- 1Fisheries College, Zhejiang Ocean University, Zhoushan, China
- 2Key Laboratory of Mariculture and Enhancement, Zhejiang Marine Fisheries Research Institute, Zhoushan, China
Soybean meal (SBM) is an acceptable replacement for unsustainable marine fish meal (FM) in aquaculture. However, we previously reported that high dietary SBM supplementation causes intestinal inflammatory injury in yellow drum (Nibea albiflora). Accordingly, a 4-week SBM-induced enteritis (SBMIE) in yellow drum trial was conducted first, followed by a 4-week additive-supplemented reparative experiment to evaluate the reparative effect of five additives on SBMIE in yellow drum. The control diet comprised 50% FM protein substituted with SBM. The additive-supplemented diet was added with 0.02% curcumin (SBMC), 0.05% berberine (SBM-BBR), 0.5% tea polyphenols (SBM-TPS), 1% taurine (SBM-TAU), or 0.8% glutamine (SBM-GLU) based on the control diet, respectively. The weight gain (WG), specific growth rate (SGR), feed efficiency ratio (FER), and survival rate (SR) of fish fed the additive-supplemented diets were significantly higher than those of fish fed the SBM diet. The WG, SGR, and FER of fish fed the SBMC, SBM-GLU and SBM-TAU diets were significantly higher than those of fish fed other diets. Moreover, fish fed the additive-supplemented diets SBMC and SBM-GLU, exhibited significantly increased intestinal villus height (IVH), intestinal muscular thickness (IMRT), and intestinal mucosal thickness (IMLT) and significantly decreased crypt depth (CD) in comparison with those fed the SBM diets. The relative expression of intestinal tight junction factors (ocln, zo1), cytoskeletal factors (f-actin, arp2/3), and anti-inflammatory cytokines (il10, tgfb) mRNA was remarkably elevated in fish fed additive-supplemented diets than those of fish fed the SBM diet. Whereas, the relative expression of intestinal myosin light chain kinase (mlck) and pro-inflammatory cytokines (il1, il6, tnfa) mRNA was markedly lower in fish fed the additive-supplemented diets. The highest relative expression of intestinal ocln, f-actin, and arp2/3 and the lowest relative expression of intestinal mlck were found in fish fed the SBMC diet. Hence, all five dietary additives effectively repaired the intestinal injury induced by SBM, with curcumin exhibiting the strongest repair effect for SBMIE in yellow drum.
1 Introduction
Soybean meal (SBM) is a good-quality plant protein source commonly used as a substitution to unsustainable fish meal (FM) in aquaculture feed because of its reasonable price, security of supply, and sustainability (1). However, the various anti-nutritional factors and unbalanced amino acid content in SBM in fish feed (2) could lead to a pro-inflammatory response in the distal intestine of carnivorous fish (3–7). Similarly, we previously reported that substituted of 45% FM protein with SBM protein reduced growth performance and induced intestinal inflammation in yellow drum (Nibea albiflora) (8). The primary associated manifestations include shortened intestinal mucosal folds, swollen of the lamina propria and subepithelial mucosa, and intense infiltration of inflammatory cells, which, collectively, can reduce the nutrient absorption capacity of the intestine and decrease growth performance (5, 9). Hence, it is particularly important to develop strategies to minimize intestinal injury caused by high proportions of SBM supplementation in marine fish.
Countermeasures, including enhancing processing technology and cultivating soybean protein-tolerant fish varieties, have been implemented for the widely use of SBM in cultivated fish varieties (10). Functional feed additives have also been served to alleviate SBM-induced enteropathy (SBMIE) in fish (11). These additives are nutritional/non-nutritional compounds provided in fish diets that enhance the physicochemical properties of diets or the performance of the target species (12, 13). Previous research suggests that a myriad of additives exhibit beneficiary properties such as anti-inflammatory, antioxidant, and immunomodulatory and may thus have the capacity to relieve SBMIE symptoms by strengthening the anti-inflammatory and immune responses of fish (14–16). Curcumin and berberine are both traditional herbal extracts, which can enhance intestinal villus development in Nile tilapia (Oreochromis niloticus) (17) and protect the intestinal barrier function of fish by regulating the intestinal microbiota (18). Meanwhile, tea polyphenols are plant extracts, which can attenuate the impairment of intestinal barrier function in fish by upregulating the expression of tight junction proteins and adhesion junction proteins (19). Taurine and glutamine are important functional amino acids, which has immune-enhancing and systemic nitrogen balance maintaining properties. Besides, taurine can improve intestinal homeostasis by regulating intestinal microbiota in mouse (20). Glutamine enhanced the intestinal tissue oxygenation and/or brush barrier function, as well as altered inflammatory processes in red drum (Sciaenops ocellatus) (21). Hence, all of these additives have great potential in the treatment of intestine injury in fish.
Yellow drum (N. albiflora) has become an important species for marine aquaculture in the East China Sea region due to its taste, rich nutrient content, and rapid growth (22). The successful artificial breeding of yellow drums has enabled their intensive cultivation, particularly in the coastal provinces of eastern China. We previously found that high dietary SBM supplementation causes intestinal inflammatory injury in yellow drum (8). Hence, in this study, we evaluated the effects of curcumin, berberine, tea polyphenols, glutamine, and taurine as potential feed additives on growth performance, whole-body nutritional composition, intestinal structure, and immune-related gene expression in yellow drum fed a high proportion of SBM diets. Collectively, this study can provide an effective solution for repairing intestinal injuries induced by SBM in marine fish.
2 Materials and methods
2.1 Experimental diets
In the current study, six isonitrogenous (49.95%) and isolipid (12.50%) diets were formulated using FM and SBM as the primary protein sources, fish oil and soybean oil as the primary lipid sources, and wheat flour as the carbohydrate source, according to the formulas described by Wang et al. (2016) (23). In the control diet, 50% of the FM protein was substituted with SBM protein (Table 1), whereas the other five treatment diets were supplemented with 0.02% curcumin (SBMC), 0.05% berberine (SBM-BBR), 0.5% tea polyphenols (SBM-TPS), 1% taurine (SBM-TAU), or 0.8% glutamine (SBM-TAU) based on the control diet.
2.2 Experimental fish and feeding management
Juvenile yellow drums obtained from the research station of the Marine Fisheries Institute of Zhejiang Province (Xixuan Island, Zhoushan, Zhejiang Province, China) were acclimated in an indoor flow-through aquaculture system for two weeks before initiating the feeding trial. A total of 450 yellow drum juveniles (initial weight: 6.65 ± 0.02 g) were transferred to 18 cylindrical fiberglass tanks (capacity: 1000 L) with 25 individuals per tank. Diets were randomly assigned to tanks in triplicates. The experiment was divided into two stages: week 1–4, when all fish were fed the SBM diet to induce intestinal inflammation; and week 4–8, when all fish, excluding the control group, were fed the additive-supplemented diets. All fish were fed twice daily at 7:00 and 14:00. The following parameters were maintained throughout the experimental period: temperature, 26–28 °C; salinity, 27.0 ± 1.0 g/L; unionized ammonia nitrogen< 0.05 mg/L; dissolved oxygen > 6.0 mg/L. The fish tanks were cleaned every two weeks, at which point the fish were removed and weighed to adjust the feeding amount.
2.3 Sample collection
After the feeding experiment, the fish were fasted for 24 h, the total number of fish in each tank was counted, and the survival rate (SR) was calculated. The weight of each tank fish was measured and then the weight gain rate (WG) and specific growth rate (SGR) of the fish were calculated. Fish were anesthetized with MS-222, and 12 individuals were randomly selected from each tank, and their total length, body length, and body weight were measured to calculate the condition factor (CF). Appropriately sized posterior intestinal tissue was cleaned with phosphate-buffered saline (PBS), and excess connective tissue was removed and fixed in 4% paraformaldehyde solution. Hindgut tissues were immersed in lyophilized vials containing RNA preservation solution (Solarbio, Beijing, China), flash-frozen in liquid nitrogen, and subsequently transferred to a −80°C refrigerator for storage. In addition, three fish were selected from each tank for the whole-body analysis.
2.4 Chemical analysis
The nutritional compositions of the experimental diets and whole fish were determined using the AOAC (1995) method. The samples were dried to a constant weight at 105 °C in a drying oven to measure the moisture content. Crude protein content was determined using a Kjeldahl nitrogen tester (BUCHI, KjeIFIex K-360, Switzerland). The crude fat content was determined using a Soxhlet extractor (FOSS Soxtec-2055, Sweden); the samples were heated in an electric furnace until they were smokeless and then cauterized to constant weight (4 h) using a Muffle furnace at 550°C to determine the ash content.
2.5 Histological analysis
The intestines and liver of three fish from each tank were collected for histometric evaluation. The fixed hindgut and liver samples were collected and dehydrated using a fully automated dehydrator. The dehydrated tissues were paraffin-embedded, and transversely cut into 5–6 μm tissue sections with a microtome and dried overnight.
The H&E (hematoxylin and eosin) staining process was divided into the following steps: dewaxing, staining, dehydration, transparency, and sealing. The sealed sections were imaged using a 250 FLASH digital pathology system (3DHISTECH, Hungary). Image-Pro Plus 6.0 software (Media Cybernetics) was used to analyze the intestinal villus height (IVH), intestinal muscle thickness (IMRT), intestinal mucosal thickness (IMLT), and crypt depth (CD) in each group (7, 24, 25).
2.6 RNA extraction and real‐time quantitative polymerase chain reaction
Total RNA was extracted and purified from distal intestinal tissue samples (approximately 50 mg) using the R1200 Total RNA Extraction Kit (Solarbio, Beijing, China) according to the manufacturer’s instructions. RNA concentration and purity were determined using an ND-2000 spectrophotometer (NanoDrop 2000; Wilmington, DE, USA). OD260/280 values between 1.8 and 2.0 indicated high-quality RNA samples, and the relative amounts of RNA and its integrity were detected by electrophoresis. The cDNA was synthesized with the PrimeScript RT reagent Kit with gDNA Eraser Kit (TaKaRa, Japan) and stored at −20°C for future use.
Primers were designed using Primer 3 (https://primer3.ut.ee/) based on the sequences of yellow drum-related genes in the yellow drum transcriptome (the target genes are shown in Table 2), and the primers were synthesized by the Zhejiang Shangya Biological Company. RT-qPCR was performed using TransStart Tip Green qPCR SuperMix (All Style Gold, Beijing, China) on a StepOnePlus real-time PCR system (Thermo Fisher Scientific, USA). The constitutively expressed ribosomal protein actb, which was shown to be stably expressed in the yellow drum, was selected as the housekeeping gene for sample normalization. After the values were normalized to actb, the fold change in transcript levels was determined by the relative quantitative method (2−ΔΔCT).
2.7 Calculations and statistical analysis
The following variables were calculated:
Survival rate (SR, %).
Weight gain (WG, %).
Specific growth rate (SGR per day, %/d).
Feed efficiency ratio (FER, %).
Hepatosomatic index HSI (%).
Viscerosomatic index (VSI, %).
Condition factor CF (g/cm3).
where Wt and W0 represent the final and initial fish weights, respectively; Nt and N0 are the final and initial numbers of fish in each tank, respectively; and t represents the trial period in days.
Data were analyzed by one-way analysis of variance (ANOVA) using SPSS23.0 software, Duncan’s multiple comparisons were performed when the data were normally distributed within groups, and a P< 0.05 was considered statistically significant. The experimental results were expressed as mean ± standard error (mean ± SEM).
3 Results
3.1 Growth, survival, and morphological indices
The fish fed additive-supplemented diets had remarkably elevated WG, SGR, SR, and FER than those of fish fed the SBM diet. The WG and SGR of fish fed SBM-TPS or SBM-BBR were remarkably lower than those of fish fed SBMC, SBM-GlU, or SBM-TAU(P< 0.05; Table 3).
The HSI and VSI differed significantly among the diets. The HSI of fish fed the SBM-BBR, SBM-TPS, and SBM-TAU diets were remarkably lower than that of fish fed the SBM diet (P< 0.05), whereas the HSI of fish fed the SBMC and SBM-GLU diets did not differ significantly from that of fish fed the SBM diet (P > 0.05). The VSI was significantly higher in fish fed the SBM-GLU diet than in those fed the SBM diet, and fish fed the SBM-TAU diet had significantly lower VSI than those fed the SBM diet.There were no significant differences between fish fed SBMC, SBM-BBR, or SBM-TPS diets and those fed SBM diets. The CF was not significantly different among the groups. (P< 0.05; Table 3).
3.2 Fish body composition
Excluding the fish in the SBM-GLU group, the fish fed the additive-supplemented diets had a remarkably lower moisture content than that of fish fed the SBM diet. The crude protein and lipid contents of fish fed the additive-supplemented diets, excluding the SBMC diet, were remarkably higher than those fed the control diet (P< 0.05; Table 4), whereas the crude lipid content of fish fed the SBMC diet did not differ significantly from that of fish fed the SBM diet (P > 0.05). Moreover, there was no significant difference in crude ash content between fish fed the additive-supplemented diets and those fed the SBM diet (P > 0.05).
3.3 Muscle amino acid composition analysis
The fish fed the additive-supplemented diets (except for the SBMC diet) had remarkably increased essential amino acid (EAA), non-essential amino acid (NEAA), and total amino acid (TAA) contents than in those fed the SBM diet (P< 0.05; Table 5). The TAA contents of fish fed the SBM-GLU and SBM-BBR diets were the highest.
3.4 Distal intestinal and liver micromorphology
Yellow drums fed the SBM diet exhibited intestinal fold atrophy and shortening, breakage, and detachment, enlargement of the intrinsic layer in the intestinal folds, and deepen the crypt depth, typical of SBMIE in the end of 8-week feeding trial. However, the intestinal morphology of the yellow drum significantly improved after consumption of the additive-supplemented diet (Figure 1). IVH, IMRT, and IMLT were significantly increased, and CD was remarkably decreased in fish fed an additive-supplemented diet, particularly the SBMC or SBM-GLU diets (P< 0.05; Table 6).
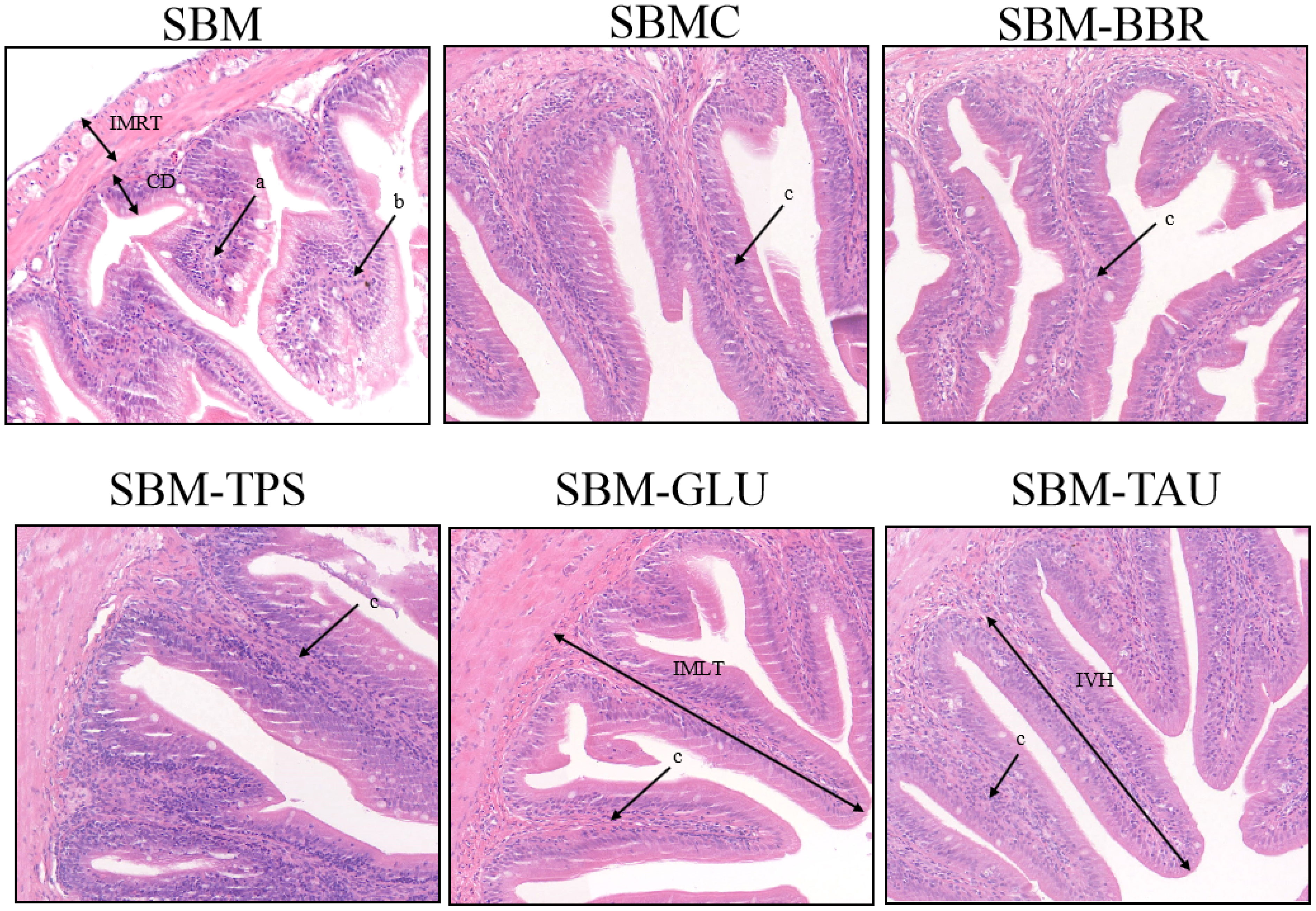
Figure 1 Representative histomorphological images from hematoxylin and eosin (H&E)-stained sections of the distal intestine. Scale Bar: 50 μm; (a) Widening of the lamina propria of the intestinal villi; (b) Intestinal villi atrophied, shortened; (c) intestinal villi are elongated and the lamina propria is thin; IVH, Intestinal villus height; IMRT, Intestinal muscular thickness; IMLT, Intestinal mucosal thickness; CD, Crypt depth.
A proportion of the hepatocytes from fish fed the SBM diet exhibited irregular geometry, severe vacuolization, intracellular localized nuclei, and solidification, whereas others lacked nuclei and exhibited broken cellular structures (Figure 2A). In contrast, fish fed the additive-supplemented diets exhibited markedly improved liver morphology, as evidenced by reduced cellular vacuolization, with most nuclei being centrally located and with intact cellular structures (Figures 2B–F). The repair effect was the most significant in the SBMC group.
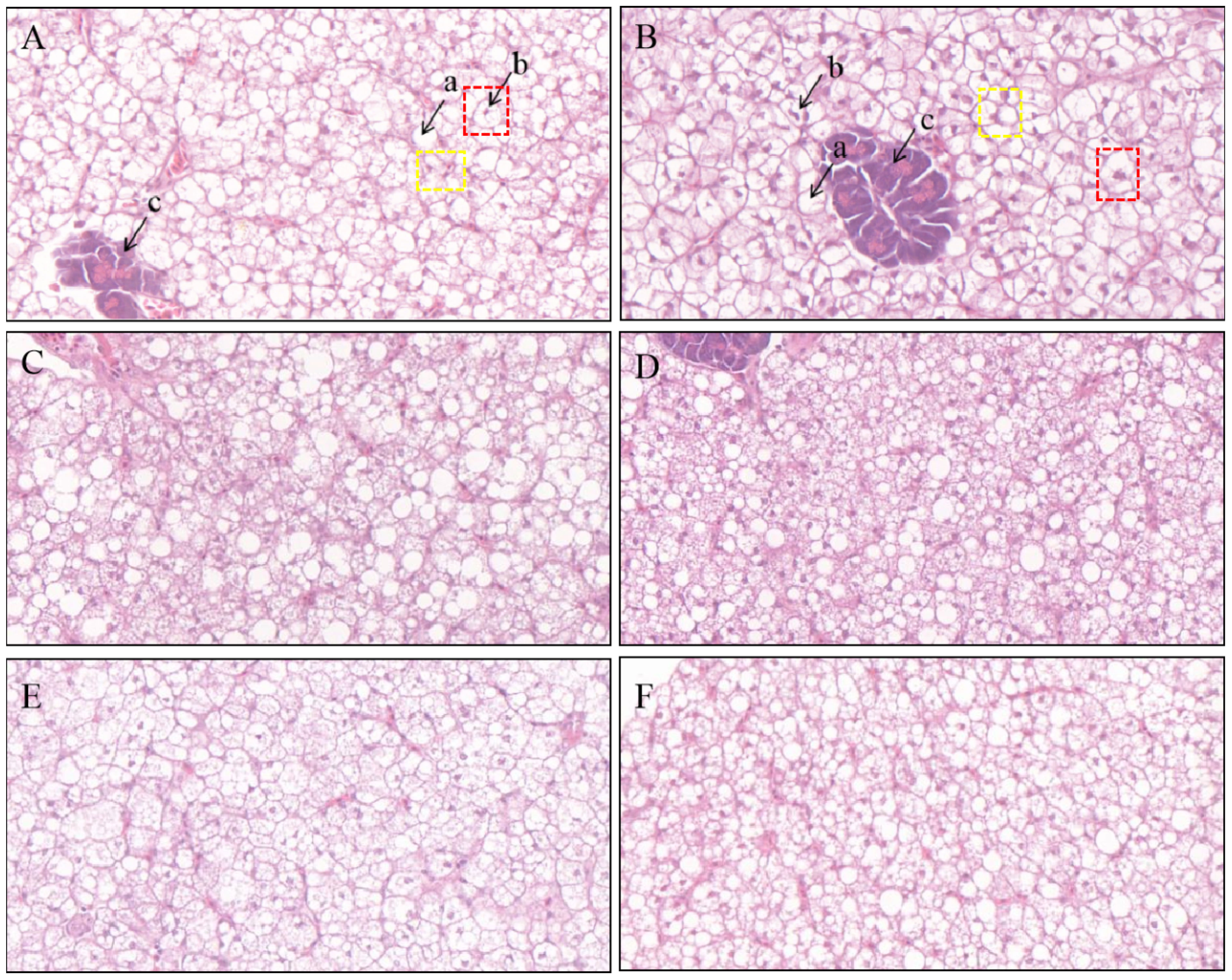
Figure 2 Representative histomorphological images from hematoxylin and eosin (H&E) stained sections of the liver. Scale Bar: 20 μm; (A) SBM; (B) SBMC; (C) SBM-BBR; (D) SBM-TPS; (E) SBM-GLU; (F) SBM-TAU; a: vacuolation; b: nucleus; c: portal vein; Red box: Normal liver cells; Yellow box: Liver cells with shifted nuclei.
3.5 Distal intestinal physical barrier-related gene expression
Overall, the expression of il1, il6, and tnfa mRNAs was significantly downregulated, whereas that of il10 and tgfb was significantly upregulated in the distal intestines of fish fed the additive-supplemented diets than that of fish fed the SBM diet (P< 0.05; Figures 3A, B). Whereas, no significant difference was observed in il6 expression or a decrease in il10 mRNA expression in fish fed SBM-BBR. Moreover, there was an elevated expression levels of tnfa mRNA but no significant different expression level of il10 mRNA in the SBM-TPS diet than those of fish fed the SBM diet (Figures 3A, B).
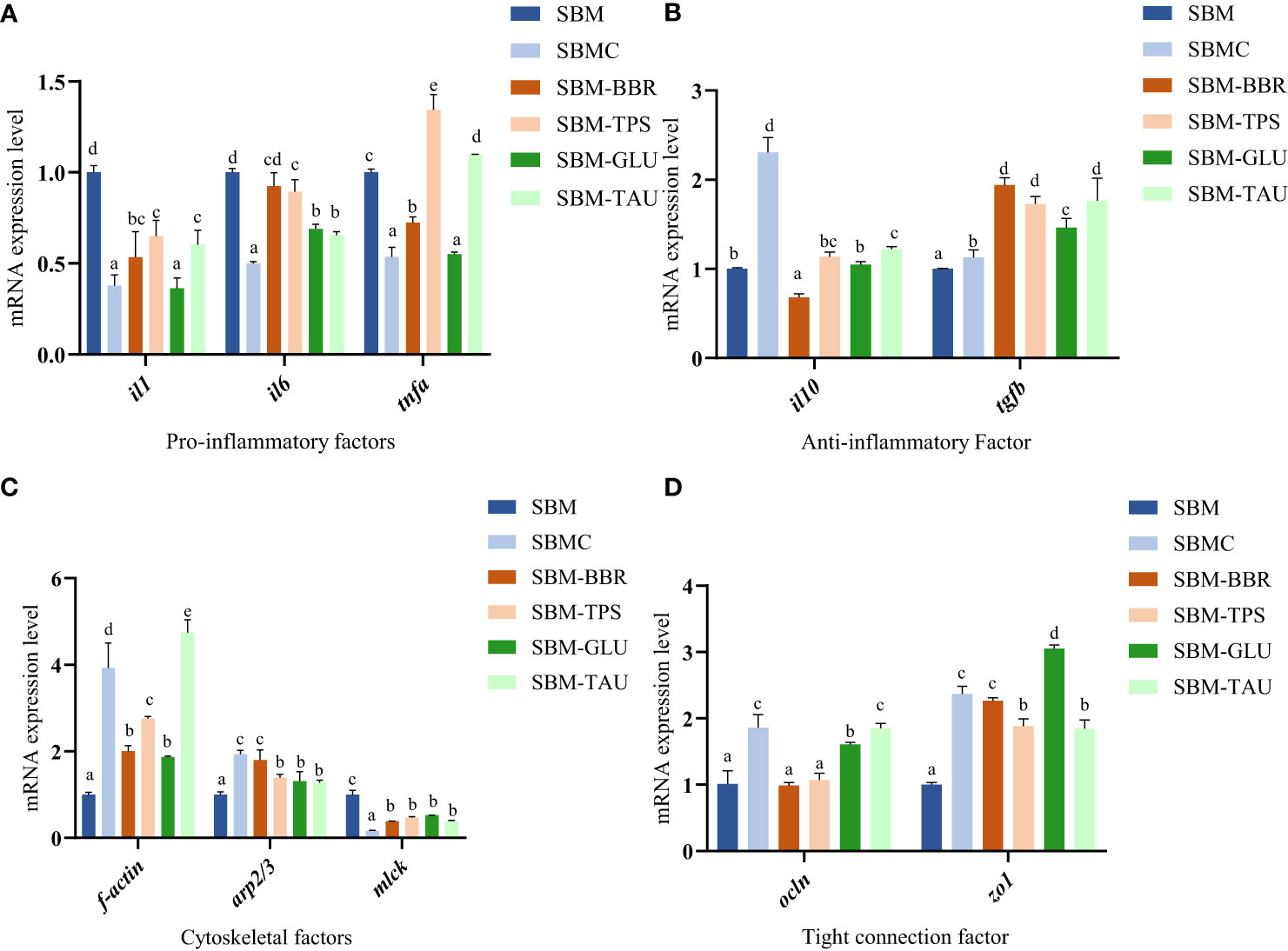
Figure 3 Relative mRNA expression of intestinal mucosal immunological barrier-related proteins in the distal intestine. Data represent as mean ± SEM (n = 3). According to the one-way ANOVA, values in the same row with different superscripts were significantly different (P< 0.05). SEM: standard error of the mean. il1, interleukin 1; il6, interleukin 6; tnfa, tumor necrosis factor alpha; il10, interleukin 10; tgfb, transforming growth factor β; f-actin, filamentous actin; arp2/3, arp2/3 complex; mlck, myosin light chain kinase; ocln, occludin; zo1, tight junction protein.
The mRNA expression levels of filamentous actin (f-actin), arp2/3 complex (arp2/3), and occludin (ocln) were remarkably higher in fish fed the additive-supplemented diets (P< 0.05; Figure 3C) than in the SBM-fed group. In particular, the increase in ocln expression was more significant in fish fed SBMC, SBM-GLU, and SBM-TAU diets. Moreover, the zonula occludens-1 (zo1) mRNA expression was significantly higher, whereas that of myosin light-chain kinase (mlck) was remarkably lower in fish fed the additive-supplemented diets than that of fish fed the SBM diet (P< 0.05; Figure 3D).
4 Discussion
In the present study, we evaluated the effectiveness of five feed additives on the repair of SBMIE in yellow drums by assessing the growth performance, intestinal histological morphology, and mRNA expression levels of intestinal barrier-related factors. High levels of SBM in feed can induce intestinal inflammation and injury in carnivorous fish, reducing the ability of the intestinal epithelium to uptake nutrients, and ultimately decreasing fish growth performance (2). However, the growth and survival of fish fed a high proportion of soybean meal diet can be significantly improved through the supplementation of functional feed additives (26, 27). In this study, the growth performance of fish fed diets provided with various additives were remarkably improved compared to those fed the SBM diet. The WG, SGR, and FER of the yellow drum fed SBMC, SBM-GlU, and SBM-TAU diets were the highest. This may be due to the additives reducing intestinal inflammation and increasing intestinal villus length and absorption area. Long villus are related to fine intestinal fitness, nutrient beneficiation, and uptake efficiency, thus improving growth performance (28, 29). This is further corroborated by the histomorphometric measurements of the intestinal tract from this study.
Replacing a significant proportion of FM protein with plant proteins can affect fish intestinal health and reduce crude protein and lipid contents, effectively diminishing the nutritional value of fish (30, 31). However, previous research has indicated that additives supplemented in high plant protein replacing fish meal diet can significantly improve fish body composition (21, 32). In the present study, the whole-body crude protein and crude lipid contents of fish fed the additive-supplemented diets were remarkably higher, whereas the moisture content was significantly lower than that of fish fed the SBM diet. This is likely due to additives that enhance the conversion of ingested food into whole-body proteins and lipids by improving intestinal morphology.
Assessing the changes in intestinal morphology is the most common and direct method for evaluating intestinal barrier function (33). Dietary SBM-induced pro-inflammatory responses are caused by injury to the physical barrier of the intestinal mucosa, subsequently exposing the otherwise protected layers of the mucosa to luminal ingredients, including pathogenic bacteria or other food antigens, thereby exacerbating the enteritis (34). Moreover, the intestine is intricately connected to the liver in terms of immunity and digestion; hence, intestinal injury can lead to liver injury, following the intestine’s exposure to harmful bacteria and metabolites (35–37). Nonetheless, the physical barrier of the intestinal mucosa can be improved by the ingestion of dietary additives that serve to repair intestinal injury (38, 39). As evidenced by the intestinal morphological indicators, the additives selected for this study significantly improved the intestinal integrity of yellow drums, which was characterized by increases in IVH, IMRT, IMLT, and CD. Similar results have been observed in Epinephelus lanceolatus (40) and Oreochromis niloticus (14), corroborating that additive-supplemented diets elicit reparative effects on the intestine injury of the yellow drum. Additionally, the degree of liver injury in fish was markedly ameliorated by the feed additives, which may be related to the repair of the intestinal physical barrier.
To further explore the reparative effects of the five selected additives on SBMIE, we assessed the mRNA expression levels of the intestinal barrier and immune-related genes. Intestinal tight junction proteins and cytoskeletal factors are crucial for protecting the integrity and function of the intestinal barrier, acting as epithelial barriers, and protecting the intestinal tract from viruses and harmful bacteria (41). Hence, intestinal tight junction injury increases the chance of intestinal inflammation. Multiprotein tight junction complexes include occludin, claudin, and ZOs (42–44). In the present study, the additives remarkably increased the mRNA expression levels of tight junction factors (ocln, zo1) in the intestine of yellow drum, while reducing that of mlck. Notably, mlck phosphorylates MLC and regulates tight junction permeability (45, 46). Hence, mlck downregulation likely reduced intestinal permeability and improved the symptoms of SBMIE. The cytoskeleton is central to maintaining cellular function and structure, and regulating adherens junctions (AJ) (47, 48). In this study, the mRNA expression of cytoskeletal factors (f-actin and arp2/3) increased in the intestines of yellow drums fed additive-supplemented diets. The upregulation of intestinal tight junctions and cytoskeletal factors suggests that intestinal barrier injury is repaired, thereby reducing the risk of intestinal inflammation (49). This is consistent with findings in other marine fish (50).
Inflammatory and anti-inflammatory cytokines secreted by the intestinal immune effector cells mediate the intestinal inflammatory response. Although proinflammatory cytokines boost the differentiation of T and B cells, leading to inflammation (51), anti-inflammatory cytokines may also reduce the probability of intestinal inflammation (52). The pro-inflammatory factors Il-1 and Tnf-α act as upstream signals for other pro-inflammatory factors, promoting their production. In contrast, Il-10 and Tgf-β are key factors in preventing enteritis development (53). The results of the current study indicated that the expression of il1, il6, and tnfa mRNA was remarkably downregulated, and il10 and tgfb were remarkably upregulated in fish fed the additive-supplemented diets compared to those fed the SBM diet. This may be due to the additives regulating immune response signaling pathways (Liu et al., 2018), which is in line with the findings of Giri et al. (2019) and Cheng et al. (2011) (26, 54, 55). However, fish fed the SBM-BBR and SBM-TPS diets did not exhibit significant changes in anti-inflammatory or pro-inflammatory cytokine levels, potentially due to the limited repair effect of berberine and tea polyphenols on yellow drum SBMIE, which resulted in incomplete intestinal injury repair.
In summary, the additives selected for this study elicited reparative effects on SBMIE symptoms in yellow drum. The SBMC diet (supplemented with curcumin) had the most reparative effect on growth performance, intestinal morphology, and gene expression of yellow drum. It is speculated that the selected additives enhance the immune function of yellow drum by regulating anti-inflammatory and pro-inflammatory factors, and improve intestinal morphology and nutrient absorption by elevating the expression of intestinal barrier related genes in yellow drum, thereby enhancing the growth performance of yellow drum and increasing its tolerance to high-level soybean meal feed. However, the specific mechanism of the reparative effects of dietary additives in treating SBMIE in yellow drum should be studied further.
Data availability statement
The original contributions presented in the study are included in the article/supplementary material. Further inquiries can be directed to the corresponding authors.
Ethics statement
The animal studies were approved by Zhejiang Ocean University’s Committee on Ethics of Animal Experiments. The studies were conducted in accordance with the local legislation and institutional requirements. Written informed consent was obtained from the owners for the participation of their animals in this study.
Author contributions
SM: Writing – original draft, Writing – review & editing. LW: Writing – original draft, Writing – review & editing. YZ: Writing – original draft. PT: Writing – original draft. RC: Writing – original draft. WH: Writing – original draft. DX: Writing – review & editing, Writing – original draft. HX: Writing – review & editing.
Funding
The author(s) declare financial support was received for the research, authorship, and/or publication of this article. This research was supported by grants from the National Natural Science Foundation of China (No. 31972785), the Distinguished Youth Fund of Zhejiang Province (No. LR21C190001), the Science and Technology Planning Project of ZhouShan city (No.2023C31040) and the Science and Technology Planning Project of Zhejiang Marine Fisheries Research Institute (No.HYS-CZ-202305).
Conflict of interest
The authors declare that the research was conducted in the absence of any commercial or financial relationships that could be construed as a potential conflict of interest.
Publisher’s note
All claims expressed in this article are solely those of the authors and do not necessarily represent those of their affiliated organizations, or those of the publisher, the editors and the reviewers. Any product that may be evaluated in this article, or claim that may be made by its manufacturer, is not guaranteed or endorsed by the publisher.
References
1. Sumon H, Rukshana KS, Subroto S, Sheikh MB, Mohammad LA, Jewel H, et al. Effects of dietary replacement of fish meal by soybean meal on growth, feed utilization, and health condition of stinging catfish, Heteropneustes fossilis. Saudi J Biol Sci (2023) 30:3. doi: 10.1016/j.sjbs.2023.103601
2. Buttle LG, Burrells AC, Good JE, Williams PD, Southgate PJ, Burrells C. The binding of soybean agglutinin (SBA) to the intestinal epithelium of Atlantic salmon, Salmo salar and rain-bow trout, Oncorhynchus mykiss, fed high levels of soybean meal. Vet Immunol Immunopathol (2001) 80:237–44. doi: 10.1016/S0165-2427(01)00269-0
3. Urán PA, Gonçalves AA, Taverne-Thiele JJ, Schrama JW, Verreth JAJ, Rombout JHWM. Soybean meal induces intestinal inflammation in common carp (Cyprinus carpio L.). Fish Shellfish Immunol (2008) 25:751–60. doi: 10.1016/j.fsi.2008.02.013
4. Lim SJ, Lee KJ. Partial replacement of fish meal by cotton-seed meal and soybean meal with iron and phytase supplementation for parrot fish Oplegnathus fasciatus. Aquaculture (2009) 290:283–9. doi: 10.1016/j.aquaculture.2009.02.018
5. Inderjit SM, Elvis MC, Elin CV, Åshild K, Anne MB. Transcriptional regulation of IL-17A and other inflammatory markers during the development of soybean meal-induced enteropathy in the distal intestine of Atlantic salmon (Salmo salar L.). Cytokine (2012) 60:186–96. doi: 10.1016/j.cyto.2012.05.027
6. Hedrera MI, Galdames JA, Jimenez-Reyes MF, Reyes AE, Avendaño-Herrera R, Romero J, et al. Soybean meal induces intestinal inflammation in zebrafish larvae. PloS One (2013) 8:e69983. doi: 10.1371/journal.pone.0069983
7. Gu M, Bai N, Zhang YQ, Åshild K. Soybean meal induces enteritis in turbot Scophthalmus maximus at high supplementation levels. Aquaculture (2016) 464:286–95. doi: 10.1016/j.aquaculture.2016.06.035
8. Wu X, Wang LG, Xie QP, Tan P. Effects of dietary sodium butyrate on growth, diet conversion, body chemical compositions and distal intestinal health in yellow drum (Nibea albiflora, Richardson). Aquacult Res (2020) 51:69–79. doi: 10.1111/are.14348
9. Deng J, Mai K, Ai QH, Zhang WB, Wang Xj, Xu W, et al. Effects of replacing fish meal with soy protein concentrate on feed intake and growth of juvenile Japanese flounder, Paralichthys Olivaceus. Aquaculture (2006) 258:503–13. doi: 10.1016/j.aquaculture.2006.04.004
10. Chen ZC, Liu Y, Li YX, Yang P, Hu HB, Yu GJ, et al. Dietary arginine supplementation mitigates the soybean meal induced enteropathy in juvenile turbot, Scophthalmus maximus L. Aquacult Res (2018) 49:1535–45. doi: 10.1111/are.13608
11. Jinho B, Ali H, Seonghun W, Wonsuk C, Sang-Gu L, Kang-Woong K, et al. Evaluation of seven different functional feed additives in a low fish meal diet for olive flounder, Paralichthys olivaceus. Aquaculture (2020) 525:735333. doi: 10.1016/j.aquaculture.2020.735333
13. Bai SC, Katya K, Yun H. Additives in aquafeed: an overview. In: Davis DA, editor. Feed and feeding practices in aquaculture. UK,Cambridge: Woodhead Publishing (2015). p. 171–91.
14. Zhu F. A review on the application of herbal medicines in the disease control of aquatic animals. Aquaculture (2020) 526:735422. doi: 10.1016/j.aquaculture.2020.735422
15. Luo QH, Qian RD, Qiu ZS, Yamamoto FY, Du YY, Lin XW, et al. Dietary α-ketoglutarate alleviates glycinin and β-conglycinin induced damage in the intestine of mirror carp (Cyprinus carpio). Front Immunol (2023) 14:1140012. doi: 10.3389/fimmu.2023.1140012
16. Luo QH, Zhou ZL, Zhao JH, Xu H, Limbu SM, Xu QY. Dietary β-conglycinin induces intestinal enteritis and affects glycerophospholipid and arginine metabolism in mirror carp (Cyprinus carpio). Aquaculture (2023) 567:739257. doi: 10.1016/j.aquaculture.2023.739257
17. Abdel-Tawwab M, Eissa ESH, Tawfik WA, Abd Elnabi HE, Saadony S, Bazina WK, et al. Dietary curcumin nanoparticles promoted the performance, antioxidant activity, and humoral immunity, and modulated the hepatic and intestinal histology of Nile tilapia fingerlings. Fish Physiol Biochem (2022) 48:585–601. doi: 10.1007/s10695-022-01066-4
18. Yu CB, Zhang J, Qin Q, Liu J, Xu JX, Xu W. Berberine improved intestinal barrier function by modulating the intestinal microbiota in blunt snout bream (Megalobrama amblycephala) under dietary high-fat and high-carbohydrate stress. Fish Shellfish Immunol (2020) 102:336–49. doi: 10.1016/j.fsi.2020.04.052
19. Ma YB, Jiang WD, Wu P, Liu Y, Jiang J, Kuang SY, et al. Tea polyphenol alleviate Aeromonas hydrophila - induced intestinal physical barrier damage in grass carp (Ctenopharyngodon idella). Aquaculture (2021) 544:737067. doi: 10.1016/j.aquaculture.2021.737067
20. Fang H, Meng FP, Piao FY, Jin B, Li M and Li WZ. Effect of taurine on intestinal microbiota and immune cells in peyer’s patches of immunosuppressive mice. Adv Exp Med Biol (2019) 1155:13–24. doi: 10.1007/978-981-13-8023-5_2
21. Cheng ZY, Buentello A, Gatlin DM. Effects of dietary arginine and glutamine on growth performance, immune responses and intestinal structure of red drum, Sciaenops ocellatus. Aquaculture (2011) 319:247–52. doi: 10.1016/j.aquaculture.2011.06.025
22. Han ZF, Xiao SJ, Li WB, Ye K, Wang ZY. The identification of growth, immune related genes and marker discovery through transcriptome in the yellow drum (Nibea albiflora). Genes Genomics (2018) 40:881–91. doi: 10.1007/s13258-018-0697-x
23. Wang LG, Lu Q, Luo SY, Zhan W, Chen RY, Lou B, et al. Effect of dietary lipid on growth performance, body composition, plasma biochemical parameters and liver fatty acids content of juvenile yellow drum Nibea albiflora. Aquacult Rep (2016) 4:10–6. doi: 10.1016/j.aqrep.2016.05.002
24. Huang JB, Zhou CP, Xu F, Luo XB, Huang XL, Huang Z, et al. Effects of partial replacement of fish meal with porcine meat meal on growth performance, antioxidant status, intestinal morphology, gut microflora and immune response of juvenile golden pompano (Trachinotus ovatus). Aquaculture (2022) 561:738646. doi: 10.1016/j.aquaculture.2022.738646
25. Femi JF, Rihanat OY, Oluwole OJ, Ibrahim A, Akeem OA, Benjamin OE. Effect of dietary polyherbal mixture on growth performance, haemato-immunological indices, antioxidant responses, and intestinal morphometry of african catfish, clarias gariepinus. Aquacult Nutr (2022) 2022:11. doi: 10.1155/2022/5502796
26. Cheng ZY, Alejandro B, Delbert MG. Effects of dietary arginine and glutamine on growth performance, immune responses and intestinal structure of red drum, Sciaenops ocellatus. Aquaculture (2011) 319:247–52. doi: 10.1016/j.aquaculture.2011.06.025
27. Sahlmann C, Sutherland BJG, Kortner TM, Koop BF, Krogdahl A, Bakke AM. Early response of gene expression in the distal intestine of Atlantic salmon (Salmo salar L.) during the development of soybean meal induced enteritis. Fish Shellfish Immunol (2013) 34:599–609. doi: 10.1016/j.fsi.2012.11.031
28. Sklan D, Prag T. Lupatsch I.Structure and function of the small intestine of the tilapia Oreochromis niloticus× Oreochromis aureus (Teleostei, Cichlidae). Aquacult Res (2004) 35:350–7. doi: 10.1111/j.1365-2109.2004.01020.x
29. Huerta-Aguirre G, Paredes-Ramos KM, Becerra-Amezcua MP, Hernández-Calderas I, Matadamas-Guzman M, Guzmán-García X. Histopathological analysis of the intestine from Mugil cephalus on environment reference sites. In: Gómez-Oliván L, editor. Pollution of water bodies in latin america. Cham: Springer Press (2019). p. 319–28.
30. Regost C, Arzel J, Kaushik SJ. Partial or totalreplacementof fishmeal by cornglu-ten meal in diet for turbot (Psetta maxima). Aquaculture (1999) 180:99–117. doi: 10.1016/S0044-8486(99)00026-5
31. Fournier V, Huelvan C, Desbruyeres E. Incorporation of a mixture of plant feed-stuffs as substitute for fish meal in diets of juvenile turbot (Psetta maxima). Aquaculture (2004) 236:451–65. doi: 10.1016/j.aquaculture.2004.01.035
32. Cheng ZY, Dmi G, Buentello A. Dietary supplementation of arginine and/or glutamine influences growth performance, immune responses and intestinal morphology of hybrid striped bass (Morone chrysops×Morone saxatilis). Aquaculture (2012) 362-363:39–43. doi: 10.1016/j.aquaculture.2012.07.015
33. Chamorro S, Romero C, Brenes A, Sanchez-Patan F, Bartolome B, Viveros A, et al. Impact of a sustained consumption of grape extract on digestion, gut microbial metabolism and intestinal barrier in broiler chickens. Food Funct (2019) 10:1444–54. doi: 10.1039/C8FO02465K
34. Romarheim OH, Øverland M, Mydland LT, Skrede A, Landsverk T. Bacteria grown on natural gas prevent soybean meal-in-duced enteritis in Atlantic salmon. J Nutr (2011) 141:124–30. doi: 10.3945/jn.110.128900
35. Zhang XL, Chen JJ, Wang GD, Chen HX, Cao JL, Xie LT, et al. Interactive effects of fluoride and seleno-l-methionine at environmental related concentrations on zebrafish (Danio rerio) liver via the gut-liver axis. Fish Shellfish Immunol (2022) 127:690–702. doi: 10.1016/j.fsi.2022.07.006
36. Miao ZY, Miao ZR, Teng XH, Xu SW. Melatonin alleviates lead-induced fatty liver in the common carps (Cyprinus carpio) via gut-liver axis. Environ Pollution (2022) 317:120730. doi: 10.1016/j.envpol.2022.120730
37. Shan JW, Wang GX, Li H, Zhao XY, Ye WD, Su L, et al. The immunoregulatory role of fish specific type II SOCS via inhibiting metaflammation in the gut-liver axis. Water Biol Secur (2023) 2:100131. doi: 10.1016/j.watbs.2022.100131
38. Torrecillas S, Montero D, Caballero MJ, Robaina L, Zamorano MJ, Sweetman J, et al. Effects of dietary concentrated mannan oligosaccharides supplementation on growth, gut mucosal immune system and liver lipid metabolism of European sea bass (Dicentrarchus labrax) juveniles. Fish Shellfish Immunol (2015) 42:508–16. doi: 10.1016/j.fsi.2014.11.033
39. Duan Y, Zhang Y, Dong H, Wang Y, Zheng X, Zhang J. Effect of dietary Clostridium butyricum on growth, intestine health status and resistance to ammonia stress in Pacific white shrimp Litopenaeus vannamei. Fish Shellfish Immunol (2017) 65:25–33. doi: 10.1016/j.fsi.2017.03.048
40. Lin Y, Cheng M. Effects of dietary organic acid supplementation on the growth, nutrient digestibility and intestinal histology of the giant grouper Epinephelus lanceolatus fed a diet with soybean meal. Aquaculture (2017) 469:106–11. doi: 10.1016/j.aquaculture.2016.11.032
41. Ulluwishew D, Anderson RC, McNabb WC, Moughan PJ, Wells JM, Roy NC. Regulation of tight junction permeability byintestinal bacteria and dietary components. J Nutr (2011) 141:769–76. doi: 10.3945/jn.110.135657
42. Tsukita S, Furuse M, Itoh M. Multifunctional strands in tight junctions. Nat Rev Mol Cell Biol (2001) 2:285–93. doi: 10.1038/35067088
43. Schneeberger EE. The tight junction: a multifunctional complex. Cell Physiol (2004) 286:1213–28. doi: 10.1152/ajpcell.00558.2003
44. Chen Q, Chen O, Martins IM, Hou H, Zhao X, Blumberg JB, et al. Collagen peptides ameliorate intestinal epithelial barrier dysfunction in immunostimulatory Caco-2 cell monolayers via enhancing tight junctions. Food Funct (2017) 8:1144–51. doi: 10.1039/C6FO01347C
45. Turner JR. Intestinal mucosal barrier function in health and dis-ease. Nat Rev Immunol (2009) 9:799–809. doi: 10.1038/nri2653
46. Marchiando AM, Shen L, Graham WV, Edelblum KL, Duckworth CA, Guan Y, et al. The epithelial barrier is main-tained by in vivo tight junction expansion during pathologic intestinal epithelial shedding. Gastroenterology (2011) 140:1–2. doi: 10.1053/j.gastro.2011.01.004
47. Turner JR. ‘Putting the squeeze’ on the tight junction: understanding cytoskeletal regulation. Semin Cell Dev Biol (2000) 11:301–8. doi: 10.1006/scdb.2000.0180
48. Weis WI, Nelson WJ. Re-solving the cadherin-catenin-actin conundrum. J Biol Chem (2006) . 281:35593–7. doi: 10.1074/jbc.R600027200
49. Rimoldi S, Finzi G, Ceccotti C, Girardello R, Grimaldi A, Ascione C, et al. Butyrate and taurine exert a mitigating effect on the inflamed distal intestine of European sea bass fed with a high percentage of soybean meal. Fisheries Aquat Sci (2016) 19:40–53. doi: 10.1186/s41240-016-0041-9
50. Terova G, Díaz N, Rimoldi S, Ceccotti C, Gliozheni E, Piferrer F. Effects of sodium butyrate treatment on histone mod-ifications and the expression of genes related to epigenetic reg-ulatory mechanisms and immune response in European sea bass (Dicentrarchus Labrax) fed a plant - based diet. PloS One (2016) 11:e0160332. doi: 10.1371/journal.pone.0160332
51. Secombes CJ, Wang T, Hong S, Peddie S, Crampe M, Laing KJ, et al. Cytokines and innate immunity of fish. Dev Comp Immunol (2001) 25:713–23. doi: 10.1016/S0145-305X(01)00032-5
52. Savan R, Sakai M. Genomics of fish cytokines. Comp Biochem Physiol Part D: Genomics Proteomics (2006) 1:89–101. doi: 10.1016/j.cbd.2005.08.005
53. Kelly A, Houston SA, Sherwood E, Casulli J, Travis MA. Regulation of innate and adaptive immunity by TGFβ. Adv Immunol (2017) 134:137–233. doi: 10.1016/bs.ai.2017.01.001
54. Liu Y, Chen ZC, Dai JH, Yang P, Hu HB, Ai QH, et al. The protective role of glutamine on enteropathy induced by high dose of soybean meal in turbot, Scophthalmus maximus L. Aquaculture (2018) 497:510–9. doi: 10.1016/j.aquaculture.2018.08.021
Keywords: Nibea albiflora, soybean meal, dietary additive, intestinal injury, reparative effect
Citation: Ma S, Wang L, Zeng Y, Tan P, Chen R, Hu W, Xu H and Xu D (2023) Reparative effect of different dietary additives on soybean meal-induced intestinal injury in yellow drum (Nibea albiflora). Front. Immunol. 14:1296848. doi: 10.3389/fimmu.2023.1296848
Received: 19 September 2023; Accepted: 17 November 2023;
Published: 08 December 2023.
Edited by:
Samad Rahimnejad, University of Murcia, SpainCopyright © 2023 Ma, Wang, Zeng, Tan, Chen, Hu, Xu and Xu. This is an open-access article distributed under the terms of the Creative Commons Attribution License (CC BY). The use, distribution or reproduction in other forums is permitted, provided the original author(s) and the copyright owner(s) are credited and that the original publication in this journal is cited, in accordance with accepted academic practice. No use, distribution or reproduction is permitted which does not comply with these terms.
*Correspondence: Ligai Wang, d2xpZ2Fpa3VhaWxlQDEyNi5jb20=; Dongdong Xu, eHVkb25nMDU4MEAxNjMuY29t