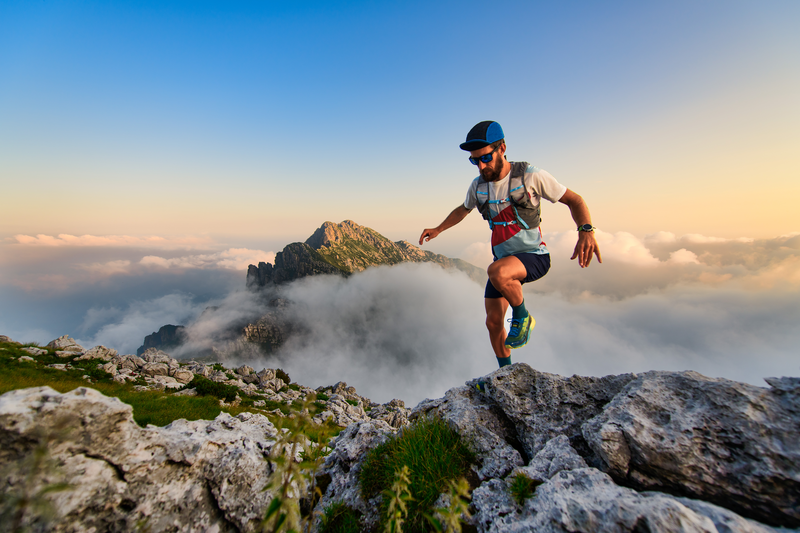
95% of researchers rate our articles as excellent or good
Learn more about the work of our research integrity team to safeguard the quality of each article we publish.
Find out more
MINI REVIEW article
Front. Immunol. , 05 December 2023
Sec. Alloimmunity and Transplantation
Volume 14 - 2023 | https://doi.org/10.3389/fimmu.2023.1296663
This article is part of the Research Topic Graft-Versus Leukemia (GVL) Effect - Immunobiology and Ways to Augment it View all 8 articles
Allogeneic hematopoietic cell transplantation (HCT) is a curative therapy for various hematologic malignancies. However, alloimmune response is a double-edged sword that mediates both beneficial graft-versus-leukemia (GVL) effects and harmful graft-versus-host disease (GVHD). Separation of GVL effects from GVHD has been a topic of intense research to improve transplant outcomes, but reliable clinical strategies have not yet been established. Target tissues of acute GVHD are the skin, liver, and intestine, while leukemic stem cells reside in the bone marrow. Tissue specific effector T-cell migration is determined by a combination of inflammatory and chemotactic signals that interact with specific receptors on T cells. Specific inhibition of donor T cell migration to GVHD target tissues while preserving migration to the bone marrow may represent a novel strategy to separate GVL from GVHD. Furthermore, tissue specific GVHD therapy, promoting tissue tolerance, and targeting of the tumor immune microenvironment may also help to separate GVHD and GVL.
Allogeneic hematopoietic cell transplantation (HCT) is a curative treatment for leukemia and other various hematologic malignancies. Its antileukemic effect is mediated by donor immune cells and refers as graft-versus-leukemia (GVL) effects. This phenomenon was recognized already in 1956; Barnes et al. reported that leukemia-bearing mice receiving allogeneic cells eventually died of GVHD without evidence of leukemia (1). Weiden et al. documented its clinical effects on preventing relapse in 1979 (2). Since then, the goal for HCT remains the enhancement of GVL effect while limiting GVHD. However, GVL activity is clearly associated with GVHD; patients with acute GVHD or chronic GVHD have a significantly lower risk of relapse after HCT compared to those without GVHD (2, 3).
Separation of GVL effects from GVHD has been a topic of intense research to improve transplant outcomes. Over the past several decades, clinical attempts to identify and separate specific immune effector mechanisms that can dissect GVHD and GVL have been unsuccessful. Current therapies for GVHD target T cells and cytokines, often antagonizing GVL effects. Acute GVHD targets specific tissues, such as the skin, gut, and liver. Recruitment of donor T cell into these tissues plays a major role in GVHD (4). On the other hand, bone marrow niche is commonly observed in various hematologic malignancies (5). Leukemia stem cells (LSCs) are resistant to chemotherapy, making them the drivers of leukemia relapse, and they reside in the bone marrow niche (6, 7). Tissue-specific effector T cell emigration is determined by a complex milieu of inflammatory and chemotactic signals that interact with specific receptors on T cell (8). Modulation of donor T cell migration may pave a new avenue to separate GVHD and GVL (Figure 1).
Figure 1 Separation of GVL from GVHD by regulating T cell trafficking to tissues. Donor derived alloreactive T cells are activated in the gut and secondary lymphoid organs, and migrate into the skin, liver, and gut. Tissue-specific effector T cell migration is determined by a combination of inflammatory and chemotactic signals that interact with specific receptors on T cells. Inhibition of donor T cell migration to GVHD target tissues without impeding T cell trafficking to the bone marrow represents a novel strategy to separate GVL from GVHD. Late-phase GVHD is maintained within affected tissues locally by tissue-resident TCF1+ pTex-like cells. Local inhibition of these cells may not impede GVL.
Similar to T cells, natural killer (NK) cells have potent anti-leukemia effector capacity, but unlike T cells, NK cells have less ability to mediate GVHD. Because of their lack of HLA-restricted specificity, allogeneic NK cells can be administered across HLA barriers without GVHD (9). Recent studies have shown that donor NK cell infusion after haploidentical bone marrow transplantation using posttransplant cyclophosphamide (PTCy-haplo) reduced relapse compared to historical controls, with an excellent safety profile (10). Donor selection according to killer immunoglobulin-like receptor (KIR) alloreactivity is associated with superior survival in PTCy-haplo (11). Maximizing donor NK alloreactivity thus holds the exciting possibility to induce GVL effect without engendering GVHD. In this review, we will discuss current attempts to separate GVHD and GVL as well as novel concepts of GVHD and GVL separation by considering the location of GVHD and GVL.
The risk of leukemia relapse was significantly higher in patients who did not develop GVHD or who received a T cell-depleted graft or a graft from patient’s identical twin (12, 13). Thus, donor T cells play a major role in mediating GVL effects. Shlomchik et al. demonstrated that naïve T cells rather than memory T cells played the major role in inducing GVHD in mice (14, 15). Clinical trial data of naïve T cell-depleted HCT demonstrated low incidences of severe acute GVHD and chronic GVHD without apparent excess risks of leukemia relapse (16, 17), but effect of naïve T cell depletion on leukemia relapse remains to be evaluated in larger studies. Unlike the memory T cells developed in donor, donor memory cells developed in the recipients after allo-SCT are involved in GVHD. It has been shown that donor stem cell-like memory cells (Tscm) persist in the recipient and maintain alloreactivity against host alloantigens in mice. Adoptive transfer of Tscm into the secondary recipients induced GVHD (18). Tscm population has been identified in human (19). A recent study showed that administration of PTCy spared Tscm that can improve GVL (20).
Donor T cells mediating both GVHD and GVL are activated primarily by recipient antigen presenting cells (APCs) in mice (21–23). Reconstituting donor hematopoietic APCs cross-present host antigens to invoke the full spectrum of GVHD and GVL (22, 24–26). Thus, separation of GVL from GVHD could not be easily achieved by the modulation of donor T cell and APC interactions.
After allogeneic HCT, host-derived alloantigen persists lifelong, which could induce T cell exhaustion. A series of experimental studies showed that T cell exhaustion is one of the chief mechanisms of tolerance induction without chronic GVHD after allogeneic HCT (27–29). T cell exhaustion is a multistep process, involving precursors of exhausted T cells (Tpex) with stem cell-like properties, transitory exhausted T cells (transitory-Tex) with potent effector-like functions and terminally differentiated exhausted T cells (terminal-Tex) with severely impaired functions (30–33). Calcineurin inhibitors inhibit T-cell exhaustion by inhibiting expression of a master regulator of T cell exhaustion, TOX (34). In experimental HCT, GVHD prophylaxis with calcineurin inhibitors suppresses differentiation of transitory-Tex to terminal-Tex, resulting in persistent alloreactivity and induction of chronic GVHD (35). T cell dysfunction of terminal-Tex is irreversible and non-responsive to immune checkpoint inhibitors (ICI), while ICI enhance proliferation and effector functions of Tpex and transitory-Tex (36). Therefore, calcineurin-induced transitory-Tex could be a promising therapeutic target to restore GVL effects by ICI but with a risk of GVHD exacerbation (35).
GVHD and GVL are mediated by donor T cells recognizing non-self-antigens expressed on host- and donor-derived APCs. In HLA-identical HCT, GVHD is induced by minor histocompatibility antigens (miHA), which are HLA-bound peptides that differ between the donor and recipient due to genetic polymorphisms (37). Although it remains unclear how many immunodominant miHA could evoke significant GVHD and GVL in humans, most miHA are ubiquitously expressed including epithelial tissues, thereby potentially inducing GVHD.
miHA with expression limited to hematopoietic cells represent attractive candidate targets for selective induction of GVL effects without causing GVHD in patients with hematologic malignancies. For example, adoptive transfer of H7a (B6dom1) specific T cells eradicates H7a-expressing leukemia efficiently in H7a-deficient mice without GVHD (38). Transfer of H60-specific CD8+ memory T cells eradiates chronic myeloid leukemia cells (39). Several studies have suggested that GVL activity is greater against hematopoietic restricted miHA by eliciting less exhaustion and activation-induced cell death of alloreactive T cells than that against ubiquitously expressed miHA (28, 39). In humans, cytotoxic T lymphocytes (CTLs) specific for male tissue specific miHA H-Y induce skin injury when co-cultured with male skin biopsy specimen, while hematopoietic system-specific miHA HA-1 and HA-2 induce little tissue injury (40). However, an inflammatory environment can render nonhematopoietic cells susceptible to T cell recognition to induce GVHD (41). It is also challenging to identify candidate miHA that can be widely applied to heterogenous patient-donor combinations. Well characterized hematopoietic miHA in humans include HA-1, HA-2, ACC-1, ACC-2, and LRH1 (40, 42–45). It remains to be elucidated whether mismatch of these miHA could reduce leukemia relapse without inducing GVHD in the presence of the other multiple miHA mismatches between the donor and recipient. Immunotherapy using specific miHA-directed T cells generated by genetic modification or vaccination is promising strategy but remains to be evaluated in prospective comparative studies.
Acute GVHD is organ-specific and principally affecting the skin, liver, and gut. Development of GVHD requires donor T cells to migrate into these tissues (4). Several tissue-specific T cell homing receptors have been identified. The α4β7 integrin is critical for T cell homing to the gastrointestinal tract and gut-associated lymphoid tissues (46). T cell migration to the skin is directed by cutaneous lymphocyte antigen, a specialized form of P-selectin glycoprotein ligand-1 (47). Regulation of effector T cell migration into target tissues occurs in a complex milieu of chemotactic signals where several receptors may be triggered simultaneously or successively (48). Inflammatory chemokines expressed in inflamed tissues upon stimulation by proinflammatory cytokines are specialized for the recruitment of donor T cells and other effector cells (8, 49). Chemokine receptors are differentially expressed on subsets of activated/effector T cells. Upon stimulation, T cells rapidly switch chemokine receptor expression and acquire new migratory capacity (50, 51). Requirement of donor T cell homing to specific tissues has profound clinical implications to modulate GVHD and GVL.
Pharmaceutical agents used for prophylaxis and treatment of GVHD such as calcineurin inhibitors, antimetabolites, and corticosteroids have considerable effects on T cell trafficking generally, thus likely suppressing GVHD and GVL at the same time (52). Corticosteroids are the first line treatment for both acute and chronic GVHD. However, higher dose and longer duration of corticosteroid therapy is associated with poor outcomes (53–55). Recently, ruxolitinib has been approved for steroid-refractory acute and chronic GVHD, ibrutinib and belumosudil for steroid-refractory chronic GVHD (56). However, it is not clear for how long they should be administered with a fear of losing GVL effects.
Although host alloantigen is essentially expressed in all tissues, target tissues of acute GVHD are the skin, gut, and liver, suggesting that donor T cells are polarized to traffic to these tissues. Accumulating evidence suggest that intestine is a critical site for alloreactive T cell activation by APCs (23, 57–59). The α4β7 integrin-MAdCAM (mucosal addressin cell adhesion molecule)-1 interactions are essential for donor T cell homing to the gut, and the subsequent development of lethal GVHD (57, 59, 60). A phase III randomized, double-blind, placebo-controlled study (GRAPHITE study) evaluated the efficacy and safety of vedolizumab, a humanized monoclonal antibody directed against α4β7 integrin, which is expressed on T cells and is essential for gastrointestinal (GI) trafficking (61). This study met its primary endpoint by demonstrating a higher lower GI-GvHD-free survival with a comparable safety profile (presented at 2023 Transplantation & Cellular Therapy Meetings; Abstract# LBA2). The impacts on skin and liver GVHD, as well as leukemia relapse, remain to be investigated.
The C–C chemokine receptor 5 (CCR5) interacts with multiple chemokine ligands that mediate the migration and function of T cells and other immune cells to the inflamed tissues (62). CCR5 is critical for donor T cell recruitment to tissues involved in visceral acute GVHD (57, 63–65). In a clinical study of CCR5 antagonist maraviroc involving 38 patients, none of the patients developed acute liver or gut GVHD (66). However, maraviroc failed to reduce GVHD in a randomized phase 2 trial (67).
The CT10 regulator of kinase (Crk) is a crucial adaptor protein for T cell migration (68). Crk deficient T cells failed to traffic GVHD target tissues but efficiently eliminate lymphoma cells in the circulation (69). However, Crk deficient T cells failed to clear the same tumor growing in the skin (69). These results suggest that Crk could be a potential target in controlling GVHD and GVL effects against circulating hematopoietic tumors.
Sphingosine-1-phosphate (S1P) is a metabolite of sphingolipid, a component of bio membrane. S1P interacts with S1P receptor types 1 to 5 (S1PR1-5) (70). S1P modulates cellular proliferation, survival, and migration. Fingolimod (FTY720), a multi-S1PR inhibitor of S1PR1 and S1PR3–5, sequestrates T cells within the secondary lymphoid organs (SLOs) (71, 72). Preclinical studies showed that administration of fingolimod ameliorated GVHD by inhibiting donor T cell infiltration to GVHD target organs and facilitating activation-induced cell death of alloreactive T cells (73, 74). Unfortunately, fingolimod exerts cardiovascular adverse effects that are accelerated in inflammatory milieu in GVHD through its affinity to S1PR3 (75). T cells primarily express S1PR1 (70). Mocravimod (KRP203), a selective agonist of S1PR1, induced apoptosis of donor T cells in the SLOs, suppressed donor T cell migration into the intestine and skin, and ameliorated GVHD (76). Importantly, mocravimod significantly preserved GVL effects compared to cyclosporine (76). In a phase 1 trial, mocravimod reduced circulating lymphocyte numbers, while increased T cell accumulation in the bone marrow (77, 78). These preliminary results suggest that selective inhibition of S1P and S1PR1 interactions inhibits donor T cell migration to the GVHD target tissues, while preserving its migration to the bone marrow. A global phase 3 study evaluating mocravimod in patients with acute myelogenous leukemias (AML) is ongoing.
Ruxolitinib, a JAK1/2 inhibitor, has been approved for the treatment of corticosteroid-refractory acute and chronic GVHD (79, 80). Ruxolitinib suppresses donor T-cell infiltration in the GVHD target organs by reducing CXCR3 expression on donor T cells (81, 82).
Such a strategy of modifying donor T cell migration may eliminate the use of broad immunosuppression, thereby minimizing infectious complications and preserving GVL effect (Figure 1).
In mouse models, alloreactive donor T cells migrate into target tissues early after allogeneic HCT. However, late-phase GVHD is maintained within affected tissues locally by tissue-resident TCF1+ pTex-like cells without migrating between target organs (83). In patients with multiple sites of GVHD, the dominant T cell receptor repertoires are not consistently observed across tissues within the same patient, raising the possibility that GVHD arose in each local tissue independently (84). Furthermore, patient-derived resident memory T cells persist for many years after HCT in association with the development of skin GVHD (85). These results suggest that tissue-resident memory T cells could be a therapeutic target for tissue-specific GVHD (Figure 1). Strategies that prevent T-cell migration into GVHD target organs could also inhibit the development of donor-derived tissue resident memory T cells. In addition, the local therapies also suppress the activities of tissue resident T cells.
While topical corticosteroids have been used for treating mild GVHD in the skin and gastrointestinal tract without blunting GVL effects, they are inadequate for treating severe GVHD. Enhancing local GVHD therapy could be the straightforward approach to dissect GVL and GVHD (Figure 2). We have recently discovered that topical ruxolitinib effectively improved skin GVHD in mice, while topical corticosteroids exhibited direct toxicity against skin stem cells (86). Long-term use of corticosteroids induces adverse effects on the skin, such as skin atrophy and delayed wound healing (87). In contrast, ruxolitinib protects skin stem cells from GVHD and facilitates wound healing (86). Ruxolitinib cream is now being tested for chronic skin GVHD in a randomized clinical trial.
Figure 2 Separation of GVL from GVHD by tissue specific GVHD therapy and modulation of TIME. (Upper panel) In GVHD target organs, donor T cells and innate immune cells such as macrophages (Mϕ) cause tissue damage via production of proinflammatory cytokines and direct cytotoxicity. Mϕ produce pro-fibrotic cytokines such as TGF-β and platelet-derived growth factor (PDGF), which activate fibroblasts and promote pathologic fibrosis and remodeling. Topical immunosuppressants such as corticosteroids and ruxolitinib suppress GVHD locally without impeding GVL. Mϕ-targeting agents such as CSF-1 receptor antibodies, and fibroblast-targeting agents such as VA-lip HSP47 may ameliorate chronic GVHD without affecting GVL effects. Growth factors for tissue stem cells or epithelial cells promote tissue tolerance, thereby suppressing tissue damage and facilitating tissue regeneration. (Lower panel) FLT3-ITD signaling produces immune-cold TIME in FLT3-ITD-expressing AML by inhibiting leukemia production of IL-15. FLT3 inhibitors restore IL-15 production and activate neighboring T cells. Mutated IDH produces 2-HG, which inhibits glycolysis and reduces cytotoxicity in neighboring T cells. IDH inhibitors activate T cells locally by reducing 2-HG and likely promote GVL effects. Red arrows indicate positive regulation, while blue arrows indicate negative regulation.
Fibrosis is an end-stage consequence of chronic inflammation in chronic GVHD. Treatment of fibrotic chronic GVHD with anti-fibrotic agents may not impede GVL (Figure 2) (88). A vitamin A-coupled liposome containing siRNA against heat-shock protein 47 (VA-lip HSP47) delivers HSP47 siRNA to pathogenic myofibroblasts in affected organs, such as the skin and salivary glands, and ameliorates fibrosis in mouse chronic GVHD (89). Macrophage-targeting therapy can also improve fibrosis by inhibiting the production of a pro-fibrotic cytokine TGF-β in mice (89, 90). Such approach likely has a minimal impact on GVL effects; axatilimab, an anti-CSF1R monoclonal antibody, is being tested in a clinical trial for chronic GVHD (91). Topical therapy using anti-fibrotic agents is also a promising treatment option. Fibrosis of the lacrimal glands leads to dry eye syndrome in chronic GVHD (92). Ocular instillation of VA-lip HSP47 ameliorates dry eye syndrome in chronic GVHD by targeting myofibroblasts in the lacrimal glands in mice (93).
Recently, ‘tissue tolerance’ has been proposed as a concept to comprehensively understand the mechanisms enhancing tissue resilience and regeneration during immune reactions (94). The sensitivity of target tissues to GVHD may be modulated by tissue-intrinsic resilience and homeostasis (95, 96). Modulation of GVHD by increasing tissue tolerance would be a promising adjunct therapy without impeding GVL (Figure 2). We and others have demonstrated that acute GVHD targets tissue epithelial stem cells in the intestine and skin, leading to prolonged and refractory GVHD (86, 95, 97, 98). Protection and stimulation of tissue stem cells to improve tissue tolerance and repair may represent a novel adjunct strategy for separating GVHD and GVL. For example, since IFN-γ damages tissue stem cells in the intestine and skin, ruxolitinib has the potential to improve GVHD by both suppressing immune reactions and protecting tissue stem cells (86, 99). R-Spondin3 is produced by intestinal stromal cells and lymphatic endothelial cells to maintain intestinal homeostasis by stimulating proliferation and differentiation of LGR5+ intestinal stem cells (100–104). Lymphatic endothelial cell injury in GVHD impairs R-Spondin3 production (102). Administration of recombinant R-Spondin1 stimulate growth and differentiation of intestinal stem cells, thus ameliorating experimental GVHD (97, 105). Similarly, administration of glucagon-like peptide-2 (GLP-2), a growth factor for intestinal stem cells produced by enteroendocrine cells in the intestine, ameliorates experimental GVHD (106). Teduglutide, a dipeptidyl peptidase inhibitor 4 (DPP4)-resistant analog of GLP-2, protects intestinal stem cells and enhances the barrier effects of the intestinal mucosa in experimental GVHD (106). IL-22 is produced by type 3 innate lymphoid cells, and its levels are reduced in acute GVHD (98). F562, a fusion protein containing two IL-22 molecules and IgG2-Fc, enhances epithelial regeneration in experimental GVHD and is currently under development in clinical studies (107, 108). Epithelial growth factors could also have beneficial effects on tissue tolerance. Administration of IL-25, a growth factor for goblet cells, mitigates the disruption of mucus layer of the intestine, and ameliorates experimental acute GVHD (109). Urinary-derived human chorionic gonadotropin/epidermal growth factor (uhCG/EGF; Pregnyl) contains abundant EGF, which protects the gut epithelium. A phase 2 trial of Pegnyl showed promising results (110). In conclusion, these therapies targeting tissue tolerance hold promise as treatment strategies for acute GVHD without inducing general immunosuppression and impeding GVL.
Internal tandem duplications (ITD) of the receptor-tyrosine kinase FLT3 gene (FLT3-ITD) are found in 20–25% of AML, providing a persistent growth stimulus. Tumor immune microenvironment (TIME) impacts on the outcome of immune-mediated treatment of various cancers. It has been shown that FLT3-ITD expressing AML has “immune cold” TIME with significantly less T and NK cell infiltration in the bone marrow compared to other types of AML (111). This is possibly due to the inhibition of IL-15 production by FLT3-ITD signaling in AML cells (112). A multi-tyrosine kinase inhibitor sorafenib restores IL-15-production in FLT3-ITD+ AML cells by inhibiting FLT3-ITD signaling (Figure 2) (112). IL-15 is a homeostatic cytokine for CD8+ T cells and plays a critical role in the survival and activation of CD8+ T cells. The biologic activities of IL-15 are uniquely mediated by the IL-15-IL15Rα complex produced by non-T cells and “trans-presented” to neighboring CD8+ T cells (113). Thus, IL-15 produced by FLT3-ITD+ AML cells can activate neighboring CD8+ T cells in the bone marrow, thereby promoting GVL effect without significant GVHD induction (112). A subsequent study demonstrated that a selective FLT3 inhibitor gilteritinib also promoted GVL effect without exacerbating GVHD through the similar mechanisms (114). However, in patients with liver involvement of FLT3-ITD+ AML, FLT3 inhibitor may exacerbate hepatic GVHD by stimulating neighboring T cells. Another immunosuppressive TIME could be produced by IDH mutation. IDH1-mutation in gliomas suppressed local expansion and cytotoxicity of CD8+ T cells by producing oncometabolite D-2-hydroxyglutarate (2-HG) (115). This localized T-cell suppression can be promptly reversed by IDH-inhibition. Although the role of IDH1 mutation on TIME is less clear in AML (116), these results suggest that IDH inhibitors may induce localized GVL effects around AML cells without affecting systemic GVHD.
Isolating GVL effects from GVHD has been a paramount issue in transplant community. However, redirecting the donor T cell alloreactivity specifically towards leukemia cells, while avoiding exacerbation of GVHD, remains challenging. In this context, the prospect lies in enhancing localized immune suppressive therapies and impeding T cell migration into GVHD target organs, while maintaining T cell migration toward the bone marrow, where niches for leukemic stem cells exist. In addition, improving our understanding of the biology of tissue stem cells in GVHD target tissues will facilitate the development of therapies aimed at promoting tissue tolerance. Counteracting immune evasion of leukemia cells stands as another avenue for enhancing GVL effects. Particularly, specific inhibitors targeting mutated molecules within leukemia may amplifying GVL effects by modulating TIME. Better understanding of the mechanisms of GVHD and GVL is essential to develop strategies for separating GVHD and GVL.
TT: Writing – original draft. DH: Writing – original draft.
The author(s) declare financial support was received for the research, authorship, and/or publication of this article. This study was supported by JSPS KAKENHI (21H02944 and 23K18296 to TT, 21K08409 to DH).
The authors declare that the research was conducted in the absence of any commercial or financial relationships that could be construed as a potential conflict of interest.
All claims expressed in this article are solely those of the authors and do not necessarily represent those of their affiliated organizations, or those of the publisher, the editors and the reviewers. Any product that may be evaluated in this article, or claim that may be made by its manufacturer, is not guaranteed or endorsed by the publisher.
1. Barnes DW, Corp MJ, Loutit JF, Neal FE. Treatment of murine leukaemia with X rays and homologous bone marrow; preliminary communication. Br Med J (1956) 2:626–7. doi: 10.1136/bmj.2.4993.626
2. Weiden PL, Flournoy N, Thomas ED, Prentice R, Fefer A, Buckner CD, et al. Antileukemic effect of graft-versus-host disease in human recipients of allogeneic-marrow grafts. N Engl J Med (1979) 300:1068–73. doi: 10.1056/NEJM197905103001902
3. Horowitz MM, Gale RP, Sondel PM, Goldman JM, Kersey J, Kolb HJ, et al. Graft-versus-leukemia reactions after bone marrow transplantation. Blood (1990) 75:555–62. doi: 10.1182/blood.V75.3.555.555
4. Sackstein R. A revision of Billingham’s tenets: the central role of lymphocyte migration in acute graft-versus-host disease. Biol Blood Marrow Transplantation: J Am Soc Blood Marrow Transplant (2006) 12:2–8. doi: 10.1016/j.bbmt.2005.09.015
5. Schepers K, Campbell TB, Passegue E. Normal and leukemic stem cell niches: insights and therapeutic opportunities. Cell Stem Cell (2015) 16:254–67. doi: 10.1016/j.stem.2015.02.014
6. Ishikawa F, Yoshida S, Saito Y, Hijikata A, Kitamura H, Tanaka S, et al. Chemotherapy-resistant human AML stem cells home to and engraft within the bone-marrow endosteal region. Nat Biotechnol (2007) 25:1315–21. doi: 10.1038/nbt1350
7. Marchand T, Pinho S. Leukemic stem cells: from leukemic niche biology to treatment opportunities. Front Immunol (2021) 12:775128. doi: 10.3389/fimmu.2021.775128
8. Springer TA. Traffic signals for lymphocyte recirculation and leukocyte emigration: the multistep paradigm. Cell (1994) 76:301–14. doi: 10.1016/0092-8674(94)90337-9
9. Ruggeri L, Capanni M, Urbani E, Perruccio K, Shlomchik WD, Tosti A, et al. Effectiveness of donor natural killer cell alloreactivity in mismatched hematopoietic transplants. Science (2002) 295:2097–100. doi: 10.1126/science.1068440
10. Ciurea SO, Kongtim P, Soebbing D, Trikha P, Behbehani G, Rondon G, et al. Decrease post-transplant relapse using donor-derived expanded NK-cells. Leukemia (2022) 36:155–64. doi: 10.1038/s41375-021-01349-4
11. Zou J, Kongtim P, Srour SA, Greenbaum U, Schetelig J, Heidenreich F, et al. Donor selection for KIR alloreactivity is associated with superior survival in haploidentical transplant with PTCy. Front Immunol (2022) 13:1033871. doi: 10.3389/fimmu.2022.1033871
12. Goldman JM, Gale RP, Horowitz MM, Biggs JC, Champlin RE, Gluckman E, et al. Bone marrow transplantation for chronic myelogenous leukemia in chronic phase. Increased risk for relapse associated with T-cell depletion. Ann Intern Med (1988) 108:806–14. doi: 10.7326/0003-4819-108-6-806
13. Marmont AM, Horowitz MM, Gale RP, Sobocinski K, Ash RC, van Bekkum DW, et al. T-cell depletion of HLA-identical transplants in leukemia. Blood (1991) 78:2120–30. doi: 10.1182/blood.V78.8.2120.2120
14. Anderson BE, McNiff J, Yan J, Doyle H, Mamula M, Shlomchik MJ, et al. Memory CD4+ T cells do not induce graft-versus-host disease. J Clin Invest (2003) 112:101–8. doi: 10.1172/JCI17601
15. Anderson BE, Taylor PA, McNiff JM, Jain D, Demetris AJ, Panoskaltsis-Mortari A, et al. Effects of donor T-cell trafficking and priming site on graft-versus-host disease induction by naive and memory phenotype CD4 T cells. Blood (2008) 111:5242–51. doi: 10.1182/blood-2007-09-107953
16. Bleakley M, Heimfeld S, Loeb KR, Jones LA, Chaney C, Seropian S, et al. Outcomes of acute leukemia patients transplanted with naive T cell-depleted stem cell grafts. J Clin Invest (2015) 125:2677–89. doi: 10.1172/JCI81229
17. Bleakley M, Sehgal A, Seropian S, Biernacki MA, Krakow EF, Dahlberg A, et al. Naive T-cell depletion to prevent chronic graft-versus-host disease. J Clin Oncol (2022) 40:1174–85. doi: 10.1200/JCO.21.01755
18. Zhang Y, Joe G, Hexner E, Zhu J, Emerson SG. Host-reactive CD8+ memory stem cells in graft-versus-host disease. Nat Med (2005) 11:1299–305. doi: 10.1038/nm1326
19. Gattinoni L, Lugli E, Ji Y, Pos Z, Paulos CM, Quigley MF, et al. A human memory T cell subset with stem cell-like properties. Nat Med (2011) 17:1290–7. doi: 10.1038/nm.2446
20. Minnie SA, Waltner OG, Ensbey KS, Nemychenkov NS, Schmidt CR, Bhise SS, et al. Depletion of exhausted alloreactive T cells enables targeting of stem-like memory T cells to generate tumor-specific immunity. Sci Immunol (2022) 7:eabo3420. doi: 10.1126/sciimmunol.abo3420
21. Shlomchik WD, Couzens MS, Tang CB, McNiff J, Robert ME, Liu J, et al. Prevention of graft versus host disease by inactivation of host antigen-presenting cells. Science (1999) 285:412–5. doi: 10.1126/science.285.5426.412
22. Reddy P, Maeda Y, Liu C, Krijanovski OI, Korngold R, Ferrara JL. A crucial role for antigen-presenting cells and alloantigen expression in graft-versus-leukemia responses. Nat Med (2005) 11:1244–9. doi: 10.1038/nm1309
23. Koyama M, Kuns RD, Olver SD, Raffelt NC, Wilson YA, Don AL, et al. Recipient nonhematopoietic antigen-presenting cells are sufficient to induce lethal acute graft-versus-host disease. Nat Med (2011) 18:135–42. doi: 10.1038/nm.2597
24. Matte CC, Liu J, Cormier J, Anderson BE, Athanasiadis I, Jain D, et al. Donor APCs are required for maximal GVHD but not for GVL. Nat Med (2004) 10:987–92. doi: 10.1038/nm1089
25. Anderson BE, McNiff JM, Jain D, Blazar BR, Shlomchik WD, Shlomchik MJ. Distinct roles for donor- and host-derived antigen-presenting cells and costimulatory molecules in murine chronic graft-versus-host disease: requirements depend on target organ. Blood (2005) 105:2227–34. doi: 10.1182/blood-2004-08-3032
26. Wang X, Li H, Matte-Martone C, Cui W, Li N, Tan HS, et al. Mechanisms of antigen presentation to T cells in murine graft-versus-host disease: cross-presentation and the appearance of cross-presentation. Blood (2011) 118:6426–37. doi: 10.1182/blood-2011-06-358747
27. Blazar BR, Carreno BM, Panoskaltsis-Mortari A, Carter L, Iwai Y, Yagita H, et al. Blockade of programmed death-1 engagement accelerates graft-versus-host disease lethality by an IFN-gamma-dependent mechanism. J Immunol (2003) 171:1272–7. doi: 10.4049/jimmunol.171.3.1272
28. Asakura S, Hashimoto D, Takashima S, Sugiyama H, Maeda Y, Akashi K, et al. Alloantigen expression on non-hematopoietic cells reduces graft-versus-leukemia effects in mice. J Clin Invest (2010) 120:2370–8. doi: 10.1172/JCI39165
29. Flutter B, Edwards N, Fallah-Arani F, Henderson S, Chai JG, Sivakumaran S, et al. Nonhematopoietic antigen blocks memory programming of alloreactive CD8+ T cells and drives their eventual exhaustion in mouse models of bone marrow transplantation. J Clin Invest (2010) 120:3855–68. doi: 10.1172/JCI41446
30. Im SJ, Hashimoto M, Gerner MY, Lee J, Kissick HT, Burger MC, et al. Defining CD8+ T cells that provide the proliferative burst after PD-1 therapy. Nature (2016) 537:417–21. doi: 10.1038/nature19330
31. Utzschneider DT, Charmoy M, Chennupati V, Pousse L, Ferreira DP, Calderon-Copete S, et al. T cell factor 1-expressing memory-like CD8(+) T cells sustain the immune response to chronic viral infections. Immunity (2016) 45:415–27. doi: 10.1016/j.immuni.2016.07.021
32. Hudson WH, Gensheimer J, Hashimoto M, Wieland A, Valanparambil RM, Li P, et al. Proliferating transitory T cells with an effector-like transcriptional signature emerge from PD-1(+) stem-like CD8(+) T cells during chronic infection. Immunity (2019) 51:1043–1058 e4. doi: 10.1016/j.immuni.2019.11.002
33. Belk JA, Daniel B, Satpathy AT. Epigenetic regulation of T cell exhaustion. Nat Immunol (2022) 23:848–60. doi: 10.1038/s41590-022-01224-z
34. Khan O, Giles JR, McDonald S, Manne S, Ngiow SF, Patel KP, et al. TOX transcriptionally and epigenetically programs CD8(+) T cell exhaustion. Nature (2019) 571:211–8. doi: 10.1038/s41586-019-1325-x
35. Senjo H, Harada S, Kubota SI, Tanaka Y, Tateno T, Zhang Z, et al. Calcineurin inhibitor inhibits tolerance induction by suppressing terminal exhaustion of donor T cells after allo-HCT. Blood (2023) 142:477–92. doi: 10.1182/blood.2023019875
36. Franco F, Jaccard A, Romero P, Yu YR, Ho PC. Metabolic and epigenetic regulation of T-cell exhaustion. Nat Metab (2020) 2:1001–12. doi: 10.1038/s42255-020-00280-9
37. Murata M, Warren EH, Riddell SR. A human minor histocompatibility antigen resulting from differential expression due to a gene deletion. J Exp Med (2003) 197:1279–89. doi: 10.1084/jem.20030044
38. Fontaine P, Roy-Proulx G, Knafo L, Baron C, Roy DC, Perreault C. Adoptive transfer of minor histocompatibility antigen-specific T lymphocytes eradicates leukemia cells without causing graft-versus-host disease. Nat Med (2001) 7:789–94. doi: 10.1038/89907
39. Li N, Matte-Martone C, Zheng H, Cui W, Venkatesan S, Tan HS, et al. Memory T cells from minor histocompatibility antigen-vaccinated and virus-immune donors improve GVL and immune reconstitution. Blood (2011) 118:5965–76. doi: 10.1182/blood-2011-07-367011
40. Dickinson AM, Wang XN, Sviland L, Vyth-Dreese FA, Jackson GH, Schumacher TN, et al. In situ dissection of the graft-versus-host activities of cytotoxic T cells specific for minor histocompatibility antigens. Nat Med (2002) 8:410–4. doi: 10.1038/nm0402-410
41. van Bergen CA, van Luxemburg-Heijs SA, de Wreede LC, Eefting M, von dem Borne PA, Balen Pv, et al. Selective graft-versus-leukemia depends on magnitude and diversity of the alloreactive T cell response. J Clin Invest (2017) 127:517–29. doi: 10.1172/JCI86175
42. de Rijke B, van Horssen-Zoetbrood A, Beekman JM, Otterud B, Maas F, Woestenenk R, et al. A frameshift polymorphism in P2X5 elicits an allogeneic cytotoxic T lymphocyte response associated with remission of chronic myeloid leukemia. J Clin Invest (2005) 115:3506–16. doi: 10.1172/JCI24832
43. Summers C, Sheth VS, Bleakley M. Minor histocompatibility antigen-specific T cells. Front Pediatr (2020) 8:284. doi: 10.3389/fped.2020.00284
44. de Bueger M, Bakker A, Van Rood JJ, van der Woude F, Goulmy E. Tissue distribution of human minor histocompatibility antigens. Ubiquitous versus restricted tissue distribution indicates heterogeneity among human cytotoxic T lymphocyte-defined non-MHC antigens. J Immunol (1992) 149:1788–94. doi: 10.4049/jimmunol.149.5.1788
45. Akatsuka Y, Nishida T, Kondo E, Miyazaki M, Taji H, Iida H, et al. Identification of a polymorphic gene, BCL2A1, encoding two novel hematopoietic lineage-specific minor histocompatibility antigens. J Exp Med (2003) 197:1489–500. doi: 10.1084/jem.20021925
46. Berlin C, Berg EL, Briskin MJ, Andrew DP, Kilshaw PJ, Holzmann B, et al. Alpha 4 beta 7 integrin mediates lymphocyte binding to the mucosal vascular addressin MAdCAM-1. Cell (1993) 74:185–95. doi: 10.1016/0092-8674(93)90305-A
47. Fuhlbrigge RC, Kieffer JD, Armerding D, Kupper TS. Cutaneous lymphocyte antigen is a specialized form of PSGL-1 expressed on skin-homing T cells. Nature (1997) 389:978–81. doi: 10.1038/40166
48. Moser B, Loetscher P. Lymphocyte traffic control by chemokines. Nat Immunol (2001) 2:123–8. doi: 10.1038/84219
49. Duffner U, Lu B, Hildebrandt GC, Teshima T, Williams DL, Reddy P, et al. Role of CXCR3-induced donor T-cell migration in acute GVHD. Exp Hematol (2003) 31:897–902. doi: 10.1016/S0301-472X(03)00198-X
50. Sallusto F, Lenig D, Forster R, Lipp M, Lanzavecchia A. Two subsets of memory T lymphocytes with distinct homing potentials and effector functions. Nature (1999) 401:708–12. doi: 10.1038/44385
51. Reinhardt RL, Khoruts A, Merica R, Zell T, Jenkins MK. Visualizing the generation of memory CD4 T cells in the whole body. Nature (2001) 410:101–5. doi: 10.1038/35065111
52. Sackstein R, Borenstein M. The effects of corticosteroids on lymphocyte recirculation in humans: analysis of the mechanism of impaired lymphocyte migration to lymph node following methylprednisolone administration. J Investig Med (1995) 43:68–77.
53. Ruutu T, Hermans J, van Biezen A, Niederwieser D, Gratwohl A, Apperley JF. How should corticosteroids be used in the treatment of acute GVHD? EBMT Chronic Leukemia Working Party. European Group for Blood and Marrow Transplantation. Bone Marrow Transplant (1998) 22:614–5. doi: 10.1038/sj.bmt.1701377
54. Stewart BL, Storer B, Storek J, Deeg HJ, Storb R, Hansen JA, et al. Duration of immunosuppressive treatment for chronic graft-versus-host disease. Blood (2004) 104:3501–6. doi: 10.1182/blood-2004-01-0200
55. Van Lint MT, Milone G, Leotta S, Uderzo C, Scime R, Dallorso S, et al. Treatment of acute graft-versus-host disease with prednisolone: significant survival advantage for day +5 responders and no advantage for nonresponders receiving anti-thymocyte globulin. Blood (2006) 107:4177–81. doi: 10.1182/blood-2005-12-4851
56. Zeiser R, Lee SJ. Three US Food and Drug Administration-approved therapies for chronic GVHD. Blood (2022) 139:1642–5. doi: 10.1182/blood.2021014448
57. Murai M, Yoneyama H, Ezaki T, Suematsu M, Terashima Y, Harada A, et al. Peyer’s patch is the essential site in initiating murine acute and lethal graft-versus-host reaction. Nat Immunol (2003) 4:154–60. doi: 10.1038/ni879
58. Koyama M, Mukhopadhyay P, Schuster IS, Henden AS, Hulsdunker J, Varelias A, et al. MHC class II antigen presentation by the intestinal epithelium initiates graft-versus-host disease and is influenced by the microbiota. Immunity (2019) 51:885–898 e7. doi: 10.1016/j.immuni.2019.08.011
59. Fu YY, Egorova A, Sobieski C, Kuttiyara J, Calafiore M, Takashima S, et al. T cell recruitment to the intestinal stem cell compartment drives immune-mediated intestinal damage after allogeneic transplantation. Immunity (2019) 51:90–103 e3. doi: 10.1016/j.immuni.2019.06.003
60. Koyama M, Hill GR. The primacy of gastrointestinal tract antigen-presenting cells in lethal graft-versus-host disease. Blood (2019) 134:2139–48. doi: 10.1182/blood.2019000823
61. Jamy O, Zeiser R, Chen YB. Novel developments in the prophylaxis and treatment of acute GVHD. Blood (2023) 142:1037–46. doi: 10.1182/blood.2023020073
62. Oppermann M. Chemokine receptor CCR5: insights into structure, function, and regulation. Cell Signal (2004) 16:1201–10. doi: 10.1016/j.cellsig.2004.04.007
63. Serody JS, Burkett SE, Panoskaltsis-Mortari A, Ng-Cashin J, McMahon E, Matsushima GK, et al. T-lymphocyte production of macrophage inflammatory protein-1alpha is critical to the recruitment of CD8(+) T cells to the liver, lung, and spleen during graft-versus-host disease. Blood (2000) 96:2973–80. doi: 10.1182/blood.V96.9.2973
64. New JY, Li B, Koh WP, Ng HK, Tan SY, Yap EH, et al. T cell infiltration and chemokine expression: relevance to the disease localization in murine graft-versus-host disease. Bone Marrow Transplant (2002) 29:979–86. doi: 10.1038/sj.bmt.1703563
65. Murai M, Yoneyama H, Harada A, Yi Z, Vestergaard C, Guo B, et al. Active participation of CCR5(+)CD8(+) T lymphocytes in the pathogenesis of liver injury in graft-versus-host disease. J Clin Invest (1999) 104:49–57. doi: 10.1172/JCI6642
66. Reshef R, Luger SM, Hexner EO, Loren AW, Frey NV, Nasta SD, et al. Blockade of lymphocyte chemotaxis in visceral graft-versus-host disease. N Engl J Med (2012) 367:135–45. doi: 10.1056/NEJMoa1201248
67. Bolanos-Meade J, Reshef R, Fraser R, Fei M, Abhyankar S, Al-Kadhimi Z, et al. Three prophylaxis regimens (tacrolimus, mycophenolate mofetil, and cyclophosphamide; tacrolimus, methotrexate, and bortezomib; or tacrolimus, methotrexate, and maraviroc) versus tacrolimus and methotrexate for prevention of graft-versus-host disease with haemopoietic cell transplantation with reduced-intensity conditioning: a randomised phase 2 trial with a non-randomised contemporaneous control group (BMT CTN 1203). Lancet Haematol (2019) 6:e132–43. doi: 10.1016/S2352-3026(18)30221-7
68. Roy NH, MacKay JL, Robertson TF, Hammer DA, Burkhardt JK. Crk adaptor proteins mediate actin-dependent T cell migration and mechanosensing induced by the integrin LFA-1. Sci Signal 11 (2018) 11:eaat3178. doi: 10.1126/scisignal.aat3178
69. Roy NH, Mammadli M, Burkhardt JK, Karimi M. CrkL is required for donor T cell migration to GvHD target organs. Oncotarget (2020) 11:1505–14. doi: 10.18632/oncotarget.27509
70. Bravo GA, Cedeno RR, Casadevall MP, Ramio-Torrenta L. Sphingosine-1-phosphate (S1P) and S1P signaling pathway modulators, from current insights to future perspectives. Cells (2022) 11:2058. doi: 10.3390/cells11132058
71. Mandala S, Hajdu R, Bergstrom J, Quackenbush E, Xie J, Milligan J, et al. Alteration of lymphocyte trafficking by sphingosine-1-phosphate receptor agonists. Science (2002) 296:346–9. doi: 10.1126/science.1070238
72. Matloubian M, Lo CG, Cinamon G, Lesneski MJ, Xu Y, Brinkmann V, et al. Lymphocyte egress from thymus and peripheral lymphoid organs is dependent on S1P receptor 1. Nature (2004) 427:355–60. doi: 10.1038/nature02284
73. Kim YM, Sachs T, Asavaroengchai W, Bronson R, Sykes M. Graft-versus-host disease can be separated from graft-versus-lymphoma effects by control of lymphocyte trafficking with FTY720. J Clin Invest (2003) 111:659–69. doi: 10.1172/JCI200316950
74. Hashimoto D, Asakura S, Matsuoka K, Sakoda Y, Koyama M, Aoyama K, et al. FTY720 enhances the activation-induced apoptosis of donor T cells and modulates graft-versus-host disease. Eur J Immunol (2007) 37:271–81. doi: 10.1002/eji.200636123
75. Tedesco-Silva H, Szakaly P, Shoker A, Sommerer C, Yoshimura N, Schena FP, et al. FTY720 versus mycophenolate mofetil in de novo renal transplantation: six-month results of a double-blind study. Transplantation (2007) 84:885–92. doi: 10.1097/01.tp.0000281385.26500.3b
76. Yokoyama E, Hashimoto D, Hayase E, Ara T, Ogasawara R, Takahashi S, et al. Short-term KRP203 and posttransplant cyclophosphamide for graft-versus-host disease prophylaxis. Bone Marrow Transplant (2020) 55:787–95. doi: 10.1038/s41409-019-0733-8
77. Dertschnig S, Passweg J, Bucher C, Medinger M, Tzankov A. Mocravimod, a S1P receptor modulator, increases T cell counts in bone marrow biopsies from patients undergoing allogeneic hematopoietic stem cell transplantation. Cell Immunol (2023) 388–9:104719. doi: 10.1016/j.cellimm.2023.104719
78. Dertschnig S, Gergely P, Finke J, Schanz U, Holler E, Holtick U, et al. Mocravimod, a selective sphingosine-1-phosphate receptor modulator, in allogeneic hematopoietic stem cell transplantation for Malignancy. Transplant Cell Ther (2023) 29:e1–41 e9. doi: 10.1016/j.jtct.2022.10.029
79. Zeiser R, von Bubnoff N, Butler J, Mohty M, Niederwieser D, Or R, et al. Ruxolitinib for glucocorticoid-refractory acute graft-versus-host disease. N Engl J Med (2020) 382:1800–10. doi: 10.1056/NEJMoa1917635
80. Zeiser R, Teshima T, Locatelli F. Ruxolitinib for chronic graft-versus-host disease. Reply N Engl J Med (2021) 385:1631–2. doi: 10.1056/NEJMc2113499
81. Carniti C, Gimondi S, Vendramin A, Recordati C, Confalonieri D, Bermema A, et al. Pharmacologic inhibition of JAK1/JAK2 signaling reduces experimental murine acute GVHD while preserving GVT effects. Clin Cancer Res: Off J Am Assoc Cancer Res (2015) 21:3740–9. doi: 10.1158/1078-0432.CCR-14-2758
82. Choi J, Ziga ED, Ritchey J, Collins L, Prior JL, Cooper ML, et al. IFNgammaR signaling mediates alloreactive T-cell trafficking and GVHD. Blood (2012) 120:4093–103. doi: 10.1182/blood-2012-01-403196
83. Sacirbegovic F, Gunther M, Greco A, Zhao D, Wang X, Zhou M, et al. Graft-versus-host disease is locally maintained in target tissues by resident progenitor-like T cells. Immunity (2023) 56:369–385 e6. doi: 10.1016/j.immuni.2023.01.003
84. DeWolf S, Elhanati Y, Nichols K, Waters NR, Nguyen CL, Slingerland JB, et al. Tissue-specific features of the T cell repertoire after allogeneic hematopoietic cell transplantation in human and mouse. Sci Trans Med (2023) 15:eabq0476. doi: 10.1126/scitranslmed.abq0476
85. Strobl J, Pandey RV, Krausgruber T, Bayer N, Kleissl L, Reininger B, et al. Long-term skin-resident memory T cells proliferate in situ and are involved in human graft-versus-host disease. Sci Trans Med (2020) 12:eabb7028. doi: 10.1126/scitranslmed.abb7028
86. Takahashi S, Hashimoto D, Hayase E, Ogasawara R, Ohigashi H, Ara T, et al. Ruxolitinib protects skin stem cells and maintains skin homeostasis in murine graft-versus-host disease. Blood (2018) 131:2074–85. doi: 10.1182/blood-2017-06-792614
87. Abraham A, Roga G. Topical steroid-damaged skin. Indian J Dermatol (2014) 59:456–9. doi: 10.4103/0019-5154.139872
88. Du J, Paz K, Flynn R, Vulic A, Robinson TM, Lineburg KE, et al. Pirfenidone ameliorates murine chronic GVHD through inhibition of macrophage infiltration and TGF-beta production. Blood (2017) 129:2570–80. doi: 10.1182/blood-2017-01-758854
89. Yamakawa T, Ohigashi H, Hashimoto D, Hayase E, Takahashi S, Miyazaki M, et al. Vitamin A-coupled liposomes containing siRNA against HSP47 ameliorate skin fibrosis in chronic graft-versus-host disease. Blood (2018) 131:1476–85. doi: 10.1182/blood-2017-04-779934
90. Alexander KA, Flynn R, Lineburg KE, Kuns RD, Teal BE, Olver SD, et al. CSF-1-dependant donor-derived macrophages mediate chronic graft-versus-host disease. J Clin Invest (2014) 124:4266–80. doi: 10.1172/JCI75935
91. Kitko CL, Arora M, DeFilipp Z, Zaid MA, Di Stasi A, Radojcic V, et al. Axatilimab for chronic graft-versus-host disease after failure of at least two prior systemic therapies: results of a phase I/II study. J Clin Oncol (2023) 41:1864–75. doi: 10.1200/JCO.22.00958
92. Ogawa Y, Yamazaki K, Kuwana M, Mashima Y, Nakamura Y, Ishida S, et al. A significant role of stromal fibroblasts in rapidly progressive dry eye in patients with chronic GVHD. Invest Ophthalmol Vis Sci (2001) 42:111–9.
93. Ohigashi H, Hashimoto D, Hayase E, Takahashi S, Ara T, Yamakawa T, et al. Ocular instillation of vitamin A-coupled liposomes containing HSP47 siRNA ameliorates dry eye syndrome in chronic GVHD. Blood Adv (2019) 3:1003–10. doi: 10.1182/bloodadvances.2018028431
94. Wu SR, Reddy P. Tissue tolerance: a distinct concept to control acute GVHD severity. Blood (2017) 129:1747–52. doi: 10.1182/blood-2016-09-740431
95. Chakraverty R, Teshima T. Graft-versus-host disease: a disorder of tissue regeneration and repair. Blood (2021) 138:1657–65. doi: 10.1182/blood.2021011867
96. Ara T, Hashimoto D. Novel insights into the mechanism of GVHD-induced tissue damage. Front Immunol (2021) 12:713631. doi: 10.3389/fimmu.2021.713631
97. Takashima S, Kadowaki M, Aoyama K, Koyama M, Oshima T, Tomizuka K, et al. The Wnt agonist R-spondin1 regulates systemic graft-versus-host disease by protecting intestinal stem cells. J Exp Med (2011) 208:285–94. doi: 10.1084/jem.20101559
98. Hanash AM, Dudakov JA, Hua G, O’Connor MH, Young LF, Singer NV, et al. Interleukin-22 protects intestinal stem cells from immune-mediated tissue damage and regulates sensitivity to graft versus host disease. Immunity (2012) 37:339–50. doi: 10.1016/j.immuni.2012.05.028
99. Takashima S, Martin ML, Jansen SA, Fu Y, Bos J, Chandra D, et al. T cell-derived interferon-gamma programs stem cell death in immune-mediated intestinal damage. Sci Immunol (2019) 4:eaay8556. doi: 10.1126/sciimmunol.aay8556
100. Wu N, Sun H, Zhao X, Zhang Y, Tan J, Qi Y, et al. MAP3K2-regulated intestinal stromal cells define a distinct stem cell niche. Nature (2021) 592:606–10. doi: 10.1038/s41586-021-03283-y
101. Shoshkes-Carmel M, Wang YJ, Wangensteen KJ, Toth B, Kondo A, Massasa EE, et al. Subepithelial telocytes are an important source of Wnts that supports intestinal crypts. Nature (2018) 557:242–6. doi: 10.1038/s41586-018-0084-4
102. Ogasawara R, Hashimoto D, Kimura S, Hayase E, Ara T, Takahashi S, et al. Intestinal lymphatic endothelial cells produce R-spondin3. Sci Rep (2018) 8:10719. doi: 10.1038/s41598-018-29100-7
103. Niec RE, Chu T, Schernthanner M, Gur-Cohen S, Hidalgo L, Pasolli HA, et al. Lymphatics act as a signaling hub to regulate intestinal stem cell activity. Cell Stem Cell (2022) 29:1067–1082 e18. doi: 10.1016/j.stem.2022.05.007
104. Goto N, Goto S, Imada S, Hosseini S, Deshpande V, Yilmaz OH. Lymphatics and fibroblasts support intestinal stem cells in homeostasis and injury. Cell Stem Cell (2022) 29:1246–1261 e6. doi: 10.1016/j.stem.2022.06.013
105. Hayase E, Hashimoto D, Nakamura K, Noizat C, Ogasawara R, Takahashi S, et al. R-Spondin1 expands Paneth cells and prevents dysbiosis induced by graft-versus-host disease. J Exp Med (2017) 214:3507–18. doi: 10.1084/jem.20170418
106. Norona J, Apostolova P, Schmidt D, Ihlemann R, Reischmann N, Taylor G, et al. Glucagon-like peptide 2 for intestinal stem cell and Paneth cell repair during graft-versus-host disease in mice and humans. Blood (2020) 136:1442–55. doi: 10.1182/blood.2020005957
107. Lindemans CA, Calafiore M, Mertelsmann AM, O’Connor MH, Dudakov JA, Jenq RR, et al. Interleukin-22 promotes intestinal-stem-cell-mediated epithelial regeneration. Nature (2015) 528:560–4. doi: 10.1038/nature16460
108. Ponce DM, Alousi AM, Nakamura R, Slingerland J, Calafiore M, Sandhu KS, et al. A phase 2 study of interleukin-22 and systemic corticosteroids as initial treatment for acute GVHD of the lower GI tract. Blood (2023) 141:1389–401. doi: 10.1182/blood.2021015111
109. Ara T, Hashimoto D, Hayase E, Noizat C, Kikuchi R, Hasegawa Y, et al. Intestinal goblet cells protect against GVHD after allogeneic stem cell transplantation via Lypd8. Sci Trans Med (2020) 12:eaaw0720. doi: 10.1126/scitranslmed.aaw0720
110. Holtan SG, Hoeschen A, Cao Q, Ustun C, Betts BC, Jurdi NE, et al. Open-label clinical trial of urinary-derived human chorionic gonadotropin/epidermal growth factor for life-threatening acute graft-versus-host disease. Transplant Cell Ther (2023) 29:509 e1–8. doi: 10.1016/j.jtct.2023.05.021
111. Dufva O, Polonen P, Bruck O, Keranen MAI, Klievink J, Mehtonen J, et al. Immunogenomic landscape of hematological Malignancies. Cancer Cell (2020) 38:380–399 e13. doi: 10.1016/j.ccell.2020.08.019
112. Mathew NR, Baumgartner F, Braun L, O’Sullivan D, Thomas S, Waterhouse M, et al. Sorafenib promotes graft-versus-leukemia activity in mice and humans through IL-15 production in FLT3-ITD-mutant leukemia cells. Nat Med (2018) 24:282. doi: 10.1038/nm.4484
113. Hong E, Usiskin IM, Bergamaschi C, Hanlon DJ, Edelson RL, Justesen S, et al. Configuration-dependent presentation of multivalent IL-15:IL-15Ralpha enhances the antigen-specific T cell response and anti-tumor immunity. J Biol Chem (2016) 291:8931–50. doi: 10.1074/jbc.M115.695304
114. Zhang Z, Hasegawa Y, Hashimoto D, Senjo H, Kikuchi R, Chen X, et al. Gilteritinib enhances graft-versus-leukemia effects against FLT3-ITD mutant leukemia after allogeneic hematopoietic stem cell transplantation. Bone Marrow Transplant (2022) 57:775–80. doi: 10.1038/s41409-022-01619-4
115. Notarangelo G, Spinelli JB, Perez EM, Baker GJ, Kurmi K, Elia I, et al. Oncometabolite d-2HG alters T cell metabolism to impair CD8(+) T cell function. Science (2022) 377:1519–29. doi: 10.1126/science.abj5104
Keywords: graft-versus-leukemia, graft-versus-host disease, intestine, tumor immune environment, tissue tolerance
Citation: Teshima T and Hashimoto D (2023) Separation of GVL from GVHD -location, location, location. Front. Immunol. 14:1296663. doi: 10.3389/fimmu.2023.1296663
Received: 18 September 2023; Accepted: 21 November 2023;
Published: 05 December 2023.
Edited by:
Olaf Penack, Charité University Medicine Berlin, GermanyReviewed by:
Luca Castagna, Azienda Ospedaliera Ospedali Riuniti Villa Sofia Cervello, ItalyCopyright © 2023 Teshima and Hashimoto. This is an open-access article distributed under the terms of the Creative Commons Attribution License (CC BY). The use, distribution or reproduction in other forums is permitted, provided the original author(s) and the copyright owner(s) are credited and that the original publication in this journal is cited, in accordance with accepted academic practice. No use, distribution or reproduction is permitted which does not comply with these terms.
*Correspondence: Takanori Teshima, dGVzaGltYUBtZWQuaG9rdWRhaS5hYy5qcA==
Disclaimer: All claims expressed in this article are solely those of the authors and do not necessarily represent those of their affiliated organizations, or those of the publisher, the editors and the reviewers. Any product that may be evaluated in this article or claim that may be made by its manufacturer is not guaranteed or endorsed by the publisher.
Research integrity at Frontiers
Learn more about the work of our research integrity team to safeguard the quality of each article we publish.