- 1Department of Medicine, The University of Colorado Anschutz Medical Campus, Aurora, CO, United States
- 2Department of Clinical Sciences, The Colorado State University Veterinary Teaching Hospital, Fort Collins, CO, United States
- 3Department of Clinical Sciences, Montclaire Animal Clinic, Denver, CO, United States
- 4Op-T, LLC, Fitzsimmons Innovation Bioscience, Aurora, CO, United States
Introduction: Canine diabetes mellitus (CDM) is a relatively common endocrine disease in dogs. Many CDM clinical features resemble human type 1 diabetes mellitus (T1DM), but lack of autoimmune biomarkers makes calling the disease autoimmune controversial. Autoimmune biomarkers linking CDM and T1DM would create an alternative model for drug development impacting both human and canine disease.
Methods: We examined peripheral blood of diagnosed CDM dog patients comparing it to healthy control (HC) dogs. Dogs were recruited to a study at the Colorado State University Veterinary Teaching Hospital and blood samples collected for blood chemistry panels, complete blood counts (CBC), and immunologic analysis. Markers of disease progression such as glycated albumin (fructosamine, the canine equivalent of human HbA1c) and c-peptide were addressed.
Results: Significant differences in adaptive immune lymphocytes, innate immune macrophages/monocytes and neutrophils and differences in platelets were detected between CDM and HC based on CBC. Significant differences in serum glucose, cholesterol and the liver function enzyme alkaline phosphatase were also detected. A systemic immune inflammation index (SII) and chronic inflammation index (CII) as measures of dynamic changes in adaptive and innate cells between inflammatory and non-inflammatory conditions were created with highly significant differences between CDM and HC. Th40 cells (CD4+CD40+ T cells) that are demonstrably pathogenic in mouse T1DM and able to differentiate diabetic from non-diabetic subjects in human T1DM were significantly expanded in peripheral blood mononuclear cells.
Conclusions: Based on each clinical finding, CDM can be categorized as an autoimmune condition. The association of significantly elevated Th40 cells in CDM when compared to HC or to osteoarthritis, a chronic but non-autoimmune disease, suggests peripheral blood Th40 cell numbers as a biomarker that reflects CDM chronic inflammation. The differences in SII and CII further underscore those findings.
Introduction
Canine diabetes mellitus (CDM) is a relatively common endocrine related disease in companion dogs (1). The prevalence ranges from 0.1 to 1.2% accounting for 165,000 diabetic dogs in the U.S., with the incidence rate increasing by 79.7% since 2006 (2) Treatment options are very limited and thus the euthanasia rate is 10% at diagnosis with an additional 10% one year later (2). As with humans, disease etiology is poorly understood. CDM involves persistent hyperglycemia resulting from insulin deficiency caused by massive beta cell loss (3). Symptoms include polyuria, polydipsia, polyphagia, weight loss, cataracts, and chronic or recurring infections as described by the American Veterinary Medical Association. In human subjects, diabetes mellitus (DM) occurs predominantly as type 1 (T1DM) or type 2 (T2DM). T2DM involves insulin resistance while T1DM is classically an autoimmune disease involving both adaptive and innate immune cells that attack the pancreatic islets leading to chronic inflammation defined as immune cell infiltrates in the islets of Langerhans, and subsequent loss of insulin production.
CDM is usually diagnosed in middle and older age adult dogs. In a study of 860 CDM dogs, the mean age of onset was 8.6 years (4). Human T1D is more common in children but the incidence is expanding in young and older adults (5). CDM can occur in any breed, but studies of breed prevalence suggest that there may be some level of genetic predisposition (4). CDM and human DM share multiple comorbidities that include diabetic ketoacidosis, a severe life-threatening metabolic derangement, cataracts, urinary tract infection (UTI), hyper-adrenocorticism, and dermatitis. CDM is a completely insulin dependent condition, but lack of biomarkers make defining it as an immune-mediated disease controversial (6). In human DM autoantibodies have been well described. In CDM autoantibodies (Aab) to insulin, GAD-65 and/or canine islet-antigen-2 (IA-2) have been reported in a small cohort of diabetic dogs (1, 7). Unfortunately, canine autoantibody detection has been notoriously unreliable. Studies showed that GAD65 antibodies were detected in 0 -13% of CD, 0 – 10% tested positive for IA2 antibodies and 0% tested positive for Zinc transporter 8 antibodies (8). A recent study used a nucleic acid programmable protein array platform to query 1700 pancreatic proteins as possible antigens for canine antibodies (9). In that study 6 autoantibodies were identified that had sensitivity greater than 10% (9). Given the difficulty with auto antibody detection in CDM, alternate diagnostic approaches should be developed.
In this report, we examined twelve diabetic companion dogs that were diagnosed at the Colorado State University Veterinary Teaching Hospital (CSU-VTH) in Fort Collins, CO and in a veterinary clinic. Based on history, physical examination, and persistent fasted hyperglycemia and glucosuria, all dogs met the veterinary criteria for CDM. Blood samples were collected for clinical laboratory and research laboratory assessment, analyzed, and compared to healthy control (HC) dogs. The total number of lymphocytes, monocytes, neutrophils, and platelets were examined. We examined serum glucose, cholesterol, and the cholestatic liver enzyme alkaline phosphatase (ALP). Various indices of dynamic leukocyte changes as possible predictors of chronic/systemic inflammation were explored. C-peptide levels as a measure of beta cell function were examined. Levels of glycosylated albumin (i.e., fructosamine) as a measure of chronic hyperglycemia were examined. We discovered that like in human T1DM and laboratory mouse autoimmune diabetes, CDM demonstrates a significant elevation in a subset of peripheral blood cells called Th40 cells (10). The discovery that Th40 cell levels in CDM dogs are substantially and significantly elevated compared to healthy controls supports the use of Th40 cell numbers as a canine biomarker for chronic inflammation. Th40 cells from CDM produce high levels of inflammatory cytokines including IL-6 and IFNγ, consistent with Th40 counterparts from mice (11) and human (12, 13). These findings further underscore that significant increases in Th40 cells correlate with chronic inflammation associated with autoimmunity in multiple species. That, in addition to other findings, supports the claim that CDM is more akin to T1DM, likely latent autoimmune diabetes in adults (LADA), than other forms of DM.
Materials and methods
Diabetic dogs
A pilot study to examine chronic inflammatory markers in canine diabetic dogs was conducted at the Colorado State University Veterinary Teaching Hospital (CSU-VTH) in Fort Collins, CO. Dogs from the small animal medicine service or from private veterinary clinics were recruited for the study. An Institutional Review Board (IRB) approval for the pilot study was instituted by the CSU-VTH IRB panel study titled “A Pilot Study comparing Canine Diabetes and Healthy Control Dogs”. At CSU-VTH, dogs that were regular, patients that had been diagnosed with diabetes were recruited; dogs from veterinary clinics were recruited by the attending veterinarians; the same consent process was used. Twelve dogs meeting the criteria for CDM were added to the study. Normal glucose at the CSU-VTH diagnostic lab is 70 – 115 mg/dl blood. Criteria for diagnosis of diabetes was a persistent fasting blood glucose greater than 120 mg/dl and detected glucosuria. Blood and urine for clinical labs, complete blood count (CBC), and research assays were obtained. An Adult Wellness Chemistry panel with SDMA and CBC was performed by a certified clinical laboratory, Antech Diagnostic Laboratory, Englewood CO. Veterinary standard of care was observed; all dogs were prescribed insulin at a dose determined by the attending veterinarian. A description of the dogs is in Table 1.
Healthy controls
A meta-analysis chart review from a Veterinary Clinic was used for healthy controls (HC). All experiments were approved by the CSU-VTH IRB as described above. Frozen peripheral blood mononuclear cell (PBMC) samples from 11 male and 8 female HC dogs that had undergone spay or neuter surgery or were seen as a healthy checkup, were used. Of those, 7 male and 5 female, had complete records (CBC and Chemistry panel). The dogs were age 9 months – 3 yrs. The breeds were mixed. An Adult Wellness Chemistry with SDMA and CBC was performed by a certified clinical laboratory, Antech Diagnostic Laboratory, Englewood CO. The data were collected from chart review. Permission from owners for access to chart data was obtained by the veterinarians. HC providing research samples were recruited by CSU-VTH veterinarians. These included client-owned dogs seen for wellness checks or minor injuries. Blood and urine samples were processed in the Clinical Pathology laboratory at the VTH. All HC dogs were covered under the approved IRB and all owners signed an approved consent form.
Research blood samples
Research blood samples, approximately 3 ml, were collected in 10 ml heparinized tubes by venipuncture for analysis. Blood was diluted 1:1 with Phosphate buffered saline (PBS, pH 7.2). Blood was layered over Ficoll-Paque Plus for density gradient separation to collect PBMC. Ficoll-Paque Plus is Ficoll PM400, a copolymerization of sucrose and epichlorohydrin sodium diatrizoate with disodium calcium EDTA. Cells were centrifuged at 1400 rpm (400xg) for 30 min. The buffy coat was removed, washed twice in 10 ml PBS and centrifuged at 300xg to collect cells and remove platelets. PBMC were suspended in a staining buffer, PBS/2 mM EDTA/0.5% bovine serum albumin (BSA) and stained for flow cytometry. Cells were aliquoted to 100,000 cells per well in a 96 well plate. Staining antibodies included: anti-CD3 (FITC) from Bio-Rad, cat# MCA1774F; anti-CD4 (APC) from Bio-Rad, cat#MCA1038APC; anti-CD40 (G28-5; Alexafluor 405) produced in house; there is no canine anti-CD40 antibody available so the G28-5 anti-CD40 that recognizes human CD40 was used. Th40 cells are defined by gating on CD3, then determining the percentage of CD4+CD40+ cells within the total CD4+ population as previously described for human T1D (13, 14).
Cytokine analysis
Frozen PBMC from nine diabetic dogs were thawed for analysis. Cells were incubated in RPMI culture medium, 5% BSA for 1 hour. Cells were stained for CD3 (FITC); CD4 (PE); CD40 (ViolBlue). Cells were fixed in 2% paraformaldehyde for 10 minutes, then incubated in 100 μl permeabilization buffer (e-Bioscience, San Diego, CA). Cells were treated with biotinylated anti-IL-6 (R&D Systems, a goat polyclonal anti-dog IL-6 IgG) or biotinylated anti-IFNγ (R&D Systems, a goat polyclonal anti-dog IFNγ IgG) for 10 mins. Cells were washed, suspended in permeabilization buffer, and incubated with APC conjugated streptavidin for 10 mins. Cells were washed and analyzed on a Beckman Coulter Cytoflex flow cytometer.
C-peptide ELISA
C-peptide levels were assayed in plasma (retrieved from the PBMC isolation) using a Canine C-peptide ELISA kit from Sigma Aldrich, cat# EZCCP-47K. The manufacturer protocol was followed. Samples were analyzed no more than one month after acquisition.
Statistical analysis
Statistical analysis was performed using GraphPad Prism 9.5.1 software. In each case, two-tailed, unpaired t-tests were performed. Statistical significance was confirmed using ANOVA when appropriate.
Ethics statement
All experiments were carried out in accordance with ARRIVE guidelines. All experiments were performed under a protocol approved by the Colorado State University Veterinary Teaching Hospital IRB. All methods were carried out in accordance with relevant guidelines and regulations.
Results
Glucose levels
Hyperglycemia and glucosuria are hallmarks for CDM and a requirement for the diagnosis and study entry. The CDM study dogs included nine new onset (diagnosed within –1 to 6 months) and three long-term (diagnosed greater than ≥18 months) diabetic dogs. The age, breed, and disease duration of participants is shown in Table 1. All diabetic dogs were greater than 5 years of age, which is typical of CDM (2). Regardless of sex or breed, all dogs demonstrated significantly (p < 0.0001) elevated blood glucose compared to HC (Figure 1A). The CDM dogs had a glucose range from 176 to 616 mg/dl, with a mean 394.0 ± 42.90 mg/dl (Figure 1A). In human subjects, diabetes is diagnosed with a sustained fasting blood glucose level of > 126 mg/dl and/or HBA1c > 6.5%., OR 2-h PG ≥200 mg/dL (11.1 mmol/L) during OGTT; Or patients with classic DM symptoms with random blood glucose > 200 mg/dl (15). In a laboratory setting, diabetes prone mice are diagnosed when blood glucose levels reach and maintain 250 mg/dl (16). Glucose was detected in the urine of all the diabetic dogs, demonstrating glucosuria, which occurs when the blood glucose concentration exceeds the renal threshold for kidney regulation; approximately 180 mg/dL in canines.
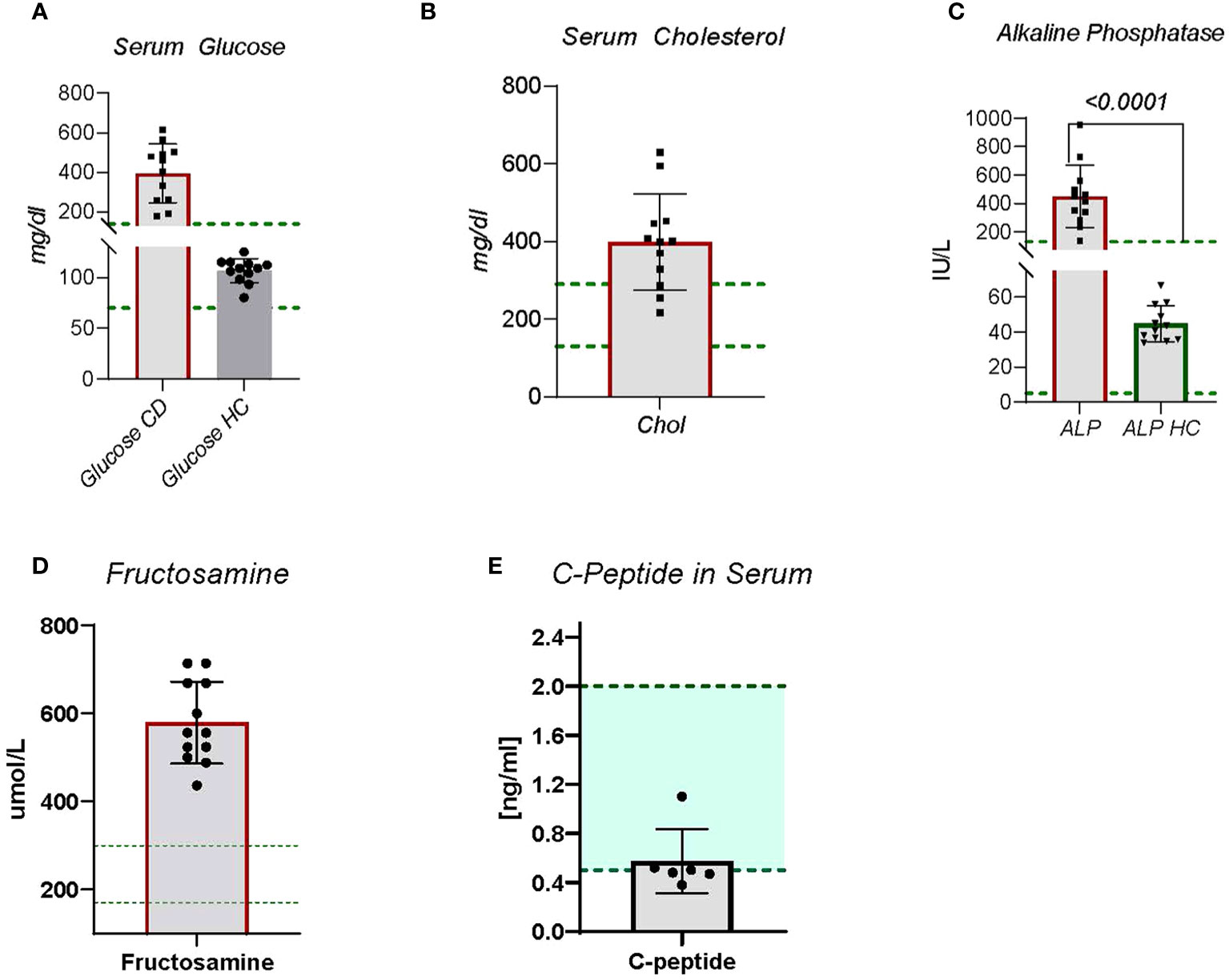
Figure 1 Blood chemistry panels were collected on CDM and HC dogs. Blood was sent to Antech Diagnostic Laboratory for analysis. (A) Serum glucose is a standard observation, (B) Serum cholesterol is not obtained on most dogs but is included in diabetic dogs due to risk of cardiovascular disease. (C) Alkaline Phosphatase, (D) Fructosamine, and (E) C-Peptide The green lines indicate established normal range. Statistics were done using GraphPad Prism using a two-tailed, unpaired t-test analysis.
Diabetic ketoacidosis
A criterion for inclusion was glucosuria. Unregulated diabetes can progress to diabetic ketoacidosis (DKA) in both canine (17) and human subjects (18). DKA clinical conditions include blood glucose greater than 250 mg/dl, the presence of ketone bodies by urinalysis, and serum bicarbonate levels less than 18 mmol/L, suggesting acidic conditions (18). Of the 12 dogs in the study, four presented with likely DKA (Table 2). They had the highest blood glucose levels, each had detectable ketone bodies in urine, each had a urine pH under physiologic 7.0 indicating acidity, and each had bicarbonate levels under 18 (Table 2). Three other dogs were at risk, with a urine pH under 7 and urine glucose ≥ 2.0. Because CDM often goes undetected for long periods of time, DKA development can be common.
Hypercholesterolemia
During inflammation the increased demand for adaptive and innate immune cells causes increased metabolic requirements. A common mechanism to satisfy metabolic demands is increased cholesterol production and metabolism (19). Cholesterol metabolism plays a key role in maintenance of innate and adaptive immune cells (19). Nine of the twelve CDM dogs demonstrated moderate to severe hypercholesterolemia (Figure 1B). Two of the dogs had normal cholesterol levels and the other was borderline high (Figure 1B). No direct correlation between serum glucose levels and cholesterol levels were observed, however.
Alkaline phosphatase
Alkaline phosphatase (ALP) is a zinc modulated enzyme involved in protein metabolism. Increases in ALP often are associated with cholestatic disease, cancers, liver problems, bone disorders, or reactive hepatopathies. In human diabetic subjects increases in ALP have been reported (20). In fact, clinically elevated ALP is associated with increased risk for cardiovascular disease and mortality (20), and for changes in bone density (21). In CDM dogs, ALP was significantly (p < 0.0001) increased compared to HC dogs (Figure 1C). All CDM were substantially outside the normal range (Figure 1C).
Fructosamine
A measure of disease control in human diabetes is glycated hemoglobin A1c (Hb A1c). Because of the rapid turnover rate for HbA1C, 14 days compared to 35 days for fructosamine (22), in veterinary patients fructosamine, glycosylated albumin, is examined (23, 24). Fructosamine levels in all diabetic dogs were substantially above the canine normal range (Figure 1D), which is consistent with unregulated disease.
C-peptide
A measure of beta cell function is detection of C-peptide, which is generated when pro-insulin is processed in the islet beta cell to mature insulin. The process creates the mature insulin A- B chain molecule and releases C-peptide in serum. When beta cell function is fully dysregulated, C-peptide can no longer be detected. The CDM dogs each had detectable C- peptide, but at levels below the established normal range (Figure 1E). This suggests some active beta cells are present in these dogs, but the activity is not sufficient to regulate the blood glucose concentration.
Lymphocyte dysregulation
Autoimmune disease necessarily involves immunocyte dysfunction, but in CDM the question arises as to whether the disease etiology is autoimmune. Focusing on complete blood counts from patients, we examined total lymphocyte counts, and innate cells including monocytes and neutrophils. In diabetic subjects, lymphocyte numbers were significantly (p < 0.001) lower than in HC dogs (Figure 2A). This observation includes both CD4 and CD8 T cells and B cells as cell type differentiation was not performed; clinical laboratories do not perform this differentiation on canine blood. A decrease in lymphocytes likely relates to changes in metabolism, to be discussed further.
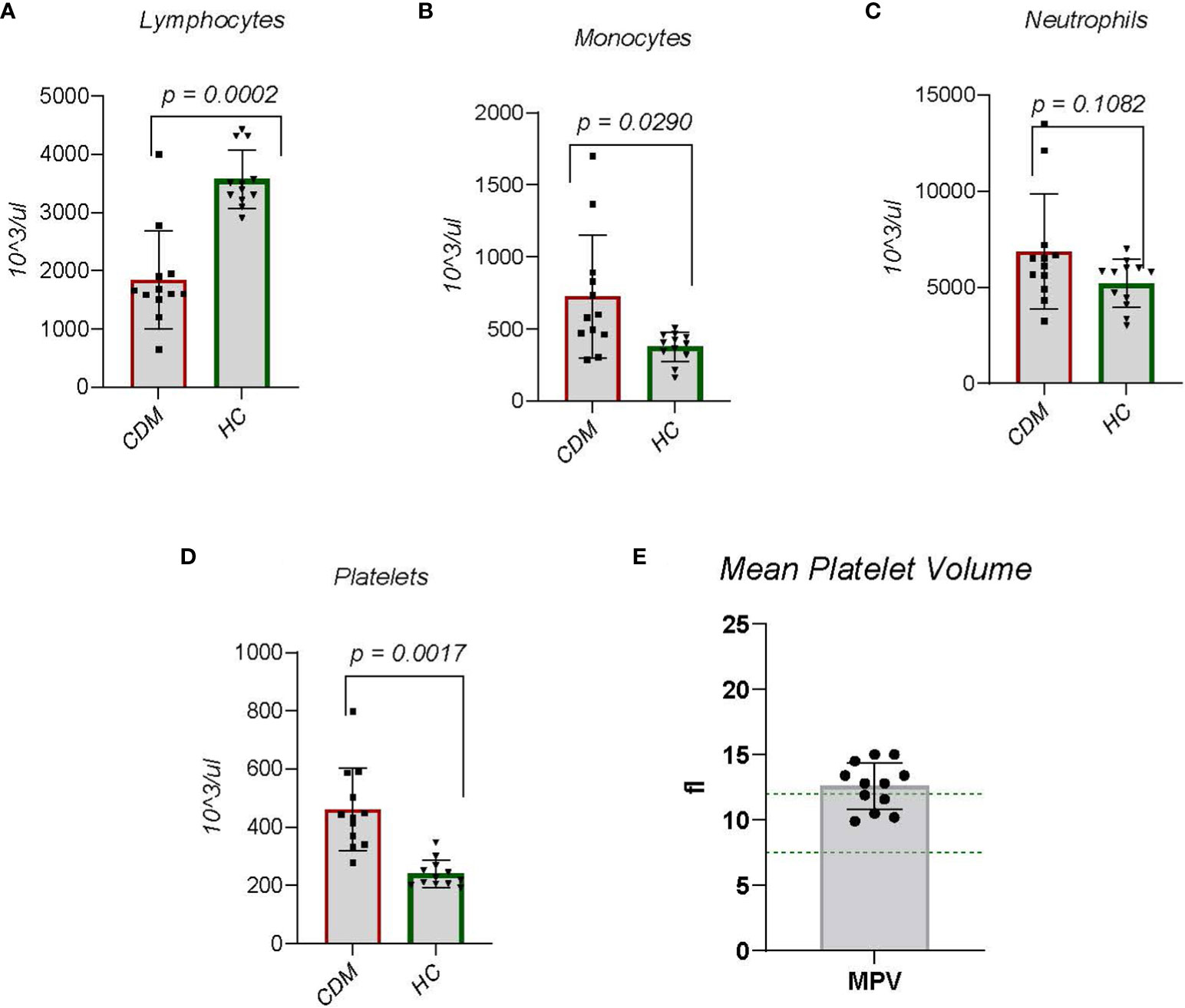
Figure 2 Data are taken from complete blood counts done on each dog; the absolute number per microliter is reported. (A) Lymphocytes, (B) Monocytes, (C) Neutrophils, (D) Platelets and (E) mean platelet volume (MPV) were done. MPVs were done only on the CDM dogs. Like serum cholesterol, MPV is a special request in the CBC. Statistics were done using GraphPad Prism using a two-tailed, unpaired t-test analysis.
Innate immune dysregulation
Monocyte numbers representing innate immune cells in CDM dogs were increased significantly (p = 0.0166) compared to HC dogs (Figure 2B). Neutrophils, another innate immune cell, produce high levels of NADPH oxidase (NOX-2) which is implicated in autoimmunity and hydrogen peroxide generated from neutrophils suppresses lymphocyte activation and cell numbers (25). While the overall number of neutrophils in CDM was increased there was no significant difference between CDM and HC neutrophil counts (Figure 2C). Interestingly, the two CDM dogs that had substantially high neutrophil numbers were new onset diabetics.
Platelet dysregulation
Platelets play a clear role in hemostasis and thrombosis, but growing evidence suggest a contributory role to chronic inflammation (26, 27). Platelets are derived from megakaryocytes and inflammatory cytokines induce rupture of megakaryocytes to increase platelet numbers (28). In both new onset and long-term CDM, platelet numbers were significantly (p = 0.0007) elevated compared to HC (Figure 2D). A feature of platelets that reflects both age of the platelet and platelet activity, i.e. more activated, is platelet size registered by mean platelet volume (MPV) (28). Mean platelet volume in 7 of the 12 CDM dogs was above normal range (Figure 2E). MPV from HC was not available.
Systemic/chronic inflammation index
Inflammation involves interactions between adaptive immune cells, and innate cells. Attempting to develop inflammatory biomarkers, an index of neutrophil to lymphocyte ratio (NLR) has been created (29, 30). The ratio accounts for dynamic changes in neutrophils and lymphocytes during chronic inflammation. NLR has been described in chronic diseases including coronary heart disease, stroke, diabetes, and cancer of solid organs (30). In one study NLR increases were significantly associated with early neurological deterioration differentiating diabetic and non-diabetic subjects (29). We compared NLR between CDM and HC dogs (Figure 3A) with significantly (p < 0.0001) striking differences. All CDM dogs had substantially greater NLR. Another approach focusing on innate to adaptive immune cell differences compared the monocyte to lymphocyte ratio (MLR). MLR is more generally associated with cancers, but has been examined in autoimmune disorders including cardiovascular disease (31). In CDM the MLR was significantly (p = 0.013) greater than in HC dogs (Figure 3B). Given the noted differences in platelets between CDM and HC we determined platelet to lymphocyte ratio (PLR). In CDM the PLR was significantly (p < 0.0001) higher than the value in HC dogs (Figure 3C).
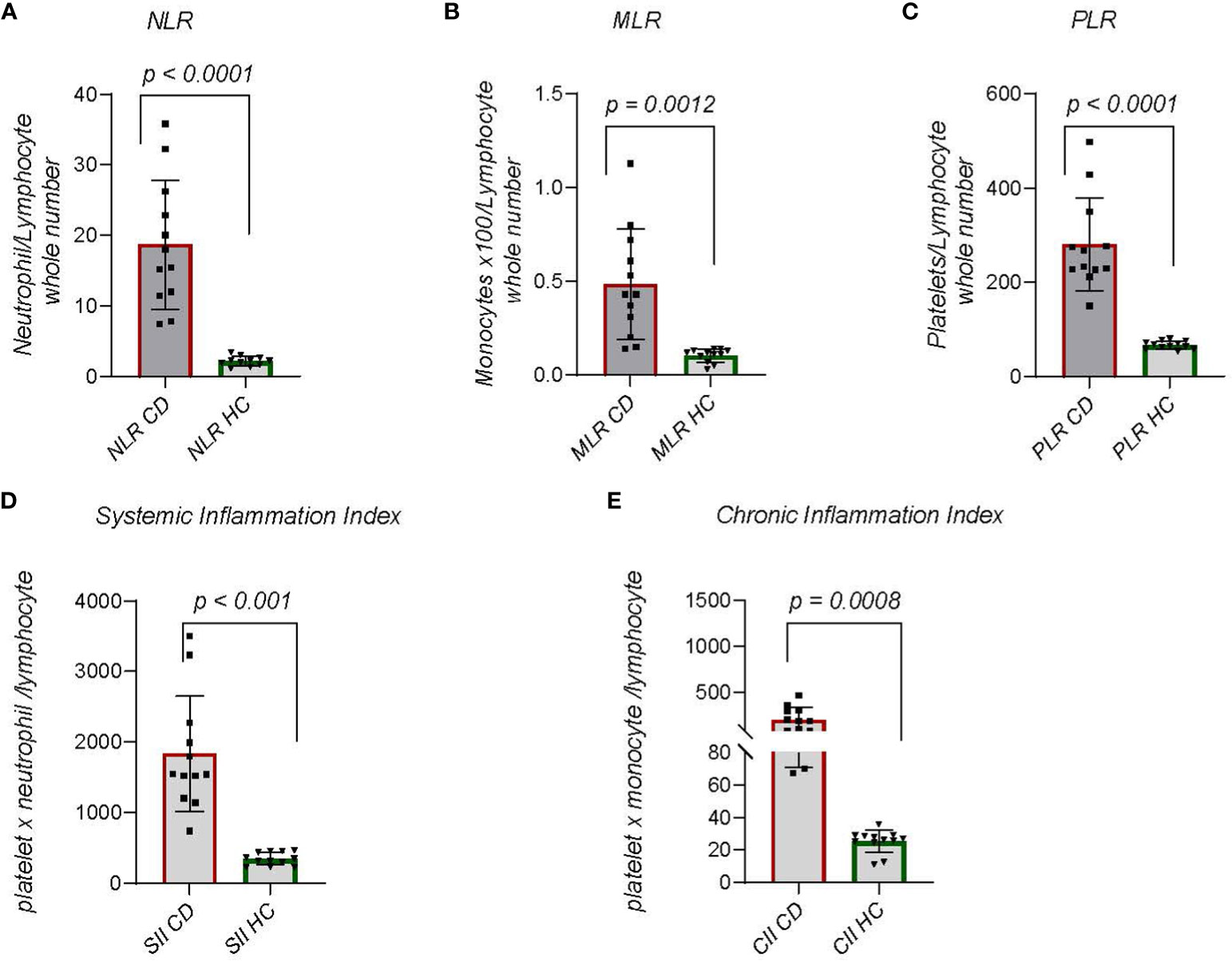
Figure 3 Indices of Chronic Inflammation: (A) Neutrophil to lymphocyte ratio (NLR) is calculated by dividing the absolute number of neutrophils per microliter by the absolute number of lymphocytes per microliter of blood. (B) Monocyte to lymphocyte ratio (MLR) is the absolute number of monocytes divided by the absolute number of lymphocytes. (C) Platelet to lymphocyte (PLR) is the total number of platelets per microliter divided by 103 times absolute number of lymphocytes. (Platelets are reported as whole number x 10^3 per microliter). (D) Systemic Immune Inflammation Index (SII) is calculated by (total number of neutrophils) x (whole number of platelets/10^3)/whole number of lymphocytes. (E) Chronic Immune Inflammation Index (CII) is calculated by: (whole number of monocytes) x (whole number of platelets/10^3)/whole number of lymphocytes. Statistics were done from GraphPad Prism using a two-tailed, unpaired t-test analysis.
Further study of dynamic changes in immune cells as a measure of chronic inflammation led to the creation of the systemic-immune-inflammation index (SII), which is derived by multiplying the total number of neutrophils and platelets then dividing by the total number of lymphocytes (32, 33). This index is used for prognosis of solid tumors but also to predict autoimmune outcomes, predominantly heart disease and rheumatoid arthritis (33). In CDM the SII was significantly (p < 0.0001) greater than that in HC dogs (Figure 3D). We considered that another analysis could be achieved by substituting monocytes for neutrophils in the index. A chronic inflammation index (CII) was derived by multiplying absolute number of monocytes and platelets then dividing by absolute number of lymphocytes (Figure 3E). The difference between CDM and HC dogs was significant (p = 0.0008) and demonstrated a narrower range than the SII.
Th40 cells indicate chronic inflammation
In previous work we showed that a T cell subset described as Th40; CD3+CD4+ helper T cells that express the CD40 receptor (10), is increased in number in murine autoimmune diabetes (16, 34, 35) and in human autoimmune (type 1) diabetes (12, 14). In the murine T1DM model, Th40 cells proved to be pathogenic in adoptive transfer studies (16, 34). In human T1DM Th40 cells were present in healthy controls and in type 2 (non-autoimmune) diabetes but at significantly lower numbers compared to T1DM (14). We compared Th40 cell numbers in CDM to HC dogs. Three of the HC dogs were seen for osteoarthritis, a chronic but not autoimmune condition, otherwise they were seen for a healthy dog check-up, vaccines, or elective surgery (spay and neuter). As reported for laboratory mice and human diabetic subjects, CDM dogs had significantly (p < 0.0001) increased Th40 cell numbers (Figure 4A). Unlike the reports for variable and highly inconsistent levels of autoantibodies in CDM (3, 9) Th40 cells were universally increased in CDM. PBMC were analyzed for CD3 and then CD4+CD40+ within the CD3 gated subset (Figure 4A for controls and 4B for CDM). In the forward versus side scatter plots, CDM demonstrated a high side scatter, lower forward scatter population (Figure 4B) compared to HC dogs (Figure 4A). In CDM the CD3 expression was lower than in HC. HC were further examined by age for Th40 cell differences, but no differences were noted (Supplementary Figure 1A). There were no differences in Th40 cell numbers when differentiated by sex (Supplementary Figure 1B).
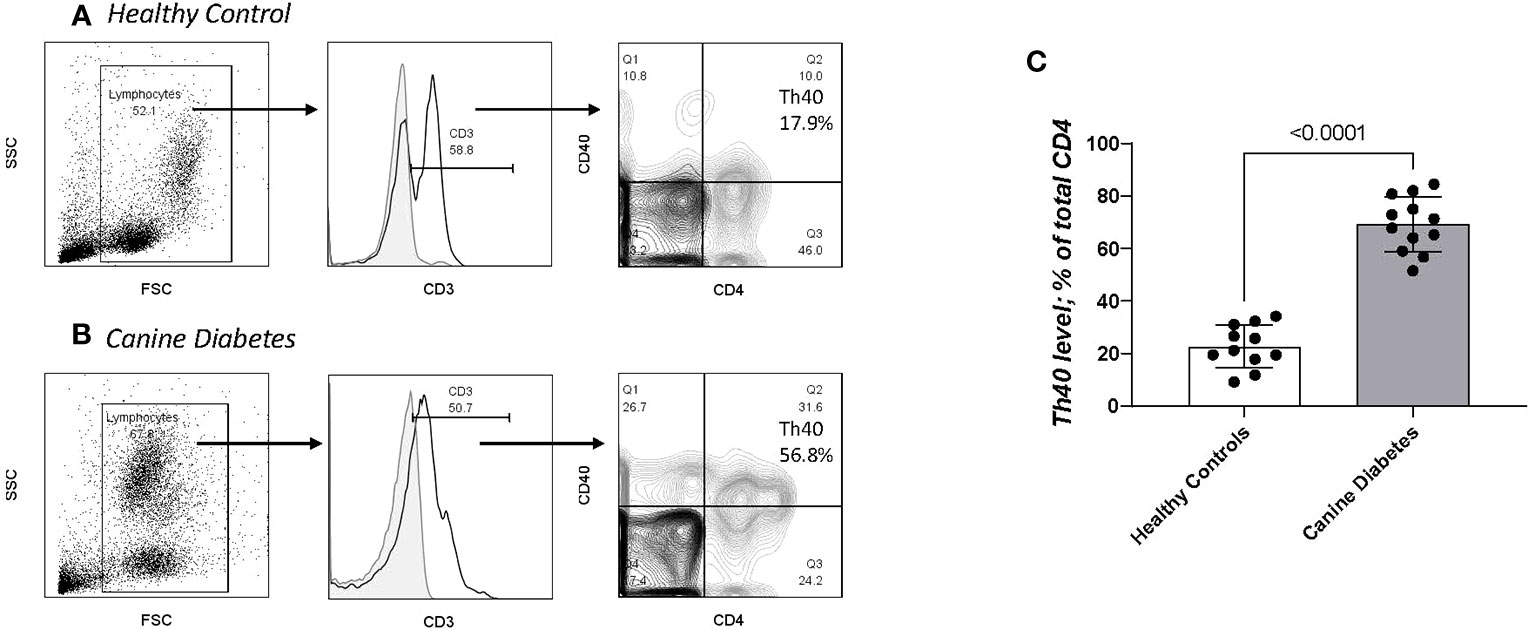
Figure 4 Th40 cell comparison in CD and HC. PBMC analyzed for CD3, CD4 and CD40 (Th40 cells). (A) Healthy controls and (B) Canine Diabetes were analyzed by dot plots to establish forward versus side scatter. Within the FSC x SSC population CD3+ cells are determined above background controls (grey solid histogram). Within the gated CD3+ population, contour plots of CD4 versus CD40 were determined. Th40 cells are defined as CD3+ then CD4+CD40+/total CD4+ cells (Q2/Q2 + Q3). (C) Th40 percentage in CD dogs compared to HC dogs. Statistics were done using Graph Pad Prism using a two-tailed, unpaired t-test analysis.
Pathogenicity of CDM Th40 cells: Pathogenicity of Th40 cells was addressed by examining intracellular cytokine levels (Figure 5). Th40 cells were gated on CD3 then CD4 versus CD40 was determined (Figure 5A) as in Figure 4. Levels of intracellular cytokines from CDM dogs were compared to HC for IL-6 (Figure 5B) and IFNγ (Figure 5C). Th40 versus conventional CD4+ T cells were compared in CDM. For both IL-6 and IFNγ levels in Th40 cells from CDM were significantly higher than levels in HC (Figures 5B, C). Likewise, cytokine levels in Th40 were significantly increased compared to levels in conventional cells from CDM (Figures 5B, C). While cytokine levels in Th40 cells from HC were significantly less than from CDM, they were increased, although not significant, when compared to conventional cells from CDM.
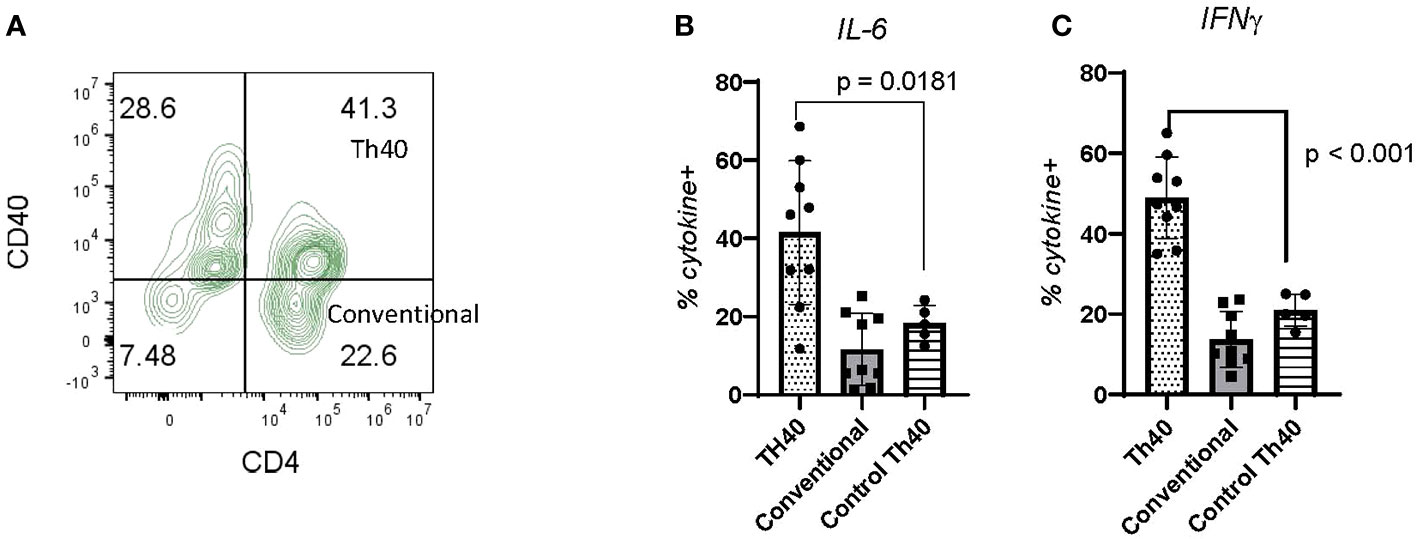
Figure 5 Inflammatory cytokine levels in CDM Th40 cells. PBMC from nine CDM and five healthy controls were analyzed. (A) Cells were stained for CD3 then gated against CD4 and CD40; dot plot is representative of CDM. Within the Th40 (CD4+CD40+) and Conventional T cell (CD4+CD40-) populations, intracellular levels of (B) IL-6 and (C) IFNγ were determined. Statistics were done from GraphPad Prism using a two-tailed, unpaired t-test analysis.
Discussion
While CDM has numerous clinical features in common with human type 1 diabetes mellitus, there are also differences (3). CDM is insulin dependent but onset is restricted to mid to late adulthood (1). Human T1D age of onset varies with the peak occurring between ages of 10 -14, mid juvenile (36). An exception is latent adult diabetes autoimmune (LADA) where the average age of onset is 45.02 yrs (37). In CDM, histopathological studies show that beta cell mass is significantly reduced (38) but the cause remains ill defined. It is certainly possible that autoimmune destruction is responsible. In human subjects the presence of autoantibodies (Aab) has been suggested to be predictive for T1DM with some limitations (39). In CDM however, autoantibodies have been disappointingly inconsistent. Reports are that GAD-65 and Islet Antigen- 2 (IA-2) antibodies can be detected, but only in a small population (40). Another study found that antibodies to pro-insulin were detected in 3 of 15 control, and 8 of 15 newly diagnosed diabetic dogs (41). In human disease, GAD and IA-2 auto antibodies are associated with LADA (37). An exploration of Aab comparing 30 healthy controls and 30 CDM dogs found that at a specificity of 90%, six autoantibodies had sensitivity greater than 10% (9). To attempt to address the lack of Aab in CDM a novel array technique that queried 1700 pancreatic proteins was only able to identify 6 autoantibodies with sensitivity greater than 10% (9). Given the reports that autoantibodies are difficult to detect and not proving predictive in CDM, we considered that alternate biomarkers would be beneficial. To that end, we chose to confirm diabetes associated markers, fructosamine, long-standing hyperglycemia and Th40 cells that were shown to be prominent biomarkers in murine (42–45) and human (12–14) T1DM including utility for predicting T1DM onset (12). Th40 cells were in fact more predictive than auto antibodies in human a pre-T1DM study (12).
Islet architecture may contribute to discrepancies between human and canine diabetes. Canine islets average 78% beta cells with cells distributed throughout the islet but concentrated at the center (46). In human pancreas, beta cells are distributed evenly throughout the islet (47). Mice islet architecture is closer to canine with beta cells localized in the center of the islet (3). During murine insulitis (immune cell infiltration of the islet) immune cells invade the poles of the islet, called peri-insulitis, then move to the center to completely engulf the islet with up to 80% of islets demonstrating infiltration (16, 35). Insulitis is rarer in both human and canine diabetes where typically only portions of the islet are infiltrated (3). For example, humans typically have less than 10% of islets infiltrated compared to NOD mice where typically 85% of islets are infiltrated. In CDM the number of immune cells that infiltrate the islet is substantially lower as well (48). In both human and canine diabetes, immune cells likely remain contributory, but not necessarily in the islet.
Another complicating issue is that diabetic dogs demonstrate islet architecture that is substantially different from normal dogs. The CDM islet has only about 30% beta cells compared to 78% beta cells in HC dogs and the islets are dispersed and difficult to identify in CDM (49). Additionally, in CDM, beta cells could be detected in acinar tissue. This leaves the question of whether beta cells were lost due to autoimmune attack, other attack, or the diabetic condition, e.g., canine genetic state causes fewer beta cells. Given that diabetes onsets so late in canines, mid to late adulthood, it is unlikely that the islet architectural differences are genetic, but rather likely occur due to some persistent stimulus, like chronic inflammation. Also, that CDM occurs in a wide range of breeds, argues against genetics as sole contributor.
Assessment of immune contributors in diabetes and particularly in CDM will require focus on circulating immune cells. Here we report that statistically significant differences in peripheral blood cell numbers occur in CDM compared to HC dogs. Monocytes (innate immune cells) were significantly increased while neutrophils trended toward increase but were not statistically different from HC. Monocytes are among the first cells to attack islets where they produce high levels of inflammatory cytokines (50). Monocytes were detected in human islets in post-mortem sections of diabetics both at time of disease (51) and later (52). Neutrophils likewise infiltrate the pancreas (53) to create neutrophil extracellular traps (NETs) which are inflammatory mediators. NETs are a network of extracellular DNA studded with histone and neutrophil granule proteins that trap and damage microbes (54). In addition, NETs create localized inflammation, impacting the inflammasome in autoimmune diseases (55). NETs are increased in serum of human T1D patients compared to healthy subjects (53).
Our observation of decrease in lymphocyte numbers initially was surprising. T cell attack of islets is well established in autoimmune diabetes. In this study and others, decreases in lymphocytes were described based on analysis of CD4+CD3+ or CD8+CD3+ T cells and CD19+CD20+ B cells done by automated clinical laboratory reports. That system does not consider T cell subsets such as Th40 cells. During autoimmune activation classic CD4 and CD8 cells begin to express CD40 (12, 14, 16). Those cells achieve an activated status and CD4 and CD3 cell surface molecules decrease (56). Th40 cells are increased during autoimmune diabetes in mice (16, 34, 35), humans (12, 14) and dogs as shown here. Th40 cells express lower CD4, TCRαβ and CD3 molecules on the cell surface, but in murine studies those molecules had become internalized (57). Thus, the low lymphocyte number seen in CDM could be illusory, in other words, the cells are present but not detected by standard CBC analysis due to the reduction in cell surface CD4, CD8, and CD3 molecules. Another explanation is that innate cell expansion is high energy demanding, lymphocyte numbers may recede due to lack of metabolic expansive energy.
Platelets were described originally for thrombosis, but more recent data show diverse activity including involvement in inflammation. Platelets interact with lymphocytes, monocytes and neutrophils (58) in each case to modulate effector functions. Platelet interaction with lymphocytes increases tissue extravasation, helper differentiation, and cytokine release (58). Platelets express high levels of CD154 (59) and in fact are the primary source of CD154. Platelets from autoimmune subjects express significantly higher levels of CD154 and other inflammatory markers compared to healthy individuals (60). CDM showed significantly increased numbers of platelets, which can drive inflammation associated processes. Platelet derived CD154 interacting with CD40 on monocytes, neutrophils, B cells and Th40 cells would lead to sustained inflammatory cytokine levels. In acute deep thrombosis studies, platelet to lymphocyte (PLR) ratio increase was associated with higher disease risk (32). In rheumatoid arthritis, a chronic systemic autoimmune disease, subjects had significantly higher PLR and NLR compared to healthy controls (61). PLR also differentiates subjects with Hashimoto’s thyroiditis, one of the fastest growing autoimmune diseases in human subjects, from HC (62). Platelets were significantly expanded in CDM as was the PLR. This finding suggests a crucial role for platelets during CDM as well as describing a potential biomarker index for disease diagnosis. High platelet numbers are a source of CD154 and increased Th40 cells are a source of CD40 providing a milieu of increased risk for inflammation development.
Human T1DM is definitionally autoimmune with adaptive immune cells, T and B cells, and innate immune cells including macrophages, dendritic cells, and neutrophils, playing substantive roles in disease development and progression. In early stages, immune cells invade the pancreatic islets (insulitis) focusing attack on the beta cells which leads to a loss of insulin. Human T1DM often includes the presence of autoantibodies (Aabs) (63). Detection of two or more Aabs in children under the age of 5 is considered predictive (39). As subjects age, the presence of Aab and disease predictability reduces substantially (64). We defined another biomarker for chronic inflammation in human T1DM, Th40 cells (12, 65). Th40 cells are CD4+ helper T cells that express the CD40 receptor. On these cells CD40 acts as a costimulus driving inflammatory cytokine production whereas CD28, another T cell costimulus, favors regulatory cytokine production (10). Th40 cells in peripheral blood of T1DM subjects were found to be elevated up to 6 standard deviations above healthy controls (12, 14). In a defined at-risk pre-T1DM cohort peripheral blood Th40 cells were also significantly increased in number (12). In peripheral blood of CDM subjects Th40 cell numbers were uniformly expanded in number, compared to HC, including patients examined for osteoarthritis, a chronic but non autoimmune disease. Given the difficulty obtaining differentiated lymphocytes, CD4, CD8, B cell, from standard CBC laboratory tests, this finding offers a direct link to measure chronic inflammation in CDM.
At present, the evidence to fully classify CDM as type 1 diabetes is insufficient. The data presented here demonstrate that CDM carries multiple indicators of chronic inflammation. Another finding is that Th40 cells directly associated with human and mouse autoimmune diabetes is significantly elevated in CDM. Human and murine T1D have definitive characteristics, immune cell infiltrations, autoantibody production, elevated numbers of Th40 cells, etc. NOD mice were genetically bred to develop T1D, and NOD mice carry a single MHC-II haplotype, I-Ag7. NOD mice clearly are a homogenous model. While proving highly useful, all the treatments developed from the NOD mouse model have yet to be translated clinically. CDM appears to be more like human T1D, perhaps more like LADA, based on islet architecture and other parameters. Generating biomarkers for chronic inflammation during diabetes is crucial to better understanding the disease. Such understanding is critical for the development of therapeutics to target chronic inflammation and the consequences of diabetes. Further work with this model is needed.
Data availability statement
The original contributions presented in the study are included in the article/Supplementary Material. Further inquiries can be directed to the corresponding author.
Ethics statement
The animal studies were approved by Colorado State University Veterinary Teaching Hospital. The studies were conducted in accordance with the local legislation and institutional requirements. Written informed consent was obtained from the owners for the participation of their animals in this study.
Author contributions
GV: Data curation, Investigation, Methodology, Writing – review & editing. TW: Data curation, Investigation, Methodology, Writing – review & editing. CW: Data curation, Investigation, Methodology, Writing – review & editing. CS: Writing – original draft. SS: Writing – original draft. DW: Investigation, Methodology, Supervision, Writing – review & editing. DHW: Conceptualization, Data curation, Formal analysis, Funding acquisition, Investigation, Resources, Supervision, Writing – original draft.
Funding
The author(s) declare financial support was received for the research, authorship, and/or publication of this article. Op-T, LLC; NIH R01DK131196. Op-T provided funding for the clinical aspects of the study, interaction with CSU Veterinary Hospital. NIH provided funding to the Wagner Lab for some of the experiments. The funder was not involved in the study design, collection, analysis, interpretation of data, the writing of this article, or the decision to submit it for publication.
Acknowledgments
The authors wish to thank Rae Isdale and Dr. Caitlin Erickson for assistance with recruitment, collection, and initial processing of healthy control samples at CSU-VTH. The authors also wish to thank Kris Kofron for assistance with collection of samples from diabetic dogs at CSU-VTH.
Conflict of interest
DHW is co-founder of OP-T, LLC a biotech company. DW is employed by Op-T, LLC.
The remaining authors declare that the research was conducted in the absence of any commercial or financial relationships that could be construed as a potential conflict of interest.
The author(s) declared that they were an editorial board member of Frontiers, at the time of submission. This had no impact on the peer review process and the final decision.
Publisher’s note
All claims expressed in this article are solely those of the authors and do not necessarily represent those of their affiliated organizations, or those of the publisher, the editors and the reviewers. Any product that may be evaluated in this article, or claim that may be made by its manufacturer, is not guaranteed or endorsed by the publisher.
Supplementary material
The Supplementary Material for this article can be found online at: https://www.frontiersin.org/articles/10.3389/fimmu.2023.1319947/full#supplementary-material
References
1. Catchpole B, Ristic JM, Fleeman LM, Davison LJ. Canine diabetes mellitus: can old dogs teach us new tricks? Diabetologia (2005) 48(10):1948–56. doi: 10.1007/s00125-005-1921-1
2. Moshref M, Tangey B, Gilor C, Papas KK, Williamson P, Loomba-Albrecht L, et al. Concise review: canine diabetes mellitus as a translational model for innovative regenerative medicine approaches. Stem Cells Transl Med (2019) 8(5):450–5. doi: 10.1002/sctm.18-0163
3. O’Kell AL, Wasserfall C, Catchpole B, Davison LJ, Hess RS, Kushner JA, et al. Comparative pathogenesis of autoimmune diabetes in humans, NOD mice, and canines: has a valuable animal model of type 1 diabetes been overlooked? Diabetes (2017) 66(6):1443–52. doi: 10.2337/db16-1551
4. Fall T, Hamlin HH, Hedhammar A, Kampe O, Egenvall A. Diabetes mellitus in a population of 180,000 insured dogs: incidence, survival, and breed distribution. J Vet Intern Med (2007) 21(6):1209–16. doi: 10.1111/j.1939-1676.2007.tb01940.x
5. Syed FZ. Type 1 diabetes mellitus. Ann Intern Med (2022) 175(3):ITC33–48. doi: 10.7326/AITC202203150
6. Ahlgren KM, Fall T, Landegren N, Grimelius L, von Euler H, Sundberg K, et al. Lack of evidence for a role of islet autoimmunity in the aetiology of canine diabetes mellitus. PloS One (2014) 9(8):e105473. doi: 10.1371/journal.pone.0105473
7. Niaz K, Maqbool F, Khan F, Hassan FI, Momtaz S, Abdollahi M. Comparative occurrence of diabetes in canine, feline, and few wild animals and their association with pancreatic diseases and ketoacidosis with therapeutic approach. Vet World (2018) 11(4):410–22. doi: 10.14202/vetworld.2018.410-422
8. Kim JH, Furrow E, Ritt MG, Utz PJ, Robinson WH, Yu L, et al. Anti-insulin immune responses are detectable in dogs with spontaneous diabetes. PloS One (2016) 11(3):e0152397. doi: 10.1371/journal.pone.0152397
9. O’Kell AL, Shome M, Qiu J, Williams S, Chung Y, LaBaer J, et al. Exploration of autoantibody responses in canine diabetes using protein arrays. Sci Rep (2022) 12(1):2490.
10. Wagner DH Jr. Overlooked mechanisms in type 1 diabetes etiology: how unique costimulatory molecules contribute to diabetogenesis. Front Endocrinol (Lausanne) (2017) 8:208. doi: 10.3389/fendo.2017.00208
11. Vaitaitis G, Waid DM, Wagner DH Jr. The expanding role of TNF-receptor super family member CD40 (tnfrsf5) in autoimmune disease: Focus on Th40 cells. Curr Immunol Rev (2010) 6(2):130–6. doi: 10.2174/157339510791111673
12. Vaitaitis GM, Rihanek M, Alkanani AK, Waid DM, Gottlieb PA, Wagner DH. Type 1 Diabetes TrialNet Study G: Biomarker discovery in pre-Type 1 Diabetes; Th40 cells as a predictive risk factor. J Clin Endocrinol Metab (2019) 104:4127–42. doi: 10.4049/jimmunol.202.Supp.115.4
13. Siebert JC, Inokuma M, Waid DM, Pennock ND, Vaitaitis GM, Disis ML, et al. An analytical workflow for investigating cytokine profiles. Cytometry A (2008) 73(4):289–98. doi: 10.1002/cyto.a.20509
14. Waid DM, Wagner RJ, Putnam A, Vaitaitis GM, Pennock ND, Calverley DC, et al. A unique T cell subset described as CD4loCD40+ T cells (TCD40) in human type 1 diabetes. Clin Immunol (2007) 124(2):138–48. doi: 10.1016/j.clim.2007.05.003
15. Sosenko JM, Skyler JS, DiMeglio LA, Beam CA, Krischer JP, Greenbaum CJ, et al. A new approach for diagnosing type 1 diabetes in autoantibody-positive individuals based on prediction and natural history. Diabetes Care (2015) 38(2):271–6. doi: 10.2337/dc14-1813
16. Waid DM, Vaitaitis GM, Wagner DH Jr. Peripheral CD4loCD40+ auto-aggressive T cell expansion during insulin-dependent diabetes mellitus. Eur J Immunol (2004) 34(5):1488–97. doi: 10.1002/eji.200324703
17. Malerba E, Cattani C, Del Baldo F, Carotenuto G, Corradini S, Golinelli S, et al. Accuracy of a flash glucose monitoring system in dogs with diabetic ketoacidosis. J Vet Intern Med (2020) 34(1):83–91. doi: 10.1111/jvim.15657
18. Eledrisi SM. Abdel-naser, elzouki: management of diabetic ketoasidosis in adults: A narrative review. Saudi J Med Med Sci (2020) 8(3):165–73.
19. Cardoso D, Perucha E. Cholesterol metabolism: a new molecular switch to control inflammation. Clin Sci (Lond) (2021) 135(11):1389–408. doi: 10.1042/CS20201394
20. Ndrepepa G, Holdenrieder S, Xhepa E, Cassese S, Lahu S, Kufner S, et al. Alkaline phosphatase and prognosis in patients with diabetes mellitus and ischemic heart disease. Clin Chim Acta (2022) 533:1–7. doi: 10.1016/j.cca.2022.05.024
21. Chen H, Li J, Wang Q. Associations between bone-alkaline phosphatase and bone mineral density in adults with and without diabetes. Med (Baltimore) (2018) 97(17):e0432. doi: 10.1097/MD.0000000000010432
22. Cohen RM, Holmes YR, Chenier TC, Joiner CH. Discordance between HbA1c and fructosamine: evidence for a glycosylation gap and its relation to diabetic nephropathy. Diabetes Care (2003) 26(1):163–7. doi: 10.2337/diacare.26.1.163
23. Loste A, Marca MC. Diabetes mellitus in dogs: a study of the critical difference for canine serum fructosamine. Vet Rec (1998) 142(20):547–8. doi: 10.1136/vr.142.20.547
24. Cartwright JA, Cobb M, Dunning MD. Pilot study evaluating the monitoring of canine diabetes mellitus in primary care practice. Vet Rec Open (2019) 6(1):e000250. doi: 10.1136/vetreco-2017-000250
25. Kramer PA, Prichard L, Chacko B, Ravi S, Overton ET, Heath SL, et al. Inhibition of the lymphocyte metabolic switch by the oxidative burst of human neutrophils. Clin Sci (Lond) (2015) 129(6):489–504. doi: 10.1042/CS20140852
26. Assinger A, Schrottmaier WC, Salzmann M, Rayes J. Platelets in sepsis: an update on experimental models and clinical data. Front Immunol (2019) 10:1687. doi: 10.3389/fimmu.2019.01687
27. Rawish E, Nording H, Munte T, Langer HF. Platelets as mediators of neuroinflammation and thrombosis. Front Immunol (2020) 11:548631. doi: 10.3389/fimmu.2020.548631
28. Handtke S, Thiele T. Large and small platelets-(When) do they differ? J Thromb Haemost (2020) 18(6):1256–67. doi: 10.1111/jth.14788
29. Fang L, Wang Y, Zhang H, Jiang L, Jin X, Gu Y, et al. The neutrophil-to-lymphocyte ratio is an important indicator correlated to early neurological deterioration in single subcortical infarct patients with diabetes. Front Neurol (2022) 13:940691. doi: 10.3389/fneur.2022.940691
30. Zahorec R. Neutrophil-to-lymphocyte ratio, past, present and future perspectives. Bratisl Lek Listy (2021) 122(7):474–88. doi: 10.4149/BLL_2021_078
31. Chen H, Li M, Liu L, Dang X, Zhu D, Tian G. Monocyte/lymphocyte ratio is related to the severity of coronary artery disease and clinical outcome in patients with non-ST-elevation myocardial infarction. Med (Baltimore) (2019) 98(26):e16267. doi: 10.1097/MD.0000000000016267
32. Tort M, Sevil FC, Sevil H, Becit N. Evaluation of systemic immune-inflammation index in acute deep vein thrombosis: a propensity-matched. J Vasc Surg Venous Lymphat Disord (2023) 11:972–7. doi: 10.1016/j.jvsv.2023.02.008
33. Satis S. New inflammatory marker associated with disease activity in rheumatoid arthritis: the systemic immune-inflammation index. Curr Health Sci J (2021) 47(4):553–7.
34. Vaitaitis GM, Poulin M, Sanderson RJ, Haskins K, Wagner DH Jr. Cutting edge: CD40-induced expression of recombination activating gene (RAG) 1 and RAG2: a mechanism for the generation of autoaggressive T cells in the periphery. J Immunol (2003) 170(7):3455–9. doi: 10.4049/jimmunol.170.7.3455
35. Wagner DH Jr., Vaitaitis G, Sanderson R, Poulin M, Dobbs C, Haskins K. Expression of CD40 identifies a unique pathogenic T cell population in type 1 diabetes. Proc Natl Acad Sci USA (2002) 99(6):3782–7. doi: 10.1073/pnas.052247099
36. Maahs DM, West NA, Lawrence JM, Mayer-Davis EJ. Epidemiology of type 1 diabetes. Endocrinol Metab Clin North Am (2010) 39(3):481–97. doi: 10.1016/j.ecl.2010.05.011
37. Gerdes C, Werner C, Kloos C, Lehmann T, Wolf G, Muller UA, et al. Progression of diabetic complications in subgroups of people with long term diabetes type 1 according to clinical course. Exp Clin Endocrinol Diabetes (2022) 130(2):101–9. doi: 10.1055/a-1192-3761
38. Gepts W, Toussaint D. Spontaneous diabetes in dogs and cats. A pathological study. Diabetologia (1967) 3(2):249–65. doi: 10.1007/BF01222202
39. Michels A, Zhang L, Khadra A, Kushner JA, Redondo MJ, Pietropaolo M. Prediction and prevention of type 1 diabetes: update on success of prediction and struggles at prevention. Pediatr Diabetes (2015) 16(7):465–84. doi: 10.1111/pedi.12299
40. Davison LJ, Weenink SM, Christie MR, Herrtage ME, Catchpole B. Autoantibodies to GAD65 and IA-2 in canine diabetes mellitus. Vet Immunol Immunopathol (2008) 126(1-2):83–90. doi: 10.1016/j.vetimm.2008.06.016
41. Davison LJ, Herrtage ME, Catchpole B. Autoantibodies to recombinant canine proinsulin in canine diabetic patients. Res Vet Sci (2011) 91(1):58–63. doi: 10.1016/j.rvsc.2010.08.007
42. Vaitaitis GM, Olmstead MH, Waid DM, Carter JR, Wagner DH Jr. A CD40-targeted peptide controls and reverses type 1 diabetes in NOD mice. Diabetologia (2014) 57(11):2366–73. doi: 10.1007/s00125-014-3342-5
43. Vaitaitis GM, Carter JR, Waid DM, Olmstead MH, Wagner DH Jr. An alternative role for Foxp3 as an effector T cell regulator controlled through CD40. J Immunol (2013) 191(2):717–25. doi: 10.4049/jimmunol.1300625
44. Vaitaitis GM, Wagner DH Jr. CD40 glycoforms and TNF-receptors 1 and 2 in the formation of CD40 receptor(s) in autoimmunity. Mol Immunol (2010) 47(14):2303–13. doi: 10.1016/j.molimm.2010.05.288
45. Vaitaitis GM, Wagner DH Jr. High distribution of CD40 and TRAF2 in Th40 T cell rafts leads to preferential survival of this auto-aggressive population in autoimmunity. PloS One (2008) 3(4):e2076. doi: 10.1371/journal.pone.0002076
46. Shields EJ, Lam CJ, Cox AR, Rankin MM, Van Winkle TJ, Hess RS, et al. Extreme beta-cell deficiency in pancreata of dogs with canine diabetes. PloS One (2015) 10(6):e0129809. doi: 10.1371/journal.pone.0129809
47. Steiner DJ, Kim A, Miller K, Hara M. Pancreatic islet plasticity: interspecies comparison of islet architecture and composition. Islets (2010) 2(3):135–45. doi: 10.4161/isl.2.3.11815
48. Campbell-Thompson ML, Atkinson MA, Butler AE, Chapman NM, Frisk G, Gianani R, et al. The diagnosis of insulitis in human type 1 diabetes. Diabetologia (2013) 56(11):2541–3. doi: 10.1007/s00125-013-3043-5
49. Pfister K, Rossi GL, Freudiger U, Bigler B. Morphological studies in dogs with chronic pancreatic insufficiency. Virchows Arch A Pathol Anat Histol (1980) 386(1):91–105. doi: 10.1007/BF00432647
50. Jansen A, Homo-Delarche F, Hooijkaas H, Leenen PJ, Dardenne M, Drexhage HA. Immunohistochemical characterization of monocytes-macrophages and dendritic cells involved in the initiation of the insulitis and beta-cell destruction in NOD mice. Diabetes (1994) 43(5):667–75. doi: 10.2337/diab.43.5.667
51. Uno S, Imagawa A, Okita K, Sayama K, Moriwaki M, Iwahashi H, et al. Macrophages and dendritic cells infiltrating islets with or without beta cells produce tumour necrosis factor-alpha in patients with recent-onset type 1 diabetes. Diabetologia (2007) 50(3):596–601. doi: 10.1007/s00125-006-0569-9
52. Willcox A, Richardson SJ, Bone AJ, Foulis AK, Morgan NG. Analysis of islet inflammation in human type 1 diabetes. Clin Exp Immunol (2009) 155(2):173–81. doi: 10.1111/j.1365-2249.2008.03860.x
53. Skoglund C, Appelgren D, Johansson I, Casas R, Ludvigsson J. Increase of neutrophil extracellular traps, mitochondrial DNA and nuclear DNA in newly diagnosed type 1 diabetes children but not in high-risk children. Front Immunol (2021) 12:628564. doi: 10.3389/fimmu.2021.628564
54. Demkow U. Molecular mechanisms of neutrophil extracellular trap (NETs) degradation. Int J Mol Sci (2023) 24(5). doi: 10.3390/ijms24054896
55. Michailidou D, Kuley R, Wang T, Hermanson P, Grayson PC, Cuthbertson D, et al. Neutrophil extracellular trap formation in anti-neutrophil cytoplasmic antibody-associated and large-vessel vasculitis. Clin Immunol (2023) 249:109274. doi: 10.1016/j.clim.2023.109274
56. Teghanemt A, Pulipati P, Misel-Wuchter K, Day K, Yorek MS, Yi R, et al. CD4 expression in effector T cells depends on DNA demethylation over a developmentally established stimulus-responsive element. Nat Commun (2022) 13(1):1477. doi: 10.1038/s41467-022-28914-4
57. Vaitaitis GM, Wagner DH Jr. Galectin-9 controls CD40 signaling through a Tim-3 independent mechanism and redirects the cytokine profile of pathogenic T cells in autoimmunity. PloS One (2012) 7(6):e38708. doi: 10.1371/journal.pone.0038708
58. Kral JB, Schrottmaier WC, Salzmann M, Assinger A. Platelet interaction with innate immune cells. Transfus Med Hemother (2016) 43(2):78–88. doi: 10.1159/000444807
59. Vanichakarn P, Blair P, Wu C, Freedman JE, Chakrabarti S. Neutrophil CD40 enhances platelet-mediated inflammation. Thromb Res (2008) 122(3):346–58. doi: 10.1016/j.thromres.2007.12.019
60. Pamuk GE, Vural O, Turgut B, Demir M, Pamuk ON, Cakir N. Increased platelet activation markers in rheumatoid arthritis: are they related with subclinical atherosclerosis? Platelets (2008) 19(2):146–54. doi: 10.1080/09537100701210057
61. Khan T, Nawal CL, Meena PD, Singh A. Study neutrophil to lymphocyte ratio and platelet to lymphocyte ratio in patient with rheumatoid arthritis. J Assoc Physicians India (2022) 70(4):11–2.
62. Erge E, Kiziltunc C, Balci SB, Atak Tel BM, Bilgin S, Duman TT, et al. A novel inflammatory marker for the diagnosis of Hashimoto’s thyroiditis: platelet-count-to-lymphocyte-count ratio. Diseases (2023) 11(1). doi: 10.3390/diseases11010015
63. Tozzoli R, Sorrentino MC, Bizzaro N. Detecting multiple autoantibodies to diagnose autoimmune co-morbidity (multiple autoimmune syndromes and overlap syndromes): a challenge for the autoimmunologist. Immunol Res (2013) 56(2-3):425–31. doi: 10.1007/s12026-013-8418-7
64. Sanyal D. Current perspective on auto-antibodies in type 1 diabetes. Indian J Endocrinol Metab (2020) 24(3):233–4. doi: 10.4103/ijem.IJEM_206_20
Keywords: canine diabetes mellitus, autoimmune, chronic inflammation, Th40 cells, inflammatory index
Citation: Vaitaitis G, Webb T, Webb C, Sharkey C, Sharkey S, Waid D and Wagner DH (2024) Canine diabetes mellitus demonstrates multiple markers of chronic inflammation including Th40 cell increases and elevated systemic-immune inflammation index, consistent with autoimmune dysregulation. Front. Immunol. 14:1319947. doi: 10.3389/fimmu.2023.1319947
Received: 23 October 2023; Accepted: 27 December 2023;
Published: 22 January 2024.
Edited by:
Soohyun Kim, Konkuk University, Republic of KoreaReviewed by:
Marcelo Maia Pinheiro, Centro Universitário de Várzea Grande, BrazilJordan Robin Yaron, Arizona State University, United States
Copyright © 2024 Vaitaitis, Webb, Webb, Sharkey, Sharkey, Waid and Wagner. This is an open-access article distributed under the terms of the Creative Commons Attribution License (CC BY). The use, distribution or reproduction in other forums is permitted, provided the original author(s) and the copyright owner(s) are credited and that the original publication in this journal is cited, in accordance with accepted academic practice. No use, distribution or reproduction is permitted which does not comply with these terms.
*Correspondence: David H. Wagner, RGF2aWQuV2FnbmVyQENVQW5zY2h1dHouZWR1