- 1Liaocheng Research Institute of Donkey High-efficiency Breeding and Ecological Feeding, Liaocheng University, Liaocheng, China
- 2College of Life Sciences, Liaocheng University, Liaocheng, China
- 3Faculty of Veterinary and Animal Sciences, University of Agriculture, Dera Ismail Khan, Pakistan
- 4College of Life Science, Anhui Agricultural University, Hefei, Anhui, China
- 5Genome Analysis Laboratory of the Ministry of Agriculture, Agricultural Genomics Institute at Shenzhen, Chinese Academy of Agricultural Sciences, Shenzhen, China
Mastitis, the inflammatory condition of mammary glands, has been closely associated with immune suppression and imbalances between antioxidants and free radicals in cattle. During the periparturient period, dairy cows experience negative energy balance (NEB) due to metabolic stress, leading to elevated oxidative stress and compromised immunity. The resulting abnormal regulation of reactive oxygen species (ROS) and reactive nitrogen species (RNS), along with increased non-esterified fatty acids (NEFA) and β-hydroxybutyric acid (BHBA) are the key factors associated with suppressed immunity thereby increases susceptibility of dairy cattle to infections, including mastitis. Metabolic diseases such as ketosis and hypocalcemia indirectly contribute to mastitis vulnerability, exacerbated by compromised immune function and exposure to physical injuries. Oxidative stress, arising from disrupted balance between ROS generation and antioxidant availability during pregnancy and calving, further contributes to mastitis susceptibility. Metabolic stress, marked by excessive lipid mobilization, exacerbates immune depression and oxidative stress. These factors collectively compromise animal health, productive efficiency, and udder health during periparturient phases. Numerous studies have investigated nutrition-based strategies to counter these challenges. Specifically, amino acids, trace minerals, and vitamins have emerged as crucial contributors to udder health. This review comprehensively examines their roles in promoting udder health during the periparturient phase. Trace minerals like copper, selenium, and calcium, as well as vitamins; have demonstrated significant impacts on immune regulation and antioxidant defense. Vitamin B12 and vitamin E have shown promise in improving metabolic function and reducing oxidative stress followed by enhanced immunity. Additionally, amino acids play a pivotal role in maintaining cellular oxidative balance through their involvement in vital biosynthesis pathways. In conclusion, addressing periparturient mastitis requires a holistic understanding of the interplay between metabolic stress, immune regulation, and oxidative balance. The supplementation of essential amino acids, trace minerals, and vitamins emerges as a promising avenue to enhance udder health and overall productivity during this critical phase. This comprehensive review underscores the potential of nutritional interventions in mitigating periparturient bovine mastitis and lays the foundation for future research in this domain.
1 Introduction
Mastitis, characterized by the inflammation of mammary glands (1, 2), is intricately associated with immune suppression and an imbalance between free radicals and antioxidants within the animal’s physiological framework (3–5). Dairy cows confront the challenge of negative energy balance (NEB) during the periparturient period, precipitating a cascade of detrimental effects such as reduced dry intake, metabolic stress, hormonal fluctuations, heightened oxidative stress, and compromised immune responses (6, 7).
This NEB triggers the mobilization of fat reserves, culminating in the dysregulation of reactive oxygen species (ROS) and reactive nitrogen species (RNS) (8), along with elevated levels of non-esterified fatty acids (NEFA) (9, 10) and β-hydroxybutyric acid (BHBA) (11). The notable concentrations of NEFA and BHB compromise the bovine immune system, rendering dairy cattle more susceptible to infections (12). Scientific investigations have demonstrated that heightened NEFA and BHBA levels exert inhibitory effects on bovine peripheral blood mononuclear cells (BPMCs) (13), stifle interferon production (14), and impede the functionality of polymorphonuclear neutrophils (PMNLs) (15). Furthermore, escalated levels of NEFA and BHB serve as indicators of mastitis susceptibility (16, 17). During the periparturient period, the heightened concentrations of NEFA and BHB emerge as pivotal factors undermining immune function, directly augmenting the vulnerability to mastitis (11).
In addition to NEB-related factors, other metabolic disorders like ketosis and hypocalcemia indirectly contribute to mastitis in dairy cattle (18–20). Hypocalcemia prompts cows to spend more time lying down, resulting in teat exposure to physical injury and facilitating pathogen entry. The compromised sphincter muscle integrity of teats and diminished immune functionality due to reduced calcium levels emerge as pivotal factors linking mastitis with hypocalcemia.
In the realm of normal physiological conditions, the intricate antioxidant system adeptly mitigates and eradicates ROS stemming from metabolic processes. Nevertheless, the transitions accompanying pregnancy and calving instigate an overabundance of ROS production (21, 22). This disruption in the equilibrium between ROS generation and the availability of antioxidants ushers in oxidative stress, rendering cattle more susceptible to a spectrum of maladies (23, 24). The unrestrained ROS production culminates in lipid peroxidation, tissue impairment, and fluctuations in reduced glutathione (GSH) levels, a pivotal constituent of glutathione metabolism (24, 25). Oxidative stress inflicts damage upon the structure and function of cellular macromolecules, including lipids, proteins, and nucleic acids, thereby inciting metabolic dysfunctions and ailments, notably mastitis in dairy cattle (6, 26). Sustaining redox homeostasis during the periparturient and peak lactation phases is of paramount importance (27–29). Oxidative stress associated with parturition may contribute to immunological and inflammatory aberrations, heightening the vulnerability to metabolic and infectious disorders (22, 30).
The metabolic stress encountered during the periparturient period stands as another pivotal factor exposing animals to immune suppression and the deviant regulation of oxidative stress. This phase instigates excessive lipid mobilization, subsequently leading to oxidative stress (16). The abnormal regulation of immunity and inflammation driven by metabolic and oxidative stress constitutes the third critical factor predisposing dairy cattle to periparturient mastitis (3, 31–33). Furthermore, oxidative and metabolic stress, negative energy balance, immune suppression, and diminished productive efficiency collectively undermine the overall productivity of these animals (34–36).
Numerous nutritional strategies have been employed to address the challenges encountered during the periparturient period in dairy cattle (37–39). Extensive research endeavors have been undertaken to probe the intricate interplay between nutrition, immune modulation, and the augmentation of antioxidant status, thereby fostering enhanced udder health during this critical phase (40–45). O’Rourke (41), in particular, highlighted that cows undergoing negative energy balance face an elevated risk of ketosis, with clinical ketosis being associated with a twofold increase in the likelihood of clinical mastitis. Furthermore, extant research underscores the pivotal role of nutritional disturbances during the periparturient period in contributing significantly to immune suppression, oxidative stress, and metabolic perturbations (46–48).
Among the pivotal nutrients that have garnered attention, specific amino acids, trace minerals, and vitamins have exhibited a pronounced impact on udder health during the periparturient period, particularly in the prevention of mastitis in dairy cattle. Consequently, this review aims to provide a comprehensive exploration of the contributions made by trace minerals, vitamins, and amino acids in bolstering udder health during the critical periparturient phase in dairy cattle.
2 Key factors associated with periparturient bovine mastitis
2.1 Metabolic stress, NEFA and BHBA
The NEFA and BHBA represent metabolites resulting from the mobilization of fat reserves triggered by NEB during the perinatal period in dairy cows, exerting detrimental effects on the cellular physiology of various bovine cell types (12). Severe NEB initiates lipid mobilization, leading to elevated circulating concentrations of NEFA and BHBA. Clinical data derived from previous studies have established a strong correlation between heightened levels of NEFA and BHBA and an increased incidence of postpartum diseases, including mastitis, ketosis, clinical endometritis, metritis, and other conditions associated with immunosuppression. These conditions have adverse repercussions on the overall health, longevity, as well as the productive and reproductive performances of dairy cows (11, 49).
Furthermore, a study conducted by Li et al. revealed that NEFA and BHBA lead to increased accumulation of malondialdehyde (MDA) and ROS, along with reduced total superoxide dismutase (T-SOD) and glutathione peroxidase (GSH-Px) activity, resulting in oxidative stress. Additionally, NEFA and BHBA stimulation led to heightened expression of inflammatory markers such as nitric oxide (NO), tumor necrosis factor-alpha (TNF-α), interleukin-6 (IL-6) and interleukin-1 beta (IL-1β). Mechanistically, their data demonstrated that NEFA and BHBA activate the mitogen-activated protein kinase (MAPK) signaling pathway, shedding light on the fact that NEFA and BHBA induce oxidative stress and an inflammatory response, potentially via the MAPK signaling pathway in BMECs (12). Similarly, another study observed that elevated NEFA levels increased ROS levels, thereby activating the MAPK signaling pathway and triggering ER stress-mediated apoptosis in BMECs (50).
2.2 Oxidative stress
Oxidative stress induced by metabolic stress is closely associated with several pathological conditions, including mastitis, during the periparturient period in dairy cattle (26, 51, 52). Consistently, Sordillo and Aitken (3) reported that oxidative stress resulting from negative energy balance is intricately linked with impaired immunity, subsequently leading to heightened inflammation, thereby rendering dairy cattle more susceptible to mastitis (3, 53; 54). In a similar vein, a study noted that selenium supplementation effectively enhanced the total antioxidant capacity of dairy cattle, consequently mitigating the risk of mastitis in periparturient dairy cattle (55). Additionally, when BMECs were exposed to lipopolysaccharide (LPS), it was observed that ROS levels increased, accompanied by inflammatory changes (56, 57). In Table 1, we present a comprehensive summary of recent published research findings that elucidate the intricate relationship between oxidative stress and mastitis in dairy cattle during the critical periparturient period. The interplay among multiple factors, including metabolic stress/oxidative stress, immunity, inflammation, NEB, and susceptibility to mastitis, has been depicted and summarized in Figure 1.
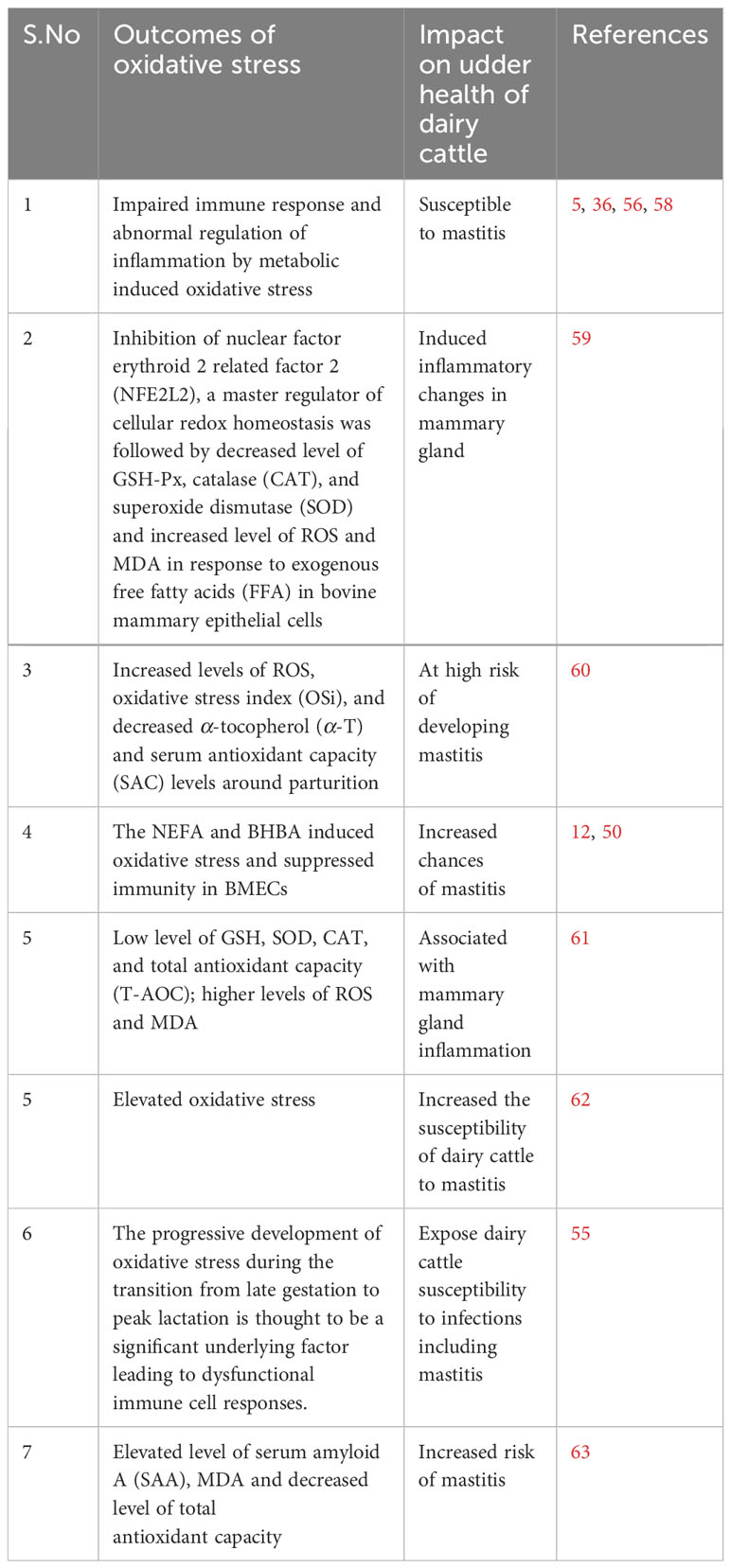
Table 1 Recent studies reported association of metabolic disturbances, suppressed immunity and oxidative stress with periparturient bovine mastitis.
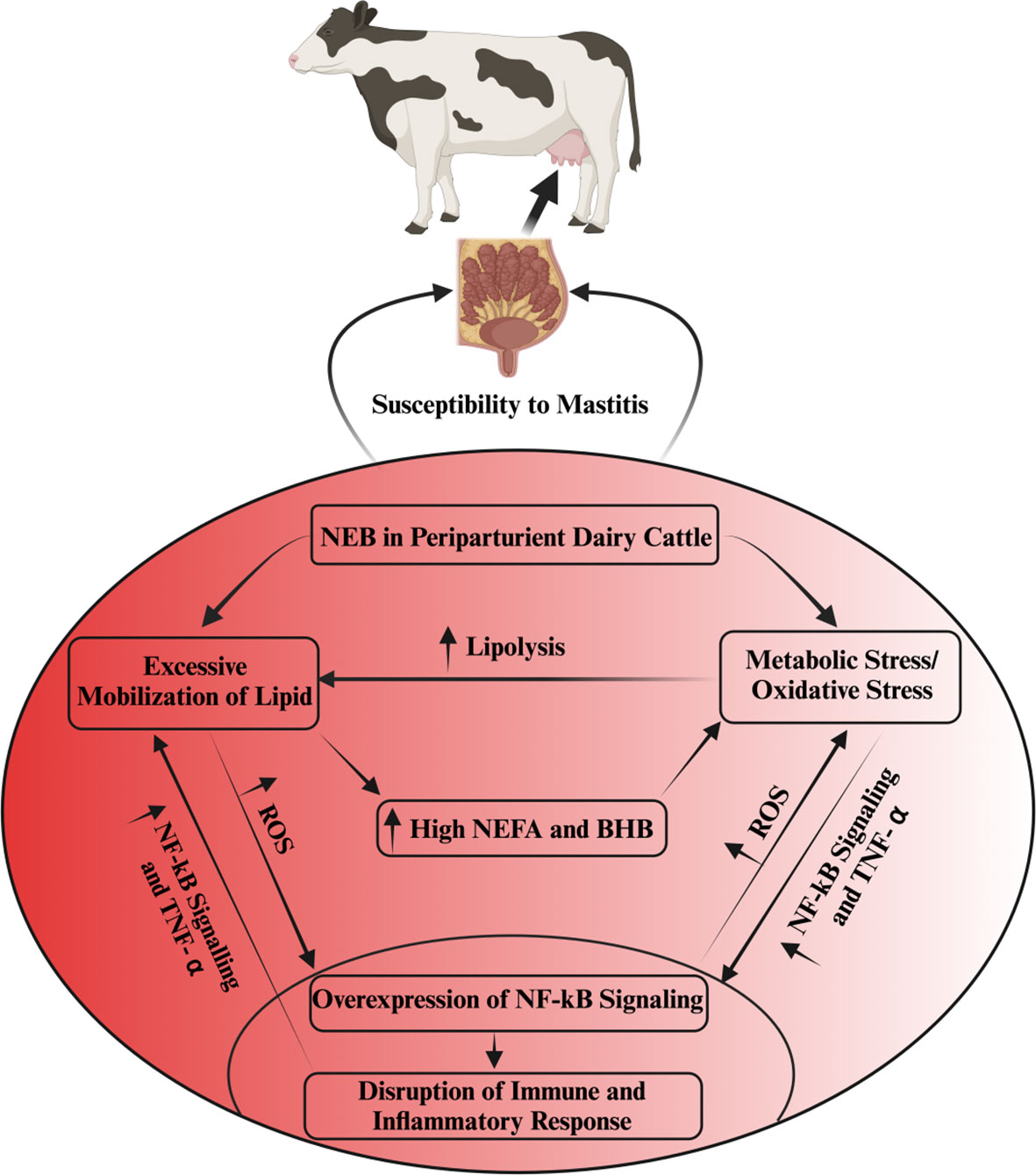
Figure 1 The interlink among metabolic stress, immunity, inflammation and mastitis susceptibility. Oxidative stress disrupts immune and inflammatory functions via the activation of NF-kB signaling. This aberrant inflammatory regulation, in turn, fosters excessive TNF-α production in non-phagocytic cells, resulting in heightened oxidative stress and increased lipolysis. The intricate interplay of oxidative stress, escalated lipid mobilization, and compromised immune and inflammatory processes is predominantly associated with NEB in periparturient dairy cattle. NEB, by triggering an excessive lipid mobilization in these cattle, elevates NEFAs, BHB and ROS, consequently inducing oxidative stress. This oxidative stress further exacerbates the disruption of immunity and inflammation regulation, rendering dairy cattle more susceptible to mastitis.
3 Trace minerals role in bovine mastitis prevention during periparturient period
3.1 Reference values for serum trace minerals in dairy cows
To establish appropriate dietary recommendations, it is essential to consider reference values for serum trace mineral concentrations in dairy cows. Notable values include: calcium (2.2–2.6 mmol/L) (64), phosphorus (1.3–2.6 mmol/L) (64), magnesium (0.75–1.0 mmol/L) (65), selenium (0.73–1.08 µmol/L) (66), copper (1–18 µmol/L) (67), and zinc (8–19 µmol/L) (68). Meeting these reference values can help optimize the mineral supply and overall health of dairy cattle.
3.2 Role of trace minerals in health regulations of dairy cattle
In the field of bovine veterinary medicine, the pivotal role of mineral deficiencies in modulating the immune system should not be underestimated. Recent investigations have elucidated that the provision of micronutrient supplements has demonstrably reduced the count of milk somatic cells (SCC), bolstered immune function, and elicited anti-inflammatory and antioxidant responses in dairy cattle during the periparturient period (69, 70). Extensive research has shown that these deficiencies can lead to immunosuppression, making the animals more susceptible to infectious diseases such as mastitis (42). Consequently, addressing mineral imbalances in cattle health becomes crucial in maintaining optimal health outcomes. Minerals typically constitute essential structural components within the body, acting as crucial cofactors for diverse enzymes and participating in vital processes such as nerve signaling, muscle contraction, and the regulation of proper keratosis. Insufficient mineral levels can result in diminished immune cell activity or disruption of innate defense mechanisms within the breast, thereby fostering the progression of mastitis (71). Recent studies have shown promising results regarding the supplementation of trace minerals and its effect on dairy cattle health by enhancing their immune and antioxidant status during the periparturient period (72–74). Trace mineral supplementation has been found to significantly enhance immune and antioxidant status, contributing to reduced levels of NEFA (75) and alleviating inflammatory changes, which are critical factors associated with clinical mastitis (76–80). These studies emphasize the potential of mineral supplementation as a strategy to improve cattle health and overall herd performance. Minerals play a fundamental role in maintaining the immune system of cattle. Recent studies have highlighted the association between serum trace mineral concentrations and their impact on bovine health. For instance, higher concentrations of serum selenium and phosphorus have been linked to the successful cure of bovine clinical mastitis (81). Similarly, research demonstrated that subcutaneous supplementation of specific minerals, such as zinc, manganese, selenium, and copper, led to increased SOD activity, decreased serum BHBA concentrations, reduced milk SCC, and a lower incidence of mastitis (82). These findings underline the vital role of trace minerals in preventing and mitigating the effects of mastitis in dairy cattle.
In clinical practice, certain mastitis cases may require supportive therapy, including the administration of calcium-containing fluids, due to the prevalent occurrence of hypocalcemia in cows with udder inflammation (83). Additionally, the supplementary use of injectable trace minerals, such as zinc, manganese, selenium, and copper, has been considered as an adjunctive tool in mastitis therapy. Clinical evidence suggests that supportive therapy involving the administration of fluids enriched with calcium can be crucial in managing mastitis in dairy cows (83). Hypocalcemia, commonly observed in cows with udder inflammation, can compromise immune function and hindering the healing process. Proper calcium supplementation has shown promise in improving the overall therapeutic outcomes in mastitis cases. Recent studies have investigated the use of injectable trace minerals as a complementary approach in the treatment of mastitis in dairy cows. Hoque et al. (84) emphasized the significance of antimicrobial therapy as the primary treatment for mastitis. However, their experiment revealed a noteworthy finding – cows that received only selenium preparations demonstrated reduced susceptibility to udder inflammation compared to the untreated control group. Ganda et al. (81) conducted a study involving the injection of trace minerals, including zinc, manganese, selenium, and copper, in dairy cows with mastitis. The results indicated a reduction in the number of chronic mastitis cases, showcasing the potential of injectable trace minerals in mitigating the severity and persistence of the disease.
Another noteworthy study by Machado et al. (85) explored the effects of injecting a multimineral preparation containing selenium, copper, zinc, and manganese. Their findings demonstrated a positive impact on udder health, leading to a decrease in linear SCC, and a reduction in the incidence of subclinical and clinical mastitis cases. Furthermore, the researchers reported an increase in serum SOD activity, indicating potential antioxidant benefits without affecting leukocyte function (82). Despite the positive effects observed in other studies, Ferreira and Petzer (86) reported no significant correlation between SCC and the levels of selenium in milk or serum among cows supplemented with selenium in various forms. This suggests that other factors might influence the relationship between SCC and selenium supplementation. A separate investigation by Bourne et al. (87) investigated the supplementation of vitamin E with selenium. The study revealed a notable 10% reduction in the risk of culling and mastitis rate, suggesting a potential synergistic effect between vitamin E and selenium in enhancing mastitis treatment outcomes. Recent research conducted by Smulski et al. (88) indicated that combining an antibiotic treatment with an antioxidant containing selenium slightly improves the overall effectiveness of clinical mastitis treatment. This highlights the importance of considering combination therapies to optimize mastitis management in dairy cows. Further investigation is necessary to gain a comprehensive understanding of its effects on cattle health and immune responses. Figure 2 illustrates the pivotal role of trace mineral supplementation in health regulation, encompassing its capacity to enhance immune function, mitigate inflammation, and bolster antioxidant responses among periparturient dairy cattle.
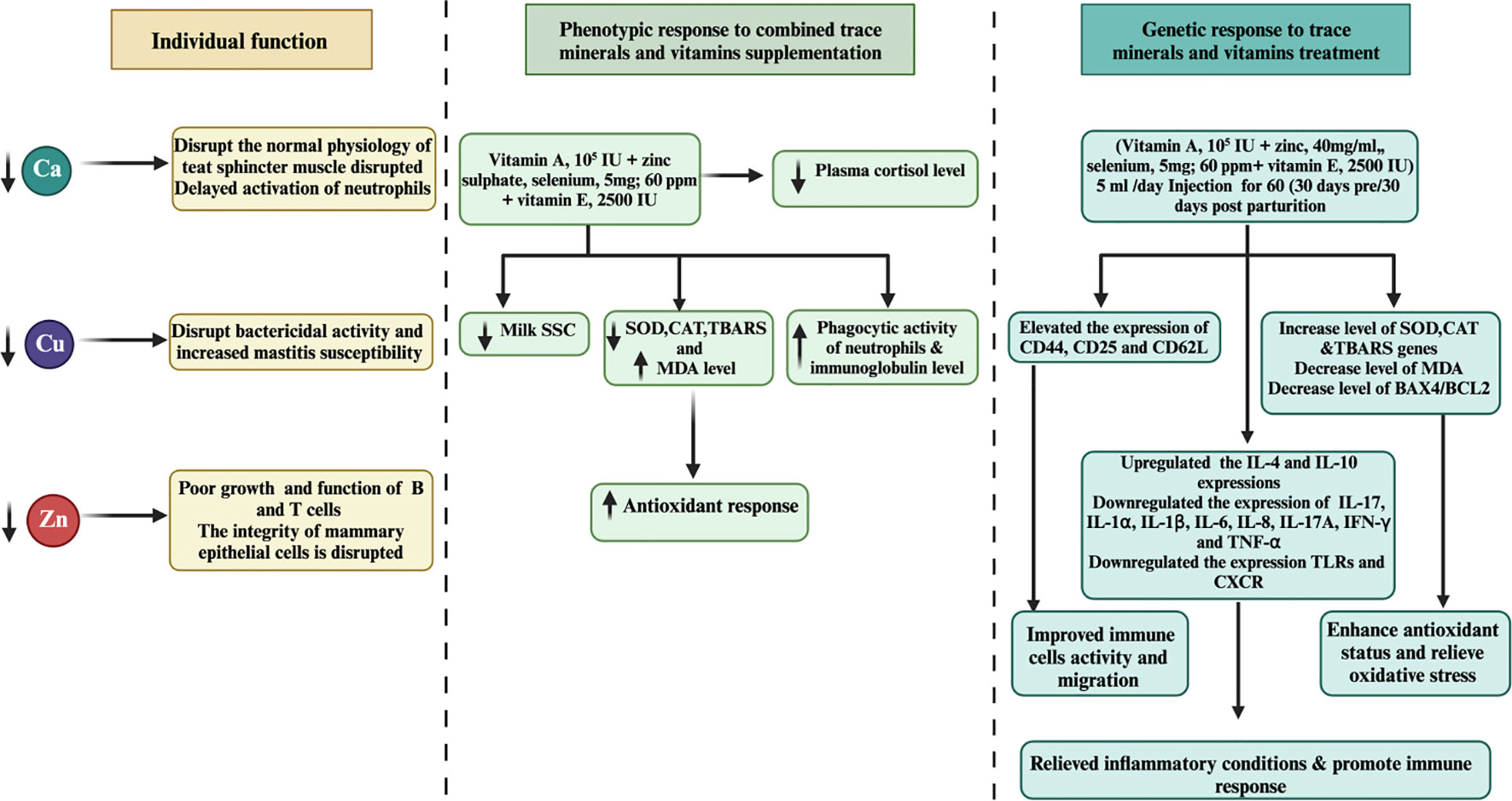
Figure 2 Associations between molecular and phenotypic factors related to immunity, apoptosis, milk SCC, anti-inflammatory responses, and antioxidant activity in dairy cattle following combined treatments with minerals and vitamins are examined. Over a 60-day duration, the combined treatment of minerals and vitamins exhibited notable downregulation of apoptosis and inflammation-related gene expression levels, concomitant with upregulation of genes associated with immunity, antioxidation, and pro-inflammatory responses in periparturient dairy cattle. Furthermore, the integrated regimen of trace minerals and vitamins demonstrated enhancements in neutrophil phagocytic activity, accompanied by reductions in plasma cortisol and milk SCC levels, suggesting a potential association with mastitis prevention during the periparturient period in dairy cattle.
3.3 Specific
3.3.1 Role of calcium, copper, zinc, and selenium in regulating anti-inflammatory and immune responses to prevent mastitis
Calcium assumes a pivotal physiological role within the biology of living organisms, functioning as an indispensable constituent of bodily structures and a critical determinant for muscle contractions, encompassing both skeletal and smooth muscles, such as the mammary gland sphincter. A prominent periparturient metabolic affliction, known as hypocalcemia, arises due to the excessive depletion of calcium in colostrum and milk. Consequently, the requisite calcium level is constrained to remain below 1.5 mmol/L to maintain normal muscle physiology, resulting in afflicted animals assuming a protracted recumbent posture (89). Furthermore, diminished calcium levels also correlate with compromised phagocytic activity of neutrophils and suppressed immunity, predisposing dairy cattle to other periparturient diseases (90, 91). In addition, the incapacity of the mammary gland sphincter muscle to contract leads to prolonged teat opening, creating favorable conditions for pathogenic microorganisms to induce mastitis (92). Notably, a study revealed lower calcium levels in acute Escherichia coli (E.coli) mastitic cows compared to healthy counterparts (93). Moreover, investigations have unveiled that certain polymorphisms (G519663A and G38819398A) within the Calcium channel, voltage-dependent, alpha-2/delta subunit 1 (CACNA2D1) gene are associated with mastitis resistance in Sahiwal cattle (94, 95).
Copper is another trace mineral, plays a crucial role in the structure and catalytic properties of cuproenzymes, which are enzymes that incorporate copper (96). Notable examples of cuproenzymes include cytochrome-c oxidase, superoxide dismutase, catechol oxidase, ceruloplasmin, and amine oxidases. Additionally, copper holds a significant position among enzymes, ranking as the second most prevalent metal after zinc and contributing to their proper functioning (97). Apart from its involvement in enzymatic functions, copper also plays a vital role in various physiological processes. It contributes to essential processes such as collagen and elastin synthesis, which are crucial for tissue structure and elasticity. Furthermore, copper is involved in myelination, a process critical for nerve impulse conduction, and hemoglobin production, which is essential for oxygen transport (96). Copper has been recognized for its antibacterial properties against bacteria commonly isolated from mastitic cows. Research by Reyes-Jara et al. (98) highlights that even low copper concentrations, as low as 250 ppm, can effectively inhibit the growth of mastitis microorganisms like E.coli and coagulase-negative Staphylococci. Supporting studies by Wernicki et al. (99) and Kalińska et al. (100) demonstrate the potent antimicrobial activity of silver and copper nanoparticles against bacteria derived from inflamed udders, indicating copper preparations as potential alternatives to dipping solutions. In vivo investigations have revealed promising results concerning the effects of copper supplementation. A 100-day dietary copper supplementation study, as conducted by Scaletti et al. (101), showed a reduced clinical response in Holstein cows experimentally intramammary infected with E. coli. The experimental group, supplemented with copper at a concentration of 20 ppm, exhibited improved outcomes compared to the control group with 6.5 ppm copper concentration. Likewise, Gakhar et al. (102) observed a decreased incidence of postpartum mastitis in cows supplemented with copper, further validating the potential benefits of copper supplementation. Copper deficiencies have been associated with impaired phagocytosis and decreased Cu, Zn-SOD (copper-zinc superoxide dismutase) activity. These findings underscore the importance of adequate copper levels for a properly functioning immune system (103). The antibacterial properties of copper are explained by the oxidation-mediated disruption of bacterial lipids, proteins, and DNA. This mechanism highlights the potential of copper as an effective antimicrobial agent (104).
Zinc is a vital trace element that plays a pivotal role in the maintenance of rumen microbiota and the synthesis of essential proteins, including collagen, glucagon, insulin, DNA, and RNA (105). It serves as an indispensable activator for a diverse array of enzymes, encompassing alkaline phosphatase, carbonic anhydrase, DNA and RNA polymerase, and, in conjunction with copper, superoxide dismutase, which assumes a key role in antioxidant processes (97). Furthermore, zinc acts as a crucial co-factor for a series of oxidoreductases and significantly contributes to keratin formation. Studies have shown that dietary supplementation of zinc can have a significant impact on the health of dairy cattle. Specifically, some research has reported that zinc supplementation results in reduced somatic cell count (106, 107) and decreased milk amyloid A levels (106). However, contrasting findings were observed by Whitaker et al. (108), where no effect of dietary zinc supplementation on SCC was found. The integrity of the intact mammary epithelium, which acts as an impermeable barrier to microorganisms, is recognized as an intrinsic component of the udder immune system. Notably, studies have demonstrated that the supplementation of zinc preparations in Holstein cows can lead to improved integrity of the mammary epithelium (109), although contradictory results have been reported by Shaffer et al. (110). Zinc is crucial for the development and proper functioning of cells involved in innate immunity, such as neutrophils. Deficiency of this essential mineral adversely affects the growth and function of T and B cells, impacting the overall immune response in dairy cattle. Zinc exhibits potent antioxidant properties and plays a pivotal role in stabilizing cellular membranes. This feature suggests its significance in preventing free-radical-induced injuries during inflammatory processes (111).While the supplementation of trace minerals has demonstrated the capacity to augment immune responses and ameliorate inflammatory alterations, it is imperative to emphasize the need for extensive and in-depth investigations aimed at elucidating the precise underlying mechanisms and exploring the potential therapeutic applications of such supplementation in the context of mitigating mastitis in dairy cattle.
3.3.2 Role of selenium of in regulation of inflammatory and immune response to prevent mastitis
Selenium has garnered considerable attention as a crucial element with antioxidant and immune-regulatory properties (36). Extensive research, encompassing in-vitro and in-vivo studies, has demonstrated that Se supplementation can alleviate inflammatory changes, oxidative stress, and mastitis caused by S. aureus in mice mammary glands (112–114). Plasma GPx-3, an extracellular antioxidant protein containing selenocysteine, plays a vital role in reducing hydrogen peroxide and lipid hydroperoxides (115). GPx-3 is essential for the antioxidant defense mechanism in dairy cattle (116). Furthermore, milk lactoserum obtained from selenium-fed cows has shown undefined antibacterial action, potentially attributed to the elevated level of GSH-Px resulting from selenium treatment (117–119). Selenium supplementation has also been found to regulate antioxidant-associated genes (SOD, GPX, TOAX, GSH, and CAT) and suppress oxidative stress in dairy cattle, thus preventing oxidative stress and subsequent infections (120). The pivotal role of selenium in regulating immunity and antioxidant activity has made it a primary focus in mastitis control research for dairy cattle. Studies have indicated a positive correlation between low levels of selenium and GPx with oxidative stress in periparturient dairy cattle. However, desired selenium supplementation in mammary epithelial cells has been found to promote anti-oxidative responses, leading to a reduction in apoptotic cells (121). Moreover, low GPx levels in blood were associated with a higher percentage of mammary infections (mastitis) in periparturient dairy cows (122). Recent research indicates that S. aureus has the ability to modulate myeloid differentiation factor 88 (MYD88) and engage the NF-kB signaling pathway, thereby initiating inflammatory responses within the mammary gland through its interaction with toll-like receptor 2 (TLR-2) (123, 124). In a recent investigation by Wei et al. (125), an elevation in MYD88, IL-1β, TNF-α, pyrin domain-containing protein 3 (NLRP), caspase-recruitment domain (ASC), and caspase-1 levels was observed in S. aureus-infected macrophages in mice. Remarkably, a 90-day regimen of selenium treatment led to a significant reduction in the expression of MYD88, IL-6, IL-1β, NLRP3, and ASC within the macrophages (125).
During instances of microbial infection and cellular damage, the NLRP3 inflammasome—a vital component of the innate immune system—maintains regulation over caspase-1 activation and the secretion of pro-inflammatory cytokines such as IL-1β/IL-18. However, S. aureus-mediated irregular control of the NLRP3 inflammasome within the mammary gland leads to an atypical inflammatory response (126, 127). Supplementing with selenium was shown to significantly curtail the expression of NLRP3 inflammasome and proinflammatory cytokines IL-1β/IL-18, thereby mitigating the abnormal inflammatory response and consequently preventing mastitis in mice (112, 127). Furthermore, Se supplementation effectively restrained the nuclear transcription factor-kappa B (NF-κB) and MAPK signaling pathways that are intricately involved in the progression of mastitis within murine macrophages (128, 129). This suggests that selenium supplementation holds potential in suppressing the inflammatory alterations elicited by S. aureus.
NF-κB and MAPK signaling pathways wield pivotal roles in initiating inflammatory transformations by bolstering cytokine production during S. aureus-induced mastitis in the mammary gland (113, 130, 131). This observation is consistently upheld by Liu et al. (113), who found that LPS supplementation led to a reduction in the recruitment of neutrophils and macrophages in mammary epithelial cells. Moreover, the overexpression of TLR2, IL-1β, TNF-α, and IL-6, coupled with the heightened phosphorylation of NF-κB and MAPKs proteins resulting from S. aureus exposure, were markedly alleviated through Se supplementation in mouse models (92, 112, 113, 132).
MerTK has been extensively documented as a key player in regulating the PI3K/AKT/mTOR pathway to enhance anti-inflammatory capabilities. Activation of the PI3K/Akt pathway within macrophages by MerTK can effectively impede NF-κB signaling (133). Furthermore, MerTK-mediated modulation of the PI3K/AKT/mTOR pathway exhibited repressive effects on TLR2-triggered immune responses, resulting in diminished inflammatory reactions and oxidative stress in U937 cells (134). S. aureus in its induction of an inflammatory response within the mammary gland of mice increased the levels of IL-1β, IL-6, and TNF-α. Notably, treatment with S. aureus led to decreased phosphorylation levels of MerTK, PI3K, AKT, and mTOR in the mouse mammary gland (114). Conversely, selenium treatment enhanced the phosphorylation levels of MerTK, PI3K, AKT, and mTOR while concurrently reducing the expression of inflammatory cytokines (IL-1β, IL-6, and TNF-α). These observations underscore selenium’s potential to augment immunity, enhance antioxidant status, and mitigate the inflammatory response within mammary glands, thereby alleviating mastitis in mice (114). In a recent in vitro experimental trail utilizing mammary alveolar cell large T antigen (MAC-T), Jing et al. (135) substantiated that selenium treatment had a marked downregulatory effect on genes (IL1B, IRAK4, MYD88, and SOCS3) linked to mastitis progression in dairy cattle. Furthermore, selenium treatment exhibited inhibitory effects on PI3K/AKT, MAPK, and NF-κB signaling pathways, while concurrently promoting the anti-inflammatory milieu through acceleration of the PI3K/Akt/mTOR pathway in dairy cattle (135). Furthermore, documented evidence indicates that Se can enhance the expression of IL10 and peroxisome-proliferator-activated receptor gamma (PPAR-γ) activity, while concurrently suppressing NF-κB and NO within the mammary gland. These effects hold true for cases of S. aureus-induced mastitis (136, 137). Additionally, selenium has been shown to alleviate the oxidative stress and inflammatory state of the mammary gland—a crucial factor in reducing the susceptibility of mice to mastitis (136; Zhang W et al., 2016). Various investigations have demonstrated positive correlations between selenium intervention and notable outcomes, including diminished SCC in milk, elevated levels of GSH-Px and reduced concentrations of MDA in plasma (138–144). Higher MDA levels are directly associated with oxidative stress, which heightens the susceptibility of periparturient dairy cattle to mastitis. Within the realm of animals, a group of twenty-five selenoproteins, twelve of which exhibit antioxidant and immunological functions, have garnered attention as promising candidates for mitigating mastitis in transition dairy cattle (135, 145, 146). The selenium content appears to be a determining factor influencing the vulnerability of mammary glands to bacterial infections during the periparturient period in dairy cattle (122). Additionally, a notable observation was made regarding selenium treatment’s efficacy in reducing SCC levels and IL-6 concentrations, while concurrently augmenting GSH-Px activity in dairy cattle (122). The potential of selenium treatment in impeding the growth of mastitis-causing bacteria, such as Streptococcus uberis (S. uberis), S. aureus, E. coli, and Streptococcus agalactiae, has been documented (147). This approach has also been associated with pronounced antibacterial effects, heightened antioxidant capacity, elevated GSH-Px activity, and lowered SCC levels in the milk of perinatal dairy cows (147). Likewise, other investigations have reported that selenium intervention, by enhancing GSH-Px activity, reduces the likelihood of mammary gland infections in dairy cattle during the transition phase (148, 149). Similarly, a distinct finding was noted, where selenium supplementation outperformed antibiotic treatment during the periparturient period in dairy cattle. To elaborate, among 36 cows, 14 still encountered infections even after antibiotic treatment, while merely 4 out of 36 cows developed mastitis in response to 4 mg of selenium supplementation during the transition phase in dairy cattle (150). Additionally, records indicate that selenium intake enhanced antioxidant capacity and concurrently regulated both innate and adaptive immunity within the mammary glands, effectively countering mastitis in dairy cattle (117). The summary of the studies discussing the association of selenium with health-enhancing phenotypic traits (antioxidant, anti-inflammation, and immunity) has been presented in Table 2. In addition, the mechanism through which selenium prevents mastitis has been highlighted in Figure 3.

Table 2 Selenium role in alleviation of mastitis by improving immunity, anti-inflammatory and antioxidant status of dairy cattle.
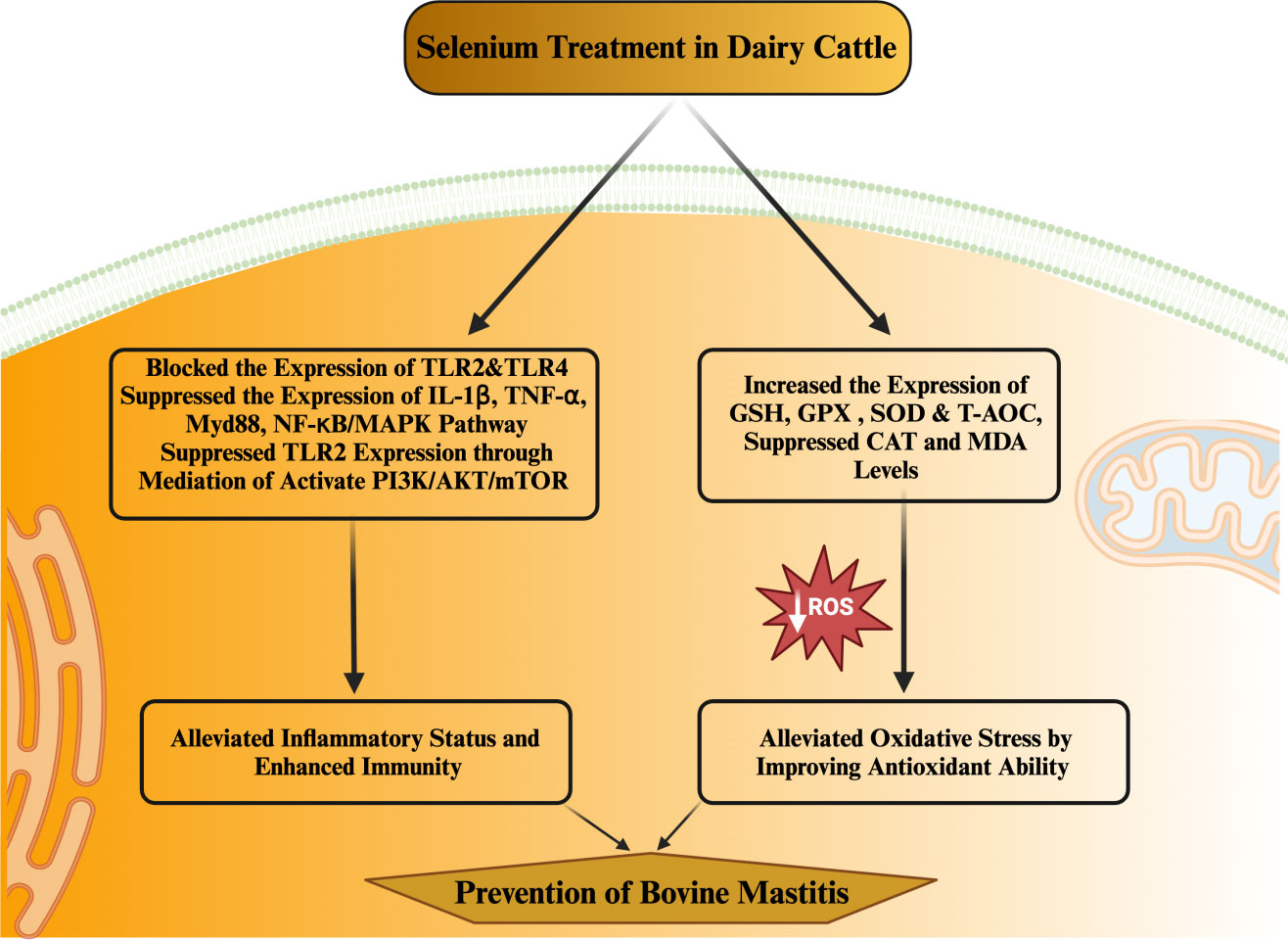
Figure 3 Mechanism through which selenium prevent mastitis in dairy cattle during periparturient period in dairy cattle.
4 Exploring the potential of mineral nanoparticles for mastitis mitigation
In animal production, minerals can be integrated into the diet or utilized in therapy through various forms, such as inorganic salts, organic forms, chelates, or nanoparticles (NPs). Nanoparticles have been studied extensively for their potential as animal growth promoters, antimicrobials, and alternatives to conventional cleaning agents (154). A significant advantage of NPs is their ability to avoid bacterial resistance by exerting toxic effects on via DNA degradation, and lipid and protein peroxidation (155, 156). Consistently, recent studies extensively described the role of different types of nanoparticles in the treatment of mastitis with positive results (157, 158). In the literature, various types of minerals nanoparticles containing copper (CuNPs), silver (AgNPs), platinum (Pt_NPs), and zinc (ZnONPs) have been described for the treatment of mastitis (100). For instance, CuNPs have shown notable inhibitory activity against various bacteria, including E. coli, Klebsiella pneumoniae, Pseudomonas aeruginosa, Propionibacterium acnes, and Salmonella typhi. Additionally, CuNPs exhibit antifungal activity against Candida species, which can cause mastitis. Moreover, AgNPs and AuNPs have demonstrated significant susceptibility against S. aureus strains isolated from clinical and subclinical mastitis cases (159). Furthermore, ZnO-NPs also exhibit antimicrobial properties against S. aureus and other pathogenic bacteria, such as E. coli and K. pneumoniae (160). AgNPs have also been considered for use in diseases caused by algae, which are associated with udder inflammation (161). According to research by Wernicki et al. (99), AgNPs and CuNPs may have a synergistic effect on various pathogens, making them potentially effective solutions in mastitis management.
5 The role of vitamins supplementation in mastitis alleviation
The significance of vitamins in their capacities as antioxidants and immune regulators has been extensively deliberated in the literature (16, 150, 162). Consequently, the inclusion of vitamin supplementation in mastitis control strategies has been well-documented (163–166).
5.1 Role of folic acid in mastitis prevention
The existing body of published evidence strongly supports the notion that folic acid supplementation yields notable improvements in metabolic function (167), while also playing a crucial role in preventing oxidative stress and enhancing immune responses (168) in periparturient dairy cattle (169). The period around calving presents a vulnerability to lowered immunity in dairy cattle due to folic acid deficiency (165). Folic acid’s pivotal contributions to immunity and anti-inflammatory processes have led to its exploration for mitigating bovine mastitis in periparturient dairy cattle (166, 170, 171). Recently a published research demonstrated that folic acid treatment significantly regulated LnRNA MSTRG.11108.1 in mastitic cows. The LncRNA MSTRG.11108.1 further regulated the genes (CXCL3, ICAM1, CXCL1, LHFPL2, LTF, ITGA9, and KIR3DL2) that were associated with immunity and inflammation (172). In addition, they also documented several immunity and immunity linked biological signaling pathways (B cell receptor signaling pathways, TNF signaling pathway, IL-17 signaling pathway and NF-κB signaling pathway) which is in consistent with findings reported previously (165, 173). By inhibiting the activation of MAPK and NF-κB, folic acid supplementation maintains an anti-inflammatory environment, consequently averting mastitis (170). Correspondingly, a study has recorded that administering folic acid (at a dosage of 120 mg per 500 kg of body weight) for 21 days led to the downregulation of several genes linked to immune function and inflammation (PIM1, SOCS3, ATP12A, KIT, LPL NFKBIA, DUSP4, ZC3H12, ESPNL, TNFAIP3) (165). These genes, found to be upregulated in S. aureus-induced mastitis during the periparturient phase in dairy cattle (174, 175) were notably downregulated.
Additionally, our prior investigation established that folic acid supplementation significantly modulated the signaling of glutathione metabolism along with its associated genes (LAP3, GSR, G6PD, GSTA4, GCLC, GPX3, PGD, IDH1, GGT1, GPX7, MGST1, and MGST2) in periparturient dairy cattle (165). Moreover, we documented that folic acid effectively enhanced dairy cattle’s antioxidant capabilities and augmented their resistance to mammary gland infections during the periparturient phase. Aligning with this, (166) recently conducted an experimental study showing that S. aureus-induced mastitis in MAC-T cells resulted in the downregulation of the noncoding RNA associated with progenitor renewal (PRANCR). Notably, in MAC-T cells treated with folic acid, this expression exhibited an increase, suggesting folic acid’s potential as a prime therapeutic agent in mastitis prevention (166). Treatment with 5 μg/mL of folic acid significantly curtailed apoptosis in Mac-T cells and offered robust defense against MRSA treatment through enhanced cytosolic DNA sensing and tightened junction signaling (173, 176). They observed the upregulation of ZBP1, IRF3, IRF7, and IFNAR2 within the cytosolic DNA-sensing pathway in folic acid-treated MAC-T cells. ZTP1, a factor associated with milk SCC (166), also assumes a critical role in activating anti-pathogenic mechanisms and inflammation (177). Furthermore, it was found that ZTP1 gene cytosolic DNA sensing pathway upregulated by folic acid treatment which has key role in activation of antipathogenic mechanism thereby enhancing the anti-inflammatory response (173). In addition, they documented that ZTP1 was significantly associated with inhibition of inflammation (177), low milk SCC and mastitis resistance (166). In light of the compiled data, we can deduce that folic acid supplementation, administered at appropriate dosages and durations, holds promise as a valuable therapeutic resource within mastitis control strategies for periparturient dairy cattle.
5.2 Role of vitamin E supplementation in periparturient bovine mastitis prevention
Vitamin E is a fat-soluble vitamin that could protect cell membrane from the action of lipid peroxidation chain reaction (178, 179). Consequently, a study found that immune cells were prone to the effects of lipid peroxidation by ROS due to the polyunsaturated fatty acids present in their cell membranes (179, 180). Furthermore, the vitamin E combats peroxyl radicals and stops the oxidation of polyunsaturated fatty acids (PUFA). In the presence of vitamin E, peroxyl radicals react with α-tocopherol rather than lipid hydroperoxide, stopping the chain reaction of peroxyl radical formation and inhibiting further oxidation of PUFAs in the membrane (181). The oxidative stress caused by aluminum was relieved by post-vitamin E injection in rats (178, 182). Furthermore, it has been demonstrated that vitamin E is important for regulating immunity and reducing oxidative stress which are the key factors for mastitis resistance/susceptibility (182–185). Additionally, vitamin E has been shown to guard against pro-oxidant-caused harm to the integrity of the bovine mammary endothelial cell barrier (186). Mokhber-Dezfouli et al. demonstrated that intramuscular vitamin E injection could lower MDA expression and lipid peroxidation and enhance plasma’s antioxidant capacity. Vitamin E supplements have been given to dairy cattle during the periparturient stage to reduce oxidative stress and maintain immunity (40). In addition, vitamin E has been extensively targeted in mastitis mitigation research in periparturient dairy cows due to its substantial role as an antioxidant and immune regulator.
Vitamin E administration has been shown to improve immunity, minimize mammary infections in dairy cattle and boost the anti-inflammatory and antioxidant functions in dairy cattle during the perinatal period (189). According to Politis et al., mastitis and oxidative stress were substantially linked to vitamin E deficiency throughout the periparturient phase (190, 191). Consequently, it has been proved experimentally that supplementation of vitamin E improved the immunity, antioxidant capacity, and anti-inflammatory ability of dairy cattle, and reduced the incidences of mastitis during the transition phase in dairy cattle (192).
Parenteral injection of 2100 mg vitamin E for 14 days before and on the day of calving significantly reduced the occurrence of periparturient mastitis in dairy cattle (87). Consistently, another study reported that supplementation of 1g vitamin E/cow/day for one month pre-calving and two months post-calving reduced the incidences of mastitis in dairy cows (193). Vitamin E has also been found effective against E. coli and S. uberis and prevented mammary gland infection during the periparturient phase in dairy cows (194, 195). Altogether, it was concluded that vitamin E is the key nutrient involved in immune regulation and relieving oxidative stress, which are the factors responsible for mastitis in periparturient dairy cattle.
5.3 Role of vitamin D supplementation in periparturient bovine mastitis prevention
Extensive research findings have demonstrated the profound impact of vitamin D (calcidiol a source of vitamin D) administration, typically within a daily dosage range of 1mg to 3mg on the regulation of various genes associated with crucial aspects of bovine immunity, inflammation, antimicrobial activity, calcium metabolism, and oxidative response (196). Notably, genes associated with immunity (CD44, ICAM1, ITGAL, ITGB1, LGALS8, SELL, NOD2, TLR2, TLR6, FOS, JUN, NFKB2), inflammation (IL1B, IL1R1, IL1RN),antimicrobial activity (CTSB, LYZ, DEFB3), calcium metabolism (TP2B1, STIM1,TRPV5, CALM3) and oxidative burst (RAC2)in periparturient dairy cattle were regulated in response to vitamin D supplementation (196). This regulatory effect is not limited to immune cells but extends to various tissues, as evidenced by the activation of 1α-hydroxylase and the subsequent regulation of calcitriol synthesis (197). Furthermore, exposure to bacterial components such as LPS and peptidoglycan has been observed to stimulate local expression of 1α-hydroxylase in peripheral blood monocytes of dairy cows. Additionally, the influence of vitamin D signaling has been elucidated in mammary gland macrophages and neutrophils when these cells are exposed to endotoxin challenge (198). In vitro experiments have also substantiated the role of vitamin D in up-regulating the mRNA activity of β-defensins and antimicrobial peptides in monocytes and milk somatic cell counts (199, 200). Importantly, studies have shown that vitamin D supplementation plays a pivotal role in reducing the incidence of mastitis and metritis in periparturient dairy cattle by mitigating oxidative stress and enhancing the immune response (201). Furthermore, vitamin D treatment has been associated with increased cell viability and the inhibition of S. aureus adhesion and invasion in bovine mammary epithelial cells, underscoring its potential significance in mastitis prevention (202). Consistently, a recent published article has demonstrated that vitamin D and its metabolites hydroxyvitamin D [25(OH)D] with a concentration of 20-400 ng/ml has shown a positive role on bovine immune cells, antioxidant response and could be consider as therapeutic agent for mastitis prevention (203–205).In light of the available literature, it is evident that vitamin D holds a central position in bolstering dairy cattle immunity and potentially alleviating bovine mastitis. However, it is worth noting that further comprehensive investigations are warranted to gain a deeper understanding of its precise mechanisms and potential therapeutic applications in this context. For ease of review, the recent findings associated with role of vitamins supplementation in boosting immune, antioxidant and anti-inflammatory response in dairy cattle during periparturient period has been summarized in Table 3. Furthermore, Figure 4 illustrates the impact of vitamin supplementation on immune, antioxidant, and anti-inflammatory responses in dairy cattle.
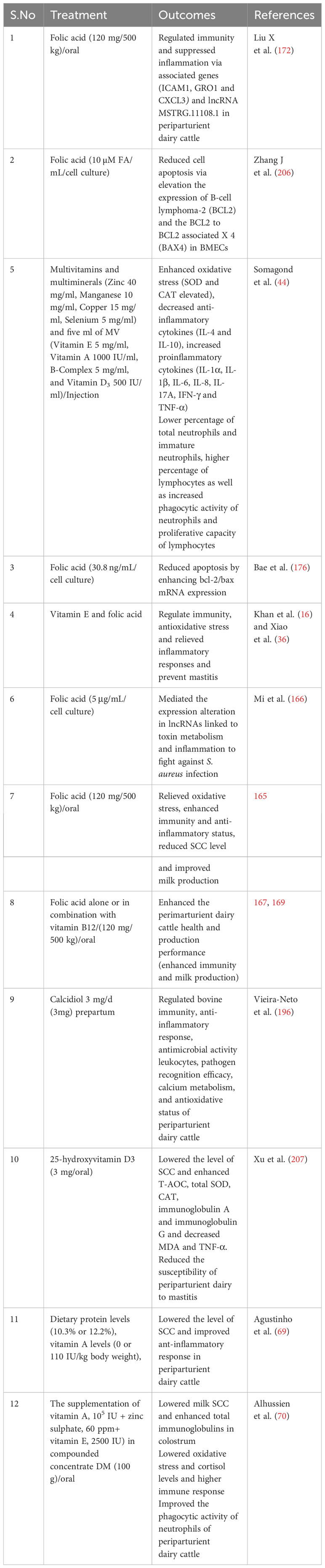
Table 3 Role of vitamins in boosting immunity, anti-inflammation and antioxidant response in periparturient dairy cattle.
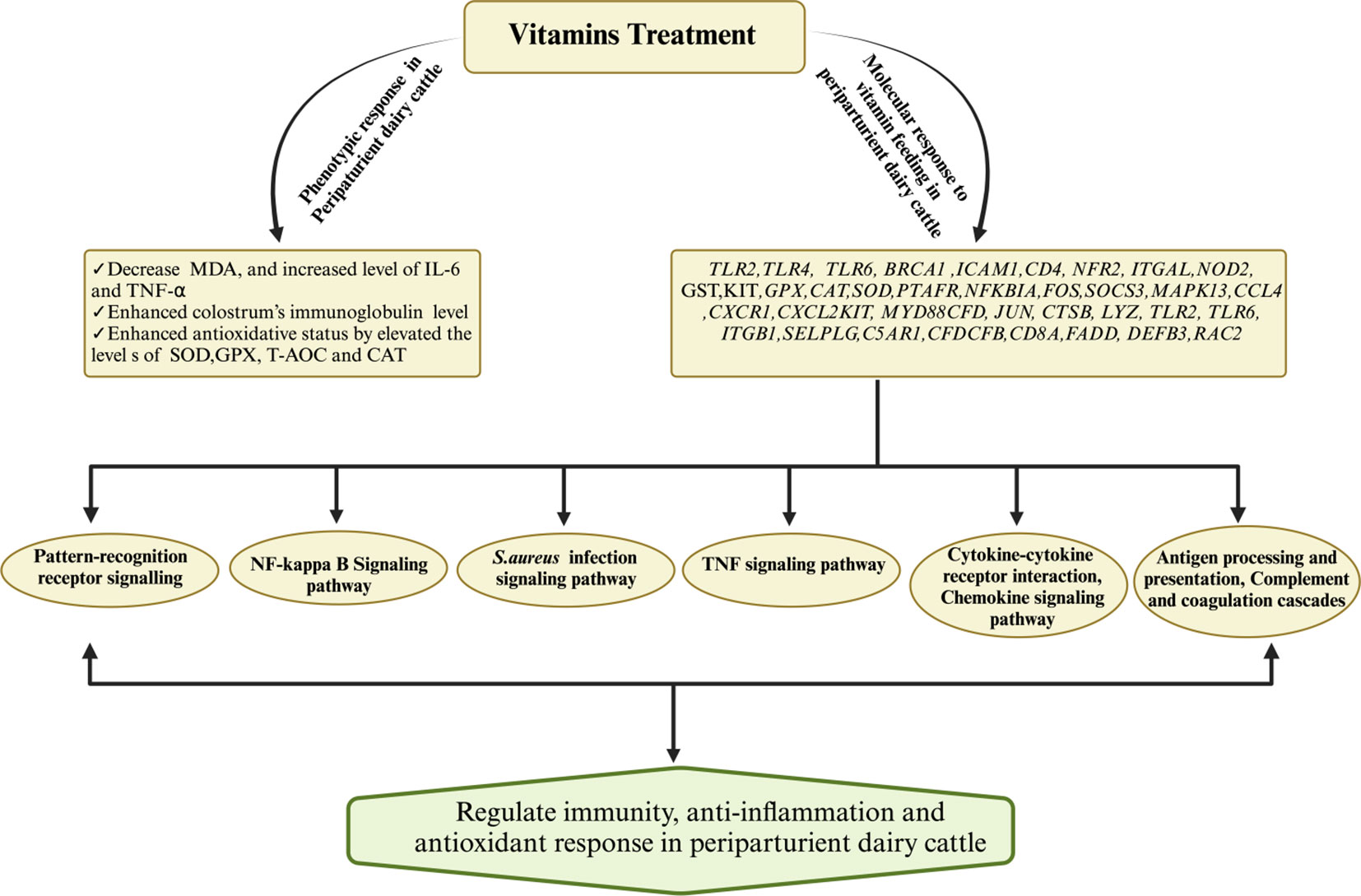
Figure 4 Mechanisms governing the regulation of immunity, antioxidant, and anti-inflammatory responses for the prevention of mastitis in periparturient dairy cattle through vitamin supplementation. Vitamin supplementation demonstrated an augmented antioxidative status by upregulating key antioxidant genes (SOD, CAT) while concurrently downregulating the levels of MDA. Additionally, vitamins exhibited regulatory effects on immunity-related and inflammatory-associated genes and their associated signaling pathways, thereby substantiating their pivotal role in mastitis resistance or susceptibility.
5 The effect of rumen-protected amino acids on immune function, oxidative and anti-inflammatory status of dairy cattle
The cellular detoxification process is facilitated by GSH through the action of GST and the neutralization of hydrogen peroxide by GSH-Px. The GSTs (EC 2.5.1.18) are pivotal antioxidant enzymes responsible for the regulation of cellular redox equilibrium, as documented by several studies (208–210). Recent evidence underscores the involvement of methionine in glutathione synthesis (211), thereby potentially enhancing the antioxidant capacity in animals and their products. This notion is corroborated by research demonstrating that methionine, in conjunction with choline, elevates glutathione and amino acid levels in perinatal dairy cows (212). Furthermore, methionine supplementation has been found to augment very-low-density lipoprotein (VLDL), facilitating the circulation of vitamin E (213). Consequently, the detrimental impact of lipid peroxidation by-products, such as MDA, can be mitigated through the administration of rumen-protected amino acids (213). Additionally, the control of ROS by antioxidant systems, categorized into enzymatic and non-enzymatic components like metabolites, has been extensively discussed in previous studies (210, 214).
Dysregulated immune function, notably in early lactation dairy cows, has been observed, adversely affecting neutrophils, circulating monocytes, and lymphocytes (6, 215 55, 216). It is postulated that complete metabolic adaptations are required to cope with the substantial nutrient demands associated with lactation initiation, contributing to immunological dysfunction (19, 217, 218). A noteworthy finding is that prolactin blockade leads to an increase in oxidative burst activity in neutrophils, resulting in an initial reduction in milk production and nutritional requirements, along with a subsequent decline in lymphocyte proliferation (219).
Empirical investigations have consistently demonstrated that methionine supplementation exerts favorable effects on the anti-inflammatory and antioxidant status in periparturient dairy cattle (220–222) and neonatal calves (223). A recent study conducted by Hu et al. has reported that the supply of methionine and arginine significantly modulates milk protein synthesis, thereby alleviating potential inflammatory and pro-oxidant conditions in transition dairy cattle (224). Moreover, emerging research has highlighted the significance of methionine supplementation in conjunction with arginine in conferring anti-inflammatory effects and enhancing the antioxidant status in transition dairy cattle. Notably, Dai et al. (225) and Batistel et al. (222) have reported the beneficial impact of methionine and arginine co-supplementation. Additionally, Abdelmegeid et al. (226) documented that the combination of choline and methionine effectively regulates antioxidative mechanisms, resulting in heightened anti-inflammatory and cytoprotective responses against oxidative stress in neonatal Holstein calves. Consistently, Zhou et al. (227) demonstrated that choline and methionine supplementation improved immunometabolic status, bolstered blood polymorphonuclear leukocyte phagocytosis capacity, promoted anti-inflammatory responses upon pathogenic challenges, and elevated antioxidative capacities in peripartal cows. In a relevant experiment, a group of cows was subjected to either methionine supplementation alone or in combination with lysine. Wang H et al. (223) found that the offspring (calves) of cows receiving rumen-protected amino acids exhibited heightened passive immunity, characterized by increased immunoglobulin G concentrations and superior growth rates compared to their counterparts in the unsupplemented amino acid group. Furthermore, they documented that methionine supplementation upregulated the expression of antioxidant-related genes, such as SOD and GSH-Px and conferred cytoprotective effects against hyperthermia (228, 229). The synergistic effects of methionine and arginine supplementation have also been observed in the regulation of immunity and the mitigation of oxidative stress induced by bacterial LPS in BMECs (225). Dai et al. (225) reported that bacterial LPS significantly down-regulated the expression of key genes associated with antioxidant responses, such as NFE2L2, NQO1, GPX1, ATG7, and GPX3, while increasing the levels of SOD2 and NOS2 in BMECs. Folic acid was found to enhance antioxidant activity, reduce the expression of inflammation-related genes, and improve udder health in dairy cattle (225). Additionally, when BMECs were challenged with gamma-d-glutamyl-meso-diaminopimelic acid (iE-DAP), a component of bacterial cell walls, it induced inflammatory changes and oxidative stress, which were effectively mitigated by arginine and methionine treatment (230). Similarly, another study demonstrated that glutamine treatment provided protective effects against the adverse effects of iE-DAP in BMECs (231). Furthermore, iE-DAP was found to elevate the expression of inflammatory markers, including NOD1, inhibitor of nuclear factor-κB (NFKBIA, IκB), nuclear factor-κB subunit p65 (RELA, NF-κB p65), IL-6, and interleukin-8 (IL-8) in cell culture (231). However, when cells treated with iE-DAP were subsequently exposed to glutamine, this intervention led to the suppression of the NOD1/NF-κB pathway and the enhancement of antioxidant protein levels (231). This aligns with previous findings that amino acids play a pivotal role in NO regulation in mammary cells, thereby exerting antibacterial activity against LPS during inflammation (232–234). In vitro experiments have consistently demonstrated that methionine supplementation effectively attenuates apoptosis, necrosis, and lipid peroxidation in bovine mammary gland cells (228, 229, 235). Moreover, these studies have shown that arginine and methionine co-supplementation enhances the expression of antioxidative genes and elevates the NFE2L2 signaling pathway in mammary cells, a crucial component of the cellular antioxidant defense system (236). Lan et al. (237) conducted experimental research revealing that pretreatment with 2 mM Met-Met had the capacity to mitigate the elevated levels of specific inflammatory markers following exposure to 1 μg/mL LPS. This included a reduction in TNF-α, IL-1β, and IL-8. Furthermore, their investigation indicated that the genes commonly affected by this treatment were primarily associated with the NF-κB, MAPK, and IL-17 pathways. Notably, the suppression of NF-κB, P38, and JNK by Met-Met appeared to occur through the Janus kinase 2-signal transducers and activators of transcription 5 (JAK2-STAT5) pathway (237). Additionally, it was observed that the Met-Met-induced reduction in LPS-triggered activation of p-IκB, NF-κB, and JNK was reversed in the presence of a JAK2 inhibitor, highlighting the intricate interplay between Met-Met and these signaling pathways (237). Furthermore, a concise summary of research endeavors investigating the impact of rumen-protected amino acids on immune function, oxidative stress, and anti-inflammatory responses in dairy cattle has been thoughtfully compiled in Table 4 for your reference and elucidation.

Table 4 Summary of studies investigating the effect of rumen-protected amino acids supplementation on immune function, oxidative and anti-inflammatory status in dairy cattle.
6 Future research direction
While this review has synthesized existing knowledge regarding the role of amino acids, vitamins, and trace minerals in mitigating periparturient mastitis, several avenues for future research emerge. Further investigation into the intricate mechanisms underlying the interactions between these nutrients, oxidative stress, and immune modulation will deepen our understanding of their synergistic effects. Incorporating molecular and cellular approaches can unravel the specific pathways through which these nutrients influence immune responses and oxidative balance. Additionally, longitudinal studies assessing the long-term effects of targeted supplementation on mastitis incidence, milk quality, and overall cow health are essential to validate the efficacy of these strategies under practical farming conditions. Furthermore, understanding the interplay between genetic factors, environmental conditions, and nutritional interventions can provide insights into personalized approaches for mastitis prevention. Moreover, exploring novel delivery mechanisms for these nutrients, such as innovative formulations or precision feeding, could optimize their absorption and utilization within the complex physiological milieu of periparturient dairy cattle. In conclusion, future research endeavors should focus on unraveling the nuances of nutrient-nutrient interactions, delineating precise molecular mechanisms, and translating these findings into practical strategies that empower dairy farmers to effectively manage periparturient mastitis, bolstering animal health and farm productivity.
7 Conclusion
In light of the intricate interplay between immune suppression, oxidative stress, and metabolic perturbations during the periparturient period, this comprehensive review underscores the paramount importance of proactive nutritional strategies in mitigating bovine mastitis. The critical vulnerabilities arising from negative energy balance, oxidative stress, and compromised immune responses underscore the need for targeted interventions to enhance udder health and overall productivity in dairy cattle. The exploration of trace minerals, vitamins, and amino acids as key mitigation factors offers promising avenues for addressing periparturient bovine mastitis. These nutritional components have demonstrated significant potential in bolstering antioxidant defenses, modulating immune responses, and preventing oxidative damage. The multifaceted roles of trace minerals, particularly copper, selenium, and calcium, have been deliberated in the context of mastitis control. Similarly, the established impact of vitamins, such as vitamin B12 and vitamin E, in enhancing metabolic function and immune responses underscores their potential in ameliorating mastitis susceptibility. Furthermore, amino acids’ pivotal role in maintaining cellular oxidative balance through their participation in crucial biosynthesis pathways presents a novel perspective in combatting mastitis-related challenges. The insights gained from this review highlight the need for a holistic approach that encompasses these nutritional factors to enhance udder health and mitigate the risks associated with periparturient mastitis.
Author contributions
MK: Conceptualization, Data curation, Formal analysis, Methodology, Supervision, Validation, Visualization, Writing – original draft, Writing – review & editing. BH: Conceptualization, Data curation, Writing – original draft, Writing – review & editing. XK: Data curation, Writing – review & editing. YC: Data curation, Writing – review & editing. HL: Data curation, Writing – review & editing. QU: Data curation, Writing – review & editing. IK: Data curation, Writing – review & editing. AK: Data curation, Writing – review & editing. WC: Data curation, Writing – review & editing. CW: Conceptualization, Data curation, Funding acquisition, Project administration, Resources, Supervision, Validation, Visualization, Writing – original draft, Writing – review & editing.
Funding
The author(s) declare financial support was received for the research, authorship, and/or publication of this article. This study was funded by the Shandong Province Modern Agricultural Technology System Donkey Industrial Innovation Team (grant number SDAIT-27), Livestock and Poultry Breeding Industry Project of the Ministry of Agriculture and Rural Affairs (grant number 19211162), Shandong Rural Revitalization Science and Technology Innovation Action Plan (Key Technology Innovation and Demonstration of Integrated Development of Dong-E Black Donkey Industry (grant number 2021TZXD012), Open Project of Liaocheng University Animal Husbandry Discipline (grant number 319312101–14), Open Project of Shandong Collaborative Innovation Center for Donkey Industry Technology (grant number 3193308), Research on Donkey Pregnancy Improvement (grant number K20LC0901), and Liaocheng University Scientific Research Fund (grant number 318052025).
Acknowledgments
We express our sincere gratitude to the Liaocheng Research Institute of Donkey High-efficiency Breeding and Ecological Feeding, Liaocheng University for providing us financial support.
Conflict of interest
The authors declare that the research was conducted in the absence of any commercial or financial relationships that could be construed as a potential conflict of interest.
Publisher’s note
All claims expressed in this article are solely those of the authors and do not necessarily represent those of their affiliated organizations, or those of the publisher, the editors and the reviewers. Any product that may be evaluated in this article, or claim that may be made by its manufacturer, is not guaranteed or endorsed by the publisher.
References
1. Khan MZ, Wang J, Ma Y, Chen T, Ma M, Ullah Q, et al. Genetic polymorphisms in immune-and inflammation-associated genes and their association with bovine mastitis resistance/susceptibility. Front Immunol (2023a) 14:1082144. doi: 10.3389/fimmu.2023.1082144
2. Khan MZ, Dari G, Khan A, Yu Y. Genetic polymorphisms of TRAPPC9 and CD4 genes and their association with milk production and mastitis resistance phenotypic traits in Chinese Holstein. Front Veterinary Science (2022b) 9:1008497. doi: 10.3389/fvets.2022.1008497
3. Sordillo LM, Aitken SL. Impact of oxidative stress on the health and immune function of dairy cattle. Vet Immunol Immunopathol (2009) 128:104–9. doi: 10.1016/j.vetimm.2008.10.305
4. Abdel-Hamied E, Mahmoud MM. Antioxidants profile, oxidative stress status, leukogram and selected biochemical indicators in dairy cows affected with mastitis. J Anim Health Prod (2020) 8(4):183–8. doi: 10.17582/journal.jahp/2020/8.4.183.188
5. Ayemele AG, Tilahun M, Lingling S, Elsaadawy SA, Guo Z, Zhao G, et al. Oxidative stress in dairy cows: insights into the mechanistic mode of actions and mitigating strategies. Antioxidants (2021) 10:1918. doi: 10.3390/antiox10121918
6. Sordillo LM. Nutritional strategies to optimize dairy cattle immunity. J dairy science (2016) 99(6):4967–82. doi: 10.3168/jds.2015-10354
7. Putman AK, Brown JL, Gandy JC, Wisnieski L, Sordillo LM. Changes in biomarkers of nutrient metabolism, inflammation, and oxidative stress in dairy cows during the transition into the early dry period. J dairy science (2018) 101(10):9350–9. doi: 10.3168/jds.2018-14591
8. Bernabucci U, Ronchi B, Lacetera N, Nardone A. Influence of body condition score on relationship between metabolic status and oxidative stress in periparturient dairy cows. J Dairy Sci (2005) 88:2017–26. doi: 10.3168/jds.S0022-0302(05)72878-2
9. Ster C, Loiselle MC, Lacasse P. Effect of postcalving serum nonesterified fatty acids concentration on the functionality of bovine immune cells. J Dairy Sci (2012) 95:708–17. doi: 10.3168/jds.2011-4695
10. Contreras GA, Strieder-Barboza C, Raphael W. Adipose tissue lipolysis and remodeling during the transition period of dairy cows. J Anim Sci Biotechnol (2017) 8:41. doi: 10.1186/s40104-017-0174-4
11. Ospina PA, McArt J, Overton T, Stokol T, Nydam D. Using nonesterified fatty acids and β-hydroxybutyrate concentrations during the transition period for herd-level monitoring of increased risk of disease and decreased reproductive and milking performance. Vet Clin N Am Food Anim Pract (2013) 29:387–412. doi: 10.1016/j.cvfa.2013.04.003
12. Li C, Huang J, Chen X, Yan Y, Li L, Zhao W. Transcriptome analysis reveals that NEFA and β-hydroxybutyrate induce oxidative stress and inflammatory response in bovine mammary epithelial cells. Metabolites (2022) 12(11):1060. doi: 10.3390/metabo12111060
13. Lacetera N, Franci O, Scalia D, Bernabucci U, Ronchi B, Nardone A. Effects of nonesterified fatty acids and beta-hydroxybutyrate on functions of mononuclear cells obtained from ewes. Am J Vet Res (2002) 63:414–8. doi: 10.2460/ajvr.2002.63.414
14. Bai H, Talukder MS, Hiroki K, Tsukino I, Manabu K, Masashi T. Evaluation of the immune status of peripheral blood monocytes from dairy cows during the periparturition period. J Reprod Dev (2019) 65:313–8. doi: 10.1262/jrd.2018-150
15. Hammon DS, Evjen IM, Dhiman TR, Goff JP, Walters JL. Neutrophil function and energy status in Holstein cows with uterine health disorders. Vet Immunol Immunopathol (2006) 113:21–9. doi: 10.1016/j.vetimm.2006.03.022
16. Khan MZ, Ma Y, Xiao J, Chen T, Ma J, Liu S, et al. Role of selenium and vitamins E and B9 in the alleviation of bovine mastitis during the periparturient period. Antioxidants (2022a) 11(4):657. doi: 10.3390/antiox11040657
17. Bouvier-Muller J, Allain C, Enjalbert F, Tabouret G, Portes D, Caubet C, et al. Response to dietary-induced energy restriction in dairy sheep divergently selected for resistance or susceptibility to mastitis. J Dairy Science (2016) 99(1):480–92. doi: 10.3168/jds.2015-9785
18. Wilde D. Influence of macro and micro minerals in the peri-parturient period on fertility in dairy cattle. Anim Reprod Sci (2006) 96(3-4):240–9. doi: 10.1016/j.anireprosci.2006.08.004
19. Aleri JW, Hine BC, Pyman MF, Mansell PD, Wales WJ, Mallard B, et al. Periparturient immunosuppression and strategies to improve dairy cow health during the periparturient period. Res Veterinary Science (2016) 108:8–17. doi: 10.1016/j.rvsc.2016.07.007
20. Fredebeul-Krein F, Schmenger A, Wente N, Zhang Y, Krömker V. Factors associated with the severity of clinical mastitis. Pathogens (2022) 11(10):1089. doi: 10.3390/pathogens11101089
21. Sordillo LM. Factors affecting mammary gland immunity and mastitis susceptibility. Livestock Production Science (2005) 98:89–99. doi: 10.1016/j.livprodsci.2005.10.017
22. Mavangira V, Sordillo LM. Role of lipid mediators in the regulation of oxidative stress and inflammatory responses in dairy cattle. Res Veterinary Science (2018) 116:4–14. doi: 10.1016/j.rvsc.2017.08.002
23. Castillo C, Hernández J, Valverde I, Pereira V, Sotillo J, Alonso ML, et al. Plasma malonaldehyde (MDA) and total antioxidant status (TAS) during lactation in dairy cows. Res Veterinary Science (2006) 80:133–9. doi: 10.1016/j.rvsc.2005.06.003
24. Mikulková K, Kadek R, Filípek J. Evaluation of oxidant/antioxidant status, metabolic profile, and milk production in cows with metritis. Irish Veterinary J (2020) 73:8. doi: 10.1186/s13620-020-00161-3
25. Wullepit N, Raes K, Beerda B, Veerkamp RF, Fremaut D, Smet S. Influence of management and genetic merit for milk yield on the oxidative status of plasma in heifers. Livestock Science (2009) 123:276–82. doi: 10.1016/j.livsci.2008.11.013
26. Sharma N, Singh N, Singh O, Pandey V, Verma P. Oxidative stress and antioxidant status during transition period in dairy cows. Asian-Australasian J Anim Sci (2011) 24:479–84. doi: 10.5713/ajas.2011.10220
27. Castillo C, Hernandez J, Bravo A, Lopez-Alonso M, Pereira V, Benedito JL. Oxidative status during late pregnancy and early lactation in dairy cows. Veterinary J (2005) 169:286–92. doi: 10.1016/j.tvjl.2004.02.001
28. Markiewicz H, Gehrke M, Malinowski E, Kaczmarowski M. Evaluating the antioxidant potential in the blood of transition cows. Medycyna Weterynaryjna (2005) 61:1382–4.
29. Kowalska J, Jankowiak D. Changes of reduction-oxidation balance in pregnant ruminants. Postępy Biochemii (2009) 55(3):323–8.
30. Mavangira V, Mangual MJ, Gandy JC, Sordillo LM. 15-F2t-isoprostane concentrations and oxidant status in lactating dairy cattle with acute coliform mastitis. J Veterinary Internal Med (2016) 30:339–47. doi: 10.1111/jvim.13793
31. Agrawal A, Khan MJ, Graugnard DE, Vailati-Riboni M, Rodriguez-Zas SL, Osorio JS, et al. Prepartal energy intake alters blood polymorphonuclear leukocyte transcriptome during the peripartal period in Holstein cows. Bioinf Biol Insights (2017) 11:1177932217704667. doi: 10.1177/1177932217704667
32. Mann S, Sipka A, Leal Yepes FA, Nydam DV, Overton TR, Wakshlag JJ. Nutrient-sensing kinase signaling in bovine immune cells is altered during the postpartum nutrient deficit: a possible role in transition cow inflammatory response. J Dairy Science (2018) 101:9360–70. doi: 10.3168/jds.2018-14549
33. Mann S, Sipka AS, Grenier JK. The degree of postpartum metabolic challenge in dairy cows is associated with peripheral blood mononuclear cell transcriptome changes of the innate immune system. Dev Comp Immunol (2019) 93:28–36. doi: 10.1016/j.dci.2018.11.021
34. Yin FG, Zhang ZZ, Ju H, Yin YL. Digestion rate of dietary starch affects systemic circulation of amino acids in weaned pigs. Br J Nutr (2010) 103(10):1404. doi: 10.1017/S0007114509993321
35. Zhou XH, He LQ, Wan D, Yang HS, Yao K, Wu GY, et al. Methionine restriction on lipid metabolism and its possible mechanisms. Amino Acids (2016) 48(7):1533–40. doi: 10.1007/s00726-016-2247-7
36. Xiao J, Khan MZ, Ma Y, Alugongo GM, Ma J, Chen T, et al. The antioxidant properties of selenium and vitamin E; their role in periparturient dairy cattle health regulation. Antioxidants (2021) 10(10):1555. doi: 10.3390/antiox10101555
37. Khan MZ, Liu S, Ma Y, Ma M, Ullah Q, Khan IM, et al. Overview of the effect of rumen-protected limiting amino acids (methionine and lysine) and choline on the immunity, antioxidative, and inflammatory status of periparturient ruminants. Front Immunol (2023b) 13:1042895. doi: 10.3389/fimmu.2022.1042895
38. Alhussien MN, Dang AK, Bu D. Strategies for mitigating the transition period stress in dairy cattle. Front Veterinary Science (2023) 10:1157526. doi: 10.3389/fvets.2023.1157526
39. Du XE, Cui Z, Zhang R, Zhao K, Wang L, Yao J, et al. The effects of rumen-protected choline and rumen-protected nicotinamide on liver transcriptomics in periparturient dairy cows. Metabolites (2023) 13(5):594. doi: 10.3390/metabo13050594
40. Spears JW, Weiss WP. Role of antioxidants and trace elements in health and immunity of transition dairy cows. Veterinary J (2008) 176:70–6. doi: 10.1016/j.tvjl.2007.12.015
41. O’Rourke D. Nutrition and udder health in dairy cows: a review. Irish Veterinary J (2009) 62:15–20. doi: 10.1186/2046-0481-62-S4-S15
42. Weiss WP. A 100-year review: from ascorbic acid to zinc—Mineral and vitamin nutrition of dairy cows. J Dairy Science (2017) 100:10045–60. doi: 10.3168/jds.2017-12935
43. Zigo F, Vasil’ M, Ondrašovičová S, Výrostková J, Bujok J, Pecka-Kielb E. Maintaining optimal mammary gland health and prevention of mastitis. Front Veterinary Science (2021) 8:607311. doi: 10.3389/fvets.2021.607311
44. Somagond YM, Alhussien MN, Dang AK. Repeated injection of multivitamins and multiminerals during the transition period enhances immune response by suppressing inflammation and oxidative stress in cows and their calves. Front Immunol (2023) 14:1059956. doi: 10.3389/fimmu.2023.1059956
45. Wang Z, Wang Q, Tang C, Yuan J, Luo C, Li D, et al. Medium chain fatty acid supplementation improves animal metabolic and immune status during the transition period: A study on dairy cattle. Front Immunol (2023) 14:1018867. doi: 10.3389/fimmu.2023.1018867
46. Ingvartsen KL, Moyes K. Nutrition, immune function and health of dairy cattle. Animal (2013) 7(s1):112–22. doi: 10.1017/S175173111200170X
47. Coleman DN, Lopreiato V, Alharthi A, Loor JJ. Amino acids and the regulation of oxidative stress and immune function in dairy cattle. J Anim Science (2020) 98(1):S175–93. doi: 10.1093/jas/skaa138
48. Mezzetti M, Bionaz M, Trevisi E. Interaction between inflammation and metabolism in periparturient dairy cows. J Anim Science (2020) 98(1):S155–74. doi: 10.1093/jas/skaa134
49. Ospina PA, Nydam DV, Stokol T, Overton TR. Association between the proportion of sampled transition cows with increased nonesterified fatty acids and β-hydroxybutyrate and disease incidence, pregnancy rate, and milk production at the herd level. J Dairy Science (2010) 93(8):3595–601. doi: 10.3168/jds.2010-3074
50. Yan Y, Huang J, Huan C, Li L, Li C. Non-esterified fatty acid induces ER stress-mediated apoptosis via ROS/MAPK signaling pathway in bovine mammary epithelial cells. Metabolites (2022) 12(9):803. doi: 10.3390/metabo12090803
51. Poławska E, Bagnicka AW, Niemczuk K, Lipińska JO. Relations between the oxidative status, mastitis, milk quality and disorders of reproductive functions in dairy cows—A review. Anim Sci Papers Rep (2012) 30(4):297–307.
52. Turk R, Juretić D, Gereš D, Svetina A, Turk N, Flegar-Meštrić Z. Influence of oxidative stress and metabolic adaptation on PON1 activity and MDA level in transition dairy cows. Anim Reprod Science (2008) 108:98–106. doi: 10.1016/j.anireprosci.2007.07.012
53. Sordillo LM, Mavangira V. The nexus between nutrient metabolism, oxidative stress and inflammation in transition cows. Anim. Prod Sci (2014) 54:1204–14. doi: 10.1071/AN14503
54. Sordillo LM, Contreras GA, Aitken SL. Metabolic factors affecting the inflammatory response of periparturient dairy cows. Anim Health Res Rev (2009) 10(1):53–63.
55. Sordillo LM, Raphael W. Significance of metabolic stress, lipid mobilization, and inflammation on transition cow disorders. Vet Clin North Am Food Anim Pract (2013) 29:267–78. doi: 10.1016/j.cvfa.2013.03.002
56. Ma N, Wei G, Zhang H, Dai H, Roy AC, Shi X, et al. Cis-9, trans-11 CLA alleviates lipopolysaccharide-induced depression of fatty acid synthesis by inhibiting oxidative stress and autophagy in bovine mammary epithelial cells. Antioxidants (2021) 11(1):55. doi: 10.3390/antiox11010055
57. Liu L, Wang B, Yang W, Jiang Q, Loor JJ, Ouyang L, et al. Sirtuin 3 relieves inflammatory responses elicited by lipopolysaccharide via the PGC1α-NFκB pathway in bovine mammary epithelial cells. J Dairy Science (2023) 106(2):1315–29. doi: 10.3168/jds.2022-22114
58. Sun X, Jia H, Xu Q, Zhao C, Xu C. Lycopene alleviates H2O2-induced oxidative stress, inflammation and apoptosis in bovine mammary epithelial cells via the NFE2L2 signaling pathway. Food Funct (2019) 10(10):6276–85. doi: 10.1039/C9FO01922G
59. Chang R, Sun X, Jia H, Xu Q, Dong Z, Tang Y, et al. Inhibiting nuclear factor erythroid 2 related factor 2-mediated autophagy in bovine mammary epithelial cells induces oxidative stress in response to exogenous fatty acids. J Anim Sci Biotechnol (2022) 13(1):1–1. doi: 10.1186/s40104-022-00695-2
60. Laliotis GP, Koutsouli P, Sotirakoglou K, Savoini G, Politis I. Association of oxidative stress biomarkers and clinical mastitis incidence in dairy cows during the periparturient period. J Veterinary Res (2020) 64(3):421. doi: 10.2478/jvetres-2020-0053
61. Lyu C, Yuan B, Meng Y, Cong S, Che H, Ji X, et al. Puerarin alleviates H2O2-induced oxidative stress and blood–milk barrier impairment in dairy cows. Int J Mol Sci (2023) 24(9):7742. doi: 10.3390/ijms24097742
62. Mahapatra A, Panigrahi S, Patra RC, Rout M, Ganguly S. A study on bovine mastitis related oxidative stress along with therapeutic regimen. Int J Curr Microbiol Appl Sci (2018) 7:247–56. doi: 10.20546/ijcmas.2018.701.027
63. Tabatabaee N, Heidarpour M, Khoramian B. Milk metabolites, proteins and oxidative stress markers in dairy cows suffering from Staphylococcus aureus subclinical mastitis with or without spontaneous cure. J Dairy Res (2021) 88(3):326–9. doi: 10.1017/S0022029921000613
64. Cozzi G. Short communication: reference values for blood parameters in holstein dairy cows: effects of parity, stage of lactation, and season of production. J Dairy Sci (2011) 94:7. doi: 10.3168/jds.2010-3687
65. Zelal A. Hypomagnesemia tetany in cattle. J Adv Dairy Res (2017) 5:10. doi: 10.4172/2329-888X.1000178
67. Laven R, Lawrence K, Livesey C. The assessment of blood copper status in cattle: A comparison of measurements of caeruloplasmin and elemental copper in serum and plasma. N Z Vet J (2007) 55:171–6. doi: 10.1080/00480169.2007.36763
68. Polders M, Holtershinken M, Meyer U, Rehage J, Flachowsky G. Assessment of reference values for copper and zinc in blood serum of first and second lactating dairy cows. Vet Med Int (2010) 2010:8.
69. Agustinho BC, Ramsey KC, Moore C, Tsai CY, Scholte CM, McGuire MA, et al. Effect of different combinations of dietary vitamin A, protein levels, and monensin on inflammatory markers and metabolites, retinol-binding protein, and retinoid status in periparturient dairy cows. Animals (2021) 11(9):2605. doi: 10.3390/ani11092605
70. Alhussien MN, Tiwari S, Panda BS, Pandey Y, Lathwal SS, Dang AK. Supplementation of antioxidant micronutrients reduces stress and improves immune function/response in periparturient dairy cows and their calves. J Trace Elements Med Biol (2021) 65:126718. doi: 10.1016/j.jtemb.2021.126718
71. Libera K, Konieczny K, Witkowska K, Żurek K, Szumacher-Strabel M, Cieslak A, et al. The association between selected dietary minerals and mastitis in dairy cows—A review. Animals (2021) 11(8):2330.
72. Khatti A, Mehrotra S, Patel PK, Singh G, Maurya VP, Mahla AS, et al. Supplementation of vitamin E, selenium, and increased energy allowance mitigates the transition stress and improves postpartum reproductive performance in the crossbred cow. Theriogenology (2017) 104:142–8. doi: 10.1016/j.theriogenology.2017.08.014
73. Bordignon R, Volpato A, Glombowsky P, Souza CF, Baldissera MD, Secco R, et al. Nutraceutical effect of vitamins and minerals on performance and immune and antioxidant systems in dairy calves during the nutritional transition period in summer. J Therm Biol (2019) 84:451–9. doi: 10.1016/j.jtherbio.2019.07.034
74. Chen YH, Chen YM, Tu PA, Lee KH, Chen JY, Hsu JT. Effect of supplementing vitamin E, selenium, copper, zinc, and manganese during the transition period on dairy cow reproductive performance and immune function. Vet Sci (2023) 10(3):225. doi: 10.3390/vetsci10030225
75. Macmillan K, Gobikrushanth M, Behrouzi A, López-Helguera I, Cook N, Hoff B, et al. The association of circulating prepartum metabolites, minerals, cytokines, and hormones with postpartum health status in dairy cattle. Res Vet Sci (2020) 130:126–32. doi: 10.1016/j.rvsc.2020.03.011
76. Mion B, Ogilvie L, Van Winters B, Spricigo JF, Anan S, Duplessis M, et al. Effects of replacing inorganic salts of trace minerals with organic trace minerals in the pre-and postpartum diets on mineral status, antioxidant biomarkers, and health of dairy cows. J Anim Sci (2023) 101:skad041. doi: 10.1093/jas/skad041
77. Osorio JS, Trevisi E, Li C, Drackley JK, Socha MT, Loor JJ. Supplementing Zn, Mn, and Cu from amino acid complexes and Co from cobalt glucoheptonate during the peripartal period benefits postpartal cow performance and blood neutrophil function. J Dairy Sci (2016) 99(3):1868–83. doi: 10.3168/jds.2015-10040
78. Ogilvie L, Spricigo JF, Mion B, Van Winters B, Karrow NA, McBride BW, et al. Neutrophil function and antibody production during the transition period: Effect of the form of supplementary trace minerals and associations with postpartum clinical disease and blood metabolites. J Dairy Sci (2022) 105(12):9944–60. doi: 10.3168/jds.2022-21909
79. Gomes da Silva GG, da Silva Dias MS, Takiya CS, Nunes AT, Del Valle TA, Grigoletto NT, et al. Feeding reduced levels of trace minerals in proteinate form and selenium-yeast to transition cows: Performance, trace minerals, and antioxidant status, peripheral neutrophil activity, and oocyte quality. J Dairy Sci (2023) 106(4):3023–42. doi: 10.3168/jds.2022-21939
80. Ogilvie L, Van Winters B, Mion B, King K, Spricigo JF, Karrow NA, et al. Effects of replacing inorganic salts of trace minerals with organic trace minerals in the diet of prepartum cows on the quality of colostrum and immunity of newborn calves. J Dairy Sci (2023) 106(5):3493–508. doi: 10.3168/jds.2022-21913
81. Ganda EK, Bisinotto RS, Vasquez AK, Teixeira AGV, MaChado VS, Foditsch C, et al. Effects of injectable trace mineral supplementation in lactating dairy cows with elevated somatic cell counts. J Dairy Sci (2016) 99:7319–29. doi: 10.3168/jds.2016-10989
82. MaChado VS, Oikonomou G, Lima SF, Bicalho ML, Kacar C, Foditsch C, et al. The effect of injectable trace minerals (Selenium, copper, zinc, and manganese) on peripheral blood leukocyte activity and serum superoxide dismutase activity of lactating holstein cows. Vet J (2014) 200(2):299–304. doi: 10.1016/j.tvjl.2014.02.026
83. Oliveira L, Ruegg PL. Treatments of clinical mastitis occurring in cows on 51 large dairy herds in wisconsin. J Dairy Sci (2014) 97:5426–36. doi: 10.3168/jds.2013-7756
84. Hoque MN, Das ZC, Rahman ANMA, Hoque MM. Effect of administration of vitamin E, selenium and antimicrobial therapy on incidence of mastitis, productive and reproductive performances in dairy cows. Int J Vet Sci Med (2016) 4:63–70. doi: 10.1016/j.ijvsm.2016.11.001
85. MaChado VS, Bicalho ML, Pereira RV, Caixeta LS, Knauer WA, Oikonomou G, et al. Effect of an injectable trace mineral supplement containing selenium, copper, zinc, and manganese on the health and production of lactating holstein cows. Vet J (2013) 197:451–6. doi: 10.1016/j.tvjl.2013.02.022
86. Ferreira GM, Petzer I-M. Injectable organic and inorganic selenium in dairy cows–effects on milk, blood and somatic cell count levels. Onderstepoort J Vet Res (2019) 86:a1664. doi: 10.4102/ojvr.v86i1.1664
87. Bourne N, Wathes DC, Lawrence KE, McGowan M, Laven RA. The effect of parenteral supplementation of vitamin E with selenium on the health and productivity of dairy cattle in the UK. Vet J (2008) 177:381–7. doi: 10.1016/j.tvjl.2007.06.006
88. Smulski S, Gehrke M, Libera K, Cieślak A, Huang H, Patra AK, et al. Effects of various mastitis treatments on the reproductive performance of cows. BMC Vet Res (2020) 16:99. doi: 10.1186/s12917-020-02305-7
89. Goff JP. The monitoring, prevention, and treatment of milk fever and subclinical hypocalcemia in dairy cows. Vet J (2008) 176:50–7. doi: 10.1016/j.tvjl.2007.12.020
90. Rodríguez EM, Arís A, Bach A. Associations between subclinical hypocalcemia and postparturient diseases in dairy cows. J Dairy Sci (2017) 100(9):7427–34. doi: 10.3168/jds.2016-12210
91. Martinez N, Sinedino LD, Bisinotto RS, Daetz R, Lopera C, Risco CA, et al. Effects of oral calcium supplementation on mineral and acid-base status, energy metabolites, and health of postpartum dairy cows. J Dairy Science. (2016) 99(10):8397–416.
92. Zhang ZB, Guo YF, Li CY, Qiu CW, Guo MY. Selenium influences mmu-miR-155 to inhibit inflammation in Staphylococcus aureus-induced mastitis in mice. Food Funct (2019) 10:6543–55. doi: 10.1039/C9FO01488H
93. Hisaeda K, Koshiishi T, Sasaki A, Shinozuka Y, Isobe N, Kawai K. Changes in ionized calcium concentration in the blood of dairy cows with peracute coliform mastitis. J Vet Med Sci (2020) 82:457–62. doi: 10.1292/jvms.19-0678
94. Deb R, Singh U, Kumar S, Kumar A, Singh R, Sengar G, et al. Genotypic to expression profiling of bovine calcium channel, voltage-dependent, alpha-2/delta subunit 1 gene, and their association with bovine mastitis among Frieswal (HFX Sahiwal) crossbred cattle of Indian origin. Anim Biotechnol (2014) 25(2):128–38. doi: 10.1080/10495398.2013.836106
95. Magotra A, Gupta ID, Verma A, Alex R, Mr V, Ahmad T. Candidate SNP of CACNA2D1 gene associated with clinical mastitis and production traits in Sahiwal (Bos taurus indicus) and Karan fries (Bos taurus taurus× Bos taurus indicus). Anim Biotechnol (2019) 30(1):75–81. doi: 10.1080/10495398.2018.1437046
96. Olivares R, Postma GC, Schapira A, Iglesias DE, Valdez LB, Breininger E, et al. Biochemical and morphological alterations in hearts of copper-deficient bovines. Biol Trace Elem Res (2019) 189:447–55. doi: 10.1007/s12011-018-1476-x
98. Reyes-Jara A, Cordero N, Aguirre J, Troncoso M, Figueroa G. Antibacterial effect of copper on microorganisms isolated from bovine mastitis. Front Microbiol (2016) 7:626. doi: 10.3389/fmicb.2016.00626
99. Wernicki A, Puchalski A, Urban-Chmiel R, Dec M. Antimicrobial properties of gold, silver, copper and platinum nanoparticles against selected microorganisms isolated from cases of mastitis in cattle. Med Weter (2014) 70(9):564–7.
100. Kalińska A, Jaworski S, Wierzbicki M, Gołębiewski M. Silver and copper nanoparticles—An alternative in future mastitis treatment and prevention? IJMS (2019) 20:1672. doi: 10.3390/ijms20071672
101. Scaletti R, Trammell DS, Smith BA, Harmon RJ. Role of dietary copper in enhancing resistance to escherichia coli mastitis. J Dairy Sci (2003) 86:1240–9. doi: 10.3168/jds.S0022-0302(03)73708-4
102. Gakhar G, Randhawa SS, Randhawa CS, Bansal BK, Singh RS. Effect of copper on the milk quality and prevention of mastitis in dairy cows. Indian J Anim Sci (2010) 80:727–8.
103. Babu U, Failla ML. Respiratory burst and candidacidal activity of peritoneal macrophages are impaired in copper-deficient rats. J Nutr (1990) 120:1692–9. doi: 10.1093/jn/120.12.1692
104. Willing BP, Pepin DM, Marcolla CS, Forgie AJ, Diether NE, Bourrie BCT. Bacterial resistance to antibiotic alternatives: A wolf in sheep’s clothing? Anim Front (2018) 8:39–47. doi: 10.1093/af/vfy003
105. Anchordoquy JM, Anchordoquy JP, Galarza EM, Farnetano NA, Giuliodori MJ, Nikoloff N, et al. Parenteral zinc supplementation increases pregnancy rates in beef cows. Biol Trace Elem Res (2019) 192:175–82. doi: 10.1007/s12011-019-1651-8
106. Cope CM, Mackenzie AM, Wilde D, Sinclair LA. Effects of level and form of dietary zinc on dairy cow performance and health. J Dairy Sci (2009) 92:2128–35. doi: 10.3168/jds.2008-1232
107. Chandra G, Aggarwal A, Singh AK, Kumar M. Effect of vitamin E and zinc supplementation on milk yield, milk composition, and udder health in sahiwal cows. Anim Nutr Feed Tech (2015) 15:67. doi: 10.5958/0974-181X.2015.00008.6
108. Whitaker DA, Eares HF, Ait K, Kelly JM. No effect of a dietary zinc proteinate on clinical mastiffs, infection rate, recovery rate and somatic cell count in dairy cows. Vet J (1997) 153:197–204. doi: 10.1016/S1090-0233(97)80040-8
109. Weng X, Monteiro APA, Guo J, Li C, Orellana RM, Marins TN, et al. Effects of heat stress and dietary zinc source on performance and mammary epithelial integrity of lactating dairy cows. J Dairy Sci (2018) 101:2617–30. doi: 10.3168/jds.2017-13484
110. Shaffer J, Pandalaneni K, Mamedova L, DeFrain J, Amamcharla J, Bradford B. Effects of dietary zinc source and level on mammary epithelia and dairy food chemistry. Kans Agric Exp Stn Res Rep (2016) 2:8. doi: 10.4148/2378-5977.1329
111. Prasad AS. Discovery of human zinc deficiency: its impact on human health and disease. Adv Nutr (2013) 4:176–90. doi: 10.3945/an.112.003210
112. Ma J, Zhu S, Guo Y, Hao M, Chen Y, Wang Y, et al. Selenium attenuates Staphylococcus aureus mastitis in mice by inhibiting the activation of the NALP3 inflammasome and NF-κB/MAPK pathway. Biol Trace Elem Res (2019) 191:159–66. doi: 10.1007/s12011-018-1591-8
113. Liu K, Ding T, Fang L, Cui L, Li J, Meng X, et al. Organic selenium ameliorates staphylococcus aureus-induced mastitis in rats by inhibiting the activation of NF-κB and MAPK signaling pathways. Front Vet Sci (2020) 7:443. doi: 10.3389/fvets.2020.00443
114. Chen SJ, Zhang CY, Yu D, Lin CJ, Xu HJ, Hu CM. Selenium alleviates inflammation in Staphylococcus aureus-induced mastitis via MerTK-dependent activation of the PI3K/Akt/mTOR pathway in mice. Biol Trace Elem Res (2022) 200:1750–62. doi: 10.1007/s12011-021-02794-z
115. Ottaviano FG, Tang SS, Handy DE, Loscalzo J. Regulation of the extracellular antioxidant selenoprotein plasma glutathione peroxidase (GPx-3) in mammalian cells. Mol Cell Biochem (2009) 327:111–26. doi: 10.1007/s11010-009-0049-x
116. Pilarczyk B, Jankowiak D, Tomza-Marciniak A, Pilarczyk R, Sablik P, Drozd R, et al. Selenium concentration and glutathione peroxidase (GSH-px) activity in serum of cows at different stages of lactation. Biol Trace Elem Res (2012) 147:91–6. doi: 10.1007/s12011-011-9271-y
117. Salman S, Khol-Parisini A, Schafft H, Lahrssen-Wiederholt M, Hulan HW, Dinse D, et al. The role of dietary selenium in bovine mammary gland health and immune function. Anim Health Res Rev (2009) 10:21–34. doi: 10.1017/S1466252308001588
118. Hall JA, Bobe G, Vorachek WR, Kasper K, Traber MG, Mosher WD, et al. Effect of supranutritional organic selenium supplementation on postpartum blood micronutrients, antioxidants, metabolites, and inflammation biomarkers in selenium-replete dairy cows. Biol Trace Elem Res (2014) 161:272–87. doi: 10.1007/s12011-014-0107-4
119. Diyabalanage S, Dangolla A, Mallawa C, Rajapakse S, Chandrajith R. Bioavailability of selenium (Se) in cattle population in Sri Lanka based on qualitative determination of glutathione peroxidase (GSH-Px) activities. Environ Geochem Health (2020) 42:617–24. doi: 10.1007/s10653-019-00395-3
120. Ahmadi K, Roshan-Milani S, Asgharzadeh F, Pourjabali M, Fard AA. In vitro and in vivo pretreatment with selenium mitigates tetrahydrocannabinol-induced testicular cell apoptosis: the role of AKT and p53 pathways. Biol Trace Elem Res (2021) 199:2278–87. doi: 10.1007/s12011-020-02322-5
121. Miranda S, Wang YJ, Purdie NG, Osborne VR, Coomber BL, Cant JP. Selenomethionine stimulates expression of glutathione peroxidase 1 and 3 and growth of bovine mammary epithelial cells in primary culture. J Dairy Sci (2009) 92:2670. doi: 10.3168/jds.2008-1901
122. Wang D, Jia D, He R, Lian S, Wang J, Wu R. Association between serum selenium level and subclinical mastitis in dairy cattle. Biol Trace Elem Res (2021) 199:1389–139. doi: 10.1007/s12011-020-02261-1
123. Khan MZ, Khan A, Xiao J, Ma J, Ma Y, Chen T, et al. Overview of research development on the role of NF-κB signaling in mastitis. Animals (2020d) 10:1625. doi: 10.3390/ani10091625
124. Akhtar M, Guo S, Guo YF, Zahoor A, Shaukat A, Chen Y, et al. Upregulated-gene expression of pro-inflammatory cytokines (TNF-α, IL-1β and IL-6) via TLRs following NF-κB and MAPKs in bovine mastitis. Acta Trop (2020) 207:105458. doi: 10.1016/j.actatropica.2020.105458
125. Wei MJ, Wang ZN, Yang Y, Zhang SJ, Tang H, Li H, et al. Selenium attenuates S. aureus-induced inflammation by regulating TLR2 signaling pathway and NLRP3 Inflammasome in RAW 264.7 Macrophages. Biol Trace Elem Res (2022) 200:761–7. doi: 10.1007/s12011-021-02676-4
126. Kelley N, Jeltema D, Duan Y, He Y. The NLRP3 inflammasome: An overview of mechanisms of activation and regulation. Int J Mol Sci (2019) 20:3328. doi: 10.3390/ijms20133328
127. Bi CL, Zhang SJ, Shen YZ, Pauline M, Li H, Tang H. Selenium plays an anti-inflammatory role by regulating NLRP3 inflammasome in Staphylococcus aureus-infected mouse mammary gland. Biol Trace Elem Res (2021) 199:604–10. doi: 10.1007/s12011-020-02166-z
128. Vunta H, Belda BJ, Arner RJ, Reddy CC, Heuvel JPV, Prabhu KS. Selenium attenuates pro-inflammatory gene expression in macrophages. Mol Nutr Food Res (2010) 52:1316–23. doi: 10.1002/mnfr.200700346
129. Bi CL, Wang H, Wang YJ, Sun J, Dong JS, Meng X, et al. Selenium inhibits Staphylococcus aureus induced inflammation by suppressing the activation of the NF-κB and MAPK signaling pathways in RAW264.7 macrophages. Eur J Pharmacol (2016) 780:159–65. doi: 10.1016/j.ejphar.2016.03.044
130. Aitken SL, Corl CM, Sordillo LM. Immunopathology of mastitis: Insights into disease recognition and resolution. J Mammary Gland Biol (2011) 16:291–304. doi: 10.1007/s10911-011-9230-4
131. Lahouassa H, Moussay E, Rainard P, Riollet C. Differential cytokine and chemokine responses of bovine mammary epithelial cells to Staphylococcus aureus and Escherichia coli. Cytokine. (2007) 38:12–21. doi: 10.1016/j.cyto.2007.04.006
132. Wang H, Bi C, Wang Y, Sun J, Meng X, Li J. Selenium ameliorates Staphylococcus aureus-induced inflammation in bovine mammary epithelial cells by inhibiting activation of TLR2, NF-κB and MAPK signaling pathways. BMC veterinary Res (2018) 14:1–8.
133. Eken C, Martin PJ, Sadallah S, Treves S, Schaller M, Schifferli JA. Ectosomes released by polymorphonuclear neutrophils induce a MerTK-dependent anti-inflammatory pathway in macrophages. J Biol Chem (2010) 285:39914–21. doi: 10.1074/jbc.M110.126748
134. Zhang B, Fang L, Wu HM, Ding PS, Xu K, Liu RY. Mer receptor tyrosine kinase negatively regulates lipoteichoic acid-induced inflammatory response via PI3K/Akt and SOCS3. Mol Immunol (2016) 76:98–107. doi: 10.1016/j.molimm.2016.06.016
135. Jing H, Chen Y, Liang W, Chen M, Qiu C, Guo MY. Effects of selenium on MAC-T cells in bovine mastitis: transcriptome analysis of exosomal mRNA interactions. Biol Trace Elem Res (2021) 199:2904–12. doi: 10.1007/s12011-020-02439-7
136. Gao X, Zhang Z, Li Y, Hu X, Shen P, Fu Y, et al. Selenium deficiency deteriorates the inflammation of S. aureus infection via regulating NF-κB and PPAR-γ in mammary gland of mice. Biol Trace Elem Res (2016) 172:140–7. doi: 10.1007/s12011-015-0563-5
137. Zhang W, Zhang R, Wang T, Jiang H, Guo M, Zhou E, et al. Selenium Inhibits LPS-Induced Pro-inflammatory Gene Expression by Modulating MAPK and NF-κB Signaling Pathways in Mouse Mammary Epithelial Cells in Primary Culture. Inflammation. (2014) 37:478–85. doi: 10.1007/s10753-013-9761-5
138. Wang L, Jing J, Yan H, Tang J, Jia G, Liu G, et al. Selenium pretreatment alleviated LPS-induced immunological stress via upregulation of several selenoprotein encoding genes in murine RAW264. 7 Cells Biol Trace Elem Res (2018) 186:505–13. doi: 10.1007/s12011-018-1333-y
139. Stewart WCG, Bobe G, Pirelli W, Mosher J. Organic and inorganic Selenium: III. Ewe progeny performance. J Anim. Sci (2012) 90:4536–43. doi: 10.2527/jas.2011-5019
140. Gong J, Ni L, Wang D, Shi B, Yan S. Effect of dietary organic Selenium on milk selenium concentration and antioxidant and immune status in midlactation dairy cows. Livest. Sci (2014) 170:84–90. doi: 10.1016/j.livsci.2014.10.003
141. Gong J, Xiao M. Selenium and antioxidant status in dairy cows at different stages of lactation. Biol Trace Elem Res (2016) 171:89–93. doi: 10.1007/s12011-015-0513-2
142. Sun P, Wang J, Liu W, Bu DP, Liu SJ, Zhang KZ. Hydroxy-selenomethionine: A novel organic selenium source that improves antioxidant status and selenium concentrations in milk and plasma of mid-lactation dairy cows. J Dairy Sci (2017) 100:9602–10. doi: 10.3168/jds.2017-12610
143. Juniper DT, Rymer C, Briens M. Bioefficacy of hydroxy-selenomethionine as a selenium supplement in pregnant dairy heifers and on the selenium status of their calves. J Dairy Sci (2019) 102:7000–10. doi: 10.3168/jds.2018-16065
144. Hemingway R. The influences of dietary Selenium and vitamin E intakes on milk somatic cell counts and mastitis in cows. Vet Res Commun (1999) 23:481–99. doi: 10.3390/nu10091203
145. Avery J, Hoffmann P. Selenium, Selenoproteins, and immunity. Nutrients. (2018) 10:1203. doi: 10.3390/nu10091203
146. Wang N, Tan H, Li S, Xu Y, Guo W, Feng Y. Supplementation of micronutrient selenium in metabolic diseases: Its role as an antioxidant. Oxid Med Cell Longev (2017) 2017:7478523. doi: 10.1155/2017/7478523
147. Ali-Vehmas T, Vikerpuur M, Fang W, Sandholm M. Giving selenium supplements to dairy cows strengthens the inflammatory response to intramammary infection and induces a growth-suppressing effect on mastitis pathogens in whey. J Vet Med Ser A. (1997) 44:559–71. doi: 10.1111/j.1439-0442.1997.tb01142.x
148. Malbe M, Klaassen E, Kaartinen L, Attila M, Atroshi F. Effects of oral selenium supplementation on mastitis markers and pathogens in Estonian cows. Vet Ther Res Appl Vet Med (2003) 4:145–54.
149. Malbe M, Attila M, Atroshi F. Possible involvement of Selenium in Staphylococcus aureus inhibition in cow’s whey. J Anim Physiol Anim Nutr (2006) 90:159–64. doi: 10.1111/j.1439-0396.2005.00582.x
150. Cui L, Li L, Tian Y, Xu F, Qiao T. Association between dietary vitamin E intake and esophageal cancer risk: an updated meta-analysis. Nutrients. (2018) 10(7):801.
151. Yang Y, Lv S, Wang Z, Liu J. Selenium ameliorates S. aureus-induced inflammation in bovine mammary epithelial cells by regulating ROS-induced NLRP3 inflammasome. Biol Trace Elem Res (2021) 200:3171–5. doi: 10.1007/s12011-021-02924-7
152. Gao X, Zhang Z, Li Y, Shen P, Hu X, Cao Y, et al. Selenium deficiency facilitates inflammation following S. aureus infection by regulating TLR2-related pathways in the mouse mammary gland. Biol Trace Elem Res (2016) 172:449–57. doi: 10.1007/s12011-015-0614-y
153. Ceballos-Marquez A, Barkema HW, Stryhn H, Dohoo IR, Keefe GP, Wichtel JJ. Milk selenium concentration and its association with udder health in Atlantic Canadian dairy herds. J Dairy Sci (2010) 93:4700–9. doi: 10.3168/jds.2010-3313
154. Hills EK, Li J. Current and future prospects for nanotechnology in animal production. J Anim Sci Biotechnol (2017) 8:26. doi: 10.1186/s40104-017-0157-5
155. Li Y, Zhang W, Niu J, Chen Y. Mechanism of photogenerated reactive oxygen species and correlation with the antibacterial properties of engineered metal-oxide nanoparticles. ACS Nano (2012) 6:5164–73. doi: 10.1021/nn300934k
156. Rai MK, Deshmukh SD, Ingle AP, Gade AK. Silver Nanoparticles: The Powerful Nanoweapon against Multidrug-Resistant Bacteria: Activity of Silver Nanoparticles against MDR Bacteria. J Appl Microbiol (2012) 112:841–52. doi: 10.1111/j.1365-2672.2012.05253.x
157. Algharib SA, Dawood A, Xie S. Nanoparticles for treatment of bovine Staphylococcus aureus mastitis. Drug Delivery (2020) 27(1):292–308. doi: 10.1080/10717544.2020.1724209
158. Sharun K, Dhama K, Tiwari R, Gugjoo MB, Iqbal Yatoo M, Patel SK, et al. Advances in therapeutic and managemental approaches of bovine mastitis: a comprehensive review. Vet Q (2021) 41(1):107–36. doi: 10.1080/01652176.2021.1882713
159. Elbehiry A, Al-Dubaib M, Marzouk E, Moussa I. Antibacterial effects and resistance induction of silver and gold nanoparticles against staphylococcus aureus-induced mastitis and the potential toxicity in rats. Microbiol Open (2019) 8:e00698. doi: 10.1002/mbo3.698
160. Hozyen HF, Ibrahim ES, Khairy EA, El-Dek SI. Enhanced antibacterial activity of capped zinc oxide nanoparticles: A step towards the control of clinical bovine mastitis. Vet World (2019) 12:1225–32. doi: 10.14202/vetworld.2019.1225-1232
161. Jagielski T, Bakuła Z, Pleń M, Kamiński M, Nowakowska J, Bielecki J, et al. The activity of silver nanoparticles against microalgae of the prototheca genus. Nanomedicine (2018) 13:1025–36. doi: 10.2217/nnm-2017-0370
162. Yang FL, Li XS. Role of antioxidant vitamins and trace elements in mastitis in dairy cows. J Adv Vet Anim. Res (2015) 2:1–9. doi: 10.5455/javar.2015.b48
163. Hogan JS, Weiss WP, Smith KL. Role of vitamin E and selenium in host defense against mastitis. J Dairy Sci (1993) 76(9):2795–803. doi: 10.3168/jds.S0022-0302(93)77618-3
164. Smith KL, Hogan JS, Weiss WP. Dietary vitamin E and selenium affect mastitis and milk quality. J Anim Sci (1997) 75:1659–65. doi: 10.2527/1997.7561659x
165. Khan MZ, Zhang Z, Liu L, Wang D, Mi S, Liu X, et al. Folic acid supplementation regulates key immunity-associated genes and pathways during the periparturient period in dairy cows. Asian Austral J Anim (2020a) 33(9):1507–19. doi: 10.5713/ajas.18.0852
166. Mi S, Tang Y, Shi L, Liu X, Si J, Yao Y, et al. Protective roles of folic acid in the responses of bovine mammary epithelial cells to different virulent Staphylococcus aureus Strains. Biology (2021b) 10(11):1164. doi: 10.3390/biology10111164
167. Khan MZ, Khan A, Xiao J, Dou J, Liu L, Yu Y. Overview of folic acid supplementation alone or in combination with vitamin B12 in dairy cattle during the periparturient period. Metabolites (2020c) 10(6):263. doi: 10.3390/metabo10060263
168. Wang B, Li H, Li Z, Wang B, Zhang H, Zhang B, et al. Integrative network analysis revealed the molecular function of folic acid on immunological enhancement in a sheep model. Front Immunol (2022) 13:913854. doi: 10.3389/fimmu.2022.913854
169. Khan MZ, Liu L, Zhang Z, Khan A, Wang D, Mi S, et al. Folic acid supplementation regulates milk production variables, metabolic associated genes and pathways in perinatal Holsteins. J Anim Physiol N (2020b) 104(2):483–92. doi: 10.1111/jpn.13313
170. Feng D, Zhou Y, Xia M, Ma J. Folic acid inhibits lipopolysaccharide-induced inflammatory response in RAW264.7 macrophages by suppressing MAPKs and NF-κB activation. Inflammation Res (2011) 60:817–22. doi: 10.1007/s00011-011-0337-2
171. Miszewski SG, Trott JF, Berryhill GE, Tat L, Green R, Borowsky AD, et al. Folate deficiency inhibits development of the mammary gland and its associated lymphatics in FVB mice. Ann N Y Acad Sci (2016) 1372:53–64.
172. Liu X, Mi S, Li W, Zhang J, Augustino S, Zhang Z, et al. Molecular regulatory mechanism of key LncRNAs in subclinical mastitic cows with folic acid supplementation. BMC Genomics (2023) 24(1):1–7. doi: 10.1186/s12864-023-09466-3
173. Mi S, Tang Y, Dari G, Shi Y, Zhang J, Zhang H, et al. Transcriptome sequencing analysis for the identification of stable lncRNAs associated with bovine Staphylococcus aureus mastitis. J Anim Sci Biotechnol (2021a) 12:120. doi: 10.1186/s40104-021-00639-2
174. Fang L, Hou Y, An J, Li B, Song M, Wang X, et al. Genome-wide transcriptional and post-transcriptional regulation of innate immune and defense responses of bovine mammary gland to Staphylococcus aureus. Front Cell Infect Microbiol (2016) 6:193. doi: 10.3389/fcimb.2016.00193
175. Kosciuczuk EM, Lisowski P, Jarczak J, Majewska A, Rzewuska M, Zwierzchowski L, et al. Transcriptome profiling of Staphylococci-infected cow mammary gland parenchyma. BMC Vet Res (2017) 13:161. doi: 10.1186/s12917-017-1088-2
176. Bae D, Chon J-W, Kim D-H, Kim H, Seo K-H. Effect of folic acid supplementation on proliferation and apoptosis in bovine mammary epithelial (MAC-T) cells. Anim Biotechnol (2022) 33:13–21. doi: 10.1080/10495398.2020.1758123
177. Lin J, Kumari S, Kim C, Van TM, Wachsmuth L, Polykratis A, et al. RIPK1 counteracts ZBP1-mediated necroptosis to inhibit inflammation. Nat Cell Biol (2016) 540:124–8. doi: 10.1038/nature20558
178. Kamal-Eldin A, Appelqvist L.Å.. The chemistry and antioxidant properties of tocopherols and tocotrienols. Lipids. (1996) 31:671–701. doi: 10.1007/BF02522884
179. Sontag TJ, Parker RS. Influence of major structural features of tocopherols and tocotrienols on their ω-oxidation by tocopherol-ω-hydroxylase. J Lipid Res (2007) 48:1090–8. doi: 10.1194/jlr.M600514-JLR200
180. Yoshida Y, Niki E, Noguchi N. Comparative study on the action of tocopherols and tocotrienols as antioxidant: Chemical and physical effects. Chem Phys Lipids (2003) 123:63–75. doi: 10.1016/S0009-3084(02)00164-0
181. Lee GY, Han SN. The role of vitamin E in immunity. Nutrients (2018) 10:1614. doi: 10.3390/nu10111614
182. El-Demerdash FM. Antioxidant effect of vitamin E and Selenium on lipid peroxidation, enzyme activities and biochemical parameters in rats exposed to aluminium. J Trace Elem Med Biol (2004) 18:113–21. doi: 10.1016/j.jtemb.2004.04.001
183. Pekmezci D. Vitamin E and immunity. Vitam Horm (2011) 86:179–215. doi: 10.1016/B978-0-12-386960-9.00008-3
184. Bivona JJ III, Patel S, Vajdy M. Induction of cellular and molecular Immunomodulatory pathways by vitamin E and vitamin C. Expert Opin Biol Ther (2017) 17:1539–51. doi: 10.1080/14712598.2017.1375096
185. Meydani SN, Beharka AA. Recent developments in vitamin E and immune response. Nutrition Reviews (1998) 56(1):S49–58.
186. Kuhn MJ, Sordillo LM. Vitamin E analogs limit in vitro oxidant damage to bovine mammary endothelial cells. J Dairy Sci (2021) 104:7154–67. doi: 10.3168/jds.2020-19675
187. Mokhber-Dezfouli MR, Rahimikia E, Asadi F, Nadalian MG. The role of route of vitamin E administration on the plasma antioxidant activity and lipid peroxidation in newborn calves. Basic Clin Pharmacol Toxicol (2008) 103:414–8. doi: 10.1111/j.1742-7843.2008.00308.x
188. Pritee G, Upadhyay AK, Gangwar NK, Rajput MKS. Relationship of mineral and vitamin supplementation with mastitis. Vet World (2008) 1:103–4.
189. Erickson KL, Medina EA, Hubbard NE. Micronutrients and innate immunity. J Infect Dis (2000) 182((Suppl. 1):S5–S10. doi: 10.1086/315922
190. Politis I, Theodorou G, Lampidonis AD, Kominakis A, Baldi A. Short communication: Oxidative status and incidence of mastitis relative to blood α-tocopherol concentrations in the postpartum period in dairy cows. J Dairy Sci (2012b) 95:7331–5. doi: 10.3168/jds.2012-5866
191. Politis I. Reevaluation of vitamin E supplementation of dairy cows: Bioavailability, animal health, and milk quality. Animal (2012a) 6:1427–34. doi: 10.1017/S1751731112000225
192. Cengiz M, Bastan A. Effectiveness of dry cow therapy antibiotic treatment, internal teat sealant, and α-tocopherol against new intramammary infections in cows. Bull Vet Inst Pulawy (2015) 59:71–8. doi: 10.1515/bvip-2015-0011
193. Singh AK, Bhakat C, Kumari T, Mandal DK, Chatterjee A, Karunakaran M, et al. Influence of pre and postpartum alpha-tocopherol supplementation on milk yield, milk quality and udder health of Jersey crossbred cows at tropical lower Gangetic region. Vet World (2020) 13:2006–11. doi: 10.14202/vetworld.2020.2006-2011
194. Sharma N, Maiti SK. Effect of dietary supplementation of vitamin E and selenium in subclinical mastitis in dairy cows. Indian J Vet Med (2005) 25:76–9.
195. Nyman A-K, Emanuelson U, Holtenius K, Ingvartsen KL, Larsen T, Waller KP. Metabolites and immune variables associated with somatic cell counts of primiparous dairy cows. J Dairy Sci (2008) 91:2996–3009. doi: 10.3168/jds.2007-0969
196. Vieira-Neto A, Poindexter MB, Marinho MN, Zimpel R, Husnain A, Silva AC, et al. Effect of source and amount of vitamin D on function and mRNA expression in immune cells in dairy cows. J Dairy Sci (2021) 104(10):10796–811. doi: 10.3168/jds.2021-20284
197. Nelson CD, Reinhardt TA, Beitz DC, Lippolis JD. In vivo activation of the intracrine vitamin D pathway in innate immune cells and mammary tissue during a bacterial infection. PloS One (2010) 5(11):e15469. doi: 10.1371/journal.pone.0015469
198. Merriman KE, Powell JL, Santos JE, Nelson CD. Intramammary 25-hydroxyvitamin D3 treatment modulates innate immune responses to endotoxin-induced mastitis. J Dairy Sci (2018) 101(8):7593–607. doi: 10.3168/jds.2017-14143
199. Merriman KE, Kweh MF, Powell JL, Lippolis JD, Nelson CD. Multiple β-defensin genes are upregulated by the vitamin D pathway in cattle. J Steroid Biochem Mol Biol (2015) 154:120–9. doi: 10.1016/j.jsbmb.2015.08.002
200. Merriman KE, Poindexter MB, Kweh MF, Santos JE, Nelson CD. Intramammary 1,25-dihydroxyvitamin D3 treatment increases expression of host-defense genes in mammary immune cells of lactating dairy cattle. J Steroid Biochem Mol Biol (2017) 173:33–41. doi: 10.1016/j.jsbmb.2017.02.006
201. Kweh MF, Merriman KE, Wells TL, Nelson CD. Vitamin D signaling increases nitric oxide and antioxidant defenses of bovine monocytes. JDS Commun (2021) 2(2):73–9. doi: 10.3168/jdsc.2020-0005
202. Yue Y, Hymøller L, Jensen SK, Lauridsen C, Purup S. Effects of vitamin D and its metabolites on cell viability and Staphylococcus aureus invasion into bovine mammary epithelial cells. Vet Microbiol (2017) 203:245–51. doi: 10.1016/j.vetmic.2017.03.008
203. Strickland JM, Wisnieski L, Mavangira V, Sordillo LM. Serum vitamin D is associated with antioxidant potential in Peri-parturient cows. Antioxidants (2021) 10(9):1420. doi: 10.3390/antiox10091420
204. Eder K, Grundmann SM. Vitamin D in dairy cows: metabolism, status and functions in the immune system. Arch Anim Nutr (2022) 76(1):1–33. doi: 10.1080/1745039X.2021.2017747
205. Reid C, Flores-Villalva S, Remot A, Kennedy E, O’farrelly C, Meade KG. Long-term in vivo vitamin D3 supplementation modulates bovine IL-1 and chemokine responses. Sci Rep (2023) 13(1):10846. doi: 10.1038/s41598-023-37427-z
206. Zhang J, Liu YP, Bu LJ, Liu Q, Pei CX, Huo WJ. Effects of dietary folic acid supplementation on lactation performance and mammary epithelial cell development of dairy cows and its regulatory mechanism. Anim Biotechnol (2023) 4:1–2. doi: 10.1080/10495398.2023.2228842
207. Xu HJ, Jiang X, Zhang CR, Ma GM, Wang LH, Zhang QY, et al. Effects of dietary 25-hydroxyvitamin D3 on the lactation performance, blood metabolites, antioxidant and immune function in dairy cows. Livest Sci (2021) 248:104497. doi: 10.1016/j.livsci.2021.104497
208. Galal AM, Walker LA, Khan IA. Induction of GST and related events by dietary phytochemicals: sources, chemistry, and possible contribution to chemoprevention. Curr Top Med Chem (2015) 14:2802–21. doi: 10.2174/1568026615666141208110721
209. Singhal SS, Singh SP, Singhal P, Horne D, Singhal J, Awasthi S. Antioxidant role of glutathione transferases: 4-hydroxynonenal, a key molecule in stress-mediated signaling. Toxicol Appl Pharmacol (2015) 289:361–70. doi: 10.1016/j.taap.2015.10.006
210. Ye ZW, Zhang J, Townsend DM, Tew KD. Oxidative stress, redox regulation and diseases of cellular differentiation. Biochim Biophys Acta (2015) 1850:1607–21. doi: 10.1016/j.bbagen.2014.11.010
211. Osorio JS, Ji P, Drackley JK, Luchini D, Loor JJ. Smartamine M and MetaSmart supplementation during the peripartal period alter hepatic expression of gene networks in 1-carbon metabolism, inflammation, oxidative stress, and the growth hormone-insulin-like growth factor 1 axis pathways. J Dairy Sci (2014a) 97:7451–64. doi: 10.3168/jds.2014-8680
212. Zhou Z, Trevisi E, Luchini DN, Loor JJ. Differences in liver functionality indexes in peripartal dairy cows fed rumen-protected methionine or choline are associated with performance, oxidative stress status, and plasma amino acid profiles. J Dairy Sci (2017) 100(8):6720–32. doi: 10.3168/jds.2016-12299
213. Sun F, Cao Y, Cai C, Li S, Yu C, Yao J. Regulation of nutritional metabolism in transition dairy cows: Energy homeostasis and health in response to post-ruminal choline and methionine. PloS One (2016) 11(8):e0160659. doi: 10.1371/journal.pone.0160659
214. Bartosz G. Non-enzymatic antioxidant capacity assays: limitations of use in biomedicine. Free Radical Res (2010) 44:711–20. doi: 10.3109/10715761003758114
215. Sordillo LM. Selenium-dependent regulation of oxidative stress and immunity in periparturient dairy cattle. Veterinary Med Int (2013) 2013. doi: 10.1155/2013/154045
216. Ingvartsen KL, Moyes KM. Factors contributing to immunosuppression in the dairy cow during the periparturient period. Japanese J Veterinary Res (2015) 63(Supplement 1):S15–24.
217. Esposito G, Irons PC, Webb EC, Chapwanya A. Interactions between negative energy balance, metabolic diseases, uterine health and immune response in transition dairy cows. Anim Reprod Sci (2014) 144(3-4):60–71. doi: 10.1016/j.anireprosci.2013.11.007
218. Esposito G, Raffrenato E, Lukamba SD, Adnane M, Irons PC, Cormican P, et al. Characterization of metabolic and inflammatory profiles of transition dairy cows fed an energy-restricted diet. J Anim Sci (2020) 98(1):skz391. doi: 10.1093/jas/skz391
219. Vanacker N, Ollier S, Beaudoin F, Blouin R, Lacasse P. Effect of inhibiting the lactogenic signal at calving on milk production and metabolic and immune perturbations in dairy cows. J Dairy Sci (2017) 100:5782–91. doi: 10.3168/jds.2017-12570
220. Osorio JS, Trevisi E, Ji P, Drackley JK, Luchini D, Bertoni G, et al. Biomarkers of inflammation, metabolism, and oxidative stress in blood, liver, and milk reveal a better immunometabolic status in peripartal cows supplemented with Smartamine M or MetaSmart. J Dairy Sci (2014b) 97:7437–50. doi: 10.3168/jds.2013-7679
221. Zhou Z, Vailati-Riboni M, Trevisi E, Drackley JK, Luchini DN, Loor JJ. Better postpartal performance in dairy cows supplemented with rumen-protected methionine compared with choline during the peripartal period. J dairy science (2016) 99(11):8716–32. doi: 10.3168/jds.2015-10525
222. Batistel F, Arroyo JM, Garces CI, Trevisi E, Parys C, Ballou MA, et al. Ethyl-cellulose rumen-protected methionine alleviates inflammation and oxidative stress and improves neutrophil function during the periparturient period and early lactation in Holstein dairy cows. J dairy science (2018) 101(1):480–90. doi: 10.3168/jds.2017-13185
223. Wang H, Elsaadawy SA, Wu Z, Bu DP. Maternal supply of ruminally-protected lysine and methionine during close-up period enhances immunity and growth rate of neonatal calves. Front Veterinary Science (2021) 8:780731. doi: 10.3389/fvets.2021.780731
224. Hu L, Chen Y, Cortes IM, Coleman DN, Dai H, Liang Y, et al. Supply of methionine and arginine alters phosphorylation of mechanistic target of rapamycin (mTOR), circadian clock proteins, and α-s1-casein abundance in bovine mammary epithelial cells. Food Funct (2020) 11(1):883–94. doi: 10.1039/C9FO02379H
225. Dai H, Coleman DN, Hu L, Martinez-Cortés I, Wang M, Parys C, et al. Methionine and arginine supplementation alter inflammatory and oxidative stress responses during lipopolysaccharide challenge in bovine mammary epithelial cells in vitro. J Dairy Sci (2020) 103(1):676–89. doi: 10.3168/jds.2019-16631
226. Abdelmegeid MK, Vailati-Riboni M, Alharthi A, Batistel F, Loor JJ. Supplemental methionine, choline, or taurine alter in vitro gene network expression of polymorphonuclear leukocytes from neonatal Holstein calves. J Dairy Sci (2017) 100:3155–65. doi: 10.3168/jds.2016-12025
227. Zhou Z, Ferdous F, Montagner P, Luchini DN, Corrêa MN, Loor JJ. Methionine and choline supply during the peripartal period alter polymorphonuclear leukocyte immune response and immunometabolic gene expression in Holstein cows. J Dairy Sci (2018) 101:10374–82. doi: 10.3168/jds.2018-14972
228. Mu T, Kong GH, Han ZY, Li HX. Cytoprotection of methionine on hyperthermia-induced damage in bovine mammary epithelial cells. Cell Biol Int (2014) 38:971–6. doi: 10.1002/cbin.10271
229. Han ZY, Mu T, Yang Z. Methionine protects against hyperthermia-induced cell injury in cultured bovine mammary epithelial cells. Cell Stress Chaperones (2015) 20:109–20. doi: 10.1007/s12192-014-0530-7
230. Dai H, Coleman DN, Lopes MG, Hu L, Martinez-Cortés I, Parys C, et al. Alterations in immune and antioxidant gene networks by gamma-d-glutamyl-meso-diaminopimelic acid in bovine mammary epithelial cells are attenuated by in vitro supply of methionine and arginine. J Dairy Science (2021) 104(1):776–85. doi: 10.3168/jds.2020-19307
231. Cheng X, Aabdin ZU, Wang Y, Ma N, Dai H, Shi X, et al. Glutamine pretreatment protects bovine mammary epithelial cells from inflammation and oxidative stress induced by γ-d-glutamyl-meso-diaminopimelic acid (iE-DAP). J Dairy Science (2021) 104(2):2123–39. doi: 10.3168/jds.2020-18402
232. Closs EI, Scheld JS, Sharafi M, Forstermann U. Substrate supply for NO synthase in macrophages and endothelial cells: role of cationic amino acid transporters. Mol Pharmacol (2000) 57:68–74.
233. Li P, Yin YL, Li DF, Kim SW, Wu G. Amino acids and immune function. Br J Nutr (2007) 98:237–52. doi: 10.1017/S000711450769936X
234. Jensen A, Figueiredo-Larsen M, Holm R, Broberg ML, Brodin B, Nielsen CU. PAT1 (SLC36A1) shows nuclear localization and affects growth of smooth muscle cells from rats. Am J Physiol-Endocrinol Metab (2014) 306(1):E65–74. doi: 10.1152/ajpendo.00322.2013
235. Han M, Zhang M. The regulatory mechanism of amino acids on milk protein and fat synthesis in mammary epithelial cells: a mini review. Anim Biotechnol (2023) 34(2):402–12. doi: 10.1080/10495398.2021.1950743
236. Ma YF, Wu ZH, Gao M, Loor JJ. Nuclear factor erythroid 2-related factor 2-antioxidant activation through the action of ataxia telangiectasia-mutated serine/threonine kinase is essential to counteract oxidative stress in bovine mammary epithelial cells. J Dairy Sci (2018) 101(6):5317–28. doi: 10.3168/jds.2017-13954
237. Lan W, Wang Z, Liu J, Liu H. Methionyl-methionine exerts anti-inflammatory effects through the JAK2-STAT5-NF-κB and MAPK signaling pathways in bovine mammary epithelial cells. J Agric Food Chem (2020) 68(47):13742–50. doi: 10.1021/acs.jafc.0c05962
238. Abreu MB, Valldecabres A, Marcondes MI, Correa A, Lobos NE, Peterson CB, et al. Implications of supplementing mid-lactation multiparous Holstein cows fed high by-product low-forage diets with rumen-protected methionine and lysine in a commercial dairy. Animal (2023) 17(4):100749. doi: 10.1016/j.animal.2023.100749
239. Gaafar HM, Basiuoni MI, Ali MF, Shitta AA, Shamas AS. Effect of zinc methionine supplementation on somatic cell count in milk and mastitis in Friesian cows. Archiva Zootechnica (2010) 13(2):36.
240. Han L, Batistel F, Ma Y, Alharthi AS, Parys C, Loor JJ. Methionine supply alters mammary gland antioxidant gene networks via phosphorylation of nuclear factor erythroid 2-like 2 (NFE2L2) protein in dairy cows during the periparturient period. J Dairy Sci (2018) 101(9):8505–12. doi: 10.3168/jds.2017-14206
241. Jacometo CB, Alharthi AS, Zhou Z, Luchini D, Loor JJ. Maternal supply of methionine during late pregnancy is associated with changes in immune function and abundance of microRNA and mRNA in Holstein calf polymorphonuclear leukocytes. J Dairy Sci (2018) 101(9):8146–58. doi: 10.3168/jds.2018-14428
242. Jacometo CB, Zhou Z, Luchini D, Corrêa MN, Loor JJ. Maternal supplementation with rumen-protected methionine increases prepartal plasma methionine concentration and alters hepatic mRNA abundance of 1-carbon, methionine, and transsulfuration pathways in neonatal Holstein calves. J Dairy Sci (2017) 100(4):3209–19. doi: 10.3168/jds.2016-11656
243. Jacometo CB, Zhou Z, Luchini D, Trevisi E, Corrêa MN, Loor JJ. Maternal rumen-protected methionine supplementation and its effect on blood and liver biomarkers of energy metabolism, inflammation, and oxidative stress in neonatal Holstein calves. J Dairy Sci (2016) 99(8):6753–63. doi: 10.3168/jds.2016-11018
244. Lan W, Ren Y, Wang Z, Liu J, Liu H. Metabolic profile reveals the immunosuppressive mechanisms of methionyl-methionine in lipopolysaccharide-induced inflammation in bovine mammary epithelial cells. Animals (2021) 11(3):833. doi: 10.3390/ani11030833
245. Lee C, Lobos NE, Weiss WP. Effects of supplementing rumen-protected lysine and methionine during prepartum and postpartum periods on performance of dairy cows. J Dairy Sci (2019) 102(12):11026–39. doi: 10.3168/jds.2019-17125
246. Li Y, Liu JX, Xiong JL, Wang YM, Zhang WX, Wang DM. Effect of hydroxyselenomethionine on lactation performance, blood profiles, and transfer efficiency in early-lactating dairy cows. J Dairy Sci (2019) 102(7):6167–73. doi: 10.3168/jds.2019-16241
247. Liang Y, Batistel F, Parys C, Loor JJ. Methionine supply during the periparturient period enhances insulin signaling, amino acid transporters, and mechanistic target of rapamycin pathway proteins in adipose tissue of Holstein cows. J Dairy Sci (2019) 102(5):4403–14. doi: 10.3168/jds.2018-15738
248. Liang SL, Wei ZH, Wu JJ, Dong XL, Liu JX, Wang DM. Effect of N-acetyl-l-methionine supplementation on lactation performance and plasma variables in mid-lactating dairy cows. J Dairy Sci (2019b) 102(6):5182–90. doi: 10.3168/jds.2018-15716
249. Lopreiato V, Vailati-Riboni M, Bellingeri A, Khan I, Farina G, Parys C, et al. Inflammation and oxidative stress transcription profiles due to in vitro supply of methionine with or without choline in unstimulated blood polymorphonuclear leukocytes from lactating Holstein cows. J Dairy Sci (2019) 102(1):10395–410. doi: 10.3168/jds.2019-16413
250. Osorio JS, Ji P, Drackley JK, Luchini D, Loor JJ. Supplemental smartamine m or MetaSmart during the transition period benefits postpartal cow performance and blood neutrophil function. J Dairy Sci (2013) 96:6248–63. doi: 10.3168/jds.2012-5790
251. Salama AA, Caja G, Albanell E, Such X, Casals R, Plaixats J. Effects of dietary supplements of zinc-methionine on milk production, udder health and zinc metabolism in dairy goats. J Dairy Res (2003) 70(1):9–17. doi: 10.1017/S0022029902005708
252. Salama AA, Duque M, Wang L, Shahzad K, Olivera M, Loor JJ. Enhanced supply of methionine or arginine alters mechanistic target of rapamycin signaling proteins, messenger RNA, and microRNA abundance in heat-stressed bovine mammary epithelial cells in vitro. J Dairy Sci (2019) 102(3):2469–80. doi: 10.3168/jds.2018-15219
253. Soder KJ, Holden LA. Lymphocyte proliferation response of lactating dairy cows fed varying concentrations of rumen-protected methionine. J dairy science. (1999) 82(9):1935–42.
254. Vailati-Riboni M, Zhou Z, Jacometo CB, Minuti A, Trevisi E, Luchini DN, et al. Supplementation with rumen-protected methionine or choline during the transition period influences whole-blood immune response in periparturient dairy cows. J Dairy Sci (2017) 100(5):3958–68. doi: 10.3168/jds.2016-11812
255. Zhao F, Wu T, Zhang H, Loor JJ, Wang M, Peng A, et al. Jugular infusion of arginine has a positive effect on antioxidant mechanisms in lactating dairy cows challenged intravenously with lipopolysaccharide. J Anim Sci (2018a) 96(9):3850–5. doi: 10.1093/jas/sky250
Keywords: periparturient period, mastitis, dairy cattle, immunity, antioxidant status, antiinflammation, amino acids, trace minerals
Citation: Khan MZ, Huang B, Kou X, Chen Y, Liang H, Ullah Q, Khan IM, Khan A, Chai W and Wang C (2024) Enhancing bovine immune, antioxidant and anti-inflammatory responses with vitamins, rumen-protected amino acids, and trace minerals to prevent periparturient mastitis. Front. Immunol. 14:1290044. doi: 10.3389/fimmu.2023.1290044
Received: 06 September 2023; Accepted: 05 December 2023;
Published: 08 January 2024.
Edited by:
Shivraj Hariram Nile, Zhejiang Chinese Medical University, ChinaReviewed by:
Alireza Haghparast, Ferdowsi University of Mashhad, IranSaima Naz, Government Sadiq College Women University, Pakistan
Mohit Kumar, National Agri-Food Biotechnology Institute, India
Copyright © 2024 Khan, Huang, Kou, Chen, Liang, Ullah, Khan, Khan, Chai and Wang. This is an open-access article distributed under the terms of the Creative Commons Attribution License (CC BY). The use, distribution or reproduction in other forums is permitted, provided the original author(s) and the copyright owner(s) are credited and that the original publication in this journal is cited, in accordance with accepted academic practice. No use, distribution or reproduction is permitted which does not comply with these terms.
*Correspondence: Changfa Wang, d2FuZ2NoYW5nZmFAbGN1LmVkdS5jbg==; Muhammad Zahoor Khan, emFob29ya2hhdHRhazkxQHlhaG9vLmNvbQ==