- 1First Clinical School of Medicine, Yunnan University of Chinese Medicine, Kunming, Yunnan, China
- 2College of Basic Medical Sciences, Yunnan University of Chinese Medicine, Kunming, Yunnan, China
- 3Yunnan Provincial Key Laboratory of Integrated Traditional Chinese and Western Medicine for Chronic Disease in Prevention and Treatment, Yunnan University of Chinese Medicine, Kunming, Yunnan, China
Inflammatory bowel disease (IBD) is a complex group of chronic intestinal diseases, the cause of which has not yet been clarified, but it is widely believed that the disorder of the intestinal microenvironment and its related functional changes are key factors in the development of the disease. Houttuynia cordata thunb. is a traditional plant with abundant resources and long history of utilization in China, which has attracted widespread attention in recent years due to its potential in the treatment of IBD. However, its development and utilization are limited owing to the aristolochic acid alkaloids contained in it. Therefore, based on the relationship between the intestinal microenvironment and IBD, this article summarizes the potential mechanisms by which the main active ingredients of Houttuynia cordata thunb., such as volatile oils, polysaccharides, and flavonoids, and related traditional Chinese medicine preparations, such as Xiezhuo Jiedu Formula, alleviate IBD by regulating the intestinal microenvironment. At the same time, combined with current reports, the medicinal and edible safety of Houttuynia cordata thunb. is explained for providing ideas for further research and development of Houttuynia chordate thunb. in IBD disease, more treatment options for IBD patients, and more insights into the therapeutic potential of plants with homology of medicine and food in intestinal diseases, and even more diseases.
1 Introduction
Inflammatory bowel disease (IBD) is an autoimmune and gut-related inflammatory disease mainly encompassing Crohn’s disease (CD) and Ulcerative colitis (UC), with chronic relapsing and remitting characteristics, characterized by inflammatory damage to the intestinal mucosa as the main pathological feature (1). Surveys by Ng SC (2) and Porter RJ (3) indicate that IBD’s prevalence is predominantly in developed regions like North America and Europe, with incidence rates exceeding 0.3%. However, since 2015, areas represented by China and Brazil, have witnessed a rapid surge in IBD cases. This uptrend, fueled by accelerated industrialization and dietary shifts, has resulted in annual growth rates peaking at 14.9%. By 2030, global IBD patient numbers are projected to reach 20.25 million.
The etiology of IBD remains unclear. Current research suggests potential causes, such as disturbances in the intestinal microenvironment, genetics, and environmental factors, all contribute to the onset and progression of IBD (4). Disturbances in the intestinal microenvironment and associated functional changes are regarded as having the closest links with the pathogenesis, recurrence, and drug resistance of this refractory disease (5). Therefore, the primary therapeutic approach for IBD focuses on inducing clinical symptom remission (6). While treatments demonstrate efficacy, nearly 30% of patients require surgical intervention due to uncontrolled disease progression. Coupled with the historically insufficient attention to IBD’s disability rates, its prevention, treatment, and cure rates lag behind other digestive diseases, posing a significant burden to global health. Hence, there is an urgent need for more safe and effective drugs to provide novel therapeutic strategies for IBD (7).
Traditional Chinese medicine classifies IBD under the categories of “diarrhea” and “prolonged dysentery”, and believes that its main causes are the accumulation of pathological products such as phlegm and blood stasis in the intestines, which then lead to the formation of heat toxin and damage to the intestinal vein. Therefore, treatment should focus on promoting blood circulation, removing blood stasis, clearing heat, and eliminating carbuncles. When selecting medications, the “three principles of adaptation” theory of traditional Chinese medicine is often used as a guide. Therefore, based on regional characteristics, it has become an effective way to prevent and treat IBD to find more suitable Chinese herbal medicines for human body constitution from natural plants that are abundant in local growth and have a long history of safe consumption, which can be used to treat IBD.
Houttuynia cordata thunb. (HC), the fresh or dried above-ground part of the plant Jicai from the Saururaceae family, primarily grows and eats in southwest China, including Sichuan, Yunnan, and Guizhou provinces (8). This dual-purpose medicinal and edible plant is recognized for its properties of clearing heat, treating diarrhea, reducing swelling, healing sores (9). According to the theory of meridian tropism, which posits that certain drugs selectively act on specific organs (10), HC mainly targets the lung meridian, emphasizing the modulation of lung function. However, in traditional Chinese medicine theory, the lungs and the large intestine share an intimate “Exterior-interior correlation” relationship (11), coupled with recent advancements in modern medicine studying the “lung-gut axis”, there has been a growing interest in utilizing HC and its active components for IBD treatment, with a focus on rectifying the imbalanced intestinal microenvironment to alleviate IBD. The clinical complete remission rate can reach 95.2%, which is superior to conventional drug intervention groups such as sulfasalazine (12).
2 The relationship between intestinal microbial environment and IBD
The intestinal microenvironment, constituted by a substantial number of microbial populations in specific proportions, plays a crucial role in protecting intestinal mucosa, absorbing and transmitting energy, and metabolizing nutrients. Alterations in this microenvironment can induce pathological reactions in the intestine (13). Recent advancements in research have confirmed that the ecological imbalance in IBD, resulting from changes in the composition and functionality of intestinal microbes, could be the pivotal trigger in the pathogenesis of IBD (14).
2.1 Relationship between intestinal flora and IBD
The gut microbiota represents a dynamic interplay determined collaboratively by the host, microbial self-selection, and environmental factors. This organic consortium is particularly crucial in host immune defense, metabolic nutrient absorption, and the processes of health maintenance and disease alleviation. It has been termed the “forgotten organ” (15) and the “eighth human organ” (16).
The human digestive tract harbors over a trillion commensal microbes, reaching concentrations of 1011 to 1012 cells per gram of luminal content, with over 99% predominantly belonging to the phyla Firmicutes, Bacteroidetes, Actinobacteria, and Proteobacteria (17, 18). The mutual interactions among these bacteria, characterized by resilience and adaptability, maintain a beneficial dynamic equilibrium in the intestinal microenvironment. Simultaneously, they promote the differentiation and maturation of intestinal epithelial cells and immune cells (18), providing a barrier against pathogenic infections, ultimately safeguarding host health (19).
However, when IBD develops, the normal structure and abundance of the intestinal microbiota are disrupted. The quantity of pathogenic bacteria, exemplified by adherent-invasive Escherichia coli, increases (20), while beneficial probiotics such as Bifidobacteria and Lactobacilli decrease (21). The mechanical barrier of the intestinal mucosa and the immune barrier are compromised during the invasion and toxin secretion by pathogenic bacteria, consequently increasing intestinal mucosal permeability, creating conditions for microbial translocation (20). The transferred bacteria and their released endotoxins further induce the expression of additional inflammatory factors, disrupting the normal immune balance in the gut and activating abnormal immune responses within the host (22), which exacerbates intestinal inflammation and mucosal tissue damage in IBD, promoting the progression and prolongation of the condition (as shown in Figure 1).
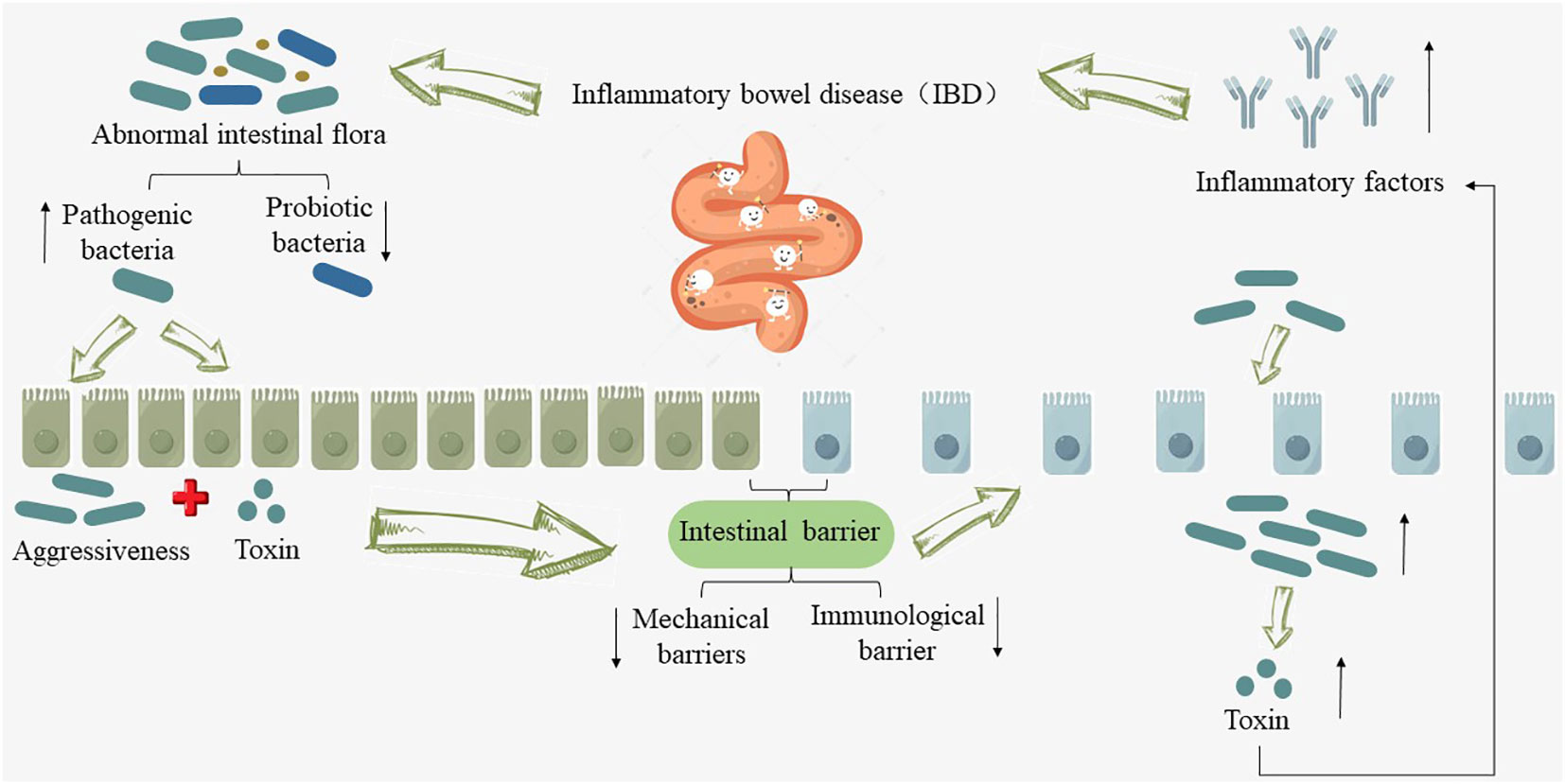
Figure 1 Relationship between intestinal flora and IBD. “↑” indicates an increase, “↓” indicates a decrease.
Herrera-deGuise C et al. (23) conducted a study involving a total of 184 volunteers who provided 111 fecal samples. These samples were collected from individuals in various health conditions, including long-term remission patients, short-term remission patients, patients in acute phase, and healthy control subjects. Following a microbiome analysis, the researchers discovered that compared with healthy control subjects, the intestinal flora abundance and diversity in IBD patients were low, while the intestinal microflora in long-term remission IBD patients had substantial recovery in abundance and diversity, and approached that of healthy individuals. However, when IBD patients underwent fecal microbiota transplantation from healthy donors, their clinical symptoms, such as diarrhea, abdominal pain, rectal bleeding, as well as intestinal mucosal inflammation and Mayo scores, significantly improved compared to the placebo group (24). Furthermore, through metagenomic techniques aimed at identifying differential microbial communities, studies by Javed NH et al. (25) and Zhang B et al. (26) have also confirmed that direct supplementation of probiotics such as Bifidobacteria can also protect the structure of the intestinal mucosal goblet cells and epithelial cell layers, thereby reducing the structural damage of the mucosal barrier, regulating the expression of immune-related cells such as Th17 cells, and thus alleviating the symptoms of IBD. These studies collectively demonstrate the close interplay between the gut microbiota and the development of IBD. Therefore, modulating the abundance and diversity of the gut microbiota to restore the dynamic balance between the gut microbiome and the host immune defense mechanisms is a critical therapeutic approach in the clinical search for methods to inhibit or slow down the occurrence and persistence of IBD (27).
2.2 Relationship between intestinal flora metabolites and IBD
The metabolites of intestinal flora originate from the small molecules produced by the intermediate or final products of intestinal flora metabolism, and are present in serum, urine, feces, and other biological tissues, exerting profound effects on the immune maturation, metabolic homeostasis, and maintenance of intestinal mucosal integrity of the body (28).
With the continuous development of genomics and metabolomics technologies, it has been observed that various gut microbiota metabolic products in individuals with IBD undergo significant alterations when compared to healthy individuals (29). These alterations can amount to over 2700 different metabolites (30). Specifically, disruptions in bile acids (BA), short-chain fatty acids (SCFAs), and tryptophan metabolism have been demonstrated to be closely associated with the occurrence and development of IBD (31). They play a role in the differentiation and functional regulation of immune cells involved in IBD development, such as Treg, Th17, CD4 cells, CD8 cells, B cells, and others (32).
2.2.1 Relationship between BA metabolism disorder and IBD
BA are amphipathic molecules with hydrophobic (β-side) and hydrophilic (α-side) surfaces. They exhibit detergent properties, generated in the liver through cholesterol metabolism and ultimately metabolized by the intestinal microbiota (33). They consist of primary bile acids (PBAs) and secondary bile acids (SBAs), participating in the host’s enterohepatic circulation and microbial metabolic pathways. They facilitate the solubilization of lipids in micelles, promoting the emulsification and absorption of dietary fatty acids and cholesterol (34). Additionally, they regulate intestinal epithelial cell proliferation, intestinal mucosal barrier function, thus contributing to host immune modulation (35).
Over the past decade, the pivotal role of gut microbiota metabolites has been highlighted and investigated in various immune-mediated diseases, particularly in the case of IBD. Long XQ et al. (34) discovered that during the pathogenesis of IBD, BA can directly or indirectly influence the abundance and diversity of the gut microbiota, subsequently modulating the host immune response to ameliorate IBD symptoms or delay disease progression. BA, such as through the activation of FXR and TGR5 expression, impact the anti-α4β7-integrin response in IBD, thereby promoting the restoration of gut microbiota homeostasis (36). This regulation extends to the balance between Th17 and Treg cells (35), ultimately suppressing or alleviating intestinal inflammation in IBD. When there is dysregulation in BA metabolism, it significantly affects the differentiation and renewal of intestinal stem cells, leading to dysbiosis in the gut microbiota. This dysbiosis results in a marked reduction in the abundance of Firmicutes, Clostridia, and an increase in pathogenic bacteria like Escherichia coli, Enterococcus, Klebsiella, and Streptococcus (37). This disruption severely impairs the function of the intestinal mucosal barrier. Additionally, it leads to decreased expression of Th17 cell-related genes, ultimately emerging as a decisive factor in the malignant progression of IBD (35). This has also been confirmed by research by Kubota H et al. (38), who showed that after oral administration of lithocholic acid, a secondary metabolite of intestinal flora, the body weight and disease activity index (DAI) of Dextran Sulfate Sodium (DSS)-induced experimental colitis mice were significantly improved, and the pathological manifestations of colon tissue, such as infiltration of inflammatory cells and loss of goblet cells, were alleviated. The mechanism is mainly that lithocholic acid can participate in maintaining the homeostasis of Treg cells by activating vitamin D receptors in immune cells, thereby inhibiting intestinal inflammatory reactions and alleviating UC (as shown in Figure 2).
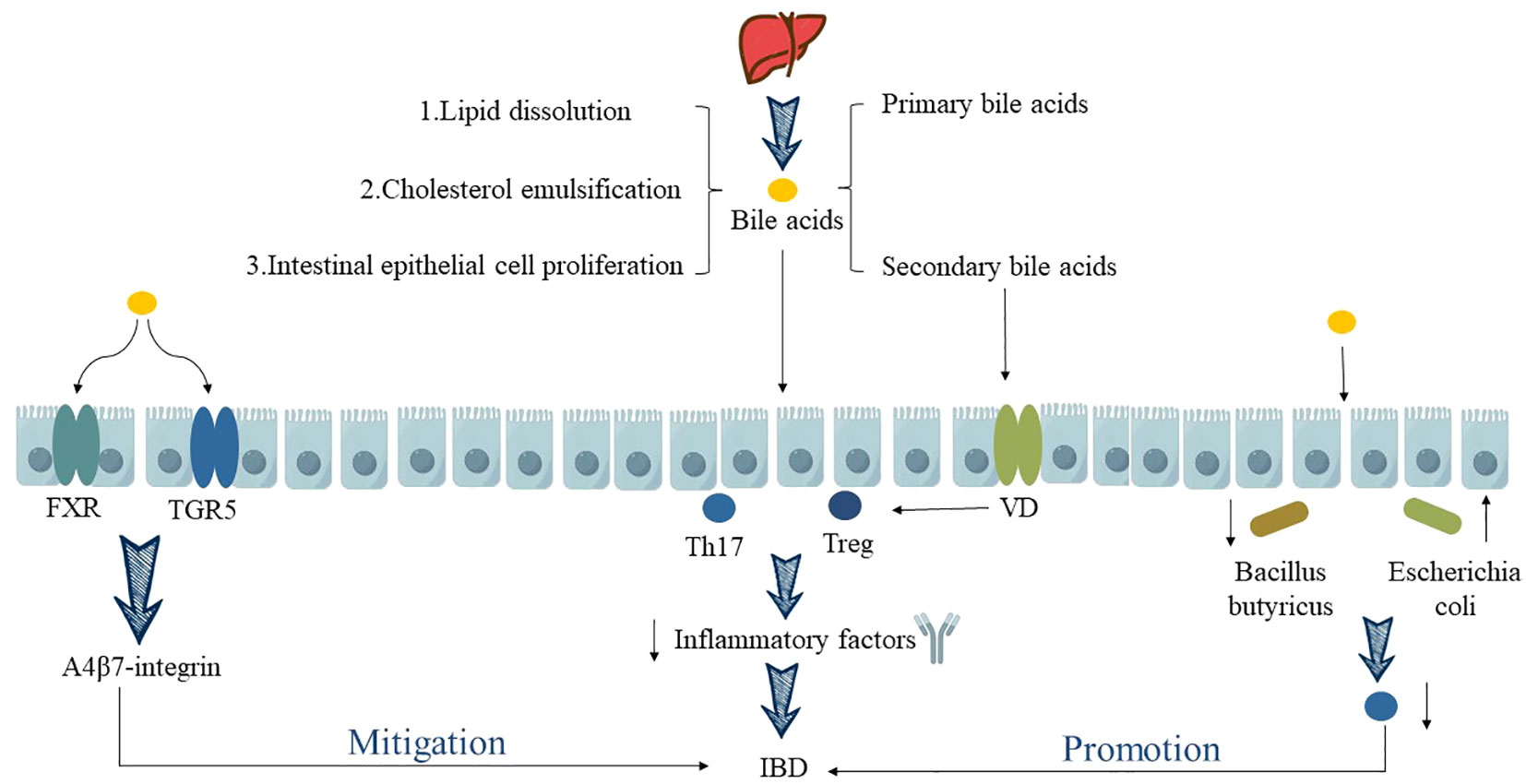
Figure 2 Relationship between BA metabolism disorder and IBD. “↑” indicates an increase, “↓” indicates a decrease.
2.2.2 Relationship between SCFAs metabolism disorder and IBD
SCFAs are a class of fatty acids with fewer than six carbon atoms produced through the fermentation of carbohydrates in the proximal colon and protein hydrolysis in the distal colon by various anaerobic bacteria in the gut. The major components of SCFAs include acetic acid, propanoic acid and butyric acid (39). Among these, butyric acid is primarily produced by Firmicutes, while acetic acid and propanoic acid are generated by Bacteroidetes (40). Research has demonstrated that SCFAs, as the predominant metabolic products of the gut microbiota, can fulfill 60%-70% of the energy requirements of colonocytes. Furthermore, butyric acid, one of the SCFAs, is involved in numerous signaling pathways in intestinal immune cells and epithelial cells. It can regulate the host’s immune homeostasis and enhance intestinal mucosal barrier function. Therefore, butyrate is emerging as a focal point in potential therapeutic strategies for IBD (41).
Macrophages are crucial components of the innate immune system and can be categorized into two main types, M1 and M2 macrophages. They play a pivotal role in shaping the inflammatory milieu in various disease contexts (42). M1 macrophages, induced by factors such as LPS, generate a plethora of pro-inflammatory cytokines which exacerbates tissue inflammation. On the other hand, M2 macrophages, induced by cytokines like IL-4 and IL-13, are characterized by the expression of Arginase-1 (Arg1) and play essential roles in mitigating tissue inflammation, promoting wound healing, and facilitating tissue repair (43). Ji J et al. (44) conducted animal experiments and found that butyric acid intervention in DSS-induced UC mice models enhances the polarization of M2-BMDM macrophages through the augmentation of IL-4-mediated STAT6 transcription and H3K9 acetylation. This process promotes Arg1 synthesis, facilitating the repair of damaged intestinal mucosal barriers and significantly reducing colonic inflammation in mice. In addition, butyrate has also been shown to promote the development of monocytes into macrophages by inhibiting histone deacetylase (HDAC), stimulate macrophages to increase the synthesis of endogenous host defense peptides (HDP), and affect intestinal immunity by maintaining the integrity of the intestinal mucosal barrier, providing epithelial energy supply, and reducing inflammatory expression, thereby alleviating IBD (45) (as shown in Figure 3).
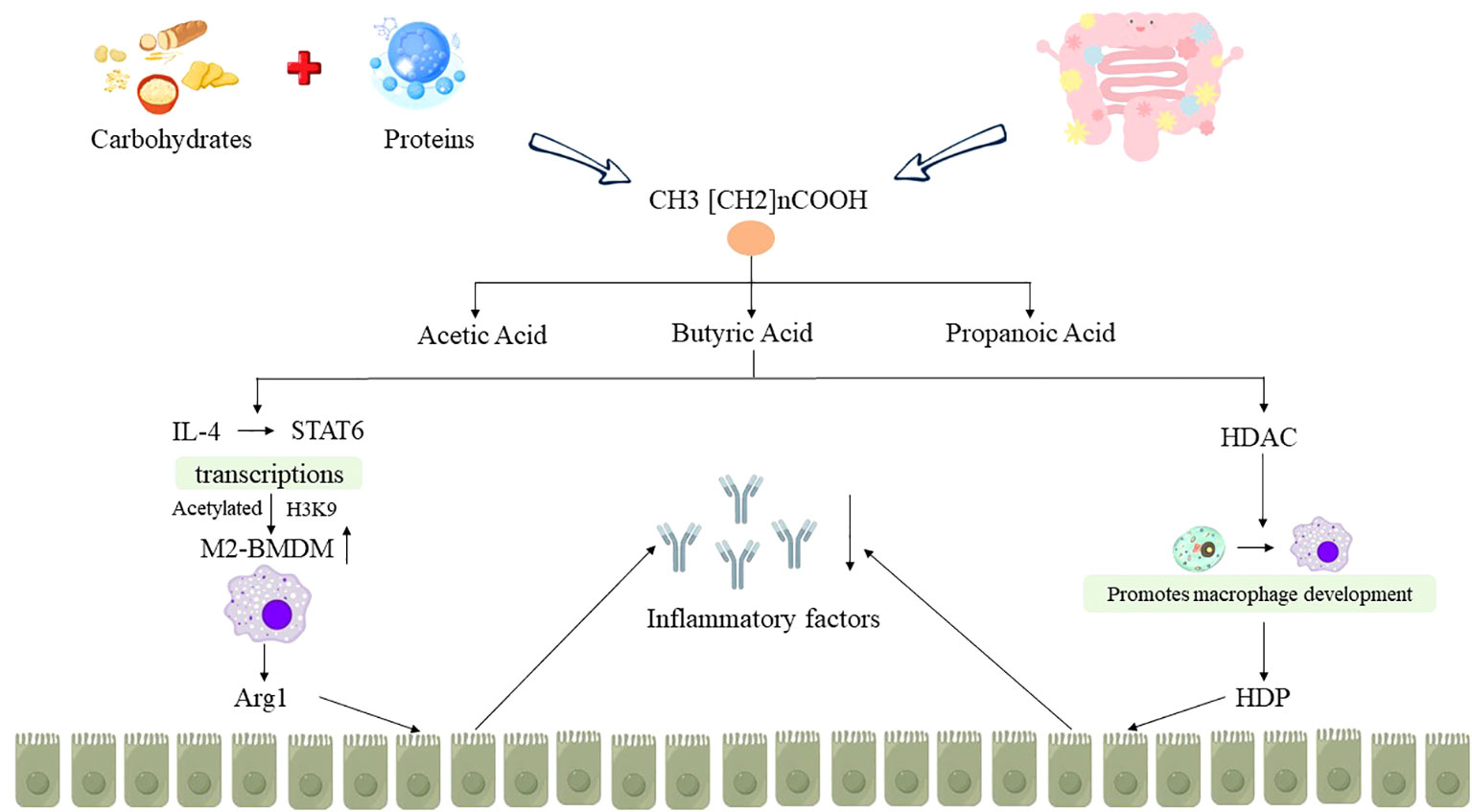
Figure 3 Relationship between SCFAs metabolism disorder and IBD. “↑” indicates an increase, “↓” indicates a decrease.
2.2.3 The relationship between dysregulation of tryptophan metabolism and IBD
Tryptophan is an essential amino acid for humans, which can be supplemented through the intake of lean meat, fish, dairy products, nuts, and seeds. It is produced in the gastrointestinal tract through three main pathways, namely the kynurenine pathway, the 5-hydroxytryptamine pathway, and the indole pathway, which play separate but complementary roles in regulating gastrointestinal function and influencing intestinal homeostasis, thereby interfering with the progression of IBD (46). Notably, research on the indole pathway in IBD has yielded more in-depth insights. It has been observed that the gut microbiota can convert 4% to 6% of intracellular tryptophan into indole and related indolic compounds, which serve as ligands for the aryl hydrocarbon receptor (AhR) and pregnane X receptor (PXR). This ligand-receptor interaction is instrumental in modulating mucosal immunity within the intestinal tract, maintaining intestinal environmental stability, and alleviating the progression of IBD (47).
Yang C (48) and Bischoff SC (49) discovered significant differences in tryptophan metabolism between UC patients and healthy volunteers through a comparative analysis. They found that UC patients had notably lower levels of tryptophan than healthy volunteers. Furthermore, in active UC patients, serum tryptophan levels were negatively correlated with the inflammatory markers ESR and CRP (P < 0.05). A large cohort study involving 535 IBD patients also yielded similar results. This study indicated that the severity of IBD disease activity was inversely related to tryptophan levels (50). Consequently, serum tryptophan levels hold monitoring value in the course of IBD and are of significant importance in IBD research.
To further investigate the mechanisms underlying tryptophan metabolism in IBD, Lamas B et al. (51) conducted relevant experiments and discovered that the potential mechanisms by which tryptophan metabolism ameliorates IBD primarily involve the AhR signaling pathway, the PXR signaling pathway, and the modulation of cytokines.
Firstly, the microbiota-AhR axis has been confirmed as a fundamental element in maintaining intestinal immune homeostasis. Tryptophan metabolites, such as indole-3-acetic acid, are potent bioactive substances influencing adaptive and innate immune responses. Their role in the body’s immune system mainly relies on the activation of the AhR signaling pathway, which is widely present in immune cells and intestinal epithelial cells and plays a regulatory role in intestinal mucosal immunity (52, 53). Therefore, after knockout of the IBD risk allele CARD9, it was observed that the metabolism of tryptophan decreased in mice, and it was difficult to catalyze tryptophan into AhR ligands, resulting in reduced release of IL-22 factors, making mice more susceptible to DSS-induced IBD. However, after inoculating mice with three strains of lactic acid bacteria that contain tryptophan or by intervening with AhR agonists, the intestinal inflammation in mice decreased, and IBD was alleviated (51).
Furthermore, the PXR signaling pathway has also been confirmed as a significant potential mechanism affecting the progression of IBD through its impact on tryptophan metabolism. PXR plays a role in regulating intestinal mucosal barrier function under homeostatic conditions and serves as a critical modulator of innate immune responses in the intestine and their responses to damage. In the absence of PXR, UC mice exhibit evident intestinal mucosal barrier “leakiness”, whereas mice without PXR defects can activate the PXR signaling pathway through tryptophan metabolite indole-3-propionic acid, resulting in reduced intestinal permeability and suppressed inflammatory responses, thereby alleviating the disease (54). Additionally, Ding X et al. (55) discovered that patients with IBD exhibit disruptions in tryptophan metabolism during the pathological process. However, by modulating tryptophan metabolism, it is possible to induce the differentiation of Treg cells through intestinal microbiota breakdown and the kynurenine pathway. This process, involving cell-cell contact and cytokine release, helps maintain the intestinal microenvironment homeostasis in IBD patients and alleviate inflammation damage to intestinal tissues (as shown in Figure 4).
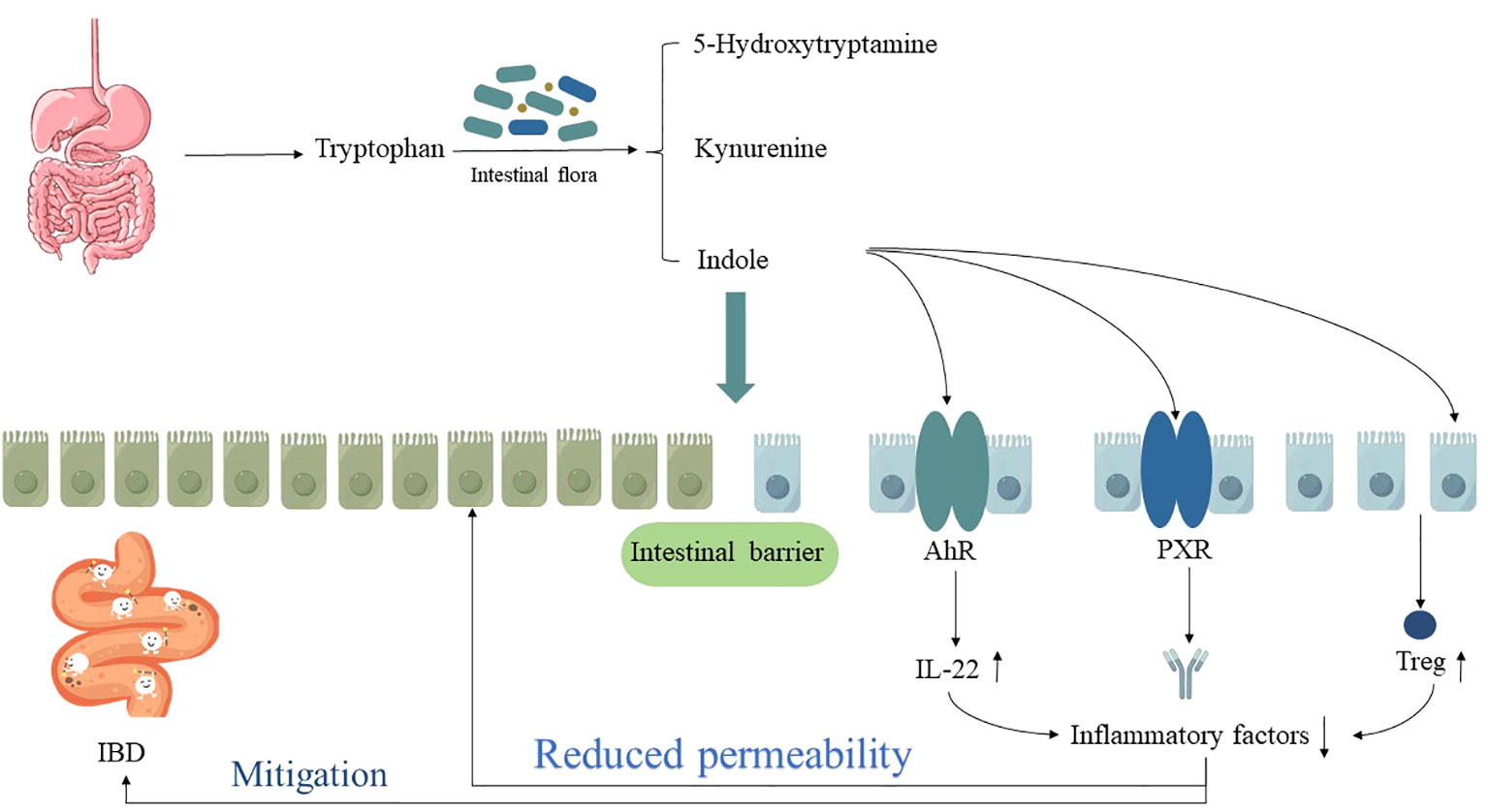
Figure 4 The relationship between dysregulation of tryptophan metabolism and IBD. “↑” indicates an increase, “↓” indicates a decrease.
However, while it has been confirmed that tryptophan metabolism is involved in the pathology and physiological processes of IBD, current research predominantly focuses on exploring the potential of tryptophan as a biomarker and its role in regulating immune and inflammatory responses (56). The specific mechanisms of tryptophan metabolism in the pathogenesis of IBD remain unclear. Additionally, further investigation is needed in the kynurenine pathway and the 5-hydroxytryptamine pathway.
3 The potential mechanism of HC in alleviating IBD through regulating the intestinal microenvironment
HC, a perennial herbaceous plant commonly associated with root nodules bacteria, derives its name from its distinctive fish-like odor permeating the entire plant. Typically thriving in wetlands, stream margins, and meadows (57), this plant usually exhibits a height ranging from 20 to 50 cm. Its leaves, either green or red, are oval or heart-shaped, measuring between 4 to 8 cm in length and 3 to 6 cm in width. The plant bears densely packed spicate flowers, with its slender rhizomes presenting nodes that are either glabrous or covered in short soft hairs (58).
HC is widely distributed in Asian regions, especially in Yunnan, Hunan, Hubei, Sichuan, and Guizhou provinces of China. It is primarily used as a food ingredient due to its richness in vitamins and minerals (59). With the enrichment of pharmacological research methods in recent years, the preparations containing HC, as well as the main chemical components and medicinal effects of HC, have been continuously explored. Among them, essential oils, flavonoids, alkaloids, and polysaccharides are the main chemical components with pharmacological activity in HC, and have been confirmed to have immune regulatory activity, anti-inflammatory, antiviral, antioxidant, and tumor cell proliferation inhibitory effects (60). They have therapeutic effects in diseases such as liver cancer (61), acute liver injury (62), avascular necrosis of the femoral head (61), asthma (63), pneumonia (64), alzheimer’s disease (65), and heart failure (66). Consequently, the plant has been recognized by the Chinese National Health Department as one of the most promising resources for both medicinal and culinary uses (67).
3.1 Essential oils
HC, often referred to as the “Chinese herbal antibiotic”, has been proven through research to possess anti-inflammatory, antioxidant, and antiviral properties. These effects have been attributed to its essential oils content. Furthermore, the essential oils constituents serve as the primary active components responsible for various pharmacological effects of the whole fish mint plant. These constituents include houttuynin, decanal, trans-caryophyllene, decanoic acid, camphene, β-pinene, lauraldehyde, α-pinene, limonene, nonanol, linalool bornyl acetate, methyl n-nonyl ketones, beta myrcene, monoterpene, 4-terpineol, caryophyllene oxide, phenylpropene derivatives, sesquiterpenes, and oxidized diterpenes (59).
Candida albicans, a common opportunistic pathogen found in the human intestinal tract, has been associated with the severity of UC. Cheng T et al. (68) found that the aldehyde and sodium bisulfite adduct of HC essential oils, sodium houttuyfonate (SH), can regulate the abundance and diversity of gut microbial flora by inhibiting harmful pathogens such as Leiberella and Bacteroides, and increasing beneficial bacteria, including bacteria producing SCFAs (Lachnospiraceae_NK4A136_group, Intestinimonas) and probiotics (Lactobacillus and Alloprevotella), thereby maintaining the stability of the intestinal microenvironment. It significantly improved the pathological signs of colon shortening and intestinal mucosal barrier injury in IBD mice infected with Candida albicans, significantly inhibiting the growth of Candida albicans. The mechanism of SH improving IBD was further confirmed in UC caused by Salmonella typhimurium, and was closely related to the inhibition of NF-κB signaling pathway (69) (as shown in Table 1).
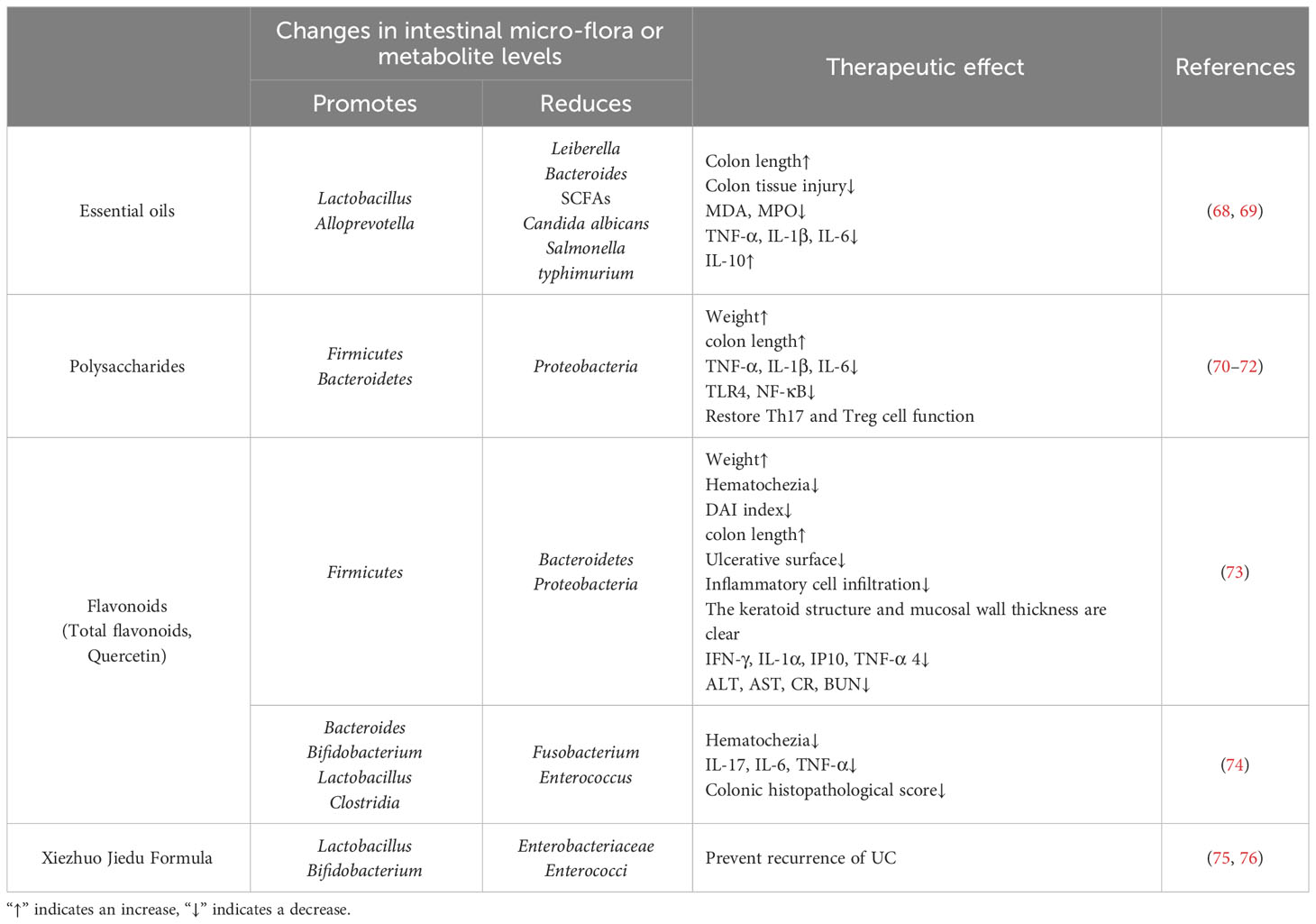
Table 1 The potential mechanism of HC in alleviating IBD through regulating the intestinal microenvironment.
3.2 Polysaccharides
Plant polysaccharides are natural macromolecular compounds derived from plants. Studies have shown that plant polysaccharides not only promote the proliferation of beneficial bacteria in the gut, but also produce organic acids through fermentation in the gut, thereby reducing the pH of the gut and making it difficult for harmful bacteria in the gut to effectively utilize plant polysaccharides, thus exerting an inhibitory effect on the growth and reproduction of pathogenic bacteria (77), restoring the diversity of gut flora, regulating the structure of gut flora, and repairing the mucosal barrier to protect the gut (78).
With the increasing attention of researchers to dual-purpose natural plants for medicine and food, as well as the diversification of extraction methods, the polysaccharides in HC have been identified as crucial pharmacologically active components. These include rhamnose, galacturonic acid, galactose, and arabinose, which possess notable immunoregulation, intestinal protection, anti-inflammatory, and antioxidant activities, demonstrating increasing advantages in the treatment of refractory diseases (79, 80).
Cen L et al. (70) established an animal model of IBD using DSS induction and then intervened with HC polysaccharides. The results showed that HC polysaccharides can regulate intestinal flora, such as increasing the abundance of Firmicutes and Bacteroidetes, reducing the abundance of Proteobacteria, improving the intestinal microenvironment, and then intervening in the expression of inflammatory signaling pathways such as NF-κB, inhibiting macrophage infiltration (71), alleviating pathological damage to the colonic mucosal mechanical barrier, thereby regulating the function of Th17 and Treg cells, protecting colonic tissue, and significantly inhibiting general signs such as weight loss and shortening of the colon length in mice. After successfully replicating the UC model, Ping W et al. (72) administered intragastric intervention with HC polysaccharides solution to mice, and the results also confirmed that HC polysaccharides can increase the species richness of intestinal bacteria in UC mice, promote the recovery of intestinal flora abundance and structure, reduce the relative abundance of Proteobacteria, inhibit the expression of pro-inflammatory cytokines in the intestine, significantly increase the level of Firmicutes, restore the microecological homeostasis in the intestine, and repair the intestinal mucosal barrier, thereby improving UC (as shown in Table 1).
3.3 Flavonoids
Flavonoids in HC, similar to the essential oils found within the plant, have been proven to possess significant anti-inflammatory and antiviral activities, representing another major active ingredient underlined in relevant studies (81). These flavonoids include quercetin, rutin, hyperin, afzelin, quercitrin, isoquercitrin, kaempferol, quercetin hexoside, avicularin, apigenin, isorhamnetin, phloridzin, and quercetin-3-O-β-D-galactoside-7-O-β-D-glucoside (82).
Letian Y et al. (73) used a 3.5% DSS solution to continuously drink water to replicate the model of UC in mice, and then used cellulase-assisted ultrasonic extraction to extract total flavonoids from HC for intervention. The results showed that total flavonoids in HC could maintain the stability of intestinal microecology by regulating the proportion of dominant bacterial groups such as Bacteroidetes, Firmicutes, and Proteobacteria to varying degrees, thereby effectively improving the body weight of UC mice, reducing hematochezia, reducing DAI index, alleviating symptoms such as shortened colon, inhibiting the expression of serum inflammatory factors, and showing a dose-dependent effect (as shown in Table 1).
In addition, quercetin in HC, a flavonoid compound widely distributed in the plant kingdom, has also been shown to play a therapeutic role in the treatment of IBD. For example, Lin R et al. (74) showed that after quercetin intervention in a Citrobacter rodentium-induced IBD mice model, intestinal microbial diversity was reshaped, and the balance between bacterial populations was regulated. The number of Bacteroides, Bifidobacterium, Lactobacillus, and Clostridia increased, while Fusobacterium and Enterococcus decreased significantly, thereby promoting the restoration of local immune homeostasis in the intestine and significantly alleviating IBD symptoms such as hematochezia (as shown in Table 1).
3.4 HC preparation alleviates IBD by regulating the intestinal microenvironment
The Xiezhuo Jiedu Formula (XZJDF) is a herbal preparation based on HC used to treat UC (75). Siyu L et al. (76) found that after intragastric administration of the XZJDF in high, medium, and low doses to UC rats induced by a mixture of 2,4,6-trinitrobenzene sulfonic acid (TNBS) and 50% ethanol, the relative expression levels of Lactobacillus and Bifidobacterium in the high dose group were higher than those in the model group, while the relative expression levels of Enterobacteriaceae and Enterococci were lower than those in the model group. Furthermore, during the second modeling stimulation, the high dose group of the XZJDF showed a lasting effect on regulating the balance and stability of intestinal flora, further improving the defensive capacity of the intestinal mucosal barrier, and significantly improving the condition of UC rats. Therefore, it can be concluded that the HC preparation XZJDF can target intestinal flora, increase the relative content of Lactobacillus and Bifidobacterium, reduce the relative content of Enterobacteriaceae and Enterococci, make the beneficial bacteria dominate over the harmful bacteria, regulate the disorder of intestinal flora, and maintain the long-term stability of the intestinal microenvironment, thus maintaining the health of the body and delaying the recurrence of UC (as shown in Table 1).
In summary, the potential mechanism of HC in alleviating IBD is mainly that the active ingredients in HC and related preparations can regulate the intestinal microenvironment, including regulating intestinal flora and its metabolites, thereby improving the body’s immunity to alleviate IBD (as shown in Figure 5). This effect has been verified not only in animal experiments, but also in clinical human drug evaluation.
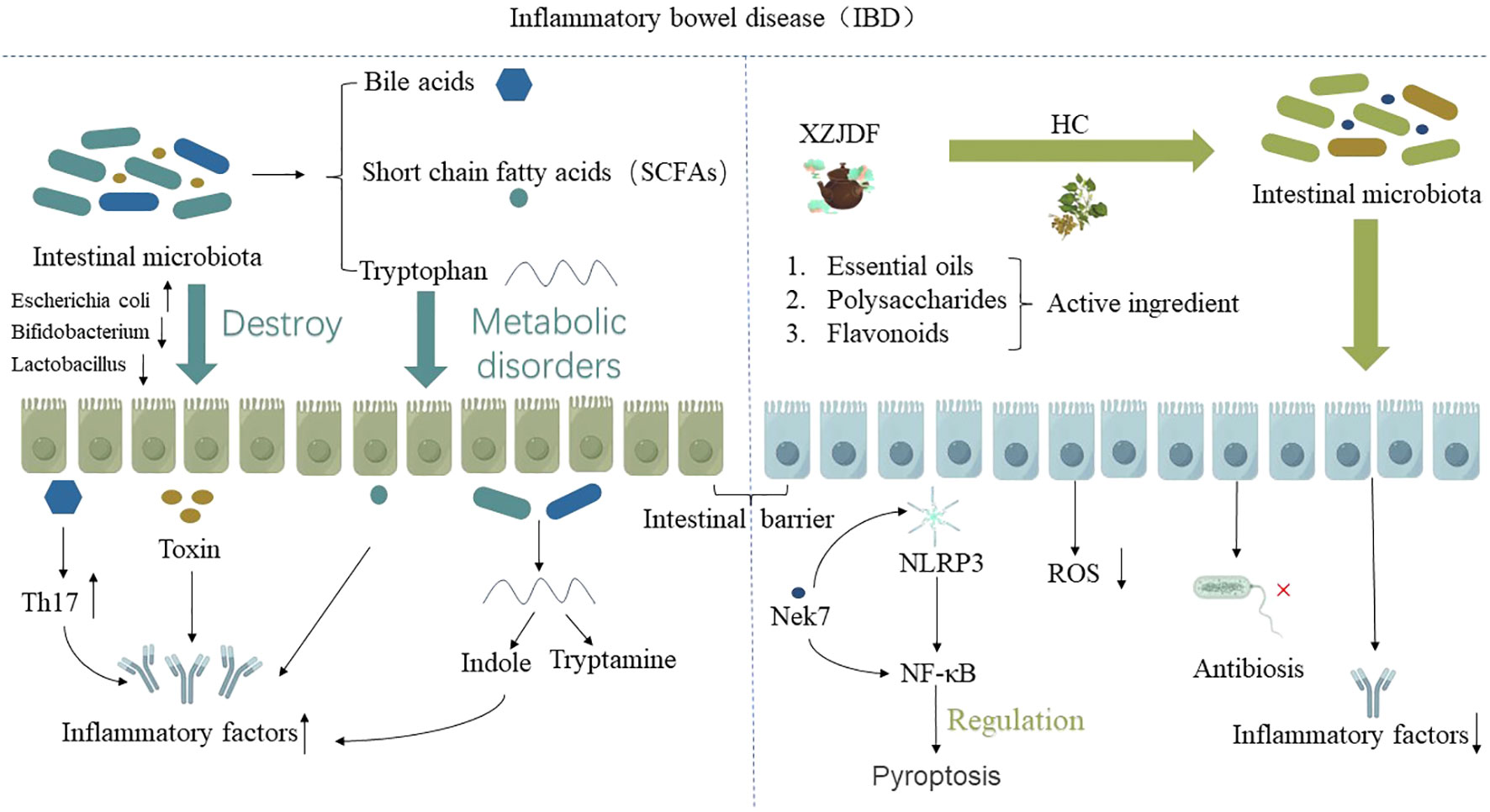
Figure 5 The potential mechanism of HC in alleviating IBD is mainly due to the active ingredients in HC, such as essential oils, polysaccharides, flavonoids, and HC-related preparations, which regulate the intestinal microenvironment, including regulating intestinal flora and its metabolites, thereby improving the body’s immunity to alleviate IBD.”↑” indicates an increase, “↓” indicates a decrease.
However, due to the presence of aristolochic acid lactam alkaloids (83), which have been confirmed to be nephrotoxic and carcinogenic, in HC, the development, research, and utilization of HC in IBD have been limited, and there is a lack of relevant literature reports. However, current research presents divergent opinions, suggesting that the nephrotoxic and carcinogenic nature of Aristolochic acid alkaloids primarily pertains to Aristolochic acid I, originating from plants within the Aristolochiaceae family such as Manshurian Dutchmanspipe Stem and Dutchmanspipe Vine. In contrast, HC belongs to the Saururaceae family, and its contained alkaloids are of the Aristolochic acid II type, including BII, AII, and FII (84–86). Their content in the total alkaloids of HC is only 0.016 g/kg, thus posing minimal risk of kidney damage and carcinogenicity (87). A cross-sectional study conducted in China to investigate the dietary habits of HC among various regional populations has affirmed this observation. Among the 3,561 volunteers included in the study, no significant correlation was found between the consumption of HC and kidney diseases or malignancies of the urinary system (R=0.988). Even after controlling for confounding factors such as age and region, the results remained negative (88). Similar animal toxicology experiments have yielded consistent findings (89).
Therefore, we should further develop the research and development of active ingredients and preparations related to HC to alleviate IBD. It is not only rich in resources and easy to obtain, but also contains many bioactive substances. In addition to the above-mentioned components, there are also chlorogenic acid, neochlorogenic acid, cryptochlorogenic acid, caffeic acid, succinic acid, β-sitosterol-4-en-3-one, β-sitosterol-3,6-dione, and daucosterol, which have great potential for development and utilization (90).
4 Summary and prospect
HC, a naturally occurring medicinal plant with dual therapeutic and dietary properties, has been substantiated through research as a beneficial supplement for the treatment of IBD. In this article, we discuss the potential mechanism of HC in the treatment of IBD based on its main active ingredients and related preparations, and emphasize its role in alleviating IBD by regulating the intestinal microenvironment and improving the body’s immunity. Although IBD is a complex disease, combined with literature reports, the active ingredients in HC and related traditional Chinese medicine preparations may regulate the intestinal microenvironment to alleviate IBD, providing a promising treatment approach for patients to alleviate the symptoms of IBD.
However, it is essential to acknowledge that current research is still in its preliminary stages and presents several unresolved mysteries and challenges. To gain a better understanding of the mechanism of action of HC, further investigations are required, including laboratory researches, clinical trials, identification of bioactive components, and optimization studies of treatment dosages and durations. Furthermore, comprehensive assessments of drug interactions and safety are also necessary to better serve patients, enhance their quality of life, and offer more avenues and options for the treatment of IBD.
Author contributions
SW: Conceptualization, Writing – original draft, Writing – review & editing. LL: Validation, Writing – original draft, Writing – review & editing. YC: Visualization, Writing – review & editing. QL: Data curation, Writing – review & editing. SZ: Visualization, Writing – review & editing. NL: Methodology, Visualization, Writing – review & editing. YW: Supervision, Writing – review & editing. JY: Funding acquisition, Supervision, Writing – review & editing.
Funding
The author(s) declare financial support was received for the research, authorship, and/or publication of this article. This study is supported by the Yunnan Provincial Major Science and Technology Special Project: Green Food Development of Regulating Intestinal Flora and Its Metabolites (202102AE090031).
Conflict of interest
The authors declare that the research was conducted in the absence of any commercial or financial relationships that could be construed as a potential conflict of interest.
Publisher’s note
All claims expressed in this article are solely those of the authors and do not necessarily represent those of their affiliated organizations, or those of the publisher, the editors and the reviewers. Any product that may be evaluated in this article, or claim that may be made by its manufacturer, is not guaranteed or endorsed by the publisher.
References
1. Zhou F, Zhang GD, Tan Y, Hu SA, Tang Q, Pei G. NOD-like receptors mediate homeostatic intestinal epithelial barrier function: promising therapeutic targets for inflammatory bowel disease. Therap Adv Gastroenterol (2023) 16:17562848231176889. doi: 10.1177/17562848231176889
2. Ng SC, Shi HY, Hamidi N, Underwood FE, Tang W, Benchimol EI, et al. Worldwide incidence and prevalence of inflammatory bowel disease in the 21st century: a systematic review of population-based studies. Lancet (2017) 390(10114):2769–78. doi: 10.1016/S0140-6736(17)32448-0
3. Porter RJ, Kalla R, Ho GT. Ulcerative colitis: Recent advances in the understanding of disease pathogenesis. F1000Res (2020) 9:1–13. doi: 10.12688/f1000research.20805.1
4. Wang Y, Li Q, Zhang J, Liu P, Zheng H, Chen L, et al. Ring1a protects against colitis through regulating mucosal immune system and colonic microbial ecology. Gut Microbes (2023) 15(2):2251646. doi: 10.1080/19490976.2023.2251646
5. Yan PG, Li JN. Advances in the understanding of the intestinal micro-environment and inflammatory bowel disease. Chin Med J (Engl) (2020) 133(7):834–41. doi: 10.1097/CM9.0000000000000718
6. Le Berre C, Honap S, Peyrin-Biroulet L. Ulcerative colitis. Lancet (2023) 402(10401):571–84. doi: 10.1016/S0140-6736(23)00966-2
7. Zhong G, He C, Wang S, Lin C, Li M. Research progress on the mechanism of cholesterol-25-hydroxylase in intestinal immunity. Front Immunol (2023) 14:1241262. doi: 10.3389/fimmu.2023.1241262
8. Shingnaisui K, Dey T, Manna P, Kalita J. Therapeutic potentials of Houttuynia cordata Thunb. against inflammation and oxidative stress: A review. J Ethnopharmacol (2018) 220:35–43. doi: 10.1016/j.jep.2018.03.038
9. Xu Z, Wang C, Bao W, Weng H, Chen D, Lu Y. In vitro nephrotoxicity and quantitative UPLC-MS analysis of three aristololactams in Houttuynia cordata. J Pharm BioMed Anal (2023) 227:115289. doi: 10.1016/j.jpba.2023.115289
10. Ho TY, Lo HY, Lu GL, Liao PY, Hsiang CY. Analysis of target organs of Houttuynia cordata: A study on the anti-inflammatory effect of upper respiratory system. J Ethnopharmacol (2023) 315:116687. doi: 10.1016/j.jep.2023.116687
11. Chen Y, Zhou D, Feng Y, Li B, Cui Y, Chen G, et al. Association of sirtuins (SIRT1-7) with lung and intestinal diseases. Mol Cell Biochem (2022) 477(11):2539–52. doi: 10.1007/s11010-022-04462-9
12. Jiang XL, Cui HF. Different therapy for different types of ulcerative colitis in China. World J Gastroenterol (2004) 10(10):1513–20. doi: 10.3748/wjg.v10.i10.1513
13. Zhang H, Sun L. When human cells meet bacteria: precision medicine for cancers using the microbiota. Am J Cancer Res (2018) 8(7):1157–75. doi: 10.1158/1538-7445.AM2018-1157
14. Haneishi Y, Furuya Y, Hasegawa M, Picarelli A, Rossi M, Miyamoto J. Inflammatory bowel diseases and gut microbiota. Int J Mol Sci (2023) 24(4):746998. doi: 10.3390/ijms24043817
15. Dalal SR, Chang EB. The microbial basis of inflammatory bowel diseases. J Clin Invest (2014) 124(10):4190–6. doi: 10.1172/JCI72330
16. Su M, Tang T, Tang W, Long Y, Wang L, Liu M. Astragalus improves intestinal barrier function and immunity by acting on intestinal microbiota to treat T2DM: a research review. Front Immunol (2023) 14:1243834. doi: 10.3389/fimmu.2023.1243834
17. Nishida A, Nishino K, Sakai K, Owaki Y, Noda Y, Imaeda H. Can control of gut microbiota be a future therapeutic option for inflammatory bowel disease? World J Gastroenterol (2021) 27(23):3317–26. doi: 10.3748/wjg.v27.i23.3317
18. Sommer F, Anderson JM, Bharti R, Raes J, Rosenstiel P. The resilience of the intestinal microbiota influences health and disease. Nat Rev Microbiol (2017) 15(10):630–8. doi: 10.1038/nrmicro.2017.58
19. Nishida A, Inoue R, Inatomi O, Bamba S, Naito Y, Andoh A. Gut microbiota in the pathogenesis of inflammatory bowel disease. Clin J Gastroenterol (2018) 11(1):1–10. doi: 10.1007/s12328-017-0813-5
20. Pickard JM, Zeng MY, Caruso R, Nunez G. Gut microbiota: Role in pathogen colonization, immune responses, and inflammatory disease. Immunol Rev (2017) 279(1):70–89. doi: 10.1111/imr.12567
21. Sanders ME, Merenstein DJ, Reid G, Gibson GR, Rastall RA. Probiotics and prebiotics in intestinal health and disease: from biology to the clinic. Nat Rev Gastroenterol Hepatol (2019) 16(10):605–16. doi: 10.1038/s41575-019-0173-3
22. Glassner KL, Abraham BP, Quigley E. The microbiome and inflammatory bowel disease. J Allergy Clin Immunol (2020) 145(1):16–27. doi: 10.1016/j.jaci.2019.11.003
23. Herrera-deGuise C, Varela E, Sarrabayrouse G, Pozuelo DRM, Alonso VR, Sainz NB, et al. Gut microbiota composition in long-remission ulcerative colitis is close to a healthy gut microbiota. Inflammation Bowel Dis (2023) 29(9):1362–9. doi: 10.1093/ibd/izad058
24. Shang S, Zhu J, Liu X, Wang W, Dai T, Wang L, et al. The impacts of fecal microbiota transplantation from same sex on the symptoms of ulcerative colitis patients. Pol J Microbiol (2023) 72(3):247–68. doi: 10.33073/pjm-2023-025
25. Javed NH, Alsahly MB, Khubchandani J. Oral feeding of probiotic bifidobacterium infantis: colonic morphological changes in rat model of TNBS-induced colitis. Scientifica (Cairo) (2016) 2016:9572596. doi: 10.1155/2016/9572596
26. Zhang B, Liu Y, Lan X, Xu X, Zhang X, Li X, et al. Oral Escherichia coli expressing IL-35 meliorates experimental colitis in mice. J Transl Med (2018) 16(1):71. doi: 10.1186/s12967-018-1441-7
27. Roy S, Dhaneshwar S. Role of prebiotics, probiotics, and synbiotics in management of inflammatory bowel disease: Current perspectives. World J Gastroenterol (2023) 29(14):2078–100. doi: 10.3748/wjg.v29.i14.2078
28. Lavelle A, Sokol H. Gut microbiota-derived metabolites as key actors in inflammatory bowel disease. Nat Rev Gastroenterol Hepatol (2020) 17(4):223–37. doi: 10.1038/s41575-019-0258-z
29. Suh JH, Degagne E, Gleghorn EE, Setty M, Rodriguez A, Park KT, et al. Sphingosine-1-phosphate signaling and metabolism gene signature in pediatric inflammatory bowel disease: A matched-case control pilot study. Inflammation Bowel Dis (2018) 24(6):1321–34. doi: 10.1093/ibd/izy007
30. Wlodarska M, Luo C, Kolde R, D'Hennezel E, Annand JW, Heim CE, et al. Indoleacrylic acid produced by commensal peptostreptococcus species suppresses inflammation. Cell Host Microbe (2017) 22(1):25–37.e6. doi: 10.1016/j.chom.2017.06.007
31. Yan Y, Lei Y, Qu Y, Fan Z, Zhang T, Xu Y, et al. Bacteroides uniformis-induced perturbations in colonic microbiota and bile acid levels inhibit TH17 differentiation and ameliorate colitis developments. NPJ Biofilms Microbiomes (2023) 9(1):56. doi: 10.1038/s41522-023-00420-5
32. Su X, Gao Y, Yang R. Gut microbiota derived bile acid metabolites maintain the homeostasis of gut and systemic immunity. Front Immunol (2023) 14:1127743. doi: 10.3389/fimmu.2023.1127743
33. Wahlstrom A, Sayin SI, Marschall HU, Backhed F. Intestinal crosstalk between bile acids and microbiota and its impact on host metabolism. Cell Metab (2016) 24(1):41–50. doi: 10.1016/j.cmet.2016.05.005
34. Long XQ, Liu MZ, Liu ZH, Xia LZ, Lu SP, Xu XP, et al. Bile acids and their receptors: Potential therapeutic targets in inflammatory bowel disease. World J Gastroenterol (2023) 29(27):4252–70. doi: 10.3748/wjg.v29.i27.4252
35. Di Ciaula A, Bonfrate L, Khalil M, Portincasa P. The interaction of bile acids and gut inflammation influences the pathogenesis of inflammatory bowel disease. Intern Emerg Med (2023) 18(8):2181–97. doi: 10.1007/s11739-023-03343-3
36. Han B, Lv X, Liu G, Li S, Fan J, Chen L, et al. Gut microbiota-related bile acid metabolism-FXR/TGR5 axis impacts the response to anti-alpha4beta7-integrin therapy in humanized mice with colitis. Gut Microbes (2023) 15(1):2232143. doi: 10.1080/19490976.2023.2232143
37. Chen ML, Takeda K, Sundrud MS. Emerging roles of bile acids in mucosal immunity and inflammation. Mucosal Immunol (2019) 12(4):851–61. doi: 10.1038/s41385-019-0162-4
38. Kubota H, Ishizawa M, Kodama M, Nagase Y, Kato S, Makishima M, et al. Vitamin D receptor mediates attenuating effect of lithocholic acid on dextran sulfate sodium induced colitis in mice. Int J Mol Sci (2023) 24(4):3517. doi: 10.3390/ijms24043517
39. Parada VD, de la Fuente MK, Landskron G, Gonzalez MJ, Quera R, Dijkstra G, et al. Short chain fatty acids (SCFAs)-mediated gut epithelial and immune regulation and its relevance for inflammatory bowel diseases. Front Immunol (2019) 10:277. doi: 10.3389/fimmu.2019.00277
40. Recharla N, Geesala R, Shi XZ. Gut microbial metabolite butyrate and its therapeutic role in inflammatory bowel disease: A literature review. Nutrients (2023) 15(10):2275. doi: 10.3390/nu15102275
41. Vereecke L, Beyaert R, van Loo G. Enterocyte death and intestinal barrier maintenance in homeostasis and disease. Trends Mol Med (2011) 17(10):584–93. doi: 10.1016/j.molmed.2011.05.011
42. Benoit M, Desnues B, Mege JL. Macrophage polarization in bacterial infections. J Immunol (2008) 181(6):3733–9. doi: 10.4049/jimmunol.181.6.3733
43. Mantovani A, Sica A, Sozzani S, Allavena P, Vecchi A, Locati M. The chemokine system in diverse forms of macrophage activation and polarization. Trends Immunol (2004) 25(12):677–86. doi: 10.1016/j.it.2004.09.015
44. Ji J, Shu D, Zheng M, Wang J, Luo C, Wang Y, et al. Microbial metabolite butyrate facilitates M2 macrophage polarization and function. Sci Rep (2016) 6:24838. doi: 10.1038/srep24838
45. Liu T, Sun Z, Yang Z, Qiao X. Microbiota-derived short-chain fatty acids and modulation of host-derived peptides formation: Focused on host defense peptides. BioMed Pharmacother (2023) 162:114586. doi: 10.1016/j.biopha.2023.114586
46. Wang S, van Schooten FJ, Jin H, Jonkers D, Godschalk R. The involvement of intestinal tryptophan metabolism in inflammatory bowel disease identified by a meta-analysis of the transcriptome and a systematic review of the metabolome. Nutrients (2023) 15(13):2886. doi: 10.3390/nu15132886
47. Roager HM, Licht TR. Microbial tryptophan catabolites in health and disease. Nat Commun (2018) 9(1):3294. doi: 10.1038/s41467-018-05470-4
48. Yang C, Zhao Y, Im S, Nakatsu C, Jones-Hall Y, Jiang Q. Vitamin E delta-tocotrienol and metabolite 13’-carboxychromanol inhibit colitis-associated colon tumorigenesis and modulate gut microbiota in mice. J Nutr Biochem (2021) 89:108567. doi: 10.1016/j.jnutbio.2020.108567
49. Bischoff SC, Kaden-Volynets V, Filipe RL, Guseva D, Seethaler B. Regulation of the gut barrier by carbohydrates from diet - Underlying mechanisms and possible clinical implications. Int J Med Microbiol (2021) 311(4):151499. doi: 10.1016/j.ijmm.2021.151499
50. Pierre JF, Hinterleitner R, Bouziat R, Hubert NA, Leone V, Miyoshi J, et al. Dietary antioxidant micronutrients alter mucosal inflammatory risk in a murine model of genetic and microbial susceptibility. J Nutr Biochem (2018) 54:95–104. doi: 10.1016/j.jnutbio.2017.12.002
51. Lamas B, Richard ML, Leducq V, Pham HP, Michel ML, Da CG, et al. CARD9 impacts colitis by altering gut microbiota metabolism of tryptophan into aryl hydrocarbon receptor ligands. Nat Med (2016) 22(6):598–605. doi: 10.1038/nm.4102
52. Wojciech L, Png CW, Koh EY, Kioh D, Deng L, Wang Z, et al. A tryptophan metabolite made by a gut microbiome eukaryote induces pro-inflammatory T cells. EMBO J (2023) 42(21):e112963. doi: 10.15252/embj.2022112963
53. Hsu CN, Tain YL. Developmental programming and reprogramming of hypertension and kidney disease: impact of tryptophan metabolism. Int J Mol Sci (2020) 21(22):8705. doi: 10.3390/ijms21228705
54. Venkatesh M, Mukherjee S, Wang H, Li H, Sun K, Benechet AP, et al. Symbiotic bacterial metabolites regulate gastrointestinal barrier function via the xenobiotic sensor PXR and Toll-like receptor 4. Immunity (2014) 41(2):296–310. doi: 10.1016/j.immuni.2014.06.014
55. Ding X, Bin P, Wu W, Chang Y, Zhu G. Tryptophan metabolism, regulatory T cells, and inflammatory bowel disease: A mini review. Mediators Inflammation (2020) 2020:9706140. doi: 10.1155/2020/9706140
56. Nikolaus S, Schulte B, Al-Massad N, Thieme F, Schulte DM, Bethge J, et al. Increased tryptophan metabolism is associated with activity of inflammatory bowel diseases. Gastroenterology (2017) 153(6):1504–1516.e2. doi: 10.1053/j.gastro.2017.08.028
57. Nanda GC, Padhi MM, Pathak NN, Choppra KK. Screening of Madhumehaghna (anti diabetic) plants in Vrihattrayee. Bull Indian Inst Hist Med Hyderabad (2000) 30(1):15–26.
58. Kumar M, Prasad SK, Hemalatha S. A current update on the phytopharmacological aspects of Houttuynia cordata Thunb. Pharmacogn Rev (2014) 8(15):22–35. doi: 10.4103/0973-7847.125525
59. Rafiq S, Hao H, Ijaz M, Raza A. Pharmacological Effects of Houttuynia cordata Thunb (H. cordata): A Comprehensive Review. Pharm (Basel) (2022) 15(9):1079. doi: 10.3390/ph15091079
60. Ma Q, Wei R, Wang Z, Liu W, Sang Z, Li Y, et al. Bioactive alkaloids from the aerial parts of Houttuynia cordata. J Ethnopharmacol (2017) 195:166–72. doi: 10.1016/j.jep.2016.11.013
61. Wang Y, Zhan Y, Ji C, Shi C, Han J. Houttuynia cordata Thunb repairs steroid-induced avascular necrosis of the femoral head through regulating NF-kappaB signaling pathway. Toxicon (2023) 233:107270. doi: 10.1016/j.toxicon.2023.107270
62. Xu X, Liu S, Zhao Y, Wang M, Hu L, Li W, et al. Combination of Houttuynia cordata polysaccharide and Lactiplantibacillus plantarum P101 alleviates acute liver injury by regulating gut microbiota in mice. J Sci Food Agric (2022) 102(15):6848–57. doi: 10.1002/jsfa.12046
63. Li M, Wang C, Xu WT, Zhong X. Sodium houttuyfonate plays a protective role in the asthmatic airway by alleviating the NLRP3-related pyroptosis and Th1/Th2 immune imbalance. Mol Immunol (2023) 160:103–11. doi: 10.1016/j.molimm.2023.06.013
64. Tang J, Zhou L, Yuan G, Liu Y, Shi X, Lu Y, et al. Therapeutic effects on H1N1-induced pneumonia in mice and intestinal bacteria biotransformation of four main flavonoids from Houttuynia cordata Thunb. J Pharm BioMed Anal (2023) 233:115469. doi: 10.1016/j.jpba.2023.115469
65. Ju IG, Lee S, Choi JG, Kim N, Huh E, Lee JK, et al. Aerial part of Houttuynia cordata reverses memory impairment by regulating amyloid beta accumulation and neuroinflammation in Alzheimer’s disease model. Phytother Res (2023) 37(7):2854–63. doi: 10.1002/ptr.7781
66. Tan R, You Q, Cui J, Wang M, Song N, An K, et al. Sodium houttuyfonate against cardiac fibrosis attenuates isoproterenol-induced heart failure by binding to MMP2 and p38. Phytomedicine (2023) 109:154590. doi: 10.1016/j.phymed.2022.154590
67. Navarro V. The world health situation. Int J Health Serv (2004) 34(1):1–10. doi: 10.2190/4HMT-KDKH-18E7-2XBE
68. Cheng T, Xu C, Wu D, Yan G, Wang C, Wang T, et al. Sodium houttuyfonate derived from Houttuynia cordata Thunb improves intestinal malfunction via maintaining gut microflora stability in Candida albicans overgrowth aggravated ulcerative colitis. Food Funct (2023) 14(2):1072–86. doi: 10.1039/d2fo02369e
69. Zhang L, Lv H, Li Y, Dong N, Bi C, Shan A, et al. Sodium houttuyfonate enhances the intestinal barrier and attenuates inflammation induced by Salmonella typhimurium through the NF-kappaB pathway in mice. Int Immunopharmacol (2020) 89(Pt A):107058. doi: 10.1016/j.intimp.2020.107058
70. Cen L, Yi T, Hao Y, Shi C, Shi X, Lu Y, et al. Houttuynia cordata polysaccharides alleviate ulcerative colitis by restoring intestinal homeostasis. Chin J Nat Med (2022) 20(12):914–24. doi: 10.1016/S1875-5364(22)60220-6
71. He X, Hu M, Song C, Ni M, Liu L, Chen C, et al. Sodium new houttuyfonate effectively improves phagocytosis and inhibitsthe excessive release of inflammatory factors by repressingTLR4/NF-Кb pathway in macrophages. Curr Pharm Biotechnol (2023) 24(15):1964–71. doi: 10.2174/1389201024666230418163100
72. Ping W, Jinqian L, Jing D, Shuo W, Zongqiang F, Zhen L, et al. lmproving effect of houttuynia cordata polysaccharide on dextran sodium sulfate-induced ulcerative colitis. Sci Technol Food Industry (2021) 42(23):362–9. doi: 10.13386/j.issn1002-0306.2021030063
73. Letian Y, Chen Y, Xiaofei Y, Chang S, Gengxin Z, Fang C. Effect of tota flavonoids from Houttuvnia cordata Thunbon the treatment of colitis in mice. J Pharm Res (2022) 41(05):287–91. doi: 10.13506/j.cnki.jpr.2022.05.002
74. Lin R, Piao M, Song Y. Dietary quercetin increases colonic microbial diversity and attenuates colitis severity in citrobacter rodentium-infected mice. Front Microbiol (2019) 10:1092. doi: 10.3389/fmicb.2019.01092
75. Xiao-meng L, Jian-ping L, Dian-gui L, Jie R, Xin K, Ming-min DU. Effects of Xiezhuo Jiedu Decoction on expressions of TLR2 mRNA and protein in colon tissue of ulcerative colitis rats. China J Tradit Chin Med Pharm (2017) 32(02):838–40.
76. Siyu L, Xiaomeng L, Jianping L, Kangxin. Preventing recurrence of Jianpi Xiezhuo Jiedu Recipe on inactive ulcerative colitisrats: based on intestinal flora. Hebei J Tradit Chin Med (2022) 44(09):1507–11. doi: 10.3969/j.issn.1002-2619.2022.09.022
77. Zhoujin T, Hai W, Fulin L, Ying C, Guangxian C, Hualing Z, et al. Effect of ultra-micro powder qiweibaishusan on the intestinal microbiota and enzyme activities in mice. Acta Ecol Sin (2012) 32(21):6856–63. doi: 10.5846/stxb201109271422
78. Li C, Zhou K, Xiao N, Peng M, Tan Z. The effect of qiweibaizhu powder crude polysaccharide on antibiotic-associated diarrhea mice is associated with restoring intestinal mucosal bacteria. Front Nutr (2022) 9:952647. doi: 10.3389/fnut.2022.952647
79. Yang L, Jiang J. Bioactive components and functional properties of Hottuynia cordata and its applications. Pharm Biol (2009) 12):1154–61. doi: 10.3109/13880200903019200
80. Zhang S, Zhang M, Li W, Ma L, Liu X, Ding Q, et al. Research progress of natural plant polysaccharides inhibiting inflammatory signaling pathways and regulating intestinal flora and metabolism to protect inflammatory bowel disease. Int J Biol Macromol (2023) 253(Pt 4):126799. doi: 10.1016/j.ijbiomac.2023.126799
81. Laldinsangi C. The therapeutic potential of Houttuynia cordata: A current review. Heliyon (2022) 8(8):e10386. doi: 10.1016/j.heliyon.2022.e10386
82. Chou SC, Su CR, Ku YC, Wu TS. The constituents and their bioactivities of Houttuynia cordata. Chem Pharm Bull (Tokyo) (2009) 57(11):1227–30. doi: 10.1248/cpb.57.1227
83. Michl J, Ingrouille MJ, Simmonds MS, Heinrich M. Naturally occurring aristolochic acid analogues and their toxicities. Nat Prod Rep (2014) 31(5):676–93. doi: 10.1039/c3np70114j
84. Probstle A, Bauer R. Aristolactams and a 4,5-dioxoaporphine derivative from Houttuynia cordata. Planta Med (1992) 58(6):568–9. doi: 10.1055/s-2006-961554
85. Bauer R, Probstle A, Lotter H, Wagner-Redecker W, Matthiesen U. Cyclooxygenase inhibitory constituents from Houttuynia cordata. Phytomedicine (1996) 2(4):305–8. doi: 10.1016/S0944-7113(96)80073-0
86. Wei Q, Hua WF, Juan L, Jinyu L. Alkaloids from Houttuynia cordata and their antiplatelet aggregation activities. Chin J Nat Med (2011) 9(06):425–8. doi: 10.3724/SP.J.1009.2011.00425
87. Mingxing Y, Jing C, Chunyang S, Wenqing W, Jianguo F. Study on the safety of Houttuynia from aristolochia lactam. Chin Tradit Herbal Drugs (2021) 52(19):6045–51. doi: 10.7501/j.issn.0253-2670.2021.19.028
88. Chao D. A cross-sectional study on consumption habits of Houttuynia cordata and chronic kidney disease. Chin J Bioprocess Eng (2019) 17(02):189–94. doi: 10.3969/j.issn.1672-3678.2019.02.012
89. Kang CK, Hah DS, Kim CH, Kim E, Kim JS. Evaluation of the genotoxicity of extracts of Houttuynia cordata Thunb. Am J Chin Med (2012) 40(5):1019–32. doi: 10.1142/S0192415X12500759
Keywords: Houttuynia cordata thunb., homology of medicine and food, intestinal microenvironment, immunity, inflammatory bowel disease
Citation: Wang S, Li L, Chen Y, Liu Q, Zhou S, Li N, Wu Y and Yuan J (2023) Houttuynia cordata thunb. alleviates inflammatory bowel disease by modulating intestinal microenvironment: a research review. Front. Immunol. 14:1306375. doi: 10.3389/fimmu.2023.1306375
Received: 03 October 2023; Accepted: 06 November 2023;
Published: 22 November 2023.
Edited by:
Xinhua Shu, Glasgow Caledonian University, United KingdomReviewed by:
Wei Liu, Zhejiang Academy of Agricultural Sciences, ChinaXuejuan Liang, Hunan Academy of Chinese Medicine, China
Haoqing Shao, Hunan University of Medicine, China
Copyright © 2023 Wang, Li, Chen, Liu, Zhou, Li, Wu and Yuan. This is an open-access article distributed under the terms of the Creative Commons Attribution License (CC BY). The use, distribution or reproduction in other forums is permitted, provided the original author(s) and the copyright owner(s) are credited and that the original publication in this journal is cited, in accordance with accepted academic practice. No use, distribution or reproduction is permitted which does not comply with these terms.
*Correspondence: Jiali Yuan, 2748132800@qq.com; Yueying Wu, misswyy@sina.cn
†These authors have contributed equally to this work and share first authorship