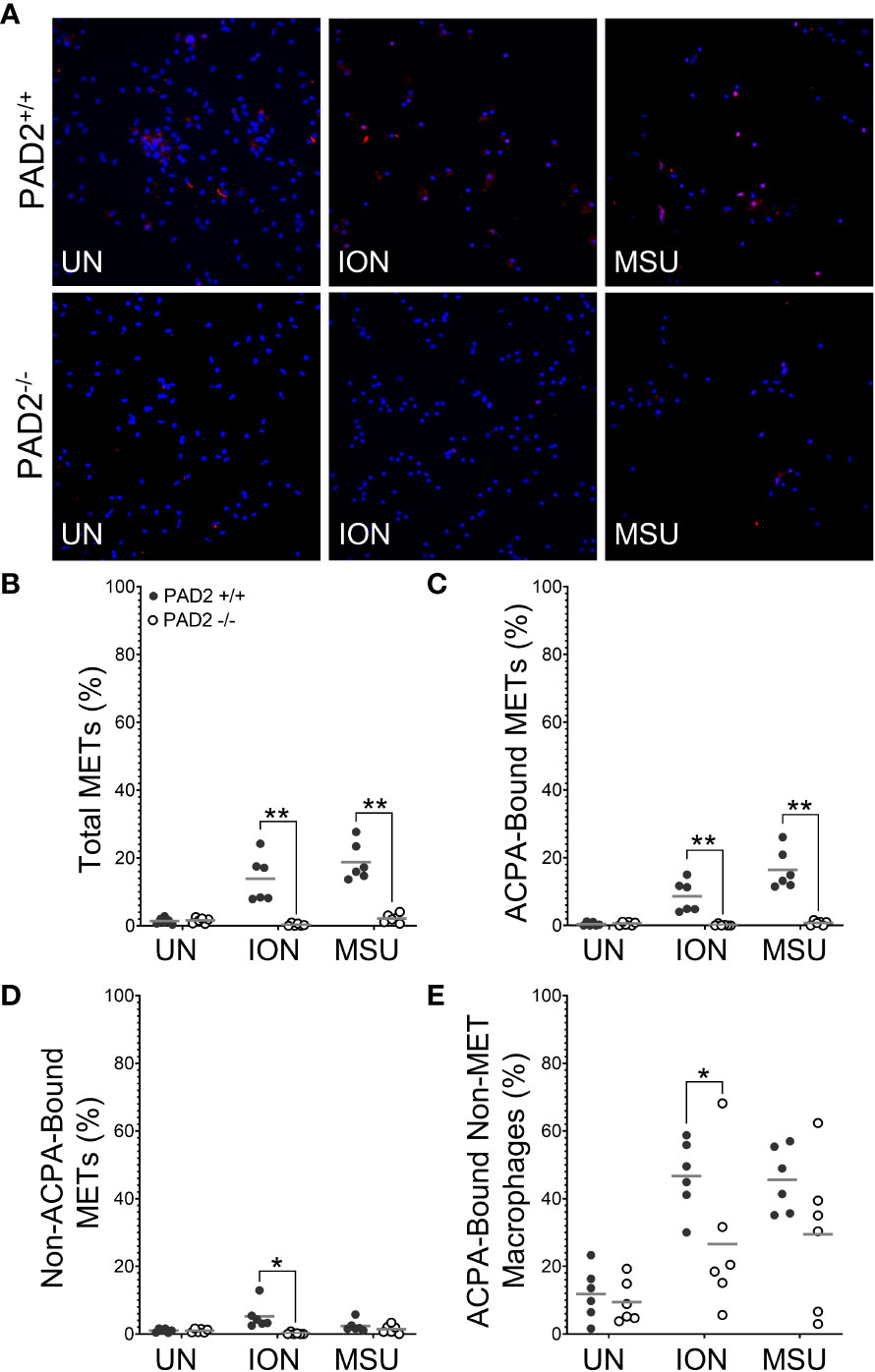
Figure 6 ACPA-bound murine METs are lost in the absence of PAD2. Peritoneal macrophages from PAD2+/+ and PAD2-/- mice were left untreated (UN) or were treated with ionomycin (ION) or monosodium urate (MSU) crystals, then fixed, incubated with ACPA+ rheumatoid arthritis sera, permeabilized, and stained with DAPI (blue) and anti-human IgG antibody (pink). (A) Representative images at 400x. Graphs depict the means and individual values for the percentage of macrophages that are total METs (B), ACPA-bound METs (C), METs not bound by ACPAs (D), and ACPA-bound non-MET macrophages (E). For all panels: PAD2 +/+ and PAD2-/- were compared by paired t-test (*p<0.05, **p<0.01) and n=6.
Finally, to provide additional human connection, we evaluated the ability of human M1 macrophages to generate ACPA targets. First, we evaluated the capacity of ionomycin, MSU, and TNFα to generate human METs (Figure 7). We found that three concentrations of MSU led to a loss of almost all human macrophages from the coverslips. However, ionomycin induced citH4+ MET-like structures that were lost with DNase, indicating true citH4+ METs. TNFα gave similar citH4+ MET results that did not achieve statistical significance. We then evaluated the ability of IgG from human ACPA+ RA sera to bind to human macrophages and METs. As shown in Figure 8, IgG from ACPA+ sera bound ionomycin-induced human METs and non-MET macrophages more than IgG from ACPA- RA sera with similar results for TNFα. Taken together, these experiments provide the first evidence that human activated macrophages and METs generate citrullinated antigens bound by human RA ACPAs.
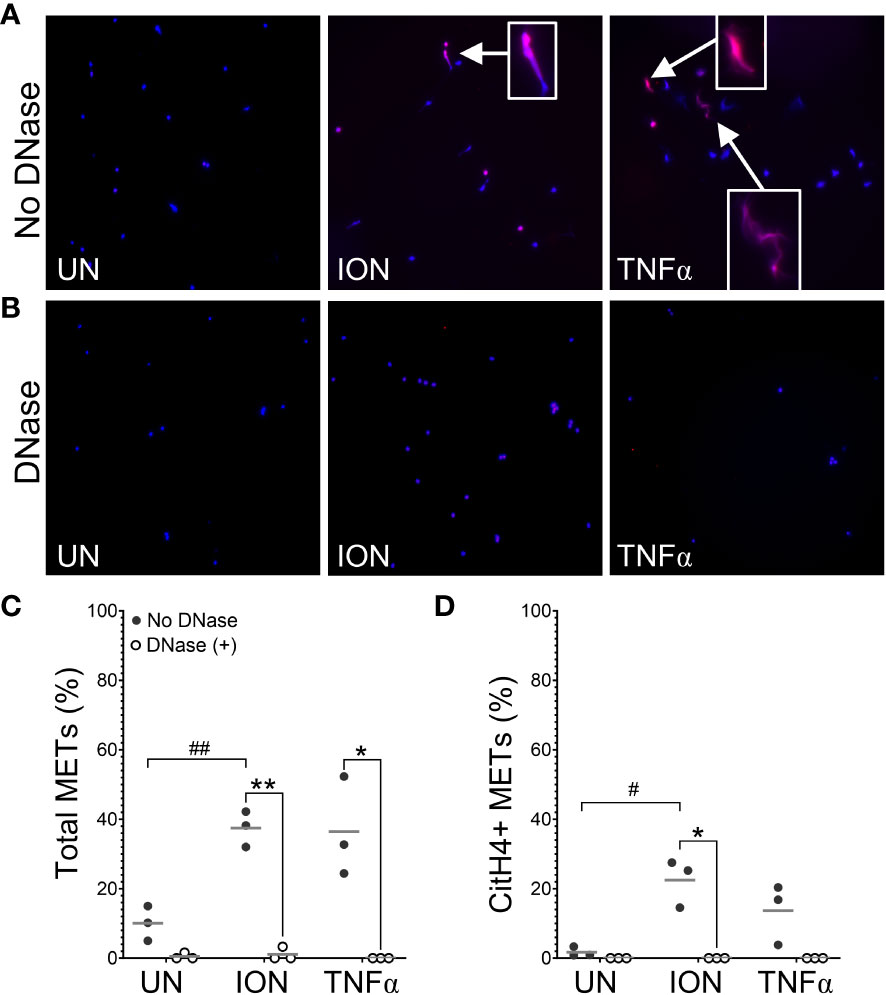
Figure 7 Human macrophages produce citrullinated histone-containing METs. Human peripheral blood-derived M1 macrophages were left untreated (UN) or were treated with ionomycin (ION) or tumor necrosis factor alpha (TNFα) followed by treatment with no DNase or DNase, then fixation and permeabilization, staining with DAPI (blue) and anti-citrullinated histone H4 (citH4) antibody (pink), imaging, and quantification of MET-like structures. Representative images at 200x of no DNase (A) or DNase (B) treated human macrophages. Arrows indicate citH4+ MET-like structures and insets are enlarged 3x. Graphs depict the means and individual values for the percent of macrophages that formed total (C) or citH4+ (D) MET-like structures for each stimulant compared to untreated for the non-DNase treated samples (#p<0.05, ##p<0.01) as well as for DNase compared to no DNase for each stimulant (*p<0.05, **p<0.01) by paired t-test (n=3).
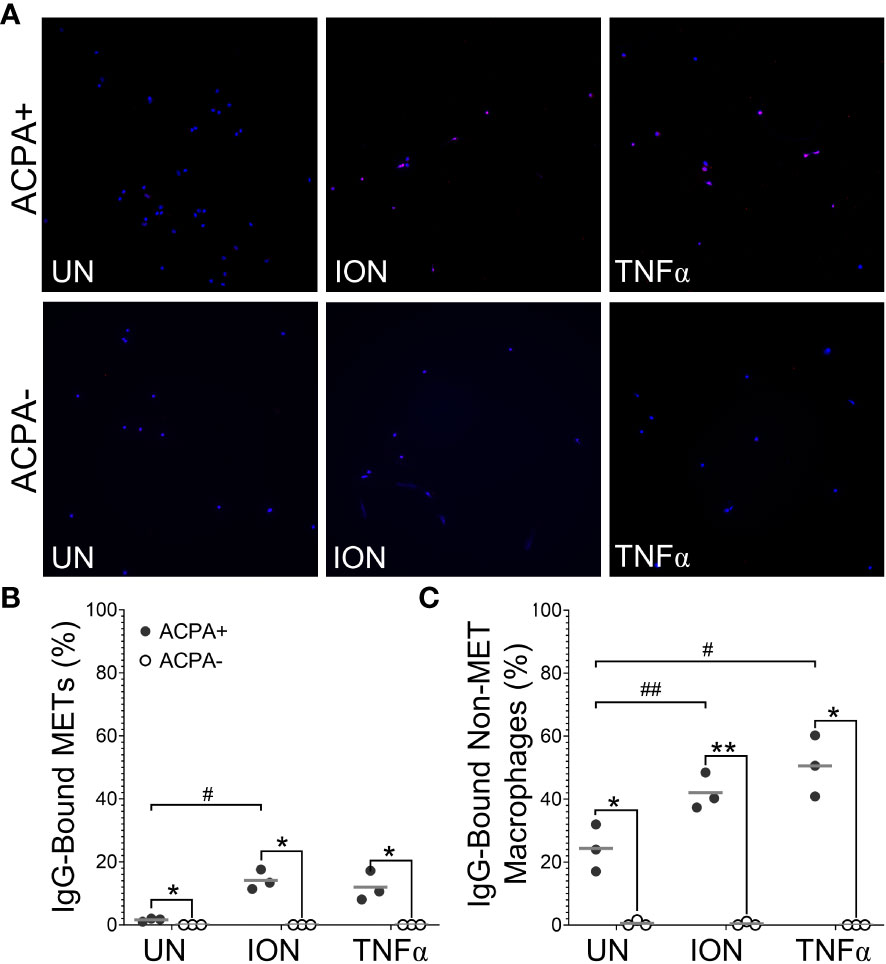
Figure 8 Anti-citrullinated protein antibodies (ACPAs) bind to human METs and activated non-MET macrophages. Untreated (UN), ionomycin (ION)-treated or tumor necrosis factor alpha (TNFα)-treated human M1 macrophages were fixed, incubated with sera from ACPA+ or ACPA- rheumatoid arthritis subjects, then permeabilized and stained with DAPI (blue) and anti-human IgG antibody (pink). (A) Representative images at 200x. Graphs depict the means and individual values for the percentage of macrophages that were IgG-bound METs (B) or IgG-bound non-MET macrophages (C) for each stimulant compared to the untreated control for ACPA+ sera (#p<0.05, ##p<0.01) and for ACPA+ versus ACPA- for each condition (*p<0.05, **p<0.01) by paired t-test (n=3).
Discussion
In this study, we report that, in response to ionomycin and MSU, murine macrophages generate citrullinated extracellular traps in a PAD4- and PAD2-dependent manner. We also show that ACPA+ sera can bind to murine and human METs, suggesting that METs can provide ACPA targets dependent upon PAD2 and PAD4.
Compared to NETs, the study of METs is only beginning. Our study investigated the ability of several stimuli to induce citH4+ and citH4- METs in thioglycolate-induced murine peritoneal macrophages (Figure 1). Although no murine model perfectly recapitulates human disease, stimulated murine peritoneal macrophages have a similar inflammatory phenotype as RA macrophages, allowing them to serve as a reasonable model of human pre-RA and RA macrophages (28, 29). In these cells, we demonstrate, for what we believe is the first time, that ionomycin, MSU, and PAF induce citH4+ METs. We also demonstrate for the first time that ionomycin induces human citH4+ METs, although a different calcium ionophore was previously shown to induce METs detected by DNA staining (30). In contrast, three concentrations of MSU led to a loss of human macrophages in general, perhaps consistent with MSU being unable to induce extracellular traps in human mononuclear cells (31).
We did not observe MET production in response to some stimuli, such as PMA or LPS, consistent with previous findings for murine peritoneal macrophages (16). This finding is in contrast to the ability of LPS and PMA to induce METs in murine splenic macrophages (19), suggesting that tissue of origin may influence METosis. Interestingly, human monocyte-derived macrophages generate METs in response to PMA (30), consistent with our previous observation that PMA induces NETs in human, but not murine, neutrophils (10). We also found that heat-killed Candida induced nuclear enlargement, but not extracellular traps. Previous studies report that murine macrophages can form METs in response to live Candida (16, 32), so perhaps live Candida induces more METosis than heat-killed Candida in murine macrophages. Finally, we found that TNFα induced primarily citH4- METs in mice. This systematic evaluation of citrullination and confirmation of extracellular structures with DNase provides new insights into murine MET activators. Similar studies are needed for human METs, given reported differences for human and murine NETs and different methodologies (10).
Using both human and murine METs, we also demonstrate that METs are a potential source of citrullinated autoantigens bound by ACPAs, similar to NETs (11, 12), although the relative contributions of METs and NETs in the initiation and propagation of RA inflammation are unknown. Others have demonstrated that human and murine METs are citrullinated (15, 19), but, to the best of our knowledge, this study is the first to demonstrate that human RA ACPAs bind to murine and human METs. IgG from ACPA+ human RA sera bound murine METs due either to conserved epitopes between mice and humans or due to the previously demonstrated multi-reactivity of ACPAs related to the recognition of very short motifs (33, 34). A caveat to our findings is the use of ACPA+ sera, not purified ACPAs; thus, we cannot exclude the possibility that a non-ACPA IgG, such as rheumatoid factor or an anti-homocitrullinated protein antibody, in the ACPA+ sera bound to the murine METs. However, multi-reactive ACPAs have rheumatoid factor activity (35) and can bind to homocitrullinated antigens (33), so eliminating all multi-reactivity while keeping ACPAs may not be possible. Nonetheless, IgG binding occurred using ACPA+ and not ACPA- RA sera, indicating that RA autoantibodies can bind METs. Thus, METs are a target of immune tolerance loss in RA, likely due to the presence of citrullinated proteins given the dependence on PAD2 and PAD4. Future studies are needed to evaluate the binding of purified ACPAs to human METs as well as to address the relative contributions of METs and NETs in the initiation and propagation of RA inflammation.
Additionally, we show that both PAD2 and PAD4 are required for the production of citrullinated, ACPA-bound murine METs. For PAD4, this finding is similar to the requirement for PAD4 in citrullinated NETs (10). However, the finding that PAD2 is required for citrullinated METs (Figures 3, 6) is, to the best of our knowledge, the first demonstration that PAD2 is required for any form of ETosis. Using knockout mice, we previously found that PAD2 was dispensable for NET formation (5, 10). Also, PADs, and PAD2 in particular, were found to be dispensable for human monocyte-derived macrophage ETosis based on inhibitor and PAD2 siRNA experiments (30). It is not clear if the discrepancy with that study is due to our complete genetic knockout of PAD2 versus incomplete PAD inhibition by Cl-amidine or siRNA, our use of peritoneal macrophages versus peripheral blood monocyte-derived macrophages, differences between mice and humans, or our quantification of METs versus representative images. A requirement for PAD2 in METosis provides a potential mechanism for the observation that PAD2 is required for the citrullination seen in murine arthritis (5) and the close correlation of synovial fluid PAD2 levels with synovial fluid PAD activity and RA disease activity (36). It will be important for future studies to thoroughly evaluate a role for PADs in human METs using a variety of methods.
A caveat to our citrullination findings is that we quantified citrullinated METs using an antibody that detects citrullination of Arg3 in histone H4, a citrulline that may specifically depend upon PAD4 (37). The use of this antibody may explain the complete loss of citH4+ METs in PAD4-/- macrophages versus the partial loss in PAD2-/- macrophages. We previously used F95 as a pan anti-citrulline antibody (10), but recent lots of F95 did not detect citrullination in our hands (data not shown). However, similar results were seen with F95 and anti-citrullinated histone H4 antibody for NETs (10). Moreover, the ACPA+ serum experiments provide similar results to the anti-citrullinated histone experiments.
Finally, we quantified citH4+ and ACPA-bound non-METs in addition to METs, providing some interesting insights. For example, ACPA-bound non-MET macrophages are not sensitive to DNase, suggesting an intact nuclear membrane (protecting DNA from DNase) and a permeable cell membrane (allowing ACPA entry to the cytoplasm). These findings could be due to the pore-forming capabilities of ionomycin and MSU (38–40) or a mechanism of ETosis in which cell membrane permeabilization is an early event as opposed to the early loss of nuclear membrane integrity in NETosis (41). Either way, non-MET macrophages might also provide some citrullinated antigen with the relative contributions of METs and activated non-MET macrophages unknown. Also, non-MET macrophages with citrullinated histone H4 are lost in the absence of PAD4, whereas they are minimally reduced in the absence of PAD2. In contrast, ACPA-bound non-MET macrophages were only minimally reduced in the absence of PAD2 or PAD4. Since the non-MET macrophages may be early or incomplete METs, PAD4 may be required early in METosis to citrullinate histone H4, whereas PAD2 is more dispensable early for histone H4 citrullination. Also, whereas PAD4 appears to be absolutely required for histone H4 citrullination, PAD2 and PAD4 can partially compensate for each other in the citrullination of other targets in early METosis. In contrast, PAD2 and PAD4 are each required independently for full METosis.
In sum, our work demonstrates that both PAD2 and PAD4 are required for the production of citrullinated METs that are bound by ACPAs. Although further work is needed to fully evaluate PAD2 and PAD4 in human METs, our findings provide a new potential source of citrullinated antigens bound by ACPAs in RA and demonstrate the importance of both PAD2 and PAD4 in the generation of those antigens.
Data availability statement
The original contributions presented in the study are included in the article/Supplementary Material. Further inquiries can be directed to the corresponding author.
Ethics statement
The studies involving humans were approved by University of Wisconsin Institutional Review Board. The studies were conducted in accordance with the local legislation and institutional requirements. The participants provided their written informed consent to participate in this study. The animal study was approved by University of Wisconsin Institutional Animal Care and Use Committee. The study was conducted in accordance with the local legislation and institutional requirements.
Author contributions
SB generated and analyzed data, prepared figures, and wrote the first draft of the manuscript. CH generated and analyzed data. MS conceptualized and oversaw the research. All authors edited the manuscript and approved the final version.
Funding
The author(s) declare financial support was received for the research, authorship, and/or publication of this article. This project was supported by the US Army Medical Research Acquisition Activity through the Peer Reviewed Medical Research Program (award W81XWH-18-1-0717 to M.A.S.) and C.L.H. was supported by the National Institutes of Health, National Heart, Lung, and Blood Institute (T32HL07899).
Acknowledgments
We thank Rozalyn Anderson and Eric McGregor for the use of and assistance with fluorescent light microscopes as well as Lance Rodenkirch and the University of Wisconsin-Madison Imaging Core for the guided use of the scanning confocal microscope.
Conflict of interest
The authors declare that the research was conducted in the absence of any commercial or financial relationships that could be construed as a potential conflict of interest.
Publisher’s note
All claims expressed in this article are solely those of the authors and do not necessarily represent those of their affiliated organizations, or those of the publisher, the editors and the reviewers. Any product that may be evaluated in this article, or claim that may be made by its manufacturer, is not guaranteed or endorsed by the publisher.
Supplementary material
The Supplementary Material for this article can be found online at: https://www.frontiersin.org/articles/10.3389/fimmu.2024.1167362/full#supplementary-material
References
1. Schellekens GA, de Jong BA, van den Hoogen FH, van de Putte LB, van Venrooij WJ. Citrulline is an essential constituent of antigenic determinants recognized by rheumatoid arthritis-specific autoantibodies. J Clin Invest (1998) 101(1):273–81. doi: 10.1172/JCI1316
2. Vossenaar ER, Radstake TR, van der Heijden A, van Mansum MA, Dieteren C, de Rooij DJ, et al. Expression and activity of citrullinating peptidylarginine deiminase enzymes in monocytes and macrophages. Ann Rheum Dis (2004) 63(4):373–81. doi: 10.1136/ard.2003.012211
3. Foulquier C, Sebbag M, Clavel C, Chapuy-Regaud S, Al Badine R, Mechin MC, et al. Peptidyl arginine deiminase type 2 (PAD-2) and PAD-4 but not PAD-1, PAD-3, and PAD-6 are expressed in rheumatoid arthritis synovium in close association with tissue inflammation. Arthritis Rheumatol (2007) 56(11):3541–53. doi: 10.1002/art.22983
4. Shelef MA, Sokolove J, Lahey LJ, Wagner CA, Sackmann EK, Warner TF, et al. Peptidylarginine deiminase 4 contributes to tumor necrosis factor α-induced inflammatory arthritis. Arthritis Rheumatol (2014) 66(6):1482–91. doi: 10.1002/art.38393
5. Bawadekar M, Shim D, Johnson CJ, Warner TF, Rebernick R, Damgaard D, et al. Peptidylarginine deiminase 2 is required for tumor necrosis factor alpha-induced citrullination and arthritis, but not neutrophil extracellular trap formation. J Autoimmun (2017) 80:39–47. doi: 10.1016/j.jaut.2017.01.006
6. Chang X, Xia Y, Pan J, Meng Q, Zhao Y, Yan X. PADI2 is significantly associated with rheumatoid arthritis. PloS One (2013) 8(12):e81259. doi: 10.1371/journal.pone.0081259
7. Suzuki A, Yamada R, Chang X, Tokuhiro S, Sawada T, Suzuki M, et al. Functional haplotypes of PADI4, encoding citrullinating enzyme peptidylarginine deiminase 4, are associated with rheumatoid arthritis. Nat Genet (2003) 34(4):395–402. doi: 10.1038/ng1206
8. Makrygiannakis D, af Klint E, Lundberg IE, Lofberg R, Ulfgren AK, Klareskog L, et al. Citrullination is an inflammation-dependent process. Ann Rheum Dis (2006) 65(9):1219–22. doi: 10.1136/ard.2005.049403
9. Li P, Li M, Lindberg MR, Kennett MJ, Xiong N, Wang Y. PAD4 is essential for antibacterial innate immunity mediated by neutrophil extracellular traps. J Exp Med (2010) 207(9):1853–62. doi: 10.1084/jem.20100239
10. Holmes CL, Shim D, Kernien J, Johnson CJ, Nett JE, Shelef MA. Insight into Neutrophil Extracellular Traps through Systematic Evaluation of Citrullination and Peptidylarginine Deiminases. J Immunol Res (2019) 2019:2160192. doi: 10.1155/2019/2160192
11. Pratesi F, Dioni I, Tommasi C, Alcaro MC, Paolini I, Barbetti F, et al. Antibodies from patients with rheumatoid arthritis target citrullinated histone 4 contained in neutrophils extracellular traps. Ann Rheum Dis (2014) 73(7):1414–22. doi: 10.1136/annrheumdis-2012-202765
12. Khandpur R, Carmona-Rivera C, Vivekanandan-Giri A, Gizinski A, Yalavarthi S, Knight JS, et al. NETs are a source of citrullinated autoantigens and stimulate inflammatory responses in rheumatoid arthritis. Sci Transl Med (2013) 5(178):178ra40. doi: 10.1126/scitranslmed.3005580
13. Rebernick R, Fahmy L, Glover C, Bawadekar M, Shim D, Holmes CL, et al. DNA area and NETosis analysis (DANA): a high-throughput method to quantify neutrophil extracellular traps in fluorescent microscope images. Biol Proced Online (2018) 20:7. doi: 10.1186/s12575-018-0072-y
14. Aulik NA, Hellenbrand KM, Czuprynski CJ. Mannheimia haemolytica and its leukotoxin cause macrophage extracellular trap formation by bovine macrophages. Infect Immun (2012) 80(5):1923–33. doi: 10.1128/IAI.06120-11
15. Wong KW, Jacobs WR. Mycobacterium tuberculosis exploits human interferon γ to stimulate macrophage extracellular trap formation and necrosis. J Infect Dis (2013) 208(1):109–19. doi: 10.1093/infdis/jit097
16. Liu P, Wu X, Liao C, Liu X, Du J, Shi H, et al. Escherichia coli and Candida albicans induced macrophage extracellular trap-like structures with limited microbicidal activity. PloS One (2014) 9(2):e90042. doi: 10.1371/journal.pone.0090042
17. Mulherin D, Fitzgerald O, Bresnihan B. Synovial tissue macrophage populations and articular damage in rheumatoid arthritis. Arthritis Rheumatol (1996) 39(1):115–24. doi: 10.1002/art.1780390116
18. Hu Y, Wang B, Shen J, Low SA, Putt KS, Niessen HWM, et al. Depletion of activated macrophages with a folate receptor-beta-specific antibody improves symptoms in mouse models of rheumatoid arthritis. Arthritis Res Ther (2019) 21(1):143. doi: 10.1186/s13075-019-1912-0
19. El Shikh MEM, El Sayed R, Nerviani A, Goldmann K, John CR, Hands R, et al. Extracellular traps and PAD4 released by macrophages induce citrullination and auto-antibody production in autoimmune arthritis. J Autoimmun (2019) 105:102297. doi: 10.1016/j.jaut.2019.06.008
20. Mohanan S, Horibata S, McElwee JL, Dannenberg AJ, Coonrod SA. Identification of macrophage extracellular trap-like structures in mammary gland adipose tissue: a preliminary study. Front Immunol (2013) 4:67. doi: 10.3389/fimmu.2013.00067
21. Edwards NJ, Hwang C, Marini S, Pagani CA, Spreadborough PJ, Rowe CJ, et al. The role of neutrophil extracellular traps and TLR signaling in skeletal muscle ischemia reperfusion injury. FASEB J (2020) 34(12):15753–70. doi: 10.1096/fj.202000994RR
22. Raijmakers R, Vogelzangs J, Raats J, Panzenbeck M, Corby M, Jiang H, et al. Experimental autoimmune encephalomyelitis induction in peptidylarginine deiminase 2 knockout mice. J Comp Neurol (2006) 498(2):217–26. doi: 10.1002/cne.21055
23. Holmes CL, Peyton CG, Bier AM, Donlon TZ, Osman F, Bartels CM, et al. Reduced IgG titers against pertussis in rheumatoid arthritis: Evidence for a citrulline-biased immune response and medication effects. PloS One (2019) 14(5):e0217221. doi: 10.1371/journal.pone.0217221
24. Boone BA, Orlichenko L, Schapiro NE, Loughran P, Gianfrate GC, Ellis JT, et al. The receptor for advanced glycation end products (RAGE) enhances autophagy and neutrophil extracellular traps in pancreatic cancer. Cancer Gene Ther (2015) 22(6):326–34. doi: 10.1038/cgt.2015.21
25. Wright B, Zeidman I, Greig R, Poste G. Inhibition of macrophage activation by calcium channel blockers and calmodulin antagonists. Cell Immunol (1985) 95(1):46–53. doi: 10.1016/0008-8749(85)90293-X
26. Yin H, Liu N, Sigdel KR, Duan L. Role of NLRP3 inflammasome in rheumatoid arthritis. Front Immunol (2022) 13:931690. doi: 10.3389/fimmu.2022.931690
27. Guo C, Fu R, Wang S, Huang Y, Li X, Zhou M, et al. NLRP3 inflammasome activation contributes to the pathogenesis of rheumatoid arthritis. Clin Exp Immunol (2018) 194(2):231–43. doi: 10.1111/cei.13167
28. Cassado Ados A, D'Imperio Lima MR, Bortoluci KR. Revisiting mouse peritoneal macrophages: heterogeneity, development, and function. Front Immunol (2015) 6:225. doi: 10.3389/fimmu.2015.00225
29. Yang X, Chang Y, Wei W. Emerging role of targeting macrophages in rheumatoid arthritis: Focus on polarization, metabolism and apoptosis. Cell Prolif (2020) 53(7):e12854. doi: 10.1111/cpr.12854
30. Rayner BS, Zhang Y, Brown BE, Reyes L, Cogger VC, Hawkins CL. Role of hypochlorous acid (HOCl) and other inflammatory mediators in the induction of macrophage extracellular trap formation. Free Radic Biol Med (2018) 129:25–34. doi: 10.1016/j.freeradbiomed.2018.09.001
31. Schorn C, Janko C, Latzko M, Chaurio R, Schett G, Herrmann M. Monosodium urate crystals induce extracellular DNA traps in neutrophils, eosinophils, and basophils but not in mononuclear cells. Front Immunol (2012) 3:277. doi: 10.3389/fimmu.2012.00277
32. Loureiro A, Pais C, Sampaio P. Relevance of macrophage extracellular traps in. Front Immunol (2019) 10:2767. doi: 10.3389/fimmu.2019.02767
33. Steen J, Forsstrom B, Sahlstrom P, Odowd V, Israelsson L, Krishnamurthy A, et al. Recognition of amino acid motifs, rather than specific proteins, by human plasma cell-derived monoclonal antibodies to posttranslationally modified proteins in rheumatoid arthritis. Arthritis Rheumatol (2019) 71(2):196–209. doi: 10.1002/art.40699
34. Zheng Z, Mergaert AM, Fahmy LM, Bawadekar M, Holmes CL, Ong IM, et al. Disordered antigens and epitope overlap between anti-citrullinated protein antibodies and rheumatoid factor in rheumatoid arthritis. Arthritis Rheumatol (2020) 72(2):262–72. doi: 10.1002/art.41074
35. Mergaert AM, Zheng Z, Denny MF, Amjadi MF, Bashar SJ, Newton MA, et al. Rheumatoid factor and anti–modified protein antibody reactivities converge on igG epitopes. Arthritis Rheumatol (2022) 74(6):984–91. doi: 10.1002/art.42064
36. Damgaard D, Senolt L, Nielsen CH. Increased levels of peptidylarginine deiminase 2 in synovial fluid from anti-CCP-positive rheumatoid arthritis patients: Association with disease activity and inflammatory markers. Rheumatol (Oxford) (2016) 55(5):918–27. doi: 10.1093/rheumatology/kev440
37. Wang Y, Wysocka J, Sayegh J, Lee YH, Perlin JR, Leonelli L, et al. Human PAD4 regulates histone arginine methylation levels via demethylimination. Science (2004) 306(5694):279–83. doi: 10.1126/science.1101400
38. Faria RX, Reis RA, Casabulho CM, Alberto AV, de Farias FP, Henriques-Pons A, et al. Pharmacological properties of a pore induced by raising intracellular Ca2+. Am J Physiol Cell Physiol (2009) 297(1):C28–42. doi: 10.1152/ajpcell.00476.2008
39. Liu X, Zhang Z, Ruan J, Pan Y, Magupalli VG, Wu H, et al. Inflammasome-activated gasdermin D causes pyroptosis by forming membrane pores. Nature (2016) 535(7610):153–8. doi: 10.1038/nature18629
40. Rashidi M, Simpson DS, Hempel A, Frank D, Petrie E, Vince A, et al. The pyroptotic cell death effector gasdermin D is activated by gout-associated uric acid crystals but is dispensable for cell death and IL-1beta release. J Immunol (2019) 203(3):736–48. doi: 10.4049/jimmunol.1900228
Keywords: macrophage extracellular trap, citrullinated antigens, anti-citrullinated protein antibodies, rheumatoid arthritis, peptidylarginine deiminase 2, peptidylarginine deiminase 4
Citation: Bashar SJ, Holmes CL and Shelef MA (2024) Macrophage extracellular traps require peptidylarginine deiminase 2 and 4 and are a source of citrullinated antigens bound by rheumatoid arthritis autoantibodies. Front. Immunol. 15:1167362. doi: 10.3389/fimmu.2024.1167362
Received: 16 February 2023; Accepted: 29 January 2024;
Published: 27 February 2024.
Edited by:
Kristen Demoruelle, University of Colorado Denver, United StatesReviewed by:
Yuko Okamoto, Tokyo Women’s Medical University, JapanI-Cheng Ho, Harvard Medical School, United States
Copyright © 2024 Bashar, Holmes and Shelef. This is an open-access article distributed under the terms of the Creative Commons Attribution License (CC BY). The use, distribution or reproduction in other forums is permitted, provided the original author(s) and the copyright owner(s) are credited and that the original publication in this journal is cited, in accordance with accepted academic practice. No use, distribution or reproduction is permitted which does not comply with these terms.
*Correspondence: Miriam A. Shelef, bXNoZWxlZkBtZWRpY2luZS53aXNjLmVkdQ==