- 1DKMS Life Science Lab, Dresden, Germany
- 2DKMS Group, Tübingen, Germany
- 3Institute for Immunology, Medical Faculty Carl Gustav Carus, University of Technology (TU) Dresden, Dresden, Germany
- 4Institute for Immunology, University Hospital Heidelberg, Heidelberg, Germany
MICA is a stress-induced ligand of the NKG2D receptor that stimulates NK and T cell responses and was identified as a key determinant of anti-tumor immunity. The MICA gene is located inside the MHC complex and is in strong linkage disequilibrium with HLA-B. While an HLA-B*48-linked MICA deletion-haplotype was previously described in Asian populations, little is known about other MICA copy number variations. Here, we report the genotyping of more than two million individuals revealing high frequencies of MICA duplications (1%) and MICA deletions (0.4%). Their prevalence differs between ethnic groups and can rise to 2.8% (Croatia) and 9.2% (Mexico), respectively. Targeted sequencing of more than 70 samples indicates that these copy number variations originate from independent nonallelic homologous recombination events between segmental duplications upstream of MICA and MICB. Overall, our data warrant further investigation of disease associations and consideration of MICA copy number data in oncological study protocols.
1 Introduction
The MICA (MHC class I polypeptide-related sequence A) gene is located on chromosome 6 within the human major histocompatibility (MHC) complex, between HLA-B and MICB (1). Despite their high structural similarity to the classical human leukocyte antigen (HLA) genes, MICA and MICB (MICA/B) do not present peptides. Upon stress-induced expression by various cell types (e.g., epithelial cells, fibroblasts), MICA/B activate the NKG2D receptor, which is primarily found on NK cells and T cell subsets, thereby promoting immune cell recognition and immune surveillance (2–4).
Similar to the HLA genes, MICA is polymorphic with 531 currently known MICA alleles, encoding for 280 distinct proteins (IMGT-IPD/HLA database; release 3.53, 07/2023) (5). These MICA variants differ in the mechanism of cell surface attachment. While some are transmembrane proteins and can be shed from the membrane by proteolytic cleavage, the most frequent MICA allele, MICA*008, and other MICA*008-related alleles, are membrane-bound via a GPI-anchor and are recruited to exosomes (6–8). Nevertheless, both variants of soluble MICA (sMICA) can bind to NKG2D, which leads to receptor internalization. Decreased MICA cell surface expression and increased sMICA levels have been associated with inferior outcome in tumor patients and may represent an important cancer immune evasion principle (7–9). Consequently, innovative therapeutic approaches aim to increase the MICA/B density on the cellular surface by enhancing MICA/B expression and/or inhibition of MICA/B shedding (10–13). Some of these approaches might also be useful against infectious diseases since multiple viruses exploit similar strategies to avoid host cell recognition by immune cells (14–16).
Studies of MICA allele disease associations are hampered by the strong linkage disequilibrium of MICA and HLA-B (17). In transplantation, increasing evidence supports independent positive effects of MICA matching on outcome (18–21). In contrast, an independent role of MICA genotypes in addition to the well-established marker HLA-B*27 in ankylosing spondylitis (AS) remains questionable (22–24).
Gene copy number variations (CNV) contribute to human genomic diversity and arise from gene duplication or gene deletion events. Similar to single nuclear polymorphisms, some CNVs can be associated with disease phenotypes while others only seem to be benign polymorphic variations (25). MICA deletions have been identified in Asian populations with allele frequencies between 0.8% and 4% (26–28). The most common deletion is linked to HLA-B*48 and results from a 100 kb deletion between HLA-B and MICB (26, 29). So far, there are only controversial or very limited reports on disease associations, in particular nasopharyngeal carcinoma in Chinese patients (28, 30) and gout in Polynesians (31). To the best of our knowledge, MICA duplications have only been described as case reports, partly linked to leukemia (28, 32, 33).
Here, we present population frequencies of MICA copy number variations based on over two million individuals and a detailed characterization of the underlying genomic reorganization.
2 Methods
2.1 Samples
Volunteers from Germany (56%), UK (18%), Poland (15%), US (6%), Chile (4%), India (1%), and South Africa (0.4%) provided over two million samples to DKMS for registration as potential stem cell donors between May 2019 and October 2021. As part of the registration process, the donors are asked to self-assign to their ethnic background. Since selectable ethnicities vary between the different DKMS donor center questionnaires, data in this study are only grouped within one donor center (e.g., samples indicated as DE_Poland or PL_Poland share the Polish ethnic background, but one group registered with DKMS Germany and the other with DKMS Poland, respectively). The genotyping is within the scope of the consent forms signed at recruitment. For whole genome sequencing, pre-selected donors provided blood and written informed consent under a protocol approved by the Ethics Committee of the Technische Universität Dresden (EK 423092019). The study was conducted in accordance with the Declaration of Helsinki.
2.2 Genotyping
High-throughput genotyping is performed for HLA-A, -B, -C, -E, -DPB1, -DQB1, -DRB1, -DPA1, -DQA1, -DRB3/4/5, MICA/B, KIR, blood groups ABO and Rh, and CCR5 as described before (34–39). In brief, DNA is isolated from buccal swabs and selected exons are amplified by PCR, followed by an additional barcoding PCR. After pooling and clean-up, the final sequencing libraries contain all amplicons of either 3480 or 7380 potential stem cell donors and are sequenced on a HiSeq 2500- (HiSeq Rapid SBS Kits V2 (500 cycles)) or NovaSeq 6000 (SP Reagent Kit (500 cycles)) instrument (Illumina, San Diego, USA), respectively. Data analysis is performed with neXtype and results are reported according to the current nomenclature (34, 40, 41). MICA and MICB are amplified in a multiplex PCR, in which the same primer pairs generate three amplicons for each gene. They cover exons 2 and 3 in separate amplicons and most bases of exons 4 and 5 in a third amplicon (35). Due to this restricted coverage, certain alleles cannot be resolved. These ambiguous genotyping results are abbreviated by using the most frequent allele of the allele group followed by a hash symbol (#): MICB*003/005/006/010 is reported as MICB*005#, MICB*004/028 is reported as MICB*004# (35). Since our sequencing data lack physical phasing, haplotype calls are based on frequency correlations from our population data (Supplementary Data 1 and 2). Consequently, rare haplotypes cannot be resolved.
Full-gene sequencing of MICA and MICB was conducted by long-range PCR with primer pairs located in the 5’- and 3’ UTR of the genes. Library preparation and sequencing was performed using SQK-LSK110 kits and MinION 9.4.1 flowcells following the manufacturer’s instructions (Oxford Nanopore Technologies, Oxford, UK). Sequences were analyzed with DR2S, an in-house developed software that is able to distinguish between alleles with varying gene copy numbers (42).
2.3 Identification of MICA copy number variations
For MICA genotyping, neXtypes algorithm first analyzes the sequencing reads of the three amplicons (exon 2, exon 3, exon 4/5) separately by calling exon allele groups (EAG) (34, 35). One EAG contains all known MICA alleles that share the same sequence in the given amplicon. These EAGs are then phased into MICA alleles under the assumption that only EAG combinations that can be found in the IPD/IMGT-HLA database are valid. For the classical HLA genes, up to two different EAGs per amplicon are allowed. For MICA, neXtype has calculated with up to three different EAGs per amplicon since May 2019. Consequently, it can detect three MICA gene copies in a sample (Supplementary Table 1, Sample B). Furthermore, in samples where only two different MICA alleles have been identified, a read coverage distribution of about 2:1 in all three amplicons also results in a genotyping result with three MICA gene copies (Supplementary Table 1, Sample C). Copy numbers of more than three can so far not be detected by neXtype.
In its current version, neXtype is only able to report hemizygous genotypes for KIR genes, but not for HLA or MIC genes. For this study, samples containing MICA deletions were identified retrospectively. For each sample, the number of mapped sequencing reads per exon was extracted from neXtype for both MICA and MICB. This number was used to calculate the ratio between MICA- and MICB sequencing reads for each exon, followed by the calculation of its mean and standard deviation (sd). All samples with a sequencing read coverage <100 in any exon or a sd >0.3, indicative of an uneven PCR amplification in one of the exons, were excluded. Additionally, samples with a heterozygous genotyping result in neXtype were excluded (n=21). In total, 2,089,638 samples (95%) passed all quality criteria and were used for further analysis (Supplementary Figure 1). For most samples, the calculated MICA/MICB ratio centered around 0.82 with only moderate variability. Two additional peaks were detected at ratios of 0.41 and 1.27 and represent MICA hemizygous (deletion) samples or samples with three MICA gene copies (duplications), respectively. All samples with a MICA/MICB ratio <0.53 were designated to be hemizygous for MICA.
2.4 Whole genome sequencing
One sample, pre-selected for harboring three different MICA alleles and HLA-B*27:02:01G, was subjected to whole genome sequencing. DNA was extracted from 4 ml blood using a chemagic™360 instrument according to the manufacturer’s instructions (PerkinElmer chemagen Technologie GmbH, Baesweiler, Germany). Small DNA was removed with Short Read Eliminator Kit XS (PacBio, Menlo Park, USA). PCR-free library preparation was performed with SQK-LSK112 kit (Oxford Nanopore Technologies, Oxford, UK) and the sequencing library was finally sequenced on MinION and PromethION flowcells with 10.3 chemistry to 20x sequencing depth (Oxford Nanopore Technologies, Oxford, UK). Assemblies were performed using shasta (43), Raven (44), miniasm/Racon (45, 46), or canu (47).
2.5 Targeted sequencing of recombination regions
PCR amplification and sequencing of the MICA duplication recombination region was performed using the primer pair MICA-Dup_M15: 5’-CAGTGCTGGATAGCATTTATGAGAC-3’and 5’-CTGCACAGTCACCCGCATGCAC-3’. 0.2 µM of each primer were mixed with genomic DNA (range: 6-300 ng), dNTPs (0.4 mM each), 1x Advantage Genomic LA Buffer and 1.25 U Advantage Genomic LA Polymerase Mix (Takara Bio, Mountain View, California) in a 25 µl reaction. PCR conditions: 94°C 1 min, 35 cycles: 98°C 10 sec/57°C 20 sec/68°C 5 min, 72°C 10 min. In the presence of a MICA duplication, a 4.9 kb fragment is amplified. Another 10 cycles of PCR were used to attach sample barcodes.
PCR amplification and sequencing of the MICA deletion recombination region was performed with primers DF (5’-AGAGTACAATCCATGTATAGAT-3’) and DR (5’-TTATCTCTTCTGTCCGTGAC-3’) according to Komatsu-Wakui et al. using the same reactions as described above (27). PCR conditions: 94°C 1 min, 35 cycles: 98°C 10 sec/55°C 20 sec/68°C 5 min, 72°C 10 min. In the presence of a MICA deletion, a 4.1 kb fragment is amplified. Another 10 cycles of PCR were used to attach custom sample barcodes. For some initial samples, primers DA and DS according to Komatsu-Wakui et al. were investigated (27). However, they could only be used for the HLA-B*48-linked MICA deletion due to primer mismatches in other haplotypes.
All PCR products were pooled and purified using 0.7x SPRIselect beads (Beckman Coulter, Brea, California). Library preparation was performed with SQK-LSK114 kits. Sequencing was performed on a GridION instrument and 10.4.1 flowcells according to the manufacturer’s instructions (Oxford Nanopore Technologies, Oxford, UK).
Sequencing reads were mapped against hypothesized references that were generated with the knowledge from our WGS sample and data from Komatsu-Wakui et al. (CLC Genomics Workbench 21 (Qiagen, Hilden, Germany)) (26, 27). These references contained the genomic region from HLA-B to MICB, using the CT dinucleotide repeat region around chr6:31389884 and chr6:31486863 as potential breakpoints (GRCh38/hg38). To exclude reads derived from unspecific amplification, the unmodified regions upstream of MICA and MICB were included in the reference. Only reads that exclusively mapped to the putative recombination region were finally used to calculate the breakpoint consensus sequence of each sample (Supplementary Data 3, GenBank accession numbers OR060976-OR061013).
2.6 Localization of the recombination site
The 20 kb upstream of MICA exon 1 and the 20 kb upstream of MICB exon 1 were extracted from publicly available genomic MHC assemblies to identify MICA and MICB specific bases in this highly homologous region. All sequences with larger assembly gaps or obvious assembly errors were excluded. Finally, 37 and 34 sequences for MICA- and MICB regions of diverse haplotypes were included, respectively (Supplementary Data 4) (48, 49). These sequences were aligned with the generated breakpoint consensus sequences of the MICA duplication- and MICA deletion samples (CLC Genomics Workbench 21 (Qiagen, Hilden, Germany)). In this alignment, a few bases could exclusively be assigned to either the region upstream of MICA or the region upstream of MICB in all available haplotypes (Supplementary Data 5). These marker SNPs were used to narrow down the presumed region of recombination for each sample.
3 Results
3.1 Detection of MICA copy number variations
We implemented amplicon-based genotyping for MICA and MICB as part of the genotyping profile for newly registered potential stem cell donors in 2017 (35). Frequently, the results were suggestive of three distinct MICA gene copies in one individual (Supplementary Table 1), which was confirmed by sequencing of the entire gene in selected samples (not shown). Consequently, we implemented the detection of three MICA gene copies into our NGS genotyping software neXtype (34).
Between May 2019 and October 2021, this workflow was used to process 2,188,836 samples. Among them were 22,880 samples (1%) with three copies of MICA. Apart from the MICA CNV, these samples had normal HLA genotyping results, including the MICA neighboring genes HLA-B and MICB.
Since neXtype cannot detect MICA deletions, we identified MICA hemizygous samples in this cohort retrospectively. In our workflow, the usage of identical primer pairs for MICA and MICB in a multiplex PCR reaction leads to a stable ratio of MICA to MICB sequencing reads. By using this MICA/MICB ratio, samples could clearly be separated according to their respective MICA gene copy number (Supplementary Figure 1). This identified 9,262 (0.44%) MICA hemizygous samples and confirmed our neXtype-based detection of MICA duplications with 97% concordance.
3.2 MICA duplications
3.2.1 Linkage to HLA-B*27:02:01G
Of the 22,880 samples with a MICA duplication, 91% share one distinct haplotype: C*02:02:02G~B*27:02:01G~MICA*007~MICA*008~MICB*005# (40). A few of these samples were sequenced at higher resolution as C*02:02:02:03~B*27:02:01:01~MICA*007:01:01~MICA*008:01:02~MICB*005:02:03. Another 705 samples (3%) share the HLA-B*27:02:01G allele, but not the HLA-C- or MICB alleles. Overall, this indicates a strong linkage between HLA-B*27:02:01G and the MICA duplication. Indeed, in our dataset, the majority (68%) of HLA-B*27:02:01G-carriers possess the MICA duplication. However, the proportion of MICA duplication carriers among HLA-B*27:02:01G carriers varies with ethnicity. While around 83% of HLA-B*27:02:01G carriers harbor the MICA duplication in Poland or South Africa, only 13% of the HLA-B*27:02:01G positive individuals carry the duplication in Chile (Figure 1A).
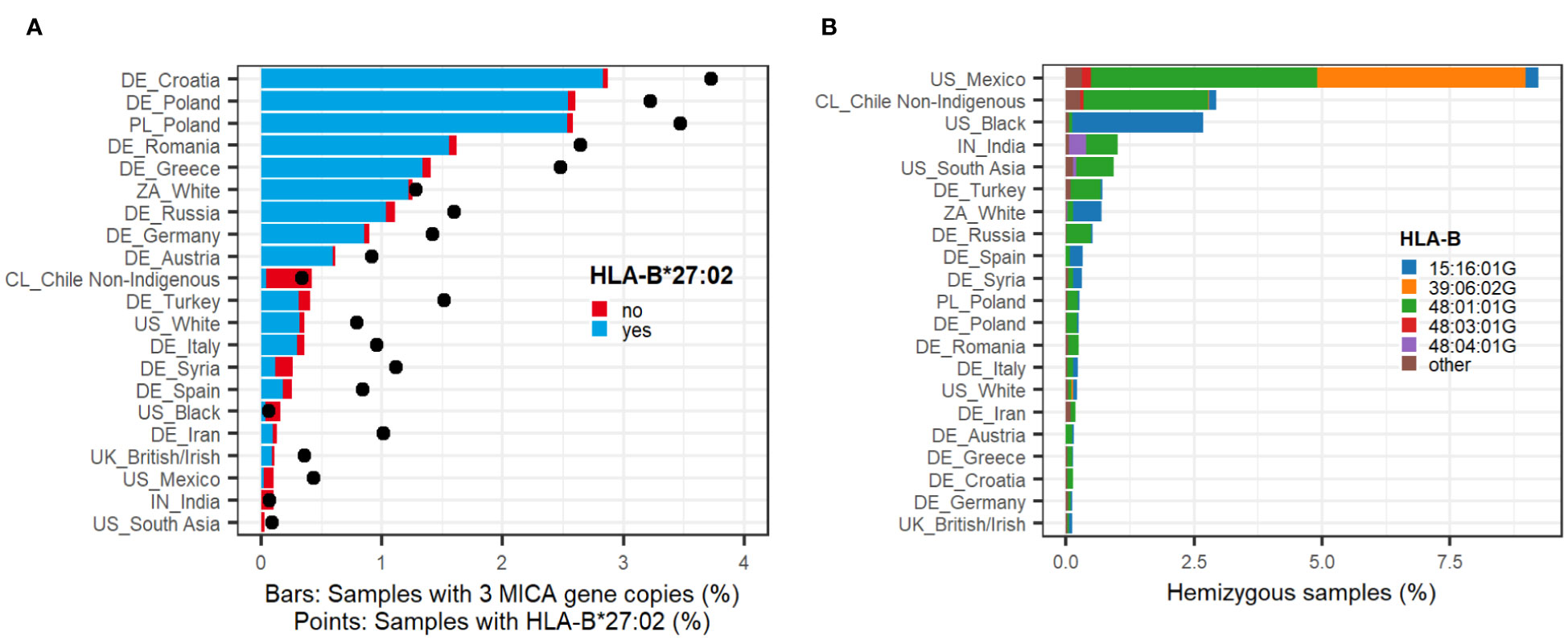
Figure 1 Population frequencies. (A) MICA duplications. Sample population frequencies of a MICA duplication in selected ethnic groups (n > 2500) (bars). Colors of the bars indicate whether samples have the common linkage to HLA-B*27:02:01G (blue) or any other haplotype (red). Black points depict the sample frequency of HLA-B*27:02:01G in the given population irrespective of MICA. All ethnic groups with n > 50 can be found in Supplementary Data 6. (B) MICA deletions. Sample population frequencies of MICA hemizygosity in selected ethnic groups (n > 2500). Colors of the bars indicate the linkage to frequently identified HLA-B alleles (Table 1). All ethnic groups with n > 50 can be found in Supplementary Data 7.
Interestingly, we also identified novel MICA alleles that were exclusively found within the described HLA-B*27:02:01G-linked MICA duplication haplotype. The most frequent one (n=50) is a variation of the MICA*007 allele and was named MICA*243 (IPD/IMGT-HLA release 3.50; Accession number OX249873).
Even though the HLA-B*27:02:01G-linked haplotype is by far dominant, we identified MICA duplications in 1,260 samples without HLA-B*27:02:01G (5.5% of samples with a detected MICA duplication; 0.06% of total cohort). In these samples, we could not find another HLA-B allele that was predominantly linked to the MICA duplication other than the extremely rare alleles HLA-B*27:30 (n=6) and HLA-B*27:83 (n=3) (Supplementary Data 1). This suggests numerous independent recombination events.
3.2.2 Population frequencies
Self-assigned ethnicities of the sample donors were used to calculate population frequencies of MICA duplications. Highest frequencies occur in the Eastern European populations with 2.9% in Croatia and 2.6% in Poland compared to 0.9% and 0.1-0.3% in Germany and Southern Europe/Great Britain, respectively (Figure 1A; Supplementary Data 6). In all studied European countries, the frequency of the MICA duplication clearly correlates with the population frequencies of HLA-B*27:02:01G, which indicates that it is predominantly driven by this one haplotype (Figure 1A). Nevertheless, MICA duplications are not completely absent in countries of non-European heritage and/or low HLA-B*27:02:01G allele frequency. For example, we identified MICA duplications in roughly 0.4% of the samples from Chileans with self-assigned non-indigenous heritage. In Mexicans, Indians, the American Black, and South Asian population, only few MICA duplications could be detected.
3.3 MICA deletions
3.3.1 Haplotypes
In our dataset of 2,089,638 samples, 9,262 samples (0.44%) were identified as MICA hemizygous. In contrast to the MICA duplication, they cannot be associated with a single major haplotype. Instead, the MICA deletion is strongly linked to several HLA-B alleles, the majority to HLA-B*48:01:01G (n=5,747, 62%) (Table 1; Supplementary Data 2). Interestingly, this haplotype does not contain any functional MIC protein since it is also linked to the MICB null allele MICB*009N. However, other MICA deletion haplotypes, including HLA-B*48:04:01G, HLA-B*15:16:01G, and HLA-B*39:06:02:02 are linked to functional MICB alleles. The strong association of HLA-B*39:06:02:02 with a MICA deletion could only be determined after full gene sequencing. While only 2% of all HLA-B*39:06:02G carriers in our cohort have a MICA deletion, 77% of the samples with US Mexican ethnicity do. Sequencing of selected samples in full length revealed that HLA-B*39:06:02:01 is the dominant HLA-B*39:06:02G allele in Europe and is not linked to a MICA deletion. In contrast, HLA-B*39:06:02:02 was identified in all MICA deleted samples from the US Mexican population.
Nevertheless, and similar to the MICA duplications, not all MICA hemizygous samples could be assigned to a frequent haplotype (n=1,034, 11%). In these samples, a great variety of different HLA-B alleles was identified. Furthermore, only a few rare HLA-B alleles could be exclusively linked to MICA hemizygosity (Supplementary Data 2). For example, 96% of the samples with HLA-B*48:01:01G are MICA hemizygous, but 4% are MICA heterozygous.
3.3.2 Population frequencies
The highest population frequencies of MICA hemizygosity were detected in people with Mexican or South American heritage. In Mexicans, who registered with DKMS in the US, a MICA deletion was identified in over 9% of the samples (Figure 1B; Supplementary Data 7). Among them are two major haplotypes, linked to either HLA-B*48:01:01G or HLA-B*39:06:02G. In Chileans, the HLA-B*48:01:01G haplotype dominates, while the US Black population is almost exclusively linked to HLA-B*15:16:01G, both with frequencies over 2.5%. In Indians and the US South Asian population, a linkage to HLA-B*48:04:01G could additionally be identified. In sharp contrast to these populations, MICA hemizygosity is consistently less frequent in European populations (around 0.3%).
3.4 Recombination
We performed long-read whole genome sequencing of a sample with three distinct MICA alleles and HLA-B*27:02:01G to examine the molecular mechanisms underlying our observations. Despite recovering all three MICA alleles in the whole genome data, assemblies with a variety of algorithms failed to reveal the genomic location of the duplication. We hypothesized that the 30 kB long segmental duplication upstream of MICA and upstream of MICB (93% sequence identity) might drive the duplication events by nonallelic homologous recombination (NAHR) (Figure 2A) (50–52). Manual inspection of the read mapping in this region indicated a potential breakpoint. To confirm this hypothesis, we sequenced a 4.9 kB PCR product spanning the predicted recombination region. In the case of a MICA duplication, a NAHR event should result in an amplicon that starts with a sequence otherwise located upstream of MICB and end with a sequence otherwise located upstream of MICA. Indeed, the obtained sequence was in full agreement with such an event.
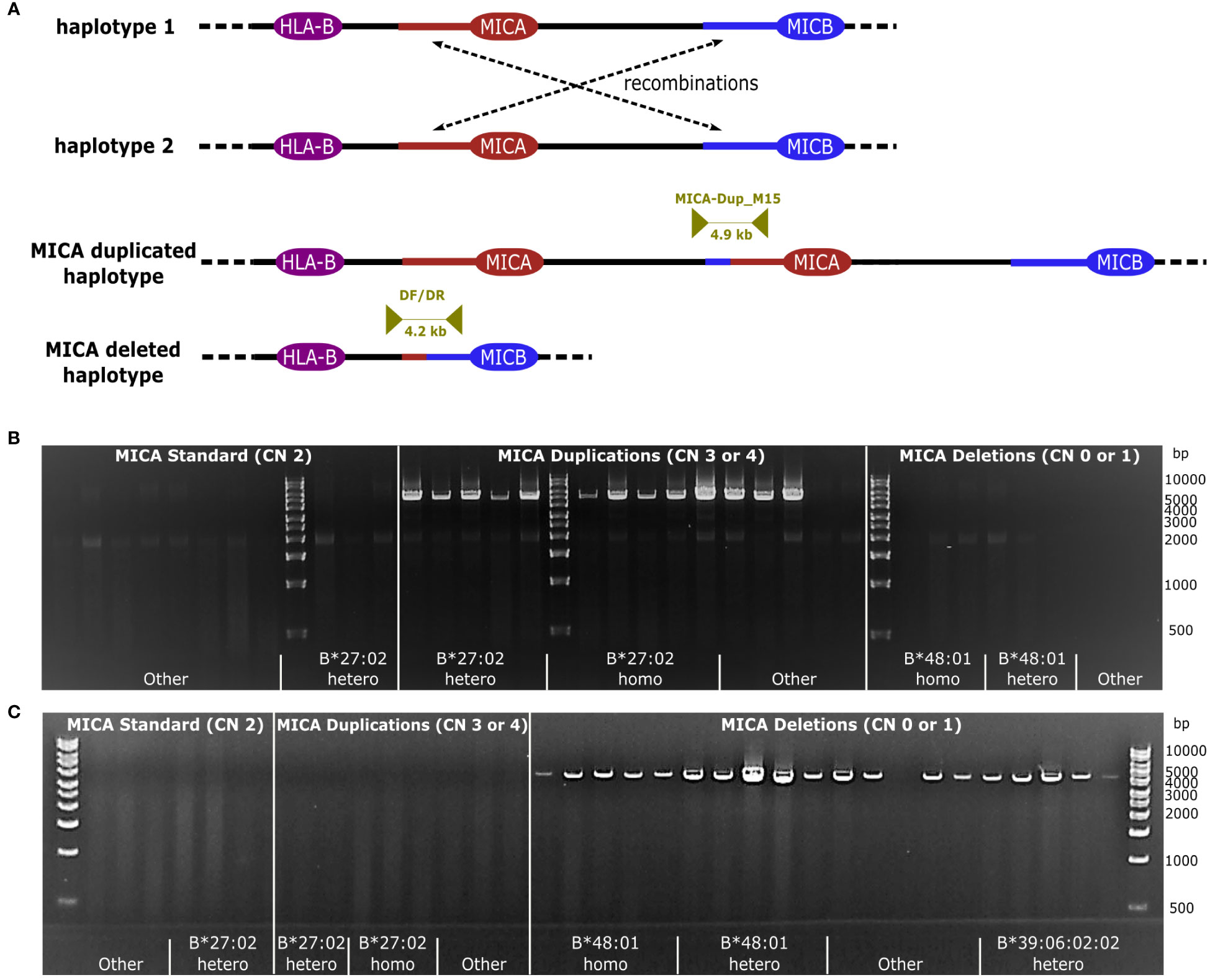
Figure 2 Recombination by NAHR. (A) Schematic representation of NAHR. Recombination takes place between two segmental duplications upstream of MICA and MICB, respectively (red/blue line). Either a MICA duplicated- or a MICA deleted haplotype can be generated. Dark yellow arrows indicate the primer pairs MICA-Dup_M15, specific for the MICA duplication, and DF/DR, specific for the MICA deletion, enclosing the anticipated recombination site. (B, C) Primer specificity. Samples with a MICA copy number (MICA CN) between 0 and 4 were subjected to a PCR with MICA-Dup_M15 (B) or DF/DR (C). Samples included homo- and heterozygous samples of the dominant haplotypes linked to HLA-B*27:02:01G and HLA-B*48:01:01G as well as randomly chosen haplotypes (designated as Other) as well as HLA-B*27:02:01G genotyped samples without a MICA duplication. PCR failures could be caused by variations in the primer binding sites due to unavailable intergenic sequence information for many MHC haplotypes.
Next, we took advantage of the large variety of MICA duplication and -deletion haplotypes described above to characterize the underlying recombination sites. Using the duplication specific PCR and a corresponding deletion specific PCR, we amplified and sequenced the predicted recombination region of 53 MICA duplicated and 23 MICA deleted samples (Figure 2; Supplementary Data 3). Due to the very high sequence identity of the two segmental duplications, it is not possible to pinpoint the breakpoint to a particular base. Nevertheless, by aligning the sequences with available MHC haplotypes, we could narrow the recombination sites for the HLA-B*27:02:01G-linked MICA duplication and the HLA-B*48-linked MICA deletion haplotype down to a region of 215 and 1,474 bases, respectively (Figure 3, Breakpoint Region Groups 1 and 13). Interestingly, these two regions do not overlap, therefore ruling out a common ancestral event. Whenever a specific haplotype was covered by multiple samples, the sequence of the recombination region was identical, pointing to a singular recombination event underlying each haplotype (Supplementary Data 3, consensus_identical_group). Despite the clear variations, all studied recombination sites fall within a stretch of 2,822 bases (upstream MICA: chr6:31388981-chr6:31391803; upstream MICB: chr6:31485960-chr6:31488704) (Figure 3; Supplementary Data 5). Within this stretch, the recombination hot spot motif 5′-CCNCCNTNNCCNC-3′ can be found (upstream MICA: chr6:31390917, upstream MICB: chr6:31487832) (53, 54).
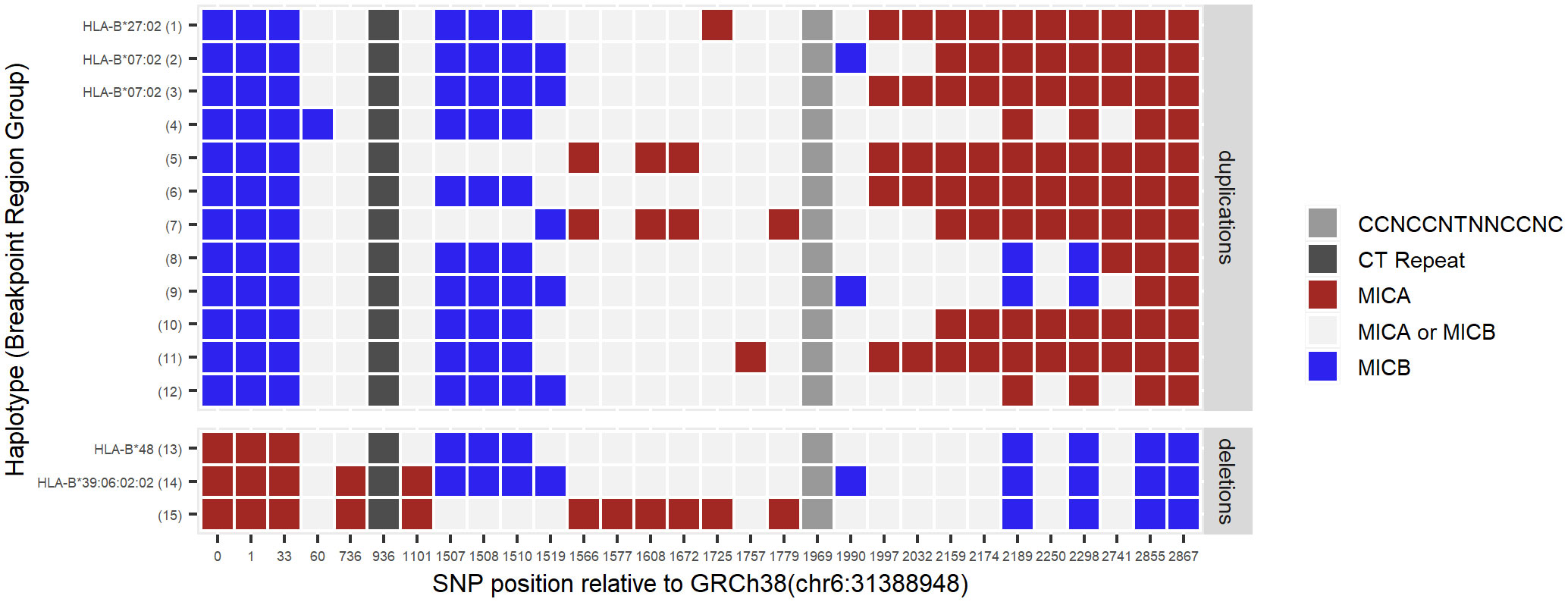
Figure 3 Localization of recombination sites. By aligning publicly available MHC haplotype sequences, upstream MICA- (red) and upstream MICB-specific (blue) marker SNP positions were identified in the segmental duplication regions. Other bases at these positions are shared between MICA- and MICB haplotypes (light grey). Identified marker SNPs were examined in the potential breakpoint regions of 53 MICA duplicated and 23 MICA deleted samples. Samples with the same pattern of the marker SNPs were grouped for better visibility (Breakpoint Region Group of individual samples: Supplementary Data 3). While Breakpoint Region Groups 1-3 and 13-14 represent common haplotypes, for groups 4-12 and 15 no haplotypes could be determined. In accordance with the proposed NAHR mechanism, MICA duplications are characterized by starting with MICB-specific bases before switching to MICA-specific bases. For MICA deletions, in full agreement with this model, it is the other way around. The switch of colors in each group narrows down the region in which the recombination has most probably happened, e.g., for the HLA-B*27:02 haplotype (Breakpoint Region Group 1) somewhere between position 1510 and 1725. Middle grey box: position of the recombination hot spot motif. Dark grey box: position of the CT dinucleotide repeat. Marker SNP positions are given relative to position chr6:31388948 of GRCh38/hg38 (Supplementary Data 5).
4 Discussion
As activating ligands of the NKG2D receptor, MIC proteins play an elaborate role in the regulation of NK and T cell function (2). This regulation is highly complex and, on the genomic level, additionally influenced by the nearly endless possible combinations of the polymorphic HLA and KIR genes (5, 38). While CNVs are common for KIR genes, germline CNVs of the classical HLA genes are rare (55). In contrast, we identified MICA CNVs in 1.4% of our samples. In some populations (e.g., Mexicans, South American populations), MICA CNV frequencies even reached 9% (56).
Despite a MICA duplication frequency of 1% in our samples, to our knowledge only a single case has previously been characterized (33). Since the most common haplotype, linked to HLA-B*27:02:01G, contains the frequent alleles MICA*008 and MICA*007 (42% and 5% allele frequency in the German population), copy number unaware genotyping will often result in an unsuspicious heterozygous result (35). In the future, the herein described strong linkage to HLA-B*27:02:01G may help to identify MICA duplications. However, it cannot substitute for copy number aware genotyping approaches, since there are HLA-B*27:02:01G haplotypes without the MICA duplication and several non-HLA-B*27:02:01G-linked MICA duplication haplotypes.
Our analysis of MICA deletions confirms the dominant HLA-B*48:01~MICB*009N associated haplotype. In Asian populations, this haplotype has previously been reported in 0.8-4% of the samples (26–28). While these numbers are supported by our data, Asian populations are not the only ones with such high frequencies (Supplementary Data 7). Especially in South American populations, the HLA-B*48:01~MICB*009N associated MICA deletion frequency is remarkably high, consistent with reports of high HLA-B*48:01 population frequencies in some indigenous populations (56–58). Individuals homozygous for this haplotype lack any functional MICA or MICB gene. In our cohort, we identified more than 50 of such homozygous individuals demonstrating that a complete absence of functional MIC proteins does not lead to major health issues, which would have prevented them from registering as potential stem cell donors (27). In addition to HLA-B*48:01:01G, we identified seven other HLA-B alleles with strong linkage to a MICA deletion that belong to the HLA-B*48, HLA-B*15, or HLA-B*39 allele groups (Table 1). The example of HLA-B*39:06:02G with neglectable linkage (2%) in the overall study population but strong linkage (77%) in the Mexican ethnicity suggests that more HLA-B linkages could be identified by higher resolution genotyping or analysis of strictly defined populations. The self-assigned populations used in this study clearly have their limitations as evident from the suspicious correlation between people self-assigned as “white” in South Africa and typical black heritage haplotypes (ZA_White, Figure 1B). Similarly, the ethnic group that self-assigned as “Chile Non-Indigenous” is confounded by some “Mapuche” heritage (59). Consequently, data from underrepresented and genetically diverse populations should be interpreted with caution.
For more than 1,000 MICA hemizygous and more than 1,200 MICA duplicated samples, we could not find any obvious recurrent haplotype due to the lack of physical intergenic phasing or available family data. In addition, despite our large cohort, we did not find some other previously reported MICA deletion linkages (e.g., HLA-B*07 (60), HLA-B*41 (60), HLA-B*46 (27), A*11:01~B*13:01~MICB*009N~DRB1*04:06 (28), or B*35:01~MICB*009N~DRB1*15:01 (28)). This supports our hypothesis that MICA deletions and -duplications are the result of frequent independent recombination events that partly may have occurred only recently.
The authors who first described the HLA-B*48:01:01G-linked MICA deletion in 1999 thoroughly mapped it to a 100 kB genomic deletion. They speculated that the underlying homologous recombination might have happened at a long CT dinucleotide repeat (26, 27). Our data for this haplotype support this possibility (Figure 3, Breakpoint Region Group 13). However, this is not the universal breakpoint for all MICA CNVs. Instead, our data show that breakpoints are scattered within a region of roughly 3 kb. This observation is expected for NAHR and further supported by the recombination hot spot motif 5′-CCNCCNTNNCCNC-3′ within this region, which is a binding site for the NAHR promoting zinc-finger protein PRDM9 (52–54, 61). Interestingly, allelic variations of PRDM9 influence the recombination hot spots activity, which could be one potential reason for our observation of more MICA CNVs without a frequent haplotype in some populations (e.g., Chileans) (62, 63).
The significant MICA CNV population frequencies we report raise the question of MICA CNV association with disease phenotypes. So far, influence of MICA duplications on overall NKG2D ligand expression, and consequently NKG2D receptor activation, is unclear. For MICA hemizygous samples, reduced serum levels of sMICA were reported (31). Ratios of membrane-bound MICA versus sMICA levels have been shown to influence the outcome in cancer patients (8, 9). An additional gene copy of MICA may result in higher levels of membrane-bound MICA and may therefore be beneficial for the recognition of tumor cells by the immune system. However, the opposite could also be true and elevated MICA expression levels would lead to an increase in sMICA. Therefore, MICA CNVs should be considered in MICA/sMICA expression studies.
A MICA deletion, presumably in most studies the HLA-B*48-linked haplotype, has been associated with several diseases, in some instances with conflicting results, e.g., nasopharyngeal carcinoma (28, 30, 31). The most prevalent MICA duplication is linked to HLA-B*27:02:01G, which is one of the risk alleles for AS (64–66). An independent contribution of MICA*007:01 to AS has been discussed, but remains questionable due to its high linkage disequilibrium with HLA-B*27 (22, 24). Since our cohort of potential bone marrow donors systematically excludes disease phenotypes, the question of MICA CNV association with disease conditions including AS cannot be settled based on our study. In conclusion, pathogenetic relevance of MICA CNVs remains a fascinating possibility that warrants assessment of MICA genotypes in disease cohorts.
Data availability statement
Sequence data that support the findings of this study have been deposited in GenBank with accession numbers OR060976-OR061013 (Supplementary Data 3). Other data are available upon reasonable request from the corresponding author.
Ethics statement
The studies involving humans were approved by Ethics Committee of the Technische Universität Dresden, Dresden, Germany. The studies were conducted in accordance with the local legislation and institutional requirements. The participants provided their written informed consent to participate in this study.
Author contributions
AK: Conceptualization, Formal Analysis, Investigation, Methodology, Project administration, Supervision, Visualization, Writing – original draft, Writing – review & editing. KP: Investigation, Methodology, Writing – review & editing. SK: Formal Analysis, Investigation, Methodology, Writing – review & editing. MK: Project administration, Writing – review & editing. JS: Formal Analysis, Investigation, Writing – review & editing. DS: Software, Writing – review & editing. GSchö: Formal Analysis, Investigation, Supervision, Writing – review & editing. CM: Formal Analysis, Writing – review & editing. GSchä: Formal Analysis, Writing – review & editing. AS: Resources, Supervision, Writing – review & editing. AR: Resources, Supervision, Writing – review & editing. VL: Conceptualization, Investigation, Resources, Supervision, Writing – original draft, Writing – review & editing.
Funding
The author(s) declare that no financial support was received for the research, authorship, and/or publication of this article.
Acknowledgments
We are grateful to all members of the DKMS Life Science Lab for their dedicated daily work that was fundamental for the analysis of all the donor samples. Furthermore, the authors would like to thank Julia Saalbach and Sandra Fritschi for their indispensable administrative assistance.
Conflict of interest
AK, KP, SK, MK, GSchö, CM, GSchä, AS, and VL are members of the DKMS Life Science Lab gGmbH, which offers commercial genotyping services.
The remaining authors declare that the research was conducted in the absence of any commercial or financial relationships that could be construed as a potential conflict of interest.
Publisher’s note
All claims expressed in this article are solely those of the authors and do not necessarily represent those of their affiliated organizations, or those of the publisher, the editors and the reviewers. Any product that may be evaluated in this article, or claim that may be made by its manufacturer, is not guaranteed or endorsed by the publisher.
Supplementary material
The Supplementary Material for this article can be found online at: https://www.frontiersin.org/articles/10.3389/fimmu.2023.1297589/full#supplementary-material
References
1. Bahram S, Bresnahan M, Geraghty DE, Spies T. A second lineage of mammalian major histocompatibility complex class I genes. Proc Natl Acad Sci (1994) 91(14):6259–63. doi: 10.1073/pnas.91.14.6259
2. Bauer S, Groh V, Wu J, Steinle A, Phillips JH, Lanier LL, et al. Activation of NK cells and T cells by NKG2D, a receptor for stress-inducible MICA. Science (1999) 285(5428):727–9. doi: 10.1126/science.285.5428.727
3. Glienke J, Sobanov Y, Brostjan C, Steffens C, Nguyen C, Lehrach H, et al. The genomic organization of NKG2C, E, F, and D receptor genes in the human natural killer gene complex. Immunogenetics (1998) 48(3):163–73. doi: 10.1007/s002510050420
4. Risti M, Bicalho M da G. MICA and NKG2D: is there an impact on kidney transplant outcome? Front Immunol (2017) 8:179. doi: 10.3389/fimmu.2017.00179
5. Robinson J, Barker DJ, Georgiou X, Cooper MA, Flicek P, Marsh SGE. IPD-IMGT/HLA database. Nucleic Acids Res (2020) 48(D1):D948–55. doi: 10.1093/nar/gkz950
6. Ashiru O, López-Cobo S, Fernández-Messina L, Pontes-Quero S, Pandolfi R, Reyburn HT, et al. A GPI anchor explains the unique biological features of the common NKG2D-ligand allele MICA*008. Biochem J (2013) 454(2):295–302. doi: 10.1042/BJ20130194
7. Ashiru O, Boutet P, Fernández-Messina L, Agüera-González S, Skepper JN, Valés-Gómez M, et al. Natural killer cell cytotoxicity is suppressed by exposure to the human NKG2D ligand MICA*008 that is shed by tumor cells in exosomes. Cancer Res (2010) 70(2):481–9. doi: 10.1158/0008-5472.CAN-09-1688
8. Schmiedel D, Mandelboim O. NKG2D ligands-critical targets for cancer immune escape and therapy. Front Immunol (2018) 9:2040. doi: 10.3389/fimmu.2018.02040
9. Zhao Y, Chen N, Yu Y, Zhou L, Niu C, Liu Y, et al. Prognostic value of MICA/B in cancers: a systematic review and meta-analysis. Oncotarget (2017) 8(56):96384–95. doi: 10.18632/oncotarget.21466
10. de Andrade LF, Tay RE, Pan D, Luoma AM, Ito Y, Badrinath S, et al. Antibody-mediated inhibition of MICA and MICB shedding promotes NK cell–driven tumor immunity. Science (2018) 359(6383):1537–42. doi: 10.1126/science.aao0505
11. Badrinath S, Dellacherie MO, Li A, Zheng S, Zhang X, Sobral M, et al. A vaccine targeting resistant tumours by dual T cell plus NK cell attack. Nature (2022) 606(7916):992–8. doi: 10.1038/s41586-022-04772-4
12. de Andrade LF, Kumar S, Luoma A, Ito Y, Alves da Silva PH, Pan D, et al. Inhibition of MICA and MICB Shedding Elicits NK cell–mediated Immunity against Tumors Resistant to Cytotoxic T cells. Cancer Immunol Res (2020) 8(6):769–80. doi: 10.1158/2326-6066.CIR-19-0483
13. Fuertes MB, Domaica CI, Zwirner NW. Leveraging NKG2D ligands in immuno-oncology. Front Immunol (2021) 12:713158. doi: 10.3389/fimmu.2021.713158
14. Matusali G, Tchidjou HK, Pontrelli G, Bernardi S, D’Ettorre G, Vullo V, et al. Soluble ligands for the NKG2D receptor are released during HIV-1 infection and impair NKG2D expression and cytotoxicity of NK cells. FASEB J (2013) 27(6):2440–50. doi: 10.1096/fj.12-223057
15. Seidel E, Dassa L, Schuler C, Oiknine-Djian E, Wolf DG, Le-Trilling VTK, et al. The human cytomegalovirus protein UL147A downregulates the most prevalent MICA allele: MICA*008, to evade NK cell-mediated killing. PloS Pathog (2021) 17(5):e1008807. doi: 10.1371/journal.ppat.1008807
16. Lanier LL. NKG2D receptor and its ligands in host defense. Cancer Immunol Res (2015) 3(6):575–82. doi: 10.1158/2326-6066.CIR-15-0098
17. Gao X, Single RM, Karacki P, Marti D, O’Brien SJ, Carrington M. Diversity of MICA and linkage disequilibrium with HLA-B in two north american populations. Hum Immunol (2006) 67(3):152–8. doi: 10.1016/j.humimm.2006.02.009
18. Fuerst D, Neuchel C, Niederwieser D, Bunjes D, Gramatzki M, Wagner E, et al. Matching for the MICA-129 polymorphism is beneficial in unrelated hematopoietic stem cell transplantation. Blood (2016) 128(26):3169–76. doi: 10.1182/blood-2016-05-716357
19. Carapito R, Jung N, Kwemou M, Untrau M, Michel S, Pichot A, et al. Matching for the nonconventional MHC-I MICA gene significantly reduces the incidence of acute and chronic GVHD. Blood (2016) 128(15):1979–86. doi: 10.1182/blood-2016-05-719070
20. Carapito R, Jung N, Untrau M, Michel S, Pichot A, Giacometti G, et al. Matching of MHC class I chain-related genes a and B is associated with reduced incidence of severe acute graft-versus-host disease after unrelated hematopoietic stem cell transplantation. Blood (2014) 124(21):664–4. doi: 10.1182/blood.V124.21.664.664
21. Carapito R, Aouadi I, Verniquet M, Untrau M, Pichot A, Beaudrey T, et al. The MHC class I MICA gene is a histocompatibility antigen in kidney transplantation. Nat Med (2022) 28, 989–98. doi: 10.1038/s41591-022-01725-2
22. Zhou X, Wang J, Zou H, Ward MM, Weisman MH, Espitia MG, et al. MICA, a gene contributing strong susceptibility to ankylosing spondylitis. Ann Rheumatic Dis (2014) 73(8):1552–7. doi: 10.1136/annrheumdis-2013-203352
23. Cortes A, Hadler J, Pointon JP, Robinson PC, Karaderi T, Leo P, et al. Identification of multiple risk variants for ankylosing spondylitis through high-density genotyping of immune-related loci. Nat Genet (2013) 45(7):730–8. doi: 10.1038/ng.2667
24. Cortes A, Gladman D, Raychaudhuri S, Cui J, Wheeler L, Brown MA. Imputation-based analysis of MICA alleles in the susceptibility to ankylosing spondylitis. Ann Rheumatic Dis (2018) 77(11):1691–2. doi: 10.1136/annrheumdis-2018-213413
25. Zhang F, Gu W, Hurles ME, Lupski JR. Copy number variation in human health, disease, and evolution. Annu Rev Genomics Hum Genet (2009) 10(1):451–81. doi: 10.1146/annurev.genom.9.081307.164217
26. Komatsu-Wakui M, Tokunaga K, Ishikawa Y, Kashiwase K, Moriyama S, Tsuchiya N, et al. MIC-A polymorphism in Japanese and a MIC-A-MIC-B null haplotype. Immunogenetics (1999) 49(7):620–8. doi: 10.1007/s002510050658
27. Komatsu-Wakui M, Tokunaga K, Ishikawa Y, Leelayuwat C, Kashiwase K, Tanaka H, et al. Wide distribution of the MICA-MICB null haplotype in East Asians. Tissue Antigens (2001) 57(1):1–8. doi: 10.1034/j.1399-0039.2001.057001001.x
28. Wang W, Tian W, Zhu F, Li L, Cai J, Wang F, et al. MICA gene deletion in 3411 DNA samples from five distinct populations in mainland China and lack of association with nasopharyngeal carcinoma (NPC) in a southern chinese han population. Ann Hum Genet (2016) 80(6):319–26. doi: 10.1111/ahg.12175
29. Shiina T, Tamiya G, Oka A, Yamagata T, Yamagata N, Kikkawa E, et al. Nucleotide Sequencing Analysis of the 146-Kilobase Segment around theIkBLandMICAGenes at the Centromeric End of the HLA Class I Region. Genomics (1998) 47(3):372–82. doi: 10.1006/geno.1997.5114
30. Tse KP, Su WH, Yang Ml, Cheng HY, Tsang NM, Chang KP, et al. A gender-specific association of CNV at 6p21.3 with NPC susceptibility. Hum Mol Genet (2011) 20(14):2889–96. doi: 10.1093/hmg/ddr191
31. Wang K, Cadzow M, Bixley M, Leask MP, Merriman ME, Yang Q, et al. A Polynesian-specific copy number variant encompassing the MICA gene associates with gout. Hum Mol Genet (2022) 31(21):3757–68. doi: 10.1093/hmg/ddac094
32. Cooper NJ, Shtir CJ, Smyth DJ, Guo H, Swafford AD, Zanda M, et al. Detection and correction of artefacts in estimation of rare copy number variants and analysis of rare deletions in type 1 diabetes. Hum Mol Genet (2015) 24(6):1774–90. doi: 10.1093/hmg/ddu581
33. Zhang A, Sun Y, Thomas D, Kawczak P, Zhang S, Askar M. Identification of three MICA alleles in the genotype of a patient with chronic lymphocytic leukemia. Tissue Antigens (2012) 79(1):64–7. doi: 10.1111/j.1399-0039.2011.01800.x
34. Lange V, Böhme I, Hofmann J, Lang K, Sauter J, Schöne B, et al. Cost-efficient high-throughput HLA typing by MiSeq amplicon sequencing. BMC Genomics (2014) 15(1):63. doi: 10.1186/1471-2164-15-63
35. Klussmeier A, Massalski C, Putke K, Schäfer G, Sauter J, Schefzyk D, et al. High-throughput MICA/B genotyping of over two million samples: workflow and allele frequencies. Front Immunol (2020) 11:314. doi: 10.3389/fimmu.2020.00314
36. Sauter J, Putke K, Schefzyk D, Pruschke J, Solloch UV, Bernas SN, et al. HLA-E typing of more than 2.5 million potential hematopoietic stem cell donors: Methods and population-specific allele frequencies. Hum Immunol (2021) 82(7):541–7. doi: 10.1016/j.humimm.2020.12.008
37. Lang K, Wagner I, Schöne B, Schöfl G, Birkner K, Hofmann JA, et al. ABO allele-level frequency estimation based on population-scale genotyping by next generation sequencing. BMC Genomics (2016) 17:374. doi: 10.1186/s12864-016-2687-1
38. Wagner I, Schefzyk D, Pruschke J, Schöfl G, Schöne B, Gruber N, et al. Allele-level KIR genotyping of more than a million samples: workflow, algorithm, and observations. Front Immunol (2018) 9:2843. doi: 10.3389/fimmu.2018.02843
39. Schöfl G, Lang K, Quenzel P, Böhme I, Sauter J, Hofmann JA, et al. 2.7 million samples genotyped for HLA by next generation sequencing: lessons learned. BMC Genomics (2017) 18:161. doi: 10.1186/s12864-017-3575-z
40. Marsh SGE, Albert ED, Bodmer WF, Bontrop RE, Dupont B, Erlich HA, et al. Nomenclature for factors of the HLA system, 2010. Tissue Antigens (2010) 75(4):291–455. doi: 10.1111/j.1399-0039.2010.01466.x
41. Marsh SGE. WHO Nomenclature Committee for Factors of the HLA System. Nomenclature for factors of the HLA system, update September 2017. HLA (2017) 90(6):391–5. doi: 10.1111/tan.13169
42. Klasberg S, Schmidt AH, Lange V, Schöfl G. DR2S: an integrated algorithm providing reference-grade haplotype sequences from heterozygous samples. BMC Bioinf (2021) 22(1):236. doi: 10.1186/s12859-021-04153-0
43. Shafin K, Pesout T, Lorig-Roach R, Haukness M, Olsen HE, Bosworth C, et al. Nanopore sequencing and the Shasta toolkit enable efficient de novo assembly of eleven human genomes. Nat Biotechnol (2020) 38(9):1044–53. doi: 10.1038/s41587-020-0503-6
44. Vaser R, Šikić M. Time- and memory-efficient genome assembly with Raven. Nat Comput Sci (2021) 1(5):332–6. doi: 10.1038/s43588-021-00073-4
45. Li H. Minimap and miniasm: fast mapping and de novo assembly for noisy long sequences. Bioinformatics (2016) 32(14):2103–10. doi: 10.1093/bioinformatics/btw152
46. Vaser R, Sović I, Nagarajan N, Šikić M. Fast and accurate de novo genome assembly from long uncorrected reads. Genome Res (2017) 27(5):737–46. doi: 10.1101/gr.214270.116
47. Koren S, Walenz BP, Berlin K, Miller JR, Bergman NH, Phillippy AM. Canu: scalable and accurate long-read assembly via adaptive k-mer weighting and repeat separation. Genome Res (2017) 27(5):722–36. doi: 10.1101/gr.215087.116
48. Norman PJ, Norberg SJ, Guethlein LA, Nemat-Gorgani N, Royce T, Wroblewski EE, et al. Sequences of 95 human MHC haplotypes reveal extreme coding variation in genes other than highly polymorphic HLA class I and II. Genome Res (2017) 27(5):813–23. doi: 10.1101/gr.213538.116
49. Houwaart T, Scholz S, Pollock NR, Palmer WH, Kichula KM, Strelow D, et al. Complete sequences of six major histocompatibility complex haplotypes, including all the major MHC class II structures. HLA (2023) 102(1):28–43. doi: 10.1111/tan.15020
50. Bailey JA, Yavor AM, Massa HF, Trask BJ, Eichler EE. Segmental duplications: organization and impact within the current human genome project assembly. Genome Res (2001) 11(6):1005–17. doi: 10.1101/gr.187101
51. Bailey JA, Gu Z, Clark RA, Reinert K, Samonte RV, Schwartz S, et al. Recent segmental duplications in the human genome. Science (2002) 297(5583):1003–7. doi: 10.1126/science.1072047
52. Carvalho CMB, Lupski JR. Mechanisms underlying structural variant formation in genomic disorders. Nat Rev Genet (2016) 17(4):224–38. doi: 10.1038/nrg.2015.25
53. Myers S, Freeman C, Auton A, Donnelly P, McVean G. A common sequence motif associated with recombination hot spots and genome instability in humans. Nat Genet (2008) 40(9):1124–9. doi: 10.1038/ng.213
54. Baudat F, Buard J, Grey C, Fledel-Alon A, Ober C, Przeworski M, et al. PRDM9 is a major determinant of meiotic recombination hotspots in humans and mice. Science (2010) 327(5967):836–40. doi: 10.1126/science.1183439
55. Jiang W, Johnson C, Jayaraman J, Simecek N, Noble J, Moffatt MF, et al. Copy number variation leads to considerable diversity for B but not A haplotypes of the human KIR genes encoding NK cell receptors. Genome Res (2012) 22(10):1845–54. doi: 10.1101/gr.137976.112
56. Aida K, Russomando G, Kikuchi M, Candia N, Franco L, Almiron M, et al. High frequency of MIC null haplotype (HLA-B48-MICA-del-MICB*0107 N) in the Angaite AmerIndian community in Paraguay. Immunogenetics (2002) 54(6):439–41. doi: 10.1007/s00251-002-0485-1
57. Tokunaga K, Ohashi J, Bannai M, Juji T. Genetic link between Asians and native Americans: evidence from HLA genes and haplotypes. Hum Immunol (2001) 62(9):1001–8. doi: 10.1016/S0198-8859(01)00301-9
58. Gonzalez-Galarza FF, McCabe A, Santos EJMD, Jones J, Takeshita L, Ortega-Rivera ND, et al. Allele frequency net database (AFND) 2020 update: gold-standard data classification, open access genotype data and new query tools. Nucleic Acids Res (2020) 48(D1):D783–8. doi: 10.1093/nar/gkz1029
59. Solloch UV, Giani AS, Pattillo Garnham MI, Sauter J, Bernas SN, Lange V, et al. HLA allele and haplotype frequencies of registered stem cell donors in Chile. Front Immunol (2023) 14:1175135. doi: 10.3389/fimmu.2023.1175135
60. Newbound C, Shamsuddin D, Kolanski S, Olafson L, Goodridge D, Lind C. P85: Deletions in MICA demonstrate haplotype diversity within the major histocompatibility complex (MHC) and possible association with specific HLA-C~B alleles. HLA (2022) 99(5):416–550. doi: 10.1111/tan.14606
61. Liu P, Lacaria M, Zhang F, Withers M, Hastings PJ, Lupski JR. Frequency of nonallelic homologous recombination is correlated with length of homology: evidence that ectopic synapsis precedes ectopic crossing-over. Am J Hum Genet (2011) 89(4):580–8. doi: 10.1016/j.ajhg.2011.09.009
62. Lam KWG, Jeffreys AJ. Processes of de novo duplication of human α-globin genes. Proc Natl Acad Sci (2007) 104(26):10950–5. doi: 10.1073/pnas.0703856104
63. Berg IL, Neumann R, Lam KWG, Sarbajna S, Odenthal-Hesse L, May CA, et al. PRDM9 variation strongly influences recombination hot-spot activity and meiotic instability in humans. Nat Genet (2010) 42(10):859–63. doi: 10.1038/ng.658
64. Wu X, Wang G, Zhang L, Xu H. Genetics of ankylosing spondylitis—Focusing on the ethnic difference between east asia and europe. Front Genet (2021) 12:863. doi: 10.3389/fgene.2021.671682
65. Cortes A, Pulit SL, Leo PJ, Pointon JJ, Robinson PC, Weisman MH, et al. Major histocompatibility complex associations of ankylosing spondylitis are complex and involve further epistasis with ERAP1. Nat Commun (2015) 6(1):7146. doi: 10.1038/ncomms8146
Keywords: MICA, copy number variation, nonallelic homologous recombination, NAHR, linkage, population frequency, haplotype, HLA-B27
Citation: Klussmeier A, Putke K, Klasberg S, Kohler M, Sauter J, Schefzyk D, Schöfl G, Massalski C, Schäfer G, Schmidt AH, Roers A and Lange V (2023) High population frequencies of MICA copy number variations originate from independent recombination events. Front. Immunol. 14:1297589. doi: 10.3389/fimmu.2023.1297589
Received: 20 September 2023; Accepted: 24 October 2023;
Published: 15 November 2023.
Edited by:
Martin Maiers, National Marrow Donor Program, United StatesReviewed by:
Raphael Carapito, Université de Strasbourg, FranceFaming Zhu, Blood Center of Zhejiang Province, China
Steven Thomas Cox, Anthony Nolan, United Kingdom
Copyright © 2023 Klussmeier, Putke, Klasberg, Kohler, Sauter, Schefzyk, Schöfl, Massalski, Schäfer, Schmidt, Roers and Lange. This is an open-access article distributed under the terms of the Creative Commons Attribution License (CC BY). The use, distribution or reproduction in other forums is permitted, provided the original author(s) and the copyright owner(s) are credited and that the original publication in this journal is cited, in accordance with accepted academic practice. No use, distribution or reproduction is permitted which does not comply with these terms.
*Correspondence: Anja Klussmeier, a2x1c3NtZWllckBka21zLWxhYi5kZQ==