- 1Department of Surgery, University of Michigan, Ann Arbor, MI, United States
- 2Department of Computational Medicine and Bioinformatics, University of Michigan, Ann Arbor, MI, United States
- 3Department of Electrical and Computer Engineering, Rice University, Houston, TX, United States
- 4Department of Surgery, Memorial Sloan Kettering Cancer Center, New York, NY, United States
- 5Department of Pathology, Memorial Sloan Kettering Cancer Center, New York, NY, United States
- 6Division of Hematology and Oncology, Department of Medicine, University of Michigan, Ann Arbor, MI, United States
- 7Department of Radiation Oncology, University of Michigan, Ann Arbor, MI, United States
- 8Department of Biomedical Engineering, University of Michigan, Ann Arbor, MI, United States
- 9Department of Biostatistics, University of Michigan, Ann Arbor, MI, United States
Introduction: Metastatic colorectal cancer (mCRC) remains a common and highly morbid disease, with a recent increase in incidence in patients younger than 50 years. There is an acute need to better understand differences in tumor biology, molecular characteristics, and other age-related differences in the tumor microenvironment (TME).
Methods: 111 patients undergoing curative-intent resection of colorectal liver metastases were stratified by age into those <50 years or >65 years old, and tumors were subjected to multiplex fluorescent immunohistochemistry (mfIHC) to characterize immune infiltration and cellular engagement.
Results: There was no difference in infiltration or proportion of immune cells based upon age, but the younger cohort had a higher proportion of programmed death-ligand 1 (PD-L1)+ expressing antigen presenting cells (APCs) and demonstrated decreased intercellular distance and increased cellular engagement between tumor cells (TCs) and cytotoxic T lymphocytes (CTLs), and between TCs and APCs. These trends were independent of microsatellite instability in tumors.
Discussion: Age-related differences in PD-L1 expression and cellular engagement in the tumor microenvironment of patients with mCRC, findings which were unrelated to microsatellite status, suggest a more active immune microenvironment in younger patients that may offer an opportunity for therapeutic intervention with immune based therapy.
Introduction
The tumor microenvironment (TME) in metastatic colorectal cancer (mCRC) is composed of both proinflammatory and immunosuppressive cells that impact disease progression and survival (1, 2). Patients with a greater proportion of cytotoxic T cells (CTLs) tend to have more favorable outcomes and lower risk of recurrence, while those with a greater proportion of immunosuppressive cells succumb to earlier disease recurrence (3–7). Programmed death-ligand 1 (PD-L1) expression has been shown to inhibit activation of granzyme-secreting CTLs (8). Tumor-resident antigen-presenting cells (APCs) that upregulate surface expression of PD-L1 directly mediate T cell suppression and are an important mechanism of tumor immune evasion (9, 10). Higher expression of PD-L1 is associated with higher stage and grade tumors, more distant metastasis, and reduced overall survival in CRC (11–13).
Not only does the composition of immune cells influence the TME and tumor biology, but characteristics of the tumor cells themselves are integral to shaping the microenvironment. For instance, defects in mismatch repair (MMR) machinery result in tumors with high mutational burden and microsatellite instability (MSI) (14, 15), which promotes both an adaptive immune response and compensatory upregulation of immune suppressive elements (16, 17). This immune state renders MMR-deficient tumors susceptible to immunotherapies targeting immunosuppressive checkpoints like PD-L1 and its receptor (18–21). However, not all patients respond to checkpoint inhibition. Immunotherapy has not yet demonstrated efficacy in clinical trials for MMR-proficient or microsatellite stable (MSS) tumors in mCRC (22, 23). These findings highlight that further understanding of the interactions between tumor cells and immune cells in the mCRC microenvironment is essential to guiding future therapeutics.
Although age is a non-modifiable risk factor for CRC incidence and mortality (24), there is a disproportionate increase in CRC incidence in patients less than 50 years of age (25). These younger patients tend to have more aggressive tumor biology and are more likely to be diagnosed at an advanced disease stage with more frequent synchronous and metachronous metastases (26). Molecular characteristics also vary between early and late onset CRC. For instance, early onset CRC is associated with higher rates of microsatellite instability and mucinous tumors (27, 28), often attributed to increased rates of hereditary syndromes, although the majority of early onset CRC is sporadic (29).
Aging significantly influences the composition of the TME. In advanced years of life, there is greater accumulation of senescent cells that secrete pro-inflammatory cytokines and chemokines, resulting in low levels of chronic inflammation, and age-related dysregulation of the immune system, which alters the TME and increases the susceptibility of tissues to metastatic seeding (30–34). Although colon cancer in younger patients appears more biologically aggressive, an in-depth analysis of the age-related differences in the TME in mCRC is lacking.
Multiplex fluorescent immunohistochemistry (mfIHC) is a technique used to provide phenotypic spatial orientation of cells within the TME, allowing quantification of cell-to-cell distance and engagement (35–39). We have previously shown using mfIHC that higher mixing of tumor cells and CTLs is associated with improved CTL engagement with tumor cells and APCs, as well as improved overall survival (35). mCRC with increased mixing of tumor cells and CTLs also demonstrated increased PD-L1+ APCs, likely a compensatory immunosuppressive response (35). We thus aimed to use mfIHC to assess age-related differences in the TME of mCRC to the liver, with the hope of informing novel approaches to immunotherapy.
Methods
Patient sample collection
The institutional review board of Memorial Sloan Kettering, New York, approved the study involving patient samples. Patient characteristics of 111 patients who underwent curative intent resection of colorectal liver metastasis were collected along with whole tissue samples. A pathologist (JS) selected 0.6 mm diameter cores from each patient block in triplicate to create a tissue microarray (TMA). Of the patients who received preoperative chemotherapy, all received standard chemotherapy treatment (40). No patients received immunotherapy.
Multiplex fluorescent immunohistochemistry staining and imaging
TMA blocks were cut into 5-micron slices and placed onto charged slides for processing. Slides were baked in a hybridization chamber for 1 hour at 60°C. Once baked, TMA slides were subjected to deparaffinization and rehydration, then fixed with formalin. Following our established protocol (35), multiplex staining was conducted on the slides through six rounds of staining. The slides were prepared for each round of staining using either an antigen retrieval buffer with pH 9 or pH 6 (AR9 and AR6 Akoya Biosciences) preceded by a primary antibody. The following primary antibodies were used—CD3, CD8, CD163, PD-L1, pancytokeratin, and FoxP3 (Supplementary Table 1) followed by secondary antibody application (Opal Polymer, Akoya Biosciences) and fluorescent tyramide signal amplification (TSA, Akoya Biosciences). Slides were counter stained using 4’,6-diamidino-2-phenylindole (DAPI), mounted, cover-slipped and left to dry overnight. Using the Mantra Quantitative Pathology WorkStation (Akoya Biosciences), cores were imaged at 20x magnification in all channels: DAPI, FITC, CY3, CY5, CY7, Texas Red, and Qdot, with an exposure of 250 ms. Composite images were created by automatically merging images from each channel, then taken for further analysis. More detailed descriptions of staining and imaging methods can be found in prior publications (35, 41).
Image analysis: phenotyping and cell-to-cell interactions
As described previously (35), InForm software (Akoya Biosciences) and novel R based programs were used to perform simple and complex phenotyping as well as cell-to-cell interactions. The following phenotypes were assigned with the previously established parameters: T cells (CD3+), regulatory T cells (Treg; CD3+CD8-FoxP3+), helper T cells (Th; CD3+CD8-FoxP3-), tumor cells (TC; PanCK+), cytotoxic T cells (CTL; CD3+CD8+), and antigen presenting cells (APC; CD163+). The nearest distance from one cell to another (nearest neighbor) and the cell-to-cell engagement was calculated as previously described (35).
Image analysis: spatial G-function
The spatial relationship between two or more types of cells was quantified by calculating the G-function to assess cellular mixing in the liver mCRC TME (35). In brief, the G-function is a function of a distance that computes the probability that cells of a reference cell type have a non-reference cell type within a certain distance. It is mathematically expressed as , where the subscripts ‘x’ and ‘y’ refer to the spatial distribution of cell type ‘y’ relative to cell type ‘x’ being calculated, ‘r’ refers to the distance from the reference cell type, and λy is the overall density of cell type ‘y’ on the slide. A Kaplan–Meier correction was applied to the G-function to correct for edge effects. As previously described (36), the G-function mathematically modeled the potential cellular interactions in the mCRC TME and the corresponding data was correlated to the patient age group.
Statistical analyses
Statistical analyses were performed using JMP Pro 13.2.0. Weighted means of the three cores were used to mitigate intratumoral heterogeneity. Differences in nearest neighbor distances and engagement were evaluated by two-sided analysis of variance (ANOVA) or bivariate analysis when appropriate. P ≤ 0.05 was considered statistically significant.
Results
Younger patients demonstrate increased infiltration of PD-L1+ APCs
To study the effect of age on the immune microenvironment in mCRC, 111 patients who underwent curative intent resection of colorectal liver metastasis were stratified by age into older (age >65 years, n=76) and younger (age <50 years, n=35) cohorts. Ages 50-65 were excluded to minimize overlap in trends between the age groups. No difference was seen between gender, disease free survival, clinical risk score, or rates of preoperative chemotherapy among the groups. Younger patients were more likely to present with smaller tumor size (L/S 3.6 vs 5.0 in the old, p=0.0221), ≥3 tumors (40% vs 20% in the old, p=0.0269) and at a higher N stage (47% N2 vs 23% in the old, p=0.0366) (Table 1).
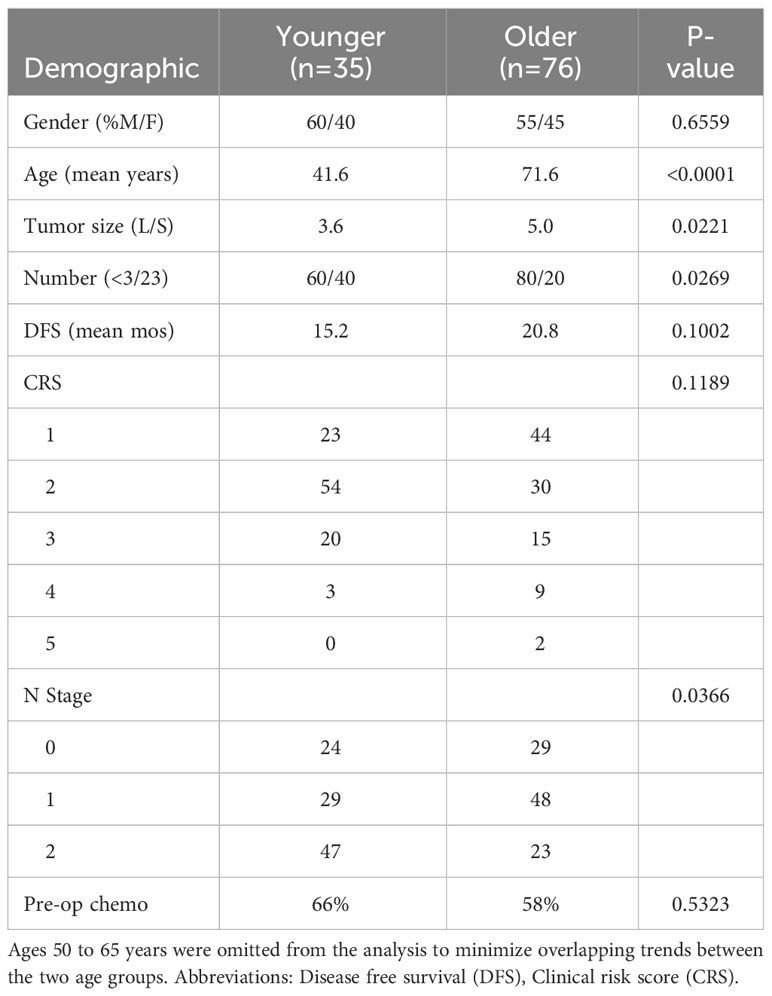
Table 1 Tumor characteristics of patients younger than 50 years of age and patients older than 65 years of age.
Tumors were subjected to mfIHC for the cell markers CD3, CD8, CD163, FoxP3, pancytokeratin, and PD-L1. InForm software (Akoya Bioscience) was used to assign a cellular phenotype and a unique spatial location within the TME as previously described (35). A representative composite mfIHC image and phenotypic map are shown in Figure 1, with representative images of an older patient >65 years in Figures 1A, B and a younger patient <50 years in Figures 1C, D. When quantifying immune composition in the TME, there was no significant difference in infiltration of APCs, T cells, CTLs, T helper cells, T regulatory cells, or the proportion of tumor cells between young and old patients. However, younger patients had a significantly higher proportion of PD-L1+ APCs of all APCs present (p=0.0387, Table 2). Representative images of PD-L1 staining on APCs and tumor cells are shown in Figures 1E, F, respectively. Although there was no difference in immune cell infiltration, this finding suggests a possible compensatory immunosuppressive TME.
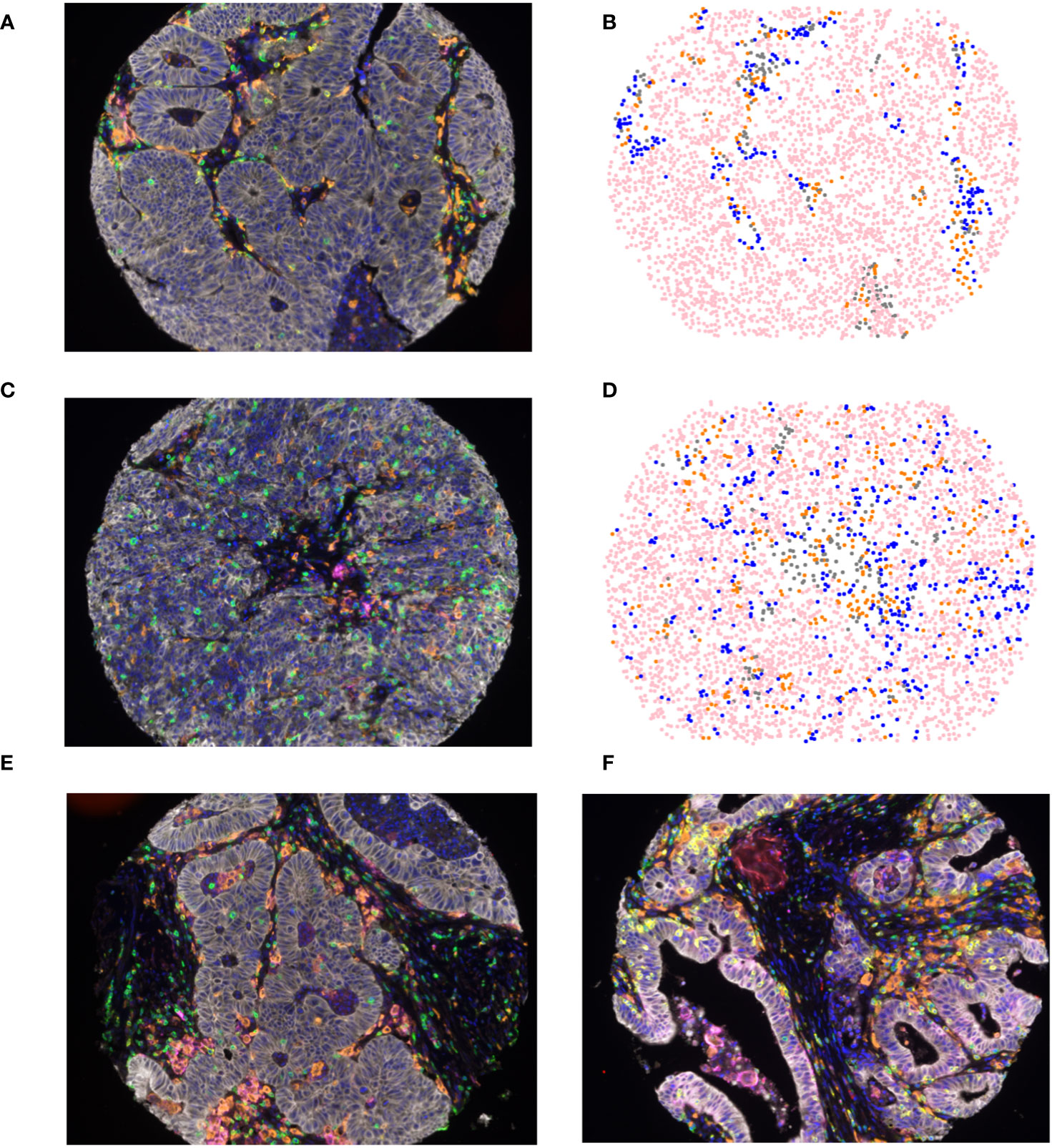
Figure 1 Multiplex fluorescent immunohistochemical (A, C) composite image of colorectal liver metastasis tumor microenvironment incorporating 7 cell types: tumor cells (white), T-cells (green), Tregs (green and red), CTLs (yellow), APCs (orange), PD-L1 (magenta), and DAPI (Blue), in (A) patients older than 65 years of age and (C) patients younger than 50 years of age; (B, D) spatial phenotype map of epithelial cells (pink), T-cells (blue), APCs (orange), and other cells (grey) in (B) patients older than 65 years of age and (D) patients younger than 50 years of age. Representative images of PD-L1 staining on APCs (E) and tumor cells (F).
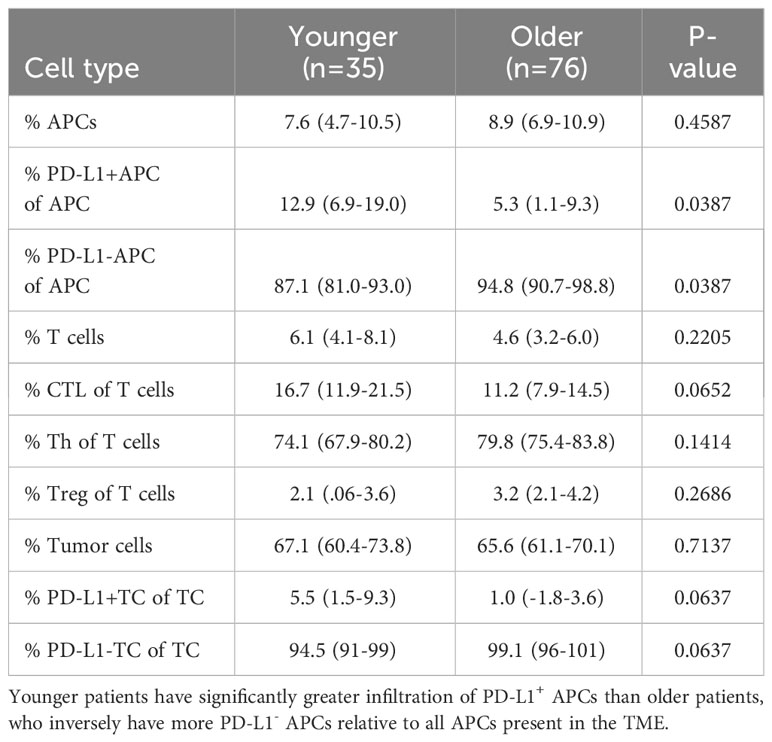
Table 2 Mean concentration of immune infiltrates in the mCRC TME in patients older than 65 years of age and patients younger than 50 years of age.
Proximity between CTLs and TCs is decreased in young patients
After unique spatial locations were assigned to phenotyped cells within the TME, cellular distance was quantified from each CTL to all other cell types. No significant difference was found between Tregs and nearest CTL (105.1µm in young vs 115.3µm in old, p=0.6373, Figure 2A), T helper cell and nearest CTL (34.4µm in young vs 29.9µm in old, p=0.9810, Figure 2B), or distance between an APC and nearest CTL (128.7µm in young vs 152.1µm in old, p=0.1742, Figure 2C). However, in young patients, the distance between both TCs and nearest CTL (152.8µm vs 194.2µm in old, p=0.0339, Figure 2D), and PD-L1- TCs and nearest CTL (158.4µm vs 196.7µm in old, p=0.0350, Figure 2E) was significantly shorter. The effect was abrogated when analyzing distance between PD-L1+ TC and nearest CTL (93.6µm in young vs 126.7µm in old, p=0.1103, Figure 2F). To evaluate this in the context of the larger cohort, spatial relationships between TCs and CTLs were evaluated against age as a continuous variable. When incorporating all ages, there continued to be a significant increase in intercellular distance (Figure 2G) and decreased cellular engagement (Figure 2H) with advancing age. These findings suggest that younger patients may have more effective infiltration of tumor antigen-specific CTLs given the decreased distances between TCs and CTLs. The abrogation of spatial proximity in the setting of PD-L1 positivity supports the hypothesis that PD-L1 expression may suppress CTL homing.
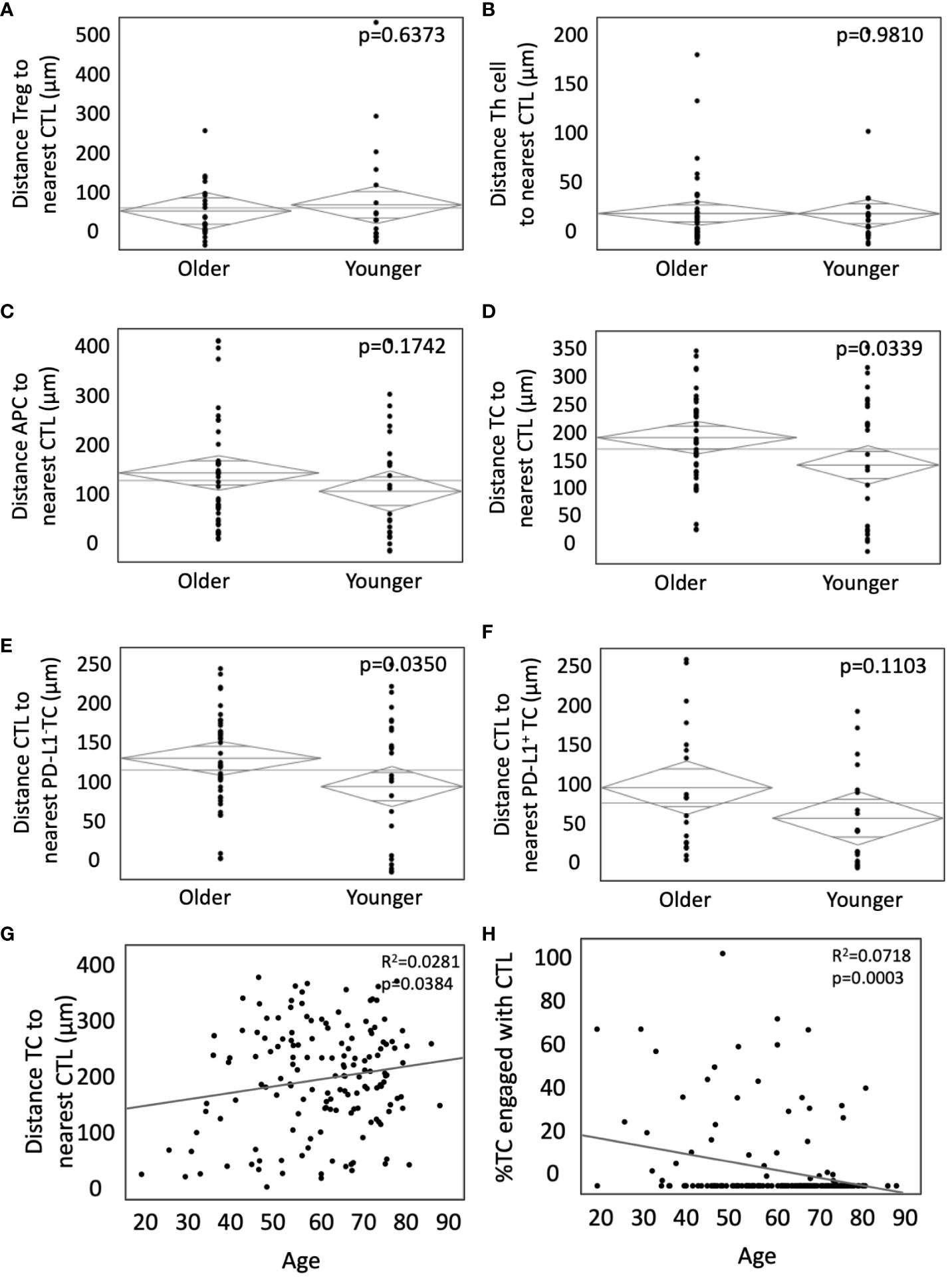
Figure 2 Younger patients present with closer TC to CTL interactions without PD-L1 expression. (A-F) ANOVA was used to compare the weighted mean distance in microns from (A) Tregs to nearest CTLs, (B) T-helper cells to nearest CTLs, (C) APCs to nearest CTLs, (D) TCs to nearest CTLs, and infiltrating CTLs relative to (E) PD-L1- TCs and (F) PD-L1+ TCs in younger patients (n=35) and older patients (n=76). Bivariate analysis of age as a continuous variable vs TC to CTL intercellular distance (G) and TC to CTL engagement (H). P values are shown for each.
CTLs are more frequently engaged with PD-L1- APCs and PD-L1- TCs in younger patients
Whereas cellular distance describes the spatial relationship between cells, cellular engagement more effectively describes physical interaction among cell types (42). We aimed to assess differences in engagement in young and old patients, as cellular engagement between CTLs and tumor cells is associated with improved survival in colorectal cancer (35). Cellular engagement was quantified by identifying instances in which a cell type was within 15µm of the center of a CTL of interest. CTLs were more likely to be engaged with APCs (35.1% in young vs 23.1% in old, p=0.0206, Figure 3A), and PD-L1- with APCs in the young (32.7% in young vs 22.6% in old, p=0.0249, Figure 3C). However, there was no difference in engagement among CTLs and PD-L1+ APCs (60.6% in young vs 54.4% in old, p=0.0980, Figure 3B). A similar trend was seen between CTL engagement with TCs, where young patients demonstrated increased engagement between CTLs and TCs (26.8% vs 15.7% in old, p=0.0016, Figure 3D) and PD-L1- TCs (24.5% vs 9.1% in old, p=0.0006, Figure 3E) but not PD-L1+ TCs (34.0% vs 27.4% in old, p=0.378, Figure 3F). Tumors were stratified into high or low CTL to TC engagement. Younger patients had a higher proportion of high CTL to TC engagement (47.6% vs 12.2% in the old, Figure 3G, p=0.0102). Because both increased infiltration and engagement of CTLs have been independently associated with improved outcomes, we next sought to determine if both factors were different in extremes of age. Indeed, young patients tended to have increased incidence of both elevated CTL infiltration and engagement (43.2% in young patients vs 8.1% in old patients, Figure 3H, p=0.0089). Increased CTL engagement with APCs and TCs may indicate increased anti-tumor immunity in younger patients. The abrogation of this effect with PD-L1 expression supports the hypothesis that PD-L1 expression is a mechanism of tumor immune evasion and may provide a link between the TME and the aggressive tumor biology in younger patients.
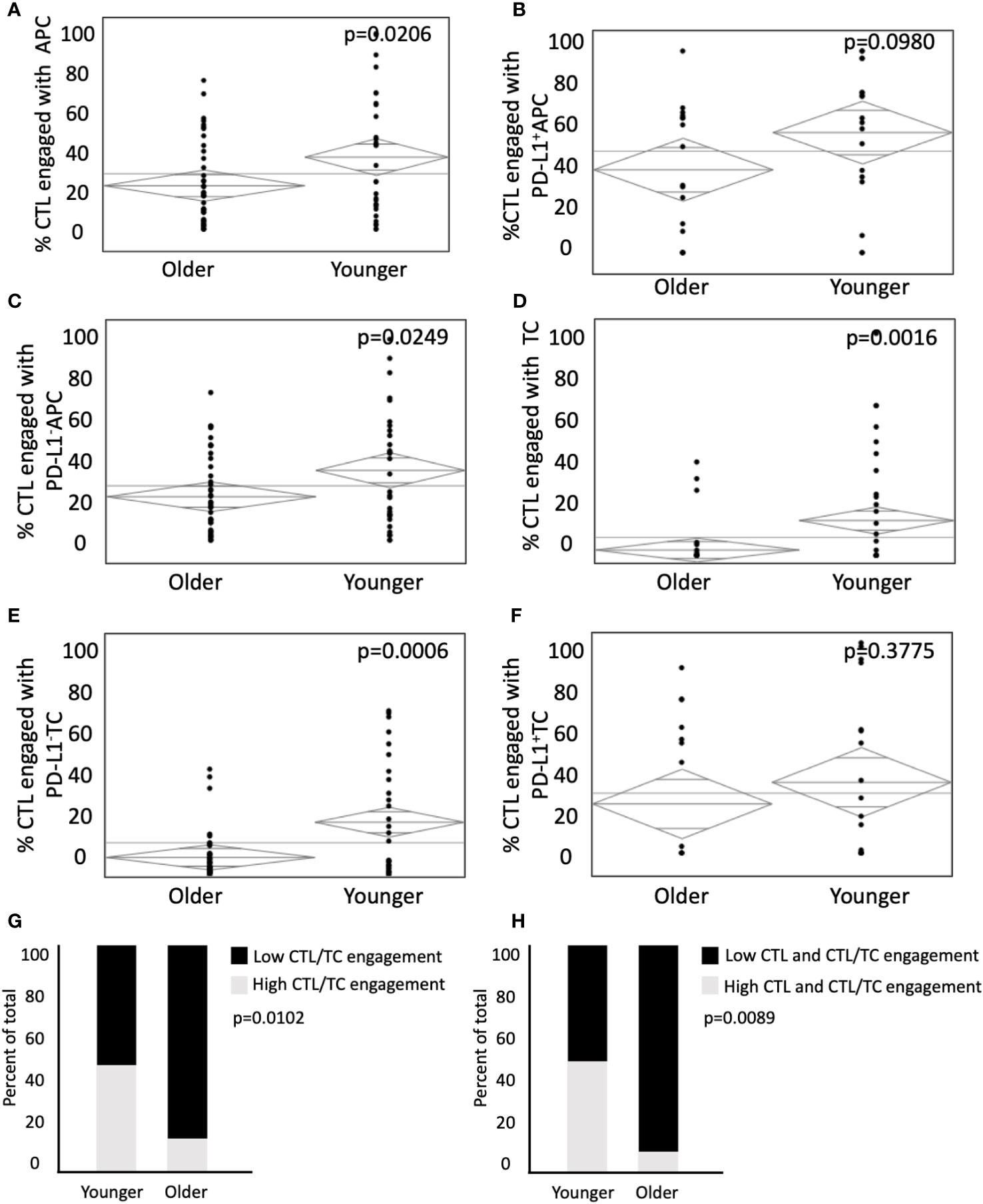
Figure 3 Younger patients present with higher CTL cellular engagement with PD-L1- APC and PD-L1- TC. Modeling predicts immune cell engagement between immune infiltrates in older and younger patients. (A-F) ANOVA analysis of CTL engagement to (A) APCs, (B) PD-L1+ APCs, (C) PD-L1- APCs, (D) TCs, (E) PD-L1- TCs, (F) PD-L1+ TCs in younger patients (n = 35) and older patients (n = 76). (G, H) ANOVA was used to compare percent of total patients between younger and older patient populations with (G) low CTL/EC engagement relative to high CTL/TC engagement and (H) Low CTL and CTL/TC engagement relative to high CTL and CTL/TC engagement. P values are shown for each.
Younger patients have increased CTL mixing and enhanced inflammation scores
Population level mixing of two or more cell types in the TME can be expressed as the tissue’s G-function (36–38). The rate of rise of the G-function correlates with the degree of cellular mixing, and therefore the area under the curve (AUC) can be used to compare the degree of cellular mixing at a fixed radius from individual cells between different tissues (36). When calculating G-function curves for CTLs and APCs, we found significantly higher mixing of these two cell types in young patients (AUC 6.7 in young vs 11.7 in old, p=0.0180, Figure 4A). Similarly, young patients had a greater degree of mixing of CTLs and TCs (AUC of 3.7 in young vs 0.97 in old, p=-.0063, Figure 4B). When stratified by the degree of CTL and TC mixing into high and low groups, younger patients had an increased percentage of high mixing (42.9% in young vs 22.4% in old, p=0.0413, Figure 4C). To evaluate potential synergistic pro-inflammatory cellular relationships, selected beneficial associations previously reported were used to calculate an immune-inflammation score (CD8-TC engagement, CD8-CD4 engagement, APC-CD8 engagement) (35, 36, 42). Younger patients had increased rates of high inflammation scores (40.0% high and 31.4% medium in young patients vs 18.4% high and 22.4% medium in older patients, p=0.0174, Figure 4D). These findings suggest that younger patients have increased mixing of both TCs and CTLs in the TME, and potentially a more immune primed environment.
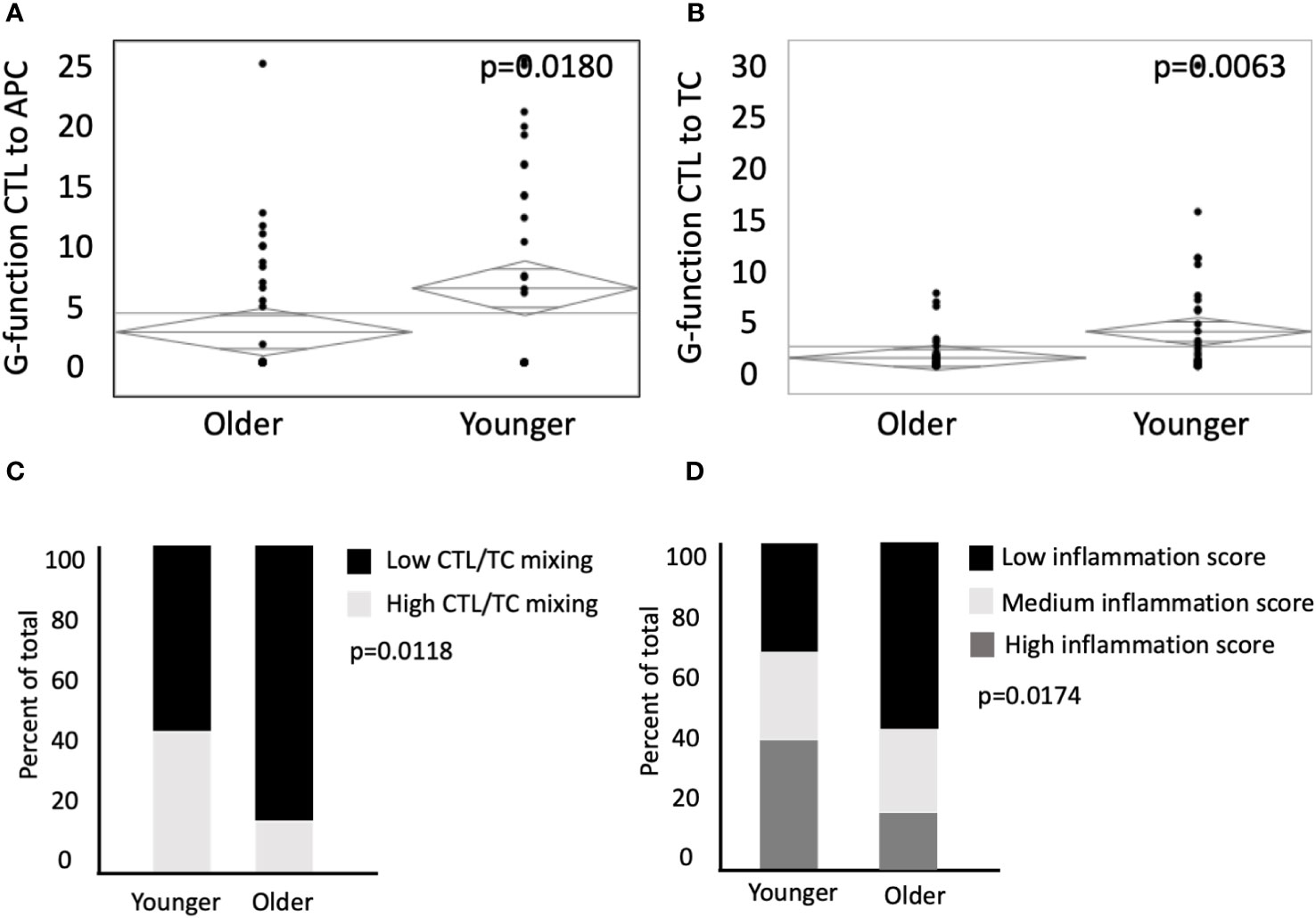
Figure 4 Younger patients have an elevated G-function, higher mixing of CTLs and enhanced inflammation scores. G-function analysis was conducted to measure mixing between CTLs to (A) APCs and (B) TCs in older patients (n=76) and younger patients (n=35). (C) ANOVA was used to compare percent of total patients between younger and older patient populations with low CTL/TC mixing relative to high CTL/TC mixing. (D) Patient inflammation scores between younger and older patients were categorized by terciles (low, medium, and high). P values are shown for each.
CTL and TC engagement remains increased in young patients with microsatellite stable tumors
Deficiencies in MMR machinery produce tumors with high mutational burden and MSI (14, 15), which leads to both an enhanced inflammatory response and upregulation of compensatory immunosuppressive elements (16, 17). Currently, MSI status is used to determine candidacy for checkpoint inhibition of PD-L1 and its receptor in mCRC (18–20), but there has yet to be significant clinical efficacy with immunotherapy in the subset of MSS patients with mCRC. To investigate age-related differences in the TME for the MSS population, we analyzed both the rates of MSI and MSS tumors by age, and cellular engagement in the TME among the subset of MSS patients (Figures 5A, B). A subset of 78 patients were subjected to IHC for MMR proteins MLH1, MSH2, MSH6, and PMS2, and deemed MSI if deficiency of one or more of the proteins were noted. Expectedly, younger patients had a higher percentage of MSI tumors (25% vs 6% in old, p=0.045, Figure 5C). In the subset of MSS patients, there was no difference in proportion of CTLs of all T cells (17.9% in young vs 14.4% in old, p=0.2786, Figure 5D). However, there was increased CTL engagement with TCs among MSS tumors in the younger population (26.9% vs 15.9% in the old, p=0.0043, Figure 5E). Stated another way, young patients with MSS tumors had increased proportion of tumors with high CTL/TC engagement than older patients with MSS tumors (33.3% in young vs 13.6% in old, p=0.0302, Figure 5F). Because of limited sample size, it was difficult to evaluate differences in spatial relationships in patients with MSI tumors. However, using the entire cohort (including those between 50 and 65), we were able to show that a similar trend was seen in MSI tumors (Supplementary Figure 1A, B). These data suggest that among MSS tumors, CTL and tumor cell interactions and anti-tumor immunity are increased in younger patients.
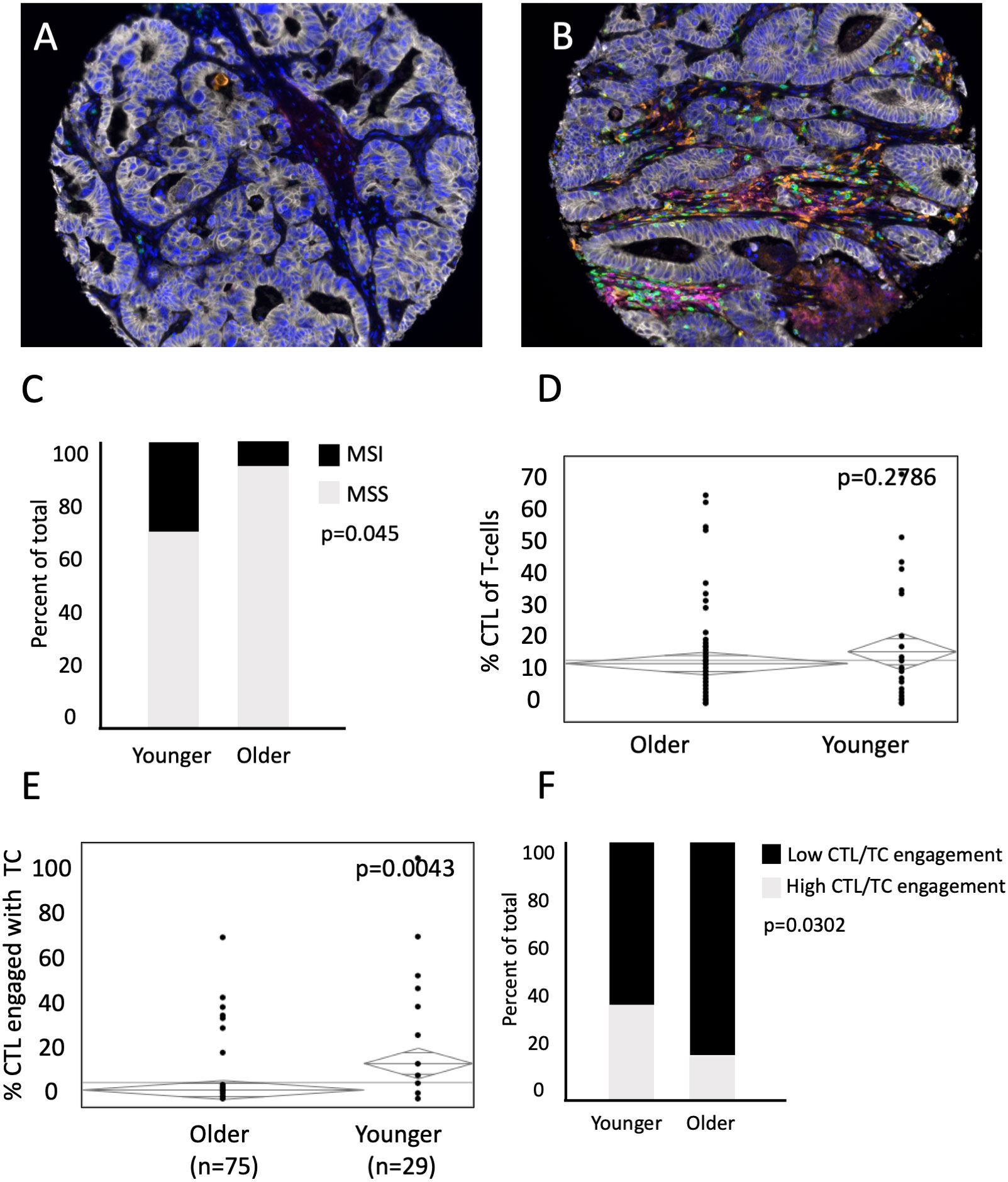
Figure 5 Representative images of MSS (A) and MSI (B) tumors. Younger patients with MSS have increased CTL/TC cellular engagement than older patients with MSS. (C) Percent of total patients between younger and older patient populations with Microsatellite Instability (MSI) and Microsatellite Stability (MSS). While there is (D) no difference in mean concentration of CTL present in the mCRC TME relative to all T-cells between younger (n=29) and older patients (n=75) with MSS, (E) percent of CTL/TC engagement in younger patients with MSS is significantly higher than in older patients with MSS. (F) ANOVA was used to compare percent of total patients excluding MSI patients with low CTL/TC mixing relative to high CTL/TC mixing.
Discussion
While age is a risk factor in the development of CRC (24), there has recently been a disproportionate increase in CRC incidence in patients under 50 years of age (25). Epidemiologic studies suggest that these younger patients tend to present with a more aggressive tumor biology, with more frequent lymphovascular invasion, synchronous tumors, and metachronous metastases (26, 43). Similarly, studies of molecular characteristics reveal differences based upon age, with younger patients more likely to have MSI high and mucinous tumors (27, 28). A recent article by Ugai et al. assessed cellular phenotypes in the TME in early-onset (<50 years), intermediate-onset (50-54 years), and later-onset CRC (≥55 years) (44). Although the differences did not reach statistical significance, there was a trend towards less tumor-infiltrating lymphocytes and peritumoral lymphocytic reactions in the early-onset compared to the later-onset group. Despite these age-related changes, complex characterization of immune infiltration in the TME of young patients with mCRC is lacking.
In this study, we performed cellular phenotyping and spatial modeling in the TME of a large cohort of patients, stratified by age, who underwent curative intent resection for colorectal liver metastasis. Although younger patients tended to have higher nodal disease burden and a higher number of metastatic deposits, all macroscopic disease was removed at the time of resection to limit disease-specific outcomes as a confounder. We identified unique characteristics present in the TME of younger patients that suggest a more immunologically active environment.
One such finding was a higher prevalence of cells expressing the immune checkpoint PD-L1. Binding of the immune checkpoint PD-1 and its ligand PD-L1 creates an immunosuppressive microenvironment by inhibiting T cell growth and CTL activation and limiting cytokine secretion (8, 45). Although it was initially thought that PD-L1 expression was important only on TCs, evidence suggests that APCs with PD-L1 expression also directly mediate T cell suppression (9, 10). Thus, over-expression of PD-1 and PD-L1 is a mechanism of tumor escape from immune surveillance (10). In CRC, PD-L1 expression is associated with worse prognosis with higher stage and grade tumors, more distant metastasis, aggressive tumor biology, and reduced overall survival in CRC (11–13, 46, 47). Our findings of a higher proportion of PD-L1+ APCs of all APCs within the younger cohort, and a trend towards increased expression on TCs, suggest a potentially more immunosuppressed TME. However, the decreased intercellular distance, increased engagement, and increased mixing between tumor cells and APCs with CTLs in the younger cohort suggest greater immune activation. While we have previously shown that increased engagement and cellular mixing of CTLs and TCs is associated with immune activation and improved disease-specific outcomes in mCRC (35, 36), it is possible that compensatory upregulation of PD-L1 related to higher CTL activity may be counteracting the beneficial CTL activity. This hypothesis is consistent with prior studies showing that PD-L1 expression has paradoxically been associated with higher CTL tumor infiltration in CRC (48, 49). It is this immune evasion that may underlie the more aggressive biology we tend to see in younger patients with colorectal cancer. This may also offer an opportunity for therapeutic intervention.
The molecular subtype in CRC is important in predicting response to immunotherapy. Defects in the MMR machinery lead to MSI and high neoantigen burden (14, 15). These MMR-deficient tumors are more susceptible to immunotherapies like the checkpoint inhibitors, which have shown great promise in treatment for advanced CRC with MSI, demonstrating a response rate of 33-55% and durable complete remissions (18–21). Recently, upfront, single-agent nivolumab has been shown to induce a complete clinical response at 6-25 month follow-up in a series of 12 patients with MMR-deficient locally advanced rectal cancer (50). However, MSI tumors comprise only 15% of CRC (51, 52). The larger population of MSS and MMR-proficient tumors are less susceptible to immunotherapy (53), associated with higher rates of local recurrence (54, 55), and worse clinical outcomes independent of disease stage (28, 56). Current treatment guidelines from the National Comprehensive Cancer Network recommend immunotherapy only for those patients with tumors demonstrating MSI (57). However, prior trials included relatively few colorectal cancers and a vast majority of patients were over 50 years of age. The true role for potential immunotherapy in young patients with MSS colorectal cancer has not been adequately studied.
When looking at only MSS tumors, increased CTL engagement with TCs persisted in the young cohort. This finding is independent of CTL infiltration, as there was no increase in the proportion of CTLs among T cells. Similarly, it is independent of APC infiltration, as we have previously shown a lack of association with MSI status (35). As the increased CTL engagement in the young cohort persisted regardless of microsatellite status, in the setting of a paradoxical increase in PD-L1 expression, a cohort of young patients with MSS disease may benefit from checkpoint inhibitor therapy.
It is possible that these differences in cellular engagement could relate to age, as aging is associated with both immunosenescence and immune dysregulation that decrease T-cell activation and proliferation (33, 58). In contrast, however, aging is also associated with low-grade chronic inflammation and increased inflammatory signaling (59), as well as increased tumor mutational burden and expression of immune checkpoint genes (60). There is also contradictory evidence of the impact of age on response to immunotherapy in clinical trials. For instance, in melanoma, two studies found a more significant survival benefit of anti-PD-1 immunotherapy in patients over 60 (61, 62). However, other meta-analyses of anti-PD-1 therapy in solid tumors did not show age-related differences in overall survival (63, 64). These findings suggest further investigation of age-related differences is needed to better understand responsiveness to immunotherapy.
Although there was no difference in the proportion of patients receiving preoperative chemotherapy, this study is limited by chemotherapy as a confounder. It is possible that age-related differences in response to chemotherapy could alter the TME and influence immune cell interactions (Supplementary Figure 1C, D). Additionally, mfIHC can only query a small panel of antibodies, limiting investigation of specialized populations like polarized dendritic cells and B lymphocytes. TMAs capture only a small portion of the tumor and although use of triplicate cores can help decrease effects of intratumoral variability, it likely falls short of whole slide imaging. Additionally, as this TMA was constructed to evaluate the broader population of patients with metastatic colon cancer, the ages are skewed towards older, which created some imbalance in sample size. Finally, because these patients were selected to undergo curative intent metastasectomy, they may not be truly representative of the general population of stage 4 colon cancer patients.
Conclusions
In this large cohort of patients undergoing curative-intent resection of colorectal liver metastasis, cellular phenotyping and spatial characterization of immune cells demonstrated decreased intercellular distance and increased cellular engagement between TCs and CTLs, as well as TCs and APCs, in young patients with mCRC. Young patients also demonstrated an increased proportion of PD-L1+ APCs of all APCs. These trends were independent of microsatellite instability. These findings may suggest a more favorable microenvironment for immune based therapy in young patients with metastatic colon cancer.
Data availability statement
The raw data supporting the conclusions of this article will be made available by the authors, without undue reservation.
Ethics statement
The studies involving humans were approved by Memorial Sloan-Kettering Cancer Center IRB. The studies were conducted in accordance with the local legislation and institutional requirements. The participants provided their written informed consent to participate in this study.
Author contributions
TF: Conceptualization, Formal analysis, Funding acquisition, Methodology, Supervision, Writing – review & editing. BG: Data curation, Investigation, Writing – original draft. JL: Data curation, Investigation, Methodology, Writing – original draft. JM: Data curation, Investigation, Writing – review & editing. SK: Data curation, Formal analysis, Methodology, Writing – original draft. MD’A: Conceptualization, Writing – review & editing. JiS: Data curation, Writing – review & editing. ID: Writing – review & editing. JE: Investigation, Writing – review & editing. AR: Conceptualization, Methodology, Supervision, Writing – review & editing. JaS: Data curation, Formal analysis, Writing – review & editing.
Funding
The author(s) declare financial support was received for the research, authorship, and/or publication of this article. This study was supported by Veterans administration - I01BX005777 - effort and resources NIH - R01DK128102 - effort and resources NIH - R01CA268426 - effort and resources NIH - U01CA274154 - effort and resources.
Conflict of interest
The authors declare that the research was conducted in the absence of any commercial or financial relationships that could be construed as a potential conflict of interest.
Publisher’s note
All claims expressed in this article are solely those of the authors and do not necessarily represent those of their affiliated organizations, or those of the publisher, the editors and the reviewers. Any product that may be evaluated in this article, or claim that may be made by its manufacturer, is not guaranteed or endorsed by the publisher.
Supplementary material
The Supplementary Material for this article can be found online at: https://www.frontiersin.org/articles/10.3389/fimmu.2023.1289402/full#supplementary-material
Supplementary Figure 1 | Bivariate analysis of age and TC/CTL intercellular distance (A) and cellular engagement (B) in patients with MSI tumors. TC/CTL engagement in young (C) and old (D) patients relative to receipt of pre-operative chemotherapy.
Supplementary Table 1 | Antibody clones, manufacturer and concentrations.
References
1. Galon J, Fridman WH, Pages F. The adaptive immunologic microenvironment in colorectal cancer: a novel perspective. Cancer Res (2007) 67(5):1883–6. doi: 10.1158/0008-5472.CAN-06-4806
2. Gout S, Huot J. Role of cancer microenvironment in metastasis: focus on colon cancer. Cancer Microenviron (2008) 1(1):69–83. doi: 10.1007/s12307-008-0007-2
3. Fridman WH, Pages F, Sautes-Fridman C, Galon J. The immune contexture in human tumours: impact on clinical outcome. Nat Rev Cancer (2012) 12(4):298–306. doi: 10.1038/nrc3245
4. Glaire MA, Domingo E, Sveen A, Bruun J, Nesbakken A, Nicholson G, et al. Tumour-infiltrating CD8(+) lymphocytes and colorectal cancer recurrence by tumour and nodal stage. Br J Cancer (2019) 121(6):474–82. doi: 10.1038/s41416-019-0540-4
5. Katz SC, Bamboat ZM, Maker AV, Shia J, Pillarisetty VG, Yopp AC, et al. Regulatory T cell infiltration predicts outcome following resection of colorectal cancer liver metastases. Ann Surg Oncol (2013) 20(3):946–55. doi: 10.1245/s10434-012-2668-9
6. Katz SC, Pillarisetty V, Bamboat ZM, Shia J, Hedvat C, Gonen M, et al. T cell infiltrate predicts long-term survival following resection of colorectal cancer liver metastases. Ann Surg Oncol (2009) 16(9):2524–30. doi: 10.1245/s10434-009-0585-3
7. Tosolini M, Kirilovsky A, Mlecnik B, Fredriksen T, Mauger S, Bindea G, et al. Clinical impact of different classes of infiltrating T cytotoxic and helper cells (Th1, th2, treg, th17) in patients with colorectal cancer. Cancer Res (2011) 71(4):1263–71. doi: 10.1158/0008-5472.CAN-10-2907
8. O’Malley G, Treacy O, Lynch K, Naicker SD, Leonard NA, Lohan P, et al. Stromal cell PD-L1 inhibits CD8(+) T-cell antitumor immune responses and promotes colon cancer. Cancer Immunol Res (2018) 6(11):1426–41. doi: 10.1158/2326-6066.CIR-17-0443
9. Curiel TJ, Wei S, Dong H, Alvarez X, Cheng P, Mottram P, et al. Blockade of B7-H1 improves myeloid dendritic cell-mediated antitumor immunity. Nat Med (2003) 9(5):562–7. doi: 10.1038/nm863
10. Lin H, Wei S, Hurt EM, Green MD, Zhao L, Vatan L, et al. Host expression of PD-L1 determines efficacy of PD-L1 pathway blockade-mediated tumor regression. J Clin Invest (2018) 128(4):1708. doi: 10.1172/JCI120803
11. Elfishawy M, Abd ESA, Hegazy A, El-Yasergy DF. Immunohistochemical expression of programmed death ligand-1 (PDL-1) in colorectal carcinoma and its correlation with stromal tumor infiltrating lymphocytes. Asian Pac J Cancer Prev (2020) 21(1):225–32. doi: 10.31557/APJCP.2020.21.1.225
12. Shan T, Chen S, Wu T, Yang Y, Li S, Chen X. PD-L1 expression in colon cancer and its relationship with clinical prognosis. Int J Clin Exp Pathol (2019) 12(5):1764–9.
13. Zhao T, Li Y, Zhang J, Zhang B. PD-L1 expression increased by IFN-gamma via JAK2-STAT1 signaling and predicts a poor survival in colorectal cancer. Oncol Lett (2020) 20(2):1127–34. doi: 10.3892/ol.2020.11647
14. Boland CR, Goel A. Microsatellite instability in colorectal cancer. Gastroenterology (2010) 138(6):2073–2087 e3. doi: 10.1053/j.gastro.2009.12.064
15. Koopman M, Kortman GA, Mekenkamp L, Ligtenberg MJ, Hoogerbrugge N, Antonini NF, et al. Deficient mismatch repair system in patients with sporadic advanced colorectal cancer. Br J Cancer (2009) 100(2):266–73. doi: 10.1038/sj.bjc.6604867
16. Deschoolmeester V, Baay M, Lardon F, Pauwels P, Peeters M. Immune cells in colorectal cancer: prognostic relevance and role of MSI. Cancer Microenviron (2011) 4(3):377–92. doi: 10.1007/s12307-011-0068-5
17. Llosa NJ, Cruise M, Tam A, Wicks EC, Hechenbleikner EM, Taube JM, et al. The vigorous immune microenvironment of microsatellite instable colon cancer is balanced by multiple counter-inhibitory checkpoints. Cancer Discovery (2015) 5(1):43–51. doi: 10.1158/2159-8290.CD-14-0863
18. Le DT, Durham JN, Smith KN, Wang H, Bartlett BR, Aulakh LK, et al. Mismatch repair deficiency predicts response of solid tumors to PD-1 blockade. Science (2017) 357(6349):409–13. doi: 10.1126/science.aan6733
19. Le DT, Uram JN, Wang H, Bartlett BR, Kemberling H, Eyring AD, et al. PD-1 blockade in tumors with mismatch-repair deficiency. N Engl J Med (2015) 372(26):2509–20. doi: 10.1056/NEJMoa1500596
20. Andre T, Shiu KK, Kim TW, Jensen BV, Jensen LH, Punt C, et al. Pembrolizumab in microsatellite-instability-high advanced colorectal cancer. N Engl J Med (2020) 383(23):2207–18. doi: 10.1056/NEJMoa2017699
21. Overman MJ, Lonardi S, Wong KYM, Lenz HJ, Gelsomino F, Aglietta M, et al. Durable clinical benefit with nivolumab plus ipilimumab in DNA mismatch repair-deficient/microsatellite instability-high metastatic colorectal cancer. J Clin Oncol (2018) 36(8):773–9. doi: 10.1200/JCO.2017.76.9901
22. Eng C, Kim TW, Bendell J, Argiles G, Tebbutt NC, Di Bartolomeo M, et al. Atezolizumab with or without cobimetinib versus regorafenib in previously treated metastatic colorectal cancer (IMblaze370): a multicentre, open-label, phase 3, randomised, controlled trial. Lancet Oncol (2019) 20(6):849–61. doi: 10.1016/S1470-2045(19)30027-0
23. Fukuoka S, Hara H, Takahashi N, Kojima T, Kawazoe A, Asayama M, et al. Regorafenib plus nivolumab in patients with advanced gastric or colorectal cancer: an open-label, dose-escalation, and dose-expansion phase ib trial (REGONIVO, EPOC1603). J Clin Oncol (2020) 38(18):2053–61. doi: 10.1200/JCO.19.03296
24. Siegel RL, Miller KD, Goding Sauer A, Fedewa SA, Butterly LF, Anderson JC, et al. Colorectal cancer statistics, 2020. CA Cancer J Clin (2020) 70(3):145–64. doi: 10.3322/caac.21601
25. Stoffel EM, Murphy CC. Epidemiology and mechanisms of the increasing incidence of colon and rectal cancers in young adults. Gastroenterology (2020) 158(2):341–53. doi: 10.1053/j.gastro.2019.07.055
26. Abdelsattar ZM, Wong SL, Regenbogen SE, Jomaa DM, Hardiman KM, Hendren S. Colorectal cancer outcomes and treatment patterns in patients too young for average-risk screening. Cancer (2016) 122(6):929–34. doi: 10.1002/cncr.29716
27. Farrington SM, McKinley AJ, Carothers AD, Cunningham C, Bubb VJ, Sharp L, et al. Evidence for an age-related influence of microsatellite instability on colorectal cancer survival. Int J Cancer (2002) 98(6):844–50. doi: 10.1002/ijc.10264
28. Gryfe R, Kim H, Hsieh ET, Aronson MD, Holowaty EJ, Bull SB, et al. Tumor microsatellite instability and clinical outcome in young patients with colorectal cancer. N Engl J Med (2000) 342(2):69–77. doi: 10.1056/NEJM200001133420201
29. Patel SG, Ahnen DJ. Colorectal cancer in the young. Curr Gastroenterol Rep (2018) 20(4):15. doi: 10.1007/s11894-018-0618-9
30. Franceschi C, Campisi J. Chronic inflammation (inflammaging) and its potential contribution to age-associated diseases. J Gerontol A Biol Sci Med Sci (2014) 69 Suppl 1:S4–9. doi: 10.1093/gerona/glu057
31. Kuilman T, Peeper DS. Senescence-messaging secretome: SMS-ing cellular stress. Nat Rev Cancer (2009) 9(2):81–94. doi: 10.1038/nrc2560
32. Leonardi GC, Accardi G, Monastero R, Nicoletti F, Libra M. Ageing: from inflammation to cancer. Immun Ageing (2018) 15:1. doi: 10.1186/s12979-017-0112-5
33. Pawelec G. Hallmarks of human “immunosenescence”: adaptation or dysregulation? Immun Ageing (2012) 9(1):15. doi: 10.1186/1742-4933-9-15
34. van Grevenstein WM, Hofland LJ, van Rossen ME, van Koetsveld PM, Jeekel J, van Eijck CH. Inflammatory cytokines stimulate the adhesion of colon carcinoma cells to mesothelial monolayers. Dig Dis Sci (2007) 52(10):2775–83. doi: 10.1007/s10620-007-9778-4
35. Lazarus J, Maj T, Smith JJ, Perusina Lanfranca M, Rao A, D’Angelica MI, et al. Spatial and phenotypic immune profiling of metastatic colon cancer. JCI Insight (2018) 3(22). doi: 10.1172/jci.insight.121932
36. Lazarus J, Oneka MD, Barua S, Maj T, Lanfranca MP, Delrosario L, et al. Mathematical modeling of the metastatic colorectal cancer microenvironment defines the importance of cytotoxic lymphocyte infiltration and presence of PD-L1 on antigen presenting cells. Ann Surg Oncol (2019) 26(9):2821–30. doi: 10.1245/s10434-019-07508-3
37. Barua S, Fang P, Sharma A, Fujimoto J, Wistuba I, Rao AUK, et al. Spatial interaction of tumor cells and regulatory T cells correlates with survival in non-small cell lung cancer. Lung Cancer (2018) 117:73–9. doi: 10.1016/j.lungcan.2018.01.022
38. Barua S, Solis L, Parra ER, Uraoka N, Jiang M, Wang H, et al. A functional spatial analysis platform for discovery of immunological interactions predictive of low-grade to high-grade transition of pancreatic intraductal papillary mucinous neoplasms. Cancer Inform (2018) 17:1176935118782880. doi: 10.1177/1176935118782880
39. Carstens JL, Correa de Sampaio P, Yang D, Barua S, Wang H, Rao A, et al. Spatial computation of intratumoral T cells correlates with survival of patients with pancreatic cancer. Nat Commun (2017) 8:15095. doi: 10.1038/ncomms15095
40. Andre T, Boni C, Mounedji-Boudiaf L, Navarro M, Tabernero J, Hickish T, et al. Multicenter International Study of Oxaliplatin/5-Fluorouracil/Leucovorin in the Adjuvant Treatment of Colon Cancer: Oxaliplatin, fluorouracil, and leucovorin as adjuvant treatment for colon cancer. N Engl J Med (2004) 350(23):2343–51. doi: 10.1056/NEJMoa032709
41. Lazarus J, Akiska Y, Perusina Lanfranca M, Delrosario L, Sun L, Long D, et al. Optimization, design and avoiding pitfalls in manual multiplex fluorescent immunohistochemistry. J Vis Exp (2019) 149. doi: 10.3791/59915
42. Griffith BD, Turcotte S, Lazarus J, Lima F, Bell S, Delrosario L, et al. MHC class II expression influences the composition and distribution of immune cells in the metastatic colorectal cancer microenvironment. Cancers (Basel) (2022) 14(17). doi: 10.3390/cancers14174092
43. O’Connell JB, Maggard MA, Liu JH, Etzioni DA, Livingston EH, Ko CY. Do young colon cancer patients have worse outcomes? World J Surg (2004) 28(6):558–62. doi: 10.1007/s00268-004-7306-7
44. Ugai T, Vayrynen JP, Lau MC, Borowsky J, Akimoto N, Vayrynen SA, et al. Immune cell profiles in the tumor microenvironment of early-onset, intermediate-onset, and later-onset colorectal cancer. Cancer Immunol Immunother (2022) 71(4):933–42. doi: 10.1007/s00262-021-03056-6
45. Payandeh Z, Khalili S, Somi MH, Mard-Soltani M, Baghbanzadeh A, Hajiasgharzadeh K, et al. PD-1/PD-L1-dependent immune response in colorectal cancer. J Cell Physiol (2020) 235(7-8):5461–75. doi: 10.1002/jcp.29494
46. Black M, Barsoum IB, Truesdell P, Cotechini T, Macdonald-Goodfellow SK, Petroff M, et al. Activation of the PD-1/PD-L1 immune checkpoint confers tumor cell chemoresistance associated with increased metastasis. Oncotarget (2016) 7(9):10557–67. doi: 10.18632/oncotarget.7235
47. Wyss J, Dislich B, Koelzer VH, Galvan JA, Dawson H, Hadrich M, et al. Stromal PD-1/PD-L1 expression predicts outcome in colon cancer patients. Clin Colorectal Cancer (2019) 18(1):e20–38. doi: 10.1016/j.clcc.2018.09.007
48. Rosenbaum MW, Bledsoe JR, Morales-Oyarvide V, Huynh TG, Mino-Kenudson M. PD-L1 expression in colorectal cancer is associated with microsatellite instability, BRAF mutation, medullary morphology and cytotoxic tumor-infiltrating lymphocytes. Mod Pathol (2016) 29(9):1104–12. doi: 10.1038/modpathol.2016.95
49. Droeser RA, Hirt C, Viehl CT, Frey DM, Nebiker C, Huber X, et al. Clinical impact of programmed cell death ligand 1 expression in colorectal cancer. Eur J Cancer (2013) 49(9):2233–42. doi: 10.1016/j.ejca.2013.02.015
50. Cercek A, Lumish M, Sinopoli J, Weiss J, Shia J, Lamendola-Essel M, et al. PD-1 blockade in mismatch repair-deficient, locally advanced rectal cancer. N Engl J Med (2022) 386(25):2363–76. doi: 10.1056/NEJMoa2201445
51. Markowitz SD, Bertagnolli MM. Molecular origins of cancer: Molecular basis of colorectal cancer. N Engl J Med (2009) 361(25):2449–60. doi: 10.1056/NEJMra0804588
52. de la Chapelle A, Hampel H. Clinical relevance of microsatellite instability in colorectal cancer. J Clin Oncol (2010) 28(20):3380–7. doi: 10.1200/JCO.2009.27.0652
53. Stein A, Folprecht G. Immunotherapy of colon cancer. Oncol Res Treat (2018) 41(5):282–5. doi: 10.1159/000488918
54. Tejpar S, Saridaki Z, Delorenzi M, Bosman F, Roth AD. Microsatellite instability, prognosis and drug sensitivity of stage II and III colorectal cancer: more complexity to the puzzle. J Natl Cancer Inst (2011) 103(11):841–4. doi: 10.1093/jnci/djr170
55. Sinicrope FA, Foster NR, Thibodeau SN, Marsoni S, Monges G, Labianca R, et al. DNA mismatch repair status and colon cancer recurrence and survival in clinical trials of 5-fluorouracil-based adjuvant therapy. J Natl Cancer Inst (2011) 103(11):863–75. doi: 10.1093/jnci/djr153
56. Popat S, Hubner R, Houlston RS. Systematic review of microsatellite instability and colorectal cancer prognosis. J Clin Oncol (2005) 23(3):609–18. doi: 10.1200/JCO.2005.01.086
57. N. C. C. Network. Clinical Practice Guidelines in Oncology: Colon Cancer (Version 3.2022). Pennsylvania, United States: National Comprehensive Cancer Network (2023).
58. Linton PJ, Haynes L, Tsui L, Zhang X, Swain S. From naive to effector–alterations with aging. Immunol Rev (1997) 160:9–18. doi: 10.1111/j.1600-065x.1997.tb01023.x
59. Franceschi C, Bonafe M, Valensin S, Olivieri F, De Luca M, Ottaviani E, et al. Inflamm-aging. An evolutionary perspective on immunosenescence. Ann N Y Acad Sci (2000) 908:244–54. doi: 10.1111/j.1749-6632.2000.tb06651.x
60. Erbe R, Wang Z, Wu S, Xiu J, Zaidi N, La J, et al. Evaluating the impact of age on immune checkpoint therapy biomarkers. Cell Rep (2021) 37(7):110033. doi: 10.1016/j.celrep.2021.110033
61. Kugel CH 3rd, Douglass SM, Webster MR, Kaur A, Liu Q, Yin X, et al. Weeraratna: age correlates with response to anti-PD1, reflecting age-related differences in intratumoral effector and regulatory T-cell populations. Clin Cancer Res (2018) 24(21):5347–56. doi: 10.1158/1078-0432.CCR-18-1116
62. Jain V, Hwang WT, Venigalla S, Nead KT, Lukens JN, Mitchell TC, et al. Association of age with efficacy of immunotherapy in metastatic melanoma. Oncologist (2020) 25(2):e381–5. doi: 10.1634/theoncologist.2019-0377
63. Elias R, Giobbie-Hurder A, McCleary NJ, Ott P, Hodi FS, Rahma O. Efficacy of PD-1 & PD-L1 inhibitors in older adults: a meta-analysis. J Immunother Cancer (2018) 6(1):26. doi: 10.1186/s40425-018-0336-8
Keywords: tumor immunology, colorectal cancer, immunotherapy, spatial relation analysis, multiplex immunohistochemistry (IHC)
Citation: Griffith BD, Lazarus J, McGue J, Krishnan S, D’Angelica MI, Shia J, Dobrosotskaya I, Shi J, Edwards J, Rao A and Frankel TL (2023) Unique characteristics of the tumor immune microenvironment in young patients with metastatic colorectal cancer. Front. Immunol. 14:1289402. doi: 10.3389/fimmu.2023.1289402
Received: 05 September 2023; Accepted: 17 November 2023;
Published: 13 December 2023.
Edited by:
Subramaniam Malarkannan, Medical College of Wisconsin, United StatesReviewed by:
Prathyusha Konda, Dana–Farber Cancer Institute, United StatesErik Hooijberg, Antoni van Leeuwenhoek Hospital, Netherlands
Copyright © 2023 Griffith, Lazarus, McGue, Krishnan, D’Angelica, Shia, Dobrosotskaya, Shi, Edwards, Rao and Frankel. This is an open-access article distributed under the terms of the Creative Commons Attribution License (CC BY). The use, distribution or reproduction in other forums is permitted, provided the original author(s) and the copyright owner(s) are credited and that the original publication in this journal is cited, in accordance with accepted academic practice. No use, distribution or reproduction is permitted which does not comply with these terms.
*Correspondence: Timothy L. Frankel, dGltb2ZyYW5AbWVkLnVtaWNoLmVkdQ==