- 1Department of Experimental and Clinical Medicine, University of Florence, Florence, Italy
- 2Flow Cytometry Diagnostic Center and Immunotherapy, Careggi University Hospital, Florence, Italy
- 3Infectious and Tropical Diseases Unit, Careggi University Hospital, Florence, Italy
- 4Immunoallergology Unit, Careggi University Hospital, Florence, Italy
- 5Hematology Unit, Careggi University Hospital, Florence, Italy
- 6Microbiology and Virology Unit, Careggi University Hospital, Florence, Italy
- 7Immunology and Cell Therapy Unit, Careggi University Hospital, Florence, Italy
Background: Patients with B-cell lymphoma are a fragile category of subjects, particularly exposed to infections and characterized by an impaired vaccination response due to the disease itself and, even more, to the chemotherapy regimen. For this reason, extensive knowledge of the immune response status of these subjects is of fundamental importance to obtain possible indications for a tailored immunization strategy.
Methods: We enrolled two cohorts of patients with B-cell lymphoma under rituximab treatment or 3–24 months after treatment. In all patients, we evaluated both humoral and cellular immunological memory toward SARS-CoV-2, after standard vaccination and upon one booster dose.
Results: We observed no Spike-specific IgG production in patients (n = 25) under anti-CD20 treatment, whereas patients (n = 16) vaccinated after the completion of chemotherapy showed a higher humoral response. Evaluating SARS-CoV-2–specific T-cell response, we found that patients in both cohorts had developed robust cellular immunity after vaccination. Of the 21 patients (51%) that experienced a breakthrough SARS-CoV-2 infection, only six patients developed severe disease. Interestingly, these six patients had all been treated with rituximab plus bendamustine. Notably, we observed that Spike-specific IgG levels in patients treated with rituximab plus bendamustine were absent or lower compared with those in patients treated with rituximab plus other chemotherapy, whereas Spike-specific T-cell response was not different based on chemotherapy regiment.
Discussion: Our results show that, in patients with B-cell lymphoma under rituximab therapy, anti–SARS-CoV-2 mRNA vaccination induces a weak or absent humoral response but a consistent T-cell response. In addition, chemotherapy regimens with bendamustine further reduce patients’ ability to mount a Spike-specific humoral response even after a long time period from chemotherapy discontinuation. These results provide evidence that different chemotherapeutics display different immunosuppressive properties that could be taken in to account in the choice of the right drug regimen for the right patient. Moreover, they question whether immunocompromised patients, particularly those treated with bendamustine, need interventions to improve vaccine-induced immune response.
Introduction
COVID-19 pandemic induced the scientific community to deeply investigate the establishment and persistence of immunological memory against SARS-CoV-2 following infection or vaccination. Most studies focused on healthy individuals. In immunocompromised subjects, such as patients with hematologic malignancy receiving immunosuppressive regimens, these aspects are still largely unexplored (1, 2), although secondary immunodeficiency is a major concern for increasing incidence and greatest risk of infection in these patients (3, 4). Rituximab (RTX)–based chemotherapy is widely used in several hematologic malignancies, i.e., non-Hodgkin lymphoma and chronic lymphocytic leukemia (5, 6). RTX is a chimeric monoclonal antibody (mAb) that targets CD20 on B lymphocytes and rapidly induces a prolonged B-cell depletion (5). Usually, circulating B cells are newly detectable 6–9 months after the end of treatment. However, kinetic of B-cell reconstitution could be affected by the concurrent administration of other hematological oncology therapies, such as alkylating agents (bendamustine, chlorambucil, and cyclophosphamide). In these cases, B-cell aplasia can be maintained even longer. Notably, in patients with hematological malignancies, anti–CD20-mAb therapy is administered at a higher dose and generally combined with intensive chemotherapy regimens, in order to enhance the anti-neoplastic effects of RTX but, as a consequence, induce a higher immunosuppression, compared with RTX regimens used in patients with autoimmune diseases (7, 8). Patients receiving these highly immunosuppressive therapies showed a significantly increased risk of infections and a more severe disease course during SARS-CoV-2 infection (9). In addition, because of compromised humoral immune response, these patients displayed impaired vaccine-induced immunity (10–13). Prior studies in patients with autoimmune rheumatic diseases demonstrated that patients treated with RTX have poor humoral immune responses after mRNA or viral vector vaccination (14), but T-cell responses resulted similar between RTX- and non–RTX-treated patients (15). Although T-cell immune response might balance ineffective humoral immunity in patients with B-cell depletion, antibodies are non-redundant and play a critical role for virus clearance. At the moment, there is no consensus on the right time to vaccinate patients with hematological malignancies. A deep analysis with a cellular biology approach will be useful to define how the timing of RTX administration could interfere with vaccine effectiveness, as well as the impact of concomitant immunosuppressive therapies. Hence, we analyzed SARS-CoV-2–specific antibody and CD4+ T-cell–mediated immune responses, following standard vaccination cycle and upon booster dose, in patients with hematological malignancy (B-cell lymphoma). Patients were divided into two cohorts: in the first cohort, anti–SARS-CoV-2 vaccination was given under RTX cycle (TP Ongoing), whereas, in the second cohort, after RTX therapy (Post TP). To assess the effect of additional immunosuppressive therapies on vaccine-induced immunity, these two groups were further divided on the basis of the regimen of chemotherapy associated with RTX.
Materials and methods
Patients
The study population included 41 patients with B-cell lymphoma recruited at the Careggi University Hospital, Florence, Tuscany, Italy, by the Centre of Research and Innovation of Myeloproliferative Neoplasms. The first cohort (TP Ongoing, n = 25) consisted of patients with B-cell lymphoma who received the first two doses of anti–SARS-CoV-2 vaccine while they were under RTX therapy, whereas the second cohort (Post TP, n = 16) included patients with B-cell lymphoma who completed RTX therapy between 3 and 12 months before anti–SARS-CoV-2 vaccination. Patients were stratified on the basis of the concomitant chemotherapy regimen received, particularly considering the administration of bendamustine. Demographic and clinical features of enrolled patients are summarized in Table 1. Additional laboratory data on hemoglobin level, leucocyte, lymphocyte, and platelet counts in recruited patients at T0 are reported in Supplementary Table 1. Patients received the first mRNA-1273 vaccine dose in March 2021, followed by a second mRNA-1273 vaccine dose 28 days later. Patients received a third vaccine dose between December 2021 and January 2022. A blood draw was performed at basal time (time 0, before the first vaccine dose), 28 days after (T1, before the second dose), 60 days after (T2), and 1 month after the third vaccine dose (T3). None of the study participants had prior PCR-confirmed SARS-CoV-2 infection, IgG antibodies against nucleoprotein and T-cell response against nucleoprotein and membrane protein (Supplementary Figure 1).
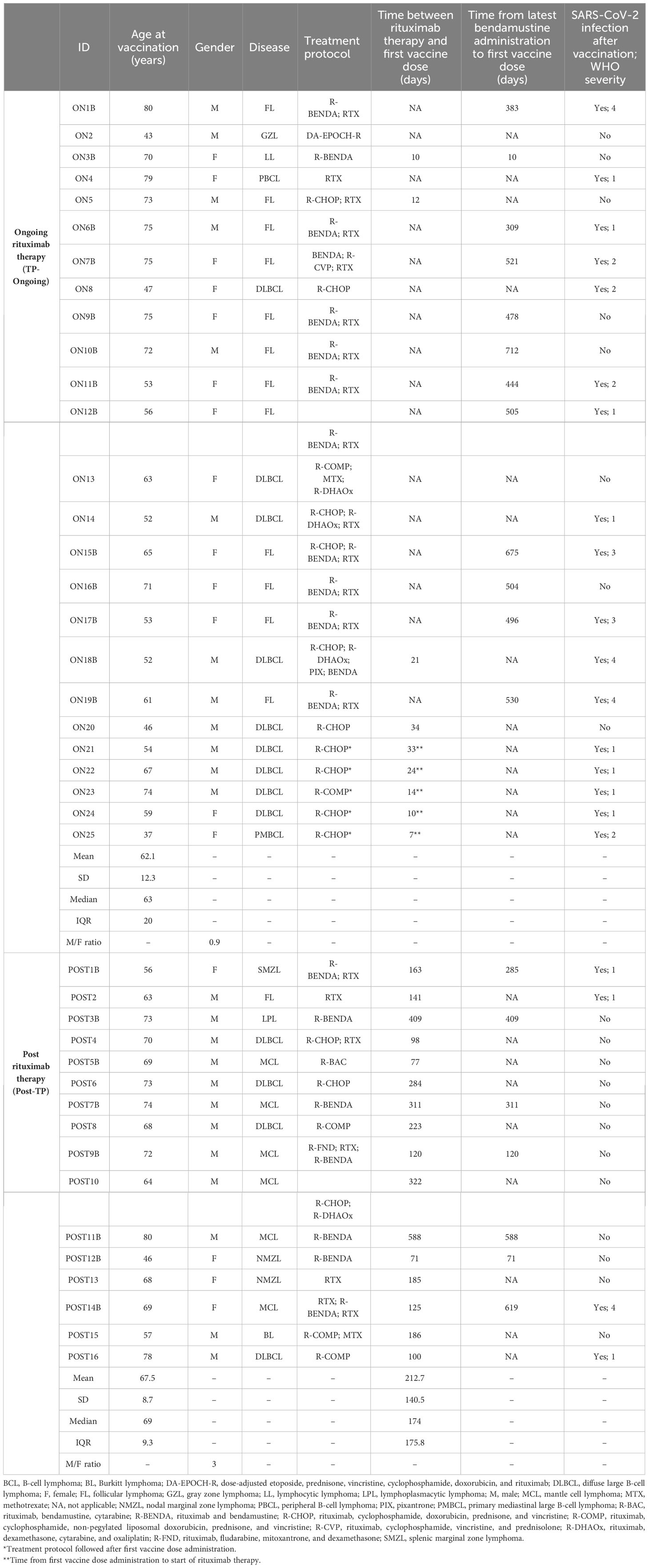
Table 1 Main demographic and clinical data of patients with B-cell lymphoma receiving anti–SARS-CoV-2 mRNA vaccination under rituximab (n = 25) or post-rituximab therapy (n = 16).
Peripheral blood mononuclear cells (PBMNCs) were obtained following density gradient centrifugation of blood samples using Lymphoprep (Axis Shield Poc As™) and were frozen in Fetal calf serum (FCS) plus 10% Dimethyl Sulfoxide (DMSO) to be stored in liquid nitrogen. For each subject, longitudinal samples were defrosted and analyzed together for T-cell evaluation. Sera were frozen and stored for Ig level evaluation.
Characteristics of study population
A total of 41 patients with B-cell lymphoma were assessed for serum antibody analysis and T-cell response evaluation (Table 1). The majority of them (n = 25) was under RTX therapy, whereas 16 patients had already completed RTX treatment. For the latter group, the median time from last therapy cycle and first vaccine dose was 174 days. The median age (range) for TP-Ongoing patients was 63 (37–80) years with a male/female ratio of 0.9, whereas, for the Post-TP group, the median age was 69 (46–80) with a male/female ratio of 3. The most common conditions were follicular lymphoma (31.7%) and diffuse large B-cell lymphoma (31.7%), followed by mantle cell lymphoma (14.6%) and nodal marginal zone lymphoma (4.9%). In the TP-Ongoing group, only one patient received RTX therapy alone, whereas the other 24 patients received RTX in combination with intensive chemotherapy regimens. In particular, 13 patients were under RTX treatment and a regimen that included bendamustine, whereas 11 patients were treated with RTX plus other chemotherapy, including R-CHOP (RTX, cyclophosphamide, doxorubicin, prednisone, and vincristine), R-COMP (RTX, cyclophosphamide, non-pegylated liposomal doxorubicin, prednisone, and vincristine), and others (Table 1). Regarding the Post-TP cohort, two patients were treated with RTX alone, eight received RTX in combination with a regimen that included bendamustine, and six were treated with RTX plus other chemotherapy (Table 1).
Evaluation of SARS-CoV-2 Spike–reactive T cells
For T-cell stimulation in vitro, 1.5 million PBMNCs were cultured in complete RPMI plus 5% human AB serum in 96-well flat bottom plates in the presence of medium alone (background, negative control) or of a pool of Spike SARS-CoV-2 peptide pools (Prot_S1, Prot_S+, and Prot_S to achieve a complete sequence coverage of the Spike protein) at 0.6 µM/peptide, according to the manufacturer’s instructions (Miltenyi Biotec). T-cell response against nucleoprotein (N) peptide pool (Miltenyi Biotec) was assessed as well. After 2 h of incubation at 37°C, 5% CO2, brefeldin A (5 µg/mL) was added, followed by additional 4h incubation. Finally, cells were fixed and stained using fluorochrome-conjugated antibodies listed in Supplementary Table 2. Samples were acquired on a BD LSR II flow cytometer (BD Biosciences) using the gating strategy showed in Supplementary Figure 2. Flow cytometry experiments were performed using published guidelines (16).
Evaluation of SARS-CoV-2–specific IgG
Evaluation of SARS-CoV-2 Spike–specific IgG (Diasorin), nucleoprotein-specific IgG (Abbott), and Receptor Binding Domain (RBD)-specific IgG (Abbott) were performed following the manufacturer’s instructions. The antibody reactivity of each specimen was expressed by the ratio between optical density and cutoff value (index) or as binding antibody unit (BAU)/ml.
Evaluation of circulating CD19+ B cells
Before T-cell evaluation, all defrosted PBMNCs were evaluated by flow cytometry for the presence of circulating CD19+ B cells. In detail, PBMNCs were stained using fluorochrome-conjugated antibodies CD3-Pacific Blue and CD19-APC-Cy7 (BD Biosciences), in order to assess the percentage of lymphocyte expressing CD19. Flow cytometry experiments were performed using the published guidelines (16).
Statistics
Unpaired Mann–Whitney test was used to compare TP-Ongoing versus Post-TP cohorts and to compare patients treated with bendamustine versus those receiving regimens without bendamustine. Wilcoxon signed-rank test was used to compare different time points of analysis in each study group. In all cases, p values ≤ 0.05 were deemed as significant.
Study approval
The procedures followed in the study were approved by the Careggi University Hospital Ethical Committee (CEAVC 23847_BIO). Written informed consent was obtained from recruited patients.
Results
Higher Spike-specific antibodies after COVID-19 vaccination in Post-TP patients compared with that in TP-Ongoing patients
Sera of both TP-Ongoing and Post-TP patients were analyzed for IgG anti-Spike and IgG anti-RBD. At baseline (T0), no antibodies against Spike or RBD were detected in all recruited patients (Figures 1A, B). Because of the complete deletion of CD20+ B cells, SARS-CoV-2–specific IgGs were undetectable in almost all patients receiving vaccination during RTX treatment (TP Ongoing) (Figures 1A, B). Interestingly, among the group of TP-Ongoing patients, two subjects at T1 (after the first dose) and three subjects at T2 (1 month after the second dose) showed detectable low levels of anti-RBD and anti-Spike IgGs (Figures 1A, B). Notably, two of these patients (ON18 and ON22) started RTX therapy shortly after the first vaccine dose (24 and 21 days, respectively) but completed the vaccination cycle while under RTX treatment (Table 1). Indeed, these two patients displayed detectable CD19+ cells at T0 (8.1% and 4.2% of CD19+ B lymphocyte, respectively), whereas no CD19+ cells were detected at T1, T2, and T3 (data not shown).
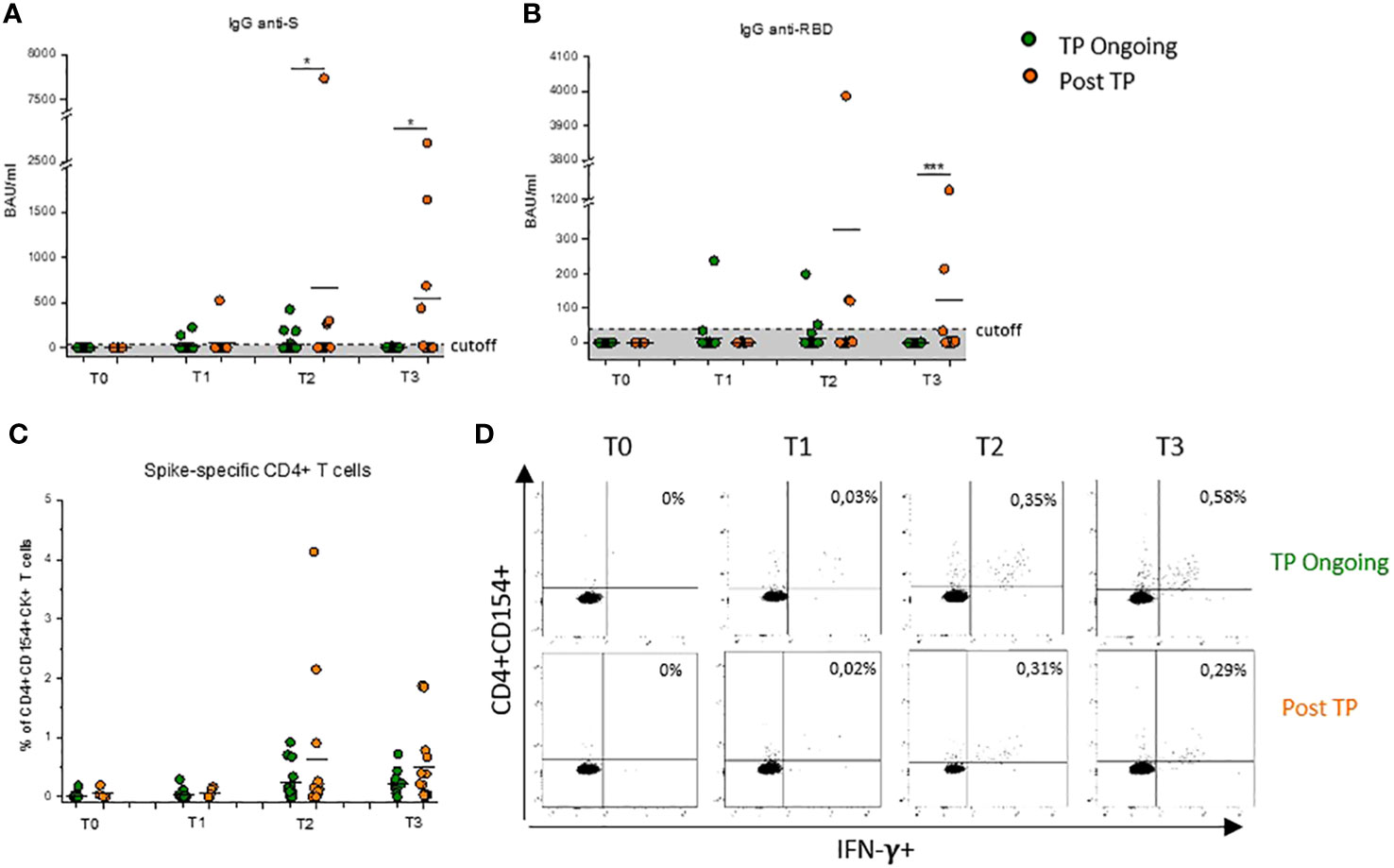
Figure 1 Evaluation of Spike-specific antibodies and CD4+ T cells after COVID-19 vaccination in Ongoing-TP and Post-TP patients. Evaluation of IgG specific for Spike protein (A) or RBD (B) in TP-Ongoing patients (green dots) at T0 (n = 24), T1 (n = 23), T2 (n = 23), and T3 (n = 10) and in Post-TP (orange dots) cohort at T0 (n = 3), T1 (n = 9), T2 (n = 13), and T3 (n = 12). (C) Frequency of CD4+ T cells reactive to SARS-CoV-2, defined by expression of CD154 and at least one cytokine among IFN-γ, IL-2, and TNF-α (CD4+CD154+CK+) in TP-Ongoing patients (green dots) at T0 (n = 14), T1 (n = 15), T2 (n = 15), and T3(n = 10) and in Post-TP (orange dots) cohort at T0 (n = 3), T1 (n = 7), T2 (n = 13), and T3 (n = 12). (D) Representative flow cytometric plots of Spike-specific CD154+CD4+IFN-γ+ T cells, at each time point, in one selected TP-Ongoing individual (upper panels) and Post-TP individual (lower panels). Black lines in (A–C) represent mean values, and gray area delimited by a dotted line in (A) and (B) represents the cutoff value. The evaluation time points are T0 (before vaccination), T1 (before the second dose), T2 (1 month after the second dose), and T3 (1 month after the third dose). *p < 0.05 and ***p < 0.001 calculated with Mann–Whitney U-test.
Conversely, patients in the Post-TP cohort, having completed the standard vaccination cycle at least 3 months after discontinuation of RTX/chemotherapy, showed appreciable humoral Spike–specific response at T2 and T3 compared with TP-Ongoing patients, reaching a statistical significance for anti-Spike IgG both at T2 and T3 and for anti-RBD IgG only at T3 (Figures 1A, B). It is important to underline that IgG levels observed in RTX-treated patients remain lower compared with those observed in healthy subjects (17). Notably, no correlation was found between time from last RTX administration and anti-Spike or anti-RBD IgG levels observed at both T2 and T3 time points (Supplementary Figure 3).
Spike-specific CD4+ T-cell response is present and intense in both Ongoing-TP and Post-TP patients after COVID-19 mRNA vaccination
RTX therapy induced a rapid B-cell depletion with no effect on T-cell numbers. Nevertheless, the majority of patients with B-cell lymphoma included in this study received intensive chemotherapy regimens in combination with RTX, to influence both quantitatively and functionally T cells. For this reason, the ability of CD4+ T cells to be re-activated in vitro upon stimulation with a pool of peptides covering the entire sequence of the Wuhan-strain (wild type) Spike was investigated. Both TP-Ongoing and Post-TP patients showed a robust cell–mediated response with high level of Spike-specific CD4+ T cells at T1, T2, and T3, with no difference between the two cohorts (Figures 1C, D). Notably, percentages of Spike-specific CD4+ T cells in RTX-treated patient were comparable to those observed in healthy subjects (Supplementary Figure 4) (17).
RTX + bendamustine–treated patients with B-cell lymphoma showed more severe COVID-19 compared with RTX + other chemotherapy–treated patients
Because of lower Spike-specific humoral response in the analyzed RTX-treated cohort compared with reported data on healthy subjects (17), we prospectively assessed the incidence and severity of breakthrough infections in the study population in 1-year follow-up after vaccination. Among all recruited patients, both Ongoing-TP and Post-TP cohorts, breakthrough SARS-CoV-2 infections were reported in 21 subjects (3–12 months after T3) with different severity; in particular, five of the 21 patients showed a more severe diseases course (Figure 2A), according to WHO COVID-19 severity index. In the TP-Ongoing cohort, 68% of patients developed COVID-19, which was most commonly mild (8 of 17) or moderate (4 of 17). Only three and two of the 17 patients developed critical disease and severe disease, respectively. On the contrary, in the Post-TP cohort, 25% of patients developed COVID-19, which, in the majority of patients (three of four), was mild. Notably, all the five patients who showed a higher WHO COVID-19 severity index (score of 3 or 4) were RTX + bendamustine–treated patients. Therefore, dividing all infected patients on the basis of bendamustine therapy, we interestingly observed that RTX + bendamustine–treated patients showed a significantly higher WHO COVID-19 severity index (mean = 2.4) compared with RTX + other chemotherapy–treated patients (mean = 1.2) (Figure 2B). No difference in the number of breakthrough infections was observed between the two analyzed cohort (data not shown).
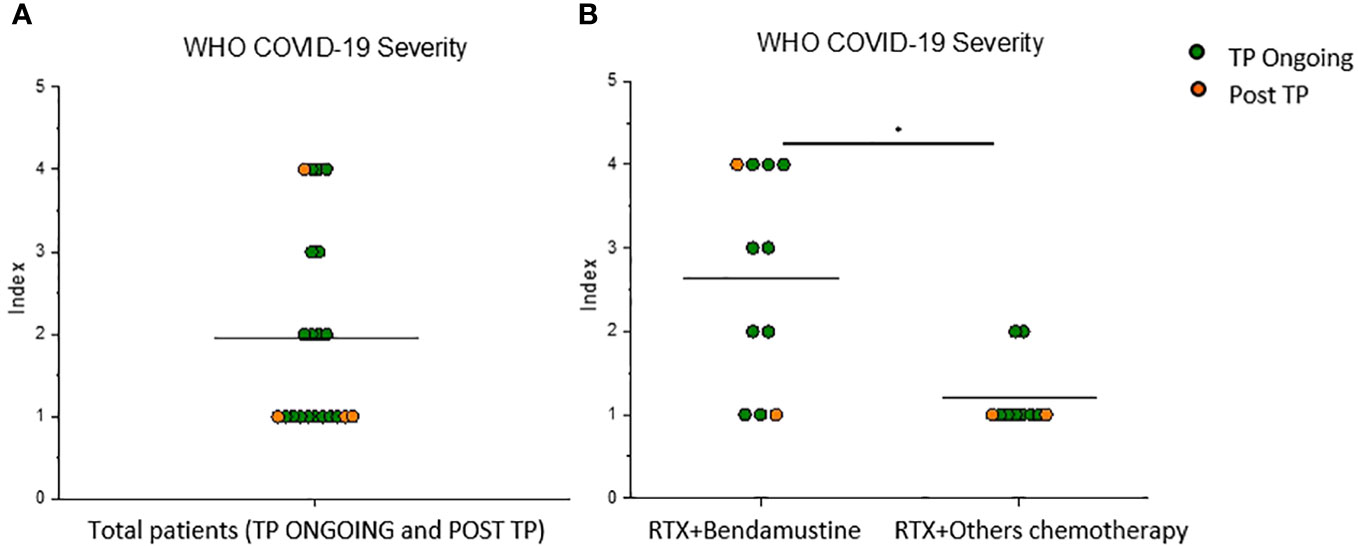
Figure 2 RTX + bendamustine–treated patients showed a more severe disease course during SARS-CoV-2 infection. (A) WHO COVID-19 severity index of 21 lymphoma B-cell patients (TP Ongoing, n = 17, green dots) (Post TP, n = 4, orange dots) infected with SARS-CoV-2 after vaccination and (B) WHO COVID-19 severity index of 11 RTX + bendamustine–treated patients and 10 RTX + other chemotherapy–treated patients. Mean values are represented as black lines. *P < 0.05 calculated with Mann–Whitney U-test.
Chemotherapy regimen associated with RTX influences the humoral vaccine–induced immune response
Given the increased risk to develop a more severe diseases course after SARS-CoV-2 infection in RTX + bendamustine–treated patients, cohorts were subsequently divided on the basis of the type of chemotherapy regimen associated with RTX. We observed that IgG levels were also influenced by the type of chemotherapy received; in particular, Post-TP patients that had been previously treated with RTX + bendamustine showed a lower or absent level of SARS-CoV-2–specific IgGs compared with Post-TP patients treated with RTX + other chemotherapy (Figures 3A, B). Interestingly, considering only patients treated with RTX + other chemotherapy, a statistically significant positive correlation between anti-Spike and anti-RBD IgG levels and time from the last RTX dose (Figures 3C, D) was found both at T2 and T3.
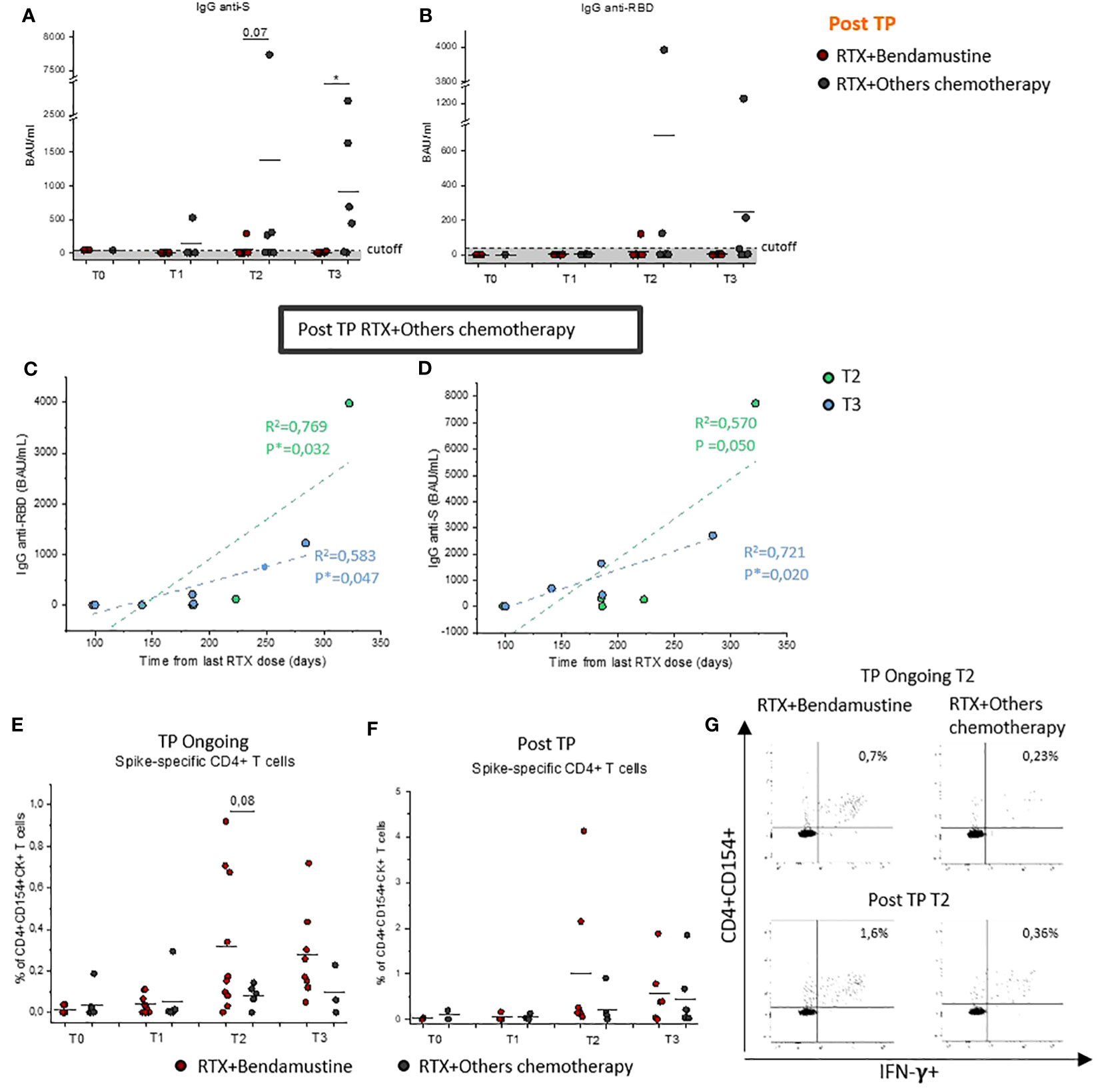
Figure 3 Evaluation of Spike-specific antibodies and CD4+ T cells after COVID-19 vaccination in bendamustine- and no-bendamustine–treated patients. Evaluation of IgG specific for Spike protein (A) or RBD (B) in Post-TP RTX + bendamustine–treated patients (red dots) at T0 (n = 2), T1 (n = 5), T2 (n = 6), and T3 (n = 6) and RTX + other chemotherapy–treated patients (gray dots) at T0 (n = 1), T1 (n = 4), T2 (n = 7), and T3 (n = 6). Correlation between IgG anti-S (C) or IgG anti-RBD (D) with time from last rituximab dose on total Post-TP RTX + other chemotherapy–treated group at T2 (n = 7; light green dots) or T3 (n = 6; light blue dots). Pearson’s correlation coefficients were used to calculate the correlations; statistical significance (*p < 0.05) is reported on each graph. (E) Frequency of CD4+ T cells reactive to SARS-CoV-2 in TP-Ongoing RTX + bendamustine–treated patients (red dots) at T0 (n = 7), T1 (n = 9), T2 (n = 10), and T3 (n = 8) and RTX + other chemotherapy–treated patients (gray dots) at T0 (n = ), T1 (n = 6), T2 (n = 5), and T3 (n = 3). (F) Frequency of CD4+ T cells reactive to SARS-CoV-2 in Post-TP RTX + bendamustine–treated patients (red dots) at T0 (n = 2), T1 (n = 3), T2 (n = 6), and T3 (n = 5) and RTX + other chemotherapy–treated patients (gray dots) at T0 (n = 1), T1 (n = 4), T2 (n = 7), and T3(n = 6). (G) Representative flow cytometric plots of Spike-specific CD154+CD4+IFN-γ+ T cells, at T2, in two selected TP-Ongoing individuals (upper panels) and in two selected Post-TP individuals (upper panels) RTX + bendamustine–treated or RTX + other chemotherapy–treated, left and right panels, respectively. Black lines in (A, B, E, F) represent mean values, and gray area delimited by a dotted line in (A) and (B) represents the cutoff value. *p < 0.05 calculated with Mann–Whitney U-test.
T-cell–mediated response was also evaluated considering the presence of bendamustine in the chemotherapy regimen. Our data highlight that T-cell–mediated response in both TP-Ongoing and Post-TP patients was not significantly influenced by chemotherapy regimen compared with the humoral response, showing that it, if anything, had an opposite trend. In fact, RTX + bendamustine–treated patients showed comparable frequency of CD4+ Spike-specific T cells compared with patients whose regimens did not include bendamustine (Figures 3E–G).
Discussion
The effectiveness of SARS-CoV-2 mRNA vaccines has been demonstrated by several studies performed throughout 2021 on different cohorts, mainly including healthy individuals (17, 18). SARS-CoV-2 infection can present asymptomatically or mildly but may also cause severe immune dysregulation, leading to hospitalization and poor outcome (19). Patients with B-cell lymphoma have an increased risk of severe COVID-19 with fatal outcome. In fact, hematological malignancies represent an independent risk factor for COVID-19 mortality (20–22). Effective vaccination against SARS-CoV-2 would contribute to the protection of these patients against severe COVID-19. There are only a few studies reporting data on the vaccine-induced immune protection in this specific population (23–25). These studies mainly focus on the effects of RTX therapy on humoral vaccine–induced response, with low or absent consideration of concomitant high immunosuppressive chemotherapy to which patients with B-cell lymphoma are exposed (25). It is therefore important to investigate whether these patients benefit from current vaccines and what additional strategy should be eventually adopted to guarantee vaccine effectiveness. To this end, in the current study, the humoral and cellular responses to two and three doses of COVID-19 mRNA vaccine in patients with B-cell lymphoma were evaluated, focusing on RTX therapy and investigating the effect of additional immunosuppressive chemotherapy on vaccine-induced response.
Our results showed, consistent with previous reports (26–28), that hematological patients who had been previously exposed to anti-CD20 mAb therapy had a poor/absent humoral response to anti–SARS-CoV-2 mRNA vaccination, very likely due to therapy-related B-cell deficiency. Interestingly, patients undergoing vaccination at least 3 months after the interruption of RTX therapy showed higher antibodies response. This result could be predictable due to the re-establishment of the B-cell compartment in RTX-treated patients 6–12 months after therapy (5). As a result, B cells could therefore respond to vaccine stimuli and start to produce antibodies. Nevertheless, in our whole cohort of Post-TP patients, no correlation was found between time from last RTX administration and antibodies titer, which was instead observed in other cohorts of patients (25, 29). Notably, this correlation is observed when we only consider patients whose chemotherapeutic regimen does not include bendamustine in association with RTX. Therefore, not only anti-CD20 mAb but also the type of chemotherapy received could influence the re-establishment of B-cell compartment and therefore humoral response to vaccines. Among administered chemotherapies, because of its efficacy and favorable toxicity profile, bendamustine has become one of the preferred therapeutic choices for the treatment of indolent B-cell lymphoma together with R-CHOP treatment (30, 31). Nevertheless, myelosuppression including lymphopenia occurs more frequently in patients receiving combinations of bendamustine and RTX compared with those in patient treated with CHOP and RTX (32–34). In agreement with these data, in our restricted cohort of Post-TP patients, we observed a lower or absent humoral response to anti–SARS-CoV-2 mRNA vaccination and no correlation between last RTX dose and antibodies titers in only patient treated with RTX + bendamustine compared with those in patient treated with RTX + other chemotherapy regimens. These data show evidence of a stronger and prolonged immunosuppression in B-cell compartment in patients receiving bendamustine in addition to anti-CD20 therapy.
Not only humoral response is essential for neutralization of virus entrance in the host cells, for virus clearance, and for preventing development of severe disease, but also T cells play a fundamental role in the prevention of more severe disease course during SARS-CoV-2 infection. In both enrolled cohorts of hematological patients, a robust Spike-specific CD4+ T-cell response to anti–SARS-CoV-2 mRNA vaccination is established and maintained, already after two vaccine doses. The observed cell-mediated response was comparable and, in some cases, even higher to previously studied healthy subjects (17). On a restricted numbers of TP-Ongoing patients at T2 and T3 (three and four patients, respectively), we had the opportunity to test CD4+ T-cell response against omicron variant (data not shown). Interestingly, the global CD4+ T-cell response of these patients is retained also against Omicron, in accordance with our previous study performed on healthy individuals (35).
Unexpectedly, T-cell response seemed not to be negatively influenced by RTX or chemotherapy regimens associated with RTX. Indeed, patients treated with RTX + bendamustine showed a comparable percentage of Spike-specific CD4+ T cells, compared with patients treated with other chemotherapy. This observation might indicate that cell-mediated response compensates, at least in part, the lack of the humoral counterpart. Nevertheless, Spike-specific T-cell response was evaluated in terms of frequency of cells and not of absolute cell number that could be lower in these immunocompromised patients given that lymphopenia occurs relatively frequently after therapy with bendamustine (36). This could be one of the reasons explaining why RTX + bendamustine–treated patients showed a higher propensity to develop more severe COVID-19, even if their percentage of Spike-specific CD4+ T-cell response was comparable to RTX + other chemotherapy–treated patients. The higher severity of SARS-CoV-2 breakthrough infection observed in RTX + bendamustine–treated cohort is in accordance with the highly immunosuppressive status caused by the association of RTX plus bendamustine, given that for other pathogens it was already reported that bendamustine-treated patients show increased risk of infection (36).
These results question whether immunocompromised patients, such as patients with B-cell lymphoma treated with RTX and associated chemotherapy regimens, need intervention to improve SARS-CoV-2 mRNA vaccine response or response to other common vaccines. These interventions could comprise improvement of the vaccination schedule by modifying time length between the second and third injection, by additional vaccine doses, or by modulation/interruption of concomitant immunosuppressive therapy, such as bendamustine. Nevertheless, whether this optimization might result in an improved level of protection remains uncertain, and further studies are essential to better define this prospective.
Conclusions
Our results show that, in hemato-oncological patients vaccinated while under RTX therapy, anti–SARS-CoV-2 mRNA vaccination induces a weak or absent humoral response, but a consistent T-cell–mediated response is present, which seems to counterbalance the lack of the humoral response. In addition, chemotherapy regimens comprising bendamustine further reduced patients’ ability to mount a Spike-specific humoral response even after a long time period from therapy discontinuation. These results question whether immunocompromised patients, particularly those treated with bendamustine, need interventions to improve vaccine-induced immune response such as additional vaccine doses over time or modulation of vaccination schedule in respect to timing of immunosuppressive therapy. Moreover, these results offer a biological base that may be useful for the clinicians, in order to better stratify the infection risk of their patients, improving the accuracy in the choice of the drugs for a tailored chemotherapeutic regimen.
Data availability statement
The original contributions presented in the study are included in the article/Supplementary Material. Further inquiries can be directed to the corresponding author.
Ethics statement
The studies involving humans were approved by Comitato Etico Regionale Area Vasta Centro Toscana, Italia. The studies were conducted in accordance with the local legislation and institutional requirements. The participants provided their written informed consent to participate in this study.
Author contributions
AV: Data curation, Formal Analysis, Investigation, Methodology, Validation, Writing – original draft. LS: Data curation, Formal Analysis, Investigation, Validation, Writing – original draft. AM: Data curation, Formal Analysis, Investigation, Methodology, Validation, Writing – original draft. GL: Data curation, Investigation, Methodology, Writing – review & editing. MCa: Data curation, Formal Analysis, Investigation, Methodology, Writing – review & editing. SF: Data curation, Formal Analysis, Investigation, Methodology, Writing – review & editing. SK: Data curation, Formal Analysis, Investigation, Methodology, Writing – review & editing. LC: Data curation, Supervision, Validation, Writing – review & editing. BP: Data curation, Supervision, Validation, Writing – review & editing. MCi: Methodology, Writing – review & editing. BS: Methodology, Writing – review & editing. GR: Data curation, Supervision, Validation, Writing – review & editing. FA: Conceptualization, Funding acquisition, Project administration, Supervision, Validation, Writing – original draft. LM: Data curation, Formal Analysis, Funding acquisition, Investigation, Validation, Writing – original draft. FL: Conceptualization, Project administration, Supervision, Validation, Writing – original draft.
Funding
The author(s) declare financial support was received for the research, authorship, and/or publication of this article. This study was supported by the University of Florence, project RICTD2122, by Tuscany Region (TagSARS CoV 2), by Ministry of Health project RF-2021-12374177 and GR-2021-12372615.
Acknowledgments
We thank all the subjects who participated in the study.
Conflict of interest
The authors declare that the research was conducted in the absence of any commercial or financial relationships that could be construed as a potential conflict of interest.
The author(s) declared that they were an editorial board member of Frontiers, at the time of submission. This had no impact on the peer review process and the final decision.
Publisher’s note
All claims expressed in this article are solely those of the authors and do not necessarily represent those of their affiliated organizations, or those of the publisher, the editors and the reviewers. Any product that may be evaluated in this article, or claim that may be made by its manufacturer, is not guaranteed or endorsed by the publisher.
Supplementary material
The Supplementary Material for this article can be found online at: https://www.frontiersin.org/articles/10.3389/fimmu.2023.1322594/full#supplementary-material
References
1. Tepasse PR, Hafezi W, Lutz M, Kühn J, Wilms C, Wiewrodt R, et al. Persisting SARS-CoV-2 viraemia after rituximab therapy: two cases with fatal outcome and a review of the literature. Br J Haematol (2020) 190(2):185–8. doi: 10.1111/bjh.16896
2. Deepak P, Kim W, Paley MA, Yang M, Carvidi AB, Demissie EG, et al. Effect of immunosuppression on the immunogenicity of mRNA vaccines to SARS-coV-2 : a prospective cohort study. Ann Intern Med (2021) 174(11):1572–85. doi: 10.7326/M21-1757
3. Shah N, Mustafa SS, Vinh DC. Management of secondary immunodeficiency in hematological Malignancies in the era of modern oncology. Crit Rev Oncol Hematol (2023) 181:103896. doi: 10.1016/j.critrevonc.2022.103896
4. Patel SY, Carbone J, Jolles S. The expanding field of secondary antibody deficiency: causes, diagnosis, and management. Front Immunol (2019) 10:33. doi: 10.3389/fimmu.2019.00033
5. Casan JML, Wong J, Northcott MJ, Opat S. Anti-CD20 monoclonal antibodies: reviewing a revolution. Hum Vaccin Immunother. (2018) 14(12):2820–41. doi: 10.1080/21645515.2018.1508624
6. Sehn LH, Gascoyne RD. Diffuse large B-cell lymphoma: optimizing outcome in the context of clinical and biologic heterogeneity. Blood (2015) 125(1):22–32. doi: 10.1182/blood-2014-05-577189
7. Pescovitz MD. Rituximab, an anti-cd20 monoclonal antibody: history and mechanism of action. Am J Transplant (2006) 6(5 Pt 1):859–66. doi: 10.1111/j.1600-6143.2006.01288.x
8. Ohmachi K, Niitsu N, Uchida T, Kim SJ, Ando K, Takahashi N, et al. Multicenter phase II study of bendamustine plus rituximab in patients with relapsed or refractory diffuse large B-cell lymphoma. J Clin Oncol (2013) 31(17):2103–9. doi: 10.1200/JCO.2012.46.5203
9. DeWolf S, Laracy JC, Perales MA, Kamboj M, van den Brink MRM, Vardhana S. SARS-CoV-2 in immunocompromised individuals. Immunity (2022) 55(10):1779–98. doi: 10.1016/j.immuni.2022.09.006
10. Mounier N, El Gnaoui T, Tilly H, Canioni D, Sebban C, Casasnovas RO, et al. Rituximab plus gemcitabine and oxaliplatin in patients with refractory/relapsed diffuse large B-cell lymphoma who are not candidates for high-dose therapy. A phase II Lymphoma Study Association trial. Haematologica (2013) 98(11):1726–31. doi: 10.3324/haematol.2013.090597
11. Aksoy S, Dizdar O, Hayran M, Harputluoğlu H. Infectious complications of rituximab in patients with lymphoma during maintenance therapy: a systematic review and meta-analysis. Leuk Lymphoma (2009) 50(3):357–65. doi: 10.1080/10428190902730219
12. Moor MB, Suter-Riniker F, Horn MP, Aeberli D, Amsler J, Möller B, et al. Humoral and cellular responses to mRNA vaccines against SARS-CoV-2 in patients with a history of CD20 B-cell-depleting therapy (RituxiVac): an investigator-initiated, single-centre, open-label study. Lancet Rheumatol (2021) 3(11):e789–97. doi: 10.1016/S2665-9913(21)00251-4
13. Mrak D, Tobudic S, Koblischke M, Graninger M, Radner H, Sieghart D, et al. SARS-CoV-2 vaccination in rituximab-treated patients: B cells promote humoral immune responses in the presence of T-cell-mediated immunity. Ann Rheum Dis (2021) 80(10):1345–50. doi: 10.1136/annrheumdis-2021-220781
14. Magliulo D, Wade SD, Kyttaris VC. Immunogenicity of SARS-CoV-2 vaccination in rituximab-treated patients: Effect of timing and immunologic parameters. Clin Immunol (2022) 234:108897. doi: 10.1016/j.clim.2021.108897
15. Jyssum I, Kared H, Tran TT, Tveter AT, Provan SA, Sexton J, et al. Humoral and cellular immune responses to two and three doses of SARS-CoV-2 vaccines in rituximab-treated patients with rheumatoid arthritis: a prospective, cohort study. Lancet Rheumatol (2022) 4(3):e177–87. doi: 10.1016/S2665-9913(21)00394-5
16. Cossarizza A, Chang HD, Radbruch A, Acs A, Adam D, Adam-Klages S, et al. Guidelines for the use of flow cytometry and cell sorting in immunological studies (second edition). Eur J Immunol (2019) 49(10):1457–973. doi: 10.1002/eji.201970107
17. Mazzoni A, Vanni A, Spinicci M, Lamacchia G, Kiros ST, Rocca A, et al. SARS-CoV-2 infection and vaccination trigger long-lived B and CD4+ T lymphocytes with implications for booster strategies. J Clin Invest (2022) 132(6):e157990. doi: 10.1172/JCI157990
18. Zhang Z, Mateus J, Coelho CH, Dan JM, Moderbacher CR, Gálvez RI, et al. Humoral and cellular immune memory to four COVID-19 vaccines. Cell (2022) 185(14):2434–2451.e17. doi: 10.1016/j.cell.2022.05.022
19. Mazzoni A, Salvati L, Maggi L, Annunziato F, Cosmi L. Hallmarks of immune response in COVID-19: Exploring dysregulation and exhaustion. Semin Immunol (2021) 55:101508. doi: 10.1016/j.smim.2021.101508
20. Bange EM, Han NA, Wileyto P, Kim JY, Gouma S, Robinson J, et al. CD8(+) T cells contribute to survival in patients with COVID-19 and hematologic cancer. Nat Med (2021) 27:1280–9. doi: 10.1038/s41591-021-01386-7
21. Vijenthira A, Gong IY, Fox TA, Booth S, Cook G, Fattizzo B, et al. Outcomes of patients with hematologic Malignancies and COVID-19: a systematic review and meta-analysis of 3377 patients. Blood. (2020) 136(25):2881–92. doi: 10.1182/blood.2020008824
22. Passamonti F, Cattaneo C, Arcaini L, Bruna R, Cavo M, Merli F, et al. Clinical characteristics and risk factors associated with COVID-19 severity in patients with haematological Malignancies in Italy: a retrospective, multicentre, cohort study. Lancet Haematol (2020) 7(10):e737–45. doi: 10.1016/S2352-3026(20)30251-9
23. Candon S, Lemee V, Leveque E, Etancelin P, Paquin C, Carette M, et al. Dissociated humoral and cellular immune responses after a three-dose schema of BNT162b2 vaccine in patients receiving anti-CD20 monoclonal antibody maintenance treatment for B-cell lymphomas. Haematologica (2022) 107(3):755–8. doi: 10.3324/haematol.2021.280139
24. Perry C, Luttwak E, Balaban R, Shefer G, Morales MM, Aharon A, et al. Efficacy of the BNT162b2 mRNA COVID-19 vaccine in patients with B-cell non-Hodgkin lymphoma. Blood Adv (2021) 5:3053–61. doi: 10.1182/bloodadvances.2021005094
25. Ishio T, Tsukamoto S, Yokoyama E, Izumiyama K, Saito M, Muraki H, et al. Anti-CD20 antibodies and bendamustine attenuate humoral immunity to COVID-19 vaccination in patients with B-cell non-Hodgkin lymphoma. Ann Hematol (2023) 12:1–11. doi: 10.1007/s00277-023-05204-7
26. Vijenthira A, Gong I, Betschel SD, Cheung M, Hicks LK. Vaccine response following anti-CD20 therapy: a systematic review and meta-analysis of 905 patients. Blood Adv (2021) 5:2624–43. doi: 10.1182/bloodadvances.2021004629
27. Cattaneo C, Cancelli V, Imberti L, Dobbs K, Sottini A, Pagani C, et al. Production and persistence of specific antibodies in COVID-19 patients with hematologic Malignancies: role of rituximab. Blood Cancer J (2021) 11:151. doi: 10.1038/s41408-021-00546-9
28. Herishanu Y, Avivi I, Aharon A, Shefer G, Levi S, Bronstein Y, et al. Efficacy of the BNT162b2 mRNA COVID-19 vaccine in patients with chronic lymphocytic leukemia. Blood (2021) 137:3165–73. doi: 10.1182/blood.2021011568
29. Bitoun S, Henry J, Desjardins D, Vauloup-Fellous C, Dib N, Belkhir R, et al. Rituximab impairs B cell response but not T cell response to COVID-19 vaccine in autoimmune diseases. Arthritis Rheumatol (2022) 74(6):927–33. doi: 10.1002/art.42058
30. Cheson BD, Wendtner CM, Pieper A, Dreyling M, Friedberg J, Hoelzer D, et al. Optimal use of bendamustine in chronic lymphocytic leukemia, non-Hodgkin lymphomas, and multiple myeloma: treatment recommendations from an international consensus panel. Clin Lymphoma Myeloma Leuk (2010) 10:21–7. doi: 10.3816/CLML.2010.n.002
31. Brugger W, Ghielmini M. Bendamustine in indolent non-Hodgkin’s lymphoma: a practice guide for patient management. Oncologist (2013) 18:954–64. doi: 10.1634/theoncologist.2013-0079
32. Rummel MJ, Niederle N, Maschmeyer G, Banat GA, von Grünhagen U, Losem C, et al. Bendamustine plus rituximab versus CHOP plus rituximab as first-line treatment for patients with indolent and mantle-cell lymphomas: an open-label, multicentre, randomised, phase 3 non-inferiority trial. Lancet (2013) 381:1203–10. doi: 10.1016/S0140-6736(12)61763-2
33. Knauf WU, Lissichkov T, Aldaoud A, Liberati A, Loscertales J, Herbrecht R, et al. Phase III randomized study of bendamustine compared with chlorambucil in previously untreated patients with chronic lymphocytic leukemia. J Clin Oncol (2009) 27:4378–84. doi: 10.1200/JCO.2008.20.8389
34. Cheson BD, Friedberg JW, Kahl BS, Van der Jagt RH, Tremmel L. Bendamustine produces durable responses with an acceptable safety profile in patients with rituximab-refractory indolent non-Hodgkin lymphoma. Clin Lymphoma Myeloma Leuk (2010) 10:452–7. doi: 10.3816/CLML.2010.n.079
35. Mazzoni A, Vanni A, Spinicci M, Capone M, Lamacchia G, Salvati L, et al. SARS-coV-2 spike-specific CD4+ T cell response is conserved against variants of concern, including omicron. Front Immunol (2022) 13:801431. doi: 10.3389/fimmu.2022.801431
Keywords: SARS-CoV-2, mRNA vaccine, B cell lymphoma, anti-CD20mAb, rituximab, bendamustine, humoral response, T cell response
Citation: Vanni A, Salvati L, Mazzoni A, Lamacchia G, Capone M, Francalanci S, Kiros ST, Cosmi L, Puccini B, Ciceri M, Sordi B, Rossolini GM, Annunziato F, Maggi L and Liotta F (2023) Bendamustine impairs humoral but not cellular immunity to SARS-CoV-2 vaccination in rituximab-treated B-cell lymphoma–affected patients. Front. Immunol. 14:1322594. doi: 10.3389/fimmu.2023.1322594
Received: 16 October 2023; Accepted: 17 November 2023;
Published: 01 December 2023.
Edited by:
Antonella Prisco, National Research Council (CNR), ItalyReviewed by:
Matteo Bulati, Mediterranean Institute for Transplantation and Highly Specialized Therapies (ISMETT), ItalyGiovanna Del Pozzo, National Research Council (CNR), Italy
Copyright © 2023 Vanni, Salvati, Mazzoni, Lamacchia, Capone, Francalanci, Kiros, Cosmi, Puccini, Ciceri, Sordi, Rossolini, Annunziato, Maggi and Liotta. This is an open-access article distributed under the terms of the Creative Commons Attribution License (CC BY). The use, distribution or reproduction in other forums is permitted, provided the original author(s) and the copyright owner(s) are credited and that the original publication in this journal is cited, in accordance with accepted academic practice. No use, distribution or reproduction is permitted which does not comply with these terms.
*Correspondence: Francesco Annunziato, ZnJhbmNlc2NvLmFubnVuemlhdG9AdW5pZmkuaXQ=